- 1Department of Psychology, University of Maryland, College Park, MD, United States
- 2Institute for Innovation and Implementation, University of Maryland School of Social Work, Baltimore, MD, United States
- 3Maryland Health Care Commission, Baltimore, MD, United States
- 4Department of Pediatrics, University of Maryland School of Medicine, Baltimore, MD, United States
- 5RTI International, Research Triangle Part, Durham, NC, United States
Early adversities, including prenatal drug exposure (PDE) and a negative postnatal emotional caregiving environment, impact children’s long-term development. The protracted developmental course of memory and its underlying neural systems offer a valuable framework for understanding the longitudinal associations of pre- and postnatal factors on children with PDE. This study longitudinally examines memory and hippocampal development in 69 parent–child dyads to investigate how the early caregiving emotional environment affects children with PDE’s neural and cognitive systems. Measures of physical health, drug exposure, caregiver stress, depression, and distress were collected between 0 and 24 months At age 14 years, adolescents completed multiple measures of episodic memory, and at ages 14 and 18 years, adolescents underwent magnetic resonance imaging (MRI) scans. Latent constructs of episodic memory and the caregiving environment were created using Confirmatory Factor Analysis. Multiple regressions revealed a negative emotional caregiving environment during infancy was associated with poor memory performance and smaller left hippocampal volumes at 14 years. Better memory performance at 14 years predicted larger right hippocampal volume at 18 years. At 18 years, the association between the emotional caregiving environment and hippocampal volume was moderated by sex, such that a negative emotional caregiving environment was associated with larger left hippocampal volumes in males but not females. Findings suggest that the postnatal caregiving environment may modulate the effects of PDE across development, influencing neurocognitive development.
Introduction
Drug use during pregnancy is a serious and increasing health problem with risks for both the mother and the unborn child (Richardson and Day, 2018; SAMHSA, 2021). Drug use has risen progressively among pregnant people in the United States, with approximately 60% of pregnant mothers reporting prescription drug use and 7.7% reporting illicit substance use during pregnancy (Traccis et al., 2020; SAMHSA, 2021).
Prenatal drug exposure (PDE, specified here as exposure to cocaine and/or heroin, commonly along with the use of other substances) is associated with adverse developmental outcomes across multiple domains (Morey et al., 2009; Buckingham-Howes et al., 2013). However, longitudinal studies of the impact of PDE on cognitive development remain limited, have shown mixed associations as children age, and have inconsistently evaluated the relative impact of the caregiving context (Ackerman et al., 2010; Riggins et al., 2012; Conradt et al., 2019). A recent systematic literature review found 27 studies on the cognitive outcomes of children with PDE beyond age two (Conradt et al., 2019). Across these studies, some reported no cognitive differences between exposed and non-exposed children, whereas others found differences in IQ and memory performance (Conradt et al., 2019). Longitudinal investigations into the role of biological sex on the impact of PDE suggest that the severity of cognitive and behavioral outcomes and neurodevelopmental consequences may be moderated by sex, such that female sex is protective against adverse cognitive and behavioral outcomes (Suffet and Brotman, 1984; Moe and Slinning, 2001; Bennett et al., 2002, 2007; Delaney-Black et al., 2004; Nair et al., 2008; Nygaard et al., 2015; Conradt et al., 2018; Skumlien et al., 2020; Traccis et al., 2020).
It is highly probable that some individual differences in the long-term outcomes of those with PDE are related to variations in the postnatal environment. The small body of literature on long-term outcomes for children with PDE increasingly points toward a complex story in which postnatal environmental interactions may emerge or recede throughout development and may be sex-dependent (Ackerman et al., 2010; Betancourt et al., 2011; Traccis et al., 2020).
As cognitive development interacts with the social environment and develops over time, understanding the impact of PDE on cognitive and neural development necessitates the longitudinal study of these constructs within their social context. Variations in caregiver emotional state and caregiving are of particular interest, as mental health is associated with variations in caregiving and both positive (e.g., sensitive, responsive, and supportive) and negative1 (e.g., intrusive, inconsistent, or insensitive) caregiving plays a role in structuring biological, behavioral, and cognitive development (Nelson, 1993; Cleveland and Reese, 2005; Farah et al., 2008; Faure et al., 2017; Botdorf et al., 2019). The current study focuses on the early emotional caregiving environment (ECE), defined as caregiver’s emotional wellbeing and emotions toward parenting. We examine the interactions between early ECE and later cognitive ability (memory) and its underlying neural correlates, which are particularly susceptible to pre- and postnatal stressors due to their protracted development. These constructs may inform our understanding of the interaction between PDE and environmental risk across development (Geng et al., 2018; Riggins et al., 2018; Botdorf et al., 2019; Canada et al., 2022).
Protracted development of memory and the hippocampus
Typical development
Episodic memory is a cornerstone ability that supports the recall of past events and their spatiotemporal context (Canada et al., 2022). Episodic memory is implicated in academic and socioemotional outcomes and is susceptible to impairment across various psychiatric and neurologic disorders (Ghetti and Bunge, 2012). Memory capacity shows a protracted, linear development course, consistently improving between ages 4 and 8 years (Canada et al., 2022) and into adolescence (Ghetti and Bunge, 2012). Memory is primarily supported by the hippocampus, which is also involved in the stress response and stress/emotion regulation (Herman et al., 2005). The hippocampus also shows a protracted developmental course, maturing and changing structurally throughout adolescence (Ghetti and Bunge, 2012; Geng et al., 2018; Riggins et al., 2018; Botdorf et al., 2019) with normative hippocampal development including both increases and decreases in volume (Botdorf et al., 2019).
The protracted developmental course of both episodic memory and hippocampal development means that differences in functionality and capacity may continue to develop until the supporting neural systems fully develop (Geng et al., 2018; Riggins et al., 2018; Botdorf et al., 2019; Canada et al., 2022). Moreover, this implies an increased time window in which memory and its underlying neural networks are susceptible to environmental influences (Gogtay et al., 2006). For example, a study examining PDE’s effects on incidental memory found that although there were only marginal differences between PDE and non-exposed groups’ performance, memory ability improved at a significantly slower rate in the PDE group (Betancourt et al., 2011). Thus, the impact of PDE on underlying systems may vary over time, suggesting that children may “grow into” or “out of” an impairment as they mature and develop. Therefore, studying the longitudinal development of memory and the hippocampus in this population may offer insight into how PDE interacts with the postnatal social environment across development.
Individuals with a history of prenatal drug exposure
To our knowledge, eight studies have specifically explored the cognitive domain of episodic memory among children with PDE histories (Guo et al., 1994; Hurt et al., 2009; Betancourt et al., 2011; Riggins et al., 2012; Sundelin Wahlsten and Sarman, 2013; Konijnenberg et al., 2016; Geng et al., 2018; Konijnenberg and Melinder, 2022). Collectively, these studies encompass an extensive age range (3–17 years), utilize eight different assessments of memory, and are characterized by mixed findings throughout development. Compared to controls, studies report differences in memory performance in early (Sundelin Wahlsten and Sarman, 2013; Konijnenberg et al., 2016) and middle childhood (Guo et al., 1994; Konijnenberg and Melinder, 2022), both differences (Riggins et al., 2012) and no differences (Hurt et al., 2009; Betancourt et al., 2011) in memory performance in early to mid-adolescence, and no differences in memory performance in late adolescence (Betancourt et al., 2011; Geng et al., 2018) (see Supplementary material for a detailed overview). Taken together, these findings suggest that the impact of PDE on memory performance may vary across development.
Both structural and functional magnetic resonance imaging (MRI) studies have reported PDE-associated differences in the neural correlates of memory utilizing the same sample as the present study. Geng et al. (2018) explored the impact of PDE on neural function during recall tasks, reporting hemispheric differences in hippocampal activation between PDE and comparison groups during memory encoding but not retrieval at age 17. Regarding hippocampal structure, Riggins et al. (2012) found PDE was associated with larger hippocampal volumes at age 14 and that larger hippocampal volumes were associated with lower memory scores in a sample of adolescents with and without histories of PDE (Riggins et al., 2012). While these studies show neural differences related to PDE, given the developmental course of memory and the hippocampus, further investigation is needed to better understand how the effects of PDE on brain structure change with age.
Overall, mixed findings across studies may be a function of differences in task performance demands or PDE’s impact changing as episodic memory and its underlying neural systems develop and interact with the social environment throughout early life. This suggests a need for research exploring these constructs more comprehensively across different time points in development.
Caregiving, memory, and the hippocampus
Typical development
Caregiving and ECE represent potential sources of variability that may contribute to individual differences in memory capacity (Farah et al., 2008; Valentino et al., 2009; Larkina and Bauer, 2010; Botdorf et al., 2019) and hippocampal volume (Rao et al., 2010; Luby et al., 2013; Blankenship et al., 2019). Disruptions in caregiving behavior associated with maternal mental health are well documented (Field, 1994; Lovejoy et al., 2000; Pettit et al., 2008; Botdorf et al., 2019; Urizar and Muñoz, 2022). Literature suggests that more negative caregiver emotional state puts a child at greater risk for experiencing negative caregiving characterized by greater negative affect, reduced support, and less sensitivity to child needs (Lovejoy et al., 2000; Dougherty et al., 2013).
Extant literature has demonstrated that memory and the hippocampus are sensitive to the influence of caregiving among children without PDE (Moore and Zoellner, 2007; Valentino et al., 2009; Valentino, 2011; Luby et al., 2013; Woody et al., 2015), and childhood may constitute a sensitive period for these influences (Rao et al., 2010; Luby et al., 2016). In studies with non-prenatally drug-exposed children, supportive and engaged caregiving has been associated with better autobiographical and general episodic memory (Valentino et al., 2009; Larkina and Bauer, 2010), whereas extreme negative caregiving and maternal depression have been linked to memory deficits such as overgeneralized autobiographical memory (Moore and Zoellner, 2007; Valentino et al., 2009; Valentino, 2011; Woody et al., 2015).
Relatedly, both positive and negative caregiving practices are thought to differentially impact hippocampal volume by altering the neurodevelopmental process of synaptogenesis (Liu et al., 2000). Early experiences of negative caregiving, maternal depression, and high stress in early childhood predict differences in hippocampal structure and function in later childhood (Blankenship et al., 2019; Luby et al., 2019). Researchers have found smaller hippocampal volumes for children who were physically abused or had greater cumulative stress exposure (Hanson et al., 2015; Humphreys et al., 2019; Botdorf et al., 2022). Another study reports significant associations between lifetime stress severity and left hippocampal volume in children younger than five, and no significant association between stress severity and hippocampal volume after age six (Humphreys et al., 2019). Moreover, stress severity in early childhood remained a significant predictor of left hippocampal volume beyond later stress severity–further suggesting evidence for a sensitive period for the effects of life stress on hippocampal volume (Humphreys et al., 2019).
Although more negative caregiving environments and more a negative caregiver emotional state may represent a significant risk factor for differences in hippocampal development, studies report that high-quality caregiving may be particularly beneficial or protective (Luby et al., 2013, 2016, 2019; Wang et al., 2014, 2019). Overall, better caregiving quality is associated with larger left hippocampi and smaller right hippocampi in infancy (Qiu et al., 2013), greater anterior functional connectivity in early childhood (Wang et al., 2019), faster growth in volume at preschool age (Luby et al., 2016), and larger volumes and functional networks during school age (Luby et al., 2013, 2016, 2019; Wang et al., 2014). Findings are mixed in adolescence and young adulthood, as histories of early life adversity and negative caregiving have been associated with both smaller (Bremner et al., 1997; Stein et al., 1997; Vythilingam et al., 2002; Buss et al., 2007; Rao et al., 2010) and larger (Rao et al., 2010) hippocampi during adolescence (Belsky and de Haan, 2011). The impact of high-quality caregiving has also been indirectly associated with hippocampal structure, with some studies showing caregiver support mediating the effects of poverty and preschool Adverse Life Experiences (ACES) on bilateral hippocampal volume (Luby et al., 2013, 2019). Moreover, sex differences have been reported in hippocampal susceptibility to prenatal stress such that male sex is associated with a greater reduction in hippocampal volume (Buss et al., 2007; Samplin et al., 2013; Teicher et al., 2018) and, relative susceptibility may be modulated by the postnatal environment in a sex-specific manner (Buss et al., 2007). This finding suggests positive caregiving may buffer systemic stressors, biological risk, and hippocampal development (Luby et al., 2013, 2019).
Overall, given the protracted development and sensitivity of memory and the hippocampus to early life stress, investigations into the longitudinal development of memory and the hippocampus may be leveraged to provide insight into the impact of postnatal social environments on cognitive development among children with PDE.
