- 1Departamento de Fisiología, Facultad de Medicina, Universidad de la República, Montevideo, Uruguay
- 2Facultad de Ciencias, Universidad de la República, Montevideo, Uruguay
The postpartum period is a demanding time during which mothers experience numerous physiological adaptations that enable them to care for their offspring while maintaining their wellbeing. Hypocretins, also known as orexins, are neuropeptides synthesized by hypothalamic neurons that play a fundamental role in several functions, including the promotion of wakefulness and motivated behaviors, such as maternal care. In this regard, several findings suggest that the activity of the hypocretinergic system increases in the early postpartum period and begins to decline as weaning approaches. In particular, hypocretins within the medial preoptic area, a crucial region during this period, modulate both maternal behavior and sleep. Although further studies are necessary to obtain a comprehensive understanding of the role of hypocretins in lactating females, current research suggests that this system participates in promoting active components of maternal behavior and regulating wakefulness and sleep adjustments during the postpartum period, potentially leading to increased wakefulness during this stage. These adaptive adjustments enable the mother to cope with the continuously changing demands of the pups.
Introduction
During the postpartum period, multiple modifications in hypothalamic neuronal systems result in physiological adaptations enabling the mother to care for her offspring while preserving her wellbeing (Russell et al., 2001; Numan and Woodside, 2010). The hypocretinergic system, is one of these networks that undergoes functional modifications during this period, suggesting its potential importance in regulating maternal physiology during lactation (Sun et al., 2003; Wang et al., 2003; España et al., 2004; Donlin et al., 2014; Diniz et al., 2018).
Hypocretins (HCRT), also known as orexins, are a pair of peptides (HCRT-1 and HCRT-2, also called orexin A and B, respectively), that originate from the same pre-pro-HCRT (de Lecea et al., 1998). The HCRT bind to two metabotropic receptors, HCRT-R1 and HCRT-R2 (de Lecea et al., 1998; Sakurai et al., 1998), that have both presynaptic and postsynaptic excitatory effects (van den Pol et al., 1998). The neurons that synthesize HCRT are situated in the posterior-lateral hypothalamus and have widespread projections throughout the brain and spinal cord (Peyron et al., 1998).
Distinct populations of HCRT neurons projecting to several areas account for the different functions of this system (Harris and Aston-Jones, 2006). Thus, although initially associated with food intake, HCRT have emerged as key regulators in a diverse range of physiological processes. Their pivotal roles encompass the promotion of wakefulness, modulation of motivated behaviors, and regulation of neuroendocrine and autonomic functions (Ferguson and Samson, 2003; Nishino and Sakurai, 2005; Li and de Lecea, 2020). Additionally, the HCRT system is critical from a medical point of view, as the dysfunction of HCRT neurons is responsible for narcolepsy, an important sleep disorder (Siegel, 1999; Seifinejad et al., 2023).
Despite its significance in motivated behaviors, the role of the HCRT system during the postpartum period has not been extensively studied. Hence, this minireview aims to explore the role of this system during the postpartum period, with a specific emphasis on how it regulates wakefulness and sleep in coordination with maternal behavior and lactation.
Hypocretinergic system during the postpartum period
Table 1 provides a summary of the main findings related to the hypocretinergic system during lactation. Wang et al. (2003) showed that rats on postpartum day (PPD) 1 have an increase in pre-pro-HCRT mRNA levels compared to rats during pregnancy and on PPD 14. Recent evidence shows an increase in the number of immunoreactive HCRT neurons during lactation on PPD 12 (Sun et al., 2003; Donlin et al., 2014) and on PPD 15 (Diniz et al., 2018) compared to non-lactating animals. This increase occurs specifically in a subpopulation of HCRT neurons located in the anterior part of the posterior hypothalamus (Donlin et al., 2014; Diniz et al., 2018). Conversely, Garcia et al. (2003) found a decrease in pre-pro-HCRT mRNA on PPD 14 in lactating rats compared to proestrus controls. This may be attributed to the specific increase in HCRT levels during the proestrus phase (Porkka-Heiskanen et al., 2004). However, other studies did not observe changes in the mRNA, pre-pro-HCRT immunoreactive neurons, and hypothalamic HCRT levels as determined by radioimmunoassay between lactating animals and controls (Brogan et al., 2000; Cai et al., 2001; Wang et al., 2003; España et al., 2004). These discrepancies between studies may be attributed to differences in the control animals used as well as the methodology employed.
