- Department of Biology, Miami University, Oxford, OH, United States
There is a striking sex bias in the prevalence and severity of autism spectrum disorder (ASD) with 80% of diagnoses occurring in males. Because the molecular etiology of ASD is likely combinatorial, including interactions across multiple genetic and environmental factors, it is difficult to investigate the physiological mechanisms driving sex-specific differences. Loss of function mutations in TSC1 result in dysregulated mTORC1 signaling and underlie a multi-system disorder known as tuberous sclerosis (TSC). Interestingly, more than 50% of individuals diagnosed with TSC are also diagnosed with ASD, making TSC mutations one of the most prevalent monogenic causes of ASD. Mice harboring targeted deletion of Tsc1 selectively in cerebellar Purkinje neurons, referred to here as Tsc1mut/mut, have multiple ASD-linked behavioral impairments, including deficits in social interactions, motor coordination, and vocalizations. However, these ASD-linked behavioral deficits have only been investigated using male Tsc1mut/mut animals. Here, we used cohorts of male and female Tsc1mut/mut animals to determine if behavioral impairments, previously identified in this model, are similar across sex. Specifically, we measured balance and motor coordination and social interaction behaviors in two age groups across sex. We determined balance and motor coordination deficits are similar in male and female Tsc1mut/mut mice, and that deficits in the firing of Tsc1mut/mut Purkinje neurons located in the cerebellar vermis are also similar across sex. However, impairments in social approach behavior were found to be significantly more severe in Tsc1mut/mut males compared to females. These results indicate the selective deletion of Tsc1 in Purkinje neurons differentially impairs cerebellar circuits based on sex.
1 Introduction
Across individuals, measures of ASD symptom manifestation and severity are heterogeneous (Geschwind, 2011), however, a highly replicated finding in ASD research is the greater number of diagnoses in males compared to females (Christensen et al., 2018). Sex-specific ASD differences have also been described but vary across age groups and symptom domains (Wijngaarden-Cremers et al., 2014; Tillmann et al., 2018; Craig et al., 2020). While the details of these sex-specific differences continue to be revealed, it remains difficult to investigate the underlying cellular and molecular mechanisms due to the etiology of ASD typically involving multiple interacting genetic and environmental factors. Here we examine if there are sex specific phenotypes in an ASD-mouse model which has selective deletion of a single ASD-linked gene, Tsc1, from a single neuronal cell type, cerebellar Purkinje neurons.
Several lines of evidence implicate cerebellar dysfunction in the development of ASD (Wang et al., 2014). Cerebellar Purkinje neurons integrate incoming information and are the sole output cells of the cerebellar cortex (Palkovits et al., 1972; Andersen et al., 1992). Reduced Purkinje neuron firing has been directly linked to ASD-like behavioral deficits in mice (Tsai et al., 2012, 2018; Kalume et al., 2007) and post-mortem investigations have revealed a reduced number and density of cerebellar Purkinje cells in individuals with ASD (Wang et al., 2014; Bailey et al., 1998; Stoodley, 2014). Tuberous sclerosis complex (TSC) is a disorder affecting multiple organ systems with severe cognitive, behavioral, and psychiatric symptoms (Crino et al., 2006). TSC is the result of loss-of-function mutations in TSC1 or TSC2 (European Chromosome 16 Tuberous Sclerosis Consortium, 1993; Au et al., 2007). Proteins encoded by these genes are necessary to form a signaling complex which drives inhibition of the mammalian target of the rapamycin complex 1 (mTORC1) pathway, which plays a major role in regulating cell growth and metabolism (Wullschleger et al., 2006). Interestingly, greater than 50% of individuals diagnosed with TSC also meet diagnostic criteria for ASD (Wiznitzer, 2004; Jeste et al., 2008), making TSC mutations one of the most prevalent monogenic causes of ASD.
To evaluate the role of Tsc1 in cerebellar PNs, Tsai et al. (2012) developed a mouse model with targeted and selective Tsc1 deletion in cerebellar Purkinje neurons using L7-Pcp2:Cre/LoxP recombination (Barski et al., 2000; Saito et al., 2005). Interestingly, this mouse model, referred to here as Tsc1mut/mut, exhibits several ASD-linked behavioral phenotypes, including deficits in motor coordination, vocalizations, social interactions, and exaggerated repetitive behaviors (Tsai et al., 2012). These behavioral impairments have been linked to both reduced repetitive firing in cerebellar Purkinje neurons, and in older animals (>10 weeks-old), to the progressive loss of Purkinje neurons via apoptosis (Tsai et al., 2012, 2018). These previous investigations of Tsc1mut/mut phenotypes, however, did not include female test subjects.
We examined if Tsc1 deletion differentially affects Purkinje neuron intrinsic excitability and ASD-linked behavioral impairments across male and female adult mice. We determined that in older (16–24 week-old) Tsc1mut/mut mice, social interaction deficits are significantly more severe in males compared to females, however, balance and motor coordination impairments in these animals are similar across sex, suggesting cerebellar circuits are differentially impaired in Tsc1mut/mut mice based on animal sex.
2 Methods
2.1 Subjects
This study used the L7-Pcp2 Cre/Tsc1 floxed animal model of autism in mice (Mus musculus) with a C57BL/J6 genetic background (Jackson Laboratories). After weaning, mice were housed with littermates of the same sex at Miami University’s Laboratory of Animal Resources facility, with controlled temperature and humidity and a 12:12 light cycle starting at 7:00 am. Mice were provided with water and a standard rodent diet ad libitum (Lab Diet 5,001, Cincinnati Lab Supply Inc., Cincinnati, OH, USA). All animal experimentation was approved by the Institutional Animal Care and Use Committee of Miami University (protocol #1044). Cre-negative Tsc1flox/flox mice were crossed with Cre-positive Tsc1flox/wt mice to generate Cre-positive Tsc1flox/wt, referred to here as Tsc1mut/wt, and Cre-positive Tsc1flox/flox mice, referred to here as Tsc1mut/mut mice for experiments. These pairings also generated Cre-negative Tsc1flox/flox mice, which were used as littermate controls in behavioral studies. L7-Pcp2 Cre-positive animals (lacking loxP sites) and Cre-negative Tsc1flox/flox have previously been shown to not differ from wild type mice in behavioral assays (Barski et al., 2000; Ehninger et al., 2008; Tsai et al., 2012, 2018). Hemizygous Cre-positive animals were also crossed with the Ai14 Cre reporter tool strain (Jackson Laboratory, strain # 007914).
2.2 Microscopy and image processing
Parasagittal cerebellar slices (25 μm) of fixed and cryoprotected brains were used for imaging the tdTomato fluorescent signal in Cre-positive Purkinje neurons. Images were acquired using a Zeiss LSM-710 confocal microscope using either a 63X oil emersion objective or 20X objective. Z-series stack images with 1 μm intervals or single plane snapshots were used to assess tdTomato expression in Cre-reporter mice.
2.3 Electrophysiology methods
Spontaneous and evoked action potentials were recorded from Purkinje neurons in parasagittal brain sections acutely isolated from 5 to 8 week-old animals. Brain sections were perfused with warmed (33–34°C) artificial cerebral spinal fluid (ACSF) that was continuously bubbled with 95% O2/5% CO2. Borosilicate glass recording pipettes were filled with an internal solution containing (in mM): 0.2 EGTA, 3 MgCl2, 10 HEPES, 8 NaCl, 4 Mg-ATP, and 0.5 Na-GTP. Recording pipette resistances were 2–4 MΩ. Electrophysiology records were acquired using a dPatch amplifier and SutterPatch acquisition software (Sutter Instrument). Evoked action potential recordings were taken following gap-free spontaneous firing recordings. For evoked firing protocols, cells were initially injected with a −0.5 nA hyperpolarizing current for 100 ms before stepping current injections to 0, 0.1, 0.2, and 0.3 nA for 700 ms. Input resistance and capacitance measurements were obtained from a whole-cell voltage-clamp recording in which membrane voltage was stepped from a −80 mV holding potential to −90 mV (for 100 ms) and to −70 mV (for 100 ms) in a subsequent sweep. Membrane capacitance was calculated by integrating the area of fast capacitive transient current during voltage steps and dividing by the change in membrane voltage (10 mV). Electrophysiology recordings were analyzed using SutterPatch (Sutter Instrument), Microsoft Excel (Microsoft), and Prism (GraphPad) software.
2.4 Behavioral tests
Behavioral tests were conducted on male and female mice in cohorts of animals that were 9–11 weeks of age and repeated on animals in the Tsc1mut/mut and control cohorts at 16–24 weeks of age. A single experimenter performed all behavioral tests for each age group. Age ranges reflect the amount of time necessary for the individual experimenter to complete these behavioral tests. For each age group, elevated balance beam tests were completed before social interaction tests. All tests were performed during the light cycle between 9:00 and 17:00.
2.4.1 Elevated balance beam
Motor coordination and balance were tested using the elevated balance beam previously described in Carter et al. (2001). The balance beam apparatus consists of an 80 cm-long flat beam 10 mm in width. A second flat beam, 8 mm in width, was also used in this assay and results from the 8 mm beam testing are reported in Supplementary Figures 1, 2. During testing, animals traversed the narrow beam to reach an enclosed goal platform which was covered by an opaque plastic goal chamber. As an incentive, food was placed inside the goal chamber. Mice were trained to cross each beam for three consecutive days prior to testing. Each training session included 2 min in which each animal explored the initial starting area of the beam, 2 min to explore the goal chamber, and a single crossing of each (10 mm and 8 mm) balance beam. After training, mice were tested individually on four consecutive days. Crossing the beam was timed from the moment the animal’s hind limbs crossed the starting line (80 cm from the goal chamber) until the animal’s hind limbs crossed entrance of the goal chamber. The time to cross the beam and the number of hindlimb foot-slips while crossing the beam were recorded and used to assess differences in fine motor coordination and balance across sex and genotype.
