- Department of Psychology and Brain Health Research Centre, University of Otago, Dunedin, New Zealand
Introduction
Neuropsychiatric disorders are poorly defined. The DSM-5 has many hundred categorical disorders (American Psychiatric Association, 2013) as does the only partially matching ICD-10 (World Health Organization, 1992). But the categories are based on symptoms (and often a required duration), not neurobiological causes, which are likely dimensional and reflected in traits (Kotov et al., 2021; Michelini et al., 2021; DeYoung et al., 2022). There can also be problems with labeling. For example, are fear and anxiety synonyms, metonyms, or antonyms (McNaughton, 2018)? With this confusion, it is no surprise that, even with broad-spectrum pharmaceuticals and well-developed psychological therapies, 30–60% of those receiving first-line treatment have continuing impairment; and the criteria for multi-treatment resistance are not well defined (Bokma et al., 2019).
These patient-level problems make it hard to construct good, matching, animal models (McNaughton and Zangrossi, 2008). Indeed, I will argue that it should be the more successful animal models that provide the basis for proper clinical diagnoses. Many models have been constructed for face validity; but have poor prediction of therapeutic drug action [e.g., the elevated plus maze does not reliably detect serotonergic drugs (Handley et al., 1993; Griebel, 1995) and a wide range of tests—e.g. holeboard, Geller-Seifter, social interaction—each has its own profile of detection (Cryan and Sweeney, 2011)] and, being based on behavior in healthy animals, no clear relationship to clinical disorder—given this discrepancy it is amazing that any animal models are predictive at all. The capacity of such animal models to predict effects on clinical disorder, is one among many reasons for taking a trait perspective—as does the anxiolytic effect in healthy people of the GABAA agonist, ethanol. “Psychiatry has proven to be among the least penetrable clinical disciplines for productively marrying knowledge of human pathology with animal behavior to develop satisfactory in vivo animal models for evaluating novel treatment approaches” (Cryan and Sweeney, 2011).
I argue that the answer lies in using strong neuropsychological theory as a basis for model construction, translation, and understanding of psychiatry disorders. As we noted elsewhere:
“Theory influences what we mean by the word “anxiety”, what we require of any animal model, and what specific theoretical constructs are embedded in any specific animal model of anxiety. We argue that, in the ideal case, the animal models we use should be embedded in a large-scale theory that integrates all of the theoretical levels of each animal model. We argue that face validity of a model should be ignored and that true predictive validity reduces ultimately to construct validity. So all models should aim to have construct validity based on strong theory. Theoretical analysis shows that anxiety should be distinguished from fear; that different anxiety disorders should be distinguished from each other; and that the components of any single apparent type of anxiety can have distinct neural control. Theory can show how a model is unsatisfactory, but it can also show that it is not the model but rather our translation from the clinical situation that is faulty. To model the many flavors of clinical disorder and variations in drug effectiveness, we must use theory to link multiple animal models, neural analysis and pharmacological analysis. The goal is to provide us with truly predictive tests that can be used for drug discovery as well as drug development. Most importantly, theory is required if we are to correctly match a particular measure from a particular model with the clinical entity we desire to model” (McNaughton and Zangrossi, 2008).
Generating Strong Neuropsychological Theory
Over 50 years ago, Gray (1970), compared the effects of the anxiolytic barbiturate sodium amylobarbitone, septal lesions, and hippocampal lesions; and proposed on the basis of similarity across only a few behavioral experiments that the septo-hippocampal system is a key site for anxiolytic (as opposed to other) drug actions. He also proposed that impairment of the theta rhythm that is seen in the hippocampus and controlled by the septum was the key to these common effects. Note that this key hypothesis was based on drugs not psychology, with the specific nature of the similarly-affected behaviors something that needed considerable work to determine.
