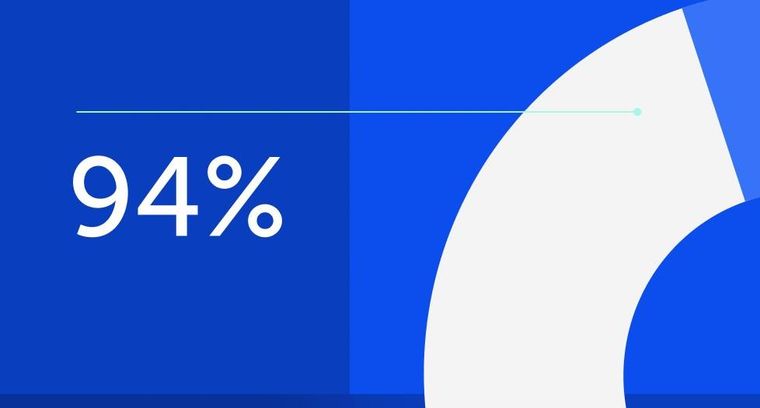
94% of researchers rate our articles as excellent or good
Learn more about the work of our research integrity team to safeguard the quality of each article we publish.
Find out more
ORIGINAL RESEARCH article
Front. Behav. Neurosci., 12 August 2022
Sec. Learning and Memory
Volume 16 - 2022 | https://doi.org/10.3389/fnbeh.2022.970304
This article is part of the Research TopicEndocrine, Developmental and Affective Control of Spatial MemoryView all 8 articles
Previous evidence indicates a link between early life stress (ELS) in humans and a predisposition to psychopathologies that are characterized in part by maladaptive habitual behaviors. Stress and anxiety influence the relative use of mammalian memory systems implicated in these disorders. Specifically, cognitive memory functions of the hippocampus are typically impaired by stress/anxiety, whereas habit memory functions of the dorsolateral striatum (DLS) are enhanced. A stress/anxiety bias toward habit memory has largely been demonstrated in adult rodents and humans, and the effects of ELS on the later use of DLS-dependent habit memory in adult rodents have not been extensively examined. The present study addressed this question by chronically elevating corticosterone (CORT) during adolescence, and investigated the effects of this treatment on DLS-dependent habit learning in adulthood. In experiment 1, adolescent rats received a single daily injection of either CORT (5 mg/kg) or vehicle (cVEH) over 5 days and then matured undisturbed before training as adults in a DLS-dependent water plus-maze task. Rats administered CORT injections during adolescence displayed a strong trend toward enhanced learning during adulthood relative to vehicle-treated rats. Adolescent CORT administration also increased anxiety-like behavior in adulthood in an elevated plus-maze. In experiment 2, adolescent CORT administration enhanced task acquisition in adulthood, and this effect was blocked by concurrent administration of the glucocorticoid antagonist mifepristone (30 mg/kg). Taken together, these findings suggest that chronic elevation of glucocorticoids during adolescence are sufficient to facilitate habit learning in adulthood, and indicate that glucocorticoid function may be a potential underlying mechanism by which ELS influences subsequent habitual behaviors.
Emotional arousal including stress and anxiety has a dramatic effect on multiple memory systems of the mammalian brain, and evidence has indicated both enhancing and impairing effects. One of the factors that determine whether emotional arousal enhances or impairs memory is the memory system being investigated (for reviews see Packard and Goodman, 2012, 2013; Schwabe, 2013; Packard et al., 2018). Specifically, previous research has indicated that stress and anxiety typically impair hippocampus-dependent cognitive memory, while enhancing dorsolateral striatum (DLS)-dependent habit memory. These effects of emotional arousal on multiple memory systems have been demonstrated in both rodents and humans. For example, in rats, several methods of inducing robust emotional arousal, including restraint stress and foot shock (Kim et al., 2001), anxiogenic drug administration (Packard and Wingard, 2004; Leong et al., 2012), and exposure to predator odor (Leong and Packard, 2014) or conditioned fear stimuli (Leong et al., 2015; Goode et al., 2016), produce a bias toward and enhancement of DLS habit memory.
The vast majority of research investigating the effects of emotional arousal within the context of multiple memory systems has examined stress exposure or anxiety that is induced during adulthood. Interestingly, extensive evidence indicates that early life stress (ELS) in rodents, usually administered within the first three post-natal weeks, is often associated with memory impairments in adulthood (for reviews, see McCormick et al., 2010; Bolton et al., 2017). For example, early maternal separation or limited bedding and nesting early in life results in reduced adult memory function across a range of tasks, including, for example, object recognition, object location, and spatial learning in the Morris water maze (Lehmann et al., 1999; Huot et al., 2002; Brunson et al., 2005; Aisa et al., 2007; Ivy et al., 2010; Molet et al., 2016). Chronic stress during adolescence also results in adult spatial memory impairments (Isgor et al., 2004; Avital and Richter-Levin, 2005). Importantly, these memory tasks require cognitive memory processes mediated in part by the hippocampus. Indeed, evidence suggests that the effects of ELS on adult cognitive memory may be attributed to deficits in hippocampal structure and function, such as reduced mossy fiber density, dendritic atrophy, and/or impaired long-term potentiation (LTP; Huot et al., 2002; Chen et al., 2004; Brunson et al., 2005). Although several studies have focused on the effects of ELS on hippocampal-dependent memory, there is some evidence that the enhanced use of DLS-dependent memory observed when emotional arousal is induced in adulthood may also be seen following ELS. In a recent study, limited bedding and nesting during post-natal days 2–9 resulted in greater habitual lever pressing in an instrumental learning task (Xu et al., 2022). Similarly, in human subjects, ELS is associated with greater adult use of habit memory strategies in solving instrumental learning tasks (Patterson et al., 2013, 2019; Gordon et al., 2020), and prenatal stress has been similarly associated with increased used of striatal learning strategies in a virtual maze (Schwabe et al., 2012a). Moreover, childhood adversity also correlates with adult scores on a self-report measure of day-to-day habitual tendencies (Creature of Habit Scale; Ersche et al., 2017).