2.4.2 Social behavior
Mice were tested for deficits in social interactions using the three-chamber social interaction task apparatus (Yang et al., 2011). The three-chamber apparatus consists of three openly connected chambers constructed of clear plexiglass. Each chamber is 20 cm by 40 cm and has 22 cm high (plexiglass) walls. Each of the two exterior chambers contained an inverted wire cup capable of holding an adult mouse comfortably. A plastic cup was placed on top of the wire cup to prevent climbing. Mice were allowed to acclimate to the testing room for 30 min in their home cage prior to testing. The test mouse was placed in the center chamber and allowed to explore the center chamber for 7 min to acclimate to the chamber environment. The assays were then conducted in three phases, each 10 min in length: an adaptation phase during which the mouse was allowed to freely roam all three chambers; a social approach phase during which one exterior chamber contained an empty wire cup and the other exterior chamber contained a cup holding an unfamiliar ‘stranger’ mouse; and a social novelty phase during which one exterior chamber contained the ‘stranger’ mouse from Session I (now considered familiar) and the previously empty chamber contained a new, unfamiliar stranger mouse. ‘Stranger’ mice were animals at least 6 weeks old and within 2 months of the age of the test mouse, the same sex as the test mouse, and Cre-negative. These animals underwent two 15-min training sessions prior to being used in testing as a ‘stranger’ to observe for any potentially disruptive behaviors. In all three sessions, the test mouse’s number of entries into each chamber, marked by the full crossing of the hind legs into the chamber, and the time spent in each chamber were scored by an observer with a stopwatch. The total number of direct contacts and the total time in direct contact with the unfamiliar object, unfamiliar mouse, and familiar mouse were also scored by the experimenter using a video recording of the session. For social approach testing, the percentage of time investigating the unfamiliar animal was calculated by dividing the total time the test animal interacted with the unfamiliar animal/cage by the sum of interaction times with the unfamiliar animal/cage and the novel object/empty cage × 100. For social novelty testing, the percentage of time investigating the unfamiliar animal was calculated by dividing the total time the test animal interacted with the unfamiliar animal/cage by the sum of interaction times with the unfamiliar animal/cage and the familiar animal/cage × 100. Direct contacts were defined as instances of 1 s or more of direct sniffing or paw-touching of the wire cage. The apparatus and both wire cages were cleaned with 70% ethanol and water between test subjects.
2.5 Statistical analysis
Statistical analyses were completed using GraphPad Prism 8 software (GraphPad Software Inc., La Jolla, CA, USA). Data from whole cell electrophysiology recording measurements were compared across sexes and genotypes using unpaired Student’s t-tests. Data from elevated balance beam experiments were compared across sexes and genotypes using repeated measures (RM) two-way ANOVA tests. Social interaction experiment results were assessed using two-way ANOVA tests; comparisons of the percent of time investigating the unfamiliar animal used sex and genotype as between-subject factors, and comparisons of the number of direct contacts used sex and chamber (containing stranger animal or containing empty cage) as between-subject factors within each genotype. Šídák’s corrections were applied in multiple comparisons tests. p < 0.05 was considered to be statistically significant. p-values for all statistical tests comparing behavioral performance measures across sex are reported in Supplementary Table 1 for the 9–11 week animal cohorts, and in Supplementary Table 2 for the 16–24 week-old animal cohorts.
3 Results
3.1 Tsc1 deletion in Purkinje neurons attenuates repetitive firing
A Cre-LoxP recombination strategy was used to selectively delete Tsc1 from mouse cerebellar Purkinje neurons. Tsc1 mutant mice with loxP sites flanking exons 17 and 18 of the Tsc1 gene (Kwiatkowski et al., 2002; Jackson laboratory, strain # 005680) were crossed with a transgenic mouse strain that expresses Cre recombinase under the control of the mouse Purkinje cell-specific L7 promoter (Jackson laboratory, strain # 010536; Figure 1A). Progeny from this cross included hemizygous Cre-positive animals with either heterozygous or homozygous Tsc1 floxed alleles, used as test group animals, and Cre-negative animals. Cre-negative animals with homozygous Tsc1 floxed alleles were used as control group animals. To verify selective Cre expression in cerebellar Purkinje neurons, hemizygous Cre-positive animals were also crossed with the Ai14 Cre reporter tool strain (Jackson Laboratory, strain # 007914; Figure 1A), which express tdTomato fluorescence following Cre-mediated recombination (Madisen et al., 2010). Parasagittal cerebellar sections from the Cre-positive progeny of this cross revealed robust tdTomato expression that was selective to Purkinje neurons in the cerebellum (Figure 1B) of both male and female animals (see Supplementary Figure 3). Cre-expressing Purkinje neurons were identified in both adult (Figure 1B) as well as neonatal (P16) animals. In these sections, no tdTomato fluorescence was identified in cells outside the Purkinje neuron layer.
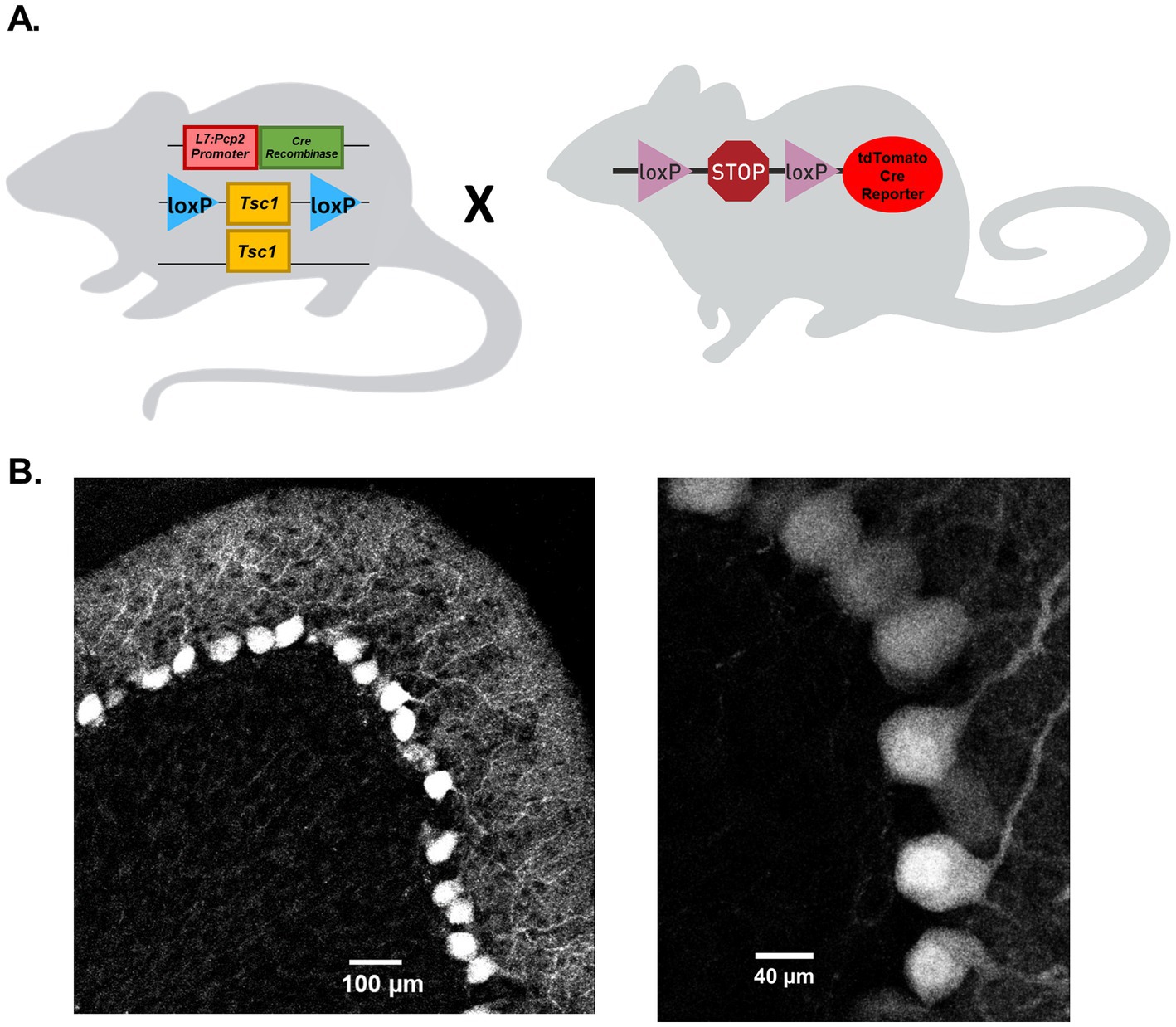
Figure 1. Cre recombinase expression on the L7-Pcp2 promoter drives selective Tsc1 deletion in mouse cerebellar Purkinje neurons. (A) Depicts the breeding strategy for Purkinje neuron-specific Tsc1 deletion. Heterozygous or homozygous animals with loxP sites flanking Tsc1 were crossed with mice hemizygous for the Cre recombinase gene under control of a L7-Pcp2 promoter. To verify the Purkinje neuron-specific expression of Cre recombinase, Cre-positive animals were crossed with a Cre reporter strain in which a loxP-flanked STOP cassette prevents expression of a CAG promoter-driven tdTomato gene. In (B), 20x (left) and 63x (right) confocal images of 25 μm parasagittal cerebellar sections were taken from adult tdTomato Cre-reporter mice expressing Cre on the L7-Pcp2 promotor.
