- 1Behavioral Neuroscience, Experimental and Biological Psychology, Faculty of Psychology, Philipps-University Marburg, Marburg, Germany
- 2Centre for Mind, Brain, and Behavior (CMBB), Philipps-University Marburg, Marburg, Germany
- 3Social and Affective Neuroscience Research Group, Laboratory of Biological Psychology, Research Unit Brain and Cognition, Faculty of Psychology and Educational Sciences, KU Leuven, Leuven, Belgium
- 4Leuven Brain Institute, KU Leuven, Leuven, Belgium
Background: Healthy brain development depends on early social practices and experiences. The risk gene CACNA1C is implicated in numerous neuropsychiatric disorders, in which key characteristics include deficits in social functioning and communication. Recently, we reported sex-dependent impairments in social behavior and ultrasonic vocalizations (USV) in juvenile heterozygous Cacna1c+/− (HET) rats. Specifically, HET females displayed increases in rough-and-tumble play that eliminated the typically observed sex difference between male and female rats. Interestingly, female wild-type Cacna1c+/+ (WT) pairs also showed a similar increase in social play when housed with HET females, suggesting their behavior may be influenced by HET cage mates. This indicates that the genetic makeup of the social environment related to Cacna1c can influence social play, yet systematic studies are lacking.
Methods: In the present study, we housed juvenile females in MIXED- or SAME-genotype cages and tested them in a social play paradigm with a same- and opposite-genotype partner.
Results: The results show that the early social environment and the genotype of the play partner influence social play and 50-kHz USV emission. Experience with a WT play partner appears necessary for HET females to show comparable levels of play and 50-kHz USV emission. Same-genotype HET pairs played less and emitted fewer 50-kHz USV than same-genotype WT or opposite-genotype pairs; however, we found that the decrease in social play and 50-kHz USV in HET pairs can be rescued by playing with a WT partner. The effect was particularly prominent when the first play partner was WT, as we found it increased play and 50-kHz USV emission in all subsequent interactions with ensuing partners.
Conclusion: These findings suggest that the genetic makeup related to the social environment and/or social peers influences social play in Cacna1c+/− haploinsufficient rats. Specifically, our results show that WT peers can rescue behavior and communication alterations in Cacna1c female rats. Our findings have important implications because they show that the genetic makeup of the social environment can divulge phenotypic changes in genetic rat models of neuropsychiatric disorders.
1. Introduction
In humans, the risk gene CACNA1C has been linked through genome-wide association studies (GWAS) to neuropsychiatric disorders with prominent social deficits, such as autism spectrum disorder (ASD) (Green et al., 2010; Cross-Disorder Group of the Psychiatric Genomics Consortium, 2013). The CACNA1C gene encodes the Cav1.2 L-type calcium channel and is an auspicious therapeutic target (Zamponi, 2016). In humans, the single-nucleotide polymorphism rs1006737 has been correlated to atypical social behavior and communication (Krug et al., 2010; Dima et al., 2013; Pasparakis et al., 2015). In genetic Cacna1c rodent models, social deficits in behavior and communication have been found in mice (Bader et al., 2011; Kabir et al., 2016) and rats (Kisko et al., 2018, 2020; Redecker et al., 2019; Wöhr et al., 2020).
Due to rats’ gregarious nature, they are a widely used model species for studying social behavior and communication (Homberg et al., 2017). As juveniles, rats interact with peers through rough-and-tumble play, also called social play (Panksepp et al., 1984; Pellis and Pellis, 2009). Rough-and-tumble play is essential for proper brain and behavioral development in rats (Pellis and Pellis, 2009; Vanderschuren and Trezza, 2013; Vanderschuren et al., 2016). Comprised of complex, fast-paced back-and-forth competition for access to each other’s nape using components such as pinning, wrestling, and chasing, social play is an intricate combination and coordination of behavior and communication that requires complex but finely timed interactions of numerous neural systems (Vanderschuren et al., 2016).
Acoustic communication through ultrasonic vocalizations (USV) is another essential factor in the social repertoire of rats. Juvenile and adult rats emit two main types of USV: aversive 22-kHz and appetitive 50-kHz calls. Aversive 22-kHz calls are associated with negative affective states and occur mainly in aversive contexts, such as exposure to predators or pain, but also during defensive and submissive displays in intermale fighting (Blanchard et al., 1991; Browning et al., 2017). On the other hand, 50-kHz USV reflect a positive affective state and is also known as “rat laughter” (Panksepp, 2005). Positive 50-kHz calls occur in appetitive and rewarding situations (Burgdorf et al., 2008; Brudzynski, 2013), most notably during rough-and-tumble play (Knutson et al., 1998). Moreover, systemic administration of psychoactive substances, such as amphetamine, can elicit 50-kHz USV at very high rates indicative of a mania-like state (Wöhr, 2022). During social play in rats, 50-kHz USV are important for facilitating and maintaining a playful mood (Himmler B. et al., 2014; Kisko et al., 2015b). Rats specifically bred for low emissions of 50-kHz USV show reduced social play behavior (Burgdorf et al., 2013), and devocalization studies show that in rats unable to emit USV, social play behavior is severely diminished (Kisko et al., 2015b).
Social behavior and USV in combination convey considerable information on the animal’s affective state and social motivation and how they respond to various social peers. Therefore, using social behavior in conjunction with USV seems to be a particularly suitable tool for studying genetic and environmental risk factors in rodent models for neuropsychiatric disorders, such as ASD. Regarding genetics, studies have shown that different rat strains display differences in play (Himmler B. et al., 2014; Lukas and Wöhr, 2015; Northcutt and Nwankwo, 2018). Moreover, breeding for different anxiety levels in rats can result in differences in play behavior (Himmler B. et al., 2014; Lukas and Wöhr, 2015; Northcutt and Nwankwo, 2018). Relating to the effects of the environment, studies show that in rats the availability and characteristics of play partners has an important role (Poole and Fish, 1975; Hol et al., 1999; van den Berg et al., 1999; Pellis et al., 2018; Stark and Pellis, 2020). For example, control rats living with devocalized cage mates play 50% less than control rats living with non-devocalized cage mates (Kisko et al., 2015b). Additionally, as adults, juvenile rats that were reared with play-deprived partners have deficits in social functions (Hol et al., 1999; van den Berg et al., 1999). These examples provide compelling evidence that the makeup of cage mates during the critical play period can influence social play, social communication and socio-cognitive skills needed in adult social interactions (Bell et al., 2010; Baarendse et al., 2013; Himmler S. et al., 2014; Kisko et al., 2015a; Schneider et al., 2016; Pellis et al., 2017).
Our heterozygous Cacna1c model exhibits several characteristics reminiscent of ASD-like social and communication deficits in juveniles and adults (Schwarting et al., 2018; Redecker et al., 2019; Kisko et al., 2020). We recently found a sex-dependent influence of Cacna1c haploinsufficiency on social behavior and emission of 50-kHz USV in juvenile rats (Kisko et al., 2020, 2021). While social play behavior was not affected in males, 50-kHz USV emission during social play was reduced in Cacna1c heterozygous (HET) rats compared to Cacna1c wildtype (WT) controls. In contrast, female HET pairs played much more than WT controls, but 50-kHz USV emission was comparable. Sex-dependent effects are not altogether surprising as there has been ample evidence for sex-specific effects of the risk gene CACNA1C in humans (Dao et al., 2010; Strohmaier et al., 2013; Heilbronner et al., 2015) and Cacna1c mouse models (Dao et al., 2010). Most strikingly, however, was a lack of general sex difference in play behavior between male and female WT rats (Kisko et al., 2020). Since all animals investigated were housed in cages with both HET and WT cage mates and exclusively played with same-genotype partners, effects due to the peers within their social environment are conceivable. Thus, the social behavior of WT females may be influenced by the presence of hyper-playful HET cage mates.
Studies in mice have shown that elements of the home cage social environment can interact with genotype to impact particular aspects of disorder-related behaviors in ASD mouse models (Yang et al., 2015). For example, social deficits in an ASD mouse model can be rescued by rearing them with a highly social mouse strain (Yang et al., 2011). Consequently, using our Cacna1c haploinsufficient rat model, we controlled the cage mate’s genotype and the play partner’s genotype in juvenile females. We then investigated the interplay between acute and long-term effects of the genetic makeup related to the social environment and social peers on rough-and-tumble play and concomitant emission of 50-kHz USV in Cacna1c haploinsufficient rats, as compared to wildtype littermate controls. Implications from our findings highlight the importance of the early environment as a factor modulating phenotypic changes in genetic rat models for neuropsychiatric disorders.
2. Materials and methods
2.1. Animals and genotyping
The interplay between acute and long-term effects of the genetic makeup related to the social environment and social peers was assessed using rough-and-tumble play and concomitant emission of 50-kHz USV in a group of juvenile female Cacna1c+/– (HET) rats (N = 40) and Cacna1c+/+ (WT) littermate controls (N = 40) by systematically manipulating the genotype of cage mates and the genotype of play partners, respectively.
As described in preceding studies (Kisko et al., 2018, 2020), HET rats were generated through zinc finger technology (Geurts et al., 2009) by SAGE Labs (now Envigo, Indianapolis, IN, USA) on a Sprague-Dawley (SD) background. HET rats carry a 4 base pair deletion at position 460 649-460 652 in genomic sequence, resulting in an early stop codon in exon 6. Genotyping was performed using DNA obtained from tail samples on a 3130xl Genetic Analyzer (Thermo Fisher Scientific, Waltham, MA, USA) with the following primers:
50-GCTGCTGAGCCTTTTATTGG-30 (Cacna1c Cel-1 F).
50-CCTCCTGGATAGCTGCTGAC-30 (Cacna1c Cel-1 R).
An established breeding protocol was applied to obtain HET and WT offspring, pairing SD females (Charles River, Sulzfeld, Germany) and male HET rats (Kisko et al., 2018). The day of birth was defined as postnatal day (PND) 0. Breeding was performed at the Faculty of Psychology, Philipps-University of Marburg, Germany.
Rats were identified by paw tattoo, using non-toxic animal tattoo ink (Ketchum permanent tattoo inks green paste, Ketchum Manufacturing Inc., Brockville, Canada). The ink was inserted subcutaneously through a 30-gauge hypodermic needle tip into the center of the paw on PND 3 ± 1. All procedures were conducted in strict accordance with the National Institutes of Health Guidelines for the Care and Use of Laboratory Animals and the relevant local or national rules and regulations of Germany and were subject to prior authorization by the local government (Tierschutzbehörde, Regierungspräsidium Gießen, Germany).
2.2. Experimental design
For our design we manipulated the genotype of the cage mate and the genotype of the play partner to fully assess the interplay between acute and long-term effects of the genetic makeup related to the social environment and social peers on rough-and-tumble play behavior and concomitant 50-kHz USV emission in juvenile Cacna1c haploinsufficient females.
2.2.1. Genotype of the cage mate (SAME- genotype vs. MIXED-genotype cage)
On the day of weaning (PND 21), juvenile females were separated into experimental housing conditions in which the genotype of the cage mate was manipulated. Experimental housing consisted of social housing in groups of 4–6 with same-sex littermates in either SAME-genotype cages (all WT females or all HET females) or MIXED-genotype cages (equal distribution of WT and HET females). All females were housed in polycarbonate Macrolon Type IV cages (Tecniplast Deutschland GmbH, Hohenpeißenberg, Germany; 58 cm × 38 cm × 20 cm, length × width × height) and kept under standard laboratory conditions (22 ± 2°C and 40–70% humidity) with free access to standard rodent chow and water.
2.2.2. Genotype of the play partner (WT vs. HET)
Forty WT and HET females from SAME- and MIXED-genotype cages were selected as focus rats and were matched with at first unfamiliar non-littermate WT and HET play partners. For each focus rat the genotype sequence of the play partners was counterbalanced so that they either played first with a WT play partner or with a HET play partner. Each focus rat played with both genotypes. Play partners were always from the same experimental housing condition as the focus rat. Play partners did not differ more than 10 g in weight.
2.2.3. Test sequence
Testing consisted of two play sequences, each composed of three play sessions that took place over consecutive days (Figure 1). During the first play sequence, the focus rat played with a WT or HET partner for three consecutive days. On the fourth day, the play partners were changed to counterbalance across subjects and then the second play sequence commenced for the next three consecutive days. Consequently, during days four to six, each focus rat interacted with the converse partner compared to the preceding the 3 days, e.g., Day 1–3: WT-WT; Day 4–6: WT-HET (Figure 1). Importantly, the focus rats were presented with the same play partner within each 3-day sequence.
