- Department of Anesthesiology, Sichuan Cancer Hospital & Institute, Sichuan Cancer Center, Affiliated Cancer Hospital of University of Electronic Science and Technology of China, Chengdu, China
Postoperative cognitive dysfunction (POCD) commonly occurs after surgery, particularly in elderly individuals. It is characterized by a notable decline in cognitive performance, encompassing memory, attention, coordination, orientation, verbal fluency, and executive function. This reduction in cognitive abilities contributes to extended hospital stays and heightened mortality. The prevalence of POCD can reach 40% within 1 week following cardiovascular surgery and remains as high as 17% 3 months post-surgery. Furthermore, POCD exacerbates the long-term risk of Alzheimer’s disease (AD). As a result, numerous studies have been conducted to investigate the molecular mechanisms underlying POCD and potential preventive strategies. This article provides a review of the research progress on POCD.
1 Introduction
Postoperative cognitive dysfunction (POCD) denotes a decline in neurocognitive function following anesthesia and surgical procedures, constituting a complication of the central nervous system (Rundshagen, 2014). Typically observed in individuals aged 65 and older (Evered and Silbert, 2018), POCD manifests primarily as reduced memory, attention, language fluency, orientation, and social skills after surgery (Needham et al., 2017). Since its inception (Rundshagen, 2014), substantial research has been conducted on POCD; however, a universally acknowledged specific pathogenesis remains elusive. Currently, it is widely accepted that POCD arises from the confluence of various factors, including patient age, surgical type, anesthesia modality, and pain intensity (Mason et al., 2010; Miller et al., 2018; Skvarc et al., 2018; Gu et al., 2019; Xiao et al., 2020). Moreover, studies indicate that POCD may persist for weeks to years, impacting patient recovery, prolonging hospitalization, and potentially leading to additional physical and mental ailments, heightened mortality, and significant burdens on patients and their families (Steinmetz et al., 2009). The ongoing trend of population aging, coupled with economic development, poses a challenge for anesthesia surgery, with an increasingly aged patient population. As patients undergoing surgery grow older, the likelihood of developing postoperative cognitive dysfunction rises. Consequently, addressing the identification and prevention of POCD has become a paramount concern. This article reviews POCD, encompassing epidemiology, risk factors, pathogenesis, and potential prevention strategies, while offering insights into future prospects for POCD prevention and treatment.
2 Definition and diagnostic criteria of POCD
Postoperative cognitive dysfunction (POCD), as per the cognitive impairment classification in the DSM-V, is characterized as a mild neurological disorder resulting from routine surgical procedures, excluding conditions like deafness, dementia, or amnesia (Edition, 2013). POCD entails a protracted cognitive decline lasting weeks, months, or even years and can be mistakenly conflated with postoperative delirium, presenting as an acute, fluctuating disturbance of consciousness typically within 1–3 days post-surgery (Steinmetz et al., 2009; Whitlock et al., 2011).
The diagnosis of POCD primarily relies on neurocognitive function scales, with widely used assessments including the Montreal Cognitive Assessment (MoCA), Wechsler Memory Scale (WMS), Wechsler Adult Intelligence Scale (WAIS), and Mini-Mental State Examination (MMSE) (Bouman et al., 2014; Chi et al., 2017; Pinto et al., 2019; Elkana et al., 2020). To mitigate biases stemming from external factors such as cultural backgrounds, education levels, and environmental or emotional states, multiple scales are often employed in combination when evaluating patients’ neurocognitive function (Ghoneim and Block, 2012; Needham et al., 2017). Following neurocognitive function evaluation, the diagnosis of POCD is commonly determined using methods like the SD method or Z-score method. The Z-score method involves selecting healthy elderly individuals for a baseline test, performing the test again after 1 week, and obtaining the mean ± standard deviation of the difference value between the two tests for healthy elderly individuals, defined as the “learning effect.” The patients’ preoperative scores are then subtracted from the postoperative scores, adjusting for the learning effect. The absolute value of the result is divided by the standard deviation of the healthy group to calculate the Z value ( , L means the learning effect, s1 means the first test points in healthy people, s2 means the test points in healthy people after 1 week, n means the number of the including people; , , S1 means the test scores in patients preoperative, S2 means the test scores in patients postoperative, SD means the standard deviation)When a patient has 2 or more Z-values greater than 1.96, the patient is considered to occur POCD (Moller et al., 1998). SD method: First, the preoperative scores of all patients included in the study are calculated to obtain the mean ± standard deviation, , , S means the test scores in patients preoperative, n means the number of the including patients). After subtracting the preoperative scores from the postoperative scores, the absolute value of the result is calculated. Postoperative deficits are identified as negative change values exceeding the standard deviation. In this context, a patient is deemed to have experienced POCD if they exhibit two or more neurocognitive test deficits (Saleh et al., 2015).
3 Epidemiology of POCD
POCD is prevalent among patients following cardiac or orthopedic surgery (Belrose and Noppens, 2019). Specifically, in individuals undergoing coronary artery bypass grafting and cardiopulmonary bypass, 50–70% develop POCD 1 week post-surgery (O'Gara et al., 2020). Furthermore, 10–30% experience long-term cognitive function effects 6 months after the procedure (Ghaffary et al., 2017). Among those undergoing hip arthroplasty, 20–50% encounter POCD within 1 week after surgery (Zhu et al., 2014; Li et al., 2019), with 10–14% still exhibiting POCD 3 months post-surgery (Krenk et al., 2014; Konishi et al., 2018).
Moreover, POCD is more prevalent in elderly patients, and its incidence increases with age (Moller et al., 1998). Studies indicate that, in non-cardiac surgery patients, those over 60 years old have a 1.5 times higher incidence of POCD compared to their younger counterparts. Additionally, the duration of POCD is longer in elderly patients than in younger ones (Monk et al., 2008).
4 Risk factors of POCD
4.1 Age
In clinical settings, the aging trend of surgical patients is evident, resulting in a relatively high proportion of elderly patients experiencing POCD post-surgery (Moller et al., 1998). Research indicates that around 30% of younger patients and approximately 40% of older patients develop POCD upon hospital discharge. Notably, 12.7% of elderly patients remain in POCD 3 months after surgery, while only 5% of their younger counterparts persist in POCD (Monk et al., 2008). The physiological decline in elderly patients, coupled with potential respiratory, cardiovascular, and cerebrovascular diseases, significantly diminishes their tolerance to anesthesia (Dodds and Allison, 1998; Ancelin et al., 2010). Consequently, the incidence of POCD is markedly higher in elderly patients.
4.2 Types of surgery
Osteoarthritis is a prevalent joint ailment in elderly patients, often addressed through hip and knee arthroplasty procedures (Abramoff and Caldera, 2020). Despite the maturity of these surgeries in enhancing joint function and alleviating pain (Canovas and Dagneaux, 2018; Lu and Phillips, 2019), the risk of POCD remains elevated compared to general surgery. This heightened risk is attributed to prolonged surgery durations, increased surgical trauma, and the potential occurrence of fat embolism (Krenk et al., 2014).
Coronary artery disease is a highly lethal condition worldwide, and its incidence and fatality rates have risen recently. Coronary artery bypass grafting is widely acknowledged as the most efficient treatment for coronary artery disease; however, it is associated with an elevated risk of POCD, particularly following coronary artery bypass grafting and cardiopulmonary bypass (CPB) (Wan et al., 2020). POCD primarily stems from inadequate perfusion during cardiac surgery, cerebral microembolism, and inflammation (Pappa et al., 2017). Within the CPB process, the cardiopulmonary bypass machine circulates microemboli like air bubbles, fat, and blood clots into the brain. Additionally, clamping the aorta during the procedure may dislodge atherosclerotic plaques, leading to embolism. A study observed minute emboli in the brains of patients who succumbed after undergoing coronary artery bypass grafting with cardiopulmonary bypass (CABG-CPB) (Moody et al., 1995). Head MRI studies have verified that around 50% of CABG recipients undergo brain microembolic infarction, resulting in mild neurological impairment (Knipp et al., 2008). It is confirmed that the immune system is activated during CPB when blood components interact with artificial materials. This activation triggers widespread systemic inflammatory responses, causing reperfusion injury to organs. Furthermore, peripheral inflammatory factors breach the blood–brain barrier, inducing central nervous system inflammation and POCD (Day and Taylor, 2005).