Individuals with a history of prenatal drug exposure
The impact of PDE on children’s neurocognitive systems varies with exposure to multiple risk and protective factors throughout development (Ackerman et al., 2010; Rao et al., 2010; Buckingham-Howes et al., 2013). A growing literature supports models of heterogeneity in susceptibility to environmental risk that examine the possibility that teratogenic and maternal risk factors interact to influence child behavioral outcomes (Konijnenberg et al., 2015; Schuetze et al., 2021). Overall, the literature suggests that a history of PDE is associated with potential risk factors that may contribute to a negative caregiving environment and, in turn, have negative developmental consequences (Conradt et al., 2023).
Drug use during pregnancy is associated with significant maternal PTSD symptoms, increased likelihood of violence exposure, and higher incidence of psychopathology (Min et al., 2018; Punamäki et al., 2021). Mothers in treatment have described worsening depression and anxiety after delivery and its detrimental effects on their recovery and self-efficacy in caring for their children (Salo et al., 2009; Martin et al., 2022). Substance use may also impact how parents process and respond to infant cues, making caregiving more difficult (Rutherford et al., 2011, 2013, 2020; Parolin and Simonelli, 2016; Kohl et al., 2017; Daigle et al., 2020; Lowell et al., 2022). In addition, infants with histories of PDE may be particularly challenging to care for, with high-pitched, piercing cries, feeding difficulties, and slow, negative responses to stimuli (Coles and Platzman, 1993; Conradt et al., 2019). Therefore, parents who may already face increased neurobiological challenges to caregiving are charged with providing care for infants who are particularly difficult to support.
For children with PDE, repeatedly impaired responses to distress during infancy may set the stage for decreased self-regulatory capacities (Baker, 2018; Morawska et al., 2019). Moreover, PDE among children is often accompanied by additional family and environmental risk factors such as poverty, maternal psychopathology, problematic parent–child interactions, and formal and informal caregiver changes, often resulting in an unstable caregiving environment (Kettinger et al., 2000; Eiden et al., 2014). These environmental risk factors may accentuate the negative effects of PDE on child development through direct or indirect mechanisms (Ackerman et al., 2010; Konijnenberg et al., 2015). Some studies have found that the caregiving environment may exacerbate or buffer against early biological vulnerability due to PDE (Konijnenberg et al., 2015; Jaekel et al., 2021). Postnatal and family factors may account for approximately half of the differences in opioid-exposed and non-exposed children’s cognitive and motor outcomes (Levine et al., 2021). Moreover, the quality of caregiving and home environment at 18 months may mediate the impact of PDE on language development at age 4.5 years (Kim et al., 2021). Overall, these findings suggest that a more favorable early caregiving environment may attenuate the impact of PDE on developmental outcomes. In contrast, a more negative caregiving environment may exacerbate the overall impact of PDE on child development (Conradt et al., 2018, 2023).
Although these findings support the developmental consequences of a significant interaction between PDE and the caregiving environment, the impact of this relationship on memory and its underlying neural structures is primarily unexplored as children age (Ackerman et al., 2010).
Of the eight studies that have specifically focused on the cognitive domain of episodic memory among those with PDE histories, only two directly explored the impact of the caregiving environment on memory outcomes (Guo et al., 1994; Konijnenberg et al., 2016). Konijnenberg et al. (2016) found a significant main effect of mother–child interaction quality on narrative memory at age 4; however, there was no interaction effect of group status and mother–child interaction, suggesting that caregiver-child interaction quality had a similar influence on narrative memory development, regardless of PDE exposure. Similarly, Guo et al. (1994) found that children with PDE histories showed similar reduced memory performance and ERP amplitude to children without PDE histories but currently living with a caregiver using opiates. This suggests that the postnatal environment may have similar adverse effects, regardless of exposure status.
Other studies covaried caregiver environment variables. Two studies found no main memory effect of parental nurturance and environmental stimulation, measured at ages 4 and 8, or maternal depression and foster care placement, measured at child age 11 (Hurt et al., 2009; Betancourt et al., 2011). One study found a significant main effect of maternal depression, measured at child age 6, on immediate recall and left hippocampal volume (Riggins et al., 2012). In this study, more significant depression was related to larger left hippocampal volumes and better immediate recall at age 14 (Riggins et al., 2012).
Collectively, the impact of PDE on child cognitive and neural development is complex, open to environmental influence, and potentially age and sex-dependent. Prior studies highlight the importance of the early caregiving environment for memory and hippocampal development, suggesting the potential of the caregiving environment to either compound or remediate the adverse impacts of PDE on developmental outcomes as children age.
The current study aims to leverage the study of memory and hippocampal development to explore whether the caregiving emotional environment may modulate the impact of PDE on neural and cognitive systems. In line with previous literature, we hypothesize that among children with PDE, a negative caregiving environment during infancy will predict worse episodic memory performance at 14 years (Guo et al., 1994; Konijnenberg et al., 2016) and smaller hippocampal volumes (Bremner et al., 1997; Stein et al., 1997; Vythilingam et al., 2002; Buss et al., 2007; Rao et al., 2010; Belsky and de Haan, 2011; Blankenship et al., 2019) at both 14 and 18 years. We further hypothesize that poor episodic memory at age 14 will be associated with variations in hippocampal volumes at ages 14 and 18 (Riggins et al., 2012). Moreover, we propose that these associations will be moderated by biological sex, such that males will have worse memory performance and larger hippocampal volumes than females (Bennett et al., 2002; Buss et al., 2007; Nair et al., 2008; Samplin et al., 2013; Traccis et al., 2020).
Methods
Participants
Phase 1 (Prenatal-24 months Postpartum)
Participants were drawn from a randomized, controlled trial of a home-based intervention for substance-using women and their infants (Nair et al., 2008). Two hundred sixty five mothers (Age M = 26.89, SD = 5.21) were recruited from an urban University Hospital and enrolled at delivery in the early 1990s (Schuler et al., 2000). Recruitment procedures have been reported previously (Schuler et al., 2002).
Parent–child dyads were randomized to intervention and control groups. Eligibility included gestational age > 32 weeks, birth weight > 1,750 g, no neonatal intensive care unit admission, and positive (cocaine/heroin) maternal/infant urine toxicology or maternal self-report of cocaine/heroin use during pregnancy. Intervention families received developmentally oriented home visits for one year. See Table 1 for complete maternal demographics.
A pediatrician reviewed neonatal medical records for head circumference, length, birth weight, gestational age, length of hospital stays, birth asphyxia, respiratory distress, sepsis, and neonatal abstinence syndrome (NAS). The mean gestational age was 38.45 (SD = 2.52) weeks, the mean length of hospital stay was 5.07 days (SD = 4.37), and the average birth weight was 2750.19 g (SD = 468.02). The total number of total neonatal problems ranged from 0 (26.01%), to 1 (18.84%), to ≥2 (26.01%), (M = 1.86, SD = 1.73), and 26 participants (37.68%) received a diagnosis of NAS. See Table 2 for birth outcomes.
Phase 2: early adolescence
Participants were recontacted during adolescence to participate in a follow-up study to assess longitudinal outcomes. Of participants retained through adolescence, 69 provided usable data (Age M = 14.24, SD = 1.14, 52.17% female). Only participants who provided data at 14 years were retained for analyses.
Of the retained children, 14.49% were exposed to cocaine, 28.99% were exposed to heroin, and 52.52% were exposed to cocaine and heroin. In most cases, exposure to cocaine and/or heroin (84%) was “heavy” as defined by a positive toxicology screen at birth and/or maternal self-reported use of 2 times or more per week during the last six months of pregnancy (i.e., 48–180 days). Consistent with previous studies (Ackerman et al., 2010), other drug use was common (i.e., cigarettes, alcohol); 87% were exposed to 3 or more substances including cocaine and heroin. See Table 2 for child exposures.
Participants in the final sample were compared to participants who were lost to follow-up on the following seven key variables: birth weight, maternal education, maternal age at first pregnancy, maternal age at the birth of the target child, neonatal abstinence scores, child gender, and receipt of public assistance. There were no significant differences between those lost and those retained. See Table 2 for child demographics.
A subset of 27 adolescents were eligible and agreed to participate in the neuroimaging sub-study (Age M = 14.51 years, SD = 1.18, 54.85% female). The demographic characteristics of participants in the neuroimaging subset were similar to those of the larger sample (Table 2).
Phase 3: late adolescence
Participants were recontacted to participate in a follow-up of the neuroimaging study. Seventeen participants were eligible and provided usable data (Age M = 18.1 years, SD = 1, 58.82% female). 64.71% of participants provided data at both 14- and 18-year scans. The demographic characteristics of the participants were similar to those of the larger sample and the phase 2 imaging participants (Table 2).
Procedures
PDE was assessed at delivery through a positive maternal toxicology screen, positive infant toxicology screen, maternal self-report, and/or notation in the mother’s medical chart (Black et al., 1993, p. 199; Schuler et al., 2000). All caregivers and their children completed a systematic protocol in the lab. The Institutional Review Boards at the University of Maryland Baltimore and the National Institute on Drug Abuse Intramural Research Program approved the study. Informed consent was obtained from all caregivers, and assent/consent was obtained at adolescence as appropriate (assent for younger than 18 years, consent for 18 years or older).
Measures
See Figure 1 for a timeline of study measures.
Phase 1 (Prenatal-24 months postpartum)
Caregiver depressive symptoms
The Center for Epidemiological Studies Depression Scale (CES-D), a 20-item self-report depressive symptom scale, was administered at 24 months (Radloff, 1977). Participants rate how often over the past week they have experienced symptoms on a 4-point scale. The CES-D includes items such as “I was bothered by things that usually do not bother me.” The CES-D has demonstrated high internal consistency, reliability, and sensitivity to differences between caregivers and non-caregivers (Pinquart and Sörensen, 2003). Scores for total depressive symptoms ranged from 0 to 39. Cronbach’s alpha for this study was 0.89. Higher scores indicate higher severity of symptoms. Scores >15 are in the clinical range (Radloff, 1977); 27.53% (n = 19) of caregivers met the clinical criteria for depression.
Caregiver stress
The Parenting Stress Index (PSI), a 101-item scale, was administered to caregivers at 18 months (Abidin, 1995). The PSI is scored on a 5-point Likert scale to tap into the domains of parent characteristics, child characteristics, and situational life stress. The scale is considered the gold standard measure for parental stress. Numerous studies provide evidence of its high internal consistency, good test–retest reliability, and validity in a wide range of at-risk youth populations (Ríos et al., 2022). The total mean parent score was used to indicate overall stress, with higher scores indicating more significant stress (Abidin, 1995). Scores >148 are in the clinical range (Abidin, 1995); 13.04% (n = 9) of caregivers met clinical criteria for parental stress.
Caregiver distress
The Child Abuse Potential Inventory (CAPI), a 160-item assessment of caregiver inclination toward abuse and neglect, was administered to caregivers at 24 months (Milner, 2004). It has shown good internal consistency and reliability across sample groups and cultures (Walker and Davies, 2010). The CAPI asks participants whether they agree or disagree with a statement to estimate the risk of a parent physically abusing a child. Higher scores indicate a greater likelihood of abuse. The distress factor score was used in analyses. Scores above 152 are in the clinical range (Milner, 2004); 21.74% (n = 15) of caregivers met clinical criteria.
Caregiver changes
Respondents reported caregiver changes at each evaluation, defined as residing with a non-maternal caregiver for ≥1 month. Caregiver changes by age seven years were summed (M = 0.94, SD = 1.7); 44.92% had no caregiver changes, 26.01% had one change, and 23.19% had ≥2 changes.
Phase 2: early adolescence
Wechsler Abbreviated Scale of Intelligence (WASI-II) is an abbreviated verbal and nonverbal intelligence measure designed for individuals 6–90 years old. The Full-Scale IQ (FSIQ-2), composed of the matrix reasoning and vocabulary sections, was used in the current study. The FSIQ-2 yields standardized scores. It has been found to have a split-half reliability coefficient of 0.89 and a test–retest reliability of 0.90 (McCrimmon and Smith, 2013).
California Verbal Learning Test–Child Version (CVLT-C; Delis et al., 1988) measures strategies and processes involved in learning and recalling verbal material. Participants are asked to remember a shopping list of 15 items (List A). For the first five trials, the same list was read to participants, and they were asked to recall words from the list after each presentation. A second interference list (List B) was then presented, and participants were asked to recall as many words from this list as possible. When the List B trial was completed, participants were again asked to recall words from List A without an additional presentation of List A. The test has shown high internal consistency and reliability (Mottram and Donders, 2005). Only scores from the free recall, short-delay portion were used in analyses to represent episodic memory.
