- 1Department of Basic Science, Medical College, Princess Nourah Bint Abdulrahman University (PNU), Riyadh, Saudi Arabia
- 2Department of Biological Science, Faculty of Science, King Abdulaziz University, Jeddah, Saudi Arabia
- 3Department of Medical Laboratory Technology, Faculty of Applied Medical Sciences, University of Tabuk, Tabuk, Saudi Arabia
- 4Department of Hematology/Pediatric Oncology, Yousef Abdullatif Jameel Chair of Prophetic Medical Applications, Faculty of Medicine, King Abdulaziz University, Jeddah, Saudi Arabia
- 5Eye Hospital, Jeddah, Ministry of Health (MOH), Jeddah, Saudi Arabia
- 6Department of Anatomy, Faculty of Medicine for Girls, Al-Azhar University, Cairo, Egypt
- 7Faculty of Nurses, National Gard, King Saud University, Jeddah, Saudi Arabia
- 8Department of Medical Histology and Cell Biology, Faculty of Medicine, Mansoura University, Mansoura, Egypt
- 9Department of Medical Histology, Faculty of Medicine, Damietta University, Damietta, Egypt
Background: Musk (Moschus moschiferus) has been described to have a significant impact on the central nervous system, as well as anticonvulsion and antidepressant effects. This study was designed to evaluate the efficacy of musk in alleviating alterations induced in olfactory bulb of depressed mice exposed to chronic stress and identify the mechanism behind it.
Methods: Fifty male albino mice were divided into five groups (n = 10 each): control, musk, chronic unpredictable mild stress (CUMS), fluoxetine-treated, and musk-treated groups were included in this study. Behavioral changes and serum levels of corticosterone and proinflammatory cytokines included tumor necrosis factor α, interleukin 6, and oxidant/antioxidant profile were assessed at the end of the experiment. Main olfactory bulb (MOB) has been processed for histopathological examination. Gene expression of caspase-3, glial fibrillary acidic protein, and Ki67 were assessed in the MOB using quantitative real-time polymerase chain reaction.
Results: The study showed that musk inhalation significantly reduced (p < 0.001) corticosterone level, immobility time, inflammatory cytokines, and oxidative stress markers in CUMS-exposed mice compared to the untreated CUMS group. Musk lessened CUMS-associated neuronal alterations in the MOB and significantly reduced apoptosis and enhanced neural cell proliferation (p < 0.001) comparable to fluoxetine. Musk significantly enhanced the level of antioxidants in the serum and significantly reduced inflammatory cytokines. The anti-inflammatory and antioxidant activity of musk and its constituents seemed to be behind its neuroprotective effect observed in this study.
Conclusion: Musk effectively ameliorated the chronic stress–induced behavioral, biochemical, and neuronal structural changes in MOB mostly through its antioxidant and anti-inflammatory effect.
Introduction
Depression is considered a worldwide health problem as more than 264 million from different age groups, all over the world, are suffering from depression. It may promote suicide, so it is considered one of the main causes of death in people 15- to 29-year-olds (WHO, 2020).
The olfactory system is a part of the limbic system. Besides its limbic connections, olfactory system has reciprocal projections with neocortical regions and several brainstem nuclei (Fudge and Tucker, 2009). The olfactory system is known as a crucial postdevelopmental neurogenesis brain region that functions throughout life in the adult mammalian brain (Cheng et al., 2016; Cersosimo, 2018). Olfactory perceptual deficits are reported in several neurodegenerative disorders as Alzheimer disease, vascular dementia, Parkinson disease, and frontotemporal dementia. These deficits appear early and preceding the classical cognitive and motor symptoms of the disease; thus, they are considered as prodromal symptoms. These deficits are also accompanied by pathology in related brain regions, for example, the limbic system, amygdala, hippocampus, and neocortical areas (Rey et al., 2018; Yoo et al., 2018). Exposure to chronic stress has been described to impair neurogenesis in hippocampus of rodents and subsequently involved in pathogenesis of neurodegenerative disorders as major depression that has been improved by use of antidepressants (Boldrini et al., 2009; Ayuob et al., 2018). It is well evidenced that chronic unpredictable mild stress (CUMS) induces olfactory bulb (OB) dysfunction in which neurogenesis and energy metabolism disturbance are involved (Cheng et al., 2016). Moreover, olfactory bulbectomy of rodents was one of the most commonly used depression models, indicating the relationship between OB and depression (Zhou et al., 2019; Nakagawasai et al., 2020). Rodents with olfactory bulbectomy showed memory dysfunction, depressive behavior, and neurochemical changes, such as reduced hippocampal monoamines, reduced neurogenesis, and brain-derived neurotrophic factor (BDNF) levels (Odaira et al., 2019).
Exposure to psychosocial stressors has been linked to stimulation of the sympathetic nervous system, secretion of adrenaline and noradrenaline, and the release of a set of proinflammatory cytokines including interleukin 6 (IL-6) (Finnell et al., 2017). It has been reported that continuous exposure to stressors along with enhanced secretion of inflammatory cytokines results in chronic neuroinflammation that triggers depression. Adding to that, neuroinflammation disturbs the balance of the antioxidant system that augments the development of depressive state (Kim et al., 2016). Therefore, inflammatory cytokines and oxidant/antioxidant status were assessed in this study.
Complementary alternative medicine has become an attractive alternate to medications. It includes the utilization of natural materials derived from animal or plant sources in managing many health problems (Tillett and Ames, 2010). It has been reported that some essential oils might be effective in relieving emotional changes in several psychological and neurodegenerative disorders such as stress and dementia (Bikmoradi et al., 2015; Yoshiyama et al., 2015).
Musk (Moschus moschiferus), a substance with a penetrating odor, belongs to the well-known and valued class of the fragrance family. Natural musk has been obtained from the male musk deer (Sytniczuk et al., 2018). Musk is a part of traditional Chinese medicine as it possesses many beneficial effects such as immunity-enhancing, antibacterial, and anti-inflammatory effects (Li et al., 2018; Jie et al., 2019). Adding to that, musk has significant effects on the central nervous system (CNS) as it has been used in treating stroke, coma, neurasthenia, convulsions, and cerebral ischemia (Ayuob, 2017; Abd El Wahab et al., 2018; Lee et al., 2019; Radnaa et al., 2020). Musky compounds, for example, androstadienone, androstenol, and muscone, were described to modulate psychological state, reducing negative mood and increasing positive mood (Jacob and McClintock, 2000; Jacob et al., 2001, 2002).
The link between depression and OB has been previously described (Zhou et al., 2019; Nakagawasai et al., 2020), but the CUMS-induced impact on the main olfactory bulb (MOB) has not been clearly discussed. Therefore, the present study was designed to evaluate the impact of musk on the structural changes induced by CUMS in MOB of mice, as well as the associated biochemical and molecular changes, and explore the possible mechanisms behind these changes.
Materials and Methods
Chemicals
Fluoxetine (Flu) (Dar Al Dawa Pharmaceuticals Co., Ltd., Jordan) was given to the mice, at a dose of 20 mg/kg per day, through intragastric gavage and dissolved in 2 mL sodium carboxymethyl cellulose (0.03%) once per day (Li et al., 2014).
Musk (M. moschiferus), obtained from one of the trusted shops for musk and perfumes in Jeddah, Kingdom of Saudi Arabia (KSA), was utilized in this study. Musk compounds have been defined by gas chromatography and mass spectrometry (GC–MS) (Agilent, Columbia, SC, United States). Just before administration, musk was diluted using propylene glycol 1.0% (vol/vol) as has been previously described (Fukayama et al., 1999).
Positive control group has been exposed to amyl acetate 5% (Sigma, St. Louis, MO, United States) through inhalation. It was selected because it was safely utilized for testing olfactory detection thresholds in humans (Walker et al., 2003) and rodents (Fleming et al., 2008), and it was reported not to have an impact on anxiety (Pavesi et al., 2011).
Animals
This experiment has been ethically approved by the Biomedical Research Ethics Committee, Faculty of Medicine, King Abdulaziz University, Jeddah, Saudi Arabia (reference no. 48-16), and followed the guidelines of animal care that has been set by King Fahd Medical Research Center (KFMRC), at King Abdulaziz University. In this study, 50 male Swiss albino mice, obtained from the animal house at KFMRC, were utilized. Their ages were ranged from 5 to 6 weeks, and their weights ranged from 30 to 40 g. They were left to acclimatize for 15 days with the laboratory conditions at 27 ± 1°C with free access to water and standard mice pellets before starting exposure to CUMS.
Mice were assigned, at random, into five groups (n = 10): control, musk, CUMS + Amyl, CUMS + Flu, and CUMS + musk groups. Each five mice were housed in a cage. The mice were exposed to CUMS protocol for 28 days according to Doron et al. (2014) followed by 2 weeks of treatment with amyl acetate, Flu, or musk. During CUMS protocol, mice were exposed to stressors at different times through the day in order to prevent adaptation to stress, for example, social stress by placing them in cages soiled by other mice, reversing the light–dark cycle, and restraining the mice. The mice were individually housed during restraint stress exposure. See the experiment timeline in Figure 1.
