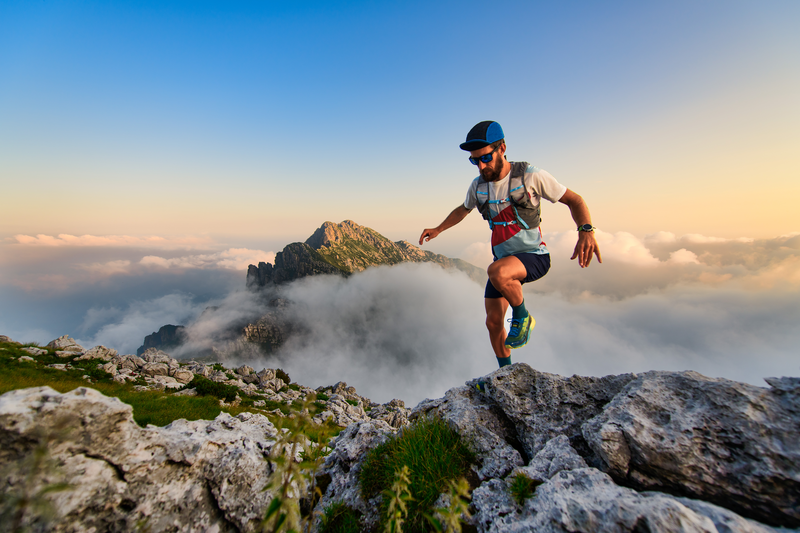
95% of researchers rate our articles as excellent or good
Learn more about the work of our research integrity team to safeguard the quality of each article we publish.
Find out more
BRIEF RESEARCH REPORT article
Front. Behav. Neurosci. , 12 September 2024
Sec. Pathological Conditions
Volume 18 - 2024 | https://doi.org/10.3389/fnbeh.2024.1445184
Anxiety is a psychiatric non-motor symptom of Parkinson’s that can appear in the prodromal period, prior to significant loss of midbrain dopamine neurons and motor symptoms. Parkinson’s-related anxiety affects females more than males, despite the greater prevalence of Parkinson’s in males. How stress, anxiety and Parkinson’s are related and the basis for a sex-specific impact of stress in Parkinson’s are not clear. We addressed this using young adult male and female mice carrying a G2019S knockin mutation of leucine-rich repeat kinase 2 (Lrrk2G2019S) and Lrrk2WT control mice. In humans, LRRK2G2019S significantly elevates the risk of late-onset Parkinson’s. To assess within-sex differences between Lrrk2G2019S and control mice in stress-induced anxiety-like behaviors in young adulthood, we used a within-subject design whereby Lrrk2G2019S and Lrrk2WT control mice underwent tests of anxiety-like behaviors before (baseline) and following a 28 day (d) variable stress paradigm. There were no differences in behavioral measures between genotypes in males or females at baseline, indicating that the mutation alone does not produce anxiety-like responses. Following chronic stress, male Lrrk2G2019S mice were affected similarly to male wildtypes except for novelty-suppressed feeding, where stress had no impact on Lrrk2G2019S mice while significantly increasing latency to feed in Lrrk2WT control mice. Female Lrrk2G2019S mice were impacted by chronic stress similarly to wildtype females across all behavioral measures. Subsequent post-stress analyses compared cFos immunolabeling-based cellular activity patterns across several stress-relevant brain regions. The density of cFos-activated neurons across brain regions in both male and female Lrrk2G2019S mice was generally lower compared to stressed Lrrk2WT mice, except for the nucleus accumbens of male Lrrk2G2019S mice, where cFos-labeled cell density was significantly higher than all other groups. Together, these data suggest that the Lrrk2G2019S mutation differentially impacts anxiety-like behavioral responses to chronic stress in males and females that may reflect sex-specific adaptations observed in circuit activation patterns in some, but not all stress-related brain regions.
Anxiety is a prevalent psychiatric non-motor symptom of genetic and idiopathic forms of Parkinson’s that can appear in the prodromal stage, before significant dopamine neuron degeneration (Shiba et al., 2000; Broen et al., 2016). Chronic behavioral stress has emerged as an important risk factor for psychiatric symptoms and Parkinson’s (Hemmerle et al., 2012). How stress, psychiatric symptoms and Parkinson’s are related mechanistically is unknown. Mice carrying gene mutations associated with an increased risk for Parkinson’s provide an opportunity to examine the intersection between genetic and experience-dependent risks.
The G2019S mutation in LRRK2 (encoding leucine-rich repeat kinase 2) is among the most common genetic risk mutations for familial and sporadic Parkinson’s (Kluss et al., 2019). LRRK2G2019S produces late-onset Parkinson’s that is clinically similar to idiopathic forms, including prodromal anxiety and depression in non-manifesting mutation carriers (Shanker et al., 2011; DeBroff et al., 2023). Genetic, pathological, and preclinical studies suggest that dysregulated LRRK2 may be part of a common pathological mechanism (Kluss et al., 2019; Rocha et al., 2022; Peter and Strober, 2023). In brain, LRRK2 protein is enriched in areas that are susceptible or can adapt negatively to stressful behavioral experiences, including medial prefrontal cortex (mPFC), amygdala, hippocampus and striatum (Simón-Sánchez et al., 2006; Giesert et al., 2013), suggesting that LRRK2 is well-positioned to modify responses to stress.
Previous work has shown that male Lrrk2G2019S knockin mice display altered social interaction and hedonic responses following periods of social defeat stress that are distinct from wildtype mice (Matikainen-Ankney et al., 2018; Guevara et al., 2020). Social defeat stress is an intense stressor that has most readily been applied to males. This leads us to ask whether such synergy between social stress and Lrrk2G019S can be generalized to different durations and forms of stress. We also address the effects of stress on female Lrrk2G2019S mice. To answer these questions, we mounted a fully powered comparison of anxiety-like behaviors and examined brain regions activated in males and females following extended exposure to a chronic variable stress protocol.
Lrrk2G2019S knockin mice (RRID:MGI:6273311) were generated by Eli Lilly and characterized previously (Matikainen-Ankney et al., 2016, 2018; Guevara et al., 2020; Hussein et al., 2022). The knockin mice were congenic on C57Bl/6NTac background, bred as homozygotes, and backcrossed to wildtype C57Bl/6NTac every fifth generation to prevent genetic drift. Age-, sex- and strain-matched wildtype mice (Lrrk2WT) were used as controls. All mice were bred in Mount Sinai’s vivarium under identical conditions. The mice were group housed and maintained on a 12 h light/dark cycle with free access to food and water unless otherwise specified. Genotypes of all animals were confirmed through PCR and sequencing. Male and female heterozygous Lrrk2G2019S mice and age- and strain-matched wildtype mice were utilized for all experiments. Heterozygotes were generated by crossing Lrrk2G2019S/G2019S mice with Lrrk2WT/WT mice. LRRK2G2019S causes autosomal dominant Parkinson’s in human carriers, with one or two copies of the mutation producing a similar disease course (Lesage et al., 2007; Healy et al., 2008; Yahalom et al., 2014). In line with this, previous studies have shown similar behavioral and electrophysiological outcomes between hetero- and homozygotes (Matikainen-Ankney et al., 2016; Guevara et al., 2020). Mice were aged postnatal day 56–60 at the start of the behavioral experiments. In the females, no attempt was made to document the stage of the estrous cycle in relation to behavioral testing. Recent studies suggest the impact of the estrous cycle on behavioral performance in tests of anxiety, including those used in this study, is negligible (Chari et al., 2020; Johnson et al., 2021; Levy et al., 2023; Li et al., 2023). On the other hand, another recent study showed that VTA dopamine neuron physiology is sensitive to phases of the estrous cycle, and that cycling sex steroids impact the stress-sensitivity of dopamine-related reward behaviors (Shanley et al., 2023). All experiments were approved by Mount Sinai’s Institutional Animal Care and Use Committee and conformed to National Institutes of Health guidelines.
