- 1Division of Pharmacology, Faculty of Pharmaceutical Sciences, Tohoku Medical and Pharmaceutical University, Sendai, Japan
- 2Department of Pharmacy, Fukushima Medical University Hospital, Fukushima, Japan
- 3Division of Clinical Pharmaceutical Therapy, Faculty of Pharmaceutical Sciences, Tohoku Medical and Pharmaceutical University, Sendai, Japan
- 4Laboratory of Forensic Toxicology, Faculty of Pharmacy, Takasaki University of Health and Welfare, Takasaki-shi, Japan
- 5Faculty of Health Science, Tohoku Fukushi University, Sendai, Japan
- 6Department of Pharmacology, School of Pharmacy, International University of Health and Welfare, Otawara, Tochigi, Japan
- 7Center for Research on Counseling and Support Services, The University of Tokyo, Bunkyō-ku, Tokyo, Japan
- 8Douglas Hospital Research Centre, McGill University, Montreal, QC, Canada
- 9Complementary and Alternative Medicine Clinical Research and Development, Graduate School of Medicine Sciences, Kanazawa University, Kanazawa, Japan
Genetic studies in humans have implicated the gene encoding neuregulin-1 (NRG-1) as a candidate susceptibility gene for schizophrenia. Furthermore, it has been suggested that NRG-1 is involved in regulating the expression and function of the N-methyl-D-aspartate receptor and the GABAA receptor in several brain areas, including the prefrontal cortex (PFC), the hippocampus, and the cerebellum. Neonatal ventral hippocampal lesioned (NVHL) rats have been considered as a putative model for schizophrenia with characteristic post-pubertal alteration in response to stress and neuroleptics. In this study, we examined NRG-1, erb-b2 receptor tyrosine kinase 4 (erbB4), and phospho-erbB4 (p-erbB4) levels in the PFC and the distribution of NRG-1 in the NVHL rats by using immunoblotting and immunohistochemical analyses. Neonatal lesions were induced by bilateral injection of ibotenic acid in the ventral hippocampus of postnatal day 7 Sprague-Dawley (SD)-rats. NVHL rats showed significantly decreased levels of NRG-1 and p-erbB4 in the PFC compared to sham controls at post-pubertal period, while the level of erbB4 did not differ between sham and NVHL rats. Moreover, microinjection of NRG-1 into the mPFC improved NVHL-induced prepulse inhibition deficits. Our study suggests PFC NRG-1 alteration as a potential mechanism in schizophrenia-like behaviors in the NVHL model.
Highlights
- NVHL rats show significantly decreased NRG-1 and p-erbB4 levels in the PFC.
- Microinjection of NRG-1 into the mPFC improved NVHL-induced PPI deficits.
Introduction
Excitotoxic neonatal ventral hippocampus lesioned (NVHL) rats have been proposed as a putative animal model for schizophrenia as these animals display post-pubertal neurochemical and behavioral abnormalities analogous to symptoms seen in this neuropsychiatric disorders (Lipska and Weinberger, 2000; Marcotte et al., 2001). For example, post-pubertal NVHL animals are hyper-reactive to stress and amphetamine, display deficits in prepulse inhibition (PPI) of startle and latent inhibition, and impaired social interaction and working memory (Sams-Dodd et al., 1997; Becker et al., 1999; Mitazaki et al., 2020).
Neuregulin-1 (NRG-1) gene and its receptor erb-b2 receptor tyrosine kinase 4 (erbB4) are significant risk factors for schizophrenia (Stefansson et al., 2002; Norton et al., 2006; Silberberg et al., 2006). In the brain, NRG-1 plays multiple roles in synapse formation, activity-dependent synaptic plasticity, and regulation of N-methyl-D-asparate, and GABAA receptor subunit expression (Ozaki et al., 1997; Rieff et al., 1999; Liu et al., 2001; Falls, 2003; Hahn et al., 2006; Woo et al., 2007). Postmortem studies have shown that schizophrenia patients have a decreased cortical NRG-1 expression (Bertram et al., 2007; Marballi et al., 2012). Moreover, mutant mice carrying mutations in the NRG-1 or erbB4 gene show schizophrenia-like behaviors such as disruption of PPI, latent inhibition and cognitive deficits (Stefansson et al., 2002; Falls, 2003; Hashimoto et al., 2004; Rimer et al., 2005; O’Tuathaigh et al., 2007). NVHL alters the development and function of the prefrontal cortex (PFC), which receives prominent ventral hippocampus projections, and is implicated in many complex behaviors disrupted by this lesion (Sams-Dodd et al., 1997; Becker et al., 1999; Lipska and Weinberger, 2000; Marcotte et al., 2001; Flores et al., 2005).
Here, we investigated whether PFC neuregulin participates in the behaviors of NVHL rats by assessing the expression of NRG-1 and its receptors, erbB4 and phospho-erbB4 (p-erbB4), in the PFC of NVHL rats using immunoblotting and immunohistochemical. In addition, we assessed the effects of intra-cortical administration of NRG-1 on PPI deficits of NVHL animals.
Materials and methods
All experiments were performed following the approval of the Ethics Committee of Animal Experiment in Tohoku Medical and Pharmaceutical University (approval numbers: A10016 and A11035) and according to the National Institutes of Health Guide for the Care and Use of Laboratory Animals. Efforts were made to minimize suffering and reduce the number of animals used. Measurements of the behaviors and post-mortem analyses were conducted by an observer blind to treatment conditions. Behavioral testing occurred between 10:00 and 18:00.
Neonatal ventral hippocampal lesion
Lesions of the ventral hippocampus in pups were performed as previously described (Flores et al., 1996). Pregnant Sprague–Dawley rats at 15 days of gestation were obtained from Japan SLC (Hamamatsu, Shizuoka, Japan), housed individually in 12-h light/dark cycle rooms and fed ad libidum. On post-natal day 7 (PD 7) male pups (15–17 g) within each litter (4–9 males/litter) were randomly divided to sham or lesion status. Pups were anesthetized by hypothermia by placing them on ice for 20 min and were immobilized on a platform fixed on a stereotaxic frame. An incision in the skin overlaying the skull was made and two 1 mm holes were drilled. A needle connected to an infusion pump through a Hamilton syringe was lowered into the ventral hippocampus at the coordinates: AP—3.0 mm ML ± 3.5 relative to bregma and—5.0 relative to the surface of the skull. Ibotenic acid (0.3 μl, 10 μg/μl; Sigma, Chemical Co, St-Louis, MO, USA) in 0.15 M phosphate-buffered saline (PBS) pH 7.4 was infused bilaterally at a flow rate of 0.15 μl/min. Sham operated animals received the same volume of PBS. The needle was withdrawn 2 min after completion of the infusion. Pups were placed under a warming lamp and then returned to their mothers. On PD 21–25, rats were weaned and grouped 2–3 per cage. Experiments were performed on post-pubertal (PD 70–80) animals.