There is accumulating evidence that altered memory system function may be relevant to understanding features of some human psychopathologies. Specifically, maladaptive DLS habit memory might be part of the neural mechanism underlying habit-like behavioral symptoms which manifest in some disorders, such as OCD, PTSD, and drug addiction and relapse (e.g., White, 1996; Everitt and Robbins, 2005; Schwabe et al., 2011; Berner and Marsh, 2014; Gillan and Robbins, 2014; Goodman et al., 2014). It is therefore tempting to speculate that the long-term effects of ELS on memory systems might be part of the mechanism through which ELS might predispose individuals toward developing psychopathologies later in life.
Therefore, the present study investigated how a chronic stress regimen [i.e., repeated corticosterone (CORT) administration] during adolescence influences DLS-dependent habit learning and anxiety in adulthood. In experiment 1, adolescent rats received a single daily injection of either CORT or vehicle over 5 days. Later, when rats reached adulthood, they received training in a habitual response learning version of the water plus-maze task. In this task, animals are trained in a water plus-maze to make the same consistently reinforced body turn response at the maze choice point. Extensive research indicates that acquisition of response learning in the plus-maze task depends on DLS function (for reviews see Packard, 2009; Goodman, 2021). We hypothesized that adolescent CORT administration would enhance acquisition of response learning in adulthood. In experiment 2, separate groups of rats received adolescent CORT administration in a manner similar to experiment 1, while some rats received concurrent administration of the glucocorticoid antagonist mifepristone to determine whether this treatment may block the effects of adolescent CORT on adult habit memory.
Adolescent male Long-Evans rats PND 28–35 on arrival were used and were pair-housed with access to food and water ad libitum throughout the study. Subjects were housed in a temperature-controlled vivarium with a 12:12 light-dark cycle (lights on 07:30). All procedures were carried out during the light-phase of the cycle. All animal procedures were carried out in accordance with the Texas A&M University Institutional Animal Care and Use Committee (IACUC).
The maze apparatus (Figure 1) was identical to that used in our previous research (e.g., Goodman and Packard, 2014; Goodman et al., 2015). Animals were trained in a black circular water maze (1.83 m in diameter, 0.58 m in height) filled to a water level of 20 cm. A clear Plexiglas plus-maze (43 cm in height, 25 cm in arm-width, and 60 cm in arm length) was inserted into the water maze. The water temperature was maintained at 25°C. A clear Plexiglas escape platform (11 cm × 14 cm) was located 1 cm below the water level in the appropriate maze goal arm.
Figure 1. The water maze apparatus and overview of the dorsolateral striatal-dependent response learning task. Starting points from the north and south arms are counterbalanced across trials and training days. Animals are trained to make a consistent body-turn response to reach the hidden escape platform (red square) for 6 trials/day over 7 consecutive training days.
The EPM consisted of three distinct zones: two opposing closed arms (41.9 cm × 13.6 cm), two opposing open arms (43.2 cm × 14.3 cm), and a center zone at the intersection, all elevated 78.7 cm above the ground. The maze was placed in the center of a novel room (i.e., different from the maze training room), and the room was evenly lit. Exploratory behavior was recorded via a webcam placed above the maze and subsequently analyzed via manual review.
Animals were handled and weighed daily for 5 days prior to initial drug administration. (CORT; Sigma-Aldrich) was dissolved in a sesame oil (Veh). The dose (5 mg/kg) was selected for its use in prior ELS models (e.g., Veenit et al., 2013) and its ability to mimic a physiological stress response in rats (Stein-Behrens et al., 1994; Venero et al., 1996). Mifepristone (MIFP; Cayman Chemical) was dissolved in a vehicle mixture (mVEH) of 90% physiological saline, 5% DMSO, and 3% Tween 80. In Experiment 1, rats received a subcutaneous (s.c.) injection of CORT (5 mg/kg; n = 12) or Veh (n = 11) once daily for 5 consecutive days. In Experiment 2, rats received a s.c. injection of (1) either MIFP (30 mg/kg) or VEH 15-min prior to subsequent administration of (2) either CORT (5 mg/kg) or cVEH. Thus, animals were divided into 4 groups based on treatment conditions: Veh + Veh (n = 10), MIFP + Veh (n = 8), MIFP + CORT (n = 10), and Veh + CORT (n = 12).
All behavioral procedures were conducted once subjects had matured into adulthood (PND 60 +), 3 weeks after the last injection(s). All behavioral procedures and training parameters were held constant across both experiments.
Rats were trained in a response learning task within the water plus-maze to reach a hidden escape platform for 6 trials/day over 7 days. Each trial began with placing the rat facing away from the maze center into either the N or S arm, pseudo-randomly counterbalanced across the 6 trials for each day of training. A maximum swim time of 60-s was allocated to reach the escape platform that was always hidden in the right arm relative to the start arm (i.e., N-start = W-goal, S-start = E-goal; Figure 1). Thus, rats were trained to make the same right body turn at the choice point to optimally reach the escape platform. Rats remained on the escape platform for 10-s before the experimenter removed them from the maze. After each trial, rats were placed in an opaque acrylic box for a 30-s inter-trial interval (ITI). Each trial was scored based on the subject’s initial full-body entry into either the correct or incorrect arms. Latency to reach the escape platform was also recorded.
A total of 48-h after the final water plus-maze training session, rats were placed in the EPM center facing an open arm to initiate the session and left to freely traverse the maze for 5-min. The total time spent within the center, open and closed arms as well as the number of entries to each zone were recorded. Time within the open and closed zones was accumulated only when all four paws were within the respective arms’ boundary.
