- 1Laboratory of Hormonal Measurement, Department of Physiology and Behavior, Federal University of Rio Grande do Norte, Natal, Brazil
- 2Postgraduate Program in Psychobiology, Federal University of Rio Grande do Norte, Natal, Brazil
- 3Brain Institute, Federal University of Rio Grande do Norte, Natal, Brazil
- 4Onofre Lopes University Hospital, Federal University of Rio Grande do Norte, Natal, Brazil
- 5National Science and Technology Institute for Translational Medicine (INCT-TM), Natal, Brazil
- 6Department of Clinical Medicine, Federal University of Rio Grande do Norte, Natal, Brazil
- 7Department of Biophysics and Pharmacology, Federal University of Rio Grande do Norte, Natal, Brazil
Sleep disturbance is a symptom consistently found in major depression and is associated with a longer course of illness, reduced response to treatment, increased risk of relapse and recurrence. Chronic insomnia has been associated with changes in cortisol and serum brain-derived neurotrophic factor (BDNF) levels, which in turn are also changed in major depression. Here, we evaluated the relationship between sleep quality, salivary cortisol awakening response (CAR), and serum BDNF levels in patients with sleep disturbance and treatment-resistant major depression (n = 18), and in a control group of healthy subjects with good (n = 21) and poor (n = 18) sleep quality. We observed that the patients had the lowest CAR and sleep duration of all three groups and a higher latency to sleep than the healthy volunteers with a good sleep profile. Besides, low CAR was correlated with more severe depressive symptoms and worse sleep quality. There was no difference in serum BDNF levels between groups with distinct sleep quality. Taken together, our results showed a relationship between changes in CAR and in sleep quality in patients with treatment-resistant depression, which were correlated with the severity of disease, suggesting that cortisol could be a physiological link between sleep disturbance and major depression.
Introduction
Changes in quality, duration, and structure of sleep are frequently observed in patients with mood disorders. In major depression, sleep alterations are part of the diagnosis, and are associated with depressed mood and loss of interest or pleasure (Sadock et al., 2016). Studies have pointed out that sleep problems usually precede other symptoms of depression and that the quality of sleep worsens before the onset of the first depressive episode (Breslau et al., 1996; Perlis et al., 1997; Ohayon and Roth, 2003). Moreover, during a depressive episode, persistent sleep disturbances are associated with more severe symptoms, worse treatment outcomes, increased risk of relapse and recurrence, and longer course of illness (Moos and Cronkite, 1999).
Among different sleep disorders, insomnia is the most frequent in patients with major depression (Harvey, 2001; Lucchesi et al., 2005). Normally, the diagnosis of insomnia is based on the difficulty to initiate or maintain sleep, which in turn results in reduced duration and quality of sleep (Schutte-Rodin et al., 2008). Some patients with depression do not present a clinical diagnosis of insomnia but show frequent nocturnal arousals, reduction in total sleep duration, non-restorative sleep, or disruptive dreams, often associated with suicidal ideation (Agargun et al., 2003; Chellappa and Araujo, 2007b). On the other hand, about 10–20% of patients with depression present hypersomnia, which is characterized by increases in night sleep duration and excessive daytime sleepiness (Pelayo, 2014).
Specific neurotransmitters and hormones, such as serotonin (Ursin, 2002), histamine and adenosine (Huang et al., 2007), cortisol (Weitzman et al., 1971; Steiger, 2003), and melatonin (Weinberg et al., 1979; Dubocovich, 2007) are associated with the modulation of sleep. The hypothalamus-pituitary-adrenal (HPA) axis plays an important role in the sleep–wake cycle. Cortisol, the final hormone of the HPA axis, presents a circadian rhythm with maximal secretion in the morning, before arousal, and lower levels at the end of the day, which are important for sleep onset (Born and Fehm, 2000; Palma et al., 2007; Gotlieb et al., 2018).
HPA axis dysregulation is commonly associated with sleep disorders (Chrousos et al., 1993; Tsuchiyama et al., 1995). Chronic insomnia is associated with circadian cortisol changes and hypercortisolemia, mainly in the evening (Vargas et al., 2018). However, enough homeostatic cortisol level has an important role in the induction and maintenance of rapid eye movement (REM) sleep (García-Borreguero et al., 2000). Moreover, hypersecretion of the corticotrophin-releasing factor (CRF)—a hormone that starts HPA axis function—is also related to sleep changes (Held et al., 2004; Holsboer and Ising, 2008).
In major depression, large changes in the HPA axis are related with chronic stress, depression severity, and duration of illness (Bhagwagar et al., 2005; Bremmer et al., 2007; Mayer et al., 2018). Increased cortisol awakening response (CAR) and hypercortisolemia are the most often reported changes in the HPA axis of patients with major depression, suggesting hyperactivity of this axis. This change could be in part due to a weak feedback sensibility and consequently CRF hypersecretion (Bhagwagar et al., 2005; Gillespie and Nemeroff, 2005; Holsboer and Ising, 2008). However, some depressive patients also have blunted CAR and hypocortisolemia (Huber et al., 2006; Bremmer et al., 2007). Both cortisol and CRF are linked with monoaminergic systems, and their changes could be related to the reduction of monoamines in the synaptic cleft, the main theory of major depression physiopathology (Steckler et al., 1999; Jeon and Kim, 2016). Therefore, since homeostatic cortisol levels and its circadian oscillations seem to be essential to a good sleep function, cortisol changes have been studied as a common physiological marker between major depression and sleep disorders (Vargas et al., 2018).
Moreover, brain-derived neurotrophic factor (BDNF), a neurotrophin associated with neuroplasticity, has recently become recognized as an indicator of sleep quality (Giese et al., 2014). Serum BDNF levels are often reduced in sleep disorders, as insomnia (Fan et al., 2018; Mikoteit et al., 2019), probably due to increased stress, which in turn reduces neurotrophic factor levels (Giese et al., 2013). The homeostatic level of cortisol is necessary for appropriate BDNF function, but higher levels of cortisol during chronic stress biding to glucocorticoid receptors reduces BDNF expression (Hansson et al., 2000; Kino et al., 2010). However, in vivo, this biphasic regulation may be more complex and depends on the brain area: in the amygdala, for instance, high levels of cortisol induce neurogenesis (Adzic et al., 2009).
BDNF has been considered an important biomarker of major depression (Polyakova et al., 2015; Peng et al., 2018). Preclinical and clinical studies of depressive patients have found low levels of blood BDNF, mainly in non-treated patients, when compared with those of healthy volunteers, and changes in BDNF levels are related to treatment response (Kreinin et al., 2015).
Despite some studies suggesting that patients with major depression have alterations in cortisol and BDNF levels (Leuchter et al., 2010; Tadić et al., 2011; Herbert, 2013), there is no consensus (Pariante et al., 2004; Molendijk et al., 2011; Elfving et al., 2012; Papakostas et al., 2013). To help clarify this issue, here we evaluated the relationship between sleep quality and disturbances, salivary CAR, and serum BDNF levels in patients with treatment-resistant major depression, compared with healthy volunteers. We hypothesize that patients will present alterations in salivary CAR and serum BDNF, which will be positively correlated with depression severity and sleep disturbances.
Materials and Methods
This study was approved by the Research Ethics Committee of the Onofre Lopes University Hospital (HUOL), Federal University of Rio Grande do Norte (UFRN), Natal, RN, Brazil (#579.479). All volunteers agreed to and signed the informed consent form prior to participation. The procedures of this study comply with the ethical standards of the relevant national and institutional committees for human experimentation and with the Declaration of Helsinki of 1975, revised in 2008.
All patients presented treatment-resistant major depression (MD group), defined here as at least two unsuccessful treatments with commercially available antidepressants from different classes. Patients (MD; 4 men and 14 women) were in a currently moderate-to-severe depressive episode during the study, according to the Hamilton Depression Scale (HAM-D; Hamilton, 1960) and presented sleep disturbances, according to the Pittsburgh Sleep Quality Index (PSQI).
Although the HPA axis and BDNF synthesis are not the main targets for antidepressants actions, they seem to modulate these two pathways (Schüle et al., 2006; Schüle, 2007; Wilkinson et al., 2018). Therefore, we required to all patients complete an average of 2-week washout period, adjusted to the half-life time of their current medications. Therefore patients were not taking antidepressants during this study. However, they were allowed to use benzodiazepines if experiencing withdrawal symptoms.
The control group of healthy volunteers (CG; 17 men and 22 women) had no previously diagnosed neurological or psychiatric disorders and did not present sleep disturbances according to the PSQI.
Patients and healthy individuals were matched by age and socio-demographic criteria. The following inclusion criteria were adopted for both groups: minimum of 18 years of age and absence of a present or past history of substance addiction. Exclusion criteria included the following: imminent suicidal risk, bipolar affective disorder, presence of psychotic symptoms, history of manic or hypomanic symptoms, and family history of schizophrenia.
Experimental Design
After screening and washout, volunteers were admitted to the hospital research unit for one overnight stay, in which they slept in a room (D1) reserved and prepared for the study. At D1, the Montgomery–Åsberg Depression Scale (MADRS) was applied to all volunteers to assess depression severity (Rush et al., 2004; Howland, 2008; Palhano-Fontes et al., 2019). The PSQI and Epworth Sleepiness Scale (ESS) were applied at D1 before sleep onset. The duration of sleep and the presence of nocturnal awakening were recorded. On the following morning, at awakening around 6 AM, saliva was collected for CAR. Additionally, blood samples were provided for serum BDNF quantification, when all subjects were fasting for at least 8 h.
Sleep Psychometric Instruments
The PSQI is a self-assessment scale that accesses sleep quality from the previous month. The questions measure seven clinical components: subjective sleep quality (C1), latency (C2), duration (C3), sleep efficiency (C4), sleep alterations (C5), medication use (C6), and daytime sleep dysfunction (C7). Overall score ranges from 0 to 21 points, which is classified as good sleep quality (from 0 to 4 points), poor sleep quality (from 5 to 10 points), and sleep disturbance (if above 10 points). Besides total PSQI score, we analyzed sleep latency and duration of sleep, measured by the PSQI.
