- Department of Biomedical Sciences, Colorado State University, Fort Collins, CO, United States
The ventral portion of the medial prefrontal cortex (vmPFC) regulates mood, sociability, and context-dependent behaviors. Consequently, altered vmPFC activity has been implicated in the biological basis of emotional disorders. Recent methodological advances have greatly enhanced the ability to investigate how specific prefrontal cell populations regulate mood-related behaviors, as well as the impact of long-term stress on vmPFC function. However, emerging preclinical data identify prominent sexual divergence in vmPFC behavioral regulation and stress responsivity. Notably, the rodent infralimbic cortex (IL), a vmPFC subregion critical for anti-depressant action, shows marked functional divergence between males and females. Accordingly, this review examines IL encoding and modulation of mood-related behaviors, including coping style, reward, and sociability, with a focus on sex-based outcomes. We also review how these processes are impacted by prolonged stress exposure. Collectively, the data suggest that chronic stress has sex-specific effects on IL excitatory/inhibitory balance that may account for sex differences in the prevalence and course of mood disorders.
Introduction
Negative mood states are a feature of numerous psychiatric conditions, including anxiety and depressive disorders. Furthermore, major depression, characterized by sadness, reduced motivation, and anhedonia, is the leading cause of years lived with disability worldwide (Friedrich, 2017). Although females are disproportionally impacted by mood disorders, preclinical studies have historically focused on male neural regulation of depression-related behaviors (Kuehner, 2017). However, recent policy and methodological advances have led to the discovery of significant sex differences in the neurobiology of mood. Here, we examine recent studies exploring sex differences in the prefrontal regulation of coping, reward, and sociability.
Clinical neuroimaging studies associate activity in the ventral medial prefrontal cortex (vmPFC) with depressive disorders, and emotional regulation broadly. The vmPFC is also essential for goal-directed and contextually-appropriate behaviors, mood, and stress responding (Nestler et al., 2002; Krishnan and Nestler, 2008; McKlveen et al., 2015). Further, in studies of males and females vmPFC activity is linked with reward processing and positively correlates with the severity of anhedonia (Keedwell et al., 2005; Green et al., 2019). In particular, the vmPFC subregion Brodmann Area 25 (BA25) has decreased volume in MDD patients across sexes, and is a target for deep brain stimulation in treatment-resistant depression (Drevets et al., 1997, 2008; Crowell et al., 2019; Sankar et al., 2019). A meta-analysis of imaging studies using males and females revealed BA25 is responsive to reward and emotional processing (Beckmann et al., 2009), as well as social exclusion (Vijayakumar et al., 2017). Though these studies indicate BA25 activity associates with depressive disorders, results examining BA25 function in depression are varied. Mixed sex studies have reported both reduced metabolic activity in MDD patients (Drevets et al., 1997), as well as hyperactivity in treatment-resistant depression measured by cerebral blood flow (Mayberg et al., 2005). Yet, both conventional antidepressant treatment and deep brain stimulation reduce BA25 activity (Mayberg et al., 2005, 2013). The heterogeneity of neural populations in BA25 may contribute to these divergent results. BA25 is principally composed of excitatory pyramidal neurons that project throughout limbic and brainstem nuclei, with a smaller but diverse population of inhibitory interneurons (Beckmann et al., 2009). Mounting evidence indicates that changes to the excitation/inhibition balance of the vmPFC relate to depressive symptomology, but the contributions of specific neural populations to behavior are difficult to address clinically (Fogaça and Duman, 2019; McKlveen et al., 2019). Further, although clinical studies commonly include both males and females, few analyze outcomes for sex differences (Vijayakumar et al., 2017). However, recent advances in neurobiology have allowed studies to establish casual roles for specific genetically-defined cell populations for processing and regulating behavior across sexes.
The infralimbic cortex (IL) is the rodent anatomical homolog of primate BA25 and is well-positioned for behavioral regulation based on projections throughout the limbic system (Vertes, 2004; Wood et al., 2018). The IL contains glutamatergic projection neurons with inhibitory interneurons providing local network regulation (McKlveen et al., 2015, 2016; Wood et al., 2018). While pharmacological and lesion studies have linked IL activity with depression-relevant behaviors, advances in cell-type specificity have identified sex-dependent roles in stress, reward, and social processes.
Negative Valence: Stress Coping
Coping with negatively-valenced stimuli involves coordinated behavioral and physiological responses to address real or perceived stressors. Ultimately, stress exposure initiates a neurohormonal cascade that leads to the synthesis of adrenal glucocorticoids that then provide feedback to the brain at glucocorticoid receptors (GR) and mineralocorticoid receptors (MR) to promote behavioral and physiological adaptation (McKlveen et al., 2013; Myers et al., 2014; Herman et al., 2016). An increasing number of studies have identified a role for the IL in stress coping (Table 1), with similarities and differences between male and female rodents.
The IL is acutely stress-responsive, as identified by histological markers of neuronal activation, and expresses both GR and MR in multiple cell types (Granholm et al., 1985; Reul and De Kloet, 1986; Cintra et al., 1994; Cullinan et al., 1995; McKlveen et al., 2013, 2016, 2019). Knockdown of male IL GR expression increases passive coping in the forced swim test (FST) and glucocorticoid responses to acute stress (McKlveen et al., 2013). However, pharmacological manipulations of male IL activity have yielded mixed results on coping behaviors. For instance, IL inactivation via GABAA receptor activation reduces FST immobility in males, an antidepressant-associated phenotype (Slattery et al., 2011). Similarly, non-specific synaptic blockade in the male IL reduces passive coping in the FST (Scopinho et al., 2010), as does long-term knockdown of IL glutamatergic output in males (Pace et al., 2020). However, both the NMDA antagonist ketamine and the muscarinic antagonist scopolamine increase IL activity and reduce male FST immobility (Fuchikami et al., 2015; Navarria et al., 2015). Further, deep brain stimulation in male rodents reduces passive coping in the FST (Hamani et al., 2010) and increases open arm time in the elevated plus maze (EPM), an anxiolytic-like phenotype (Shimizu et al., 2018). The mixed outcome of pharmacological interventions highlights the need to determine endogenous neural activity patterns during behavior, as well as more temporally- and genetically-specific modulation of neural activity.
In vivo recordings indicate the male IL processes anxiogenic stimuli and acute stressors. IL neural activity, measured by multiunit electrodes, increases in the seconds preceding entry into the open arms of the EPM (Shimizu et al., 2018). More generally, multiunit electrode array recordings of male mPFC neurons at the border between the IL and prelimbic (PL) area have variable activity in response to FST. Although many neurons are inhibited during FST, a large portion have selectivity for immobile vs. mobile periods and the majority of those increase activity during mobile periods (Warden et al., 2012). The heterogeneity of cellular responses underscores the need to examine the contributions of specific IL neural populations. Advances in optogenetics have permitted temporally-precise and cell-type specific modulation of IL activity. Activation of male IL glutamatergic neurons 24 h before testing reduces FST passive coping (Fuchikami et al., 2015), suggesting IL stimulation induces pyramidal neuron plasticity. Although, it remains to be determined how this stimulation may regulate IL efferent activity. Optogenetic modulation also permits synaptic stimulation, which indicates output targets differentially influence behavioral outcomes. For instance, Warden et al. stimulated male mPFC (IL and PL) glutamatergic neurons without affecting FST behavior. However, evoked glutamate release from mPFC terminals in the dorsal raphe increased active coping, while projections to the lateral habenula decreased active coping (Warden et al., 2012). Taken together, these results indicate differing behavioral outcomes from modulating male IL activity, likely relating to the cellular specificity of interventions and/or the differential engagement of output targets.