Child Memory Scales (CMS, Cohen, 1997) measures learning and memory across various dimensions. Participants were read two short stories and asked to recall them immediately and after a 15-min delay. Only scores from the delayed verbatim recall were used in analyses to represent episodic memory. This assessment resulted in measures of immediate and delayed recall of verbatim and thematic information and delayed recognition. CMS has shown high test–retest and split-half reliability and moderate to high concurrent validity (Cohen, 2011).
Rey–Osterrieth Complex Figure (ROCF; Rey and Osterrieth, 1941) has been used to evaluate non-verbal memory and viso-constructional ability for decades. Participants copy complex geometric shapes and reproduce them from memory in delayed recall trials. The Taylor 1959 scoring method was used to evaluate each unit’s accuracy and the figure’s relative position, reflecting the degree of similarity between the original image and the reconstruction (Frisk et al., 2005). Each element of the ROCF was scored between 0 and 2 points. Total accuracy scores range from 0 to 36. Delayed recall scores were used for analyses. The ROFC has shown good clinical practicality, high inter-rater reliability, and good concurrent and content validity (Davies et al., 2011; Zhang et al., 2021).
Phases 2 and 3: ealy and late adolescence
Hippocampal volume
A 3-T Siemens Allegra scanner was used to acquire a whole-brain oblique axial T1-weighted structural image (MPRAGE) for anatomical evaluation (1-mm3 isotropic voxels: TR = 2.5 s; TE = 4.38 ms; FA = 80°). Cortical reconstruction and volumetric segmentation were performed using AFNI (Analysis of Functional Neuro-Imaging; Cox, 1996) and the Freesurfer image analysis suite. The technical details of the Freesurfer pipeline are described in prior publications (Dale and Sereno, 1993; Dale et al., 1999; Fischl et al., 1999, 2001, 2002, 2004; Han et al., 2006, p. 200). Freesurfer morphometric procedures have shown good test–retest reliability across scanner manufacturers and field strengths (Han et al., 2006). Freesurfer has been validated against manual measurements and has shown a reliable ability to detect differences in hippocampal volume (Morey et al., 2009).
Analytic approach
Emotional caregiver environment
Confirmatory factor analysis (CFA) with Mplus 7.11 was used to construct a latent variable of emotional caregiver environment (ECE), composed of three dimensions from measures collected in Phase 1: maternal depression (CES-D, M = 14.41, SD = 10.74), maternal stress (PSI: Parent Score, M = 131.02, SD = 19.43), and caregiver distress (CAPI: Distress Score, CAPI; M = 83.06, SD = 71.04). Scores were standardized and utilized in a one-factor, just-identified model. Standardized factor loadings range from 0.67–0.80 (caregiver depression: 0.67, p < 0.001, caregiver stress: 0.71, p < 0.001, caregiver distress: 0.080, p < 0.001) (See Figure 2A). High scores represent a negative caregiver emotional state.
Episodic memory
CFA was used to construct a latent variable of adolescent memory from three different delayed free-recall tasks from three separate Phase 2 measures: the CVLT-C (M = 10.38, SD = 2.38), the CMS (M = 26.18, SD = 14.04), and the ROCF task (M = 11.64, SD = 6.15). Scores were standardized and utilized in a one-factor, just-identified model. Standardized factor loadings range from 0.54 to 0.66 (CVLT-C: 0.66, p < 0.001, CMS: 0.57, p < 0.001, ROCF: 0.54, p < 0.001) (see Figure 2B). High scores indicate better memory performance.
Hippocampal volume adjustment
Hippocampal volumes were adjusted for age, sex, and intracranial volume (ICV) following methods detailed by Keresztes et al. (2017). In analyses exploring potential moderation by sex, hippocampal volumes were only adjusted for age and ICV so as not to remove for sex-dependent differences.
Analytic plan
Factor scores for latent variables were extracted for analyses. Multiple linear regressions were used to test the associations between the three constructs (Figure 3). Given the relations between the constructs, analyses utilizing episodic memory controlled for FSIQ-2 to obtain a more precise measurement of memory.
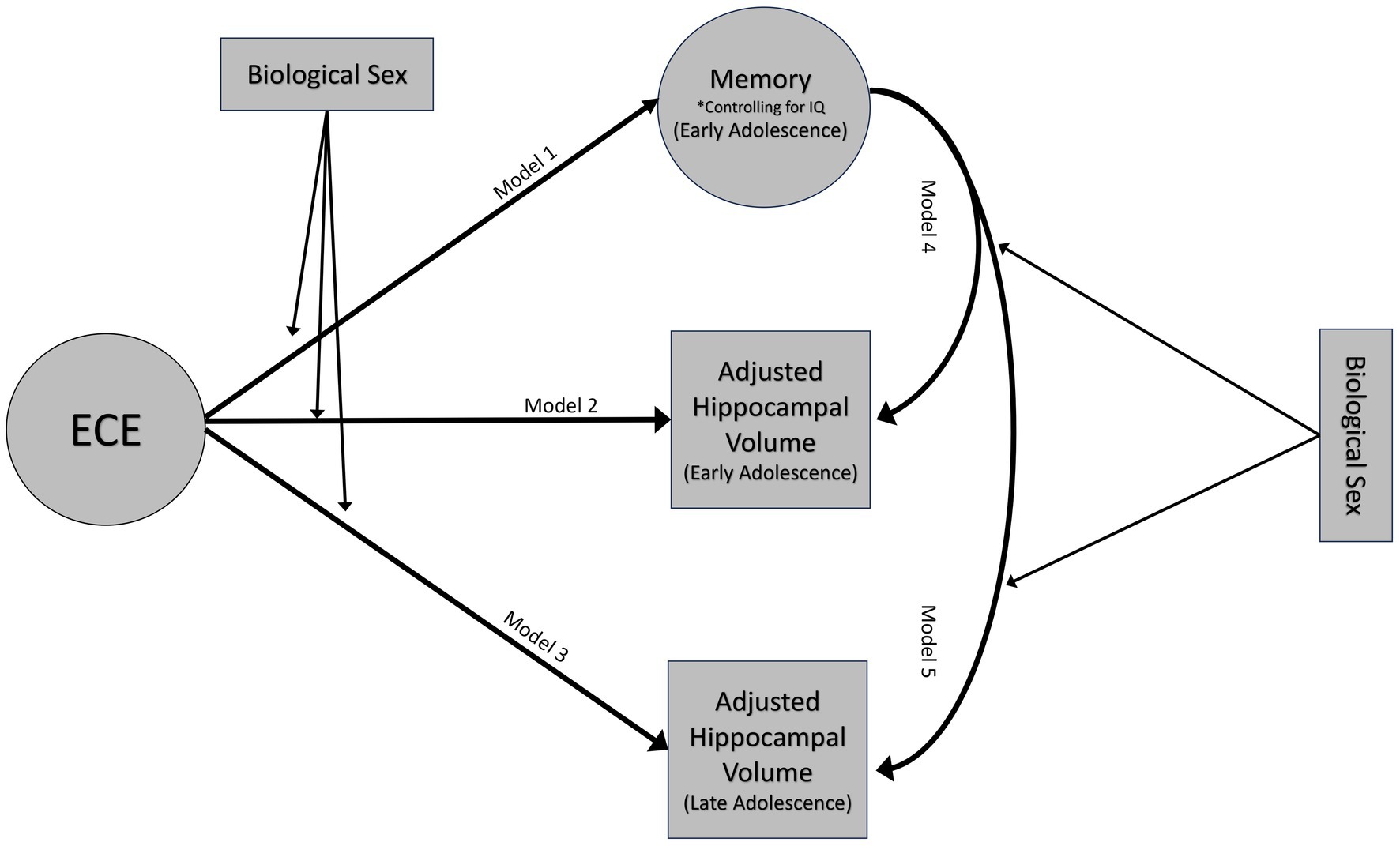
Figure 3. Conceptual model. Emotional caregiving environment will predict episodic memory performance at 14 years (model 1) and hippocampal volumes at both 14 (model 2) and 18 years (model 3). Episodic memory at age 14 will be associated with variations in hippocampal volumes at ages 14 (model 4) and 18 (model 5). These associations will be moderated by biological sex. Moderation analyses only adjusted hippocampal volumes for age and ICV.
After testing the initial relationships, follow up analyses were performed to assess biological sex as a potential moderator.
Results
Results of analyses outlined in the analytic plan are reflected in Figure 4.
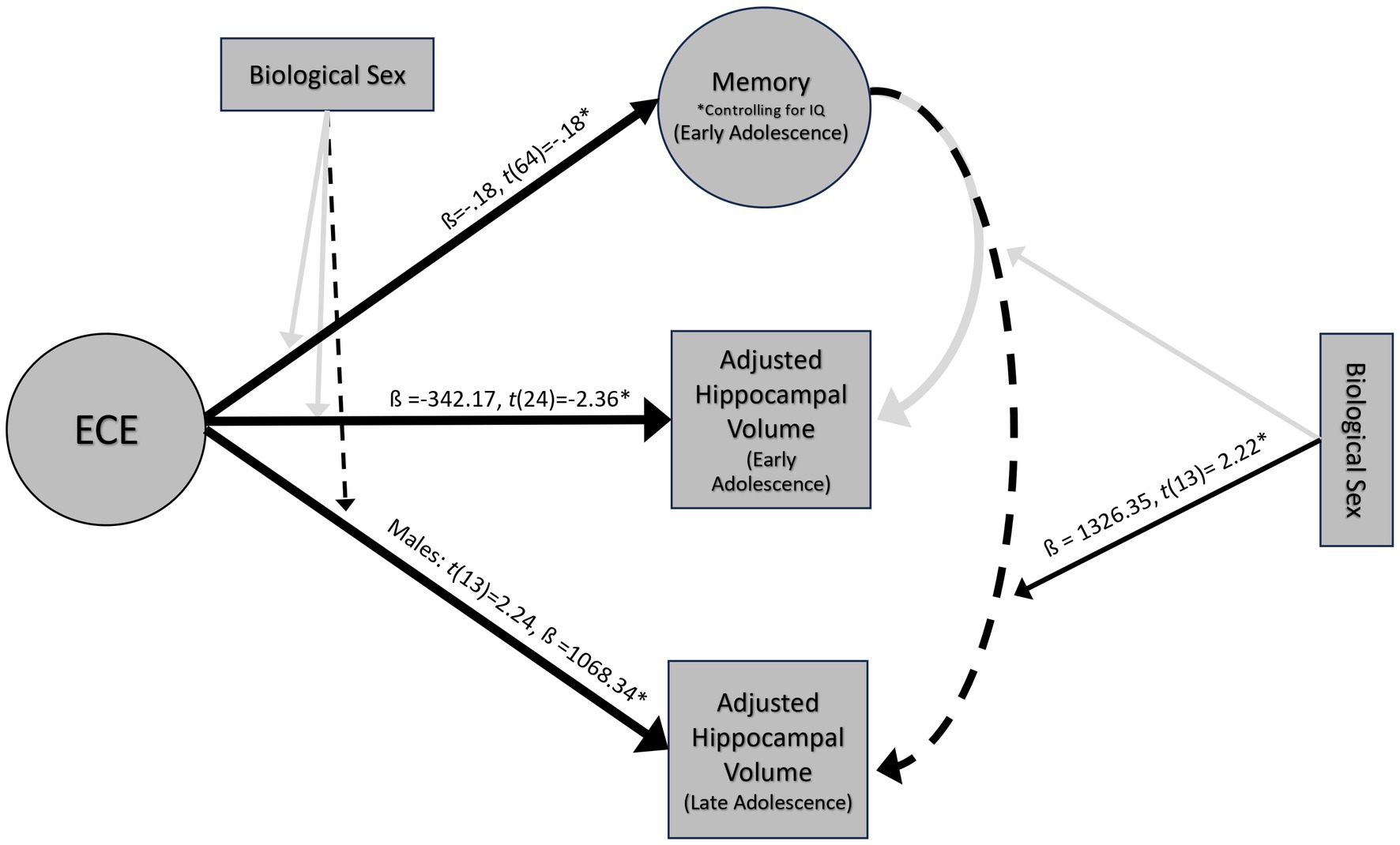
Figure 4. Results of multiple linear regressions at 14 and 18 years. *ps < 0.05, dashed lines indicate marginal significance ps < 0.10.
