- 1Graduate Program in Biomedical Gerontology, School of Medicine, Pontifical Catholic University of Rio Grande do Sul (PUCRS), Porto Alegre, Brazil
- 2Neuroplasticity and Rehabilitation Research Group (NEUROPLAR), Pontifical Catholic University of Rio Grande do Sul (PUCRS), Porto Alegre, Brazil
- 3Developmental Cognitive Neuroscience Lab (DCNL), Pontifical Catholic University of Rio Grande do Sul (PUCRS), Porto Alegre, Brazil
- 4School of Health and Life Sciences, Pontifical Catholic University of Rio Grande do Sul (PUCRS), Porto Alegre, Brazil
Introduction: Skilled walking is influenced by memory, stress, and anxiety. While this is evident in cases of neurological disorders, memory, and anxiety traits may predict skilled walking performance even in normal functioning. Here, we address whether spatial memory and anxiety-like behavior can predict skilled walking performance in mice.
Methods: A cohort of 60 adult mice underwent a behavioral assessment including general exploration (open field), anxiety-like behavior (elevated plus maze), working and spatial memory (Y-maze and Barnes maze), and skilled walking performance (ladder walking test). Three groups were established based on their skilled walking performance: superior (SP, percentiles ≥75), regular (RP, percentiles 74–26), and inferior (IP, percentiles ≤25) performers.
Results: Animals from the SP and IP groups spent more time in the elevated plus maze closed arms compared to the RP group. With every second spent in the elevated plus maze closed arms, the probability of the animal exhibiting extreme percentiles in the ladder walking test increased by 1.4%. Moreover, animals that spent 219 s (73% of the total time of the test) or more in those arms were 4.67 times more likely to exhibit either higher or lower percentiles of skilled walking performance.
Discussion: We discuss and conclude anxiety traits may influence skilled walking performance in facility-reared mice.
1. Introduction
Skilled walking is a highly specialized behavior involving the ability to generate steps, maintain postural balance and adjust movements to accomplish behavioral and contextual/environmental-related demands (Balasubramanian et al., 2014; Geerse et al., 2018). This behavior is controlled by complex sensory-cognitive-motor processes and requires that neural networks overcome the contextual challenges encountered while an individual is moving (Yogev-Seligmann et al., 2008).
The cognitive-motor interplay in humans and animal models is well studied, particularly in stress and neurological disorders (Silva and Frussa-Filho, 2000). For instance, attention and executive functioning deficits are associated with a higher risk of skilled walking impairment (Yogev-Seligmann et al., 2008). Moreover, spatial memory deficits reduce skilled walking performance and increase the likelihood of falls in older adults (Strekalova et al., 2005; Brody and Holtzman, 2006; Kalueff et al., 2007; Anguera et al., 2011) while anxiety can influence skilled movement in people living with Parkinson’s disease (Ehgoetz Martens et al., 2018; Nodehi et al., 2021). Chronic stress can also induce either hyperactivity or hypoactivity in some animals (Heiderstadt et al., 2000). Interestingly, a slight food restriction generated anxiety-like behavior and reduced the movement accuracy in reaching and grasping tasks (Smith and Metz, 2005). Eysenck et al. (2007) found anxiety increased attention regarding perceived threats, but reduced attention when executing a simultaneous task.
Evidence suggests spatial tasks influence postural control, which is the act of maintaining, obtaining, or regaining balance throughout any posture or activity (Pollock et al., 2000). Moreover, previous research in a cross-species model of dual-task walking in young and older humans and rats suggested spatial memory performance is linked with cognitive-motor performance. The findings revealed aged animals and humans performed worse in cognitive-motor tasks that require spatial memory when compared to their younger peers, as observed in dual-task combining object discrimination with the alternation task (Hernandez et al., 2020). Within a broader cognitive-motor interplay, spatial memory contributes to motor control by improving the success of an intended movement in a spatial context (Langan and Seidler, 2011). Moreover, spatial working memory is a good predictor regarding speed of learning when a new skilled motor task is acquired. This is particularly important in the sensorimotor adaptation and in the sequence of a task learning (Anguera et al., 2011).
Although there is a clear link between memory, anxiety disorders and impaired skilled movement, this connection may also be present under physiological conditions. For example, anxiety traits can impact motor performance when healthy people are under pressure to perform an important task/activity (Hutchinson and Cotten, 1973; Wankel, 2014), e.g., when anxiety leads pianists to perform imprecise movements (Kotani and Furuya, 2018). Thus, we hypothesize that extreme percentiles of memory and anxiety-like behavior may be able to predict skilled walking performance. A better understanding of this functional relationship might help predict cognitive-motor disorders, such as Alzheimer (Buchman and Bennett, 2011) or Parkinson-related dementia (Tan et al., 2020). A similar situation could occur among individuals exhibiting borderline memory functioning and anxiety (McDonald, 2011; Gulpers et al., 2016, 2019; Fernandez-Baizan et al., 2019).
To the best of our knowledge, no previous study has addressed whether the spatial memory and anxiety-like behavior can predict skilled walking performance in facility-reared mice. Using the elevated plus maze (to test anxiety-like behavior), Y- and Barnes mazes (to test working and spatial memory), and the ladder walking test (to assess skilled walking performance), we hypothesize that anxiety-like traits might play a role in fine movements, such as those involved in skilled walking. To assess the skilled walking performance, the quality of each paw placement is examined frame-by-frame during the ladder walking test trials and graded using the foot-fault score (Metz and Whishaw, 2002, 2009), thus providing a skilled walking performance metric. Overall, by classifying animals according to their natural skilled walking performance distribution (percentiles ≥75, 74–26, and ≤25), we can determine whether spatial memory or anxiety-like behavior could predict skilled walking performance. Thereby, this research intended to provide essential information for further investigations focused on discovering how neurochemical and genetic factors modulate cognitive-motor interplay in skilled walking.
2. Materials and methods
2.1. Subjects
We used 60 mice (Balb/cJ, weight, 25–35 g) male (n = 28) and female (n = 32) acquired from our local colony (CEMBE/PUCRS). The animals were housed in standard lab conditions: same-sex littermates in 3–4 per cage (Tecniplast GM500: 391 mm × 199 mm × 160 mm); water and food ad libitum; temperature 23 ± 1°C and 12 h light/dark cycle. This study was approved by the Ethics Committee for Animal Research at the (PUCRS) (number 8955) and was conducted in accordance with the Ethics Guidelines of ICSS and National Institutes of Health Guide for the Care and Use of Laboratory Animals (Russell and Burch, 1959).
2.2. Procedure
The experiments were replicated twice, using half the sample each time. Animals were tested during the same period of the day (morning) in an experimental room with controlled temperature (23 ± 1°C) and lighting (500 lux). Before starting the behavioral assessment, the animals underwent 7 days of acclimation with the researchers (a daily 5-min handling session per animal). After, a behavioral test battery was performed from post-natal day (P) P60 to P72. Mice performed the open field (P60), elevated plus maze (P61), Y-maze (P62), and ladder walking (P63) tests. The day after (P64), mice began the first of the three stages of the Barnes maze test, which ended on P72. After each trial, all the apparatus were cleaned using a 70% alcohol solution. On each day, the behavioral battery lasted from 4 to 6 h for the entire sample, depending on the scheduled tests. Mice remained in their habitual housing room and were transferred to the testing room in their home cages approximately 30 min before being assessed (the between-room distance was ∼5 m, located on the same floor). The order in which behavioral tests were conducted was based on the degree of complexity of the tests, from lower to higher in accordance with other studies (Võikar et al., 2004; Lad et al., 2010). At P73, the rodents were euthanized by cervical dislocation. Supplementary Figure 1 illustrates the study design.
2.3. Apparatus
The following behavioral tests were used in this study:
2.3.1. Open field (spontaneous locomotor activity)
Animals were placed in the center of a squared Plexiglas box (33 × 33 cm) and allowed to explore the apparatus for 5 min. Video recordings were analyzed using the AnyMaze Software (Stoelting Co., Wood Dale, IL, USA), which divided the field into 16 squares (4 central and 12 peripheral). The total distance traveled, time spent in the central and peripheral zones and number of entrances in each zone were measured (Carola et al., 2002; Kraeuter et al., 2019b; Wearick-Silva et al., 2019).
2.3.2. Elevated plus maze (anxiety-like behavior)
The apparatus, elevated 50 cm above the ground, comprised two open (30 cm × 5 cm) and two closed (30 cm × 5 cm × 15 cm) arms accessed from a central platform (5 cm × 5 cm) (Gomes et al., 2022). Animals were placed individually in the center of the maze facing the open arms and allowed to explore for 5 min (Komada et al., 2008; Kraeuter et al., 2019a; Wearick-Silva et al., 2019). The following outcome measures were analyzed using the AnyMaze Software (Stoelting Co., Wood Dale, IL, USA): the distance traveled; the number of entries in each arm (open and closed arms); the time spent in the open arms, closed arms and in the center of the maze. The avoidance index (AI) was also calculated. AI is defined as a percentage measure of the avoidance of the open arms, calculated as previously described (Trullas and Skolnick, 1993). Briefly, the higher the AI the greater the avoidance behavior.
