- 1Department of Molecular and Cellular Biology, University of California, Berkeley, Berkeley, CA, United States
- 2Helen Wills Neuroscience Institute, University of California, Berkeley, Berkeley, CA, United States
- 3Department of Psychology, University of California, Berkeley, Berkeley, CA, United States
Dispersal from the natal site or familial group is a core milestone of adolescent development in many species. A wild species of mouse, Mus spicilegus, presents an exciting model in which to study adolescent development and dispersal because it shows different life history trajectory depending on season of birth. M. spicilegus born in spring and summer on long days (LD) disperse in the first 3 months of life, while M. spicilegus born on shorter autumnal days (SD) delay dispersal through the wintertime. We were interested in using these mice in a laboratory context to compare age-matched mice with differential motivation to disperse. To first test if we could find a proxy for dispersal related behavior in the laboratory environment, we measured open field and novel object investigation across development in M. spicilegus raised on a LD 12 h:12 h light:dark cycle. We found that between the first and second month of life, distance traveled and time in center of the open field increased significantly with age in M. spicilegus. Robust novel object investigation was observed in all age groups and decreased between the 2nd and 3rd month of life in LD males. Compared to male C57BL/6 mice, male M. spicilegus traveled significantly longer distances in the open field but spent less time in the center of the field. However, when a novel object was placed in the center of the open field, Male M. spicilegus, were significantly more willing to contact and mount it. To test if autumnal photoperiod affects exploratory behavior in M. spicilegus in a laboratory environment, we reared a cohort of M. spicilegus on a SD 10 h:14 h photoperiod and tested their exploratory behavior at P60-70. At this timepoint, we found SD rearing had no effect on open field metrics, but led to reduced novel object investigation. We also observed that in P60-70 males, SD reared M. spicilegus weighed less than LD reared M. spicilegus. These observations establish that SD photoperiod can delay weight gain and blunt some, but not all forms of exploratory behavior in adolescent M. spicilegus.
Introduction
Exploratory behavior during adolescence is a topic of great interest to psychology, psychiatry, and public health as well as integrated biology. While exploratory behavior may serve many functions, we were particularly interested in identifying exploratory behavior in rodents in a laboratory environment that could be related to natal dispersal.
Natal dispersal is a dramatic behavior observed in most vertebrates that often occurs during adolescence. Dispersal occurs when animals, typically males, but sometimes females, leave their site of birth (or hatching) to roam more broadly or to inhabit a new site where reproduction will occur. By definition, dispersing individuals increase their spatial exploration and with this exploration they encounter new and potentially threatening novel objects in their environment. Natal dispersal typically occurs before reproductive maturity, but not in all species. Hypotheses for the ultimate evolutionary causes of dispersal include competition for mates, competition for resources, and inbreeding avoidance (Greenwood and Harvey, 1982; Pusey and Wolf, 1996; Alonso et al., 1998; Long et al., 2008).
It is not well understood what proximate neural mechanisms drive natal dispersal on an acute timescale, although attainment of sufficient weight, gonadal and adrenal hormones are thought to play a role (Howard, 1960; Holekamp, 1984; O’Riain et al., 1996; Dufty and Belthoff, 2001; Duckworth, 2009; Duckworth and Sockman, 2012). External triggers may include social factors such as parental and sibling aggression (Gerlach, 1990). Dispersal in some species is regulated by photoperiodism, where day length can modulate physiological factors to trigger dispersal (Walton et al., 2011).
Mus spicilegus is a wild mouse species of particular interest to studies of adolescent development due to its seasonally dependent life history as well as its interesting mound building behavior (Poteaux et al., 2008; Simeonovska-Nikolova and Mehmed, 2009; Tong and Hoekstra, 2012; Couger et al., 2018). M. spicilegus are primarily found in agricultural ecosystems throughout Eastern Europe. Depending on the time of year in which they are born, M. spicilegus have different timelines for dispersal and mating as well as different social structures. Mice born in spring and early summer, a long day (LD) cohort, disperse from their natal nest and mate after 2–3 months; they do not live through the winter and have shorter lives compared to their autumnal-born counterparts (Gouat et al., 2003b; Poteaux et al., 2008). Conversely, the autumnal or short day (SD) cohort, born in late summer and fall, cooperatively build large, earthen mounds made from foraged materials in which they overwinter with related kin; delaying dispersal and reproduction 6–8 months until the following spring (Poteaux et al., 2008; Szenczi et al., 2011; Csanády et al., 2020). Thus, mice born in these different seasons have considerably different life trajectories. Based on these observations in the wild, we postulated that dispersal in M. spicilegus could be regulated by photoperiod and sought to test effects of photoperiod on exploration in a laboratory environment. We postulated that M. spicilgeus reared on LD light would express motivation to disperse during the first 2–4 months of life while those reared on SD light would not express the same level of motivation in this time frame. In this initial study we decided to use measures of exploratory behavior that are commonly assessed in domesticated rodents in the laboratory environment but that also may be putatively related to dispersal. We reasoned that if these behaviors served as a reliable proxy metric for the motivation to disperse, they would show A) increases with development in the first 2–4 months of life in LD light cycles, and B) relative suppression in SD reared compared to LD reared M. spicilegus.
To test (A) we first measured distance traveled in an open field and novel object investigation behavior in male and female M. spicilegus born on a LD 12 h day length. For reference, we also compared male M. spicilegus exploratory behavior to male C57 BL/6 mice. To test (B), we examined if a SD rearing reduced the expression of exploratory behavior at a peak adolescent timepoint in male M. spicilegus.
Materials and methods
Animals
Male and female M. spicilegus mice (derived from Kalomoyevka, Ukraine) were maintained as a colony from a limited founder population obtained from France (courtesy of the lab of François Bonhomme).
M. spicilegus were reared in one of two different photoperiods meant to mimic spring/summer and autumnal conditions. One cohort of M. spicilegus was maintained on a 12 h:12 h light:dark cycle (LD) mimicking spring/summer conditions (12 h day length is commonly employed in animal facilities and reflects daylight hours first reached in March in Ukraine. A limitation of this study is that 12 h daylight is also experienced in Ukraine in September). A second cohort of M. spicilegus was reared on a shorter 10:14 h light:dark cycle (SD) mimicking autumnal conditions (day length is 10 h in late October in Ukraine). To attain mice born on the 10:14 h light cycle, some dams were moved to this light cycle pregnant and others bred on this light cycle. To study weight at P60-79 on the LD and SD light cycles, we used data from mice who ran through the battery of tasks as well as data from mice who were only weighed.