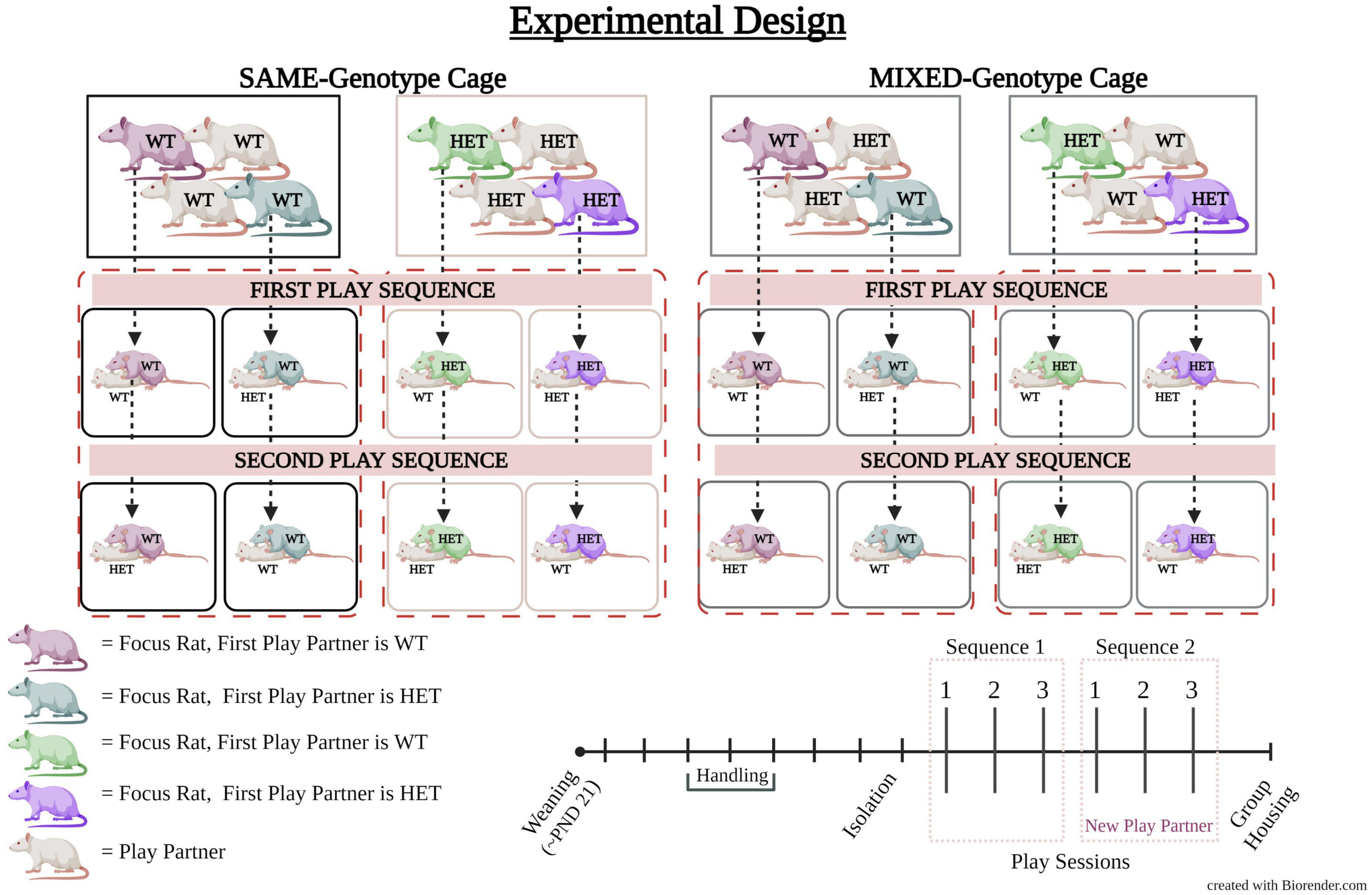
Figure 1. Experimental design of cage mate genotype and play partner genotype. The experimental design involved cages with female rats of the same genotype (SAME-genotype cage, shown on the left side). These cages consist of either all wildtype (WT) females (represented by black boxes) or all heterozygous (HET) females (represented by tan boxes). On the right side, there are the cages with a mix of genotypes (MIXED-genotype cages), with an equal representation of HET and WT females (represented by gray boxes). In the first play sequence, a focus rat is paired with either a WT or HET partner. In the second play sequence, the same focus rat interacts with a partner opposite to the one it played with in the first sequence. As indicated in the legend (bottom left) the color of each focus rat represents its first play partner. Play partners are shown in white. For example, the dark purple WT rat plays first with a WT play partner; the dark green WT rat plays first with a HET play partner; the purple HET rat plays first with a HET play partner; and the light green rat plays first with a WT play partner. Females from SAME-genotype cages always play with a partner from a SAME-genotype cage while females from MIXED-genotype cages always play with a partner from a MIXED-genotype cage. The timeline for the experiment is shown (bottom right) in which all animals were weaned around postnatal day (PND) 21 and put into manipulated housing conditions. All animals were handled and then isolated prior to the start of play testing. Play testing consisted of two play sequences each composed of three play sessions. A new play partner was given for the second play sequence. Following the last play session animals were placed back into group housing.
2.3. Rough-and-tumble play
Testing took place between PND 31 and 36. All rats were isolated the day prior to their first play session in a Makrolon type III cage (265 mm × 150 mm × 425 mm, plus high stainless-steel covers; Techniplast Deutschland GmbH) to enhance social motivation. In line with previous experiments (Kisko et al., 2018, 2020), play sessions were initialized by a 2-min anticipation phase, during which the focus rat habituated to the observation arena (35 cm × 35 cm, with Plexiglas walls; floor covered with 1 cm of fresh bedding). The anticipation phase was followed by a 5-min play phase, where focus and partner rats were allowed to socially interact. Before each play pair, the observation arena was cleaned thoroughly with an acetic acid solution (0.1%) and new bedding was added. Testing was conducted under red light conditions (∼28 lux). A digital camera (TK-1281 Color Video Camera, JVC, Yokohama, Japan) was used to record rats for behavioral analyses. Focus rats were marked with a non-toxic commercial marker, to enable the observer to distinguish the rats on the video.
Ultrasonic vocalizations recordings were taken during the play phase using UltraSoundGate Condenser CM16 Microphones connected to an UltraSoundGate 416H USB audio device (Avisoft Bioacoustics) placed 35 cm above the floor of the center of the observation arena. Briefly, acoustic data was recorded with a sampling rate of 250 000 Hz (recording range: 0–125 kHz; 16 bit) by Avisoft RECORDER USGH and transferred to Avisoft SASLab Pro for acoustical analysis. Start of behavioral recording was acoustically labeled by an audible beep signal from a stopwatch to synchronize audio and video recordings.
2.3.1. Analysis of social play behaviors
The following behavioral measures were scored as play components or non-play components by a trained observer blind to the experimental conditions using the Observer XT (Noldus, Wageningen, Netherlands): Play components included pinning, wrestling, chasing, evasion, self-pinning, crawl-over, biting and nape attack. Non-play components consisted of sniffing, physical contact, self-grooming and grooming of the partner (see Table 1 for detailed descriptions). While evasion, self-pinning, crawl-over and biting were observed, they did not occur with sufficient frequency or specific playful meaning to contribute to the findings or to allow meaningful statistical analysis. Therefore, apart from being added to the total duration of play behaviors, they were not analyzed or discussed further.
2.3.2. Analysis of USV
Using Avisoft SASLab Pro, 50-kHz and 22-kHz calls were manually counted for the entire 5-min play period in 20-s time bins by a trained observer. If two 50-kHz USV elements were at least 10 ms apart, two independent 50-kHz USV were counted. A frequency threshold of 32 kHz was defined to distinguish 50-kHz and 22-kHz USV. While atypical 50-kHz calls (Kisko et al., 2018) and 22-kHz calls were emitted, they did not occur with sufficient frequency and therefore were not analyzed or discussed further.
2.4. Statistical analysis
For comparing rough-and-tumble play behaviors and pro-social 50-kHz USV between genotype of the cage mates and genotype of the partner, analysis of variances (ANOVA) for repeated measurements were calculated with the between-subject factor genotype of the cage mates (H), genotype of the focus rat (G), genotype sequence of the play partner (i.e., WT or HET first) (SQ) and the within-subject factors genotype of the play partner (i.e., same or opposite) (P), play session (SE), rough-and-tumble play component (i.e., pinning, wrestling, chasing and nape attack) (C). Post hoc assessment of significant main effects was calculated using independent or paired t-tests, where appropriate. A significance threshold was set at p < 0.050.
3. Results
In the current study, we explored the interplay between acute and long-term effects of the genetic makeup related to the social environment and social peers on rough-and-tumble play and concomitant emission of 50-kHz USV during the critical developmental period in juvenile female rats. To this aim, we used a well-established genetic Cacna1c rat model and manipulated the genotype of the cage mates (SAME- vs. MIXED-genotype cages) and the genotype of the play partner (WT vs. HET play partner). Effects of Cacna1c haploinsufficiency were assessed in female constitutive heterozygous Cacna1c+/– rats (HET: N = 40) and compared to wildtype Cacna1c+/+ littermate controls (WT: N = 40).
3.1. Rough-and-tumble play
For juvenile WT and HET females, the amount of time spent engaged in rough-and-tumble play averaged across all play sessions was not dependent on the genotype of the cage mates, i.e., housing [PxH: F(1,32) = 0.000, p = 0.990]. Because the genotype of the cage mate had no effect on play, the following results (Figures 2, 3) depict pooled housing conditions. However, the duration of rough-and-tumble play was dependent on the genotype of the play partner [PxG: F(1,32) = 4.316, p = 0.046; Figure 2A and Table 2A]. Importantly, the effect of the play partner’s genotype on the time spent playing was strongly modulated by the sequence of the play partner (i.e., WT or HET play partner first).
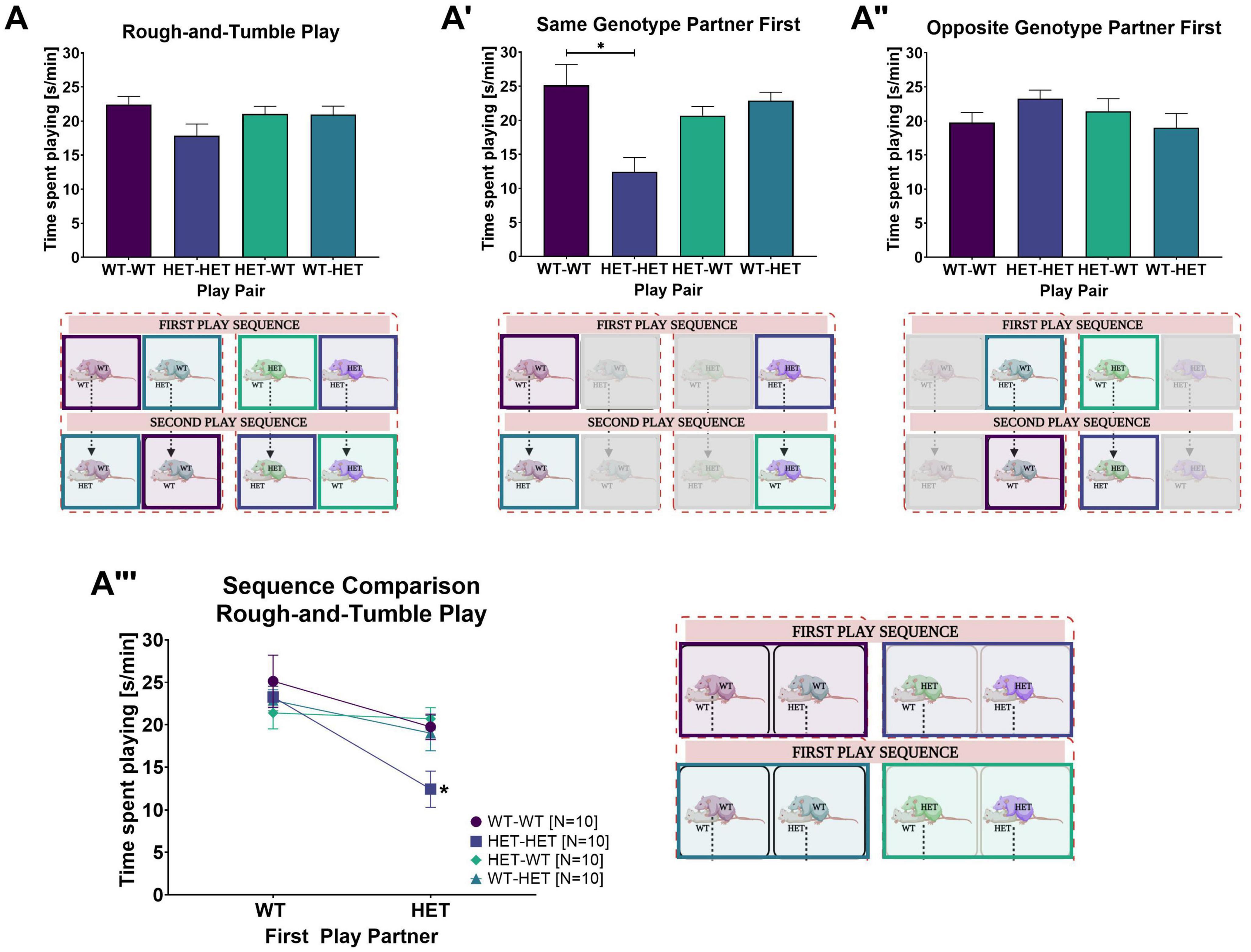
Figure 2. Rough-and-tumble play behavior and effects of the first play partner. The following data are pooled for genotype of the cage mate. Pair data included in the graphs are represented by the equivalent colored boxes surrounding the pairs of female rats in the representative design figure placed below or beside each graph (WT-WT = Dark Purple, HET-HET = Purple, HET-WT = Light Green, WT-HET = Dark Green, Greyed out boxes = not included). (A) The total averaged time spent playing for all six play sessions in pairs of female WT-WT (Dark purple bars, N = 20), HET-HET (Purple bars, N = 20), HET-WT (Light green bars, N = 20), and WT-HET (Dark green bars, N = 20). (A’,A”) The total averaged time spent playing across all three play sessions with a same- or opposite genotype play partner in pairs of female WT-WT (Dark purple bars, N = 10), HET-HET (Purple bars, N = 10), HET-WT (Light green bars, N = 10) and WT-HET (Dark green bars, N = 10). (A”’) The sequence comparison of time spent playing depending on genotype of the first play partner within female pairs of WT-WT (Dark purple circles, N = 10), HET-HET (Purple boxes, N = 10), HET-WT (Green diamonds, N = 10) and WT-HET (Dark green triangles, N = 10). Data are presented as mean + SEM. *p < 0.05.
