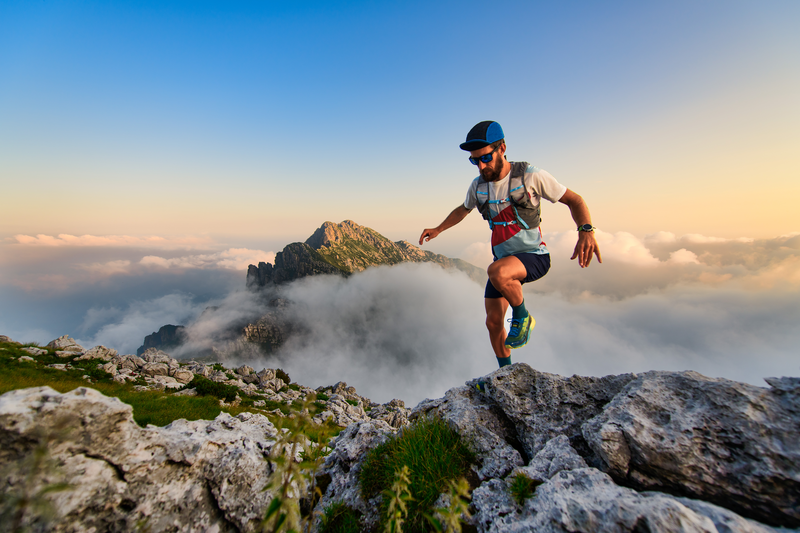
94% of researchers rate our articles as excellent or good
Learn more about the work of our research integrity team to safeguard the quality of each article we publish.
Find out more
MINI REVIEW article
Front. Behav. Neurosci. , 06 January 2023
Sec. Individual and Social Behaviors
Volume 16 - 2022 | https://doi.org/10.3389/fnbeh.2022.1103192
This article is part of the Research Topic Expert Opinion in Environmental and Genetic Factors Impacting Functional Brain Lateralization in Development and Evolution View all 9 articles
Evidence of sensory experience influencing the development of lateralized brain and behavior is reviewed. The epigenetic role of light exposure during two specific stages of embryonic development of precocial avian species is a particular focus of the research discussed. Two specific periods of light sensitivity (in early versus late incubation), each depending on different subcellular and cellular processes, affect lateralized behavior after hatching. Auditory and olfactory stimulation during embryonic development is also discussed with consideration of interactions with light-generated visual lateralization.
Most research on laterality of brain and behavior in non-human species has been conducted on adults, but research on lateralization in domestic chicks is an exception. Since avian embryos develop in eggs, it is possible to manipulate their pre-hatching, as well as post-hatching, sensory experience much more easily than can be achieved in mammals. Consequently, the chick (Gallus gallus) has become a model for elucidating genetic and epigenetic influences on the development of lateralized brain and behavior. A similar ease of studying epigenetic influences on the development of lateralization applies to other avian species although, so far, the pigeon and the quail have been the only other avian species studied in this regard (quail, Casey and Sleigh, 2014; Harshaw et al., 2021; pigeon, Güntürkün and Ocklenburg, 2017; Letzner et al., 2017). In fact, precocial avian species, such as the chick and quail, have an exceptional attribute aiding study of development; both before and after hatching their development passes through a number of distinct phases, each quite separate and of short duration. These phases can be intercepted and manipulated separately or in sequence to reveal outcomes on behavior after hatching, making it possible to investigate the influence of sensory experience on brain function.
This paper discusses what is known about the effect of sensory stimulation on development of lateralized brain and behavior in precocial, avian species (domestic chick and quail).
As a body of research has shown, newly hatched chicks process information differently in each hemisphere and each hemisphere controls different patterns of behavior (Rogers and Anson, 1979; Vallortigara and Rogers, 2005). These lateral differences include specialization of the right hemisphere for attention to novel and unexpected events (Rogers, 2000), expression of sexual behavior and aggression (Rogers et al., 1985), attention to geometric spatial information (Tommasi and Vallortigara, 2001; Tommasi et al., 2003), including attention to spatial relationships between objects (Morandi-Raikova and Mayer, 2021), social behavior (Deng and Rogers, 2002; Daisley et al., 2009; Rosa Salva et al., 2012) and, as an aspect of the latter, attention to the movement of living beings (Rugani et al., 2015). By contrast, the left hemisphere is specialized to categorize stimuli (e.g., grains versus small pebbles) and follow learned rules of behavior (Rogers, 1982; Rogers et al., 2013). Sensory stimulation during embryonic development affects the development of several of these lateralized functions. In other words, sensory input interplays dynamically with a genetic program to achieve different lateral outcomes.
