- 1Research Group Neuropharmacology, Addiction and Behavior, Department of Pharmaceutical Biosciences, Uppsala University, Uppsala, Sweden
- 2Division of Anatomy and Physiology, Department of Anatomy, Physiology and Biochemistry, Swedish University of Agricultural Sciences, Uppsala, Sweden
Early-life stress and its possible correlations to genes, environment, and later health outcomes can only be studied retrospectively in humans. Animal models enable the exploration of such connections with prospective, well-controlled study designs. However, with the recent awareness of replicability issues in preclinical research, the reproducibility of results from animal models has been highlighted. The present study aims to reproduce the behavioral effects of maternal separation (MS) previously observed in the multivariate concentric square fieldTM (MCSF) test. A second objective was to replicate the adolescent behavioral profiles previously described in the MCSF test. Male rats, subjected to short or prolonged MS or standard rearing, were subjected to behavioral testing in early adolescence and early adulthood. As seen in previous studies, the behavioral effects of MS in the MCSF were small at both tested time points. When tested in early adolescence, the animals exhibited a similar behavioral profile as previously seen, and the finding of adolescent behavioral types was also reproduced. The distribution of animals into the behavioral types was different than in the initial study, but in a manner consistent with developmental theories, as the current cohort was younger than the previous. Notably, the Shelter seeker behavioral type persisted through development, while the Explorer type did not. The lack of basal behavioral effect after MS is in line with the literature on this MS paradigm; the working hypothesis is that the prolonged MS gives rise to a phenotype predisposed to negative health outcomes but which is not apparent without additional provocation.
Introduction
Early-life stress has evident adverse effects on several physiological systems, but the most emphasized is the increased vulnerability to develop psychiatric disorders (Ehlert, 2013; Maniam et al., 2014; Bick and Nelson, 2016). Various animal models have been developed to study this relationship, each with different types of stressors and developmental timings. Maternal separation (MS) is such a category of models that manipulates the early postnatal environment of the offspring. Prolonged MS, where the offspring is removed from its caretaker for between 3 and 6 h daily, is considered an early-life stress condition. However, the reported effects of prolonged MS vary considerably; some studies report increased impulsiveness/hyperactivity (Brake et al., 2004; Colorado et al., 2006), others increased anxiety- and/or depression-like behavior (Kalinichev et al., 2002; Jin et al., 2018), and yet others only limited or no effects (Shalev and Kafkafi, 2002; Marmendal et al., 2004; Farkas et al., 2009). Factors contributing to these discrepancies are probably differences in the MS methodology, model organism, the outcome assessment method and timing, and even the choice of control group (Lehmann and Feldon, 2000; Nylander and Roman, 2013; Vetulani, 2013).
Behavioral tests constitute an important part of animal models of psychiatric disorders (Brown and Bolivar, 2018; Bale et al., 2019). Recently, behavioral neuroscience has been mentioned as an example in the reproducibility crisis discussion (Kafkafi et al., 2018). Therefore, the choice of behavioral test and its validity and reproducibility have been emphasized. The multivariate concentric square fieldTM (MCSF) test is an ethologically founded behavioral test that is unbiased by underlying mental condition, as it seeks to establish a behavioral profile of the tested animal (Meyerson et al., 2006). The MCSF has been validated for use in adult (Meyerson et al., 2006, 2013) and adolescent (Lundberg et al., 2019) rats. It has also been shown to have a greater sensitivity than classical behavioral tests in detecting effects of both pharmacological and non-pharmacological interventions (Roman et al., 2006; Meyerson et al., 2013; Karlsson and Roman, 2016).
The aim of the present study was to evaluate the behavioral outcome after prolonged MS compared to short MS and standard rearing at two developmental time points. Behavioral assessment was made with the MCSF test at 4 (early adolescence) and 9 (early adulthood) weeks of age, to study the short-term behavioral outcome and the effect of development.
Materials and Methods
An overview of the experimental timeline can be seen in Figure 1A, and a detailed description of the methods can be found in Comasco et al. (2015) regarding the MS procedure and in Meyerson et al. (2006) and Roman and Colombo (2009) for the MCSF procedure. Previously published results from the animal cohort (developmental body weights, voluntary ethanol consumption, and later genetic and epigenetic results) can be found in Bendre et al. (2015, 2018), Comasco et al. (2015); Nylander et al. (2016), Todkar et al. (2016); Granholm et al. (2017), and Vrettou et al. (2017).
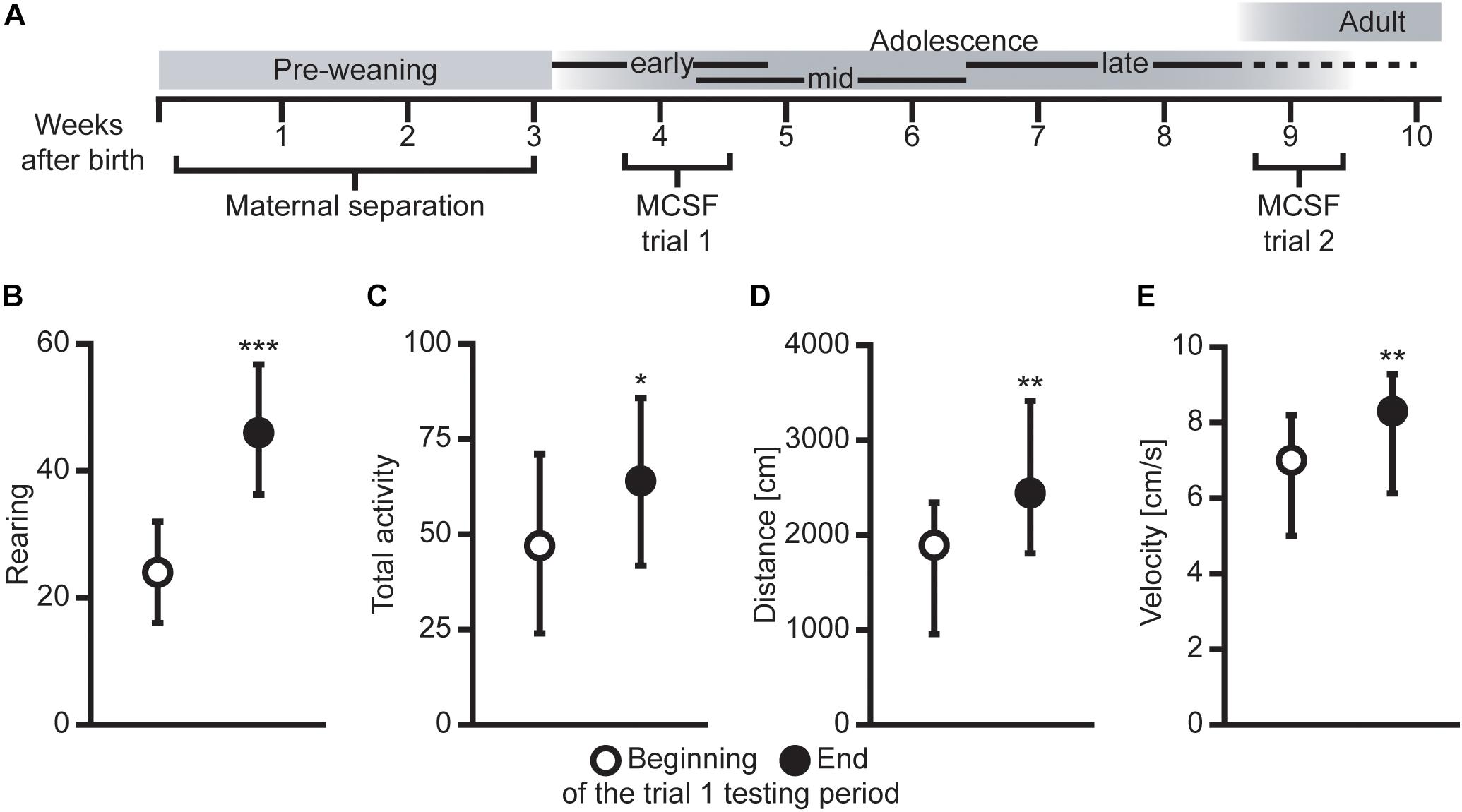
Figure 1. (A) Overview of the experimental outline with age in weeks and approximate developmental stages [adopted from Lundberg et al. (2019) with permission]. Difference in (B) rearing, (C) total activity (i.e., sum of all frequencies), (D) total distance traveled (cm), and (E) mean velocity (cm/s) between animals tested in the beginning (first half, n = 39) compared to the end (second half, n = 32) of the MCSF trial 1 testing period. Data presented as median with upper and lower quartiles. *P < 0.05, **P < 0.01, ***P < 0.001, Mann–Whitney U test.