To develop a full theory from this hypothesis, the neural basis of anxiolytic action, and the psychological nature of the drugs' effects, then received 30 years of progressive development (Gray, 1982; Gray and McNaughton, 2000; McNaughton and Gray, 2000) that retained the underlying hypothetical bedrock while elaborating on the superstructure. Importantly, despite the appearance of completely new classes of drugs (benzodiazepines1, buspirone—a serotonin1A agonist, specific serotonin reuptake inhibitors, pregabalin—a calcium channel agent, and ketamine, the anxiolytic mechanism of which is unknown), the positive and negative predictions of the theory remained intact. For example, both benzodiazepines and buspirone impair control of hippocampal theta rhythm (Zhu and McNaughton, 1991, 1995a), impair hippocampus-sensitive learning in the Morris water maze (McNaughton and Morris, 1987, 1992), and impair hippocampus-sensitive behavioral inhibition (Gray and McNaughton, 1983; Zhu and McNaughton, 1995b). We have proved that the benzodiazepines affect behavioral inhibition via an action on one of the locations that controls theta rhythm (Woodnorth and McNaughton, 2002) and that theta rhythmicity, in and of itself, is important for spatial learning (McNaughton et al., 2006; Ruan et al., 2011).
This may seem a long road to have traveled but, as we will see, it leads to more than one desirable destination. A good theory, properly applied, solves many problems—and, as Newton showed, need not be complicated in its core elements even though their working out can be another matter (as shown by the 3-body problem).
From Theory to Construct Validity
It is important to note that the anxiety-septohippocampal-theta theory implies construct validity for some models and not others. Operant tests of “behavioral inhibition” have long had anxiolytic predictive validity; and fell out of favor because of their cost. But the role of goal conflict in the theory (Gray and McNaughton, 2000) gives the bulk of them stronger construct validity; and also explains the lack of effect of anxiolytics on action inhibition (McNaughton et al., 2013; Shadli et al., 2015), which is functionally distinct but lexically confusable. Similarly, the theory provides a good theoretical basis for contextual conditioning (Luyten et al., 2011), and the elevated T-maze (McNaughton and Zangrossi, 2008) and but less so the elevated plus maze (Pellow et al., 1987) unless ethological measures are used (Cole and Rodgers, 1994, 1995; Rodgers and Cole, 1994).
Here it is worth noting that the only current model of clinical anxiolytic action that has no false positives nor false negatives (McNaughton et al., 2007) in 40 years of testing (McNaughton and Sedgwick, 1978) is reticular stimulation elicited hippocampal theta rhythm. This is, of course, one of the key foundational elements of the theory. But it has also withstood the challenge of the progressive appearance of new classes of anxiolytic and of recent predictive tests (Engin et al., 2009; Siok et al., 2009). Importantly for its construct validity, specific manipulations of the theta control system alter behavior in a manner consistent with the psychological aspects of the theory when they alter theta in a fashion consistent with theta changes being the basis for their behavioral actions.
From Model to Translational Test
It might seem difficult to take a rat model that uses depth stimulation and recording in rats and use it to develop an equivalent human test. This is where theory can provide a bridge.
In the theory (Gray and McNaughton, 2000) hippocampal theta is necessary but not sufficient for goal conflict processing and will be present even when the hippocampus has no functional output. The prefrontal cortex can show its own forms of theta (Mitchell et al., 2008) but becomes synchronous with hippocampal theta during, e.g., risk assessment behavior (Young and McNaughton, 2009) and novelty detection (Park et al., 2021); and theta (and other rhythms) changes across the hippocampus-amygdala-prefrontal network in response to stress (Merino et al., 2021). This suggests that we should be able to use prefrontal scalp EEG to record theta rhythmicity that is functionally equivalent to the hippocampal theta in the rat model.
The psychological core of the theory is goal conflict (Gray and McNaughton, 2000). We therefore tested for frontal theta rhythmicity linked to goal conflict in a simple gain/loss-based approach/avoidance task in student participants (Neo and McNaughton, 2011; Neo et al., 2020) and found a conflict-related power increase at the right-frontal site, F8. Right frontal cortex, and the right inferior frontal gyrus in particular, are involved in stopping (a key output of the goal conflict system) in the stop signal task (SST). So, we used the SST and found that it generated a goal-conflict specific “theta” rhythmicity (GCSR) at F8 (Neo et al., 2011).