Light exposure of the developing chick embryo has a critical role in generating lateralization of brain structure and a range of different brain functions, as will be discussed. However, before focusing on the latter, it is worth noting that light stimulation is not required for development of all aspects of the lateralized brain: e.g., asymmetry of neural activity in the pre-optic region of the brain is present in chicks not exposed to light (Lorenzi et al., 2019) and lateralization of choice to approach a familiar versus and unfamiliar chick is present in chicks lacking exposure to light (Deng and Rogers, 2002).
Stimulation by light during incubation is by far the most studied example of sensory influence on lateralized behavior after hatching. There are two periods when light exposure affects development of visual lateralization. The first falls within the first three days of incubation (Chiandetti et al., 2013) and the second occurs during the last three days before hatching (Rogers, 1982, 1990). During natural incubation, hens leave the nest for longer periods exactly at these two times of light-sensitivity (Archer and Mench, 2014) thereby exposing the embryos to light, which indicates that the laboratory studies are relevant to development in natural conditions.
Exposure to light during the first three days of incubation (early period) has some effects similar to those generated by light exposure during the last three days (late period); viz., both early and late light-exposure cause the chick to peck at an array of grains with a leftwards bias (Chiandetti et al., 2013) and both times of exposure suppress a preference to pay more attention to distracting stimuli on the chick’s left side (Chiandetti et al., 2017). However, another measure of post-hatching behavior separates the effects of early versus late exposure to light (Chiandetti and Vallortigara, 2019): chicks tested binocularly with scattered grain and pebbles perseverate by repeatedly pecking at the same pebble provided they have been exposed to light during late incubation but not if they have been exposed to light during early incubation, or if they have been incubated in the dark. Note that the repeated pecking at pebbles after late exposure to light does not mean that these chicks cannot discriminate grain from pebbles (discussed in section “2.2 Light exposure at the end of embryonic development”).
It is, of course, not surprising that the early and late periods of sensitivity to light have different effects on post-hatching behavior since each must rely on different cellular and subcellular processes. The early period happens well before the embryo’s visual system is fully functional: not until day 18 of incubation can an electroretinogram be recorded (Rogers, 1995). It is, however, on day 2 of incubation that the embryo adopts an asymmetrical posture with its left side against the yolk. This early stage of development involves left-right differences in the expression of genes, including Lefty and Nodal (Levin et al., 1995; Nakamura and Hamada, 2012). Chiandetti et al. (2013) have hypothesized that the early effect of light may be mediated via undifferentiated cells of photosensitive regions, but what might those cells be? Pigmentation of the eyes begins on day 3 of incubation and on day 3 amacrine cells begin to form in the retina (summarized in Rogers, 1995). Using a marker for photoreceptive cone cells, Visinin, Doh et al. (2010) were able to detect retinal photoreceptor cells on day 4 of incubation. Possibly these cells could develop even earlier since day 4 was the earliest day of incubation examined. Although it is not until day 10 that Visinin-labeled cells in the retina peak in number (Doh et al., 2010) and by day 6 of incubation axons from the ganglion cells of the retina start arriving at the optic tecta of the brain [summarized in Rogers (1995)], on day 3 there is a detectable increase in spontaneous motor activity of the embryo in response to light exposure (Wu et al., 2001). In other words, the early embryo can detect and respond to light stimulation, possibly via either the first formed retinal photoceptors or via photoreceptors in the developing pineal and parapineal organs (Kuo et al., 2003), both of which are known to establish asymmetry in zebrafish (Guglielmotti and Cristino, 2006; Andrew, 2009; Concha et al., 2009) and to do so by asymmetry of Nodal signaling (Concha et al., 2000; Liang et al., 2000).
The second period of light-sensitivity (last 3 days of incubation: Rogers, 1982) occurs at a time when the chick embryo adopts an asymmetric posture inside the egg so that the left eye is occluded by the chick’s body and the right eye is next to the shell and membranes (Kovach, 1968; Rogers, 1990). This genetically determined postural turning allows the right eye only to be stimulated by light passing through the shell and membranes. From 96 to 98 percent of chick embryos are oriented in this way (Olsen and Byerly, 1935; Butcher and Nilipour, 2002). At this late stage of embryonic development, the retinas are functional and light-stimulated nerve impulses are sent to the brain for processing (summarized in Rogers, 1995). Since the optic nerves decussate completely, light stimulation of the right eye relays visual inputs to the left side of the midbrain.