We used a within-subject design to evaluate the effects of 28d of variable stress on different measures of anxiety-like behaviors. To establish a pre-stress baseline of responses, behaviorally naïve Lrrk2G2019S and Lrrk2WT mice were tested on a battery of standard tests on days 1–3. Twenty-four hours later, mice were subjected to 28d of variable stress. Twenty-four hours after the last day of the stress paradigm, mice were re-tested on the same daily pre-stress behavioral battery to establish post-stress effects for comparison (Figure 1). The pre- and post-stress behavioral assays comprised an open field test, a social interaction test with a same-sex conspecific, and a novelty-suppressed feeding test. One test was conducted per day and animals were allowed to habituate to the testing room for 1 h prior to testing. Equipment was cleaned with 70% ethanol in between testing sessions. Mice were group-housed throughout the entire experiment (Westenbroek et al., 2005; Manouze et al., 2019).
Figure 1. Schematic showing timeline of behavioral tests prior to (baseline) and following a 28d variable stress paradigm, with subsequent cFos immunohistochemistry (IHC). OFT, Open Field Test; SIT, Social Interaction Test; NSF, Novelty Suppressed Feeding.
Mice were placed in an open arena (40 × 40 × 30 cm) under white light and allowed to explore for 5 min (Cunningham et al., 2021). We designated a 20.3 × 20.3 cm square centrally located within the arena as the “center” with the rest of the arena designated as the “periphery.” Fusion Software (v5.0) (Omnitech Electronics) was used to quantify time spent in the center and periphery of the open arena as an indicator of anxiety (Badimon et al., 2020), and to monitor total distance traveled.
Social interaction (SI) was assessed in two 150 s phases, separated by a duration of 30 s, either in the absence or presence of a novel conspecific social target under red lights (Muir et al., 2020; Tsyglakova et al., 2021). SI behavior was calculated as an SI Ratio = tpresent/tabsent, where tpresent and tabsent represent the times spent in the interaction zone in the presence and absence of the novel social target. Time and location in the arena were recorded by video tracking software (Ethovision 10.0, Noldus).
NSF tests were conducted as described (Freire-Cobo and Wang, 2020; Johnson et al., 2021). Briefly, mice were food-deprived the night before the test. On the day of testing, mice were placed in an arena containing a single food pellet in the center. The latency to take a bite from the piece of food was scored manually for a maximum of 10 min. The test was conducted under red light. All mice were weighed in the evening prior to food restriction and the next morning prior to the NSF test.
The variable stress paradigm was performed as previously described (Freire-Cobo and Wang, 2020; Johnson et al., 2021). Mice were subjected to one of three stressors in the following order for 1 h per day: 100 random foot shocks of 0.45 mA for 3 s each (day 1), tail suspension (day 2), and restraint by placing the animal in a ventilated 50-mL conical tube (day 3). Stressors were administered and repeated in this order over 28d. Mice were returned to their homecage and group-housed at the end of each stressor.
Twenty-four hours following the last behavioral assay, 4–5 mice of each sex and genotype were subjected to restraint stress for 1 h then returned to their homecage for 90 min followed by transcardial perfusion with 4% paraformaldehyde in phosphate buffered saline (PBS, pH7.3) (Figure 1). Following 6 h post-fixation, brains were sectioned coronally at 50 μM on a freezing sliding microtome (Leica). Immunocytochemical localization of cFos was performed on every sixth section (approximately every 300 μm). Free-floating sections were incubated in 2% bovine serum albumin (BSA) in 0.3% Tween-20 PBS for 1 h then incubated in rabbit anti-c-Fos antibody (1:500, Synaptic Systems, catalog # 226–003, RRID: AB_2231974) diluted in the same solution for 72 h at 4°C. Sections were washed, then incubated in goat anti-rabbit Alexa 647-conjugated antibodies (1:500, Abcam catalog #150076, RRID: AB_2782993) diluted in 2% BSA in 0.3% Tween-20 PBS for 1 h and then stained with 0.005% DAPI in PBS for 20 min. Finally, sections were slide-mounted and coverslipped with Mowiol.
Stress is a significant risk factor for anxiety and depression in humans (Hemmerle et al., 2012), and a large number of brain regions have been implicated in anxiety- and depression-like behaviors (Russo and Nestler, 2013). We chose a subset of potential brain regions for cFos analysis that included mPFC (areas PL and IL in mice), dorsal striatum (dStr), nucleus accumbens (NAc), basolateral amygdala (BLA), dorsal hippocampal area CA1 (dHPC) and ventral hippocampal area CA1 (vHPC). This subset of areas met two criteria for analysis: they respond to stress and/or have been implicated in anxiety-like or depression-like behaviors in mice and humans (Furman et al., 2011; Gabbay et al., 2013; Kerestes et al., 2015; Cooper et al., 2017; Muir et al., 2020; Kim and Chung, 2021; Rainville et al., 2022; Giovanniello et al., 2023) and they exhibit significant LRRK2 expression (Taymans et al., 2006; Giesert et al., 2013; Huntley and Benson, 2020).
Single-plane, tiled images of entire cFos-immunolabeled brain sections were captured on a Leica DMi8 widefield microscope equipped with a 10X objective lens, a SpectraX fluorescence illuminator, and a Leica DFC9000GT monochrome camera in the Microscopy and Advanced Bioimaging Core at Mount Sinai. Anatomical regions were identified using Nissl staining as a guide, and a region of interest (ROI) of the same size was generated in each section across mice. In layered structures (PL, IL, hippocampus), ROIs included all layers.
Density of cFos-labeled cells was quantified using the “Cell Counter” plug-in on ImageJ/Fiji. Thresholding limits were determined using the “Moments” setting on ImageJ/Fiji. cFos density was calculated as # cFos-labeled cells/area of ROI (μm2).
The numbers of animals used for behavioral and cFos mapping experiments are given in the relevant figure legends. Statistical comparisons of normally distributed data between two groups were conducted using two-tailed Student’s t-tests. To compare parametric data from multiple groups, we used two-way ANOVA, but for within-subject comparisons of parametric data from multiple groups, we used two-way repeated-measures ANOVA followed by Šidák’s post-hoc test for multiple comparisons. For nonparametric data between two groups, a Mann–Whitney U test was used. For all datasets, we tested for outliers using Grubb’s test, but no animal was excluded because no single animal was an outlier on all behavioral tests. Analyses were conducted using GraphPad Prism (v8.2.1), with p < 0.05 considered significant. Data are presented as means ± SEM.
We subjected young adult male and female Lrrk2WT and heterozygous Lrrk2G2019S knockin mice to a variable stress (VS) paradigm for 28 days (28d-VS) to assess the impact of stress, sex and genetic risk for Parkinson’s on measures of anxiety-like behaviors in mice using a within-subject design to compare baseline (pre-stress) and post-stress responses (Figure 1).
In an OFT, reduced time spent in the center of an open arena is interpreted as increased anxiety-like behavior (Simon et al., 1994; Harro, 2018). At baseline, we found no significant genotype differences among the male and female cohorts in the time spent in the center (Figures 2A,D) or in total distance traveled, indicating that the mutation alone does not significantly affect anxiety-like behavior or locomotor activity in this test in males or females, as expected (Yue et al., 2015). After 28d-VS, male Lrrk2WT and Lrrk2G2019S mice exhibited no significant stress-related changes in the time spent in the center (Figure 2A). However, in contrast to males, both Lrrk2WT and Lrrk2G2019S female mice showed a main effect of stress by a significantly decreased time in center following 28d-VS (Figure 2D). There were no significant effects of stress on locomotor activity in males or females (males: main effect of stress, F1,18 = 1.267, p = 0.054, main effect of genotype, F1,18 = 0.1268, p = 0.7259; females: main effect of stress, F1,17 = 1.807, p = 0.1966, main effect of genotype, F1,17 = 1.063, p = 0.3169).