The SST has the advantage, for clinical work, that it does not use monetary gain and loss. We, therefore, proceeded to validate GCSR within the theory by showing that it is sensitive to the three main types of specifically anxiolytic (i.e. not antipanic) drugs (McNaughton et al., 2013), similarly validated an improved version of the task (Shadli et al., 2015, 2020), demonstrated its relation to handedness (Shadli et al., 2021a), and demonstrated that its value is high in a subgroup of those with high trait anxiety scores and DSM “anxiety disorder” diagnoses (Shadli et al., 2021b).
Discussion
It is tempting to ask, at this point, “What sort of DSM anxiety does GCSR represent?”. However, as a biomarker for a causal agent (equivalent to SARS-Cov-2), it will be linked to a wide range of symptomatic expressions of its disorder (equivalent to COVID-19) and show both positive and negative discrepancies from any DSM symptom-based class (equivalent to “flu-like respiratory infection”). The key original problem is that current diagnoses do not map to the underlying biological disorder that generates the symptoms.
Figures 1A,B show that, despite very similar scores on the trait scale of the Spielberger State Trait Anxiety Inventory (Spielberger et al., 1983), the diagnostic groups differ in a non-categorical way in terms of GCSR. This fits both previous doubts about the current categories and the recent move to a trait perspective on psychopathology (Kotov et al., 2021; Michelini et al., 2021; DeYoung et al., 2022). It also fits the nosological mapping of the neuropsychological theory (Gray and McNaughton, 2000; McNaughton and Corr, 2004; McNaughton, 2020) summarized in Figure 1C. The first important feature of this nosology is that theta is a modulator across the range of conflict control structures, with its strongest effects in the middle of the hierarchy (see shading in figure). Thus, the disorder for which GCSR is a biomarker will represent only one trait component of the possible conflict-related disorders. The second important feature is that within the separate repulsion system, there are distinct areas controlling obsession (and so linked to obsessive compulsive disorder) and panic (and so linked to panic disorder); each with their own pharmacological sensitivities and so capacity for distinct contributions to disorder; while, conversely, serotonergic modulation can impact on all systems; as can noradrenergic modulation, which is also involved in anxiety control but to a more limited extent (Gray, 1982; Gray and McNaughton, 2000; McNaughton and Gray, 2000). These are all immediate targets for the search for relevant models that will deliver new biomarkers. A third particularly important feature is that this neuropsychology implies only a loose connection between causes of disorder and symptoms. Pathological panic can generate anxiety in an otherwise normal anxiety system, and vice versa, with the capacity for a vicious cycle (McNaughton and Corr, 2016); while a dysfunctional serotonin system could generate a combination of pathological panic and pathological anxiety.