Related to the HCRT activity during the postpartum period, using c-Fos expression as a marker of neuronal activity, two studies found changes during this period. Specifically, España et al. (2004) observed an increase in HCRT neuronal activity on PPD 8 in comparison to cycling virgin mice, whereas in rats, Diniz et al. (2018) found an increase in HCRT activity on PPD15 compared to proestrus and diestrus in a dorsomedial subpopulation. This latter activity was followed by a significant reduction toward the end of lactation (PPD 21). Interestingly, this decrease is modulated by the suckling stimulus of the pups, as interrupting suckling reduces the decline (Diniz et al., 2018).
In summary, these results suggest that the HCRT system is most active during the early postpartum period and that this activity gradually decreases as the postpartum period advances.
Regarding HCRT receptor expression, Wang et al. (2003) reported a significant increase in the mRNA expression of HCRT-R1 in the hypothalamus of female rats on PPD 1 compared to those on PPD 14 and during pregnancy. They also observed an increase in the number of HCRT-R1 immunoreactive cells on PPD 1 in the magnocellular neurons of the supraoptic and paraventricular nuclei, where oxytocinergic neurons are located. However, no significant changes were found in the mRNA expression of HCRT-R2 (Wang et al., 2003). These findings suggest that the expression of HCRT-R1, but not HCRT-R2, is upregulated in the early postpartum period, particularly in putative oxytocinergic neurons.
Hypocretins regulate maternal behavior
Maternal behavior is crucial for ensuring the survival of offspring by providing warmth, shelter, defense, nourishment, and affect (Stern, 1989). It encompasses a spectrum of behavioral adaptations governed by intricate neuroendocrine mechanisms, enabling the female to effectively meet the demanding of the offspring (Bridges, 2020). Hormonal shifts occurring during late gestation and parturition facilitate the onset of maternal behavior (Bridges, 1984). Once established, maternal behavior is primarily regulated by stimuli originating from the pups (Rosenblatt, 1980).
Given that the HCRT system regulates a range of motivated behaviors (Mahler et al., 2014), it is not surprising that it also affects maternal behavior. In fact, D’Anna and Gammie (2006) demonstrated that the administration of intermediate doses of HCRT-1 into the brain’s ventricles of postpartum mice led to an increase in pup-licking and grooming, while high doses resulted in reduced pup retrieval, nursing, and nesting behavior. Furthermore, they found that the systemic administration of the HCRT-R1 antagonist tended to decrease certain active maternal behaviors while increasing nursing. Hence, optimal levels of HCRT-1 are necessary for the proper display of maternal behavior.
Hypocretins regulate maternal behavior and lactation through the preoptic area