All mice were weaned at P21 and housed with 2–3 same-sex siblings and nesting material. For age comparison, LD reared mice were tested in 4 age group bins: P21-33; P35-45; P60-79; P80-125. Our age bins were informed by pubertal ages in C57 BL/6 mice who are reaching the first estrus ∼P35 and breeding ∼P60 and field work in M. spicilegus that suggests dispersal occurs 2–3 months after birth on LD photoperiods (Lafaille et al., 2015). Our largest sample came from LD reared male M. spicilegus: P21-P33 (n = 18), P35-P45 (n = 11), P60-P79 (n = 29), and P80-P125 (n = 11). Female LD reared M. spicilegus were tested across a comparable age range and binned into the same four age groups: P21-P33 (n = 7), P35-P45 (n = 7), P60-P79 (n = 10), and P80-P125 (n = 12). Male C57 BL/6 were tested across the same age range: P21-P33 (n = 9), P35-P45 (n = 15), P60-P79 (n = 9), and P80-P125 (n = 8). Male C57BL/6 mice were housed on a 12 h:12 h reverse light cycle. We did not study female C57 BL/6 mice.
All behavioral procedures were approved by the Animal Care and Use Committee at The University of California, Berkeley.
Open field
Mice were placed in the center of a transparent acrylic arena with 42 cm by 42 cm floor dimensions, 30.5 cm high walls, and a removable, perforated lid (Figure 1A). The arena was housed within a sound-attenuated chamber (Med Associates; Fairfax, VT) with lights and a fan in the interior. Mice were allowed to explore the arena undisturbed for 15 min. Movement was monitored in the arena using infrared beam brakes (Versamax, AccuScan Instruments; Columbus, OH). Behavioral metrics analyzed included total distance traveled (cm) and time in center (center defined as > 7.875 cm from the walls of the arena). The arena was wiped down with 70% ethanol and allowed to dry before adding each animal. Mice were only tested once.
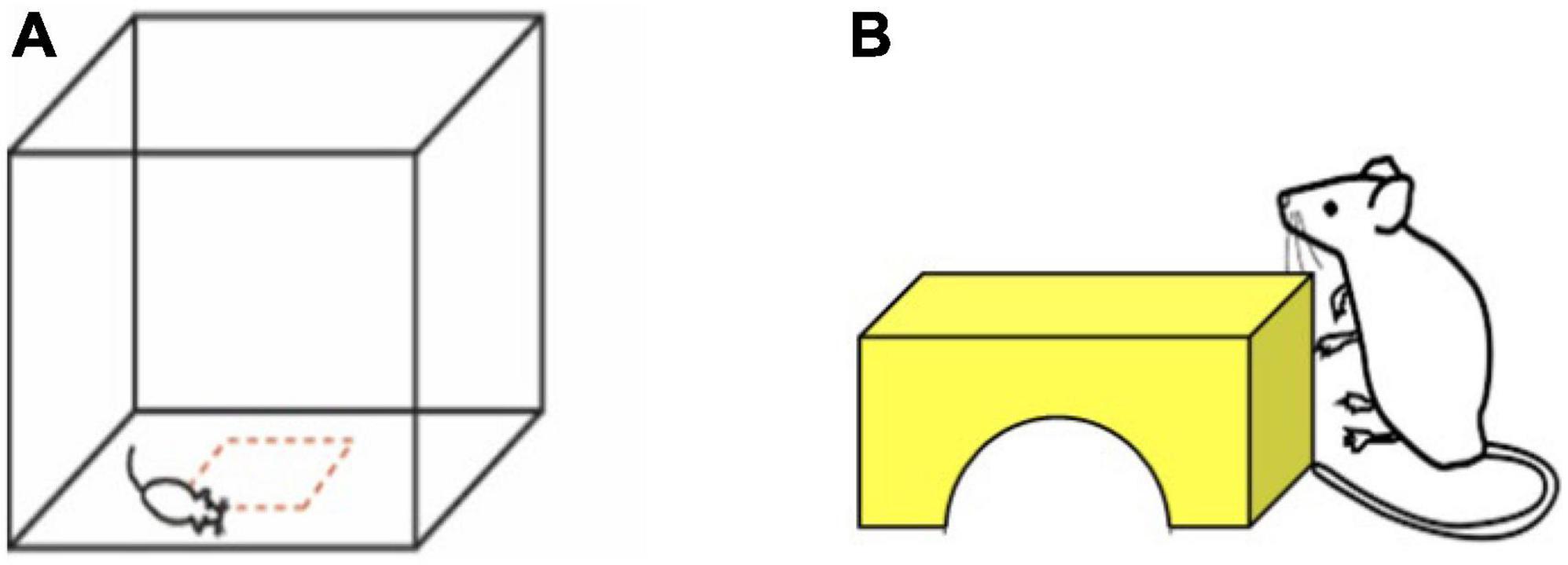
Figure 1. Open field and novel object investigation were used to measure exploratory behavior in the laboratory environment. (A) Open field area with center area defined in red. We measured distance traveled in the arena and time in center in a 15 min test. (B) Novel object investigation. We measured time contacting object and time on top of the object in a 5 min test. The shape, color, and size of the object was kept consistent.
Novel object investigation
Immediately following testing in the open field, mice were momentarily covered with a large plastic cup and gently moved to one corner of the arena. A 2 inch high yellow block bridge was placed in the center of the arena facing the corner in which the mouse was covered (Figure 1B). The cup was then removed, the doors to the sound-attenuated chamber were closed, and mice were given 5 min to explore the open field arena with the novel object present. Mice were removed from the open field arena after 5 min, weighed, and returned to their home cage. The placement of the object in the center of the arena, as well as novelty of the object both likely contribute to the challenge of this task. Novel object investigation behavior was analyzed using the Boris animal tracking software (Friard and Gamba, 2016). We scored time contacting the object and time on top of the object.