Targeted deletion of Tsc1 from male (mouse) cerebellar Purkinje neurons has previously been shown to result in attenuated repetitive firing in adult Purkinje neurons (Tsai et al., 2012). To determine if Cre-positive Tsc1flox/flox progeny (described above), referred to here as Tsc1mut/mut, have similar deficits in repetitive firing, and to test if changes in membrane excitability is conserved across Tsc1mut/mut Purkinje neurons from male and female animals, we performed whole cell patch current-clamp recordings from adult (5–8 week-old) Tsc1mut/mut and wild type Purkinje neurons in acutely isolated cerebellar sections (see Methods). These recordings were performed at near physiological temperatures (33–34°C) and revealed the spontaneous repetitive firing frequency in Tsc1mut/mut Purkinje neurons is significantly (p < 0.001, Student’s t-test) reduced compared to age-matched wild type cells (see Figures 2A,B). Reduced repetitive firing frequencies measured in Tsc1mut/mut Purkinje neurons were not, however, significantly different between cells isolated from male and female mice (Figure 2C). Depolarizing current injections after a common hyperpolarizing (−0.5 nA) current step revealed similar impaired evoked firing in Tsc1mut/mut Purkinje neurons, and no difference between male and female Tsc1mut/mut Purkinje neurons (Figures 2D,E). Measures of membrane capacitance were also not significantly different across male and female Tsc1mut/mut Purkinje neurons, however, membrane input resistance was found to be significantly (p = 0.02, Student’s t-test) lower in male Tsc1mut/mut Purkinje neurons compared to cells from Tsc1mut/mut females (Figure 2F). No sex-specific differences in capacitance and input resistance were measured in wild type controls cells (data not shown).
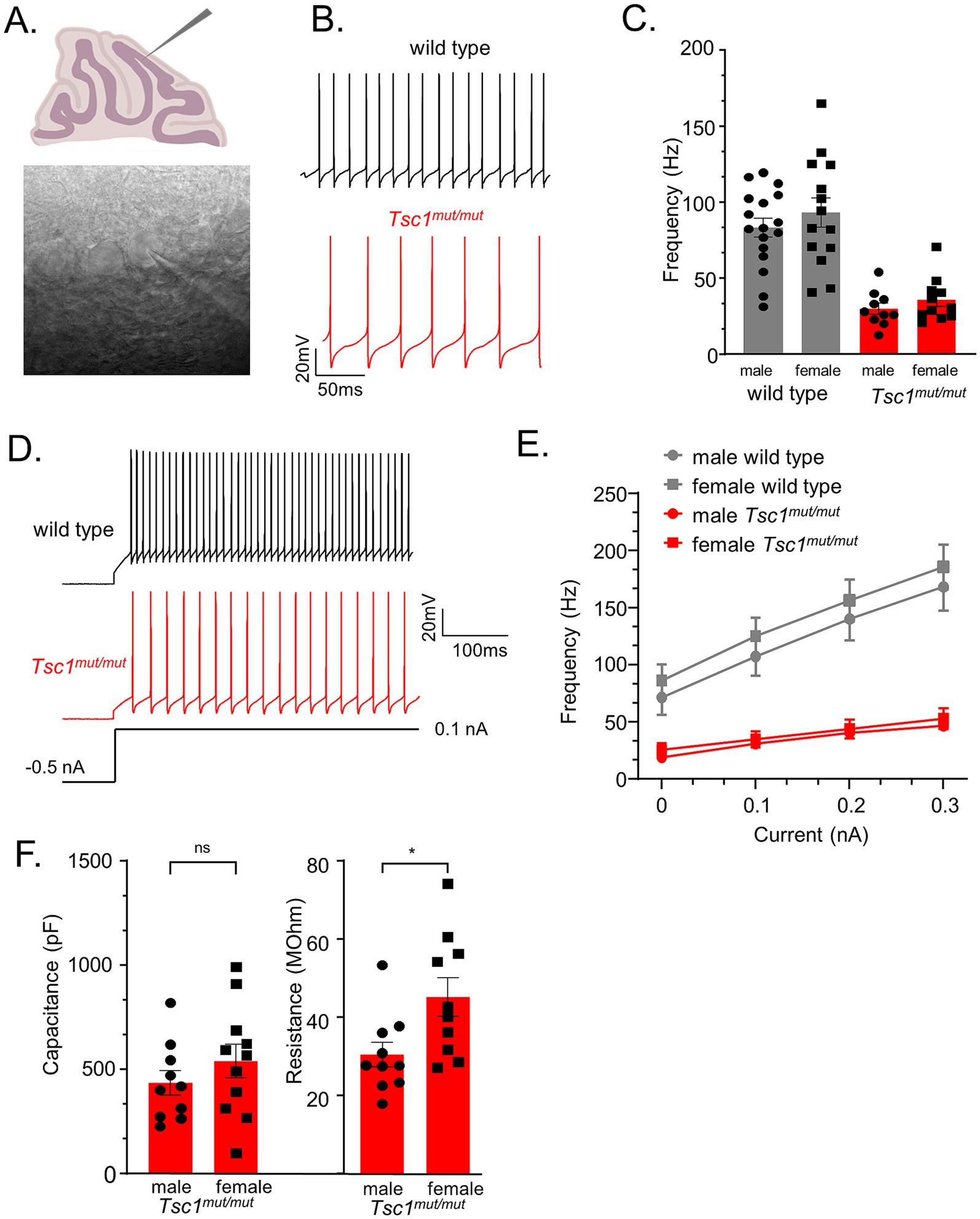
Figure 2. Tsc1 deletion results in reduced membrane excitability in Purkinje neurons from male and female mice. (A) 350 μm parasagittal cerebellar sections were acutely isolated for whole cell patch-clamp recordings. In panel (B), representative simple spike activity is presented from wild type and Tsc1mu/mut adult Purkinje neurons. (C) In Tsc1mut/mut Purkinje neurons, mean ± SEM spontaneous firing frequency is significantly lower than wild type Purkinje neurons. Within each genotype, mean ± SEM firing frequency is not significantly different across sex. Evoked firing was measured during 0.1–0.3 nA depolarizing current injections after a common −0.5 nA hyperpolarizing step (D). (E) Compared to wild type controls, mean ± SEM evoked firing frequencies were significantly lower in Tsc1mut/mut cells, and not different across male and female cells from either genotype. (F) Mean ± SEM measures of capacitance were not significantly different across male and female Tsc1mut/mut cells. Mean input resistance was determined to be significantly lower in male Tsc1mut/mut Purkinje neurons. Input resistance and capacitance did not differ between wild type male and female Purkinje neurons (data not shown).
3.2 Tsc1 deletion from Purkinje neurons impairs motor coordination similarly in males and females
To examine potential sex-specific differences in motor coordination and balance resulting from the targeted deletion of Tsc1 in Purkinje neurons, the elevated balance beam behavioral assay (Carter et al., 2001) was performed on 9–11 week-old and 16–24 week-old mice. For each age-group, the balance beam test was performed across four consecutive testing days. The amount of time it took the animal to traverse a 10 mm wide beam (see Figure 3A), and the number of hindlimb foot-slips while traversing the beam (see Methods) were recorded. The mean (±SEM) time to cross and hindlimb foot-slips are plotted in Figure 3 for each of the four testing days. Results for the testing of 9–11 week-old animals are plotted in Figures 3B,C, and again for the 16–24 week-old age group in Figures 3D,E. These data revealed that at both ages (9–11 week-old and 16–24 week-old) Tsc1mut/mut animals have impaired motor performance compared to control (Tsc1flox/flox; Cre -) mice, taking significantly (Figures 3B,D; p < 0.0001, RM two-way ANOVA) longer to cross the 10 mm balance beam and having significantly (Figures 3C,E; p < 0.0001, RM two-way ANOVA) more hindlimb foot-slips while traversing the 10 mm beam. Animals with targeted deletion of a single Tsc1 allele (Tsc1mut/wt) performed similarly to control animals (p = 0.9866, RM two-way ANOVA, Figure 3B). For all genotypes tested on the 10 mm balance beam, no significant differences were recorded across sex (within a genotype). These data suggest there are no significant differences between sexes in ASD-associated deficits in fine motor coordination and balance. This testing paradigm was also performed using a more challenging, 8 mm balance beam, and similar to the 10 mm balance beam, revealed no significant differences across sex within a given genotype (see Supplementary Figures 1, 2).