The ESS was applied to evaluate daytime sleepiness. ESS consists of eight statements rated from 0 to 3. A null score implies no chances of taking a nap, whereas scores of 1, 2, and 3 mean small, moderate and high chance of a nap throughout the day, respectively.
Salivary Cortisol Awakening Response
Saliva was used to analyze the CAR. It was obtained through a Salivette (Sarstedt AG & Co., KG Werk Hemer, Germany), a device specifically used for saliva collection composed of a cotton swab and a plastic tube. Three samples of saliva were collected: immediately at awakening, 30 and 45 min after awakening. Volunteers were not allowed to move excessively, brush their teeth, eat, or drink before or during saliva collection. Saliva samples were centrifuged (15 min, 4°C to 3,000 rpm) and stored in aliquots of 0.300 ml, at −80°C. Salivary cortisol was measured with competitive ELISA kit DGR-SLV 4635.
Serum Brain-Derived Neurotrophic Factor
Blood collection was performed with a disposable syringe by trained professionals from the HUOL clinical analysis laboratory. Blood samples were centrifuged (10 min, 4°C to 3,000 rpm), and the serum samples were stored in aliquots of 0.300 ml at −80°C. BDNF quantification was performed by sandwich ELISA with the Merck Millipore CYT306 kit.
Statistical Analysis
For CAR statistical analysis, the area under the curve (AUC) was calculated in cm3, using the three cortisol concentration measurements. Quantitative dependent variables investigated in this study are as follows: total PSQI score and its seven components, previous month sleep latency and sleep duration measured by the PSQI, daytime sleepiness, sleep duration at D1, CAR, and serum BDNF levels. PSQI, previous month sleep duration, sleep duration at D1, CAR, and serum BDNF levels were normalized by logarithmic transformation. The nocturnal awakening at D1 (present or not) was treated as a categorical dependent variable.
All volunteers (CG and MD) were categorized with respect to sleep quality, according to the PSQI total score, into three groups: good sleep (GS), poor sleep (PS), and sleep disturbance (SD). To analyze the influence of previous antidepressants treatments, patients were split into three groups, which were included as categorical independent variables: the number of unsuccessful previous antidepressant treatments with different drug classes, G1 (two or three previous treatments, n = 10), G2 (four or five previous treatments, n = 5), and G3 (six to nine previous treatments, n = 3). These groups and gender were included as categorical independent variables.
The influence of gender and sleep quality (GS, PS, and SD) on PSQI scores and CAR was explored with a general linear model (GLM) and Fisher post hoc test. For BDNF, we applied the analysis of covariance (ANCOVA) using groups (GS, PS, and SD) and gender as independent variables and the levels of platelets as covariate. A one-way analysis of variance (one-way ANOVA) and a Fisher post hoc test were used to investigate possible differences in sleep duration according to sleep quality (GS, PS, and SD) and to CAR and BDNF levels with respect to the number of unsuccessful previous treatments.
A Student’s t-test was used to investigate possible differences in PSQI, CAR, and BDNF with respect to comorbid personality and anxiety disorders. To analyze daytime sleepiness and previous month sleep latency with respect to group sleep quality (GS, PS, and SD), we applied the Kruskal–Wallis test. The chi-square test was used to cross the nocturnal awakenings at D1 with respect to sleep quality (GS, PS, and SD). Multiple regression was applied to analyze the modulation of the patients’ previous benzodiazepines treatment (yes or no) on CAR and BDNF levels.
Spearman’s correlation was applied to analyze possible correlations between PSQI, and its seven components, previous month sleep latency and sleep duration, daytime sleepiness, sleep duration at D1, CAR and serum BDNF levels, and them with MADRS scores, considering the groups together (GS, PS, and SD).
Statistical analysis was conducted in SPSS 20. Graphics were built in R 3.4.1 (RStudio). The level of significance was set at p ≤ 0.05 (two-tailed).
Results
All volunteers were Brazilians adults (MD, 39.83 ± 11.6 years old; CG, 31.6 ± 9.6 years old), and the majority were women (MD, 78%; CG, 56.4%). Compared with controls, who had higher education (MD, 16.67%; CG, 33.33%), patients had predominantly secondary education (MD, 61.11%; CG, 17.94%) and lower income (MD, 83.33%; CG, 51.28%).
On average, patients presented 8.11 ± 7.10 years of depression, with 13.94 ± 17.07 previous episodes and an average MADRS score of 33.27 ± 6.14 at the time of the study. Patients had been previously treated on average with 4.05 ± 1.98 different types of antidepressant medications.
All patients (100%) had been treated already with a selective serotonin reuptake inhibitor (SSRI), 50% also used tricyclic antidepressants (TCAs), 44.44% used serotonin–norepinephrine reuptake inhibitor (SNRI), and 16.67% had tried norepinephrine–dopamine reuptake inhibitor (NDRI). The percentage of antidepressants used does not add up to 100% owing to the overlapping with previous antidepressant treatments. All patients were using benzodiazepines hypnotics/sedatives (alprazolam, n = 2; bromazepam, n = 2; and diazepam, n = 2) and antiepileptic (clonazepam, n = 12). Nine patients were already treated with benzodiazepines before this study and nine were not.
Pittsburgh Sleep Quality Index
All MD patients showed sleep disturbances. In the control group, 18 individuals had poor sleep quality, while 21 showed good sleep. With respect to gender, 4 men and 14 women showed sleep disturbance; 7 men and 11 women presented poor sleep and 10 men and 11 women had good sleep. The sleep disturbance group presented higher total PSQI scores than did the poor sleep and good sleep groups (GLM; Group*: F = 122.67, df = 2, p < 0.001; Fisher post hoc, SD*PS, p < 0.001; SD*GS, p < 0.001); and the PS group presented higher PSQI scores than the GS (Fisher post hoc, p < 0.001; Figure 1). There were no significant differences observed in PSQI scores with respect to gender and to the interaction between group and gender. For all statistical values of main effects and interactions of GLM and for descriptive statistic, see Supplementary Tables S1, S2.
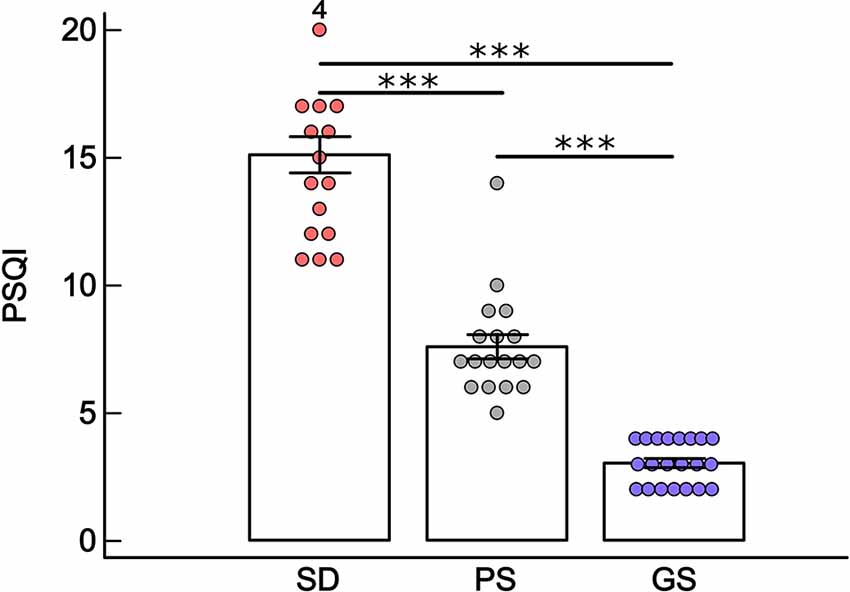
Figure 1. Mean ± standard error of Pittsburgh Sleep Quality Index (PSQI) in the sleep disturbance (SD: n = 18 depressive patients), poor sleep (PS: n = 18 healthy volunteers) and good sleep (GS: n = 21 healthy volunteers) groups. ***p < 0.001, general linear model (GLM) and Fisher post hoc. The number 4 on first bar indicates volunteers that have score higher than 20.
Three patients had comorbid diabetes mellitus, but all showed controlled levels of glycemia (according to reference values) during the study, analyzed by fast glucose levels. Half of the patients had comorbid anxiety disorder (n = 9) and 77% had a comorbid personality disorder (13 patients with personality disorder in cluster B and one patient in cluster A). No significant differences were found in PSQI with respect to comorbid anxiety disorder (Student’s t-test: t = −0.94, df = 16, p = 0.35) and comorbid personality disorder in cluster B (Student’s t-test: t = 0.67, df = 15, p = 0.50; Supplementary Table S3).
The SD group presented higher previous month sleep latency than did the GS group (Kruskal–Wallis; H = 12.67, df = 2, p < 0.001; SD*GS, p < 0.001). A trend toward significance was observed in latency to sleep between the SD and PS groups (p = 0.08) but no significant difference between the GS and PS groups (p = 0.56; Figure 2A, Supplementary Table S2). Two outlier patients in the SD group who showed 3 and 4 h of sleep latency were excluded from the statistical analysis.