2.3.3. Y-maze (working memory)
The Y-shaped apparatus has three plexiglass arms (35 cm long, 5 cm wide, and 10 cm high, at 120° angle from each other) (Kraeuter et al., 2019c; Viola et al., 2019; Orso et al., 2021). Animals were placed individually in arm B and explored arms A and B for 5 min while arm C (the novel arm) remained closed. After, animals were individually placed in the apparatus (arm B) for 2 min with all arms available for exploration. The phases were separated by a 1-min interval (Morgan et al., 2018). Two raters manually evaluated the number of entries and recorded the exploration time in the arms (A, B, and C) (number, time spent, and percentage). The Y-maze is considered as reliable when the test protocols are followed (Gawel et al., 2019). In addition, the intraclass correlation index showed the results obtained by the two raters were reliable (data not shown). The exploration preference was calculated, as follows:
Spontaneous alternation was considered to have occurred when a mouse entered each of the 3 arms consecutively, not necessarily observing any particular order (Miedel et al., 2017). The percentage (%) of spontaneous alternation was calculated, as follows:
2.3.4. Ladder walking test (skilled walking performance)
The apparatus consisted of two sidewalls made of clear Plexiglas (1 m) and metal rungs to create a horizontal ladder (Metz and Whishaw, 2002). While crossing the ladder, mice were filmed using a GoPro Hero 4 (12 Mp/240 frames-per-second). The first two trials were considered habituation and the third trial was analyzed using the foot fault score system (Metz and Whishaw, 2002; Wearick-Silva et al., 2019) by two independent raters. The inter and intra-rater reliability for the ladder walking test are excellent using both rats and mice models (Martins et al., 2022). The position of the metal rungs across the trials (asymmetrical-only pattern) was modified, except for the first habituation trial. The performance scores were calculated as follows:
A normalized total score (%) lower than 100% means the animal performed worse in the ladder walking test when compared to the cohort mean.
2.3.5. Barnes maze (spatial memory)
The apparatus comprised a circular platform (91 cm diameter), with 20 holes spaced uniformly around the perimeter. The Barnes maze is raised 90 cm above the ground, with a moveable escape box hidden under one hole. Visual cues are used to help the animals locate the escape box. The test is divided into three stages: (a) adaptation; (b) acquisition; and (c) testing. During adaptation, the escape box is kept in the same position and rodents typically adopt three different strategies to find it: random, serial, and spatial. During the 4-day adaptation period, once a day, the mice were placed in the center of the apparatus and given 5 min to find the escape box. On the last day of adaptation, all the animals were allowed to remain in the escape box for 2 min. If they failed to enter the escape box within the 5-min period, they were gently placed there for 2 min. The acquisition stage lasted an additional 4 days. Two trials were carried out daily at 15-min intervals. In each trial, the animals were given 3 min to locate the escape box and allowed to stay there for 1 min. If they failed to enter the escape tunnel within the 3-min period, they were gently placed there for 1 min. Primary errors, total errors, primary latency and total latency were counted in each trial by the ratters executing the test. In terms of reliability the Barnes maze is considered valid when the recommended test protocols are followed (Gawel et al., 2019). In the test stage (probe trial), the escape box was removed and the mice were given 180 s to locate the place where the box had been. The number of pokes in each hole, latency until finding the hole where the box had been and errors were counted (Gawel et al., 2019).
2.4. Statistical analysis
To calculate the sample size, we used the previous study by Wearick-Silva et al. (2019). The animals were divided into three groups based on the percentiles of performance in the ladder walking test: (1) superior performer (SP, percentiles ≥75) (n = 25; female = 13, male = 12), (2) regular performer (RP, percentiles 74–26) (n = 16; female = 9, male = 7), and (3) inferior performer (IP, percentiles ≤25) (n = 19; female = 10, male = 9). Between-group comparisons were made using the one-way ANOVA and Tukey’s post-hoc test (for parametric data) or Kruskal–Wallis and Mann–Whitney tests (for non-parametric data), according to the data distribution. The potential sex-related differences in the studied sample were also assessed using one-way ANOVA (unadjusted analysis) and by entering “sex” as an independent variable in the regression models (adjusted analysis).
In this study, extreme skilled walking performance (higher or lower performance percentiles in the ladder walking test) was defined by collapsing the SP and IP groups (percentiles ≥75 and percentiles ≤25) (n = 44; female = 23, male = 21). The predictive accuracy of the measurements of spatial memory and anxiety-related behavior in determining skilled walking performance was evaluated using the receiver operating characteristic (ROC) curve. Quantitative and qualitative Poisson regressions were used to assess the predictive potential of anxiety-like behavior and spatial memory in determining skilled walking performance. This step-by-step approach allowed us to explore the current research question. Statistical analysis was performed using the Statistical Package for the Social Sciences (SPSS) 20.0 (IBM, NY, USA) and Prism GraphPad 6.0 software (La Jolla, CA, USA). p ≤ 0.05 was considered significant.
3. Results
The overall mice cohort characterization is shown in Supplementary Table 1. No statistically significant differences were found when we tested for possible sex-related behavioral bias using a between-group comparison (ANOVA) (Supplementary Table 2 and Supplementary Figure 2). Nevertheless, the sex was included as an independent variable in the regression models.
3.1. Ladder walking test
Between-group differences were observed among SP, RP, and IP groups regarding the “combined limbs performance score” (F(2,59) = 67.74; p = 0.0001). When the “forelimb performance score” was analyzed (F(2,59) = 12.51; p = 0.0001), we noticed the SP and IP (p = 0.0001) and RP and IP (p = 0.005) groups differ. In the “hindlimb performance score” (F(2,59) = 10.39; p = 0.0001), the SP was different from IP (p = 0.0001) and RP (p = 0.020). However, the IP group did not differ from the RP (p = 0.366) (Figure 1). As expected, these results suggest classifying the mice according to their “combined limbs” skilled walking performance percentiles (≥75, 74–26, ≤25) was statistically significant.
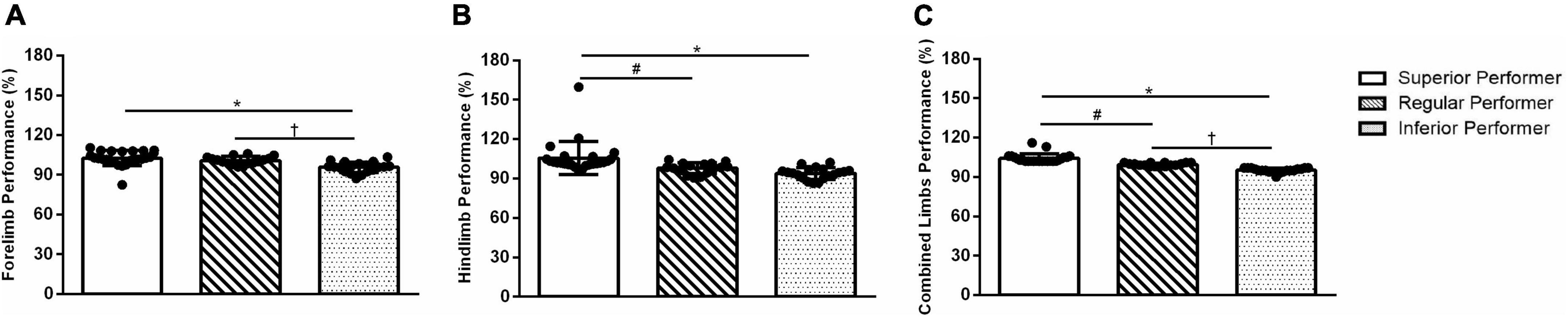
Figure 1. Walking adaptability performance in the ladder walking test. (A) Forelimb performance, (B) hindlimb performance, and (C) combined limbs performance. Data are expressed as mean ± SD. One-way ANOVA and Tukey’s post-hoc tests were used. *Superior performers vs. inferior performers; #superior performers vs. regular performers; †regular performers vs. inferior performers.
3.2. Open field test
No statistically significant between-group differences in the open field test variables were found when classifying mice according to their skilled walking performance (percentiles ≥75, 74–26, ≤25 in the ladder walking test) (Supplementary Figure 3). This suggests skilled walking performance distribution was unrelated with any exploratory behavioral pattern in the open field test.
3.3. Elevated-plus-maze
A statistically significant overall difference for “time in the closed arms” was found (KW(2,59) = 7.57; p = 0.023) (Figure 2E). The SP and IP groups were different from RP (p = 0.008 and p = 0.031, respectively). There was no difference between the SP and IP groups (p = 0.696) (Figure 2E). All the other assessed outcomes in the elevated plus maze did not differ among the studied groups (Figures 2A–G). In summary, these findings suggest mice exhibiting the higher or lower skilled walking performance percentiles, i.e., extreme skilled walking performance, showed higher levels of anxiety-like behavior in facility-reared mice. Attentional overload can offset some negative effects of anxiety, in which case a task will be adequately performed but at a higher cognitive cost (Smith et al., 2001; Nguyen, 2006). Conversely, anxiety can also make task-irrelevant stimuli more distracting and reduce attentional control, thus resulting in reduced processing efficiency when planning and executing skilled movements (Wilson, 2012). Hence mice exhibiting higher levels of anxiety can exhibit superior or inferior performance in the ladder walking test, which is in line with the literature regarding the attentional control theory. This evidence provide support to collapse SP and IP groups for a preliminary, exploratory analysis.