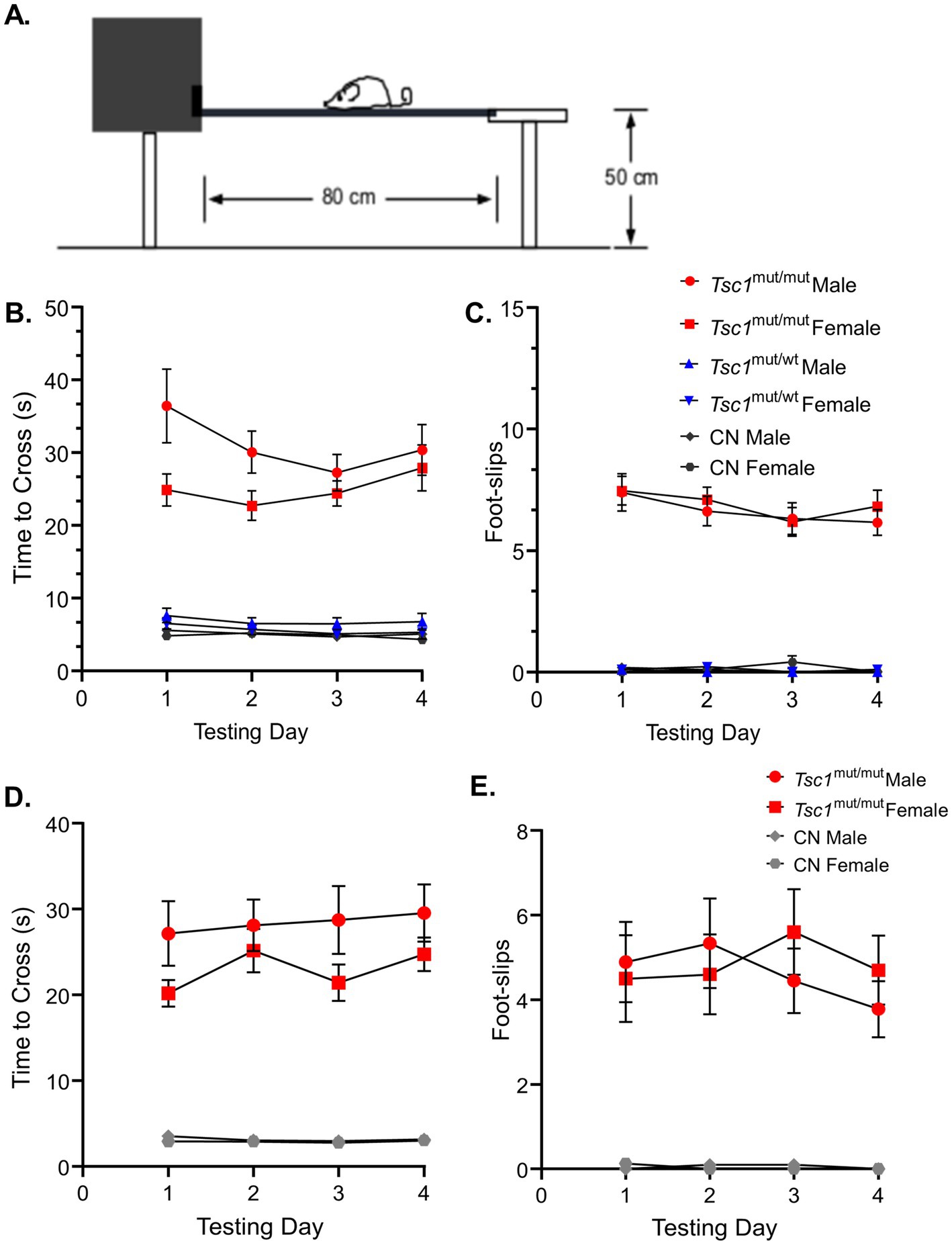
Figure 3. No sex-specific differences were found in performance on the 10 mm elevated balance beam in 9–11 week-old and 16–24 week-old mice. (A) A cartoon depiction of the elevated balance beam testing setup is shown. (B) 9–11 week-old Tsc1mut/mut (N = 13 males and N = 11 females), Tsc1mut/wt (N = 10 males and N = 10 females), and control (CN, N = 11 males and N = 10 females) mice were tested on a 10 mm (width) elevated balance beam. Across the four testing days, the mean time to cross of Tsc1mut/mut mice (of both sexes) took significantly (p < 0.0001, RM two-way ANOVA) longer to cross than Tsc1mut/wt and control mice. (C) The same was true for the number of hindlimb foot-slips (p < 0.0001, RM two-way ANOVA). Tsc1mut/wt and control cohorts performed similarly. Within each genotype, male and female animals performed similarly across the four testing days. Mean ± SEM values are plotted. Mean ± SEM results are plotted for 16–24 week-old Tsc1mut/mut (N = 9 males and N = 10 females) and control (N = 10 males and N = 8 females) mice on the 10 mm (width) elevated balance beam in D-E. Within each genotype, male and female animals performed similarly across the four testing days. (D) Tsc1mut/mut mice took significantly longer to cross (p < 0.001, RM two-way ANOVA) than CN mice across testing days. (E) Tsc1mut/mut mice had significantly more hindlimb foot-slips (p < 0.001, RM two-way ANOVA) than CN mice across testing days.
3.3 Social approach behavior is differentially affected in male and female Tsc1mut/mut mice
A three-chamber apparatus was used to measure differences between sexes in impairments for social approach preference and social novelty preference in Tsc1mut/mut mice. The three-chamber apparatus consisted of an empty center chamber and two exterior chambers with wire cups large enough to comfortably hold an adult mouse. In this assay, preference for social approach is reflected as an increase in the investigation of an unfamiliar animal (in one of the wire cups) compared to the alternate empty wire cup (Figure 4A). In Figure 4B, the mean (±SEM) percentage values for the time spent investigating the unfamiliar mouse, as a percentage of the total time spent investigating the unfamiliar mouse and the empty wire cup, are plotted across sex and genotype for 9–11 week-old cohorts. Results from these tests reveal Tsc1mut/mut mice spend a significantly (p < 0.05, two-way ANOVA) lesser percentage of time investigating the unfamiliar animal compared to the control (Tsc1flox/flox/Cre-negative) cohort. In this analysis, animals with targeted deletion of one Tsc1 allele (Tsc1mut/wt) were not significantly different from Tsc1mut/mut or control animal cohorts. Similar to the results from the elevated balance beam assay, within each tested genotype (Tsc1mut/mut, Tsc1mut/wt, and control), we found no significant difference in these values based on sex (p = 0.2681, two-way ANOVA, Supplementary Table 1).
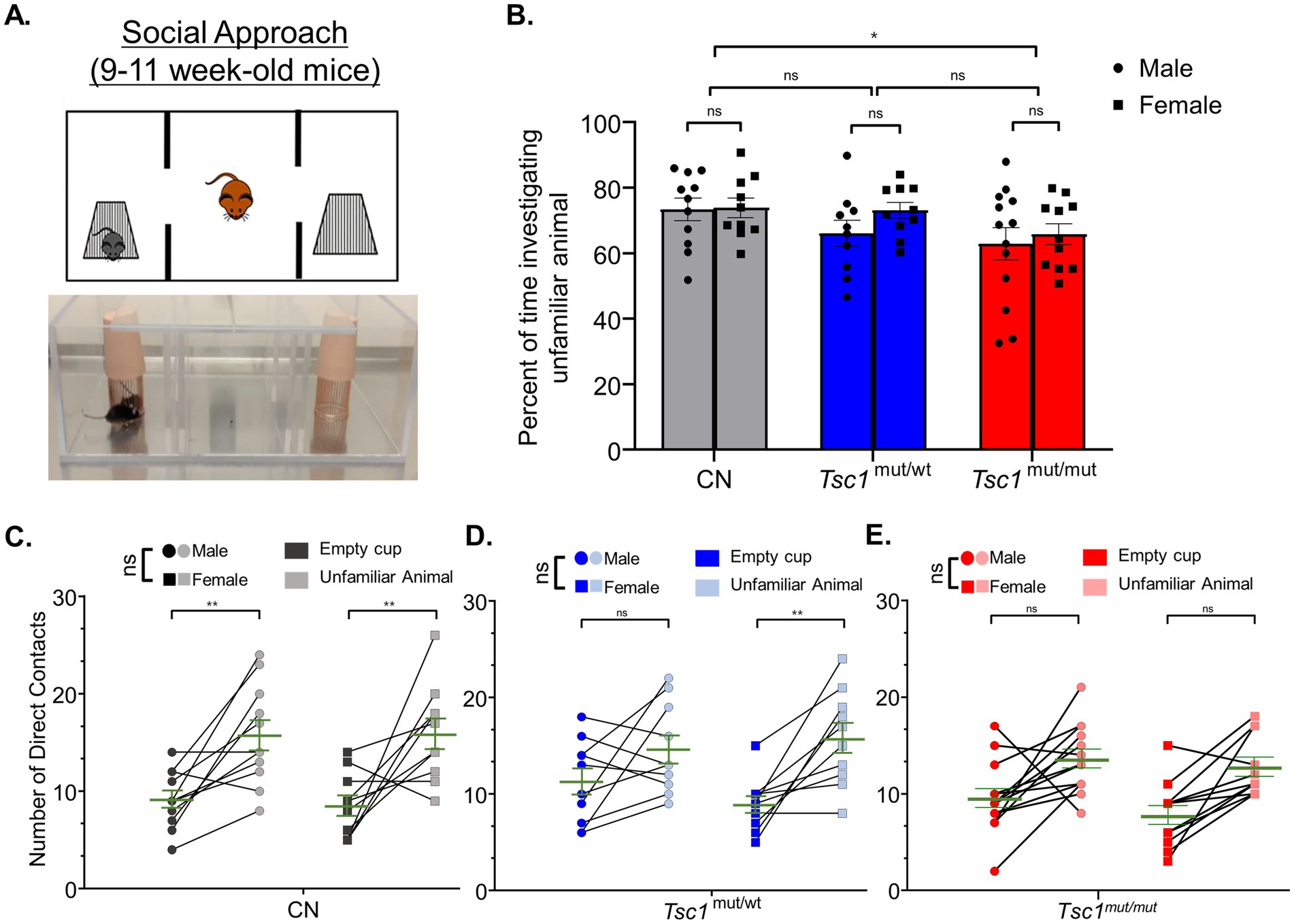
Figure 4. Social approach deficits are similar across males and females in control and Tsc1 mutant animals. 9–11 week-old male and female Tsc1mut/mut (N = 13 males and N = 11 females), Tsc1mut/wt (N = 10 males and N = 10 females), and control (CN, N = 11 males and N = 10 females) mice were tested for social approach preference using the three-chamber apparatus depicted in in panel (A). Photograph and cartoon depictions show the test animal, unfamiliar stranger mouse (in the left chamber), and empty wire cup (in the right chamber). (B) Tsc1mut/mut mice spent a significantly (p < 0.05, two-way ANOVA) smaller proportion of time investigating the unfamiliar animal compared to Tsc1mut/wt and control mice. No differences were recorded between sexes. In panels (C–E), the number of contacts with the empty cup and the unfamiliar animal is plotted for each test mouse for males and females of each genotype. Within each genotype, no significant difference was measured across sex for preference for contact with the unfamiliar animal. Individual values are plotted with the mean ± SEM shown in green (ns, not significant, *p < 0.05, **p < 0.01, Šídák’s corrected multiple comparisons tests used in two-way ANOVAs).
As another method to quantify the performance on the social approach behavioral test, the number of the test mouse’s direct contacts with the cage holding the unfamiliar animal and the number of direct contacts with the empty cage are plotted in Figures 4C–E, across sexes, for Tsc1mut/mut, Tsc1mut/wt, and control genotypes, respectively. These data reveal control mice of both sexes exhibit social approach preference (p < 0.01, two-way ANOVA, Figure 4C), having a higher number of direct contacts with the unfamiliar animal compared to the empty cage. Interestingly, while male Tsc1mut/mut and Tsc1mut/wt mice did not exhibit significant preference for the unfamiliar animal over the empty cage (Tsc1mut/mut: p = 0.1627, Tsc1mut/wt: p = 0.1065, two-way ANOVA, Figures 4D,E), indicating impaired social approach behavior in these genotypes, female Tsc1mut/wt mice did exhibit significant preference for the unfamiliar animal (p < 0.01, two-way ANOVA, Figure 4D) similar to control animals; a phenotype not shared by Tsc1mut/wt males.
