- 1Graduate School of Education and Psychology, Pepperdine University, Los Angeles, CA, United States
- 2Center for Neuropsychology and Consciousness, Miami, FL, United States
The best predictor of functional outcome in victims of traumatic brain injury (TBI) is a neuropsychological evaluation. An exponential growth of research into TBI has focused on diagnosis and treatment. Extant literature lacks a comprehensive neuropsychological review that is simultaneously scholarly and practical. In response, our group included, and went beyond a general overview of TBI's, which commonly include definition, types, severity, and pathophysiology. We incorporate reasons behind the use of particular neuroimaging techniques, as well as the most recent findings on common neuropsychological assessments conducted in TBI cases, and their relationship to outcome. In addition, we include tables outlining estimated recovery trajectories of different age groups, their risk factors and we encompass phenomenological studies, further covering the range of existing—promising tools for cognitive rehabilitation/remediation purposes. Finally, we highlight gaps in current research and directions that would be beneficial to pursue.
Introduction
It is possible to speculate that the non-linear path of evolution and its violent history, may have led to the development of brains that favored protection and recovery from brain injuries. To name a few, human brains are protected by the cranium, underneath which are three core layers of protective membranes, in addition to being immersed in cerebrospinal fluid capable of absorbing impact. Indeed, recovery has been found through neuroplasticity and neurogenesis, which appear to be far more advanced than previously believed. At some point however, there was a trade-off, the advancement of brain structure and function, for a brain more susceptible to damage through impact. This adaptation functioned because it was predicated on the utility of complex cognitive abilities to offset and/or treat the consequences of Traumatic Brain Injuries (TBI). One way this is expressed in modern day is the development of neuropsychological assessments in service of evaluating, predicting, and improving the outcome of a TBI.
While TBIs were likely around since the dawn of humankind, it is also likely that our brains did not evolve to withstand the impact of a car crash. Nonetheless, when viewing incidence rates, it is clear that comparable injuries our ancestors might have sustained (e.g., from falls, sports, or violence) continues to be a major problem. Worldwide, traumatic brain injuries (TBI) constitute one of the leading causes of injury-related deaths and disability (Maas et al., 2022). TBIs are responsible for ~30% of all injury-related deaths in the United States and are a leading cause of mortality and disability (Kaur and Sharma, 2018). Closed-head injuries (CHI) account for about 75% of TBIs, while penetrating head injuries (PHI) account for around 25% (CDC, 2016). There were ~223,125 TBI-related hospitalizations in 2019 and 64,362 TBI-related deaths in 2020 (CDC, 2022). TBI is the leading cause of death and disability in people younger than age 35 in the US (Popescu et al., 2015). Falls lead to nearly half of the TBI-related hospitalizations and are now the leading cause of TBI, overtaking road traffic accidents (Roozenbeek et al., 2013). Firearm-related suicide is the most common cause of TBI related deaths in the US. In the United States, around 1.7 million people suffer TBI, with older adolescents (ages 15–19 years) and older adults (ages 65 years and older) among the most likely to sustain a TBI (Georges and Das, 2023).
Traumatic Brain Injury (TBI) refers to penetrating, blunt, or acceleration/deceleration force-derived craniocerebral injury. TBI often results in cognitive deficits in memory, attention, processing speed, word finding, planning, and problem-solving. From a behavioral perspective, difficulties such as lack of initiative, irritability, and poor temper control may be present. Somatic symptoms may include headaches, dizziness, fatigue, sleep disturbance, poor balance, and coordination. TBI can also result in psychological symptoms (e.g., anxiety and depression). These difficulties usually resolve to some degree, but could persist in many cases, even decades after injury. Thus, ongoing assessment and tailored interventions are crucial for effectively managing TBI. Neuropsychological assessments are essential for identifying deficits and understanding the extent of functional loss. These assessments predict outcomes and guide treatment, aiming to improve patients' functional abilities while mitigating further cognitive decline. The specific characteristics of a TBI, including the nature and extent of the damage, help determine the type of neuropsychological deficits that may arise. Understanding these characteristics will assist in distinguishing between different forms of head injuries and their impacts.
Types of traumatic brain injuries
Head injuries can be classified into two broad categories: closed head injury (CHI) and penetrating head injury (PHI) (Kaur and Sharma, 2018). CHI is more common and complicated than PHI; over 75% of all brain injuries are CHIs. CHI occurs when an external force impacts the skull, causing damage to the brain without penetrating the skull. Swift forward or backward movement and shaking of the brain inside the cranium are common causes of this type of damage, which results in hemorrhage and the tearing of brain tissue and blood vessels (Vieira et al., 2016). CHIs can affect various areas of the skull, including the frontal bone, temporal bone, parietal bone, and occipital bone, depending on the specific circumstances of the injury (Jeyaraj, 2019). Paradoxically, while the cranium is meant to protect the brain, in certain circumstances, parts of it may cause damage. One such area is the crista galli, a protruding triangular surface rising from the ethmoid bone that plays a role in attaching the dura mater (one of the protective membranes mentioned earlier).
On the other hand, PHI occurs when a foreign object, such as a bullet or a sharp projectile, penetrates the skull and directly damages the brain tissue. It is important to note that PHI can cause significant damage to the brain tissue, as the object can cause both primary and secondary injuries to the brain. Primary and secondary injuries are two distinct phases of TBI that can cause damage to the brain tissue (Ng and Lee, 2019). Primary injury occurs immediately after the traumatic event, caused by the direct physical forces applied to the brain tissue. Secondary injury occurs after the primary injury and can be caused by various processes, including inflammation, oxidative stress, excitotoxicity, and others. These processes may worsen the symptoms of the initial injury and inflict more harm on the brain tissue. Secondary injuries can occur over a period of hours or days after the initial trauma and can contribute significantly to the long-term effects of TBI.
CHI can range in severity from mild concussions to severe TBI. In mild cases, the individual may experience symptoms such as headaches, dizziness, and confusion but may not lose consciousness. Severe CHI can result in long-term neurological deficits and disability. Symptoms of closed head injuries can include loss of consciousness, memory loss, difficulty concentrating, seizures, and changes in personality or behavior.
In contrast, PHI is typically more severe and life-threatening than CHI. The severity of a PHI depends on the location and extent of the damage caused by the foreign object. In general, PHIs result in more localized brain damage, whereas CHIs may result in diffuse damage to the brain. PHIs may thus yield a neurocognitive profile with more targeted deficits. This may however, be complicated by hemorrhaging, infection, and swelling, further damaging the brain tissue. Treatment for penetrating head injuries often involves surgical removal of the foreign object, followed by intensive medical care to manage the resulting brain damage and complications. Recognizing the distinction between closed and penetrating head injuries provides context regarding the diverse nature of TBI and their specific effects on brain regions, with these varying impacts directly influencing cognitive and functional outcomes.
Multiple levels of analysis
The short and long-term sequelae of PHIs and CHIs depend on severity and may be analyzed on multiple levels. Linear and rotational acceleration of the brain can result in mild TBI (mTBI) if a significant amount of force is applied. Blennow et al. (2016) have shown that in these instances, the lower sulci located in the frontal, parietal, and temporal lobes receive higher levels of stress and strain induced by TBI. Cortices and white matter tracts also receive the brunt of the damage induced by mTBI. White matter tracts, which send neuronal signals to nearby neurons and are located in subcortical regions, corpus callosum, fornices, and cerebellum, are more prone to damage upon the initial impact of mTBI.
One of the most common symptoms caused by TBI is cerebral edema. This symptom occurs after the injury and is triggered by an inflammatory response (Arulsamy et al., 2018). Cortical swelling is typically increased in the prefrontal and temporal cortices (Linden et al., 2019; Dall'Acqua et al., 2017). Prolonged and sufficient damage from TBI has the potential to induce an inflammatory response (Bigler, 2013; Johnson et al., 2013) with acute swelling potentially leading to chronic secondary injuries (Ma et al., 2016). That is why, when swelling is severe enough, a portion of the skull may be removed (i.e., craniectomy) to enable the inflammatory processes to take their course, and naturally subside without risking further damage to the brain. Compared with controls, once swelling has subsided, many individuals diagnosed with TBI exhibit reduced brain volume in the temporal, hippocampal, and frontal regions (Bigler, 2013).
Upon impact, axonal shearing may occur near the primary site of injury (Govindarajan et al., 2016). Occurring in areas of the brain initially injured, axonal shearing has been identified as a precursor to the buildup of beta-amyloid plaques, apoptosis, and oxidative stress (Ma et al., 2016). Damage to these areas plays a large role in the symptoms that are experienced. TBI has been shown to produce emotional deficits, challenges with working memory, and other executive dysfunctions. The hippocampus has been correlated to memory related processes and aids in the regulation of emotions. Damage to this area caused by TBI can result in decreased memory capacity and emotional functioning. However, studies have shown that promoting neurogenesis within this region can reduce negative symptoms (Peng and Bonaguidi, 2018).
Moderate to severe TBI significantly reduces cortical thickness (Vedung et al., 2022). Differences in cortical thickness in acute and chronic stages of TBI demonstrate how an injury in the frontal-temporal region correlates to neurodegeneration across the hemispheres. For example, a study was conducted comparing the cortical thickness of mTBI patients where a baseline of decreased cortical thickness was established compared to healthy controls (Govindarajan et al., 2016). Research conducted by Govindarajan et al. (2016) demonstrated that cortical thinning is associated with mTBI primarily in the frontal, temporal, and parietal regions. Follow up examinations of cortical thickness in these subjects revealed the thinning had spread to include some areas of the insula and cingulate cortex (Govindarajan et al., 2016). Research has shown that cortical thickness decreases in adolescents who tested positive for TBI in the prefrontal cortex (Linden et al., 2019). Vedung et al. (2022) established a correlation between a decrease in cortical thickness and an increase in TBI symptom severity, in particular, individuals with moderate to severe TBI exhibit increases in cortical thinning compared with control groups. Within their study, Govindarajan et al. (2016) provided treatment for an mTBI subgroup, participants did not show a significant reduction in cortical thickness after obtaining treatment.
Mild TBI also commonly decreases. However, in some instances, sporadic increases in cortical thickness have been shown to occur post-mTBI, but the increase is not significant compared to cortical thinning (Govindarajan et al., 2016). Individuals who have worse outcomes long term, over 3 months, after the initial injury have lower volumes of gray and white matter and increased cortical thickness compared with healthy controls (Dall'Acqua et al., 2017). The subsequent increase of cortical thickness in mTBI patients has not been well-established, but it could indicate an increase in swelling and trauma.
White and gray matter are largely affected by TBI (Vedung et al., 2022; Dall'Acqua et al., 2017). A decrease in white and gray matter volume inhibits homeostasis as these regions are responsible for neuronal communication and processing. The continuous degeneration of white and gray matter is also found in the neuropathology of neurodegenerative diseases suggesting that secondary injuries of TBI correlate to those diseases (Jang et al., 2017). Jang et al. (2017) found a relationship between the degeneration of white and gray matter tracts and Alzheimer's, Subcortical Vascular Dementia, and mixed dementia, with the highest level of white matter degeneration occurring in Subcortical Vascular Dementia and mixed dementia. The varied impact of TBI on specific brain areas results in diverse experiences and outcomes, with differences observed in cortical thickness, white and gray volume, and the manifestation of secondary injuries.
Findings on how TBIs may impact cortical thickness, white and gray matter, major lobes of the brain, the hemispheres and particular regions may orient the clinician toward specific functions that may be anticipated to be impacted. Yet, real-time dysfunction may be more accurately depicted by a network approach, analyzing neurodynamic imbalances between networks within the brain. Recognizing how TBI disrupts these interconnected networks informs the injury's impact on both localized brain functions and broader cognitive processes.
Neural networks and TBIs
While there are many neural networks, there are three of particular interest, the Default Mode Network (DMN), the Salience Network (SN) and the Central Executive Network (CEN). The DMN is a network related to mind wandering, autobiographical recall, prospection, self referential processing, and social cognition. The SN determines the significance or salience of external or internal stimuli in any particular moment. It also acts as a toggle between the DMN and CEN. The CEN is a network dedicated to goal-directed tasks and executive functioning. Mutual inhibition typifies the relationship between the DMN and CEN (Chan, 2021). These three networks are typically working together in neurodynamic balance, as the individual shifts their focus toward the external world to focus on a task (CEN) or pauses to reflect on themselves and an interaction that occurred (DMN). In TBIs, intra-network and inter-network disruptions result in broad imbalances and cognitive dysfunction.
From the intra-network perspective, Zhou et al. (2012) have shown that mTBI leads to altered connectivity within the Default Mode Network (DMN), marked by reduced connectivity in posterior regions like the posterior cingulate cortex (PCC) and increased connectivity in the medial prefrontal cortex (mPFC). This imbalance between anterior and posterior regions of the DMN was closely linked to deficits in executive function and mental flexibility, suggesting that such network disruptions may be at the root of some cognitive difficulties commonly observed in mTBI patients. The hyperconnectivity within the mPFC demonstrated an inverse relationship with mood related symptoms such as depression and anxiety. It was further interpreted that the mPFC may initially compensate to sustain cognitive abilities; however, over time, this could contribute to the emergence of psychological symptoms like anxiety and depression.
From an inter-network perspective, Liu et al. (2024) note that it is typical for there to be increased dysconnectivity between the DMN and CEN in mTBI, which has been correlated to reduced working memory performance; abnormal coupling between the CEN and SN which has related to increased emotional dysregulation and internetwork irregularities between the SN and DMN, which has led to disinhibition. Their novel insights come from analyzing the neurodynamic imbalances from a temporal perspective. In comparison to healthy controls, the mTBI group spent the most time in a state characterized by reduced connectivity between the DMN and SN (state 1), whose length of time correlated to reduced scores on the Montreal Cognitive Assessment. Significantly less time was found in a state characterized by higher DMN connectivity, and negative correlation between the DMN and SN (state 3) whom the authors hypothesized may relate to reduced social cognitive abilities. Finally, mTBI participants also demonstrated overall reductions in the amount of transitions between networks.
Disruptions in other networks such as the SN and CEN have also been observed in mTBI. Liu et al. (2020) documented that mTBI patients frequently exhibit hyper-connectivity between the DMN and SN, potentially acting as a compensatory strategy to preserve cognitive performance in the wake of injury. However, this hyper-connectivity may eventually become maladaptive, contributing to further network imbalances and cognitive decline over time. The impact of these network disruptions is not confined to isolated cognitive functions. Research by Li et al. (2020), Li F. et al. (2023), Li X. et al. (2023), and Li C. et al. (2023) demonstrates that mTBI can disrupt connectivity across multiple networks, including the DMN, SN, CEN, and SMN. These disruptions are strongly associated with impairments in attention, executive function, and memory, highlighting the role of network integrity in the cognitive recovery process following injury. Specifically, decreased connectivity between the SN and executive control regions, such as the superior frontal gyrus, relate to the challenges mTBI patients face in maintaining cognitive performance.