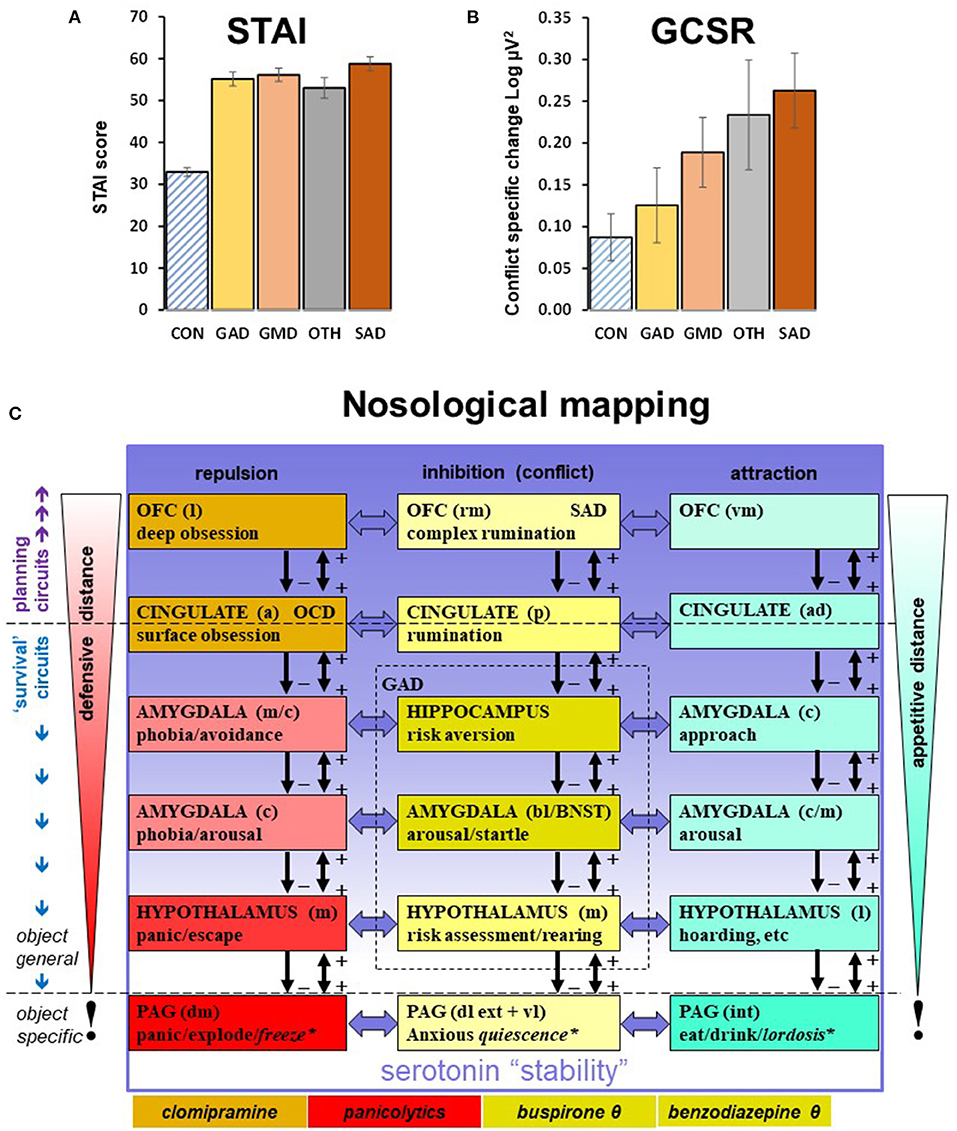
Figure 1. (A) The relationship of Spielberger State Trait Anxiety Inventory (Spielberger et al., 1983) trait values (STAI) to DSM (American Psychiatric Association, 2013) diagnosis in the same groups as B. Bars are ±SEM. (B) The relationship of goal conflict specific rhythmicity (GCSR, 4–7 Hz maximum value) to DSM diagnosis. The data are from (Shadli et al., 2021b) with permission of the author. Note that the trait anxiety scores are high (clinical cases are usually >45), and very similar across the groups. GCSR appears elevated across all diagnoses but varies across the groups (there is no obvious factor controlling this variation). CON, community control; GAD, generalized anxiety disorder; GMD, GAD + major depression; SAD, social anxiety disorder; OTH, mixed other anxiety-related diagnoses with small N per diagnosis. (C) Nosological mapping to hierarchical systems. Goal attraction, goal repulsion, and goal inhibition (activated by conflict between goals) are each controlled by systems in which modules are organized hierarchically in relation to motivational distance (from contacting to distant) and neural location (caudal to rostral). Conservation of modulatory control during phylogeny (McNaughton, 2020) means that hormonal compounds, e.g., benzodiazepine receptor ligands, and neuromodulators, e.g., serotonin, can target all the modules of a specific system (as with benzodiazepines and goal inhibition; yellow highlight) or all the modules of several systems (as with serotonin). Note that in the case of serotonin (most obviously via specific serotonin reuptake inhibitors), its effects (indicated by the gradation of the purple shading) appear to be to shift control from lower to higher levels of the systems (Carver et al., 2008) rather than to increase or decrease activity across an entire system. There is also the capacity for more localized dysfunction and pharmacological specificity, as with obsession (orange highlight) and panic (red highlight). Figure and legend based on (McNaughton et al., 2016; Silva and McNaughton, 2019; McNaughton, 2020) with permission of the author. a, anterior; b, basal; c, central; d, dorsal; ext, external; int, internal; l, lateral; m, medial; p, posterior v, ventral; BNST, bed nucleus of the stria terminalis; SAD, Social Anxiety Disorder; OCD, obsessive compulsive disorder; OFC, orbitofrontal cortex; PAG, periaqueductal gray; θ, these compounds affect the system as a whole by reducing theta rhythmic input.