In line with regulating coping behavior, the IL also mediates physiological responses to acute challenges. Viral-mediated knockdown of male pyramidal neuron vesicular glutamate transporter 1 (vGluT1) reduces glutamate release and increases hypothalamic-pituitary-adrenal (HPA) axis, heart rate, and blood pressure responses to restraint (Myers et al., 2017; Schaeuble et al., 2019). Similarly, optogenetic stimulation of male IL glutamatergic neurons reduces both corticosterone and glucose responses to restraint stress, as well as heart rate and blood pressure responses to a novel environment. In contrast, optogenetic stimulation of female IL glutamate neurons increases glucose responses to restraint and heart rate reactivity to a novel environment (Wallace et al., 2021). Collectively, these data suggest that male IL glutamatergic neurons are both necessary and sufficient to reduce autonomic and neuroendocrine responses to stress, while female IL glutamate neurons facilitate stress reactivity. It remains to be determined what mechanisms account for sex differences in IL function. While IL c-Fos expression following FST is similar in males and females, males have greater activation following acute restraint, suggesting differences in stress reactivity may be stimuli-specific (Sood et al., 2018). Further, ovarian hormones may be involved as lateral ventricle infusion of corticotrophin releasing hormone leads to negative correlations between IL c-Fos expression and grooming behavior in both male and diestrus female rats. However, IL activity positively associates with grooming in proestrus females (Wiersielis et al., 2016). Overall IL neural populations signal distinct aspects of stressors, while male IL glutamatergic neural activity constrains the physiological stress response and bidirectionally regulates coping style depending upon projection sites. In contrast, female IL glutamatergic neural activity facilitates the physiological stress response and divergent IL responses to stressors may relate to ovarian hormone signaling.
Positive Valence: Reward
The IL has a prominent role in coordinating context-appropriate reward-seeking behaviors (Table 2). Pharmacological inhibition of the male rat IL with combined GABAA and GABAB agonists reduces inhibitory control in a food reward-seeking task (Capuzzo and Floresco, 2020), as well as extinction and renewal of context-conditioned food reward (Eddy et al., 2016). Moorman and Aston-Jones (2015) used a similar pharmacological approach and found that IL inhibition reduces both lever presses to a reward-associated stimulus and extinction of reward-seeking after the stimulus is no longer paired with reward. Furthermore, male IL multiunit potential recordings found that putative pyramidal neurons heterogeneously respond (significantly increase or decrease activity) to cue-evoked reward-seeking and extinction (Moorman and Aston-Jones, 2015). Additionally, male IL neurons have prolonged firing in response to rewarded but not unrewarded operant responses and IL inhibition increases the latency to reward acquisition (Burgos-Robles et al., 2013). IL pyramidal neuron regulation of midbrain dopamine signaling may be important for effects on reward and motivation. Ferenczi et al. utilized a stable-step function opsin (SSFO) to optogentically increase male IL glutamate neuron excitability and found reduced sucrose preference. In females, the SSFO approach also reduces the rewarding quality of ventral tegmental area stimulation in a real-time place preference assay (Ferenczi et al., 2016), suggesting IL inhibition of dopamine signaling may contribute to anhedonia. In contrast, SSFO-induced increases in the excitability of IL GABAergic/vasoactive intestinal peptide (VIP)-expressing interneurons reduces high-calorie palatable food consumption without impacting food reward motivation or low-calorie chow intake (Newmyer et al., 2019). Overall, these studies indicate that male IL neurons signal multiple aspects of food reward acquisition, including contextually-appropriate reward-seeking and behavioral inhibition. Although, specific IL cell populations likely have opposing effects on hedonic feeding.
Fewer publications have investigated the role of the female rodent IL in reward seeking and motivational behaviors. To date, evidence suggests that the female IL may have more limited involvement in reward processing and positive affect. For instance, optogenetic activation of the glutamatergic IL to nucleus accumbens shell (NAcSh) pathway following conditioned taste aversion reduces aversive taste reactivity in males but not females. However, both sexes lever press for IL-NAcSh stimulation and a prior history of IL-NAcSh stimulation increases sucrose preference in males and females (Hurley and Carelli, 2020). Further, optogenetic stimulation of IL glutamatergic neurons induces a real-time place preference in males without affecting place preference or aversion in females, suggesting a positive valence to IL glutamatergic activity in males but not females (Wallace et al., 2021). These studies collectively indicate that, in males, IL activity is necessary for contextual appraisal during reward acquisition and that glutamatergic activity has positive valence. In contrast, current evidence suggests that female IL glutamatergic activity does not affect place preference or conditioned aversion, although activity in specific projections may be rewarding.
Social Behavior
Reduced sociability is a common symptom of mood disorders. Additionally, decreased motivation for social interaction further worsens the course of depressive illness (Kupferberg et al., 2016). Consequently, determining how neural circuits encode and regulate social behavior is a critical area for investigation. Growing evidence indicates that male IL neural output regulates the affective and motivational processes that underly social interaction (Table 3), possibly through descending limbic integration (Vertes, 2004; Wood et al., 2018). Indeed, pharmacological inactivation of the IL reduces both the frequency and duration of social play in adolescent male rats (Van Kerkhof et al., 2013). Furthermore, Minami et al. (2017) conducted electrical recordings of IL activity during social behavior and found that male IL neurons increase firing during the termination of social behavior, an effect absent in isolation-reared rats suggesting experience-dependent social encoding. SSFO enhancement of male IL glutamatergic neuron excitability reduces social interaction with a juvenile interactor (Ferenczi et al., 2016). In contrast, acute optogenetic stimulation of male IL glutamate neurons increases conspecific social motivation (Wallace et al., 2021), providing further evidence for contextual factors impacting IL-mediated behaviors. Increasing the excitability of male IL GABAergic VIP interneurons reduces novel social investigation, as well as novel object interactions (Newmyer et al., 2019). Other interneuronal investigations examined GABAergic parvalbumin (PV) neurons in the male and female mouse mPFC and found that PV neural activity increases in both sexes during social interactions compared to novel object interactions, a phenotype missing in the CNTNAP2 knockout autism model. Further, SSFO-increased excitability of PV interneurons rescues social deficits in the CNTNAP2 model, without impacting sociability in wildtype mice (Selimbeyoglu et al., 2017). Ultimately, these studies highlight the need to further investigate how specific interneuronal populations within the mPFC differentially encode and modulate social behavior.
Currently, evidence suggests the female IL may have a different role in social behavior. Optogenetic stimulation of IL glutamatergic neurons does not alter female social motivation or social novelty prefrence (Wallace et al., 2021). Further, the female IL appears to be less responsive to social interaction with conspecifics as female rats have less c-Fos expression compared to males after social interaction (Mikosz et al., 2015). Moreover, male rats have greater c-Fos responses to a previously-stressed interactor than an unstressed conspecific, an effect that does not occur in females. This sex difference may be independent of ovarian hormones as both intact cycling females and ovariectomized (OVX) females have similar IL c-Fos following social interaction (Mikosz et al., 2015). However, specific projection-defined IL neurons are necessary for social motivation. Huang et al. (2020) chemogenetically inactivated female basolateral amygdala-projecting IL neurons and abolished social preference in a 3-chamber social test. Overall, the data suggest that the male IL is more responsive to conspecific social interaction and that increasing male IL glutamatergic activity can bidirectionally modulate social motivation, dependent upon the method of stimulation, while interneuron stimulation produces opposing effects. Additionally, the female IL has less neural activity than males after conspecific interaction and stimulation of female IL glutamate neurons does not alter sociability. Although, amygdala-projecting female IL neurons are necessary for social preference.