In short, pregnant dams [n = 25, RccHanTM WI, Harlan (currently Envigo), Horst, Netherlands] arrived at gestational day 15. Litters were sexed and cross-fostered on the day of birth [postnatal day (PND) 0] and randomized into one of three rearing conditions: daily separations on PND 1–21 for 360 (MS360) or 15 (MS15) minutes or kept according to standard animal facility rearing (AFR).
At PND 22, the litters were weaned, and only male offspring continued in the study (MS360, n = 30; MS15, n = 21; AFR, n = 21). The light cycle was reversed, and the animals were group housed three per cage with littermates.
In early adolescence (PND 26–31; 4–9 days after weaning), the animals were tested for the first time in the MCSF (trial 1). The second MCSF trial was performed 34–35 days later (at 61–65 days of age, i.e., in early adulthood). Both tests were performed during the dark period of the light/dark cycle.
The MCSF Test
The MCSF arena is 100 cm × 100 cm with 10 defined zones: the center with central circle, three corridors used to move between the different parts of the arena, a covered shelter called the dark corner room (DCR), the slightly raised hurdle with hole board for nose poking, the slope leading up to the bridge entrance, and the illuminated bridge [for illustration see Lundberg et al. (2019)].
The animals were brought directly from their home cages and placed into the center of the arena, facing the wall not leading to a corridor. The trial started immediately as the animal was released into the arena and lasted for 20 min. The animals were recorded from above by a video camera and observed from an adjacent room. Direct observation was used to score number of rearing, grooming, and stretched attend postures (SAPs). After each trial, the number of urinations and fecal boli in the arena were counted, and the number of nose pokes in the hole board in the hurdle was read from a photocell counter. Score 3.3 (Soldis, Uppsala, Sweden) was used to manually score latency (L, s), frequency (F), and duration (D, s) of visits to the zones of the MCSF arena; EthoVision 2.3 (Noldus Inc., Wageningen, Netherlands) was used for automatic tracking of distance (total, cm) and velocity (mean, cm/s) in the arena. Additional parameters were derived as previously described (Lundberg et al., 2019).
Statistical Analysis
Classical statistical analysis was carried out in Statistica 13 (TIBCO Software Inc., Tulsa, OK, United States). The data were examined for normality with Shapiro–Wilk’s test; the majority of the parameters were not normally distributed, and thus, non-parametric statistics were used. Between-group differences in continuous parameters were analyzed with Mann–Whitney U test (two initial groups) or Kruskal–Wallis ANOVA by ranks with post hoc Mann–Whitney U test (more than two initial groups); categorical parameters were analyzed with the maximum-likelihood chi-square test. Within-group differences in continuous parameters were analyzed with Wilcoxon-matched pairs test and categorical parameters with McNemar chi-square test. Effects sizes were calculated for significant differences according to Fritz et al. (2012).
Multivariate data analysis in the form of principal component analysis (PCA) was carried out in SIMCA 15 (Sartorius Stedim Data Analytics AB, Umeå, Sweden). Autofit was used to generate each model; this produces the maximum number of significant components. The components were inspected and excluded if they had eigenvalues <2 or large negative Q2 values. The latency, percental frequency, and percental duration parameters were not included in the PCA, and other parameters were excluded when advised by the software due to minimal variance.
Rank Order Procedures—Adult Trend Analysis and Adolescent Type Scores
Two rank-order procedures to summarize related parameters into compound variables were applied. For adult animals, the trend analysis (Meyerson et al., 2013) was used. It groups, ranks, and sums parameters into five different behavioral categories: general activity, exploratory activity, risk assessment, risk taking, and shelter seeking. However, the trend analysis has previously been shown to be invalid for animals tested in mid-adolescence, and instead, a separation of individuals into behavioral types called Explorers (characterized by high activity), Shelter seekers (high shelter-seeking behavior and low activity and risk-taking behavior), and Main type (intermediates) have been described (Lundberg et al., 2019). To quantify the outlying types, the same method as the trend analysis was applied on the significant parameters characterizing the behavioral types, creating compound variables called type scores. In the type score exploration, the following parameters are included: distance, velocity, and D/F center (negative relationship, ranking reversed before summarizing). In the type score shelter seeking, D DCR, D/F DCR, and nose pokes have a positive relationship with the behavioral type, and total activity, velocity, distance, D center, D bridge entrance, and D bridge have a negative relationship (Lundberg et al., 2019).
Results
The behavioral profile of male MS360, MS15, and AFR offspring was assessed with the MCSF test at 4 (trial 1, early adolescence) and 9 (trial 2, early adulthood) weeks of age. One animal was excluded from trial 1 and three from trial 2 due to technical issues during testing. One animal was euthanized, due to malocclusion, between trials 1 and 2.
The effect of order in the two MCSF trials was examined by comparing the animals tested in the beginning (first half) of each testing period with the animals tested in the end (second half) of the corresponding testing period. As seen in Figures 1B–E, in trial 1, the activity was increased in animals tested in the end compared to the beginning of the testing period in all measures of activity, i.e., rearing, total activity, distance traveled, and velocity. Some additional activity-associated parameters were increased in the animals tested in the end compared to the beginning of the testing period in trial 1 (statistics not shown, Supplementary Data Sheet S1). This pattern was not seen in trial 2, where only a few mixed parameters were different between the animals tested in the beginning compared to the end of the testing period (statistics not shown, Supplementary Data Sheet S1).