In parallel, Rigon et al. (2016) found that mTBI leads to significant reductions in inter-hemispheric functional connectivity (FC) within externally oriented networks (EONs) such as the fronto-parietal network (FPN) and executive control network (ECN), rather than within the DMN or sensorimotor network (SMN). These specific disruptions in inter hemispheric connectivity were associated with impairments in visuospatial and organizational skills, as evidenced by poorer performance on the Rey-Osterrieth Complex Figure Test (ROCFT), implicating inter-hemispheric FC within executive control and flexibility (Rigon et al., 2016).
Structural damage, such as diffuse axonal injury (DAI), significantly impairs key neural networks like the DMN, SN, and CEN. DAI, which occurs in about half of all severe head trauma cases, involves extensive white matter damage that is correlated with cognitive deficits (Aquino et al., 2014). The severity of DAI correlates with its location: mild cases typically involve the frontal and temporal lobar white matter, moderate cases affect the corpus callosum, and severe cases extend to the dorsolateral midbrain. This damage to white matter tracts, particularly in regions like the corpus callosum and midbrain, is a primary contributor to the network disruptions observed in mTBI, leading to cognitive impairments (Aquino et al., 2014).
While the previous studies focused on spatial dynamics between and within networks of the brain, Alhourani et al. (2016) explored temporal dynamics, focusing on frequency-specific changes in connectivity following mTBI. Their study found that mTBI reduces alpha band connectivity and generates slow delta waves, both associated with white matter deafferentation and subsequent cognitive impairments. These frequency-specific disruptions, particularly within the DMN, are linked to deficits in higher cognitive functions such as memory and attention, which are commonly reported post-concussion (Alhourani et al., 2016). Additionally, the observed network topology changes, including the loss of inter-hemispheric connections, may be related to DAI's impact on white matter tracts like the corpus callosum, further exacerbating cognitive deficits in mTBI patients (Alhourani et al., 2016).
Neuropsychological functions and TBIs
In the context of such foundations as what TBIs are, how they are classified, and how the brain is typically impacted from multiple perspectives, specific neuropsychological functions may now be reviewed in depth. Damage to specific areas (i.e., frontal-temporal cortices and hippocampus) produce the deficits associated with sustaining TBI. As the previously mentioned areas are most vulnerable, the following impaired functions discussed are hallmarks of TBI.
Wang et al. (2021) found support through their research that information processing, memory, and attention are impaired. Individuals with TBI show hyperactivation in the prefrontal cortex, which can lead to cognitive fatigue compared with healthy controls (Gillis and Hampstead, 2015). A review by Blennow et al. (2016) found that impairment of the prefrontal cortex presents difficulty concentrating and poor memory. Additional symptoms related to all severity levels of TBI include nausea, dizziness, vomiting, sensitivity to light, and headaches. Mood changes can also be seen in TBI patients such as an increase in irritability. The treatment of cortical and structural areas resulting in improved functioning that had been damaged by TBI reinforces the role the brain regions play in a healthy brain and what processes are disrupted upon injury.
The prefrontal cortex connects to the limbic system and facilitates top-down processing; damage of these connections correlates to deficits in emotional processing (van der Horn et al., 2015). Emotional impairments are common symptoms of TBI and deficits in processing positively correlate with impairments in accurately identifying negative emotions in individuals with TBI (Rosenberg et al., 2015). Individuals may experience decreased emotional responses or inability to control stronger emotions such as anger (Rassovsky et al., 2015). Axonal shearing of white matter tracts is related to a decrease in general processing speed (Boccia et al., 2022; Ferraracci et al., 2021). This disruption may correlate to damage to the prefrontal cortex. Individuals with TBI compared with healthy controls have demonstrated slower processing speeds (Dymowski et al., 2015). In addition, slowed processing speed, induced by TBI, can affect working memory (Gorman et al., 2016).
TBI may also lead to affective disorders, with anxiety, depression, and PTSD being the most common. Anxiety symptoms, irritability, fatigue, and cognitive deficits persist well-beyond 3 months after the initial impact of TBI (Lamontagne et al., 2022; McMahon et al., 2014). Deficits in language and verbal memory occur consistently with TBI (Wang et al., 2021; Ryan et al., 2015b). The duration of these deficits may depend on the age of the individual when they sustained a TBI and the severity classification. Adults aged 18–24 with TBI had language processing return to normal functioning by 6 months post-injury (Coffey et al., 2021). However, other studies suggest that patients/individuals aged 5–15 years may suffer from language deficits for up to 2 years after the initial impact of TBI (Ryan et al., 2015b). The severity of TBI correlates to the severity of symptoms experienced. Age and severity are variables affecting the degree of potential recovery from damage caused to the brain. Continued research in this area could be useful in determining rehabilitation techniques to reduce the duration of deficits after TBI.
Classifying the severity of TBIs
The severity of a TBI is a significant factor that affects both the outcomes and therapies for patients (Rauchman et al., 2022). The degree of severity may increase the risk for cognitive deficits, motor impairment, and emotional difficulties (depression, anxiety, aggression, impulse control, etc.), both temporarily and permanently (Mckee and Daneshvar, 2015). Understanding the similarities and differences between these severity levels is crucial for accurate diagnosis, prognosis, and potential treatment methods. Table 1 is a table which his typically used to help classify the severity of a brain injury. Neuropsychologists are responsible for determining the length of Post-Traumatic Amnesia (PTA), a state of discontinuous cognitive functioning, classically characterized by anterograde and retrograde amnesia. One common task administered is the Galveston Orientation and Amnesia Test (GOAT), whereby the TBI patient must receive a score of 75 or greater on three consecutive trials on independent days to be determined out of PTA.
Imaging tools play a crucial role in determining the severity of TBI and guiding appropriate treatment strategies. These tools provide valuable insights into the structural and functional changes that occur in the brain following an injury (Mckee and Daneshvar, 2015). By visualizing the affected areas and their extent, imaging techniques enable healthcare professionals to accurately assess the severity of TBI, identify potential complications, and monitor the progression of the condition over time. The following table (Table 2) provides a summary of commonly used techniques and their advantages.
Severity of TBIs and neuropsychological profile
The complexity of TBI-related impairments encompasses cognitive deficits, functional limitations, and behavioral changes. Cognitive deficits, including attention, memory, and executive function impairments, pose challenges in cognitive processes. Functional limitations affect individuals' ability to perform daily activities independently, while behavioral changes can have implications for emotional wellbeing and social interactions (Devi et al., 2020). Behavioral problems following TBI present a significant challenge, yet interventions targeting these problems have received limited attention compared with cognitive and functional deficits (Yeates et al., 2017). Treatment approaches primarily focus on addressing the cognitive and functional aspects of TBI, which can have a profound impact on an individual's daily functioning, work performance, and overall quality of life. Various injury-related factors, such as TBI severity, complications, pre-existing injuries to other body regions, and the duration of the injury, influence the manifestation of TBI symptoms (Rabinowitz and Levin, 2014).
Traumatic brain injury ranges from mild, moderate to severe. Mild TBI (mTBI) is more commonly referred to as concussions. Symptoms experienced in mTBI typically do not exceed 3 months, but they tend to subside within 7–10 days. In cases of mild TBI, individuals may experience temporary cognitive impairments, including difficulties with attention, memory, and information processing speed. Additionally, they may encounter mild functional limitations, such as changes in coordination, balance, and fine motor skills. Fortunately, these deficits are usually transient and tend to resolve relatively quickly. Understanding the neurobiological underpinnings of cognitive impairments and emotional changes following TBI provides valuable insights into the mechanisms involved. Research suggests that mTBI can lead to alterations in synaptic function and plasticity. Disruptions in synaptic strength, which refers to the ability of neurons to communicate effectively, can impair neural communication and impact cognitive processes and overall brain function (Witowski et al., 2019). Rapid changes in synaptic strength may be a contributing factor to attention deficits, memory problems, and learning difficulties commonly observed following mTBI.
In moderate TBI cases, cognitive impairments tend to be more pronounced and long lasting, involving attention and memory difficulties, executive function deficits, and reduced information processing speed. Functional impairments in moderate cases may include persistent motor coordination difficulties, challenges in performing activities of daily living, and emotional and behavioral changes. Severe TBI often leads to severe and persistent cognitive impairments affecting multiple domains, such as attention, memory, language, problem-solving, and executive functions. Furthermore, functional impairments in severe cases can manifest as severe physical disabilities, significant limitations in self-care tasks, difficulties with speech and swallowing, and cognitive and behavioral impairments (Mckee and Daneshvar, 2015). TBI also disrupts the balance of neurotransmitters in the brain, leading to alterations in mood regulation and cognitive functioning (Ahmed et al., 2017). Severe TBI can disrupt emotional contagion, making it difficult for individuals to empathize with others' feelings and maintain social relationships (Rushby et al., 2013). This means they may struggle to pick up on non-verbal cues, misunderstand emotional expressions, and react inappropriately in social situations. This impairment is linked to damage in key brain regions responsible for emotional contagion, like the amygdala and prefrontal cortex (Rushby et al., 2013).
When assessing TBI, different approaches can be taken. As previously discussed, imaging tools are a proficient manner in which to examine this condition and see physical damage to the brain. Another approach to gauging the severity of TBI is to specifically look for difficulties with attention, memory, processing speed, working memory, coordination, and executive functioning. Pen and paper, and computer tests that are administered by a trained professional can acquire such information. These tests inform the clinician to what degree the patient may be experiencing cognitive impairments. Deficits in cognitive functioning are seen in acute and chronic phases of TBI (Tsai et al., 2021).
As research accumulates, so does the opportunity exist for the synthesis of literature that may enable a convenient path for more precise neuropsychological testing. As an example, previous meta-analytic work demonstrates that particular neuropsychological functions were strongly correlated with functional outcome. Allanson et al. (2017) found that delayed verbal memory, visuo-spatial construction, set shifting, and generativity particularly stood out as significant predictors of functional outcome. A more recent meta-analysis supports and builds upon these findings (Krynicki et al., 2023), some of which is presented in Table 3.
TBI impairs various cognitive functions including memory and executive functions. Alongside these cognitive deficits, TBI has been associated with altered affect and subjective emotional states. Forceful trauma may disrupt neural substrates and subsequently neurotransmitters altering emotions (Ahmed et al., 2017). Often, emotional disruptions experienced following TBI are displayed as behaviors and emotional reactions that cannot be accurately classified by the Diagnostic and Statistical Manual for Mental Disorders (DSM-V) (Shields et al., 2015). Decreased executive functioning coupled with the disruption of neurotransmitters as a result of TBI can have profound effects on emotional regulation. Large correlations are shown between emotional regulation and executive functioning self and informant report forms in an acquired brain injury sample of 64% were a result of TBI (Stubberud et al., 2020). Screening for emotional deficits after TBI improves the care clinicians provide. Emotional measures adequate for assessing mood disruptions in TBI populations is provided in Table 4.
Recovery in children, adolescents, adults, and elderly
The trajectory of healing after traumatic brain injury (TBI) is significantly influenced by an individual's developmental stage. These stages—childhood (ages < 10), adolescence (ages 10–17), adulthood (ages 18–64), and late adulthood (ages 65+)—mark distinct phases characterized by differing resilience levels and recovery challenges post-TBI. Tailoring interventions to suit these stages optimizes rehabilitation by addressing specific developmental needs and challenges, ensuring more effective functional outcomes and enhanced quality of life.
Many studies suggest that TBI sustained in early childhood tends to have more profound and persistent effects on neuropsychological, psychosocial, and educational outcomes compared with TBI experienced in later childhood (Treble-Barna et al., 2017; Sariaslan et al., 2016; Wade et al., 2016). Younger age and greater TBI severity are linked to poorer functional outcomes (Treble-Barna et al., 2017; Sariaslan et al., 2016; Wade et al., 2016). Age of injury is a critical factor as young children have an increased vulnerability to diffuse brain injury and the harmful effects that such injury may have on their growth and development (Treble-Barna et al., 2017). It is suggested that skills that are rapidly developing during the phase of injury are more susceptible to compromise. Consequently, childhood TBI may pose an increased risk of enduring impairments. TBI severity is another critical factor in functional outcomes, as greater severity is linked to lifelong impairments (Treble-Barna et al., 2017; Sariaslan et al., 2016; Wade et al., 2016). Children with moderate to severe TBI tend to display poorer functioning in different domains, including academic performance, community engagement, interpersonal behavior, emotional state, and cognitive processing (Wade et al., 2016). Sariaslan et al. (2016) found that the risks of disability pension and psychiatric inpatient hospitalization were increased by 106 and 92%, respectively, for individuals who had experienced moderate to severe TBI during their childhood, compared with their unaffected siblings. Another study indicated that children who sustained a severe TBI during childhood displayed impairments in adaptive functioning, based on neuropsychological tests and interviews, 6.9 years post-injury (Tomar et al., 2018). These children displayed deficits in fluid reasoning and processing speed, which predicted risk of poorer outcomes in adulthood. Deficiencies in fluid reasoning may hinder an individual's ability to generate solutions and adapt their thinking to navigate daily problems. At the same time, a decrease in processing speed may interfere with daily tasks like interpreting verbal orders or dealing with the demands of a rapid work environment (Treble-Barna et al., 2017). Jones et al. (2018) found that predictors of poor cognitive function in children 12 months post-injury include low socioeconomic status, male gender, living in rural areas, and having experienced a non-accidental injury.
Within 1 month post-injury, children with mild TBI experienced minimal changes in cognitive recovery and quality-of-life (QoL) (Jones et al., 2018). However, by the 6-month mark, there was observable progress in behavioral adjustment, reflecting improvements from baseline. Similarly, 12 months post-injury, children displayed significant cognitive, behavioral, and QoL improvements.
Studies on moderate-to-severe TBI tend to be grouped together. Kennedy et al. (2022) found that older age at the time of trauma correlated with increased mortality and unfavorable overall recovery in children with moderate-to-severe TBI at 6 months post-injury. However, other studies have distinguished the differences between moderate and severe TBI. Those with severe TBI at the 6-month mark show a deterioration in the ability to copy or memorize complex visual materials, suggesting an increased susceptibility to challenging perceptual tasks (Recla et al., 2013). At 24 months post-injury, children with moderate TBI often face difficulties with short and long-term memory in both verbal and visual domains (Catroppa and Anderson, 2007). Whereas, those with severe TBI functioning worsened during the first year, as shown by their accelerated growth curves, indicating an increased presence of executive function dysfunction, specifically related to emotional control, inhibition, and working memory (Keenan et al., 2021). Severe TBI symptoms also displayed a secondary worsening at 24 months.