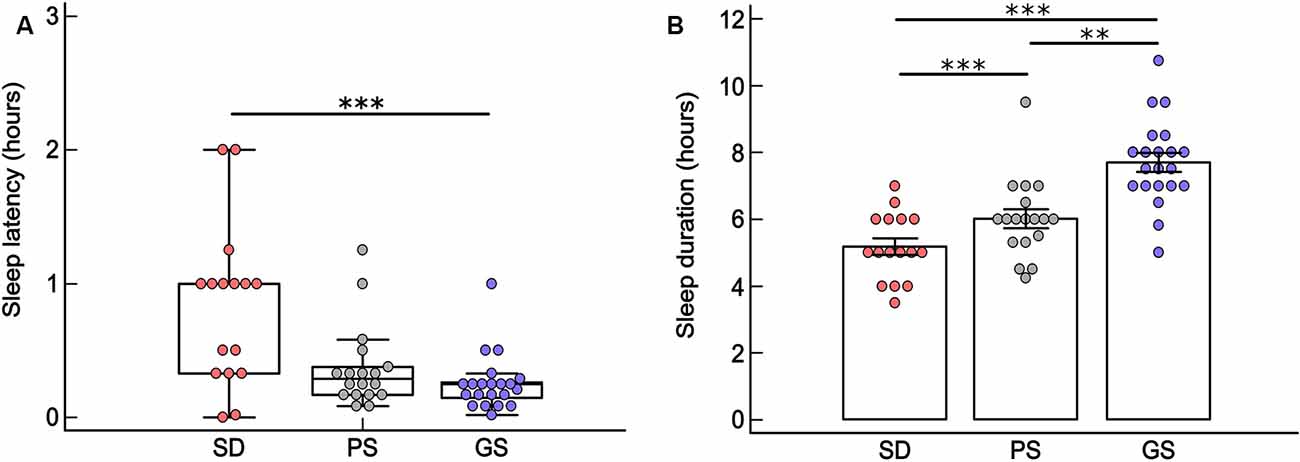
Figure 2. (A) Box plot (median ± Q75% and Q25%) of last month sleep latency (in hours) according to the Pittsburgh Sleep Quality Index (PSQI) in the sleep disturbance (SD: n = 16), poor sleep (PS: n = 18) and good sleep (GS: n = 21) groups. ***p < 0.001, Kruskal–Wallis test. (B) Mean ± standard error of the previous month sleep duration (in hours) according to the PSQI, in SD (n = 16) PS (n = 18), and GS (n = 21) groups. ***p < 0.001 and **p < 0.05 one-way analysis of variance (ANOVA) and Fisher post hoc. Two outlier patients of SD were excluded from both statistical analyses.
The GS group showed longer sleep duration during the previous month than the SD and PS groups (one-way ANOVA: F = 22.02, df = 2, p < 0.001; Fisher post hoc, GS*PS: p < 0.05; GS*SD, p < 0.001). The PS group showed longer sleep duration during the previous month than did the SD group (Fisher post hoc; PS*SD, p < 0.001). Two outlier patients in the SD group who showed 10 and 12 h of sleep duration were excluded from the statistical analysis (Figure 2B, Supplementary Table S2).
Considering all groups together (SD, PS, and GS), significantly positive correlations were found between MADRS scores, and a) PSQI total score (Spearman rho = 0.72, p ≤ 0.05) and b) previous month sleep latency (Spearman rho = 0.52, p ≤ 0.05). Moreover, significant negative correlations were found between MADRS scores and previous month sleep duration (Spearman rho = −0.31, p ≤ 0.05). All results of statistical Spearman correlation are in Table 1.
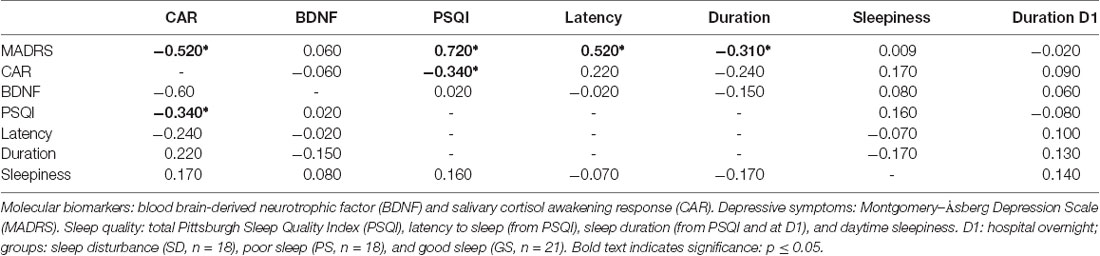
Table 1. Statistical values of Spearman correlation tests between quantitative dependent variables considering all groups together [good sleep (GS), poor sleep (PS) and sleep disturbance (SD)].
Daytime Sleepiness (by Epworth Sleepiness Scale)
No statistically significant differences were observed for daytime sleepiness between groups (Kruskal–Wallis; H = 2.80, df = 2, p = 0.24; Figure 3 and Supplementary Table S2).
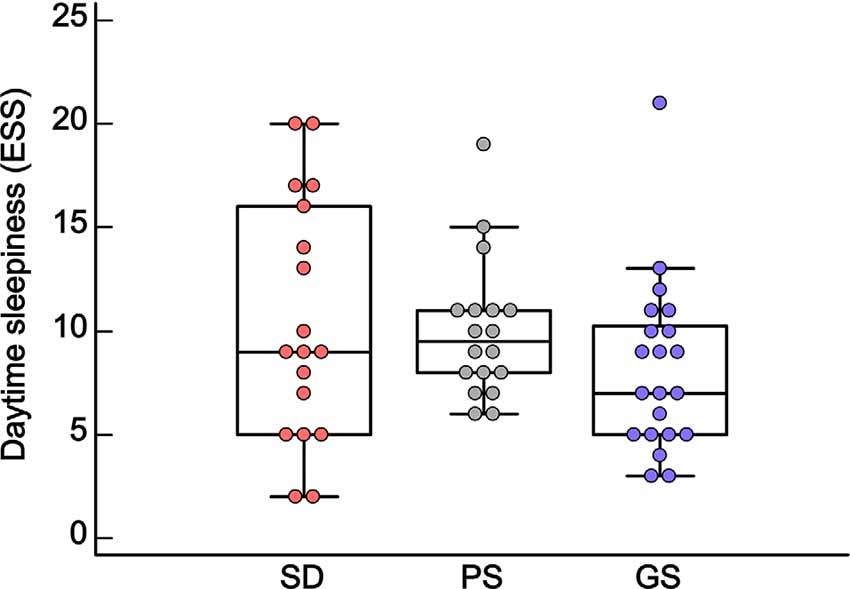
Figure 3. Box plot of daytime sleepiness (median ± Q75% and Q25%), according to the Epworth Sleepiness Scale (ESS), in the sleep disturbance (SD: n = 18), poor sleep (PS: n = 18) and good sleep (GS: n = 21) groups. p ≥ 0.05, Kruskal–Wallis test.
Considering all groups together (SD, PS, and GS), no significant correlations were found between scores of daytime sleepiness and the other quantitative dependent variables: MADRS, CAR, BDNF, PSQI, sleep latency, and sleep duration (Spearman p ≥ 0.05; Table 1).
Sleep Duration at D1
No statistically significant differences were observed regarding sleep duration at D1 between SD, PS, and GS (one-way ANOVA; F = 0.42, df = 2, p = 0.65; Figure 4 and Supplementary Table S2).
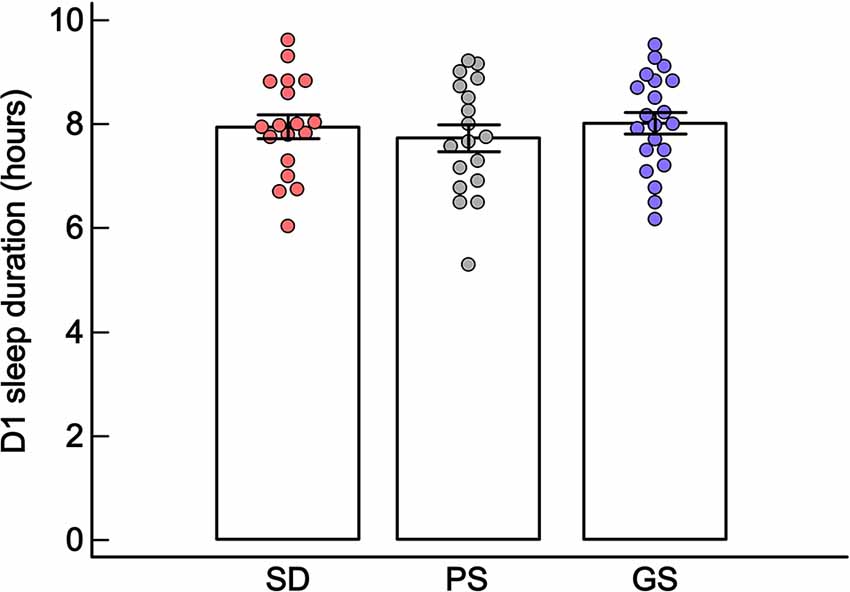
Figure 4. Mean ± standard error of sleep duration (in hours) at D1, in the sleep disturbance (SD: n = 18), poor sleep (PS: n = 18) and good sleep (GS: n = 21) groups. p ≥ 0.05, one-way ANOVA.
Considering all groups together (SD, PS, and GS), no significant correlations were found between sleep duration at D1 and the other quantitative dependent variables: MADRS, CAR, BDNF, PSQI, sleep latency, and sleep duration (Spearman p ≥ 0.05; Table 1).
Nocturnal Awakening at D1
Sleep quality at D1 showed that nocturnal awakening was present in 50% of the individuals with sleep disturbance (n = 9), in 42.85% (n = 9) of the individuals with poor sleep quality, and in 38.8% (n = 7) of the individuals with good sleep quality. No statistically significant differences were observed for nocturnal awakening between groups (chi-square: χ2 = 6.36, p = 1).
Salivary Cortisol Awakening Response
Volunteers with sleep disturbance had lower salivary CAR than subjects with poor sleep (F = 7.31, df = 2, p < 0.001, Fisher post hoc, p < 0.001) and good sleep quality (Fisher post hoc, p < 0.001). There was no difference in CAR between PS and GS (Fisher post hoc, p = 0.69). No differences in CAR were observed with respect to gender and for the interaction between group and gender (Figure 5). For all statistical values of main effects and interactions of GLM and for descriptive statistic, see Supplementary Tables S1, S2.