MCSF Performance by Rearing Condition
The result from the MCSF tests analyzed by rearing condition can be seen in Figure 2A and Supplementary Tables S1, S2. Only a few parameters differed depending on the rearing condition; in trial 1, MS360 and MS15 rats behaved differently in the center zone of the MCSF (lower duration, duration per visit, and percental duration and frequency) compared to AFR animals. MS360 rats had higher duration per visit on the bridge than both MS15 and AFR rats and had lower distance traveled than AFR rats. No difference in the type scores was observed among the rearing conditions.
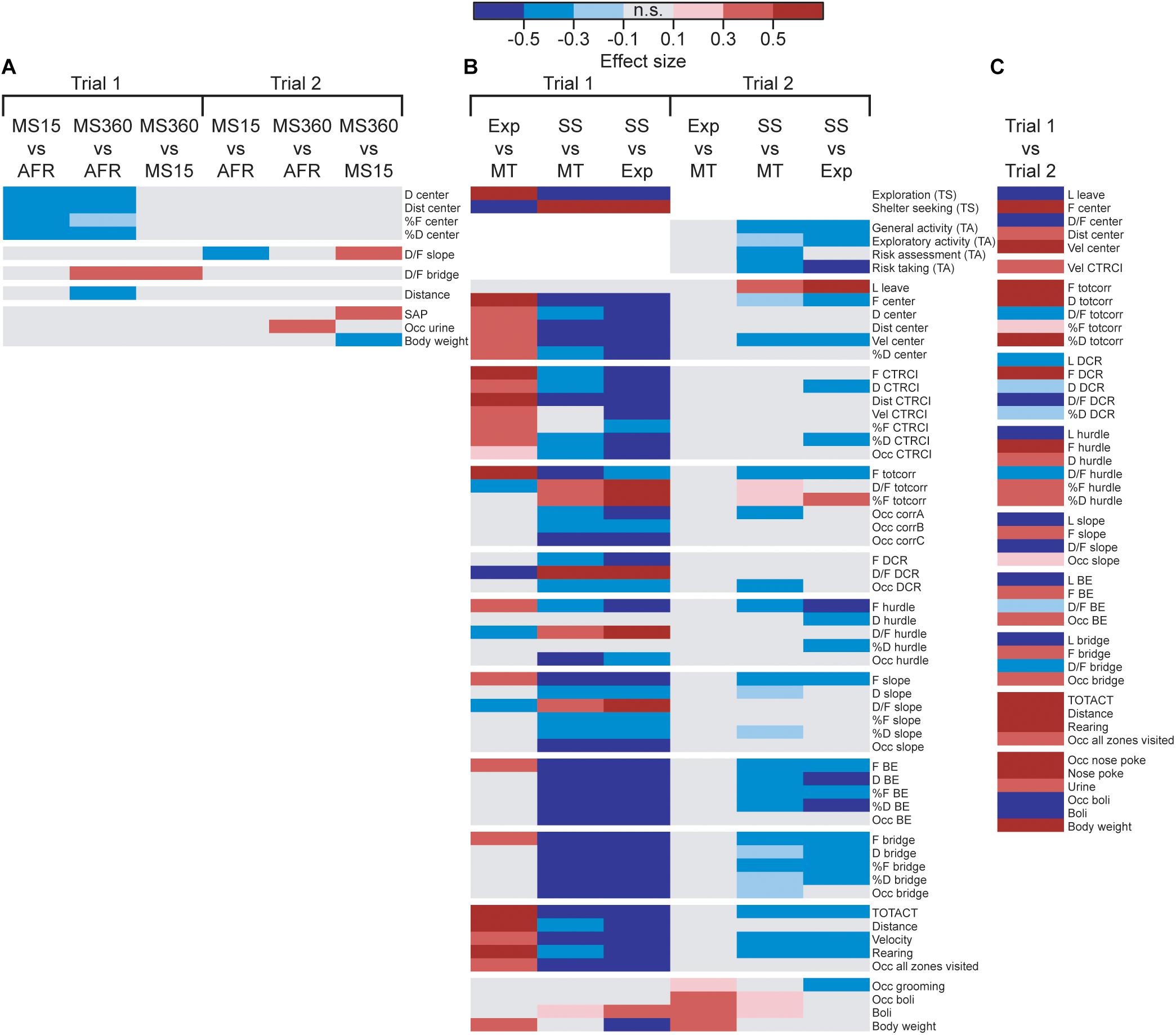
Figure 2. Heatmap of effect sizes for the significant differences comparing the (A) rearing conditions in trials 1 and 2 (MS15, ntrials1,2 = 21; MS360, ntrial1 = 30, ntrial2 = 28; AFR, ntrial1 = 20, n = trial 2 = 19), (B) behavioral types in trials 1 and 2 (Explorers, ntrial1 = 9, ntrial2 = 8; Shelter seekers, ntrial1,2 = 20; Main type ntrial1 = 42, ntrial2 = 39), and (C) trial 1 (n = 71) with trial 2 (n = 68) in the whole cohort. The parameters are sorted as followed: rank order procedures (type scores and trend analysis categories), zone measures (center, CTRCI, totcorr, DCR, hurdle, slope, BE, and bridge), activity parameters, and miscellaneous parameters. Parameters without any significant difference are not shown in the figure. AFR, animal facility reared; BE, bridge entrance; CTRCI, central circle; corr, corridor; D, duration; DCR, dark corner room; D/F, duration per visit; Dist, total distance; F frequency; MS15, maternal separation 15 min; MS360, maternal separation 360 min; n.s., non-significant; Occ, occurrence; SAP, stretched attend posture; TA, trend analysis; TOTACT, total activity; totcorr, total corridor; TS, type score; Vel, mean velocity.
In trial 2 (Figure 2A and Supplementary Table S2), MS15 rats had lower duration per visit to the slope than MS360 and AFR rats, MS360 rats performed more SAPs than MS15 rats, and a higher proportion of MS360 rats urinated in the arena compared to AFR rats. At the second trial, MS360 rats weighed less than the MS15 rats; no other body weight difference among the different rearing conditions was detected. No difference in the trend analysis categories was observed among the rearing conditions.
That the rats from the different rearing conditions have similar behavioral profiles can also be seen in the PCA analyses (Figures 3A,B, 4A,B) where the individuals in the three rearing conditions are evenly spread out in the score plots both when tested in early adolescence (Figure 3B) and early adulthood (Figure 4B).