Figure 2. Effects of 28d-VS (variable stress) on measures of anxiety-like behavior, social interaction and hyponeophagia in males (top row) and females (bottom row). (A) Male Lrrk2WT (WT) control and Lrrk2G2019S (GS) mice showed no changes to time in center following 28d-VS in comparison with their baseline measures (p = 0.4293, F1,18 = 0.6539 for main effect of stress). (B) WT and GS males both exhibited an increased social interaction (SI) ratio following 28d-VS in comparison with baseline SI ratios (p = 0.0201, F1,17 = 6.572 for main effect of stress). (C) In an NSF test, GS males were unaffected by 28d-VS compared to their baseline latencies. In contrast, WT males showed a significantly increased latency to feed (p = 0.0099), which was significantly longer than post-stress latencies of GS males (p = 0.0001) (p = 0.0199, F1,18 = 0.2994 for interaction effect; p = 0.0014, F1,18 = 14.21 for main effect of genotype). (D) Both WT and GS females show a decreased time in center following 28d-VS (p = 0.046, F1,17 = 4.635 for main effect of stress). (E) WT and GS females both showed an increased SI ratio following 28d-VS (p = 0.0036, F1,17 = 11.42 for main effect of stress); with GS females showing a significantly increased SI ratio compared to their baseline measures (p = 0.019). (F) Both WT and GS female mice exhibited a similarly increased latency to feed following 28d-VS (p = <0.0001) (p = <0.0001, F1,17 = 143.7 for main effect of stress). n = 10 WT male mice, 10 WT female mice, 13 GS male mice, and 9 GS female mice. Statistical tests used: 2-way repeated measures ANOVA with post hoc Šidák test for multiple comparisons. Data presented as mean ± s.e.m. *p < 0.05; **p < 0.01; ***p < 0.001, ****p < 0.001.
Mice were tested for social interaction (SI) behavior with an age- and sex-matched conspecific because social avoidance is a measure of anxiety- and depression-like states (Golden et al., 2011). There were no baseline genotype differences in SI ratios in males or females (Figures 2B,E), as expected (Matikainen-Ankney et al., 2018; Guevara et al., 2020). However, there was a main effect of 28d-VS on SI behavior in both genotypes and sexes (Figures 2B,E). In males and females, we found that 28d-VS significantly increased social interaction in Lrrk2WT and Lrrk2G2019S mice. Post hoc analysis of the female cohort showed that female Lrrk2G2019S mice had a significantly greater increase in SI ratios following 28d-VS compared to their baselines (Figure 2E).
Mice were food-restricted overnight and subsequently placed in a novel arena. Their latency to feed on a new food pellet was measured. Increased latency to feed is interpreted as a measure of heightened anxiety-like behavior because this measure is reduced by anxiolytic drugs (Bodnoff et al., 1989). Under baseline conditions, there were no genotype differences in males or females in response latencies to feed (Figures 2C,F). In males, post hoc analyses showed that following 28d-VS, Lrrk2WT mice exhibited significantly increased latencies to feed compared to their pre-stress baseline responses (Figure 2C). In contrast, male Lrrk2G2019S showed no significant differences between unstressed and post-stress latencies to feed (Figure 2C), suggesting a lack of anxiety-like effect in this test driven by 28d-VS compared to a significant elevation in anxiety-like behavior of Lrrk2WT mice. We verified that in the male mice, there were no genotype differences in the percent body weight lost after overnight fasting, either under baseline or post-28d-VS conditions (repeated-measures two-way ANOVA, main effect of genotype, F1,18 = 0.2795, p = 0.6035), ruling out potential differences between genotypes in weight lost as a factor accounting for different post-stress effects on latency to feed.
In female mice, there was a significant effect of stress on latencies to feed that was evident in both genotypes, with Lrrk2WT and Lrrk2G2019S female mice exhibiting significantly increased latencies to feed following 28d-VS compared to their unstressed baseline latencies (Figure 2F). Comparison of overnight fasting-induced body weight loss showed that at baseline, female Lrrk2G2019S mice lost a significantly greater percentage of body weight compared to baseline measured in female wildtype mice (two-way repeated-measures two-way ANOVA, main effect of genotype, F1,17 = 9.262, p = 0.0073), but there were no significant differences between genotypes in body weight lost following 28d-VS. As the latencies to feed were similar between genotypes under both baseline and post-stress conditions, the significance of a greater percentage of body weight lost at baseline in the Lrrk2G2019S females is not clear. In sum, following stress, latency to feed was increased significantly in both male and female wildtype mice and in female Lrrk2G2019S mice. Male Lrrk2G2019S mice were unaffected.
A summary of behavioral results, following 28d-VS in males and females, is shown in Figure 3.
Figure 3. Summary of effects of 28d-VS on WT and GS male and female mice across all behavioral measures. Directionality of change in behavioral performance following 28d-VS is indicated by color and direction of arrows. Red downward arrow = decreased measure of behavior performance following 28d-VS compared to baseline measures; green upward arrow = increased measure of behavioral performance following 28d-VS compared to baseline measures; horizontal line = no change in measure of behavioral performance following 28d-VS. More than one arrow = significance in post-hoc comparison.
To assay sex and genetic differences in regional activation patterns of the stress-network following stress-experience, we used immunolabeling for the immediate early gene cFos as a neural activity marker (Morgan and Curran, 1989) (Figure 4A). We analyzed a subset of brain regions implicated in anxiety-like behaviors and that exhibit high levels of LRRK2 expression (see Materials and Methods).
Figure 4. cFos immunolabeling-based cellular activity patterns in male and female mice following stress. cFos immunofluorescent labeling of perfused brain sections was used as an activity marker in stress-relevant brain regions following stress-experience. (A) A Nissl-stained series of frontal sections (top row) through the brain of a wildtype mouse post-stress with regions in green shading highlighting those analyzed for cFos immunolabeling. Representative confocal microscope images through mPFC of a male WT mouse (bottom row, left) or of a male GS mouse (bottom row, right) showing examples of cFos immunofluorescently-labeled cells (boxed regions show higher-magnification images). mPFC, medial prefrontal cortex; dStr, dorsal striatum; NAc, nucleus accumbens; BLA, basolateral amygdala; dHPC, dorsal hippocampus; vHPC, ventral hippocampus. Following stress, mice exhibited differential genotype and sex differences in most areas examined. (B) Analysis of cFos-labeled cell density in mPFC post-stress (p = 0.0085, F1,14 = 9.345 for genotype effect; p = 0.0006, F1,14 = 19.06 for main effect of sex); with GS males (p = 0.0258) and WT females (p = 0.0057) showing decreased density of cFos-labeled cells compared to WT males, and GS females showing decreased density of cFos-labeled cells compared to GS males (p = 0.0442). (C) Analysis of cFos-labeled cell density in dStr post-stress (p = 0.0657, F1,14 = 3.985 for main effect of sex). (D) Analysis of cFos-labeled cell density in NAc post-stress (p = 0.0037, F1,14 = 12.05 for interaction effect; p = 0.0672, F1,14 = 3.936 for genotype effect; p = 0.0024, F1,14 = 13.68 for main effect of sex); with GS males (p = 0.0022) showing increase cFos-labeled cell density compared to WT males, and GS females showing decreased density of cFos-labeled cells compared to GS males (p = 0.0003). (E) Analysis of cFos-labeled cell density in BLA post-stress (p = 0.0088, F1,14 = 9.261 for genotype effect); with GS females showing a significantly decreased density of cFos-labeled cells compared to WT females (p = 0.0474). (F) Analysis of cFos-labeled cell density in dHPC post-stress (p = 0.0667, F1,13 = 4.005 for main effect of genotype). (G) Analysis of cFos-labeled cell density in vHPC post-stress mice (p = 0.62, F(1,14) = 0.25 for interaction effect; p = 0.72, F(1,14) = 0.13 for genotype effect; p = 0.33, F(1,14) = 0.10 for sex effect). For all experiments, n = average of 2 brain slices per mouse / 4–5 mice per group. Statistical tests used: 2-way ANOVA with post hoc Šidák test for multiple comparisons. Data presented as mean ± s.e.m. #p = 0.05–0.07, *p < 0.05; **p < 0.01; ***p < 0.001.