On this view, problems with previous animal models of psychiatric disorder may have resulted from both inappropriate assumptions behind perception of face validity and inappropriate relation of symptoms to diagnostic categories. Strong theory that accounts for the fundamental similarities of species, while allowing for their species-specific superficial expression should provide a way forward.
Author Contributions
The author confirms being the sole contributor of this work and has approved it for publication.
Conflict of Interest
The author declares that the research was conducted in the absence of any commercial or financial relationships that could be construed as a potential conflict of interest.
Publisher's Note
All claims expressed in this article are solely those of the authors and do not necessarily represent those of their affiliated organizations, or those of the publisher, the editors and the reviewers. Any product that may be evaluated in this article, or claim that may be made by its manufacturer, is not guaranteed or endorsed by the publisher.
Footnotes
1. ^The benzodiazepines act on the same GABAA receptor as do the barbiturates, meprobamate, and other classical anxiolytics—but they do so by changing the way the GABA site works (Haefely, 1990), not through a site that acts directly to open the channel. This was a breakthrough for toxicity and related side effects.
References
American Psychiatric Association, (2013). Diagnostic and statistical manual of mental disorders, fifth edition, American Psychiatric Association, Arlington, VA. doi: 10.1176/appi.books.9780890425596
Bokma, W. A., Wetzer, G. A., Gehrels, J. B., Penninx, B. W., Batelaan, N. M., and van Balkom, A. L. (2019). Aligning the many definitions of treatment resistance in anxiety disorders: A systematic review. Depress. Anxiety 36, 801–812. doi: 10.1002/da.22895
Carver, C. S., Johnson, S. L., and Joormann, J. (2008). Serotonergic function, two-mode models of self-regulation, and vulnerability to depression: What depression has in common with impulsive aggression. Psychol. Bull. 134, 912–943. doi: 10.1037/a0013740
Cole, J. C., and Rodgers, R. J. (1994). Ethological evaluation of the effects of acute and chronic buspirone treatment in the murine elevated plus-maze test: comparison with haloperidol. Psychopharmacology. 114, 288–296. doi: 10.1007/BF02244851
Cole, J. C., and Rodgers, R. J. (1995). Ethological comparison of the effects of diazepam and acute/chronic imipramine on the behaviour of mice in the elevated plus- maze. Pharmacol. Biochem. Behav. 52, 473–478. doi: 10.1016/0091-3057(95)00163-Q
Cryan, J. F., and Sweeney, F. F. (2011). The age of anxiety: role of animal models of anxiolytic action in drug discovery. Br. J. Pharmacol. 164, 1129–1161. doi: 10.1111/j.1476-5381.2011.01362.x
DeYoung, C. G., Chmielewski, M., Clark, L. A., Condon, D. M., Kotov, R., Krueger, R. F., et al. (2022). The distinction between symptoms and traits in the Hierarchical Taxonomy of Psychopathology (HiTOP). J. Pers. 90, 20–33. doi: 10.1111/jopy.12593
Engin, E., Treit, D., and Dickson, C. T. (2009). Anxiolytic- and antidepressant-like properties of ketamine in behavioral and neurophysiological animal models. Neuroscience. 161, 359–369. doi: 10.1016/j.neuroscience.2009.03.038
Gray, J. A. (1970). Sodium amobarbital, the hippocampal theta rhythm and the partial reinforcement extinction effect. Psychol. Rev. 77, 465–480. doi: 10.1037/h0029804
Gray, J. A. (1982). “The Neuropsychology of Anxiety: An enquiry in to the functions of the septo-hippocampal system”, Oxford University Press, Oxford. doi: 10.1017/S0140525X00013066
Gray, J. A., and McNaughton, N. (1983). Comparison between the behavioural effect of septal and hippocampal lesions: a review. Neurosci. Biobehav. Rev. 7, 119–188. doi: 10.1016/0149-7634(83)90014-3
Gray, J. A., and McNaughton, N. (2000). “The Neuropsychology of Anxiety: An enquiry into the functions of the septo-hippocampal system (2 ed.)”, Oxford University Press, Oxford.