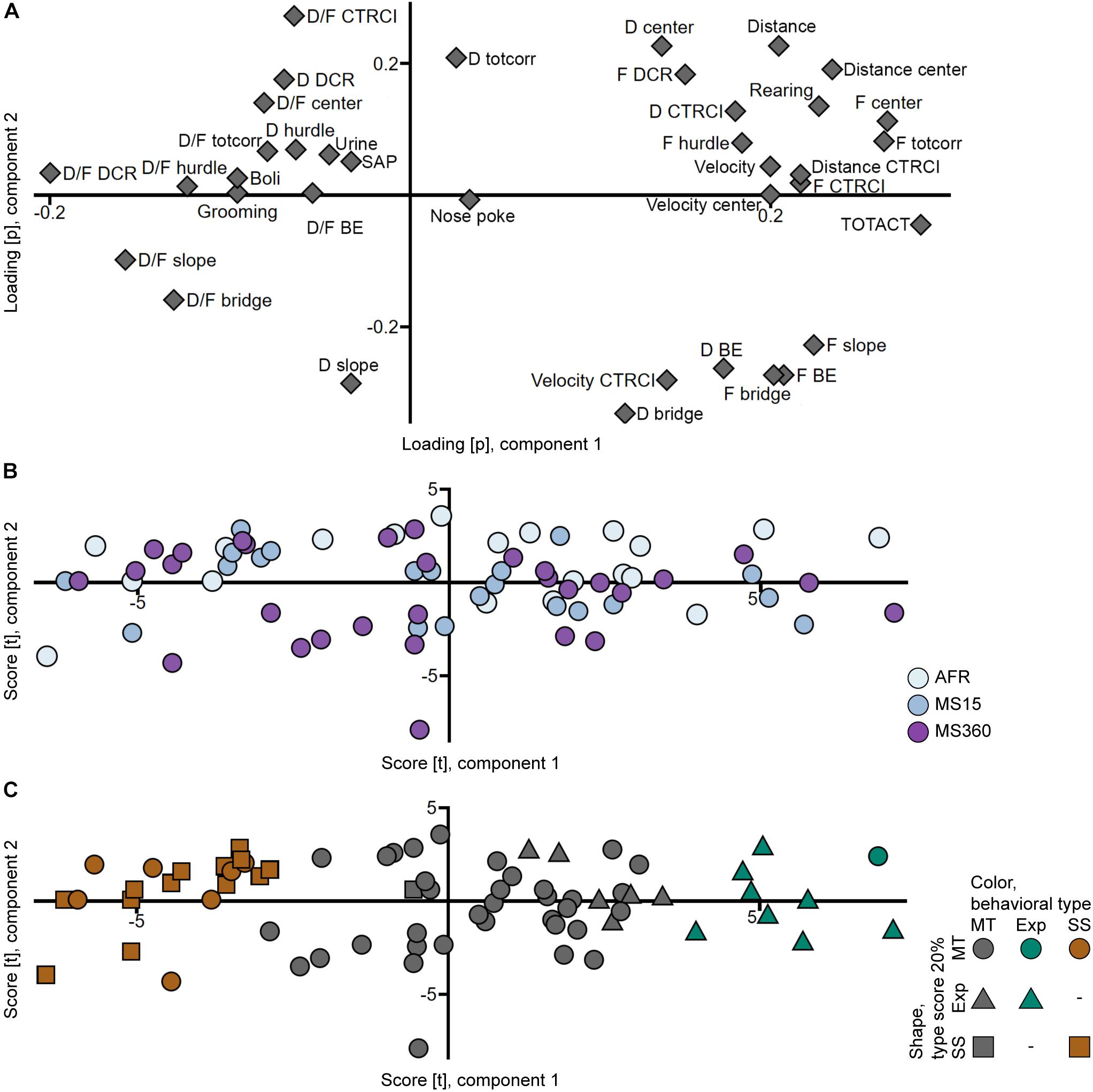
Figure 3. Scatter plots of the (A) parameter loadings and (B,C) individual scores from the adolescent PCA (n = 71, two components, R2X = 0.439, Q2 = 0.314). (B) is colored according to rearing condition and (C) according to behavioral type, and the shapes show the top 20% of each type score (exploration and shelter seeking). AFR, animal facility reared; BE, bridge entrance; CTRCI, central circle; D, duration; DCR, dark corner room; D/F, duration per visit; Exp, Explorer/exploration; F, frequency; MS15, maternal separation 15 min; MS360, maternal separation 360 min; MT, Main type; SAP, stretched attend posture; SS, Shelter seeker/seeking; TOTACT, total activity; totcorr, total corridor.
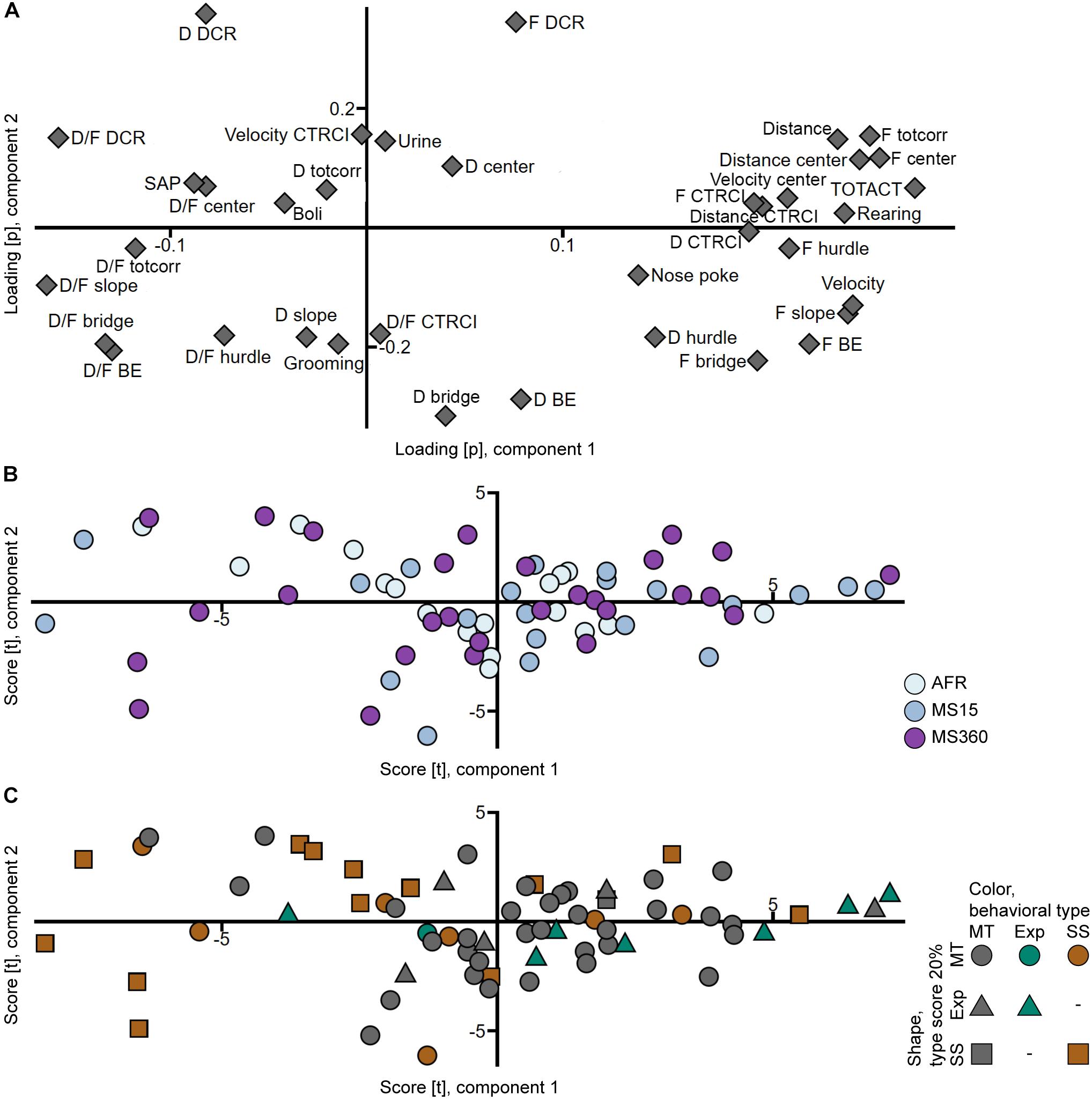
Figure 4. Scatter plots of the (A) parameter loadings and (B,C) individual scores from the adult PCA (n = 67, two components, R2X = 0.447, Q2 = 0.290). (B) is colored according to rearing condition and (C) according to behavioral type, and the shapes show the top 20% of each type score (exploration and shelter seeking). AFR, animal facility reared; BE, bridge entrance; CTRCI, central circle; D, duration; DCR, dark corner room; D/F, duration per visit; Exp, Explorer/exploration; F, frequency; MS15, maternal separation 15 min; MS360, maternal separation 360 min; MT, Main type; SAP, stretched attend posture; SS, Shelter seeker/seeking; TOTACT, total activity; totcorr, total corridor.