This asymmetrical stimulation of the visual pathways leads to asymmetry of visual behavior after the chick has hatched. For example, male chicks hatched from eggs exposed to light during the last three days of incubation are able to learn to discriminate food grains from a background of small pebbles provided that they are using their right eye (left hemisphere), but not if they are using their left eye (right hemisphere) (Rogers, 1982). By contrast, chicks hatched from eggs kept in darkness during the last 3 days show no such laterality (Rogers and Anson, 1979; Rogers, 1982, 1997; Mench and Andrew, 1986), even if they have been exposed to light up until day 17 of incubation (Zappia and Rogers, 1983). Lateralization of other types of visual behavior also depends on light-exposure during this period; viz., attack and copulation (Rogers, 1982, 1990), discrimination of left from right position (Chiandetti and Vallortigara, 2009), attention to spatial information (Chiandetti et al., 2005), attention to biological motion (Rugani et al., 2015) and competition within a social group (Rogers and Workman, 1989).
Although light exposure in ovo enables use of the right eye and left hemisphere in learning to find grain scattered amongst pebbles, exposure to light also alters functions controlled by the right hemisphere (e.g., attack and copulation, Rogers, 1982; Bullock and Rogers, 1986), possibly via interhemispheric communication which releases the right hemisphere from inhibition by the left hemisphere and, hence, elevates attack and copulation. A role of interhemispheric communication has also been shown in a task requiring chicks to locate food using patterned or spatial cues (Chiandetti et al., 2005). When tested monocularly on this task, chicks exposed to light before hatching are able to use both hemispheres to process visual information, likely because, in this case, the exposure to light enhances interhemispheric communication. This contrasts to chicks incubated in the dark, which can use only the hemisphere opposite to their open eye. Indeed, study of lateralized development in the pigeon has shown that interhemispheric communication is enhanced following exposure of embryos to light throughout incubation (Manns and Römling, 2012; Letzner et al., 2014).
Along with the behavioral asymmetries dependent on light stimulation, there are left-right asymmetries in the number of neural projections from the thalamus to the visual Wulst/hyperpallium region of the forebrain (Rogers and Deng, 1999), asymmetries in some, but not all, aspects of neural responses in the visual Wulst (Costalunga et al., 2022) and left-right differences in the number of synapses per neuron in the Wulst (Stewart et al., 1992).
Although most studies have used the procedure of exposing the embryo to light from day 19 of incubation until hatching on day 21, as little as 4 to 6 h of light exposure on day 19 of incubation is sufficient to generate the lateralization of visual behavior (Zappia and Rogers, 1983; Rogers, 1990). During this short sensitive period, genetic processes that generate postural asymmetry of the head and body provide a foundation for the epigenetic effect of light stimulation on the development of lateralized brain function (for more, see Versace et al., 2022).
It is now worth investigating whether the two different periods of sensitivity to light stimulation during embryonic development interact in terms of their effects on behavior after hatching.
By removing the egg shell and membranes of quail embryos 24 to 36 h before hatching, Lickliter (1990) studied the influence of patterned visual stimulation on choices made after hatching. Quail chicks that had received the visual experience prior to hatching integrated visual with auditory information when tested soon after hatching, whereas chicks hatched normally without pre-hatching visual stimulation responded only to auditory inputs. Although this study did not assess lateralization, a study by Casey and Lickliter (1998) did show that the visual stimulation pre-hatching enhances lateralization, measured as turning bias after hatching (a type of visuo-motor laterality). This suggests that visual experience prior to hatching may enhance integration of sensory inputs via an effect on brain lateralization.
Processing of auditory information by the domestic chick is lateralized. During the first week after hatching, chicks turn their right ear toward an auditory sound source (Andrew and Dharmaretnam, 1991), showing a preference to use the left hemisphere. This finding is supported by evidence that disrupting left-hemisphere function impairs auditory habituation, whereas the same treatment of the right hemisphere has no effect (Rogers and Anson, 1979; Howard et al., 1980).
Exposing chick embryos to auditory experience during the final days of incubation does not appear to alter laterality, at least in tasks that rely primarily on visual behavior (Zappia and Rogers, 1983). Also, auditory stimulation during the final 3-4 days of incubation has no effect on lateralization of habituation to an auditory stimulus (Zappia and Rogers, 1983), and light-induced lateralization is not affected by exposing the late-stage embryos to sounds at the same time as the light exposure (Zappia and Rogers, 1983). Similarly, Casey and Lickliter (1998) found that exposing quail embryos to the sounds made by embryos just prior to hatching had no affect on turning bias of the chicks after hatching, whereas exposure to light did enhance side-bias of turning.