Table 1 compare the incidence of POCD in various age groups following cardiac and non-cardiac surgeries. Due to a lower frequency of cardiac surgeries in younger individuals, Table 2 exclusively presents the morbidity of POCD in elderly patients.
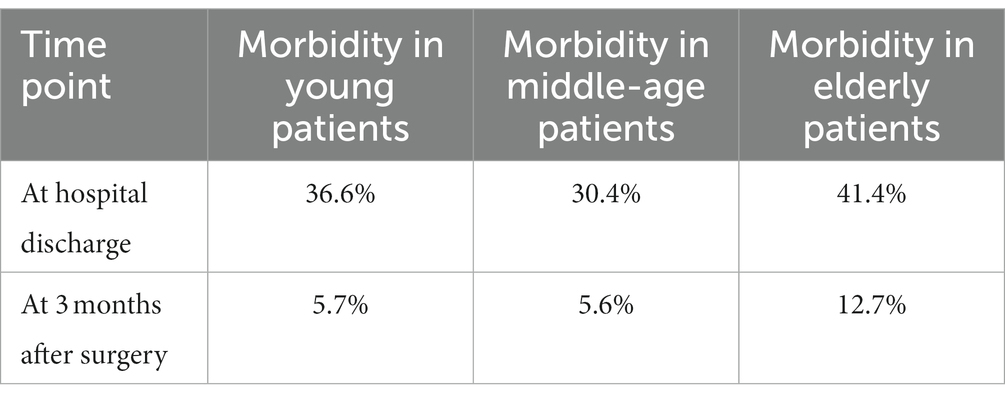
Table 1. Morbidity in age-matched patients (non-cardiac surgery) (Monk et al., 2008).
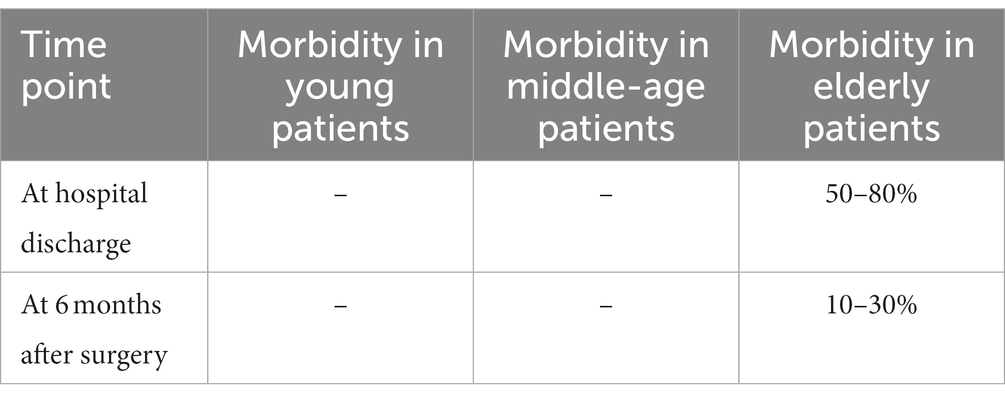
Table 2. Morbidity in elderly patients (cardiac surgery) (Ghaffary et al., 2017).
4.3 Types of anesthesia
According to the surgical requirements, the anesthesiologist will opt for either intravertebral anesthesia or general anesthesia. Previous research indicates that there is no discernible difference in the occurrence of POCD between knee arthroplasty patients undergoing either general or intravertebral anesthesia 1 week and 6 months post-surgery (Williams-Russo et al., 1995). In a study, patients undergoing hip arthroplasty were randomly assigned to three groups receiving sedation with midazolam, dexmedetomidine, or propofol under spinal-epidural block. The incidence of POCD in the propofol group 1 week after surgery was notably lower than in the midazolam and dexmedetomidine groups (Li et al., 2019). The combination of midazolam and isoflurane is a widely employed anesthesia approach. An investigation utilizing this combination for rat anesthesia revealed widespread apoptosis of hippocampal neurons and dysfunction in hippocampal synapses, potentially contributing to cognitive function decline (Jevtovic-Todorovic et al., 2003). A meta-analysis of 28 Randomized Controlled Trials (RCTs) concluded that the incidence of POCD is lower in surgeries maintaining intravenous anesthesia with propofol compared to those using inhalation anesthesia with isoflurane or sevoflurane (Miller et al., 2018).
4.4 Pain management
After surgery, postoperative pain is a common issue, mainly stemming from substantial surgical trauma or the potential for wound infection. Consequently, prophylactic antibiotics are administered following certain operations, and patients are equipped with analgesia pumps to alleviate pain. A study revealed that utilizing a postoperative patient-controlled analgesia pump independently increased the risk of POCD. In comparison to those who received intravenous opioids through a patient-controlled analgesia device, individuals who orally received postoperative analgesia faced a significantly lower risk of developing POCD (Wang et al., 2007). Su et al. demonstrated that incorporating dexmedetomidine as an adjunct, as opposed to routine postoperative analgesia, could reduce pain and enhance postoperative cognitive function (Su et al., 2022). Additionally, meta-analyses on pain indicate that persistent pain may lead to a decline in patients’ cognitive abilities, attention, memory, and information processing (Berryman et al., 2013; Mazza et al., 2018).
4.5 Education level
Individuals with higher education levels may experience a lower occurrence of POCD. In this educated demographic, the brain consistently engages in challenging intellectual tasks, bolstering neuronal reserve and synaptic function. In instances of surgical trauma, a robust brain reserve could mitigate hippocampal damage, consequently diminishing cognitive decline post-surgery (Kotekar et al., 2018). Higher-educated patients exhibit enhanced tolerance to pathological changes induced by neurodegenerative diseases. Additionally, those with greater cultural reserve demonstrate a deceleration in the aging process and cognitive function decline (Stern, 2012).
5 Pathogenesis of POCD
5.1 Central inflammation
The emergence of POCD is now associated with the inflammatory response within the central nervous system. Studies affirm that, following surgical trauma, peripheral inflammatory factors breach the blood–brain barrier (BBB), causing central nervous system inflammation (Alam et al., 2018; Zhu et al., 2018). Cibelli et al. observed that IL-1 receptor knockout mice, subjected to tibial fracture, maintained unimpaired cognitive function with no increase in IL-1β levels. They proposed that surgery-induced innate immune responses activate IL-1β-mediated inflammatory reactions in the hippocampus, resulting in cognitive impairment (Cibelli et al., 2010). Feng et al. demonstrated that mice with depleted microglia did not develop POCD after tibial fracture, and hippocampal inflammation remained unaltered. This suggests that surgery-induced microglia activation contributes to hippocampal inflammation, with activated microglia promoting the entry of peripheral inflammatory factors into the nervous system and exacerbating inflammation levels (Feng et al., 2017). Bowman et al. hypothesized that BBB injury induces POCD in patients. Those with BBB injury exhibited a generally increased concentration of inflammatory factors in cerebrospinal fluid, strongly implicating nervous system inflammation in the genesis of POCD (Bowman et al., 2018).