Statistical analyses
Statistical comparisons were made using Graphpad Prism software. We examined main effects of age and sex (or age and species) and their interactions using 2 way ANOVA. We used Sidak’s multiple comparison test to compare age groups within sex or species and report adjusted p-values. LD and SD males were compared using Mann-Whitney U-test.
Results
Long day male and female Mus spicilegus show increases in exploratory behavior in the open field during the first 3 months of life
In our first experiment, LD male and female M. spicilegus underwent open-field testing followed by a novel object investigation test. Each individual was tested only once. We found a significant main effect of age but not of sex on distance traveled [two-way ANOVA, age: F(3, 97) = 14.9, p < 0.0001; sex: F(1, 97) = 0.70, p = 0.41] (Figure 2A). We found no significant interaction between age and sex [F(3, 97) = 1.44, p = 0.24]. Post hoc comparisons revealed that distance traveled was significantly lower at P21-P33 compared to older ages in males and females (21-33 vs. P35-P45: male p = 0.0002, female p = 0.31; P21-33 vs. P60-79: male p < 0.0001, female p < 0.0001; P21-33 vs. P80-125: male p = 0.0006, female p = 0.018). Other age comparisons were non-significant. In the heavily sampled P60-79 male group, there was no significant linear relationship between weight and distance traveled (R2 = 0.01, p = 0.78).
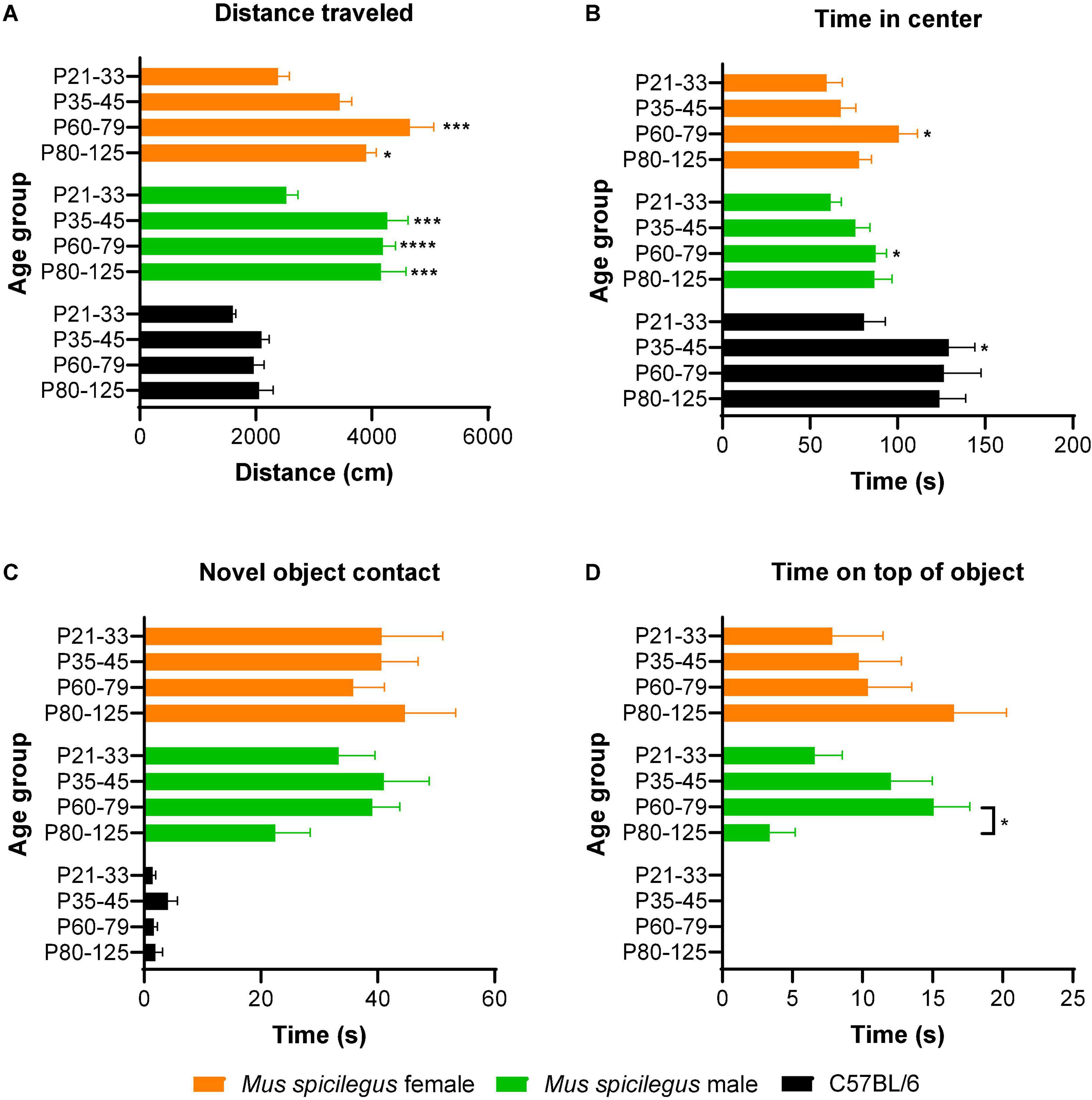
Figure 2. Male and female Mus spicilegus reared on LD photoperiod show comparable adolescent development of exploratory behavior that differs from that seen in C57BL/6 males. (A) Distance traveled in the open field. Male M. spicilegus showed a significant increase in distance traveled in the open field in the transition between the end of the first month (P21-33) and second month of life (P35 +) (P < 0.001). In female M. spicilegus, there was a significant increase in distance traveled between P21-P33 and P60-P79 (p < 0.0001). No main effect of sex was observed. Comparisons between males of two species showed a significant main effect of age as well as species on distance traveled (age: p = 0.0002; species: p < 0.0001). Male M. spicilegus traveled more than C57 BL/6 males in the open field (species: p < 0.0001). No significant changes with age were observed in this metric in C57 BL/6 males. (B) Time in center of open field. There was no effect of sex but a significant effect of age on time in center of the open field in M. spicilegus. Male and female M. spicilegus showed a significant increase in time in center of the open field in the transition between the end of the first month (P21-33) and end of the second month of life (P60-79) (P < 0.05). No main effect of sex was observed. Comparisons between males of two species showed a significant main effect of age as well as species on time in the center (age: p = 0.007; species: p < 0.0001). C57 Bl/6 showed a gain in this metric from P21-33 to P35-45 P = 0.03). (C) Novel object contact. There was no significant main effect of sex or age on novel object contact in M. spicilegus. Species comparison showed a significant main effect of species but not age on novel object contact (age: p = 0.37; species: p < 0.0001). (D) Time on top of novel object (mounting). There was no significant main effect of sex but a significant effect of age on time on top of novel object in M. spicilegus groups. Male M. spicilegus showed a significant decrease in time spent on top of the novel object between P60-P79 and P80-P125 (p = 0.04). While M. spicilegus readily mounted the object even at the youngest ages, male C57/Bl/6 largely did not express this behavior in the ages sampled (age: p = 0.11; species: p < 0.0001). *p < 0.05, ***p < 0.001, ****p < 0.0001 (All * without lines are relative to P21-33 group).