Drugs
NRG-1 (500 ng or 1000 ng/0.5 μl; R&D Systems, Minneapolis, MN, USA) was dissolved in 0.15 M PBS (pH 7.4), and infused bilaterally in the prelimbic cortex (PrL) (AP + 2.8 mm ML ± 0.5 relative to bregma and—5.0 relative to the surface of the skull) at a flow rate of 0.25 μl/min under anesthesia with pentobarbital Na (50 mg/kg, intraperitoneally administration; Kyoritsu Seiyaku, Tokyo, Japan). The dose for NRG-1 used was calculated based on a previous report (Jalilzad et al., 2019).
Histology
To assess the location of the lesion, adult rats were sacrificed by decapitation and brains were removed and frozen in 2-methylbutane at –40°C and stored at –80°C until sectioning using a cryostat. We visually confirmed the ventral hippocampal location (Bregma from −4.6 to −6.0 mm) using rat brain stereotaxic coordinates (Paxinos and Franklin, 2013). Coronal sections (Bregma −4.8 mm, 20 μm thick) were mounted onto gelatin-coated slides and stained with cresyl violet. Lesion size was confirmed by digital camera. As shown in Figure 1, bilateral damages including neuronal loss, atrophy and cavitation of the ventral hippocampus was observed in ibotenic acid-treated rats. Animals exhibiting damage in the dorsal hippocampus, thalamus or cortex were excluded from the study. Sham control animals did not show any obvious damage in hippocampal areas.
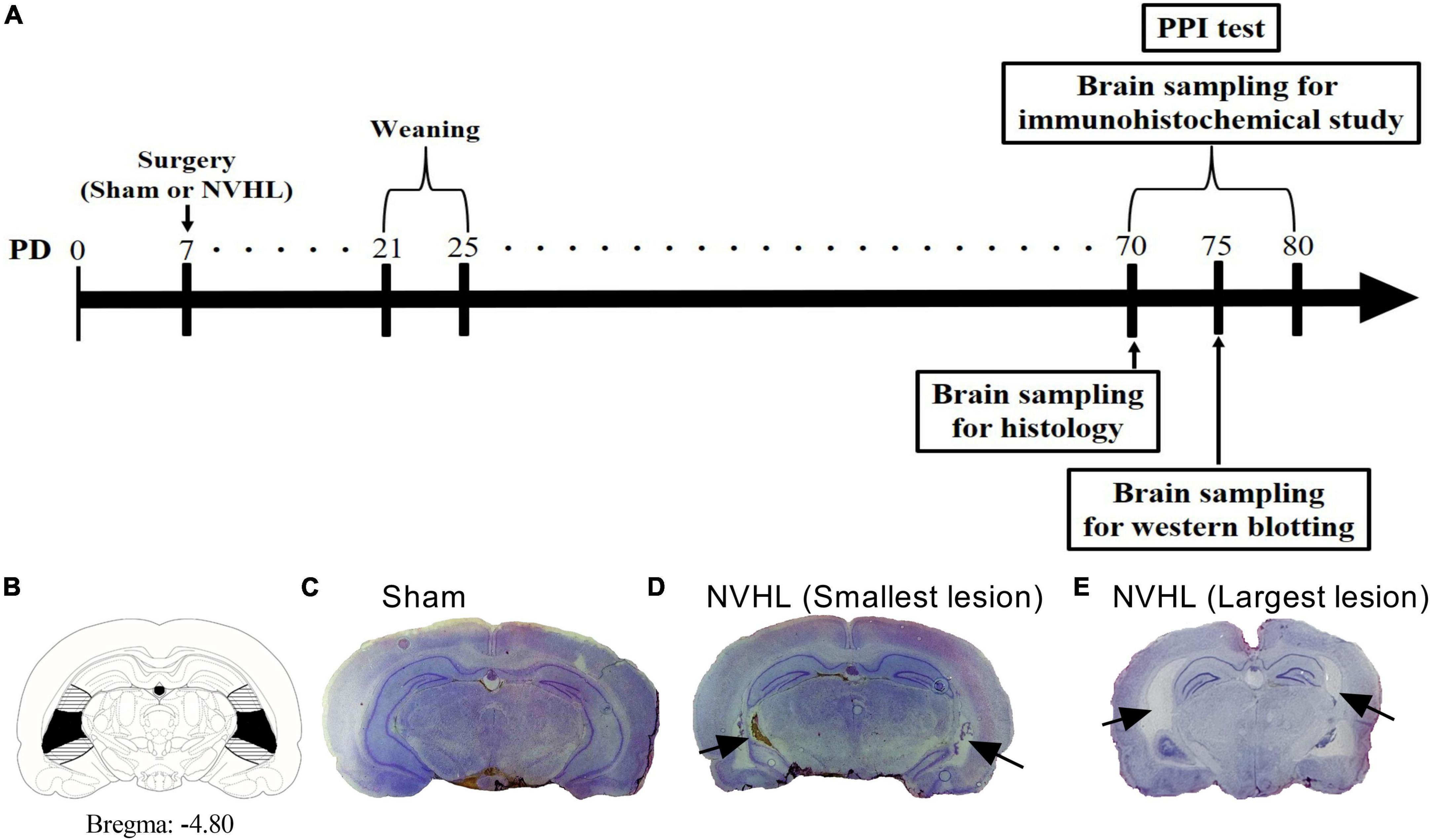
Figure 1. Experimental time course for protocol (A). (B) Diagram showing the dorsal to ventral hippocampus. Horizontal bars and blackened areas indicate the largest and smallest lesions, respectively. (C) Sham rat. (D) NVHL rat (smallest lesion). (E) NVHL rat (largest lesion). A typical cresyl violet stained coronal sections at the level of ventral hippocampus of a PD 70 rat indicating ibotenic acid induced neuron loss (arrow) in the ventral hippocampus (D,E).