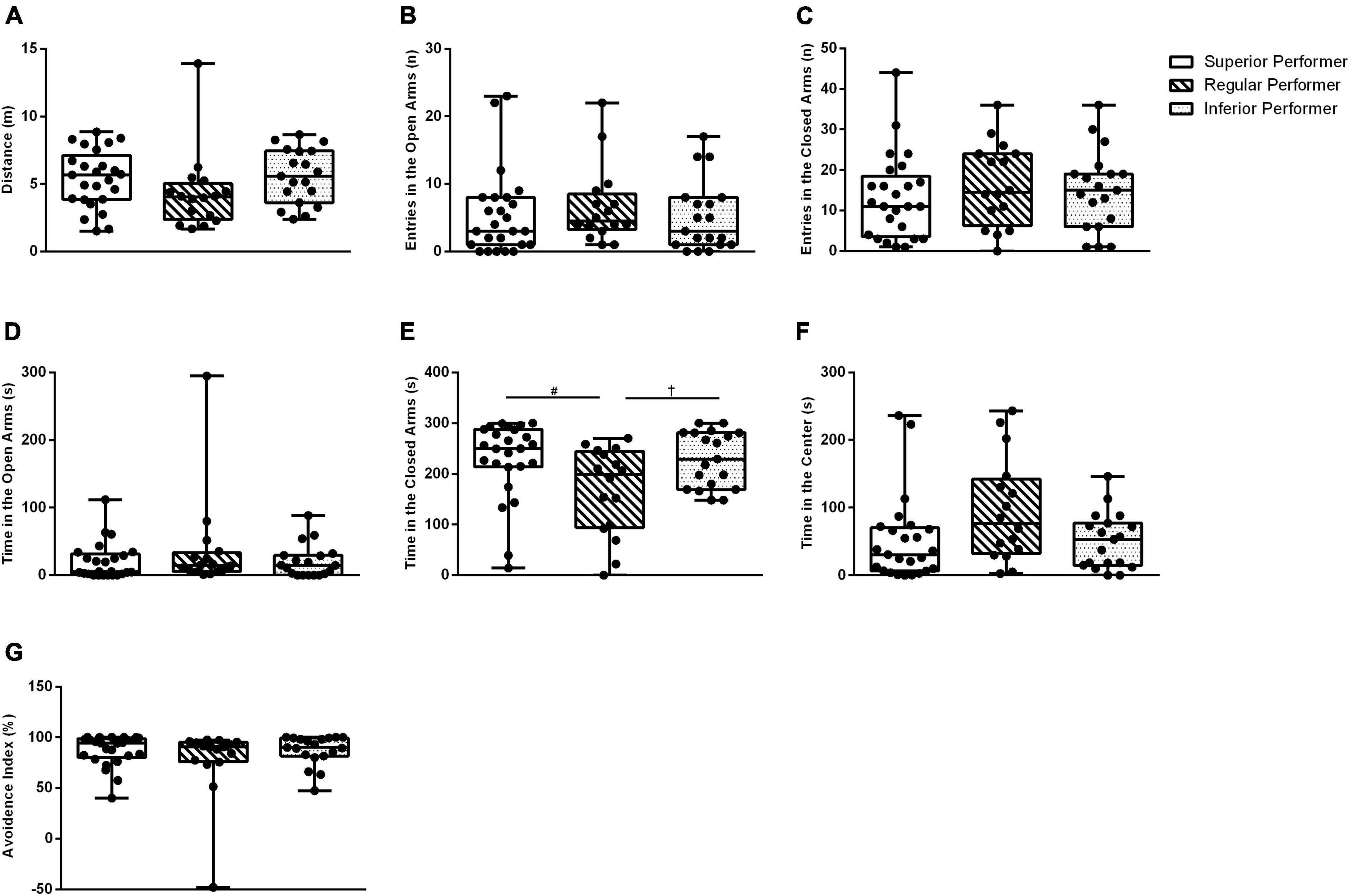
Figure 2. Anxiety-like behavior in the elevated plus maze test. (A) Distance traveled, (B) number of entries in the open arms, (C) number of entries in the closed arms, (D) time spent in the open arms, (E) time spent in the closed arms, (F) time spent in the center, and (G) avoidance index. Data are expressed in 25–50–75 percentile and range (minimum and maximum). Kruskal–Wallis and Mann–Whitney tests were used. #Superior performers vs. regular performers; †regular performers vs. inferior performers.
3.4. Y-maze and Barnes maze
No statistically significant between-group differences were found in the Y-maze-assessed outcomes when classifying mice according to their skilled walking performance (percentiles ≥75, 74–26, ≤25 in the ladder walking test) (Figure 3).
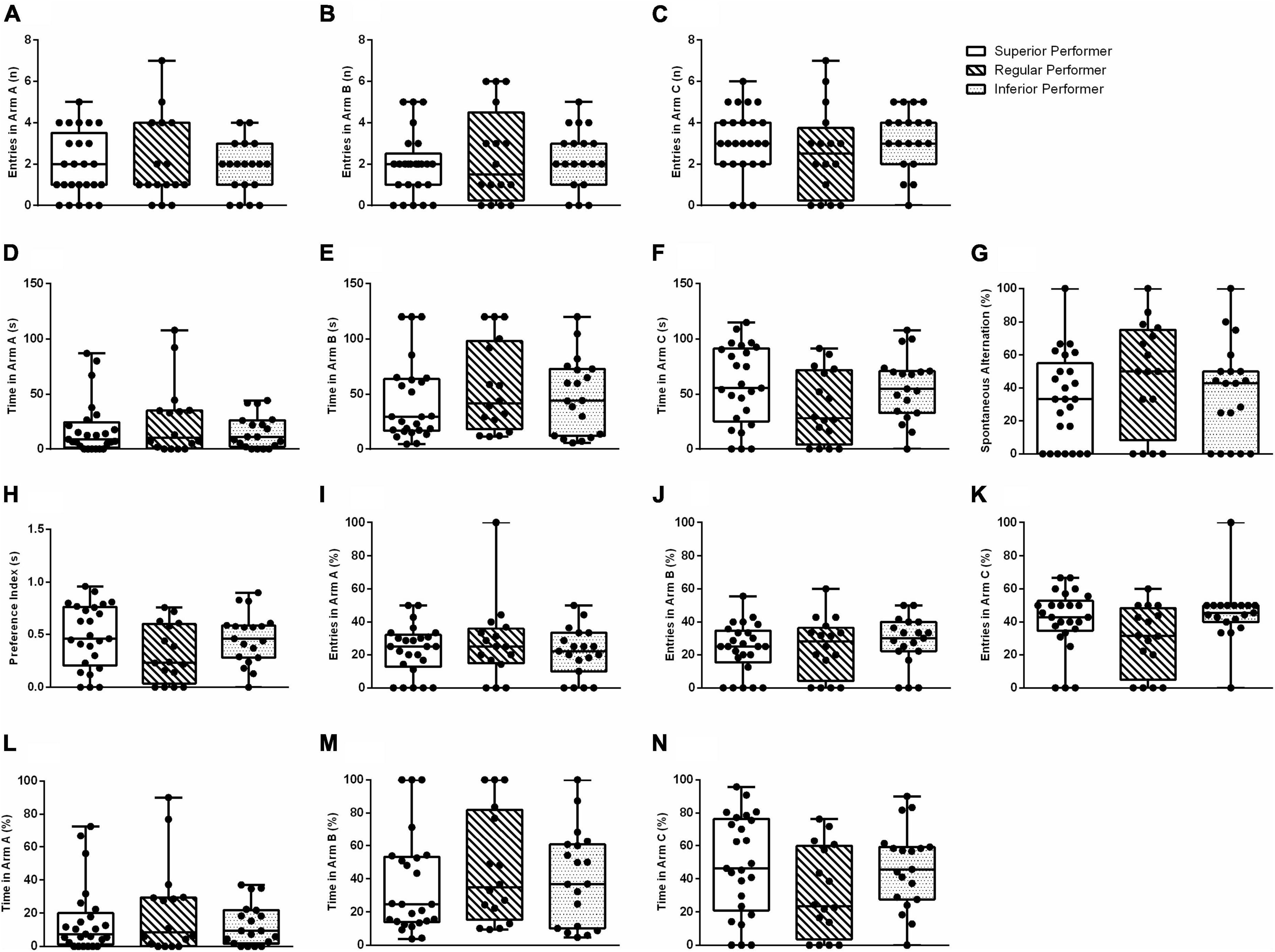
Figure 3. Spatial memory in the Y-maze test. (A) Entries in arm A, (B) entries in arm B, (C) entries in arm C, (D) time spent in arm A, (E) time spent in arm B, (F) time spent in arm C, (G) % spontaneous alternation, (H) preference index, (I) % entries in arm A, (J) % entries in arm B, (K) % entries in arm C, (L) % time spent in arm A, (M) % time spent in arm B, and (N) % time spent in arm C. Data are expressed in 25–50–75 percentile and range (minimum and maximum). The Kruskal–Wallis and Mann–Whitney tests were used.
The Barnes maze results are shown in Supplementary Figure 4. While we observed a within-group learning effect over time for averaged primary latency (F(4,59) = 11.48; p = 0.0001), primary error (F(4,59) = 7.65; p = 0.0001), total latency (F(4,59) = 16.93; p = 0.0001), as expected, no between-group differences were found when the mice were divided into skilled walking performance percentiles (≥75, 74–26, ≤25 in the ladder walking test). Together, these findings revealed spatial memory did not differ when comparing the mice in terms of skilled walking performance.
3.5. Predictive analyses (ROC analysis and Poisson regression)
The accuracy of spatial memory or anxiety-like behavior in predicting regular (RP) or extreme skilled walking performance (SP and IP groups collapsed) were tested using the ROC curve. As mentioned above, the extreme performance category was created because the SP and IP groups did not differ regarding spatial memory or anxiety-like behavior (see group analyses in section “2.4. Statistical analysis”). Moreover, we had an a priori suspicion anxiety might play a dual-effect role in skilled walking performance, i.e., higher levels of anxiety-like behavior can trigger different cognitive-motor mechanisms, thus resulting in better or worse skilled movements.
When building the regression models, we tested the outcomes exhibiting p-values ≤ 0.20 for group effects. The findings show the elevated plus maze “time in the closed arms” and the Y-maze “entries in arm C (%)” were able to predict the mouse performance in the ladder walking test (p < 0.05) (Table 1).