Both male and female Lrrk2G2019S mice had a significantly decreased density of cFos immunofluorescent cells in mPFC following stress compared to their respective stressed Lrrk2WT controls (Figure 4B), with combined males having a significantly higher cFos-labeled cell density overall in comparison with combined females (Figure 4B). In dStr (Figure 4C), there was a trending main effect of sex with combined males having higher densities of cFos-labeled cells compared to combined females. In NAc (Figure 4D), there was a significant increase in the density of cFos immunolabeled cells in male Lrrk2G2019S mice compared to male Lrrk2WT mice, but no significant differences in cFos-labeled cell density between Lrrk2G2019S and Lrrk2WT female mice (Figure 4D). Together, these data support a main effect of genotype, sex and their interaction. In BLA (Figure 4E), there was a main effect of genotype, with lower cFos-labeled cell densities in Lrrk2G2019S mice compared to Lrrk2WT mice and significantly lower cFos-labeled cell density in stressed Lrrk2G2019S female mice compared to stressed female Lrrk2WT mice. In dHPC (Figure 4F), there was a trending main effect of genotype with higher densities of cFos-labeled cells in Lrrk2G2019S mice compared to Lrrk2WT controls, while in vHPC, there were no genotype or sex-related differences in cFos-labeled cells following stress (Figure 4G). Together, these data suggest that the recruitment of neural networks across some stress-responsive brain regions differs between male and female mice. c-Fos-based activation levels appeared to be generally higher in male than in female mice. Most striking was the selectively increased activation of NAc in male Lrrk2G2019S mice because, outside of this, the mutation appeared to mostly dampen activation.
The purpose of this study was to better understand how the Parkinson’s Lrrk2G2019S risk mutation impacted stress-induced anxiety-like behaviors in young adulthood in males and females. We subjected male and female Lrrk2G2019S heterozygous knockin and Lrrk2WT control mice to a 28d chronic variable stress paradigm to assess within-sex differences between Lrrk2G2019S mice and Lrrk2WT controls. Using several tests of anxiety-like behaviors, we found that in males, chronic variable stress affected behavioral performance of Lrrk2WT and Lrrk2G2019S mice similarly with the exception of an NSF test, where stress had no impact on Lrrk2G2019S mice while significantly affecting wildtypes. Female Lrrk2G2019S mice were impacted by chronic stress similarly to Lrrk2WT control females across all behavioral measures. Subsequent post-stress analyses compared cFos immunolabeling-based cellular activity patterns across several stress-relevant brain regions. We found that in male Lrrk2G2019S mice, post-stress patterns of cFos-labeled cells in several areas, including mPFC and the NAc, differed significantly from those found in either stressed Lrrk2WT males or stressed Lrrk2G2019S females. In contrast, stressed female Lrrk2G2019S mice showed cFos-labeling patterns that were mostly similar to stressed female wildtypes with the exception of the BLA, which had significantly fewer cFos-labeled neurons in stressed female Lrrk2G2019S mice compared to stressed female Lrrk2WT mice. Together, these data suggest that the Lrrk2G2019S mutation differentially impacts anxiety-like behavioral responses to chronic stress in male and female mutation carriers. This may reflect sex-specific adaptations in circuit activation patterns in many, but not all, stress-related brain regions. More broadly, our findings suggest a potential basis for differences in the onset and/or severity of psychiatric symptoms in male and female Parkinson’s patients.
We used a within-subject longitudinal behavioral testing design because it greatly increases statistical power over a between-subjects design (Partridge et al., 2023). Moreover, a within-subject behavioral testing design has been used in several previous studies of the effects of stress on anxiety-like measures in mice, including some of the measures used in the present study (Muir et al., 2020; Morel et al., 2022). It has been shown that some behavioral tests (e.g., elevated plus maze) are sensitive to repeated exposure (McIlwain et al., 2001) and inter-exposure interval (Paylor et al., 2006). While we cannot rule out the possibility of effects on post-stress behaviors following the initial baseline exposure, the possibility of significant repeat-exposure effects on post-stress behaviors is unlikely. First, the effects of 28d-VS on anxiety-like behaviors of our Lrrk2WT control mice were similar to previous studies of wildtype mice that used a between-subject design. For example, we found a main effect of stress in the significantly longer latencies to feed in the NSF test in male and female Lrrk2WT mice as reported previously with shorter (Bittar et al., 2021) or identical periods of exposure to similar stressors (Johnson et al., 2021). Similarly, social interaction time with a sex-matched, conspecific target increased in both male and female Lrrk2WT mice following 28d-VS as reported previously (Zhao et al., 2020; LeClair et al., 2021; Li et al., 2023). Second, all mice were subjected to the identical protocol, so any effect of prior test exposure would be expected to affect all mice. Importantly, in the absence of stress, we found no within-sex differences between Lrrk2G2019S knockin mice and their wildtype controls at baseline. This observation indicates that the mutation does not, in and of itself, drive aberrant anxiety-like, depression-like or anhedonia-like behaviors in either male or female Lrrk2G2019S mice at the young adult ages examined, as shown previously (Yue et al., 2015; Volta et al., 2017; Matikainen-Ankney et al., 2018; Guevara et al., 2020). While there were no baseline behavioral effects of the mutation at young adult ages, it is possible that in the absence of stress, mutation-driven effects on anxiety- or depression-like behaviors would emerge with advancing age. In a study using BAC transgenic mice overexpressing human LRRK2G2019S, anxiety- and depression-like behaviors that were absent in young adulthood first appeared in both male and female mutant mice at about 1 year of age—in the absence of overt stress—and persisted at older ages (Lim et al., 2018). Moreover, such age-dependent effects on psychiatric-like behaviors in these transgenic mice were correlated with contemporaneous changes in hippocampal serotonin levels and altered serotonergic receptor expression (Lim et al., 2018). Setting aside significant differences in the genetics of the two mouse models, it may be that the knockin mice may gradually show an age-dependent effect on anxiety-like behaviors in the absence of stress. Future studies will be needed to examine this and to see if serotonergic innervation becomes compromised with advancing age in the knockin mice. In middle-aged human LRRK2G2019 carriers, alterations in serotonin transporter binding have been reported (Wile et al., 2017). Nevertheless, it is important to emphasize that this and other Lrrk2G2019S knockin lines of mice do not exhibit age-related dopamine neuron degeneration nor do they exhibit age-related motor deficits mimicking human Parkinson’s (Longo et al., 2014; Yue et al., 2015; Volta et al., 2017; Matikainen-Ankney et al., 2018; Guevara et al., 2020). The mice are thus better models of some non-motor symptoms, exhibiting for example fronto-striatal based cognitive deficits (Hussein et al., 2022) and psychiatric-like behaviors such as those studied here and previously (Matikainen-Ankney et al., 2018; Guevara et al., 2020).