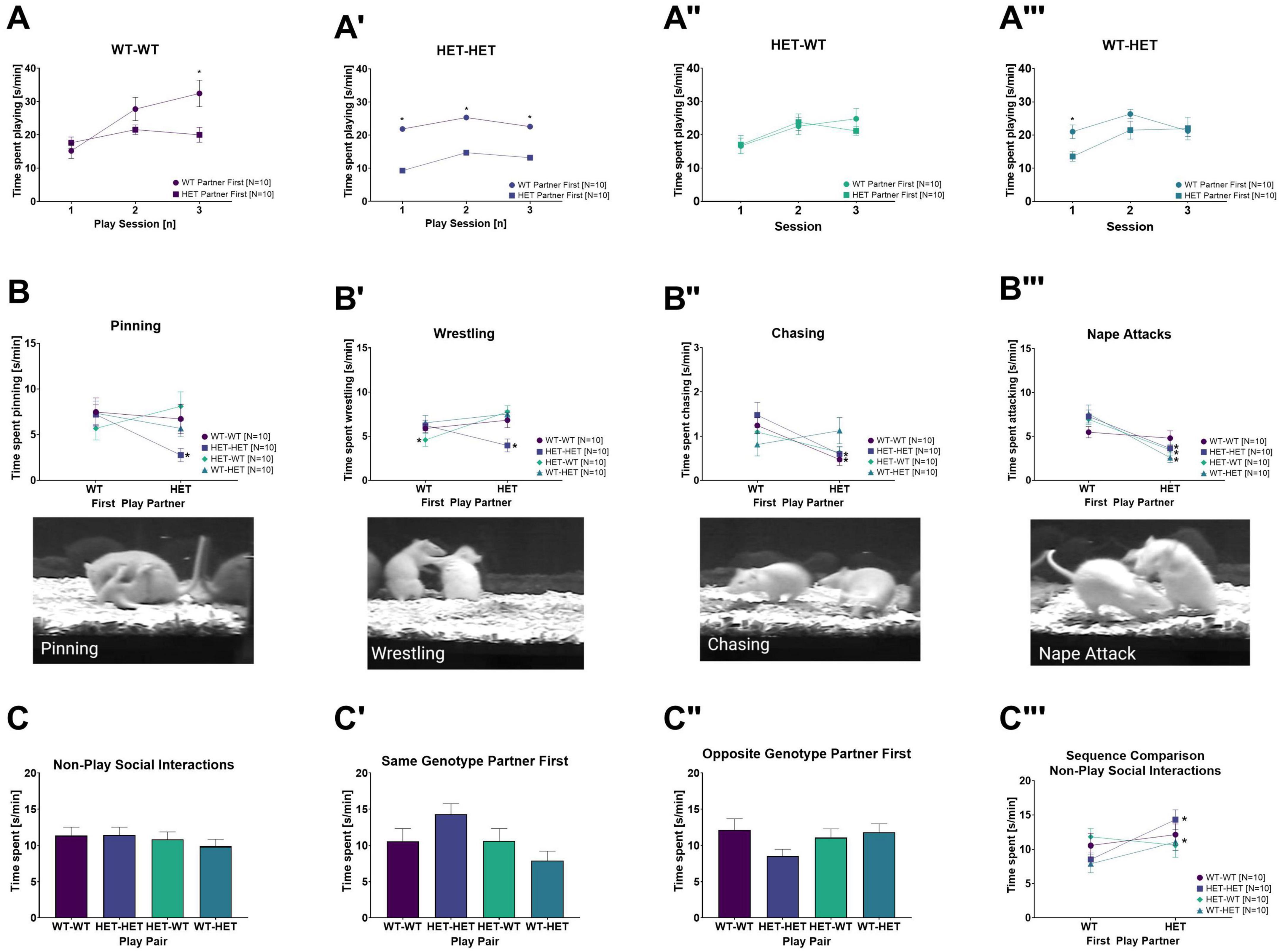
Figure 3. Rough-and-tumble play rough-and-tumble play components and non-play social interactions across play sessions. The following data are pooled for genotype of the cage mate. The total time spent playing across each individual play session depending on the genotype of the first play partner in (A) WT-WT, (A’) HET-HET, (A”) HET-WT and (A”’) WT-HET female pairs. The total time spent engaged and exemplary images for specific rough-and-tumble play components depending on genotype of the first play partner in female pairs of WT-WT (Dark purple circles), HET-HET (Purple boxes), HET-WT (Green diamonds) and WT-HET (Dark green triangles) for (B) pinning, (B’) wrestling, (B”) chasing and (B”’) nape attacks. (C–C”’) Non-play social interactions. (C) Total averaged time spent in non-play social interactions across all play session in pairs of female WT-WT (Dark purple bars, N = 20), HET-HET (Purple bars, N = 20), HET-WT (Light green bars, N = 20) and WT-HET (Dark green bars, N = 20). The total time spent averaged across play sessions in non-play social interactions with a (C’) same- or (C”) opposite genotype play partner in pairs of female WT-WT (Dark purple bars, N = 10), HET-HET (Purple bars, N = 10), HET-WT (Light green bars, N = 10), and WT-HET (Dark green bars, N = 10). (C”’) The sequence comparison of time spent in non-play social interactions depending on genotype of the first play partner in female pairs of WT-WT (Dark purple circles), HET-HET (Purple boxes), HET-WT (Green diamonds) and WT-HET (Dark green triangles). Data are presented as mean + SEM. *p < 0.05.
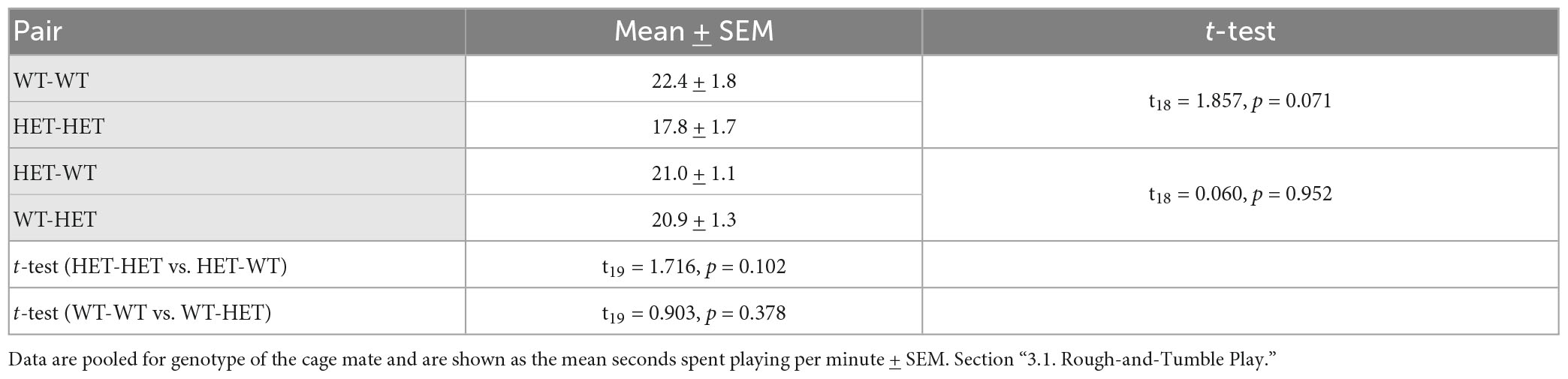
Table 2A. Post-hoc t-test results for rough-and-tumble play behavior in pairs of juvenile female HET and WT rats.
3.1.1. Effects of the first play partner on rough-and-tumble play
When the first play partner was the same genotype, the time spent playing was much lower in same-genotype HET pairs than same-genotype WT pairs (WT-WT vs. HET-HET: Table 2B and Figure 2A’). In contrast, there was no significant difference between same-genotype HET pairs and same-genotype WT pairs when the first play partner was the opposite genotype (WT-WT vs. HET-HET: Table 2B and Figure 2A”), suggesting that playing first with a WT partner leads to an increase in the time spent playing in same-genotype HET pairs.
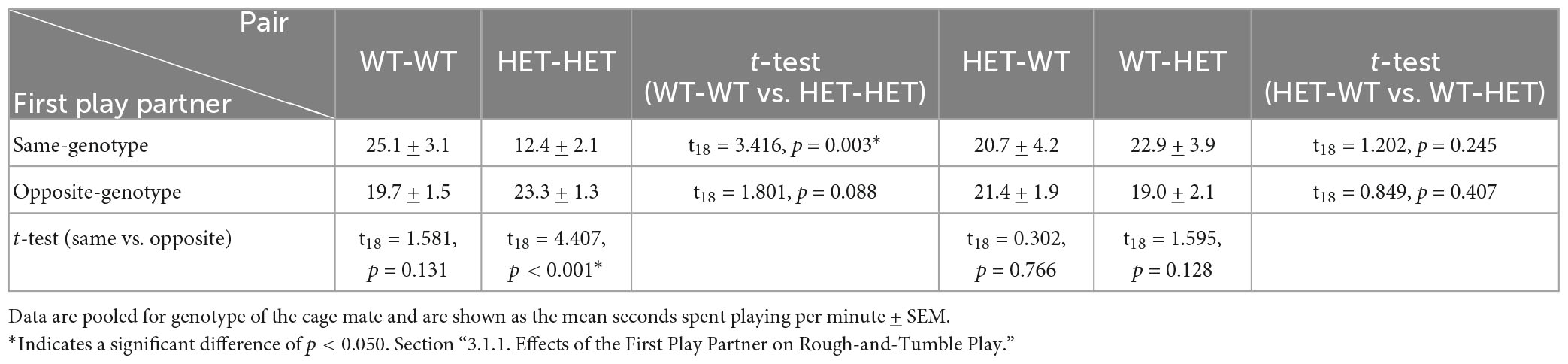
Table 2B. Post-hoc t-test results for rough-and-tumble play behavior in pairs of juvenile female HET and WT rats.
Indeed, the most important factor appears to be the sequence in which the focus rat plays with a same-or opposite-genotype partner [GxSQ: F(1,32) = 10.694, p = 0.003; PxGxSQ: F(1,32) = 6.682, p = 0.015]. A prominent rescue effect was found within same-genotype HET pairs. For same-genotype HET pairs the duration of play was higher when the first play partner was a WT compared to a HET (WT vs. HET: Table 2B and Figure 2A”’). In contrast, for same-genotype WT pairs, there was no difference in the time spent playing whether the first partner was a WT or a HET (WT vs. HET: Table 2B and Figure 2A”’). Within opposite-genotype pairs, no sequence effects were found (WT vs. HET: Table 2B and Figures 2A’–A”’). Of note, no main effects of genotype, housing, sequence, or other interactions were found (Supplementary Table 1A and Supplementary Figure 1C).
3.1.2. Rough-and-tumble play across play sessions
A closer inspection of the time spent playing across individual play sessions between same- and opposite-genotype pairs showed an increase of the time spent playing across play sessions [SE: F(2,64) = 26.584, p < 0.001]. For juvenile female WT and HET rats, the increase in play duration across sessions was dependent on the genotype and sequence of the play partners [PxSExG: F(2,64) = 5.185, p = 0.008; PxSExSQ: F(2,64) = 13.445, p < 0.001; PxSExGxSQ: F(2,64) = 3.731, p = 0.029, a representation of unsorted and pooled sequence data across all six play sessions is shown in Supplementary Figure 1A].
3.1.2.1. Effects of the first play partner on rough-and-tumble play across play sessions
In HET females playing with a same-genotype partner (HET-HET), the duration of playful interactions was nearly 50% higher in each individual play session (i.e., session 1, session 2, and session 3) when the first play partner was a WT compared to a HET (Table 2C and Figure 3A’). In HET females playing with an opposite-genotype partner (HET-WT), the time spent playing across sessions did not differ dependent on first play partner’s genotype (Table 2C and Figure 3A”).
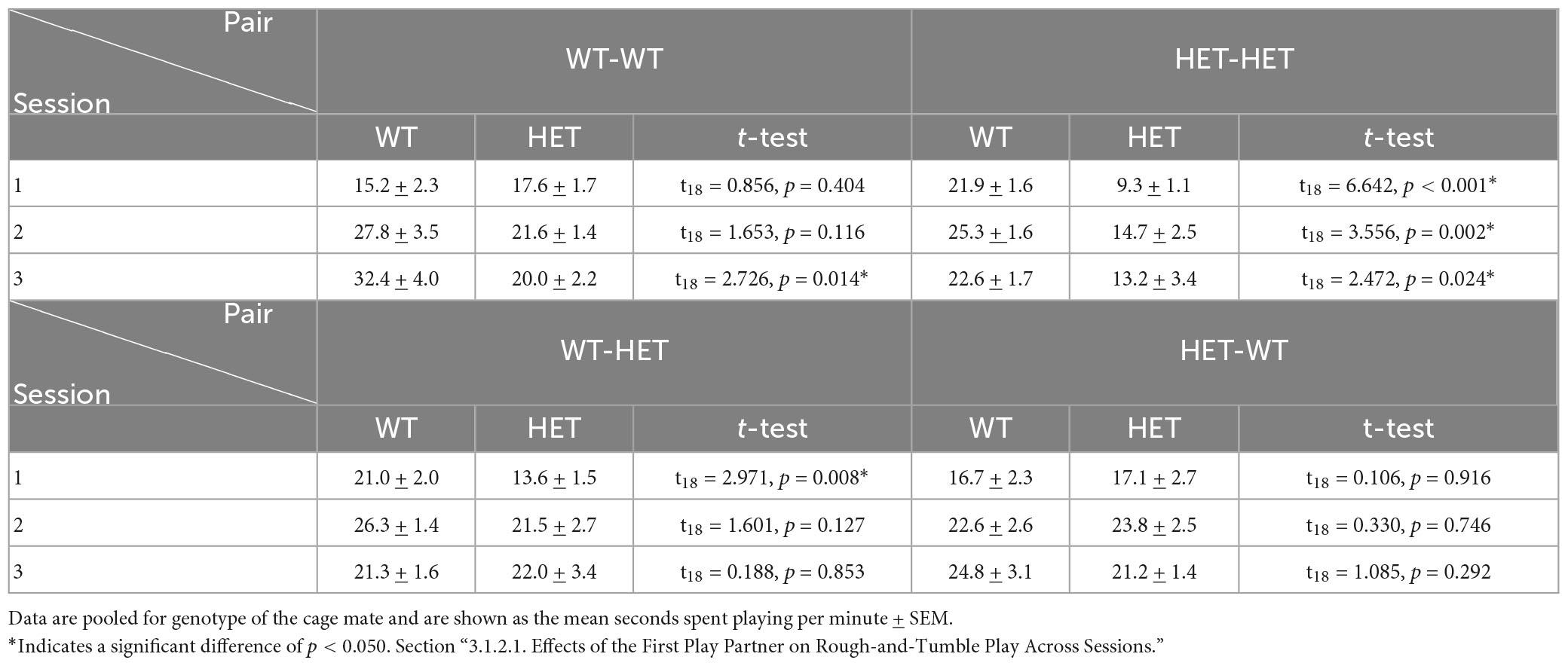
Table 2C. Post-hoc t-test results for rough-and-tumble play behavior in pairs of juvenile female HET and WT rats.
In WT females playing with a same-genotype partner (WT-WT), more time was spent playing during the third play session when the first play partner was WT compared to a HET, but no effects were present in the first or second play sessions (Table 2C and Figure 3A). In WT females playing with an opposite genotype partner (WT-HET), less time was spent playing only in the first play session when the first play partner was a HET compared to a WT (Table 2C and Figure 3A”’).