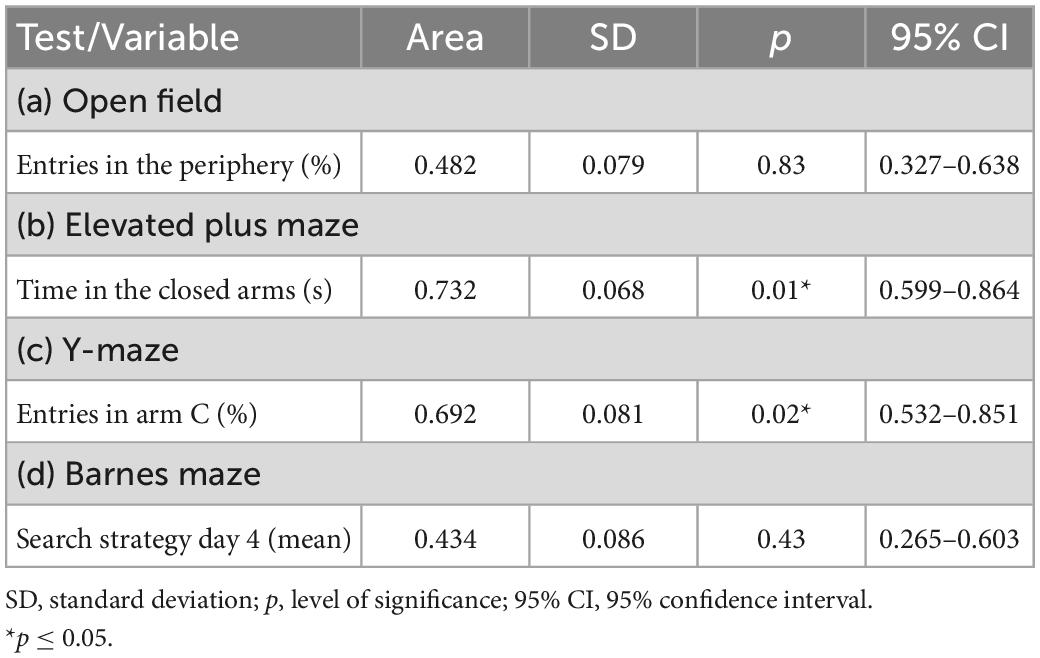
Table 1. Receiver operating characteristic curve statistics to detect the extreme behaviors in the ladder walking test.
Thereafter, the quantitative Poisson regression model was applied (Table 2). Statistical significance was observed for the variables of the elevated plus maze “time in the closed arms” (p = 0.0004) and the Y-maze “entries in arm C (%)” (p = 0.002). The quantitative regression findings showed each additional second the mouse spent in the elevated plus maze closed arms increased 1.4% its probability to exhibit an extreme performance in the ladder walking test. Moreover, each additional percentage of entries in Y-maze novel arm increased 4.6% the mouse probability to exhibit an extreme performance in the ladder walking test. The most balanced cut-off points (sensitivity vs. specificity) identified in the ROC curve (Supplementary Tables 3, 4) were used in the qualitative Poisson regression (Table 3). The cut-off point of 219 s (73% of the total time of the test) for the elevated plus maze “time in the closed arms” (sensitivity of 63.6% and specificity of 68.7%) and 42% Y-maze “entries in arm C” (novel arm) (sensitivity 61.4% and specificity 68.7%) were adopted. In the qualitative regression model, the animals that spent 73% of the total time of the test or more in the elevated plus maze closed arms increased by 4.67 times their probability of exhibiting an extreme performance in the ladder walking test. No significant effects were found for the Y-maze “entries in arm C.”
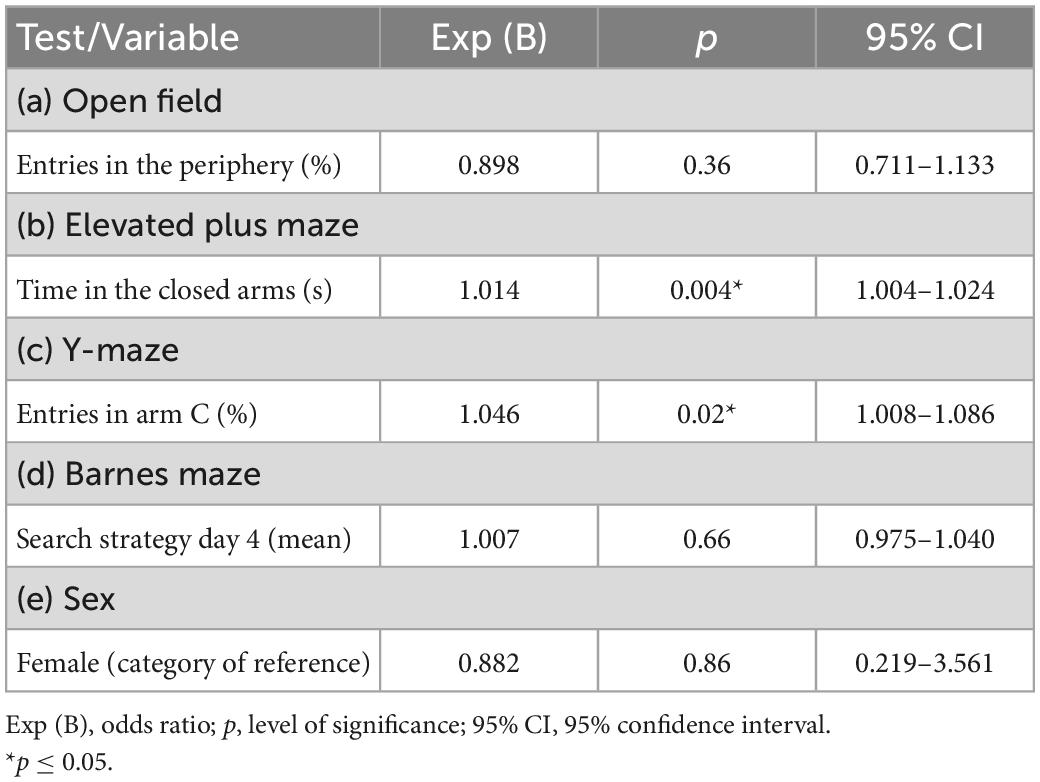
Table 2. Quantitative regression model to test the relationship between variables of interest with the extreme behaviors in the ladder walking test.
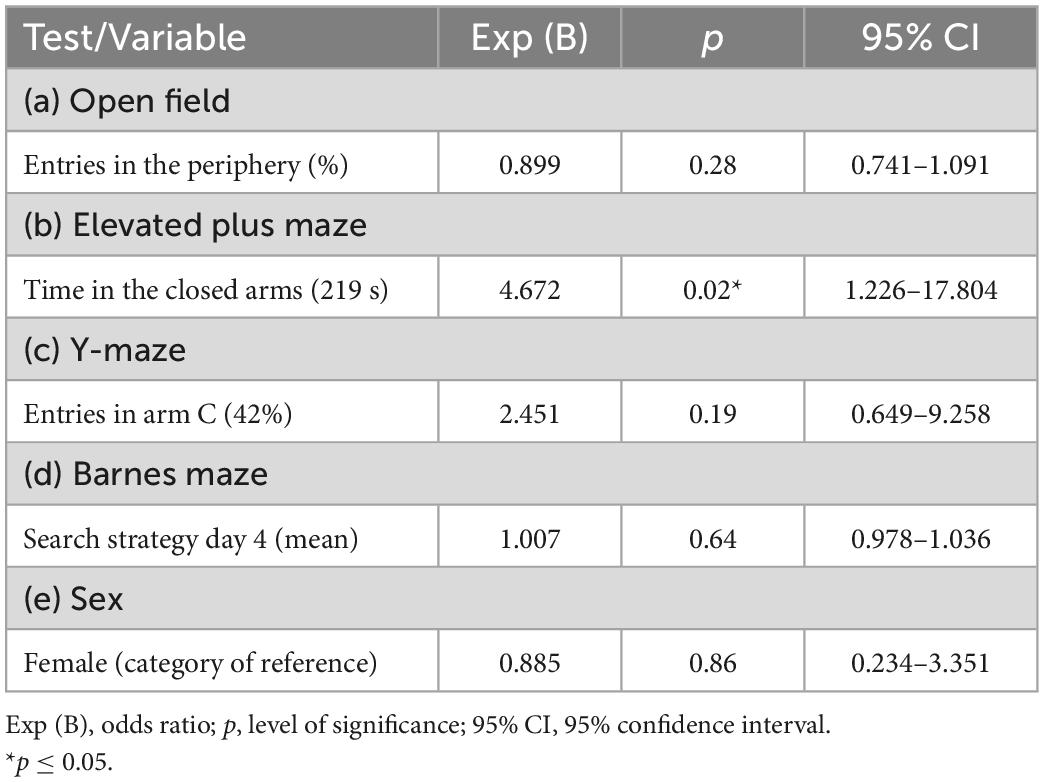
Table 3. Qualitative regression model to test the relationship between the variables of interest and the extreme behaviors of the ladder walking test.
4. Discussion
This exploratory, preliminary study sought to assess whether the anxiety-like behavior and spatial memory could be predictors of skilled walking performance in mice. To the best of our knowledge, this is the first study to explore the issue in a facility-reared mice cohort. Studying a reared-mice cohort is an interesting strategy to explore how spatial memory and anxiety might influence the motor planning needed for controlling skilled walking movements.
Our exploratory, preliminary findings suggest animals exhibiting higher levels of anxiety-like traits are more prone to show extreme skilled walking performance (lower or higher percentiles in the ladder walking test). Thus, the subtle variability in the skilled walking performance in facility-reared mice may be influenced, at least in part, by anxiety-related traits. This is of importance because skilled walking could be impaired by well-known anxiety disorders (Young et al., 2012), but this is the first study to show the anxiety may also influence the control of skilled movements in facility-reared mice.
Increased anxiety levels might produce a dual effect in skilled walking performance due to the anticipatory and/or attentional mechanisms. Some individuals overuse the anxiety-driven cognitive anticipatory mechanism and thus generate a motor plan more likely to fail when facing an irregular walkway/unpredictable obstacle. However, others use anxiogenic inputs to improve their attentional capacity, which results in higher performance levels (Eysenck et al., 2007). The determinants of which individual strategy will be adopted is still unclear.