MCSF Performance by Behavioral Type
The top 20% individuals in each type score were identified in the trial 1 PCA score plot (Figure 3C), with the aim to replicate the finding of the behavioral types from Lundberg et al. (2019). Corresponding clusters were found but with different proportions; the 20% of individuals with the highest shelter seeking score loaded together but with additional individuals interspersed; only one individual with high shelter seeking score loaded away from this cluster. On the other hand, the 20% of individuals with the highest exploration score did not load together; some loaded separate from the Main type cluster, but several loaded intermingled with the main body of individuals. These clusters were used to classify the animals into the behavioral types Shelter seekers (20 individuals, 28%), Explorers (9 individuals, 13%), and Main type (42 individuals, 59%).
The behavioral types differed in their type scores, with Explorers having higher exploration score than Main type and Shelter seekers having lower exploration score than both Explorers and Main types. The reverse was seen for the shelter seeking score; Shelter seekers had higher shelter seeking score than both Explorers and Main type, while Explorers scored lower than the Main type (Figure 2B and Supplementary Table S3). The rearing condition did not affect which behavioral type an individual was classified as (Supplementary Table S1). However, a higher proportion of the individuals tested in the beginning of the trial 1 testing period was classified as Shelter seekers and fewer as Explorers than among the individuals tested at the end of the period (Supplementary Table S3).
When analyzing trial 1 by behavioral type, profound differences were found (see Figure 2B and Supplementary Table S3); 46 of the 75 parameters had some behavioral-type-dependent difference. Explorers had higher activity in the arena in general (higher total activity, distance, velocity, rearing, and a larger proportion that visited all zones of the arena) and in the central parts (higher frequency, duration, distance, and velocity at the center and central circle, and higher proportion that visited the central circle) than Main type animals. Explorers had higher number of visits than the Main type animals to all zones except the DCR and had lower average duration in the corridors, DCR, hurdle, and slope. Shelter seekers, on the other hand, had lower activity in the arena (lower total activity, distance, velocity, rearing, and a smaller proportion that visited all zones of the arena) than both Main type and Explorers and lower activity in the central parts of the arena (lower frequency, duration, and distance at the center and central circle) and on the entire bridge construction (lower frequency, duration, and proportion visiting the slope, bridge entrance, and bridge) than the other types. Instead, Shelter seekers had longer average visits to the corridors, DCR, hurdle, and slope, as well as fewer visits to the corridors in absolute numbers; but when correcting for the lower number of zone transits, they did percentage-wise more visits to the corridors than Main types and Explorers.
In trial 2, the Explorer behavioral type did not persist, while the Shelter seeker profile can be identified in the classical statistical analysis (Figure 2B and Supplementary Table S4), although with lower effect sizes and the clustering in the PCA score plot (Figure 4C) is not visible. However, in the PCA, most of the Shelter seekers still tend to load with lower contribution of component 1 than the other individuals (Figure 4C). In the trend analysis (Figure 2B and Supplementary Table S4), Explorers did not differ from the Main type animals, while Shelter seekers had lower general activity, exploratory activity, and risk taking than both Main type and Explorers, and lower risk assessment than Main type animals. Notably, the trend analysis category shelter seeking did not differ between the behavioral types.
MCSF Performance With Repeated Testing
The effect of repeated testing/development can be seen in Figure 2C and Supplementary Table S5. The analysis was conducted with rearing conditions collapsed since the effect of rearing condition was small in both trials. Over time, the activity increased (higher total activity, distance, rearing, and proportion visiting all zones) and the latency to leave the center and latency to the other zones (except the central circle) were lower in trial 2 than in trial 1. The duration in the corridors and in the hurdle increased, the time spent in the DCR decreased, and the duration in the other zones were unchanged between the trials. The average duration decreased between trials 1 and 2 in all zones, except the central circle where it was unchanged. In the second trial, more individuals performed nose pokes, and the number of nose pokes also increased. More urinations but fewer defecations were observed, and the proportion of individuals defecating also decreased in trial 2 relative to trial 1.
Discussion
The present study describes the MCSF behavioral profiles at 4 and 9 weeks of age in male rats subjected to different rearing conditions in the preweaning period. Only minor differences were found depending on the rearing condition, which replicates previous studies in both adolescent (Palm et al., 2013) and adult (Roman et al., 2006) male rats tested in the MCSF after MS. However, within the large individual variation in the test in early adolescence, it was possible to identify the same behavioral types as have been previously reported for adolescent animals (Lundberg et al., 2019). The present study advances the behavioral type concept further by developing, in correspondence to the trend analysis for adult animals (Meyerson et al., 2013), compound variables quantifying these behavioral types with the so-called type scores. This procedure will hopefully aid the interpretation of future studies, especially studies with a smaller number of animals, where the identification of the behavioral types can be more difficult as the number of animals in each behavioral type decreases.