The effects of adolescent administration of CORT on response learning in adulthood are illustrated in Figure 2. A significance level of p < 0.05 was employed for all tests. A two-way repeated-measures ANOVA (Group X Day) showed no Group X Day interaction on accuracy scores; F(6,126) = 1.473, p > 0.05. As expected, both groups showed improved accuracy in task performance over the seven training days, Day, F(3.273,68.73) = 17.57, p < 0.0001. There was also a strong trend for group differences; Group effect F(1,21) = 4.31, p = 0.0504, consistent with the hypothesis of enhanced response learning in adulthood in the CORT group. To provide a measure of the magnitude of the treatment effect, we next calculated effect sizes for group differences over days using Cohen’s d (Cohen, 1988), where d = 0.50 and d = 0.80 are considered medium and large effect sizes, respectively. This analysis revealed large effect sizes for the difference between CORT and vehicle animals that increased across Day 4 (d = 0.88) and Day 5 (d = 1.39) of training, consistent with an enhancing effect of CORT on task acquisition.
Figure 2. Effect of CORT administration during adolescence on response learning in adulthood (Mean Percent Correct ± SEM). Exogenous CORT administration significantly enhanced task acquisition.
Elevated plus-maze exploratory behavior between groups was assessed by the time spent in each zone over 5-min (300-s) and is illustrated in Figure 3. Adult rats that had received CORT during adolescence spent less time in the open zones relative to controls, t(13.98) = 2.34, p < 0.05. No significant differences were observed for time spent in the center, t(17.28) = 0.62, p > 0.05, or closed arms, t(20.88) = 1.32, p > 0.05. There were no significant differences in the number of entries into each zone between groups. The EPM findings indicate that chronic adolescent CORT administration can increase anxiety-like behavior in adulthood.
Figure 3. Effect of CORT administration during adolescence on anxiety-like behavior in EPM adulthood. Time spent in the Closed, Center, and Open arms of the apparatus. CORT administration reduced the time spent in the open arms. *p < 0.05.
The effects of adolescent administration of CORT on response learning in adulthood, along with the effects of concurrent administration of mifepristone are illustrated in Figure 4. In view of the early training variability inherent with the larger number of groups in experiment 2, the percent correct data was normalized by calculating the difference between percent correct on each training day and percent correct on day one of training. As such, we analyzed the relative rate of increase in habit memory expression in the maze as compared to the first day of training. A two-way repeated-measures ANOVA (Group X Day) revealed a significant group effect, F(3,36) = 2.464, p < 0.001. The Group X Day interaction was non-significant, F(18,216) = 1.428, p > 0.05, and a significant effect of Day revealed that all groups improved in task accuracy over the seven training days, F(3.811, 137.2) = 27.91, p < 0.001.
Figure 4. Effects of CORT and mifepristone (MIFP) administration during adolescence on response learning in adulthood (Mean Percent Correct ± SEM). CORT administration enhanced task acquisition and this effect was blocked by concurrent MIFP.
We next employed Tukey’s post-hoc tests and also calculated Cohen’s d for effect size in order to compare group differences in task acquisition. Animal’s receiving Veh + CORT were significantly enhanced in task acquisition relative to Veh + Veh controls, t(20) = 3.09, p < 0.05; d = 1.79. Animals receiving Veh + CORT were also enhanced in task acquisition relative to those administered MIFP + CORT, t(20) = 3.37, p < 0.01; d = 1.95, indicating that the enhancing effect of CORT on response learning was blocked by administration of MIFP. Finally, animals receiving MIFP alone (MIFP + Veh), were not significantly different than the vehicle group (Veh + Veh), t(20) = 0.84, n.s.; d = 0.40, indicating that MIFP at this dose does not by itself affect task acquisition.
Taken together, these findings indicate animals receiving CORT injections displayed facilitated response learning in adulthood, consistent with the effect of CORT observed in experiment 1. Concurrent injections of the CORT antagonist mifepristone during adolescence blocked the enhancing effect of CORT on response learning, and mifepristone did not affect task acquisition when administered alone.
The present study investigated the effects of adolescent ELS exposure on anxiety and DLS-dependent habit memory in adulthood. Chronic systemic CORT administration during adolescence was used as a model of ELS, based on previous research (e.g., Veenit et al., 2013) and its ability to mimic a physiological stress response in rats (Stein-Behrens et al., 1994; Venero et al., 1996). The present findings indicate that this regimen of CORT administration in adolescence increased anxiety-like behavior during adulthood in an elevated plus-maze. The findings also indicated that chronic CORT treatment during adolescence enhanced acquisition in a DLS-dependent response learning water plus-maze task. Moreover, the effect of adolescent CORT on acquisition was blocked by concurrent administration of glucocorticoid receptor antagonist mifepristone, suggesting the effects of CORT may depend, in part, on glucocorticoid receptor activation.
The findings are largely consistent with extensive prior evidence demonstrating that stress and anxiety may enhance the memory processes of the DLS (for reviews, see Packard and Goodman, 2012; Schwabe, 2013; Packard et al., 2018). Most of this prior research involved the use of stressors and/or anxiogenic drugs administered specifically during adulthood, as opposed to adolescence. Adult administration of several behavioral stressors, such as chronic restraint stress, exposure to predator odor, or anxiogenic drugs enhances the acquisition of habit memory and biases animals toward the use of habit strategies in tasks that may be solved adequately with an alternative strategy (Packard et al., 2018). Consistent with the present findings, CORT administration during adulthood also enhances memory in habitual response learning tasks in rodents (Quirarte et al., 2009; Goodman et al., 2015; Siller-Pérez et al., 2017). In addition, hydrocortisone administration in adult human subjects similarly enhances habit memory in both instrumental learning and virtual navigation tasks (Schwabe et al., 2010, 2012b; Guenzel et al., 2014).
