- Department of Neuroscience, Medical University of South Carolina, Charleston, SC, United States
Drug addiction is a neuropsychiatric disorder with grave personal consequences that has an extraordinary global economic impact. Despite decades of research, the options available to treat addiction are often ineffective because our rudimentary understanding of drug-induced pathology in brain circuits and synaptic physiology inhibits the rational design of successful therapies. This understanding will arise first from animal models of addiction where experimentation at the level of circuits and molecular biology is possible. We will review the most common preclinical models of addictive behavior and discuss the advantages and disadvantages of each. This includes non-contingent models in which animals are passively exposed to rewarding substances, as well as widely used contingent models such as drug self-administration and relapse. For the latter, we elaborate on the different ways of mimicking craving and relapse, which include using acute stress, drug administration or exposure to cues and contexts previously paired with drug self-administration. We further describe paradigms where drug-taking is challenged by alternative rewards, such as appetitive foods or social interaction. In an attempt to better model the individual vulnerability to drug abuse that characterizes human addiction, the field has also established preclinical paradigms in which drug-induced behaviors are ranked by various criteria of drug use in the presence of negative consequences. Separation of more vulnerable animals according to these criteria, along with other innate predispositions including goal- or sign-tracking, sensation-seeking behavior or impulsivity, has established individual genetic susceptibilities to developing drug addiction and relapse vulnerability. We further examine current models of behavioral addictions such as gambling, a disorder included in the DSM-5, and exercise, mentioned in the DSM-5 but not included yet due to insufficient peer-reviewed evidence. Finally, after reviewing the face validity of the aforementioned models, we consider the most common standardized tests used by pharmaceutical companies to assess the addictive potential of a drug during clinical trials.
Introduction
“All models are wrong, some models are useful”.
George Box
The main difficulty with modeling drug addiction using nonhuman animals is capturing an inherently complex behavioral pathology using relatively simple behavioral protocols (Spanagel, 2017; Müller, 2018). Environmental circumstances, behavioral traits and genetic factors all interplay with one another and affect an individual’s susceptibility to acquiring and maintaining the use of an addictive substance, as well as relapse propensity (Everitt and Robbins, 2016). Regardless, creating better preclinical models of drug addiction is essential for elucidating the neurobiological mechanisms that contribute to addiction-related behaviors, and creating better treatment options for those afflicted with addiction. In this review article, we build on the existing literature (García Pardo et al., 2017; Lynch, 2018) and discuss the various models that exist for studying addiction-related behaviors in animals, including individual variation in addiction-related behaviors, and commonalities between drug addiction and certain behavioral addictions. We then focus on how these models are used to assess abuse potential by pharmaceutical companies.
In models such as behavioral sensitization and conditioned place preference (CPP), an animal is non-contingently administered a drug, allowing drug delivery independent from the motivation to take the drug to be assessed. More commonly used are drug self-administration (SA) models, whereby drug delivery is contingent upon the animal’s motivation to take the drug. SA models are constantly evolving, with the emphasis being placed on the importance of the duration of drug experience (i.e., session length) and the temporal pattern of drug delivery as a means to better model the transition to addiction. The motivation for drug-taking can also be assessed using multisymptomatic training paradigms and behavioral economics. Factors that contribute to reinstated drug-seeking behavior, including environmental contexts, cues, stressors and the drug itself are briefly discussed, along with procedures where drugs are challenged by alternative rewards.
Independent of modeling addiction-related behaviors, we emphasize the importance and advantages of modeling individual variation within these behaviors, as is seen in humans. For example, measures of impulsivity have been shown to predict addiction liability. We also discuss two well-established models that capture individual variation in addiction-related behaviors: the high-responder/low-responder model, and the sign-tracker/goal-tracker model. While the high-responder/low-responder model captures individual variation in the acquisition of drug-taking behavior, the sign-tracker/goal-tracker model captures individual variation in relapse propensity. Thus, the two models independently capture two different phases of addiction.
Next, we discuss behavioral addictions that share several similarities with drug addiction, with an emphasis on gambling disorder. There is a high prevalence rate between those diagnosed with gambling disorder and those with a substance abuse disorder (17% for illicit drugs, 28% for alcohol dependence according to Lorains et al., 2011) and not surprisingly the criteria to diagnose the two are very similar (for review see Rash et al., 2016). As with drug addiction, accurately modeling behaviors associated with gambling disorder using rodents are complex. The growing prevalence and efforts to assess and diagnose exercise addiction are also briefly discussed.
We end this review with a discussion on the models used by pharmaceutical companies to assess the abuse potential of possible medications for the treatment of addiction. It is for this reason that critical evaluation of models used to assess addiction-related behaviors is particularly necessary, as these models are being used to evaluate treatment efficacy.
Non-Contingent Models: Behavioral Sensitization, Conditioned Place Preference and Runway Model
Models based on non-contingent (i.e., experimenter-administered) drug exposure are simple and quick to set up. Because of these advantages, many studies have used them to identify key reward-related neurobiological substrates and how drug exposure alters them. See Table 1 for a succinct summary of advantages and limitations of these models.
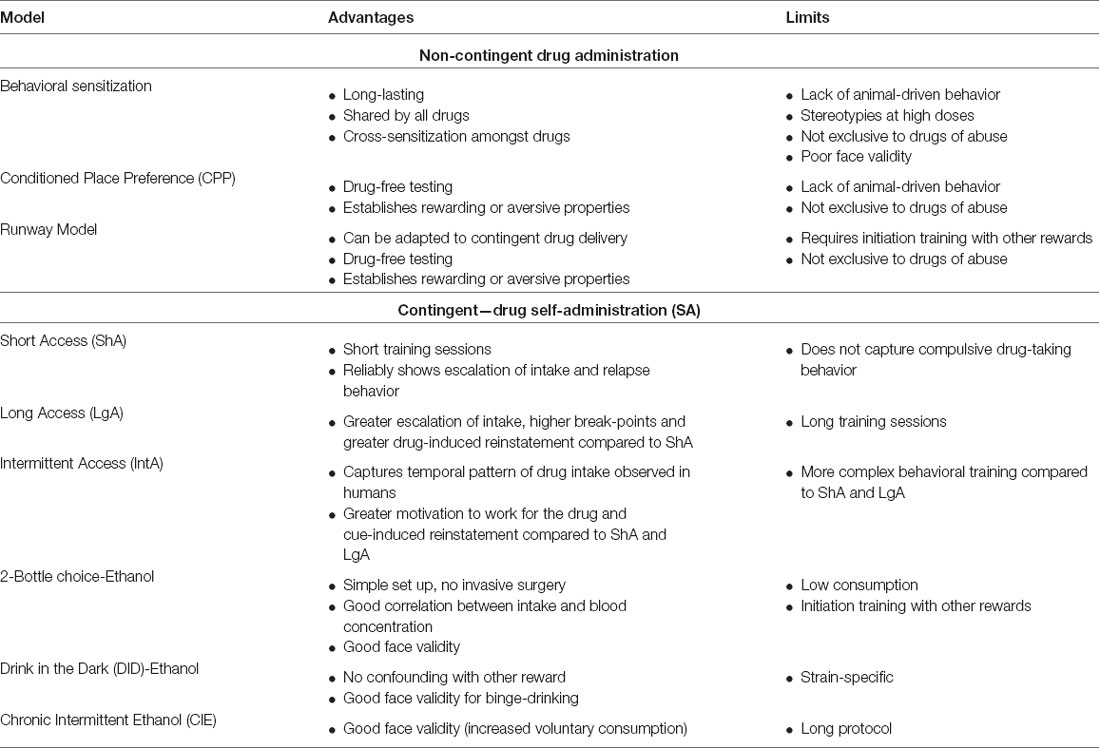
Table 1. Brief summary of preclinical behavioral models most currently used to study the neurobiology of addiction: non-contingent drug administration and contingent drug self-administration (SA).
Behavioral Sensitization
The behavioral sensitization model is at the heart of the incentive-sensitization theory of addiction developed by Robinson and Berridge (1993). The model is based on the potentiation of drug-induced locomotion after repeated non-contingent exposure to a constant drug dose. Behavioral sensitization is usually divided into two phases: the induction (or initiation) and the expression phase. During the induction phase, it is possible to measure the molecular and cellular modifications directly induced by drug exposure. The expression, tested with a drug challenge and after a variable withdrawal, is generally attributed to the long-term effects of the aforementioned drug-induced changes. Behavioral sensitization requires D1-dopaminergic receptor activation in the ventral tegmental area (VTA; Vezina, 1996) and AMPA-mediated glutamatergic transmission in the nucleus accumbens (NAc), the latter being shared with most models of drug seeking (Bell and Kalivas, 1996; Pierce et al., 1996; Famous et al., 2008). Sensitization to all drugs of abuse has also been shown to correlate with a sustained hyper-reactivity of noradrenergic and serotonergic systems in the locus coeruleus and dorsal raphe, respectively (Tassin, 2008; Doucet et al., 2013).
Behavioral sensitization has many advantages. First, drug delivery is simple, as it relies on experimenter-administered intraperitoneal injections. Depending on the experimental timeline and withdrawal periods, sensitization can be rapidly induced since potentiation can be measured after only a few drug injections, or even a single injection (Magos, 1969; Robinson et al., 1982; Vanderschuren et al., 1999a; Valjent et al., 2010). However, it is possible to use sensitization to study the long-term effects of chronic drug-exposure, one rat study showing amphetamine sensitization lasting up to a year (Paulson et al., 1991). But beyond these technical strengths, an important characteristic of behavioral sensitization is that most drugs of abuse, including cocaine (Post and Rose, 1976), amphetamine (Segal and Mandell, 1974), morphine (Babbini and Davis, 1972), ethanol [in certain mouse strains only, never shown in rats (Didone et al., 2008; Bahi and Dreyer, 2012a,b)], and nicotine (Clarke and Kumar, 1983), induce sensitization in rodents. Notably, no behavioral sensitization has been shown with Δ9-tetrahydrocannabinol (THC; Varvel et al., 2007). Throughout the drug spectrum, sensitization remains sensitive to individual vulnerabilities to stress and genetics factors, as demonstrated by the wide range of behaviors observed with different rodent strains (Phillips et al., 1997).
Most importantly, drugs often cross-sensitize, which means that potentiated response to one drug is observed after induction with another drug. Cross-sensitization has indeed been observed between psychostimulants such as amphetamine and cocaine (Bonate et al., 1997; Vanderschuren et al., 1999a), but also across different drug classes like amphetamine and morphine (Vezina and Stewart, 1990; Vanderschuren et al., 1997), with the exception of one study in rats where cross-sensitization was lacking (Vanderschuren et al., 1999b). Surprisingly, although THC does not induce behavioral sensitization as mentioned earlier (Varvel et al., 2007), it cross-sensitizes with opioids (Lamarque et al., 2001; Cadoni et al., 2008) and amphetamine (Lamarque et al., 2001) in rats. However, cannabinoid agonist HU 210 failed to cross-sensitize with morphine or alcohol (Hagues et al., 2008). Interestingly, pre-exposing animals to drugs, thus inducing sensitization, potentiates CPP (Lett, 1989; Gaiardi et al., 1991; Shippenberg and Heidbreder, 1995; Shippenberg et al., 1996; Meririnne et al., 2001; Harris and Aston-Jones, 2003a,b) and SA behaviors (Horger et al., 1990, 1992; Piazza et al., 1990; Valadez and Schenk, 1994; Pierre and Vezina, 1998; Covington and Miczek, 2001) compared to non-sensitized controls. These results support the idea that chronic non-contingent drug exposure used in the sensitization model allows studying the neurobiological pathways shared by all drugs.
Despite all the advantages of this model, behavioral sensitization faces major downsides that contributed to the field increasingly shifting towards other models. Before all else, the face validity of this model is limited as sensitization in humans is challenging to demonstrate, although some studies measured a potentiation of certain symptoms such as activity and energy levels, mood or speech quantity after repeated amphetamine challenges that could be compared to behavioral sensitization (Strakowski et al., 1996; Strakowski and Sax, 1998; Boileau et al., 2006). Chronic amphetamine use has also been linked to progressive augmentation in paranoid behaviors (Kalivas and Stewart, 1991). Another characteristic of this model contrasting with the clinic resides in the fact that only a few drug injections can induce sensitization in rodents, whereas in humans a large number of exposures over time often precede abuse. Because of this, it has been argued that this model can be useful to better understand the initial phases of drug intake that influence, but does not provide a complete picture, of the transition to substance use disorder (Vanderschuren and Pierce, 2010). Finally, cross-sensitization is not limited to drugs, but also extends to stressors such as foot shock (Herman et al., 1984; Sorg, 1992), restrain stress (Robinson et al., 1985) or tail pinch (Antelman et al., 1980). In addition to stress, amphetamine-sensitized animals show facilitation of sexual behavior (Fiorino and Phillips, 1999a,b), whereas rats sensitized to morphine display increased interest for food, as well as sexual and social behaviors (Nocjar and Panksepp, 2007). These results suggest that drug-sensitization induces an unspecific activation of the reward pathways, which contradicts Diagnostic and Statistical Manual (DSM) criteria of addicted individual’s disinterest for natural, social or professional rewards (APA, 2013).