The medial preoptic area (mPOA) is a crucial brain region where hormones and neuromodulators regulate the maternal care of the pups (Numan, 1974). In our studies, summarized in Figure 1, we found that the microinjections of HCRT-1 into the mPOA of mother rats increased active maternal behaviors such as licking and retrieval, whereas microinjections of an HCRT-R1 antagonist reduced active components of maternal behavior and promoted nursing (Rivas et al., 2016). These results are consistent with those described by D’Anna and Gammie (2006). Together, these findings indicate that the effects of HCRT and the HCRT-R1 antagonist on maternal behavior are mediated, at least in part, by the mPOA. In contrast, HCRT-1 administration in the mPOA did not affect nursing time in our study, whereas D’Anna and Gammie (2006) reported that high doses of HCRT-1 administered intracerebroventricularly, decreased nursing. This discrepancy may be attributed to the different doses and routes of administration used in these studies.
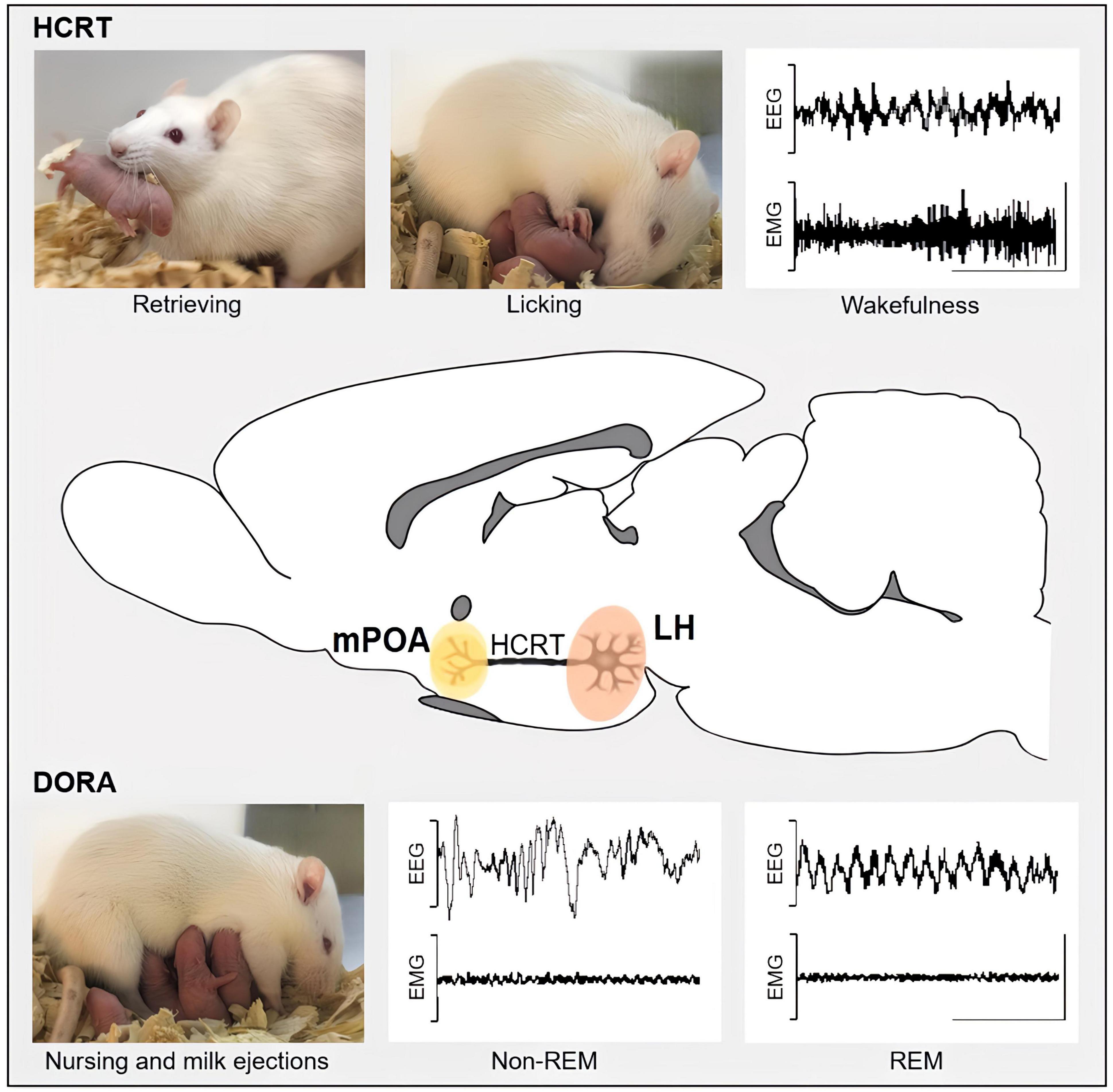
Figure 1. This schematic representation depicts the potential roles of projections from hypocretinergic neurons located in the posterior lateral hypothalamus (LH, highlighted in pink) to the medial preoptic area (mPOA, highlighted in yellow) in the regulation of both sleep and maternal behavior, as demonstrated by intra-mPOA administration of HCRT-1 and the dual hypocretin receptors antagonists (DORA). HCRT-1 intra-mPOA enhances active maternal behaviors, such as licking and retrieval, as well as wakefulness, while DORA promotes nursing along with NREM and REM sleep. EEG, electroencephalogram; EMG, electromyogram. An EEG with high-frequency and low-amplitude waves and an EMG with moderate or high activity characterizes wakefulness. Sleep contains two main stages: REM (rapid eye movement), characterized by high-frequency EEG, with characteristic theta waves in rodents, and muscle atony in EMG; and non-REM sleep, characterized by EEG with low-frequency and high-amplitude waves, and low-activity EMG. Calibration bars: 1 mV and 1 ms.
Moreover, the effect of HCRT-1 within the mPOA was more pronounced in the second week of the postpartum period compared to that found in the first week (Rivas et al., 2016). Conversely, the antagonist had a greater effect during the first postpartum week, suggesting that endogenous levels of HCRT-1 are higher in the first compared to the second postpartum week. These findings are consistent with the study of Wang et al. (2003), which reported that hypothalamic levels of pre-pro-HCRT mRNA in lactating rats are elevated on PPD 1 compared to levels observed in the second postpartum week. However, Grieb et al. (2018) did not find variation in HCRT-1 levels within the mPOA analyzed by enzyme immunoassay along the postpartum stage. Nonetheless, the large individual variability found in the study, as well as potential differences between rat strains could account for this discrepancy.
Blocking both HCRT receptors has been found to increase the time that lactating rats spent nursing and in contact with their pups (Rivas et al., 2021). Similarly, Grieb et al. (2018) observed a negative correlation between HCRT levels in the mPOA and the frequency of contact with pups when animals were grouped. Specifically, lower HCRT levels within the mPOA were associated with increased time spent with the pups. This relationship may be due to an increased wakefulness in mothers treated with higher HCRT-1 levels, which may reduce the time they spend in contact with their pups.