Griebel, G. (1995). 5-hydroxytryptamine-interacting drugs in animal models of anxiety disorders: more than 30 years of research. Pharmacol. Ther. 65, 319–395. doi: 10.1016/0163-7258(95)98597-J
Haefely, W. (1990). “The GABAa-benzodiazepine receptor: Biology and Pharmacology”, in G.D. Burrows, M. Roth, and R. Noyes, (Eds.), Handbook of Anxiety: The Neurobiology of Anxiety, Elsevier Science Publishers B.V., pp. 165–187.
Handley, S. L., McBlane, J. W., Critchley, M. A. E., and Njung'e, K. (1993). Multiple serotonin mechanisms in animal models of anxiety: environmental, emotional and cognitive factors. Behav. Brain Res. 58, 203–210. doi: 10.1016/0166-4328(93)90104-X
Kotov, R., Krueger, R. F., Watson, D., Cicero, D. C., Conway, C. C., DeYoung, C. G., et al. (2021). The Hierarchical Taxonomy of Psychopathology (HiTOP): a quantitative nosology based on consensus of evidence. Annu. Rev. Clin. Psychol. 17, 83–108. doi: 10.1146/annurev-clinpsy-081219-093304
Luyten, L., Vansteenwegen, D., van Kuyck, K., Gabriëls, L., and Nuttin, B. (2011). Contextual conditioning in rats as an animal model for generalized anxiety disorder. Cogn. Affect. Behav. Neurosci. 11, 228–244. doi: 10.3758/s13415-011-0021-6
McNaughton, N. (2018). What do you mean “anxiety”? Developing the first anxiety syndrome biomarker. J. Roy. Soc. New Zealand. 48, 177–190. doi: 10.1080/03036758.2017.1358184
McNaughton, N. (2020). Personality neuroscience and psychopathology: should we start with biology and look for neural-level factors? Personal. Neurosci. 3, e4. doi: 10.1017/pen.2020.5
McNaughton, N., and Corr, P. J. (2004). A two-dimensional neuropsychology of defense: fear/anxiety and defensive distance. Neurosci. Biobehav. Rev. 28, 285–305. doi: 10.1016/j.neubiorev.2004.03.005
McNaughton, N., and Corr, P. J. (2016). Mechanisms of comorbidity, continuity, and discontinuity in anxiety-related disorders. Dev. Psychopathol. 28, 1053–1069. doi: 10.1017/S0954579416000699
McNaughton, N., DeYoung, C. G., and Corr, P. J. (2016). “Approach/avoidance”, in: J.R. Absher, and J. Cloutier, (Eds.), Neuroimaging personality, social cognition and character, Elsevier, San Diego, pp. 25–49. doi: 10.1016/B978-0-12-800935-2.00002-6
McNaughton, N., and Gray, J. A. (2000). Anxiolytic action on the behavioural inhibition system implies multiple types of arousal contribute to anxiety. J. Affect. Disord. 61, 161–176. doi: 10.1016/S0165-0327(00)00344-X
McNaughton, N., Kocsis, B., and Hajós, M. (2007). Elicited hippocampal theta rhythm: a screen for anxiolytic and procognitive drugs through changes in hippocampal function? Behav. Pharmacol. 18, 329–346. doi: 10.1097/FBP.0b013e3282ee82e3
McNaughton, N., and Morris, R. G. M. (1987). Chlordiazepoxide, an anxiolytic benzodiazepine, impairs place navigation in rats. Behav. Brain Res. 24, 39–46. doi: 10.1016/0166-4328(87)90034-9
McNaughton, N., and Morris, R. G. M. (1992). Buspirone produces a dose-related impairment in spatial navigation. Pharmacol. Biochem. Behav. 43, 167–171. doi: 10.1016/0091-3057(92)90653-W