The absence of any effect of auditory stimulation in these experiments could be due to the late stage when the exposure to sounds was applied. Chick embryos already respond to low frequency auditory stimulation on day 12 of incubation (Rogers, 1995), well before the visual system becomes functional. Hence, it is possible that auditory stimulation may influence the development of auditory lateralization if it is applied during a sensitive period midway through incubation instead of just before hatching. This has not yet been tested.
A complication of empirically testing the effects of auditory stimulation on development of lateralization before hatching is the interaction between visual and auditory experience (Lickliter and Lewkowicz, 1995). Testing quails, Foushée and Lickliter (2002) showed that visual experience prior to hatching interferes with auditory learning of species-typical vocalizations. This could involve the right hemisphere since, as shown recently, this hemisphere processes and integrates visual and auditory inputs (Harshaw et al., 2021).
Regardless of which hemisphere is involved, it is it worth considering that, if lateralization of the auditory system develops in response to auditory stimulation midway through incubation, this particular laterality may be enhanced or suppressed by the later development in ovo of visual asymmetry.
Detection and response to odors is lateralized in the domestic chick, as it is also in other species (Cavelius et al., 2022). Chicks respond to lower doses of odors when they detect them using their right nostril than they do when using their left nostril (Vallortigara and Andrew, 1994; Burne and Rogers, 2002). Since each nostril sends its primary inputs to its ipsilateral hemisphere, this means that the right hemisphere processes olfactory information.
Although the olfactory system develops rather early during incubation, it is unlikely that it is functional until day 20 of incubation when the nares are freed of obstructing material (Rogers, 1995). Dissimilar to visual and auditory stimulation, which in late incubation are lateralized to the right eye and ear respectively, it seems rather unlikely that olfactory stimulation of the embryo is temporarily restricted to one nostril, unless the obstructing material of one nostril is cleared in advance of the other. Just before hatching the embryo can certainly detect odors and this leads to development of olfactory preferences after hatching (Tolhurst and Vince, 1976; Burne and Rogers, 1999) but there has been no investigation of whether this pre-hatching olfactory stimulation is lateralized.
At the current stage of knowledge, it seems that lateralization of olfactory processing is genetically determined and not affected by epigenetic influences during development of the embryo. Nevertheless, lateralized olfactory behavior after hatching is influenced by visual inputs. For example, chicks perform head shaking after they have pecked an attractive blue bead coupled with clove oil odor provided that they use the right nostril and not when they use the left nostril (Rogers et al., 1998). However, if the same odor is coupled with a less attractive red bead, no laterality is manifested: the chicks head-shake to the same amount when the odor is presented to the right or the left nostril (Rogers et al., 1998). In other words, there are left-right differences in integration of visual and olfactory inputs. Visual inputs compete with olfactory inputs from the left nostril to the left hemisphere but this competition does not occur in the right hemisphere, indicating a clear link between lateralized processing of visual information and processing of olfactory information. It is perhaps worth mentioning here that sensory responses of zebrafish to light and odor are lateralized to opposite sides of the epithalamic region of the brain and changing the laterality of one changes laterality of the other (Dreosti et al., 2014).
Using the precocial avian embryo as a model for study, an epigenetic influence of light exposure on the development of lateralized visual behavior occurs during two sensitive periods that lead to lateralized visual processing in hatched chicks. The generated visual lateralities also interact with both auditory and olfactory stimulation after hatching and may enhance or mask lateralities in these modalities. Further research is needed to determine whether auditory or olfactory stimulation of the embryo plays a direct role or interactive role in the development of lateralization in these sensory modalities. So far, it seems that, after hatching, laterality of processing visual information plays a key role, possibly and over-riding one, in laterality of the chick’s response to sounds and odors.
Asymmetric tactile stimulation is another potential epigenetic influence on development so far not investigated. It may have an influence given the asymmetrical posture adopted by the embryo, especially in the early stages of incubation when the embryo lies with its left side against the egg yolk and membranes. Since tactile sensitivity has been recorded as early as on day 6 of incubation (Hamburger and Balaban, 1963; Freeman and Vince, 1974; Rogers, 1995), any lateralized tactile influence on development is expected to occur quite early in incubation.