Administering HCRT-1 into the mPOA of lactating rats also reduced litter weight gain, despite not affecting nursing time (Rivas et al., 2021). Electrical stimulation of the mPOA has been shown to induce prolactin release (Tindal and Knaggs, 1977), and this area is known to be part of the neural pathway that regulates prolactin release in lactating rats (Tindal and Knaggs, 1977), suggesting that mPOA plays a role in the regulation of prolactin release. Considering that GABAergic neurons in the mPOA inhibit HCRT neurons (Saito et al., 2013), a possible mechanism could be based on the stimulation of the former neurons through intra mPOA administration of HCRT would affect HCRT release, which in turn could reduce prolactin secretion (Fujisawa et al., 2019), resulting in decreased milk production and reduced pup growth. However, as discussed in a following section, prolactin regulation by HCRT is complex and the existing evidence is contradictory.
Hypocretins regulate wakefulness and sleep during the postpartum period
The behavioral states of sleep and wakefulness with cyclic rhythmicity are well-established in all mammals (Siegel, 2005). Sleep comprises two distinct sleep states known as rapid eye movement (REM) sleep and non-rapid eye movement (NREM) sleep. These behavioral states are accompanied by distinct electrophysiological correlates that enable their identification (Figure 1).
The postpartum period is characterized by significant disruptions in sleep patterns (Stremler et al., 2017). Mothers of diverse species, including humans and rats, exhibit heightened wakefulness to attend to the demands of their offspring, leading to instances of partial sleep deprivation or fragmented sleep (Nishihara et al., 2001; Sivadas et al., 2016; Benedetto et al., 2017; Toth et al., 2020). As HCRT play an essential role in regulating sleep and wakefulness states (Adamantidis et al., 2007; Hung and Yamanaka, 2023), we hypothesized that these peptides contribute to some of the sleep changes that occur during the postpartum period. HCRT neurons have direct projections to critical regions that are involved in generating sleep and wakefulness, such as the thalamus, hypothalamus, and mesopontine reticular (Peyron et al., 1998; Costa et al., 2020). Specifically, projections toward the mPOA may promote wakefulness and active maternal behavior, leading to heightened alertness focused on maternal care of the pups.
While increased levels of HCRT during pregnancy have been linked to poor sleep quality (Qin et al., 2023), the impact of changes in the HCRT system during the postpartum period on maternal sleep remains unclear. Currently, the only study that investigated the effects of HCRT on maternal sleep has focused on its actions through the mPOA (Rivas et al., 2021). In this sense, administering HCRT-1 within the mPOA of lactating rats promotes wakefulness and reduces both NREM and REM sleep (Figure 1), which is consistent with the evidence found in male rats where HCRT-1 had a similar effect (Espana et al., 2001). Furthermore, microinjecting the dual orexin receptor antagonist (DORA) within the mPOA of lactating rats increased both NREM and REM sleep (Figure 1; Rivas et al., 2021).
Hypocretins regulate wakefulness and maternal behavior through the medial preoptic area
Since lactating rats primarily sleep while nursing (Benedetto et al., 2017), we investigated the concurrent effects of HCRT on wakefulness and sleep, as well as maternal behavior and lactation. The sleep-promoting effects of DORA were accompanied by an increase in nursing time and the number of milk ejections. In contrast, even if the wake-promoting result of HCRT-1 did not lead to changes in nursing time, it still caused a reduction in litter weight gain. As highlighted in Figure 1, these findings suggest a functional integration between sleep, maternal behavior and lactation, regulated by the coordinated actions of HCRT neuronal projections toward the mPOA (Rivas et al., 2021). It could be suggested that HCRT likely acts on specific subpopulations of mPOA neurons to modulate these behaviors. This is supported by extracellular in vivo recordings of mPOA neurons in lactating rats, which showed that HCRT-1 increased activity in one group of neurons, while decreasing it in another (Rivas et al., 2022).
Hypocretins regulate prolactin secretion
HCRT neurons have been implicated in the control of hormone secretion by the anterior pituitary (Taheri, 2006). The effects of HCRT on prolactin synthesis and secretion remain uncertain and subject to debate as limited research has been conducted in the context of lactation. To our knowledge, only one study, conducted by Molik et al. (2008), has investigated the impact of HCRT-1 on prolactin release using in vitro pituitary explants from lactating sheep. The results suggest that HCRT-1 directly stimulates prolactin release from lactotroph cells (Molik et al., 2008). On the other hand, in non-lactating females, while there is evidence that HCRT stimulate prolactin release (Kohsaka et al., 2001), it has also been shown that HCRT reduce the expression of this hormone in lactotrope cells (Fujisawa et al., 2019). These findings indicate that the effects of HCRT on prolactin regulation may vary according to reproductive status.