Importantly, the present study adds to emerging evidence that these commonly observed effects of adult stress on DLS habit memory may be mimicked by ELS. Consistent with the present findings, limited bedding, and nesting during post-natal days 2–9 results in greater habitual lever pressing in an instrumental learning task (Xu et al., 2022). Moreover, social isolation during adolescence in mice also enhanced habitual responding to the instrumental task during adulthood (Shan et al., 2022). Our findings are also consistent with accumulating evidence from human research linking ELS to increased use of DLS-dependent habitual responding in adulthood (Patterson et al., 2013, 2019; Gordon et al., 2020). Similar observations have also been made for human prenatal stress, which is associated with enhanced habit memory in adulthood in a virtual-navigation task (Schwabe et al., 2012a). In addition, childhood adversity (as measured by the Childhood Trauma Questionnaire; Bernstein et al., 2003) has been positively correlated with self-report measures of habitual responding in adulthood (measured using the Creature of Habit Scale; Ersche et al., 2017).
While adult administration of glucocorticoids has been associated with enhanced DLS habit memory in prior research, our present findings are the first to our knowledge linking ELS CORT to enhanced habit memory in adulthood. Chronic stimulation of glucocorticoid receptors in adolescence may permanently alter the function of memory systems in the brain favoring habit memory, and this effect may be achieved via different mechanisms. Though administered systemically, CORT readily crosses the blood-brain barrier and may bind to high-affinity mineralocorticoid and low-affinity glucocorticoid receptors across the rodent brain (Reul and de Kloet, 1985; de Kloet et al., 1993). Importantly, the DLS may be an important site of action for the effects of adolescent CORT, considering that this brain region richly expresses glucocorticoid receptors; however, this brain region is relatively devoid of mineralocorticoid receptors (Ahima and Harlan, 1990, 1991; Morimoto et al., 1996). As noted previously, the DLS is critically involved in the acquisition, consolidation, and retrieval of habit memory, including the acquisition of habit memory in the specific response learning plus-maze task employed in the present experiments (for review, see Goodman, 2021). It is possible that, in the present study, chronic CORT influenced habit learning directly via stimulation of glucocorticoid receptors in the DLS. Indeed, adult administration of CORT directly into the DLS is sufficient to enhance habit memory (Quirarte et al., 2009; Siller-Pérez et al., 2017), and the effects of glucocorticoid administration on DLS memory processes depend on interactions with other neurotransmitter systems, such as endocannabinoid (Siller-Pérez et al., 2019), cholinergic (Sánchez-Resendis et al., 2012), and adrenergic systems (Goodman et al., 2015). The extent to which the effects of adolescent chronic exposure to glucocorticoids observed in the present study may be explained by similar mechanisms may be investigated in future research.
Considering the long-term effects of ELS observed in this study, it is reasonable that our chronic CORT treatment during adolescence resulted in long-term functional and/or structural changes in one or more memory systems of the brain to produce the observed effects on habit memory. This explanation would be consistent with recent evidence showing that behavioral stressors during childhood/adolescence may enhance habit memory during adulthood via altering structure and function of the dorsal striatum. Limited bedding and nesting during childhood in mice boosts habitual responding later in life, and this enhancement was associated with the increased post-synaptic density of neurons in the DLS (Xu et al., 2022). Moreover, ELS may be associated with suppression of the striatonigral direct pathway of the dorsomedial striatum (DMS; Shan et al., 2022), a brain region implicated in goal-directed action-outcome learning as opposed to DLS-mediated habitual stimulus-response learning (Yin and Knowlton, 2006; Dezfouli and Balleine, 2012). Importantly, chemogenetic activation of direct pathway DMS neurons erases the enhancing effect of ELS on habit memory and shifts animals away from DLS-habitual responding and toward goal-directed responding (Shan et al., 2022). Future research is required to determine whether the ELS treatment employed in the present experiments (i.e., chronic CORT treatment) enhances habit memory via similar structural and functional changes in the dorsal striatum. Considering that CORT was administered systemically, it should be noted that additional brain regions outside the dorsal striatum may also be involved.
Chronic administration of CORT (similar to behavioral stressors) may have a global impact on the brain, affecting changes in multiple brain areas, including those supporting learning and memory. In addition to structural and functional changes in the dorsal striatum, it is possible that ELS may enhance habit memory indirectly via changes to the hippocampus. While the hippocampus does not mediate habit memory, evidence suggests that the hippocampus may interact with the DLS, and in some learning situations, these memory systems compete for control of behavioral output (for reviews, see Poldrack and Packard, 2003; Packard and Goodman, 2013; Goodman, 2021). For instance, extensive evidence suggests that hippocampal impairments (e.g., reversible lesion) can lead to enhancement of DLS-dependent habit memory (Packard et al., 1989), and this effect is mimicked by stress and anxiety-induced impairments to the hippocampus (e.g., Kim et al., 2001; Wingard and Packard, 2008). A competitive interaction between the hippocampus and DLS may be especially relevant to understanding the effects of ELS on habit memory, considering evidence that ELS also impairs the structure and function of the hippocampus. For example, ELS is associated with adult reduction of mossy fiber density, dendritic atrophy, and impaired LTP in the hippocampus (Huot et al., 2002; Chen et al., 2004; Brunson et al., 2005) as well as impairments in hippocampus-dependent declarative/spatial memory (Lehmann et al., 1999; Huot et al., 2002; Isgor et al., 2004; Avital and Richter-Levin, 2005; Brunson et al., 2005; Aisa et al., 2007; Ivy et al., 2010; Molet et al., 2016). Importantly, when stress is administered during adulthood, stress-induced impairments in hippocampal LTP and declarative memory are also associated with enhanced habit memory (e.g., Kim et al., 2001). Thus, ELS-induced hippocampal impairment may be part of the mechanism through which ELS enhances habit memory.