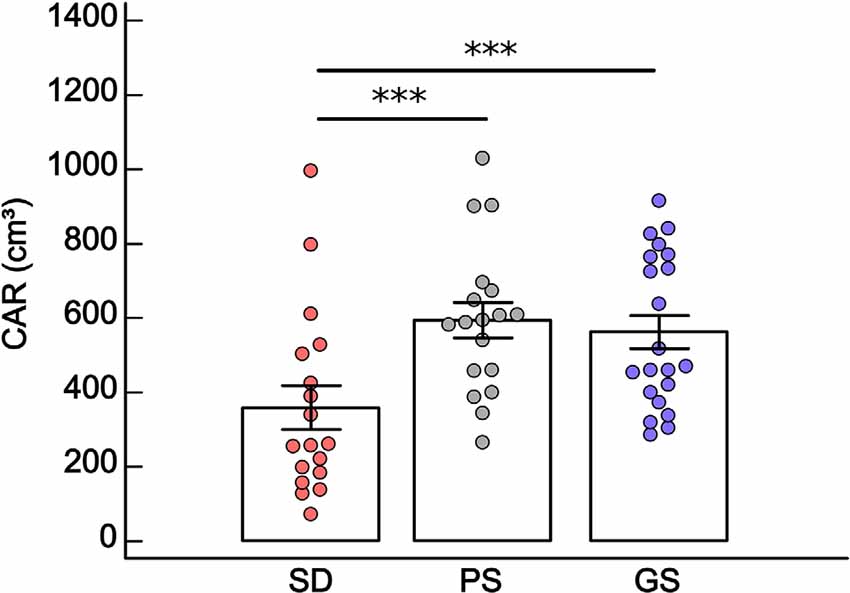
Figure 5. Mean ± standard error of salivary cortisol awakening response (CAR: cm3) in the sleep disturbance (SD: n = 18), poor sleep (PS: n = 18) and good sleep (GS: n = 21) groups. ***p < 0.001, general linear model (GLM) and Fisher post hoc. Two patients in SD showed close values, one of them being superimposed on the graph.
No differences were observed in CAR with respect to the number of previous antidepressant treatments (one-way ANOVA: F = 0.28, df = 2, p = 0.75; G1: n = 10, μ = 354.4 ± 80.9 cm3; G2: n = 5, μ = 296.2 ± 114.5 cm3; G3: n = 3, μ = 479.4 ± 147.8 cm3; Supplementary Figure S1). Moreover, previous treatment with benzodiazepines did not predict CAR (multiple regression: AICC = 198.86, p = 0.08).
No difference was found in CAR between patients with and without comorbid anxiety disorder (t-test: t = −0.12, df = 16, p = 0.90) and comorbid personality disorder (t-test: t = −0.82, df = 15, p = 0.42; Supplementary Figure S2, Supplementary Table S3).
Considering all groups together (GS, PS, and SD), there were significant negative correlations between CAR and MADRS scores (Spearman rho = −0.52, p ≤ 0.05) and PSQI total score (Spearman rho = −0.34, p ≤ 0.05; Table 1). Moreover, negative significant correlations were observed between CAR and some clinical components of the PSQI; subjective sleep quality (C1), sleep efficiency (C4), sleep alterations (C5), and medication use (C6; Supplementary Table S4). Higher scores in these components mean worse sleep quality.
Serum Brain-Derived Neurotrophic Factor Levels
There were no significant differences in BDNF levels between the SD, PS, and GS groups (ANCOVA: F = 0.19, df = 2, p = 0.82). Platelets were statistically significant as covariable (ANCOVA: F = 6.49, df = 1, p = 0.01), and a positive correlation for serum BDNF levels and platelets was observed (Spearman rho = 0.29 p ≤ 0.05). No significant differences were observed in BDNF levels with respect to gender nor for the interaction between group and gender (Figure 6). For all statistical values of main effects and interactions of GLM and for descriptive statistic, see Supplementary Tables S1, S2.
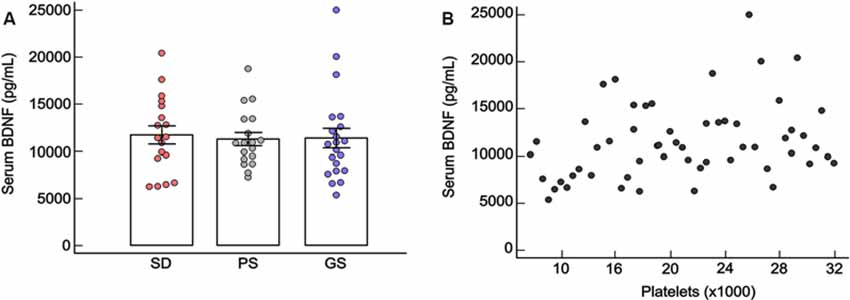
Figure 6. (A) Mean ± standard error of serum blood brain-derived neurotrophic factor (BDNF) levels (pg/ml) in the sleep disturbance (SD: n = 18), poor sleep (PS: n = 18) and good sleep (GS: n = 21). p ≥ 0.05, ANCOVA. (B) Statistically significant Spearman correlation between serum BDNF levels and platelets (rho = 0.29, p ≤ 0.05).
No significant differences were found for BDNF levels with respect to previous antidepressant treatments (one-way ANOVA: F = 2.63, df = 2, p = 0.10; G1: n = 10, μ = 13,355.23 ± 1,967.26 pg/ml; G2: n = 5, μ = 11,222.27 ± 2,782.12 pg/ml; G3: n = 3, μ = 8,648.96 ± 3,591.71 pg/ml; Supplementary Figure S3). Additionally, previous treatments with benzodiazepines did not predict BDNF (multiple regression: AICC = 198.86, p = 0.08).
No differences were observed for BDNF levels between patients with and without comorbid anxiety disorder (t-test: t = −1.13, df = 16, p = 0.27) and comorbid personality disorder (t-test: t = 2.09, df = 15, p = 0.055; Supplementary Figure S4, Supplementary Table S3).
Considering all groups together (GS, PS, and SD), there were no statistically significant correlations to BDNF and CAR, MADRS, or dependent variables of sleep quality (Table 1, Supplementary Table S4).
Discussion
In this study, we observed that all patients with major depression had sleep disturbances, whereas 54% of the healthy controls had poor sleep quality. We found that patients had shorter time of total sleep and higher sleep latency than control volunteers with good sleep quality, which were associated with more severe symptoms of depression. Moreover, patients showed lower salivary CAR than did healthy controls, which was correlated with more severe depressive symptoms and worse sleep quality. Comorbid anxiety and personality disorders did not modulate the sleep quality of the patients, CAR, or BDNF levels. Moreover, the number of unsuccessful previous antidepressant treatments with different drug classes and previous treatments with benzodiazepines were not related with CAR and BDNF levels.
The higher number of women than men in our sample reflects the prevalence of major depression in the general population (Panagiotakopoulos and Neigh, 2014; Kuehner, 2017). However, gender did not modulate salivary CAR nor serum BDNF levels in our sample, although sex hormones have been shown to modulate both BDNF transcription and cortisol secretion (Kudielka and Kirschbaum, 2005; Atwi et al., 2014; Kreinin et al., 2015; Glud et al., 2019). Therefore, the link between these biomarkers, sleep disturbance and major depression, with respect to gender, requires further evaluation (Kreinin et al., 2015; Galvão et al., 2018; Almeida et al., 2019).
Our results show the presence of insomnia in the patients, particularly of initial insomnia, as our patients had higher latency to sleep than healthy controls with good sleep, and the lowest sleep duration with respect to controls with good and poor sleep. These results corroborate the literature that suggests the link between sleep disturbances, mainly insomnia, and major depression (Thase, 1999; Taylor et al., 2005; Alvaro et al., 2013). A previous study assessed 70 patients with major depression (44 women and 26 men) and found out that 70% of them had insomnia, with a significant positive correlation between PSQI scores and depression severity (Chellappa and Araujo, 2007a). Insomnia complaints are frequent in major depression (Taylor et al., 2005; Alvaro et al., 2013; Gebara et al., 2018), especially initial insomnia, which has been correlated with anxiety and personality traits (Chellappa and Araujo, 2007a; Harvey et al., 2014; Van Veen et al., 2017). In fact, most of our patients had comorbid personality disorder and/or anxiety disorder. However, none of these modulated PSQI.
Herein, we did not observe significant differences in daytime sleepiness when comparing patients with sleep disturbance and healthy controls with poor or good sleep quality. Moreover, sleep quality at the night spent in the hospital show that patients with sleep disturbances and healthy controls had similar nocturnal awakenings and sleep duration. Patients’ mean sleep duration was 2 h longer than recorded in the previous month by the PSQI, whereas healthy subjects showed approximately 40 min longer sleep duration. Since most volunteers had low socioeconomic status, the room conditions offered were probably more comfortable than what they had at their homes, which may have influenced positively their sleep duration and quality.
The relationship between sleep disturbances and major depression is complex, mainly in treatment-resistant patients, as is the case of our sample; however, usually, sleep alterations are a core symptom of the disease than a side effect of antidepressant medication (Kurian et al., 2010). Patients with residual symptoms of sleep disorders, as insomnia, have higher chances of recurrence than those without persistent sleep disturbances (Judd et al., 1998; Kurian et al., 2010). Likewise, the absence of response to pharmacological treatment and recurrence are related to chronic sleep disturbances (Fawcett et al., 1990; Buysse et al., 1997, 1999). It is important to highlight that the literature appoints to a good validity of the PSQI when compared with more objective measurements such as polysomnography, which is considered the gold standard in the field (Mollayeva et al., 2016). However, in some cases, subjective changes in sleep have been present even without changes measured by polysomnography (Argyropoulos et al., 2003). Therefore, our findings corroborate the relevance of using subjective sleep evaluation scales, such as the PSQI, as a clinical tool for sleep investigation in major depression, because polysomnography analyses require a sophisticated laboratory setup as well as subjects’ availability to sleep in the study setting.