This paper has focused on development of lateralization in precocial avian species but the epigenetic effect of light stimulation has also been studied in the pigeon, an altricial species, revealing similar, though not identical, effects of light on lateralized behavior and asymmetry of visual pathways (Manns and Römling, 2012; Manns and Ströckens, 2014; Letzner et al., 2020; Manns, 2021; Pusch et al., 2022). Light exposure also alters the development of lateralization in the zebrafish (Andrew et al., 2009; Budaev and Andrew, 2009). It makes sense, therefore, to predict that light stimulation might influence the development of laterality in mammalian species (Rogers, 2020). Asymmetry of sensory inputs in other modalities might also affect development of lateralization: in fact, asymmetrical vestibular inputs have recently been shown to affect motor asymmetry in mice, leading to prediction that early, inner ear imbalance may contribute to the development of handedness in humans (Antoine et al., 2018).
The author confirms being the sole contributor of this work and has approved it for publication.
The author declares that the research was conducted in the absence of any commercial or financial relationships that could be construed as a potential conflict of interest.
All claims expressed in this article are solely those of the authors and do not necessarily represent those of their affiliated organizations, or those of the publisher, the editors and the reviewers. Any product that may be evaluated in this article, or claim that may be made by its manufacturer, is not guaranteed or endorsed by the publisher.
Andrew, R. J., and Dharmaretnam, M. (1991). “A timetable of development,” in Neural and behavioural plasticity: The use of the domestic chick as a model, ed. R. J. Andrew (Oxford: Oxford University Press), 166–176.
Andrew, R. J., Osorio, D., and Budaev, S. (2009). Light during embryonic development modulates patterns of lateralization strongly and similarly in both zebrafish and chick. Phil. Trans. R. Soc. 364, 983–989. doi: 10.1098/rstb.2008.0241
Antoine, M. W., Zhu, X., Dieterich, M., Brandt, T., Vijayakumar, S., McKeehan, N., et al. (2018). Early uneven ear input induces long-lasting differences in left-right motor function. PLoS Biol. 16:e2002988. doi: 10.1371/journal.pbio.2002988
Archer, G. S., and Mench, J. A. (2014). Natural incubation patterns and the effects of exposing eggs to light at various times during incubation on post-hatch fear and stress responses in broiler (meat) chickens. Appl. Anim. Behav. Sci. 152, 44–51.
Budaev, S. V., and Andrew, R. J. (2009). Shyness and behavioural asymmetries in larval zebrafish (Brachydanio rerio) developed in light and dark. Behaviour 146, 1037–1052.
Bullock, S. P., and Rogers, L. J. (1986). Glutamate-induced asymmetry in the sexual and aggressive behaviour of young chickens. Pharm. Biochem. Behav. 24, 549–554. doi: 10.1016/0091-3057(86)90556-3
Burne, T. H. J., and Rogers, L. J. (1999). Changes in olfactory responsiveness by the domestic chick following early exposure to odorants. Anim. Behav. 58, 329–336. doi: 10.1006/anbe.1999.1151
Burne, T. H. J., and Rogers, L. J. (2002). Chemosensory input and lateralization of brain function in the domestic chick. Behav. Brain Res. 133, 293–300. doi: 10.1016/s0166-4328(02)00009-8
Butcher, G. D., and Nilipour, A. H. (2002). Chicken embryo malpositions and deformities. Gainesville, FL: University of Florida.
Casey, M. B., and Lickliter, R. (1998). Prenatal visual experience influences the development of turning bias in bobwhite quail chicks (Colinus virginianus). Dev. Psychobiol. 32, 327–338.
Casey, M. B., and Sleigh, M. J. (2014). Prenatal visual experience induces postnatal motor laterality in Japanese quail chicks (Coturnix japonica). Dev. Psychobiol. 56, 489–497. doi: 10.1002/dev.21116
Cavelius, M., Brunel, T., and Didier, A. (2022). Lessons from behavioral lateralization in olfaction. Brain Struct. Funct. 227, 685–696. doi: 10.1007/s00429-021-02390-w
Chiandetti, C., and Vallortigara, G. (2009). Effects of embryonic light stimulation on the ability to discriminate left from right in the domestic chick. Behav. Brain Res. 198, 240–246. doi: 10.1016/j.bbr.2008.11.018
Chiandetti, C., and Vallortigara, G. (2019). Distinct effect of early and late embryonic light-stimulation on chicks’ lateralization. Neuroscience 414, 1–7. doi: 10.1016/j.neuroscience.2019.06.036
Chiandetti, C., Galliussi, J., Andrew, R. J., and Vallortigara, G. (2013). Early-light embryonic stimulation suggests a second route, via gene activation, to cerebral lateralizationin vertebrates. Sci. Rep. 3:2701. doi: 10.1038/srep02701
Chiandetti, C., Lemaire, B., Versace, E., and Vallortigara, G. (2017). Early- and late-light embryonic stimulation modulates similarly chicks’ ability to filter out distractors. Symmetry 9:84. doi: 10.3390/sym9060084
Chiandetti, C., Regolin, L., Rogers, L. J., and Vallortigara, G. (2005). Effects of light stimulation in embryo on the use of position-specific and object-specific cues in binocular and monocular chicks (Gallus gallus). Behav. Brain Res. 163, 10–17. doi: 10.1016/j.bbr.2005.03.024
Concha, M. L., Burdine, R. D., Russell, C., Schier, S. W., and Wilson, S. W. (2000). A nodal signaling pathway regulates the laterality of neuroanatomical asymmetries in the zebrafish forebrain. Neuron 28, 399–409.