ECE and episodic memory (Model 1)
The full model explained significant variance in episodic memory scores, R2 = 14.11%, F(2, 64) = 5.26, p = 0.01. Negative ECE was associated with low memory scores when controlling for IQ, ß = −0.18, t(64) = −0.18, p = 0.04 (Figure 5A). This significant association held after controlling for the number of caregiver changes ß = −0.18, t(59) = −0.18, p = 0.04. Moderation analyses revealed no significant interaction between ECE and biological sex in predicting episodic memory, F(1, 62) = −0.16, p = 0.35. Based on a Bayes Factor Analysis, the null hypothesis is 3.64 times more likely than the alternative; thus, we conclude that biological sex does not significantly moderate the association between ECE and episodic memory.
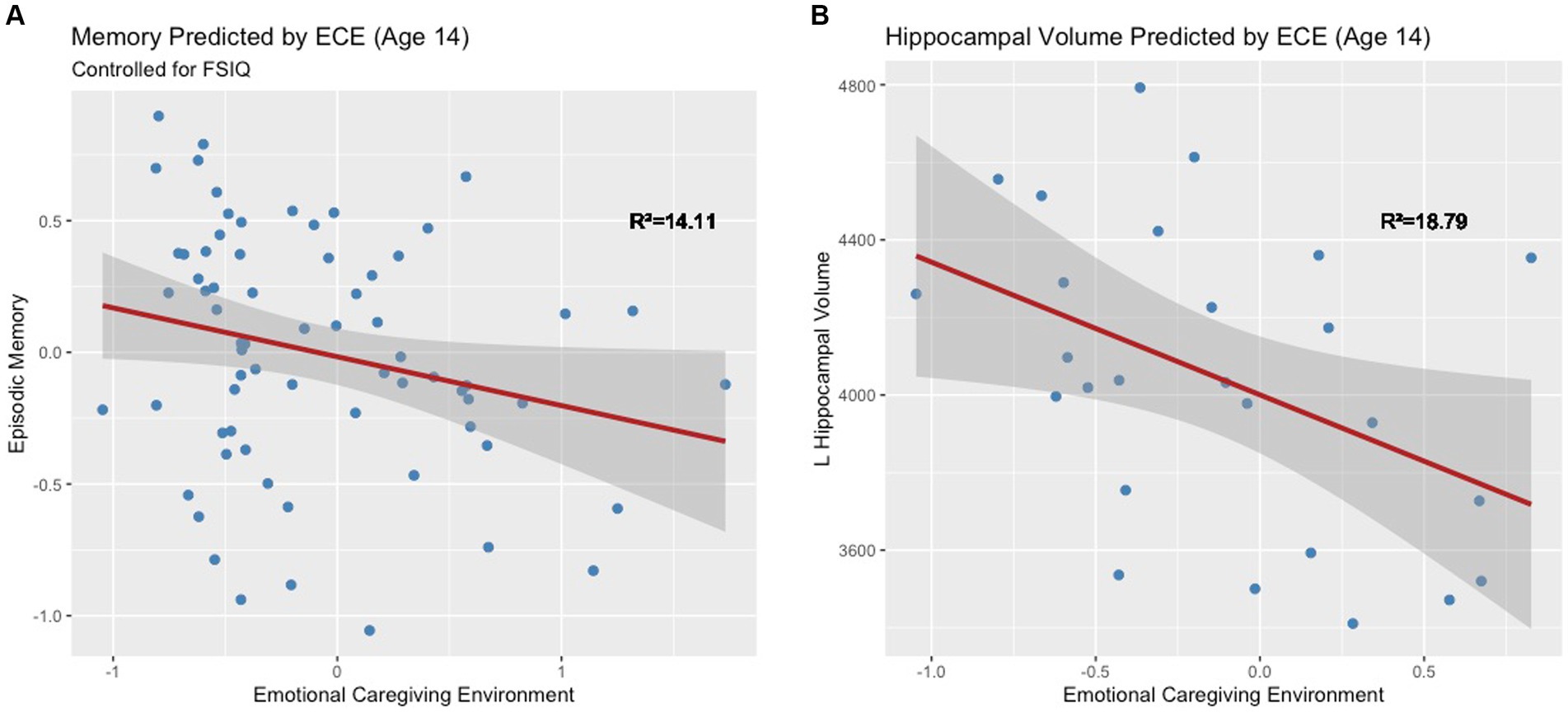
Figure 5. (A) Early emotional caregiving environment predicts episodic memory at age 14. (B) Early emotional caregiving environment predicts left hippocampal volume at age 14.
ECE and early adolescent hippocampal volume (Model 2)
ECE explained significant variance in adjusted left hippocampal volume at age 14, R2 = 18.79%, F(1, 24) = 5.55, p = 0.03. Negative ECE was associated with smaller adjusted left hippocampal volume, ß = −342.17, t(24) = −2.36, p = 0.03 (Figure 5B). This significant association held after controlling for the number of caregiver changes ß = -343.55, t(23) = −2.3, p = 0.03. ECE did not explain significant variance in right hippocampal volume (R2 = 6.87%, F(1, 24) = 1.77, p = 0.20). Moderation analyses revealed that there was not a significant interaction between ECE and biological sex in predicting adjusted left hippocampal volume, F(1, 23) = 0.37, p = 0.55. Based on a Bayes Factor Analysis, the null hypothesis is 1.92 times more likely than the alternative; thus, we conclude that biological sex does not significantly moderate the association between ECE and left hippocampal volume.
ECE and late adolescent hippocampal volume (Model 3)
ECE did not explain significant variance in right (R2 = 0.59%, F(1, 15) = 0.09, p = 0.77), or left hippocampal volume (R2 = 6.28%, F(1, 15) = 1.00, p = 0.33) at age 18. Moderation analyses revealed that there was not a significant interaction between ECE and biological sex in predicting bilateral (ß = −357.7, t(13) = −0.40, p = 0.69) or right hippocampal volume (ß =755.6, t(13) = 1.3, p = 0.22). There was a marginally significant interaction between ECE and left hippocampal volume (ß = −1096.7, t(13) = −2.0, p = 0.06). A negative ECE was associated with larger left hippocampal volumes in males (ß =1068.34, p = 0.04), but not in females (ß = -28.35, p = 0.92) (Figure 6). In analyses controlling for the interaction, there was a significant main effect of ECE on left hippocampal volume (ß = 1068.3, t(13) = 2.24, p = 0.04), such that a negative ECE was associated with larger left hippocampal volume.
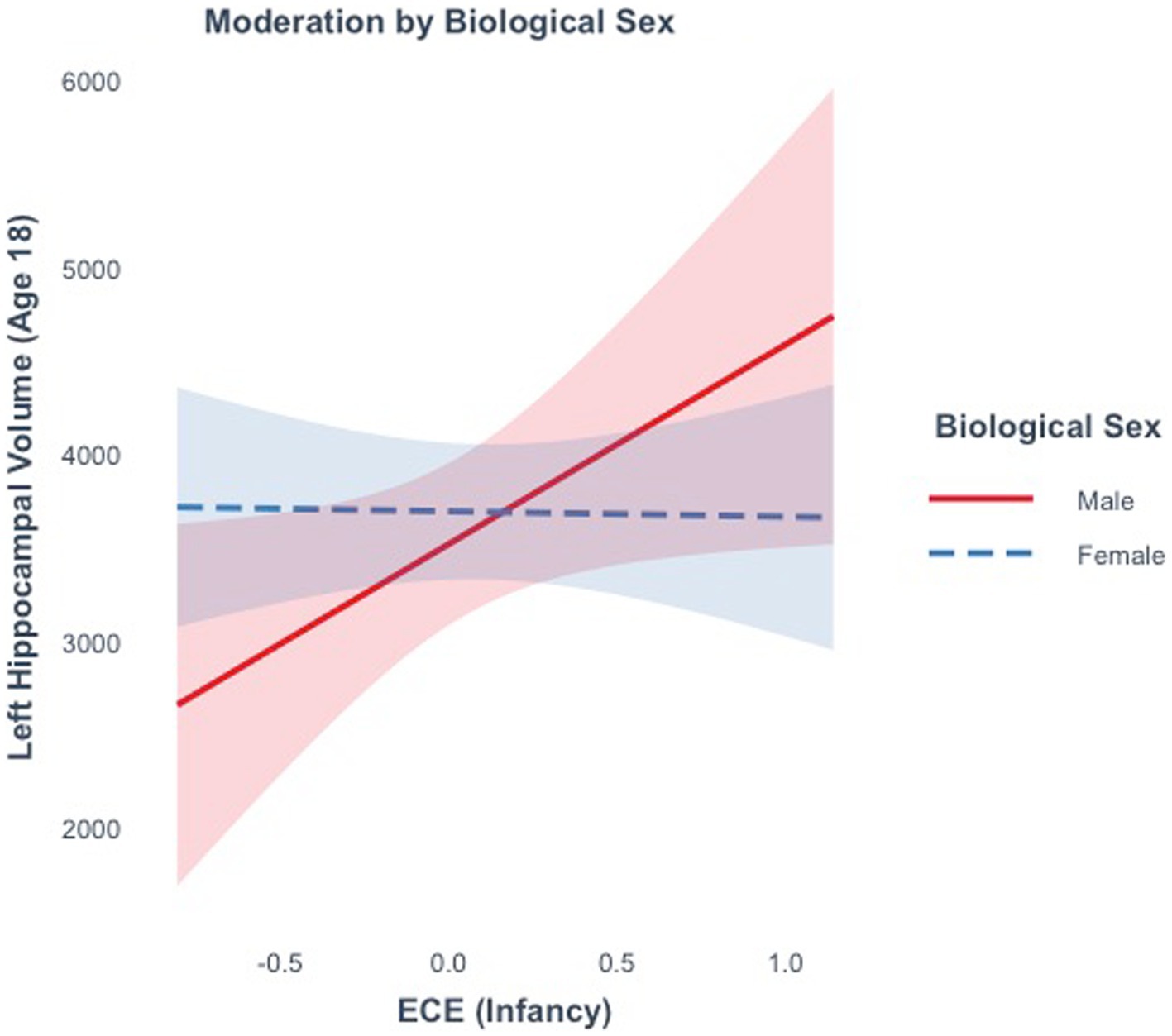
Figure 6. The effect of the early emotional caregiving environment on hippocampal volume at age 18 is moderated by biological sex.
Episodic memory and early adolescent hippocampal volume (Model 4)
The full model did not explain a significant amount of variance in right (R2 = 2.36%, F(2, 23) = 0.28, p = 0.76) or left hippocampal volume (R2 = 3.88%, F(2, 23) = 0.46, p = 0.63) at age 14. Episodic memory (t(23) = 0.89, p = 0.38) did not significantly predict adjusted left hippocampal volume in early adolescence. Moderation analyses revealed that there was not a significant interaction between memory and biological sex in predicting left hippocampal volume, (F(1, 23) = 0.32, p = 0.55). Based on a Bayes Factor Analysis, the null hypothesis is 7.05 times more likely than the alternative; thus, we conclude that biological sex does not significantly moderate the association between episodic memory and left hippocampal volume.
Episodic memory and late adolescent hippocampal volume (Model 5)
The full model explained a significant amount of variance in right hippocampal volume (R2 = 52.8%, F(2, 13) = 7.27, p = 0.01) at age 18. The main effect of memory in this model was marginal, (ß = 431.71, t(13) = 1.93, p = 0.07), such that better memory scores were marginally associated with larger right hippocampal volumes (Figure 7). The model did not explain a significant amount of variance in bilateral (R2 = 32.02%, F(2, 13) = 3.06, p = 0.08) or left hippocampal volume (R2 = 5.78%, F(2, 13) = 0.40, p = 0.68). Moderation analyses revealed no significant interaction between memory and biological sex in predicting bilateral (ß =767.8, t(13) = 0.80, p = 0.44) or right hippocampal volume (ß = −400.9, t(13) = −0.62, p = 0.55). There was a significant interaction between memory and biological sex in predicting left hippocampal volume (ß = 1326.35, t(13) = 2.22, p = 0.04). Better memory was marginally associated with smaller hippocampal volumes in males (ß = 800.12, p = 0.07), but not in females (ß = 526.23, p = 0.25) at age 18 (Figure 7).