The attentional control theory states anxiety may cause attentional bias when judging real task-related threats and irrelevant stimuli. As a result, the anxiety-related influences in performance might reflect relative inefficiency in the attentional processes (Eysenck et al., 2007). Higher levels of anxiety may change movement control and compromise movement smoothness (Lohse et al., 2011) as well as impair divided attention tasks in older adults (Hogan, 2003), thus changing the attentional efficiency required to deal with targets in the walkway (Gage et al., 2003). Moreover, there is a strong relationship between stepping inaccuracy and self-reported anxiety. Increased anxiety levels may also influence stepping accuracy indirectly by provoking maladaptive visual sampling strategies (Young et al., 2012). Conversely, some anxiety levels may help improve attention and movement accuracy. One of our most interesting findings is that those mice that stayed 219 s or longer in the elevated plus maze closed arms (longer than the RP group mean) were more likely to exhibit extreme skilled walking performance. This finding is in line with the literature, thus reinforcing that anxiety traits could also influence motor control during skilled walking movements.
Rodents have been used to provide insights into normal and pathological anxiety-like behavior (Van der Staay, 2006). Anxiety is present in both humans and rodents and plays a role in individual defense and survival (Fraser et al., 2010). However, the extent/intensity of anxiety behavior could be influenced by both genetic and phenotype profiles (Smoller and Tsuang, 1998; Meier et al., 2019). Thus, using isogenic mice models (that are genetically identical) may be the best approach to control genetic-based influences. Hence, because we used isogenic mice in this study, we can assume the differences found in anxiety-like behavior are related to the individual’s phenotype. Therefore, the current findings suggest skilled movement is influenced by anxiety-like traits determined by the individual’s phenotype. This also occurs in anxiety disorders, but to a greater extent. Overall, our exploratory, preliminary findings indicate anxiety-like behavior may contribute to determine skilled movement performance. Notwithstanding, fluctuations in anxiety levels may be related to the test conditions or based on intra-individual characteristics (Ramos, 2008). The literature suggests open field, elevated plus maze and light-dark box outcomes may not measure the same type of anxiety-like behavior (Cryan and Holmes, 2005). Similarly, the strain of mice and the apparatus used in the experiments could also influence the measured outcomes (An et al., 2011). For instance, Balb/c mice were found to behave more “anxiously” in the elevated plus maze when compared with the open field test (Trullas and Skolnick, 1993; Carola et al., 2002). Hence, it is not completely unusual to find a lack of concordance between these two tests (Trullas and Skolnick, 1993; Rogers et al., 1999). Moreover, while the open field and elevated plus maze tests are both valuable when assessing anxiety-like behavior, their paradigms are quite different. While open field could be used to assess anxiety-like behavior in general, it is designed to measure exploratory/general locomotion. By contrast, the elevated plus maze is specifically designed to assess anxiety-like behavior (Fraser et al., 2010). Additionally, rearing and grooming were not analyzed in the present study because they could be considered unspecific to identify anxiety levels. While some studies suggest greater rearing counts are associated with anxiety-like behavior in mice, other studies show the opposite (Costall et al., 1989; Borta and Schwarting, 2005). A similar lack of specificity has been reported for grooming counts (Kalueff, 2000; Kalueff and Tuohimaa, 2005).
In this study, the animals were assessed in controlled experimental rooms dedicated to behavioral studies, in the same period of the day, interacting with the same researchers, in accordance with guidelines found in the international literature. Regarding the time spent in different maze zones, the Balb/c mice would naturally be expected to spend more time, on average, avoiding potential risks by remaining more time in the closed arms/peripheral zones of the of the elevated plus maze and open field, respectively (Rodgers and Johnson, 1995; Rodgers and Dalvi, 1997). This may explain the reduced number of crossings from the areas where animals feel safe to areas where they feel more exposed. Different results could have been found if anxiety/stress-induced protocols, anxiolytic treatments, or other mice strains had been used.
The ability to cope with environmental circumstances depends on learning and memory (Vorhees and Williams, 2014). The retention and processing of visuospatial information involves spatial working memory (Fenner et al., 2000). When navigating in a new environment, visuospatial information needs to be temporarily stored and used to locate objects or reach targets, thereby inhibiting distracting stimuli (Flouri et al., 2019). Spatiotemporal parameters of gait are also influenced by working memory (Eysenck et al., 2007) and could be modified during brain aging (Ayoubi et al., 2015). Here, working memory exhibited a subtle capacity to predict skilled walking performance. This may be attributed to the features of the ladder walking test that provide insufficient spatial memory challenges compared with those observed when the rodent is inserted in its ecological context. Although the ladder walking test provides for an asymmetrical pattern, all the rungs have the same diameter, shape and placement level, thus facilitating the animal’s navigation. In addition, the fact the animals underwent three trials on the ladder walking test might have facilitated the creation of an internal image of the test, therefore reducing the demand on the spatial memory (Sorrentino et al., 2019).
This study was designed to minimize biases when establishing behavioral battery assessments, following previously published recommendations (Saré et al., 2021). First, all the experiments were performed in the Center for Experimental Biological Models (Cembe), a reference in animal care and research in southern Brazil. The Center has standard rooms designed and fully dedicated to the study of behavior in mice. Second, all animals undergo a familiarization protocol with the researchers prior to testing; lighting was standardized during the tests and the same researchers conducted all the behavioral tests. Third, a seminal paper from Paylor et al. (2006) using different mice strains demonstrated a 1-day test interval is sufficient to minimize behavioral testing-related effects in comparison with a 1-week test interval, thus supporting the current study design. Finally, several studies have used a similar sequence of behavioral testing (Võikar et al., 2004; Wearick-Silva et al., 2019). Hence, an a priori suspicion regarding test interval effects on the current behavioral protocol seems unlikely. Nevertheless, to the best of our knowledge, no previous studies have compared 1-day vs. longer between-test intervals using the same test sequence we adopted.
This study has some limitations. First, skilled walking performance, anxiety, and spatial memory were assessed using different and non-simultaneous tasks. Thus, fluctuations in attention and anxiety-like behavior may have occurred across the tests. Nevertheless, to the best of our knowledge, there is no validated behavioral apparatus capable of simultaneously assessing skilled walking performance, spatial memory, and anxiety-like behavior in rodents. Secondly, we have not compared facility-reared mice with those genetically modified to exhibit anxiety-related disorders. Although such a comparison might be interesting, the link between pathological anxiety and cognitive-motor performance has been well studied (Runswick et al., 2018; Nolte et al., 2019). In addition, brain tissue-related analyses were not possible (due to limited research funding in Brazil), thus the brain mechanisms underlying skilled walking performance were not addressed in this study. Nevertheless, here, our main research goal was to explore whether subtle differences in skilled walking performance could be influenced by anxiety traits. This research contributes to motor control theories, particularly those that sustain personal traits influence the movement strategies adopted to perform some tasks (Bolmont, 2005; Gatto et al., 2011; Berret et al., 2018). Further studies properly designed to compare normal and pathological levels of anxiety-like behavior in mice models using anxiolytic drugs or non-pharmacological strategies may help move the field forward. The influence of anxiety levels on skilled walking performance might differ in physiological and pathological conditions and should be interpreted with caution.
When looking for potential sex-related behavioral bias, we found no changes in the statistical analyses we ran, which is partially consistent with other mouse models (An et al., 2011; Hunsaker et al., 2011). Despite sex (being male or female) having been included as variable in the regression models (see Tables 2, 3), animals were categorized without considering their hormonal status due to the exploratory nature of the study. Nevertheless, we cannot exclude the possibility that sex-related hormones might have influenced the present findings. Although our regression models included “sex” as an independent variable (which was shown not to be significant in the regression models), we did not control the phases of the female estrous cycle. To ensure the acquired data from the female mice would properly represent the behavioral test/test phase under the potential influence of a specific estrous cycle day (proestrus, estrus, metestrus, and diestrus), considering a 13-day long behavioral battery, a very large number of animals would be necessary. Notwithstanding, addressing specific hormonal influences on the different behaviors (independent factors) that may predict walking adaptability performance (dependent factor) could be worthwhile. Hence, our findings could be considered as a starting point to encourage further studies covering this issue.
Additionally, the clustered analysis of males and females, the number of animals used, and the lack of an anxiolytic treatment without motor effects, e.g., allopregnanolone (Hovakimyan et al., 2013; Leppä et al., 2016; Diviccaro et al., 2022) or chrysin (Rodríguez-Landa et al., 2021) could also explain the main results of this preliminary, exploratory study. Hence, further studies properly designed to test the influence of the estrous cycle and anxiolytic-related drugs on anxiety levels and skilled walking performance are required to confirm the current exploratory findings. Several studies have reported that the estrous cycle influences EPM-related behavior (Mora et al., 1996; Galeeva and Tuohimaa, 2001; Marcondes et al., 2001; An et al., 2011; Hunsaker et al., 2011; Scholl et al., 2019). The data analysis in the present study does not measure these cycle phases of the female in the sample, therefore, the current results should be interpreted with caution. In our study, when we assessed a potential between-sex difference for the number of entries in the EPM open arms, a borderline statistical significance was found (p = 0.06) (see Supplementary Table 2). When designing trials on anxiety and walking adaptability, verifying within-sex rather than between-sex differences would be advisable (Bale and Epperson, 2017). Our findings, together with those in the literature, suggest the need for further trials addressing sex and hormonal influences on anxiety and skilled walking-related outcomes.