5.2 Neuronal apoptosis
Neurons form the foundation of cognitive function. Cognitive decline, memory loss, diminished learning abilities, and emotional changes in patients can result from brain damage caused by neuronal apoptosis. While neurons in the adult brain possess a self-healing capacity through regeneration, aging diminishes this ability (Vasic et al., 2019). Sun et al. observed a significantly higher apoptosis index of hippocampal neurons in AD mice compared to the wild-type control group, as indicated by TUNEL staining. Treatment of AD mice with dexmedetomidine led to a reduction in hippocampal neuronal apoptosis and an improvement in cognitive function (Sun et al., 2020). Yuan et al., in their POCD model using aged mice with the left extrahepatic lobe resected, proposed that the activation of the NF-κB pathway in neurons triggers neuronal apoptosis and autophagy, resulting in cognitive impairment (Yuan et al., 2022). Shao et al. induced the POCD model through sevoflurane and discovered that Chikusetsu saponin IVa (ChIV) exerts a neuroprotective effect against sevoflurane-induced neuroinflammation and cognitive impairment by inhibiting the NLRP3/caspase-1 pathway. This suggests that ChIV could be a promising clinical strategy for treating anesthesia-induced POCD in elderly patients (Shao et al., 2020).
5.3 Synaptic plasticity impairment
Synaptic plasticity denotes the autonomous adjustment of connection strength between nerve cell synapses, leading to short-term (inhibition, facilitation, enhancement) and long-term (long-term potentiation, long-term inhibition) changes in synaptic morphology and function (Magee and Grienberger, 2020). This phenomenon forms the neural basis for memory and learning mechanisms (Martin et al., 2000; Magee and Grienberger, 2020). Post-synaptic density protein 95 (PSD95) plays a crucial role in synaptic plasticity. Gao et al. observed that surgical and anesthesia stimulation led to a reduction in synaptophysin and PSD95 in the mouse hippocampus, impairing postoperative cognitive function (Gao et al., 2021). In a POCD model induced by exploratory laparotomy under anesthesia in aged mice, Li et al. identified increased expression of histone deacetylase 3 in the dorsal hippocampus, accompanied by significantly decreased levels of dendritic spine density and synaptic plasticity-related proteins. Selective blockade of histone deacetylase 3 in the dorsal hippocampus restored dendritic spine density and synaptic plasticity-related proteins, resulting in the reversal of postoperative cognitive decline (Yang et al., 2022). Similarly, Luo et al. discovered that surgery elevated the activity of histone deacetylase 2 and decreased dendritic arborization and spine density in the hippocampus of POCD mice. Intraperitoneal injection of suberanilohydroxamic acid, a relatively specific inhibitor of histone deacetylase 2, reversed these surgery-induced effects (Luo et al., 2020). Xiao et al. reported that inhibiting prostaglandin E2-receptor3 through dorsal hippocampus stereotaxic virus injection rescued the expression of plasticity-related proteins, including cAMP response element-binding protein and activity-regulated cytoskeletal-associated protein, alleviating surgery-induced cognitive decline (Xiao et al., 2018).
5.4 Abnormal modification of tau protein
Tau protein, a key member of the tubulin family, plays a crucial role in microtubule assembly and forms the structural foundation of the neural cytoskeleton (Ukmar-Godec et al., 2020). In physiological conditions, Tau protein undergoes a dynamic balance of phosphorylation and dephosphorylation, maintaining the stability of the neural cytoskeleton structure. Hyperphosphorylation of Tau protein results in the formation of neurofibrillary tangles, triggering neuronal apoptosis and contributing to neurodegenerative diseases (Avila et al., 2004; Pîrşcoveanu et al., 2017). Yan et al. observed a significant increase in phosphorylated Tau protein levels in the hippocampal tissue and cultured neurons of an aged POCD mouse model (Yan et al., 2020). Mattsson et al. established a correlation between the levels of total tau protein and phosphorylated tau protein in cerebrospinal fluid and the disease state of AD. These proteins can serve as biomarkers predicting the onset of AD (Mattsson et al., 2017). Dai et al. isolated phosphorylated Tau protein from the brains of deceased AD patients and injected it into the hippocampus of AD mice. In half of the mice, monoclonal antibody 43D was administered intravenously to eliminate pathological tau protein. Treatment with the 43D monoclonal antibody effectively hindered the abnormal modification and aggregation of Tau protein, blocking its spread to the contralateral hippocampus. This finding suggests that phosphorylated Tau protein may play a crucial role in the progression of AD (Dai et al., 2018).
5.5 Pain
Pain, a multifaceted physiological and psychological experience, is a prevalent clinical symptom with potential links to the onset of POCD. Berryman et al. discovered that individuals experiencing chronic pain exhibited poorer performance on working memory tests compared to healthy controls (Berryman et al., 2013). Simultaneously, executive abilities were also observed to be diminished in patients with chronic pain compared to their healthy counterparts (Berryman et al., 2014). Liu et al. identified severe memory impairment in patients with chronic pain, suggesting that pain might induce distraction and reduce memory capacity (Liu et al., 2014, 2021). Veldhuijzen et al. noted impaired problem-solving skills and decreased speed in individuals with fibromyalgia (Veldhuijzen et al., 2012). Establishing a rat model of sciatic nerve chronic constriction injury (CCI), Zhang et al. observed the development of POCD persisting for at least 21 days post-surgery in the CCI group (Zhang et al., 2021). Ding et al. reported compromised learning and memory abilities in a mouse model of sciatic nerve CCI, suggesting that CCI-induced chronic pain might exacerbate POCD through alterations in neurotransmitter levels and inflammatory factors (Ding et al., 2021). Pain significantly influences various neurodegenerative diseases, including AD, often underestimated due to communication challenges faced by AD patients (Cravello et al., 2019). Notably, pain levels in AD patients positively correlate with disease severity (Scherder et al., 2008; Whitlock et al., 2017). Shared pathological mechanisms, such as abnormal noradrenergic system function, microglial activation in the frontal cortex, and heightened inflammation, underlie both AD and pain (Salter and Stevens, 2017; Hayashida and Obata, 2019). Chronic pain and cognitive decline share intertwined pathological pathways, with the progression of pain intricately linked to the development of cognitive impairment.
5.6 Mitochondrial metabolic disorder
Aging, a natural biological process, is marked by the decline of bioenergy (López-Otín et al., 2013). Mitochondrial metabolism, a central process in bodily capacitation, is closely linked to diseases associated with aging (Natarajan et al., 2020). Yang et al. observed increased mitochondrial fragmentation in the hippocampus of POCD mice, linking neuroinflammation to mitochondrial dysfunction in primary cells. Enhancing mitochondrial function effectively improved postoperative cognitive function in mice (Yang et al., 2021). In a rat model of POCD, Netto et al. identified neuroinflammation and oxidative stress as sources of free radicals that activate the central nervous immune system, impair mitochondrial function, influence the production of brain-derived neurotrophic factor (BDNF), and contribute to the occurrence of POCD (Netto et al., 2018). Han et al. demonstrated that high expression of PGC-1α in the hippocampus improves cognitive function in mice. This increase in expression enhances mitochondrial antioxidant activity, inhibits neuroinflammation and reactive oxygen species production, boosts neuronal metabolic activity, and reduces mitochondrial damage, presenting a potential target for POCD treatment (Han et al., 2020).
5.7 Neurotrophic factor
Brain-derived neurotrophic factor (BDNF) exerts neurotrophic effects, with widespread expression of BDNF and its receptors in the central nervous system. Their primary role involves enhancing synaptic plasticity and promoting neurogenesis in the hippocampus, crucial for brain learning and memory function (Lu et al., 2013). In patients with vascular cognitive impairment, Wang et al. identified reduced BDNF expression in the serum. Cell experiments revealed that negative regulation of BDNF expression triggered nerve cell apoptosis, leading to cognitive impairment (Wang et al., 2020). Qiu et al. observed that anesthesia-induced neuroinflammation resulted in dysregulation of the BDNF/TrkB signaling pathway, causing dendritic spine loss and apoptosis, leading to POCD in mice (Qiu et al., 2020). Amidfar et al. found that beta-amyloid inhibits BDNF expression, contributing to cognitive decline (Amidfar et al., 2020). Successfully constructing a dementia model through entorhinal cortex synapse damage, Ando et al. overexpressed the BDNF gene in the entorhinal cortex, demonstrating that high BDNF expression could reverse cognitive decline (Ando et al., 2002).