The sleep–wake cycle is regulated by the interaction between homeostatic and circadian processes (Borb and Achermann, 1999), and changes in these mechanisms can induce sleep-related complaints and disorders. The suprachiasmatic nucleus of the hypothalamus regulates the oscillating pattern of cortisol release, with high levels before awakening and low levels at night. Disturbances in circadian rhythms of cortisol levels can change the 24-h sleep–wake cycle, which in turn has been related to the etiology of mood disorders (Luca et al., 2013). We found lower salivary CAR in the group of patients with sleep disturbances, than in healthy subjects with good and bad sleep quality. No difference was observed in CAR levels between healthy controls with good and bad sleep. Moreover, lower CAR was positively correlated with depression severity and worse sleep quality, inferred by total PSQI score and some of its components such as subjective sleep quality, sleep efficiency, sleep alterations, and medication use.
Some studies showed that sleep disturbances, like insomnia, are correlated with reduced CAR in subjects without psychiatry disorders (Backhaus et al., 2004). Hypocortisolemic addisonian patients treated with hydrocortisone reverted the sleep abnormalities, suggesting that homeostatic cortisol levels have permissive action on sleep (Gan and Pearce, 2016). Despite these associations, it is uncertain whether sleep disturbances alter cortisol levels or vice versa. However, we observed that sleep duration and nocturnal awakenings at D1 did not modulate salivary CAR on the next morning. Studies relating CAR to specific characteristics of the previous night have been very inconsistent (Elder et al., 2016). Some report that acute changes in sleep do not immediately modulate CAR, which tends to follow a more stable pattern of response (Dettenborn et al., 2007; Fries et al., 2009).
Disrupted HPA axis, advancement or delay in circadian rhythm of cortisol, and disturbance in upper and lower bounds of cortisol range are also observed in major depression (Abell et al., 2016; Starr et al., 2017). In some cases, depression is accompanied by hypocortisolemia (Bremmer et al., 2007; Vreeburg et al., 2013; Moreira et al., 2016; Galvão et al., 2018), which may be induced by prolonged use of particular type of antidepressants, such as mirtazapine—a selective antagonism at 5-HT2 receptors (Schüle et al., 2006). In other cases, prolonged exposure to stressors can induce high and chronic reactivity of the HPA axis, followed by adrenal exhaustion (Pariante et al., 2004; Wu et al., 2008; Leuchter et al., 2010). However, as our patients were under washout to antidepressants and the number of unsuccessful previous antidepressant treatments with different drug classes did not modulate CAR, we propose that hypocortisolemia found here is probably a symptom of the disease rather than a result of the antidepressant medication. However, it has been suggested that cortisol changes are a state of major depression, not a trait, owing to its dependence on disease’s phase, duration, and severity (Gillespie and Nemeroff, 2005).
Moreover, it is important to highlight that all patients were under benzodiazepines during this study and that half of them were already using them before the study. However, previous treatment with benzodiazepines did not predict CAR, corroborating some similar studies that did not observe changes in CAR induced by benzodiazepines (McIntyre et al., 1993; Manthey et al., 2010). Benzodiazepine modulation on the HPA axis is conflicting and dependent on the type of drug, dose, type of biological sample, and study design (Laakmann et al., 1984; Kalogeras et al., 1990; Pomara et al., 2005; Fries et al., 2006).
Nevertheless, as the normalization of the HPA function seems to be a prerequisite for the remission of depression, and it is important to sleep homeostasis, new treatments with focus in glucocorticoid stabilization should be stimulated (Steckler et al., 1999; Galvão et al., 2018). Depressive patients treated with antagonist drugs of the CRF receptor showed improvement in depressive symptoms and sleep disorders (Held et al., 2004; Holsboer and Ising, 2008).
Contrary to our hypothesis, there was no statistically significant difference in serum BDNF levels between groups with distinct sleep quality. Although not unanimous, studies have been consistently reporting low serum BDNF in subjects with chronic insomnia, which is correlated to poor subjective sleep quality and decreased REM sleep (Giese et al., 2013, 2014; Klein et al., 2013; Rethorst et al., 2015; Mikoteit et al., 2019). However, it has also been suggested that BDNF is not directly correlated to a specific sleep disorder, or sleep duration, but with the proportion of sleep stages and REM sleep (Deuschle et al., 2018). Therefore, our result might be partially contaminated by the short washout period and long-term use of antidepressants (Dubovsky, 2018; Wilkinson et al., 2018). The number of unsuccessful previous antidepressant treatments with different drug classes did not modulate BDNF levels. Furthermore, previous treatment with benzodiazepines did not predict BDNF, and in some cases, benzodiazepines are associated with memory impairment and reduction of BDNF expression in the hippocampus; thus, further studies should analyze this topic (Zafra et al., 1991; Licata et al., 2013).
We found a positive significant correlation between serum BDNF and platelets, and it is known that in the periphery, BDNF is mainly produced by platelets (Nurden et al., 2008). Nevertheless, we did not observe correlations between CAR and serum BDNF, although the literature appoints a biphasic model for the interaction between the HPA system and BDNF synthesis, besides the importance of cortisol to BDNF receptor activation (Kunugi et al., 2010; Giese et al., 2013; Schmitt et al., 2016).
It is essential to point out some limitations of this study. First, with respect to the sample size, our study was carried out with a modest number of patients, with treatment-resistant depression and some cases of comorbid personality and anxiety disorder. Although patients were under washout to antidepressants, they were under treatment with benzodiazepines. With respect to the study design, sleep was not assessed with polysomnography. Only one biological sample per volunteer was collected and pro-BDNF and mature BDNF molecules, which are more specific than total BDNF, were not dosed (Angoa-Pérez et al., 2017). Thus, further studies that address these limitations are necessary to obtain potentially relevant additional results.
Conclusion
Our results suggest a relationship between changes in CAR and sleep quality in patients with treatment-resistant major depression, and changes in both were correlated with the severity of depression, suggesting that cortisol could be a potential physiological link between sleep disturbance and major depression.
Data Availability Statement
The datasets generated for this study are available on request to the corresponding author.
Ethics Statement
The studies involving human participants were reviewed and approved by the Research Ethics Committee of the Onofre Lopes University Hospital (HUOL), Federal University of Rio Grande do Norte (UFRN), Natal-RN, Brazil (#579.479), and was registered at http://clinicaltrials.gov (NCT02914769). The patients/participants provided their written informed consent to participate in this study.
Author Contributions
NG-C, BL-S, DA, JM, and FP-F designed the experiments. RA and AC measured the hormonal data. FP-F, AG, and GS collected the experimental data, carried out the statistical analysis, and prepared the figures. NG-C, BL-S, DA, SM-R, FP-F, AM, and GS prepared the manuscript.
Funding
This study was funded by the Brazilian National Council for Scientific and Technological Development (CNPq, grants #466760/2014 and #479466/2013) and by Coordenação de Aperfeiçoamento de Pessoal de Nível Superior—Brasil (CAPES)—Finance Code 001.
Conflict of Interest
The authors declare that the research was conducted in the absence of any commercial or financial relationships that could be construed as a potential conflict of interest.
Acknowledgments
We would like to express our gratitude to all volunteers of this study and to the Hospital Universitário Onofre Lopes, from the Federal University of Rio Grande do Norte, Brazil, for institutional support.
Abbreviations
AUC, area under the curve; CAR, salivary cortisol awakening response; C1, subjective sleep quality; C2, sleep latency; C3, sleep duration; C4, sleep efficiency; C5, sleep alterations; C6, medication use; C7, daytime sleep dysfunction; D1, day which the volunteers slept; ESS, Epworth Sleepiness Scale; GC, control group; GS, volunteers with good sleep in PSQI; HUOL, Onofre Lopes University Hospital; MD, patients with treatment-resistant major depression; NDRI, norepinephrine–dopamine reuptake inhibitor; PS, volunteers with poor sleep in PSQI; PSQI, Pittsburgh Sleep Quality Index; REM, rapid eye movement; SD, volunteers with sleep disturbance in PSQI; SNRI, serotonin–norepinephrine reuptake inhibitor; SSRI, selective serotonin reuptake inhibitor; TCAs, tricyclic antidepressants; UFRN, Federal University of Rio Grande do Norte.
Supplementary Material
The Supplementary Material for this article can be found online at: https://www.frontiersin.org/articles/10.3389/fnbeh.2020.00044/full#supplementary-material.