TBI presents with considerable heterogeneity across different individuals, as factors such as the severity of the injury, location of brain damage, age, sex, and pre-existing health conditions can all influence the manifestation and outcome of the injury, leading to highly variable outcomes (Walker et al., 2018).
Studies have found that TBIs during adolescence may be associated with more difficulties in recovery than in young children and adults because adolescence is known as a critical window for plasticity, substantial maturation, and growth (Mulligan et al., 2021). During adolescence, cortical structures undergo extensive remodeling through the process of synaptic pruning, corresponding to a decrease in cortical gray matter, acceleration of myelination of axons, increased axon density, and increased white matter volume. These changes allow the acquisition of higher cognitive functions, such as improved cognitive control, enhanced behavioral regulation, and better social cognition (Mulligan et al., 2021). The development of social skills during adolescence is critical for group membership and connection to others, which can significantly impact psychological wellbeing (Di Battista et al., 2014; Mulligan et al., 2021).
Because adolescence represents a crucial stage in brain development and maturation, any disruption to this process may have far-reaching consequences beyond the initial injury phase, leading to lasting impairment. The long-term effects of adolescent mTBI can involve attention and executive function deficits (Gutiérrez-Ruiz et al., 2022). Gutiérrez-Ruiz et al. (2022) found that adolescents with mTBI showed lower cognitive processing speeds and a decreased capacity for selective and sustained attentional tasks. These adolescents also showed an increase in symptoms related to anxiety, depression, withdrawal, and social issues (Gutiérrez-Ruiz et al., 2022). Adolescents with moderate or severe TBI experience continuous and significant declines in overall quality of life due to the impact of TBI on important domains of life, such as school, career opportunities, work functioning, and social interactions (Mulligan et al., 2021). Moreover, alterations in self-perception were observed, encompassing feelings of inferior intelligence and self-consciousness resulting in diminished social identity while increasing their reliance on others. These findings are similar to research on adult survivors of adolescent TBI, who reported “poorer school performance, greater employment difficulties, poor health-related quality of life (HRQoL), and increased risk of mental health problems” (Di Battista et al., 2014, p. 1).
With TBI recovery in adolescents, Rivara et al. (2011) found that those with mild TBI, 3 months post-injury, showed a minor decline in quality-of-life (QoL). During follow up periods, at 12 and 24 months, these patients exhibited lower QoL scores, reaching statistical significance without reaching clinically significant levels. In another study, Ryan et al. (2015a) found that adolescents with mild TBI at 12 months post-injury exhibited minimal issues with social functioning, and their social abilities remained relatively stable over time. These studies suggest that sustaining mild TBI during adolescence results in more favorable recovery outcomes.
Adolescents with moderate-to-severe TBI, 3 months post-injury, showed a significant reduction in the range of activities, social and community-based, that they could engage in. Additionally, they experienced a reduction in their communication and self-care abilities compared to their initial baseline measurements (Rivara et al., 2011). At 12 months post-injury, adolescents with moderate-to-severe TBI showed improvement in the range of activities they could participate in, although significant impairments still persisted. Additionally, at the 24- month mark, there were some minor improvements; however, their Pediatric Quality of Life Inventory scores remained significantly lower when compared to baseline. Upon further observation, these patients' communication and self-care abilities showed no significant improvements (Rivara et al., 2011). Between the 12- and 24-month post-injury period, adolescents with severe TBI experienced a significant increase in social problems (Ryan et al., 2015a).
The factors associated with the healing trajectory for TBIs in adulthood include patient characteristics, such as a history of recurrent mTBIs, younger age, and greater educational attainment (List et al., 2015; Rabinowitz et al., 2018). List et al. (2015) found that adult patients with recurrent TBIs had a higher prevalence of cognitive deficits for those who had sustained three or more mTBIs compared with those who had experienced 1 to 2 mTBIs.
Recurrent mTBIs are seen as a risk factor that contributes to the development of dementia later in life. In some individuals, mTBI may even lead to an earlier onset of Alzheimer's disease (AD). The increased risk of AD may be due to accelerated neurodegeneration caused by TBI-induced neurotoxic processes, inflammatory processes, and the accumulation of hyperphosphorylated tau. However, younger adult age has been found to be correlated with more rapid improvement than older age (Rabinowitz et al., 2018). Education has also been indicated as a moderating factor in post-injury function. Rabinowitz et al. (2018) found that participants' education level had a significant effect on their processing speed (PS), executive function (EF), and verbal learning (VL) but did not have an impact on their recovery trajectory. In comparison, younger age was correlated with better recovery of both simple and complex PS and EF. This may indicate that age is a protective factor in the recovery of TBI. However, recurrent injury leads to dose-dependent cortical thinning, resulting in detrimental effects on cognitive function that can contribute to the development of AD (List et al., 2015).
With TBI recovery in adults, Othman et al. (2022) found that 19.2% of adults with mild TBI, 3 months post-injury, showed cognitive impairment, while 19.2% exhibited neuropsychiatric manifestations. At 6 months post-injury, patients continued to experience persistent cognitive impairment, while the remaining majority showed signs of recovery. In another study, Scholten et al. (2015) found that at 6 and 7 months post-injury, adults with mild TBI exhibited significantly more favorable outcomes in various domains, such as Physical Component Summary (PCS) scores, physical functioning, role physical, social functioning, and role emotional. At 12 months post-injury, patients demonstrated outcomes that were similar to population norms, indicating a substantial level of recovery.
In adults with moderate TBI, 3 months post-injury, 39.3% of patients presented cognitive impairment, while 25% exhibited neuropsychiatric manifestations (Othman et al., 2022). At 6 months post-injury, no patients exhibited persistent cognitive impairment or neuropsychiatric manifestations. However, the study by Scholten et al. (2015) found that functional outcome and Health-Related Quality of Life (HRQL) were notably lower when compared to the outcomes observed after mild TBI. Notably, when examined 12 months post-injury, patients with moderate TBI scored higher on physical functioning, general health, and vitality on the Short-Form Health Survey (SF-36; reflecting physical, mental, and social functioning) than those with mild TBI. However, Glasgow Outcome Scale Extended (GOSE) scores were significantly lower than for those with mild TBI (Scholten et al., 2015).
Wilkins et al. (2019) found that in adults with severe TBI, 3–6 months post injury, 43% of survivors demonstrate improvement in their outcome, transitioning from unfavorable to favorable. Interestingly, 6 months post-injury, severe TBI patients scored higher in nearly all SF-36 domains and reported higher PQoL scores than moderate TBI (Scholten et al., 2015). This could be attributed to survivors of severe injuries perceiving some of their challenges as less difficult or their gratitude for being alive potentially outweighing concerns about their functional abilities. However, their functional outcome and HRQL were notably lower compared to the outcomes observed after mild TBI (Scholten et al., 2015). Twelve months post-injury, patients with severe TBI had significantly lower outcomes than mild TBI in the SF-36 domains of PCS, physical functioning, role physical, social functioning, and role emotional. Additionally, GOSE was significantly lower for severe TBI than mild TBI (Scholten et al., 2015). This observation aligns with the study by Wilkins et al. (2019), which reported that 38% of patients with severe TBI improved from unfavorable to favorable outcomes from 12 to 24 months.
When examining traumatic brain injuries (TBIs) in late adulthood, it becomes apparent that the healing trajectory is worse. The majority of the literature indicates an increase in mortality rates and poor functional outcomes. Elderly adults experience worse outcomes after TBIs due to their age, which is found to be an independent risk factor (Prasad et al., 2018; Toth et al., 2021). As individuals age, the brain undergoes atrophy, which leads to an increase in the distance between the brain and skull, making the dural vessels more susceptible to shearing damage. Many elderly patients may live with medical conditions that are masked by TBIs, and their decreased cerebral reserve makes them more vulnerable to minor injuries (Prasad et al., 2018). For instance, if an individual with advanced dementia suffers a head injury, it can lead to cognitive impairments that may prevent independent living. There is a lack of clear guidelines for the treatment of elderly TBI, and the existing guidelines are primarily based on studies of younger adults. This contributes to higher mortality rates and poor functional outcomes in elderly patients. Prasad et al. (2018) further found that, compared with younger patients, elderly patients typically have more extended rehabilitation stays, higher total rehabilitation costs, and a lower rate of improvement in functional measures. Aging and TBI also increase the risk of developing cerebral microbleeds (CMBs). Toth et al. (2021) found that both aging and TBIs can cause CMBs through mechanisms such as cerebrovascular oxidative stress, matrix metalloproteinase activation, and changes in the cerebrovascular wall. CMBs impact the healing trajectory of the elderly because they may lead to “cognitive impairments, psychiatric disorders, and gait dysfunction” (p. 1).
The prognosis for recovery following traumatic brain injury in older adults is generally poor, with substantial evidence indicating high mortality rates and limited functional independence. TBI recovery in older adults shows that those with mild TBI, 3 months post injury, exhibited lower cognitive functioning and performed worse on neuropsychological tests compared to non-injured peers (Hume et al., 2023). At 6 months post-injury, 14% of older adult patients with mild TBI died (Utomo et al., 2009). Thompson et al. (2020) compared older and younger adults with mild TBI, finding that from 1 week to 1 year post-injury, older adults consistently reported poorer overall physical Health-Related Quality of Life (HRQOL) than their younger counterparts.
Older adults with moderate TBI, 3 months post-injury, experience significantly poorer functional health status, with an average Glasgow Outcome Scale Extended (GOSE) score of 5.1, indicating a greater degree of disability following a moderate TBI compared to younger adults (Thompson et al., 2020). At 6 months post-injury, 47.8% of patients with moderate TBI died (Utomo et al., 2009). One-year post-injury, older adults with moderate TBI continued to report an ongoing average of 3.9 symptoms out of 17 symptoms assessed, with a higher likelihood of experiencing balance and coordination issues (Thompson et al., 2020).
Older adults with severe TBI, 6 months post-injury, with a Glasgow score of <9 exhibited unfavorable outcomes, with a significant mortality rate of 83.3% (Utomo et al., 2009). Similarly, Maiden et al. (2020) found that 6 months post-injury, 85% had died, 47% were living dependently, and 6% had recovered to functional independence. By 12 months post injury, 86.3% had died, 6.4% were living dependently, and 7.3% had recovered to functional independence. Overall, the data reveals that older adults with severe TBI face a substantial risk of death, with only a minority regaining functional independence. The following figure (Figure 1) shows children, adolescent, adult, and elderly TBI risk factors.
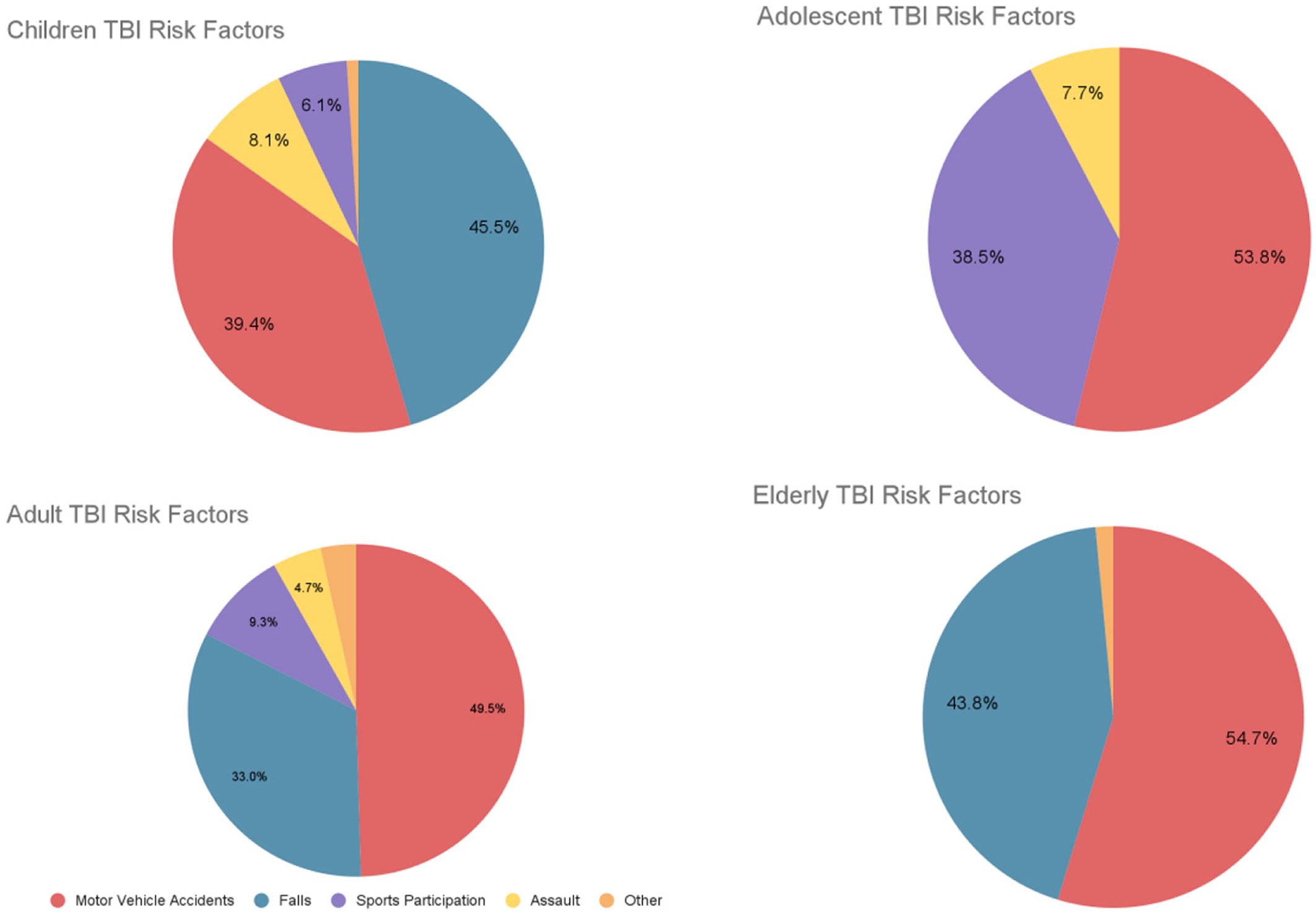
Figure 1. Children, adolescents, adults, and elderly TBI risk factors. Based on data from Keenan et al. (2021), Mulligan et al. (2021), Scholten et al. (2015), and Prasad et al. (2018).