Notably, a recent study suggested that ELS-induced enhancement of DLS and impairment of the hippocampus may both be associated with habit memory enhancement in adulthood (Xu et al., 2022). In this study, ELS increased post-synaptic density and the number of thin and mushroom-shaped spines in the DLS, whereas opposite effects were found in the CA1 region of the hippocampal neurons (Xu et al., 2022). These structural changes were correlated with greater reliance on habitual responding in an instrumental learning task and impaired spatial memory in a novel object location task (Xu et al., 2022). Thus, consistent with a competitive interaction between memory systems (Poldrack and Packard, 2003), it is possible that ELS-induced structural changes to the hippocampus and DLS may similarly account for, in part, the enhancing effects of ELS on habit memory observed in the present experiments. Further research will be required to investigate these and other potential mechanisms.
Research on ELS is highly relevant to understanding how childhood or adolescent stressful life events, trauma, and abuse can result in the development of psychiatric disorders later in life (Carr et al., 2013; Syed and Nemeroff, 2017). In light of the present findings, it is possible that ELS may lead to heightened DLS habit memory in adult human psychiatric patients. Multiple investigators have suggested that stress and anxiety may be, in part, associated with the development and expression of habit-like behavioral symptoms observed in multiple disorders, such as obsessive-compulsive disorder, PTSD, and drug addiction, among other disorders (White, 1996; Everitt and Robbins, 2005; Schwabe et al., 2011; Goodman et al., 2012, 2014; Berner and Marsh, 2014; Gillan and Robbins, 2014; Goodman and Packard, 2016). ELS has been associated with the development of similar disorders in adulthood (e.g., addiction and PTSD, and other anxiety disorders; Enoch, 2011; Nugent et al., 2011). Therefore, ELS-induced changes to memory systems that lead to enhanced habit memory in adulthood may partially account for the habit-like symptoms observed in these adult psychiatric disorders (e.g., avoidance symptoms in PTSD, drug-seeking and relapse, compulsive behaviors, repetitive and stereotyped behaviors, etc.). It is important to emphasize that ELS has a dramatic impact on multiple brain areas and circuits that may be related to adult psychopathology (Gutman and Nemeroff, 2002; Heim and Nemeroff, 2002; Bremner and Vaccarino, 2015; Agorastos et al., 2019; Kirsch et al., 2020), and enhanced DLS habit memory represents only one of the many neurobehavioral outcomes of ELS. Nevertheless, considering how ELS-induced enhancements of habit memory could critically explain some of the hallmark features of adult human psychopathology, this effect and its underlying mechanisms warrant further investigation in future research.
The original contributions presented in this study are included in the article/supplementary material, further inquiries can be directed to the corresponding author.
The animal study was reviewed and approved by the IACUC Texas A&M University.
All authors listed have made a substantial, direct, and intellectual contribution to the work, and approved it for publication.
This work was partially supported by a grant from the National Institutes of Health (P20GM103653).
The authors declare that the research was conducted in the absence of any commercial or financial relationships that could be construed as a potential conflict of interest.
All claims expressed in this article are solely those of the authors and do not necessarily represent those of their affiliated organizations, or those of the publisher, the editors and the reviewers. Any product that may be evaluated in this article, or claim that may be made by its manufacturer, is not guaranteed or endorsed by the publisher.
Agorastos, A., Pervanidou, P., Chrousos, G. P., and Baker, D. G. (2019). Developmental trajectories of early life stress and trauma: a narrative review on neurobiological aspects beyond stress system dysregulation. Front. Psychiatry 10:118. doi: 10.3389/fpsyt.2019.00118
Ahima, R. S., and Harlan, R. E. (1990). Charting of type II glucocorticoid receptor-like immunoreactivity in the rat central nervous system. Neuroscience 39, 579–604. doi: 10.1016/0306-4522(90)90244-x
Ahima, R. S., and Harlan, R. E. (1991). Differential corticosteroid regulation of type II glucocorticoid receptor-like immunoreactivity in the rat central nervous system: topography and implications. Endocrinology 129, 226–236. doi: 10.1210/endo-129-1-226
Aisa, B., Tordera, R., Lasheras, B., Del Río, J., and Ramírez, M. J. (2007). Cognitive impairment associated to HPA axis hyperactivity after maternal separation in rats. Psychoneuroendocrinology 32, 256–266. doi: 10.1016/j.psyneuen.2006.12.013
Avital, A., and Richter-Levin, G. (2005). Exposure to juvenile stress exacerbates the behavioural consequences of exposure to stress in the adult rat. Int. J. Neuropsychopharmacol. 8, 163–173. doi: 10.1017/S1461145704004808
Berner, L. A., and Marsh, R. (2014). Frontostriatal circuits and the development of bulimia nervosa. Front. Behav. Neurosci. 8:395. doi: 10.3389/fnbeh.2014.00395
Bernstein, D. P., Stein, J. A., Newcomb, M. D., Walker, E., Pogge, D., Ahluvalia, T., et al. (2003). Development and validation of a brief screening version of the childhood trauma questionnaire. Child Abuse Negl. 27, 169–190. doi: 10.1016/S0145-2134(02)00541-540
Bolton, J. L., Molet, J., Ivy, A., and Baram, T. Z. (2017). New insights into early-life stress and behavioral outcomes. Curr. Opin. Behav. Sci. 14, 133–139. doi: 10.1016/j.cobeha.2016.12.012
Bremner, J. D., and Vaccarino, V. (2015). “Neurobiology of early life stress in women,” in Psychosocial Stress and Cardiovascular Disease in Women, eds K. Orth-Gomér, N. Schneiderman, V. Vaccarino, and H. C. Deter (Cham: Springer).