The detected rearing-condition-dependent differences were few and are therefore difficult to interpret and draw conclusions from. However, the significant parameters are associated with some of the same behavioral categories as the previously described differences: risk taking in adolescent animals (Palm et al., 2013) and risk assessment in adults (Roman et al., 2006). What can be concluded from the present study is that, in early adolescence, MS males, independent of separation length, seem to have an avoidance of open areas, as indicated by the lower duration, distance, and percental duration and frequency to the center of the MCSF arena. This is in agreement with, for the MS360 animals, previous findings of behavior in the elevated plus maze (Ploj et al., 2002). However, as this is not supported by a corresponding difference among central circle parameters in the present study, the interpretation becomes less clear, and thus, the difference could also indicate decreased thigmotaxic behavior in both short and prolonged MS animals relative to AFR.
The behavioral effects of MS vary considerably between studies in both adolescence and adulthood; prolonged MS has been associated with as disparate behavioral characteristics as impulsiveness/hyperactivity (Brake et al., 2004; Colorado et al., 2006) and high anxiety- and/or depression-like behavior (Kalinichev et al., 2002; Jin et al., 2018), but no or small behavioral effects have also been reported (Shalev and Kafkafi, 2002; Marmendal et al., 2004; Farkas et al., 2009). These discrepancies can be due to the different behavioral tests used (Hånell and Marklund, 2014) and methodological differences in the MS protocols (Lehmann and Feldon, 2000; Pryce and Feldon, 2003; Nylander and Roman, 2012, 2013; Vetulani, 2013). Banqueri et al. (2017) suggested, in a direct comparison between different developmental lengths of separation, that MS limited to the first half of the preweaning period is responsible for the impulsive behavioral outcome, while MS throughout the preweaning period leads to an anxiety-like behavioral profile. Although, in our hands, using a consistent MS paradigm with separations throughout the preweaning period, we have consistently seen minor basal behavioral effects in male offspring, independent of testing age and behavioral test (Ploj et al., 2002; Roman et al., 2006; Palm et al., 2013; Lundberg et al., 2017). This is in line with the working hypothesis that this type of MS (daily 360 min separations throughout the preweaning period) leads to a phenotype predisposed to develop stress-related disorders but not overt changes in physiology and behavior without additional provocations (Nylander and Roman, 2012; Palm et al., 2013). This is supported by findings of dysregulated hypothalamic pituitary adrenal (HPA) axis reactivity in both young (Lundberg et al., 2017) and adult (Roman et al., 2006) MS360 males. However, the dysregulation seems to differ between young and adult individuals; shortly after weaning, MS360 males have increased baseline and recovery corticosterone levels (Lundberg et al., 2017), while in adulthood, it is stress-induced corticosterone levels that are blunted (Roman et al., 2006).
The fact that the identification of behavioral types in adolescent animals in the MCSF could be replicated from Lundberg et al. (2019) lends further support for this finding. In the present study, the proportion of the behavioral types differs from the initial finding; this is, however, not surprising since the present cohort is 1–2 weeks younger than in the previous study, and a higher proportion of Shelter seekers in a younger cohort is in agreement with behavioral developmental theories (Yurgelun-Todd, 2007; Doremus-Fitzwater et al., 2010; Sturman and Moghaddam, 2011). The Shelter seekers identified in early adolescence were still separable from the rest of the population in early adulthood, while the Explorer behavioral type was not. These results, together with the finding that Shelter seekers in early adulthood differed in all the trend analysis categories except precisely shelter seeking, are interesting new findings regarding how individual behavioral profiles develop with maturation. Important to note is that the trend analysis category shelter seeking only include DCR-related parameters, while the behavioral type encompass both increased shelter-seeking behavior as well as decreased activity and risk-taking behavior (Lundberg et al., 2019). That only the activity- and risk-associated differences characterizing Shelter seekers persists throughout adolescence is noteworthy and an interesting phenomenon for continued study.
What should be noted in the present study is that the light/dark cycle reversal together with weaning preceded the behavioral test in early adolescence with only 4–9 days. It is likely that these procedures affected the animals to some degree and may have played a part in the detected differences between the animals tested in the beginning and at the end of the trial 1 testing period, together with the age of the animal.
In the present study, it is impossible to detangle what differences between trials 1 and 2 that are age-dependent and what are due to repeated testing and thus established memory of the arena. Still, there were more differences in the present study between trials 1 and 2 than between individuals tested repeatedly with 1 week intertest interval in adolescence (Lundberg et al., 2019). This indicates that the present finding could be more due to developmental effects since the memory effect can be assumed to be stronger with 1 week intertest interval than with 5 weeks, even though the memory effect in adulthood has been described with testing intervals between 1 and 6 weeks (Meyerson et al., 2006; Roman et al., 2007; Karlsson et al., 2009; Roman and Colombo, 2009; Magara et al., 2015).
The effect of age in the MCSF has only been examined in a few previous studies. A comparison between males aged 5–6 weeks (mid-adolescence) and a literature cohort of males aged 10–11 weeks (early adulthood) (Lundberg et al., 2019) found clear differences between the age groups. However, the specific findings are partially contradicting to the present; mainly, the increase in activity seen in the present study is not evident in the previous work (Lundberg et al., 2019). This could, however, be attributed to the memory effect of repeated testing as discussed above. Another study, more similar to the present, found only minor-age-dependent differences following repeated testing in the MCSF after MS at week 4 (early adolescence) and week 15 (adulthood) of age (Palm et al., 2013). However, in Palm et al. (2013), the animals were housed individually during the intertest interval, which has been demonstrated to have a profound influence on behavior (Haller et al., 2014) and adolescent social isolation has also been shown to interact with MS (Biggio et al., 2014), possibly altering the behavioral outcome. It is also possible that the discrepancies between these three studies are due, in part, to an inverted u-shaped developmental trajectory making early adolescents and fully adult individuals more similar in their behavior than the intermediate developmental stages.
In conclusion, the present study replicates previous findings both regarding behavior in the MCSF after MS and adolescent behavior in the MCSF in general. The study also adds new information about developmental effects on behavior as reflected by the performance in the MCSF test.
Data Availability Statement
All datasets generated for this study are included in the article/Supplementary Material.
Ethics Statement
The animal study was reviewed and approved by the Uppsala Animal Ethical Committee in accordance with the Swedish Legislation on Animal Experimentation (Animal Welfare Act SFS1998:56) and the European Communities Council Directive (86/609/EEC).
Author Contributions
ER and IN contributed to conceptualization and design of the study and provided the funding. SL performed the statistical analysis and wrote the first draft of the manuscript. All authors contributed to manuscript revision and read and approved the submitted version.
Funding
This study was supported by funds from the Alcohol Research Council of the Swedish Alcohol Retailing Monopoly (2014-0004 to IN), the Swedish Research Council (K2012-61X-22090-01-3 to IN), and the Facias and Gahlins Foundations (to ER). The funding sources had no role in the study design; in the collection, analysis, and interpretation of the data; the writing of this report; nor the decision to submit this report for publication.