Conditioned Place Preference (CPP)
CPP (also known as place preference conditioning, or PPC) allows testing the rewarding or aversive properties of an experience or a stimulus. A multitude of variations of the CPP model exist, yet the standard use remains to associate an experience, such as non-contingent drug delivery, to a recognizable context, often a chamber with definitive cues identifiable by the animal. In parallel, animals are also exposed to a different neutral context. After a first phase of pre-conditioning to assert no innate preference of the animal for one of the contexts, the acquisition phase consists of pairing the drug to one context. Pairing can be achieved after single or repeated exposures, depending on the drug doses or timing exposures. On test day, or post-conditioning phase, the animal is allowed to explore the contexts freely. When the time spent in the paired context is longer to the time spent in the unpaired one, the stimulus is concluded to be rewarding. The opposite result grants aversive effects to the stimulus, defined as Conditioned Place Aversion (CPA). CPP was first shown with morphine (Beach, 1957), and a multitude of studies later showed that all drugs of abuse, including cocaine, amphetamine, methamphetamine, nicotine, alcohol and cannabis also induced CPP (Bardo and Bevins, 2000; García Pardo et al., 2017). Along with SA, CPP is the most commonly used model to test abuse potential of a new drug during clinical trials (see later section). The popularity and abundant use of this model relies on its simplicity and promptness. Moreover, the drug of interest is typically not administered on testing day, thus allowing the determination of its rewarding properties and measurement of enduring neurobiological changes induced by the drug without causing massive neurotransmitter release. It also provides a tool to definitively establish aversive effects through avoidance, which lacks in the self-administering paradigm (i.e., lack of responding to a drug can be interpreted as the drug having aversive or neutral properties). Importantly, individual differences and susceptibilities to the rewarding effects of drugs can be measured using this paradigm. While reviewing how the novelty-seeking endophenotype impacts psychostimulant responses, Arenas et al. (2016) summarized the potentiated responses of high responding rats to sub-threshold doses of amphetamine and cocaine compared to low responding animals (see the “Individual Variation in Addiction-Related Behaviors” section below for more detailed definition of the High-responder/Low-responder model). This potentiated response could be linked to the corticotropin-releasing factor (CRF) since a study found that mice continually overexpressing CRF show potentiated cocaine CPP only in mice displaying low behavioral reactivity to novelty in contrast to mice with high locomotor response to novelty (Kasahara et al., 2015). More recently, a study established a positive correlation between rats exhibiting risk-taking behaviors and methamphetamine-induced CPP (Takahashi et al., 2019), corroborating the ability to detect individual differences using this paradigm. However, CPP is not specific to abused substances, since natural rewards such as food (Cason et al., 2010), novelty (Klebaur and Bardo, 1999), physical exercise (wheel running; Antoniadis et al., 2000) and sexual behavior (Paredes, 2009) induce robust CPP. Similar to the behavioral sensitization and SA models, CPP heavily relies on motor capacities, thus requiring appropriate controls to assess possible sedative or anxiolytic effects of the drug that are not present when testing the animal in a drug-free state.
Using receptor-specific agonists and antagonists, many studies (for an extensive review see Tzschentke, 2007) have established a necessary role of the usual suspects within reward circuitry (dopaminergic, glutamatergic, GABergic, cholinergic, noradrenergic and serotonergic systems) in the induction and maintenance of CPP. Consistent with this idea of shared reward-related mechanisms, inducing CPP often potentiates the behavior observed in other models of addiction as seen in a study where expression of cocaine behavioral sensitization is only observed in the compartment paired with the drug during CPP, and not in a novel compartment (Duvauchelle et al., 2000). Using CPP and CPA can also help elucidate heterogeneous behaviors in other models. Supporting this, the authors of a study comparing animals self-administering cocaine at a high and stable level to animals failing to do so elegantly show that self-administering animals exhibit cocaine CPP, while non-self-administering ones show CPA (Rademacher et al., 2000). These results argue that cocaine effects are appetitive for some animals but aversive for others. However, inducing CPP with one reward does not always predict a potentiated reward-induced behavior in other models, as reported in a study that found no correlation between the magnitude of novelty-induced CPP and the degree of amphetamine SA (Klebaur et al., 2001). Another study reported that ethanol consumption during the SA pre-exposure phase negatively correlates with ethanol-induced CPP in mice (Nocjar et al., 1999).
In some studies, CPP is also used to model relapse and dissect the neurobiology of drug-seeking (for extensive review, see Aguilar et al., 2009). Prior to CPP reinstatement, animals undergo extinction training, consisting in either exposing the animal to the previously reward-paired context without administering the reward or administering sham injections in the paired and unpaired contexts (Epstein et al., 2006; Aguilar et al., 2009). CPP reinstatement, shown for cocaine, amphetamine, methamphetamine, morphine, heroin, nicotine, ethanol and MDMA, can be induced by a priming dose of drug or different types of stress, including footshock, immobilization and forced swim (Liu et al., 2008; Aguilar et al., 2009). A study further demonstrated robust cocaine CPP reinstatement induced by conditioned fear stimuli, in this case an odor or a tone previously paired with footshock (Sanchez and Sorg, 2001). In a study testing the establishment, maintenance, extinction and reinstatement of cocaine CPP, authors showed that once developed, place preference endures for several weeks and is rapidly reinstated after extinction training following cocaine priming injections (Mueller and Stewart, 2000). Similar to the induction and maintenance part of CPP, several neurotransmitters have been shown to drive reinstatement, including glutamatergic, dopaminergic and noradrenergic transmissions. The role of these systems seems to be highly dependent on reinstatement modality, i.e., drug priming or stress (Aguilar et al., 2009).
The Runway Model
The runway model has been used to evaluate opponent aversive and rewarding components of drugs. It was developed to study goal-directed behaviors with natural rewards (Hull, 1934; Crespi, 1942; Miller, 1944). From the start compartment, animals learn to cross a 6-foot long straight corridor (the runway) to reach the goal compartment, where the reward is delivered (Ettenberg, 2004, 2009). Prior to drug exposure, food-deprived animals are trained to enter the goal compartment for food pellets. Subsequently, entering the goal compartment is associated with drug delivery. The run time, i.e., the time the trained animal takes to reach the goal compartment, is the resultant of approach and avoidance behaviors and is interpreted as an index of motivation/aversion for the reward. While in some cases the drug-seeking is delivered in a noncontingent fashion, the model has been adapted to more recent techniques such as i.v. drug self-administration (Geist and Ettenberg, 1990) or optogenetics (Jhou et al., 2013). The runway model allows characterization of the ambivalent properties of drugs, which have been shown with most drugs including cocaine, amphetamine, heroin, morphine, MDMA and ethanol (for review see Ettenberg, 2009).
Contingent Models of Addiction-Related Behaviors
The models presented here rely on operant learning during repeated exposure to the drug of interest. During SA sessions an animal performs an action (e.g., lever deflection or nose port entry) in order to receive an infusion of the drug. There is consensus in the addiction field that initial exposures to the drug mainly impact the prefrontal cortex (PFC), driving goal-directed behavior, and the mesolimbic regions, including the NAc, are key regions in the integration of reinforcing stimuli (Hopf and Lesscher, 2014). As the training develops, the dorsal striatum has been shown to take a major role in maintaining drug intake (Belin and Everitt, 2008). Below we discuss the main SA paradigms currently used in drug addiction research, followed by a discussion on the various ways to assess the motivation for taking a drug and relapse propensity. See Table 1 for a brief summary of advantages and limitations of drug self-administration models and Table 2 for models of motivation and drug-seeking and relapse models mentioned below.
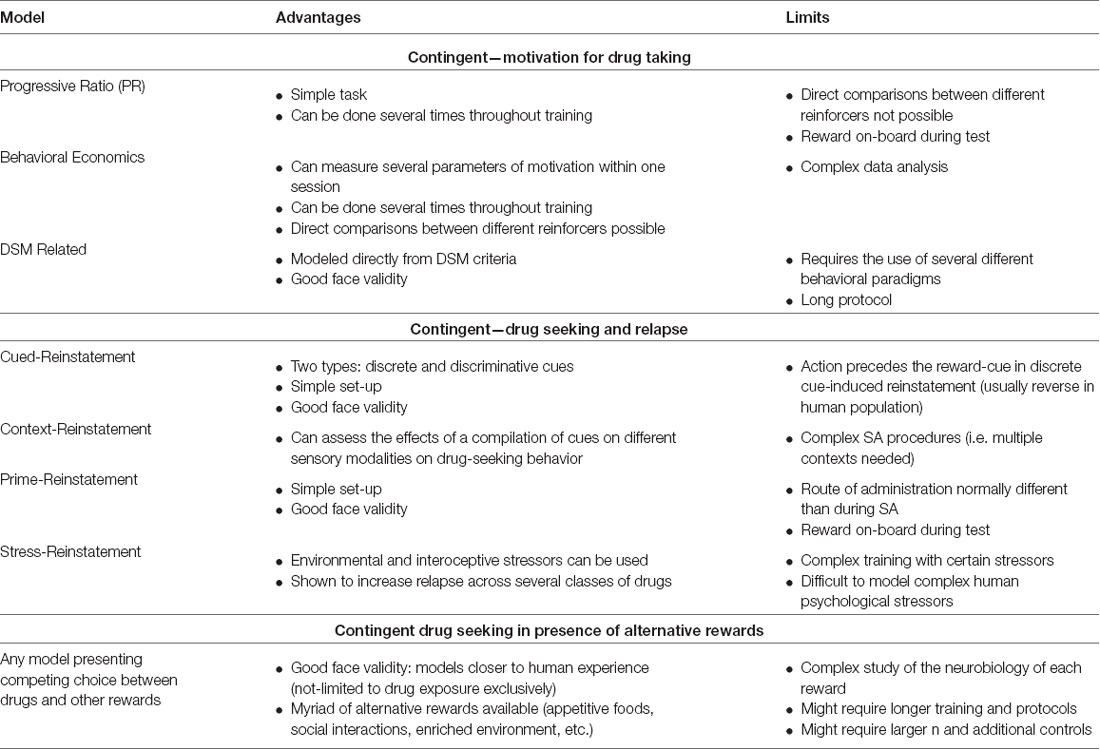
Table 2. Brief summary of preclinical behavioral models most currently used to study the neurobiology of addiction: motivation and seeking in contingent models, models including alternative rewards.
Drug Self-administration Models
Animal drug SA paradigms have significantly evolved since the inception of the technique in 1962 (Weeks, 1962), as more attempts are being put forth to more accurately model drug-taking behavior in humans. A commonly used paradigm, short-access (ShA) training, involves SA sessions that generally last between 1 and 3 h. ShA paradigms reliably show an increase in drug-taking behavior and reinstatement of drug-seeking behavior, two features of human addiction. However, despite its proliferous use, arguments have been made that ShA sessions capture drug-taking behavior, but not behavior that is representative of the transition to addiction (Ahmed and Koob, 1998). That is to say, ShA may only address recreational drug use, and not the escalation to compulsive drug taking that is seen in human addicts (for review see Roberts et al., 2007). To address this limitation, a long-access (LgA; Spanagel et al., 1996) training procedure was developed that consists of SA training sessions lasting a minimum of 6 h (Ahmed and Koob, 1998), with some lasting as long as 12 h (Lucantonio et al., 2015; Cocker et al., 2019). Compared to ShA sessions, rats undergoing LgA training show greater escalation in drug-taking behavior (Ahmed and Koob, 1998; Mantsch et al., 2004; Mandt et al., 2015), are more motivated to work for an infusion of cocaine (Paterson and Markou, 2003; Hao et al., 2010), and show greater cocaine-primed drug-seeking behavior (Mantsch et al., 2004; Knackstedt and Kalivas, 2007). The neurobiological mechanisms mediating behavior as a result of these two training paradigms also appear to differ, specifically in respect to neuroplasticity within the striatum (Purgianto et al., 2013; Ducret et al., 2016).