In older (16–24 week-old) animals, however, the social approach testing paradigm revealed a clear difference between sexes in Tsc1mut/mut mice (Figures 5A,B). Male Tsc1mut/mut mice spent a significantly smaller proportion of time than female test animals investigating the unfamiliar mouse (mean difference: -18.43 ± 5.1, p < 0.01, N = 8 males and N = 9 females, two-way ANOVA, Figure 5B). No difference between sexes was measured in control mice (p = 0.99, N = 8 males and N = 6 females, two-way ANOVA, Figure 5B). In Figures 5C,D, the number of the test mouse’s direct contacts with the cage holding the unfamiliar animal versus the empty cage are plotted between sexes for control and Tsc1mut/mut animals, respectively. Consistent with the results presented in Figure 5B, control test mice from both sexes exhibited a significant preference for contacts with the unfamiliar animal compared to the empty cage (p = <0.0001, two-way ANOVA, Figure 5C). In control mice, we measured no significant difference in preference between males and females (p = 0.1123, two-way ANOVA, Figure 5C). Conversely, male Tsc1mut/mut mice exhibited a significantly (p = 0.0117, two-way ANOVA, Figure 5D) lesser preference for contacting the unfamiliar animal compared to female Tsc1mut/mut mice. Differences in the number of contacts (with the empty cage or with the unfamiliar mouse cage) may be driven by sex-specific differences in the total time spent in each chamber. We explored this possibility by analyzing the total time spent in each chamber for male and female animals across genotypes during the social approach and social novelty tasks (see Supplementary Figures 4, 5). These data reveal that in older (16–24 week-old) control and Tsc1mut/mut cohorts, male and female mice did not significantly differ in the amounts of time in each chamber.
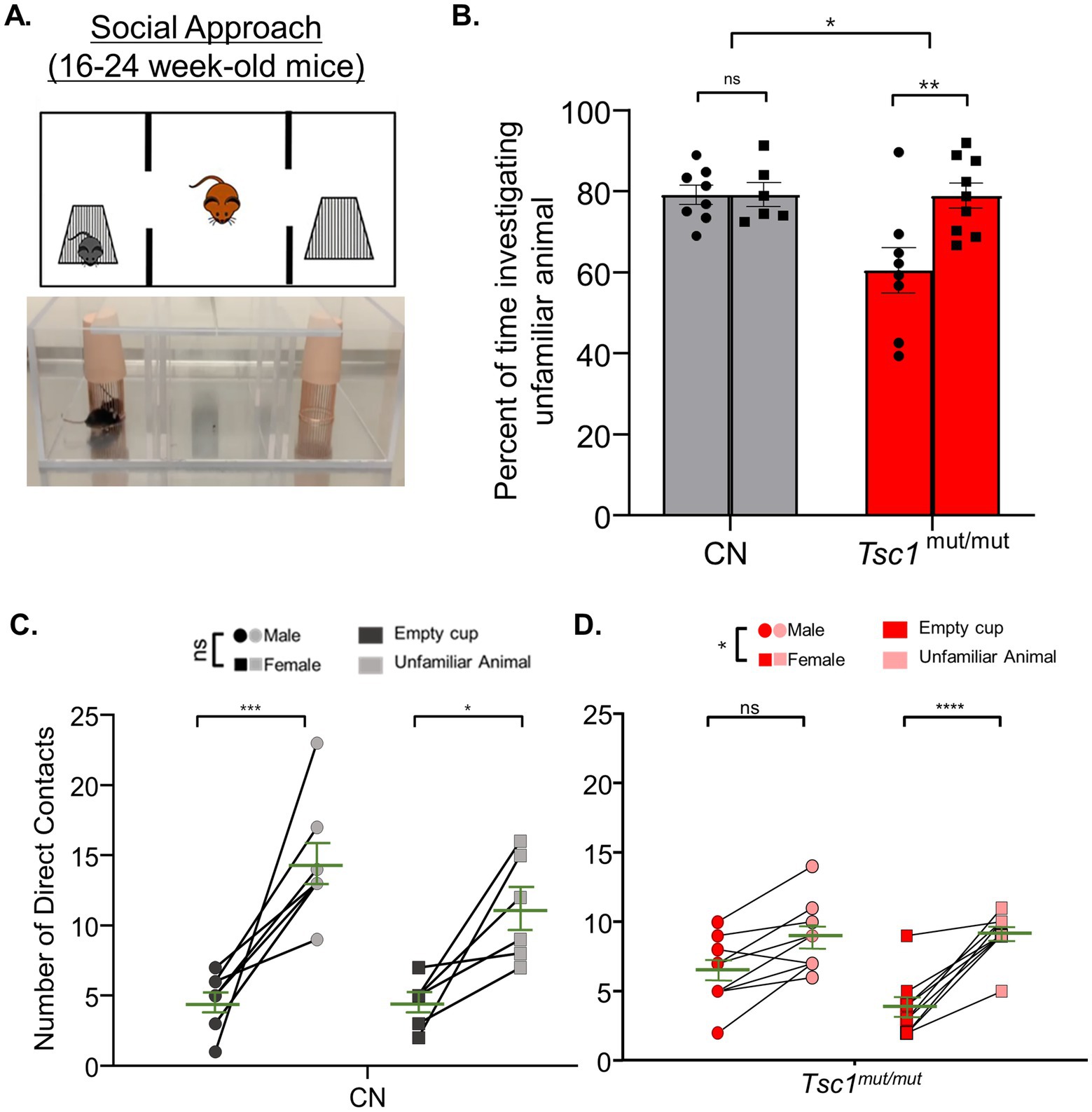
Figure 5. In 16–24 week-old Tsc1mut/mut mice, social approach deficits are more severe in males compared to females. 16–24 week-old male and female Tsc1mut/mut (N = 8 males and N = 9 females) and control (CN, N = 8 males and N = 6 females) mice were tested using the three-chamber apparatus for social approach preference depicted in panel (A,B). Tsc1mut/mut mice spent a significantly (p < 0.05, two-way ANOVA) lesser percentage of time investigating the unfamiliar animal compared to controls. Male Tsc1mut/mut mice spent a significantly (p < 0.05, two-way ANOVA) lesser percentage of time investigating the unfamiliar animal compared to Tsc1mut/mut females. In panels (C,D), the number of contacts with the empty cup and the unfamiliar animal is plotted for male and female test mice of control and Tsc1mut/mut genotypes. Male Tsc1mut/mut mice showed a significantly (p < 0.05, two-way ANOVA) lesser preference for contact with the unfamiliar animal than Tsc1mut/mut females. No difference between sexes was recorded in the control group. Individual values are plotted with the mean ± SEM shown in green (ns, not significant, *p < 0.05, **p < 0.01, ***p < 0.001; ****p < 0.0001, Šídák’s corrected multiple comparison tests used in two-way ANOVAs).
3.4 Sex specific differences in social approach behaviors were identified in both control and Tsc1mut/mut mice
To test for a preference for social novelty across sexes, the three-chamber apparatus was used to measure the preference of test animals for investigating an unfamiliar animal (in one chamber) compared to a familiar animal (in the alternative chamber). An image and cartoon depiction of this testing paradigm is presented in Figure 6A. Social novelty preference is measured as the preference for investigating the unfamiliar animal compared to the familiar animal. These tests were performed directly after social approach testing (see Methods). Also similar to our previous behavioral tests, in 9–11 week-old animals, a cohort of Tsc1mut/wt animals was also tested. Interestingly, in these social novelty preference tests, we measured no significant difference in preference for investigating the unfamiliar animal across (9–11 week-old) control, Tsc1mut/wt, and Tsc1mut/mut genotypes (p = 0.2972, two-way ANOVA, Figure 6B). This is in contrast to previous findings in which Tsc1 mutant mice were found to exhibit no preference for investigating the socially novel animal (Tsai et al., 2012). Interestingly, within each genotype, control and Tsc1mut/wt genotypes were found to have significant differences in social novelty preference based on sex. For instance, in control mice, female test animals investigated the unfamiliar animal a significantly lower percentage of time compared to male test animals (mean difference: 18.9 ± 5.8, p = 0.0055, two-way ANOVA, Figure 6B). These differences in preference based on sex were not, however, measured in the Tsc1mut/mut cohort (p = 0.9986, two-way ANOVA, Figure 6B).