Although there are no functional studies relating HCRT and prolactin, given that prolactin regulates maternal behavior (Bridges, 2020; Georgescu et al., 2021), it could be speculated that HCRT could indirectly influence this behavior through the regulation of prolactin.
Additionally, as prolactin promotes sleep (Roky et al., 1995; Frieboes et al., 1998), HCRT may also modulate sleep indirectly through prolactin regulation. In this sense, the reduction of prolactin expression that results from HCRT administration (Fujisawa et al., 2019), may lead to a decrease in sleep. However, to confirm this hypothesis, further investigation is required.
Hypocretins may regulate food intake during the postpartum period
To meet the high energy demands of lactation, mothers increase their food intake, which can be partially regulated by HCRT due to its ability to stimulate feeding behavior (Gao and Horvath, 2016). However, Garcia et al. (2003) reported that pre-pro-HCRT mRNA levels in lactating rats on PPD14 did not change after 48 or 72 h of fasting. Conversely, while the expression of pre-pro-HCRT or hypothalamic HCRT-1 levels did not differ between lactating and virgin rats subjected to food restriction or bromocriptine treatment (which inhibits milk production), HCRT-2 levels increased in lactating rats on PPD14 subjected to food restriction (Cai et al., 2001). This finding suggests that HCRT-2 may play a significant role in regulating food intake in lactating rats. Nevertheless, the precise mechanism underlying the hyperphagia during lactation remains unknown.
Conclusion and future directions
In recent years, there has been a growing interest in studying the HCRT system during the postpartum period. According to the available evidence, the HCRT system increased its activity during the early postpartum period, with levels gradually returning to those of pre-lactation as weaning approaches. These findings suggest a functional role of the HCRT system during this specific period. One function of this system is the promotion of maternal behavior, as optimal brain levels of HCRT-1 are necessary for displaying this behavior correctly. Furthermore, HCRT-1 may play a significant role in regulating wakefulness and sleep adjustment during the postpartum period, potentially leading to increased wakefulness during this stage. These functions are regulated by HCRT, at least in part, through the mPOA. Furthermore, the broad range of functions regulated by the HCRT evidenced in males and cycling females suggests that it may also regulate physiology and behavior during the postpartum period, which has not been fully explored yet. For example, HCRT play an important role in endocrine regulation, indicating that it may participate in hormonal adjustments during lactation.
In addition, to gain a more complete understanding of the role of the HCRT system in lactating females, it would be crucial to conduct studies using advanced techniques such as optogenetics and chemogenetics, to modulate the activity of the HCRT neurons during this period.
Of note, the Food and Drug Administration (FDA) has approved DORA for the treatment of insomnia (Yang, 2014; Scott, 2020), but its effects on lactating mothers and their babies, since this drug has been detected in cord blood and breast milk (Koreki et al., 2023), are still unknown. Therefore, additional research is needed to better understand the impact of these drugs during this critical stage of life, as well as to ensure their safety for use in lactating women.
Author contributions
MR collected the studies and wrote the first draft of the manuscript. LB, PT, and AF contributed to the writing and provided the critical comments. All authors agreed on the submitted version.
Funding
This research was partially supported by PEDECIBA, ANII, and the grant CSIC-I+D groups 2022- group ID-22620220100148.
Conflict of interest
The authors declare that the research was conducted in the absence of any commercial or financial relationships that could be construed as a potential conflict of interest.
Publisher’s note
All claims expressed in this article are solely those of the authors and do not necessarily represent those of their affiliated organizations, or those of the publisher, the editors and the reviewers. Any product that may be evaluated in this article, or claim that may be made by its manufacturer, is not guaranteed or endorsed by the publisher.
References
Adamantidis, A. R., Zhang, F., Aravanis, A. M., Deisseroth, K., and de Lecea, L. (2007). Neural substrates of awakening probed with optogenetic control of hypocretin neurons. Nature 450, 420–424. doi: 10.1038/nature06310
Benedetto, L., Rivas, M., Pereira, M., Ferreira, A., and Torterolo, P. (2017). A descriptive analysis of sleep and wakefulness states during maternal behaviors in postpartum rats. Arch. Ital. Biol. 155, 99–109.
Bridges, R. S. (1984). A quantitative analysis of the roles of dosage, sequence, and duration of estradiol and progesterone exposure in the regulation of maternal behavior in the rat. Endocrinology 114, 930–940.