6 Prevention of POCD
Current research indicates that POCD results from various factors, with hospitals generally lacking awareness of early prevention strategies for the condition. Therefore, early prevention emerges as a crucial strategy to reduce the incidence of POCD.
6.1 Identify biomarkers
Early prediction of POCD is pivotal for proactively addressing potential issues and formulating preventive plans. Duan et al. discovered that serum glial cell line-derived neurotrophic factor (GDNF) serves as a predictive biomarker for POCD diagnosis. ΔGDNF on the second day postoperatively demonstrated early predictive efficacy for POCD, with a threshold set at 49.1 (Duan et al., 2018). Liu et al. established a link between the occurrence of POCD and the concentration of peripheral inflammatory factors, identifying C-reactive protein and IL-6 as the most significant markers in this context (Liu et al., 2018). Given the variety of studies on POCD-related biomarkers, selecting appropriate biomarkers can enhance predictive accuracy for the onset of POCD.
6.2 Cognitive function training
Saleh et al. demonstrated that preoperative cognitive function training, such as the Method of Loci (MoL), could effectively prevent the occurrence of POCD (Saleh et al., 2015). O’Gara et al. employed a mobile application to train patients in memory, attention, problem-solving, flexibility, and processing speed until 1 month post-surgery. Although there was no significant difference in the incidence of POCD between the cognitive training group and the routine nursing group, it was noted that the complexity of the training might have reduced patient compliance. However, the cognitive training group exhibited significantly better memory and cognitive abilities than the routine nursing group. Hence, there is a need for further development and design of appropriate cognitive training programs to maximize benefits (O'Gara et al., 2020). Humeidan et al. utilized electronic tablets for preoperative cognitive exercises targeting memory, speed, attention, flexibility, and problem-solving functions. The intervention was found to reduce the risk of delirium in patients who were minimally compliant at the very least (Humeidan et al., 2021).
6.3 Rational use of drug in the perioperative period
Liang et al. demonstrated that perioperative administration of ulinastatin could reduce postoperative inflammation levels and lower the incidence of POCD (Lv et al., 2016; Liang et al., 2021). Wang et al. found that patients receiving lidocaine exhibited a reduced occurrence of POCD, potentially attributable to lidocaine’s neuroprotective effects (Wang et al., 2002). Similarly, Chen et al. reported the effectiveness of continuous lidocaine infusion during surgery in reducing POCD incidence among elderly patients undergoing spinal surgery (Chen et al., 2015). However, Mathew et al. found no significant difference in POCD incidence between the lidocaine treatment group and the placebo treatment group (Mathew et al., 2009). Su et al. observed that prophylactic low-dose dexmedetomidine during the perioperative period resulted in a lower incidence of postoperative delirium and fewer adverse cardiovascular events, affirming the safety and efficacy of dexmedetomidine in preventing cognitive decline (Su et al., 2016). Conversely, Deiner et al. reported that intraoperative dexmedetomidine did not reduce the incidence of POCD (Deiner et al., 2017). Valentin et al. found that intravenous dexamethasone administered before general anesthesia effectively reduced the incidence of POCD, particularly in the memory and executive cognitive domains (Valentin et al., 2016). Papadopoulos et al. discovered that postoperative administration of anstenchon could reduce the incidence of POCD after hip arthroplasty, providing relief from patient pain (Papadopoulos et al., 2014). It is evident that current research on perioperative medication to reduce the incidence of POCD remains controversial, as the drug concentration, timing of use, and duration of administration all play crucial roles.
6.4 Multidisciplinary collaboration
Multidisciplinary collaboration holds significant value in preventing POCD. The synergy between anesthesiologists and surgeons fosters effective communication and cooperation, aiding in the selection of optimal anesthesia and surgical plans. This collaborative effort enables cautious use of anesthesia drugs, optimization of patient admission and operation times, and reduction of postoperative complications. Timely communication between anesthesiologists and surgical departments enhances postoperative pain management, nutrition management, and rehabilitation, contributing to improved patient outcomes. Interaction between anesthesiologists and patients, along with their families, encourages swift reintegration into society. Family support helps alleviate post-surgery anxiety in patients, reducing the likelihood of POCD occurrence (Egerod et al., 2010; Laalou et al., 2011).
7 Discussion
POCD is a multifactorial neurodegenerative disease influenced by various factors, including patient age, surgery type, anesthesia plan, postoperative pain, and educational level, all of which contribute to the risk of POCD. This condition commonly manifests after cardiac and orthopedic surgeries, often persisting for weeks to months, with some cases enduring for several years, impacting the recovery process and increasing mortality rates.
While neurocognitive evaluation remains the optimal method for identifying POCD, patient compliance may sometimes be challenging. Consequently, the search for a highly specific and sensitive biomarker as a predictor of POCD becomes imperative. Identification of high-risk patients enables surgeons and anesthesiologists to exercise greater caution during surgery, minimizing the likelihood of POCD occurrence. Current research on POCD has delved into its pathogenesis, implicating factors such as central nervous system inflammation, neuronal apoptosis, synaptic plasticity damage, abnormal modification of Tau protein, chronic pain, and mitochondrial metabolic disorders. Several studies have confirmed the efficacy of certain drugs and cognitive training in preventing POCD. Appropriate and timely application of these interventions proves effective in reducing the incidence of POCD.
In contemporary research, the construction of the POCD model has reached a mature stage, employing various methods such as unilateral nephrectomy under anesthesia in aged mice (Liu et al., 2023), exploratory laparotomy under anesthesia in aged mice (Qiu et al., 2020), resection of the left extrahepatic lobe in aged mice (Yuan et al., 2022), and inducing a tibia fracture via orthopedic surgery in middle-aged rats (Garrone et al., 2021). Cognitive function assessment involves tests such as the fear conditioning test, open field test, Morris Water Maze test, Y-Maze test, among others. Through extensive animal experiments, scientists have identified various signal pathways associated with POCD, including the NF-κB pathway in central inflammation, the caspase protein family in neuronal apoptosis, and TLRs in inflammation and pain (Chen et al., 2019).
As of now, substantial progress has been made in understanding POCD, and effective methods for its prevention and treatment have been identified. The anticipation is that future research will delve further into POCD treatment. Drawing from the current knowledge of pathogenic pathways, targeted therapy drugs may be developed to address POCD. Potential targets include Tau protein, NF-κB pathway, caspase protein family, IL-1β, and Aβ. Such targeted therapies hold promise in safeguarding the well-being of patients affected by POCD.
Author contributions
QZ: Funding acquisition, Writing – original draft, Writing – review & editing. HW: Software, Writing – original draft, Writing – review & editing. HP: Software, Writing – review & editing. YX: Funding acquisition, Writing – review & editing.
Funding
The author(s) declare financial support was received for the research, authorship, and/or publication of this article. This study was funded by the Sichuan Cancer Hospital Youth Foundation (No. YB2023021 to QZ).
Conflict of interest
The authors declare that the research was conducted in the absence of any commercial or financial relationships that could be construed as a potential conflict of interest.
Publisher’s note
All claims expressed in this article are solely those of the authors and do not necessarily represent those of their affiliated organizations, or those of the publisher, the editors and the reviewers. Any product that may be evaluated in this article, or claim that may be made by its manufacturer, is not guaranteed or endorsed by the publisher.