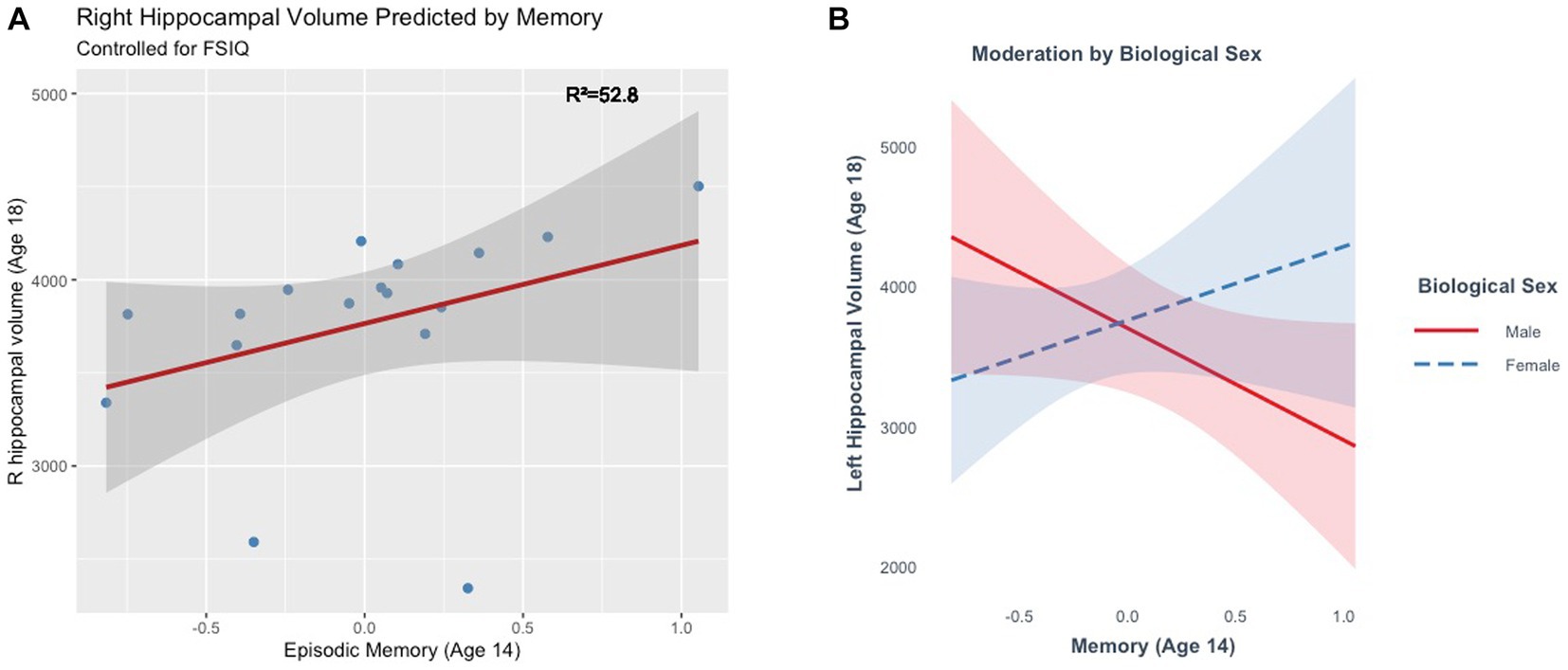
Figure 7. (A) Episodic memory at age 14 marginally predicts right hippocampal volume at age 18. (B) The effect of episodic memory at age 14 on hippocampal volume at age 18 is moderated by biological sex.
Additional analyses
Pearson’s product moment correlations examining the relationship between neonatal abstinence syndrome (NAS) and outcome variables were nonsignificant. At 14 years, NAS was not significantly correlated with memory r(67) = −0.09, p = 0.47, ECE r(67) = 0.14, p = 0.24, bilateral hippocampal volume r(24) = 0.129, p = 0.53, left hippocampal volume r(24) = 0.12, p = 0.56, or right hippocampal volume r(24) = 0.13, p = 0.525. Similarly, at 18 years, NAS was not significantly correlated with bilateral hippocampal volume r(15) = 0.27, p = 0.3, left hippocampal volume r(15) = 0.21, p = 0.42, or right hippocampal volume r(15) = 0.19, p = 0.46.
Pearson’s product moment correlations examining the relationship between intervention group status and outcome variables were nonsignificant. Intervention status was not significantly correlated with ECE, r(67) = 0.04, p = 0.72. At 14 years, intervention status was not significantly correlated with memory, r(67) = −0.09, p = 0.45, right hippocampal volume, r(24) = −0.013, p = 0.52, or left hippocampal volume r(24) = −0.19, p = 0.36. At 18 years, intervention status was not significantly correlated with right hippocampal volume r(15) = −0.19, p = 0.46, or left hippocampal volume, r(15) = 0.17, p = 0.50.
Discussion
This study explored whether the emotional caregiving environment during infancy may modulate the impact of PDE on neural and cognitive systems at 14 and 18 years. Results show significant associations between early emotional caregiving environment, memory, and hippocampal volume among participants with a history of PDE. In line with the hypotheses and previous literature (Guo et al., 1994; Konijnenberg et al., 2016), at 14 years, a negative emotional caregiving environment during infancy was associated with poor memory capacity, even after controlling for IQ, and with smaller hippocampal volumes in the left hemisphere. Better memory performance at 14 years predicted larger right hippocampal volume at 18 years. At 18 years, the association between the early emotional caregiving environment and hippocampal volume was moderated by sex, such that a negative emotional caregiving environment was associated with larger left hippocampal volumes in males but not females. Taken together, these findings suggest that PDE and the postnatal caregiving environment work together across development to influence neurocognitive systems. Such work is critical as it sheds light on modifiable factors that can buffer the effects of PDE across development.
Several findings are consistent with previous literature. First, in line with Guo et al. (1994) and Konijnenberg et al. (2016), negative ECE during infancy was associated with poorer memory capacity at 14 years, even after controlling for IQ. Second, findings are consistent with previous studies indicating that early ECE is related to smaller hippocampal volumes at 14 years (Bremner et al., 1997; Stein et al., 1997; Vythilingam et al., 2002; Buss et al., 2007; Rao et al., 2010; Belsky and de Haan, 2011; Blankenship et al., 2019). Finally, in line with emerging findings suggesting sex differences in relation to ECE and hippocampal volume, we found that biological sex moderated the association between ECE and hippocampal volume, as well as memory performance and hippocampal volume at age 18 (Moe and Slinning, 2001; Bennett et al., 2002, 2007; Delaney-Black et al., 2004; Buss et al., 2007; Nair et al., 2008; Samplin et al., 2013).
In contrast, some aspects of our findings are contrary to our hypotheses and previous literature. At age 18, our findings did not support a significant association between early emotional caregiving environment and hippocampal volume. However, follow-up moderation analyses found a marginally significant interaction between ECE and left hippocampal volume such that, after controlling for the interaction between ECE and left hippocampal, the main effect of ECE was significant, and a negative ECE was associated with larger left hippocampal volume. The direction of this effect was in the opposite direction than predicted for age 18. This finding contributes to the mixed literature related to the direction of the effect, in line with findings from Rao et al. (2010) but contrary to others who have suggested greater that early-life adversity is associated with smaller hippocampal volumes at age 18 (Bremner et al., 1997; Stein et al., 1997; Vythilingam et al., 2002; Buss et al., 2007; Rao et al., 2010; Belsky and de Haan, 2011; Blankenship et al., 2019). The mixed literature may result from a myriad of factors (see Belsky and de Haan, 2011 for a review). Notably, the studies that have shown an association are not homogenous in their age range, covariates, or conception of early-life stress (e.g., parental psychopathology, abuse, or neglect), which may contribute to some of the variability in findings. Moreover, it is possible that the children who remained in the present study through the 18-year timepoint had the most involved caregivers. This possibility may introduce further variability, as differential effects of optimal positive caregiving versus overprotection have been found on adult hippocampal volume (Wang et al., 2017).
Overall, the finding that negative ECE was associated with smaller left hippocampal volume at age 14 and larger left hippocampal volume in males at age 18 only further supports the theory that the hippocampus remains susceptible to environmental influence throughout adolescent development and the effects of the early social environment may be age-related (Gogtay et al., 2006). The variation of impact as a function of time has clinical implications, as children and adolescents with PDE histories may “grow into” or “out of” impairment throughout development. Additional research with a larger sample size and a comprehensive measure of the social environment beyond ECE could help inform the timing and targets of interventions for children with PDE histories.
Second, counter to hypotheses and prior literature suggesting that female sex is associated with better cognitive outcomes (Moe and Slinning, 2001; Bennett et al., 2002, 2007; Delaney-Black et al., 2004; Buss et al., 2007; Nair et al., 2008; Samplin et al., 2013; Traccis et al., 2020), the associations between hippocampal volume, ECE, and memory were moderated by sex at age 18 but not age 14. Little is known about how the effects of PDE may vary by biological sex throughout development (Conradt et al., 2018). Contrary to our findings, some have suggested that sex differences in outcomes may decline with age and are more prominent earlier in development (Traccis et al., 2020). Literature has linked estrogen to the promotion of hippocampal neurogenesis and synapse formation, suggesting a potentially protective role of the hormone on hippocampal development (Cooke and Woolley, 2005; Satterthwaite et al., 2014; Damme et al., 2020). To our knowledge, the association between estrogen and hippocampal development in samples of children with PDE has yet to be studied; however, given that the current study spans the pubertal period, fluctuations in estrogen may account for some sex differences. Overall, our findings suggest an enduring interaction between PDE biological sex, necessitating further research on sex-dependent outcomes.
Lastly, although we did see an association between memory performance at age 14 and hippocampal volume at age 18, we did not replicate previous findings that memory performance was associated with left hippocampal volume at age 14 (Riggins et al., 2012). One possible explanation may be using a comprehensive measure of memory, including verbal and nonverbal memory tasks, in the current study. It is also possible that potential variations in hippocampal volume were obscured because of the choice to estimate whole hippocampal volumes, rather than hippocampal subregions (Canada et al., 2022). An alternative explanation may be that current measures lack the specificity to show mechanistic differences in retrieval. For example, an fMRI study in this sample found differences between children with PDE and controls in memory encoding but not retrieval or performance (Geng et al., 2018). This finding suggests that overall performance may not differ between groups, but the neural resources necessary to support memory differ as a function of exposure. A better understanding of the consequences of exposure-dependent differences in neural resources and the relative impact of PDE on different stages in the memory process (e.g., encoding and retrieval) could inform future intervention targets.
Strengths and limitations
The current study adds to the limited literature exploring the long-term impacts of PDE on outcomes in memory and its associated neural structure. It adds a critical piece – the impact of the early social world (early caregiving emotional environment). Moreover, the use of neural assessment at multiple time points and the investigation into potential sex differences provides further insight into why past findings may be mixed, suggesting that the effects of PDE may be age and sex-dependent.
Although our study significantly contributes to the literature in support of the combined risk model, suggesting that teratogenic and maternal risk factors interact to influence child behavioral outcomes (Konijnenberg et al., 2015; Schuetze et al., 2021), it also has methodological limitations that necessitate caution in the interpretation of results. The sample size was small, particularly at age 18, limiting statistical power; however, the sample size at age 14 is consistent with prospective longitudinal, neuroimaging studies of high-risk children, and attrition analyses demonstrated few differences in demographic characteristics, reducing the likelihood of bias. Although the sample is homogeneous, which limits our ability to generalize findings beyond this group, this homogeneity increases our ability to control for the confounds of racial discrimination, socioeconomic resources, and neonatal problems.
The present study is further limited by assessing outcomes only for children with PDE. A community comparison group was recruited around the six-year time point; however, comparison participants were not included in analyses due to the lack of measurement for the early caregiving environment. Another limitation of the present study is the high incidence of polysubstance use. 87% of participants were exposed to three or more substances, including opioids and stimulants. Although polysubstance use complicates our ability to attribute findings to a specific drug, 85% of longitudinal studies of PDE consist of polysubstance-exposed children, which is consistent with typical substance use behavior (Lester et al., 1998; Ackerman et al., 2010; Jaekel et al., 2021). Therefore, while it is impossible to separate out specific substances in this sample, findings from this study have high ecological validity and can be generalized to many other studies of children with PDE.
Additionally, it was outside of the scope of the present paper to evaluate the influence of adolescent peer relationships on outcomes. However, it is worth noting that the adolescent period is a time where peers become increasingly influential in development (Telzer et al., 2018). Literature has demonstrated the importance of peers influence on adolescent risk-taking behavior (Albert and Steinberg, 2011); however, to our knowledge, studies have not explored the impact of peers on memory development. Future studies should evaluate the relative impact of peer and caregiver socialization on longitudinal memory development.
This study’s construct validity benefits from using latent variable structures. ECE comprises parent-report measures using multiple gold-standard assessments, which increases the likelihood that the overall factor score represents a valid assessment of the environment. Moreover, the use of multiple, standardized measures of memory, including verbal and nonverbal indices, furthers past research that has focused mainly on individual subtests of larger cognitive assessments, single measures, or one type of recall (i.e., verbal) (Guo et al., 1994; Sundelin Wahlsten and Sarman, 2013; Konijnenberg et al., 2016; Konijnenberg and Melinder, 2022). To our knowledge, the latent variable created for this study is the most comprehensive measure of episodic memory among the existent eight studies of PDE and memory outcomes, affording more confidence in statistical conclusions regarding the construct.