Concha, M. L., Signore, I. A., and Colombo, A. (2009). Mechanisms of directional asymmetry in the zebrafish epithalamus. Semin. Cell Dev. Biol. 20, 498–509.
Costalunga, G., Kobylkov, D., Rosa-Salva, O., Vallortigara, G., and Mayer, U. (2022). Light-incubation effects on lateralisation of single unit responses in the visual Wulst of domestic chicks. Brain Struct. Funct. 227, 497–513. doi: 10.1007/s00429-021-02259-y
Daisley, J. N., Mascalzoni, E., Salva, O. R., Rugani, R., and Regolin, L. (2009). Lateralization of social cognition in the domestic chicken (Gallus gallus). Phil. Trans. R. Soc. Lond. B Biol. Sci. 364, 965–981. doi: 10.1098/rstb.2008.0229
Deng, C., and Rogers, L. J. (2002). Social recognition and approach in the chick: Lateralization and effect of visual experience. Anim. Behav. 63, 697–706.
Doh, S. T., Hao, H., Loh, S. C., Patel, T., Tawil, H. Y., Chen, D. K., et al. (2010). Analysis of retinal cell development in chick embryo by immunohistochemistry and in ovo electroporation techniques. BMC Dev. Biol. 10:8. doi: 10.3390/sym13101815
Dreosti, E., Llopis, N. V., Carl, M., Yaksi, E., and Wilson, S. W. (2014). Left-right asymmetry is required for the habenulae to respond to both visual and olfactory stimuli. Curr. Zool. 24, 440–445. doi: 10.1016/j.cub.2014.01.016
Foushée, R. D., and Lickliter, R. (2002). Early visual experience affects postnatal auditory responsiveness in bobwhite quail (Colinus virginianus). J. Comp. Psych. 116, 369–380. doi: 10.1037/0735-7036.116.4.369
Guglielmotti, V., and Cristino, L. (2006). The interplay between the pineal complex and the habenular nuclei in lower vertebrates in the context of the evolution of cerebral asymmetry. Brain Res. Bull. 69, 475–488. doi: 10.1016/j.brainresbull.2006.03.010
Hamburger, V., and Balaban, M. (1963). Observations and experiments on spontaneous rhythmical behaviour in the chick embryo. Dev. Biol. 7, 533–545.
Harshaw, C., Ford, C. B., and Lickliter, R. (2021). Hearing better with the right eye? The lateralization of multisensory processing affects auditory learning in Northern bobwhite quail (Colinus virginianus) chicks. Appl. Anim. Behav. Sci. 236:105274. doi: 10.1016/j.applanim.2021.105274
Howard, K. J., Rogers, L. J., and Boura, A. L. A. (1980). Functional lateralisation of the chicken fore-brain revealed by use of intracranial glutamate. Brain Res. 188, 369–382. doi: 10.1016/0006-8993(80)90038-4
Kovach, J. K. (1968). Spatial orientation of the chick embryo during the last five days of incubation. J. comp. Physiol. Psychol. 66, 283–288. doi: 10.1037/h0026374
Kuo, C., Tamoku, S., Morita, Y., Akiyama, M., and Miki, N. (2003). Presence of retina-specific proteins in the lamprey pineal complex. Brain Res. 442, 147–151. doi: 10.1016/0006-8993(88)91442-4
Letzner, S., Güntürkün, O., Lor, S., Pawlik, R. J., and Manns, M. (2017). Visuospatial attention in the lateralised brain of pigeons–a matter of ontogenetic light experiences. Sci. Rep. 7:15547. doi: 10.1038/s41598-017-15796-6
Letzner, S., Manns, M., and Güntürkün, O. (2020). Light-dependent development of the tectorotundal projection in pigeons. Eur. J. Neurosci. 52, 3561–3571. doi: 10.1111/ejn.14775
Letzner, S., Patzke, N., Verhaal, J., and Manns, M. (2014). Shaping a lateralized brain: Asymmetrical light experience modulates access to visual interhemispheric information in pigeons. Sci. Rep. 4:4253. doi: 10.1038/srep04253
Levin, M., Johnson, R. L., Sten, C. D., Kuehn, M., and Tabin, C. (1995). A molecular pathway determining left-right asymmetry in chick embryogenesis. Cell 82, 803–814.