References
Abramoff, B., and Caldera, F. E. (2020). Osteoarthritis: pathology, diagnosis, and treatment options. Med. Clin. North Am. 104, 293–311. doi: 10.1016/j.mcna.2019.10.007
Alam, A., Hana, Z., Jin, Z., Suen, K. C., and Ma, D. (2018). Surgery, neuroinflammation and cognitive impairment. EBioMedicine 37, 547–556. doi: 10.1016/j.ebiom.2018.10.021
Amidfar, M., de Oliveira, J., Kucharska, E., Budni, J., and Kim, Y. K. (2020). The role of CREB and BDNF in neurobiology and treatment of Alzheimer's disease. Life Sci. 257:118020. doi: 10.1016/j.lfs.2020.118020
Ancelin, M. L., de Roquefeuil, G., Scali, J., Bonnel, F., Adam, J. F., Cheminal, J. C., et al. (2010). Long-term post-operative cognitive decline in the elderly: the effects of anesthesia type, apolipoprotein E genotype, and clinical antecedents. J. Alzheimers Dis. 22 Suppl 3, 105–113. doi: 10.3233/jad-2010-100807
Ando, S., Kobayashi, S., Waki, H., Kon, K., Fukui, F., Tadenuma, T., et al. (2002). Animal model of dementia induced by entorhinal synaptic damage and partial restoration of cognitive deficits by BDNF and carnitine. J. Neurosci. Res. 70, 519–527. doi: 10.1002/jnr.10443
Avila, J., Lucas, J. J., Perez, M., and Hernandez, F. (2004). Role of tau protein in both physiological and pathological conditions. Physiol. Rev. 84, 361–384. doi: 10.1152/physrev.00024.2003
Belrose, J. C., and Noppens, R. R. (2019). Anesthesiology and cognitive impairment: a narrative review of current clinical literature. BMC Anesthesiol. 19:241. doi: 10.1186/s12871-019-0903-7
Berryman, C., Stanton, T. R., Bowering, K. J., Tabor, A., McFarlane, A., and Moseley, G. L. (2014). Do people with chronic pain have impaired executive function? A meta-analytical review. Clin. Psychol. Rev. 34, 563–579. doi: 10.1016/j.cpr.2014.08.003
Berryman, C., Stanton, T. R., Jane Bowering, K., Tabor, A., McFarlane, A., and Lorimer Moseley, G. (2013). Evidence for working memory deficits in chronic pain: a systematic review and meta-analysis. Pain 154, 1181–1196. doi: 10.1016/j.pain.2013.03.002
Bouman, Z., Hendriks, M. P., Aldenkamp, A. P., and Kessels, R. P. (2014). Clinical validation of the WMS-IV-NL brief cognitive status exam (BCSE) in older adults with MCI or dementia. Int. Psychogeriatr. 27, 221–229. doi: 10.1017/s1041610214001471
Bowman, G. L., Dayon, L., Kirkland, R., Wojcik, J., Peyratout, G., Severin, I. C., et al. (2018). Blood-brain barrier breakdown, neuroinflammation, and cognitive decline in older adults. Alzheimers Dement. 14, 1640–1650. doi: 10.1016/j.jalz.2018.06.2857
Canovas, F., and Dagneaux, L. (2018). Quality of life after total knee arthroplasty. Orthop. Traumatol. Surg. Res. 104, S41–s46. doi: 10.1016/j.otsr.2017.04.017
Chen, C., Gao, R., Li, M., Wang, Q., Chen, H., Zhang, S., et al. (2019). Extracellular RNAs-TLR3 signaling contributes to cognitive decline in a mouse model of postoperative cognitive dysfunction. Brain Behav. Immun. 80, 439–451. doi: 10.1016/j.bbi.2019.04.024
Chen, K., Wei, P., Zheng, Q., Zhou, J., and Li, J. (2015). Neuroprotective effects of intravenous lidocaine on early postoperative cognitive dysfunction in elderly patients following spine surgery. Med. Sci. Monit. 21, 1402–1407. doi: 10.12659/msm.894384
Chi, Y. L., Li, Z. S., Lin, C. S., Wang, Q., and Zhou, Y. K. (2017). Evaluation of the postoperative cognitive dysfunction in elderly patients with general anesthesia. Eur. Rev. Med. Pharmacol. Sci. 21, 1346–1354.
Cibelli, M., Fidalgo, A. R., Terrando, N., Ma, D., Monaco, C., Feldmann, M., et al. (2010). Role of interleukin-1beta in postoperative cognitive dysfunction. Ann. Neurol. 68, 360–368. doi: 10.1002/ana.22082
Cravello, L., Di Santo, S., Varrassi, G., Benincasa, D., Marchettini, P., de Tommaso, M., et al. (2019). Chronic Pain in the elderly with cognitive decline: a narrative review. Pain Ther. 8, 53–65. doi: 10.1007/s40122-019-0111-7
Dai, C. L., Hu, W., Tung, Y. C., Liu, F., Gong, C. X., and Iqbal, K. (2018). Tau passive immunization blocks seeding and spread of Alzheimer hyperphosphorylated tau-induced pathology in 3 × Tg-AD mice. Alzheimers Res. Ther. 10:13. doi: 10.1186/s13195-018-0341-7
Day, J. R., and Taylor, K. M. (2005). The systemic inflammatory response syndrome and cardiopulmonary bypass. Int. J. Surg. 3, 129–140. doi: 10.1016/j.ijsu.2005.04.002
Deiner, S., Luo, X., Lin, H. M., Sessler, D. I., Saager, L., Sieber, F. E., et al. (2017). Intraoperative infusion of Dexmedetomidine for prevention of postoperative delirium and cognitive dysfunction in elderly patients undergoing major elective noncardiac surgery: a randomized clinical trial. JAMA Surg. 152:e171505. doi: 10.1001/jamasurg.2017.1505
Ding, X., Gao, X., Wang, Z., Jiang, X., Lu, S., Xu, J., et al. (2021). Preoperative chronic and acute Pain affects postoperative cognitive function mediated by neurotransmitters. J. Mol. Neurosci. 71, 515–526. doi: 10.1007/s12031-020-01673-x
Dodds, C., and Allison, J. (1998). Postoperative cognitive deficit in the elderly surgical patient. Br. J. Anaesth. 81, 449–462. doi: 10.1093/bja/81.3.449
Duan, X., Zhu, T., Chen, C., Zhang, G., Zhang, J., Wang, L., et al. (2018). Serum glial cell line-derived neurotrophic factor levels and postoperative cognitive dysfunction after surgery for rheumatic heart disease. J. Thorac. Cardiovasc. Surg. 155, 958–965.e1. doi: 10.1016/j.jtcvs.2017.07.073
Edition, F. (2013). Diagnostic and statistical manual of mental disorders. Am. Psychiatr. Assoc. 21, 591–643.
Egerod, I., Rud, K., Specht, K., Jensen, P. S., Trangbaek, A., Rønfelt, I., et al. (2010). Room for improvement in the treatment of hip fractures in Denmark. Dan. Med. Bull. 57:A4199.