While the female IL may be less involved in conspecific interaction, Pereira and Morrell (2020) demonstrated that the IL plays a critical role in maternal behaviors. Using a conditioned place preference paradigm, new mother rats spend equivalent time in chambers associated with cocaine reward and pups. However, blockade of sodium conductance in the female IL leads to an exclusive preference for the cocaine-paired chamber. Furthermore, IL inactivation reduces maternal behaviors including nest building and retrievals. In fact, none of the IL-inactivated mothers fully retrieved all pups, while all vehicle-treated females did (Pereira and Morrell, 2020). Further, histological evidence indicates that the female IL shows greater activation following exposure to newborns than juvenile pups (Pose et al., 2019). Thus, the female IL may be more tuned to facilitate pup rearing than conspecific social interactions.
Gonadal Hormone Influences
The impact of gonadal hormones on neural activity may contribute to sexually divergent IL function. Gonadal hormones influence circuit regulation through both organizational effects in development as well as activational effects in adulthood. The importance of gonadal hormones for mPFC development, synapse formation, and pruning has been recently reviewed (Premachandran et al., 2020; Delevich et al., 2021). In adult rodents, estrogen receptors α and β (ERα and ERβ) are present in the male rodent mPFC (Figure 1), distributed broadly across cortical layers in both pyramidal and non-pyramidal putative interneurons (Montague et al., 2008). Further, ultrastructural analysis found ERα, ERβ, and G protein-coupled estrogen receptor 1 (GPER1) in the female mPFC. Interestingly, GPER1 is expressed at over twice the levels of ERα and ERβ, suggesting a significant fast-acting component to ER signaling (Almey et al., 2014). Moreover, ER localization is largely extranuclear, with most receptors located axonally (Almey et al., 2014). Growing evidence suggests that ER signaling plays an important role in mPFC regulation of female behavior. For instance, 17β-estradiol (E2) localized to the female PL/IL junction shifts the cognitive strategy used for maze navigation (Almey et al., 2014). Further, ERα and ERβ agonist treatment in diestrus females potentiates the antidepressant-like effect of ketamine (Dossat et al., 2018). Recent evidence from OVX females also indicates that E2 increases the excitability of IL pyramidal neurons in slice and enhances extinction of reward-seeking (Yousuf et al., 2019). While more research is needed to determine the mechanisms by which estrogens regulate prefrontal function, these studies suggest that cyclic fluctuations in intrinsic network activity impact depression-related behaviors. Considerably less is known about the effects of cortical progesterone signaling. The female rodent frontal cortex expresses both progesterone receptor a (PRa) and b (PRb), with PRb levels decreasing during estrus (Guerra-Araiza et al., 2003). Although there are no reports, to our knowledge, of PR expression in males, repeated progesterone administration increases GABAA receptor subunit α1 expression in the mPFC of both sexes (Andrade et al., 2012). Thus, cyclic increases in progesterone likely affect mPFC E/I balance.
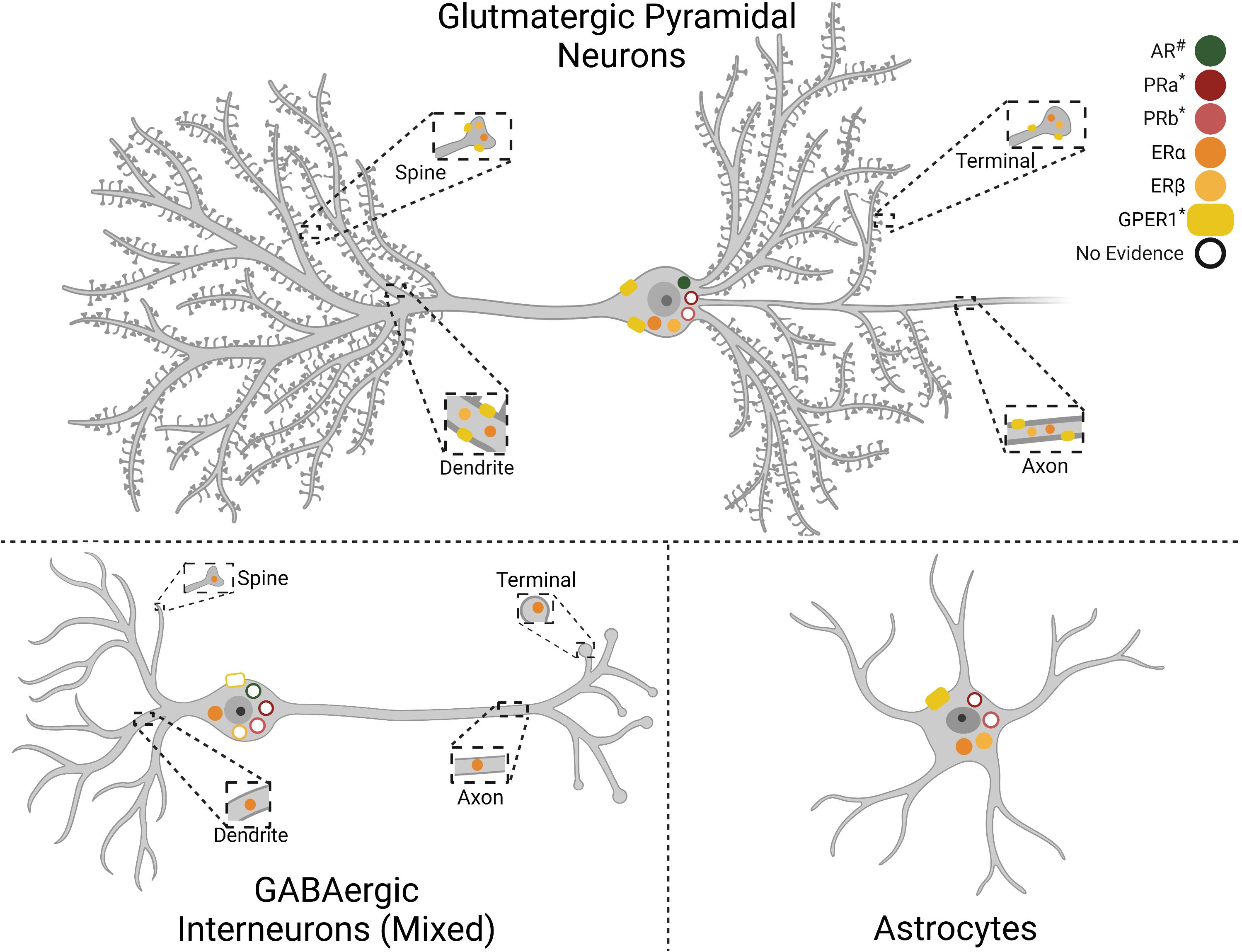
Figure 1. Gonadal hormone receptor expression in infralimbic cortex (IL) cell types. Depiction of gonadal hormone receptor expression and localization. Filled symbols indicate evidence for expression in the cell type and cellular compartment. Empty symbols denote lack of information as to the presence or absence of the receptor. Progesterone receptor cell-type specifity has not been reported. Androgen Receptor (AR), Progesterone Receptor A (PRa), Progesterone Receptor B (PRb), Estrogen Receptor α (ERα), Estrogen Receptor β (ERβ), G protein-coupled estrogen receptor 1 (GPER1). #AR expression is higher in males than females. *PRa, PRb, and GPER1 expression has only been reported in females. Created with BioRender.com.