References
Abell, J. G., Shipley, M. J., Ferrie, J. E., Kivimäki, M., and Kumari, M. (2016). Recurrent short sleep, chronic insomnia symptoms and salivary cortisol: a 10-year follow-up in the Whitehall II study. Psychoneuroendocrinology 68, 91–99. doi: 10.1016/j.psyneuen.2016.02.021
Adzic, M., Djordjevic, J., Djordjevic, A., Niciforovic, A., Demonacos, C., Radojcic, M., et al. (2009). Acute or chronic stress induce cell compartment-specific phosphorylation of glucocorticoid receptor and alter its transcriptional activity in Wistar rat brain. J. Endocrinol. 202, 87–97. doi: 10.1677/joe-08-0509
Agargun, M. Y., Kara, H., Özer, Ö. A., Selvi, Y., Kiran, Ü., and Özer, B. (2003). Clinical importance of nightmare disorder in patients with dissociative disorders. Psychiatry Clin. Neurosci. 57, 575–579. doi: 10.1046/j.1440-1819.2003.01169.x
Almeida, R. N., Galvão, A. C. D. M., da Silva, F. S., Silva, E. A. D. S., Palhano-Fontes, F., Maia-de-Oliveira, J. P., et al. (2019). Modulation of serum brain-derived neurotrophic factor by a single dose of ayahuasca: observation from a randomized controlled trial. Front. Psychol. 10:1234. doi: 10.3389/fpsyg.2019.01234
Alvaro, P. K., Roberts, R. M., and Harris, J. K. (2013). A systematic review assessing bidirectionality between sleep disturbances, anxiety, and depression. Sleep 36, 1059–1068. doi: 10.5665/sleep.2810
Angoa-Pérez, M., Anneken, J. H., and Kuhn, D. M. (2017). The role of brain-derived neurotrophic factor in the pathophysiology of psychiatric and neurological disorders. J. Psychiatry Psychiatr. Disord. 1, 252–269. doi: 10.26502/jppd.2572-519x0025
Argyropoulos, S. V., Hicks, J. A., Nash, J. R., Bell, C. J., Rich, A. S., Nutt, D. J., et al. (2003). Correlation of subjective and objective sleep measurements at different stages of the treatment of depression. Psychiatry Res. 120, 179–190. doi: 10.1016/s0165-1781(03)00187-2
Atwi, S., McMahon, D., Scharfman, H., and MacLusky, N. J. (2014). Androgen modulation of hippocampal structure and function. Neuroscientist 22, 46–60. doi: 10.1177/1073858414558065
Backhaus, J., Junghanns, K., and Hohagen, F. (2004). Sleep disturbances are correlated with decreased morning awakening salivary cortisol. Psychoneuroendocrinology 29, 1184–1191. doi: 10.1016/j.psyneuen.2004.01.010
Bhagwagar, Z., Hafizi, S., and Cowen, P. J. (2005). Increased salivary cortisol after waking in depression. Psychopharmacology 182, 54–57. doi: 10.1007/s00213-005-0062-z
Borb, A. A., and Achermann, P. (1999). Sleep homeostasis and models of sleep regulation. J. Biol. Rhythms 14, 559–570. doi: 10.1177/074873099129000894
Born, J., and Fehm, H. L. (2000). The neuroendocrine recovery function of sleep. Noise Health 2, 25–37.
Bremmer, M. A., Deeg, D. J., Beekman, A. T., Penninx, B. W., Lips, P., and Hoogendijk, W. J. (2007). Major depression in late life is associated with both hypo-and hypercortisolemia. Biol. Psychiatry 62, 479–486. doi: 10.1016/j.biopsych.2006.11.033
Breslau, N., Roth, T., Rosenthal, L., and Andreski, P. (1996). Sleep disturbance and psychiatric disorders: a longitudinal epidemiological study of young adults. Biol. Psychiatry 39, 411–418. doi: 10.1016/0006-3223(95)00188-3
Buysse, D. J., Frank, E., Lowe, K. K., Cherry, C. R., and Kupfer, D. J. (1997). Electroencephalographic sleep correlates of episode and vulnerability to recurrence in depression. Biol. Psychiatry 41, 406–418. doi: 10.1016/s0006-3223(96)00041-8
Buysse, D. J., Tu, X. M., Cherry, C. R., Begley, A. E., Kowalski, J., Kupfer, D. J., et al. (1999). Pretreatment REM sleep and subjective sleep quality distinguish depressed psychotherapy remitters and nonremitters. Biol. Psychiatry 45, 205–213. doi: 10.1016/s0006-3223(98)00198-x
Chellappa, S. L., and Araujo, J. F. (2007a). O sono e os transtornos do sono na depressão. Arch. Clin. Psychiatry 34, 285–289. doi: 10.1590/s0101-60832007000600005
Chellappa, S. L., and Araujo, J. F. (2007b). Qualidade subjetiva do sono em pacientes com transtorno depressivo. Estud. Psicol. 12, 269–274. doi: 10.1590/s1413-294x2007000300009
Chrousos, G. A., Kattah, J. C., Beck, R. W., Cleary, P. A., Beck, R., Cleary, P., et al. (1993). Side effects of glucocorticoid treatment: experience of the Optic Neuritis Treatment Trial. JAMA 269, 2110–2112. doi: 10.1001/jama.1993.03500160080036
Dettenborn, L., Rosenloecher, F., and Kirschbaum, C. (2007). No effects of repeated forced wakings during three consecutive nights on morning cortisol awakening responses (CAR): a preliminary study. Psychoneuroendocrinology 32, 915–921. doi: 10.1016/j.psyneuen.2007.06.011
Deuschle, M., Schredl, M., Wisch, C., Schilling, C., Gilles, M., Geisel, O., et al. (2018). Serum brain-derived neurotrophic factor (BDNF) in sleep-disordered patients: relation to sleep stage N3 and rapid eye movement (REM) sleep across diagnostic entities. J. Sleep Res. 27, 73–77. doi: 10.1111/jsr.12577
Dubocovich, M. L. (2007). Melatonin receptors: role on sleep and circadian rhythm regulation. Sleep Med. 8, 34–42. doi: 10.1016/j.sleep.2007.10.007
Dubovsky, S. L. (2018). What is new about new antidepressants? Psychother. Psychosom. 87, 129–139. doi: 10.1159/000488945
Elder, G. J., Ellis, J. G., Barclay, N. L., and Wetherell, M. A. (2016). Assessing the daily stability of the cortisol awakening response in a controlled environment. BMC Psychol. 4:3. doi: 10.1186/s40359-016-0107-6
Elfving, B., Buttenschøn, H. N., Foldager, L., Poulsen, P. H., Andersen, J. H., Grynderup, M. B., et al. (2012). Depression, the Val66Met polymorphism, age, and gender influence the serum BDNF level. J. Psychiatr. Res. 46, 1118–1125. doi: 10.1016/j.jpsychires.2012.05.003
Fan, T. T., Chen, W. H., Shi, L., Lin, X., Tabarak, S., Chen, S. J., et al. (2018). Objective sleep duration is associated with cognitive deficits in primary insomnia: BDNF may play a role. Sleep 42:192. doi: 10.1093/sleep/zsy192
Fawcett, J., Scheftner, W. A., Fogg, L., Clark, D. C., and Young, M. A. (1990). Time-related predictors of suicide in major affective disorder. Am. J. Psychiatry 147, 1189–1194. doi: 10.1176/ajp.147.9.1189
Fries, E., Dettenborn, L., and Kirschbaum, C. (2009). The cortisol awakening response (CAR): facts and future directions. Int. J. Psychophysiol. 72, 67–73. doi: 10.1016/j.ijpsycho.2008.03.014
Fries, E., Hellhammer, D. H., and Hellhammer, J. (2006). Attenuation of the hypothalamic-pituitary-adrenal axis responsivity to the Trier Social Stress Test by the benzodiazepine alprazolam. Psychoneuroendocrinology 31, 1278–1288. doi: 10.1016/j.psyneuen.2006.09.009
Galvão, A. C. M., Almeida, R. N., Silva, E. S., Freire, F. A. M., Palhano-Fontes, F., Onias, H., et al. (2018). Cortisol modulation by ayahuasca in patients with treatment resistant depression and healthy controls. Front. Psychiatry 9:185. doi: 10.3389/fpsyt.2018.00185
Gan, E. H., and Pearce, S. H. (2016). Management of endocrine disease: regenerative therapies in autoimmune Addison’s disease. Eur. J. Endocrinol. 176, R123–R135. doi: 10.1530/eje-16-0581
García-Borreguero, D., Wehr, T. A., Larrosa, O., Granizo, J. J., Hardwick, D., Chrousos, G. P., et al. (2000). Glucocorticoid replacement is permissive for rapid eye movement sleep and sleep consolidation in patients with adrenal insufficiency. J. Clin. Endocrinol. Metab. 85, 4201–4206. doi: 10.1210/jc.85.11.4201
Gebara, M. A., Siripong, N., DiNapoli, E. A., Maree, R. D., Germain, A., Reynolds, C. F., et al. (2018). Effect of insomnia treatments on depression: a systematic review and meta-analysis. Depress. Anxiety 35, 717–731. doi: 10.1002/da.22776
Giese, M., Unternaehrer, E., Brand, S., Calabrese, P., Holsboer-Trachsler, E., and Eckert, A. (2013). The interplay of stress and sleep impacts BDNF level. PLoS One 8:e76050. doi: 10.1371/journal.pone.0076050
Giese, M., Unternährer, E., Hüttig, H., Beck, J., Brand, S., Calabrese, P., et al. (2014). BDNF: an indicator of insomnia? Mol. Psychiatry 19, 151–152. doi: 10.1038/mp.2013.10
Gillespie, C. F., and Nemeroff, C. B. (2005). Hypercortisolemia and depression. Psychosom. Med. 67, S26–S28. doi: 10.1097/01.psy.0000163456.22154.d2
Glud, M., Christiansen, T., Larsen, L. H., Richelsen, B., and Bruun, J. M. (2019). Changes in circulating bdnf in relation to sex, diet, and exercise: a 12-week randomized controlled study in overweight and obese participants. J. Obes. 2019:4537274. doi: 10.1155/2019/4537274
Gotlieb, N., Moeller, J., and Kriegsfeld, L. J. (2018). Circadian control of neuroendocrine function: implications for health and disease. Curr. Opin. Physiol. 5, 133–140. doi: 10.1016/j.cophys.2018.11.001
Hamilton, M. (1960). A rating scale for depression. J. Neurol. Neurosurg. Psychiatry 23, 56–62. doi: 10.1136/jnnp.23.1.56
Hansson, A. C., Cintra, A., Belluardo, N., Sommer, W., Bhatnagar, M., Bader, M., et al. (2000). Gluco-and mineralocorticoid receptor-mediated regulation of neurotrophic factor gene expression in the dorsal hippocampus and the neocortex of the rat. Eur. J. Neurosci. 12, 2918–2934. doi: 10.1046/j.1460-9568.2000.00185.x