Bridges, R. S. (2020). The behavioral neuroendocrinology of maternal behavior: past accomplishments and future directions. Horm. Behav. 120:104662. doi: 10.1016/j.yhbeh.2019.104662
Brogan, R. S., Grove, K. L., and Smith, M. S. (2000). Differential regulation of leptin receptor but not orexin in the hypothalamus of the lactating rat. J. Neuroendocrinol. 12, 1077–1086. doi: 10.1046/j.1365-2826.2000.00559.x
Cai, X. J., Denis, R., Vernon, R. G., Clapham, J. C., Wilson, S., Arch, J. R., et al. (2001). Food restriction selectively increases hypothalamic orexin-B levels in lactating rats. Regul. Pept. 97, 163–168. doi: 10.1016/s0167-0115(00)00209-3
Costa, A., Monti, J., and Torterolo, P. (2020). Hypocretin (orexin) immunoreactivity in the feline midbrain: relevance for the generation of wakefulness. J. Chem. Neuroanat. 105, 101769. doi: 10.1016/j.jchemneu.2020.101769
D’Anna, K. L., and Gammie, S. C. (2006). Hypocretin-1 dose-dependently modulates maternal behaviour in mice. J. Neuroendocrinol. 18, 553–566.
de Lecea, L., Kilduff, T. S., Peyron, C., Gao, X., Foye, P. E., Danielson, P. E., et al. (1998). The hypocretins: hypothalamus-specific peptides with neuroexcitatory activity. Proc. Natl. Acad. Sci. U.S.A. 95, 322–327.
Diniz, G. B., Candido, P. L., Klein, M. O., Alvisi, R. D., Presse, F., Nahon, J. L., et al. (2018). The weaning period promotes alterations in the orexin neuronal population of rats in a suckling-dependent manner. Brain Struct. Funct. 223, 3739–3755.
Donlin, M., Cavanaugh, B. L., Spagnuolo, O. S., Yan, L., and Lonstein, J. S. (2014). Effects of sex and reproductive experience on the number of orexin A-immunoreactive cells in the prairie vole brain. Peptides 57, 122–128. doi: 10.1016/j.peptides.2014.05.004
Espana, R. A., Baldo, B. A., Kelley, A. E., and Berridge, C. W. (2001). Wake-promoting and sleep-suppressing actions of hypocretin (orexin): basal forebrain sites of action. Neuroscience 106, 699–715.
España, R. A., Berridge, C. W., and Gammie, S. C. (2004). Diurnal levels of Fos immunoreactivity are elevated within hypocretin neurons in lactating mice. Peptides 25, 1927–1934.
Ferguson, A. V., and Samson, W. K. (2003). The orexin/hypocretin system: a critical regulator of neuroendocrine and autonomic function. Front. Neuroendocrinol. 24:141–150. doi: 10.1016/s0091-3022(03)00028-1
Frieboes, R. M., Murck, H., Stalla, G. K., Antonijevic, I. A., and Steiger, A. (1998). Enhanced slow wave sleep in patients with prolactinoma. J. Clin. Endocrinol. Metab. 83, 2706–2710.
Fujisawa, S., Komatsubara, M., Ogura-Ochi, K., Tsukamoto-Yamauchi, N., Toma, K., Inagaki, K., et al. (2019). Orexin A modulates prolactin production by regulating BMP-4 activity in rat pituitary lactotorope cells. Peptides 113, 35–40. doi: 10.1016/j.peptides.2019.01.002
Gao, X. B., and Horvath, T. L. (2016). Feeding behavior: hypocretin/orexin neurons act between food seeking and eating. Curr. Biol. 26, R845–R847. doi: 10.1016/j.cub.2016.07.069
Garcia, M. C., Lopez, M., Gualillo, O., Seoane, L. M., Dieguez, C., and Senaris, R. M. (2003). Hypothalamic levels of NPY, MCH, and prepro-orexin mRNA during pregnancy and lactation in the rat: role of prolactin. FASEB J. 17, 1392–1400.
Georgescu, T., Swart, J. M., Grattan, D. R., and Brown, R. S. E. (2021). The prolactin family of hormones as regulators of maternal mood and behavior. Front. Glob. Womens Health 2:767467. doi: 10.3389/fgwh.2021.767467
Grieb, Z. A., Holschbach, M. A., and Lonstein, J. S. (2018). Interaction between postpartum stage and litter age on maternal caregiving and medial preoptic area orexin. Physiol. Behav. 194, 430–436.