McNaughton, N., Ruan, M., and Woodnorth, M. A. (2006). Restoring theta-like rhythmicity in rats restores initial learning in the Morris water maze. Hippocampus. 16, 1102–1110. doi: 10.1002/hipo.20235
McNaughton, N., and Sedgwick, E. M. (1978). Reticular stimulation and hippocampal theta rhythm in rats: effects of drugs. Neuroscience. 3, 629–632. doi: 10.1016/0306-4522(78)90004-0
McNaughton, N., Swart, C., Neo, P. S. H., Bates, V., and Glue, P. (2013). Anti-anxiety drugs reduce conflict-specific “theta”—a possible human anxiety-specific biomarker. J. Affect. Disord. 148, 104–111. doi: 10.1016/j.jad.2012.11.057
McNaughton, N., and Zangrossi, H. (2008). Theoretical approaches to the modeling of anxiety in animals”, in R.J. Blanchard, D.C. Blanchard, G. Griebel, and D.J. Nutt, (Eds.), Handbook of Anxiety and Fear, Elsevier B.V., Oxford, UK, pp. 11–27. doi: 10.1016/S1569-7339(07)00002-1
Merino, E., Raya-Salom, D., Teruel-Mart,í, V., Adell, A., Cervera-Ferri, A., and Martínez-Ricós, J. (2021). Effects of acute stress on the oscillatory activity of the hippocampus–amygdala–prefrontal cortex network. Neuroscience 476, 72–89. doi: 10.1016/j.neuroscience.2021.09.009
Michelini, G., Palumbo, I. M., DeYoung, CG, Latzman, RD, and Kotov, R. (2021). Linking RDoC and HiTOP: A new interface for advancing psychiatric nosology and neuroscience. Clin. Psychol. Rev. 86, 102025. doi: 10.1016/j.cpr.2021.102025
Mitchell, D. J., McNaughton, N., Flanagan, D., and Kirk, I. J. (2008). Frontal-midline theta from the perspective of hippocampal “theta”. Prog. Neurobiol. 86, 156–185. doi: 10.1016/j.pneurobio.2008.09.005
Neo, P. S. H., and McNaughton, N. (2011). Frontal theta power linked to neuroticism and avoidance. Cognit. Affect. Behav. Neurosci. 11, 396–403. doi: 10.3758/s13415-011-0038-x
Neo, P. S. H., Thurlow, J., and McNaughton, N. (2011). Stopping, goal-conflict, trait anxiety and frontal rhythmic power in the stop-signal task. Cognit. Affect. Behav. Neurosci. 11, 485–493. doi: 10.3758/s13415-011-0046-x
Neo, P. S. H., Tinker, J., and McNaughton, N (2020). Goal-conflict EEG theta and biased economic decisions: a role for a second negative motivation system. Front. Neurosci. 14, e342. doi: 10.3389/fnins.2020.00342
Park, A. J., Harris, A. Z., Martyniuk, K. M., Chang, C. Y., Abbas, A. I., Lowes, D. C., et al. (2021). Reset of hippocampal–prefrontal circuitry facilitates learning. Nature. 591, 615–619. doi: 10.1038/s41586-021-03272-1
Pellow, S., Johnston, A. L., and File, S. E. (1987). Selective agonists and antagonists for 5- hydroxytryptamine receptor subtypes and interactions with yohimbine and FG 7142 using the elevated plus-maze test in the rat. J. Pharm. Pharmacol. 39, 917–928. doi: 10.1111/j.2042-7158.1987.tb03129.x
Rodgers, R. J., and Cole, J. C. (1994). “The elevated plus-maze: Pharmacology, methodology and ethology”, in S.J. Cooper, and C.A. Hendrie, (Eds.), Ethology and Psychopharmacology, John Wiley and Sons Ltd, pp. 9–44.