There was a significant main effect of age but not of sex on time spent in the center of the open field [two-way ANOVA, age: F(3, 97) = 5.57, p = 0.001; sex: F(1, 97) = 0.06, p = 0.81], and no significant interaction between age and sex [F(3, 97) = 0.8, p = 0.50] (Figure 2B). Post hoc comparisons revealed there was a significant increase in time in center between P21-33 and P60-79 (male p = 0.03, female p = 0.04) (Figure 2B). There were no significant differences in time spent in the center of the open field between other groups (p > 0.14). In the heavily sampled P60-79 male group, there was no significant linear relationship between weight and time in center (R2 = 0.02, p = 0.34).
There was no significant main effect of age or sex on time spent contacting the novel object [two-way ANOVA, age: F(3, 97) = 0.29, p = 0.83; sex: F(1, 97) = 1.49, p = 0.23] (Figure 2C). There was no interaction between age and sex [two-way ANOVA: F(3, 97) = 1.28, p = 0.29] (Figure 2C). In the heavily sampled P60-79 male group there was no significant linear relationship between weight and novel object contact (R2 = 0.04, p = 0.66).
There was no significant main effect of age or sex on time on top of the novel object [two-way ANOVA, age: F(3, 97) = 1.05, p = 0.37; sex: F(1, 97) = 0.63, p = 0.43] (Figure 2D). There was a significant interaction between age and sex [two-way ANOVA: F(3, 97) = 3.16, p = 0.028] (Figure 2D). Post hoc comparisons revealed in males that the P60-P79 group spent significantly greater time on top of the novel object than the P80-P125 group (p = 0.04). Post hoc comparisons showed no significant difference between other age groups in metrics of time on top of the novel object (p > 0.12). In the heavily sampled P60-79 male group, there was no significant linear relationship between weight and distance traveled (R2 < 0.01, p = 0.99).
Male Mus spicilegus exploratory behavior differs from C57BL/6
Next we compared the open field and novel object investigation performance of C57BL/6 males with M. spicilegus males across development. We ran a two-way ANOVA to assess the effect of age and species on the four exploratory metrics (distance traveled, time in center, time contacting object and time on top of the object).
M. spicilegus males traveled further in the open field than C57BL/6 males (Figure 2A). There was a significant main effect of species as well as age on distance traveled [two-way ANOVA, age: F(3, 102) = 7.35, p = 0.0002; species: F(1, 102) = 87.43, p < 0.0001] (Figure 2A). There was no significant interaction between age and species [two-way ANOVA: F(3, 102) = 2.56, p = 0.06] (Figure 2A).
Conversely, male C57BL/6 spent more time in the center of the open field than male M. spicilegus at all age groups (Figure 2B). There was a significant main effect of species as well as age on time in the center [two-way ANOVA, age: F(3, 102) = 4.26, p = 0.007; species: F(1, 102) = 20.0, p < 0.0001] (Figure 2B). There was no significant interaction between age and species [two-way ANOVA: F(3, 102) = 0.750, p = 0.53] (Figure 2B). Post hoc comparisons revealed that within the C57 BL/6 males, there was a significant increase in time in center between P21-33 and P35-45 (p = 0.03).
M. spicilegus males contacted the novel object more than male C57BL/6 for all 4 age bins (Figure 2C). There was a significant main effect of species but not age on novel object contact [two-way ANOVA, age: F(3, 102) = 1.06, p = 0.37; species: F(1, 102) = 58.4, p < 0.0001] (Figure 2C). There was no significant interaction between age and species [two-way ANOVA: F(3, 102) = 0.80, p = 0.50] (Figure 2C).
No C57BL/6 ever climbed on top of the novel object whereas a majority of the M. spicilegus did (Figure 2D). There was a significant main effect of species but not age on time spent on top of the novel object [two-way ANOVA, age: F(3, 102) = 2.06, p = 0.11; species: F(1, 102) = 26.1, p < 0.0001] (Figure 2D). There was no significant interaction between age and species [two-way ANOVA: F(3, 102) = 2.06, p = 0.11] (Figure 2D).
In P60-79 males, short day photoperiod rearing affected weight and novel object investigation
In our final experiment we sought to use photoperiod to delay or blunt the increase in exploratory behavior in Mus spicilegus. We reasoned that if M. spicilegus born in SD autumnal photoperiod delay their dispersal in the wild, an autumnal photoperiod in the lab might delay an increase in exploratory behavior seen in the first months of life in LD conditions. To this end, we reared M. spicilegus in a SD 10 h:14 h light:dark cycle in the laboratory (Figure 3A).