Phenomenology of TBIs
Phenomenological studies of TBI healing progression can provide valuable insights into the subjective experiences of individuals with TBI and the factors that shape their recovery journey. By understanding the individual's perspective on their recovery, healthcare professionals can better tailor their treatment approaches and support the individual in their efforts to recover and return to their everyday lives.
Many phenomenological studies of TBI have interviewed patients during their recovery to assess their functional outcomes and wellbeing over time. Wellbeing is typically categorized as psychological, physical, and social, but there is often overlap between these categories in the literature on TBI healing progression.
Visser et al. (2021) conducted a study examining patients' wellbeing after injury, from their experiences in the emergency room to discharge and rehabilitation. Patients who were severely injured reported feeling a fear of dying while being treated in the ambulance and emergency room. For instance, one patient (female, >16 years) feared she was going to die after seeing blood spouting from her leg, thinking it was arterial bleeding (Visser et al., 2021). Patients who were sedated, unconscious, or experiencing post-traumatic amnesia during treatment often reported feelings of confusion and anxiety about the events of their injury. The realization that they had survived the injury often came to patients during hospitalization and recovery, and this realization was frequently accompanied by a fear of permanent physical limitation, replacing the earlier fear of dying. These findings highlight the initial emotional turmoil and evolving fears faced by patients after severe injuries.
Many patients reported experiencing symptoms of acute stress disorder (ASD) during hospitalization and post-traumatic stress disorder (PTSD) during rehabilitation. Stenberg et al. (2022) followed up with patients 1 and 7 years after TBI and found that wellbeing resulted from adaptation to a recovered or changed life situation. Those with moderate to severe disabilities reported poor wellbeing because adaptation was an ongoing process. However, patients reported that adaptation and wellbeing were facilitated by factors such as leading a purposeful daily life, maintaining an optimistic perspective, and employing adaptive strategies, such as applying knowledge gained from previous hardships. For instance, when asked about the ability to adapt, one patient (Female, 36 years) expressed that despite her permanent injuries, she focused on choosing to be positive and appreciating the good things in life rather than being sad about the negative things (Stenberg et al., 2022). Developing resilience and a positive outlook helped mitigate the negative emotional impact of TBIs in patients' lives.
Experiences related to physical function also contributed to patients' wellbeing. Visser et al. (2021) found that patients often reported that the time needed for recovery was much longer than they anticipated. The desire to feel autonomous rather than helpless often motivated severely injured patients to push through physical limitations during rehabilitation. Stenberg et al. (2022) found that patients with severe TBI reported suffering from life long limitations that impact their health and wellbeing. Overcoming obstacles such as the denial of one's disabilities, feelings of guilt, shame, loneliness, and isolation, and avoidant behavior were all described as necessary for adaptation and wellbeing by severely injured patients. For instance, when asked about difficulties in adapting, one patient (Male, 27 years) expressed increasing depression and frustration due to the persistent impact of his injuries on his life (Stenberg et al., 2022). These experiences reveal the persistent challenges and emotional struggles that accompany physical recovery.
Social support is another critical aspect of TBI recovery, as it is known to improve mental health and overall wellbeing. Visser et al. (2021) found that while it was challenging to rely on others, patients were thankful for the assistance they received from loved ones. In addition, patients believed that their friends' and family's support could aid their recovery. Stenberg et al. (2022) found that living with a severe disability impacted patients' social wellbeing. Patients coping with a severe disability described it as frustrating and leading to loneliness in daily life, as it resulted in exclusion from work and social networks, and the inability to participate in leisure activities. For instance, when asked about living with a disability, one patient (Male, 57 years) described feeling extremely lonely and frustrated by his inability to communicate and participate in activities, ultimately accepting his situation with difficulty (Stenberg et al., 2022). Social support is crucial in mitigating the isolation and emotional distress experienced by TBI patients.
Defense or deficit?
Phenomenological studies help “humanize” TBI accounts, especially in such reviews as this. The difficulties mentioned in previous examples, in combination with cognitive deficits summarized throughout lead to another important consideration for all neuropsychologists, especially when involved in therapeutic intervention (directly or indirectly). This is the development of the knowledge and skills necessary to help determine whether the patient's presentation is a psychological defense or deficit. These defenses can be a false positive, masking a cognitive deficit, or the cognitive deficit may mimic a defense. The following are some examples where defenses and deficits may be confused:
1. Anosagnosia, or a lack of insight into ones own impairments, may be confused with denial.
2. Executive dysfunction may lead to misattribution of blame, disinhibition and lack of self insight which could be confused with projection, acting out or displacement.
3. Rationalization may also stem from impaired judgment due to frontal compromise, as opposed to a personality type, or emotional reaction.
4. Repression or lack of details related to PTSD could be confused with significant reductions in memory processes related to TBI.
5. Reaction formation or when a polarization of belief occurs, specifically one that was opposite to what the individual previously identified with could arise from deficits in memory, and emotion related systems.
In these instances, a neuropsychological evaluation will play an important role for clinical judgment. TBI victims in inpatient units are typically physically and emotionally vulnerable, creating an environment conducive to the expression of defenses. Outpatients will likely be easier to determine, given discharge is normally associated with a level of stability that improves the likelihood of a comprehensive evaluation, consistency and increased number of sessions.
Treatment and rehabilitation
Once the patient has been assessed, and deficits ascertained, what can be done? Treatment and rehabilitation for TBI is dependent on the recovery stage of the patient. In the acute stage, in the hours immediately following injury, the main focus of treatment is to stabilize the patient and focus on preventing further injury. Typically, treatment is carried out at an intensive care unit, followed by a neurosurgical ward. For chronic stages of recovery, rehabilitation is the main treatment, and a variety of rehabilitation and treatment protocols exist.
The Rancho Los Amigos Scale-Revised (RLAS-R) is an assessment tool used to describe the cognitive and behavioral patterns found in brain injury patients as they recover from injury. The scale consists of ten levels, with the first level representing the lowest level of functioning, and the tenth level representing the highest. As a patient progresses through higher levels, they demonstrate an improved capacity toward achieving greater independence. Overall, the scale helps facilitate communication among treating healthcare professionals and aids in treatment planning; individualized treatment interventions can be administered depending on the patient's level of functioning and impairments. While individuals move through the different levels in a sequential pattern, the amount of time spent in each level and the maximum level achieved varies by individual. Additionally, levels can be skipped during their recovery, and behaviors can overlap between two different levels. The following table (Table 5) shows the RLAS-R.
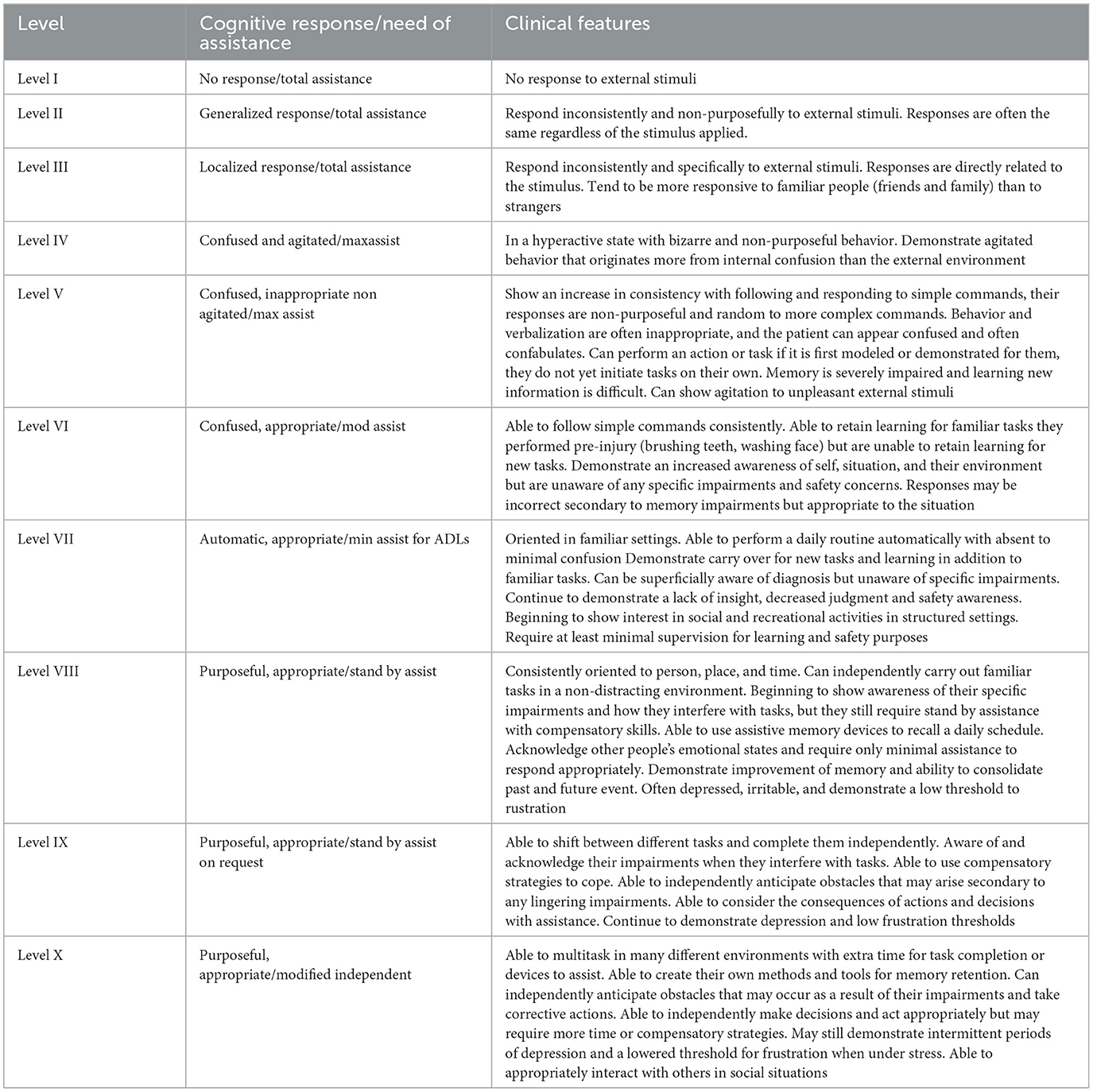
Table 5. Rancho Los Amigos (revised) (Hagen et al., 1972; Lin and Wroten, 2023).
The RLAS-R is typically paired with the GCS to assist in determining the TBI patient's responsiveness. However, unlike the GCS, the RLAS-R is usually used throughout the recovery period, and is not limited to the initial assessment. Earlier screening of cognitive function is important for the prediction of recovery outcomes and facilitates rehabilitation planning.
The administration of the RLAS-R can also be used in conjunction with the Montreal Cognitive Assessment (MoCA). The MoCA is a cognitive assessment that is administered in roughly 10 min and scored on a maximum of 30 points. The domains of function measured includes visuospatial/executive functioning, memory, language, attention, concentration, working memory, and orientation. Individuals with mild and moderate TBI typically have better performances on the MoCA than those with severe TBI (de Guise et al., 2013), and the MoCA can reliably detect impairment in mild TBI and differentiate cognitive disabilities between mild to severe TBI (Mishra et al., 2020).
Once a patient is medically stable following the TBI, they may be transferred to a subacute rehabilitation unit of a medical center, or to an independent rehabilitation hospital. Typically, a multidisciplinary approach is utilized to optimize patient outcomes. The target of rehabilitation treatment will depend on the injury sustained and the associated neurological deficits; as such, treatment may include (but not limited to) physiotherapy, speech and language therapy, cognitive rehabilitation therapy, and occupational therapy.
Cognitive rehabilitation typically consists of training various aspects of cognitive functioning, including learning and memory, visual attention and auditory attention, psychomotor function, and executive functioning. Restorative interventions aim to restore impaired functionality, while compensatory interventions (i.e., cognitive remediation) assist in the development of strategies to minimize the functional impact of the impairment. Neuropsychologists can assess for TBI-induced deficits utilizing a wide-variety of assessment tools, and can prescribe various forms of training to restore or remediate cognitive functioning.
Attention-related difficulties are common among individuals with TBI and can manifest in a variety of ways. Computer-mediated tasks designed to retrain attention can lead to gains on the trained tasks with generalization to similar cognitive measures. However, the results do not always generalize to everyday attentional behavior. Engaging in metacognition, which includes self-reflective capacities and an increase in self-awareness and self-monitoring, has been found to compensate for attentional problems in adults (Ponsford et al., 2014). Metacognitive can also help with problem solving in adults (Tate et al., 2014).
Memory problems can be compensated for in various ways; internal memory strategies, such as mnemonics, visual imagery, and self-instructional methods have been shown to enhance performance on neuropsychological tests. Other helpful memory supports include diaries, notebooks, smart phones, and electronic calendars. Additionally, self-instructional methods that aim to enhance the individual's understanding of manifestations of their memory problems have been shown to improve prospective memory (Velikonja et al., 2014).
TBI often results in communication disturbances that can lead to impaired social competence for both children and adults. Social communication training in group formats has been found to be an effective intervention for individuals 6–24 months post injury. Such training can incorporate everyday communication with partners, and individuals are encouraged to practice the skills they learn beyond the period of training. Social communication interventions can be provided in the natural environment of the person's everyday life (Togher et al., 2014).
Several non-invasive brain stimulation technologies have been developed that can lead to reduced symptomology for TBI patients. The most common technologies include transcranial magnetic stimulation (TMS) and transcranial direct current stimulation (tDCS). tDCS has been shown to reduce TBI-associated depression, tinnitus, neglect, memory deficits, and attention disorders (Dhaliwal et al., 2015). Repetitive transcranial magnetic stimulation (rTMS) is another non-invasive, easily operated treatment that has been shown to improve depression and increase cognitive function after TBI (Neville et al., 2015).
Virtual Reality (VR) has been shown to be an effective rehabilitation tool and can function both as an assessment instrument and as a therapeutic intervention. Dahdah et al. (2017) found that VR can enhance executive functions and information processing in the sub-acute phase of TBI. It has also been used for attention training in severe TBI (Dvorkin et al., 2013), and has been effective in addressing and treating balance deficits (Cuthbert et al., 2014).
Digital brain games overall have yielded mixed results. Some however have demonstrated to efficiently improve cognition among individuals with TBI. BrainHQ is a common and frequently used online platform in which users can play games to train their memory, attention, cognitive speed, interpersonal skills, intelligence, and navigation. Research has found that BrainHQ training can result in both objective and subjective improvements on cognitive measures, which correlates with changes in functional connectivity between the DMN and other resting-state networks in adults with chronic TBI (Lindsey et al., 2022).