Immunohistochemical procedure
The immunohistochemical analysis of NRG-1 was performed on PD 70-80 rats. The rats were anesthetized with Na pentobarbital and perfused through the heart with ice-cold PBS (pH 7.4), immediately followed by a fixative containing 4% paraformaldehyde (Sigma−Aldrich) and 0.2% glutaraldehyde (Nacalai Tesque, Osaka, Japan) in PBS. The brain was then postfixed with the same fixative solution at 4°C for 1 h and then placed in a 20% sucrose-buffered solution at 4°C for 12 h. The tissue was frozen on dry ice and cut into 20-μm-thick coronal sections (Bregma from +4.20 to +2.70 mm) on a cryostat (Leitz, Stuttgart, Germany). The immunohistochemical staining procedure was carried out as previously described (Sutoo et al., 2001; Yabuki et al., 2013). For immunohistochemical staining, sections were incubated with NRG-1 antibody (1:500; Santa Cruz Biotechnology, Santa Cruz, CA, USA, Cat# sc-348) was diluted in PBS containing 1% normal goat serum and 0.1% Triton-X 100 at 4°C for 12 h. This was followed with incubation for 3 h with the secondary antibody FITC-labeled anti-rabbit IgG goat serum (1:200; BioSource International, Inc., Camarillo, CA, USA). The stained sections were mounted in 10% glycerin-PBS, and kept at 4°C in a dark room. The distribution of NRG-1 immunofluorescence intensities was quantitatively analyzed using a modified brain mapping analyzer system (Yamato Scientific Co., Inc., Tokyo, Japan). The background value, such as non-specific fluorescence originating from glutaraldehyde, was subtracted photometrically from the total fluorescence intensity value at each measuring point. Immunohistochemical fluorescence intensities obtained for the regions were indicated relative to that of standard 1mM quinine sulfate. The average NRG-1 fluorescence intensity in each region was obtained from 2 to 3 sections per animal.
Western blotting
Western immunoblotting analysis was performed using medial PFC samples from rats that had not undergone any behavioral testing. Brain was removed and sectioned on ice, using a rat brain slicer (Muromachi Kikai, Tokyo, Japan), to produce 1 mm thick coronal sections. We visually confirmed the medial PFC location using rat brain stereotaxic coordinates (Paxinos and Franklin, 2013). Protein isolation and western blots were performed as described previously (Takahashi et al., 2018). The blots were incubated with a blocking solution (25 mM Tris-HCl pH 7.4, 137 mM NaCl, 2.68 mM KCl, and 10% skim milk) for 4 h and then with NRG-1 antibody (1:1000; Santa Cruz Biotechnology; Cat# sc-348), erbB4 (1:1000; Santa Cruz Biotechnology; Cat# sc-18), p-erbB4 (1:5000; Santa Cruz Biotechnology; Cat# sc-33040), or β-actin to control for protein loading (1:1000; Cell Signaling Technology; Cat# 4967) overnight at 4°C. The membrane was then washed with blocking solution without skim milk, and horseradish peroxidase-linked secondary antibody (goat anti-rabbit IgG HRP: 1:1000) for 1 h. Protein amount was analyzed by enhanced chemiluminescence with an ECL plus Western blotting detection system (Amersham, Arlington Height, IL, USA). The band density was measured by densitometry (Image-J 1.43μ, National Institute of Health).
PPI of the acoustic startle response
Prepulse inhibition tests were performed using the SR-LAB system (SR-LAB, San Diego Instruments, San Diego, CA, USA). Rats were placed in the Plexiglas enclosure 24 h after vehicle or NRG-1 administration. Ventilation was provided by a small electric fan that also generated a 70 dB background noise. Measures of both acoustic startle response (ASR) and PPI were obtained in a single session. Rats were allowed to acclimatize to the environment for 5 min before being tested during 42 discrete trials. On the first two trials, the magnitude of the ASR to a 120 dB noise-burst lasting 50 ms was measured. These first two startle noise-bursts were presented in order to habituate the animals to the testing procedure. Therefore, the ASR magnitude of these two trials was omitted from the statistical analysis of the mean ASR amplitude. On the subsequent 40 trials, the startle noise-burst was either presented alone or 100 ms after the presentation of a 30 ms duration prepulse. Prepulse intensity ranged from 3 to 15 dB above background noise and was varied randomly between trials in 3 dB steps. Measures were taken at each of the five prepulse intensities during five trials; animals were randomly presented with the startle noise-burst alone during another ten trials; null trials (background noise alone) were conducted during the other five trials. The same stimulus condition was never presented on more than two consecutive trials. The interval between each trial was programmed to a variable time schedule with an average duration of 15 s (range 5–30 s). A measure of startle response amplitude was derived from the mean of 100 digitized data points collected from stimulus onset at a rate of 1 kHz. PPI was expressed as a percentage based on the mean amplitude of response to the startle pulse-alone trials relative to those recorded under the five prepulse conditions: PPI = 100 − [(mean startle amplitude for prepulse + pulse trials/mean startle amplitude for pulse-alone trials) × 100]%. PPI under the five different prepulse intensities were averaged and used for statistical analysis.
Statistical analysis
Results are expressed as mean ± standard error of the mean (SEM). The significance of differences was determined by the Student’s t-test for two-group comparison, and by a two-way analysis of variance (ANOVA), followed by Tukey–kramer test for the startle amplitude and average PPI data for multigroup comparisons.
Results
Histological examination of the ventral hippocampal lesion
Experimental time course for protocol is shown in Figure 1A. As shown in Figures 1B–E, NVHL rats showed bilateral cell loss and cavitation of the ventral hippocampus, while sham-lesioned control animals did not show any obvious damage in ventral hippocampal areas.
Distribution of NRG-1 fluorescence intensity in the PFC
As shown in Figures 2E, F, NVHL rats at post-puberty showed a significant decrease in NRG-1 protein level in the PFC [Student’s t-test: t(5) = 2.98, p < 0.05, Figure 2F]. In addition, as shown in Figures 2A–C, immunohistochemical analyses showed a marked decrease in NRG-1 fluorescence intensity the prelimbic cortex (PrL), cigulated cortex area 1 (Cg1), secondary motor cortex (M2), and primary motor cortex (M1) in the NVHL group compared with the sham group of animals [Student’s t-test: PrL: t(27) = 2.31, p < 0.05, Cg1: t(27) = 3.0, p < 0.01, M2: t(27) = 3.26, p < 0.01, M1: t(27) = 3.013, p < 0.01, Figure 2D].