Harvey, A. G. (2001). Insomnia: symptom or diagnosis? Clin. Psychol. Rev. 21, 1037–1059. doi: 10.1016/s0272-7358(00)00083-0
Harvey, C. J., Gehrman, P., and Espie, C. A. (2014). Who is predisposed to insomnia: a review of familial aggregation, stress-reactivity, personality and coping style. Sleep Med. Rev. 18, 237–247. doi: 10.1016/j.smrv.2013.11.004
Held, K., Künzel, H., Ising, M., Schmid, D. A., Zobel, A., Murck, H., et al. (2004). Treatment with the CRH1-receptor-antagonist R121919 improves sleep-EEG in patients with depression. J. Psychiatr. Res. 38, 129–136. doi: 10.1016/s0022-3956(03)00076-1
Herbert, J. (2013). Cortisol and depression: three questions for psychiatry. Psychol. Med. 43, 449–469. doi: 10.1017/s0033291712000955
Holsboer, F., and Ising, M. (2008). Central CRH system in depression and anxiety—evidence from clinical studies with CRH1 receptor antagonists. Eur. J. Pharmacol. 583, 350–357. doi: 10.1016/j.ejphar.2007.12.032
Howland, R. H. (2008). Sequenced treatment alternatives to relieve depression (STAR* D)—part 2: study outcomes. J. Psychosoc. Nurs. Ment. Health Serv. 46, 21–24. doi: 10.3928/02793695-20081001-05
Huang, Z. L., Urade, Y., and Hayaishi, O. (2007). Prostaglandins and adenosine in the regulation of sleep and wakefulness. Curr. Opin. Pharmacol. 7, 33–38. doi: 10.1016/j.coph.2006.09.004
Huber, T. J., Issa, K., Schik, G., and Wolf, O. T. (2006). The cortisol awakening response is blunted in psychotherapy inpatients suffering from depression. Psychoneuroendocrinology 31, 900–904. doi: 10.1016/j.psyneuen.2006.03.005
Jeon, S. W., and Kim, Y. K. (2016). Molecular neurobiology and promising new treatment in depression. Int. J. Mol. Sci. 17:381. doi: 10.3390/ijms17030381
Judd, L. L., Akiskal, H. S., Maser, J. D., Zeller, P. J., Endicott, J., Coryell, W., et al. (1998). Major depressive disorder: a prospective study of residual subthreshold depressive symptoms as predictor of rapid relapse. J. Affect. Disord. 50, 97–108. doi: 10.1016/s0165-0327(98)00138-4
Kalogeras, K. T., Calogero, A. E., Kuribayiashi, T., Khan, I., Gallucci, W. T., Kling, M. A., et al. (1990). In vitro and in vivo effects of the triazolobenzodiazepine alprazolam on hypothalamic pituitary-adrenal function: pharmacological and clinical implications. J. Clin. Endocrinol. Metab. 70, 1462–1471. doi: 10.1210/jcem-70-5-1462
Kino, T., Jaffe, H., Amin, N. D., Chakrabarti, M., Zheng, Y. L., Chrousos, G. P., et al. (2010). Cyclin-dependent kinase 5 modulates the transcriptional activity of the mineralocorticoid receptor and regulates expression of brain-derived neurotrophic factor. Mol. Endocrinol. 24, 941–952. doi: 10.1210/me.2009-0395
Klein, A. B., Jennum, P., Knudsen, S., Gammeltoft, S., and Mikkelsen, J. D. (2013). Increased serum brain-derived neurotrophic factor (BDNF) levels in patients with narcolepsy. Neurosci. Lett. 544, 31–35. doi: 10.1016/j.neulet.2013.03.031
Kreinin, A., Lisson, S., Nesher, E., Schneider, J., Bergman, J., Farhat, K., et al. (2015). Blood BDNF level is gender specific in severe depression. PLoS One 10:e0127643. doi: 10.1371/journal.pone.0127643
Kudielka, B. M., and Kirschbaum, C. (2005). Sex differences in HPA axis responses to stress: a review. Biol. Psychol. 69, 113–132. doi: 10.1016/j.biopsycho.2004.11.009
Kuehner, C. (2017). Why is depression more common among women than among men? Lancet Psychiatry 4, 146–158. doi: 10.1016/s2215-0366(16)30263-2
Kunugi, H., Hori, H., Adachi, N., and Numakawa, T. (2010). Interface between hypothalamic-pituitary-adrenal axis and brain-derived neurotrophic factor in depression. Psychiatry Clin. Neurosci. 64, 447–459. doi: 10.1111/j.1440-1819.2010.02135.x
Kurian, B. T., Greer, T. L., and Trivedi, M. H. (2010). Strategies to enhance the therapeutic efficacy of antidepressants: targeting residual symptoms. Expert Rev. Neurother. 9, 975–984. doi: 10.1586/ern.09.53
Laakmann, G., Wittmann, M., Gugath, M., Mueller, O. A., Treusch, J., Wahlster, U., et al. (1984). Effects of psychotropic drugs (desimipramine, chlorimipramine, sulpiride and diazepam) on the human HPA axis. Psychopharmacology 84, 66–70. doi: 10.1007/bf00432027
Leuchter, A. F., Cook, I. A., Hamilton, S. P., Narr, K. L., Toga, A., Hunter, A. M., et al. (2010). Biomarkers to predict antidepressant response. Curr. Psychiatry Rep. 12, 553–562. doi: 10.1007/s11920-010-0160-4
Licata, S. C., Shinday, N. M., Huizenga, M. N., Darnell, S. B., Sangrey, G. R., Rudolph, U., et al. (2013). Alterations in brain-derived neurotrophic factor in the mouse hippocampus following acute but not repeated benzodiazepine treatment. PLoS One 8:e84806. doi: 10.1371/journal.pone.0084806
Luca, A., Luca, M., and Calandra, C. (2013). Sleep disorders and depression: brief review of the literature, case report, and nonpharmacologic interventions for depression. Clin. Interv. Aging 8, 1033–1039. doi: 10.2147/cia.s47230
Lucchesi, L. M., Pradella-Hallinan, M., Lucchesi, M., and Moraes, W. A. D. S. (2005). O sono em transtornos psiquiátricos. Braz. J. Psychiatry 27, 27–32. doi: 10.1590/S1516-44462005000500006
Manthey, L., Giltay, E. J., van Veen, T., Neven, A. K., Vreeburg, S. A., Penninx, B. W., et al. (2010). Long-term benzodiazepine use and salivary cortisol: the Netherlands Study of Depression and Anxiety (NESDA). J. Clin. Psychopharmacol. 30, 160–168. doi: 10.1097/jcp.0b013e3181d41f41
Mayer, S. E., Lopez-Duran, N. L., Sen, S., and Abelson, J. L. (2018). Chronic stress, hair cortisol and depression: a prospective and longitudinal study of medical internship. Psychoneuroendocrinology 92, 57–65. doi: 10.1016/j.psyneuen.2018.03.020
McIntyre, I. M., Norman, T. R., Burrows, G. D., and Armstrong, S. M. (1993). Alterations to plasma melatonin and cortisol after evening alprazolam administration in humans. Chronobiol. Int. 10, 205–213. doi: 10.3109/07420529309073889
Mikoteit, T., Brand, S., Eckert, A., Holsboer-Trachsler, E., and Beck, J. (2019). Brain-derived neurotrophic factor is a biomarker for subjective insomnia but not objectively assessable poor sleep continuity. J. Psychiatr. Res. 110, 103–109. doi: 10.1016/j.jpsychires.2018.12.020
Molendijk, M. L., Bus, B. A., Spinhoven, P., Penninx, B. W., Kenis, G., Prickaerts, J., et al. (2011). Serum levels of brain-derived neurotrophic factor in major depressive disorder: state-trait issues, clinical features and pharmacological treatment. Mol. Psychiatry 16, 1088–1095. doi: 10.1038/mp.2010.98
Mollayeva, T., Thurairajah, P., Burton, K., Mollayeva, S., Shapiro, C. M., and Colantonio, A. (2016). The Pittsburgh sleep quality index as a screening tool for sleep dysfunction in clinical and non-clinical samples: a systematic review and meta-analysis. Sleep Med. Rev. 25, 52–73. doi: 10.1016/j.smrv.2015.01.009
Moos, R. H., and Cronkite, R. C. (1999). Symptom-based predictors of a 10-year chronic course of treated depression. J. Nerv. Ment. Dis. 187, 360–368. doi: 10.1097/00005053-199906000-00005
Moreira, M. A., Guerra, R. O., do Nascimento Falcão Freire, A., Dos Santos Gomes, C., and Maciel, Á. C. (2016). Depressive symptomatology and cortisol concentrations in elderly community residents: a cross-sectional study. Aging Clin. Exp. Res. 28, 131–137. doi: 10.1007/s40520-015-0374-8
Nurden, A. T., Nurden, P., Sanchez, M., Andia, I., and Anitua, E. (2008). Platelets and wound healing. Front. Biosci. 13, 3532–3548. doi: 10.2741/2947
Ohayon, M. M., and Roth, T. (2003). Place of chronic insomnia in the course of depressive and anxiety disorders. J. Psychiatr. Res. 37, 9–15. doi: 10.1016/s0022-3956(02)00052-3
Palhano-Fontes, F., Barreto, D., Onias, H., Andrade, K. C., Novaes, M. M., Pessoa, J. A., et al. (2019). Rapid antidepressant effects of the psychedelic ayahuasca in treatment-resistant depression: a randomized placebo-controlled trial. Psychol. Med. 49, 655–663. doi: 10.1017/s0033291718001356
Palma, B. D., Tiba, P. A., Machado, R. B., Tufik, S., and Suchecki, D. (2007). Repercussões imunológicas dos distúrbios do sono: o eixo hipotálamo-pituitária-adrenal como fator modulador. Braz. J. Psychiatry 29, s33–s38. doi: 10.1590/S1516-44462007000500007
Panagiotakopoulos, L., and Neigh, G. N. (2014). Development of the HPA axis: where and when do sex differences manifest? Front. Neuroendocrinol. 35, 285–302. doi: 10.1016/j.yfrne.2014.03.002
Papakostas, G. I., Shelton, R. C., Kinrys, G., Henry, M. E., Bakow, B. R., Lipkin, S. H., et al. (2013). Assessment of a multi-assay, serum-based biological diagnostic test for major depressive disorder: a pilot and replication study. Mol. Psychiatry 18, 332–339. doi: 10.1038/mp.2011.166
Pariante, C. M., Thomas, S. A., Lovestone, S., Makoff, A., and Kerwin, R. W. (2004). Do antidepressants regulate how cortisol affects the brain? Psychoneuroendocrinology 29, 423–447. doi: 10.1016/j.psyneuen.2003.10.009
Pelayo, R. (2014). Excessive daytime sleepiness. Encycl. Neurol. Sci. 27, 233–235. doi: 10.1016/b978-0-12-385157-4.00552-2
Peng, S., Li, W., Lv, L., Zhang, Z., and Zhan, X. (2018). BDNF as a biomarker in diagnosis and evaluation of treatment for schizophrenia and depression. Discov. Med. 26, 127–136.