3.1.3. Rough-and-tumble play components
Detailed analysis of the specific play components showed that time spent pinning was most prevalent, followed by wrestling, nape attacks and chasing [C: F(3,108) = 60.678, p < 0.001]. Genotype of the play partner (i.e., same- or opposite-genotype) did not affect the prevalence of the individual play components [P: F(1,36) = 2.017, p = 0.164], yet there was an effect for the sequence of the play partner [PxSQ: F(1,36) = 19.773, p < 0.001]. Moreover, an interaction between genotype of the play partner and genotype of the focus rat and sequence of play partner was also found [PxGxSQ: F(1,36) = 5.375, p = 0.026]. Importantly, the duration for specific play components was dependent on the sequence of the play partner [CxGxSQ: F(3,108) = 6.309, p < 0.001; PxCxSQ: F(3,108) = 2.720, p = 0.048; PxCxGxSQ: F(3,108) = 4.718, p = 0.004].
3.1.3.1. Effects of the first play partner on rough-and-tumble play components
Like the duration of rough-and-tumble play, in HET females playing with a same-genotype partner (HET-HET), the duration of all playful components (i.e., pinning, wrestling, chasing, and nape attacks) was about 50% higher when the first play partner was a WT compared to a HET (Figures 3B–B”’ and Table 2D). When a HET female was playing with an opposite-genotype partner (HET-WT), less time was spent wrestling and more time was spent attacking the partner’s nape when the first play partner was a WT compared to a HET (Figures 3B–B”’ and Table 2D), while the duration of pinning and chasing was unaffected by the genotype of the first play partner.
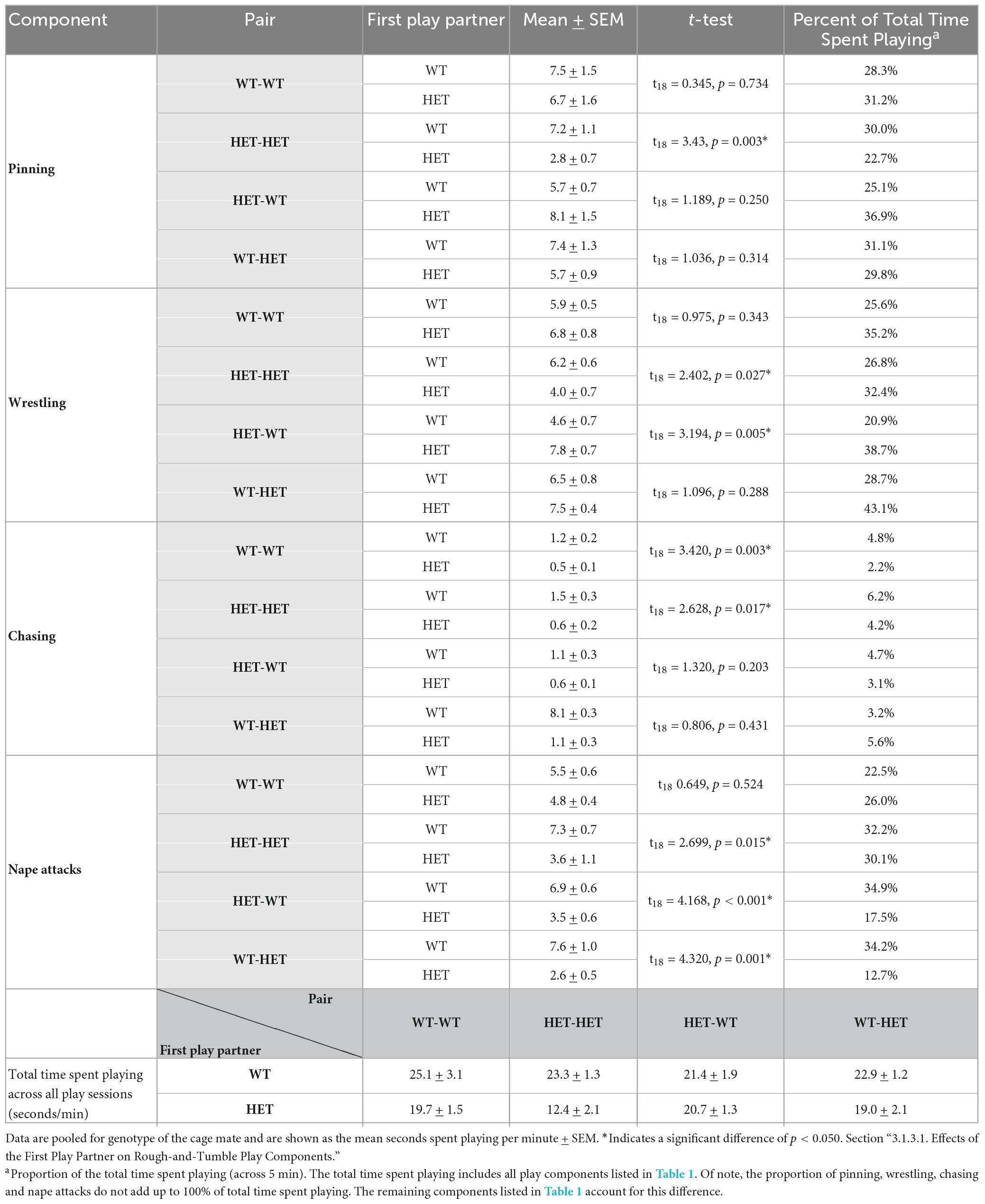
Table 2D. Post-hoc t-test results for rough-and-tumble play behavior in pairs of juvenile female HET and WT rats.
For WT females, only one play component appeared to be affected by the sequence of play partner. For same-genotype WT pairs (WT-WT), the duration of chasing was lower when the first play partner was a HET compared to a WT (Figures 3B–B”’ and Table 2D). When WT females played with an opposite-genotype partner (WT-HET), the time spent attacking the nape was reduced when the first play partner was a HET compared to a WT. The remaining play components were unaffected (Figures 3B–B”’ and Table 2D).
Between subjects, effects for rough-and-tumble play components between same- and opposite-genotype pairs showed an interaction effect of focus rat genotype and sequence of the play partner [GxSQ: F(1,36) = 9.889, p = 0.003] but no main effect for the genotype of the focus rat or sequence of the play partner was observed [G: F(1,36) = 1.672, p = 0.204; SQ: F(1,36) = 0.329, p = 0.570].
3.2. Non-play social interactions
Within play sessions, the duration of non-play social interactions in WT and HET females depended on the play partner’s sequence [PxSQ: F(1,32) = 7.909, p = 0.008]. However, with a closer look at the duration of non-play social behavior between pairs playing first with a same- or an opposite-genotype partner, no differences were found and are therefore not reported (Figures 3C–C”). As there were no significant differences in the duration of non-play social behaviors in same-and opposite-genotype pairs apart from being added to the total duration of non-play social interactions, individual components (Table 1) were not analyzed or discussed further. Additionally, the genotype of the cage mate had no effect on non-play social interactions and therefore the following results and representative figures (Figures 3C–C”’) are pooled for housing composition.
3.2.1. Effects of the first play partner on non-play social interactions
When comparing the sequence of play partners within same- and opposite-genotype pairs for non-play social interactions, we observed that in HET females playing with a same-genotype partner (HET-HET) and WT females playing with an opposite-genotype partner (WT-HET), more time was spent in non-play social interactions when the first play partner was HET compared to WT (Table 2E and Figure 3C”’). No other effects of the first play partner were found in WT females playing with a same-genotype play partner (WT-WT) or in HET females playing with an opposite-genotype partner (HET-WT).
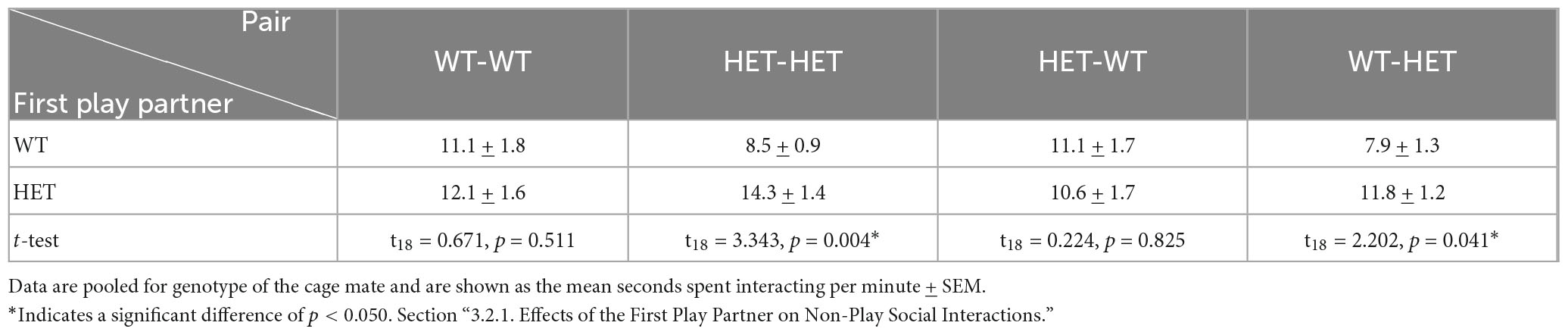
Table 2E. Post-hoc t-test results for rough-and-tumble play behavior in pairs of juvenile female HET and WT rats.
Between subjects, effects showed that the duration of non-play social interactions was dependent on the focus rats genotype and the play partner’s sequence [GxSQ: F(1,32) = 4.610, p = 0.039]. However, the main effects for the genotype of the focus rat, the genotype of the cage mates and the genotype and sequence of the play partner did not affect non-play social interactions (Supplementary Table 1B).
3.3. Rough-and-tumble induced 50-kHz USV
In slight contrast to rough-and-tumble play behavior, the total 50-kHz USV emission averaged across all play sessions in WT and HET females was not dependent on the genotype of the play partner [PxG: F(1,31) = 0.717, p = 0.404, Figure 3A]. Instead, the emission of 50-kHz USV during rough-and-tumble play in same- and opposite-genotype pairs was dependent on the play partner’s sequence [PxSQ: F(1,31) = 20.460, p < 0.001]. The genetic composition of the cage mates had no effect on the total averaged number of 50-kHz USV emissions across all play sessions. Accordingly, the post-hoc t-test results (Table 3A) and representative figures (Figures 4, 5B–B”’) for 50-kHz USV are pooled housing conditions.
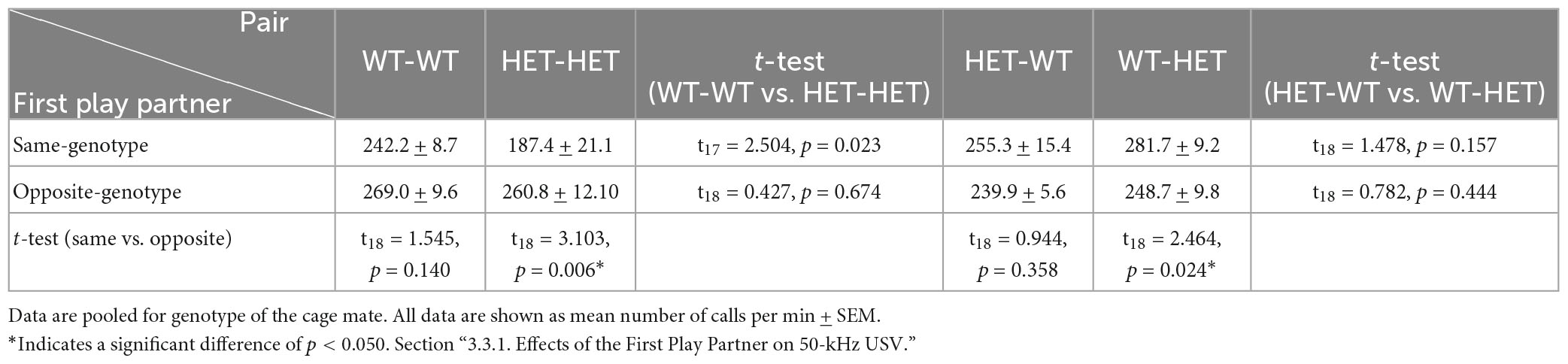
Table 3A. Post-hoc t-test results for Rough-and-Tumble Play induced 50-kHz USV in pairs of juvenile female HET and WT rats.