Functional electrical stimulation (FES) refers to a process whereby a low-frequency pulse current is used to stimulate limb or organ dysfunction, thereby replacing or correcting lost function in limbs and organs. FES has been shown to have a positive effect on a TBI patient suffering from dysphagia (Calabrò et al., 2016). Though this study only included one individual, the results demonstrated that FES led to significantly improved swallowing functionality in this patient, who could eventually eat solid food safely after the treatment. This suggests that FES may constitute a promising effective treatment for improving lost functionality after TBI, and future research should continue to investigate its potential therapeutic effects with larger sample sizes. There is also evidence that FES can lead to cortical reorganization among individuals with TBI. Milosevic et al. (2021) found that FES treatment led to cortical reorganization and motor improvements in a male participant with chronic TBI suffering from mild motor impairment affecting his right upper-limb. Widespread changes were observed in the motor, premotor, sensory, and parietal cortices in both contralateral and ipsilateral hemispheres and his drawing test performance showed improvements after the intervention and during 3-month follow ups. Again, while this study only included one individual, the results suggest that FES may be an effective tool to improve motor functionality and elicit cortical re-organization and highlight the necessity for future research with larger cohorts of patients.
Hyperbaric Oxygen Therapy (HBOT) refers to the inhalation of 100% at higher than atmospheric pressure and has been used as a treatment for a variety of neurological conditions. HBOT can lead to greatly improved symptomology and a reduction in TBI-related cognitive deficits (Harch et al., 2012). In severe TBI, HBOT has been shown to reduce mortality and enhance functional outcomes in both adults (Lv et al., 2011) and children (Prakash et al., 2012). There is also evidence that HBOT may suppress activation of inflammation signals which TBI induces (Meng et al., 2016). However, it must be noted that despite the published literature supporting HBOT's efficacy for TBI, there have been limited double-blind placebo-controlled trials, and thus HBOT is not an FDA-approved therapy for TBI.
Severe TBI can lead to cerebral edema, which can precipitate intracranial hypertension. The treatment of cerebral edema involves cerebrospinal fluid (CSF) drainage, administration of osmolar agents, and/or craniectomy. The prevention of the development of severe oedema can be targeted by emerging treatments such as glibenclamide, which selectively acts on specific ion channel openings in the brain that can prevent the build-up of fluid (Blennow et al., 2016).
Future considerations
Future research on traumatic brain injury (TBI) should prioritize the development of multidimensional outcome assessments to more accurately capture individual impairments and establish precise prognostic endpoints. Traditional global or unidimensional instruments may not fully capture the nuances of TBI-related disabilities. Cognitive impairments and mental health outcomes distinguish between patients with TBI on different levels of disability severity. Cognitive impairment often distinguishes between those with moderate and severe disabilities, while adverse mental health outcomes differentiate patients with higher levels of functional outcomes. Multidimensional outcome assessment can utilize unidimensional instruments such as the Rivermead Post-Concussion Symptoms Questionnaire by reconfiguring a general factor of severity of symptoms into core dimensions of psychopathology (e.g., internalizing factors, somatic symptoms) (Nelson et al., 2021). The individual impact of TBI is inextricably related to individual factors (e.g., preinjury mental health, coping skills), as well as the larger socioeconomic context of access to healthcare. Future studies warrant sample cohorts that are large and heterogeneous in order to distinguish factors and contribute toward a multifactorial (bio-psycho-socio-ecological) model of TBI. The multifactorial model, encompassing biological, psychological, interpersonal, and contextual dynamics, clarifies the relationship between individual and socio-economic determinants of health. This model views health as a dynamic interaction of various systems evolving over time. Following a TBI, biological responses like immune system activation shift alongside changes in interpersonal and psychological dynamics. For instance, in the acute phase, biological factors such as inflammation may dominate, while psychological factors like coping strategies become central during rehabilitation (Lehman et al., 2017). Interpersonal dynamics, such as family support, also play a pivotal role throughout the recovery process.
Neuropsychological research should continue distinguishing the efficacy of particular assessments in distinct contexts (e.g.. sex, education, culture, age, etc.). This promotes a more effective selection of batteries that can be tailored to patients. It will also help direct novices in the field in knowing what variables to pay attention to. In addition, approaching TBIs from a neurophenomenological approach may be greatly beneficial. For instance, in major traumatic brain injuries, global dysfunction (e.g., PTA) undergoes a particular temporal sequence toward recovery. This sequence will vary depending on individual differences, yet it is also possible for there to be underlying commonalities more deeply embedded in the brains organic recovery process. A potential sequence may be reflected in a temporospatial model that tracks the development and maturation of emotion and thoughts (Chan et al., 2022). A more nuanced understanding of this sequence may yield important results that may enrich treatment.
Research on TBI should also increasingly address the chronic nature of TBI symptoms given that TBI symptoms can persist or worsen over time and that a majority of moderate to severe TBI patients experience a decline in functioning and an estimated 20 percent die within 5 years of injury (Whiteneck et al., 2018). An individual's functional outcomes at 2 and 5 years after an injury are greatly influenced by their functional status at the first year. However, several factors, such as age, race, payor source (referring to the source of funding for healthcare expenses), length of rehabilitation stay, and cognitive and motor scores, also have a significant impact on how their condition progresses over time (Dams-O'Connor et al., 2020). Current TBI care in the USA frequently fails to meet the needs of individuals, families, and communities impacted by TBI (National Academies of Sciences, 2022). Long-term problems can arise beyond the period of post-acute care: fatigue, sleep disturbance, cognitive impairments affecting memory, attention, and executive function, anxiety, and depression, which can affect individuals' ability to work, study, and drive for at least 5–10 years post-injury (Maas et al., 2022). Although international guidelines for treatment of cognitive and communication impairments have been updated in 2022, more large-scale controlled studies are required to validate new interventions (Bayley et al., 2023).
Furthermore, future research should explore the prevalence and long-term effects of post concussion-like symptoms in patients with different types of injuries. Post-concussion-like symptoms are common among all injury patients, and not just those with head injuries. Understanding the risk factors for developing post-concussion symptoms, such as low educational level, road traffic accidents, chronic diseases, and hospitalization, is also important for early detection and prevention (van der Vlegel et al., 2021). Further studies should also investigate the impact and treatment of post-concussion-like symptoms on the quality of life of patients, healthcare utilization, and return to work rates, as these factors can inform the development of supportive care programs.
The use of biomarkers in classifying injury severity of TBI shows a promising path toward identifying specific patient groups related to disease endotypes. Recent research has assessed certain endotypes (e.g., pH, lactate, blood glucose, and platelet count) (Åkerlund et al., 2022), which may correlate with established measures of TBI impact such as the Glasgow Coma Scale score and degree of metabolic derangement. Other circulating biomarkers like amyloid beta (Hossain et al., 2020) and heart fatty acid binding protein (Lagerstedt et al., 2020) are also being studied to be able to categorize subgroups of TBI patients with different inflammatory endotypes or detect the presence of autoantibodies against brain and extracranial antigens (Maas et al., 2022). As these biomarkers may reflect the body's inflammatory response post-injury, clinicians can more appropriately select and implement anti-inflammatory and immunomodulatory therapies with this data. Future identification of new biomarkers will involve advanced analytic techniques, including artificial intelligence and machine learning, and may help refine important medical decisions throughout the treatment and rehabilitation process. The use of fMRI (Madhavan et al., 2019) and MR spectroscopy (Nadel et al., 2021) as research techniques show promise as future clinical tools for assessing TBI patients. Advanced MRI techniques in combination with volumetric analyses (Stein et al., 2021) have demonstrated increased sensitivity to detecting specific injuries, e.g., traumatic axonal injury, which would typically not be detectable by visual inspection. For mTBI patients, such injuries may not present on CT imagery but are relevant for both persistent post-concussion symptoms and long-term disability. There is also an increasing interest in utilizing MRI to assess the chronic phase of TBI because brain injury biomarkers identified by MRI techniques may predict late brain volume loss and reflect accelerated brain aging (Gan et al., 2021; Yin et al., 2019).
While the field of neurorehabilitation is growing, there is still a lack of support concerning what combinations of treatments may be best depending on the type and severity of TBI. Moreover, the use of computerized interventions has also been on the rise, yet research appears to be mixed. Further specificity as to how these differ from each other may lead to the development of more efficacious programs. Beyond programs focused on cognitive restoration, progress on the development of new compensatory strategies have also appeared to stagnate. It will be beneficial for the development of new strategies considering how much science and technology have advanced (e.g., since birth of ideas such as chunking, visualization or method of the loci). In addition, while individualizing such techniques for everyday experiences is commonly done by practitioners involved with neurorehabilitation, it would be of benefit to identify their efficacy under different contexts. The future of rehabilitation will likely be combined with technological advancements. A deeper examination of roles between clinician and technology will give way to the optimal use of new technologies. An example of this might be the importance of the clinician assisting patients in the translation of skills from virtual brain games to everyday life.
Another route of investigation, more biologically oriented relates to mechanisms underlying the long-term effects of TBI, including the role of neurotoxic processes, inflammatory processes, and the accumulation of hyperphosphorylated tau in the development of dementia and AD. Additionally, more research is needed to understand the psychological effects of TBI, including the prevalence and long-term effects of affective disorders such as anxiety, depression, and PTSD. TBIs can have significant psychological consequences, as they can disrupt the brain's functioning and alter neural pathways involved in emotional regulation. This research may involve longitudinal studies that track individuals with TBIs over an extended period, assessing their psychological wellbeing and identifying risk factors that contribute to the development of affective disorders. By gaining a better understanding of these psychological effects, healthcare professionals can develop more targeted interventions and support systems to improve the overall outcomes and quality of life for individuals with TBIs.
Conclusion
The assessment and treatment of TBIs provides a unique window into the brain's capabilities and vulnerabilities. Similar to how brain lesions provide a deeper understanding of their corresponding functions, TBIs may be a lens into the underlying processes of cognition, emotion and personality. TBIs are a multidimensional, complex injuries that can result in a range of physical, cognitive, and emotional deficits. The brain areas most vulnerable to injury are the frontal and temporal cortices leading to deficits in executive functioning, attention, memory, language, and emotional processing. Deficits in processing correlate with impairments in identifying negative emotions, affective disorders such as anxiety, depression, and PTSD. Mild TBI, accounting for the majority of cases, can cause temporary post-concussive symptoms that may resolve within a few months and temporary cognitive symptoms within a few days. However, even a single mTBI or recurrent mTBI can result in the emergence of depressive-like behaviors up to 90 days following the injury. On the other hand, moderate and severe TBIs are more serious and can lead to long-term complications, even death.
The trajectory of healing for TBI varies depending on the developmental stage of the individual. Adolescents who sustain TBI may have more difficulties in recovery than young children and adults due to their critical window for plasticity, substantial maturation, and growth. Several factors influence the healing process of traumatic brain injuries (TBIs) in adults. These factors include patient characteristics like a history of repeated TBIs, younger age, and higher levels of education. Individuals who have experienced multiple TBIs are more likely to have cognitive deficits compared with those who have had fewer incidents. Recurrent TBIs are also associated with an increased risk of developing dementia later in life. In certain cases, mTBI may even accelerate the onset of Alzheimer's disease.
Evolution has left humans with the cognitive ability to generate sophisticated methods for the assessment and treatment of TBIs. Grounded in research, neuropsychological assessments are themselves a product of evolutionary necessity, offsetting our brains vulnerability by providing critical and nuanced information that can be used practically. At its core, neuropsychological results provide (1) viable predictions on functional outcome that can assist with expectation management, (2) strengths that can be used to compensate for challenges, (3) weaknesses that are specifically targeted for restoration/compensation, and (4) an optimal path for recovery and adaptation.
Author contributions
AC: Conceptualization, Funding acquisition, Methodology, Resources, Supervision, Writing – original draft, Writing – review & editing. JO: Supervision, Writing – original draft, Writing – review & editing. KN: Writing – original draft. AJ: Writing – original draft. SB: Writing – original draft. RK: Supervision, Writing – review & editing.
Funding
The author(s) declare that no financial support was received for the research, authorship, and/or publication of this article.
Conflict of interest
The authors declare that the research was conducted in the absence of any commercial or financial relationships that could be construed as a potential conflict of interest.
Publisher's note
All claims expressed in this article are solely those of the authors and do not necessarily represent those of their affiliated organizations, or those of the publisher, the editors and the reviewers. Any product that may be evaluated in this article, or claim that may be made by its manufacturer, is not guaranteed or endorsed by the publisher.