Elkana, O., Soffer, S., Eisikovits, O. R., Oren, N., Bezalel, V., and Ash, E. L. (2020). WAIS information subtest as an indicator of crystallized cognitive abilities and brain reserve among highly educated older adults: a three-year longitudinal study. Appl. Neuropsychol. Adult 27, 525–531. doi: 10.1080/23279095.2019.1575219
Evered, L. A., and Silbert, B. S. (2018). Postoperative cognitive dysfunction and noncardiac surgery. Anesth. Analg. 127, 496–505. doi: 10.1213/ane.0000000000003514
Feng, X., Valdearcos, M., Uchida, Y., Lutrin, D., Maze, M., and Koliwad, S. K. (2017). Microglia mediate postoperative hippocampal inflammation and cognitive decline in mice. JCI Insight 2:e91229. doi: 10.1172/jci.insight.91229
Gao, S., Zhang, S., Zhou, H., Tao, X., Ni, Y., Pei, D., et al. (2021). Role of mTOR-regulated autophagy in synaptic plasticity related proteins downregulation and the reference memory deficits induced by anesthesia/surgery in aged mice. Front. Aging Neurosci. 13:628541. doi: 10.3389/fnagi.2021.628541
Garrone, B., Durando, L., Prenderville, J., Sokolowska, E., Milanese, C., Di Giorgio, F. P., et al. (2021). Paracetamol (acetaminophen) rescues cognitive decline, neuroinflammation and cytoskeletal alterations in a model of post-operative cognitive decline (POCD) in middle-aged rats. Sci. Rep. 11:10139. doi: 10.1038/s41598-021-89629-y
Ghaffary, S., Ghaeli, P., Talasaz, A. H., Karimi, A., Noroozian, M., Salehiomran, A., et al. (2017). Effect of memantine on post-operative cognitive dysfunction after cardiac surgeries: a randomized clinical trial. Daru 25:24. doi: 10.1186/s40199-017-0190-0
Ghoneim, M. M., and Block, R. I. (2012). Clinical, methodological and theoretical issues in the assessment of cognition after anaesthesia and surgery: a review. Eur. J. Anaesthesiol. 29, 409–422. doi: 10.1097/EJA.0b013e328356bd6e
Gu, H., Deng, X., Lv, Y., Chen, Q., and Yu, W. (2019). Preoperational chronic pain impairs the attention ability before surgery and recovery of attention and memory abilities after surgery in non-elderly patients. J. Pain Res. 12, 151–158. doi: 10.2147/jpr.s178118
Han, B., Jiang, W., Liu, H., Wang, J., Zheng, K., Cui, P., et al. (2020). Upregulation of neuronal PGC-1α ameliorates cognitive impairment induced by chronic cerebral hypoperfusion. Theranostics 10, 2832–2848. doi: 10.7150/thno.37119
Hayashida, K. I., and Obata, H. (2019). Strategies to treat chronic Pain and strengthen impaired descending noradrenergic inhibitory system. Int. J. Mol. Sci. 20:822. doi: 10.3390/ijms20040822
Humeidan, M. L., Reyes, J. C., Mavarez-Martinez, A., Roeth, C., Nguyen, C. M., Sheridan, E., et al. (2021). Effect of cognitive Prehabilitation on the incidence of postoperative delirium among older adults undergoing major noncardiac surgery: the Neurobics randomized clinical trial. JAMA Surg. 156, 148–156. doi: 10.1001/jamasurg.2020.4371
Jevtovic-Todorovic, V., Hartman, R. E., Izumi, Y., Benshoff, N. D., Dikranian, K., Zorumski, C. F., et al. (2003). Early exposure to common anesthetic agents causes widespread neurodegeneration in the developing rat brain and persistent learning deficits. J. Neurosci. 23, 876–882. doi: 10.1523/jneurosci.23-03-00876.2003
Knipp, S. C., Matatko, N., Wilhelm, H., Schlamann, M., Thielmann, M., Lösch, C., et al. (2008). Cognitive outcomes three years after coronary artery bypass surgery: relation to diffusion-weighted magnetic resonance imaging. Ann. Thorac. Surg. 85, 872–879. doi: 10.1016/j.athoracsur.2007.10.083
Konishi, Y., Evered, L. A., Scott, D. A., and Silbert, B. S. (2018). Postoperative cognitive dysfunction after sevoflurane or propofol general anaesthesia in combination with spinal anaesthesia for hip arthroplasty. Anaesth. Intensive Care 46, 596–600. doi: 10.1177/0310057x1804600610
Kotekar, N., Shenkar, A., and Nagaraj, R. (2018). Postoperative cognitive dysfunction – current preventive strategies. Clin. Interv. Aging 13, 2267–2273. doi: 10.2147/cia.s133896
Krenk, L., Kehlet, H., Bæk Hansen, T., Solgaard, S., Soballe, K., and Rasmussen, L. S. (2014). Cognitive dysfunction after fast-track hip and knee replacement. Anesth. Analg. 118, 1034–1040. doi: 10.1213/ane.0000000000000194
Laalou, F. Z., Jochum, D., and Pain, L. (2011). Postoperative cognitive dysfunction (POCD): strategy of prevention, assessment and management. Ann. Fr. Anesth. Reanim. 30, e49–e53. doi: 10.1016/j.annfar.2011.08.009
Li, W. X., Luo, R. Y., Chen, C., Li, X., Ao, J. S., Liu, Y., et al. (2019). Effects of propofol, dexmedetomidine, and midazolam on postoperative cognitive dysfunction in elderly patients: a randomized controlled preliminary trial. Chin. Med. J. 132, 437–445. doi: 10.1097/cm9.0000000000000098
Liang, Z., Xu, X., Qi, X., and Zhang, F. (2021). Efficacy and safety of ulinastatin on cognitive dysfunction after general anesthesia in elderly patients: a protocol for systematic review and meta-analysis. Medicine (Baltimore) 100:e24814. doi: 10.1097/md.0000000000024814
Liu, X., Li, L., Tang, F., Wu, S., and Hu, Y. (2014). Memory impairment in chronic pain patients and the related neuropsychological mechanisms: a review. Acta Neuropsychiatr. 26, 195–201. doi: 10.1017/neu.2013.47
Liu, C., Wu, J., Li, M., Gao, R., Zhang, X., Ye-Lehmann, S., et al. (2023). Smad7 in the hippocampus contributes to memory impairment in aged mice after anesthesia and surgery. J. Neuroinflammation 20:175. doi: 10.1186/s12974-023-02849-z
Liu, X., Yang, H., Li, S., Wan, D., Deng, Y., Fu, Y., et al. (2021). Mediating effects of working memory on the relationship between chronic pain and overgeneral autobiographical memory. Memory 29, 298–304. doi: 10.1080/09658211.2021.1889606
Liu, X., Yu, Y., and Zhu, S. (2018). Inflammatory markers in postoperative delirium (POD) and cognitive dysfunction (POCD): a meta-analysis of observational studies. PLoS One 13:e0195659. doi: 10.1371/journal.pone.0195659
López-Otín, C., Blasco, M. A., Partridge, L., Serrano, M., and Kroemer, G. (2013). The hallmarks of aging. Cell 153, 1194–1217. doi: 10.1016/j.cell.2013.05.039
Lu, B., Nagappan, G., Guan, X., Nathan, P. J., and Wren, P. (2013). BDNF-based synaptic repair as a disease-modifying strategy for neurodegenerative diseases. Nat. Rev. Neurosci. 14, 401–416. doi: 10.1038/nrn3505
Lu, M., and Phillips, D. (2019). Total hip arthroplasty for posttraumatic conditions. J. Am. Acad. Orthop. Surg. 27, 275–285. doi: 10.5435/jaaos-d-17-00775
Luo, F., Min, J., Wu, J., and Zuo, Z. (2020). Histone deacetylases may mediate surgery-induced impairment of learning, memory, and dendritic development. Mol. Neurobiol. 57, 3702–3711. doi: 10.1007/s12035-020-01987-2