In idiopathic Parkinson’s, symptoms of anxiety and depression are more prevalent in women than in men (Leentjens et al., 2011; Cui et al., 2017). Although the prevalence of anxiety and depression in human LRRK2 mutation carriers is similar to that in idiopathic Parkinson’s (Gaig et al., 2014; San Luciano et al., 2017; Chen et al., 2020; DeBroff et al., 2023), it is less clear whether there are sex differences in psychiatric symptoms among manifesting LRRK2G2019S carriers. One study suggests that depression severity may be similar in male and female mutation carriers (San Luciano et al., 2017), which aligns with the equal penetrance of the mutation in male and female carriers (Marder et al., 2015). Our data in the young adult Lrrk2G2019S mice suggest that behavioral stress impacts anxiety-like behaviors in females more significantly than in males, bearing some similarity to idiopathic Parkinson’s. Elevated LRRK2 kinase activity, in the absence of genetic mutation, is found in sporadic Parkinson’s (Kluss et al., 2019; Rocha et al., 2022). As such, behavioral outcomes and regional cell activation analyses of stress effects in Lrrk2G2019S mice may have broad relevance to Parkinson’s generally.
Despite broadly similar anxiety-like behavioral responses to 28-VS between Lrrk2G2019S and wildtype mice, the densities of cFos-labeled cells across brain regions activated under such conditions showed both genotype-related and sex-specific differences. In stressed male and female Lrrk2G2019S mice, densities of cFos-labeled cells were affected in mostly similar ways compared to stressed Lrrk2WT mice—lower (mPFC, dStr and BLA), slightly higher (dHPC) or unchanged (vHPC). The exception to this pattern was in the NAc. In this structure, cFos densities in male LrrkG2019S mice were significantly higher than all other groups, whereas in female LrrkG2019S mice, cFos density was similar to male and female Lrrk2WT mice. It is possible that in male LrrkG2019S mice, the unique combination of dysregulated top-down control of NAc circuitry by mPFC (Britt et al., 2012) and/or the BLA (Stuber et al., 2011) suggested by lowered cFos densities in those structures, coupled with heightened activation of NAc neurons suggested by the elevated density of cFos-labeled cells, reflects abnormal network activity that ultimately increases motivation to feed and/or blunts anxiety associated with the novel arena. Previous studies of wildtype mice have shown that NSF produces elevated densities of cFos-labeled cells in NAc (in comparison with controls exploring an open-field arena), while optogenetically activating an anterior paraventricular thalamus-to-NAc projection during the NSF test increases motivation to consume food in a novel environment and increases time spent in the open arms of an elevated plus maze (Cheng et al., 2018), commonly interpreted as lowered anxiety. Additionally, other forms of chronic stress in wildtype mice increase glutamate release from mPFC terminals onto a subpopulation of BLA neurons that is correlated with heightened anxiety-like behaviors. Decreasing such stress-induced glutamate release reduces anxiety-like behaviors (Liu et al., 2020). Future studies could test the significance of the sex- and genotype-specific cFos labeling patterns described here by interrogating circuit-based contributions to behavioral outcomes.
One limitation in the design of the cFos labeling study is that while 28d-VS animals were sacrificed following a final restraint-stress session, there was no comparative group receiving only restraint-stress without prior 28d-VS, leaving some uncertainty as to whether differential cFos labeling patterns between Lrrk2G2019S and Lrrk2WT mice were driven by the cumulative effect of chronic stress, or were driven entirely by an acute episode of restraint stress. Previous studies of wildtype mice and rats have shown that a single episode of acute restraint stress generally increases cFos labeling density in many brain regions (Hsu et al., 2007; Kovács et al., 2018; Kim and Chung, 2021; Han et al., 2023). However, it should be emphasized that the goal here was to compare between genotypes how stressful experience shapes cFos labeling patterns, with less emphasis on exactly what the stressful experience was in either type or duration. Thus, the conclusion stands that a stressful experience drives cFos labeling patterns that differ between sex and genotype. Future studies could address how single episodes of any of the three stressors used here differentially and acutely impact cFos-labeling patterns between genotype.
The mechanistic basis for genotype- and sex-specific differences in anxiety-induced behavioral measures and cellular activation patterns remain speculative. Previous studies of Lrrk2WT and Lrrk2G2019S male mice subjected to 1-day social defeat stress show significant genotype-specific differences in synaptic properties and intrinsic excitability (Guevara et al., 2020), suggesting that the Lrrk2G2019S mutation drives different cellular and synaptic adaptations to other types or durations of behavioral stress. While we did not examine cellular or synaptic properties electrophysiologically or neurochemically in the present study, a previous study of wildtype mice exposed to sub-chronic (6d) variable stress using the same stressors showed significant changes in molecular markers of excitatory synapses in the NAc of female, but not male mice (Brancato et al., 2017). Thus, it is likely that 28d-VS drives both genotype- and sex-specific differences in synaptic and non-synaptic adaptations in several, if not all of the stress-relevant brain regions.
Finally, Lrrk2G2019S may be differentially influencing stress-relevant brain circuits through exogenous inflammatory mediators. Cells of the peripheral innate immune system express high levels of LRRK2 (Ahmadi Rastegar and Dzamko, 2020), many of which gain access to the brain following periods of behavioral stress, where they play significant roles in modulating cellular and synaptic function in stress-relevant brain regions (Hodes et al., 2015; Weber et al., 2017).
The original contributions presented in the study are included in the article/supplementary material, further inquiries can be directed to the corresponding authors.
The animal study was approved by Institutional Animal Care and Use Committee of the Icahn School of Medicine at Mount Sinai. The study was conducted in accordance with guidelines established by the National Institutes of Health and in accordance with and institutional requirements.
CG: Conceptualization, Data curation, Formal analysis, Investigation, Methodology, Validation, Writing – original draft, Writing – review & editing. KA: Data curation, Formal analysis, Investigation, Writing – review & editing. SG: Data curation, Formal analysis, Investigation, Writing – review & editing. RT: Investigation, Writing – review & editing. PV: Investigation, Writing – review & editing. AM: Investigation, Writing – review & editing. DB: Conceptualization, Formal analysis, Funding acquisition, Investigation, Methodology, Project administration, Supervision, Writing – review & editing. GH: Conceptualization, Funding acquisition, Project administration, Supervision, Writing – original draft, Writing – review & editing.
The author(s) declare that financial support was received for the research, authorship, and/or publication of this article. This research was supported by the NIH-NINDS R01NS107512 and R01NS135728 (GWH, DLB); NIH-NIMH T32MH087004 (CAG); NIH-NIA T32AG049688 (ARM); Rainwater Charitable Foundation (CAG, SG) and grants from the Parkinson’s Foundation, the Pritzker Pucker Family Foundation and Columbia University CCE-WEP Grant (KA).
We thank Matthew Fields, Kyomi Blake, Jamal Magoti, and Amelia Wieland for assistance in behavioral and cFos experiments.
The authors declare that the research was conducted in the absence of any commercial or financial relationships that could be construed as a potential conflict of interest.
All claims expressed in this article are solely those of the authors and do not necessarily represent those of their affiliated organizations, or those of the publisher, the editors and the reviewers. Any product that may be evaluated in this article, or claim that may be made by its manufacturer, is not guaranteed or endorsed by the publisher.