Androgens may also play a role in IL functional differences as androgen receptors (AR) are expressed in the frontal cortex of male and female rodents (DonCarlos et al., 2006). Expression is higher in males than females and current evidence indicates little to no astrocyte expression, though this could be age-dependent (Feng et al., 2010). Further, AR expression in midbrain-projecting neurons suggests putative pyramidal expression (Aubele and Kritzer, 2012; Low et al., 2017). In addition, androgens regulate dopamine (DA) inputs to the male rodent mPFC. Orchiectomy increases DA axonal density and extracellular DA levels within the mPFC, an effect reversed by testosterone administration (Kritzer, 2003; Aubele and Kritzer, 2012). Further, a large portion of ventral tegmental area-projecting IL neurons express AR. Taken together, these results suggest a bidirectional interaction between androgen signaling and mesocortical DA circuitry that may influence IL network excitability as well as mood and behavior. Further evidence for gonadal hormone regulation of mPFC activity comes from studies indicating that androgens and estrogens have opposing effects on mPFC metabolism of DA, norepinephrine, and serotonin during a novel environment stressor (Handa et al., 1997). Collectively, this evidence suggests gonadal hormones modify IL function. Due to the widespread expression of these receptors in mPFC cell populations and varied actions on neural activity, considerable work remains to understand how hormonal fluctuations across the lifespan impact prefrontal network function.
Chronic Stress Impacts
The two greatest predictors of depressive outcomes are cumulative lifetime traumas and severe life stressors (Cassileth et al., 1984), indicating that the neural consequences of repeated or severe stress dictate disease burden. Mood-related symptoms, including negative affect, anhedonia, despair, and social withdrawal, are also frequently initiated and/or exacerbated by prolonged stress (Kennedy and Adolphs, 2012; Lupien et al., 2009). Accordingly, chronic stress exposure has been a primary preclinical paradigm for studying depression and mood disorders in animal models. In recent years, there has been growing interest in the sexual basis of chronic stress impacts on limbic structures. Multiple excellent reviews have covered the topic in-depth (McLaughlin et al., 2009; Bourke et al., 2012; Shansky and Woolley, 2016; Shors, 2016; Shepard and Coutellier, 2018; Fogaça and Duman, 2019; Moench et al., 2019; Page and Coutellier, 2019). Here, we review IL-specific effects.
Chronic stress-induced IL pyramidal neuron dendritic hypotrophy has been consistently reported in male rodents, though this varies based on projection targets (Cerqueira et al., 2005; Goldwater et al., 2009; Shansky et al., 2009; Luczynski et al., 2015; Czéh et al., 2018). Further, measurement of the long-term activation marker ΔFosB indicates the male IL is responsive to chronic stress exposure, an effect not present in other frontal regions such as the anterior cingulate or orbital cortices (Flak et al., 2012; Pace et al., 2020). However, studies of chronic stress effects on male IL glutamatergic excitability have yielded mixed results, contributing to opposing hypothesis of either hyper- or hypo-inhibition. McKlveen et al. (2016) found that IL pyramidal neurons of male rats exposed to a 2-week variable stress paradigm had increased inhibitory currents and more GABAergic synaptic appositions, suggesting increased inhibition of IL glutamatergic neurons. GR was also reduced specifically in PV interneurons (McKlveen et al., 2016), pointing to the importance of glucocorticoid feedback for regulating local inhibition. In support of hyper-inhibition, chemogenetic inhibition of male mouse IL PV interneurons during CVS reduces passive coping in FST (Nawreen et al., 2020). Furthermore, chronic stress increases GAD67 mRNA in the male mouse IL (Shepard et al., 2016). Additionally, 3 weeks of daily restraint stress in male mice increases dendritic arborization of GAD67-positive interneurons but reduces GAD67-positive somas (Gilabert-Juan et al., 2013). In support of hypo-inhibition, Czéh et al. (2018) found reductions in both IL interneuron populations and inhibitory currents in an anhedonic subpopulation of male rats exposed to 9 weeks of variable stress (Czéh et al., 2018). Similarly, 3 weeks of chronic unpredictable stress reduces GAD67 mRNA in male rats, although vGluT1 mRNA is also reduced (Ghosal et al., 2020). Thus, there are data to support both increased and decreased inhibition of male IL pyramidal neurons after chronic stress. Multiple factors including differences in methodology, stress paradigms, and temporal factors may contribute to the discrepant findings. Further, how these post-mortem changes affect neural network activity and behavioral outcomes remains to be determined. In vivo, electrophysiology studies indicate male IL neurons increase firing during a shock-predicting cue; however, this effect is not present after repeated stress (Wilber et al., 2011). Taken together, chronic stress reduces male IL glutamatergic dendritic complexity and spine density, yet the chronic stress effects on inhibitory neural populations are mixed and have yet to reach a consensus.
Numerous female studies suggest that estrogen may be protective against chronic stress effects on IL function. Though not specific to the IL, Wei et al. (2014) found that repeated restraint stress reduced mPFC miniature excitatory postsynaptic current (mEPSC) amplitude and frequency in males but not females. The decreased excitability was accompanied by a male-specific reduction in glutamate receptor surface proteins. However, ER antagonism in females unmasked stress effects on mEPSC frequency and glutamate receptors, suggesting ER prevents excitatory hypofunction following stress. Intriguingly, estrogen delivery in males is sufficient to prevent stress effects on mEPSC amplitude and frequency, as well as partially restore glutamate receptor surface protein expression (Wei et al., 2014). Moreover, female IL neurons generally do not show stress-induced dendritic remodeling. However, female IL pyramidal neurons projecting to the basolateral amygdala have estrogen-dependent increases in dendritic branching after repeated restraint stress (Shansky et al., 2010). Further, repeated stress increases spine density in this projection regardless of estrogen treatment (Shansky et al., 2010). Others have reported sex-specific effects of chronic variable stress based on IL projection target. Here, chronically-stressed female mice have increased EPSCs in the IL-NAc projection, while males have greater loss of dendritic complexity in VTA-projecting IL neurons. Additionally, chemogenetic inhibition of NAc-projecting IL neurons rescues chronic stress-induced behaviors only in females (Bittar et al., 2021). In contrast to pyramidal cells, PV interneurons appear to be more stress susceptible in females than males. Female IL PV mRNA increases following 2 weeks of daily stress exposure, with a further increase at 4 weeks. Females at 4 weeks also have increased PV neuron density and reduced IL c-Fos expression following open-field, effects that are absent in males (Shepard et al., 2016). However, both male and female mice have increased c-Fos in PV cells (Page et al., 2019), indicating increased interneuron activity. Although, chemogenetic activation of IL PV interneurons induces anxiety-like behavior only in females. Overall, these results indicate that estrogen is protective for female IL glutamatergic neurons, sex differences in chronic stress effects are projection-dependent, and interneuron populations are more susceptible to chronic stress in females.
Conclusion
The increased attention on females in preclinical research and the rapid development of neurobiological techniques with enhanced genetic and temporal specificity have isolated sex-specific regulatory roles of IL neural populations. Manipulations that induce long-term changes in pyramidal E/I balance (SSFO-mediated hyperexcitability or lentiviral knockdown of glutamate release) can lead to divergent and sometimes contradictory behavioral outcomes. However, acutely increasing activity of IL glutamate neurons regulates numerous stress coping, motivational, and social behaviors in male rodents. The aggregate data suggest these cells promote active coping, context-appropriate reward-seeking, motivation, and sociability. Although, specific projections may have differing or even opposing actions. Less work has examined stress coping in females, but IL glutamate neurons have sexually divergent effects on physiological stress responses. Female IL pyramidal neurons may also play a smaller role in reward-seeking and motivational behaviors. In terms of sociability, the female IL seems less involved in conspecific interactions, with significant involvement in maternal behaviors. Many of these differences may be mediated by gonadal hormone signaling in different components of the IL neural and glial network. Generally, estrogens seem to protect glutamate neurons from the effects of chronic stress while androgens modulate cortical dopamine function. The sex-specific functions of the numerous IL interneuron subtypes remain to be determined. Further, the effects of chronic stress on IL cellular excitability are mixed for both sexes. Ultimately, complex interactions between sex and stress impact many aspects of vmPFC local networks and, consequently, brain-wide synaptic signaling. Determining the mechanistic basis of E/I balance in these cell groups is likely to significantly push forward our understanding of mood disorders and identify sex-specific treatment options to improve health outcomes.