Lv, Z. T., Huang, J. M., Zhang, J. M., Zhang, J. M., Guo, J. F., and Chen, A. M. (2016). Effect of Ulinastatin in the treatment of Postperative cognitive dysfunction: review of current literature. Biomed. Res. Int. 2016, 1–9. doi: 10.1155/2016/2571080
Magee, J. C., and Grienberger, C. (2020). Synaptic plasticity forms and functions. Annu. Rev. Neurosci. 43, 95–117. doi: 10.1146/annurev-neuro-090919-022842
Martin, S. J., Grimwood, P. D., and Morris, R. G. (2000). Synaptic plasticity and memory: an evaluation of the hypothesis. Annu. Rev. Neurosci. 23, 649–711. doi: 10.1146/annurev.neuro.23.1.649
Mason, S. E., Noel-Storr, A., and Ritchie, C. W. (2010). The impact of general and regional anesthesia on the incidence of post-operative cognitive dysfunction and post-operative delirium: a systematic review with meta-analysis. J. Alzheimers Dis. 22 Suppl 3, 67–79. doi: 10.3233/jad-2010-101086
Mathew, J. P., Mackensen, G. B., Phillips-Bute, B., Grocott, H. P., Glower, D. D., Laskowitz, D. T., et al. (2009). Randomized, double-blinded, placebo controlled study of neuroprotection with lidocaine in cardiac surgery. Stroke 40, 880–887. doi: 10.1161/strokeaha.108.531236
Mattsson, N., Schöll, M., Strandberg, O., Smith, R., Palmqvist, S., Insel, P. S., et al. (2017). (18)F-AV-1451 and CSF T-tau and P-tau as biomarkers in Alzheimer's disease. EMBO Mol. Med. 9, 1212–1223. doi: 10.15252/emmm.201707809
Mazza, S., Frot, M., and Rey, A. E. (2018). A comprehensive literature review of chronic pain and memory. Prog. Neuro-Psychopharmacol. Biol. Psychiatry 87, 183–192. doi: 10.1016/j.pnpbp.2017.08.006
Miller, D., Lewis, S. R., Pritchard, M. W., Schofield-Robinson, O. J., Shelton, C. L., Alderson, P., et al. (2018). Intravenous versus inhalational maintenance of anaesthesia for postoperative cognitive outcomes in elderly people undergoing non-cardiac surgery. Cochrane Database Syst. Rev. 2018:Cd012317. doi: 10.1002/14651858.CD012317.pub2
Moller, J. T., Cluitmans, P., Rasmussen, L. S., Houx, P., Rasmussen, H., Canet, J., et al. (1998). Long-term postoperative cognitive dysfunction in the elderly ISPOCD1 study. ISPOCD investigators. International study of post-operative cognitive dysfunction. Lancet 351, 857–861. doi: 10.1016/s0140-6736(97)07382-0
Monk, T. G., Weldon, B. C., Garvan, C. W., Dede, D. E., van der Aa, M. T., Heilman, K. M., et al. (2008). Predictors of cognitive dysfunction after major noncardiac surgery. Anesthesiology 108, 18–30. doi: 10.1097/01.anes.0000296071.19434.1e
Moody, D. M., Brown, W. R., Challa, V. R., Stump, D. A., Reboussin, D. M., and Legault, C. (1995). Brain microemboli associated with cardiopulmonary bypass: a histologic and magnetic resonance imaging study. Ann. Thorac. Surg. 59, 1304–1307. doi: 10.1016/0003-4975(95)00057-r
Natarajan, V., Chawla, R., Mah, T., Vivekanandan, R., Tan, S. Y., Sato, P. Y., et al. (2020). Mitochondrial dysfunction in age-related metabolic disorders. Proteomics 20:e1800404. doi: 10.1002/pmic.201800404
Needham, M. J., Webb, C. E., and Bryden, D. C. (2017). Postoperative cognitive dysfunction and dementia: what we need to know and do. Br. J. Anaesth. 119, i115–i125. doi: 10.1093/bja/aex354
Netto, M. B., de Oliveira Junior, A. N., Goldim, M., Mathias, K., Fileti, M. E., da Rosa, N., et al. (2018). Oxidative stress and mitochondrial dysfunction contributes to postoperative cognitive dysfunction in elderly rats. Brain Behav. Immun. 73, 661–669. doi: 10.1016/j.bbi.2018.07.016
O'Gara, B. P., Mueller, A., Gasangwa, D. V. I., Patxot, M., Shaefi, S., Khabbaz, K., et al. (2020). Prevention of early postoperative decline: a randomized, controlled feasibility trial of perioperative cognitive training. Anesth. Analg. 130, 586–595. doi: 10.1213/ane.0000000000004469
Papadopoulos, G., Pouangare, M., Papathanakos, G., Arnaoutoglou, E., Petrou, A., and Tzimas, P. (2014). The effect of ondansetron on postoperative delirium and cognitive function in aged orthopedic patients. Minerva Anestesiol. 80, 444–451.
Pappa, M., Theodosiadis, N., Tsounis, A., and Sarafis, P. (2017). Pathogenesis and treatment of post-operative cognitive dysfunction. Electron. Physician 9, 3768–3775. doi: 10.19082/3768
Pinto, T. C. C., Machado, L., Bulgacov, T. M., Rodrigues-Júnior, A. L., Costa, M. L. G., Ximenes, R. C. C., et al. (2019). Is the Montreal cognitive assessment (MoCA) screening superior to the Mini-mental state examination (MMSE) in the detection of mild cognitive impairment (MCI) and Alzheimer's disease (AD) in the elderly? Int. Psychogeriatr. 31, 491–504. doi: 10.1017/s1041610218001370
Pîrşcoveanu, D. F. V., Pirici, I., Tudorică, V., Bălşeanu, T. A., Albu, V. C., Bondari, S., et al. (2017). Tau protein in neurodegenerative diseases – a review. Romanian J. Morphol. Embryol. 58, 1141–1150.
Qiu, L. L., Pan, W., Luo, D., Zhang, G. F., Zhou, Z. Q., Sun, X. Y., et al. (2020). Dysregulation of BDNF/TrkB signaling mediated by NMDAR/ca(2+)/calpain might contribute to postoperative cognitive dysfunction in aging mice. J. Neuroinflammation 17:23. doi: 10.1186/s12974-019-1695-x
Rundshagen, I. (2014). Postoperative cognitive dysfunction. Dtsch. Arztebl. Int. 111, 119–125. doi: 10.3238/arztebl.2014.0119
Saleh, A. J., Tang, G. X., Hadi, S. M., Yan, L., Chen, M. H., Duan, K. M., et al. (2015). Preoperative cognitive intervention reduces cognitive dysfunction in elderly patients after gastrointestinal surgery: a randomized controlled trial. Med. Sci. Monit. 21, 798–805. doi: 10.12659/msm.893359
Salter, M. W., and Stevens, B. (2017). Microglia emerge as central players in brain disease. Nat. Med. 23, 1018–1027. doi: 10.1038/nm.4397
Scherder, E. J., Eggermont, L., Plooij, B., Oudshoorn, J., Vuijk, P. J., Pickering, G., et al. (2008). Relationship between chronic pain and cognition in cognitively intact older persons and in patients with Alzheimer's disease. The need to control for mood. Gerontology 54, 50–58. doi: 10.1159/000113216
Shao, A., Fei, J., Feng, S., and Weng, J. (2020). Chikusetsu saponin IVa alleviated sevoflurane-induced neuroinflammation and cognitive impairment by blocking NLRP3/caspase-1 pathway. Pharmacol. Rep. 72, 833–845. doi: 10.1007/s43440-020-00078-2
Skvarc, D. R., Berk, M., Byrne, L. K., Dean, O. M., Dodd, S., Lewis, M., et al. (2018). Post-operative cognitive dysfunction: an exploration of the inflammatory hypothesis and novel therapies. Neurosci. Biobehav. Rev. 84, 116–133. doi: 10.1016/j.neubiorev.2017.11.011
Steinmetz, J., Christensen, K. B., Lund, T., Lohse, N., and Rasmussen, L. S. (2009). Long-term consequences of postoperative cognitive dysfunction. Anesthesiology 110, 548–555. doi: 10.1097/ALN.0b013e318195b569
Stern, Y. (2012). Cognitive reserve in ageing and Alzheimer's disease. Lancet Neurol. 11, 1006–1012. doi: 10.1016/s1474-4422(12)70191-6