References
Åkerlund, C. A. I., Holst, A., Stocchetti, N., Steyerberg, E. W., Menon, D. K., Ercole, A., et al. (2022). Clustering identifies endotypes of traumatic brain injury in an intensive care cohort: a CENTER-TBI study. Crit. Care 26:228. doi: 10.1186/s13054-022-04079-w
Aguerrevere, L. E., Greve, K. W., Bianchini, K. J., and Ord, J. S. (2011). Classification accuracy of the Millon Clinical Multiaxial Inventory–III modifier indices in the detection of malingering in traumatic brain injury. J. Clin. Exp. Neuropsychol. 33, 497–504. doi: 10.1080/13803395.2010.535503
Ahmed, A., Venigalla, H., Mekala, H., Dar, S., Hassan, M., and Ayub, S. (2017). Traumatic brain injury and neuropsychiatric complications. Indian J. Psychol. Med. 39, 114–121. doi: 10.4103/0253-7176.203129
Alhourani, A., Wozny, T. A., Krishnaswamy, D., Pathak, S., Walls, S. A., Ghuman, A. S., et al. (2016). Magnetoencephalography-based identification of functional connectivity network disruption following mild traumatic brain injury. J. Neurophysiol. 116, 1840–1847. doi: 10.1152/jn.00513.2016
Allanson, F., Pestell, C., Gignac, G., et al. (2017). Neuropsychological predictors of outcome following traumatic brain injury in adults: a meta-analysis. Neuropsychol. Rev. 27, 187–201. doi: 10.1007/s11065-017-9353-5
Anderson, L. B., Jaroh, R., Smith, H., Strong, C.-A. H., and Donders, J. (2017). Criterion validity of the D-KEFS color–word and verbal fluency switching paradigms following traumatic brain injury. J. Clin. Exp. Neuropsychol. 39, 890–899. doi: 10.1080/13803395.2016.1277513
Aquino, C., Woolen, S., and Steenburg, S. D. (2014). Magnetic resonance imaging of traumatic brain injury: a pictorial review. Emerg. Radiol. 22, 65–78. doi: 10.1007/s10140-014-1226-0
Arulsamy, A., Teng, J., Colton, H., Corrigan, F., and Collins-Praino, L. (2018). Evaluation of early chronic functional outcomes and their relationship to pre-frontal cortex and hippocampal pathology following moderate-severe traumatic brain injury. Behav. Brain Res. 348, 127–138. doi: 10.1016/j.bbr.2018.04.009
Ashendorf, L. (2019). Rey Auditory Verbal Learning Test and Rey–Osterrieth Complex Figure Test performance validity indices in a VA Polytrauma sample. Clin. Neuropsychol. 33, 1388–1402. doi: 10.1080/13854046.2018.1543454
Aslaksen, P. M., Ørbo, M., Elvestad, R., Schäfer, C., and Anke, A. (2013). Prediction of on-road driving ability after traumatic brain injury and stroke. Eur. J. Neurol. 20, 1227–1233. doi: 10.1111/ene.12172
Azeredo, A., Geraldo, A., Moreira, D., and Barbosa, F. (2024). Traumatic brain injury and malingering: a systematic review. Psychol. Neurosci. 17, 61–88. doi: 10.1037/pne0000331
Bayley, M. T., Janzen, S., Harnett, A., Bragge, P., Togher, L., Kua, A., et al. (2023). INCOG 2.0 guidelines for Cognitive Rehabilitation following traumatic brain injury: what's changed from 2014 to now? J. Head Trauma Rehabil. 38, 1–6. doi: 10.1097/htr.0000000000000826
Bell, D. R., Guskiewicz, K. M., Clark, M. A., and Padua, D. A. (2011). Systematic review of the balance error scoring system. Sports Health 3, 287–295. doi: 10.1177/1941738111403122
Ben-David, B., Nguyen, L., and Van Lieshout, P. (2011). Stroop effects in persons with traumatic brain injury: selective attention, speed of processing, or color-naming? A meta-analysis. J. Int. Neuropsychol. Soci. 17, 354–363. doi: 10.1017/S135561771000175X
Beng, J. F., Caroselli, J. S., and Temple, R. O. (2007). Wisconsin Card Sorting Test: factor structure and relationship to productivity and supervision needs following severe traumatic brain injury. Brain Inj. 21, 395–400. doi: 10.1080/02699050701311091
Bigler, E. D. (2013). Traumatic brain injury, neuroimaging, and neurodegeneration. Front. Hum. Neurosci. 7, 1–15. doi: 10.3389/fnhum.2013.00395
Blennow, K., Brody, D. L., Kochanek, P. M., Levin, H., McKee, A., Ribbers, G. M., et al. (2016). Traumatic brain injuries. Nat. Rev. Dis. Prim. 2:16084. doi: 10.1038/nrdp.2016.84
Boccia, M., Barbetti, S., Valentini, F., De Angelis, C., Tanzilli, A., Fabio, V., et al. (2022). Neural underpinnings of the slowness of information processing in patients with traumatic brain injury: Insights from tract-based spatial statistics. Neurol. Sci. 43, 5083–5086. doi: 10.1007/s10072-022-06150-4
Calabrò, R. S., Nibali, V. C., Naro, A., Floridia, D., Pizzimenti, M., Salmeri, L., et al. (2016). Is non-invasive neuromuscular electrical stimulation effective in severe chronic neurogenic dysphagia? Reporton a post-traumatic brain injury patient. NeuroRehabilitation 38, 53–57. doi: 10.3233/nre-151295
Carlozzi, N. E., Grech, J., and Tulsky, D. S. (2013). Memory Functioning in individuals with traumatic brain injury: An Examination of the Wechsler Memory Scale-Fourth Edition (WMS IV). J. Clin. Exp. Neuropsychol. 35, 906–914. doi: 10.1080/13803395.2013.833178
Carlozzi, N. E., Kirsch, N. L., Kisala, P. A., and Tulsky, D. S. (2015). An Examination of the wechsler adult intelligence scales, fourth edition (WAIS-IV) in individuals with complicated mild, moderate and severe traumatic brain injury (TBI). Clin. Neuropsychol. 29, 21–37. doi: 10.1080/13854046.2015.1005677
Catroppa, C., and Anderson, V. (2007). Recovery in memory function, and its relationship to academic success, at 24 months following pediatric TBI. Child Neuropsychol. 13, 240–261. doi: 10.1080/09297040600837362
CDC (2016). Traumatic Brain Injury in the United States: Emergency Department Visits, Hospitalizations and Deaths 2002-2006 (Blue Book). Centers for Disease Control and Prevention. Available at: https://www.cdc.gov/traumaticbraininjury/tbi_ed.html (accessed April 22, 2023).
CDC (2022). TBI Data. Centers for Disease Control and Prevention. Available at: https://www.cdc.gov/TraumaticBrainInjury/data/index.html (accessed April 22, 2023).
Cermak, C. A., Scratch, S. E., Kakonge, L., and Beal, D. S. (2021). The effect of childhood traumatic brain injury on verbal fluency performance: a systematic review and meta-analysis. Neuropsychol. Rev. 31, 1–13. doi: 10.1007/s11065-020-09475-z
Chan, A. (2021). Reassembling Models of Reality: Theory and Clinical Practice. New York, NY: W. W. Norton.
Chan, A., Northoff, G., Karasik, R., Ouyang, J., and Williams, K. (2022). Flights and perchings of the brainmind: a temporospatial approach to psychotherapy. Front. Psychol. 13:828035. doi: 10.3389/fpsyg.2022.828035
Coffey, B. J., Threlkeld, Z. D., Foulkes, A. S., Bodien, Y. G., and Edlow, B. L. (2021). Reemergence of the language network during recovery from severe traumatic brain injury: A pilot functional MRI study. Brain Inj. 35, 1552–1562. doi: 10.1080/02699052.2021.1972455
Cuthbert, J. P., Staniszewski, K., Hays, K., Gerber, D., Natale, A., and O'Dell, D. (2014). Virtual reality-based therapy for the treatment of balance deficits in patients receiving inpatient rehabilitation for Traumatic Brain Injury. Brain Inj. 28, 181–188. doi: 10.3109/02699052.2013.860475
Dahdah, M. N., Bennett, M., Prajapati, P., Parsons, T. D., Sullivan, E., and Driver, S. (2017). Application of virtual environments in a multi-disciplinary day neurorehabilitation program to improve executive functioning using the Stroop task. NeuroRehabilitation 41, 721–734. doi: 10.3233/nre-172183
Dall'Acqua, P., Johannes, S., Mica, L., Simmen, H.-P., Glaab, R., Fandino, J., et al. (2017). Prefrontal cortical thickening after mild traumatic brain injury: A one-year magnetic resonance imaging study. J. Neurotrauma 34, 3270–3279. doi: 10.1089/neu.2017.5124
Dams-O'Connor, K., Ketchum, J. M., Cuthbert, J. P., Corrigan, J. D., Hammond, F. M., Haarbauer-Krupa, J., et al. (2020). Functional outcome trajectories following inpatient rehabilitation for TBI in the United States: A NIDILRR TBIMS and CDC Interagency Collaboration. J. Head Trauma Rehabil. 35, 127–139. doi: 10.1097/HTR.0000000000000484
de Guise, E., Alturki, A. Y., LeBlanc, J., Champoux, M.-C., Couturier, C., Lamoureux, J., et al. (2013). The Montreal Cognitive Assessment in persons with traumatic brain injury. Appl. Neuropsychol. 21, 128–135. doi: 10.1080/09084282.2013.778260
de Guise, E., Gosselin, N., LeBlanc, J., Champoux, M.-C., Couturier, C., Lamoureux, J., et al. (2011). Clock drawing and mini-mental state examination in patients with traumatic brain injury. Appl. Neuropsychol. 18, 179–190. doi: 10.1080/09084282.2011.595444
Devi, Y., Khan, S., Rana, P., and Dhandapani, M. (2020). Cognitive, behavioral, and functional impairments among traumatic brain injury survivors: impact on caregiver burden. J. Neurosci. Rural Pract. 11, 629–635. doi: 10.1055/s-0040-1716777
Dhaliwal, S. K., Meek, B. P., and Modirrousta, M. M. (2015). Non-invasive brain stimulation for the treatment of symptoms following traumatic brain injury. Front. Psychiatry 6:119. doi: 10.3389/fpsyt.2015.00119
Di Battista, A., Godfrey, C., Soo, C., Catroppa, C., and Anderson, V. (2014). Depression and health related quality of life in adolescent survivors of a traumatic brain injury: a pilot study. PLoS ONE 9:e0101842. doi: 10.1371/journal.pone.0101842
Donders, J., Forness, K., Anderson, L. B., Gillis, J. R., and Benedict, R. H. B. (2022). Performance on, and correlates of, the Brief Visuospatial Memory Test—Revised after traumatic brain injury. J. Clin. Exp. Neuropsychol. 44, 42–49. doi: 10.1080/13803395.2022.2069231
Douglas, D. B., Iv, M., Douglas, P. K., Anderson, A., Vos, S. B., Bammer, R., et al. (2015). Diffusion tensor imaging of TBI potentials and challenges. Top. Magn. Reson. Imaging 24, 241–251. doi: 10.1097/RMR.0000000000000062
Dvorkin, A. Y., Ramaiya, M., Larson, E. B., Zollman, F. S., Hsu, N., Pacini, S., et al. (2013). A “virtually minimal” visuo-haptic training of attention in severe traumatic brain injury. J. Neuroeng. Rehabil. 10:92. doi: 10.1186/1743-0003-10-92
Dymowski, A. R., Owens, J. A., Ponsford, J. L., and Willmott, C. (2015). Speed of processing and strategic control of attention after traumatic brain injury. J. Clin. Exp. Neuropsychol. 37, 1024–1035. doi: 10.1080/13803395.2015.1074663
Ferraracci, J., Anzalone, C., Bridges, R. M., Moore, R. D., and Decker, S. L. (2021). QEEG correlates of cognitive processing speed in children and adolescents with traumatic brain injuries. Applied Neuropsychology: Child 10, 247–257. doi: 10.1080/21622965.2019.1675523
French, L., Taber, K., Hemlock, K., Hurley, R., and Warden, D. (2015). “Traumatic brain injury in the military population,” in Combat and Operational Behavioral Health (Government Printing Office, Illustrated edition), 225–242.
Gan, S., Shi, W., Wang, S., Sun, Y., Yin, B., Bai, G., et al. (2021). Accelerated brain aging in mild traumatic brain injury: longitudinal pattern recognition with white matter integrity. J. Neurotrauma 38, 2549–2559. doi: 10.1089/neu.2020.7551
Gillis, M. M., and Hampstead, B. M. (2015). A two-part preliminary investigation of encoding related activation changes after moderate to severe traumatic brain injury: hyperactivation, repetition suppression, and the role of the prefrontal cortex. Brain Imaging Behav. 9, 801–820. doi: 10.1007/s11682-014-9337-5
Gómez-de-Regil, L. (2020). Assessment of executive function in patients with traumatic brain injury with the wisconsin card-sorting test. Brain Sci. 10:699. doi: 10.3390/brainsci10100699
Gorman, S., Barnes, M. A., Swank, P. R., Prasad, M., Cox, C. S. Jr., and Ewing-Cobbs, L. (2016). Does processing speed mediate the effect of pediatric traumatic brain injury on working memory? Neuropsychology 30, 263–273. doi: 10.1037/neu0000214
Gosset, A., Wagman, H., Pavel, D., Cohen, P., Tarzwell, R., Bruin, S., et al. (2022). Using single-photon emission computerized tomography on patients with positive quantitative electroencephalogram to evaluate chronic mild traumatic brain injury with persistent symptoms. Front. Neurol. 13:704844. doi: 10.3389/fneur.2022.704844
Govindarajan, K. A., Narayana, P. A., Hasan, K. M., Wilde, E. A., Levin, H. S., Hunter, J. V., et al. (2016). Cortical thickness in mild traumatic brain injury. J. Neurotrauma 33:4253. doi: 10.1089/neu.2015.4253
Gutiérrez-Ruiz, K., Audivett, D. L., and Mosquera-Valoy, Y. (2022). Health-related quality of life, neuropsychological functions and emotional state between children and adolescents with mild traumatic brain injury and healthy controls. Rev. Psicopatol. Psicol. Clín. 27, 13–24. doi: 10.5944/rppc.27891
Hagen, C., Malkmus, D., and Durham, P. (1972). Levels of Cognitive Functioning. Downey, CA: Rancho Los Amigos Hospital.