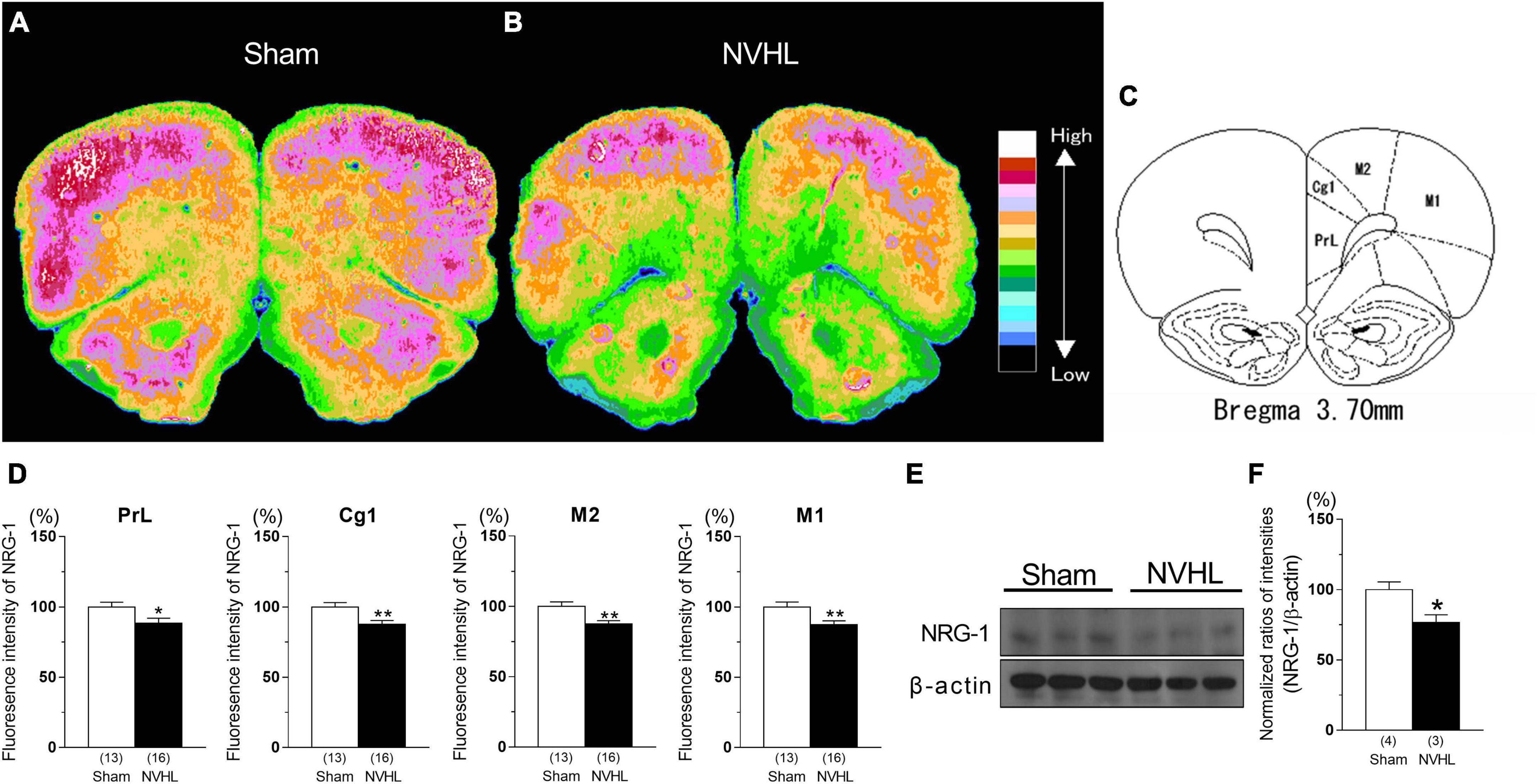
Figure 2. Reduced NRG-1 levels in the PFC in the NVHL rats at post-pubertal period. Immunohistochemical fluorescence intensity for NRG-1 in PFC. (A) Sham rat. (B) NVHL rat. (C) Diagram showing the prelimbic cortex (PrL), cingulated cortex area 1 (Cg1), secondary motor cortex (M2), and primary motor cortex (M1). (D) Values for the immunofluorescence intensity for NRG-1 in PrL, Cg1, M2, and M1. The distribution of NRG-1 fluorescence intensity in each rat PFC section was determined by microphotometry and classified into 16 levels [as indicated by color in panel (A,B), with lowest concentration in black and highest concentration in white]. Representative immunoblots probed with antibodies against NRG-1 and β-actin, as indicated (E). Quantification of the normalized values of NRG-1 levels with β-actin (F). Numbers in square brackets indicate the number of animals in each group. Bars represent means ± SEM. *p < 0.05 and **p < 0.01 vs. sham group.
Change in phosphorylation of erbB4 level in the PFC of NVHL rats
As shown in Figure 3, the immunocontent of phosphorylation of erbB4 level in the PFC of NVHL rats at post-puberty was significantly decreased compared to sham group, while erbB4 level was unchanged between sham and NVHL rats [Student’s t-test: t(6) = 0.22, p = 0.83, Figure 3A; t(7) = 2.67, p < 0.05, Figure 3B].