Harris, G. C., and Aston-Jones, G. (2006). Arousal and reward: A dichotomy in orexin function. Trends Neurosci. 29, 571–577. doi: 10.1016/j.tins.2006.08.002
Hung, C., and Yamanaka, A. (2023). The role of orexin neuron activity in sleep/wakefulness regulation. Peptides 165:171007. doi: 10.1016/j.peptides.2023.171007
Kohsaka, A., Watanobe, H., Kakizaki, Y., Suda, T., and Schioth, H. B. (2001). A significant participation of orexin-A, a potent orexigenic peptide, in the preovulatory luteinizing hormone and prolactin surges in the rat. Brain Res. 898, 166–170. doi: 10.1016/s0006-8993(01)02157-6
Koreki, A., Mashima, Y., Oda, A., Koizumi, T., Koyanagi, K., and Onaya, M. (2023). You are already dead: case report of nihilistic delusions regarding others as one representation of Cotard’s syndrome. Psychiatry Clin. Neurosci. Rep. 2:e93. doi: 10.1002/pcn5.93
Li, S. B., and de Lecea, L. (2020). The hypocretin (orexin) system: from a neural circuitry perspective. Neuropharmacology 167:107993. doi: 10.1016/j.neuropharm.2020.107993
Mahler, S. V., Moorman, D. E., Smith, R. J., James, M. H., and Aston-Jones, G. (2014). Motivational activation: a unifying hypothesis of orexin/hypocretin function. Nat. Neurosci. 17, 1298–1303. doi: 10.1038/nn.3810
Molik, E., Zieba, D. A., Misztal, T., Romanowicz, K., Wszola, M., Wierzchos, E., et al. (2008). The role of orexin A in the control of prolactin and growth hormone secretions in sheep–in vitro study. J. Physiol. Pharmacol. 59(Suppl. 9), 91–100.
Nishihara, K., Horiuchi, S., Eto, H., and Uchida, S. (2001). Comparisons of sleep patterns between mothers in post-partum from 9 to 12 weeks and non-pregnant women. Psychiatry Clin. Neurosci. 55, 227–228. doi: 10.1046/j.1440-1819.2001.00835.x
Nishino, S., and Sakurai, T. (2005). The orexin/hypocretin system: physiology and pathophysiology. Berlin: Springer Science and Business Media.
Numan, M. (1974). Medial preoptic area and maternal behavior in the female rat. J. Comp. Physiol. Psychol. 87, 746–759.
Numan, M., and Woodside, B. (2010). Maternity: neural mechanisms, motivational processes, and physiological adaptations. Behav. Neurosci. 124, 715–741.
Peyron, C., Tighe, D. K., van den Pol, A. N., de Lecea, L., Heller, H. C., Sutcliffe, J. G., et al. (1998). Neurons containing hypocretin (orexin) project to multiple neuronal systems. J. Neurosci. 18, 9996–10015.
Porkka-Heiskanen, T., Kalinchuk, A., Alanko, L., Huhtaniemi, I., and Stenberg, D. (2004). Orexin A and B levels in the hypothalamus of female rats: the effects of the estrous cycle and age. Eur. J. Endocrinol. 150, 737–742. doi: 10.1530/eje.0.1500737
Qin, L., Luo, Y., Chang, H., Zhang, H., Zhu, Z., Du, Y., et al. (2023). The association between serum orexin-A levels and sleep quality in pregnant women. Sleep Med. 101, 93–98.
Rivas, M., Serantes, D., Pascovich, C., Pena, F., Ferreira, A., Torterolo, P., et al. (2022). Electrophysiological characterization of medial preoptic neurons in lactating rats and its modulation by hypocretin-1. Neurosci. Res. 184, 19–29. doi: 10.1016/j.neures.2022.08.009
Rivas, M., Serantes, D., Pena, F., Gonzalez, J., Ferreira, A., Torterolo, P., et al. (2021). Role of hypocretin in the medial preoptic area in the regulation of sleep, maternal behavior and body temperature of lactating rats. Neuroscience 475, 148–162. doi: 10.1016/j.neuroscience.2021.08.034
Rivas, M., Torterolo, P., Ferreira, A., and Benedetto, L. (2016). Hypocretinergic system in the medial preoptic area promotes maternal behavior in lactating rats. Peptides 81, 9–14.
Roky, R., Obal, F., Jr., Valatx, J. L., Bredow, S., Fang, J., Pagano, L. P., et al. (1995). Prolactin and rapid eye movement sleep regulation. Sleep 18, 536–542.
Rosenblatt, J. S. (1980). Hormonal and nonhormonal regulation of maternal behavior: a theoretical survey. Reprod. Nutr. Dev. 20, 791–800.
Russell, J. A., Douglas, A. J., and Ingram, C. D. (2001). Brain preparations for maternity–adaptive changes in behavioral and neuroendocrine systems during pregnancy and lactation. An overview. Prog. Brain Res. 133, 1–38. doi: 10.1016/s0079-6123(01)33002-9
Saito, Y. C., Tsujino, N., Hasegawa, E., Akashi, K., Abe, M., Mieda, M., et al. (2013). GABAergic neurons in the preoptic area send direct inhibitory projections to orexin neurons. Front. Neural Circ. 7:192. doi: 10.3389/fncir.2013.00192
Sakurai, T., Amemiya, A., Ishii, M., Matsuzaki, I., Chemelli, R. M., Tanaka, H., et al. (1998). Orexins and orexin receptors: a family of hypothalamic neuropeptides and G protein-coupled receptors that regulate feeding behavior. Cell 92, 573–585.