Lastly, the present study does not include a measure of continued maternal substance use or ECE as participants age, making it difficult to determine the impact of the concurrent caregiving environment on child outcomes beyond infancy; however, exploratory analyses revealed that the effects of ECE were still significant after controlling for the number of caregiver changes between birth and age 7, suggesting that the early caregiving environment continues to be important, even after controlling for instability. Future research should evaluate the relative impact of early-life experiences and later-life experiences on memory ability and hippocampal volume.
Notably, the measures of ECE used in this study range from negative to neutral. While a more negative caregiving emotional environment may pose a risk to child development, it is important to acknowledge that many potential factors can support resilience. Interventions for at-risk children have shown efficacy in improving caregiver-child attachment relationships and parental sensitivity and positively influencing child behavior and biology (Bick and Dozier, 2013; Bernard et al., 2017; Roben et al., 2017). Overall, ECE represents a modifiable construct that may, in turn, influence the developmental consequences of PDE. Future research should explore sources of resilience and more positive indicators of ECE.
Conclusion
Results from the present study highlight the interaction between prenatal and postnatal environments, suggesting a negative emotional caregiving environment may accentuate the effects of PDE on neurocognitive development, and these effects may be moderated by biological sex. This study contributes to the limited literature on the impact of pre- and postnatal factors on memory development in this population. Further, it extends past findings by including a neuroimaging assessment at 18 years of age. Although the sample size is small, we demonstrate that the effects of the interaction between pre- and postnatal factors vary from early-mid adolescence to late adolescence. Our finding that these effects are sex-dependent in late adolescence, but not mid-adolescence adds to the limited literature examining the interaction between PDE and sex through adolescence and into early adulthood. Findings can potentially influence intervention efforts for parent–child dyads coping with PDE significantly. The result sheds light on potential modifiable mechanisms of intergenerational transmission of risk. By supporting maternal functioning and the early caregiving environment, we may buffer against neurocognitive developmental risks associated with PDE.
Data availability statement
The original contributions presented in the study are included in the article/Supplementary material, further inquiries can be directed to the corresponding author.
Ethics statement
The studies involving humans were approved by Institutional Review Boards at the University of Maryland Baltimore and the National Institute on Drug Abuse Intramural Research Program. The studies were conducted in accordance with the local legislation and institutional requirements. Written informed consent for participation in this study was provided by the participants’ legal guardians/next of kin.
Author contributions
BHK, TR, and MAC contributed to the conception and design of the study. BHK performed statistical analyses with contributions from ZC. BHK wrote the first draft of the manuscript. All authors contributed to the manuscript revision, read, and approved the submitted version.
Funding
This research was supported in part by the Intramural Research Program of the NIH, NIDA, and grants DA07432-05 (Nair), DA02105-09 (Black), and DA029113 (Riggins).
Acknowledgments
We are grateful to the families and staff who made this study possible. We would like to thank Jade Dunstan (University of Maryland, College Park) for her tireless support, friendship, and peer mentorship. We would also like to thank Prasanna Nair (University of Maryland School of Medicine), without whom this study would not be possible.
Conflict of interest
The authors declare that the research was conducted in the absence of any commercial or financial relationships that could be construed as a potential conflict of interest.
Publisher’s note
All claims expressed in this article are solely those of the authors and do not necessarily represent those of their affiliated organizations, or those of the publisher, the editors and the reviewers. Any product that may be evaluated in this article, or claim that may be made by its manufacturer, is not guaranteed or endorsed by the publisher.
Supplementary material
The Supplementary material for this article can be found online at: https://www.frontiersin.org/articles/10.3389/fnbeh.2023.1238172/full#supplementary-material
Footnotes
1. ^While the constructs of positive and negative parenting are commonly used in the literature, it is important to acknowledge that these concepts are influenced by environmental demands and societal constructs. Therefore, negative parenting may be adaptive or protective in some contexts (Baumrind, 1972; Rious et al., 2019).
References
Abidin, R. R. (1995). “Parenting stress index manual” in Psychological assessment resources, Eds. C. P. Zalaquett and R. J. Wood (Wuhan: Scientific Research Publishing)
Ackerman, J. P., Riggins, T., and Black, M. M. (2010). A review of the effects of prenatal cocaine exposure among school-aged children. Pediatrics 125, 554–565. doi: 10.1542/peds.2009-0637
Albert, D., and Steinberg, L. (2011). “Peer influences on adolescent risk behavior” in Inhibitory control and drug abuse prevention: From research to translation. eds. M. T. Bardo, D. H. Fishbein, and R. Milich (London: Springer)
Baker, S. (2018). “The effects of parenting on emotion and self-regulation” in Handbook of parenting and child development across the lifespan. eds. M. R. Sanders and A. Morawska (London: Springer International Publishing)
Baumrind, D. (1972). An exploratory study of socialization effects on Black children: some Black-white comparisons. Child Dev. 43, 261–267. doi: 10.2307/1127891
Belsky, J., and de Haan, M. (2011). Annual research review: parenting and children’s brain development: the end of the beginning. J. Child Psychol. Psychiatry 52, 409–428. doi: 10.1111/j.1469-7610.2010.02281.x
Bennett, D., Bendersky, M., and Lewis, M. (2002). Children’s intellectual and emotional-behavioral adjustment at 4 years as a function of cocaine exposure, maternal characteristics, and environmental risk. Dev. Psychol. 38, 648–658. doi: 10.1037//0012-1649.38.5.648
Bennett, D., Bendersky, M., and Lewis, M. (2007). Preadolescent health risk behavior as a function of prenatal cocaine exposure and gender. J. Dev. Behav. Pediatr. 28, 467–472. doi: 10.1097/DBP.0b013e31811320d8
Bernard, K., Lee, A. H., and Dozier, M. (2017). Effects of the ABC intervention on Foster Children’s receptive vocabulary: follow-up results from a randomized clinical trial. Child Maltreat. 22, 174–179. doi: 10.1177/1077559517691126
Betancourt, L. M., Yang, W., Brodsky, N. L., Gallagher, P. R., Malmud, E., Giannetta, J. M., et al. (2011). Adolescents with and without gestational cocaine exposure: longitudinal analysis of inhibitory control, memory and receptive language. Neurotoxicol. Teratol. 33, 36–46. doi: 10.1016/j.ntt.2010.08.004
Bick, J., and Dozier, M. (2013). The effectiveness of an attachment-based intervention in PROMOTING foster mothers’ sensitivity toward foster infants. Infant Ment. Health J. 34, 95–103. doi: 10.1002/imhj.21373
Black, M. M., Schuler, M. E., and Nair, P. (1993). Prenatal drug exposure: neurodevelopmental outcome and parenting environment. J. Pediatr. Psychol. 18, 605–620. doi: 10.1093/jpepsy/18.5.605
Blankenship, S. L., Chad-Friedman, E., Riggins, T., Dougherty, L. R., Dougherty, L. R., and Dougherty, L. R. (2019). Early parenting predicts hippocampal subregion volume via stress reactivity in childhood. Dev. Psychobiol. 61, 125–140. doi: 10.1002/dev.21788
Botdorf, M., Canada, K. L., and Riggins, T. (2022). A meta-analysis of the relation between hippocampal volume and memory ability in typically developing children and adolescents. Hippocampus 32, 386–400. doi: 10.1002/hipo.23414
Botdorf, M., Riggins, T., and Dougherty, L. R. (2019). Early positive parenting and maternal depression history predict children’s relational binding ability at school-age. Dev. Psychol. 55, 2417–2427. doi: 10.1037/dev0000803
Bremner, J. D., Randall, P., Vermetten, E., Staib, L., Bronen, R. A., Mazure, C., et al. (1997). Magnetic resonance imaging-based measurement of hippocampal volume in posttraumatic stress disorder related to childhood physical and sexual abuse—a preliminary report. Biol. Psychiatry 41, 23–32. doi: 10.1016/s0006-3223(96)00162-x
Buckingham-Howes, S., Berger, S. S., Scaletti, L. A., and Black, M. M. (2013). Systematic review of prenatal cocaine exposure and adolescent development. Pediatrics 131, e1917–e1936. doi: 10.1542/peds.2012-0945
Buss, C., Lord, C., Wadiwalla, M., Hellhammer, D. H., Lupien, S. J., Meaney, M. J., et al. (2007). Maternal care modulates the relationship between prenatal risk and hippocampal volume in women but not in men. J. Neurosci. Off. J. Soc. Neurosci. 27, 2592–2595. doi: 10.1523/JNEUROSCI.3252-06.2007
Canada, K. L., Hancock, G. R., and Riggins, T. (2022). Developmental changes in episodic memory across early- to mid-childhood: insights from a latent longitudinal approach. Memory 30, 248–261. doi: 10.1080/09658211.2021.2006233
Cleveland, E. S., and Reese, E. (2005). Maternal structure and autonomy support in conversations about the past: contributions to Children’s autobiographical memory. Dev. Psychol. 41, 376–388. doi: 10.1037/0012-1649.41.2.376
Cohen, M. J. (1997). Children’s memory scale (CMS). San Antonio, Texas: The Psychological Corporation.
Cohen, M. (2011). “Children’s Memory Scale,” in Encyclopedia of Clinical Neuropsychology. Eds. J. S. Kreutzer, J. DeLuca, and B. Caplan Springer. 556–559.