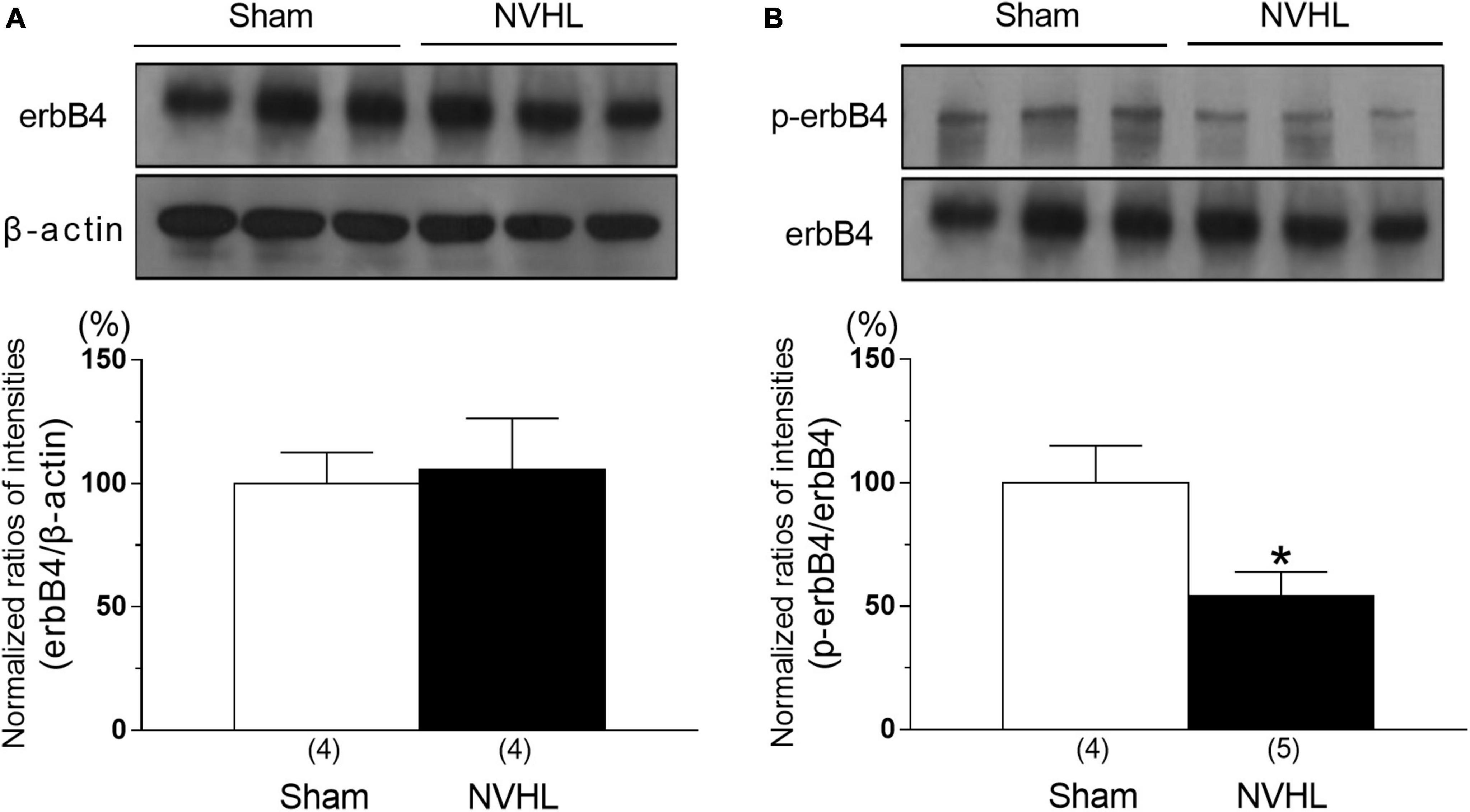
Figure 3. Alternation of erbB4 and p-erbB4 level in the PFC in the NVHL rats at post-pubertal period. Representative immunoblots probed with antibodies against β-actin, erbB4 (A), and p-erbB4 (B), as indicated. Quantification of the normalized values of erbB4 levels with β-actin (A) and p-erbB4 levels with erbB4 (B), respectively. Numbers in square brackets indicate the number of animals in each group. Bars represent means ± SEM. *p < 0.05 vs. sham group.
Effects of intra-cortical microinjection of NRG-1 on PPI in post-pubertal NVHL rats
Neonatal ventral hippocampal lesioned rats showed a decreased average PPI compared to sham control groups, while this change was reversed by microinjection of NRG-1 (1000 ng/0.5 μl) into the PFC of NVHL rats [Two-way ANOVA: group: F(1,48) = 53.55, p < 0.01, treatment: F(2,48) = 2.81, p < 0.05, group × treatment: F(2,48) = 2.74, p < 0.05; Post-hoc test (average PPI): 500 ng/0.5 μL: p = 0.081; 1000 ng/0.5 μL: p < 0.05, Figure 4B]. Regarding startle amplitude, it was unchanged among all groups [Two-way ANOVA: group: group: F(1,48) = 0.49, p = 0.49, treatment: F(2,48) = 0.57, p = 0.57, group × treatment: F(2,48) = 0.039, p = 0.96, Figure 4C].
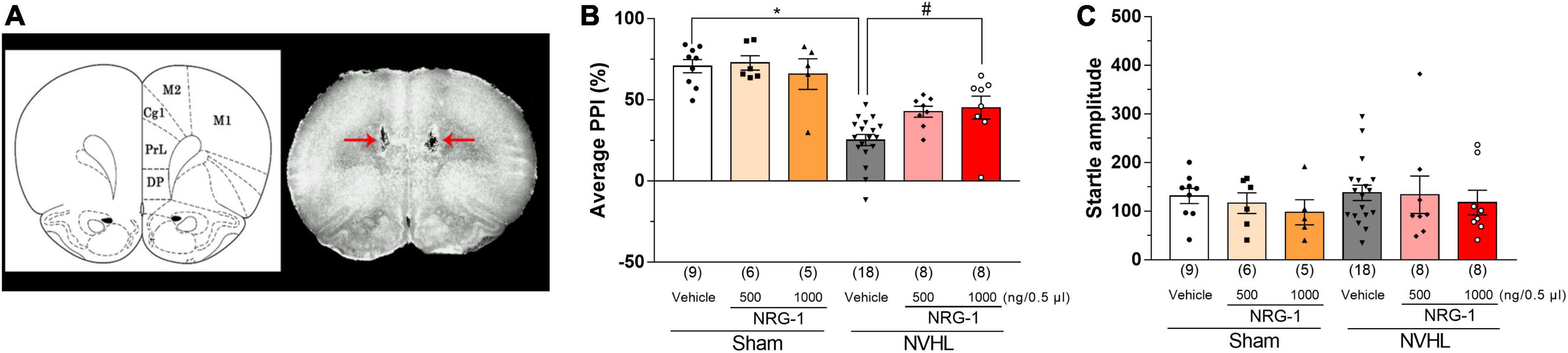
Figure 4. Intra-cortical microinjection of NRG-1 improves NVHL-induced PPI deficits in rats. (A) Photograph of cortical microinjection sites of NRG-1. Schematic drawing of coronal brain sections and photograph of a frontal section of the brain in an animal with microinjection are shown in panel (A). Red arrows indicate the microinjection sites of NRG-1 into the mPFC. Effect of NRG-1 on average PPI (B) and startle amplitude (C) in sham and NVHL rats. Numbers in square brackets indicate the number of animals in each group. Bars represent means ± SEM. *p < 0.05 vs. sham group. #p < 0.05 vs. NVHL group.
Discussion
In the present study, we have demonstrated that post-pubertal NVHL rats exhibit a decrease in the levels of both NRG-1 and p-erbB4 in the PFC. Moreover, we have observed that intra-cortical microinjection of NRG-1 was able to attenuate the PPI deficits in post-pubertal NVHL rats.