Ruan, M., Young, C. K., and McNaughton, N. (2011). Minimal driving of hippocampal theta by the supramammillary nucleus during water maze learning. Hippocampus. 21, 1074–1081. doi: 10.1002/hipo.20821
Shadli, S. M., Ando, L. C., McIntosh, J., Lodhia, V., Russell, B. R., Kirk, I. J., et al. (2021b). Right frontal anxiolytic-sensitive EEG ‘theta' rhythm in the stop-signal task is a theory-based anxiety disorder biomarker. Sci. Rep. 11, 19746. doi: 10.1038/s41598-021-99374-x
Shadli, S. M., Glue, P., McIntosh, J., and McNaughton, N. (2015). An improved human anxiety process biomarker: characterization of frequency band, personality and pharmacology. Transl. Psychiatry. 5, e699. doi: 10.1038/tp.2015.188
Shadli, S. M., High, O., Byers, B., Gibbs, P., Steller, R., and Glue, P. (2020). Human anxiety-specific “theta” occurs with selective stopping and localizes to right inferior frontal gyrus. Behav. Neurosci. 134, 547–555. doi: 10.1037/bne0000316
Shadli, S. M., Tewari, V., Holden, J., and McNaughton, N. (2021a). Laterality of an EEG anxiety disorder biomarker largely follows handedness. Cortex. 140, 210–221. doi: 10.1016/j.cortex.2021.03.025
Silva, C., and McNaughton, N. (2019). Are periaqueductal gray and dorsal raphe the foundation of appetitive and aversive control? A comprehensive review. Prog. Neurobiol. 177, 33–72. doi: 10.1016/j.pneurobio.2019.02.001
Siok, C. J., Taylor, C. P., and Hajós, M. (2009). Anxiolytic profile of pregabalin on elicited hippocampal theta oscillation. Neuropharmacology. 56, 379–385. doi: 10.1016/j.neuropharm.2008.09.013
Spielberger, C. D., Gorusch, R. L., Lushene, R., Vagg, P. R., and Jacobs, G. A. (1983). “Manual for the STATE-TRAIT ANXIETY INVENTORY (Form Y)”, Consulting Psychologists Press, Palo Alto. CA94306.
Woodnorth, M. A., and McNaughton, N. (2002). Similar effects of medial supramammillary or systemic injection of chlordiazepoxide on both theta frequency and fixed-interval responding. Cognit. Affect. Behav. Neurosci. 2, 76–83. doi: 10.3758/CABN.2.1.76
World Health Organization, (1992). The ICD-10 Classification of Mental and Behavioural Disorders, World Health Organization, Geneva.
Young, C. K., and McNaughton, N. (2009). Coupling of theta oscillations between anterior and posterior midline cortex and with the hippocampus in freely behaving rats. Cereb. Cortex. 19, 24–40. doi: 10.1093/cercor/bhn055
Zhu, X. O., and McNaughton, N. (1991). Effects of long-term administration of anxiolytics on reticular-elicited hippocampal rhythmical slow activity. Neuropharmacology. 30, 1095–1099. doi: 10.1016/0028-3908(91)90138-2
Zhu, X. O., and McNaughton, N. (1995a). Minimal changes with long-term administration of anxiolytics on septal driving of hippocampal rhythmical slow activity. Psychopharmacology. 118, 93–100. doi: 10.1007/BF02245254
Keywords: animal model, anxiety, disorder, clinical translation, neuropsychology, theory, biomarker
Citation: McNaughton N (2022) Neuropsychological Theory as a Basis for Clinical Translation of Animal Models of Neuropsychiatric Disorder. Front. Behav. Neurosci. 16:877633. doi: 10.3389/fnbeh.2022.877633
Received: 17 February 2022; Accepted: 05 April 2022;
Published: 10 May 2022.
Edited by:
Boriss Sagalajev, University Hospital of Cologne, GermanyReviewed by:
Andrea Bari, Aelis Farma, FranceKarolina Noworyta, Polish Academy of Sciences (PAN), Poland
Copyright © 2022 McNaughton. This is an open-access article distributed under the terms of the Creative Commons Attribution License (CC BY). The use, distribution or reproduction in other forums is permitted, provided the original author(s) and the copyright owner(s) are credited and that the original publication in this journal is cited, in accordance with accepted academic practice. No use, distribution or reproduction is permitted which does not comply with these terms.
*Correspondence: Neil McNaughton, bmVpbC5tY25hdWdodG9uQG90YWdvLmFjLm56