Brunson, K. L., Kramár, E., Lin, B., Chen, Y., Colgin, L. L., Yanagihara, T. K., et al. (2005). Mechanisms of late-onset cognitive decline after early-life stress. J. Neurosci. 25, 9328–9338.
Carr, C. P., Martins, C. M. S., Stingel, A. M., Lemgruber, V. B., and Juruena, M. F. (2013). The role of early life stress in adult psychiatric disorders: a systematic review according to childhood trauma subtypes. J. Nerv. Ment. Dis. 201, 1007–1020. doi: 10.1097/NMD.0000000000000049
Chen, Y., Bender, R. A., Brunson, K. L., Pomper, J. K., Grigoriadis, D. E., Wurst, W., et al. (2004). Modulation of dendritic differentiation by corticotropin-releasing factor in the developing hippocampus. Proc. Natl. Acad. Sci. U S A. 101, 15782–15787. doi: 10.1073/pnas.0403975101
Cohen, J. (1988). Statistical Power Analysis for the Behavioral Sciences, 2nd Edn. New Jersey, NJ: Lawrence Erlbaum Associates.
de Kloet, E. R., Oitzl, M. S., and Joëls, M. (1993). Functional implications of brain corticosteroid receptor diversity. Cell Mol. Neurobiol. 13, 433–455.
Dezfouli, A., and Balleine, B. W. (2012). Habits, action sequences and reinforcement learning. Eur. J. Neurosci. 35, 1036–1051. doi: 10.1111/j.1460-9568.2012.08050.x
Enoch, M.-A. (2011). The role of early life stress as a predictor for alcohol and drug dependence. Psychopharmacology 214, 17–31. doi: 10.1007/s00213-010-1916-1916
Ersche, K. D., Lim, T.-V., Ward, L. H. E., Robbins, T. W., and Stochl, J. (2017). Creature of Habit: a self-report measure of habitual routines and automatic tendencies in everyday life. Pers. Individ. Dif. 116, 73–85. doi: 10.1016/j.paid.2017.04.024
Everitt, B. J., and Robbins, T. W. (2005). Neural systems of reinforcement for drug addiction: from actions to habits to compulsion. Nat. Neurosci. 8, 1481–1489. doi: 10.1038/nn1579
Gillan, C. M., and Robbins, T. W. (2014). Goal-directed learning and obsessive-compulsive disorder. Philos. Trans. R. Soc. Lond. B Biol. Sci. 369:20130475. doi: 10.1098/rstb.2013.0475
Goode, T. D., Leong, K.-C., Goodman, J., Maren, S., and Packard, M. G. (2016). Enhancement of striatum-dependent memory by conditioned fear is mediated by beta-adrenergic receptors in the basolateral amygdala. Neurobiol. Stress 3, 74–82. doi: 10.1016/j.ynstr.2016.02.004
Goodman, J. (2021). Place vs. response learning: history, controversy, and neurobiology. Front. Behav. Neurosci. 14:598570. doi: 10.3389/fnbeh.2020.598570
Goodman, J., Leong, K.-C., and Packard, M. G. (2012). Emotional modulation of multiple memory systems: implications for the neurobiology of post-traumatic stress disorder. Rev. Neurosci. 23, 627–643. doi: 10.1515/revneuro-2012-2049
Goodman, J., Leong, K. C., and Packard, M. G. (2015). Glucocorticoid enhancement of dorsolateral striatum-dependent habit memory requires concurrent noradrenergic activity. Neuroscience 311, 1–8. doi: 10.1016/j.neuroscience.2015.10.014
Goodman, J., Marsh, R., Peterson, B. S., and Packard, M. G. (2014). Annual research review: the neurobehavioral development of multiple memory systems–implications for childhood and adolescent psychiatric disorders. J. Child Psychol. Psychiatry 55, 582–610. doi: 10.1111/jcpp.12169
Goodman, J., and Packard, M. G. (2014). Peripheral and intra-dorsolateral striatum injections of the cannabinoid receptor agonist WIN 55,212-2 impair consolidation of stimulus-response memory. Neuroscience 274, 128–137. doi: 10.1016/j.neuroscience.2014.05.007
Goodman, J., and Packard, M. G. (2016). Memory systems and the addicted brain. Front. Psychiatry 7:24. doi: 10.3389/fpsyt.2016.00024
Gordon, A. L., Patterson, T. K., and Knowlton, B. J. (2020). Early-life stress is associated with a preponderance of habitual responding in a novel instrumental avoidance learning paradigm. Neurobiol. Learn. Mem. 175:107316. doi: 10.1016/j.nlm.2020.107316
Guenzel, F. M., Wolf, O. T., and Schwabe, L. (2014). Sex differences in stress effects on response and spatial memory formation. Neurobiol. Learn. Mem. 109, 46–55. doi: 10.1016/j.nlm.2013.11.020
Gutman, D. A., and Nemeroff, C. B. (2002). Neurobiology of early life stress: rodent studies. Semin. Clin. Neuropsychiatry 7, 89–95. doi: 10.1053/scnp.2002.31781
Heim, C., and Nemeroff, C. B. (2002). Neurobiology of early life stress: clinical studies. Semin. Clin. Neuropsychiatry 7, 147–159. doi: 10.1053/scnp.2002.33127
Huot, R. L., Plotsky, P. M., Lenox, R. H., and McNamara, R. K. (2002). Neonatal maternal separation reduces hippocampal mossy fiber density in adult Long Evans rats. Brain Res. 950, 52–63. doi: 10.1016/s0006-8993(02)02985-2982
Isgor, C., Kabbaj, M., Akil, H., and Watson, S. J. (2004). Delayed effects of chronic variable stress during peripubertal-juvenile period on hippocampal morphology and on cognitive and stress axis functions in rats. Hippocampus 14, 636–648. doi: 10.1002/hipo.10207
Ivy, A. S., Rex, C. S., Chen, Y., Dubé, C., Maras, P. M., Grigoriadis, D. E., et al. (2010). Hippocampal dysfunction and cognitive impairments provoked by chronic early-life stress involve excessive activation of CRH receptors. J. Neurosci. 30, 13005–13015. doi: 10.1523/JNEUROSCI.1784-10.2010
Kim, J. J., Lee, H. J., Han, J. S., and Packard, M. G. (2001). Amygdala is critical for stress-induced modulation of hippocampal long-term potentiation and learning. J. Neurosci. 21, 5222–5228.