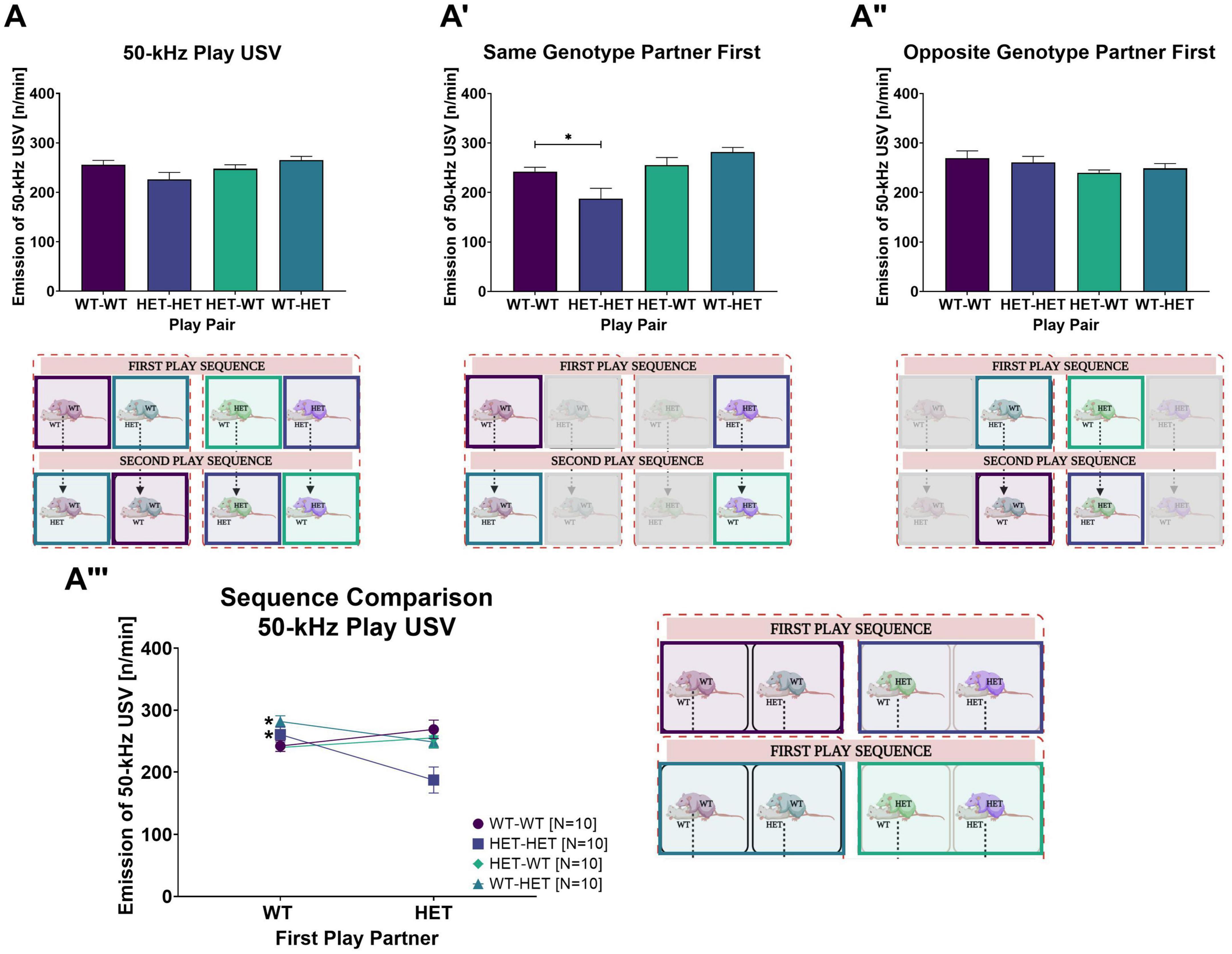
Figure 4. Rough-and-Tumble induced 50-kHz USV. The following data are pooled for genotype of the cage mate. Pair data included in the graphs are represented by the equivalent-colored boxes surrounding the pairs of female rats in the representative design figure placed below or beside each graph (WT-WT = Dark Purple, HET-HET = Purple, HET-WT = Light Green, WT-HET = Dark Green, Greyed out boxes = not included). (A) The total 50-kHz USV emissions averaged across all sessions in pairs of female WT-WT (Dark purple bars, N = 20), HET-HET (Purple bars, N = 20), HET-WT (Light green bars, N = 20) and WT-HET (Dark green bars, N = 20); (A’,A”) The number of 50-kHz USV emission (n/min) averaged across all sessions while playing with a same- or opposite genotype play partner in pairs of female WT-WT (Dark purple bars, N = 10), HET-HET (Purple bars, N = 10), HET-WT (Light green bars, N = 10) and WT-HET (Dark green bars, N = 10). (A”’) The sequence comparison of 50-kHz USV (n/min) emissions averaged across all sessions during social play depending on genotype of the first play partner in female pairs of WT-WT (Dark purple circles), HET-HET (Purple boxes), HET-WT (Green diamonds), and WT-HET (Dark green triangles). Data are presented as mean + SEM. *p < 0.05.
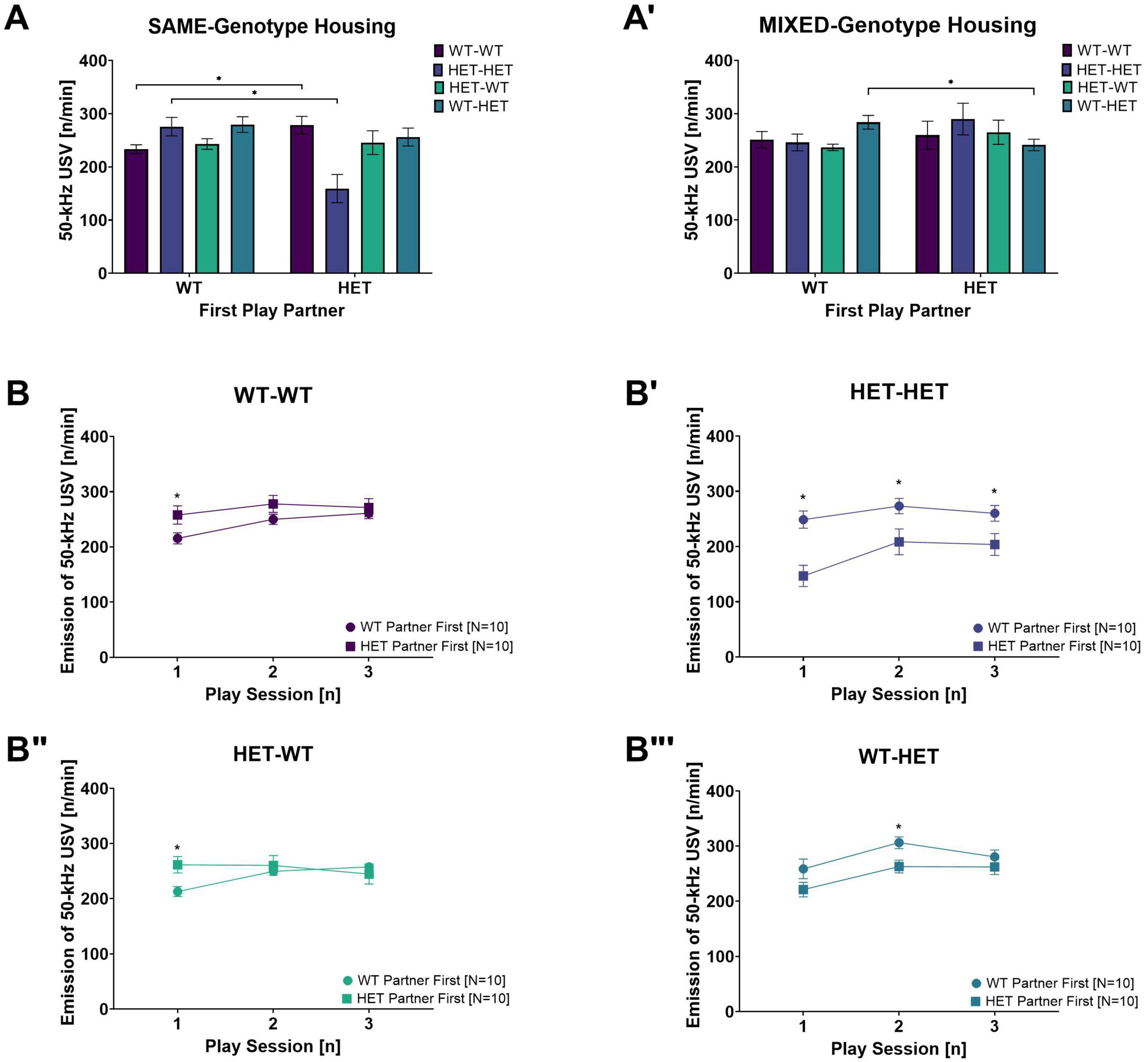
Figure 5. Rough-and-Tumble Induced 50-kHz USV across sessions and effects of the first play partner and housing. The total number of 50-kHz USV emissions per min averaged across all play sessions depending on the genotype of the first play partner in (A) SAME-genotype housing and (A’) MIXED-genotype housing for pairs of female WT-WT (Dark purple bars, N = 5), HET-HET (Purple bars, N = 5), HET-WT (Light green bars, N = 5) and WT-HET (Dark green bars, N = 5). (B–B”’) Data are pooled for genotype of the cage mate. The emission of 50-kHz USV across each individual play session depending on genotype of the first play partner (WT partner first = circles and HET partner first = squares) in female pairs of (B) WT-WT, (B’) HET-HET, (B”) HET-WT and (B”’) WT-HET; Data are presented as mean + SEM. *p < 0.05.
3.3.1. Effects of the first play partner on 50-kHz USV
When the first play partner was the same genotype, the total 50-kHz USV averaged across all play sessions was lower in same-genotype HET pairs than same-genotype WT pairs (WT-WT vs. HET-HET: Table 3A and Figure 4A’). No effects of the first play partner on 50-kHz USV emissions was found in opposite-genotype pairs (WT-HET vs. HET-WT, Table 3A and Figure 4A”). Like play behavior, playing with a WT play partner appears to rescue the diminished 50-kHz USV emissions during rough-and-tumble play in same-genotype HET pairs.
Within each pair constellation, we saw that for same-genotype HET females (HET-HET), the decrease in 50-kHz USV was rescued when the first play partner was WT compared to the HET (WT vs. HET: Table 3A and Figure 4A”’). Notably, for pairs of HET females playing with a WT play partner (HET-WT), there was no differences in 50-kHz USV emissions, even if the first play partner was a HET compared to a WT (WT vs. HET: Table 3A and Figure 4A”’), strongly indicating that playing with a WT play partner is necessary for normalizing 50-kHz USV emissions during rough-and-tumble play in juvenile HET females. For WT females playing with a same-genotype partner (WT-WT), playing first with a WT or a HET does not affect the emission of 50-kHz USV (WT vs. HET: Table 3A and Figure 4A”’). However, in WT females playing with opposite-genotype play partners (WT-HET) higher 50-kHz USV emissions were seen when the first play partner was a WT compared to a HET (WT vs. HET: Table 3A and Figure 4A”’).
Between-subject comparisons showed a main genotype effect [G: F(1,31) = 6.785, p = 0.014], with fewer 50-kHz calls associated with HET females compared to WT females. Furthermore, an interaction effect was observed between the cage mates’ genotype and the play partner’s sequence [HxSQ: F(1,31) = 4.397, p = 0.044].
3.3.2. Effects of the first play partner and genotype of the cage mate on 50-kHz USV
In SAME-genotype cages, the emission of 50-kHz calls in same-genotype pairs was higher when the first play partner was the opposite-genotype for both same-genotype WT and same-genotype HET pairs (WT-WT and HET-HET: Table 3B and Figure 5A). In contrast, no difference in 50-kHz USV emissions was observed in opposite-genotype pairs due to the sequence of play partner (WT-HET and HET-WT: Table 3B and Figure 5A). In MIXED-genotype cages, the opposite effect was found, same-genotype pairs showed no difference because of the sequence of the play partner (WT-WT and HET-HET: Table 3B and Figure 5A’), but opposite-genotype pairs showed higher 50-kHz USV emissions when their first play partner was of the same genotype (WT-HET and HET WT: Table 3B and Figure 5A’). The effect appears to be driven by opposite-genotype WT (WT-HET) pairs as the opposite-genotype HET (HET-WT) pairs, from MIXED-genotype cages, show no differences in 50-kHz USV emissions due to the first play partner (Table 3B and Figure 5A’).
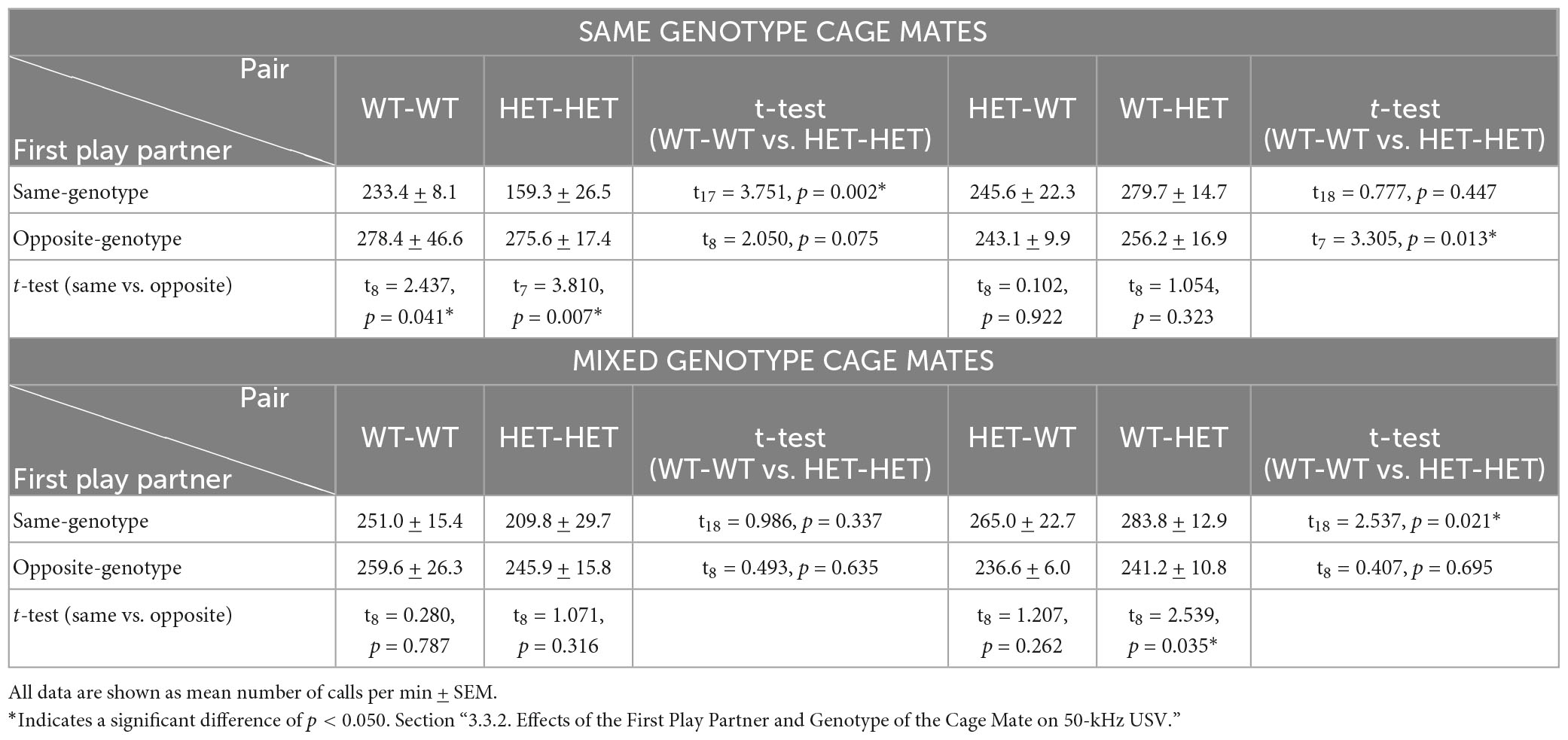
Table 3B. Post-hoc t-test results for Rough-and-Tumble Play induced 50-kHz USV in pairs of juvenile female HET and WT rats.
No main effect for the genotype of the cage mate or sequence of the play partner was found for same- and opposite-genotype pairs in the emission of 50-kHz USV (Supplementary Table 1C and Supplementary Figure 1D).