Coles, C. D., and Platzman, K. A. (1993). Behavioral development in children prenatally exposed to drugs and alcohol. Int. J. Addict. 28, 1393–1433. doi: 10.3109/10826089309062192
Conradt, E., Camerota, M., Maylott, S., and Lester, B. M. (2023). Annual research review: prenatal opioid exposure – a two-generation approach to conceptualizing neurodevelopmental outcomes. J. Child Psychol. Psychiatry 64, 566–578. doi: 10.1111/jcpp.13761
Conradt, E., Crowell, S. E., and Lester, B. M. (2018). Early life stress and environmental influences on the neurodevelopment of children with prenatal opioid exposure. Neurobiol. Stress 9, 48–54. doi: 10.1016/j.ynstr.2018.08.005
Conradt, E., Flannery, T., Aschner, J. L., Annett, R. D., Croen, L. A., Duarte, C. S., et al. (2019). Prenatal opioid exposure: neurodevelopmental consequences and future research priorities. Pediatrics 144:e20190128. doi: 10.1542/peds.2019-0128
Cooke, B. M., and Woolley, C. S. (2005). Gonadal hormone modulation of dendrites in the mammalian CNS. J. Neurobiol. 64, 34–46. doi: 10.1002/neu.20143
Cox, R. W. (1996). AFNI: Software for analysis and visualization of functional magnetic resonance neuroimages. Computers and Biomedical Research, an International Journal, 29, 162–173. doi: 10.1006/cbmr.1996.0014
Daigle, K. M., Heller, N. A., Sulinski, E. J., Shim, J., Lindblad, W., Brown, M. S., et al. (2020). Maternal responsivity and oxytocin in opioid-dependent mothers. Dev. Psychobiol. 62, 21–35. doi: 10.1002/dev.21897
Dale, A. M., Fischl, B., and Sereno, M. I. (1999). Cortical surface-based analysis I. Segmentation and surface reconstruction. NeuroImage 9, 179–194. doi: 10.1006/nimg.1998.0395
Dale, A. M., and Sereno, M. I. (1993). Improved Localizadon of cortical activity by combining EEG and MEG with MRI cortical surface reconstruction: a linear approach. J. Cogn. Neurosci. 5, 162–176. doi: 10.1162/jocn.1993.5.2.162
Damme, K. S. F., Ristanovic, I., Vargas, T., and Mittal, V. A. (2020). Timing of menarche and abnormal hippocampal connectivity in youth at clinical-high risk for psychosis. Psychoneuroendocrinology 117:104672. doi: 10.1016/j.psyneuen.2020.104672
Davies, S. R., Field, A. R. J., Andersen, T., and Pestell, C. (2011). The ecological validity of the Rey–Osterrieth complex figure: predicting everyday problems in children with neuropsychological disorders. J. Clin. Exp. Neuropsychol. 33, 820–831. doi: 10.1080/13803395.2011.574608
Delaney-Black, V., Covington, C., Nordstrom, B., Ager, J., Janisse, J., Hannigan, J. H., et al. (2004). Prenatal cocaine: quantity of exposure and gender moderation. J. Dev. Behav. Pediatr. 25, 254–263. doi: 10.1097/00004703-200408000-00005
Delis, D. C., Freeland, J., Kramer, J. H., and Kaplan, E. (1988). Integrating clinical assessment with cognitive neuroscience: construct validation of the California verbal learning test. J. Consult. Clin. Psychol. 56, 123–130. doi: 10.1037/0022-006X.56.1.123
Dougherty, L. R., Tolep, M. R., Bufferd, S. J., Olino, T. M., Dyson, M., Traditi, J., et al. (2013). Preschool anxiety disorders: comprehensive assessment of clinical, demographic, temperamental, familial, and life stress correlates. J. Clin. Child Adolesc. Psychol. 42, 577–589. doi: 10.1080/15374416.2012.759225
Eiden, R. D., Coles, C. D., Schuetze, P., and Colder, C. R. (2014). Externalizing behavior problems among polydrug cocaine-exposed children: indirect pathways via maternal harshness and self-regulation in early childhood. Psychol. Addict. Behav. 28, 139–153. doi: 10.1037/a0032632
Farah, M. J., Betancourt, L., Shera, D. M., Savage, J. H., Giannetta, J. M., Brodsky, N. L., et al. (2008). Environmental stimulation, parental nurturance and cognitive development in humans. Dev. Sci. 11, 793–801. doi: 10.1111/j.1467-7687.2008.00688.x
Faure, N., Habersaat, S., Harari, M. M., Müller-Nix, C., Borghini, A., Ansermet, F., et al. (2017). Maternal sensitivity: a resilience factor against internalizing symptoms in early adolescents born very preterm? J. Abnorm. Child Psychol. 45, 671–680. doi: 10.1007/s10802-016-0194-0
Field, T. (1994). The effects of mother’s physical and emotional unavailability on emotion regulation. Monogr. Soc. Res. Child Dev. 59, 208–227. doi: 10.1111/j.1540-5834.1994.tb01286.x
Fischl, B., Liu, A., and Dale, A. M. (2001). Automated manifold surgery: constructing geometrically accurate and topologically correct models of the human cerebral cortex. IEEE Trans. Med. Imaging 20, 70–80. doi: 10.1109/42.906426
Fischl, B., Salat, D. H., Busa, E., Albert, M., Dieterich, M., Haselgrove, C., et al. (2002). Whole brain segmentation: automated labeling of neuroanatomical structures in the human brain. Neuron 33, 341–355. doi: 10.1016/s0896-6273(02)00569-x
Fischl, B., Salat, D. H., van der Kouwe, A. J. W., Makris, N., Ségonne, F., Quinn, B. T., et al. (2004). Sequence-independent segmentation of magnetic resonance images. NeuroImage 23, S69–S84. doi: 10.1016/j.neuroimage.2004.07.016
Fischl, B., Sereno, M. I., and Dale, A. M. (1999). Cortical surface-based analysis. II: inflation, flattening, and a surface-based coordinate system. NeuroImage 9, 195–207. doi: 10.1006/nimg.1998.0396
Frisk, V., Jakobson, L. S., Knight, R. M., and Robertson, B. (2005). Copy and recall performance of 6–8-year-old children after standard vs. step-by-step Administration of the Rey-Osterrieth Complex Figure. Child Neuropsychol. 11, 135–152. doi: 10.1080/092970490911289
Geng, F., Salmeron, B. J., Ross, T. J., Black, M. M., and Riggins, T. (2018). Long-term effects of prenatal drug exposure on the neural correlates of memory at encoding and retrieval. Neurotoxicol. Teratol. 65, 70–77. doi: 10.1016/j.ntt.2017.10.008
Ghetti, S., and Bunge, S. A. (2012). Neural changes underlying the development of episodic memory during middle childhood. Dev. Cogn. Neurosci. 2, 381–395. doi: 10.1016/j.dcn.2012.05.002
Gogtay, N., Nugent, T. F., Herman, D. H., Ordonez, A., Greenstein, D., Hayashi, K. M., et al. (2006). Dynamic mapping of normal human hippocampal development. Hippocampus 16, 664–672. doi: 10.1002/hipo.20193
Guo, X., Spencer, J. W., Suess, P. E., Hickey, J. E., Better, W. E., and Herning, R. I. (1994). Cognitive brain potential alterations in boys exposed to opiates: in utero and lifestyle comparisons. Addict. Behav. 19, 429–441. doi: 10.1016/0306-4603(94)90065-5
Han, X., Jovicich, J., Salat, D., van der Kouwe, A., Quinn, B., Czanner, S., et al. (2006). Reliability of MRI-derived measurements of human cerebral cortical thickness: the effects of field strength, scanner upgrade and manufacturer. NeuroImage 32, 180–194. doi: 10.1016/j.neuroimage.2006.02.051
Hanson, J. L., Nacewicz, B. M., Sutterer, M. J., Cayo, A. A., Schaefer, S. M., Rudolph, K. D., et al. (2015). Behavioral problems after early life stress: contributions of the Hippocampus and amygdala. Biol. Psychiatry 77, 314–323. doi: 10.1016/j.biopsych.2014.04.020
Herman, J. P., Ostrander, M. M., Mueller, N. K., and Figueiredo, H. (2005). Limbic system mechanisms of stress regulation: Hypothalamo-pituitary-adrenocortical axis. Prog. Neuro-Psychopharmacol. Biol. Psychiatry 29, 1201–1213. doi: 10.1016/j.pnpbp.2005.08.006
Humphreys, K. L., King, L. S., Sacchet, M. D., Camacho, M. C., Colich, N. L., Ordaz, S. J., et al. (2019). Evidence for a sensitive period in the effects of early life stress on hippocampal volume. Dev. Sci. 22:e12775. doi: 10.1111/desc.12775
Hurt, H., Betancourt, L. M., Malmud, E. K., Shera, D. M., Giannetta, J. M., Brodsky, N. L., et al. (2009). Children with and without gestational cocaine exposure: a neurocognitive systems analysis. Neurotoxicol. Teratol. 31, 334–341. doi: 10.1016/j.ntt.2009.08.002
Jaekel, J., Kim, H. M., Lee, S. J., Schwartz, A., Henderson, J. M. T., and Woodward, L. J. (2021). Emotional and behavioral trajectories of 2 to 9 years old children born to opioid-dependent mothers. Res. Child Adolesc. Psychopathol. 49, 443–457. doi: 10.1007/s10802-020-00766-w
Keresztes, A., Bender, A. R., Bodammer, N. C., Lindenberger, U., Shing, Y. L., and Werkle-Bergner, M. (2017). Hippocampal maturity promotes memory distinctiveness in childhood and adolescence. Proc. Natl. Acad. Sci. 114, 9212–9217. doi: 10.1073/pnas.1710654114
Kettinger, L. A., Nair, P., and Schuler, M. E. (2000). Exposure to environmental risk factors and parenting attitudes among substance-abusing women. Am. J. Drug Alcohol Abuse 26, 1–11. doi: 10.1081/ada-100100586
Kim, H. M., Bone, R. M., McNeill, B., Lee, S. J., Gillon, G., and Woodward, L. J. (2021). Preschool language development of children born to women with an opioid use disorder. Children 8:268. doi: 10.3390/children8040268
Kohl, J., Autry, A. E., and Dulac, C. (2017). The neurobiology of parenting: a neural circuit perspective. BioEssays 39, 1–11. doi: 10.1002/bies.201600159
Konijnenberg, C., Lund, I. O., and Melinder, A. (2015). Behavioural outcomes of four-year-old children prenatally exposed to methadone or buprenorphine: a test of three risk models. Early Child Dev. Care 185, 1641–1657. doi: 10.1080/03004430.2015.1016506
Konijnenberg, C., and Melinder, A. (2022). Verbal and nonverbal memory in school-aged children born to opioid-dependent mothers. Early Hum. Dev. 171:105614. doi: 10.1016/j.earlhumdev.2022.105614
Konijnenberg, C., Sarfi, M., and Melinder, A. (2016). Mother-child interaction and cognitive development in children prenatally exposed to methadone or buprenorphine. Early Hum. Dev. 101, 91–97. doi: 10.1016/j.earlhumdev.2016.08.013
Larkina, M., and Bauer, P. J. (2010). The role of maternal verbal, affective, and behavioral support in preschool children’s independent and collaborative autobiographical memory reports. Cogn. Dev. 25, 309–324. doi: 10.1016/j.cogdev.2010.08.008
Lester, B. M., LaGasse, L. L., and Seifer, R. (1998). Cocaine exposure and children: the meaning of subtle effects. Science 282, 633–634. doi: 10.1126/science.282.5389.633
Levine, T. A., Davie-Gray, A., Kim, H. M., Lee, S. J., and Woodward, L. J. (2021). Prenatal methadone exposure and child developmental outcomes in 2-year-old children. Dev. Med. Child Neurol. 63, 1114–1122. doi: 10.1111/dmcn.14808
Liu, D., Diorio, J., Day, J. C., Francis, D. D., and Meaney, M. J. (2000). Maternal care, hippocampal synaptogenesis and cognitive development in rats. Nat. Neurosci. 3, 799–806. doi: 10.1038/77702
Lovejoy, M. C., Graczyk, P. A., O’Hare, E., and Neuman, G. (2000). Maternal depression and parenting behavior: a meta-analytic review. Clin. Psychol. Rev. 20, 561–592. doi: 10.1016/S0272-7358(98)00100-7
Lowell, A. F., Morie, K., Potenza, M. N., Crowley, M. J., and Mayes, L. C. (2022). An intergenerational lifespan perspective on the neuroscience of prenatal substance exposure. Pharmacol. Biochem. Behav. 219:173445. doi: 10.1016/j.pbb.2022.173445
Luby, J., Belden, A., Botteron, K., Marrus, N., Harms, M. P., Babb, C., et al. (2013). The effects of poverty on childhood brain development: the mediating effect of caregiving and stressful life events. JAMA Pediatr. 167, 1135–1142. doi: 10.1001/jamapediatrics.2013.3139
Luby, J., Belden, A., Harms, M. P., Tillman, R., and Barch, D. M. (2016). Preschool is a sensitive period for the influence of maternal support on the trajectory of hippocampal development. Proc. Natl. Acad. Sci. 113, 5742–5747. doi: 10.1073/pnas.1601443113
Luby, J., Tillman, R., and Deanna, M. (2019). Association of Timing of adverse childhood experiences and caregiver support with regionally specific brain development in adolescents. JAMA Netw. Open 2:e1911426. doi: 10.1001/jamanetworkopen.2019.11426
Martin, C. E., Almeida, T., Thakkar, B., and Kimbrough, T. (2022). Postpartum and addiction recovery of women in opioid use disorder treatment: a qualitative study. Subst. Abus. 43, 389–396. doi: 10.1080/08897077.2021.1944954
McCrimmon, A. W., and Smith, A. D. (2013). Review of the Wechsler abbreviated scale of intelligence, second edition (WASI-II). J. Psychoeduc. Assess. 31, 337–341. doi: 10.1177/0734282912467756
Milner, J. S. (2004). The child abuse potential (CAP) inventory. In comprehensive handbook of psychological assessment, Personality assessment John Wiley & Sons, Inc.