NRG-1 expression in the central nervous system has been detected in many regions including the PFC, hippocampus, cerebellum, and substantia nigra, in both humans and rodents (Chaudhury et al., 2003; Law et al., 2004; Bertram et al., 2007). ErbB4 is the only receptor with a high affinity for NRG-1 (Mei and Xiong, 2008), and is mostly located in the GABAergic neurons in the PFC (Bean et al., 2014). Postmortem study has showed that cortical NRG-1 level is decreased in schizophrenia patients (Bertram et al., 2007; Marballi et al., 2012). NRG-1 or erbB4 knock out mice exhibit schizophrenia-like behaviors such as disruption of PPI, latent inhibition and cognitive deficits (Stefansson et al., 2002; Falls, 2003; Hashimoto et al., 2004; Rimer et al., 2005; O’Tuathaigh et al., 2007). From these findings, dysfunction of NRG-1/erbB4 signaling may be associated with development of schizophrenia. In the present study, NVHL rats, which also exhibit schizophrenia-like behaviors (Sams-Dodd et al., 1997; Becker et al., 1999; Mitazaki et al., 2020), showed significant reduction in NRG-1 and p-erbB4 levels in the PFC (Figures 2, 3), suggesting that NRG-1/erbB4 pathway in the PFC of NVHL rats was impaired. Previous study showed that nrg-1 gene in the mPFC was unchanged between sham and NVHL rats (Swerdlow et al., 2013). These paradoxical results may be attributed to different methods of NRG-1 quantification. The brain mapping analyzer is equipped with a photomultiplier tube as a detector and outperforms the quantitative linearity of the image analyzer used in high-sensitivity TV cameras by more than two orders of magnitude and the sensitivity of high-performance liquid chromatography with electrochemical detector by more than three orders of magnitude (Sutoo et al., 1988). Glutamatergic neurotransmission regulates shedding of the ectodomain of the NRG-1 precursor, and releases the activated NRG-1 through activation of glutamate receptors/protein kinase C (PKC)/a disintegrin and metalloproteinase families (Iwakura et al., 2017). Our previous studies showed that NVHL rats show decreased phosphorylation of glutamate receptors and PKC in the PFC (Yabuki et al., 2013, 2019). Thus, we think that the reduction of NRG-1 in the PFC of NVHL rats may be associated with dysfunction of glutamatergic system in the PFC. Previous studies have suggested that NRG-1 levels in the PFC are significantly correlated with a number of genes, including csnk1e (casein kinase 1 epsilon), grid2 (glutamate receptor delta-2), comt (catechol-O-methyltransferase) in the PFC and NGR-1 levels in other brain regions such as the nucleus accumbens and ventral hippocampus (Swerdlow et al., 2012). Therefore, it is possible that reduced NRG-1 in the PFC does not directly contribute to NVHL-induced PPI impairment, but rather may result by affecting the expression of these genes or by affecting the expression of NRG-1 in other brain regions. This possibility is the subject of future research. Administration of NRG-1 has been found to rapidly promote GABA release in the cortex, and modulates functions of glutamatergic neurons (Woo et al., 2007; Vullhorst et al., 2015). Activation of GABAergic system in the PFC has been reported to improve PPI deficits in animal models for schizophrenia (Bortolato et al., 2007; Fejgin et al., 2009). Disruption of PPI is a translational endophenotype of schizophrenia modeling the pre-attentional and sensori-motor impairments (Lipska et al., 1995). A previous study reported that NVHL rats have a reduction in GAD67 gene expression, a GABA neuron marker, in the PFC (Lipska et al., 2003). Thus, we hypothesized that intra-cortical microinjection of NRG-1 may improve NVHL-induced PPI deficits. Our study showed that the microinjection of NRG-1 (1000 ng/0.5 μl) into the PFC (Figure 4A) partially reversed NVHL-induced PPI deficits (Figure 4B). These present results and previous studies suggest that decreased the levels of NRG-1 and p-erbB4 in the PFC may be involved in PPI deficits in post-pubertal NVHL rats, and this mechanism may be associated with GABAergic system. However, we did not assess the changes in GABA release in the PFC of NVHL rats after NRG-1 administration which we will examine in a future study. Moreover, previous study has reported a correlation between lesion size and PPI deficits (Swerdlow et al., 2013). However, we did not perform the quantification of lesion size and assessment of the relationship between lesion size and NRG-1/erbB4 effects, and these questions also need to be addressed in future studies.
Animal models of psychiatric disorders have many limitations and cannot fully reflect the conditions observed in humans (Białoń and Wa̧sik, 2022). The NVHL model is inadequate for assessing the functionality of protein changes observed in schizophrenia patients, and future studies are needed to determine whether similar results can be obtained in transgenic animals.
In summary, this study revealed that alteration of NRG-1/p-erbB4 in PFC may play a role in abnormal behaviors observed in post-pubertal NVHL rats. Based on our data in this putative animal model for schizophrenia-like phenotype, we speculate that at least some of the sensorimotor gating deficits in schizophrenia patients may be due to reduction in NRG-1 in the PFC.
Data availability statement
The original contributions presented in this study are included in the article/supplementary material, further inquiries can be directed to the corresponding author.
Ethics statement
This animal study was reviewed and approved by Ethics Committee of Animal Experiment in Tohoku Medical and Pharmaceutical University.
Author contributions
KW, ON, KT, and LS: writing—review and editing. KW, ON, and KT: conceptualization. S-iK, SM, HO, K-iW, KT-N, and MI: methodology. KW, SM, and HO: formal analysis. KW and KT: visualization. ON: resource. LS, RQ, and TT: supervision. All authors interpreted the results and read and approved the final version of the manuscript.
Funding
This study was supported in part by JSPS KAKENHI (Grant numbers: 20790309 and 21K15351).
Conflict of interest
The authors declare that the research was conducted in the absence of any commercial or financial relationships that could be construed as a potential conflict of interest.
Publisher’s note
All claims expressed in this article are solely those of the authors and do not necessarily represent those of their affiliated organizations, or those of the publisher, the editors and the reviewers. Any product that may be evaluated in this article, or claim that may be made by its manufacturer, is not guaranteed or endorsed by the publisher.