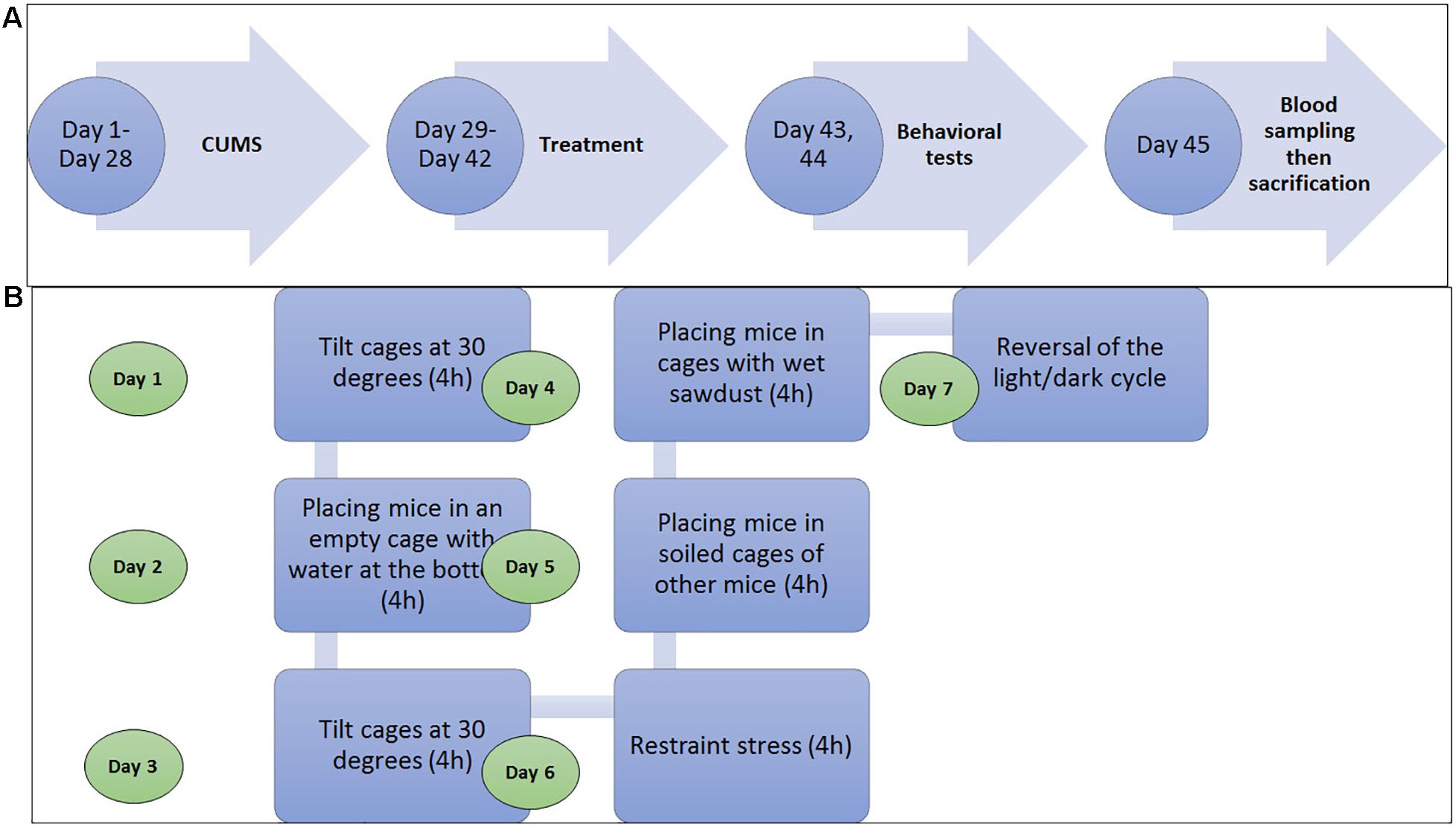
Figure 1. (A) Diagram shows that the experimental timeline shows that mice were exposed to either chronic unpredictable mild stress (CUMS) for 28 days and then treatment with amyl acetate, fluoxetine (Flu), or muck for 14. On days 43 and 44, the behavioral tests were sequentially done for all groups, and then the blood sample was obtained on the day 45, and finally, the mice were sacrificed. (B) Diagram shows the stressor to which the mice were exposed during the CUMS procedure in 1 week at different time points during the day. It was repeated for 4 weeks (Doron et al., 2014.
Administration of musk and amyl acetate has been performed through inhalation in an odor-isolated chamber (32 cm × 24 cm × 32 cm), as described (Chioca et al., 2013). Inhalation was performed once per day immediately after the CUMS procedure and lasted for 15 min, where the mice were individually placed in cages.
Identification of the Constituents of Musk
The components of musk have been identified by using GC–MS (Thermo Scientific, Austin, TX, United States) with a direct capillary column TR-5MS (30 m 0.25 μm 9 0.25-μm film thickness).
Behavioral Assessment
Behavior changes were assessed at the end of the experiment in the morning (8:00–11:30 AM) through forced swimming test (FST) and elevated plus maze test (EPM) separated by 24 h (Mineur et al., 2006).
The FST is described to be sensitive to all available antidepressant drugs; therefore, it is commonly used to test the antidepressant effect of new drugs (Jeong et al., 2015). The EPM is a validated, widely used behavioral assay in rodents used to assess the antianxiety effects of pharmacological agents and to study the mechanisms underlying anxiety-related behavior (Walf and Frye, 2007). Hence, both tests were selected in this study.
Regarding FST, the total time of immobility spent by the mouse during 6 min of observation was documented in seconds. During EPM, the number of entries to closed arms was counted in 6 min, and the time spent in the open arms was estimated in seconds for each mouse using Noldus Information Technology, EthoVision XT®.
Biochemical Assessment
Twenty-four hours after finishing the behavioral tests, mice were anesthetized with 4% isoflurane (SEDICO Pharmaceuticals Company, Cairo, Egypt) in 100% oxygen. Blood samples were collected from the retro-orbital venous plexus of the mice in tubes with EDTA and then centrifuged for 15 min to be kept at −80°C for biochemical assessment. Serum samples, obtained by centrifugation as previously described, were run in duplicate in order to provide enough data for statistical validation of the results. Variation coefficient, the ratio of the standard deviation to the mean, of duplicates was accepted at ≤20%.
Corticosterone level has been assessed in the serum, based on the manufacturer instructions, in order to confirm induction of depression using enzyme-linked immunosorbent assay (ELISA) kits (ALPCO Diagnostics, Orangeburg, NY, United States). Tumor necrosis factor α (TNF-α) and IL-6 (Quantikine; R&D Systems, USA Kit) were measured in the serum using ELISA as described in the manufacturer instructions.
Oxidant/antioxidant profile in the serum has been assessed through measuring malonaldehyde (MDA) spectrophotometrically at 535 nm using the thiobarbituric acid reactive substances assay kit (Biodiagnostic; Egypt) as previously described (Gamal et al., 2018). Superoxide dismutase (SOD) activity was assessed using the relevant kit (Biodiagnostic; Egypt) as has been described by Packer (2002). Glutathione peroxidase (GPX) kits (Randox Labs, Crumlin, United Kingdom) and catalase (CAT) kits (Biodiagnostic; Egypt) were also used as was described by Gamal et al. (2018).
After obtaining the blood sampling, mice were immediately euthanized by cervical dislocation, and the whole brain with the OB has been carefully dissected out, sectioned into two hemispheres, then fixed in 10% neutral-buffered formalin, and routinely processed into paraffin blocks.
Expression of Ki67, caspase-3, and glial fibrillary acidic protein (GFAP) genes in MOB was assessed using quantitative real-time polymerase chain reaction (qRT-PCR). Extraction of total RNA using Trizol from 100 mg of paraffin-processed samples of MOB was performed based on the supplier instruction (Invitrogen Life Technologies, Carlsbad, CA, United States). The methods used were previously described (Ayuob et al., 2020). The primers used are shown in Supplementary Table 1. Results are presented as the ratio of mRNA level of the gene to that of corresponding β-actin.
Histological Findings
Paraffin-embedded brain hemispheres including the MOB were sectioned at 5-μm thickness and stained with hematoxylin-eosin (H&E) to be examined histologically using the light microscope (LM). Another set of paraffin sections was immunohistochemically stained using the peroxidase-labeled streptavidin–biotin technique. Anti-GFAP antibody (at dilution 1:1,000; Dako Cytomation, Minneapolis, MN, United States) was utilized for identification of astrocytes. Anti–caspase-3 antibody (at dilution 1:1,000; Santa Cruz Biotechnology, Santa Cruz, CA, United States) was utilized for identification of apoptosis. Anti-Ki67 antibody (at dilution 1:100; Abcam, Cambridge, United Kingdom) was used for identification of cell proliferation, whereas anti–double-cortin (DCX) (at dilution 1:100; Santa Cruz Biotechnology) has been used for neuroblast identification.
Specificity of the primary antibody was verified by preparing a negative control section with the primary antibody omitted. Slides were examined and photographed using LM connected with camera (Olympus, BX-61, Los Angeles, CA, United States). ImageJ 1.52a (National Institutes of Health, United States) was used for semiquantitative assessment of antibody immunoexpression. Area percent of GFAP immune expression, the number of caspase-3– and Ki67-positive cells, and the thickness of mitral cell layer were assessed in five fields (×400)/slide, and five slides/mouse were examined.