Rats have continuous access to a drug during LgA and ShA training, however, the temporal pattern of drug delivery has been argued to also play a critical role in the transition to addiction. Human addicts have been reported to take cocaine intermittently whereby a large quantity of cocaine is consumed within a short time span, followed by a period of no drug use before consuming cocaine again (Allain et al., 2015). This intermittent pattern of drug-taking is believed to cause a constant spiking of brain-cocaine concentration levels and contribute to addiction-related behaviors (Zimmer et al., 2011). The intermittent access (IntA) training procedure emulates this behavior in a rodent model. During this task, rats are allowed to self-administer during 5 min bins that are separated by 25 min periods where the drug is not available (Zimmer et al., 2011). This procedure results in a high level of consumption during drug-available periods (Allain et al., 2018) and fluctuations in brain-cocaine concentration levels (Zimmer et al., 2012). While rats trained to self-administer cocaine using LgA procedures consume more drug, rats trained using IntA show greater motivation to work for cocaine compared to rats in LgA or ShA training (Zimmer et al., 2012). Rats also show greater cue-induced drug-seeking behavior following IntA training compared to LgA or ShA (Kawa et al., 2016, 2019). IntA results in greater dopamine concentrations within the NAc core following a single infusion of cocaine compared to LgA training, and dopamine levels correlated with several measures of motivation for cocaine (Kawa et al., 2019). IntA training, but not LgA or ShA, using psychostimulants also results in sensitization of NAc dopamine transports (Calipari et al., 2013, 2014). Taken together, it is apparent that while ShA, LgA and IntA all produce drug-taking and drug-seeking behavior, the behavioral paradigms differ in several other measures of motivation for a drug and resultant neurobiological effects, both of which are factors that should be taken into consideration during experimental design.
Motivation for Drug-Taking Behavior
Motivation for a drug can be measured independent of the quantity of drug consumed or pattern of intake. Progressive ratio tests and behavioral economics can be used to assess the reinforcing properties of a reward as the price and/or demand for the drug is manipulated. Adapting DSM criteria on substance use disorders to behavioral tests are also used to more directly translate data from rodent models to human addiction.
Progressive Ratio
Progressive ratio schedules are within-session procedures where the cost of a reward exponentially increases with each subsequent trial (Hodos, 1961; Roberts and Richardson, 1992; Richardson and Roberts, 1996). Using this paradigm, the motivation of the animal to work for a reward can be measured, with the maximum number of responses an animal makes in order to receive the reward referred to as the “break-point” (BP). BPs can be taken at several time points in an experiment, yielding insight into how the reinforcing properties of a drug change over the course of drug SA training. Behavior during this test is particularly sensitive to drug dose (Roberts et al., 1989), injection speed (Woolverton and Wang, 2004; Liu et al., 2005), and availability of drug during SA as well as length of forced abstinence (Morgan et al., 2002, 2005). Due to these factors, comparing BPs for the same reward between studies is often difficult. Furthermore, BPs are not comparable between different reinforcers, as they are not standardized to a baseline threshold, as is common using demand curves (discussed below). Nevertheless, a progressive ratio test is a useful tool to assess the motivation of an animal to work for a drug, and thus track the transition to addiction in animal models (for review see Roberts et al., 2007).
Behavioral Economics: Demand Curve Analysis
Behavioral economics approaches, specifically demand curve analyses, have become more widely used due to their unique ability to measure several parameters of motivation for a drug during SA (Bickel et al., 1993, 2011; Hursh and Winger, 1995). A demand curve is the effort an individual is willing to expend for a reward at various prices (Hursh, 1980), and so the cost or price of a reward is a function of that effort (Hursh et al., 1988). Demand curves can be generated within a single SA session using a threshold procedure (Oleson and Roberts, 2009; Oleson et al., 2011; Bentzley et al., 2013). These sessions typically last 110 min, and every 10 min the dose of drug available decreases according to a quarter logarithmic scale. At the conclusion of the session, a demand curve is fit to the data, and several variables are generated that yield insight into the reinforcing properties of the reward (Hursh and Silberberg, 2008). Recent models of demand curve analysis have used a focused fitting approach, whereby data points that are generated when brain-cocaine concentrations greatly fluctuate, generally at the start of the session when the animal is “loading” on the drug or toward the end of the session when the price is beyond what the animal is willing to work for the drug, are removed from analysis (Bentzley et al., 2013). This has been shown to result in a demand curve that more accurately represents the behavior of the subject (Bentzley et al., 2013).
The following variables are calculated from the demand curve: Qo, Pmax, Omax and α. Q0 is a measure of the “hedonic set point” (Ahmed and Koob, 1998, 1999), or the drug intake when the effort to acquire the drug is low. It thus acts as a general measure of consummatory behavior (Oleson et al., 2011). Because the price of the reward is low, Qo is a function of demand only. In contrast, Pmax is a function of elasticity. Elasticity refers to the rate at which the slope of the demand curve changes as the price for the reward increases (Hursh, 1980). A demand curve showing more elasticity is indicative of an individual showing less effort to consume the reward as the price increases. Pmax is the maximum price (responses/mg reward) an individual will pay to consume the reward, or rather the maximum effort the animal will expel to maintain its hedonic set point (i.e., Qo; Hursh, 1991). It is not too surprising then that Pmax values have been shown to correlate with the BP in a progressive ratio test (Rodefer and Carroll, 1997; Bickel and Madden, 1999; Lenoir and Ahmed, 2008; Oleson and Roberts, 2009). The Omax value, or the maximum number of responses made at Pmax, is a function of both demand and elasticity (Hursh and Winger, 1995). This value is unique in that it is the only variable generated from a demand curve that reliably predicts the success of drug addiction treatment (MacKillop and Murphy, 2007). The last variable, α, is known as the “essential value” of a reward and is the slope of the demand curve (Hursh and Silberberg, 2008). The motivation to continue to work for the drug as price increases is inversely related to α, such that rewards with a higher essential value have smaller α values, and show less elasticity (Bentzley et al., 2013). Alpha can also be tracked across several time periods throughout SA training to see how the essential value of the drug changes with increased drug experience (Christensen et al., 2008b). An advantage of α in comparison to the other variables is that it is inherently normalized to Qo, allowing for α values to be directly compared between reinforcers. In fact, food as a reward has a greater essential value compared to both cocaine (Christensen et al., 2008a) and methamphetamine (Galuska et al., 2011). Though Pmax and Omax are not normalized to Qo, they can manually be such that these values are also able to be compared across different rewards (Ko et al., 2002; Winger et al., 2006; Wade-Galuska et al., 2007). Overall, though data analysis is complex, demand curve analysis affords researchers the ability to parse the multiple components of motivation for drug-taking behavior within a single session and allows for the direct comparison of these components between different rewards.
Modeling DSM-Related Drug Addiction Behaviors
Another consideration when modeling addiction-related behaviors in animal models is incorporating the diagnostic criteria within the DSM for substance use disorders in humans. To that end, efforts have been put forth to model some of the criteria in animal models in order to create better preclinical models of drug addiction (Deroche-Gamonet et al., 2004; for review see Belin-Rauscent et al., 2016). Criterion often modeled includes: compulsive drug-seeking behavior when the drug is not available, high levels of motivation for the drug, and continuing to take drug despite the co-occurrence of adverse consequences (Deroche-Gamonet et al., 2004). In a rodent model, these criteria are applied by measuring drug-seeking behavior during periods of signaled no drug availability, using progressive ratio tests, and pairing a foot shock with reward consumption (for review see Belin-Rauscent et al., 2016). By using this multi-symptomatic model, rats can be separated based on the number of criteria met for a substance use disorder diagnosis, and interestingly the percent of rats that meet all criteria is very similar to the percent of human drug addicts that meet DSM criteria, further strengthening the validity of using this model (Deroche-Gamonet et al., 2004). Another advantage of this model is the ability to assess individual differences in behavioral traits and neurobiological factors that may contribute to an addicted phenotype (Belin et al., 2008, 2009, 2011; Kasanetz et al., 2010; Kawa et al., 2016).
Models of Reinstatement of Drug-Seeking Behavior
The biggest obstacle in the treatment of drug addiction is the high rates of relapse following exposure to environmental stimuli (e.g., cues, contexts, stressors) associated with prior drug-taking behavior (Shaham et al., 2003; Bossert et al., 2013). The most common methods for examining relapse behavior in animal models is via tests for cue-induced [both discrete (Meil and See, 1996) and discriminative (Weiss et al., 2000)], context-induced (Crombag and Shaham, 2002), drug-primed (de Wit and Stewart, 1981) and stress-induced (Shaham and Stewart, 1995) reinstatement. Tests for reinstatement generally occur following a period of abstinence, such as forced abstinence, voluntary abstinence, extinction training, or a combination of these procedures. While each reinstatement test isolates a specific factor that contributes to drug-seeking behavior, in humans, several of these factors likely co-occur and result in relapse. However, by studying each of these models separately, we are able to assess similarities and differences in the neurobiological mechanisms that mediate each mode of relapse (for review see Crombag et al., 2008; Bossert et al., 2013).
Cues associated with the drug-taking experience can result in craving (Childress et al., 1988, 1993) and ultimately drug relapse (see Bossert et al., 2013). There are two common types of cues used during SA training that differ based on their contingency of presentation: a discrete cue and a discriminative stimulus (DS). A discrete cue is one that is localizable and directly tied to operant responding for drug delivery (e.g., light above the lever), thus presentation of a discrete cue is contingent upon drug-taking behavior. During discrete cue-induced reinstatement, the action during SA that resulted in drug delivery and presentation of the discrete drug-associated cue now only results in the presentation of the cue. Therefore, during these tests, the conditioned reinforcing property, or the ability of the cue to invigorate ongoing behavior, of the reward-paired cue is being assessed. In humans, however, though presentation of the reward-cue invigorates drug-seeking behavior, oftentimes drug-seeking behavior precedes presentation of a reward-cue. While using a single discrete cue can evoke drug-seeking behavior, using a compound discrete stimulus, such as a cue-light and tone pairing, results in a more robust reinstatement (Kruzich et al., 2001). In contrast to a discrete cue, a DS signals when the reward is, or is not, available during SA training sessions. That is, a DS operates as an “occasion setter” (Crombag et al., 2008), signaling when operant responding for the drug will (positive DS), or will not be (negative DS), reinforced (e.g., house light turning on and off). Thus, presentation of the DS is not contingent upon drug-taking behavior. During discriminative cue-induced reinstatement tests, the positive or negative DS is noncontingently presented, and the ability of the DS to affect subsequent drug-seeking behavior is analyzed. While the use of a DS is oftentimes classified as a type of cue-induced reinstatement, some have argued that it shares more properties with context-induced reinstatement (Weiss, 2005; Trask et al., 2017).
Like cues, exposure to contexts associated with the drug-taking experience can result in relapse in humans (Wikler, 1973; O’Brien et al., 1992). A context is a compilation of several cues where no single cue predicts drug availability more than another. To test the ability of a context to invigorate drug-seeking behavior, animals are trained to self-administer drugs in one context (Context A), extinguished in a separate context (Context B), and then reintroduced to Context A for the reinstatement test (Bouton and Bolles, 1979; Crombag and Shaham, 2002; Fuchs et al., 2005). In addition to environmental cues and contexts evoking drug-seeking behavior, interoceptive cues associated with the drug experience following abstinence can also result in craving (Jaffe et al., 1989) and elevated intake (de Wit and Chutuape, 1993). Tests for drug-primed reinstatement involve the non-contingent delivery of the drug prior to being tested in extinction conditions, thus the reinstating properties of a drug are being tested while the drug is on board. In contrast to intravenous delivery as during SA, the drug prime is usually administered subcutaneously or intraperitoneally, leading to possible pharmacokinetic differences in the drug’s effect. Regardless, prime reinstatement remains the only test that isolates the ability of the drug itself to invigorate drug-seeking behavior.
The ability of different environmental and interoceptive stressors to invigorate drug-seeking behavior has also been explored. During these tests, a stressor is generally administered to the animal prior to the session starting. The main stressors used are food deprivation (Shalev et al., 2000), intermittent foot-shock (Shaham and Stewart, 1995), and administration of yohimbine (Shepard et al., 2004), a drug that causes anxiety- and stress-like effects. While it is difficult to model the complex psychological and physical stressors relevant to the human condition using animal models, the aforementioned stressors have been shown to potentiate drug-seeking behavior across several classes of drugs (for review see Mantsch et al., 2016).