Perlis, M. L., Giles, D. E., Buysse, D. J., Tu, X., and Kupfer, D. J. (1997). Self-reported sleep disturbance as a prodromal symptom in recurrent depression. J. Affect. Disord. 42, 209–212. doi: 10.1016/s0165-0327(96)01411-5
Polyakova, M., Stuke, K., Schuemberg, K., Mueller, K., Schoenknecht, P., and Schroeter, M. L. (2015). BDNF as a biomarker for successful treatment of mood disorders: a systematic and quantitative meta-analysis. J. Affect. Disord. 174, 432–440. doi: 10.1016/j.jad.2014.11.044
Pomara, N., Willoughby, L. M., Sidtis, J. J., Cooper, T. B., and Greenblatt, D. J. (2005). Cortisol response to diazepam: its relationship to age, dose, duration of treatment, and presence of generalized anxiety disorder. Psychopharmacology 178, 1–8. doi: 10.1007/s00213-004-1974-8
Rethorst, C. D., Greer, T. L., Toups, M. S. P., Bernstein, I., Carmody, T. J., and Trivedi, M. H. (2015). IL-1β and BDNF are associated with improvement in hypersomnia but not insomnia following exercise in major depressive disorder. Transl. Psychiatry 5:e611. doi: 10.1038/tp.2015.104
Rush, A. J., Fava, M., Wisniewski, S. R., Lavori, P. W., Trivedi, M. H., Sackeim, H. A., et al. (2004). Sequenced treatment alternatives to relieve depression (STAR*D): rationale and design. Control. Clin. Trials 25, 119–142. doi: 10.1016/s0197-2456(03)00112-0
Sadock, B. J., Sadock, V. A., and Ruiz, P. (2016). Compêndio de Psiquiatria: Ciência do Comportamento e Psiquiatria Clínica. Porto Alegre: Artmed Editora.
Schmitt, K., Holsboer-Trachsler, E., and Eckert, A. (2016). BDNF in sleep, insomnia, and sleep deprivation. Ann. Med. 48, 42–51. doi: 10.3109/07853890.2015.1131327
Schüle, C. (2007). Neuroendocrinological mechanisms of actions of antidepressant drugs. J. Neuroendocrinol. 19, 213–226. doi: 10.1111/j.1365-2826.2006.01516.x
Schüle, C., Baghai, T. C., Eser, D., Zwanzger, P., Jordan, M., Buechs, R., et al. (2006). Time course of hypothalamic-pituitary-adrenocortical axis activity during treatment with reboxetine and mirtazapine in depressed patients. Psychopharmacology 186, 601–611. doi: 10.1007/s00213-006-0382-7
Schutte-Rodin, S., Broch, L., Buysse, D., Dorsey, C., and Sateia, M. (2008). Clinical guideline for the evaluation and management of chronic insomnia in adults. J. Clin. Sleep Med. 4, 487–504. doi: 10.5664/jcsm.27286
Starr, L. R., Dienes, K., Stroud, C. B., Shaw, Z. A., Li, Y. I., Mlawer, F., et al. (2017). Childhood adversity moderates the influence of proximal episodic stress on the cortisol awakening response and depressive symptoms in adolescents. Dev. Psychopathol. 29, 1877–1893. doi: 10.1017/s0954579417001468
Steckler, T., Holsboer, F., and Reul, J. M. (1999). Glucocorticoids and depression. Baillieres Best Pract. Res. Clin. Endocrinol. Metab. 13, 597–614. doi: 10.1053/beem.1999.0046
Steiger, A. (2003). Sleep and endocrinology. J. Intern. Med. 254, 13–22. doi: 10.1046/j.1365-2796.2003.01175.x
Tadić, A., Wagner, S., Gorbulev, S., Dahmen, N., Hiemke, C., Braus, D. F., et al. (2011). Peripheral blood and neuropsychological markers for the onset of action of antidepressant drugs in patients with major depressive disorder. BMC Psychiatry 11:16. doi: 10.1186/1471-244x-11-16
Taylor, D. J., Lichstein, K. L., Durrence, H. H., Reidel, B. W., and Bush, A. J. (2005). Epidemiology of insomnia, depression and anxiety. Sleep 28, 1457–1464. doi: 10.1093/sleep/28.11.1457
Thase, M. E. (1999). Antidepressant treatment of the depressed patient with insomnia. J. Clin. Psychiatry 60, 28–31.
Tsuchiyama, Y., Uchimura, N., Sakomoto, T., Maeda, H., and Kotorii, T. (1995). Effects of hCRH on sleep and body temperature rhythms. Psychiatry Clin. Neurosci. 49, 299–304. doi: 10.1111/j.1440-1819.1995.tb01906.x
Vargas, I., Vgontzas, A. N., Abelson, J. L., Faghih, R. T., Morales, K. H., and Perlis, M. L. (2018). Altered ultradian cortisol rhythmicity as a potential neurobiologic substrate for chronic insomnia. Sleep Med. Rev. 41, 234–243. doi: 10.1016/j.smrv.2018.03.003
Van Veen, M. M., Karsten, J., and Lancel, M. (2017). Poor sleep and its relation to impulsivity in patients with antisocial or borderline personality disorders. Behav. Med. 43, 218–226. doi: 10.1080/08964289.2017.1313719
Vreeburg, S. A., Hoogendijk, W. J., DeRijk, R. H., van Dyck, R., Smit, J. H., Zitman, F. G., et al. (2013). Salivary cortisol levels and the 2-year course of depressive and anxiety disorders. Psychoneuroendocrinology 38, 1494–1502. doi: 10.1016/j.psyneuen.2012.12.017
Weinberg, U., D’Eletto, R. D., Weitzman, E. D., Erlich, S., and Hollander, C. S. (1979). Circulating melatonin in man: episodic secretion throughout the light-dark cycle. J. Clin. Endocrinol. Metab. 48, 114–118. doi: 10.1210/jcem-48-1-114
Weitzman, E. D., Fukushima, D., Nogeire, C., Roffwarg, H., Gallagher, T. F., and Hellman, L. (1971). Twenty-four hour pattern of the episodic secretion of cortisol in normal subjects. J. Clin. Endocrinol. Metab. 33, 14–22. doi: 10.1210/jcem-33-1-14
Wilkinson, S. T., Kiselycznyk, C., Banasr, M., Webler, R. D., Haile, C., and Mathew, S. J. (2018). Serum and plasma brain-derived neurotrophic factor and response in a randomized controlled trial of riluzole for treatment resistant depression. J. Affect. Disord. 241, 514–518. doi: 10.1016/j.jad.2018.08.075
Wu, J. Y., Hsu, S. C., Ku, S. C., Ho, C. C., Yu, C. J., and Yang, P. C. (2008). Adrenal insufficiency in prolonged critical illness. Crit. Care 12:R65. doi: 10.1186/cc6895
Zafra, F., Castrén, E., Thoenen, H., and Lindholm, D. (1991). Interplay between glutamate and gamma-aminobutyric acid transmitter systems in the physiological regulation of brain-derived neurotrophic factor and nerve growth factor synthesis in hippocampal neurons. Proc. Natl. Acad. Sci. U S A 88, 10037–10041. doi: 10.1073/pnas.88.22.10037
Keywords: pittsburgh sleep quality index, brain-derived neurotrophic factor, salivary cortisol awakening response, sleep disturbance, treatment-resistant depression
Citation: Santiago GTP, Galvão ACM, de Almeida RN, Mota-Rolim SA, Palhano-Fontes F, Maia-de-Oliveira JP, de Araújo DB, Lobão-Soares B and Galvão-Coelho NL (2020) Changes in Cortisol but Not in Brain-Derived Neurotrophic Factor Modulate the Association Between Sleep Disturbances and Major Depression. Front. Behav. Neurosci. 14:44. doi: 10.3389/fnbeh.2020.00044
Received: 27 August 2019; Accepted: 13 March 2020;
Published: 28 April 2020.
Edited by:
Tamas Kozicz, Mayo Clinic, United StatesReviewed by:
Piray Atsak, Radboud University, NetherlandsAxel Steiger, Ludwig Maximilian University of Munich, Germany
Copyright © 2020 Santiago, Galvão, de Almeida, Mota-Rolim, Palhano-Fontes, Maia-de-Oliveira, de Araújo, Lobão-Soares and Galvão-Coelho. This is an open-access article distributed under the terms of the Creative Commons Attribution License (CC BY). The use, distribution or reproduction in other forums is permitted, provided the original author(s) and the copyright owner(s) are credited and that the original publication in this journal is cited, in accordance with accepted academic practice. No use, distribution or reproduction is permitted which does not comply with these terms.
*Correspondence: Nicole Leite Galvão-Coelho, bmljb2xlbGdhbHZhb2NvZWxob0BnbWFpbC5jb20=