While other behavioral tests could have been adopted in this study, we decided to use only exploratory-based tests without any aversive stimuli. We know from the literature that using aversive stimuli, as seen in Sidman avoidance task or Vogel conflict task, could potentially change mice behavior in other exploratory-based tests (Hiew et al., 2020; Lucantonio et al., 2021). Therefore, when designing a behavioral battery, the minimum number of tests should be used in the same sample (Hånell and Marklund, 2014), as subjecting a sample to an excessive number of tests has been shown to change neurochemical and behavioral findings (Barchas et al., 1978). With this in mind, we considered the widely used elevated plus maze was the most appropriate to assess anxiety-like behavior in the current study (Carola et al., 2002; Yilmazer-Hanke et al., 2003; An et al., 2011; Medeiros et al., 2018; Kraeuter et al., 2019a). Further trials addressing how aversive experiences influence skilled walking performance might benefit from adopting aversive-based tests.
Finally, the lack of any between-group difference in the open field or differences in the time spent in the center or open arms of the elevated plus maze suggest there are subtle anxiety-like behavior changes in facility-reared mice. On the one hand, this is expected because we are not working with models of anxiety disorder but, on the other hand, the lack of consistency in the adopted tests can be considered a study limitation. Further study replication is needed to fully clarify this issue and move the field forward.
5. Conclusion
We conclude higher anxiety traits might have a dual effect on skilled walking performance in facility-reared mice, thus predicting, at least in part, the individuals exhibiting superior or inferior performance. This finding agrees with previous research suggesting anxiety traits can modulate cognitive-motor planning when adapting movements such as the ability to adapt walking on an irregular footpath. This exploratory, preliminary study may constitute an important step to encourage further research properly designed to understand the neurobiological mechanisms of skilled walking performance.
Data availability statement
The raw data supporting the conclusions of this article will be made available by the authors, without undue reservation.
Ethics statement
This animal study was reviewed and approved by the Ethics Committee for Animal Research at the Pontifical Catholic University of Rio Grande do Sul.
Author contributions
AS, LM, LW-S, RO, LX, and RM contributed to the conception and design of the study. AS, LM, and RM organized the data and wrote the manuscript. AS and RM performed the statistical analysis. All authors contributed to the article and approved the submitted version.
Funding
This study was supported by the Coordination of Improvement of Higher Education Personnel – Brazil (CAPES) – Financing Code 001 and the National Council for Scientific and Technological Development (CNPq).
Conflict of interest
The authors declare that the research was conducted in the absence of any commercial or financial relationships that could be construed as a potential conflict of interest.
Publisher’s note
All claims expressed in this article are solely those of the authors and do not necessarily represent those of their affiliated organizations, or those of the publisher, the editors and the reviewers. Any product that may be evaluated in this article, or claim that may be made by its manufacturer, is not guaranteed or endorsed by the publisher.
Supplementary material
The Supplementary Material for this article can be found online at: https://www.frontiersin.org/articles/10.3389/fnbeh.2023.1059029/full#supplementary-material
References
An, X. L., Zou, J. X., Wu, R. Y., Ying, Y., Tai, F. D., Zeng, S. Y., et al. (2011). Strain and sex differences in anxiety-like and social behaviors in C57Bl/6J and BALB/cJ Mice. Exp. Anim. 60, 111–123. doi: 10.1538/expanim.60.111
Anguera, J. A., Reuter-Lorenz, P. A., Willingham, D. T., and Seidler, R. D. (2011). Failure to engage spatial working memory contributes to age-related declines in visuomotor learning. J. Cogn. Neurosci. 23, 11–25. doi: 10.1162/jocn.2010.21451
Ayoubi, F., Launay, C. P., Annweiler, C., and Beauchet, O. (2015). Fear of falling and gait variability in older adults: A systematic review and meta-analysis. J. Am. Med. Dir. Assoc. 16, 14–19. doi: 10.1016/j.jamda.2014.06.020
Balasubramanian, C. K., Clark, D. J., and Fox, E. J. (2014). Walking adaptability after a stroke and its assessment in clinical settings. Stroke Res. Treat. 2014:591013. doi: 10.1155/2014/591013
Bale, T. L., and Epperson, C. N. (2017). Sex as a biological variable: Who, what, when, why, and how. Neuropsychopharmacology 42, 386–396. doi: 10.1038/NPP.2016.215
Barchas, J. D., Akil, H., Elliott, G. R., Holman, R. B., and Watson, S. J. (1978). Behavioral neurochemistry: Neuroregulators and behavioral states. Science 200, 964–973. doi: 10.1126/SCIENCE.25486
Berret, B., Castanier, C., Bastide, S., and Deroche, T. (2018). Vigour of self-paced reaching movement: Cost of time and individual traits. Sci. Rep. 8, 1–14. doi: 10.1038/s41598-018-28979-6
Bolmont, B. (2005). “Role and influence of moods including anxiety on motor control. - PsycNET,” in Causes, role and influence of mood states, ed. A. V. Clark (New York, NY: Nova Biomedical Books), 57–73.
Borta, A., and Schwarting, R. K. W. (2005). Inhibitory avoidance, pain reactivity, and plus-maze behavior in Wistar rats with high versus low rearing activity. Physiol. Behav. 84, 387–396. doi: 10.1016/J.PHYSBEH.2005.01.009
Brody, D. L., and Holtzman, D. M. (2006). Morris water maze search strategy analysis in PDAPP mice before and after experimental traumatic brain injury. Exp. Neurol. 197, 330–340. doi: 10.1016/j.expneurol.2005.10.020
Buchman, A. S., and Bennett, D. A. (2011). Loss of motor function in preclinical Alzheimer’s disease. Expert Rev. Neurother. 11, 665–676. doi: 10.1586/ern.11.57
Carola, V., D’Olimpio, F., Brunamonti, E., Mangia, F., and Renzi, P. (2002). Evaluation of the elevated plus-maze and open-field tests for the assessment of anxiety-related behaviour in inbred mice. Behav. Brain Res. 134, 49–57. doi: 10.1016/S0166-4328(01)00452-1
Costall, B., Jones, B. J., Kelly, M. E., Naylor, R. J., and Tomkins, D. M. (1989). Exploration of mice in a black and white test box: Validation as a model of anxiety. Pharmacol. Biochem. Behav. 32, 777–785. doi: 10.1016/0091-3057(89)90033-6
Cryan, J. F., and Holmes, A. (2005). Model organisms: The ascent of mouse: Advances in modelling human depression and anxiety. Nat. Rev. Drug Discov. 4, 775–790. doi: 10.1038/nrd1825
Diviccaro, S., Cioffi, L., Falvo, E., Giatti, S., and Melcangi, R. C. (2022). Allopregnanolone: An overview on its synthesis and effects. J. Neuroendocrinol. 34, 1–17. doi: 10.1111/JNE.12996
Ehgoetz Martens, K. A., Silveira, C. R. A., Intzandt, B. N., and Almeida, Q. J. (2018). Overload from anxiety: A non-motor cause for gait impairments in Parkinson’s Disease. J. Neuropsychiatry Clin. Neurosci. 30, 77–80. doi: 10.1176/APPI.NEUROPSYCH.16110298
Eysenck, M. W., Derakshan, N., Santos, R., and Calvo, M. G. (2007). Anxiety and cognitive performance: Attentional control theory. Emotion 7, 336–353. doi: 10.1037/1528-3542.7.2.336
Fenner, J., Heathcote, D., and Jerrams-Smith, J. (2000). The development of wayfinding competency: Asymmetrical effects of visuo-spatial and verbal ability. J. Environ. Psychol. 20, 165–175. doi: 10.1006/jevp.1999.0162
Fernandez-Baizan, C., Diaz-Caceres, E., Arias, J. L., and Mendez, M. (2019). Egocentric and allocentric spatial memory in healthy aging: Performance on real-world tasks. Braz. J. Med. Biol. Res. 52:e8041. doi: 10.1590/1414-431X20198041
Flouri, E., Papachristou, E., and Midouhas, E. (2019). The role of neighbourhood greenspace in children’s spatial working memory. Br. J. Educ. Psychol. 89, 359–373. doi: 10.1111/bjep.12243
Fraser, L. M., Brown, R. E., Hussin, A., Fontana, M., Whittaker, A., O’leary, T. P., et al. (2010). Measuring anxiety-and locomotion-related behaviours in mice: A new way of using old tests. Psychopharmacology 211, 99–112. doi: 10.1007/s00213-010-1873-0
Gage, W. H., Sleik, R. J., Polych, M. A., McKenzie, N. C., and Brown, L. A. (2003). The allocation of attention during locomotion is altered by anxiety. Exp. Brain Res. 150, 385–394. doi: 10.1007/s00221-003-1468-7
Galeeva, A., and Tuohimaa, P. (2001). Analysis of mouse plus-maze behavior modulated by ovarian steroids. Behav. Brain Res. 119, 41–47. doi: 10.1016/S0166-4328(00)00341-7