3.3.3. 50-kHz USV across play sessions
Detailed investigation of the emission of 50-kHz USV across individual play sessions between same- and opposite-genotype pairs showed an increase of 50-kHz USV across all play sessions [SE: F(1,62) = 36.819, p < 0.001].
3.3.3.1. Effects of the cage mates genotype on 50-kHz USV across play sessions
The emission of 50-kHz USV across play sessions further showed an interaction effect for cage mate’s genotype, the focus rat’s genotype, and the play partner’s sequence [SExHxGxSQ: F(1,62) = 3.671, p = 0.031]. In particular, the effect appears to be driven by higher emission rates of 50-kHz USV when the first play partner was the opposite-genotype in females with SAME-genotype cage mates. The effect is most prominent in the same-genotype HET pairs, which produced more rough-and-tumble 50-kHz calls when the first play partner was a WT (HET-HET: Table 3C). While in same-genotype WT pairs this effect was not significant (WT-WT: Table 3C). In pairs of juvenile females from MIXED-genotype cages, the emission of 50-kHz USV in same- and opposite-genotype pairs is roughly equivalent whether playing with the same-or opposite-genotype partner across most play sessions (Table 3C).
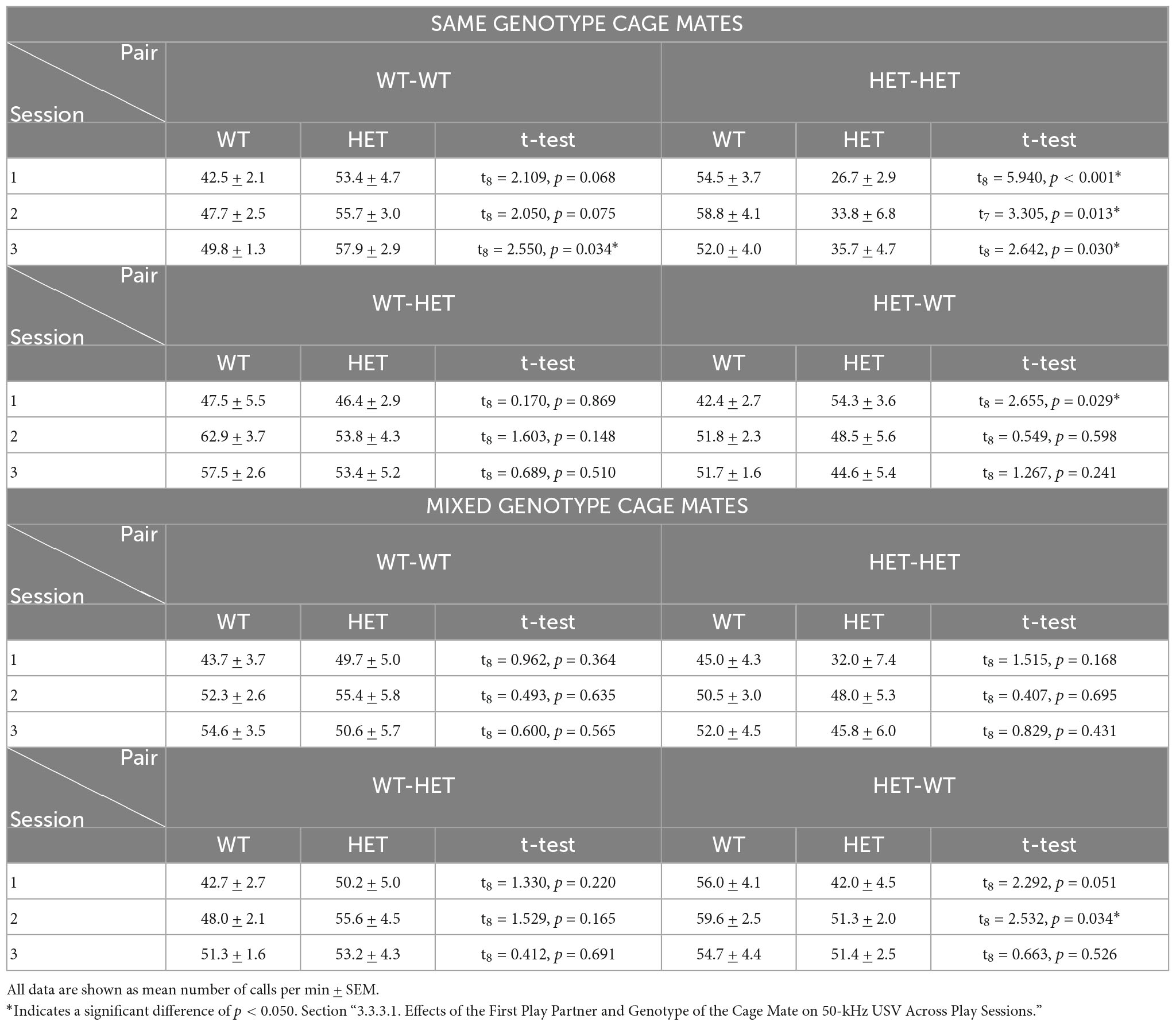
Table 3C. Post-hoc t-test results for Rough-and-Tumble Play induced 50-kHz USV in pairs of juvenile female HET and WT rats.
3.3.3.2. Effects of the first play partner on 50-kHz USV across sessions
Like play behavior, the emission of 50-kHz USV across individual play sessions was dependent on the play partner’s genotype and sequence [PxSExSQ: F(2,62) = 8.647, p < 0.001]. In same-genotype pairs (WT-WT and HET-HET), the emission of 50-kHz USV appears to be higher when the first play partner was the opposite-genotype compared to the same-genotype. Specifically, when the first play partner was a WT, the HET females playing with same-genotype partners showed higher 50-kHz USV emissions across three all play sessions (HET-HET: Table 3D and Figure 5B’). For same-genotype WT pairs a difference in emission of 50-kHz USV was evident only during the first play session when the first play partner was a HET compared to a WT (WT-WT: Table 3D and Figure 5B).
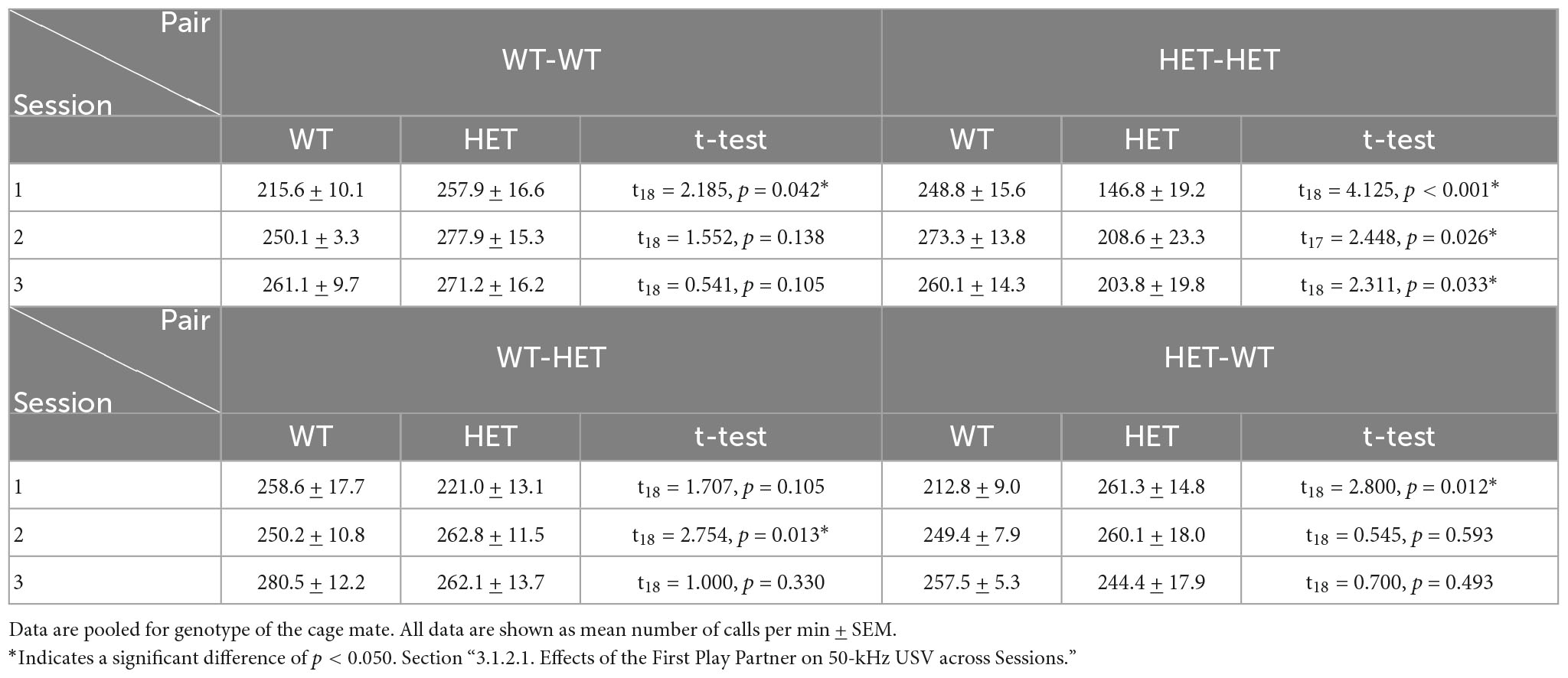
Table 3D. Post-hoc t-test results for Rough-and-Tumble Play induced 50-kHz USV in pairs of juvenile female HET and WT rats.
In contrast, the opposite-genotype pairs (WT-HET and HET-WT) showed higher 50-kHz USV emissions across each individual play session when the first play partner was the same-genotype. Within the pair constellations, the HET females playing with an opposite-genotype partner showed higher 50-kHz USV emissions only during the first play session, when the first play partner was a HET compared to a WT (HET-WT: Table 3D and Figure 5B”). In WT females playing with an opposite-genotype partner, there was a difference in 50-kHz USV emissions only during the second play session (WT-HET: Table 3D and Figure 5B”’).
4. Discussion
This study examined the interplay between acute and long-term effects of the genetic makeup related to the social environment and social peers on rough-and-tumble play and concomitant 50-kHz USV emission in juvenile female Cacna1c haploinsufficient rats and wildtype littermate controls. In contrast to what we expected based on our previous study (Kisko et al., 2020), we found that the cage mate’s genotype and the focus rat’s genotype had no main effects on play behavior and 50-kHz USV. What we found to be more important is the genotype and sequence of the play partner. Specifically, we found that same-genotype HET play pairs engage less in play behavior and emit fewer 50-kHz USV than same-genotype WT play pairs when first playing with a HET partner. Importantly, WT play partners can rescue social play and 50-kHz USV deficits in same-genotype HET pairs. The prominent rescue effect in HET rats by WT play partners indicates that early social environment and social peers significantly influence social behavior and communication development in juvenile female Cacna1c haploinsufficient rats.
4.1. Rough-and-tumble play
Play behavior is essential to development (Pellis and Pellis, 2009; Vanderschuren and Trezza, 2013; Vanderschuren et al., 2016). Juvenile rats are highly motivated to play and in the present study all pairs engaged in rough-and-tumble play, suggesting that motivation to play was present both in Cacna1c haploinsufficient females and wildtype littermate controls, irrespective of genetic makeup related to the social environment and social peers. However, we saw a reduced amount of time playing in same-genotype HET pairs, particularly if their first play partner was a HET. Interestingly, the time spent playing was rescued to levels equivalent to the same-genotype WT control pairs when a HET female played with a WT play partner regardless of sequence. Notably, this was evident not only in the average duration of play across all play sessions but also within each individual play session and relevant play components, i.e., pinning, wrestling, chasing and nape attacks. The WT females, therefore, can enhance the playful motivation of their HET play partners acutely and long-term, as the effect is persistent when the subsequent play partner is another HET.
The influence of the WT female rats on the HET contrasts our initial hypothesis that the hyper-playful HET females were responsible for increasing the playful motivation of their WT cage mates (Kisko et al., 2020). In rats, play partners and early social environment can affect the overall playful and social characteristics established during the critical period (Varlinskaya et al., 1999; Burgdorf et al., 2005; Himmler S. et al., 2014; Kisko et al., 2015a,b; Burke et al., 2017; Redecker et al., 2019; Stark and Pellis, 2020). Devocalization studies have shown that control rats living with devocalized cage mates spent less time playing than controls housed with other controls (Kisko et al., 2015b). Additionally, when rats are reared with adults or non-playful cage mates as juveniles, they show impairments in socio-cognitive functions (Bell et al., 2010; Baarendse et al., 2013; Himmler S. et al., 2014; Schneider et al., 2016; Pellis et al., 2017; Stark and Pellis, 2020) and are less socially competent when navigating adult social interactions (Kisko et al., 2015a; Stark and Pellis, 2020). In the current study, manipulating the post-weaning social environment had no significant main effects on the time spent playing for pairs of juvenile female rats. While it is important to acknowledge that the post-weaning social environment could have potentially influenced the individual play partners, it would be remiss not to consider its impact. During rough-and-tumble play in situations where it is possible, participants adapt their play behavior to their partner (Varlinskaya et al., 1999; Burgdorf et al., 2005; Himmler S. et al., 2014; Kisko et al., 2015b). In this manner, each partner benefits from the interaction. In adult Cacna1c haploinsufficient rats, we have found that the HET females will adapt their social behavior based on their partner’s genotype (Redecker et al., 2019). Our results indicate that the juvenile females adjust their behavior to suit their play partner’s genotype. However, it would be important to examine each playmate closely, particularly in opposite-genotype pairs. Additionally, the sequence in which the partners genotypes are presented plays a role in determining how playful the pair will be. For instance, HET females appear to be more playful when the first play partner was a WT, while WT females show more subtle effects when playing first with a HET play partner, i.e., spending less time playing only in certain play sessions and within select play components.
4.2. Non-play social behavior
In same-genotype HET and opposite-genotype WT pairs, there is an increase in non-play social behaviors when the genotype of the first play partner was a HET. In a study by Varlinskaya et al. (1999), the authors showed that in juvenile rats, the isolated, hyper-playful partner would adapt their behavior if their partner was reluctant to play and instead engage in more non-play-directed social behaviors (Varlinskaya et al., 1999). Therefore, it may be that the HET females are not as motivated to engage in rough-and-tumble play behaviors but rather prefer more non-play social interactions. If this were the case, we would also expect to see no difference in 50-kHz USV emissions, especially during periods of play, compared to non-play social interactions. At this point, however, we cannot say whether the HET individual drives the duration of non-play social interactions. We also cannot say during which behaviors 50-kHz USV are emitted, suggesting a more in-depth look and a detailed assessment of the individual play partners in combination with 50-kHz USV emissions.