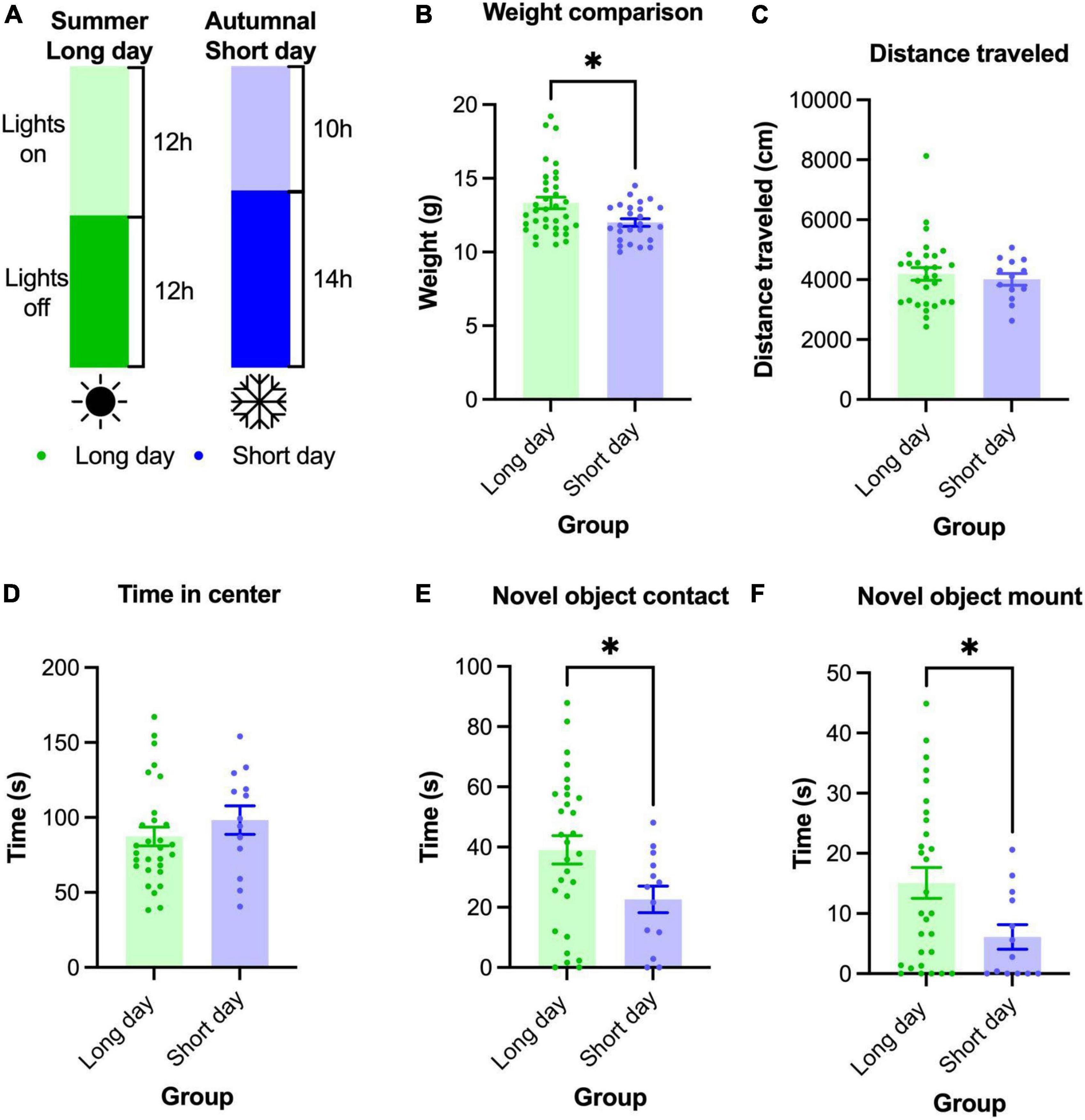
Figure 3. Adolescent (P60-P70) male M. spicilegus reared in SD photoperiod weighed less and investigated the novel object less than those reared in LD photoperiod. (A) Male M. spicilegus were reared in two different photoperiods. (B) Weight data from SD 14 h:10 h and LD 12 h:12 h reared male mice. SD males weighed significantly less than LD males (p = 0.024) (C) There was no significant difference in distance traveled in the open field between animals reared in SD and LD (p = 0.78). (D) There was no significant difference in time spent in the center of the open field between SD and LD (p = 0.28). (E) SD males contacted the novel object significantly less than LD males (p = 0.037). (F) SD males spent less time on top of the novel object than LD males (p = 0.037). These data demonstrate that photoperiod can significantly affect adolescent development in M. spicilegus reared in the laboratory. *p < 0.05 Mann-Whitney U-test.
Our first assay of mice reared in the SD protocol was body weight (the sample size for this group was larger than the sample size we were able to test in behavior). We found that SD reared mice showed significantly lower body weight than LD reared mice (SD 12.0 ± 0.25 g, n = 25; LD 13.3 ± 0.39 g, n = 35 (U = 288, p = 0.024) (Figure 3B).
We were able to test n = 13 male M. spicilegus reared on SD light and compare them to n = 29 male M. spicilegus reared on LD light (data shown above in age and sex comparisons). Contrary to our prediction, we found no significant difference between groups in distance traveled in the open field (U = 178, p = 0.78) and no significant difference in time spent in the center of the open field between males reared in LD and SD photoperiod (U = 148, p = 0.28) (Figures 3C,D). In the novel object test, P60-P79 male M. spicilegus reared in the SD photoperiod contacted the novel object for significantly less time than those reared in the LD photoperiod (U = 112, p = 0.037) (Figure 3E). Adolescent male M. spicilegus reared in SD photoperiod also spent significantly less time on top of the novel object than those reared in the longer photoperiod (U = 113, p = 0.037) (Figure 3F). These data establish novel object investigation was blunted by exposure to the SD photoperiod in male M. spicilegus.
Discussion
Here we examined the maturation of exploratory behavior in lab based male and female M. spicilegus and tested if a photoperiod manipulation could blunt exploration behaviors. We found that distance traveled in the open field increased significantly between P21-P33 and P60-P79 in both male and female M. spicilegus reared in LD 12 h:12 h light:dark cycle in our lab. Robust novel object investigation was also observed in developing male and female M. spicilegus but did not show significant increases over the developmental time period we sampled. Our LD data showing increased in distance traveled are possibly consistent with motivation to disperse. In “the field,” M. spicilegus dispersal has been determined to occur in the first 2–3 months of life on long days (Lafaille et al., 2015) and in tests of exploratory behavior in the lab with M. spicilegus reared on a LD laboratory environment (Lafaille and Féron, 2014). However, alternate explanations for the observed developmental increases should also be considered (see more on this below).