References
Bean, J. C., Lin, T. W., Sathyamurthy, A., Liu, F., Yin, D. M., Xiong, W. C., et al. (2014). Genetic labeling reveals novel cellular targets of schizophrenia susceptibility gene: Distribution of GABA and non-GABA ErbB4-positive cells in adult mouse brain. J. Neurosci. 34, 13549–13566. doi: 10.1523/jneurosci.2021-14.2014
Becker, A., Grecksch, G., Bernstein, H. G., Höllt, V., and Bogerts, B. (1999). Social behaviour in rats lesioned with ibotenic acid in the hippocampus: Quantitative and qualitative analysis. Psychopharmacology 144, 333–338. doi: 10.1007/s002130051015
Bertram, I., Bernstein, H. G., Lendeckel, U., Bukowska, A., Dobrowolny, H., Keilhoff, G., et al. (2007). Immunohistochemical evidence for impaired neuregulin-1 signaling in the prefrontal cortex in schizophrenia and in unipolar depression. Ann. N Y Acad. Sci. 1096, 147–156. doi: 10.1196/annals.1397.080
Białoń, M., and Wa̧sik, A. (2022). Advantages and limitations of animal schizophrenia models. Int. J. Mol. Sci. 23:5968. doi: 10.3390/ijms23115968
Bortolato, M., Frau, R., Orrù, M., Piras, A. P., Fà, M., Tuveri, A., et al. (2007). Activation of GABA(B) receptors reverses spontaneous gating deficits in juvenile DBA/2J mice. Psychopharmacology 194, 361–369. doi: 10.1007/s00213-007-0845-5
Chaudhury, A. R., Gerecke, K. M., Wyss, J. M., Morgan, D. G., Gordon, M. N., and Carroll, S. L. (2003). Neuregulin-1 and erbB4 immunoreactivity is associated with neuritic plaques in Alzheimer disease brain and in a transgenic model of Alzheimer disease. J. Neuropathol. Exp. Neurol. 62, 42–54. doi: 10.1093/jnen/62.1.42
Falls, D. L. (2003). Neuregulins: Functions, forms, and signaling strategies. Exp. Cell Res. 284, 14–30. doi: 10.1016/s0014-4827(02)00102-7
Fejgin, K., Pålsson, E., Wass, C., Finnerty, N., Lowry, J., and Klamer, D. (2009). Prefrontal GABA(B) receptor activation attenuates phencyclidine-induced impairments of prepulse inhibition: Involvement of nitric oxide. Neuropsychopharmacology 34, 1673–1684. doi: 10.1038/npp.2008.225
Flores, G., Barbeau, D., Quirion, R., and Srivastava, L. K. (1996). Decreased binding of dopamine D3 receptors in limbic subregions after neonatal bilateral lesion of rat hippocampus. J. Neurosci. 16, 2020–2026. doi: 10.1523/jneurosci.16-06-02020.1996
Flores, G., Silva-Gómez, A. B., Ibáñez, O., Quirion, R., and Srivastava, L. K. (2005). Comparative behavioral changes in postpubertal rats after neonatal excitotoxic lesions of the ventral hippocampus and the prefrontal cortex. Synapse 56, 147–153. doi: 10.1002/syn.20140
Hahn, C. G., Wang, H. Y., Cho, D. S., Talbot, K., Gur, R. E., Berrettini, W. H., et al. (2006). Altered neuregulin 1-erbB4 signaling contributes to NMDA receptor hypofunction in schizophrenia. Nat. Med. 12, 824–828. doi: 10.1038/nm1418
Hashimoto, R., Straub, R. E., Weickert, C. S., Hyde, T. M., Kleinman, J. E., and Weinberger, D. R. (2004). Expression analysis of neuregulin-1 in the dorsolateral prefrontal cortex in schizophrenia. Mol. Psychiatry 9, 299–307. doi: 10.1038/sj.mp.4001434
Iwakura, Y., Wang, R., Inamura, N., Araki, K., Higashiyama, S., Takei, N., et al. (2017). Glutamate-dependent ectodomain shedding of neuregulin-1 type II precursors in rat forebrain neurons. PLoS One 12:e0174780. doi: 10.1371/journal.pone.0174780
Jalilzad, M., Jafari, A., and Babaei, P. (2019). Neuregulin1beta improves both spatial and associative learning and memory in Alzheimer model of rats possibly through signaling pathways other than Erk1/2. Neuropeptides 78:101963. doi: 10.1016/j.npep.2019.101963
Law, A. J., Shannon Weickert, C., Hyde, T. M., Kleinman, J. E., and Harrison, P. J. (2004). Neuregulin-1 (NRG-1) mRNA and protein in the adult human brain. Neuroscience 127, 125–136. doi: 10.1016/j.neuroscience.2004.04.026
Lipska, B. K., and Weinberger, D. R. (2000). To model a psychiatric disorder in animals: Schizophrenia as a reality test. Neuropsychopharmacology 23, 223–239. doi: 10.1016/s0893-133x(00)00137-8
Lipska, B. K., Lerman, D. N., Khaing, Z. Z., Weickert, C. S., and Weinberger, D. R. (2003). Gene expression in dopamine and GABA systems in an animal model of schizophrenia: Effects of antipsychotic drugs. Eur. J. Neurosci. 18, 391–402. doi: 10.1046/j.1460-9568.2003.02738.x
Lipska, B. K., Swerdlow, N. R., Geyer, M. A., Jaskiw, G. E., Braff, D. L., and Weinberger, D. R. (1995). Neonatal excitotoxic hippocampal damage in rats causes post-pubertal changes in prepulse inhibition of startle and its disruption by apomorphine. Psychopharmacology 122, 35–43. doi: 10.1007/bf02246439
Liu, Y., Ford, B., Mann, M. A., and Fischbach, G. D. (2001). Neuregulins increase alpha7 nicotinic acetylcholine receptors and enhance excitatory synaptic transmission in GABAergic interneurons of the hippocampus. J. Neurosci. 21, 5660–5669. doi: 10.1523/jneurosci.21-15-05660.2001
Marballi, K., Cruz, D., Thompson, P., and Walss-Bass, C. (2012). Differential neuregulin 1 cleavage in the prefrontal cortex and hippocampus in schizophrenia and bipolar disorder: Preliminary findings. PLoS One 7:e36431. doi: 10.1371/journal.pone.0036431
Marcotte, E. R., Pearson, D. M., and Srivastava, L. K. (2001). Animal models of schizophrenia: A critical review. J. Psychiatry Neurosci. 26, 395–410.
Mei, L., and Xiong, W. C. (2008). Neuregulin 1 in neural development, synaptic plasticity and schizophrenia. Nat. Rev. Neurosci. 9, 437–452. doi: 10.1038/nrn2392
Mitazaki, S., Nakagawasai, O., Onogi, H., Watanabe, K., Takahashi, K., Tan-No, K., et al. (2020). Role of prefrontal cortical 5-HT2A receptors and serotonin transporter in the behavioral deficits in post-pubertal rats following neonatal lesion of the ventral hippocampus. Behav. Brain Res. 377:112226. doi: 10.1016/j.bbr.2019.112226
Norton, N., Moskvina, V., Morris, D. W., Bray, N. J., Zammit, S., Williams, N. M., et al. (2006). Evidence that interaction between neuregulin 1 and its receptor erbB4 increases susceptibility to schizophrenia. Am. J. Med. Genet. B Neuropsychiatr. Genet. 141b, 96–101. doi: 10.1002/ajmg.b.30236
O’Tuathaigh, C. M., Babovic, D., O’meara, G., Clifford, J. J., Croke, D. T., and Waddington, J. L. (2007). Susceptibility genes for schizophrenia: Characterisation of mutant mouse models at the level of phenotypic behaviour. Neurosci. Biobehav. Rev. 31, 60–78. doi: 10.1016/j.neubiorev.2006.04.002
Ozaki, M., Sasner, M., Yano, R., Lu, H. S., and Buonanno, A. (1997). Neuregulin-beta induces expression of an NMDA-receptor subunit. Nature 390, 691–694. doi: 10.1038/37795
Paxinos, G., and Franklin, K. B. J. (2013). The rat brain in stereotaxic coordinates. San Diego, CA: Academic Press.