4.3. 50-kHz rough-and-tumble USV
Like play behavior, the sequence of play partner affected the emission of 50-kHz USV in same-genotype HET pairs. When the first play partner was a WT, the same-genotype HET pairs emitted more 50-kHz USV and consequently showed no difference to the same-genotype WT control pairs. For the opposite-genotype pairs, the sequence of play partner had no effect on 50-kHz USV emissions and as such were comparable to same-genotype WT control pairs. Thus, for juvenile female rats the WT play partner appears to increase the emission of 50-kHz USV to control levels and appears to rescue deficits in 50-kHz USV emission in same-genotype HET pairs. Higher 50-kHz USV emissions in the same-genotype HET pairs after playing first with a WT or in opposite-genotype pairs are not altogether surprising, as they also spend more time playing. It is well-known that high rates of social play are associated with high rates of 50-kHz USV emissions in juvenile rats (Knutson et al., 1998; Burgdorf et al., 2005).
For same-genotype WT pairs, we again see mild effects of the first play partner. In fact, sequence effects are only evident within individual play sessions for 50-kHz USV emissions in WT females playing with a same- or opposite-genotype partner. Specifically, during the first play session same-genotype WT pairs emit more 50-kHz USV when the first play partner was a HET compared to a WT. In opposite-genotype WT pairs 50-kHz USV are reduced when the first play partner was a HET compared to WT, particularly within the second play session. The findings in WT pairs with the same and opposite-genotype partners are not too surprising because they can be attributed to the influence of the first play partner and the lasting effects it may have on play behavior. For instance, in half of the same-genotype WT pairs, the first play partner is another WT, while in the other half, they initially played with a partner of different genotype (HET). It is understandable, therefore, that they might be accustomed to playing with the HET partner in such cases. The reason for this is that the difference in behavior only occurs during the first play session. We can speculate that WT females may produce more 50-kHz USV initially to create a playful atmosphere possibly out of habitual tendency, even when they are paired with a new play partner. However, in subsequent sessions, they learn that this behavior is not necessary. Regarding the opposite-genotype WT pairs, the difference in 50-kHz USV emissions occurs only in the second play session. This might also be attributed to the influence of the previous play partner. In half of the pairs, the first play interaction involves a HET partner, whereas in the other half, they have already played with a WT partner for 3 days. As a result, they may still be highly motivated to play and, therefore, emit more 50-kHz USV to encourage their new HET play partner to engage in higher levels of playful behavior.
We know from devocalization studies that a vocal rat playing with a devocalized partner will increase the emission of 50-kHz USV to be comparable to pairs of two vocal rats leading to an increase in the playful mood and higher rates of play (Burke et al., 2017). Although, there is no way to say if the WT females are calling more than the HET females within the opposite-genotype pairs. However, it does not seem so unlikely based on the lower emission rates in same-genotype HET pairs.
Interestingly, while the genotype of cage mates did not affect play behavior, we found an interaction between the genotype of the cage mate and the sequence of the first play partner within 50-kHz USV emissions. In the females from SAME-genotype cages, the emission of 50-kHz USV was higher when the first play partner was the opposite genotype. Sequence effects in SAME-genotype cages are interesting as they reinforce the impression that WT females emit more 50-kHz USV when paired with HET play partners and that the HET females may adapt not only their behavior but also their social communication to complement the play partner leading to an increase in play and 50-kHz USV emissions in the subsequent play partners. In the MIXED-genotype housing condition, we see only an effect in the WT-HET pairs with higher 50-kHz USV when playing with a same-genotype partner first, which is likely driven by the increase in 50-kHz USV during the second play session. Thus, while not a primary effect, the genotype of the cage mate is not wholly unimportant as it provides the initial play experiences with and for both genotypes. For HET females, this is particularly important as it may rescue deficits that would be more prominent if housed with only other SAME-genotype HET cage mates.
There is strong evidence for social learning from conspecifics in various species, including rats (Carcea and Froemke, 2019). This includes social learning of food preferences (Galef Bennet, 2012) and observational fear learning (Kim et al., 2019). Moreover, we recently obtained evidence suggesting that juvenile rats may learn the appropriate use of USV within social contexts from their cage mates and play peers (Kisko et al., 2015a). By removing the ability to produce USV through devocalization, we found that devocalized juvenile rats reared with only other devocalized cage mates are not able to appropriately navigate ambiguous social interactions as adults, leading to increased aggression (Kisko et al., 2015a). In the present study, it is possible that the HET females may have learned from their WT cage mates, and vice versa.
Studies in mice have shown that social deficits in an ASD mouse model can be rescued by rearing them with a highly social mouse strain (Yang et al., 2011). From our study, similar effects might also occur in genetic rat models. The early social environment, through social peers and genotype of the cage mates, influences social communication in our Cacna1c juvenile HET and WT females. It provides further evidence to support findings in mice (Yang et al., 2015) that elements from the social environment, particularly the home cage, can interact with genotype to impact particular aspects of a disease model. Our findings reinforce the importance of the early social environment as a factor in phenotypic changes modulating genetic rat models of neuropsychiatric disorders.
In contrast to the current study, Yang et al. (2015) showed that mutant mice reared in mixed-genotype cages had fewer social interaction-induced USV than the wildtype controls, yet the mutant mice and wildtype controls reared in same-genotype cages did not differ in social interaction-induced USV emissions. The authors suggest that the genetic mutation is not necessarily causing the results but may be due to the mutant mouse’s subordinate status, which is eliminated in the same-genotype cage conditions (Yang et al., 2015). In Cacna1c adult females reared in mixed-genotype cages, we found reduced 50-kHz USV in same-genotype HET compared to same-genotype WT control and opposite-genotype pairs (Redecker et al., 2019). Although, in the social dominance tube test, the HET females were dominant over the WT females (Redecker et al., 2019), speaking somewhat against the results of our study being due to dominant-and subordinate roles within MIXED-genotype cages.
While we did not see any main effect of manipulating the cage mate’s genotype on the play behaviors, we did see an effect on 50-kHz USV emissions. Thus, the effects on behavior may be more subtle and become prominent when looking at the individual rats in each pair and how they respond to one another when initiating or responding to a playful attack. Social dominance in juvenile rats is typically measured through pinning behavior, with the more dominant rat pinning more (Panksepp et al., 1985). In the current study, the duration of play and play components are expressed as a value for the pair. Consequently, we cannot exclusively say at this moment what each specific partner is doing and if one is more dominant and thus pinning more than the other, but the analysis is on-going to establish the behavior of each rat. Furthermore, evidence from Pellis et al. (2022) shows that high and low-playing rats emit the same number of 50-kHz calls yet show differences in the call subtypes and associated behaviors. Thus, detailed investigations for the emission of 50-kHz USV during play would also provide more insight into the playful motivation and how they may use different communication signals depending on the partner’s genotype and the genotype of their cage mates.
4.4. Translational relevance
In humans, the CACNA1C gene has been linked through GWAS to an increased risk of ASD (Li et al., 2015), partly characterized by social and communication impairments. In the current study, the same-genotype HET pairs show social behavior and communication deficits reflected by reduced social play and 50-kHz USV emissions. However, there is a prominent rescue effect for the HET females by the WT play partners in behavior and 50-kHz USV. Our previous findings indicated that juvenile HET females have a hyper-playful repertoire and had male typical 50-kHz USV emission patterns (Kisko et al., 2020, 2021) yet still showed social communication impairments, particularly in aspects related to social incentive (Kisko et al., 2020). As adults, the HET females displayed further ASD-like social deficits and more repetitive behaviors than the WT controls (Redecker et al., 2019). Late emerging ASD-like phenotypes suggest first that rearing WT and HET juveniles together masks social deficits due to the rescue effect by WT cage mates and the innate drive for juveniles to engage in social play during this critical development period. Secondly, like humans (Baron-Cohen, 2002), ASD-like social impairments in Cacna1c haploinsufficient rats appears to manifest differently in males and females. It has been suggested that in humans autistic females may have fewer social impairments and show higher levels of social motivation than males (Head et al., 2014; Hiller et al., 2014), which is similar to what we have observed in the juvenile Cacna1c haploinsufficient rats.
Additionally, in female children a diagnosis of attention-deficit-hyperactivity disorder is often diagnosed years before a correct or accompanying diagnosis of ASD is recognized, due to the masking of social deficits (Rommelse et al., 2010). Thus, the alteration in Cav1.2 protein levels may exert similar sex differences regarding ASD-like phenotypes in Cacna1c haploinsufficient juvenile rats, which may be supplemented by housing them with WT and HET cage mates in females. Furthermore, mixed-genotype housing in males may mask behavioral social deficits but lead to more noticeable deficits in social communication through USV, resulting in decreased social motivation and reduced 50-kHz USV emissions (Kisko et al., 2018). The housing arrangement with HET and WT cages mates, therefore, may have inadvertently rescued and potentially concealed social play and communication deficits in the HET females.
5. Conclusion
While the anticipated influence of the genetic composition of the social environment on rough-and-tumble play behavior was not observed, our findings demonstrate that MIXED-genotype housing provides an optimal context for HET females to engage in natural rough-and-tumble play interactions with WT partners. This valuable interaction effectively mitigates social deficits resulting from Cacna1c haploinsufficiency. Our study emphasizes the crucial role of genetic factors and social peers in shaping early social environments and their significant impact on the development of social behavior and communication in a rat model exhibiting ASD-like deficits.
Data availability statement
The raw data supporting the conclusions of this article will be made available by the authors, without undue reservation.
Ethics statement
The animal study was reviewed and approved by the Tierschutzbehörde, Regierungspräsidium Gießen, Germany.
Author contributions
TK conceived the study with the help of MW. RK and TK performed the experiments. RB and TK analyzed the data. RB and TK wrote the manuscript with the help of MW, RS, and RK. RS and MW acquired funding. All authors contributed to the article and approved the submitted version.
Funding
This work was part of the Forschergruppe “Neurobiology of affective disorders: Translational perspectives on brain structure and function” (FOR2107) and was supported by grants from the Deutsche Forschungsgemeinschaft to RS (DFG SCHW 559/14-1 and SCHW 559/14-2) and MW (DFG WO 1732/4-1 and WO 1732/4-2), also supported by the Fonds Wetenschappelijk Onderzoek—Vlaanderen (FWO; Research Foundation—Flanders) through a grant from the 2021 ERA-NET Neuron “Neurodevelopmental Disorders” awarded to MW (PXF-E2090-G0G4421N ERA-NET MINERVA) and the Interne Fondsen KU Leuven (Internal Funds KU Leuven) through a BOFZAP Starting Grant to MW (PXF-E0120-STG/20/062).
Acknowledgments
We wish to thank Christine Hohmeyer. Marcella Rietschel, Stephanie Witt, Tobias Redecker, A. Özge Sungur, Robert Hoffmann, Alexandra Miller, and Nivethini Sangarapillai for their help in this project.
Conflict of interest
The authors declare that the research was conducted in the absence of any commercial or financial relationships that could be construed as a potential conflict of interest.
Publisher’s note
All claims expressed in this article are solely those of the authors and do not necessarily represent those of their affiliated organizations, or those of the publisher, the editors and the reviewers. Any product that may be evaluated in this article, or claim that may be made by its manufacturer, is not guaranteed or endorsed by the publisher.
Supplementary material
The Supplementary Material for this article can be found online at: https://www.frontiersin.org/articles/10.3389/fnbeh.2023.1190272/full#supplementary-material
References
Baarendse, P., Counotte, D., O’Donnell, P., and Vanderschuren, L. (2013). Early social experience is critical for the development of cognitive control and dopamine modulation of prefrontal cortex function. Neuropsychopharmacol 38, 1485–1494. doi: 10.1038/npp.2013.47
Bader, P., Faizi, M., Kim, L., Owen, S., Tadross, M., Alfa, R., et al. (2011). Mouse model of timothy syndrome recapitulates triad of autistic traits. Proc. Natl. Acad. Sci. U.S.A. 108, 15432–15437. doi: 10.1073/pnas.1112667108
Bell, H., Pellis, S., and Kolb, B. (2010). Juvenile peer play experience and the development of the orbitofrontal and medial prefrontal cortices. Behav. Brain Res. 207, 7–13. doi: 10.1016/j.bbr.2009.09.029
Blanchard, R., Blanchard, D., Agullana, R., and Weiss, S. (1991). Twenty-two kHz alarm cries to presentation of a predator, by laboratory rats living in visible burrow systems. Physiol. Behav. 50, 967–972. doi: 10.1016/0031-9384(91)90423-l
Browning, J., Whiteman, A., Leung, L., Lu, X., and Shear, D. (2017). Air-puff induced vocalizations: a novel approach to detecting negative affective state following concussion in rats. J. Neurosci. Methods 275, 45–49. doi: 10.1016/j.jneumeth.2016.10.017
Brudzynski, S. (2013). Ethotransmission: communication of emotional states through ultrasonic vocalization in rats. Curr. Opin. Neurobiol. 23, 310–317. doi: 10.1016/j.conb.2013.01.014
Burgdorf, J., Kroes, R., Moskal, J., Pfaus, J., Brudzynski, S., and Panksepp, J. (2008). Ultrasonic vocalizations of rats (Rattus norvegicus) during mating, play, and aggression: behavioral concomitants, relationship to reward, and self-administration of playback. J. Comp. Psychol. 122, 357–367. doi: 10.1037/a0012889
Burgdorf, J., Moskal, J., Brudzynski, S., and Panksepp, J. (2013). Rats selectively bred for low levels of play-induced 50kHz vocalizations as a model for autism spectrum disorders: a role for NMDA receptors. Behav. Brain Res. 251, 18–24. doi: 10.1016/j.bbr.2013.04.022
Burgdorf, J., Panksepp, J., Brudzynski, S., Kroes, R., and Moskal, J. (2005). Breeding for 50-kHz positive affective vocalization in rats. Behav. Genet. 35, 67–72. doi: 10.1007/s10519-004-0856-5
Burke, C., Kisko, T., Pellis, S., and Euston, D. (2017). Avoiding escalation from play to aggression in adult male rats: the role of ultrasonic calls. Behav. Processes 144, 72–81. doi: 10.1016/j.beproc.2017.09.014
Carcea, I., and Froemke, R. (2019). Biological mechanisms for observational learning. Curr. Opin. Neurobiol. 54, 178–185.