Statistical Assessment
Statistical Package for the Social Sciences (SPSS, version 22) software was used to analyze the raw data of this study. Data normality was tested using Kolmogorov–Smirnov test, and it was noticed that the data followed the normal distribution. The study variables were affected by two independent factors: stress exposure and treatment; therefore, analysis using a mixed-model two-way analysis of variance (ANOVA) based on Bonferroni post hoc was performed. p < 0.05 was considered significant.
Results
Compounds Detected in Musk Using GC–MS
The main compounds detected in musk, used in this study, included steroids (13.80%), essential oils (12.45%), and organic compound (41.52%), besides other compounds (Table 1). Musk compounds that were reported to have anti-inflammatory effects included steroids, α-cedrol, 5,6-dihydroaaptamine, and nerolidol, whereas those with antioxidant effects included 2, 4-di-tert-butylphenol, benzyl benzoate, isophytol, spirostan-23-ol, and others (Table 1).
Behavioral Changes Induced by CUMS Exposure
In this study, statistical significance was assessed using a mixed-model two-way ANOVA to investigate the effects of two independent variables, the exposure to stress and the effect of treatment. Assessment of the behavioral changes of the mice was performed at the end of the experiment in order to confirm the occurrence of depression-like behavior after exposing mice to CUMS for 28 days. It has been found that musk inhalation did not significantly affect the behavior of the control mice during the FST or EPM test. On the other hand, CUMS significantly prolonged the immobility time (F = 6.34, p < 0.001, n = 10/group) during the FST. Mice of Flu-treated (F = 7.09, p = 0.03, n = 10/group) and musk-treated (F = 7.09, p = 0.01, n = 10/group) groups showed a significant reduction in immobility time compared to the CUMS group (Table 2).
The time spent in the open arms of EPM showed a significant decrease (F = 24.49, p < 0.001, n = 10/group), whereas the number of entries to the closed arms showed a significant increase (F = 25.44, p < 0.001, n = 10/group) in the CUMS group compared to the untreated CUMS group. Administration of Flu and musk, separately, induced a significant increase (F = 8.04, p = 0.01, p = 0.02, n = 10/group) in the time spent inside the open arms and a significant reduction (F = 30.09 p < 0.001, n = 10/group) in the number of entries to the closed arm compared to untreated CUMS group, respectively (Table 2).
Biochemical Changes Induced by CUMS Exposure
Effect of Musk on Serum Corticosterone Level
It has been observed that musk inhalation did not significantly affect the serum corticosterone level of the control group, whereas it was significantly higher (F = 454.84, p < 0.001, n = 10/group) in the CUMS group than that of the control group. On the other hand, serum corticosterone was significantly lower (F = 399.40, p < 0.001, n = 10/group) in both Flu and musk groups compared to the untreated CUMS group (Figure 2).
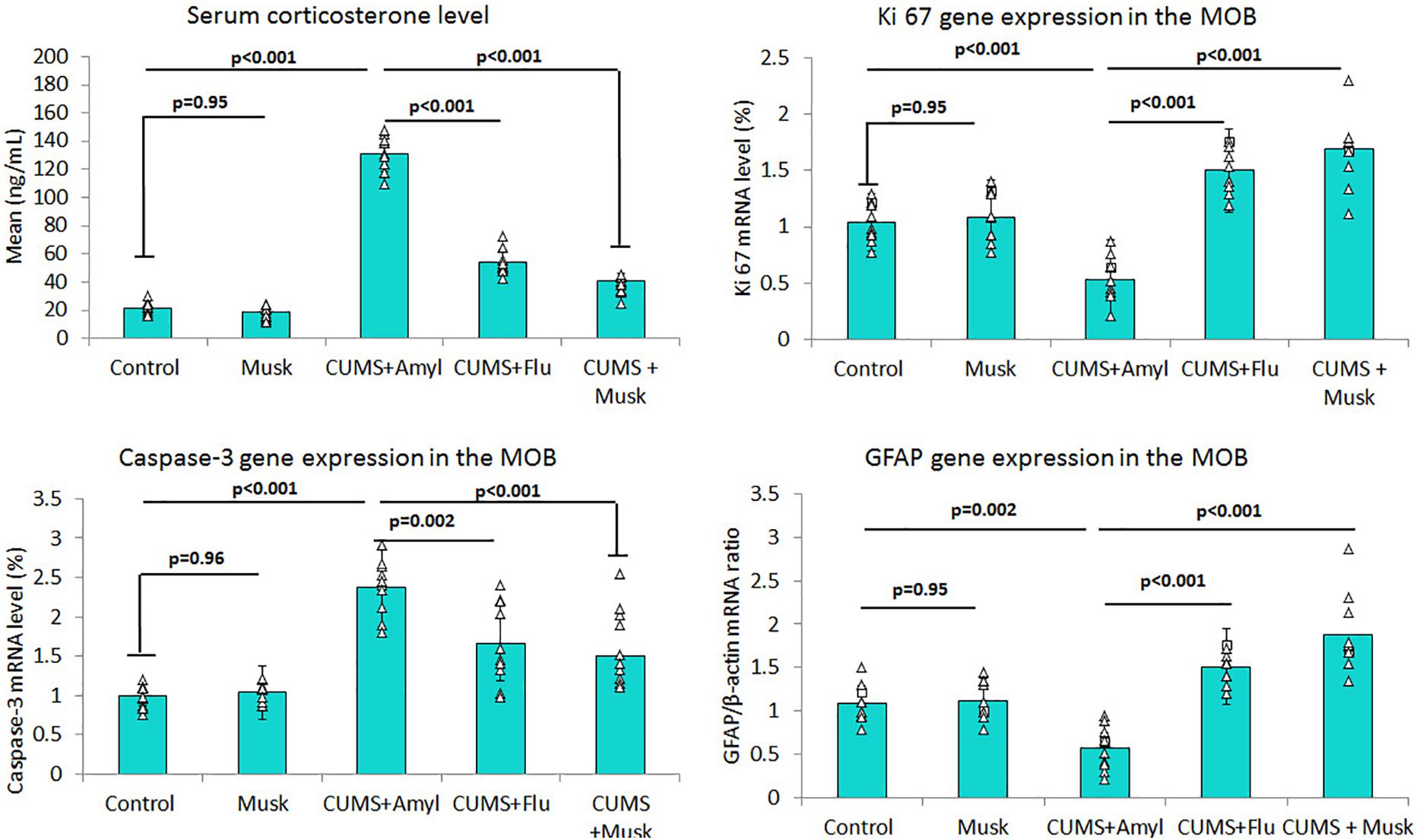
Figure 2. The effect of Flu and musk on serum corticosterone level, Ki67, caspase-3, and GFAP gene expression in MOB. Data are analyzed using a mixed-model two-way ANOVA based on Bonferroni post hoc was used to analyze the data. Results are expressed as mean ± SD (n = 10). p < 0.05 was considered significant. MOB, main olfactory bulb; CUMS, chronic unpredictable mild stress; Flu, fluoxetine.
Effect of Musk on Inflammatory Cytokines
The anti-inflammatory effect of musk has been assessed by measuring the proinflammatory cytokines levels in the serum. Although serum TNF-α and IL-6 levels did not show significant change in control mice inhaled musk, they were significantly increased (F = 102.67, F = 152.62, p < 0.001, n = 10/group) in the CUMS group compared to the control, respectively, whereas their levels showed no significant difference in the Flu-treated group. On the other hand, serum TNF-α and IL-6 levels were significantly lower (F = 79.07, F = 134.16, p < 0.001, n = 10/group) in the musk-treated group than those of the untreated CUMS group (Table 2).
Effect of Musk on Antioxidant Status
Serum SOD, GPX, and CAT levels showed an insignificant increase in control mice inhaled musk compared to the control group. CUMS-induced depression, in this study, was accompanied with a significant elevation (F = 5.75, p = 0.003, n = 10/group) in the MDA level, as well as a significant reduction in serum SOD (F = 7.35, p < 0.001, n = 10/group), GPX (F = 20.57, p < 0.001, n = 10/group), and CAT (F = 11.02, p = 0.002, n = 10/group) compared to the control. Whereas the musk-treated group showed significantly increased serum level of SOD (F = 4.77, p = 0.04), GPX (F = 8.92, p = 0.004), and CAT (F = 11.88, p = 0.001), the Flu-treated group did not show any significant change in their levels (Table 2).
Effect of Musk on Gene Expression
Levels of mRNA of caspase-3, GFAP, and Ki67 were assessed using qRT-PCR. It has been noticed that exposing mice to CUMS induced a significant up-regulation (F = 32.46, p < 0.001, n = 10/group) of mRNA level of caspase-3 in the MOB, while administering Flu (F = 13.77, p = 0.002) and musk (F = 13.77, p = 0.001) after exposure to CUMS induced a significant down-regulation in caspase-3 mRNA compared to the untreated group (Figure 2).