Su, X., Meng, Z. T., Wu, X. H., Cui, F., Li, H. L., Wang, D. X., et al. (2016). Dexmedetomidine for prevention of delirium in elderly patients after non-cardiac surgery: a randomised, double-blind, placebo-controlled trial. Lancet 388, 1893–1902. doi: 10.1016/s0140-6736(16)30580-3
Su, C., Ren, X., Wang, H., Ding, X., and Guo, J. (2022). Changing Pain management strategy from opioid-centric towards improve postoperative cognitive dysfunction with Dexmedetomidine. Curr. Drug Metab. 23, 57–65. doi: 10.2174/1389200222666211118115347
Sun, W., Zhao, J., and Li, C. (2020). Dexmedetomidine provides protection against hippocampal neuron apoptosis and cognitive impairment in mice with Alzheimer's disease by mediating the miR-129/YAP1/JAG1 Axis. Mol. Neurobiol. 57, 5044–5055. doi: 10.1007/s12035-020-02069-z
Ukmar-Godec, T., Wegmann, S., and Zweckstetter, M. (2020). Biomolecular condensation of the microtubule-associated protein tau. Semin. Cell Dev. Biol. 99, 202–214. doi: 10.1016/j.semcdb.2019.06.007
Valentin, L. S., Pereira, V. F., Pietrobon, R. S., Schmidt, A. P., Oses, J. P., Portela, L. V., et al. (2016). Effects of single low dose of dexamethasone before noncardiac and nonneurologic surgery and general anesthesia on postoperative cognitive dysfunction-a phase III double blind, randomized clinical trial. PLoS One 11:e0152308. doi: 10.1371/journal.pone.0152308
Vasic, V., Barth, K., and Schmidt, M. H. H. (2019). Neurodegeneration and neuro-regeneration-Alzheimer's disease and stem cell therapy. Int. J. Mol. Sci. 20:4272. doi: 10.3390/ijms20174272
Veldhuijzen, D. S., Sondaal, S. F., and Oosterman, J. M. (2012). Intact cognitive inhibition in patients with fibromyalgia but evidence of declined processing speed. J. Pain 13, 507–515. doi: 10.1016/j.jpain.2012.02.011
Wan, J., Luo, P., Du, X., and Yan, H. (2020). Preoperative red cell distribution width predicts postoperative cognitive dysfunction after coronary artery bypass grafting. Biosci. Rep. 40:BSR20194448. doi: 10.1042/bsr20194448
Wang, J., Niu, Y., Tao, H., Xue, M., and Wan, C. (2020). Knockdown of lncRNA TUG1 inhibits hippocampal neuronal apoptosis and participates in aerobic exercise-alleviated vascular cognitive impairment. Biol. Res. 53:53. doi: 10.1186/s40659-020-00320-4
Wang, Y., Sands, L. P., Vaurio, L., Mullen, E. A., and Leung, J. M. (2007). The effects of postoperative pain and its management on postoperative cognitive dysfunction. Am. J. Geriatr. Psychiatry 15, 50–59. doi: 10.1097/01.JGP.0000229792.31009.da
Wang, D., Wu, X., Li, J., Xiao, F., Liu, X., and Meng, M. (2002). The effect of lidocaine on early postoperative cognitive dysfunction after coronary artery bypass surgery. Anesth. Analg. 95, 1134–1141. doi: 10.1097/00000539-200211000-00002
Whitlock, E. L., Diaz-Ramirez, L. G., Glymour, M. M., Boscardin, W. J., Covinsky, K. E., and Smith, A. K. (2017). Association between persistent Pain and memory decline and dementia in a longitudinal cohort of elders. JAMA Intern. Med. 177, 1146–1153. doi: 10.1001/jamainternmed.2017.1622
Whitlock, E. L., Vannucci, A., and Avidan, M. S. (2011). Postoperative delirium. Minerva Anestesiol. 77, 448–456.
Williams-Russo, P., Sharrock, N. E., Mattis, S., Szatrowski, T. P., and Charlson, M. E. (1995). Cognitive effects after epidural vs general anesthesia in older adults. A randomized trial. JAMA 274, 44–50. doi: 10.1001/jama.1995.03530010058035
Xiao, Q. X., Liu, Q., Deng, R., Gao, Z. W., and Zhang, Y. (2020). Postoperative cognitive dysfunction in elderly patients undergoing hip arthroplasty. Psychogeriatrics 20, 501–509. doi: 10.1111/psyg.12516
Xiao, J. Y., Xiong, B. R., Zhang, W., Zhou, W. C., Yang, H., Gao, F., et al. (2018). PGE2-EP3 signaling exacerbates hippocampus-dependent cognitive impairment after laparotomy by reducing expression levels of hippocampal synaptic plasticity-related proteins in aged mice. CNS Neurosci. Ther. 24, 917–929. doi: 10.1111/cns.12832
Yan, J., Luo, A., Sun, R., Tang, X., Zhao, Y., Zhang, J., et al. (2020). Resveratrol mitigates hippocampal tau acetylation and cognitive deficit by activation SIRT1 in aged rats following anesthesia and surgery. Oxidative Med. Cell. Longev. 2020, 4635163–4635114. doi: 10.1155/2020/4635163
Yang, L., Hao, J. R., Gao, Y., Yang, X., Shen, X. R., Wang, H. Y., et al. (2022). HDAC3 of dorsal hippocampus induces postoperative cognitive dysfunction in aged mice. Behav. Brain Res. 433:114002. doi: 10.1016/j.bbr.2022.114002
Yang, Y., Liu, Y., Zhu, J., Song, S., Huang, Y., Zhang, W., et al. (2021). Neuroinflammation-mediated mitochondrial dysregulation involved in postoperative cognitive dysfunction. Free Radic. Biol. Med. 178, 134–146. doi: 10.1016/j.freeradbiomed.2021.12.004
Yuan, N., Wang, X., Zhang, Y., Kong, L., Yuan, L., and Ge, Y. (2022). Intervention of NF-Κb signaling pathway and preventing post-operative cognitive dysfunction as well as neuronal apoptosis. Iran. J. Public Health 51, 124–132. doi: 10.18502/ijph.v51i1.8303
Zhang, G. F., Zhou, Z. Q., Guo, J., Gu, H. W., Su, M. Z., Yu, B. C., et al. (2021). Histone deacetylase 3 in hippocampus contributes to memory impairment after chronic constriction injury of sciatic nerve in mice. Pain 162, 382–395. doi: 10.1097/j.pain.0000000000002056
Zhu, S. H., Ji, M. H., Gao, D. P., Li, W. Y., and Yang, J. J. (2014). Association between perioperative blood transfusion and early postoperative cognitive dysfunction in aged patients following total hip replacement surgery. Ups. J. Med. Sci. 119, 262–267. doi: 10.3109/03009734.2013.873502
Keywords: POCD, review, risk factors, pathogenesis, prevention
Citation: Zhao Q, Wan H, Pan H and Xu Y (2024) Postoperative cognitive dysfunction—current research progress. Front. Behav. Neurosci. 18:1328790. doi: 10.3389/fnbeh.2024.1328790
Edited by:
Miou Zhou, Western University of Health Sciences, United StatesReviewed by:
Pingping Zhao, University of California, Los Angeles, United StatesThaddee Valdelievre, Baylor Scott and White Health, United States
Copyright © 2024 Zhao, Wan, Pan and Xu. This is an open-access article distributed under the terms of the Creative Commons Attribution License (CC BY). The use, distribution or reproduction in other forums is permitted, provided the original author(s) and the copyright owner(s) are credited and that the original publication in this journal is cited, in accordance with accepted academic practice. No use, distribution or reproduction is permitted which does not comply with these terms.
*Correspondence: Hui Pan, d29wYW5odWlAMTI2LmNvbQ==; Yiquan Xu, eHV5cXFpbmdAMTYzLmNvbQ==
†These authors have contributed equally to this work and share first authorship