There are several similarities and differences between the neurobiological mechanisms that mediate drug-seeking behavior using the different models of reinstatement. However, such detail is not within the scope of the current review, but several reviews exist that proficiently address the neurobiology associated with different animal relapse models (see Crombag et al., 2008; Bossert et al., 2013; Mantsch et al., 2016).
Alternative Rewards
Most operant models currently used to dissect the neurobiology underlying abuse disorders present very restricted options to animals; they can choose between self-administering the reward or not. However, a growing number of studies prove that when given broader choices, the vast majority of animals recoil from drug rewards.
Early in the development of the addiction field, a few rare studies measured the desire of dogs or chimpanzees pre-treated with drugs to choose cocaine or morphine over food (Tatum and Seevers, 1929; Spragg, 1940), in an attempt to model addiction-like phenotypes in animals. More recently, Lenoir et al. (2007) published an elegant study that surprised many: when given the mutually exclusive option between saccharin-sweetened water or cocaine, 94% of the animals preferred the sweet water over intravenous cocaine. Rats established this preference after multiple cocaines and sweet water samplings, consistently over 15 days of training. Importantly, when cocaine was present, maximal lever sampling and locomotor sensitization confirmed its rewarding and locomotor effects. Based on these results and subsequent work, Ahmed et al. (2013) argued that the field might be limited by using models lacking competing choices to study addiction, a disorder altering value-based decision-making. To study craving after experiencing several rewards, one study tested reinstating animals after they underwent food and cocaine SA, followed by choice tests and extinction training (Tunstall and Kearns, 2014). While the majority of rats chose to self-administer food, cocaine-primed reinstatement induced a significant increase in lever pressing of the cocaine-associated lever. Footshock and food-primed induced reinstatements however only induced a mild, non-specific increase of responding in both levers. The authors conclude that cocaine seeking can prevail over food seeking when cocaine is on board during primed reinstatement. A follow-up study established that when choosing between cocaine or grain pellets, rats still preferred pellets. However, cue-induced reinstatement following extinction training showed cocaine craving, as measured by a significant increase in lever pressing for the previously cocaine-associated lever (Tunstall and Kearns, 2016). When choosing between grain and sucrose pellets, the majority of rats self-administered sucrose over grain pellets and also responded more to the sucrose-associated lever during cued-reinstatement. The cocaine/sucrose paradigm was not tested. These results argue for a strengthening of the cocaine-associated cue despite cocaine not being the preferred option during SA.
Recently, Venniro et al. (2018) elegantly developed an operant model of choice between drugs and social interaction and showed that operant social reward prevented methamphetamine and heroin SA, even in rats exhibiting a high addiction score (Deroche-Gamonet et al., 2004). It also prevented methamphetamine incubation of craving and relapse, through protein kinase C-δ -expressing neurons in the central amygdala and inhibition of activity in the anterior ventral insular cortex (Venniro et al., 2018). These results are consistent with what is observed in humans, where greater social support and integration predicted lower risk of relapse for alcohol, opiates and cigarette smoking (Havassy et al., 1991). Another innovative study showed how social interactions profoundly affect decision-making and firing of dopaminergic cells in the VTA by analyzing the behavior of mice living in Souris City, a large environment shared by a large community of peers (Torquet et al., 2018). Based on measurements obtained after experimenter-induced social re-organizations, the authors highlight the importance of social environments on animals’ individual profiles and goal-directed decision-making.
The effects of alternative rewards are not only observed in contingent models. Solinas et al. (2009) showed that upgrading mice home cages to an enriched environment not only reduced the reinforcing effect of psychostimulants, as it had been previously shown (Bardo et al., 2001; Bezard et al., 2003; El Rawas et al., 2009; Solinas et al., 2009), but completely eliminated cocaine-induced behavioral sensitization and CPP (Solinas et al., 2008). These results were later extended to additional drugs including methamphetamine, heroin and nicotine (Sikora et al., 2018).
Despite the fact that exposing animals to multiple rewards makes dissecting the neurobiological effects of each reward more complex, it brings preclinical models closer to the intricate human experience. Akin to Portugal, a few countries combat addiction and the social marginalization associated with it by offering treatment, support services and enforcing harm reduction policies (Cabral, 2017). These could be considered the clinical equivalent of enriched environment or social interaction, and the success of such drug policy supports the idea that, by implementing similar strategies, drug abuse can be greatly decreased.
Models of Alcohol Intake
Similar to other drugs, alcohol abuse is a complex disorder impacted by social, economic and neurobiological factors (Goltseker et al., 2019). Aside from a few examples (Augier et al., 2014; de Guglielmo et al., 2017), voluntary alcohol consumption is typically weak, and often requires water-depriving the animals to incentivize drinking or initially pairing ethanol with a more salient reward, such as sucrose (Koob and Weiss, 1990; Becker, 2013; Goltseker et al., 2019). Since this “initiation training” introduces animals to multiple rewards and this can be problematic (see “Alternative Rewards” section above), a few rodent strains showing high preference for alcohol have been selectively bred (Li et al., 1979; Stewart and Li, 1997; Bell et al., 2006). Despite low levels of behavior, many studies use two-bottle choice and drinking in the dark (DID) models, both based on voluntary consumption. In the two-bottle choice model, animals are usually first presented with two bottles of water, later replacing one water solution bottle by another containing increasing percentages of alcohol (García Pardo et al., 2017). Access to alcohol can be continuous or intermittent, presenting the alcohol bottle only every other day (Brancato et al., 2016). Two-hours alcohol exposure is sufficient to measure significant correlations between alcohol intake and blood ethanol concentrations (Griffin, 2014). Several studies show that long-term exposure to intermittent alcohol access induces binge-drinking, potentiated alcohol preference and high blood alcohol concentrations (Wise, 1973; Carnicella et al., 2014). Because of these behaviors, this model mimics closely what is observed in humans, for whom the drinking pattern is a key factor in the development of alcohol use disorder (Kranzler and Soyka, 2018).
The DID model (Rhodes et al., 2005) takes advantage of rodent’s nocturnal activity to replace the home cage water bottle by a bottle containing a high concentration (20%) ethanol solution for a short period of time (2–4 h). This ethanol exposure promotes binge drinking and pharmacologically relevant blood ethanol concentrations, high enough to cause behavioral evidence of intoxication (Thiele and Navarro, 2014). This model aims to mimic the rapid and massive consumption most often observed in adolescent alcohol drinking. It does not require any modification of the alcohol solutions with other rewards or progressive increase of alcohol percentages, and binge drinking can be observed in 4 days, making it a simple and prompt model to use. However, the model seems to be somewhat restricted by mouse strain specificity. In the original study presenting the model (Rhodes et al., 2005), the DID paradigm induced binge drinking in the high ethanol drinking strain (C57BL/6J), yet the behavior was not observed in any of the other 11 inbred mice strains tested.
Chronic intermittent ethanol (CIE) is another recent model of alcohol use disorder gaining popularity (Griffin, 2014). This paradigm combines voluntary drinking and repeated exposure to alcohol vapor. After a 4-week training period of daily 2 h voluntary alcohol drinking, mice enter a cycle of 16 h vapor exposure, followed by 8 h of control air exposure (Lopez and Becker, 2005). After repeating the cycle 4–5 times (one cycle is enough to measure significant effects, but repeated cycles potentiate the behavioral outcome), animals are then tested in limited access sessions, similar to the ones performed during the training phase. CIE-animals exhibit a significant increase in voluntary ethanol drinking compared to controls, thus modeling the increase experienced by humans developing alcohol use disorder, that have been shown to be driven by neuroadaptations in glutamatergic and CRF signaling (Griffin, 2014). Since low voluntary alcohol consumption in most rodent strains is a notable limitation to study binge drinking (see discussion above), a non-contingent version of the CIE model, the Chronic-Intermittent Ethanol Administration (CIEA) paradigm, has been developed in rats (Nogales et al., 2014; Contreras et al., 2019) and mice (Sanchez-Roige et al., 2014; Lacaille et al., 2015; Monleón et al., 2019). The protocol follows the CIE timeline or a variation of it, i.e., repeated cycles of exposure to i.p. ethanol injections (3–4 g/kg) for several consecutive days intertwined with repeated days of non-exposure. CIEA is easy and inexpensive, and in combination with simple behavioral paradigms such as locomotion or elevated plus-maze, allows studying the neurobiology of binge drinking.
Alternative models focus on ethanol seeking behavior as a way to replicate relapse. Similar to other drugs, seeking behavior can be induced by priming injections of ethanol, stress, ethanol-paired cues or a combination of these factors (Le et al., 1998; Liu and Weiss, 2002; LeCocq et al., 2018). Using an alcohol-preferring rat strain, Giuliano et al. (2015) developed a different model of cue-induced alcohol-seeking. The procedure begins with a long exposure (18 sessions) to a 2-bottle choice procedure, followed by training to instrumental response to access alcohol paired with an alcohol-associated conditioned stimuli (CS). Alcohol seeking is measured during 20 min cycles where the drug is no longer present and contingent presentations of the CS act as a reinforcer. At the end of the seeking period, ethanol is re-introduced to avoid the CS losing its reinforcing properties. This experimental design aims to model alcohol craving by creating unusually high levels of alcohol-seeking behavior induced by the CS, followed by high alcohol consumption following the craving, thus closely mimicking craving leading to ethanol consumption in humans. Other models focus on compulsive-like alcohol intake, by incorporating aversive consequences to consumption, such as pairing alcohol intake with bitter quinine or footshocks (Hopf and Lesscher, 2014). These paradigms aim to model drug use despite negative consequences, one of the key symptoms listed in the DSM-5 to characterize substance abuse (APA, 2013). Quinine-resistant alcohol consumption seems to require long periods of ethanol exposure to develop, since it is measured after long cycles (8 months) of free access to alcohol (Spanagel et al., 1996; Fachin-Scheit et al., 2006; for review see Hopf and Lesscher, 2014) or after at least 3 months of intermittent access (Hopf et al., 2010). The models pairing footshocks to ethanol intake in alcohol-preferring rats show that the punishment context-dependently decreases subsequent alcohol SA (Marchant et al., 2013), however, some rats show footshock-resistant alcohol intake (Seif et al., 2013).
Individual Variation in Addiction-Related Behaviors
It is widely acknowledged that regardless of the class of addictive drug, a minority of people who use the drug develop compulsive drug-seeking behaviors indicative of substance use disorder. Appropriately modeling this variation in animals can yield powerful insight into the neurobiological mechanisms that mediate addiction propensity. Fortunately, animal models exist that capture the individual variation inherently present in the human population. Impulsivity, a trait associated with addiction liability, can be assessed using an array of behavioral paradigms. Individual variation in the acquisition of drug-taking behavior can be captured using the high-responder (HR)/low-responder (LR) model, while individual variation in relapse propensity can be assessed using the sign-tracker (ST)/goal-tracker (GT) model. The HR/LR and ST/GT models are particularly useful as they capture individual variation in two distinct phases of drug addiction: acquisition and relapse, respectively.
Models of Impulsivity
Although not specific to addiction, impulsivity, or the tendency to act prematurely without foresight, is often impaired in individuals with substance abuse disorders (Dalley et al., 2011; Ersche et al., 2012; Kaiser et al., 2016) and constitutes one of the risk factors for addictive behaviors (Dalley et al., 2007; Voon and Dalley, 2016; Kozak et al., 2019). This is particularly the case in adolescence and young adulthood, a critical period of substance experimentation, brain development and elevated impulsive behavior (Rømer Thomsen et al., 2018). Similarly, in preclinical models, adolescent animals (largely defined as postnatal day 21–60), tend to display more impulsive patterns of responding compared to adults (Burton and Fletcher, 2012; Hunt et al., 2016).