Author Contributions
Both authors contributed to the conceptualization of the review. TW performed the initial literature search, wrote the first draft of the manuscript, and created the illustrations in BioRender. BM contributed additional literature and revised the manuscript. Both authors approved the submitted version.
Funding
This work was supported by NIH R01 HL150559 to BM.
Conflict of Interest
The authors declare that the research was conducted in the absence of any commercial or financial relationships that could be construed as a potential conflict of interest.
Publisher’s Note
All claims expressed in this article are solely those of the authors and do not necessarily represent those of their affiliated organizations, or those of the publisher, the editors and the reviewers. Any product that may be evaluated in this article, or claim that may be made by its manufacturer, is not guaranteed or endorsed by the publisher.
References
Almey, A., Cannell, E., Bertram, K., Filardo, E., Milner, T. A., and Brake, W. G. (2014). Medial prefrontal cortical estradiol rapidly alters memory system bias in female rats: ultrastructural analysis reveals membrane-associated estrogen receptors as potential mediators. Endocrinology 155, 4422–4432. doi: 10.1210/en.2014-1463
Andrade, S., Arbo, B. D., Batista, B. A. M., Neves, A. M., Branchini, G., Brum, I. S., et al. (2012). Effect of progesterone on the expression of GABA A receptor subunits in the prefrontal cortex of rats: implications of sex differences and brain hemisphere. Cell Biochem. Funct. 30, 696–700. doi: 10.1002/cbf.2854
Aubele, T., and Kritzer, M. F. (2012). Androgen influence on prefrontal dopamine systems in adult male rats: localization of cognate intracellular receptors in medial prefrontal projections to the ventral tegmental area and effects of gonadectomy and hormone replacement on glutamate-stimulated extracellular dopamine level. Cereb. Cortex 22, 1799–1812. doi: 10.1093/cercor/bhr258
Beckmann, M., Johansen-Berg, H., and Rushworth, M. F. S. (2009). Connectivity-based parcellation of human cingulate cortex and its relation to functional specialization. J. Neurosci. 29, 1175–1190. doi: 10.1523/JNEUROSCI.3328-08.2009
Bittar, T. P., Pelaez, M. C., Hernandez Silva, J. C., Quessy, F., Lavigne, A. A., Morency, D., et al. (2021). Chronic stress induces sex-specific functional and morphological alterations in corticoaccumbal and corticotegmental pathways. Biol. Psychiatry 90:14. doi: 10.1016/j.biopsych.2021.02.014
Bourke, C. H., Harrell, C. S., and Neigh, G. N. (2012). Stress-induced sex differences: adaptations mediated by the glucocorticoid receptor. Horm. Behav. 62, 210–218. doi: 10.1016/j.yhbeh.2012.02.024
Burgos-Robles, A., Bravo-Rivera, H., and Quirk, G. J. (2013). Prelimbic and infralimbic neurons signal distinct aspects of appetitive instrumental behavior. PLoS One 8:57575. doi: 10.1371/journal.pone.0057575
Capuzzo, G., and Floresco, S. B. (2020). Prelimbic and infralimbic prefrontal regulation of active and inhibitory avoidance and reward-seeking. J. Neurosci. 40, 4773–4787. doi: 10.1523/JNEUROSCI.0414-20.2020
Cassileth, B. R., Lusk, E. J., Strouse, T. B., Miller, D. S., Brown, L. L., Cross, P. A., et al. (1984). Psychosocial status in chronic illness. A comparative analysis of six diagnostic groups. N. Engl. J. Med. 311, 506–511. doi: 10.1056/NEJM198408233110805
Cerqueira, J. J., Pêgo, J. M., Taipa, R., Bessa, J. M., Almeida, O. F. X., and Sousa, N. (2005). Morphological correlates of corticosteroid-induced changes in prefrontal cortex-dependent behaviors. J. Neurosci. 25, 7792–7800. doi: 10.1523/JNEUROSCI.1598-05.2005
Cintra, A., Zoli, M., Rosén, L., Agnati, L. F., Okret, S., Wikström, A. C., et al. (1994). Mapping and computer assisted morphometry and microdensitometry of glucocorticoid receptor immunoreactive neurons and glial cells in the rat central nervous system. Neuroscience 62, 843–897. doi: 10.1016/0306-4522(94)90481-2
Crowell, A. L., Riva-Posse, P., Holtzheimer, P. E., Garlow, S. J., Kelley, M. E., Gross, R. E., et al. (2019). Long-term outcomes of subcallosal cingulate deep brain stimulation for treatment-resistant depression. Am. J. Psychiatry 176, 949–956. doi: 10.1176/appi.ajp.2019.18121427
Cullinan, W. E., Herman, J. P., Battaglia, D. F., Akilt, H., and Watsont, S. J. (1995). Pattern and time course of immediate early gene expression in rat brain following acute stress. Neuroscience 64, 477–505.
Czéh, B., Vardya, I., Varga, Z., Febbraro, F., Csabai, D., Martis, L. S., et al. (2018). Long-term stress disrupts the structural and functional integrity of GABAergic neuronal networks in the medial prefrontal cortex of rats. Front. Cell. Neurosci. 12:148. doi: 10.3389/fncel.2018.00148
Delevich, K., Klinger, M., Okada, N. J., and Wilbrecht, L. (2021). Coming of age in the frontal cortex: the role of puberty in cortical maturation. Semin. Cell Dev. Biol. doi: 10.1016/J.SEMCDB.2021.04.021 [Epub ahead of print].