On the other hand, mRNA levels of GFAP (F = 43.38, p < 0.001) and Ki67 (F = 52.73, p < 0.001) in MOB were significantly lower in the CUMS group than those of the control group, respectively, whereas they were significantly higher in the Flu (GFAP; F = 61.30, p = 0.01, Ki67; F = 75.54, p = 0.002) and musk (F = 61.30, F = 75.54, p < 0.001) groups compared to the untreated CUMS group, respectively (Figure 2).
Effect of Musk on Histopathological Changes Induced by CUMS Exposure
Histopathological examination of the H&E-stained sections in MOB of control mice revealed regular multilayered organization of the bulb. The six distinct layers of the MOB were easily distinguished and including from inward outward: the granular cell layer, the internal plexiform layer, the mitral cell layer, the external plexiform layer, the glomerular layer, and the olfactory nerve layer, which is the outmost layer. The mitral cells, the largest cells in MOB, were triangular or multipolar nerve cells arranged in one row in the mitral cell layer. They had abundant granular basophilic cytoplasm and large pale vesicular nuclei with prominent nucleoli. There were scattered smaller granule nerve cells between the mitral cells (Figure 3). It was observed that musk inhalation did not affect the MOB of control mice (data not shown).
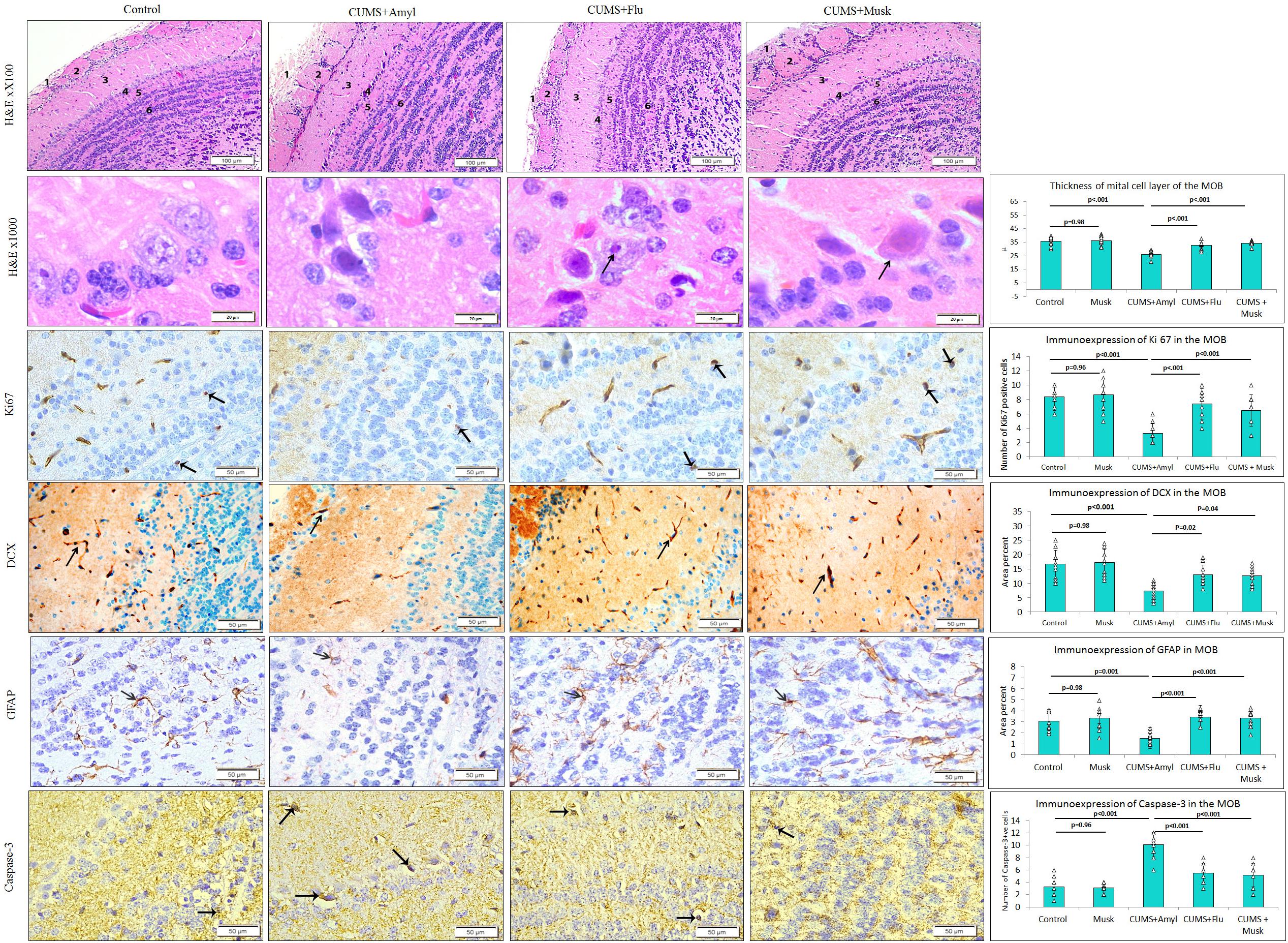
Figure 3. The effect of Flu and musk on the histological structure and immunoexpression of GFAP, caspase-3, Ki67, and DCX in the main olfactory bulb (MOB) of the studied groups. The MOB shows six layers; nerve fiber layer (1), glomerular layer (2), external plexiform layer (3), mitral layer (4), internal plexiform layer (5), and granular layers (6). Note the affected mitral cells in the mitral layer indicated by the arrow and surrounded by a wide pericellular space around them (arrowhead). Data are analyzed using a mixed-model two-way ANOVA based on Bonferroni post hoc was used to analyze the data. Results are expressed as mean ± SD (n = 10). p < 0.05 was considered significant (df = 39). CUMS, chronic unpredictable mild stress; Flu, fluoxetine.
On the other hand, mitral cells of CUMS-exposed mice were mostly affected as they appeared distorted, shrunken with dark cytoplasm, and deeply stained pyknotic nuclei. Widening of pericellular spaces around mitral cells has been also observed (Figure 3). The thickness of the mitral cell layer was significantly reduced (F = 29.28, p < 0.001) in the CUMS group compared to that of the control mice. Flu- and musk-treated groups showed that most mitral cells restored their normal appearance except few cells that were distorted. There was a significant increase (p < 0.001) in the thickness of the mitral cell layer in Flu- (F = 24.87, p < 0.001) and musk-treated (F = 24.87, p < 0.001) groups compared to the CUMS group (Figure 3).
Immunoexpression of Caspase-3
The number of caspase-3–positive cells, observed in all layers of the MOB, was significantly higher (F = 39.63, p < 0.001) in the CUMS group compared to the control, whereas it was significantly lower (F = 28.07, p < 0.001) in Flu- or musk-treated groups compared to the untreated CUMS group with no significant difference between the two treated groups (Figure 3).
Immunoexpression of Ki67 and DCX
The number of Ki67- and CDX-positive cells was markedly reduced (F = 16.99, F = 14.48, p < 0.001) in MOB of CUMS-exposed mice compared to that of the control, respectively, whereas it was significantly higher in Flu (Ki67; F = 14.27, p < 0.001, CDX; F = 6.96, p = 0.01) and musk (Ki67; F = 14.27, p < 0.001, CDX; F = 6.96, p = 0.02) groups compared to the untreated CUMS-exposed mice, with no significant difference between the two treated groups (Figure 3).
Immunoexpression of GFAP
It has been observed that CUMS group showed a significant lower (F = 8.45, p < 0.001, n = 10/group) GFAP immunoexpression in MOB compared to the control, while it was significantly higher (F = 11.28, p < 0.001) in Flu- and musk-treated groups. No statistical significant difference existed between the two groups receiving treatment (Figure 3).
Discussion
The olfactory sense could have unbelievable attributes if we consider its capacity to modulate human behaviors. It has determinant roles in the evolution of human habitat and in the social behavior (Sarafoleanu et al., 2009). The link between olfactory function and depression has been established. Many studies reported that decline in olfactory functions is the earliest biological marker of motor dysfunction or cognitive impairments appearing in the neurodegenerative disorders such as that occurs in Parkinson disease and Alzheimer disease (Meyer et al., 2018; Zheng et al., 2019). In this study, we hypothesized that inhalation of musk improves CUMS-induced structural changes in MOB. For confirmation of this hypothesis, mice were exposed to CUMS for a month in order to induce depression followed by exposure to musk inhalation for 2 weeks, and then CUMS-induced behavioral, biochemical, and MOB structural changes were investigated. It has been noted that exposing mice to CUMS induced a depressive state evident by prolonged immobility time during the FST and reduced time spent in the open arms of the EPM and has been verified by increased level of serum corticosterone. These findings were consistent with those previously reported (Liu et al., 2014; CB Filho et al., 2015). CUMS has been reported to increase serum corticosterone level mostly as a result of dysfunction of hypothalamic–pituitary–adrenal (HPA) axis as previously mentioned (Menard et al., 2017).
Both TNF-α and IL-6 were reported to be linked to depression pathogenesis (Taraz et al., 2015; Pedraz-Petrozzi et al., 2020); therefore, these cytokines were assessed, in this study, in order to investigate the anti-inflammatory effect of musk. We found that exposing mice to CUMS induced marked increase in inflammatory cytokines TNF-α and IL-6. This finding has been endorsed by Kim et al. (2016), who reported that the pathophysiological changes occur in depression including up-regulated proinflammatory cytokine secretion and functional resistance of glucocorticoid receptor in the hippocampus.