Liang, J. O., Etheridge, A., Hantsoo, L., Rubinstein, A. L., Nowak, S. J., Izpisá Belmonte, J. C., et al. (2000). Asymmetric nodal signaling in the zebrafish diencephalon positions the pineal organ. Development 127, 5101–5112.
Lickliter, R. (1990). Premature visual stimulation accelerates intersensory functioning in bobwhite quail neonates. Dev. Psychobiol. 22, 651–667. doi: 10.1002/dev.420230103
Lickliter, R., and Lewkowicz, D. J. (1995). Intersensory experience and early perceptual development: Attenuated prenatal sensory stimulation affects postnatal auditory and visual responsiveness in bobwhite quail chicks (Colinus virginianus). Dev. Psychobiol. 31, 609–618.
Lorenzi, E., Mayer, U., Rosa-Salva, O., Morandi-Raikova, A., and Vallortigara, G. (2019). Spontaneous and light-induced lateralization of immediate early genes expression in domestic chicks. Behav. Brain Res. 368:111905. doi: 10.1016/j.bbr.2019.111905
Manns, M., and Römling, J. (2012). The impact of asymmetrical light input on cerebral hemispheric specialization and interhemispheric cooperation. Nat. Commun. 3:696. doi: 10.1038/ncomms1699
Manns, M., and Ströckens, F. (2014). Functional and structural comparison of visual lateralization in birds-similar but still different. Front. Psychol. 5:206. doi: 10.3389/fpsyg.2014.00206
Mench, J. A., and Andrew, R. J. (1986). Lateralization of a food search task in the domestic chick. Behav. Neural Biol. 46, 107–114.
Morandi-Raikova, A., and Mayer, U. (2021). Selective activation of the right hippocampus during navigation by spatial cues in domestic chicks (Gallus gallus). Neurobiol. Learn. Memory 177:107344. doi: 10.1016/j.nlm.2020.107344
Nakamura, T., and Hamada, H. (2012). Left-right patterning: Conserved and divergent mechanisms. Development 139, 3257–3262. doi: 10.1242/dev.061606
Olsen, M. W., and Byerly, T. (1935). The orientation of the embryo in the egg of the domestic fowl. Poult. Sci. 14, 46–53.
Pusch, R., Clark, W., Rose, J., and Güntürkün, O. (2022). Visual categories and concepts in the avian brain. Anim. Cogn. doi: 10.1007/s10071-022-01711-8
Rogers, L. J. (1982). Light experience and asymmetry of brain function in chickens. Nature 297, 223–225.
Rogers, L. J. (1990). Light input and the reversal of functional lateralization in the chicken brain. Behav. Brain Res. 38, 211–221.
Rogers, L. J. (1995). The development of brain and behaviour in the chicken. Wallingford: CAB International.
Rogers, L. J. (1997). Early experiential effects on laterality: Research on chicks has relevance to other species. Laterality 2, 199–219. doi: 10.1080/713754277
Rogers, L. J. (2000). Evolution of hemispheric specialisation: Advantages and disadvantages. Brain Lang. 73, 236–253.
Rogers, L. J. (2020). Asymmetry of motor behavior and sensory perception: Which comes first? Symmetry 12:690. doi: 10.3390/sym12050690
Rogers, L. J., and Anson, J. M. (1979). Lateralisation of function in the chicken fore-brain. Pharm. Biochem. Behav. 10, 679–686.
Rogers, L. J., and Deng, C. (1999). Light experience and lateralization of the two visual pathways in the chick. Behav. Brain Res. 98, 277–287.
Rogers, L. J., and Workman, L. (1989). Light exposure during incubation affects competitive behaviour in domestic chicks. Appl. Anim. Behav. Sci. 23, 187–198.