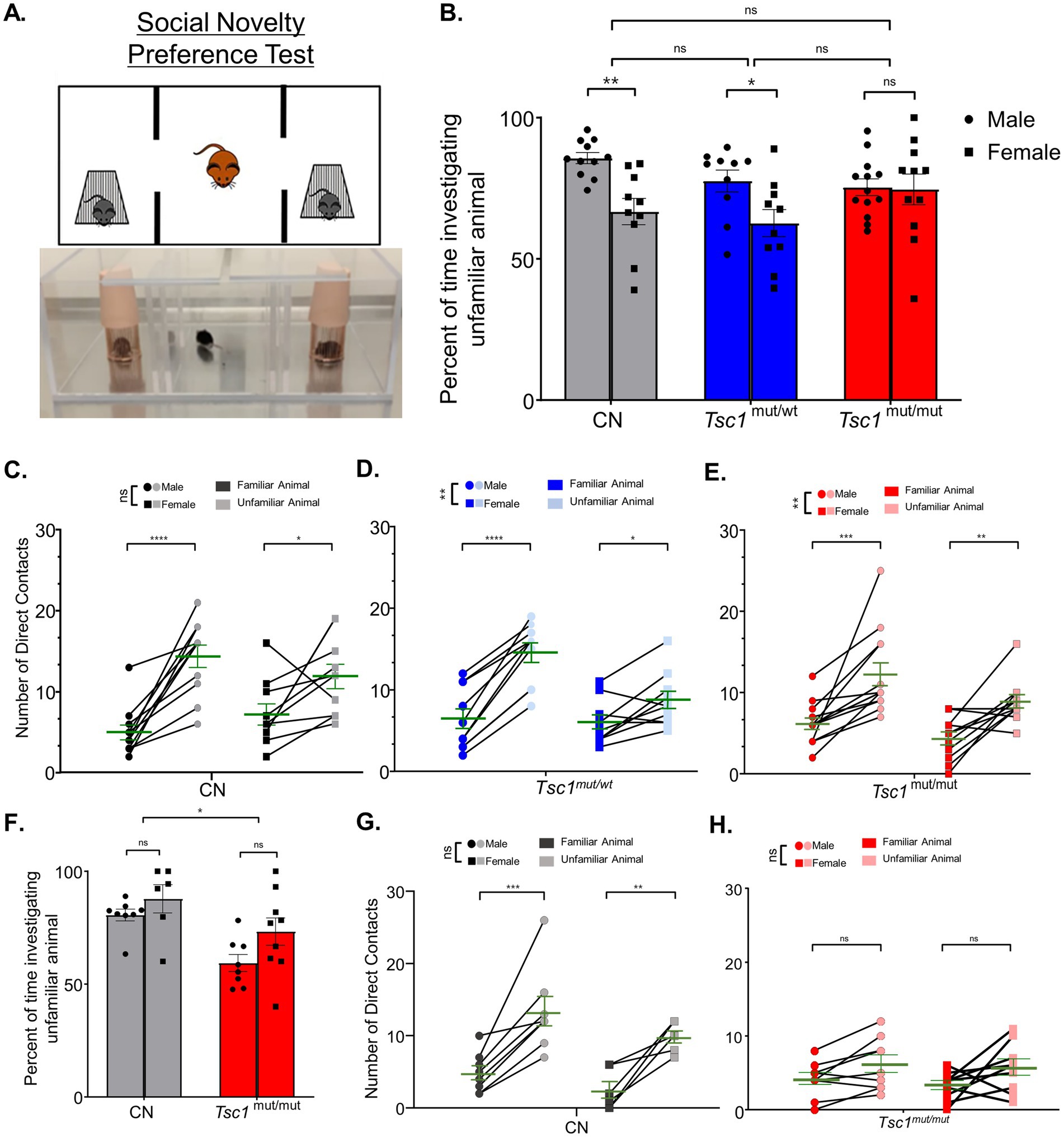
Figure 6. Sex-specific differences in social novelty preference occur in Tsc1 mutant and control groups. 9–11 week-old male and female Tsc1mut/mut (N = 13 males and N = 11 females), Tsc1mut/wt (N = 10 males and N = 10 females), and control (CN, N = 11 males and N = 10 females) mice were tested for preference for social novelty using the three-chamber apparatus depicted in panel (A). In the photograph and cartoon depiction, the test mouse in the center chamber is free to interact with an unfamiliar or familiar animal in the two side chambers. Panels B-E. show results for 9–11 week-old animal testing and panels (F–H). show results for 16–24 week-old animal tests. (B) Female control and Tsc1mut/wt mice investigated the unfamiliar animal a significantly lesser percentage of time (CN: p < 0.001, two-way ANOVA, Tsc1mut/wt: p < 0.01, two-way ANOVA) compared to males. No significant differences were measured between genotypes. (C) For the Tsc1mut/mut and Tsc1mut/wt groups, females had significantly (p < 0.01, two-way ANOVA) lower preference for direct contact with the unfamiliar animal compared to males. Mean ± SEM values are plotted in (B). In panel (C), individual values are plotted with the mean ± SEM shown in green (ns, not significant, *p < 0.05, **p < 0.01, *** p < 0.001; ****p < 0.0001, Šídák’s corrected multiple comparison tests used in two-way ANOVAs). (F) In 16–24 week-old Tsc1mut/mut (N = 8 males and N = 9 females) and control (N = 8 males and N = 6 females) cohorts, Tsc1mut/mut mice spent significantly (p < 0.05, two-way ANOVA) less time investigating the unfamiliar mouse than controls. (G,H) Tsc1mut/mut mice had no preference for direct contact with the familiar mouse. No differences between sex were measured in control (G) or Tsc1mut/mut (H) animals. Mean ± SEM values are plotted in F. In panel G-H., individual values are plotted with the mean ± SEM shown in green (ns, not significant, * p < 0.05, ** p < 0.01, *** p < 0.001, Šídák’s corrected multiple comparison tests used in two-way ANOVAs).
Interestingly, when number of cage contacts was used to assess social novelty preference in the 9–11 week-old animals, we measured no sex-specific differences in control animals (p = 0.9222, two-way ANOVA, Figure 6C), however, in Tsc1mut/wt (Figure 6D) and Tsc1mut/mut (Figure 6E) cohorts, there were significant differences based on sex, with females in both Tsc1mut/wt and Tsc1mut/mut cohorts contacting the unfamiliar animal cage less than males of the respective cohort (Figures 6D,E). Together, these data suggest female test animals, from all genotypes tested, exhibit a lower preference for social novelty compared to male test animals; importantly, this sex-specific difference was measured in control animals, which makes assessing sex-specific effects of Tsc1 deletion difficult. This difference between sexes was not found to be present in the Tsc1mut/mut group. A previous study which compared social behavioral phenotypes of prairie voles to C57BL/6 mice also identified sex-specific differences in wild type C57BL/6 mice social novelty preference (Wu et al., 2022). Similar to the results presented here, Wu et al. (2022) determined male mice exhibit significantly greater social novelty preference (Figure 6B) compared to female mice.
Social novelty performance was assessed again in 16–24 week old control and Tsc1mut/mut cohorts, with results presented in Figures 6F–H. 16–24 week-old Tsc1mut/mut mice spent a significantly smaller percentage of time investigating the unfamiliar animal than control mice (p = 0.0438, two-way ANOVA, Figure 6F), revealing a deficit in typical (control) social novelty preference. No significant differences between male and female test animals of either genotype were measured (control-p = 0.5644, Tsc1mut/mut-p = 0.0917, two-way ANOVA, Figures 6G,H). Male and female control mice exhibited a significant preference for contacting the socially novel animal (p < 0.0001, two-way ANOVA, Figure 6G), and, in contrast to the results from the 9–11 week-old cohorts, Tsc1mut/mut mice did not exhibit preference for the unfamiliar mouse based on contact number (males: p = 0.1889, females: 0.0942, two-way ANOVA, Figure 6H). These data suggest older (16–24 week-old) Tsc1mut/mut develop an impairment in social novelty preference that is not significant at younger (9–11 week-old) ages.
4 Discussion
Studies investigating sexual dimorphism in ASD symptoms in humans often result in inconclusive or contradictory findings. For example, there are reported sex differences in repetitive behaviors (Tillmann et al., 2018) and in fine motor control and social communication abilities (Craig et al., 2020), however, other investigations found no significant differences in these symptom domains across sexes (Andersson et al., 2013; Reinhardt et al., 2015). This emphasizes the need for a more targeted study of ASD symptomatology across sexes in animal models in which the underlying cell-and circuit-level drivers of behavioral phenotypes are well-established. The use of animal models to investigate ASD sex differences has only recently garnered increased interest; previous preclinical animal studies often only used males to avoid physiological variability linked to the estrous cycle of female rodents (Jeon et al., 2018). Interestingly, however, a study on of the effect of the female estrous cycle on behavioral testing, including social interaction assays, found very few parameters with significant differences between estrus and diestrus female mice (Zeng et al., 2023).
Here, we tested if the selective deletion of Tsc1 in cerebellar Purkinje neurons differentially affects Purkinje neuron intrinsic excitability, as well as ASD-related behaviors in male and female mice. We used L7-Pcp2:Cre/LoxP recombination to selectively delete Tsc1 in Purkinje neurons. While selective for cerebellar Purkinje neurons, Cre-recombinase expression driven by the L7-Pcp2 promotor has not been shown to occur differentially across sex. For instance, Jahncke and Wright (2024) recently characterized the timeline of Cre-recombinase expression in L7-Pcp2 Cre-positive mice using both male and female animals. In this study, the authors did not note or discuss any sex-specific differences in Cre-recombinase expression, although a sex-specific comparison was not presented in the results. In a mutant mouse model with inactivation of the Pcp2 allele, sex-specific effects were measured in anxiety behaviors (Iscru et al., 2009), however, these phenotypes were not linked to sex-specific differences in Pcp2 expression. Using crosses with the Ai14 Cre-reporter line, we determined Cre-recombinase expression is robust and specific to the Purkinje neuron layer of both male and female L7-Pcp2 Cre-positive animals (Supplementary Figure 3). There remains a possibility that there are sex-specific differences in the expression of Purkinje neuron specific Cre-recombinase and/or the frequency of Cre-mediated recombination throughout cerebellar circuits. However, the finding that impairments in repetitive firing (Figure 2) and balance and motor coordination (Figure 3) are similar in male and female Tsc1mut/mut mice suggests Purkinje neuron specific Tsc1 deletion occurs similarly across sex. We tested animals in two (9–11 week-old and 16–24 week-old) age groups for social interaction and balance and motor coordination behaviors. Results from these studies revealed more severe deficits in older (16–24 week-old) male, compared to female, Tsc1mut/mut mice. These differences were measured using the 3-chamber social interaction assay. Measurements in this assay, which are typical for quantifying social approach and social novelty behaviors (Nadler et al., 2004), could be affected by differences in animal aggression, exploratory behavior or reactions to new environments. In future work, it will be useful to assess sociability in a home cage environment (Kim et al., 2019), which may reduce these potential confounds.
The effect of sex on social approach behavior (Figure 5) is similar to the more severe social interaction deficits identified in males (compared to females) of a Shank3 mutant mouse model of ASD (Matas et al., 2021), although this mutant model relied on a global mutation, making it difficult to confidently attribute the sexual dimorphism to an effect on Purkinje neurons. Interestingly, we measured no significant differences between Tsc1mut/mut males and females on the elevated balance beam assay in both age groups, suggesting Purkinje neuron-specific Tsc1 deletion affects cerebellar circuits that contribute to somatosensory function similarly across males and females; and conversely, in cerebellar circuits that contribute to social interaction behaviors, the selective deletion of Tsc1 has a more severe effect in males compared to females.