When we compared male LD reared M. spicilegus behavior to C57 Bl/6 mice reared on the a 12 h:12 h light:dark cycle, we found M. spicilegus traveled longer distances than C57BL/6 lab mice; yet avoided the center of the empty open field more than their C57BL/6 counterparts. LD M. spicilegus were also bolder in their interactions with a novel object. Here species differences are also entwined with domestication history. We speculate less time spent in center in M. spicilegus reflect a strategic, predation-avoidant behavior that may have been lost over time in captivity in C57 Bl/6 mice.
Next, we used a photoperiod manipulation to test if rearing mice on SD light 10 h:14 h light: Dark in the lab was sufficient to blunt weight gain or exploratory behavior in male adolescent M. spicilegus. We found SD reared males achieved significantly lower weights at P60-70 and showed lower metrics of novel object investigation than LD reared M. spicilegus. These data suggest a photoperiod manipulation is sufficient to impact some aspects of physiology and behavior in M. spicilegus in the laboratory. This is an important first milestone and establishes that we can use photoperiod to regulate development in mice in the laboratory. This is significant because it is thought not to be possible in most inbred mouse lines due to their lack of melatonin (Kennaway, 2019).
While these results did uncover some developmental and photoperiod sensitive increases in exploratory behaviors, they did not fully meet the two pre-conditions we laid out for our goal. We found one set of behavioral metrics, open field distance traveled, that increased with development, but it was another set of metrics, novel object contact and mounting, that were blunted by SD photoperiod. We therefore cannot logically consider open field exploration or novel object investigation metrics as a stand-alone proxy for adolescent motivation to disperse. A battery of behavioral assays, or more ethological assays may serve as better indicators or markers.
As we develop a broader battery, we must also consider the emergence of behavioral changes related to mound building in M. spicilgeus. Given that wild living M. spicilegus born in the fall or winter months engage in mound building before overwintering and delaying dispersal until the following spring (Simeonovska-Nikolova and Mehmed, 2009; Szenczi et al., 2011; Hurtado et al., 2013), it is possible that adolescent SD M. spicilegus develop some form of exploratory behavior at the same age as LD M. spicilegus, to support mound building, or travel to mounds, but not dispersal over longer distances or toward reproductive ends. Again, further research using more ethological assays (see Hurtado et al., 2013) and photoperiod manipulations may help inform this question of the meaning of the expression of behavior in the lab.
Lack of sex differences on long day photoperiod
The lack of sex differences in our LD experiments is also of significant interest as a finding. Previous studies have differed in their observation of sex differences in this species but this may be explained by age and light cycle (Garza et al., 1997; Simeonovska-Nikolova, 2000; Poteaux et al., 2008; Groo et al., 2013; Lafaille and Féron, 2014). In Groo et al. (2013) evaluated dispersal timing in LD 12 h:12 h light:dark laboratory conditions by modeling dispersal with an apparatus that required mice to cross a pool of water. Animals were tested up to the age of P120 and no significant differences between sexes were observed. Lafaille and Féron (2014) reared M. spicilegus in LD 14 h:10 h light:dark conditions and tested them in an open field test, elevated plus maze, and novel object investigation test. They found young mice (2 months) and old mice (24 months) to be similarly hyper-exploratory in both sexes when reared in constant LD summer-like photoperiod (Lafaille and Féron, 2014). However, by 6 months, females showed reduced exploration while male behavior remained unchanged. Simeonovska-Nikolova (2000) studied M. spicilegus caught directly from wild populations between spring and fall and found that males more actively explored their environment than females. The exact ages were unknown but all individuals were sexually mature adults. Since all animals were sexually mature, it is reasonable to assume they were over 2–3 months old and likely even older. These results may then corroborate exploratory measurements from Lafaille and Féron (2014) in suggesting exploration begins to decline with age earlier in females than in males for the cohort born in summer. In combination, these studies suggest that in M. spicilegus, the initial emergence of exploratory behavior gains for summer-born individuals is symmetric between sexes (in first 3–4 months), but may diverge at older ages.
Different mating systems are typically associated with different, sex specific dispersal trends (Dieckmann et al., 1999). M. spicilegus are primarily thought to be socially monogamous, as they exhibit characteristics such as pair bonding, considerable olfactory bulb neurogenesis in response to their mate, biparental care of young, and heightened aggression toward unfamiliar conspecifics (Patris and Baudoin, 2000; Baudoin et al., 2005). Others have argued for a polygynous mating system in M. spicilegus, though evidence for this has only been observed in the early spring, directly following dispersal (Gouat et al., 2003a; Bardet et al., 2007; Poteaux et al., 2008). It is possible that the sex differences in dispersal behavior in the LD born M. spicilegus cohort differ from the SD overwintering M. spicilegus. There is good evidence of male biased dispersal from mounds following overwintering in the wild (Greenwood, 1980; Poteaux et al., 2008; Simeonovska-Nikolova, 2012; Csanády et al., 2020). Genetic studies of wild populations have shown that females remain near the mounds after overwintering while males disperse greater distances (Poteaux et al., 2008; Simeonovska-Nikolova, 2012). Comparable comprehensive genetic studies of the summer cohort dispersal dynamics have not been conducted to our knowledge. Based on these data, we predict that sex differences may emerge in a laboratory based study of M. spicilegus raised on SD light cycles at older ages.
We also consider that social factors may also contribute to seasonal sex differences in dispersal. The sexual maturity of females has been found to be impacted by social environment (Féron and Gheusi, 2003; Gouat et al., 2003b). In the summer, females are particularly aggressive toward other females, and pregnant females demonstrate heightened levels of aggression compared to non-pregnant females (Simeonovska-Nikolova, 2012; Ambaryan et al., 2019). In the summer, there is no evidence of group living or cooperation other than between mated pairs (Milishnikov et al., 1998). In the wild, these changing environmental and social conditions may differentially impact males and females and social partners may need to be included to capture the role of these influences on studies dispersal in the laboratory.