DonCarlos, L. L., Sarkey, S., Lorenz, B., Azcoitia, I., Garcia-Ovejero, D., Huppenbauer, C., et al. (2006). Novel cellular phenotypes and subcellular sites for androgen action in the forebrain. Neuroscience 138, 801–807. doi: 10.1016/j.neuroscience.2005.06.020
Dossat, A. M., Wright, K. N., Strong, C. E., and Kabbaj, M. (2018). Behavioral and biochemical sensitivity to low doses of ketamine: influence of estrous cycle in C57BL/6 mice. Neuropharmacology 130, 30–41. doi: 10.1016/j.neuropharm.2017.11.022
Drevets, W. C., Price, J. L., Simpson, J. R., Todd, R. D., Reich, T., Vannier, M., et al. (1997). Subgenual prefrontal cortex abnormalities in mood disorders. Nature 386, 824–827. doi: 10.1038/386824a0
Drevets, W. C., Savitz, J., and Trimble, M. (2008). The subgenual anterior cingulate cortex in mood disorders. CNS Spectr. 13, 663–681. doi: 10.1017/S1092852900013754
Eddy, M. C., Todd, T. P., Bouton, M. E., and Green, J. T. (2016). Medial prefrontal cortex involvement in the expression of extinction and ABA renewal of instrumental behavior for a food reinforcer. Neurobiol. Learn Mem. 128, 33-39. doi: 10.1016/j.nlm.2015.12.003
Feng, Y., Weijdegård, B., Wang, T., Egecioglu, E., Fernandez-Rodriguez, J., Huhtaniemi, I., et al. (2010). Spatiotemporal expression of androgen receptors in the female rat brain during the oestrous cycle and the impact of exogenous androgen administration: a comparison with gonadally intact males. Mol. Cell. Endocrinol. 321, 161–174. doi: 10.1016/j.mce.2010.02.029
Ferenczi, E. A., Zalocusky, K. A., Liston, C., Grosenick, L., Warden, M. R., Amatya, D., et al. (2016). Prefrontal cortical regulation of brainwide circuit dynamics and reward-related behavior. Science 351:aac9698. doi: 10.1126/science.aac9698
Flak, J. N., Solomon, M. B., Jankord, R., Krause, E. G., and Herman, J. P. (2012). Identification of chronic stress-activated regions reveals a potential recruited circuit in rat brain. Eur. J. Neurosci. 36, 2547–2555. doi: 10.1111/j.1460-9568.2012.08161.x
Fogaça, M. V., and Duman, R. S. (2019). Cortical GABAergic dysfunction in stress and depression: new insights for therapeutic interventions. Front. Cell. Neurosci. 13:87. doi: 10.3389/fncel.2019.00087
Friedrich, M. J. (2017). Depression is the leading cause of disability around the world. JAMA 317:1517. doi: 10.1001/jama.2017.3826
Fuchikami, M., Thomas, A., Liu, R., Wohleb, E. S., Land, B. B., DiLeone, R. J., et al. (2015). Optogenetic stimulation of infralimbic PFC reproduces ketamine’s rapid and sustained antidepressant actions. Proc. Natl. Acad. Sci. U.S.A. 112, 8106–8111. doi: 10.1073/pnas.1414728112
Ghosal, S., Duman, C. H., Liu, R. J., Wu, M., Terwilliger, R., Girgenti, M. J., et al. (2020). Ketamine rapidly reverses stress-induced impairments in GABAergic transmission in the prefrontal cortex in male rodents. Neurobiol. Dis. 134:104669. doi: 10.1016/j.nbd.2019.104669
Gilabert-Juan, J., Castillo-Gomez, E., Guirado, R., Moltó, M. D., and Nacher, J. (2013). Chronic stress alters inhibitory networks in the medial prefrontal cortex of adult mice. Brain Struct. Funct. 218, 1591–1605. doi: 10.1007/s00429-012-0479-1
Goldwater, D. S., Pavlides, C., Hunter, R. G., Bloss, E. B., Hof, P. R., McEwen, B. S., et al. (2009). Structural and functional alterations to rat medial prefrontal cortex following chronic restraint stress and recovery. Neuroscience 164, 798–808. doi: 10.1016/j.neuroscience.2009.08.053
Granholm, L., Wikström, A. C., Wikström, A. C., Okret, S., Gustafsson, J. Å, Agnati, L. F., et al. (1985). Mapping of glucocorticoid receptor immunoreactive neurons in the rat tel- and diencephalon using a monoclonal antibody against rat liver glucocorticoid receptor. Endocrinology 117, 1803–1812. doi: 10.1210/endo-117-5-1803
Green, I. W., Pizzagalli, D. A., Admon, R., and Kumar, P. (2019). Anhedonia modulates the effects of positive mood induction on reward-related brain activation HHS public access. Neuroimage 193, 115–125. doi: 10.1016/j.neuroimage.2019.02.063
Guerra-Araiza, C., Villamar-Cruz, O., González-Arenas, A., Chavira, R., and Camacho-Arroyo, I. (2003). Changes in progestrone receptor isoforms content in the rat brain during the oestrous cycle and after oestradiol and progesterone treatments. J. Neuroendocrinol. 15, 984–990. doi: 10.1046/j.1365-2826.2003.01088.x
Hamani, C., Diwan, M., Macedo, C. E., Brandão, M. L., Shumake, J., Gonzalez-Lima, F., et al. (2010). Antidepressant-like effects of medial prefrontal cortex deep brain stimulation in rats. Biol. Psychiatry 67, 117–124. doi: 10.1016/j.biopsych.2009.08.025
Handa, R. J., Hejna, G. M., and Lorens, S. A. (1997). Androgen inhibits neurotransmitter turnover in the medial prefrontal cortex of the rat following exposure to a novel environment. Brain Res. 751, 131–138. doi: 10.1016/S0006-8993(96)01394-7
Herman, J. P., McKlveen, J. M., Ghosal, S., Kopp, B., Wulsin, A., Makinson, R., et al. (2016). Regulation of the hypothalamic-pituitary-adrenocortical stress response. Compr. Physiol. 6, 603–621. doi: 10.1002/cphy.c150015
Huang, W. C., Zucca, A., Levy, J., and Page, D. T. (2020). Social behavior is modulated by valence-encoding mPFC-Amygdala Sub-circuitry. Cell Rep. 32:107899. doi: 10.1016/j.celrep.2020.107899
Hurley, S. W., and Carelli, R. M. (2020). Activation of infralimbic to nucleus accumbens shell pathway suppresses conditioned aversion in male but not female rats. J. Neurosci. doi: 10.1523/JNEUROSCI.0137-20.2020 [Epub ahead of print].
Keedwell, P. A., Andrew, C., Williams, S. C. R., Brammer, M. J., and Phillips, M. L. (2005). The neural correlates of anhedonia in major depressive disorder. Biol. Psychiatry 58, 843–853. doi: 10.1016/j.biopsych.2005.05.019
Kennedy, D. P., and Adolphs, R. (2012). The social brain in psychiatric and neurological disorders. Trends Cogn. Sci. 16, 559–572. doi: 10.1016/j.tics.2012.09.006
Krishnan, V., and Nestler, E. J. (2008). The molecular neurobiology of depression. Nature 455, 894–902. doi: 10.1038/nature07455
Kritzer, M. F. (2003). Long-term gonadectomy affects the density of tyrosine hydroxylase- but not dopamine-β- hydroxylase-, choline acetyltransferase- or serotonin-immunoreactive axons in the medial prefrontal cortices of adult male rats. Cereb. Cortex 13, 282–296. doi: 10.1093/cercor/13.3.282
Kuehner, C. (2017). Why is depression more common among women than among men? Lancet Psychiatry 4, 146–158. doi: 10.1016/S2215-0366(16)30263-2
Kupferberg, A., Bicks, L., and Hasler, G. (2016). Social functioning in major depressive disorder. Neurosci. Biobehav. Rev. 69, 313–332. doi: 10.1016/j.neubiorev.2016.07.002
Low, K. L., Ma, C., and Soma, K. K. (2017). Tyramide signal amplification permits immunohistochemical analyses of androgen receptors in the rat prefrontal cortex. J. Histochem. Cytochem. 65, 295–308. doi: 10.1369/0022155417694870
Luczynski, P., Moquin, L., and Gratton, A. (2015). Chronic stress alters the dendritic morphology of callosal neurons and the acute glutamate stress response in the rat medial prefrontal cortex. Stress 18, 654–667. doi: 10.3109/10253890.2015.1073256
Lupien, S. J., McEwen, B. S., Gunnar, M. R., and Heim, C. (2009). Effects of stress throughout the lifespan on the brain, behaviour and cognition. Nat. Rev. Neurosci. 10, 434–445. doi: 10.1038/nrn2639
Mayberg, H. S., Liotti, M., Brannan, S. K., McGinnis, S., Mahurin, R. K., and Jerabek, P. A. (eds) (2013). “Reciprocal limbic-cortical function and negative mood: converging PET findings in depression and normal sadness,” in Depression: The Science of Mental Health, (Milton Park: Taylor and Francis), 245–253.