Adapted from a human task, the 5-choice serial reaction time task (5-CSRTT) rodent model was originally presented by Carli et al. (1983) in rats and has since become one of the most commonly used models to study attentional performance and motor impulsivity in rodents (Higgins and Silenieks, 2017). Rodents are first trained (20–30 sessions, around 100 trials/session) to respond to a visual stimulus on top of one of the five nose-pokes arranged on one wall of the testing chamber. Responding to the stimulus by poking the associated nose-poke is rewarded by food or liquid reward. This task requires the animal to maintain attention to the five nose-pokes and their corresponding visual cues. Poking during inter-trial intervals, i.e., between the last nose-poke and the presentation of a new stimulus, is recorded as a premature and impulsive response that is not rewarded and followed by a time-out. One of the advantages of this test resides in the possibility to control nearly every parameter, from the randomization of the visual stimuli to the length of the limited hold of the nose-poke and time out periods (Higgins and Silenieks, 2017). However, interpretation of the data requires researchers to take into account the extensive influence of attention when drawing conclusions, as any disorder disrupting attention might heavily alter the results. In this regard, using mice in this task could appear more delicate than using rats, since mice have shorter attention spans (Kentros et al., 2004; Hok et al., 2016). However, mice performances in this task are equivalent to rats, a few studies showing indeed superior motor control of task performance (Humby et al., 1999; Sanchez-Roige et al., 2012; Cope et al., 2016; Higgins and Silenieks, 2017). Exposure to drugs of abuse is typically correlated to robust increases in impulsive behavior, often specifically in adolescent rodents but not in adults, measured with the 5-CSRTT or the two-choice reaction time task, a simplified version of the former (Burton and Fletcher, 2012; Siemian et al., 2017; Moazen et al., 2018; Xue et al., 2018). Interestingly, a study compared cocaine and a cocaine-associated cue to compete for attention, and concluded that while cocaine severely disrupted the well-learned sustained attention task in rats, the cocaine-associated cue induced cocaine seeking but failed to impair the task (Pitchers et al., 2017c).
Another model focuses on response inhibition, i.e., the ability to inhibit a pre-potent (planned or already initiated) action by measuring action restraint and/or cancellation (Bari and Robbins, 2013). The animal is required to withhold responding for a set duration in order to receive a reward. Any premature response during the waiting interval resets the waiting time and increases the delay to reward.
Using these models, the field has established that impairments of the PFC, that undergoes profound pruning during adolescence (Drzewiecki et al., 2016), are both risk factors and consequences of impulse-control disorders, akin to what is observed in substance abuse (Goldstein and Volkow, 2011). Other studies show a role of VTA dopamine, locus coeruleus norepinephrine neurons and cholinergic neurotransmission on attention, impulsive and motivational control (Balachandran et al., 2018; Fitzpatrick et al., 2019; Sarter and Lustig, 2019).
High-Responder/Low-Responder Model
Rats are characterized as HRs or LRs based on their cumulative locomotor movements during a locomotor test in a novel, inescapable environment, with HRs showing greater locomotor activity compared to LRs. This separates rats based on novelty-induced “sensation-seeking” behavior, a trait associated with drug addiction (Piazza et al., 1989; Dellu et al., 1996). This model captures individual variation in the acquisition of drug-taking behavior, specifically psychostimulants. Relative to LRs, HRs acquire cocaine (Piazza et al., 2000; Mantsch et al., 2001; Ferris et al., 2013), amphetamine (Piazza et al., 1989, 1990, 1991, 1998; Klebaur et al., 2001; Cain et al., 2008) and nicotine SA (Suto et al., 2001) at a faster rate. HRs also show greater behavioral sensitization to repeated amphetamine injections compared to LRs (Hooks et al., 1992). Despite acquiring drug-taking at different rates, outbred HRs and LRs do not differ in other addiction-related behaviors following prolonged cocaine SA, including the motivation to work for the drug, or drug-seeking behavior during tests of cocaine-primed and cue-induced reinstatement (Deroche-Gamonet et al., 2004). However, work using a model of rats selectively bred based on locomotor response to a novel environment [bred high-responder (bHR)/bred low-responder (bLR) model] has challenged this view, as these two phenotypes do differ in several addiction-related traits. Compared to bLR, bHR show higher levels of impulsivity (Flagel et al., 2010), attribute incentive motivational value to food and cocaine cues (Flagel et al., 2010), and acquire cocaine SA at a faster rate (Davis et al., 2008; Flagel et al., 2016). Recent work has shown that after prolonged SA training bHR initially acquires cocaine SA at a faster rate and show greater compulsive drug-seeking behavior when drug is not available compared to bLRs (Flagel et al., 2016). bHRs also show greater drug-seeking behavior during tests of cocaine-primed and cue-induced reinstatement compared to bLRs (Flagel et al., 2016). While the data from the selectively bred rat line (Flagel et al., 2016) contrasts those of an outbred population of rats (Deroche-Gamonet et al., 2004), it appears that this model may still be relevant for assessing individual variation in addiction-related behavior beyond the acquisition of drug-taking.
Work focusing on the neurobiological mechanisms underlying phenotypic differences between HRs and LRs has focused mainly on the mesolimbic dopamine system. Following drug experience, both outbred and selectively bred HRs and LRs differ in several dopamine parameters within the NAc (Rougé-Pont et al., 1993; Chefer et al., 2003; Flagel et al., 2010; Ferris et al., 2013; Waselus et al., 2013; Mabrouk et al., 2018), as well as dopamine firing rates in the VTA (McCutcheon et al., 2009, HRs and LRs only). Differences also exist in basal levels of epigenetic modification within the NAc in bHRs and bLRs (Chaudhury et al., 2014), and these differences persist following cocaine experience (Flagel et al., 2016). In addition to the mesolimbic dopamine system, HRs and LR differentially engage the hypothalamic-pituitary-adrenal axis (Piazza et al., 1991; Kabbaj et al., 2007), which is also believed to contribute to differences in addiction-related behaviors between the two phenotypes.
Sign-Tracker/Goal-Tracker Model
The sign-tracker (ST)/goal-tracker (GT) model is used to assess individual variation in the motivational value of a reward-paired cue during a Pavlovian conditioned approach (PavCA) task (Flagel et al., 2007; Robinson and Flagel, 2009; Meyer et al., 2012). During Pavlovian learning, a once neutral stimulus that reliably precedes the delivery of a reward becomes attributed with a predictive value and is transformed into a CS (Pavlov, 1927). However, in addition to a predictive value, the CS can also be attributed with an incentive motivational value and invigorate behavior on its own (Robinson and Berridge, 1993; Berridge, 2001). During PavCA training, rats that attribute a predictive value to the CS are called GTs, whereas those that attribute both a predictive and incentive motivational value to the CS are STs. Using this model, the neurobiological mechanisms underlying the predictive vs. the incentive motivational value of a reward cue have been explored. STs engage regions within the “motive circuit” (Kalivas and Volkow, 2005) to a greater extent than GTs in response to both food (Flagel et al., 2011a; Haight et al., 2017) and drug-paired cues (Yager et al., 2015). Dopamine transmission within the NAc core (Flagel et al., 2007, 2011b; Saunders and Robinson, 2012) and PFC (Pitchers et al., 2017b) also mediates sign-tracking behavior, whereas cholinergic transmission within the PFC mediates goal-tracking behavior (Pitchers et al., 2017b). Collectively, work has shown that goal-tracking behavior is reliant on “top-down” cortical processing (for review see Kuhn et al., 2018a; Sarter and Phillips, 2018; Campus et al., 2019), whereas sign-tracking behavior engages “bottom-up” subcortical processing (for review see Flagel and Robinson, 2017; Kuhn et al., 2018a). It is proposed that the imbalance between “top-down” and “bottom-up” processing results in the behavioral differences between the phenotypes.
It has been postulated that attributing an excessive incentive motivational value to a reward-cue can lead to maladaptive behaviors such as drug addiction. In fact, STs and GTs differ in several addiction-related behaviors. For example, in addition to sign-tracking to cues associated with a food reward, STs also sign-track to cues associated with cocaine (Uslaner et al., 2006; Yager and Robinson, 2013) and opioid (Yager et al., 2015) reward delivery. Relative to GTs, STs are also more impulsive (Flagel et al., 2010; Lovic et al., 2011) and will work harder for an infusion of cocaine (Saunders and Robinson, 2011). However, the two phenotypes do not differ in the rate of cocaine SA (Saunders and Robinson, 2010; but see Beckmann et al., 2011; Saunders et al., 2013; Kawa et al., 2016; Kuhn et al., 2018b) or operant extinction (Saunders and Robinson, 2011; Kawa et al., 2016; Kuhn et al., 2018b), but do differ in reinstatement of drug-seeking behavior. STs show greater rates of both cocaine-primed (Saunders and Robinson, 2011) and cue-induced (Saunders et al., 2013; but see Kawa et al., 2016) reinstatement of drug-seeking behavior compared to GTs following ShA training. Work has shown that enhanced dopamine transmission within the NAc core contributes to higher cue-induced drug-seeking behavior observed in STs (Saunders et al., 2013). Additionally, the paraventricular nucleus of the thalamus, a region within the motive circuitry (Kelley et al., 2005) that has recently gained attention for mediating motivated behaviors including addiction-related behaviors (for review see Millan et al., 2017), is also a key node regulating this individual variation in cue-induced drug-seeking behavior (Kuhn et al., 2018b).
Though STs are more susceptible to both cocaine-primed and cue-induced reinstatement, GTs show greater drug-seeking behavior during a test for context-induced reinstatement (Saunders et al., 2014), and in response to discriminative stimuli associated with reward delivery, an effect mediated by cholinergic transmission within the PFC (Pitchers et al., 2017a). Compared to STs, GTs more readily utilize cortical processing (for review see Sarter and Phillips, 2018), and it is postulated that this cortical engagement allows them to disentangle the complex nature of contexts and discriminative stimuli better than STs, resulting in more drug-seeking behavior. This difference in relapse propensity between STs and GTs relative to the type of reinstatement implies that both phenotypes are sensitive to addiction-related behaviors; however, the environmental contingencies and neurobiological mechanisms mediating these effects differ. These findings suggest that in contrast to the HR/LR model, the main strength of the ST/GT model is elucidating the neurobiological mechanisms associated with individual variation in relapse propensity. This model also has translational validity, as work in humans has shown sign- and goal-tracking behavior (Garofalo and di Pellegrino, 2015; Joyner et al., 2018; Schad et al., 2019), though linking a specific conditioned response in humans with addiction-related behaviors has yet to be explored.
Additional Models of Behavioral Addictions
Gambling Disorder
Due to its diagnostic similarities with substance-use disorders, gambling disorder (GD; Langdon et al., 2019) was moved to a new category entitled “Substance-related and Addictive Disorders” in the most recent DSM (APA, 2013). In fact, GD is currently the only behavioral addiction that is diagnosable in the DSM, and interestingly there is a high level of comorbidity between individuals diagnosed with GD and drug addiction (Lorains et al., 2011). GD is characterized as compulsive gambling behavior that results in distress and causes disruptions to an individual’s personal and professional life (APA, 2013). The Iowa Gambling Task (IGT), a neuropsychological battery used to assess decision-making strategies, is clinically used to study GD. During this task, an individual selects a card from one of four decks, each of which has a different probability of a reward to punishment ratio assigned to it resulting in “safe” and “risky” options (Bechara et al., 1994). Individuals with GD choose cards from riskier decks more often than healthy control, resulting in fewer winnings (Cavedini et al., 2002). In fact, performance on this task has been associated with treatment success (Alvarez-Moya et al., 2011).
The rodent gambling task (rGT; Zeeb et al., 2009) is the most commonly used adaptation of the IGT. During this task, rats have the option to poke into four different ports, and each port has a specific reward (e.g., sugar pellets) and punishment (e.g., time-out period between trials) ratio associated with it. Rats are given a fixed amount of time to complete the task and choosing from the riskier ports (i.e., more sugar pellets but a longer time-out period) results in a lower net gain compared to selecting the safer ports. Studies have found that the orbitofrontal cortex (OFC) and the basolateral amygdala (BLA) mediate the acquisition of adaptive decision making during this task (Zeeb and Winstanley, 2011, 2013). However, only the BLA is needed for the continued expression of the adaptive strategy suggesting that the OFC is initially recruited to establish the behavior, but the BLA maintains it (Zeeb and Winstanley, 2011; Zeeb et al., 2015). The insular cortex has also been shown to mediate decision making during the rGT (Ishii et al., 2015; Pushparaj et al., 2015; Daniel et al., 2017). These data complement studies in humans demonstrating that various regions of the PFC are recruited during the IGT (Fellows and Farah, 2005; Tanabe et al., 2007; Lawrence et al., 2009; Power et al., 2012), and the amygdala mediates GD in humans (Bechara et al., 1999; Takeuchi et al., 2019).