Kirsch, D., Nemeroff, C. M., and Lippard, E. T. C. (2020). Early life stress and substance use disorders: underlying neurobiology and pathways to adverse outcomes. Adv. Res. Sci. 1, 29–47. doi: 10.1007/s42844-020-00005-7
Lehmann, J., Pryce, C. R., Bettschen, D., and Feldon, J. (1999). The maternal separation paradigm and adult emotionality and cognition in male and female Wistar rats. Pharmacol. Biochem. Behav. 64, 705–715.
Leong, K.-C., Goodman, J., and Packard, M. G. (2012). Buspirone blocks the enhancing effect of the anxiogenic drug RS 79948-197 on consolidation of habit memory. Behav. Brain Res. 234, 299–302. doi: 10.1016/j.bbr.2012.07.009
Leong, K.-C., Goodman, J., and Packard, M. G. (2015). Post-training re-exposure to fear conditioned stimuli enhances memory consolidation and biases rats toward the use of dorsolateral striatum-dependent response learning. Behav. Brain Res. 291, 195–200. doi: 10.1016/j.bbr.2015.05.022
Leong, K.-C., and Packard, M. G. (2014). Exposure to predator odor influences the relative use of multiple memory systems: role of basolateral amygdala. Neurobiol. Learn. Mem. 109, 56–61. doi: 10.1016/j.nlm.2013.11.015
McCormick, C. M., Mathews, I. Z., Thomas, C., and Waters, P. (2010). Investigations of HPA function and the enduring consequences of stressors in adolescence in animal models. Brain Cogn. 72, 73–85. doi: 10.1016/j.bandc.2009.06.003
Molet, J., Maras, P. M., Kinney-Lang, E., Harris, N. G., Rashid, F., Ivy, A. S., et al. (2016). MRI uncovers disrupted hippocampal microstructure that underlies memory impairments after early-life adversity. Hippocampus 26, 1618–1632. doi: 10.1002/hipo.22661
Morimoto, M., Morita, N., Ozawa, H., Yokoyama, K., and Kawata, M. (1996). Distribution of glucocorticoid receptor immunoreactivity and mRNA in the rat brain: an immunohistochemical and in situ hybridization study. Neurosci. Res. 26, 235–269. doi: 10.1016/S0168-0102(96)01105-1104
Nugent, N. R., Tyrka, A. R., Carpenter, L. L., and Price, L. H. (2011). Gene-environment interactions: early life stress and risk for depressive and anxiety disorders. Psychopharmacology 214, 175–196.
Packard, M. G. (2009). Anxiety, cognition, and habit: a multiple memory systems perspective. Brain Res. 1293, 121–128.
Packard, M. G., and Goodman, J. (2012). Emotional arousal and multiple memory systems in the mammalian brain. Front. Behav. Neurosci. 6:14. doi: 10.3389/fnbeh.2012.00014
Packard, M. G., and Goodman, J. (2013). Factors that influence the relative use of multiple memory systems. Hippocampus 23, 1044–1052. doi: 10.1002/hipo.22178
Packard, M. G., Goodman, J., and Ressler, R. L. (2018). Emotional modulation of habit memory: neural mechanisms and implications for psychopathology. Curr. Opin. Behav. Sci. 20, 25–32. doi: 10.1016/j.cobeha.2017.09.004
Packard, M. G., Hirsh, R., and White, N. M. (1989). Differential effects of fornix and caudate nucleus lesions on two radial maze tasks: evidence for multiple memory systems. J. Neurosci. 9, 1465–1472. doi: 10.1523/JNEUROSCI.09-05-01465.1989
Packard, M. G., and Wingard, J. C. (2004). Amygdala and “emotional” modulation of the relative use of multiple memory systems. Neurobiol. Learn. Mem. 82, 243–252. doi: 10.1016/j.nlm.2004.06.008
Patterson, T. K., Craske, M. G., and Knowlton, B. J. (2013). The effect of early-life stress on memory systems supporting instrumental behavior. Hippocampus 23, 1025–1034. doi: 10.1002/hipo.22174
Patterson, T. K., Craske, M. G., and Knowlton, B. J. (2019). Enhanced avoidance habits in relation to history of early-life stress. Front. Psychol. 10:1876. doi: 10.3389/fpsyg.2019.01876
Poldrack, R. A., and Packard, M. G. (2003). Competition among multiple memory systems: converging evidence from animal and human brain studies. Neuropsychologia 41, 245–251. doi: 10.1016/s0028-3932(02)00157-4
Quirarte, G. L., de la Teja, I. S. L., Casillas, M., Serafín, N., Prado-Alcalá, R. A., and Roozendaal, B. (2009). Corticosterone infused into the dorsal striatum selectively enhances memory consolidation of cued water-maze training. Learn. Mem. 16, 586–589. doi: 10.1101/lm.1493609
Reul, J. M., and de Kloet, E. R. (1985). Two receptor systems for corticosterone in rat brain: microdistribution and differential occupation. Endocrinology 117, 2505–2511. doi: 10.1210/endo-117-6-2505
Sánchez-Resendis, O., Medina, A. C., Serafín, N., Prado-Alcalá, R. A., Roozendaal, B., and Quirarte, G. L. (2012). Glucocorticoid-cholinergic interactions in the dorsal striatum in memory consolidation of inhibitory avoidance training. Front. Behav. Neurosci. 6:33. doi: 10.3389/fnbeh.2012.00033
Schwabe, L. (2013). Stress and the engagement of multiple memory systems: integration of animal and human studies. Hippocampus 23, 1035–1043. doi: 10.1002/hipo.22175