Conflict of Interest
The authors declare that the research was conducted in the absence of any commercial or financial relationships that could be construed as a potential conflict of interest.
Acknowledgments
The authors are grateful for the skillful technical assistance of Dr. Linnea Granholm and Dr. Lova Segerström.
Supplementary Material
The Supplementary Material for this article can be found online at: https://www.frontiersin.org/articles/10.3389/fnbeh.2020.00037/full#supplementary-material
References
Bale, T. L., Abel, T., Akil, H., Carlezon, W. A. Jr., Moghaddam, B., Nestler, E. J., et al. (2019). The critical importance of basic animal research for neuropsychiatric disorders. Neuropsychopharmacology 44, 1349–1353. doi: 10.1038/s41386-019-0405-9
Banqueri, M., Méndez, M., and Arias, J. L. (2017). Behavioral effects in adolescence and early adulthood in two length models of maternal separation in male rats. Behav. Brain Res. 324, 77–86. doi: 10.1016/j.bbr.2017.02.006
Bendre, M., Comasco, E., Nylander, I., and Nilsson, K. W. (2015). Effect of voluntary alcohol consumption on Maoa expression in the mesocorticolimbic brain of adult male rats previously exposed to prolonged maternal separation. Transl. Psychiatry 5:e690. doi: 10.1038/tp.2015.186
Bendre, M., Granholm, L., Drennan, R., Meyer, A., Yan, L., Nilsson, K. W., et al. (2018). Early life stress and voluntary alcohol consumption in relation to Maoa methylation in male rats. Alcohol 79, 7–16. doi: 10.1016/j.alcohol.2018.11.001
Bick, J., and Nelson, C. A. (2016). Early adverse experiences and the developing brain. Neuropsychopharmacology 41, 177–196. doi: 10.1038/npp.2015.252
Biggio, F., Pisu, M. G., Garau, A., Boero, G., Locci, V., Mostallino, M. C., et al. (2014). Maternal separation attenuates the effect of adolescent social isolation on HPA axis responsiveness in adult rats. Eur. Neuropsychopharmacol. 24, 1152–1161. doi: 10.1016/j.euroneuro.2014.03.009
Brake, W. G., Zhang, T. Y., Diorio, J., Meaney, M. J., and Gratton, A. (2004). Influence of early postnatal rearing conditions on mesocorticolimbic dopamine and behavioural responses to psychostimulants and stressors in adult rats. Eur. J. Neurosci. 19, 1863–1874. doi: 10.1111/j.1460-9568.2004.03286.x
Brown, R. E., and Bolivar, S. (2018). The importance of behavioural bioassays in neuroscience. J. Neurosci. Methods 300, 68–76. doi: 10.1016/j.jneumeth.2017.05.022
Colorado, R. A., Shumake, J., Conejo, N. M., Gonzalez-Pardo, H., and Gonzalez-Lima, F. (2006). Effects of maternal separation, early handling, and standard facility rearing on orienting and impulsive behavior of adolescent rats. Behav. Process. 71, 51–58. doi: 10.1016/j.beproc.2005.09.007
Comasco, E., Todkar, A., Granholm, L., Nilsson, K. W., and Nylander, I. (2015). A lpha 2a-adrenoceptor gene expression and early life stress-mediated propensity to alcohol drinking in outbred rats. Int. J. Environ. Res. Public Health 12, 7154–7171. doi: 10.3390/ijerph120707154
Doremus-Fitzwater, T. L., Varlinskaya, E. I., and Spear, L. P. (2010). Motivational systems in adolescence: possible implications for age differences in substance abuse and other risk-taking behaviors. Brain Cogn. 72, 114–123. doi: 10.1016/j.bandc.2009.08.008
Ehlert, U. (2013). Enduring psychobiological effects of childhood adversity. Psychoneuroendocrinology 38, 1850–1857. doi: 10.1016/j.psyneuen.2013.06.007
Farkas, J., Reglodi, D., Gaszner, B., Szogyi, D., Horvath, G., Lubics, A., et al. (2009). Effects of maternal separation on the neurobehavioral development of newborn Wistar rats. Brain Res. Bull. 79, 208–214. doi: 10.1016/j.brainresbull.2008.12.011
Fritz, C. O., Morris, P. E., and Richler, J. J. (2012). Effect size estimates: current use, calculations, and interpretation. J. Exp. Psychol. Gen. 141, 2–18. doi: 10.1037/a0024338
Granholm, L., Todkar, A., Bergman, S., Nilsson, K., Comasco, E., and Nylander, I. (2017). The expression of opioid genes in non-classical reward areas depends on early life conditions and ethanol intake. Brain Res. 1668, 36–45. doi: 10.1016/j.brainres.2017.05.006
Haller, J., Harold, G., Sandi, C., and Neumann, I. D. (2014). Effects of adverse early-life events on aggression and anti-social behaviours in animals and humans. J. Neuroendocrinol. 26, 724–738. doi: 10.1111/jne.12182
Hånell, A., and Marklund, N. (2014). Structured evaluation of rodent behavioral tests used in drug discovery research. Front. Behav. Neurosci. 8:252. doi: 10.3389/fnbeh.2014.00252
Jin, S., Zhao, Y., Jiang, Y., Wang, Y., Li, C., Zhang, D., et al. (2018). Anxiety-like behaviour assessments of adolescent rats after repeated maternal separation during early life. Neuroreport 29, 643–649. doi: 10.1097/WNR.0000000000001010
Kafkafi, N., Agassi, J., Chesler, E. J., Crabbe, J. C., Crusio, W. E., Eilam, D., et al. (2018). Reproducibility and replicability of rodent phenotyping in preclinical studies. Neurosci. Biobehav. Rev. 87, 218–232. doi: 10.1016/j.neubiorev.2018.01.003
Kalinichev, M., Easterling, K. W., Plotsky, P. M., and Holtzman, S. G. (2002). Long-lasting changes in stress-induced corticosterone response and anxiety-like behaviors as a consequence of neonatal maternal separation in Long–Evans rats. Pharmacol. Biochem. Behav. 73, 131–140. doi: 10.1016/S0091-3057(02)00781-5
Karlsson, O., and Roman, E. (2016). Dose-dependent effects of alcohol administration on behavioral profiles in the MCSF test. Alcohol 50, 51–56. doi: 10.1016/j.alcohol.2015.10.003
Karlsson, O., Roman, E., and Brittebo, E. B. (2009). Long-term cognitive impairments in adult rats treated neonatally with β-N-Methylamino-L-Alanine. Toxicol. Sci. 112, 185–195. doi: 10.1093/toxsci/kfp196
Lehmann, J., and Feldon, J. (2000). Long-term biobehavioral effects of maternal separation in the rat: consistent or confusing? Rev. Neurosci. 11, 383–408.