Gatto, N. M., Bordelon, Y., Gatz, M., and Ritz, B. (2011). Personality characteristics and motor skills attributed to occupations in Parkinson disease. Cogn. Behav. Neurol. 24, 18–25. doi: 10.1097/WNN.0B013E318218C5EB
Gawel, K., Gibula, E., Marszalek-Grabska, M., Filarowska, J., and Kotlinska, J. H. (2019). Assessment of spatial learning and memory in the Barnes maze task in rodents-methodological consideration. Naunyn Schmiedebergs Arch. Pharmacol. 392, 1–18. doi: 10.1007/s00210-018-1589-y
Geerse, D. J., Roerdink, M., Marinus, J., and van Hilten, J. J. (2018). Assessing walking adaptability in Parkinson’s Disease: “The Interactive Walkway.”. Front. Neurol. 9:1096. doi: 10.3389/fneur.2018.01096
Gomes, M. G. S., Tractenberg, S. G., Orso, R., Viola, T. W., and Grassi-Oliveira, R. (2022). Sex differences in risk behavior parameters in adolescent mice: Relationship with brain-derived neurotrophic factor in the medial prefrontal cortex. Neurosci. Lett. 766. doi: 10.1016/J.NEULET.2021.136339
Gulpers, B. J. A., Oude Voshaar, R. C., van Boxtel, M. P. J., Verhey, F. R. J., and Köhler, S. (2019). Anxiety as a risk factor for cognitive decline: A 12-year follow-up cohort study. Am. J. Geriatr. Psychiatry 27, 42–52. doi: 10.1016/J.JAGP.2018.09.006
Gulpers, B., Ramakers, I., Hamel, R., Köhler, S., Oude Voshaar, R., and Verhey, F. (2016). Anxiety as a predictor for cognitive decline and dementia: A systematic review and meta-analysis. Am. J. Geriatr. Psychiatry 24, 823–842. doi: 10.1016/J.JAGP.2016.05.015
Hånell, A., and Marklund, N. (2014). Structured evaluation of rodent behavioral tests used in drug discovery research. Front. Behav. Neurosci. 8:252. doi: 10.3389/FNBEH.2014.00252
Heiderstadt, K. M., McLaughlin, R. M., Wright, D. C., Walker, S. E., and Gomez-Sanchez, C. E. (2000). The effect of chronic food and water restriction on open-field behaviour and serum corticosterone levels in rats. Lab. Anim. 34, 20–28. doi: 10.1258/002367700780578028
Hernandez, A. R., Winesett, S. P., Federico, Q. P., Williams, S. A., Burke, S. N., and Clark, D. J. (2020). A cross-species model of dual-task walking in young and older humans and rats. Front. Aging Neurosci. 12:276. doi: 10.3389/fnagi.2020.00276
Hiew, L. F., Khairuddin, S., Aquili, L., Koh, J., Fung, M. L., Lim, W. L., et al. (2020). Behavioural responses of anxiety in aversive and non-aversive conditions between young and aged Sprague-Dawley rats. Behav. Brain Res. 385:112559. doi: 10.1016/J.BBR.2020.112559
Hogan, M. J. (2003). Divided attention in older but not younger adults is impaired by anxiety. Exp. Aging Res. 29, 111–136. doi: 10.1080/03610730303712
Hovakimyan, M., Maass, F., Petersen, J., Holzmann, C., Witt, M., Lukas, J., et al. (2013). Combined therapy with cyclodextrin/allopregnanolone and miglustat improves motor but not cognitive functions in Niemann-Pick Type C1 mice. Neuroscience 252, 201–211. doi: 10.1016/J.NEUROSCIENCE.2013.08.001
Hunsaker, M. R., von Leden, R. E., Ta, B. T., Goodrich-Hunsaker, N. J., Arque, G., Kim, K., et al. (2011). Motor deficits on a ladder rung task in male and female adolescent and adult CGG knock-in mice. Behav. Brain Res. 222, 117–121. doi: 10.1016/j.bbr.2011.03.039
Hutchinson, V. Q., and Cotten, D. J. (1973). Effects of audience and anxiety level on learning and performance of a complex gross motor skill by college women. Percept. Mot. Skills 36, 1103–1108. doi: 10.2466/PMS.1973.36.3C.1103
Kalueff, A. V. (2000). Measuring grooming in stress and comfort | Request PDF. Available online at: https://www.researchgate.net/publication/285772797_Measuring_grooming_in_stress_and_comfort (accessed October 24, 2022).
Kalueff, A. V., and Tuohimaa, P. (2005). The grooming analysis algorithm discriminates between different levels of anxiety in rats: Potential utility for neurobehavioural stress research. J. Neurosci. Methods 143, 169–177. doi: 10.1016/J.JNEUMETH.2004.10.001
Kalueff, A. V., Fox, M. A., Gallagher, P. S., and Murphy, D. L. (2007). Hypolocomotion, anxiety and serotonin syndrome-like behavior contribute to the complex phenotype of serotonin transporter knockout mice. Genes Brain Behav. 6, 389–400. doi: 10.1111/j.1601-183X.2006.00270.x
Komada, M., Takao, K., and Miyakawa, T. (2008). Elevated plus maze for mice protocol protocol. J. Vis. Exp. 22:1088. doi: 10.3791/1088
Kotani, S., and Furuya, S. (2018). State anxiety disorganizes finger movements during musical performance. J. Neurophysiol. 120, 439–451. doi: 10.1152/JN.00813.2017
Kraeuter, A. K., Guest, P. C., and Sarnyai, Z. (2019b). “The open field test for measuring locomotor activity and anxiety-like behavior,” in Methods in molecular biology, ed. P. Guest (Totowa, NJ: Humana Press Inc), 99–103. doi: 10.1007/978-1-4939-8994-2_9
Kraeuter, A. K., Guest, P. C., and Sarnyai, Z. (2019a). “The elevated plus maze test for measuring anxiety-like behavior in rodents,” in Methods in molecular biology, ed. P. Guest (Totowa, NJ: Humana Press Inc), 69–74. doi: 10.1007/978-1-4939-8994-2_4
Kraeuter, A. K., Guest, P. C., and Sarnyai, Z. (2019c). “The Y-maze for assessment of spatial working and reference memory in mice,” in Methods in molecular biology, ed. P. Guest (Totowa, NJ: Humana Press Inc), 105–111. doi: 10.1007/978-1-4939-8994-2_10
Lad, H. V., Liu, L., Paya-Cano, J. L., Parsons, M. J., Kember, R., Fernandes, C., et al. (2010). Behavioural battery testing: Evaluation and behavioural outcomes in 8 inbred mouse strains. Physiol. Behav. 99, 301–316. doi: 10.1016/J.PHYSBEH.2009.11.007
Langan, J., and Seidler, R. D. (2011). Age differences in spatial working memory contributions to visuomotor adaptation and transfer. Behav. Brain Res. 225, 160–168. doi: 10.1016/j.bbr.2011.07.014
Leppä, E., Linden, A. M., Aller, M. I., Wulff, P., Vekovischeva, O., Luscher, B., et al. (2016). Increased motor-impairing effects of the neuroactive steroid pregnanolone in mice with targeted inactivation of the GABAA Receptor γ2 subunit in the cerebellum. Front. Pharmacol. 7:403. doi: 10.3389/FPHAR.2016.00403
Lohse, K. R., Sherwood, D. E., and Healy, A. F. (2011). Neuromuscular effects of shifting the focus of attention in a simple force production task. J. Mot. Behav. 43, 173–184. doi: 10.1080/00222895.2011.555436
Lucantonio, F., Kim, E., Su, Z., Chang, A. J., Bari, B. A., and Cohen, J. Y. (2021). Aversive stimuli bias corticothalamic responses to motivationally significant cues. eLife 10:e57634. doi: 10.7554/ELIFE.57634
Marcondes, F. K., Miguel, K. J., Melo, L. L., and Spadari-Bratfisch, R. C. (2001). Estrous cycle influences the response of female rats in the elevated plus-maze test. Physiol. Behav. 74, 435–440. doi: 10.1016/S0031-9384(01)00593-5
Martins, L. A., Schiavo, A., Xavier, L. L., and Mestriner, R. G. (2022). The foot fault scoring system to assess skilled walking in rodents: A reliability study. Front. Behav. Neurosci. 16:892010. doi: 10.3389/FNBEH.2022.892010
McDonald, W. M. (2011). Defining neurocognitive disorders. Am. J. Geriatr. Psychiatry 19, 909–914. doi: 10.1097/JGP.0B013E31823497C7
Medeiros, F. M., de Carvalho Myskiw, J., Baptista, P. P. A., Neves, L. T., Martins, L. A., Furini, C. R. G., et al. (2018). Can an aversive, extinction-resistant memory trigger impairments in walking adaptability? An experimental study using adult rats. Neurosci. Lett. 665, 224–228. doi: 10.1016/j.neulet.2017.12.017
Meier, S. M., Trontti, K., Purves, K. L., Als, T. D., Grove, J., Laine, M., et al. (2019). Genetic variants associated with anxiety and stress-related disorders: A genome-wide association study and mouse-model study. JAMA Psychiatry 76, 924–932. doi: 10.1001/JAMAPSYCHIATRY.2019.1119
Metz, G. A., and Whishaw, I. Q. (2002). Cortical and subcortical lesions impair skilled walking in the ladder rung walking test: A new task to evaluate fore- and hindlimb stepping, placing, and co-ordination. J. Neurosci. Methods 115, 169–179. doi: 10.1016/S0165-0270(02)00012-2
Metz, G. A., and Whishaw, I. Q. (2009). The ladder rung walking task: A scoring system and its practical application. J. Vis. Exp. 28:1204. doi: 10.3791/1204
Miedel, C. J., Patton, J. M., Miedel, A. N., Miedel, E. S., and Levenson, J. M. (2017). Assessment of spontaneous alternation, novel object recognition and limb clasping in transgenic mouse models of amyloid-β and tau neuropathology. J. Vis. Exp. 123:55523. doi: 10.3791/55523
Mora, S., Dussaubat, N., and Díaz-Véliz, G. (1996). Effects of the estrous cycle and ovarian hormones on behavioral indices of anxiety in female rats. Psychoneuroendocrinology 21, 609–620. doi: 10.1016/S0306-4530(96)00015-7