Ahmadi Rastegar, D., and Dzamko, N. (2020). Leucine rich repeat kinase 2 and innate immunity. Front. Neurosci. 14:193. doi: 10.3389/fnins.2020.00193
Badimon, A., Strasburger, H. J., Ayata, P., Chen, X., Nair, A., Ikegami, A., et al. (2020). Negative feedback control of neuronal activity by microglia. Nature 586, 417–423. doi: 10.1038/s41586-020-2777-8
Bittar, T. P., Pelaez, M. C., Hernandez Silva, J. C., Quessy, F., Lavigne, A.-A., Morency, D., et al. (2021). Chronic stress induces sex-specific functional and morphological alterations in Corticoaccumbal and Corticotegmental pathways. Biol. Psychiatry 90, 194–205. doi: 10.1016/j.biopsych.2021.02.014
Bodnoff, S. R., Suranyi-Cadotte, B., Quirion, R., and Meaney, M. J. (1989). A comparison of the effects of diazepam versus several typical and atypical anti-depressant drugs in an animal model of anxiety. Psychopharmacology 97, 277–279. doi: 10.1007/BF00442264
Brancato, A., Bregman, D., Ahn, H. F., Pfau, M. L., Menard, C., Cannizzaro, C., et al. (2017). Sub-chronic variable stress induces sex-specific effects on glutamatergic synapses in the nucleus accumbens. Neuroscience 350, 180–189. doi: 10.1016/j.neuroscience.2017.03.014
Britt, J. P., Benaliouad, F., McDevitt, R. A., Stuber, G. D., Wise, R. A., and Bonci, A. (2012). Synaptic and behavioral profile of multiple glutamatergic inputs to the nucleus accumbens. Neuron 76, 790–803. doi: 10.1016/j.neuron.2012.09.040
Broen, M. P. G., Narayen, N. E., Kuijf, M. L., Dissanayaka, N. N. W., and Leentjens, A. F. G. (2016). Prevalence of anxiety in Parkinson’s disease: a systematic review and meta-analysis. Mov. Disord. 31, 1125–1133. doi: 10.1002/mds.26643
Chari, T., Griswold, S., Andrews, N. A., and Fagiolini, M. (2020). The stage of the Estrus cycle is critical for interpretation of female mouse social interaction behavior. Front. Behav. Neurosci. 14:113. doi: 10.3389/fnbeh.2020.00113
Chen, Y., Gu, X., Ou, R., Zhang, L., Hou, Y., Liu, K., et al. (2020). Evaluating the role of SNCA, LRRK2, and GBA in Chinese patients with early-onset Parkinson’s disease. Mov. Disord. 35, 2046–2055. doi: 10.1002/mds.28191
Cheng, J., Wang, J., Ma, X., Ullah, R., Shen, Y., and Zhou, Y.-D. (2018). Anterior paraventricular thalamus to nucleus Accumbens projection is involved in feeding behavior in a novel environment. Front. Mol. Neurosci. 11:202. doi: 10.3389/fnmol.2018.00202
Cooper, S. E., Kechner, M., Caraballo-Pérez, D., Kaska, S., Robison, A. J., and Mazei-Robison, M. S. (2017). Comparison of chronic physical and emotional social defeat stress effects on mesocorticolimbic circuit activation and voluntary consumption of morphine. Sci. Rep. 7:8445. doi: 10.1038/s41598-017-09106-3
Cui, S.-S., Du, J.-J., Fu, R., Lin, Y.-Q., Huang, P., He, Y.-C., et al. (2017). Prevalence and risk factors for depression and anxiety in Chinese patients with Parkinson disease. BMC Geriatr. 17:270. doi: 10.1186/s12877-017-0666-2
Cunningham, A. M., Walker, D. M., Ramakrishnan, A., Doyle, M. A., Bagot, R. C., Cates, H. M., et al. (2021). Sperm transcriptional state associated with paternal transmission of stress phenotypes. J. Neurosci. 41, 6202–6216. doi: 10.1523/JNEUROSCI.3192-20.2021
DeBroff, J., Omer, N., Cohen, B., Giladi, N., Kestenbaum, M., Shirvan, J. C., et al. (2023). The influence of GBA and LRRK2 on mood disorders in Parkinson’s disease. Mov Disord Clin Pract 10, 606–616. doi: 10.1002/mdc3.13722
Freire-Cobo, C., and Wang, J. (2020). Dietary phytochemicals modulate experience-dependent changes in Neurexin gene expression and alternative splicing in mice after chronic variable stress exposure. Eur. J. Pharmacol. 883:173362. doi: 10.1016/j.ejphar.2020.173362
Furman, D. J., Hamilton, J. P., and Gotlib, I. H. (2011). Frontostriatal functional connectivity in major depressive disorder. Biol Mood Anxiety Disord 1:11. doi: 10.1186/2045-5380-1-11
Gabbay, V., Ely, B. A., Li, Q., Bangaru, S. D., Panzer, A. M., Alonso, C. M., et al. (2013). Striatum-based circuitry of adolescent depression and anhedonia. J. Am. Acad. Child Adolesc. Psychiatry 52, 628–641.e13. doi: 10.1016/j.jaac.2013.04.003
Gaig, C., Vilas, D., Infante, J., Sierra, M., García-Gorostiaga, I., Buongiorno, M., et al. (2014). Nonmotor symptoms in LRRK2 G2019S associated Parkinson’s disease. PLoS One 9:e108982. doi: 10.1371/journal.pone.0108982
Giesert, F., Hofmann, A., Bürger, A., Zerle, J., Kloos, K., Hafen, U., et al. (2013). Expression analysis of Lrrk1, Lrrk2 and Lrrk2 splice variants in mice. PLoS One 8:e63778. doi: 10.1371/journal.pone.0063778
Giovanniello, J. R., Paredes, N., Wiener, A., Ramírez-Armenta, K., Oragwam, C., Uwadia, H. O., et al. (2023). A dual-pathway architecture enables chronic stress to promote habit formation. Biorxiv. doi: 10.1101/2023.10.03.560731
Golden, S. A., Covington, H. E., Berton, O., and Russo, S. J. (2011). A standardized protocol for repeated social defeat stress in mice. Nat. Protoc. 6, 1183–1191. doi: 10.1038/nprot.2011.361
Guevara, C. A., Matikainen-Ankney, B. A., Kezunovic, N., LeClair, K., Conway, A. P., Menard, C., et al. (2020). LRRK2 mutation alters behavioral, synaptic, and nonsynaptic adaptations to acute social stress. J. Neurophysiol. 123, 2382–2389. doi: 10.1152/jn.00137.2020
Han, Y.-Y., Zhou, J.-W., Guo, Z.-W., Wu, Z.-Q., Zhang, Z.-Y., Liu, D.-X., et al. (2023). Multiple brain regions are involved in reaction to acute restraint stress in CYLD-knockout mice. Stress 26:2228925. doi: 10.1080/10253890.2023.2228925
Harro, J. (2018). Animals, anxiety, and anxiety disorders: how to measure anxiety in rodents and why. Behav. Brain Res. 352, 81–93. doi: 10.1016/j.bbr.2017.10.016
Healy, D. G., Falchi, M., O’Sullivan, S. S., Bonifati, V., Durr, A., Bressman, S., et al. (2008). Phenotype, genotype, and worldwide genetic penetrance of LRRK2-associated Parkinson’s disease: a case-control study. Lancet Neurol. 7, 583–590. doi: 10.1016/S1474-4422(08)70117-0
Hemmerle, A. M., Herman, J. P., and Seroogy, K. B. (2012). Stress, depression and Parkinson’s disease. Exp. Neurol. 233, 79–86. doi: 10.1016/j.expneurol.2011.09.035
Hodes, G. E., Kana, V., Menard, C., Merad, M., and Russo, S. J. (2015). Neuroimmune mechanisms of depression. Nat. Neurosci. 18, 1386–1393. doi: 10.1038/nn.4113
Hsu, H.-R., Chen, T.-Y., Chan, M.-H., and Chen, H.-H. (2007). Acute effects of nicotine on restraint stress-induced anxiety-like behavior, c-Fos expression, and corticosterone release in mice. Eur. J. Pharmacol. 566, 124–131. doi: 10.1016/j.ejphar.2007.03.040