While the rGT emulates aspects of GD and appears to have translational significance, it has been criticized for only modeling poor decision-making strategies, and not behaviors exclusively associated with GD (for review see Winstanley and Clark, 2016). To better address this, additional rodent models have been developed to model specific aspects of GD. For example, the rodent betting task models “escalation of commitment”, or rather the phenomenon that people become more cautious as the stakes get higher (Staw, 1981). Like humans, as stakes get higher rats will more often select a certain reward as opposed to the chance of receiving a higher reward with the risk of receiving nothing at all (Cocker et al., 2012). The BLA (Tremblay et al., 2014), regions within the PFC (Barrus et al., 2017), and dorsal striatal dopamine levels (Cocker et al., 2012) appear to mediate behavior during this task. Gambling tasks have also been created to mimic the behavior of “loss-chasing,” or continuing to gamble in an effort to earn back previous losses, which is commonly observed in individuals with GD (Toce-Gerstein et al., 2003; Strong and Kahler, 2007). During this paradigm, rats intermittently must choose between withholding responding during a time-out period, or to gamble with the chance of avoiding the time-out period with the risk of doubling it (Rogers et al., 2013). The BLA (Tremblay et al., 2014), as well as serotonin and dopamine transmission (Rogers et al., 2013), have been shown to mediate behavior during this task. Lastly, a task known as the rat slot machine task has been used to model the “near-miss effect” (Peters et al., 2010) seen in humans whereby barely losing a gamble motivates continual gambling (Kassinove and Schare, 2001; Clark et al., 2009). During this task, rats must first poke into all available ports, after which the lights within each port either turn on or stay off. If all lights come on, that is considered a “win” trial and the rat must press on a lever in order to receive the reward. Compared to other “loss” trials, rats show greater lever pressing when a “near miss” occurs (e.g., lights in all ports come on except one vs. only one light coming on; Winstanley et al., 2011). Dopamine signaling has been implicated in mediating behavior during this task (Winstanley et al., 2011; Cocker et al., 2014, 2017), particularly within the insular cortex (Cocker et al., 2016).
Other factors that affect gambling behavior in humans are also being taken into consideration when modeling GD, such as the motivational salience of audiovisual cues that are commonly present in casinos. Similar to behavior in drug addiction, cues and contexts associated with gambling have been shown to affect gambling behavior (for review see Barrus et al., 2016), and behavioral paradigms in rodents have been created to emulate these effects (Barrus and Winstanley, 2016; Adams et al., 2017; Langdon et al., 2019). Taken together, it is evident that no single behavioral paradigm can capture the complexity of GD in rodents. Thus, similar to studying drug addiction-related behaviors in animal models, a battery of assessments are used in order to better model and assess the neurobiological underpinnings of GD.
Exercise Addiction
Though not included in the DSM, exercise addiction (EA) has been reported to affect 0.3–0.5% of the general population and 1.9–3.2% of individuals who regularly exercise (Berczik et al., 2012; Griffiths et al., 2015). The main issue in diagnosing individuals with EA is that diagnostic criteria have not yet been agreed upon. Several screening tools have been developed to assess EA in humans, including the Exercise Addiction Inventory and the more commonly used Exercise Dependence Scale (for review see Hausenblas et al., 2017). Several studies have proposed that criteria for EA be adapted from those used to diagnose substance use disorders per the DSM, including measures of tolerance, withdrawal, and decreased involvement in other activities (for review see Freimuth et al., 2011). Independent of diagnostic criteria, studies have identified behavioral traits associated with EA, such as obsessive-compulsive behavior, loneliness and anxiety (Macfarlane et al., 2016; Lukács et al., 2019). Several other disorders, including substance use disorders, eating disorder, and other behavior addictions such as shopping and sex addiction have been found to co-occur with EA (for review see Freimuth et al., 2011). Despite not having a standardized diagnostic procedure, rodent models of EA exist, primarily composed of assessing wheel-running behavior. In fact, several studies using rodent wheel-running models have yielded evidence of behaviors akin to symptoms of EA aligning with those observed in substance use disorders (for review see Richter et al., 2014). Though still being fully conceptualized, it is evident that EA is garnering more attention and is being more rigorously assessed in both clinical and preclinical models.
Models Used by Pharmaceutical Companies to Assess Abuse Potential and Their Face Validity
Regardless of the scientific question, all animal models strive to represent part of the neurobiological mechanisms that guide human behavior. Models are limited in this regard, but their use is needed to assess the abuse potential of new drugs. We describe in this section a general overview of the procedures commonly used by pharmaceutical companies during a new drug safety evaluation to assess that risk. In 2017, the Food and Drug Administration agency (FDA) released an updated guide for industry gathering nonbinding recommendations on how to evaluate whether a new drug product acting on the central nervous system (CNS) has abuse potential and required to be subject to control under the Controlled Substances Act (CSA; U.S. Department of Health and Human Services, 2017). FDA does not recommend that every drug under development undergo an evaluation of abuse potential, but proposes focusing on the new CNS-active molecular entities that have not previously been assessed by FDA for abuse potential. After assessing if a new drug, or any of its major metabolites, is CNS-active through chemistry, pharmacokinetics and receptor-binding studies, the next step includes abuse-related animal behavioral studies, most commonly performed in rats. A first set of safety studies measure the effects of the drug on general behavior, such as a motor performance test. As an example, drug-induced hyperactivity is recorded as an abuse-related signal. Specific abuse-related studies evaluate: (1) the rewarding and reinforcing properties of the drug; and (2) the similarity of the effects of the new drug to established drugs of abuse, defined as drug discrimination. The rewarding and reinforcing properties are measured using SA starting on fixed ratio 1, which increases with continual training. Drug discrimination generally involves training animals to self-administer a training drug, usually a drug of abuse of similar classification than the newly tested drug, with a known mechanism of action. When the new drug induces the same SA behavior than the training drug, it is then hypothesized that drugs share a pharmacological mechanism of action (Carter and Griffiths, 2009). However, since certain classes of drugs, such as hallucinogenic 5HT2A agonists or cannabinoids, are poorly self-administered if at all (Yanagita, 1986; Fantegrossi et al., 2004; Heal et al., 2018), conditioned place-preference studies are alternatively recommended to establish potential rewarding properties of a new drug (Heal et al., 2018). These studies are usually performed at the end of phase 2 of the clinical trial, using the final therapeutic doses previously selected. When the studies establishing drug reward properties are completed, the FDA additionally recommends performing a physical dependence study, to identify potential withdrawal syndromes and behavioral disruptions upon abrupt drug discontinuation.
The decision to test the abuse potential of a new drug in humans depends on the results of the above-mentioned animal studies, a thorough comparison of the preclinical and clinical studies related to the drug and profiles of abuse- and euphoria-related adverse events established in healthy individuals and individuals with the disease of study. If all of these markers point to abuse-related signals, the drug is then tested in humans reporting numerous recent recreational experiences with drugs in the same drug class as the tested drug, through human abuse potential additional studies.
The two major models used to classify and regulate drug use, SA and CPP, present different levels of face validity. SA, including multiple-choice SA procedures (Griffiths et al., 1993), presents a high level of face validity and predictive validity (Haney and Spealman, 2008; Carter and Griffiths, 2009) and drugs self-administered in rodents correspond with those having reinforcing properties in humans (Schuster and Thompson, 1969; Griffiths and Ator, 1980; Haney and Spealman, 2008; Carter and Griffiths, 2009). In several studies, preclinical identification of drugs reducing craving and/or intake successfully translated to humans (Heilig et al., 2016). This was the case for the α2-adrenergic agonist clonidine that reduced cocaine and heroin craving in a human laboratory setting and clinical trial (Jobes et al., 2011; Kowalczyk et al., 2015). Similarly, glucocorticoid receptor antagonist mifepristone was found to decrease alcohol intake in rats, and also lessened cue-induced alcohol craving and intake in individuals with alcohol use disorder (Vendruscolo et al., 2012, 2015).
It is critical to remember that although useful, simple rodent models fail to encapsulate the complexity of human life. Factors such as drug availability, economic and socio-cultural levels influence the opportunities and experiences available to individuals and thus impact the risk of abuse. Preclinical models offering alternative rewards or including behavioral economics tackle some of these factors (see above), but these models are mostly limited to laboratory use currently and are not yet mainstreamed for pharmacological testing. Some authors additionally argue that, albeit the current models used to assess abuse potential are valid and reliable, they often lack accuracy assessing the propensity to develop addiction and the severity in which it will manifest, two parameters deeply influenced by individual vulnerability (Conway et al., 2010). They argue the need for better indices accounting for the complexity and multifactorial traits that impact the development of addiction in humans, in order to develop improved prevention approaches and treatment strategies.
One way to address these limitations is to introduce genetic variability in rodent models. While genetic factors contribute to susceptibility to drug addiction (for review see Nestler, 2000; Bevilacqua and Goldman, 2009), the heritability of drug use varies with hallucinogens showing the lowest level of heritability and cocaine showing the highest (Goldman et al., 2005). Rat lines exist that inherently capture genetic diversity, such as the heterogeneous stock rat, allowing for better representation of genetic and behavioral variation present in the human population (Solberg Woods, 2014; Woods and Mott, 2017). For a more targeted assessment of the role a gene may have in a behavioral trait, transgenic models, specifically genetic knockout and overexpressing models, can be used. In contrast to manipulating the genetic background of an animal to assess changes in behaviors, models can also be created by selectively breeding animals based on a specific behavioral trait, such as in the bHR/bLR model, and then assessing genetic differences between phenotypes. Lastly, to gain a broader understanding of the genetic differences that may contribute to certain phenotypic traits in animal models, quantitative trait loci analysis can be used. Using this technique, genetic variants can be identified in animals that differ in behaviors associated with addiction. While genetics plays a role in the predisposition for addiction-related behaviors, previous work has also focused on the epigenetic effects drugs of abuse have on the long-term transcriptional regulation of genes and how this contributes to addiction (Robison and Nestler, 2011). By focusing on the genetic basis for addiction-related behaviors, in conjunction with implementing better preclinical models of addiction, pharmaceutical companies can identify more successful therapeutics interventions in the treatment of drug addiction.
Conclusion
Despite the complexity of substance-related and addictive disorders, we have highlighted in this review currently used preclinical models aiming to mimic as closely as possible the behaviors observed in humans. As models become more complex, the tools used to study the underlying neurobiological substrates also improve, moving the field forward towards future therapeutic opportunities.
Author Contributions
All authors listed have made a substantial, direct and intellectual contribution to the work, and approved it for publication.
Funding
This research was supported by grant numbers T32DA007288-27 (BK), DA003906, DA12513, DA04530001A1, DA046373-01 and BX004727 (PK), and DA046522 (A-CB).
Abbreviations
5-CSRTT, 5-Choice Serial Reaction Time Task; AMPA, Receptor: α-amino-3-hydroxy-5-methyl-4-isoxazolepropionic acid receptor; BLA, Basolateral amygdala; bHR, Bred high-responder; bLR, Bred low-responder; BP, Break-point; CIE, Chronic intermittent ethanol; CNS, Central nervous system; CPA, Conditioned Place Aversion; CPP, Conditioned place preference; CS, Conditioned stimulus; CSA, Controlled Substances Act; DID, Drinking In the Dark; DS, Discriminative stimulus; DSM, Diagnostic and Statistical Manual; EA, Exercise addiction; GD, Gambling disorder; GT, Goal-tracker; FDA, Food and Drug Administration agency; HR, High-responder; IGT, Iowa gambling task; IntA, Intermittent access training; LgA, Long-access training; LR, Low-responder; NAc, Nucleus accumbens; OFC, Orbitofrontal cortex; PavCA, Pavlovian conditioned approach training; PFC, Prefrontal cortex; rGT, Rodent gambling task; SA, Self-administration; ShA, Short-access training; ST, Sign-tracker; THC, Δ9-tetrahydrocannabinol; VTA, Ventral tegmental area.
Conflict of Interest
The authors declare that the research was conducted in the absence of any commercial or financial relationships that could be construed as a potential conflict of interest.