Stress increased oxygen free radicals in brain, which is more vulnerable to oxidative damage than other organs in addition to its limited antioxidant capacity. This resulted in damage of macromolecules such as lipid, protein, and nucleic acids, leading to neuronal dysfunction and atrophy (Freitas et al., 2014; CB Filho et al., 2015). In this study, CUMS-induced depressive-like status has been associated with disturbed antioxidant profile manifested by up-regulation of MDA and down-regulation of SOD, CAT, and GPX in the serum. Similar changes were reported by Nabavi et al. (2015) following exposure of animals to CUMS. They added that these changes were associated with oxidative stress and impairment of HPA. Liu et al. (2015) also reported that the development of depression may be due to low antioxidants content in the body.
The link between corticosterone levels, brain oxidative stress, and neuroinflammatory has been previously reported. Maternal separation model of early life stress has been reported to result in increased corticosterone levels and alterations of brain lipid peroxidation (Pinheiro et al., 2011). Brain oxidative damage has been reported to be involved in the development of CNS pathologies of neurodegenerative diseases and psychiatric disorders, in both animal models and patients (Sorce and Krause, 2009). Adding to that, reactive oxygen species (ROS) were reported to trigger alterations of brain morphology neuroinflammation and neuronal death (Gu et al., 2011).
Mitral cells of the MOB were grouped into three subtypes according to cell body shape: triangular, round, and fusiform type (Kikuta et al., 2013). Axons of mitral cells send projection to piriform cortex, the largest component of the primary olfactory cortex (Patel and Pinto, 2014). The CUMS mice, in the present work, showed structural changes in the OB in the form of mitral cell shrinkage that could be the explanation of the atrophy seen in this work in addition to other studies. Negoias et al. (2010) and Marsden (2013) reported that exposure to CUMS resulted in atrophy of limbic brain regions included hippocampus and MOB with subsequent olfactory dysfunction. CUMS exposure, in this study, led to reduction in immune and gene expression of GFAP in MOB, the selective astrocyte marker. This finding was consistent with what has been reported by Li et al. (2013), who mentioned that atrophy of microglia contributes to pathogenesis of depression.
In this study, exposing mice to CUMS induced suppression of neural cell proliferation evidenced by reduction of Ki67-positive proliferating cells as well as DCX-positive neuroblast in MOB together with the significant down-regulation of Ki67 gene expression compared to the control. Yang et al. (2011a) and Siopi et al. (2016) reported similar results in MOB using different cell proliferation markers as PSANCAM, DCX, and BrdU. Marked inhibition of neurogenesis has also been reported in the hippocampus of mice model of depression (Ayuob et al., 2018; Qi et al., 2018). This observation might be caused by elevation of plasma corticosterone in CUMS mice, which has antiproliferative effects (Ali et al., 2017; Tong et al., 2019).
Increased neuronal apoptosis has been observed in CUMS mice, in this study, manifested by numerous caspase-3–positive cells in addition to overexpression of caspase-3 gene in MOB. Previous studies have also reported similar results in rat MOB in CUMS models (Yang et al., 2011b) and in the hippocampal regions of mice subjected to CUMS (Abd El Wahab et al., 2018; Qi et al., 2018).
Fluoxetine, an approved antidepressant, has been used in this study, to relieve the depressive-like status in mice for pharmacological validation of musk. We observed that Flu attenuated the structural alteration that has been observed in MOB of CUMS mice, as well as the biochemical and the behavioral changes. Previous research reported that Flu relieved the behavioral changes and improved the structural deteriorations that occurred in the hippocampal regions of mice exposed to CUMS in accordance with our results (CB Filho et al., 2015; Ali et al., 2017; Ayuob et al., 2018). Although some studies have reported that Flu has anti-inflammatory activity (Kostadinov et al., 2015) and antioxidant properties (Caiaffo et al., 2016), in this study, administration of Flu has not been associated with a significant changes in proinflammatory cytokines or antioxidant levels. This might be attributed to the relatively short time of Flu administration.
In the present study, musk inhalation significantly improved the depressive-like behavioral changes and reduced serum corticosterone level. It also improved the neuronal affection in MOB after CUMS exposure mainly in the mitral cells in a way similar to that achieved by FLU. Thus, musk can reduce neuronal atrophy and restore astrocytes in MOB of mice. In addition, musk decreased neuronal and glial apoptosis and stimulated neural cell proliferation in mice MOB, evident by the decreased number of apoptotic cells and increased number of proliferating cells.
Similar results were reported by Abd El Wahab et al. (2018) and Ayuob et al. (2016) in the hippocampus of CUMS mice model, which is an important site for postdevelopmental neurogenesis together with MOB. Wang et al. (2015) and Lee et al. (2019) said that musk also had neuroprotective effect against focal cerebral ischemia in cases of ischemia/reperfusion injury.
These findings were also supported by those previously reported on muscone, the active ingredient of musk, which has been reported to exert antidementia (Liu et al., 2017), anti–cerebral ischemia (Surinkaew et al., 2018), and neuroprotective effects (Zhou et al., 2020). These activities and its underlying mechanisms are involved in promoting cell proliferation, ameliorating the loss of cell viability, preventing mitochondrial membrane potential collapse, lactate dehydrogenase, Ca2+ overload, ROS generation, and reducing the release of inflammatory factors, such as IL-1β, TNF-α, prostaglandin E2, and myeloperoxidase (Liu et al., 2017; Surinkaew et al., 2018; Zhou et al., 2020). Wei et al. (2012) and Zhang et al. (2020) reported that muscone can inhibit apoptosis via inhibiting expression of caspase 8 and Fas in the cortex and stimulate neural stem cells proliferation transforming them into neurons in ischemia/reperfusion rats. Muscone has also been suggested to mitigate hyperglycemia-induced Schwann cell autophagy and apoptosis partially by activating the Akt/mTOR signaling pathway (Dong et al., 2019).
This neuroprotective effect of musk might be attributed to its anti-inflammatory activity proven by the significant reduction in proinflammatory cytokines. Musk anti-inflammatory effect might be caused by its bioactive compounds detected in the present study such as steroids, α-cedrol, 5,6-dihydroaaptamine, and nerolidol. The antioxidant activity of musk, confirmed in this study, could be behind its neuroprotective effect. The compounds with antioxidant effect detected in musk, in this study, included 2,4-di-tert-butylphenol, benzyl benzoate, isophytol, spirostan-23-ol, and others. It has been reported that reduction of the antioxidants in the body may trigger the occurrence of depression (Liu et al., 2015). Therefore, restoring or enhancing the antioxidants capacity could exert a beneficial effect in preventing or treating depression.
Nerolidol, one of the compounds detected in musk in this study, has been reported to have antioxidant, anti-inflammatory, sedative, and neuroprotective effect, as it protects against hippocampal neuronal damages that occur in psychosocial disorders (Nogueira Neto et al., 2013). Nerolidol suppressed proinflammatory cytokines and inflammatory mediator release. It increased the level of SOD, CAT, and GSH and decreased the level of MDA; therefore, nerolidol treatment attenuated rotenone-induced dopaminergic neurodegeneration (Javed et al., 2016).
Among the limitations of the study was the inability to explore, in-depth, the mechanism through which musk exerts its neuroprotective effect on OB of depressed mice, although the study gave some evidence that confirmed this effect and provided some clues about the mechanism. Future study to identify the detailed mechanism is encouraged, and testing the efficacy of musk in volunteer depressed patients is also encouraged.
In conclusion, this study revealed that musk relieved behavioral depressive changes, as well as the biochemical changes observed in mice exposed to chronic mild stress. Musk also reduced apoptosis and enhanced neural cell proliferation in the MOB evidenced by underexpression of caspase-3 and enhanced expression of Ki67 gene.
Data Availability Statement
The original contributions presented in the study are included in the article/Supplementary Material, further inquiries can be directed to the corresponding author.
Ethics Statement
The animal study was reviewed and approved by Biomedical Research Ethics Committee, Faculty of Medicine, King Abdulaziz University, Jeddah, Saudi Arabia.
Author Contributions
HA, AB, and ZM supervised the experiment, collect data of the investigation, and participated in results interpretation. SA, SM, MA, AA, and NA designed the experimental protocol, involved in the implementation of the overall study, performed the statistical analysis of the study, researched the data, and wrote the manuscript. All authors contributed to the revision of the manuscript and approval of the final manuscript.
Conflict of Interest
The authors declare that the research was conducted in the absence of any commercial or financial relationships that could be construed as a potential conflict of interest.
Publisher’s Note
All claims expressed in this article are solely those of the authors and do not necessarily represent those of their affiliated organizations, or those of the publisher, the editors and the reviewers. Any product that may be evaluated in this article, or claim that may be made by its manufacturer, is not guaranteed or endorsed by the publisher.
Acknowledgments
This research was funded by the Deanship of Scientific Research at Princess Nourah Bint Abdulrahman University through the Fast-track Research Funding Program.