Huntley, G. W., and Benson, D. L. (2020). Origins of Parkinson’s disease in brain development: insights from early and persistent effects of LRRK2-G2019S on striatal circuits. Front. Neurosci. 14:265. doi: 10.3389/fnins.2020.00265
Hussein, A., Tielemans, A., Baxter, M. G., Benson, D. L., and Huntley, G. W. (2022). Cognitive deficits and altered cholinergic innervation in young adult male mice carrying a Parkinson's disease Lrrk2(G2019S) knockin mutation. Exp. Neurol. 355:114145. doi: 10.1016/j.expneurol.2022.114145
Johnson, A., Rainville, J. R., Rivero-Ballon, G. N., Dhimitri, K., and Hodes, G. E. (2021). Testing the limits of sex differences using variable stress. Neuroscience 454, 72–84. doi: 10.1016/j.neuroscience.2019.12.034
Kerestes, R., Harrison, B. J., Dandash, O., Stephanou, K., Whittle, S., Pujol, J., et al. (2015). Specific functional connectivity alterations of the dorsal striatum in young people with depression. Neuroimage Clin. 7, 266–272. doi: 10.1016/j.nicl.2014.12.017
Kim, W., and Chung, C. (2021). Brain-wide cellular mapping of acute stress-induced activation in male and female mice. FASEB J. 35:e22041. doi: 10.1096/fj.202101287R
Kluss, J. H., Mamais, A., and Cookson, M. R. (2019). LRRK2 links genetic and sporadic Parkinson’s disease. Biochem. Soc. Trans. 47, 651–661. doi: 10.1042/BST20180462
Kovács, L. Á., Schiessl, J. A., Nafz, A. E., Csernus, V., and Gaszner, B. (2018). Both basal and acute restraint stress-induced c-Fos expression is influenced by age in the extended amygdala and brainstem stress Centers in male rats. Front. Aging Neurosci. 10:248. doi: 10.3389/fnagi.2018.00248
LeClair, K. B., Chan, K. L., Kaster, M. P., Parise, L. F., Burnett, C. J., and Russo, S. J. (2021). Individual history of winning and hierarchy landscape influence stress susceptibility in mice. eLife 10:e71401. doi: 10.7554/eLife.71401
Leentjens, A. F. G., Dujardin, K., Marsh, L., Martinez-Martin, P., Richard, I. H., and Starkstein, S. E. (2011). Symptomatology and markers of anxiety disorders in Parkinson’s disease: a cross-sectional study. Mov. Disord. 26, 484–492. doi: 10.1002/mds.23528
Lesage, S., Leclere, L., Lohmann, E., Borg, M., Ruberg, M., Durr, A., et al. (2007). Frequency of the LRRK2 G2019S mutation in siblings with Parkinson’s disease. Neurodegener. Dis. 4, 195–198. doi: 10.1159/000101844
Levy, D. R., Hunter, N., Lin, S., Robinson, E. M., Gillis, W., Conlin, E. B., et al. (2023). Mouse spontaneous behavior reflects individual variation rather than estrous state. Curr. Biol. 33, 1358–1364.e4. doi: 10.1016/j.cub.2023.02.035
Li, L., Durand-de Cuttoli, R., Aubry, A. V., Burnett, C. J., Cathomas, F., Parise, L. F., et al. (2023). Social trauma engages lateral septum circuitry to occlude social reward. Nature 613, 696–703. doi: 10.1038/s41586-022-05484-5
Lim, J., Bang, Y., Choi, J.-H., Han, A., Kwon, M.-S., Liu, K. H., et al. (2018). LRRK2 G2019S induces anxiety/depression-like behavior before the onset of motor dysfunction with 5-HT1A receptor upregulation in mice. J. Neurosci. 38, 1611–1621. doi: 10.1523/JNEUROSCI.4051-15.2017
Liu, W.-Z., Zhang, W.-H., Zheng, Z.-H., Zou, J.-X., Liu, X.-X., Huang, S.-H., et al. (2020). Identification of a prefrontal cortex-to-amygdala pathway for chronic stress-induced anxiety. Nat. Commun. 11:2221. doi: 10.1038/s41467-020-15920-7
Longo, F., Russo, I., Shimshek, D. R., Greggio, E., and Morari, M. (2014). Genetic and pharmacological evidence that G2019S LRRK2 confers a hyperkinetic phenotype, resistant to motor decline associated with aging. Neurobiol. Dis. 71, 62–73. doi: 10.1016/j.nbd.2014.07.013
Manouze, H., Ghestem, A., Poillerat, V., Bennis, M., Ba-M’hamed, S., Benoliel, J., et al. (2019). Effects of single cage housing on stress, cognitive, and seizure parameters in the rat and mouse pilocarpine models of epilepsy. eNeuro 6:ENEURO.0179-18.2019. doi: 10.1523/ENEURO.0179-18.2019
Marder, K., Wang, Y., Alcalay, R. N., Mejia-Santana, H., Tang, M.-X., Lee, A., et al. (2015). Age-specific penetrance of LRRK2 G2019S in the Michael J. Fox Ashkenazi Jewish LRRK2 consortium. Neurology 85, 89–95. doi: 10.1212/WNL.0000000000001708
Matikainen-Ankney, B. A., Kezunovic, N., Menard, C., Flanigan, M. E., Zhong, Y., Russo, S. J., et al. (2018). Parkinson’s disease-linked LRRK2-G2019S mutation alters synaptic plasticity and promotes resilience to chronic social stress in young adulthood. J. Neurosci. 38, 9700–9711. doi: 10.1523/JNEUROSCI.1457-18.2018
Matikainen-Ankney, B. A., Kezunovic, N., Mesias, R. E., Tian, Y., Williams, F. M., Huntley, G. W., et al. (2016). Altered development of synapse structure and function in striatum caused by Parkinson’s disease-linked LRRK2-G2019S mutation. J. Neurosci. 36, 7128–7141. doi: 10.1523/JNEUROSCI.3314-15.2016
McIlwain, K. L., Merriweather, M. Y., Yuva-Paylor, L. A., and Paylor, R. (2001). The use of behavioral test batteries: effects of training history. Physiol. Behav. 73, 705–717. doi: 10.1016/s0031-9384(01)00528-5
Morel, C., Montgomery, S. E., Li, L., Durand-de Cuttoli, R., Teichman, E. M., Juarez, B., et al. (2022). Midbrain projection to the basolateral amygdala encodes anxiety-like but not depression-like behaviors. Nat. Commun. 13:1532. doi: 10.1038/s41467-022-29155-1
Morgan, J. I., and Curran, T. (1989). Stimulus-transcription coupling in neurons: role of cellular immediate-early genes. Trends Neurosci. 12, 459–462. doi: 10.1016/0166-2236(89)90096-9
Muir, J., Tse, Y. C., Iyer, E. S., Biris, J., Cvetkovska, V., Lopez, J., et al. (2020). Ventral hippocampal afferents to nucleus Accumbens encode both latent vulnerability and stress-induced susceptibility. Biol. Psychiatry 88, 843–854. doi: 10.1016/j.biopsych.2020.05.021
Partridge, K. J., Olson, R., and Hillhouse, T. M. (2023). Methodological approach: using a within-subjects design in the marble burying assay. Behav. Pharmacol. 34, 494–499. doi: 10.1097/FBP.0000000000000752
Paylor, R., Spencer, C. M., Yuva-Paylor, L. A., and Pieke-Dahl, S. (2006). The use of behavioral test batteries, II: effect of test interval. Physiol. Behav. 87, 95–102. doi: 10.1016/j.physbeh.2005.09.002
Peter, I., and Strober, W. (2023). Immunological features of LRRK2 function and its role in the gut-brain Axis governing Parkinson’s disease. J. Parkinsons Dis. 13, 279–296. doi: 10.3233/JPD-230021