Cross-Disorder Group of the Psychiatric Genomics Consortium (2013). Identification of risk loci with shared effects on five major psychiatric disorders: a genome-wide analysis. Lancet 381, 1371–1379. doi: 10.1016/S0140-6736(12)62129-1
Dao, D., Mahon, P., Cai, X., Kovacsics, C., Blackwell, R., Arad, M., et al. (2010). Mood disorder susceptibility Gene CACNA1C modifies mood-related behaviors in mice and interacts with sex to influence behavior in mice and diagnosis in humans. Biol. Psychiatry 68, 801–810. doi: 10.1016/j.biopsych.2010.06.019
Dima, D., Jogia, J., Collier, D., Vassos, E., Burdick, K., and Frangou, S. (2013). Independent modulation of engagement and connectivity of the facial network during affect processing by CACNA1C and ANK3 risk genes for bipolar disorder. JAMA Psychiatry 70, 1303–1311. doi: 10.1001/jamapsychiatry.2013.2099
Galef Bennet, G. (2012). “Social learning in rats: historical context and experimental findings,” in The Oxford handbook of comparative cognition, eds T. R. Zentall and E. A. Wasserman (Oxford: Oxford University Press).
Geurts, A., Cost, G., Freyvert, Y., Zeitler, B., Miller, J., Choi, V., et al. (2009). Knockout rats via embryo microinjection of zinc-finger nucleases. Science 325, 433–433. doi: 10.1126/science.1172447
Green, E., Grozeva, D., Jones, I., Jones, L., Kirov, G., Caesar, S., et al. (2010). The bipolar disorder risk allele at CACNA1C also confers risk of recurrent major depression and of schizophrenia. Mol. Psychiatry 15, 1016–1022. doi: 10.1038/mp.2009.49
Head, A., McGillivray, J., and Stokes, M. (2014). Gender differences in emotionality and sociability in children with autism spectrum disorders. Mol. Autism 5:19. doi: 10.1186/2040-2392-5-19
Heilbronner, U., Malzahn, D., Strohmaier, J., Maier, S., Frank, J., Treutlein, J., et al. (2015). A common risk variant in CACNA1C supports a sex-dependent effect on longitudinal functioning and functional recovery from episodes of schizophrenia-spectrum but not bipolar disorder. Eur. Neuropsychopharmacol. 25, 2262–2270. doi: 10.1016/j.euroneuro.2015.09.012
Hiller, R., Young, R., and Weber, N. (2014). Sex differences in autism spectrum disorder based on DSM-5 criteria: evidence from clinician and teacher reporting. J. Abnorm. Child Psychol. 42, 1381–1393. doi: 10.1007/s10802-014-9881-x
Himmler, B., Kisko, T., Euston, D., Kolb, B., and Pellis, S. (2014). Are 50-kHz calls used as play signals in the playful interactions of rats? I. Evidence from the timing and context of their use. Behav. Processes 106, 60–66. doi: 10.1016/j.beproc.2014.04.014
Himmler, S., Modlinska, K., Stryjek, R., Himmler, B., Pisula, W., and Pellis, S. (2014). Domestication and diversification: a comparative analysis of the play fighting of the Brown Norway, Sprague-Dawley, and Wistar laboratory strains of (Rattus norvegicus). J. Comp. Psychol. 128, 318–327. doi: 10.1037/a0036104
Hol, T., Van den Berg, C., Van Ree, J., and Spruijt, B. (1999). Isolation during the play period in infancy decreases adult social interactions in rats. Behav. Brain Res. 100, 91–97. doi: 10.1016/s0166-4328(98)00116-8
Homberg, J., Wöhr, M., and Alenina, N. (2017). Comeback of the rat in biomedical research. ACS Chem. Neurosci. 8, 900–903. doi: 10.1021/acschemneuro.6b00415
Kabir, Z., Lee, A., and Rajadhyaksha, A. (2016). L-type Ca 2+ channels in mood, cognition and addiction: integrating human and rodent studies with a focus on behavioural endophenotypes. J. Physiol. 594, 5823–5837. doi: 10.1113/JP270673
Kim, A., Keum, S., and Shin, H. (2019). Observational fear behavior in rodents as a model for empathy. Genes Brain Behav. 18:e12521. doi: 10.1111/gbb.12521
Kisko, T., Braun, M., Michels, S., Witt, S., Rietschel, M., Culmsee, C., et al. (2018). Cacna1c haploinsufficiency leads to pro-social 50-kHz ultrasonic communication deficits in rats. Dis. Model Mech. 11:dmm034116. doi: 10.1242/dmm.034116
Kisko, T., Braun, M., Michels, S., Witt, S., Rietschel, M., Culmsee, C., et al. (2020). Sex-dependent effects of Cacna1c haploinsufficiency on juvenile social play behavior and pro-social 50-kHz ultrasonic communication in rats. Genes Brain Behav. 19:e12552. doi: 10.1111/gbb.12552
Kisko, T., Himmler, B., Himmler, S., Euston, D., and Pellis, S. (2015b). Are 50-kHz calls used as play signals in the playful interactions of rats? II. Evidence from the effects of devocalization. Behav. Processes 111, 25–33. doi: 10.1016/j.beproc.2014.11.011
Kisko, T., Euston, D., and Pellis, S. (2015a). Are 50-khz calls used as play signals in the playful interactions of rats? III. The effects of devocalization on play with unfamiliar partners as juveniles and as adults. Behav. Processes 113, 113–121. doi: 10.1016/j.beproc.2015.01.016
Kisko, T., Schwarting, R., and Wöhr, M. (2021). Sex differences in the acoustic features of social play-induced 50-kHz ultrasonic vocalizations: a detailed spectrographic analysis in wild-type Sprague–Dawley and Cacna1c haploinsufficient rats. Dev. Psychobiol. 63, 262–276. doi: 10.1002/dev.21998
Knutson, B., Burgdorf, J., and Panksepp, J. (1998). Anticipation of play elicits high-frequency ultrasonic vocalizations in young rats. J. Comp. Psychol. 112, 65–73. doi: 10.1037/0735-7036.112.1.65
Krug, A., Nieratschker, V., Markov, V., Krach, S., Jansen, A., Zerres, K., et al. (2010). Effect of CACNA1C rs1006737 on neural correlates of verbal fluency in healthy individuals. Neuroimage 49, 1831–1836. doi: 10.1016/j.neuroimage.2009.09.028
Li, J., Zhao, L., You, Y., Lu, T., Jia, M., Yu, H., et al. (2015). Schizophrenia related variants in CACNA1C also confer risk of autism. PLoS One 10:e0133247. doi: 10.1371/journal.pone.0133247
Lukas, M., and Wöhr, M. (2015). Endogenous vasopressin, innate anxiety, and the emission of pro-social 50-kHz ultrasonic vocalizations during social play behavior in juvenile rats. Psychoneuroendocrinology 56, 35–44. doi: 10.1016/j.psyneuen.2015.03.005
Northcutt, K. V., and Nwankwo, V. (2018). Sex differences in juvenile play behavior differ among rat strains. Dev. Psychobiol. 60, 903–912. doi: 10.1002/dev.21760
Panksepp, J. (2005). Beyond a joke: from animal laughter to human joy? Science 308, 62–63. doi: 10.1126/science.111206
Panksepp, J., Jalowiec, J., DeEskinazi, F., and Bishop, P. (1985). Opiates and play dominance in juvenile rats. Behav. Neurosci. 99, 441–453. doi: 10.1037/0735-7044.99.3.441
Panksepp, J., Siviy, S., and Normansell, L. (1984). The psychobiology of play: theoretical and methodological perspectives. Neurosci. Biobehav. Rev. 8, 465–492. doi: 10.1016/0149-7634(84)90005-8
Pasparakis, E., Koiliari, E., Zouraraki, C., Tsapakis, E., Roussos, P., Giakoumaki, S., et al. (2015). The effects of the CACNA1C rs1006737 A/G on affective startle modulation in healthy males. Eur. J. Psychiatry 30, 492–498. doi: 10.1016/j.eurpsy.2015.03.004
Pellis, S., Burke, C., Kisko, T., and Euston, D. (2018). 50-kHz vocalizations, play and the development of social competence. Handb. Behav. Neurosci. 25, 117–126. doi: 10.1098/rstb.2021.0184
Pellis, S., and Pellis, V. (2009). The playful brain: venturing to the limits of neuroscience. London: Oneworld Publications.
Pellis, S., Pellis, V., Burke, C., Stark, R., Ham, J., Euston, D., et al. (2022). Measuring play fighting in rats: a multilayered approach. Curr. Protoc. 2:e337. doi: 10.1002/cpz1.337
Pellis, S., Williams, L., and Pellis, V. (2017). Adult-juvenile play fighting in rats: insight into the experiences that facilitate the development of socio-cognitive skills. Int. J. Comp. Psychol. 30:34346. doi: 10.46867/ijcp.2017.30.00.14
Poole, T., and Fish, J. (1975). An investigation of playful behaviour in Rattus norvegicus and Mus musculus (Mammalia). J. Zool. 175, 61–71. doi: 10.1111/j.1469-7998.1975.tb01391.x
Redecker, T., Kisko, T., Schwarting, R., and Wöhr, M. (2019). Effects of Cacna1c haploinsufficiency on social interaction behavior and 50-kHz ultrasonic vocalizations in adult female rats. Behav. Brain Res. 367, 35–52. doi: 10.1016/j.bbr.2019.03.032
Rommelse, N., Franke, B., Geurts, H., Hartman, C., and Buitelaar, J. (2010). Shared heritability of attention-deficit/hyperactivity disorder and autism spectrum disorder. Eur. Child Adolesc. Psychiatry 19, 281–295. doi: 10.1007/s00787-010-0092-x
Schneider, P., Bindila, L., Schmahl, C., Bohus, M., Meyer-Lindenberg, A., Lutz, B., et al. (2016). Adverse social experiences in adolescent rats result in enduring effects on social competence, pain sensitivity and endocannabinoid signaling. Front. Behav. Neurosci. 10:203. doi: 10.3389/fnbeh.2016.00203
Schwarting, R., Kisko, T., and Wöhr, M. (2018). Playback of ultrasonic vocalizations to juvenile and adult rats: behavioral and neuronal effects. Handb. Behav. Neurosci. 25, 357–369.
Stark, R., and Pellis, S. (2020). Male long evans rats reared with a Fischer-344 peer during the juvenile period show deficits in social competency: a role for play. Int. J. Play 9, 76–91. doi: 10.1080/21594937.2020.1720142
Strohmaier, J., Amelang, M., Hothorn, L., Witt, S., Nieratschker, V., Gerhard, D., et al. (2013). The psychiatric vulnerability gene CACNA1C and its sex-specific relationship with personality traits, resilience factors and depressive symptoms in the general population. Mol. Psychiatry 18, 607–613. doi: 10.1038/mp.2012.53
Van den Berg, C. L., Hol, T., Van Ree, J. M., Spruijt, B. M., Everts, H., and Koolhaas, J. M. (1999). Play is indispensable for an adequate development of coping with social challenges in the rat. Dev. Psychobiol. 34, 129–138.
Vanderschuren, L., Achterberg, E., and Trezza, V. (2016). The neurobiology of social play and its rewarding value in rats. Neurosci. Biobehav. Rev. 70, 86–105. doi: 10.1016/j.neubiorev.2016.07.025
Vanderschuren, L., and Trezza, V. (2013). What the laboratory rat has taught us about social play behavior: role in behavioral development and neural mechanisms. Curr. Top. Behav. Neurosci. 16, 189–212. doi: 10.1007/7854_2013_268
Varlinskaya, E., Spear, L., and Spear, N. (1999). Social behavior and social motivation in adolescent rats. Physiol. Behav. 67, 475–482.
Wöhr, M. (2022). Measuring mania-like elevated mood through amphetamine-induced 50-kHz ultrasonic vocalizations in rats. Br. J. Pharmacol. 179, 4201–4219. doi: 10.1111/bph.15487
Wöhr, M., Willadsen, M., Kisko, T., Schwarting, R., and Fendt, M. (2020). Sex-dependent effects of Cacna1c haploinsufficiency on behavioral inhibition evoked by conspecific alarm signals in rats. Prog. Neuropsychopharmacol. Biol. Psychiatry 99:109849. doi: 10.1016/j.pnpbp.2019.109849
Yang, M., Lewis, F., Foley, G., and Crawley, J. (2015). In tribute to bob blanchard: divergent behavioral phenotypes of 16p11.2 deletion mice reared in same-genotype versus mixed-genotype cages. Physiol. Behav. 146, 16–27. doi: 10.1016/j.physbeh.2015.04.023
Yang, M., Perry, K., Weber, M., Katz, A., and Crawley, J. (2011). Social peers rescue autism-relevant sociability deficits in adolescent mice. Autism Res. 4, 17–27. doi: 10.1002/aur.163
Keywords: Cav1.2, calcium, rough-and-tumble play, ultrasonic communication, social environment, development
Citation: Bogdan R, Kayumova R, Schwarting RKW, Wöhr M and Kisko TM (2023) Wildtype peers rescue social play and 50-kHz ultrasonic vocalization deficits in juvenile female Cacna1c heterozygous rats. Front. Behav. Neurosci. 17:1190272. doi: 10.3389/fnbeh.2023.1190272
Received: 20 March 2023; Accepted: 23 June 2023;
Published: 03 August 2023.
Edited by:
Marjorie C. Gondré-Lewis, Howard University, United StatesReviewed by:
Marijke Achterberg, Utrecht University, NetherlandsCheryl Brandenburg, University of Maryland, United States
Adam Hamed, Polish Academy of Sciences, Poland
Copyright © 2023 Bogdan, Kayumova, Schwarting, Wöhr and Kisko. This is an open-access article distributed under the terms of the Creative Commons Attribution License (CC BY). The use, distribution or reproduction in other forums is permitted, provided the original author(s) and the copyright owner(s) are credited and that the original publication in this journal is cited, in accordance with accepted academic practice. No use, distribution or reproduction is permitted which does not comply with these terms.
*Correspondence: Theresa M. Kisko, theresa.kisko@kuleuven.be
†Present addresses: Rukhshona Kayumova, Functional Architecture of Memory Department, Leibniz Institute for Neurobiology, Magdeburg, Germany
‡These authors share last authorship