4.1 Cell and circuit etiology of Tsc1mut/mut behavioral impairments
Numerous ASD-linked behavioral deficits were originally identified in (male) Tsc1mut/mut mice and these phenotypes were linked to two types of cerebellar impairment. In older (>10 week-old) Tsc1mut/mut animals, Tsai et al. (2012) measured reduced numbers of Purkinje neurons in the cerebellar vermis, as well as a significant increase in the proportion of Purkinje neurons expressing apoptotic factors TUNEL and Caspase 9. No changes in Purkinje neuron number were measured in younger (6 week-old) animals, however, there were marked deficits in Purkinje neuron repetitive firing properties (Tsai et al., 2012), similar to the results reported here (Figure 2). Because impaired behaviors were also recorded in younger (~6 week-old animals) it’s likely that Tsc1 deletion causes cerebellar circuit deficits through both impaired Purkinje neuron excitability, and, as the animals become older, by way of Purkinje neuron apoptosis. The stand-out result from the current investigation is that in older Tsc1mut/mut animals, impaired social interaction behavior (social approach preference) is more severe in Tsc1mut/mut males compared to females, and importantly, this sex-specific difference is not present in behaviors related to balance and motor coordination. This result indicates the sex-specific impairments vary across distinct cerebellar circuits. However, the progression of Purkinje neuron apoptosis in Tsc1mut/mut animals has not been measured and directly compared across males and females.
Previous work has also suggested the selective deletion of Tsc1 from Purkinje neurons differentially affects distinct cerebellar circuits. Rapamycin treatment, which has been shown to rescue deficits associated with Tsc1 deletion (Meikle et al., 2008; Zhou et al., 2009), was administered at various ages of Tsc1mut/mut mice to determine at which ages the treatment was effective in rescuing behavioral impairments. Interestingly, the ages at which rapamycin was effective at alleviating behavioral impairments were dependent on the type of behavior being rescued. For instance, rapamycin treatment in 10 week-old animals rescued deficits in motor function (measured via the rotarod test), but deficits in social interaction behaviors were not improved (Tsai et al., 2018). A clear hypothesis for this difference in rescue is that rapamycin-mediated rescue is no longer possible if Purkinje neurons in the relevant cerebellar circuit have undergone apoptosis. Extended to the work presented here, it may be that in male Tsc1mut/mut animals, there is greater apoptosis and Purkinje neuron loss in circuits contributing to social interaction behaviors compared to Tsc1mut/mut females, and that this sex-specific difference does not occur in cerebellar circuits contributing to balance and motor coordination. It will be interesting to test these ideas directly. Purkinje neurons functional in circuits dedicated to balance and motor coordination have been shown to localize to spinocerebellar (vermis and paravermis) regions (Unverdi and Alsayouri, 2024); while Purkinje neurons functional in higher order behaviors, such as social interactions have been associated with Purkinje neurons localized in hemispheric Crus I and Crus II cerebellar subregions (Matas et al., 2021; Stoodley et al., 2017; Van Overwalle et al., 2020).
In addition, or perhaps in place of sex-specific differences in Purkinje neuron apoptosis, there may be sex-specific and circuit-specific differences in the deficits in Purkinje neuron membrane excitability of Tsc1mut/mut animals. It’s of note that the sex-specific difference in social approach behaviors of Tsc1mut/mut animals was not measured in younger (9–11 week-old) animals. This result is in line with the progressive nature of behavioral deficits in Tsc1mut/mut animals (Tsai et al., 2018; 2012), which also may result from progressive Purkinje neuron apoptosis that occurs differentially across distinct cerebellar circuits. Beyond identifying the cellular and circuit-level substrates responsible for the sexual dimorphism in this ASD model, it will be interesting to also investigate the physiological drivers of such differences. For instance, there is some evidence that female sex hormones may provide a neuroprotective function in neurodegenerative disorders (Sumien et al., 2021; Túnez et al., 2006; Henderson et al., 1994; Garcia-Segura et al., 2001), resulting in sex-specific outcomes, although differences in the expression properties of sex chromosomes and/or sex hormone receptor expression (Ng and Hazrati, 2022) may also contribute to these differences. In future work aimed at effectively linking Tsc1mut/mut behavioral impairments with impairments in cerebellar functioning, it will be necessary to categorize experiments based on animal sex and age, and in cellular-based experiments, careful localization and targeting of Purkinje neurons should be used to link the functioning of discrete cerebellar circuits with the relevant behavioral phenotype.
Data availability statement
The original contributions presented in the study are included in the article/Supplementary material, further inquiries can be directed to the corresponding author.
Ethics statement
The animal study was approved by Miami University Animal Care and Use Program. The study was conducted in accordance with the local legislation and institutional requirements.
Author contributions
RL: Conceptualization, Data curation, Formal analysis, Investigation, Methodology, Project administration, Writing – original draft, Writing – review & editing. NL: Data curation, Formal analysis, Investigation, Writing – review & editing. SB: Data curation, Formal analysis, Investigation, Methodology, Project administration, Writing – review & editing. AJ: Investigation, Visualization, Writing – review & editing. JO: Investigation, Visualization, Writing – review & editing. JR: Conceptualization, Data curation, Formal analysis, Funding acquisition, Methodology, Project administration, Resources, Supervision, Validation, Visualization, Writing – original draft, Writing – review & editing.
Funding
The author(s) declare that financial support was received for the research, authorship, and/or publication of this article. This work was supported by startup funds from the Miami University College of Arts and Sciences and by the NINDS at the National Institutes of Health: Award 1R15NS125560.
Acknowledgments
The authors gratefully acknowledge and thank personnel of the Miami University Laboratory Animal Resources (LAR) and Center for Advanced Microscopy and Imaging (CAMI). The authors also thank A. Radke for advice on behavior testing and statistical analyses.
Conflict of interest
The authors declare that the research was conducted in the absence of any commercial or financial relationships that could be construed as a potential conflict of interest.
Publisher’s note
All claims expressed in this article are solely those of the authors and do not necessarily represent those of their affiliated organizations, or those of the publisher, the editors and the reviewers. Any product that may be evaluated in this article, or claim that may be made by its manufacturer, is not guaranteed or endorsed by the publisher.
Supplementary material
The Supplementary material for this article can be found online at: https://www.frontiersin.org/articles/10.3389/fnbeh.2024.1474066/full#supplementary-material
References
Andersen, B. B., Korbo, L., and Pakkenberg, B. (1992). A quantitative study of the human cerebellum with unbiased stereological techniques. J. Comp. Neurol. 326, 549–560. doi: 10.1002/cne.903260405
Andersson, G. W., Gillberg, C., and Miniscalco, C. (2013). Pre-school children with suspected autism Spectrum disorders: do girls and boys have the same profiles? Res. Dev. Disabil. 34, 413–422. doi: 10.1016/j.ridd.2012.08.025
Au, K. S., Williams, A. T., Steve Roach, E., Batchelor, L., Sparagana, S. P., Delgado, M. R., et al. (2007). Genotype/phenotype correlation in 325 individuals referred for a diagnosis of tuberous sclerosis complex in the United States. Genet. Med. 9, 88–100. doi: 10.1097/GIM.0b013e31803068c7
Bailey, A., Luthert, P., Dean, A., Harding, B., Janota, I., Montgomery, M., et al. (1998). A Clinicopathological study of autism. Brain J. Neurol. 121 May, 889–905. doi: 10.1093/brain/121.5.889
Barski, J. J., Dethleffsen, K., and Meyer, M. (2000). Cre recombinase expression in cerebellar purkinje cells. Genesis 28, 93–98.
Carter, R. J., Morton, J., and Dunnett, S. B. (2001). Motor coordination and balance in rodents. Curr. Prot. Neurosci. 15:8.12. doi: 10.1002/0471142301.ns0812s15
Christensen, Deborah L., Braun, Kim Van Naarden, Baio, Jon, Bilder, Deborah, Charles, Jane, Constantino, John N., et al. (2018). “Prevalence and characteristics of autism spectrum disorder among children aged 8 years - autism and developmental disabilities monitoring network, 11 sites, United States, 2012.” Morbidity and mortality weekly report. Surveillance summaries (Washington, D.C.: 2002) 65 (13): 1–23.