Min, M. O., Minnes, S., Park, H., Ridenour, T., Ridenour, T., Ridenour, T. A., et al. (2018). Developmental trajectories of externalizing behavior from ages 4 to 12: prenatal cocaine exposure and adolescent correlates. Drug Alcohol Depend. 192, 223–232. doi: 10.1016/j.drugalcdep.2018.08.007
Moe, V., and Slinning, K. (2001). Children prenatally exposed to substances: gender-related differences in outcome from infancy to 3 years of age. Infant Ment. Health J. 22, 334–350. doi: 10.1002/imhj.1005
Moore, S. A., and Zoellner, L. A. (2007). Overgeneral autobiographical memory and traumatic events: an evaluative review. Psychol. Bull. 133, 419–437. doi: 10.1037/0033-2909.133.3.419
Morawska, A., Dittman, C. K., and Rusby, J. C. (2019). Promoting self-regulation in young children: the role of parenting interventions. Clin. Child. Fam. Psychol. Rev. 22, 43–51. doi: 10.1007/s10567-019-00281-5
Morey, R. A., Petty, C. M., Xu, Y., Hayes, J. P., Wagner, H. R., Lewis, D. V., et al. (2009). A comparison of automated segmentation and manual tracing for quantifying hippocampal and amygdala volumes. NeuroImage 45, 855–866. doi: 10.1016/j.neuroimage.2008.12.033
Mottram, L., and Donders, J. (2005). Construct validity of the California verbal learning test—Children’s version (CVLT-C) after pediatric traumatic brain injury. Psychol. Assess. 17, 212–217. doi: 10.1037/1040-3590.17.2.212
Nair, P., Black, M. M., Ackerman, J. P., Schuler, M. E., and Keane, V. (2008). Children’s cognitive-behavioral functioning at age 6 and 7: prenatal drug exposure and caregiving environment. Ambul. Pediatr. 8, 154–162. doi: 10.1016/j.ambp.2008.02.002
Nelson, K. (1993). The psychological and social origins of autobiographical memory. Psychol. Sci. 4, 7–14. doi: 10.1111/j.1467-9280.1993.tb00548.x
Nygaard, E., Moe, V., Slinning, K., and Walhovd, K. B. (2015). Longitudinal cognitive development of children born to mothers with opioid and polysubstance use. Pediatr. Res. 78, 330–335. doi: 10.1038/pr.2015.95
Parolin, M., and Simonelli, A. (2016). Attachment theory and maternal drug addiction: the contribution to parenting interventions. Front. Psych. 7:152. doi: 10.3389/fpsyt.2016.00152
Pettit, J. W., Olino, T. M., Roberts, R. E., Seeley, J. R., and Lewinsohn, P. M. (2008). Intergenerational transmission of internalizing problems: effects of parental and grandparental major depressive disorder on child behavior. J. Clin. Child Adolesc. Psychol. 37, 640–650. doi: 10.1080/15374410802148129
Pinquart, M., and Sörensen, S. (2003). Differences between caregivers and noncaregivers in psychological health and physical health: a meta-analysis. Psychol. Aging 18, 250–267. doi: 10.1037/0882-7974.18.2.250
Punamäki, R.-L., Flykt, M., Belt, R., Lindblom, J., and Lindblom, J. (2021). Maternal substance use disorder predicting children’s emotion regulation in middle childhood: the role of early mother-infant interaction. Heliyon 7:e06728. doi: 10.1016/j.heliyon.2021.e06728
Qiu, A., Rifkin-Graboi, A., Chen, H., Chong, Y.-S., Kwek, K., Gluckman, P. D., et al. (2013). Maternal anxiety and infants’ hippocampal development: timing matters. Transl. Psychiatry 3:e306. doi: 10.1038/tp.2013.79
Radloff, L. S. (1977). The CES-D scale: a self-report depression scale for research in the general population. Appl. Psychol. Meas. 1, 385–401. doi: 10.1177/014662167700100306
Rao, H., Betancourt, L., Giannetta, J. M., Brodsky, N. L., Korczykowski, M., Avants, B. B., et al. (2010). Early parental care is important for hippocampal maturation: evidence from brain morphology in humans. NeuroImage 49, 1144–1150. doi: 10.1016/j.neuroimage.2009.07.003
Rey, A., and Osterrieth, P. A. (1941). Rey-Osterrieth complex figure copying test. APA PsycTests. doi: 10.1037/t07717-000
Richardson, G. A., and Day, N. L. (2018). “Longitudinal Studies of the Effects of Prenatal Cocaine Exposure on Development and Behavior,” in Handbook of Developmental Neurotoxicology, 2nd Edition, Eds. W. Slikker, M. G. Paule, and C. Wang Academic Press. 379–388.
Riggins, T., Cacic, K., Buckingham-Howes, S., Scaletti, L. A., Jo Salmeron, B., and Black, M. M. (2012). Memory ability and hippocampal volume in adolescents with prenatal drug exposure. Neurotoxicol. Teratol. 34, 434–441. doi: 10.1016/j.ntt.2012.05.054
Riggins, T., Geng, F., Botdorf, M., Canada, K., Cox, L., and Hancock, G. R. (2018). Protracted hippocampal development is associated with age-related improvements in memory during early childhood. NeuroImage 174, 127–137. doi: 10.1016/j.neuroimage.2018.03.009
Ríos, M., Zekri, S., Alonso-Esteban, Y., and Navarro-Pardo, E. (2022). Parental stress assessment with the parenting stress index (PSI): a systematic review of its psychometric properties. Children 9:1649. doi: 10.3390/children9111649
Rious, J. B., Cunningham, M., and Beale Spencer, M. (2019). Rethinking the notion of “hostility” in African American parenting styles. Res. Hum. Dev. 16, 35–50. doi: 10.1080/15427609.2018.1541377
Roben, C. K. P., Dozier, M., Caron, E. B., and Bernard, K. (2017). Moving an evidence-based parenting program into the community. Child Dev. 88, 1447–1452. doi: 10.1111/cdev.12898
Rutherford, H., Potenza, M. N., and Mayes, L. C. (2013). “The neurobiology of addiction and attachment” in Parenting and substance abuse: Developmental approaches to intervention Eds. N. E. Suchman, M. Pajulo, and L. C. Mayes (Oxford: Oxford University Press) 3–23.
Rutherford, H., Williams, S., Moy, S., Mayes, L., and Johns, J. (2011). Disruption of maternal parenting circuitry by addictive process: rewiring of reward and stress systems. Front. Psychol. 2:e0037. doi: 10.3389/fpsyt.2011.00037
Rutherford, H., Yip, S. W., Worhunsky, P. D., Kim, S., Strathearn, L., Potenza, M. N., et al. (2020). Differential responses to infant faces in relation to maternal substance use: an exploratory study. Drug Alcohol Depend. 207:107805. doi: 10.1016/j.drugalcdep.2019.107805
Salo, S., Kivistö, K., Korja, R., Biringen, Z., Tupola, S., Kahila, H., et al. (2009). Emotional availability, parental self-efficacy beliefs, and child development in caregiver-child relationships with buprenorphine-exposed 3-year-olds. Parenting 9, 244–259. doi: 10.1080/15295190902844563
SAMHSA (2021). Section 8 PE Tables Results from the 2021 National Survey on drug use and health: detailed tables, SAMHSA, CBHSQ Available at: https://www.samhsa.gov/data/sites/default/files/reports/rpt39441/NSDUHDetailedTabs2021/NSDUHDetailedTabs2021/NSDUHDetTabsSect8pe2021.htm#tab8.25a (accessed May 26, 2023).
Samplin, E., Ikuta, T., Malhotra, A. K., Szeszko, P. R., and DeRosse, P. (2013). Sex differences in resilience to childhood maltreatment: effects of trauma history on hippocampal volume, general cognition and subclinical psychosis in healthy adults. J. Psychiatr. Res. 47, 1174–1179. doi: 10.1016/j.jpsychires.2013.05.008
Satterthwaite, T. D., Vandekar, S., Wolf, D. H., Ruparel, K., Roalf, D. R., Jackson, C., et al. (2014). Sex differences in the effect of puberty on hippocampal morphology. J. Am. Acad. Child Adolesc. Psychiatry 53, 341–350.e1. doi: 10.1016/j.jaac.2013.12.002
Schuetze, P., Godleski, S., and Sassaman, J. (2021). Prenatal exposure to opioids: associations between the caregiving environment and externalizing behaviors. Neurotoxicol. Teratol. 87:107019. doi: 10.1016/j.ntt.2021.107019
Schuler, M. E., Nair, P., and Black, M. (2002). Ongoing maternal drug use, parenting attitudes, and a home intervention: effects on mother-child interaction at 18 months. J. Dev. Behav. Pediatr. 23, 87–94. doi: 10.1097/00004703-200204000-00004
Schuler, M. E., Nair, P., Black, M. M., and Kettinger, L. (2000). Mother–infant interaction: effects of a home intervention and ongoing maternal drug use. J. Clin. Child Psychol. 29, 424–431. doi: 10.1207/S15374424JCCP2903_13
Skumlien, M., Ibsen, I. O., Kesmodel, U. S., and Nygaard, E. (2020). Sex differences in early cognitive development after prenatal exposure to opioids. J. Pediatr. Psychol. 45, 475–485. doi: 10.1093/jpepsy/jsaa008
Stein, M. B., Koverola, C., Hanna, C., Torchia, M. G., and McClarty, B. (1997). Hippocampal volume in women victimized by childhood sexual abuse. Psychol. Med. 27, 951–959. doi: 10.1017/s0033291797005242
Suffet, F., and Brotman, R. (1984). A comprehensive care program for pregnant addicts: obstetrical, neonatal, and child development outcomes. Int. J. Addict. 19, 199–219. doi: 10.3109/10826088409057176
Sundelin Wahlsten, V., and Sarman, I. (2013). Neurobehavioural development of preschool-age children born to addicted mothers given opiate maintenance treatment with buprenorphine during pregnancy. Acta Paediatr. 102, 544–549. doi: 10.1111/apa.12210
Teicher, M. H., Anderson, C. M., Ohashi, K., Khan, A., McGreenery, C. E., Bolger, E. A., et al. (2018). Differential effects of childhood neglect and abuse during sensitive exposure periods on male and female hippocampus. NeuroImage 169, 443–452. doi: 10.1016/j.neuroimage.2017.12.055
Telzer, E. H., van Hoorn, J., Rogers, C. R., and Do, K. T. (2018). Social influence on positive youth development: a developmental neuroscience perspective. Adv. Child Dev. Behav. 54, 215–258. doi: 10.1016/bs.acdb.2017.10.003
Traccis, F., Frau, R., and Melis, M. (2020). Gender differences in the outcome of offspring prenatally exposed to drugs of abuse. Front. Behav. Neurosci. 14:e0072. doi: 10.3389/fnbeh.2020.00072
Urizar, G. G., and Muñoz, R. F. (2022). Role of maternal depression on child development: a prospective analysis from pregnancy to early childhood. Child Psychiatry Hum. Dev. 53, 502–514. doi: 10.1007/s10578-021-01138-1
Valentino, K. (2011). A developmental psychopathology model of overgeneral autobiographical memory. Dev. Rev. 31, 32–54. doi: 10.1016/j.dr.2011.05.001
Valentino, K., Toth, S. L., and Cicchetti, D. (2009). Autobiographical memory functioning among abused, neglected, and nonmaltreated children: the overgeneral memory effect. J. Child Psychol. Psychiatry 50, 1029–1038. doi: 10.1111/j.1469-7610.2009.02072.x
Vythilingam, M., Heim, C., Newport, J., Miller, A. H., Anderson, E., Bronen, R., et al. (2002). Childhood trauma associated with smaller hippocampal volume in women with major depression. Am. J. Psychiatry 159, 2072–2080. doi: 10.1176/appi.ajp.159.12.2072
Walker, C. A., and Davies, J. (2010). A critical review of the psychometric evidence base of the child abuse potential inventory. J. Fam. Violence 25, 215–227. doi: 10.1007/s10896-009-9285-9
Wang, Y., Buckingham-Howes, S., Nair, P., Zhu, S., Magder, L. S., Black, M. M., et al. (2014). Prenatal drug exposure, behavioral problems, and drug experimentation among African-American urban adolescents. J. Adolesc. Health 55, 423–431. doi: 10.1016/j.jadohealth.2014.02.021
Wang, Y., Song, Y., Li, X., Zhang, L., and Liu, J. (2017). Influence of parental care on offspring hippocampal volume in young adults varies as a function of overprotection. Sci. Rep. 7:46429. doi: 10.1038/srep46429
Wang, Q., Zhang, H., Wee, C.-Y., Lee, A., Poh, J. S., Chong, Y.-S., et al. (2019). Maternal sensitivity predicts anterior hippocampal functional networks in early childhood. Brain Struct. Funct. 224, 1885–1895. doi: 10.1007/s00429-019-01882-0
Woody, M. L., Burkhouse, K. L., and Gibb, B. E. (2015). Overgeneral autobiographical memory in children of depressed mothers. Cognit. Emot. 29, 130–137. doi: 10.1080/02699931.2014.891972
Keywords: caregiving, prenatal drug exposure, development, memory, hippocampus, adolescent brain
Citation: Kohn BH, Cui Z, Candelaria MA, Buckingham-Howes S, Black MM and Riggins T (2023) Early emotional caregiving environment and associations with memory performance and hippocampal volume in adolescents with prenatal drug exposure. Front. Behav. Neurosci. 17:1238172. doi: 10.3389/fnbeh.2023.1238172
Edited by:
Gayathri Dowling, National Institute on Drug Abuse (NIH), United StatesReviewed by:
Devon L. Graham, Florida State University, United StatesJulie M. Croff, Oklahoma State University Center for Health Sciences, United States
Copyright © 2023 Kohn, Cui, Candelaria, Buckingham-Howes, Black and Riggins. This is an open-access article distributed under the terms of the Creative Commons Attribution License (CC BY). The use, distribution or reproduction in other forums is permitted, provided the original author(s) and the copyright owner(s) are credited and that the original publication in this journal is cited, in accordance with accepted academic practice. No use, distribution or reproduction is permitted which does not comply with these terms.
*Correspondence: Tracy Riggins, cmlnZ2luc0B1bWQuZWR1