Limitations and future directions
Our study used highly simplified metrics of exploration to assay behavior. More ethological tasks have been invented to study M. spicilegus behavior (Groo et al., 2013; Hurtado et al., 2013) and could be applied with photoperiod manipulation to test how photoperiod regulates dispersal related behavior in laboratory conditions. Future experiments may also consider: (1) More accurate mimicry of season through longer LD day length, changing day length over time and including a temperature manipulation as well as a photoperiod manipulation; (2) studying behavior over time periods longer than 3 months, (3) studying females more closely; and (4) allowing social factors to influence behavior. Furthermore, measures of gonadal hormonal status and corticosterone may also help inform our understanding of adolescent development and photoperiod in M spicilegus.
Conclusion
We examined the adolescent development of exploratory behavior M. spicilegus, a mouse model which may offer an exciting opportunity to use photoperiod to control the emergence of dispersal behavior. In this wild-derived mouse reared on LD photoperiods, we observed high levels of novel object investigation at juvenile and adolescent ages and robust increases in exploratory behavior in the open field over the first 3 months of life. When we manipulated photoperiod to mimic SD “autumnal” conditions that delay dispersal in the wild, we found we significantly blunted weight gain and novel object investigation metrics, but not open field metrics. These data establish that photoperiod can impact the adolescent development of male M. spicilegus weight and exploratory behavior in captivity. The relevance of these changes to dispersal and mound building behavior will need to be informed by further work using more ethological assays.
Data availability statement
The raw data supporting the conclusions of this article are available in Supplementary materials.
Ethics statement
This animal study was reviewed and approved by the Animal Care and Use Committee, University of California, Berkeley.
Author contributions
NC conceptualized experiments, ran the experiments, analyzed the data, and wrote the manuscript. GP and WL conceptualized experiments, ran experiments, supervised experiments, and contributed to the analyses and manuscript. OK, WC, and NM ran experiments and contributed to the analyses and manuscript. LW conceptualized and supervised experiments, data analyses, and wrote the manuscript. All authors contributed to the article and approved the submitted version.
Funding
This work was supported by a Miller Professorship from the Miller Institute for Basic Science Research at University of California, Berkeley. Publication was supported in part by the Berkeley Research Impact Initiative (BRII) sponsored by the UC Berkeley Library.
Acknowledgments
We thank François Bonhomme for providing the mice, Lance Kriegsfeld, Juliana Chase, Albert Qu, and Fernanda Castro for discussion.
Conflict of interest
The authors declare that the research was conducted in the absence of any commercial or financial relationships that could be construed as a potential conflict of interest.
Publisher’s note
All claims expressed in this article are solely those of the authors and do not necessarily represent those of their affiliated organizations, or those of the publisher, the editors and the reviewers. Any product that may be evaluated in this article, or claim that may be made by its manufacturer, is not guaranteed or endorsed by the publisher.
Supplementary material
The Supplementary Material for this article can be found online at: https://www.frontiersin.org/articles/10.3389/fnbeh.2022.988033/full#supplementary-material
References
Alonso, J. C., Martín, E., Alonso, J. A., and Morales, M. B. (1998). Proximate and ultimate causes of natal dispersal in the great bustard Otis tarda. Behav. Ecol. 9, 243–252.
Ambaryan, A. V., Voznessenskaya, V. V., and Kotenkova, E. V. (2019). Mating behavior differences in monogamous and polygamous sympatric closely related species. Mus musculus and Mus spicilegus and their role in behavioral precopulatory isolation. Russ. J. Theriol. 18, 67–79.
Bardet, J., Essen, D. K., Féron, C., and Gouat, P. (2007). Evaluation of the social bond: A new method tested in Mus spicilegus. C. R. Biol. 330, 837–843. doi: 10.1016/j.crvi.2007.08.006
Baudoin, C., Busquet, N., Dobson, F. S., Gheusi, G., Feron, C., Durand, J.-L., et al. (2005). Male-female associations and female olfactory neurogenesis with pair bonding in Mus spicilegus. Biol. J. Linn. Soc. 84, 323–334.
Couger, M. B., Arévalo, L., and Campbell, P. (2018). A high quality genome for Mus spicilegus, a close relative of house mice with unique social and ecological adaptations. G3 8, 2145–2152. doi: 10.1534/g3.118.200318
Csanády, A., Stanko, M., and Mošanskı, L. (2020). First knowledge of spring-summer demographic structure and reproductive characteristics of Mus spicilegus from Slovakia. Biologia 75, 927–933.
Dieckmann, U., O’Hara, B., and Weisser, W. (1999). The evolutionary ecology of dispersal. Trends Ecol. Evol. 14, 88–90. doi: 10.1016/S0169-5347(98)01571-7
Duckworth, R. A. (2009). Maternal effects and range expansion: A key factor in a dynamic process? Philos. Trans. R. Soc. B Biol. Sci. 364, 1075–1086. doi: 10.1098/rstb.2008.0294
Duckworth, R. A., and Sockman, K. W. (2012). Proximate mechanisms of behavioural inflexibility: Implications for the evolution of personality traits. Funct. Ecol. 26, 559–566.
Dufty, A. M. Jr., and Belthoff, J. R. (2001). “Proximate mechanisms of natal dispersal: The role of body condition and hormones,” in Dispersal, eds J. Clobert, E. Danchin, A. A. Dhondt, and J. D. Nichols (New York, NY: Oxford University Press), 217–229.
Féron, C., and Gheusi, G. (2003). Social regulation of reproduction in the female mound-builder mouse (Mus spicilegus). Physiol. Behav. 78, 717–722. doi: 10.1016/s0031-9384(03)00044-1
Friard, O., and Gamba, M. (2016). BORIS: A free, versatile open-source event-logging software for video/audio coding and live observations. Methods Ecol. Evol. 7, 1325–1330. doi: 10.1111/2041-210X.12584
Garza, J. C., Dallas, J., Duryadi, D., Gerasimov, S., Croset, H., and Boursot, P. (1997). Social structure of the mound-building mouse Mus spicilegus revealed by genetic analysis with microsatellites. Mol. Ecol. 6, 1009–1017. doi: 10.1046/j.1365-294x.1997.00278.x
Gerlach, G. (1990). Dispersal mechanisms in a captive wild house mouse population (Mus domesticus Rutty). Biol. J. Linn. Soc. 41, 271–277.