Lundberg, S., Högman, C., and Roman, E. (2019). Adolescent exploratory strategies and behavioral types in the multivariate concentric square field(TM) test. Front. Behav. Neurosci. 13:41. doi: 10.3389/fnbeh.2019.00041
Lundberg, S., Martinsson, M., Nylander, I., and Roman, E. (2017). Altered corticosterone levels and social play behavior after prolonged maternal separation in adolescent male but not female Wistar rats. Horm. Behav. 87, 137–144. doi: 10.1016/j.yhbeh.2016.11.016
Magara, S., Holst, S., Lundberg, S., Roman, E., and Lindskog, M. (2015). Altered explorative strategies and reactive coping style in the FSL rat model of depression. Front. Behav. Neurosci. 9:89. doi: 10.3389/fnbeh.2015.00089
Maniam, J., Antoniadis, C., and Morris, M. J. (2014). Early-life stress, HPA axis adaptation, and mechanisms contributing to later health outcomes. Front. Endocrinol. 5:73. doi: 10.3389/fendo.2014.00073
Marmendal, M., Roman, E., Eriksson, C. J. P., Nylander, I., and Fahlke, C. (2004). Maternal separation alters maternal care, but has minor effects on behavior and brain opioid peptides in adult offspring. Dev. Psychobiol. 45, 140–152. doi: 10.1002/dev.20027
Meyerson, B. J., Augustsson, H., Berg, M., and Roman, E. (2006). The concentric square field: a multivariate test arena for analysis of explorative strategies. Behav. Brain Res. 168, 100–113. doi: 10.1016/j.bbr.2005.10.020
Meyerson, B. J., Jurek, B., and Roman, E. (2013). A rank-order procedure applied to an ethoexperimental behavior model - the Multivariate Concentric Square Field (MCSF) test. J. Behav. Brain Sci. 3, 350–361. doi: 10.4236/jbbs.2013.34035
Nylander, I., and Roman, E. (2012). Neuropeptides as mediators of the early-life impact on the brain; implications for alcohol use disorders. Front. Mol. Neurosci. 5:77. doi: 10.3389/fnmol.2012.00077
Nylander, I., and Roman, E. (2013). Is the rodent maternal separation model a valid and effective model for studies on the early-life impact on ethanol consumption? Psychopharmacology 229, 555–569. doi: 10.1007/s00213-013-3217-3
Nylander, I., Todkar, A., Granholm, L., Vrettou, M., Bendre, M., Boon, W., et al. (2016). Evidence for a link between Fkbp5/FKBP5, early life social relations and alcohol drinking in young adult rats and humans. Mol. Neurobiol. 54, 6225–6234. doi: 10.1007/s12035-016-0157-z
Palm, S., Daoura, L., Roman, E., and Nylander, I. (2013). Effects of rearing conditions on behaviour and endogenous opioids in rats with alcohol access during adolescence. PLoS One 8:e76591. doi: 10.1371/journal.pone.0076591
Ploj, K., Roman, E., and Nylander, I. (2002). Effects of maternal separation on brain nociceptin/orphanin FQ peptide levels in male Wistar rats. Pharmacol. Biochem. Behav. 73, 123–129. doi: 10.1016/s0091-3057(02)00778-5
Pryce, C. R., and Feldon, J. (2003). Long-term neurobehavioural impact of the postnatal environment in rats: manipulations, effects and mediating mechanisms. Neurosci. Biobehav. Rev. 27, 57–71. doi: 10.1016/s0149-7634(03)00009-5
Roman, E., and Colombo, G. (2009). Lower risk taking and exploratory behavior in alcohol-preferring sP rats than in alcohol non-preferring sNP rats in the multivariate concentric square field (MCSF) test. Behav. Brain Res. 205, 249–258. doi: 10.1016/j.bbr.2009.08.020
Roman, E., Gustafsson, L., Berg, M., and Nylander, I. (2006). Behavioral profiles and stress-induced corticosteroid secretion in male Wistar rats subjected to short and prolonged periods of maternal separation. Horm. Behav. 50, 736–747. doi: 10.1016/j.yhbeh.2006.06.016
Roman, E., Meyerson, B. J., Hyytiä, P., and Nylander, I. (2007). The multivariate concentric square field test reveals different behavioural profiles in male AA and ANA rats with regard to risk taking and environmental reactivity. Behav. Brain Res. 183, 195–205. doi: 10.1016/j.bbr.2007.06.009
Shalev, U., and Kafkafi, N. (2002). Repeated maternal separation does not alter sucrose-reinforced and open-field behaviors. Pharmacol. Biochem. Behav. 73, 115–122. doi: 10.1016/S0091-3057(02)00756-6
Sturman, D. A., and Moghaddam, B. (2011). The neurobiology of adolescence: changes in brain architecture, functional dynamics, and behavioral tendencies. Neurosci. Biobehav. Rev. 35, 1704–1712. doi: 10.1016/j.neubiorev.2011.04.003
Todkar, A., Granholm, L., Aljumah, M., Nilsson, K. W., Comasco, E., and Nylander, I. (2016). HPA axis gene expression and DNA methylation profiles in rats exposed to early life stress, adult voluntary ethanol drinking and single housing. Front. Mol. Neurosci. 8:90. doi: 10.3389/fnmol.2015.00090
Vetulani, J. (2013). Early maternal separation: a rodent model of depression and a prevailing human condition. Pharmacol. Rep. 65, 1451–1461. doi: 10.1016/S1734-1140(13)71505-6
Vrettou, M., Granholm, L., Todkar, A., Nilsson, K. W., Wallén-Mackenzie, Å., Nylander, I., et al. (2017). Ethanol affects limbic and striatal presynaptic glutamatergic and DNA methylation gene expression in outbred rats exposed to early-life stress. Addict. Biol. 22, 369–380. doi: 10.1111/adb.12331
Keywords: adolescence, adult, behavior, early-life stress, handling, juvenile, maternal deprivation, multivariate concentric square field
Citation: Lundberg S, Nylander I and Roman E (2020) Behavioral Profiling in Early Adolescence and Early Adulthood of Male Wistar Rats After Short and Prolonged Maternal Separation. Front. Behav. Neurosci. 14:37. doi: 10.3389/fnbeh.2020.00037
Received: 05 December 2019; Accepted: 21 February 2020;
Published: 19 March 2020.
Edited by:
Carla Cannizzaro, University of Palermo, ItalyReviewed by:
Olga M. Bazanova, State Scientific-Research Institute of Physiology & Basic Medicine, RussiaSara Palumbo, University of Pisa, Italy
Copyright © 2020 Lundberg, Nylander and Roman. This is an open-access article distributed under the terms of the Creative Commons Attribution License (CC BY). The use, distribution or reproduction in other forums is permitted, provided the original author(s) and the copyright owner(s) are credited and that the original publication in this journal is cited, in accordance with accepted academic practice. No use, distribution or reproduction is permitted which does not comply with these terms.
*Correspondence: Stina Lundberg, U3RpbmEuTHVuZGJlcmdAZmFybWJpby51dS5zZQ==