Supplementary Material
The Supplementary Material for this article can be found online at: https://www.frontiersin.org/articles/10.3389/fnbeh.2021.704180/full#supplementary-material
Supplementary Table 1 | The primers used in this study to assess gene expression through quantitative real-time polymerase chain reaction (qRT-PCR). They were obtained from Metabion International AG, Semmelweisstraße, Germany.
Abbreviations
CUMS, chronic unpredictable mild stress; MOB, main olfactory bulb; GFAP, glial fibrillary acidic protein; BDNF, brain-derived neurotrophic factor; GC–MS, gas chromatography and mass spectrometry; FST, forced swimming test; EPM, elevated plus maze; MDA, malonaldehyde; SOD, superoxide dismutase; GPX, glutathione peroxidase; CAT, catalase.
References
Abd El Wahab, M. G., Ali, S. S., and Ayuob, N. N. (2018). The role of musk in relieving the neurodegenerative changes induced after exposure to chronic stress. Am. J. Alzheimers Dis. Other. Demen. 33, 221–231. doi: 10.1177/1533317518755993
Al-Harthy, T., Zoghaib, W. M., Pfluger, M., Schöpel, M., Önder, K., Reitsammer, M., et al. (2016). Design, synthesis, and cytotoxicity of 5-fluoro-2-methyl-6-(4-aryl-piperazin-1-yl) benzoxazoles. Molecules 21:1290. doi: 10.3390/molecules21101290
Ali, S. S., Abd El Wahab, M. G., Ayuob, N. N., and Suliaman, M. (2017). The antidepressant-like effect of Ocimum basilicum in an animal model of depression. Bio. Histochem. 92, 390–401. doi: 10.1080/10520295.2017.1323276
Almoulah, N. F. (2017). Phytochemical Study and Biological Evaluation of Five Plants Belonging to the Family Solanaceae. France: Université de Lorraine.
Ayuob, N. N. (2017). Evaluation of the antidepressant-like effect of musk in an animal model of depression: how it works. Anat. Sci. Int. 92, 539–553. doi: 10.1007/s12565-016-0357-7
Ayuob, N. N., Abd El Wahab, M. G., Ali, S. S., and Abdel-Tawab, H. S. (2018). Ocimum basilicum improve chronic stress-induced neurodegenerative changes in mice hippocampus. Metab. Brain Dis. 33, 795–804. doi: 10.1007/s11011-017-0173-3
Ayuob, N. N., Ali, S. S., Suliaman, M., El Wahab, M. G. A., and Ahmed, S. M. (2016). The antidepressant effect of musk in an animal model of depression: a histopathological study. Cell Tissue Res. 366, 271–284. doi: 10.1007/s00441-016-2468-9
Ayuob, N. N., Balgoon, M. J., Ali, S., Alnoury, I. S., Almohaimeed, H. M., and Abdelfattah, A. A. (2020). Ocimum basilicum (basil) modulates apoptosis and neurogenesis in olfactory pulp of mice exposed to chronic unpredictable mild stress. Front. Psychiatry 11:569711. doi: 10.3389/fpsyt.2020.569711
Bikmoradi, A., Seifi, Z., Poorolajal, J., Araghchian, M., Safiaryan, R., and Oshvandi, K. (2015). Effect of inhalation aromatherapy with lavender essential oil on stress and vital signs in patients undergoing coronary artery bypass surgery: a single-blinded randomized clinical trial. Complement Ther. Med. 23, 331–338. doi: 10.1016/j.ctim.2014.12.001
Boldrini, M., Underwood, M. D., Hen, R., Rosoklija, G. B., Dwork, A. J., John Mann, J., et al. (2009). Antidepressants increase neural progenitor cells in the human hippocampus. Neuropsychopharmacology 34, 2376–2389. doi: 10.1038/npp.2009.75
Caiaffo, V., Oliveira, B. D. R., De Sá, F. B., and Evencio Neto, J. (2016). Anti-inflammatory, antiapoptotic, and antioxidant activity of fluoxetine. Pharmacol. Res. Perspect. 4:e00231.
CB Filho, J. C., Donato, F., Giacomeli, R., Del Fabbro, L., Da Silva Antunes, M., De Gomes, M., et al. (2015). Chronic unpredictable mild stress decreases BDNF and NGF levels and Na (+), K (+)-ATPase activity in the hippocampus and prefrontal cortex of mice: antidepressant effect of chrysin. Neuroscience 289, 367–380. doi: 10.1016/j.neuroscience.2014.12.048
Cersosimo, M. G. (2018). Propagation of alpha-synuclein pathology from the olfactory bulb: possible role in the pathogenesis of dementia with Lewy bodies. Cell Tissue Res. 373, 233–243. doi: 10.1007/s00441-017-2733-6
Chen, X., Shen, J., Zhao, J.-M., Guan, J., Li, W., Xie, Q.-M., et al. (2020). Cedrol attenuates collagen-induced arthritis in mice and modulates the inflammatory response in LPS-mediated fibroblast-like synoviocytes. Food Funct. 11, 4752–4764. doi: 10.1039/d0fo00549e
Cheng, K., Li, J., Yang, D., Yang, Y., Rao, C., Zhang, S., et al. (2016). 2D-gel based proteomics unravels neurogenesis and energetic metabolism dysfunction of the olfactory bulb in CUMS rat model. Behav. Brain Res. 313, 302–309. doi: 10.1016/j.bbr.2016.05.026
Chioca, L. R., Ferro, M. M., Baretta, I. P., Oliveira, S. M., Silva, C. R., Ferreira, J., et al. (2013). Anxiolytic-like effect of lavender essential oil inhalation in mice: participation of serotonergic but not GABAA/benzodiazepine neurotransmission. J. Ethnopharmacol. 147, 412–418. doi: 10.1016/j.jep.2013.03.028
Dong, J., Li, H., Bai, Y., and Wu, C. (2019). Muscone ameliorates diabetic peripheral neuropathy through activating AKT/mTOR signalling pathway. J. Pharmacy Pharmacol. 71, 1706–1713. doi: 10.1111/jphp.13157
Doron, R., Lotan, D., Einat, N., Yaffe, R., Winer, A., Marom, I., et al. (2014). A novel herbal treatment reduces depressive-like behaviors and increases BDNF levels in the brain of stressed mice. Life Sci. 94, 151–157. doi: 10.1016/j.lfs.2013.10.025
Elsharkawy, E., Elshathely, M., Jaleel, G. A., and Al-Johar, H. I. (2013). Anti-inflammatory effects of medicinal plants mixture used by Bedouin people in Saudi Arabia. Herba Polonica 59, 76–87. doi: 10.2478/hepo-2013-0018
Finnell, J. E., Lombard, C. M., Padi, A. R., Moffitt, C. M., Wilson, L. B., Wood, C. S., et al. (2017). Physical versus psychological social stress in male rats reveals distinct cardiovascular, inflammatory and behavioral consequences. PLoS One 12:e0172868. doi: 10.1371/journal.pone.0172868
Fleming, S. M., Tetreault, N. A., Mulligan, C. K., Hutson, C. B., Masliah, E., and Chesselet, M. F. (2008). Olfactory deficits in mice overexpressing human wildtype α−synuclein. Eur. J. Neurosci. 28, 247–256. doi: 10.1111/j.1460-9568.2008.06346.x
Freitas, A. E., Bettio, L. E., Neis, V. B., Santos, D. B., Ribeiro, C. M., Rosa, P. B., et al. (2014). Agmatine abolishes restraint stress-induced depressive-like behavior and hippocampal antioxidant imbalance in mice. Prog. Neuropsychopharmacol. Biol. Psychiatry 50, 143–150. doi: 10.1016/j.pnpbp.2013.12.012
Fristiyohadi, A., Wahyuni, W., Kalimin, W. O., Permana, L. O., Saripuddin, S., and Sahidin, I. (2019). Anti-inflammatory activity of marine sponge aaptos sp. to the plasma interleukin-1β level in wistar male rats. Pharmacol. Clin. Pharmacy Res. 4, 35–39. doi: 10.15416/pcpr.v4i2.24269
Fudge, J. L., and Tucker, T. (2009). Amygdala projections to central amygdaloid nucleus subdivisions and transition zones in the primate. Neuroscience 159, 819–841. doi: 10.1016/j.neuroscience.2009.01.013
Fukayama, M. Y., Easterday, O. D., Serafino, P. A., Renskers, K. J., North-Root, H., and Schrankel, K. R. (1999). Subchronic inhalation studies of complex fragrance mixtures in rats and hamsters. Toxicol. Lett. 111, 175–187. doi: 10.1016/s0378-4274(99)00179-4
Gamal, M., Moawad, J., Rashed, L., Morcos, M. A., and Sharawy, N. (2018). Possible involvement of tetrahydrobiopterin in the disturbance of redox homeostasis in sepsis–induced brain dysfunction. Brain Res. 1685, 19–28. doi: 10.1016/j.brainres.2018.02.008
Gu, Y., Dee, C. M., and Shen, J. (2011). Interaction of free radicals, matrix metalloproteinases and caveolin-1 impacts blood-brain barrier permeability. Front. Biosci. 3:1216–1231. doi: 10.2741/s222
Jacob, S., Garcia, S., Hayreh, D., and Mcclintock, M. K. (2002). Psychological effects of musky compounds: comparison of androstadienone with androstenol and muscone. Hormones Behav. 42, 274–283. doi: 10.1006/hbeh.2002.1826
Jacob, S., Hayreh, D. J., and Mcclintock, M. K. (2001). Context-dependent effects of steroid chemosignals on human physiology and mood. Physiol. Behav. 74, 15–27. doi: 10.1016/s0031-9384(01)00537-6
Jacob, S., and McClintock, M. K. (2000). Psychological state and mood effects of steroidal chemosignals in women and men. Hormones Behav. 37, 57–78. doi: 10.1006/hbeh.1999.1559
Javed, H., Azimullah, S., Abul Khair, S. B., Ojha, S., and Haque, M. E. (2016). Neuroprotective effect of nerolidol against neuroinflammation and oxidative stress induced by rotenone. BMC Neurosci. 17:58.