References
Adams, W. K., Barkus, C., Ferland, J. N., Sharp, T., and Winstanley, C. A. (2017). Pharmacological evidence that 5-HT2C receptor blockade selectively improves decision making when rewards are paired with audiovisual cues in a rat gambling task. Psychopharmacology 234, 3091–3104. doi: 10.1007/s00213-017-4696-4
Aguilar, M. A., Rodríguez-Arias, M., and Miñarro, J. (2009). Neurobiological mechanisms of the reinstatement of drug-conditioned place preference. Brain Res. Rev. 59, 253–277. doi: 10.1016/j.brainresrev.2008.08.002
Ahmed, S. H., and Koob, G. F. (1998). Transition from moderate to excessive drug intake: change in hedonic set point. Science 282, 298–300. doi: 10.1126/science.282.5387.298
Ahmed, S. H., and Koob, G. F. (1999). Long-lasting increase in the set point for cocaine self-administration after escalation in rats. Psychopharmacology 146, 303–312. doi: 10.1007/s002130051121
Ahmed, S. H., Lenoir, M., and Guillem, K. (2013). Neurobiology of addiction versus drug use driven by lack of choice. Curr. Opin. Neurobiol. 23, 581–587. doi: 10.1016/j.conb.2013.01.028
Allain, F., Bouayad-Gervais, K., and Samaha, A. N. (2018). High and escalating levels of cocaine intake are dissociable from subsequent incentive motivation for the drug in rats. Psychopharmacology 235, 317–328. doi: 10.1007/s00213-017-4773-8
Allain, F., Minogianis, E. A., Roberts, D. C., and Samaha, A. N. (2015). How fast and how often: the pharmacokinetics of drug use are decisive in addiction. Neurosci. Biobehav. Rev. 56, 166–179. doi: 10.1016/j.neubiorev.2015.06.012
Alvarez-Moya, E. M., Ochoa, C., Jiménez-Murcia, S., Aymamí, M. N., Gómez-Peña, M., Fernández-Aranda, F., et al. (2011). Effect of executive functioning, decision-making and self-reported impulsivity on the treatment outcome of pathologic gambling. J. Psychiatry Neurosci. 36, 165–175. doi: 10.1503/jpn.090095
Antelman, S. M., Eichler, A. J., Black, C. A., and Kocan, D. (1980). Interchangeability of stress and amphetamine in sensitization. Science 207, 329–331. doi: 10.1126/science.7188649
Antoniadis, E. A., Ko, C. H., Ralph, M. R., and McDonald, R. J. (2000). Circadian rhythms, aging and memory. Behav. Brain Res. 114, 221–233. doi: 10.1016/s0166-4328(00)00290-4
APA. (2013). Diagnostic and Statistical Manual of Mental Disorders. Washington, DC: American Psychiatric Press.
Arenas, M. C., Aguilar, M. A., Montagud-Romero, S., Mateos-García, A., Navarro-Francés, C. I., Miñarro, J., et al. (2016). Influence of the novelty-seeking endophenotype on the rewarding effects of psychostimulant drugs in animal models. Curr. Neuropharmacol. 14, 87–100. doi: 10.2174/1570159x13666150921112841
Augier, E., Flanigan, M., Dulman, R. S., Pincus, A., Schank, J. R., Rice, K. C., et al. (2014). Wistar rats acquire and maintain self-administration of 20% ethanol without water deprivation, saccharin/sucrose fading, or extended access training. JAMA Cardiol. 231, 4561–4568. doi: 10.1007/s00213-014-3605-3
Babbini, M., and Davis, W. M. (1972). Time-dose relationships for locomotor activity effects of morphine after acute or repeated treatment. Br. J. Pharmacol. 46, 213–224. doi: 10.1111/j.1476-5381.1972.tb06866.x
Bahi, A., and Dreyer, J. L. (2012a). Involvement of nucleus accumbens dopamine D1 receptors in ethanol drinking, ethanol-induced conditioned place preference, and ethanol-induced psychomotor sensitization in mice. Psychopharmacology 222, 141–153. doi: 10.1007/s00213-011-2630-8
Bahi, A., and Dreyer, J. L. (2012b). Involvement of tissue plasminogen activator “tPA” in ethanol-induced locomotor sensitization and conditioned-place preference. Behav. Brain Res. 226, 250–258. doi: 10.1016/j.bbr.2011.09.024
Balachandran, R. C., Sieg, M. L., Tran, C. T. Q., Clancy, B. M., Beaudin, S. A., and Eubig, P. A. (2018). Cholinergic and dopaminergic interactions alter attention and response inhibition in Long-Evans rats performing the 5-choice serial reaction time task. Pharmacol. Biochem. Behav. 175, 160–173. doi: 10.1016/j.pbb.2018.10.006
Bardo, M. T., and Bevins, R. A. (2000). Conditioned place preference: what does it add to our preclinical understanding of drug reward? Psychopharmacology 153, 31–43. doi: 10.1007/s002130000569
Bardo, M. T., Klebaur, J. E., Valone, J. M., and Deaton, C. (2001). Environmental enrichment decreases intravenous self-administration of amphetamine in female and male rats. Psychopharmacology 155, 278–284. doi: 10.1007/s002130100720
Bari, A., and Robbins, T. W. (2013). Inhibition and impulsivity: behavioral and neural basis of response control. Prog. Neurobiol. 108, 44–79. doi: 10.1016/j.pneurobio.2013.06.005
Barrus, M. M., Cherkasova, M., and Winstanley, C. A. (2016). Skewed by cues? The motivational role of audiovisual stimuli in modelling substance use and gambling disorders. Curr. Top. Behav. Neurosci. 27, 507–529. doi: 10.1007/7854_2015_393
Barrus, M. M., Hosking, J. G., Cocker, P. J., and Winstanley, C. A. (2017). Inactivation of the orbitofrontal cortex reduces irrational choice on a rodent Betting Task. Neuroscience 345, 38–48. doi: 10.1016/j.neuroscience.2016.02.028
Barrus, M. M., and Winstanley, C. A. (2016). Dopamine D3 receptors modulate the ability of win-paired cues to increase risky choice in a rat gambling task. J. Neurosci. 36, 785–794. doi: 10.1523/JNEUROSCI.2225-15.2016
Beach, H. D. (1957). Morphine addiction in rats. Can. J. Psychol. 11, 104–112. doi: 10.1037/h0083703
Bechara, A., Damasio, A. R., Damasio, H., and Anderson, S. W. (1994). Insensitivity to future consequences following damage to human prefrontal cortex. Cognition 50, 7–15. doi: 10.1016/0010-0277(94)90018-3
Bechara, A., Damasio, H., Damasio, A. R., and Lee, G. P. (1999). Different contributions of the human amygdala and ventromedial prefrontal cortex to decision-making. J. Neurosci. 19, 5473–5481. doi: 10.1523/JNEUROSCI.19-13-05473.1999
Becker, H. C. (2013). Animal models of excessive alcohol consumption in rodents. Curr. Top. Behav. Neurosci. 13, 355–377. doi: 10.1007/7854_2012_203
Beckmann, J. S., Marusich, J. A., Gipson, C. D., and Bardo, M. T. (2011). Novelty seeking, incentive salience and acquisition of cocaine self-administration in the rat. Behav. Brain Res. 216, 159–165. doi: 10.1016/j.bbr.2010.07.022
Belin, D., Balado, E., Piazza, P. V., and Deroche-Gamonet, V. (2009). Pattern of intake and drug craving predict the development of cocaine addiction-like behavior in rats. Biol. Psychiatry 65, 863–868. doi: 10.1016/j.biopsych.2008.05.031
Belin, D., Berson, N., Balado, E., Piazza, P. V., and Deroche-Gamonet, V. (2011). High-novelty-preference rats are predisposed to compulsive cocaine self-administration. Neuropsychopharmacology 36, 569–579. doi: 10.1038/npp.2010.188
Belin, D., and Everitt, B. J. (2008). Cocaine seeking habits depend upon dopamine-dependent serial connectivity linking the ventral with the dorsal striatum. Neuron 57, 432–441. doi: 10.1016/j.neuron.2007.12.019
Belin, D., Mar, A. C., Dalley, J. W., Robbins, T. W., and Everitt, B. J. (2008). High impulsivity predicts the switch to compulsive cocaine-taking. Science 320, 1352–1355. doi: 10.1126/science.1158136
Belin-Rauscent, A., Fouyssac, M., Bonci, A., and Belin, D. (2016). How preclinical models evolved to resemble the diagnostic criteria of drug addiction. Biol. Psychiatry 79, 39–46. doi: 10.1016/j.biopsych.2015.01.004
Bell, K., and Kalivas, P. W. (1996). Context-specific cross-sensitization between systemic cocaine and intra-accumbens AMPA infusion in the rat. Psychopharmacology 127, 377–383. doi: 10.1007/bf02806018
Bell, R. L., Rodd, Z. A., Lumeng, L., Murphy, J. M., and McBride, W. J. (2006). The alcohol-preferring P rat and animal models of excessive alcohol drinking. Addict. Biol. 11, 270–288. doi: 10.1111/j.1369-1600.2005.00029.x
Bentzley, B. S., Fender, K. M., and Aston-Jones, G. (2013). The behavioral economics of drug self-administration: a review and new analytical approach for within-session procedures. Psychopharmacology 226, 113–125. doi: 10.1007/s00213-012-2899-2
Berczik, K., Szabo, A., Griffiths, M. D., Kurimay, T., Kun, B., Urban, R., et al. (2012). Exercise addiction: symptoms, diagnosis, epidemiology, and etiology. Subst. Use Misuse 47, 403–417. doi: 10.3109/10826084.2011.639120
Berridge, K. C. (2001). “Reward learning: reinforcement, incentives, and expectations,” in Psychology of Learning and Motivation (Vol. 40), ed. D. L. Medin (New York, NK: Academic Press), 223–278.