Gouat, P., Féron, C., and Demouron, S. (2003b). Seasonal reproduction and delayed sexual maturity in mound-building mice Mus spicilegus. Reprod. Fertil. Dev. 15, 187–195. doi: 10.1071/rd02105
Gouat, P., Katone, K., and Poteaux, C. (2003a). Is the socio-spatial distribution of mound-building mice, Mus spicilegus, compatible with a monogamous mating system ? Mammalia 67, 15–24. doi: 10.1515/mamm.2003.67.1.15
Greenwood, P. J. (1980). Mating systems, philopatry and dispersal in birds and mammals. Anim. Behav. 28, 1140–1162.
Greenwood, P. J., and Harvey, P. H. (1982). The natal and breeding dispersal of birds. Ann. Rev. Ecol. Syst. 13, 1–21. doi: 10.1146/annurev.es.13.110182.000245
Groo, Z., Szenczi, P., Banszegi, O., and Altbaecker, V. (2013). Natal dispersal in two mice species with contrasting social systems. Behav. Ecol. Sociobiol. 67, 235–242.
Holekamp, K. E. (1984). Natal dispersal in Belding’s ground squirrels (Spermophilus beldingi). Behav. Ecol. Sociobiol. 16, 21–30.
Howard, W. E. (1960). Innate and environmental dispersal of individual vertebrates. Am. Midl. Nat. 63, 152–161.
Hurtado, M. J., Fénéron, R., and Gouat, P. (2013). Specialization in building tasks in the mound-building mouse. Mus spicilegus. Anim. Behav. 85, 1153–1160. doi: 10.1016/j.anbehav.2013.03.020
Kennaway, D. J. (2019). Melatonin research in mice: A review. Chronobiol. Int. 36, 1167–1183. doi: 10.1080/07420528.2019.1624373
Lafaille, M., and Féron, C. (2014). U-shaped relationship between ageing and risk-taking behaviour in a wild-type rodent. Anim. Behav. 97, 45–52.
Lafaille, M., Gouat, P., and Féron, C. (2015). Efficiency of delayed reproduction in Mus spicilegus. Reprod. Fertil. Dev. 27, 491–496.
Long, E. S., Diefenbach, D. R., Rosenberry, C. S., and Wallingford, B. D. (2008). Multiple proximate and ultimate causes of natal dispersal in white-tailed deer. Behav. Ecol. 19, 1235–1242.
Milishnikov, A. N., Rafiev, A. N., and Muntianu, A. I. (1998). [Genotypic variability in populations of the mound-builder mouse Mus spicilegus Pet., 1882, at various life cycle stages]. Genetika 34, 947–952.
O’Riain, M. J., Jarvis, J. U., and Faulkes, C. G. (1996). A dispersive morph in the naked mole-rat. Nature 380, 619–621.
Patris, B., and Baudoin, C. (2000). A comparative study of parental care between two rodent species: Implications for the mating system of the mound-building mouse Mus spicilegus. Behav. Process. 51, 35–43. doi: 10.1016/s0376-6357(00)00117-0
Poteaux, C., Busquet, N., Gouat, P., Katona, K., and Baudoin, C. (2008). Socio-genetic structure of mound-building mice, Mus spicilegus, in autumn and early spring. Biol. J. Linn. Soc. 93, 689–699.
Pusey, A., and Wolf, M. (1996). Inbreeding avoidance in animals. Trends Ecol. Evol. 11, 201–206. doi: 10.1016/0169-5347(96)10028-8
Simeonovska-Nikolova, D. (2000). Strategies in open field behaviour of Mus spicilegus and Mus musculus musculus. Belg. J. Zool. 130, 119–124.
Simeonovska-Nikolova, D. (2012). Neighbour relationships and spacing behaviour of mound-building mouse, Mus spicilegus (Mammalia: Rodentia) in summer. Acta Zool. Bulg. 64, 135–143.
Simeonovska-Nikolova, D., and Mehmed, Sh (2009). Behavior of mound building mouse, Mus Spicilegus during autumn-winter period in captivity. Biotechnol. Biotechnol. Equip. 23, 180–183. doi: 10.1080/13102818.2009.10818395
Szenczi, P., Bánszegi, O., Dúcs, A., Gedeon, C. I., Markó, G., Németh, I., et al. (2011). Morphology and function of communal mounds of overwintering mound-building mice (Mus spicilegus). J. Mammal. 92, 852–860. doi: 10.1644/10-mamm-a-258.1
Tong, W., and Hoekstra, H. (2012). Mus spicilegus. Curr. Biol. 22, R858–R859. doi: 10.1016/j.cub.2012.08.054
Keywords: dispersal, seasonality, adolescence, open field, novel object, wild mouse
Citation: Cryns NG, Lin WC, Motahari N, Krentzman OJ, Chen W, Prounis G and Wilbrecht L (2022) The maturation of exploratory behavior in adolescent Mus spicilegus on two photoperiods. Front. Behav. Neurosci. 16:988033. doi: 10.3389/fnbeh.2022.988033
Received: 06 July 2022; Accepted: 10 October 2022;
Published: 04 November 2022.
Edited by:
Laurence Coutellier, The Ohio State University, United StatesReviewed by:
Peter Szenczi, National Institute of Psychiatry Ramon de la Fuente Muñiz (INPRFM), MexicoNicole Ferrara, Rosalind Franklin University of Medicine and Science, United States
Copyright © 2022 Cryns, Lin, Motahari, Krentzman, Chen, Prounis and Wilbrecht. This is an open-access article distributed under the terms of the Creative Commons Attribution License (CC BY). The use, distribution or reproduction in other forums is permitted, provided the original author(s) and the copyright owner(s) are credited and that the original publication in this journal is cited, in accordance with accepted academic practice. No use, distribution or reproduction is permitted which does not comply with these terms.
*Correspondence: Linda Wilbrecht, d2lsYnJlY2h0QGJlcmtlbGV5LmVkdQ==
†Present address: Noah G. Cryns, Memory and Aging Center, University of California, San Francisco, San Francisco, CA, United States; Oliver J. Krentzman, Washington University School of Medicine, St. Louis, MO, United States