Schwabe, L., Bohbot, V. D., and Wolf, O. T. (2012a). Prenatal stress changes learning strategies in adulthood. Hippocampus 22, 2136–2143. doi: 10.1002/hipo.22034
Schwabe, L., Tegenthoff, M., Höffken, O., and Wolf, O. T. (2010). Concurrent glucocorticoid and noradrenergic activity shifts instrumental behavior from goal-directed to habitual control. J. Neurosci. 30, 8190–8196. doi: 10.1523/JNEUROSCI.0734-10.2010
Schwabe, L., Tegenthoff, M., Höffken, O., and Wolf, O. T. (2012b). Simultaneous glucocorticoid and noradrenergic activity disrupts the neural basis of goal-directed action in the human brain. J. Neurosci. 32, 10146–10155. doi: 10.1523/JNEUROSCI.1304-12.2012
Schwabe, L., Dickinson, A., and Wolf, O. T. (2011). Stress, habits, and drug addiction: a psychoneuroendocrinological perspective. Exp. Clin. Psychopharmacol. 19, 53–63. doi: 10.1037/a0022212
Shan, Q., Yu, X., and Tian, Y. (2022). Adolescent social isolation shifts the balance of decision-making strategy from goal-directed action to habitual response in adulthood via suppressing the excitatory neurotransmission onto the direct pathway of the dorsomedial striatum. Cereb. Cortex. Online ahead of print. doi: 10.1093/cercor/bhac158
Siller-Pérez, C., Fuentes-Ibañez, A., Sotelo-Barrera, E. L., Serafín, N., Prado-Alcalá, R. A., Campolongo, P., et al. (2019). Glucocorticoid interactions with the dorsal striatal endocannabinoid system in regulating inhibitory avoidance memory. Psychoneuroendocrinology 99, 97–103. doi: 10.1016/j.psyneuen.2018.08.021
Siller-Pérez, C., Serafín, N., Prado-Alcalá, R. A., Roozendaal, B., and Quirarte, G. L. (2017). Glucocorticoid administration into the dorsolateral but not dorsomedial striatum accelerates the shift from a spatial toward procedural memory. Neurobiol. Learn. Mem. 141, 124–133. doi: 10.1016/j.nlm.2017.03.020
Stein-Behrens, B., Mattson, M. P., Chang, I., Yeh, M., and Sapolsky, R. (1994). Stress exacerbates neuron loss and cytoskeletal pathology in the hippocampus. J. Neurosci. 14, 5373–5380.
Syed, S. A., and Nemeroff, C. B. (2017). Early life stress, mood, and anxiety disorders. Chronic Stress (Thousand Oaks) 1:2470547017694461. doi: 10.1177/2470547017694461
Veenit, V., Cordero, M. I., Tzanoulinou, S., and Sandi, C. (2013). Increased corticosterone in peripubertal rats leads to long-lasting alterations in social exploration and aggression. Front. Behav. Neurosci. 7:26. doi: 10.3389/fnbeh.2013.00026
Venero, C., Guaza, C., and Sandi, C. (1996). Regional and temporal modulation of brain glycoprotein synthesis by corticosterone. Neuroreport 7, 2819–2822.
White, N. M. (1996). Addictive drugs as reinforcers: multiple partial actions on memory systems. Addiction 91:921-49; discussion 951.
Wingard, J. C., and Packard, M. G. (2008). The amygdala and emotional modulation of competition between cognitive and habit memory. Behav. Brain Res. 193, 126–131.
Xu, B., Zhang, X., He, Y., Liu, C., Li, L., Liu, Q., et al. (2022). The impacts of early-life adversity on striatal and hippocampal memory functions. Neuroscience 490, 11–24. doi: 10.1016/j.neuroscience.2022.02.029
Keywords: early life stress (ELS), memory, habits, learning, striatum, corticosterone
Citation: Gadberry TM, Goodman J and Packard MG (2022) Chronic corticosterone administration in adolescence enhances dorsolateral striatum-dependent learning in adulthood. Front. Behav. Neurosci. 16:970304. doi: 10.3389/fnbeh.2022.970304
Received: 15 June 2022; Accepted: 28 July 2022;
Published: 12 August 2022.
Edited by:
Robert James McDonald, University of Lethbridge, CanadaReviewed by:
Roberto A. Prado-Alcalá, National Autonomous University of Mexico, MexicoCopyright © 2022 Gadberry, Goodman and Packard. This is an open-access article distributed under the terms of the Creative Commons Attribution License (CC BY). The use, distribution or reproduction in other forums is permitted, provided the original author(s) and the copyright owner(s) are credited and that the original publication in this journal is cited, in accordance with accepted academic practice. No use, distribution or reproduction is permitted which does not comply with these terms.
*Correspondence: Mark G. Packard, bWFya3BhY2thcmRAdGFtdS5lZHU=
Disclaimer: All claims expressed in this article are solely those of the authors and do not necessarily represent those of their affiliated organizations, or those of the publisher, the editors and the reviewers. Any product that may be evaluated in this article or claim that may be made by its manufacturer is not guaranteed or endorsed by the publisher.
Research integrity at Frontiers
Learn more about the work of our research integrity team to safeguard the quality of each article we publish.