Jeong, H.-J., Kim, J.-H., Kim, N.-R., Yoou, M.-S., Nam, S.-Y., Kim, K.-Y., et al. (2015). Antidepressant effect of stillenTM. Arch. Pharmacal. Res. 38, 1223–1231. doi: 10.1007/s12272-014-0472-8
Jie, H., Zhao, G. J., Zeng, D. J., Feng, X. L., Zhang, C. L., Liang, Z. J., et al. (2019). [Microbial diversity of musk across its maturation process in forest musk deer]. Zhongguo Zhong Yao Za Zhi 44, 4448–4453.
Kadhim, M. J., Al-Rubaye, A. F., and Hameed, I. H. (2017). Determination of bioactive compounds of methanolic extract of vitis vinifera using GC-MS. Int. J. Toxicol. Pharmacol. Res. 9, 113–126.
Kikuta, S., Fletcher, M. L., Homma, R., Yamasoba, T., and Nagayama, S. (2013). Odorant response properties of individual neurons in an olfactory glomerular module. Neuron 77, 1122–1135. doi: 10.1016/j.neuron.2013.01.022
Kim, N.-R., Kim, H.-Y., Kim, M.-H., Kim, H.-M., and Jeong, H.-J. (2016). Improvement of depressive behavior by Sweetme Sweet PumpkinTM and its active compound, β-carotene. Life Sci. 147, 39–45. doi: 10.1016/j.lfs.2016.01.036
Kostadinov, I., Delev, D., Petrova, A., Stanimirova, I., Draganova, K., Kruzliak, P., et al. (2015). Study on anti-inflammatory and immunomodulatory effects of fluoxetine in rat models of inflammation. Eur. J. Inflam. 13, 173–182. doi: 10.1177/1721727x15618671
Lee, C.-L., Hwang, T.-L., Yang, J.-C., Cheng, H.-T., He, W.-J., Yen, C.-T., et al. (2014). Anti-inflammatory spirostanol and furostanol saponins from Solanum macaonense. J. Nat. Products 77, 1770–1783. doi: 10.1021/np500057b
Lee, D., Kim, Y. S., Song, J., and Kim, H. (2019). Neuroprotective effects of musk of muskrat on transient focal cerebral ischemia in rats. Evid Based Complement Alternat Med. 2019:9817949.
Li, L. F., Yang, J., Ma, S. P., and Qu, R. (2013). Magnolol treatment reversed the glial pathology in an unpredictable chronic mild stress-induced rat model of depression. Eur. J. Pharmacol. 711, 42–49. doi: 10.1016/j.ejphar.2013.04.008
Li, M., Fu, Q., Li, Y., Li, S., Xue, J., and Ma, S. (2014). Emodin opposes chronic unpredictable mild stress induced depressive-like behavior in mice by upregulating the levels of hippocampal glucocorticoid receptor and brain-derived neurotrophic factor. Fitoterapia 98, 1–10. doi: 10.1016/j.fitote.2014.06.007
Li, Y., Zhang, T., Qi, L., Yang, S., Xu, S., Cha, M., et al. (2018). Microbiota changes in the musk gland of male forest musk deer during musk maturation. Front. Microbiol. 9:3048.
Liu, D., Xie, K., Yang, X., Gu, J., Ge, L., Wang, X., et al. (2014). Resveratrol reverses the effects of chronic unpredictable mild stress on behavior, serum corticosterone levels and BDNF expression in rats. Behav. Brain Res. 264, 9–16. doi: 10.1016/j.bbr.2014.01.039
Liu, L., Guo, Y. H., Xin, H. L., Nie, Y., Han, T., Qin, L. P., et al. (2012). Antiosteoporotic effects of benzyl benzoate glucosides from Curculigo orchioides in Ovariectomized rats. Zhong Xi Yi Jie He Xue Bao 10, 1419–1426. doi: 10.3736/jcim20121214
Liu, T., Zhong, S., Liao, X., Chen, J., He, T., Lai, S., et al. (2015). A meta-analysis of oxidative stress markers in depression. PLoS One 10:e0138904. doi: 10.1371/journal.pone.0138904
Liu, Z., Zhou, T., Ziegler, A. C., Dimitrion, P., and Zuo, L. (2017). Oxidative stress in neurodegenerative diseases: from molecular mechanisms to clinical applications. Oxid. Med. Cell. Longev. 2017:2525967.
Marsden, W. N. (2013). Synaptic plasticity in depression: molecular, cellular and functional correlates. Prog. Neuropsychopharmacol. Biol. Psychiatry 43, 168–184. doi: 10.1016/j.pnpbp.2012.12.012
Menard, C., Pfau, M. L., Hodes, G. E., and Russo, S. J. (2017). Immune and neuroendocrine mechanisms of stress vulnerability and resilience. Neuropsychopharmacology 42, 62–80. doi: 10.1038/npp.2016.90
Meyer, A., Glaser, A., Brauer, A. U., Wree, A., Strotmann, J., Rolfs, A., et al. (2018). Olfactory performance as an indicator for protective treatment effects in an animal model of neurodegeneration. Front. Integr. Neurosci. 12:35.
Mineur, Y. S., Belzung, C., and Crusio, W. E. (2006). Effects of unpredictable chronic mild stress on anxiety and depression-like behavior in mice. Behav. Brain Res. 175, 43–50. doi: 10.1016/j.bbr.2006.07.029
Nabavi, S. F., Braidy, N., Habtemariam, S., Orhan, I. E., Daglia, M., Manayi, A., et al. (2015). Neuroprotective effects of chrysin: from chemistry to medicine. Neurochem. Int. 90, 224–231. doi: 10.1016/j.neuint.2015.09.006
Nakagawasai, O., Yamada, K., Odaira, T., Takahashi, K., Nemoto, W., Sakuma, W., et al. (2020). Liver hydrolysate improves depressive-like behavior in olfactory bulbectomized mice: Involvement of hippocampal neurogenesis through the AMPK/BDNF/CREB pathway. J. Pharmacol. Sci. 143, 52–55. doi: 10.1016/j.jphs.2020.01.002
Negoias, S., Croy, I., Gerber, J., Puschmann, S., Petrowski, K., Joraschky, P., et al. (2010). Reduced olfactory bulb volume and olfactory sensitivity in patients with acute major depression. Neuroscience 169, 415–421. doi: 10.1016/j.neuroscience.2010.05.012
Nogueira Neto, J. D., De Almeida, A. A. C., Da Silva Oliveira, J., Dos Santos, P. S., De Sousa, D. P., and De Freitas, R. M. (2013). Antioxidant effects of nerolidol in mice hippocampus after open field test. Neurochem. Res. 38, 1861–1870. doi: 10.1007/s11064-013-1092-2
Norata, G. D., Cattaneo, P., Poletti, A., and Catapano, A. L. (2010). The androgen derivative 5α-androstane-3β, 17β-diol inhibits tumor necrosis factor α and lipopolysaccharide induced inflammatory response in human endothelial cells and in mice aorta. Atherosclerosis 212, 100–106. doi: 10.1016/j.atherosclerosis.2010.05.015
Odaira, T., Nakagawasai, O., Takahashi, K., Nemoto, W., Sakuma, W., Lin, J.-R., et al. (2019). Mechanisms underpinning AMP-activated protein kinase-related effects on behavior and hippocampal neurogenesis in an animal model of depression. Neuropharmacology 150, 121–133. doi: 10.1016/j.neuropharm.2019.03.026
Patel, R. M., and Pinto, J. M. (2014). Olfaction: anatomy, physiology, and disease. Clin. Anatomy 27, 54–60. doi: 10.1002/ca.22338
Pavesi, E., Canteras, N. S., and Carobrez, A. P. (2011). Acquisition of Pavlovian fear conditioning using β-adrenoceptor activation of the dorsal premammillary nucleus as an unconditioned stimulus to mimic live predator-threat exposure. Neuropsychopharmacology 36, 926–939. doi: 10.1038/npp.2010.231
Pedraz-Petrozzi, B., Neumann, E., and Sammer, G. (2020). Pro-inflammatory markers and fatigue in patients with depression: a case-control study. Sci. Rep. 10, 1–12. doi: 10.1155/2015/758314
Pinheiro, M. L., Ferraz-De-Paula, V., Ribeiro, A., Sakai, M., Bernardi, M. M., and Palermo-Neto, J. (2011). Long-term maternal separation differentially alters serum corticosterone levels and blood neutrophil activity in A/J and C57BL/6 mouse offspring. Neuroimmunomodulation 18, 184–190. doi: 10.1159/000323516
Qi, X., Xu, H., Wang, L., and Zhang, Z. (2018). Comparison of therapeutic effects of TREK1 blockers and fluoxetine on chronic unpredicted mild stress sensitive rats. ACS chemical neuroscience 9, 2824–2831. doi: 10.1021/acschemneuro.8b00225
Radnaa, G., Oyuntsetseg, N., Dagvatseren, B., Bat-Erdene, J., Lkhagvasuren, E., and Chimedragchaa, C. (2020). The effect of musk on neurons in the pattern of ischemic stroke. Central Asian J. Med. Sci. 6, 66–73.