Mayberg, H. S., Lozano, A. M., Voon, V., McNeely, H. E., Seminowicz, D., Hamani, C., et al. (2005). Deep brain stimulation for treatment-resistant depression. Neuron 45, 651–660. doi: 10.1016/j.neuron.2005.02.014
McKlveen, J. M., Moloney, R. D., Scheimann, J. R., Myers, B., and Herman, J. P. (2019). “Braking” the prefrontal cortex: the role of glucocorticoids and interneurons in stress adaptation and pathology. Biol. Psychiatry 86, 669–681. doi: 10.1016/j.biopsych.2019.04.032
McKlveen, J. M., Morano, R. L., Fitzgerald, M., Zoubovsky, S., Cassella, S. N., Scheimann, J. R., et al. (2016). Chronic stress increases prefrontal inhibition: a mechanism for stress-induced prefrontal dysfunction. Biol. Psychiatry 80, 754–764. doi: 10.1016/j.biopsych.2016.03.2101
McKlveen, J. M., Myers, B., Flak, J. N., Bundzikova, J., Solomon, M. B., Seroogy, K. B., et al. (2013). Role of prefrontal cortex glucocorticoid receptors in stress and emotion. Biol. Psychiatry 74, 672–679. doi: 10.1016/j.biopsych.2013.03.024
McKlveen, J. M., Myers, B., and Herman, J. P. (2015). The medial prefrontal cortex: coordinator of autonomic, neuroendocrine and behavioural responses to stress. J. Neuroendocrinol. 27, 446–456. doi: 10.1111/jne.12272
McLaughlin, K. J., Baran, S. E., and Conrad, C. D. (2009). Chronic stress- and sex-specific neuromorphological and functional changes in limbic structures. Mol. Neurobiol. 40, 166–182. doi: 10.1007/s12035-009-8079-7
Mikosz, M., Nowak, A., Werka, T., and Knapska, E. (2015). Sex differences in social modulation of learning in rats. Sci. Rep. 5:18114. doi: 10.1038/srep18114
Minami, C., Shimizu, T., and Mitani, A. (2017). Neural activity in the prelimbic and infralimbic cortices of freely moving rats during social interaction: effect of isolation rearing. PLoS One 12:e0176740. doi: 10.1371/journal.pone.0176740
Moench, K. M., Breach, M. R., and Wellman, C. L. (2019). Chronic stress produces enduring sex- and region-specific alterations in novel stress-induced c-Fos expression. Neurobiol. Stress 10:100147. doi: 10.1016/j.ynstr.2019.100147
Montague, D., Weickert, C. S., Tomaskovic-Crook, E., Rothmond, D. A., Kleinman, J. E., and Rubinow, D. R. (2008). Oestrogen receptor α localisation in the prefrontal cortex of three mammalian species. J. Neuroendocrinol. 20, 893–903. doi: 10.1111/j.1365-2826.2008.01743.x
Moorman, D. E., and Aston-Jones, G. (2015). Prefrontal neurons encode context-based response execution and inhibition in reward seeking and extinction. Proc. Natl. Acad. Sci. U.S.A. 112, 9472–9477. doi: 10.1073/pnas.1507611112
Myers, B., McKlveen, J. M., and Herman, J. P. (2014). Glucocorticoid actions on synapses, circuits, and behavior: implications for the energetics of stress. Front. Neuroendocrinol. 35, 180–196. doi: 10.1016/j.yfrne.2013.12.003
Myers, B., McKlveen, J. M., Morano, R., Ulrich-Lai, Y. M., Solomon, M. B., Wilson, S. P., et al. (2017). Vesicular glutamate transporter 1 knockdown in infralimbic prefrontal cortex augments neuroendocrine responses to chronic stress in male rats. Endocrinology 158, 3579–3591. doi: 10.1210/en.2017-00426
Navarria, A., Wohleb, E. S., Voleti, B., Ota, K. T., Dutheil, S., Lepack, A. E., et al. (2015). Rapid antidepressant actions of scopolamine: role of medial prefrontal cortex and M1-subtype muscarinic acetylcholine receptors. Neurobiol. Dis. 82, 254–261. doi: 10.1016/j.nbd.2015.06.012
Nawreen, N., Cotella, E. M., Morano, R., Mahbod, P., Dalal, K. S., Fitzgerald, M., et al. (2020). Chemogenetic inhibition of infralimbic prefrontal cortex gabaergic parvalbumin interneurons attenuates the impact of chronic stress in male mice. eNeuro 7:ENEURO.423-19.2020. doi: 10.1523/ENEURO.0423-19.2020
Nestler, E. J., Barrot, M., DiLeone, R. J., Eisch, A. J., Gold, S. J., and Monteggia, L. M. (2002). Neurobiology of depression. Neuron 34, 13–25. doi: 10.1016/S0896-6273(02)00653-0
Newmyer, B. A., Whindleton, C. M., Klein, P. M., Beenhakker, M. P., Jones, M. K., and Scott, M. M. (2019). VIPergic neurons of the infralimbic and prelimbic cortices control palatable food intake through separate cognitive pathways. JCI Insight 4:e126283. doi: 10.1172/jci.insight.126283
Pace, S. A., Christensen, C., Schackmuth, M. K., Wallace, T., McKlveen, J. M., Beischel, W., et al. (2020). Infralimbic cortical glutamate output is necessary for the neural and behavioral consequences of chronic stress. Neurobiol. Stress 13:100274. doi: 10.1016/j.ynstr.2020.100274
Page, C. E., and Coutellier, L. (2019). Prefrontal excitatory/inhibitory balance in stress and emotional disorders: evidence for over-inhibition. Neurosci. Biobehav. Rev. 105, 39–51. doi: 10.1016/j.neubiorev.2019.07.024
Page, C. E., Shepard, R., Heslin, K., and Coutellier, L. (2019). Prefrontal parvalbumin cells are sensitive to stress and mediate anxiety-related behaviors in female mice. Sci. Rep. 9:19772. doi: 10.1038/s41598-019-56424-9
Pereira, M., and Morrell, J. I. (2020). Infralimbic cortex biases preference decision making for offspring over competing cocaine-associated stimuli in new mother rats. eNeuro 7, 1–13. doi: 10.1523/ENEURO.0460-19.2020
Pose, S., María, L., Zuluaga, J., Ferreño, M., Agrati, D., Bedó, G., et al. (2019). Raising overlapping litters: differential activation of rat maternal neural circuitry after interacting with newborn or juvenile pups. J. Neuroendocrinol. 31:e12701. doi: 10.1111/jne.12701
Premachandran, H., Zhao, M., and Arruda-Carvalho, M. (2020). Sex differences in the development of the rodent corticolimbic system. Front. Neurosci. 14:583477. doi: 10.3389/FNINS.2020.583477
Reul, J. M. H. M., and De Kloet, E. R. (1986). Anatomical resolution of two types of corticosterone receptor sites in rat brain with in vitro autoradiography and computerized image analysis. J. Steroid Biochem. 24, 269–272. doi: 10.1016/0022-4731(86)90063-4
Sankar, T., Chakravarty, M. M., Jawa, N., Li, S. X., Giacobbe, P., Kennedy, S. H., et al. (2019). Neuroanatomical predictors of response to subcallosal cingulate deep brain stimulation for treatment-resistant depression. J. Psychiatry Neurosci. 44, 1–10. doi: 10.1503/jpn.180207
Schaeuble, D., Packard, A. E. B., McKlveen, J. M., Morano, R., Fourman, S., Smith, B. L., et al. (2019). Prefrontal cortex regulates chronic stress-induced cardiovascular susceptibility. J. Am. Heart Assoc. 8:e014451. doi: 10.1161/JAHA.119.014451