Harch, P. G., Andrews, S. R., Fogarty, E. F., Amen, D., Pezzullo, J. C., and Lucarini, J. (2012). A phase I study of low pressure hyperbaric oxygen therapy for blast-induced post-concussion syndrome and post traumatic stress disorder. J. Neurotrauma 29, 168–185. doi: 10.1089/neu.2011.1895
Henry, J., and Crawford, J. (2004). A meta-analytic review of verbal fluency performance in patients with traumatic brain injury. Neuropsychology 18, 621–628. doi: 10.1037/0894-4105.18.4.621
Hossain, I., Mohammadian, M., Takala, R. S. K., Tenovuo, O., Azurmendi Gil, L., Frantzén, J., et al. (2020). Admission levels of total tau and β-amyloid isoforms 1-40 and 1-42 in predicting the outcome of mild traumatic brain injury. Front. Neurol. 11:325. doi: 10.3389/fneur.2020.00325
Huang, C. X., Li, Y. H., Lu, W., Huang, S. H., Li, M. J., Xiao, L. Z., et al. (2022). Positron emission tomography imaging for the assessment of mild traumatic brain injury and chronic traumatic encephalopathy: recent advances in radiotracers. Neural Regen. Res. 17, 74–81. doi: 10.4103/1673-5374.314285
Hume, C., Mitra, B., Wright, B., and Kinsella, G. J. (2023). Cognitive performance in older people after mild traumatic brain injury: trauma effects and other risk factors. J. Int. Neuropsychol. Soc. 29, 651–661. doi: 10.1017/S1355617722000674
Jacobs, M. L., and Donders, J. (2007). Criterion validity of the California Verbal Learning Test Second Edition (CVLT-II) after traumatic brain injury. Arch. Clin. Neuropsychol. 22, 143–149. doi: 10.1016/j.acn.2006.12.002
Jang, H., Kwon, H., Yang, J. J., and Lee, J. M. (2017). Correlations between grey matter and white matter degeneration in pure alzheimer's disease, pure subcortical vascular dementia, and mixed dementia. Sci. Rep. 7:9541. doi: 10.1038/s41598-017-10074-x
Jeyaraj, P. (2019). Frontal bone fractures and frontal sinus injuries: treatment paradigms. Ann. Maxillofac. Surg. 9, 261–282. doi: 10.4103/ams.ams_151_19
Johnson, V. E., Stewart, J. E., Begbie, F. D., Trojanowski, J. Q., Smith, D. H., and Stewart, W. (2013). Inflammation and white matter degeneration persist for years after a single traumatic brain injury. Brain 136, 28–42. doi: 10.1093/brain/aws322
Jones, K. M., Barker-Collo, S., Parmar, P., Starkey, N., Theadom, A., Ameratunga, S., et al. (2018). Trajectories in health recovery in the 12 months following a mild traumatic brain injury in children: Findings from the bionic study. J. Prim. Health Care 10:81. doi: 10.1071/hc17038
Joseph, A.-L. C., Lippa, S. M., McNally, S. M., Garcia, K. M., Leary, J. B., Dsurney, J., et al. (2019). Estimating premorbid intelligence in persons with traumatic brain injury: an examination of the Test of Premorbid Functioning. Appl. Neuropsychol. 28, 535–543. doi: 10.1080/23279095.2019.1661247
Kaur, P., and Sharma, S. (2018). Recent advances in pathophysiology of traumatic brain injury. Curr. Neuropharmacol. 16, 1224–1238. doi: 10.2174/1570159x15666170613083606
Keenan, H. T., Clark, A. E., Holubkov, R., Cox, C. S., and Ewing-Cobbs, L. (2021). Trajectories of children's executive function after traumatic brain injury. JAMA Netw. Open 4:2624. doi: 10.1001/jamanetworkopen.2021.2624
Kennedy, L., Nuno, M., Gurkoff, G. G., Nosova, K., and Zwienenberg, M. (2022). Moderate and severe TBI in children and adolescents: the effects of age, sex, and injury severity on patient outcome 6 months after injury. Front. Neurol. 13:41717. doi: 10.3389/fneur.2022.741717
Krynicki, C. R., Jones, C. A., and Hacker, D. A. (2023). A meta-analytic review examining the validity of executive functioning tests to predict functional outcomes in individuals with a traumatic brain injury. Appl. Neuropsychol. 1–18. doi: 10.1080/23279095.2023.2225666
Laccarino, C., Carretta, A., Nicolosi, F., and Morselli, C. (2018). Epidemiology of severe traumatic brain injury. J Neurosurgeon Sci. 62, 525–541. doi: 10.23736/S0390-5616.18.04532-0
Lagerstedt, L., Azurmendi, L., Tenovuo, O., Katila, A. J., Takala, R. S. K., Blennow, K., et al. (2020). Interleukin 10 and heart fatty acid-binding protein as early outcome predictors in patients with traumatic brain injury. Front. Neurol. 11:376. doi: 10.3389/fneur.2020.00376
Lamontagne, G., Belleville, G., Beaulieu-Bonneau, S., Souesme, G., Savard, J., Sirois, M.-J., et al. (2022). Anxiety symptoms and disorders in the first year after sustaining mild traumatic brain injury. Rehabil. Psychol. 67, 90–99. doi: 10.1037/rep0000422
Lee, H., Yang, Y., Xu, J., Ware, J. B., and Liu, B. (2021). Use of magnetic resonance imaging in acute traumatic brain injury patients is associated with lower inpatient mortality. J. Clin. Imaging Sci. 11:53. doi: 10.25259/JCIS_148_2021
Lehman, B. J., David, D., and Gruber, J. A. (2017). Rethinking the biopsychosocial model of health: Understanding health as a dynamic system. Soc. Personal. Psychol. Compass 11:12328. doi: 10.1111/spc3.12328
Li, C., Hua, R., Gao, D., Zheng, F., and Xie, W. (2023). Cognitive decline before and during COVID-19 pandemic among older people with multimorbidity: a longitudinal study. J. Post Acute Long Term Care Med. doi: 10.1016/j.jamda.2023.01.001
Li, F., Lu, L., Li, H., Liu, Y., Chen, H., Yuan, F., et al. (2023). Disrupted resting-state functional connectivity and network topology in mild traumatic brain injury: an arterial spin labelling study. Brain Commun. 5:fcad254. doi: 10.1093/braincomms/fcad254
Li, F., Lu, L., Shang, S., Hu, L., Chen, H., Wang, P., et al. (2020). Disrupted functional network connectivity predicts cognitive impairment after acute mild traumatic brain injury. CNS Neurosci. Ther. 26, 1083–1091. doi: 10.1111/cns.13430
Li, X., Jia, X., Liu, Y., Bai, G., Pan, Y., Ji, Q., et al. (2023). Brain dynamics in triple-network interactions and its relation to multiple cognitive impairments in mild traumatic brain injury. Cereb. Cort. 33, 6620–6632. doi: 10.1093/cercor/bhac529
Linden, C. V., Verhelst, H., Verleysen, G., Caeyenberghs, K., Deblaere, K., and Vingerhoets, G. (2019). Prefrontal and temporal cortical thickness in adolescents with traumatic brain injury. Dev. Med. Child Neurol. 61, 672–679. doi: 10.1111/dmcn.14100
Lindsey, H. M., Lazar, M., Mercuri, G., Rath, J. F., Bushnik, T., Flanagan, S., et al. (2022). The effects of plasticity-based cognitive rehabilitation on resting-state functional connectivity in Chronic Traumatic Brain Injury: a pilot study. NeuroRehabilitation 51, 133–150. doi: 10.3233/nre-210264
List, J., Ott, S., Bukowski, M., Lindenberg, R., and Flöel, A. (2015). Cognitive function and brain structure after recurrent mild traumatic brain injuries in young-to-middle-aged adults. Front. Human Neurosci. 9:228. doi: 10.3389/fnhum.2015.00228
Liu, H., Zhang, G., Zheng, H., Tan, H., Zhuang, J., Li, W., et al. (2024). Dynamic dysregulation of the triple network of the brain in mild traumatic brain injury and its relationship with cognitive performance. J. Neurotrauma 41, 879–886. doi: 10.1089/neu.2022.0257
Liu, Y., Wu, W., Chen, X., Wu, M., Hu, G., Zhou, G., et al. (2020). Aberrant correlation between the default mode and salience networks in mild traumatic brain injury. Front. Comput. Neurosci. 14:68. doi: 10.3389/fncom.2020.00068
Lolli, V., Pezzullo, M., Delpierre, I., and Sadeghi, N. (2016). MDCT imaging of traumatic brain injury. Br. J. Radiol. 89:20150849. doi: 10.1259/bjr.20150849
Loring, D. W., and Larrabee, G. J. (2006). Sensitivity of the Halstead and Wechsler Test Batteries to brain damage: evidence from Reitan's original validation sample. Clin. Neuropsychol. 20, 221–229. doi: 10.1080/13854040590947443
Lv, L.-Q., Hou, L.-J., Yu, M.-K., Ding, X.-H., Qi, X.-Q., and Lu, Y.-C. (2011). Hyperbaric oxygen therapy in the management of paroxysmal sympathetic hyperactivity after severe traumatic brain injury: a report of 6 cases. Arch. Phys. Med. Rehabil. 92, 1515–1518. doi: 10.1016/j.apmr.2011.01.014
Ma, J., Zhang, K., Wang, Z., and Chen, G. (2016). Progress of research on diffuse axonal injury after traumatic brain injury. Neural Plast. 2016:9746313. doi: 10.1155/2016/9746313
Maas, A. I., Menon, D. K., Manley, G. T., Abrams, M., Åkerlund, C., Andelic, N., et al. (2022). Traumatic brain injury: progress and challenges in prevention, Clinical Care, and research. Lancet Neurol. 21, 1004–1060. doi: 10.1016/s1474-4422(22)00309-x
Madhavan, R., Joel, S. E., Mullick, R., Cogsil, T., Niogi, S. N., Tsiouris, A. J., et al. (2019). Longitudinal resting state functional connectivity predicts clinical outcome in mild traumatic brain injury. J. Neurotrauma 36, 650–660. doi: 10.1089/neu.2018.5739
Maiden, M. J., Cameron, P. A., Rosenfeld, J. V., Cooper, D. J., McLellan, S., and Gabbe, B. J. (2020). Long-term outcomes after severe traumatic brain injury in older adults. A registry-based Cohort Study. Am. J. Respir. Crit. Care Med. 201, 167–177. doi: 10.1164/rccm.201903-0673oc
Mckee, A., and Daneshvar, D. (2015). The neuropathology of traumatic brain injury. Hands Clin. Neurol. 127, 45–66. doi: 10.1016/B978-0-444-52892-6.00004-0
McMahon, P. J., Hricik, A., Yue, J. K., Puccio, A. M., Inoue, T., Lingsma, H. F., et al. (2014). Symptomatology and functional outcome in mild traumatic brain injury: results from the prospective track-tbi study. J. Neurotrauma 31, 26–33. doi: 10.1089/neu.2013.2984
Meng, X. E., Zhang, Y., Li, N., Fan, D. F., Yang, C., Li, H., et al. (2016). Effects of hyperbaric oxygen on the Nrf2 signaling pathway in secondary injury following traumatic brain injury. Genet. Mol. Res.15:16933. doi: 10.4238/gmr.15016933
Milosevic, M., Nakanishi, T., Sasaki, A., Yamaguchi, A., Nomura, T., Popovic, M. R., et al. (2021). Cortical re-organization after traumatic brain injury elicited using functional electrical stimulation therapy: a case report. Front. Neurosci. 15:693861. doi: 10.3389/fnins.2021.693861
Mishra, K., Purohit, D., and Sharma, S. (2020). Montreal Cognitive Assessment Score: A screening tool for cognitive function in traumatic brain injury (TBI) population. J. Neurol. Med. 5, 35–39. doi: 10.29245/2572.942x/2020/3.1238
Mulligan, T., Barker-Collo, S., Gibson, K., and Jones, K. (2021). You only get one brain: Adult reflections on the long-term impacts of traumatic brain injury in adolescence. Brain Impair.23, 278–295. doi: 10.1017/brimp.2021.22
Nadel, J., McNally, J. S., DiGiorgio, A., and Grandhi, R. (2021). Emerging utility of applied magnetic resonance imaging in the management of traumatic brain injury. Med. Sci. 9:10. doi: 10.3390/medsci9010010
National Academies of Sciences, Engineering, and Medicine. (2022). Traumatic Brain Injury: A Roadmap for Accelerating Progress. Washington, DC: The National Academies Press.
Nelson, L. D., Kramer, M. D., Joyner, K. J., Patrick, C. J., Stein, M. B., Temkin, N., et al. (2021). Relationship between transdiagnostic dimensions of psychopathology and traumatic brain injury (TBI): A TRACK-TBI study. J. Abnorm. Psychol. 130, 423–434. doi: 10.1037/abn0000672
Neville, I. S., Hayashi, C. Y., El Hajj, S. A., Zaninotto, A. L., Sabino, J. P., Sousa, L. M., et al. (2015). Repetitive transcranial magnetic stimulation (rtms) for the cognitive rehabilitation of Traumatic Brain Injury (TBI) victims: Study protocol for a randomized controlled trial. Trials 16. doi: 10.1186/s13063-015-0944-2
Ng, S., and Lee, A. (2019). Traumatic brain injuries: pathophysiology and potential therapeutic targets. Front. Cell. Neurosci. 13:528. doi: 10.3389//fncel.2019.00528
Othman, A., Idris, Z., Rosman, A. K., Abdullah, J. M., Ghan, I. A. R. I., and Zakaria, A. Z. (2022). Cognitive impairment and neuropsychiatry manifestation following mild and moderate traumatic brain injury at 3 months and 6 months. Malays. J. Med. Sci. 29, 48–58. doi: 10.21315/mjms2022.29.5.6
Palmer, G. A., and Palmer, D. G. (2021). Anxiety screening in polytrauma patients by use of single-item reporting with the neurobehavioral symptom inventory: how brief is too brief? Psychol. Rep. 125, 2922–2935. doi: 10.1177/00332941211031789
Peng, L., and Bonaguidi, M. A. (2018). Function and dysfunction of adult hippocampal neurogenesis in regeneration and disease. Am. J. Pathol. 188, 23–28. doi: 10.1016/j.ajpath.2017.09.004
Ponsford, J., Bayley, M., Wiseman-Hakes, C., Togher, L., Velikonja, D., McIntyre, A., et al. (2014). Incog recommendations for management of cognition following traumatic brain injury, part II. J. Head Trauma Rehabil. 29, 321–337. doi: 10.1097/htr.0000000000000072
Popescu, C., Anghelescu, A., Daia, C., and Onose, G. (2015). Actual data on epidemiological evolution and prevention endeavours regarding traumatic brain injury. J. Med. Life 8, 272–277.