Rey, N. L., Wesson, D. W., and Brundin, P. (2018). The olfactory bulb as the entry site for prion-like propagation in neurodegenerative diseases. Neurobiol. Dis. 109, 226–248. doi: 10.1016/j.nbd.2016.12.013
Sarafoleanu, C., Mella, C., Georgescu, M., and Perederco, C. (2009). The importance of the olfactory sense in the human behavior and evolution. J. Med. Life 2, 196–198.
Sing, P., and Paul, K. (2006). A simple synthesis of 5-spirobarbituric acids and transformations of spirocyclopropane barbiturates to 5-substitutated barbiturates. Indian J. Chem. B 45, 247–251.
Siopi, E., Denizet, M., Gabellec, M. M., De Chaumont, F., Olivo-Marin, J. C., Guilloux, J. P., et al. (2016). Anxietyand depression-like states lead to pronounced olfactory deficits and impaired adult neurogenesis in mice. J. Neurosci. 36, 518–531. doi: 10.1523/jneurosci.2817-15.2016
Sokmen, B. B., Ugras, S., Sarikaya, H. Y., Ugras, H. I., and Yanardag, R. (2013). Antibacterial, antiurease, and antioxidant activities of some arylidene barbiturates. Appl. Biochem. Biotechnol. 171, 2030–2039. doi: 10.1007/s12010-013-0486-6
Sorce, S., and Krause, K.-H. (2009). NOX enzymes in the central nervous system: from signaling to disease. Antiox. Redox Sign. 11, 2481–2504. doi: 10.1089/ars.2009.2578
Sriramavaratharajan, V., and Murugan, R. (2018). Chemical profile of leaf essential oil of Cinnamomum walaiwarense and comparison of its antioxidant and hypoglycemic activities with the major constituent benzyl benzoate. Nat. Product Commun. 13, 1934578X1801300633.
Surinkaew, P., Sawaddiruk, P., Apaijai, N., Chattipakorn, N., and Chattipakorn, S. C. (2018). Role of microglia under cardiac and cerebral ischemia/reperfusion (I/R) injury. Metab. Brain Dis. 33, 1019–1030. doi: 10.1007/s11011-018-0232-4
Sytniczuk, A., Leszczynska, A., Kajetanowicz, A., and Grela, K. (2018). Preparation of musk-smelling macrocyclic lactones from biomass: looking for the optimal substrate combination. ChemSusChem 11, 3157–3166. doi: 10.1002/cssc.201801463
Taraz, M., Taraz, S., and Dashti-Khavidaki, S. (2015). Association between depression and inflammatory/anti-inflammatory cytokines in chronic kidney disease and end-stage renal disease patients: a review of literature. Hemodialysis Int. 19, 11–22. doi: 10.1111/hdi.12200
Tillett, J., and Ames, D. (2010). The uses of aromatherapy in women’s health. J. Perinat. Neonatal. Nurs. 24, 238–245. doi: 10.1097/jpn.0b013e3181ece75d
Tong, J., Zhou, Z., Qi, W., Jiang, S., Yang, B., Zhong, Z., et al. (2019). Antidepressant effect of helicid in chronic unpredictable mild stress model in rats. Int. Immunopharmacol. 67, 13–21. doi: 10.1016/j.intimp.2018.11.052
Varsha, K. K., Devendra, L., Shilpa, G., Priya, S., Pandey, A., and Nampoothiri, K. M. (2015). 2, 4-di-tert-butyl phenol as the antifungal, antioxidant bioactive purified from a newly isolated Lactococcus sp. Int. J. Food Microbiol. 211, 44–50. doi: 10.1016/j.ijfoodmicro.2015.06.025
Walf, A. A., and Frye, C. A. (2007). The use of the elevated plus maze as an assay of anxiety-related behavior in rodents. Nat. Protocols 2, 322–328. doi: 10.1038/nprot.2007.44
Walker, J. C., Hall, S. B., Walker, D. B., Kendal-Reed, M. S., Hood, A. F., and Niu, X.-F. (2003). Human odor detectability: new methodology used to determine threshold and variation. Chem. Senses 28, 817–826. doi: 10.1093/chemse/bjg075
Wang, B., Cao, H., Shen, T., Li, M., Li, Y. L., Cui, C. L., et al. (2015). Mechanism of musk and borneol on inflammatory of cerebral ischemia and reperfusion injury at different time points of acute phase in rats. Zhong Yao Cai 38, 2139–2143.
Wei, G., Chen, D. F., Lai, X. P., Liu, D. H., Deng, R. D., Zhou, J. H., et al. (2012). Muscone exerts neuroprotection in an experimental model of stroke via inhibition of the fas pathway. Nat. Prod. Commun. 7, 1069–1074.
WHO (2020). Who Health Organization. Available online at: https://www.who.int/news-room/fact-sheets/detail/depression (accessed January 7, 2021).
Yang, D., Li, Q., Fang, L., Cheng, K., Zhang, R., Zheng, P., et al. (2011a). Reduced neurogenesis and pre-synaptic dysfunction in the olfactory bulb of a rat model of depression. Neuroscience 192, 609–618. doi: 10.1016/j.neuroscience.2011.06.043
Yang, D., Liu, X., Zhang, R., Cheng, K., Mu, J., Fang, L., et al. (2011b). Increased apoptosis and different regulation of pro-apoptosis protein bax and anti-apoptosis protein bcl-2 in the olfactory bulb of a rat model of depression. Neurosci. Lett. 504, 18–22. doi: 10.1016/j.neulet.2011.08.046
Yoo, H. S., Jeon, S., Chung, S. J., Yun, M., Lee, P. H., Sohn, Y. H., et al. (2018). Olfactory dysfunction in Alzheimer’s disease- and lewy body-related cognitive impairment. Alzheimers Dement 14, 1243–1252. doi: 10.1016/j.jalz.2018.05.010
Yoshiyama, K., Arita, H., and Suzuki, J. (2015). The effect of aroma hand massage therapy for people with dementia. J. Altern. Complement Med. 21, 759–765. doi: 10.1089/acm.2015.0158
Zhang, C., Liao, Y., Liu, L., Sun, Y., Lin, S., Lan, J., et al. (2020). A Network pharmacology approach to investigate the active compounds and mechanisms of musk for ischemic stroke. Evid Based Complement Alternat Med. 2020:4063180.
Zheng, R., Zhang, Z. H., Zhao, Y. X., Chen, C., Jia, S. Z., Cao, X. C., et al. (2019). Transcriptomic insights into the response of the olfactory bulb to selenium treatment in a mouse model of Alzheimer’s disease. Int. J. Mol. Sci. 20:2998. doi: 10.3390/ijms20122998
Zhou, L.-Y., Yao, M., Tian, Z.-R., Liu, S.-F., Song, Y.-J., Ye, J., et al. (2020). Muscone suppresses inflammatory responses and neuronal damage in a rat model of cervical spondylotic myelopathy by regulating Drp1-dependent mitochondrial fission. J. Neurochem. 155, 154–176. doi: 10.1111/jnc.15011
Keywords: musk, olfactory bulb, depression, caspase-3, neurogenesis, GFAP, chronic stress
Citation: Almohaimeed HM, Batawi AH, Mohammedsaleh ZM, Al Jaouni S, Mutlq Alsawat SA, Abd El Wahab MG, AbdElfattah AA and Ayuob NN (2021) Musk (Moschus moschiferus) Attenuates Changes in Main Olfactory Bulb of Depressed Mice: Behavioral, Biochemical, and Histopathological Evidence. Front. Behav. Neurosci. 15:704180. doi: 10.3389/fnbeh.2021.704180
Received: 01 May 2021; Accepted: 29 July 2021;
Published: 27 August 2021.
Edited by:
Matthew J. Robson, University of Cincinnati, United StatesReviewed by:
Shiho Kitaoka, Hyogo College of Medicine, JapanAnilkumar Pillai, University of Texas Health Science Center at Houston, United States
Copyright © 2021 Almohaimeed, Batawi, Mohammedsaleh, Al Jaouni, Mutlq Alsawat, Abd El Wahab, AbdElfattah and Ayuob. This is an open-access article distributed under the terms of the Creative Commons Attribution License (CC BY). The use, distribution or reproduction in other forums is permitted, provided the original author(s) and the copyright owner(s) are credited and that the original publication in this journal is cited, in accordance with accepted academic practice. No use, distribution or reproduction is permitted which does not comply with these terms.
*Correspondence: Nasra N. Ayuob, nasraayuob@gmail.com; nasraayuob@du.edu.eg