Craig, F., Crippa, A., De Giacomo, A., Ruggiero, M., Rizzato, V., Lorenzo, A., et al. (2020). Differences in developmental functioning profiles between male and female preschoolers children with autism spectrum disorder. Autism Res. 13, 1537–1547. doi: 10.1002/aur.2305
Crino, P. B., Nathanson, K. L., and Henske, E. P. (2006). The tuberous sclerosis complex. N. Engl. J. Med. 355, 1345–1356. doi: 10.1056/NEJMra055323
Ehninger, D., Han, S., Carrie Shilyansky, Y., Zhou, W. L., Kwiatkowski, D. J., Ramesh, V., et al. (2008). Reversal of learning deficits in a Tsc2+/− mouse model of tuberous sclerosis. Nat. Med. 14, 843–848. doi: 10.1038/nm1788
European Chromosome 16 Tuberous Sclerosis Consortium (1993). Identification and characterization of the tuberous sclerosis gene on chromosome 16. Cell 75, 1305–1315. doi: 10.1016/0092-8674(93)90618-Z
Garcia-Segura, L. M., Azcoitia, I., and DonCarlos, L. L. (2001). Neuroprotection by estradiol. Prog. Neurobiol. 63, 29–60. doi: 10.1016/S0301-0082(00)00025-3
Geschwind, D. H. (2011). Genetics of autism Spectrum disorders. Trends Cogn. Sci. 15, 409–416. doi: 10.1016/j.tics.2011.07.003
Henderson, V. W., Paganini-Hill, A., Emanuel, C. K., Dunn, M. E., and Galen Buckwalter, J. (1994). Estrogen replacement therapy in older women: comparisons between Alzheimer’s disease cases and nondemented control subjects. Arch. Neurol. 51, 896–900. doi: 10.1001/archneur.1994.00540210068014
Iscru, E., Serinagaoglu, Y., Schilling, K., Tian, J., Bowers-Kidder, S. L., Zhang, R., et al. (2009). Sensorimotor enhancement in mouse mutants lacking the Purkinje cell-specific Gi/o modulator, Pcp2(L7). Mol. Cell. Neurosci. 40, 62–75. doi: 10.1016/j.mcn.2008.09.002
Jahncke, J. N., and Wright, K. M. (2024). Tools for Cre-mediated conditional deletion of Floxed alleles from developing cerebellar Purkinje cells. eNeuro 11:ENEURO.0149-24.2024. doi: 10.1523/ENEURO.0149-24.2024
Jeon, S. J., Gonzales, E. L., Darine, F. N., Mabunga, S. T., Valencia, D. G., Kim, Y. K., et al. (2018). Sex-specific behavioral features of rodent models of autism Spectrum disorder. Exp. Neurobiol. 27, 321–343. doi: 10.5607/en.2018.27.5.321
Jeste, S. S., Sahin, M., Bolton, P., Ploubidis, G. B., and Humphrey, A. (2008). Characterization of autism in Young children with tuberous sclerosis complex. J. Child Neurol. 23, 520–525. doi: 10.1177/0883073807309788
Kalume, F., Yu, F. H., Westenbroek, R. E., Scheuer, T., and Catterall, W. A. (2007). Reduced sodium current in Purkinje neurons from Nav1.1 mutant mice: implications for Ataxia in severe myoclonic epilepsy in infancy. J. Neurosci. 27, 11065–11074. doi: 10.1523/JNEUROSCI.2162-07.2007
Kim, D. G., Gonzales, E. L., Kim, S., Kim, Y., Adil, K. J., Jeon, S. J., et al. (2019). Social interaction test in home cage as a novel and ethological measure of social behavior in mice. Exp. Neurobiol. 28, 247–260. doi: 10.5607/en.2019.28.2.247
Kwiatkowski, D. J., Zhang, H., Bandura, J. L., Heiberger, K. M., Glogauer, M., El-Hashemite, N., et al. (2002). A mouse model of TSC1 reveals sex-dependent lethality from liver hemangiomas, and up-regulation of p70S6 kinase activity in Tsc1 null cells. Hum. Mol. Genet. 11, 525–534. doi: 10.1093/hmg/11.5.525
Madisen, L., Zwingman, T. A., Sunkin, S. M., Seung Wook, O., Zariwala, H. A., Hong, G., et al. (2010). A robust and high-throughput Cre reporting and characterization system for the whole mouse brain. Nat. Neurosci. 13, 133–140. doi: 10.1038/nn.2467
Matas, E., Maisterrena, A., Thabault, M., Balado, E., Francheteau, M., Balbous, A., et al. (2021). Major motor and gait deficits with sexual dimorphism in a Shank3 mutant mouse model. Mol. Autism 12:2. doi: 10.1186/s13229-020-00412-8
Meikle, L., Pollizzi, K., Egnor, A., Kramvis, I., Lane, H., Sahin, M., et al. (2008). Response of a neuronal model of tuberous sclerosis to mammalian target of rapamycin (mTOR) inhibitors: effects on mTORC1 and Akt signaling lead to improved survival and function. J. Neurosci. 28, 5422–5432. doi: 10.1523/JNEUROSCI.0955-08.2008
Nadler, J. J., Moy, S. S., Dold, G., Trang, D., Simmons, N., Perez, A., et al. (2004). Automated apparatus for quantitation of social approach behaviors in mice. Genes Brain Behav. 3, 303–314. doi: 10.1111/j.1601-183X.2004.00071.x
Ng, M., and Hazrati, L.-N. (2022). Evidence of sex differences in cellular senescence. Neurobiol. Aging 120, 88–104. doi: 10.1016/j.neurobiolaging.2022.08.014
Overwalle, V., Frank, M. M., Cattaneo, Z., Clausi, S., Ferrari, C., Gabrieli, J. D. E., et al. (2020). Consensus paper: cerebellum and social cognition. Cerebellum (London, England) 19, 833–868. doi: 10.1007/s12311-020-01155-1
Palkovits, M., Magyar, P., and Szentágothai, J. (1972). Quantitative histological analysis of the cerebellar cortex in the cat. IV. Mossy Fiber-Purkinje cell numerical transfer. Brain Res. 45, 15–29. doi: 10.1016/0006-8993(72)90213-2
Reinhardt, V. P., Wetherby, A. M., Schatschneider, C., and Lord, C. (2015). Examination of sex differences in a large sample of young children with autism spectrum disorder and typical development. J. Autism Dev. Disord. 45, 697–706. doi: 10.1007/s10803-014-2223-6
Saito, H., Tsumura, H., Otake, S., Nishida, A., Furukawa, T., and Suzuki, N. (2005). L7/Pcp-2-specific expression of Cre recombinase using Knock-in approach. Biochem. Biophys. Res. Commun. 331, 1216–1221. doi: 10.1016/j.bbrc.2005.04.043
Stoodley, C. J. (2014). Distinct regions of the cerebellum show gray matter decreases in autism, ADHD, and developmental dyslexia. Front. Syst. Neurosci. 8:92. doi: 10.3389/fnsys.2014.00092
Stoodley, C. J., D’Mello, A. M., Ellegood, J., Jakkamsetti, V., Liu, P., Nebel, M. B., et al. (2017). Altered cerebellar connectivity in autism spectrum disorders and rescue of autism-related behaviors in mice. Nat. Neurosci. 20, 1744–1751. doi: 10.1038/s41593-017-0004-1
Sumien, N., Thomas Cunningham, J., Davis, D. L., Engelland, R., Fadeyibi, O., Farmer, G. E., et al. (2021). Neurodegenerative disease: roles for sex, hormones, and oxidative stress. Endocrinology 162:bqab185. doi: 10.1210/endocr/bqab185
Tillmann, J., Ashwood, K., Absoud, M., Bölte, S., Bonnet-Brilhault, F., Buitelaar, J. K., et al. (2018). Evaluating sex and age differences in ADI-R and ADOS scores in a large European multi-site sample of individuals with autism spectrum disorder. J. Autism Dev. Disord. 48, 2490–2505. doi: 10.1007/s10803-018-3510-4
Tsai, P. T., Hull, C., Chu, Y. X., Greene-Colozzi, E., Sadowski, A. R., Leech, J. M., et al. (2012). Autistic-like behaviour and cerebellar dysfunction in Purkinje cell Tsc1 mutant mice. Nature 488, 647–651. doi: 10.1038/nature11310
Tsai, P. T., Rudolph, S., Guo, C., Ellegood, J., Gibson, J. M., Schaeffer, S. M., et al. (2018). Sensitive periods for cerebellar-mediated autistic-like behaviors. Cell Rep. 25, 357–367.e4. doi: 10.1016/j.celrep.2018.09.039
Túnez, I., Collado, J. A., Medina, F. J., Peña, J., María Del, C., Muñoz, I. J., et al. (2006). 17 Beta-estradiol may affect vulnerability of striatum in a 3-Nitropropionic acid-induced experimental model of Huntington’s disease in ovariectomized rats. Neurochem. Int. 48, 367–373. doi: 10.1016/j.neuint.2005.11.011
Unverdi, Mahmut, and Alsayouri, Khalid. (2024). Neuroanatomy, cerebellar dysfunction. Treasure Island (FL): StatPearls Publishing.
Wang, S. S.-H., Kloth, A. D., and Badura, A. (2014). The cerebellum, sensitive periods, and autism. Neuron 83, 518–532. doi: 10.1016/j.neuron.2014.07.016
Wijngaarden-Cremers, V., Patricia, J. M., van Eeten, E., Groen, W. B., Van Deurzen, P. A., Oosterling, I. J., et al. (2014). Gender and age differences in the core triad of impairments in autism spectrum disorders: a systematic review and Meta-analysis. J. Autism Dev. Disord. 44, 627–635. doi: 10.1007/s10803-013-1913-9
Wiznitzer, M. (2004). Autism and tuberous sclerosis. J. Child Neurol. 19, 675–679. doi: 10.1177/08830738040190090701
Wu, R., Jiang, X., Xueyan, W., Pang, J., Tang, Y., Ren, Z., et al. (2022). Interspecific differences in sociability, social novelty preference, anxiety-and depression-like behaviors between Brandt’s voles and C57BL/6J mice. Behav. Process. 197:104624. doi: 10.1016/j.beproc.2022.104624
Wullschleger, S., Loewith, R., and Hall, M. N. (2006). TOR signaling in growth and metabolism. Cell 124, 471–484. doi: 10.1016/j.cell.2006.01.016
Yang, M., Silverman, J. L., and Crawley, J. N. (2011). Automated three-chambered social approach task for mice. Curr. Protoc. Neurosci. 8:26. doi: 10.1002/0471142301.ns0826s56
Zeng, P.-Y., Tsai, Y.-H., Lee, C.-L., Ma, Y.-K., and Kuo, T.-H. (2023). Minimal influence of estrous cycle on studies of female mouse behaviors. Front. Mol. Neurosci. 16:1146109. doi: 10.3389/fnmol.2023.1146109
Keywords: tuberous sclerosis, TSC, cerebellum, social interaction, motor coordination, sexual dimorphism
Citation: Lawson RJ, Lipovsek NJ, Brown SP, Jena AK, Osko JJ and Ransdell JL (2024) Selective deletion of Tsc1 from mouse cerebellar Purkinje neurons drives sex-specific behavioral impairments linked to autism. Front. Behav. Neurosci. 18:1474066. doi: 10.3389/fnbeh.2024.1474066
Edited by:
Martine Ammassari-Teule, National Research Council (CNR), ItalyReviewed by:
Paola Sgadò, University of Trento, ItalyJessica Burket, Christopher Newport University, United States
Copyright © 2024 Lawson, Lipovsek, Brown, Jena, Osko and Ransdell. This is an open-access article distributed under the terms of the Creative Commons Attribution License (CC BY). The use, distribution or reproduction in other forums is permitted, provided the original author(s) and the copyright owner(s) are credited and that the original publication in this journal is cited, in accordance with accepted academic practice. No use, distribution or reproduction is permitted which does not comply with these terms.
*Correspondence: Joseph L. Ransdell, cmFuc2RlamxAbWlhbWlvaC5lZHU=