Scopinho, A. A., Scopinho, M., Lisboa, S. F., Correa, F. M., de, A., Guimarães, F. S., et al. (2010). Acute reversible inactivation of the ventral medial prefrontal cortex induces antidepressant-like effects in rats. Behav. Brain Res. 214, 437–442. doi: 10.1016/j.bbr.2010.06.018
Selimbeyoglu, A., Kim, C. K., Inoue, M., Lee, S. Y., Hong, A. S. O., Kauvar, I., et al. (2017). Modulation of prefrontal cortex excitation/inhibition balance rescues social behavior in CNTNAP2-deficient mice. Sci. Transl. Med. 9:eaah6733. doi: 10.1126/scitranslmed.aah6733
Shansky, R. M., Hamo, C., Hof, P. R., Lou, W., McEwen, B. S., and Morrison, J. H. (2010). Estrogen promotes stress sensitivity in a prefrontal cortex-amygdala pathway. Cereb. Cortex 20, 2560–2567. doi: 10.1093/cercor/bhq003
Shansky, R. M., Hamo, C., Hof, P. R., McEwen, B. S., and Morrison, J. H. (2009). Stress-induced dendritic remodeling in the prefrontal cortex is circuit specific. Cereb. Cortex 19, 2479–2484. doi: 10.1093/cercor/bhp003
Shansky, R. M., and Woolley, C. S. (2016). Considering sex as a biological variable will be valuable for neuroscience research. J. Neurosci. 36, 11817–11822. doi: 10.1523/JNEUROSCI.1390-16.2016
Shepard, R., and Coutellier, L. (2018). Changes in the prefrontal glutamatergic and parvalbumin systems of mice exposed to unpredictable chronic stress. Mol. Neurobiol. 55, 2591–2602. doi: 10.1007/s12035-017-0528-0
Shepard, R., Page, C. E., and Coutellier, L. (2016). Sensitivity of the prefrontal GABAergic system to chronic stress in male and female mice: relevance for sex differences in stress-related disorders. Neuroscience 332, 1–12. doi: 10.1016/j.neuroscience.2016.06.038
Shimizu, T., Minami, C., and Mitani, A. (2018). Effect of electrical stimulation of the infralimbic and prelimbic cortices on anxiolytic-like behavior of rats during the elevated plus-maze test, with particular reference to multiunit recording of the behavior-associated neural activity. Behav. Brain Res. 353, 168–175. doi: 10.1016/j.bbr.2018.07.005
Shors, T. J. (2016). A trip down memory lane about sex differences in the brain. Philos. Trans. R. Soc. B Biol. Sci. 371:20150124. doi: 10.1098/rstb.2015.0124
Slattery, D. A., Neumann, I. D., and Cryan, J. F. (2011). Transient inactivation of the infralimbic cortex induces antidepressant-like effects in the rat. J. Psychopharmacol. 25, 1295–1303. doi: 10.1177/0269881110368873
Sood, A., Chaudhari, K., and Vaidya, V. A. (2018). Acute stress evokes sexually dimorphic, stressor-specific patterns of neural activation across multiple limbic brain regions in adult rats. Stress 21, 136–150. doi: 10.1080/10253890.2017.1422488
Van Kerkhof, L. W., Damsteegt, R., Trezza, V., Voorn, P., and Vanderschuren, L. J. (2013). Social play behavior in adolescent rats is mediated by functional activity in medial prefrontal cortex and striatum. Neuropsychopharmacology 38, 1899–1909. doi: 10.1038/npp.2013.83
Vertes, R. P. (2004). Differential projections of the infralimbic and prelimbic cortex in the rat. Synapse 51, 32–58. doi: 10.1002/syn.10279
Vijayakumar, N., Cheng, T. W., and Pfeifer, J. H. (2017). Neural correlates of social exclusion across ages: a coordinate-based meta-analysis of functional MRI studies. Neuroimage 153, 359–368. doi: 10.1016/j.neuroimage.2017.02.050
Wallace, T., Schaeuble, D., Pace, S. A., Schackmuth, M. K., Hentges, S. T., Chicco, A. J., et al. (2021). Sexually divergent cortical control of affective-autonomic integration. Psychoneuroendocrinology 129:105238. doi: 10.1016/j.psyneuen.2021.105238
Warden, M. R., Selimbeyoglu, A., Mirzabekov, J. J., Lo, M., Thompson, K. R., Kim, S. Y., et al. (2012). A prefrontal cortex-brainstem neuronal projection that controls response to behavioural challenge. Nature 492, 428–432. doi: 10.1038/nature11617
Wei, J., Yuen, E. Y., Liu, W., Li, X., Zhong, P., Karatsoreos, I. N., et al. (2014). Estrogen protects against the detrimental effects of repeated stress on glutamatergic transmission and cognition. Mol. Psychiatry 19, 588–598. doi: 10.1038/mp.2013.83
Wiersielis, K. R., Wicks, B., Simko, H., Cohen, S. R., Khantsis, S., Baksh, N., et al. (2016). Sex differences in corticotropin releasing factor-evoked behavior and activated networks. Psychoneuroendocrinology 73, 204–216. doi: 10.1016/j.psyneuen.2016.07.007
Wilber, A. A., Walker, A. G., Southwood, C. J., Farrell, M. R., Lin, G. L., Rebec, G. V., et al. (2011). Chronic stress alters neural activity in medial prefrontal cortex during retrieval of extinction. Neuroscience 174, 115–131. doi: 10.1016/j.neuroscience.2010.10.070
Wood, M., Adil, O., Wallace, T., Fourman, S., Wilson, S. P., Herman, J. P., et al. (2018). Infralimbic prefrontal cortex structural and functional connectivity with the limbic forebrain: a combined viral genetic and optogenetic analysis. Brain Struct. Funct. 13:100274. doi: 10.1007/s00429-018-1762-6
Yousuf, H., Smies, C. W., Hafenbreidel, M., Tuscher, J. J., Fortress, A. M., Frick, K. M., et al. (2019). Infralimbic estradiol enhances neuronal excitability and facilitates extinction of cocaine seeking in female rats via a BDNF/TrkB mechanism. Front. Behav. Neurosci. 13:168. doi: 10.3389/fnbeh.2019.00168
Keywords: coping, depression, gonadal hormones, infralimbic cortex, reward, sociability, valence
Citation: Wallace T and Myers B (2021) Effects of Biological Sex and Stress Exposure on Ventromedial Prefrontal Regulation of Mood-Related Behaviors. Front. Behav. Neurosci. 15:737960. doi: 10.3389/fnbeh.2021.737960
Received: 07 July 2021; Accepted: 06 August 2021;
Published: 26 August 2021.
Edited by:
Mario G. Oyola, Uniformed Services University of the Health Sciences, United StatesReviewed by:
Krystle Frahm, University of Pittsburgh, United StatesMichael Baum, Boston University, United States
Copyright © 2021 Wallace and Myers. This is an open-access article distributed under the terms of the Creative Commons Attribution License (CC BY). The use, distribution or reproduction in other forums is permitted, provided the original author(s) and the copyright owner(s) are credited and that the original publication in this journal is cited, in accordance with accepted academic practice. No use, distribution or reproduction is permitted which does not comply with these terms.
*Correspondence: Brent Myers, YnJlbnQubXllcnNAY29sb3N0YXRlLmVkdQ==