Rainville, J. R., Lipuma, T., and Hodes, G. E. (2022). Translating the transcriptome: sex differences in the mechanisms of depression and stress, revisited. Biol. Psychiatry 91, 25–35. doi: 10.1016/j.biopsych.2021.02.003
Rocha, E. M., Keeney, M. T., Di Maio, R., De Miranda, B. R., and Greenamyre, J. T. (2022). LRRK2 and idiopathic Parkinson’s disease. Trends Neurosci. 45, 224–236. doi: 10.1016/j.tins.2021.12.002
Russo, S. J., and Nestler, E. J. (2013). The brain reward circuitry in mood disorders. Nat. Rev. Neurosci. 14, 609–625. doi: 10.1038/nrn3381
San Luciano, M., Wang, C., Ortega, R. A., Giladi, N., Marder, K., Bressman, S., et al. (2017). Sex differences in LRRK2 G2019S and idiopathic Parkinson’s disease. Ann. Clin. Transl. Neurol. 4, 801–810. doi: 10.1002/acn3.489
Shanker, V., Groves, M., Heiman, G., Palmese, C., Saunders-Pullman, R., Ozelius, L., et al. (2011). Mood and cognition in leucine-rich repeat kinase 2 G2019S Parkinson’s disease. Mov. Disord. 26, 1875–1880. doi: 10.1002/mds.23746
Shanley, M. R., Miura, Y., Guevara, C. A., Onoichenco, A., Kore, R., Ustundag, E., et al. (2023). Estrous cycle mediates midbrain neuron excitability altering social behavior upon stress. J. Neurosci. 43, 736–748. doi: 10.1523/JNEUROSCI.1504-22.2022
Shiba, M., Bower, J. H., Maraganore, D. M., McDonnell, S. K., Peterson, B. J., Ahlskog, J. E., et al. (2000). Anxiety disorders and depressive disorders preceding Parkinson’s disease: a case-control study. Mov. Disord. 15, 669–677. doi: 10.1002/1531-8257(200007)15:4<669::aid-mds1011>3.0.co;2-5
Simon, P., Dupuis, R., and Costentin, J. (1994). Thigmotaxis as an index of anxiety in mice. Influence of dopaminergic transmissions. Behav. Brain Res. 61, 59–64. doi: 10.1016/0166-4328(94)90008-6
Simón-Sánchez, J., Herranz-Pérez, V., Olucha-Bordonau, F., and Pérez-Tur, J. (2006). LRRK2 is expressed in areas affected by Parkinson’s disease in the adult mouse brain. Eur. J. Neurosci. 23, 659–666. doi: 10.1111/j.1460-9568.2006.04616.x
Stuber, G. D., Sparta, D. R., Stamatakis, A. M., van Leeuwen, W. A., Hardjoprajitno, J. E., Cho, S., et al. (2011). Excitatory transmission from the amygdala to nucleus accumbens facilitates reward seeking. Nature 475, 377–380. doi: 10.1038/nature10194
Taymans, J.-M., Van den Haute, C., and Baekelandt, V. (2006). Distribution of PINK1 and LRRK2 in rat and mouse brain. J. Neurochem. 98, 951–961. doi: 10.1111/j.1471-4159.2006.03919.x
Tsyglakova, M., Huskey, A. M., Hurst, E. H., Telep, N. M., Wilding, M. C., Babington, M. E., et al. (2021). Sex and region-specific effects of variable stress on microglia morphology. Brain Behav. Immun. Health 18:100378. doi: 10.1016/j.bbih.2021.100378
Volta, M., Beccano-Kelly, D. A., Paschall, S. A., Cataldi, S., MacIsaac, S. E., Kuhlmann, N., et al. (2017). Initial elevations in glutamate and dopamine neurotransmission decline with age, as does exploratory behavior, in LRRK2 G2019S knock-in mice. eLife 6:e28377. doi: 10.7554/eLife.28377
Weber, M. D., Godbout, J. P., and Sheridan, J. F. (2017). Repeated social defeat, Neuroinflammation, and behavior: monocytes carry the signal. Neuropsychopharmacology 42, 46–61. doi: 10.1038/npp.2016.102
Westenbroek, C., Snijders, T. A. B., den Boer, J. A., Gerrits, M., Fokkema, D. S., and Ter Horst, G. J. (2005). Pair-housing of male and female rats during chronic stress exposure results in gender-specific behavioral responses. Horm. Behav. 47, 620–628. doi: 10.1016/j.yhbeh.2005.01.004
Wile, D. J., Agarwal, P. A., Schulzer, M., Mak, E., Dinelle, K., Shahinfard, E., et al. (2017). Serotonin and dopamine transporter PET changes in the premotor phase of LRRK2 parkinsonism: cross-sectional studies. Lancet Neurol. 16, 351–359. doi: 10.1016/S1474-4422(17)30056-X
Yahalom, G., Orlev, Y., Cohen, O. S., Kozlova, E., Friedman, E., Inzelberg, R., et al. (2014). Motor progression of Parkinson’s disease with the leucine-rich repeat kinase 2 G2019S mutation. Mov. Disord. 29, 1057–1060. doi: 10.1002/mds.25931
Yue, M., Hinkle, K. M., Davies, P., Trushina, E., Fiesel, F. C., Christenson, T. A., et al. (2015). Progressive dopaminergic alterations and mitochondrial abnormalities in LRRK2 G2019S knock-in mice. Neurobiol. Dis. 78, 172–195. doi: 10.1016/j.nbd.2015.02.031
Keywords: anxiety, depression, mice, open-field, novelty-suppressed feeding, social interaction, cFos, activity-mapping
Citation: Guevara CA, Alloo K, Gupta S, Thomas R, del Valle P, Magee AR, Benson DL and Huntley GW (2024) Parkinson’s LRRK2-G2019S risk gene mutation drives sex-specific behavioral and cellular adaptations to chronic variable stress. Front. Behav. Neurosci. 18:1445184. doi: 10.3389/fnbeh.2024.1445184
Received: 06 June 2024; Accepted: 27 August 2024;
Published: 12 September 2024.
Edited by:
Yasuhiro Kosuge, Nihon University, JapanReviewed by:
Aki Takahashi, University of Tsukuba, JapanCopyright © 2024 Guevara, Alloo, Gupta, Thomas, del Valle, Magee, Benson and Huntley. This is an open-access article distributed under the terms of the Creative Commons Attribution License (CC BY). The use, distribution or reproduction in other forums is permitted, provided the original author(s) and the copyright owner(s) are credited and that the original publication in this journal is cited, in accordance with accepted academic practice. No use, distribution or reproduction is permitted which does not comply with these terms.
*Correspondence: George W. Huntley, Z2VvcmdlLmh1bnRsZXlAbXNzbS5lZHU=; Deanna L. Benson, ZGVhbm5hLmJlbnNvbkBtc3NtLmVkdQ==
†ORCID: Christopher A. Guevara, orcid.org/0000-0001-6755-9317
Swati Gupta, orcid.org/0000-0003-1558-0469
Pamela del Valle, orcid.org/0000-0003-2053-8011
Alexandra R. Magee, orcid.org/0000-0003-2950-9268
Deanna L. Benson, orcid.org/0000-0001-7037-1147
George W. Huntley, orcid.org/0000-0003-3645-6192
Disclaimer: All claims expressed in this article are solely those of the authors and do not necessarily represent those of their affiliated organizations, or those of the publisher, the editors and the reviewers. Any product that may be evaluated in this article or claim that may be made by its manufacturer is not guaranteed or endorsed by the publisher.
Research integrity at Frontiers
Learn more about the work of our research integrity team to safeguard the quality of each article we publish.