Power, S. P., Moloney, F., Twomey, M., James, K., O'Connor, O. J., and Maher, M. M. (2016). Computed tomography and patient risk: facts, perceptions and uncertainties. World J. Radiol. 8, 902–915. doi: 10.4329/wjr.v8.i12.902
Prakash, A., Parelkar, S. V., Oak, S. N., Gupta, R. K., Sanghvi, B. V., Bachani, M., et al. (2012). Role of hyperbaric oxygen therapy in severe head injury in children. J. Pediatr. Neurosci. 7:4. doi: 10.4103/1817-1745.97610
Prasad, G. L., Anmol, N., and Menon, G. R. (2018). Outcome of traumatic brain injury in the elderly population: a tertiary center experience in a developing country. World Neurosurg. 111:34. doi: 10.1016/j.wneu.2017.12.034
Rabinowitz, A. R., Hart, T., Whyte, J., and Kim, J. (2018). Neuropsychological recovery trajectories in moderate to severe traumatic brain injury: Influence of patient characteristics and diffuse axonal injury — Erratum. J. Int. Neuropsychol. Soc. 25:998. doi: 10.1017/s1355617718000346
Rabinowitz, A. R., and Levin, H. (2014). Cognitive sequelae of traumatic brain injury. Psychiatr Chin North Am. doi: 10.1016/j.psc.2013.11.004
Rassovsky, Y., Levi, Y., Agranov, E., Sela-Kaufman, M., Sverdlik, A., and Vakil, E. (2015). Predicting long-term outcome following traumatic brain injury (TBI). J. Clin. Exp. Neuropsychol. 37, 354–366. doi: 10.1080/13803395.2015.1015498
Rauchman, S., Albert, J., Pinkhasov, A., and Reiss, A. (2022). Mild-to-moderate traumatic brain injury: a review with focus on the visual system. Neural Int. 14, 453–470. doi: 10.3390/neurolint14020038
Recla, M., Bardoni, A., Galbiati, S., Pastore, V., Dominici, C., Tavano, A., et al. (2013). Cognitive and adaptive functioning after severe TBI in school-aged children. Brain Inj. 27, 862–871. doi: 10.3109/02699052.2013.775499
Rigon, A., Duff, M. C., McAuley, E., Kramer, A. F., and Voss, M. W. (2016). Is traumatic brain injury associated with reduced inter-hemispheric functional connectivity? A study of large scale resting state networks following traumatic brain injury. J. Neurotrauma 33, 977–989. doi: 10.1089/neu.2014.3847
Rivara, F. P., Koepsell, T. D., Wang, J., Temkin, N., Dorsch, A., Vavilala, M. S., et al. (2011). Disability 3, 12, and 24 months after traumatic brain injury among children and adolescents. Pediatrics 128, e1129–e1138. doi: 10.1542/peds.2011-0840
Roozenbeek, B., Maas, A. I., and Menon, D. K. (2013). Changing patterns in the epidemiology of Traumatic Brain Injury. Nat. Rev. Neurol. 9, 231–236. doi: 10.1038/nrneurol.2013.22
Rosenberg, H., Dethier, M., Kessels, R. P., Westbrook, R. F., and McDonald, S. (2015). Emotion perception after moderate–severe traumatic brain injury: the valence effect and the role of working memory, processing speed, and nonverbal reasoning. Neuropsychology 29, 509–521. doi: 10.1037/neu0000171
Rushby, J., McDonald, S., Randall, R., Sousa, A., Trimmer, E., and Fisher, A. (2013). Impaired emotional contagion following severe traumatic brain injury. Int. J. Psychophysiol. 89, 466–474. doi: 10.1016/j.ijpsycho.2013.06.013
Ryan, N. P., Bijnen, L., Catroppa, C., Beauchamp, M. H., Crossley, L., Hearps, S., et al. (2015a). Longitudinal outcome and recovery of social problems after Pediatric Traumatic Brain Injury (TBI): Contribution of Brain Insult and family environment. Int. J. Dev. Neurosci. 49, 23–30. doi: 10.1016/j.ijdevneu.2015.12.004
Ryan, N. P., Catroppa, C., Beare, R., Coleman, L., Ditchfield, M., Crossley, L., et al. (2015b). Predictors of longitudinal outcome and recovery of pragmatic language and its relation to externalizing behaviour after pediatric traumatic brain injury. Brain Lang. 142, 86–95. doi: 10.1016/j.bandl.2015.01.007
Sariaslan, A., Sharp, D. J., D'Onofrio, B. M., Larsson, H., and Fazel, S. (2016). Long-term outcomes associated with traumatic brain injury in childhood and adolescence: a nationwide Swedish cohort study of a wide range of medical and social outcomes. PLoS Med. 13:e1002103. doi: 10.1371/journal.pmed.1002103
Scheibel, R. S. (2017). Functional magnetic resonance imaging of cognitive control following traumatic brain injury. Front. Neurol. 8:352. doi: 10.3389/fneur.2017.00352
Scholten, A. C., Haagsma, J. A., Andriessen, T. M. J. C., Vos, P. E., Steyerberg, E. W., van Beeck, E. F., et al. (2015). Health-related quality of life after mild, moderate and severe traumatic brain injury: patterns and predictors of suboptimal functioning during the first year after injury. Injury 46, 616–624. doi: 10.1016/j.injury.2014.10.064
Schroeder, R. W., Baade, L. E., Peck, C. P., VonDran, E. J., Brockman, C. J., et al. (2012). Validation of MMPI-2-RF validity scales in criterion group neuropsychological samples. Clin. Neuropsychol. 26, 129–146. doi: 10.1080/13854046.2011.639314
Schweitzer, A. D., Niogi, S. N., Whitlow, C. T., and Tsiouris, A. J. (2019). Traumatic brain injury: imaging patterns and complications. Radiographics 39, 1571–1595. doi: 10.1148/rg.2019190076
Shields, C., Ownsworth, T., O'Donovan, A., and Fleming, J. (2015). A transdiagnostic investigation of emotional distress after traumatic brain injury. Neuropsychol. Rehabil. 26, 410–445. doi: 10.1080/09602011.2015.1037772
Stein, M. B., Yuh, E., Jain, S., Okonkwo, D. O., Mac Donald, C. L., Levin, H., et al. (2021). Smaller regional brain volumes predict posttraumatic stress disorder at 3 months after mild traumatic brain injury. Biol. Psychiatry Cogn. Neurosci. Neuroimaging 6, 352–359. doi: 10.1016/j.bpsc.2020.10.008
Stenberg, M., Stålnacke, B.-M., and Saveman, B.-I. (2022). Health and well-being of persons of working age up to seven years after severe traumatic brain injury in northern Sweden: A mixed method study. J. Clin. Med. 11:1306. doi: 10.3390/jcm11051306
Stubberud, J., Lovstad, M., Solbakk, A., Schanke, A., and Tornas, S. (2020). Emotional regulation following acquired brain injury: associations with executive functioning in daily life and symptoms of anxiety and depression. Front. Neurol. 11:1011. doi: 10.3389/fneur.2020.01011
Sweeney, J. E., and Johnson, A. M. (2017). Halstead-Reitan characteristics of nonimpact and impact mTBI litigants and insurance claimants. Appl. Neuropsychol. 26, 65–75. doi: 10.1080/23279095.2017.1359783
Tate, R., Kennedy, M., Ponsford, J., Douglas, J., Velikonja, D., Bayley, M., et al. (2014). Incog recommendations for management of cognition following traumatic brain injury, part III. J. Head Trauma Rehabil. 29, 338–352. doi: 10.1097/htr.0000000000000068
Thaler, N. S., Allen, D. N., Hart, J. S., Boucher, J. R., McMurray, J. C., and Mayfield, J. (2013). Neurocognitive correlates of the trail making test for older children in patients with traumatic brain injury. Arch. Clin. Neuropsychol. 27, 446–452. doi: 10.1093/arclin/acs042
Thompson, H. J., Rivara, F. P., and Wang, J. (2020). Effect of age on longitudinal changes in symptoms, function, and outcome in the first year after mild-moderate traumatic brain injury. J. Neurosci. Nurs. 52, 46–52. doi: 10.1097/JNN.0000000000000498
Togher, L., Wiseman-Hakes, C., Douglas, J., Stergiou-Kita, M., Ponsford, J., Teasell, R., et al. (2014). Incog recommendations for management of cognition following traumatic brain injury, part IV. J. Head Trauma Rehabil. 29, 353–368. doi: 10.1097/htr.0000000000000071
Tomar, S., Sharma, A., Jain, A., Sinha, V., and Gupta, I. (2018). Study of fatigue and associated factors in traumatic brain injury and its correlation with insomnia and depression. As. J. Neurosurg. doi: 10.4103/ajns.AJNS_89_17
Toth, L., Czigler, A., Horvath, P., Szarka, N., Kornyei, B., Toth, A., et al. (2021). The effect of mild traumatic brain injury on cerebral microbleeds in aging. Front. Aging Neurosci. 13:717391. doi: 10.3389/fnagi.2021.717391
Treble-Barna, A., Zang, H., Zhang, N., Taylor, H. G., Yeates, K. O., and Wade, S. (2017). Long term neuropsychological profiles and their role as mediators of adaptive functioning after traumatic brain injury in early childhood. J. Neurotrauma 34, 353–362. doi: 10.1089/neu.2016.4476
Tsai, Y.-C., Liu, C.-J., Huang, H.-C., Lin, J.-H., Chen, P.-Y., Su, Y.-K., et al. (2021). A meta-analysis of dynamic prevalence of cognitive deficits in the acute, subacute, and chronic phases after traumatic brain injury. J. Neurosci. Nurs. 53, 63–68. doi: 10.1097/JNN.0000000000000570
Ubukata, S., Ueda, K., Fujimoto, G., Ueno, S., Murai, T., and Oishi, N. (2021). Extracting apathy from depression syndrome in traumatic brain injury by using a clustering method. J. Neuropsychiatry Clin. Neurosci. 34, 158–167. doi: 10.1176/appi.neuropsych.21020046
Utomo, W. K., Gabbe, B. J., Simpson, P. M., and Cameron, P. A. (2009). Predictors of in-hospital mortality and 6-month functional outcomes in older adults after moderate to severe traumatic brain injury. Injury 40, 973–977. doi: 10.1016/j.injury.2009.05.034
van der Horn, H. J., Liemburg, E. J., Aleman, A., Spikman, J. M., and van der Naalt, J. (2015). Brain networks subserving emotion regulation and adaptation after mild traumatic brain injury. J. Neurotrauma 33, 1–9. doi: 10.1089/neu.2015.3905
van der Vlegel, M., Polinder, S., Toet, H., Panneman, M. J. M., and Haagsma, J. A. (2021). Prevalence of post-concussion-like symptoms in the general injury population and the association with health-related quality of life, health care use, and return to work. J. Clin. Med. 10:806. doi: 10.3390/jcm10040806
Vedung, F., Fahlström, M., Wall, A., Antoni, G., Lubberink, M., Johansson, J., et al. (2022). Chronic cerebral blood flow alterations in traumatic brain injury and sports-related concussions. Brain Inj. 36, 948–960. doi: 10.1080/02699052.2022.2109746
Velikonja, D., Tate, R., Ponsford, J., McIntyre, A., Janzen, S., and Bayley, M. (2014). INCOG recommendations for management of cognition following traumatic brain injury, part V. J. Head Trauma Rehabil. 29, 369–386. doi: 10.1097/htr.0000000000000069
Vieira, R., Paiva, W., Oliveira, D., Teixeira, M., Andrade, A., and Sousa, R. (2016). Diffuse axonal injury: epidemiology, outcome and associated risk factors. Front. Neurol. 7:178doi: 10.3389/fneur.2016.00178
Visser, E., Den Oudsten, B. L., Traa, M. J., Gosens, T., and De Vries, J. (2021). Patients' experiences and wellbeing after injury: A Focus Group Study. PLoS ONE 16:e024. doi: 10.1371/journal.pone.024
Wade, S. L., Zhang, N., Yeates, K. O., Stancin, T., and Taylor, H. G. (2016). Social environmental moderators of long-term functional outcomes of early childhood brain injury. JAMA Pediatr. 170:343. doi: 10.1001/jamapediatrics.2015.4485
Walker, W. C., Stromberg, K. A., Marwitz, J. H., Sima, A. P., Agyemang, A. A., Graham, K. M., et al. (2018). predicting long-term global outcome after traumatic brain injury: development of a practical prognostic tool using the traumatic brain injury model systems national database. J. Neurotrauma 35, 1587–1595. doi: 10.1089/neu.2017.5359
Wang, T., Hu, Y., Wang, D., Liu, J., Sun, J., Wei, C., et al. (2021). Arcuate fasciculus subsegment impairments distinctly associated with memory and language deficits in acute mild traumatic brain injury patients. J. Neurotrauma 38, 3279–3287. doi: 10.1089/neu.2021.0267
Whiteneck, G. G., Eagye, C. B., Cuthbert, J. P., Corrigan, J. D., Bell, J. M., Haarbauer-Krupa, J. K., et al. (2018). One and Five Year Outcomes After Moderate-to-Severe Traumatic Brain Injury Requiring Inpatient Rehabilitation : Traumatic Brain Injury Report. Centers for Disease Control and Prevention (U.S.); National Center for Injury Prevention and Control (U.S.); United States. Administration for Community Living; National Institute on Disability, Independent Living and Rehabilitation Research (U.S.). Available at: https://stacks.cdc.gov/view/cdc/59524
Wilkins, T. E., Beers, S. R., Borrasso, A. J., Brooks, J., Mesley, M., Puffer, R., et al. (2019). Favorable functional recovery in severe traumatic brain injury survivors beyond six months. J. Neurotrauma 36, 3158–3163. doi: 10.1089/neu.2018.6153
Williams, C. N., McEvoy, C. T., Lim, M. M., Shea, S. A., Kumar, V., Nagarajan, D., et al. (2022). Sleep and executive functioning in pediatric traumatic brain injury survivors after critical care. Children 9:748. doi: 10.3390/children9050748
Witowski, E., Gao, Y., Gavsyuk, A., Maor, I., DeWatt, G., Eldred, W., et al. (2019). Rapid changes in synaptic strength after mild traumatic brain injury. Front. Cell. Neurosci. 13:166. doi: 10.3389/fncel.2019.00166
Yeates, K., Levin, H., and Ponsford, J. (2017). The neuropsychology of traumatic brain injury: looking back, peering ahead. J. Int. Neuropsychol. Soc. 23, 806–817. doi: 10.1017/S1355617717000686
Yin, B., Li, D. D., Huang, H., Gu, C. H., Bai, G. H., Hu, L. X., et al. (2019). Longitudinal changes in diffusion tensor imaging following mild traumatic brain injury and correlation with outcome. Front. Neural Circuits 13:28. doi: 10.3389/fncir.2019.00028
Zane, K., Gfeller, J., Roskos, P., and Bucholz, R. (2016). The clinical utility of the conners' continuous performance test-II in traumatic brain injury. Arch. Clin. Neuropsychol. doi: 10.1093/arclin/acw078
Keywords: traumatic brain injury (TBI), neuropsychology, review, assessment, head injury, trauma, neuroscience, psychology
Citation: Chan A, Ouyang J, Nguyen K, Jones A, Basso S and Karasik R (2024) Traumatic brain injuries: a neuropsychological review. Front. Behav. Neurosci. 18:1326115. doi: 10.3389/fnbeh.2024.1326115
Received: 22 October 2023; Accepted: 20 September 2024;
Published: 08 October 2024.
Edited by:
Patricio Huerta, Feinstein Institute for Medical Research, United StatesReviewed by:
Andrew P. Lavender, Federation University Australia, AustraliaMargit Jehna, Medical University of Graz, Austria
Copyright © 2024 Chan, Ouyang, Nguyen, Jones, Basso and Karasik. This is an open-access article distributed under the terms of the Creative Commons Attribution License (CC BY). The use, distribution or reproduction in other forums is permitted, provided the original author(s) and the copyright owner(s) are credited and that the original publication in this journal is cited, in accordance with accepted academic practice. No use, distribution or reproduction is permitted which does not comply with these terms.
*Correspondence: Aldrich Chan, YWxkcmljaCYjeDAwMDQwO2RyY2hhbmNuYy5jb20=