Scott, L. J. (2020). Lemborexant: first approval. Drugs 80, 425–432. doi: 10.1007/s40265-020-01276-1
Seifinejad, A., Ramosaj, M., Shan, L., Li, S., Possovre, M. L., Pfister, C., et al. (2023). Epigenetic silencing of selected hypothalamic neuropeptides in narcolepsy with cataplexy. Proc. Natl. Acad. Sci. U.S.A. 120:e2220911120. doi: 10.1073/pnas.2220911120
Siegel, J. M. (1999). Narcolepsy: a key role for hypocretins (orexins). Cell 98, 409–412. doi: 10.1016/s0092-8674(00)81969-8
Sivadas, N., Radhakrishnan, A., Aswathy, B. S., Kumar, V. M., and Gulia, K. K. (2016). Dynamic changes in sleep pattern during post-partum in normal pregnancy in rat model. Behav. Brain Res. 320, 264–274.
Stern, J. M. (1989). “Maternal behavior: sensory, hormonal, and neural determinants,” in Psychoendocrinology, eds F. R. Brush and S. Levine (Amsterdam: Elsevier), 105–226.
Stremler, R., Sharkey, K. M., and Wolfson, A. R. (2017). “Postpartum period and early motherhood,” in Principles and practice of sleep medicine, eds M. Kryger, T. Roth, and W. C. Dement (Amsterdam: Elsevier), 1547–1552.e1544.
Sun, G., Narita, K., Murata, T., Honda, K., and Higuchi, T. (2003). Orexin-A immunoreactivity and prepro-orexin mRNA expression in hyperphagic rats induced by hypothalamic lesions and lactation. J. Neuroendocrinol. 15, 51–60. doi: 10.1046/j.1365-2826.2003.00862.x
Taheri, S. (2006). “Neuroendocrine role of the orexins (hypocretins),” in The Orexin/Hypocretin System, eds S. Nishino and T. Sakurai (Berlin: Springer), 119–130.
Tindal, J. S., and Knaggs, G. S. (1977). Pathways in the forebrain of the rat concerned with the release of prolactin. Brain Res. 119, 211–221. doi: 10.1016/0006-8993(77)90101-9
Toth, A., Petho, M., Keseru, D., Simon, D., Hajnik, T., Detari, L., et al. (2020). Complete sleep and local field potential analysis regarding estrus cycle, pregnancy, postpartum and post-weaning periods and homeostatic sleep regulation in female rats. Sci. Rep. 10:8546.
van den Pol, A. N., Gao, X. B., Obrietan, K., Kilduff, T. S., and Belousov, A. B. (1998). Presynaptic and postsynaptic actions and modulation of neuroendocrine neurons by a new hypothalamic peptide, hypocretin/orexin. J. Neurosci. 18, 7962–7971.
Wang, J. B., Murata, T., Narita, K., Honda, K., and Higuchi, T. (2003). Variation in the expression of orexin and orexin receptors in the rat hypothalamus during the estrous cycle, pregnancy, parturition, and lactation. Endocrine 22, 127–134. doi: 10.1385/ENDO:22:2:127
Keywords: hypocretin (orexin), neuropeptide, hypothalamus, medial preoptic area (mPOA), wakefulness, sleep, maternal behavior, lactation
Citation: Rivas M, Ferreira A, Torterolo P and Benedetto L (2023) Hypocretins, sleep, and maternal behavior. Front. Behav. Neurosci. 17:1184885. doi: 10.3389/fnbeh.2023.1184885
Received: 12 March 2023; Accepted: 15 June 2023;
Published: 30 June 2023.
Edited by:
Walter Adriani, National Institutes of Health (ISS), ItalyReviewed by:
Aldo B. Lucion, Federal University of Rio Grande do Sul, BrazilGiovanni Laviola, National Institutes of Health (ISS), Italy
Copyright © 2023 Rivas, Ferreira, Torterolo and Benedetto. This is an open-access article distributed under the terms of the Creative Commons Attribution License (CC BY). The use, distribution or reproduction in other forums is permitted, provided the original author(s) and the copyright owner(s) are credited and that the original publication in this journal is cited, in accordance with accepted academic practice. No use, distribution or reproduction is permitted which does not comply with these terms.
*Correspondence: Mayda Rivas, mrivas@fmed.edu.uy