Morgan, J. A., Singhal, G., Corrigan, F., Jaehne, E. J., Jawahar, M. C., and Baune, B. T. (2018). The effects of aerobic exercise on depression-like, anxiety-like, and cognition-like behaviours over the healthy adult lifespan of C57BL/6 mice. Behav. Brain Res. 337, 193–203. doi: 10.1016/j.bbr.2017.09.022
Nguyen, P. V. (2006). Comparative plasticity of brain synapses in inbred mouse strains. J. Exp. Biol. 209, 2293–2303. doi: 10.1242/jeb.01985
Nodehi, Z., Mehdizadeh, H., Azad, A., Mehdizadeh, M., Reyhanian, E., Saberi, Z. S., et al. (2021). Anxiety and cognitive load affect upper limb motor control in Parkinson’s disease during medication phases. Ann. N. Y. Acad. Sci. 1494, 44–58. doi: 10.1111/NYAS.14564
Nolte, E. D., Nolte, K. A., and Yan, S. S. (2019). Anxiety and task performance changes in an aging mouse model. Biochem. Biophys. Res. Commun. 514, 246–251. doi: 10.1016/J.BBRC.2019.04.049
Orso, R., Creutzberg, K. C., Lumertz, F. S., Wearick-Silva, L. E., Sanches, E. F., Mestriner, R. G., et al. (2021). Early environmental enrichment rescues memory impairments provoked by mild neonatal hypoxia-ischemia in adolescent mice. Behav. Brain Res. 407:113237. doi: 10.1016/J.BBR.2021.113237
Paylor, R., Spencer, C. M., Yuva-Paylor, L. A., and Pieke-Dahl, S. (2006). The use of behavioral test batteries, II: Effect of test interval. Physiol. Behav. 87, 95–102. doi: 10.1016/J.PHYSBEH.2005.09.002
Pollock, A. S., Durward, B. R., Rowe, P. J., and Paul, J. P. (2000). What is balance? Clin. Rehabil. 14, 402–406. doi: 10.1191/0269215500cr342oa
Ramos, A. (2008). Animal models of anxiety: Do I need multiple tests? Trends Pharmacol. Sci. 29, 493–498. doi: 10.1016/j.tips.2008.07.005
Rodgers, R. J., and Dalvi, A. (1997). Anxiety, defence and the elevated plus-maze. Neurosci. Biobehav. Rev. 21, 801–810. doi: 10.1016/S0149-7634(96)00058-9
Rodgers, R. J., and Johnson, N. J. T. (1995). Factor analysis of spatiotemporal and ethological measures in the murine elevated plus-maze test of anxiety. Pharmacol. Biochem. Behav. 52, 297–303. doi: 10.1016/0091-3057(95)00138-M
Rodríguez-Landa, J. F., Guillén-Ruiz, G., Hernández-López, F., Cueto-Escobedo, J., Rivadeneyra-Domínguez, E., Bernal-Morales, B., et al. (2021). Chrysin reduces anxiety-like behavior through actions on GABA A receptors during metestrus-diestrus in the rat. Behav. Brain Res. 397:112952. doi: 10.1016/j.bbr.2020.112952
Rogers, D. C., Jones, D. N. C., Nelson, P. R., Jones, C. M., Quilter, C. A., Robinson, T. L., et al. (1999). Use of SHIRPA and discriminant analysis to characterise marked differences in the behavioural phenotype of six inbred mouse strains. Behav. Brain Res. 105, 207–217. doi: 10.1016/S0166-4328(99)00072-8
Runswick, O. R., Roca, A., Williams, A. M., Bezodis, N. E., and North, J. S. (2018). The effects of anxiety and situation-specific context on perceptual-motor skill: A multi-level investigation. Psychol. Res. 82, 708–719. doi: 10.1007/S00426-017-0856-8
Russell, W., and Burch, R. (1959). The principles of humane experimental technique. Available online at: http://117.239.25.194:7000/jspui/bitstream/123456789/1342/1/PRILIMINERY%20%20AND%20%20CONTENTS.pdf (accessed January 24, 2021).
Saré, R. M., Lemons, A., Smith, C. B., and Smith-Hicks, C. (2021). Behavior testing in rodents: Highlighting potential confounds affecting variability and reproducibility section on neuroadaptation and protein metabolism. Brain Sci. 11:522. doi: 10.3390/brainsci11040522
Scholl, J. L., Afzal, A., Fox, L. C., Watt, M. J., and Forster, G. L. (2019). Sex differences in anxiety-like behaviors in rats. Physiol. Behav. 211:112670. doi: 10.1016/J.PHYSBEH.2019.112670
Silva, R. H., and Frussa-Filho, R. (2000). The plus-maze discriminative avoidance task: A new model to study memory-anxiety interactions. Effects of chlordiazepoxide and caffeine. J. Neurosci. Methods 102, 117–125. doi: 10.1016/S0165-0270(00)00289-2
Smith, L. K., and Metz, G. A. (2005). Dietary restriction alters fine motor function in rats. Physiol. Behav. 85, 581–592. doi: 10.1016/j.physbeh.2005.06.013
Smith, N. C., Bellamy, M., Collins, D. J., and Newell, D. (2001). A test of processing efficiency theory in a team sport context. J. Sports Sci. 19, 321–332. doi: 10.1080/02640410152006090
Smoller, J. W., and Tsuang, M. T. (1998). Panic and phobic anxiety: Defining phenotypes for genetic studies. Am. J. Psychiatry 155, 1152–1162. doi: 10.1176/AJP.155.9.1152
Sorrentino, P., Lardone, A., Pesoli, M., Liparoti, M., Montuori, S., Curcio, G., et al. (2019). The development of spatial memory analyzed by means of ecological walking task. Front. Psychol. 10:728. doi: 10.3389/fpsyg.2019.00728
Strekalova, T., Spanagel, R., Dolgov, O., and Bartsch, D. (2005). Stress-induced hyperlocomotion as a confounding factor in anxiety and depression models in mice. Behav. Pharmacol. 16, 171–180. doi: 10.1097/00008877-200505000-00006
Tan, S., Hong, C. T., Chen, J. H., Chan, L., Chi, W. C., Yen, C. F., et al. (2020). Hand fine motor skill disability correlates with cognition in patients with moderate-to-advanced Parkinson’s Disease. Brain Sci. 10:337. doi: 10.3390/brainsci10060337
Trullas, R., and Skolnick, P. (1993). Differences in fear motivated behaviors among inbred mouse strains. Psychopharmacology 111, 323–331. doi: 10.1007/BF02244948
Van der Staay, F. J. (2006). Animal models of behavioral dysfunctions: Basic concepts and classifications, and an evaluation strategy. Brain Res. Rev. 52, 131–159.
Viola, T. W., Creutzberg, K. C., Zaparte, A., Kestering-Ferreira, É, Tractenberg, S. G., Centeno-Silva, A., et al. (2019). Acute neuroinflammation elicited by TLR-3 systemic activation combined with early life stress induces working memory impairments in male adolescent mice. Behav. Brain Res. 376:112221. doi: 10.1016/J.BBR.2019.112221
Võikar, V., Vasar, E., and Rauvala, H. (2004). Behavioral alterations induced by repeated testing in C57BL/6J and 129S2/Sv mice: Implications for phenotyping screens. Genes Brain Behav. 3, 27–38. doi: 10.1046/j.1601-183X.2003.0044.x
Vorhees, C. V., and Williams, M. T. (2014). Assessing spatial learning and memory in rodents. ILAR J. 55, 310–332. doi: 10.1093/ilar/ilu013
Wankel, L. M. (2014). Audience size and trait anxiety effects upon state anxiety and motor performance. Res. Q. 48, 181–186. doi: 10.1080/10671315.1977.10762168
Wearick-Silva, L. E., Orso, R., Martins, L. A., Creutzberg, K. C., Centeno-Silva, A., Xavier, L. L., et al. (2019). Dual influences of early life stress induced by limited bedding on walking adaptability and Bdnf/TrkB and Drd1/Drd2 gene expression in different mouse brain regions. Behav. Brain Res. 359, 66–72. doi: 10.1016/j.bbr.2018.10.025
Wilson, M. R. (2012). “Anxiety: Attention, the brain, the body, and performance,” in The Oxford handbook of sport and performance psychology, ed. S. M. Murphy (Oxford: Oxford University Press), 173–190. doi: 10.1093/OXFORDHB/9780199731763.013.0009
Yilmazer-Hanke, D. M., Roskoden, T., Zilles, K., and Schwegler, H. (2003). Anxiety-related behavior and densities of glutamate, GABAA, acetylcholine and serotonin receptors in the amygdala of seven inbred mouse strains. Behav. Brain Res. 145, 145–159. doi: 10.1016/S0166-4328(03)00107-4
Yogev-Seligmann, G., Hausdorff, J. M., and Giladi, N. (2008). The role of executive function and attention in gait. Mov. Disord. 23, 329–342. doi: 10.1002/mds.21720
Keywords: skilled walking, gait, motor control, anxiety-like, spatial memory, mice
Citation: Schiavo A, Martins LA, Wearick-Silva LE, Orso R, Xavier LL and Mestriner RG (2023) Can anxiety-like behavior and spatial memory predict the extremes of skilled walking performance in mice? An exploratory, preliminary study. Front. Behav. Neurosci. 17:1059029. doi: 10.3389/fnbeh.2023.1059029
Received: 30 September 2022; Accepted: 26 January 2023;
Published: 28 February 2023.
Edited by:
Juan Francisco Rodríguez-Landa, Universidad Veracruzana, MexicoReviewed by:
Jovana Joksimovic Jovic, University of Kragujevac, SerbiaMarta Méndez-Couz, Ruhr University Bochum, Germany
Jonathan Cueto-Escobedo, Universidad Veracruzana, Mexico
Copyright © 2023 Schiavo, Martins, Wearick-Silva, Orso, Xavier and Mestriner. This is an open-access article distributed under the terms of the Creative Commons Attribution License (CC BY). The use, distribution or reproduction in other forums is permitted, provided the original author(s) and the copyright owner(s) are credited and that the original publication in this journal is cited, in accordance with accepted academic practice. No use, distribution or reproduction is permitted which does not comply with these terms.
*Correspondence: Régis Gemerasca Mestriner, regis.mestriner@pucrs.br; orcid.org/0000-0001-9837-1691