Rieff, H. I., Raetzman, L. T., Sapp, D. W., Yeh, H. H., Siegel, R. E., and Corfas, G. (1999). Neuregulin induces GABA(A) receptor subunit expression and neurite outgrowth in cerebellar granule cells. J. Neurosci. 19, 10757–10766. doi: 10.1523/jneurosci.19-24-10757.1999
Rimer, M., Barrett, D. W., Maldonado, M. A., Vock, V. M., and Gonzalez-Lima, F. (2005). Neuregulin-1 immunoglobulin-like domain mutant mice: Clozapine sensitivity and impaired latent inhibition. Neuroreport 16, 271–275. doi: 10.1097/00001756-200502280-00014
Sams-Dodd, F., Lipska, B. K., and Weinberger, D. R. (1997). Neonatal lesions of the rat ventral hippocampus result in hyperlocomotion and deficits in social behaviour in adulthood. Psychopharmacology 132, 303–310. doi: 10.1007/s002130050349
Silberberg, G., Darvasi, A., Pinkas-Kramarski, R., and Navon, R. (2006). The involvement of ErbB4 with schizophrenia: Association and expression studies. Am. J. Med. Genet. B Neuropsychiatr. Genet. 141b, 142–148. doi: 10.1002/ajmg.b.30275
Stefansson, H., Sigurdsson, E., Steinthorsdottir, V., Bjornsdottir, S., Sigmundsson, T., Ghosh, S., et al. (2002). Neuregulin 1 and susceptibility to schizophrenia. Am. J. Hum. Genet. 71, 877–892. doi: 10.1086/342734
Sutoo, D., Akiyama, K., and Maeda, I. (1988). [The development of a high sensitivity and high linearity fluorescence microphotometry system for distribution analysis of neurotransmitter in the brain]. Nihon Yakurigaku Zasshi 91, 173–180. doi: 10.1254/fpj.91.173
Sutoo, D., Akiyama, K., and Yabe, K. (2001). Quantitative imaging of tyrosine hydroxylase and calmodulin in the human brain. J. Neurosci. Res. 63, 369–376. doi: 10.1002/1097-4547(20010301)63:5<369::Aid-jnr1031<3.0.Co;2-3
Swerdlow, N. R., Powell, S. B., Breier, M. R., Hines, S. R., and Light, G. A. (2013). Coupling of gene expression in medial prefrontal cortex and nucleus accumbens after neonatal ventral hippocampal lesions accompanies deficits in sensorimotor gating and auditory processing in rats. Neuropharmacology 75, 38–46. doi: 10.1016/j.neuropharm.2013.06.003
Swerdlow, N. R., Shilling, P. D., Breier, M., Trim, R. S., Light, G. A., and Marie, R. S. (2012). Fronto-temporal-mesolimbic gene expression and heritable differences in amphetamine-disrupted sensorimotor gating in rats. Psychopharmacology 224, 349–362. doi: 10.1007/s00213-012-2758-1
Takahashi, K., Nakagawasai, O., Nemoto, W., Odaira, T., Sakuma, W., and Tan-No, K. (2018). Antidepressant-like effect of aripiprazole via 5-HT(1A), D(1), and D(2) receptors in the prefrontal cortex of olfactory bulbectomized mice. J. Pharmacol. Sci. 137, 241–247. doi: 10.1016/j.jphs.2018.06.006
Vullhorst, D., Mitchell, R. M., Keating, C., Roychowdhury, S., Karavanova, I., Tao-Cheng, J. H., et al. (2015). A negative feedback loop controls NMDA receptor function in cortical interneurons via neuregulin 2/ErbB4 signalling. Nat. Commun. 6:7222. doi: 10.1038/ncomms8222
Woo, R. S., Li, X. M., Tao, Y., Carpenter-Hyland, E., Huang, Y. Z., Weber, J., et al. (2007). Neuregulin-1 enhances depolarization-induced GABA release. Neuron 54, 599–610. doi: 10.1016/j.neuron.2007.04.009
Yabuki, Y., Nakagawasai, O., Moriguchi, S., Shioda, N., Onogi, H., Tan-No, K., et al. (2013). Decreased CaMKII and PKC activities in specific brain regions are associated with cognitive impairment in neonatal ventral hippocampus-lesioned rats. Neuroscience 234, 103–115. doi: 10.1016/j.neuroscience.2012.12.048
Keywords: neonatal ventral hippocampal lesion, neuregulin-1, erbB4, prefrontal cortex, prepulse inhibition, schizophrenia
Citation: Watanabe K, Nakagawasai O, Kanno S-i, Mitazaki S, Onogi H, Takahashi K, Watanabe K-i, Tan-No K, Ishikawa M, Srivastava LK, Quirion R and Tadano T (2022) Alterations in prefrontal cortical neuregulin-1 levels in post-pubertal rats with neonatal ventral hippocampal lesions. Front. Behav. Neurosci. 16:1008623. doi: 10.3389/fnbeh.2022.1008623
Received: 01 August 2022; Accepted: 07 December 2022;
Published: 22 December 2022.
Edited by:
Patricio Huerta, Feinstein Institutes for Medical Research, United StatesReviewed by:
Hiroki Toyoda, Osaka University, JapanNeal R. Swerdlow, University of California, San Diego, United States
Alain Louilot, INSERM U1114, France
Copyright © 2022 Watanabe, Nakagawasai, Kanno, Mitazaki, Onogi, Takahashi, Watanabe, Tan-No, Ishikawa, Srivastava, Quirion and Tadano. This is an open-access article distributed under the terms of the Creative Commons Attribution License (CC BY). The use, distribution or reproduction in other forums is permitted, provided the original author(s) and the copyright owner(s) are credited and that the original publication in this journal is cited, in accordance with accepted academic practice. No use, distribution or reproduction is permitted which does not comply with these terms.
*Correspondence: Osamu Nakagawasai, ✉ osamun@tohoku-mpu.ac.jp