Rogers, L. J., Andrew, R. J., and Burne, T. H. J. (1998). Light exposure of the embryo and development of behavioural lateralisation in chicks, I: Olfactory responses. Behav. Brain Res. 97, 195–200.
Rogers, L. J., Vallortigara, G., and Andrew, R. J. (2013). Divided brains: The biology and behaviour of brain asymmetries. Cambridge: Cambridge University Press.
Rogers, L. J., Zappia, J. V., and Bullock, S. P. (1985). Testosterone and eye-brain asymmetry for copulation in chickens. Experientia 41, 1447–1449.
Rosa Salva, O., Mascalzoni, E., Regolin, L., and Vallortigara, G. (2012). Cerebral and behavioural asymmetries in animal social recognition. Comp. Cogn. Behav. Rev. 7:10. doi: 10.3819/ccbr.2012.70006
Rugani, R., Rosa Salva, O., Regolin, L., and Vallortigara, G. (2015). Brain asymmetry modulates perception of biological motion in newborn chicks (Gallus gallus). Behav. Brain Res. 290, 1–7. doi: 10.1016/j.bbr.2015.04.032
Stewart, M. G., Rogers, L. J., Davies, H. A., and Bolden, S. W. (1992). Structural asymmetry in the thalamofugal visual projections in the 2-day-old chick is correlated with a hemispheric difference in synaptic number in the hyperstriatum accessorium. Brain Res. 585, 381–385. doi: 10.1016/0006-8993(92)91241-6
Tolhurst, B. E., and Vince, M. A. (1976). Sensitivity to odours in the embryo of the domestic fowl. Anim. Behav. 24, 772–779.
Tommasi, L., and Vallortigara, G. (2001). Encoding of geometric and landmark information in the left and right hemispheres of the avian brain. Behav. Neurosci. 115, 602–613.
Tommasi, L., Gagliardo, A., Andrew, R. J., and Vallortigara, G. (2003). Separate processing mechanisms for encoding of geometric and landmark information in the avian hippocampus. Eur. J. Neurosci. 17, 1695–1702. doi: 10.1046/j.1460-9568.2003.02593.x
Vallortigara, G., and Andrew, R.J. (1994). Olfactory lateralization in the chick. Neuropsychologia 32, 417–423.
Vallortigara, G., and Rogers, L. J. (2005). Survival with an asymmetrical brain: Advantages and disadvantages of cerebral lateralization. Behav. Brain Sci. 28, 575–633. doi: 10.1017/S0140525X05000105
Versace, E., Sgado, P., George, J., Loveland, J. L., Ward, J., Thorpe, P., et al. (2022). Light-induced asymmetries in embryonic retinal gene expression are mediated by the vascular system and extracellular matrix. Sci. Rep. 12:12086. doi: 10.1038/s41598-022-14993-8
Wu, K., Streicher, J., Lee, M. L., Hall, B. K., and Müller, G. B. (2001). Role of motility in embryonic development I: Embryo movements and amnion contractions in the chick and the influence of illumination. J. Exp. Zool. 291, 186–194. doi: 10.1002/jez.1068
Keywords: lateralization, development, sensory stimulation, avian embryo, vision, audition, olfaction, behavior
Citation: Rogers LJ (2023) Unfolding a sequence of sensory influences and interactions in the development of functional brain laterality. Front. Behav. Neurosci. 16:1103192. doi: 10.3389/fnbeh.2022.1103192
Received: 20 November 2022; Accepted: 16 December 2022;
Published: 06 January 2023.
Edited by:
Luca Tommasi, University of Studies G. d’Annunzio Chieti and Pescara, ItalyReviewed by:
Anastasia Morandi-Raikova, University of Trento, ItalyCopyright © 2023 Rogers. This is an open-access article distributed under the terms of the Creative Commons Attribution License (CC BY). The use, distribution or reproduction in other forums is permitted, provided the original author(s) and the copyright owner(s) are credited and that the original publication in this journal is cited, in accordance with accepted academic practice. No use, distribution or reproduction is permitted which does not comply with these terms.
*Correspondence: Lesley J. Rogers, bHJvZ2Vyc0B1bmUuZWR1LmF1
Disclaimer: All claims expressed in this article are solely those of the authors and do not necessarily represent those of their affiliated organizations, or those of the publisher, the editors and the reviewers. Any product that may be evaluated in this article or claim that may be made by its manufacturer is not guaranteed or endorsed by the publisher.
Research integrity at Frontiers
Learn more about the work of our research integrity team to safeguard the quality of each article we publish.