Bevilacqua, L., and Goldman, D. (2009). Genes and addictions. Clin. Pharmacol. Ther. 85, 359–361. doi: 10.1038/clpt.2009.6
Bezard, E., Dovero, S., Belin, D., Duconger, S., Jackson-Lewis, V., Przedborski, S., et al. (2003). Enriched environment confers resistance to 1-methyl-4-phenyl-1,2,3,6-tetrahydropyridine and cocaine: involvement of dopamine transporter and trophic factors. J. Neurosci. 23, 10999–11007. doi: 10.1523/JNEUROSCI.23-35-10999.2003
Bickel, W. K., Jarmolowicz, D. P., Mueller, E. T., and Gatchalian, K. M. (2011). The behavioral economics and neuroeconomics of reinforcer pathologies: implications for etiology and treatment of addiction. Curr. Psychiatry Rep. 13, 406–415. doi: 10.1007/s11920-011-0215-1
Bickel, W. K., DeGrandpre, R. J., and Higgins, S. T. (1993). Behavioral economics: a novel experimental approach to the study of drug dependence. Drug Alcohol Depend. 33, 173–192. doi: 10.1016/0376-8716(93)90059-y
Bickel, W. K., and Madden, G. J. (1999). A comparison of measures of relative reinforcing efficacy and behavioral economics: cigarettes and money in smokers. Behav. Pharmacol. 10, 627–637. doi: 10.1097/00008877-199911000-00009
Boileau, I., Dagher, A., Leyton, M., Gunn, R. N., Baker, G. B., Diksic, M., et al. (2006). Modeling sensitization to stimulants in humans: an [11C]raclopride/positron emission tomography study in healthy men. Arch. Gen. Psychiatry 63, 1386–1395. doi: 10.1001/archpsyc.63.12.1386
Bonate, P. L., Swann, A., and Silverman, P. B. (1997). Context-dependent cross-sensitization between cocaine and amphetamine. Life Sci. 60, PL1–PL7. doi: 10.1016/s0024-3205(96)00591-7
Bossert, J. M., Marchant, N. J., Calu, D. J., and Shaham, Y. (2013). The reinstatement model of drug relapse: recent neurobiological findings, emerging research topics, and translational research. Psychopharmacology 229, 453–476. doi: 10.1007/s00213-013-3120-y
Bouton, M. E., and Bolles, R. C. (1979). Contextual control of the extinction of conditioned fear. Learn. Motiv. 10, 445–466. doi: 10.1016/0023-9690(79)90057-2
Brancato, A., Plescia, F., Lavanco, G., Cavallaro, A., and Cannizzaro, C. (2016). Continuous and intermittent alcohol free-choice from pre-gestational time to lactation: focus on drinking trajectories and maternal behavior. Front. Behav. Neurosci. 10:31. doi: 10.3389/fnbeh.2016.00031
Burton, C. L., and Fletcher, P. J. (2012). Age and sex differences in impulsive action in rats: the role of dopamine and glutamate. Behav. Brain Res. 230, 21–33. doi: 10.1016/j.bbr.2012.01.046
Cabral, T. S. (2017). The 15th anniversary of the Portuguese drug policy: its history, its success and its future. Drug Sci. Policy Law 3:2050324516683640. doi: 10.1177/2050324516683640
Cadoni, C., Valentini, V., and Di Chiara, G. (2008). Behavioral sensitization to Δ 9-tetrahydrocannabinol and cross-sensitization with morphine: differential changes in accumbal shell and core dopamine transmission. J. Neurochem. 106, 1586–1593. doi: 10.1111/j.1471-4159.2008.05503.x
Cain, M. E., Denehy, E. D., and Bardo, M. T. (2008). Individual differences in amphetamine self-administration: the role of the central nucleus of the amygdala. Neuropsychopharmacology 33, 1149–1161. doi: 10.1038/sj.npp.1301478
Calipari, E. S., Ferris, M. J., Siciliano, C. A., Zimmer, B. A., and Jones, S. R. (2014). Intermittent cocaine self-administration produces sensitization of stimulant effects at the dopamine transporter. J. Pharmacol. Exp. Ther. 349, 192–198. doi: 10.1124/jpet.114.212993
Calipari, E. S., Ferris, M. J., Zimmer, B. A., Roberts, D. C., and Jones, S. R. (2013). Temporal pattern of cocaine intake determines tolerance vs sensitization of cocaine effects at the dopamine transporter. Neuropsychopharmacology 38, 2385–2392. doi: 10.1038/npp.2013.136
Campus, P., Covelo, I. R., Kim, Y., Parsegian, A., Kuhn, B. N., Lopez, S. A., et al. (2019). The paraventricular thalamus is a critical mediator of top-down control of cue-motivated behavior in rats. Elife 8:e49041. doi: 10.7554/eLife.49041
Carli, M., Robbins, T. W., Evenden, J. L., and Everitt, B. J. (1983). Effects of lesions to ascending noradrenergic neurones on performance of a 5-choice serial reaction task in rats; implications for theories of dorsal noradrenergic bundle function based on selective attention and arousal. Behav. Brain Res. 9, 361–380. doi: 10.1016/0166-4328(83)90138-9
Carnicella, S., Ron, D., and Barak, S. (2014). Intermittent ethanol access schedule in rats as a preclinical model of alcohol abuse. Alcohol 48, 243–252. doi: 10.1016/j.alcohol.2014.01.006
Carter, L. P., and Griffiths, R. R. (2009). Principles of laboratory assessment of drug abuse liability and implications for clinical development. Drug Alcohol Depend. 105, S14–S25. doi: 10.1016/j.drugalcdep.2009.04.003
Cason, A. M., Smith, R. J., Tahsili-Fahadan, P., Moorman, D. E., Sartor, G. C., and Aston-Jones, G. (2010). Role of orexin/hypocretin in reward-seeking and addiction: implications for obesity. Physiol. Behav. 100, 419–428. doi: 10.1016/j.physbeh.2010.03.009
Cavedini, P., Riboldi, G., Keller, R., D’Annucci, A., and Bellodi, L. (2002). Frontal lobe dysfunction in pathological gambling patients. Biol. Psychiatry 51, 334–341. doi: 10.1016/s0006-3223(01)01227-6
Chaudhury, S., Aurbach, E. L., Sharma, V., Blandino, P. Jr., Turner, C. A., Watson, S. J., et al. (2014). FGF2 is a target and a trigger of epigenetic mechanisms associated with differences in emotionality: partnership with H3K9me3. Proc. Natl. Acad. Sci. U S A 111, 11834–11839. doi: 10.1073/pnas.1411618111
Chefer, V. I., Zakharova, I., and Shippenberg, T. S. (2003). Enhanced responsiveness to novelty and cocaine is associated with decreased basal dopamine uptake and release in the nucleus accumbens: quantitative microdialysis in rats under transient conditions. J. Neurosci. 23, 3076–3084. doi: 10.1523/JNEUROSCI.23-07-03076.2003
Childress, A., Ehrman, R., McLellan, A. T., and O’Brien, C. P. (1988). Conditioned craving and arousal in cocaine addiction: a preliminary report. NIDA Res. Monogr. 81, 74–80.
Childress, A., Ehrman, R., McLellan, A. T., and O’Brien, C. P. (1993). “Classically conditioned factors in drug dependenc,” in Comprehensive Textbook of Substance Abuse, eds J. Lowinson and R. P. Millman (Baltimore: Williams and Wilkins), 56–69.
Christensen, C. J., Silberberg, A., Hursh, S. R., Huntsberry, M. E., and Riley, A. L. (2008a). Essential value of cocaine and food in rats: tests of the exponential model of demand. Psychopharmacology 198, 221–229. doi: 10.1007/s00213-008-1120-0
Christensen, C. J., Silberberg, A., Hursh, S. R., Roma, P. G., and Riley, A. L. (2008b). Demand for cocaine and food over time. Pharmacol. Biochem. Behav. 91, 209–216. doi: 10.1016/j.pbb.2008.07.009
Clarke, P. B., and Kumar, R. (1983). Characterization of the locomotor stimulant action of nicotine in tolerant rats. Br. J. Pharmacol. 80, 587–594. doi: 10.1111/j.1476-5381.1983.tb10733.x
Clark, L., Lawrence, A. J., Astley-Jones, F., and Gray, N. (2009). Gambling near-misses enhance motivation to gamble and recruit win-related brain circuitry. Neuron 61, 481–490. doi: 10.1016/j.neuron.2008.12.031
Cocker, P. J., Dinelle, K., Kornelson, R., Sossi, V., and Winstanley, C. A. (2012). Irrational choice under uncertainty correlates with lower striatal D(2/3) receptor binding in rats. J. Neurosci. 32, 15450–15457. doi: 10.1523/JNEUROSCI.0626-12.2012
Cocker, P. J., Le Foll, B., Rogers, R. D., and Winstanley, C. A. (2014). A selective role for dopamine D4 receptors in modulating reward expectancy in a rodent slot machine task. Biol. Psychiatry 75, 817–824. doi: 10.1016/j.biopsych.2013.08.026
Cocker, P. J., Lin, M. Y., Barrus, M. M., Le Foll, B., and Winstanley, C. A. (2016). The agranular and granular insula differentially contribute to gambling-like behavior on a rat slot machine task: effects of inactivation and local infusion of a dopamine D4 agonist on reward expectancy. Psychopharmacology 233, 3135–3147. doi: 10.1007/s00213-016-4355-1
Cocker, P. J., Rotge, J. Y., Daniel, M. L., Belin-Rauscent, A., and Belin, D. (2019). Impaired decision making following escalation of cocaine self-administration predicts vulnerability to relapse in rats. Addict. Biol. doi: 10.1111/adb.12738 [Epub ahead of print].
Cocker, P. J., Tremblay, M., Kaur, S., and Winstanley, C. A. (2017). Chronic administration of the dopamine D2/3 agonist ropinirole invigorates performance of a rodent slot machine task, potentially indicative of less distractible or compulsive-like gambling behaviour. Psychopharmacology 234, 137–153. doi: 10.1007/s00213-016-4447-y
Contreras, A., Morales, L., and Del Olmo, N. (2019). The intermittent administration of ethanol during the juvenile period produces changes in the expression of hippocampal genes and proteins and deterioration of spatial memory. Behav. Brain Res. 372:112033. doi: 10.1016/j.bbr.2019.112033
Conway, K. P., Levy, J., Vanyukov, M., Chandler, R., Rutter, J., Swan, G. E., et al. (2010). Measuring addiction propensity and severity: the need for a new instrument. Drug Alcohol Depend. 111, 4–12. doi: 10.1016/j.drugalcdep.2010.03.011
Cope, Z. A., Halberstadt, A. L., van Enkhuizen, J., Flynn, A. D., Breier, M., Swerdlow, N. R., et al. (2016). Premature responses in the five-choice serial reaction time task reflect rodents’ temporal strategies: evidence from no-light and pharmacological challenges. Psychopharmacology 233, 3513–3525. doi: 10.1007/s00213-016-4389-4
Covington, H. E. III., and Miczek, K. A. (2001). Repeated social-defeat stress, cocaine or morphine. Effects on behavioral sensitization and intravenous cocaine self-administration “binges”. Psychopharmacology 158, 388–398. doi: 10.1007/s002130100858
Crespi, L. P. (1942). Quantitative variation of incentive and performance in the white rat. Am. J. Psychol. 55, 467–517. doi: 10.2307/1417120
Crombag, H. S., Bossert, J. M., Koya, E., and Shaham, Y. (2008). Review. Context-induced relapse to drug seeking: a review. Philos. Trans. R. Soc. Lond. B Biol. Sci. 363, 3233–3243. doi: 10.1098/rstb.2008.0090
Crombag, H. S., and Shaham, Y. (2002). Renewal of drug seeking by contextual cues after prolonged extinction in rats. Behav. Neurosci. 116, 169–173. doi: 10.1037/0735-7044.116.1.169
Dalley, J. W., Everitt, B. J., and Robbins, T. W. (2011). Impulsivity, compulsivity, and top-down cognitive control. Neuron 69, 680–694. doi: 10.1016/j.neuron.2011.01.020
Dalley, J. W., Fryer, T. D., Brichard, L., Robinson, E. S., Theobald, D. E., Lääne, K., et al. (2007). Nucleus accumbens D2/3 receptors predict trait impulsivity and cocaine reinforcement. Science 315, 1267–1270. doi: 10.1126/science.1137073
Daniel, M. L., Cocker, P. J., Lacoste, J., Mar, A. C., Houeto, J. L., Belin-Rauscent, A., et al. (2017). The anterior insula bidirectionally modulates cost-benefit decision-making on a rodent gambling task. Eur. J. Neurosci. 46, 2620–2628. doi: 10.1111/ejn.13689
Davis, B. A., Clinton, S. M., Akil, H., and Becker, J. B. (2008). The effects of novelty-seeking phenotypes and sex differences on acquisition of cocaine self-administration in selectively bred High-Responder and Low-Responder rats. Pharmacol. Biochem. Behav. 90, 331–338. doi: 10.1016/j.pbb.2008.03.008
de Guglielmo, G., Kallupi, M., Cole, M. D., and George, O. (2017). Voluntary induction and maintenance of alcohol dependence in rats using alcohol vapor self-administration. Psychopharmacology 234, 2009–2018. doi: 10.1007/s00213-017-4608-7
de Wit, H., and Chutuape, M. A. (1993). Increased ethanol choice in social drinkers following ethanol preload. Behav. Pharmacol. 4, 29–36. doi: 10.1097/00008877-199302000-00003
de Wit, H., and Stewart, J. (1981). Reinstatement of cocaine-reinforced responding in the rat. Psychopharmacology 75, 134–143. doi: 10.1007/bf00432175
Dellu, F., Piazza, P. V., Mayo, W., Le Moal, M., and Simon, H. (1996). Novelty-seeking in rats–biobehavioral characteristics and possible relationship with the sensation-seeking trait in man. Neuropsychobiology 34, 136–145. doi: 10.1159/000119305
Deroche-Gamonet, V., Belin, D., and Piazza, P. V. (2004). Evidence for addiction-like behavior in the rat. Science 305, 1014–1017. doi: 10.1126/science.1099020
Didone, V., Quoilin, C., Tirelli, E., and Quertemont, E. (2008). Parametric analysis of the development and expression of ethanol-induced behavioral sensitization in female Swiss mice: effects of dose, injection schedule, and test context. Psychopharmacology 201, 249–260. doi: 10.1007/s00213-008-1266-9
Doucet, E. L., Bobadilla, A. C., Houades, V., Lanteri, C., Godeheu, G., Lanfumey, L., et al. (2013). Sustained impairment of α2A-adrenergic autoreceptor signaling mediates neurochemical and behavioral sensitization to amphetamine. Biol. Psychiatry 74, 90–98. doi: 10.1016/j.biopsych.2012.11.029
Drzewiecki, C. M., Willing, J., and Juraska, J. M. (2016). Synaptic number changes in the medial prefrontal cortex across adolescence in male and female rats: a role for pubertal onset. Synapse 70, 361–368. doi: 10.1002/syn.21909
Ducret, E., Puaud, M., Lacoste, J., Belin-Rauscent, A., Fouyssac, M., Dugast, E., et al. (2016). N-acetylcysteine facilitates self-imposed abstinence after escalation of cocaine intake. Biol. Psychiatry 80, 226–234. doi: 10.1016/j.biopsych.2015.09.019
Duvauchelle, C. L., Ikegami, A., and Castaneda, E. (2000). Conditioned increases in behavioral activity and accumbens dopamine levels produced by intravenous cocaine. Behav. Neurosci. 114, 1156–1166. doi: 10.1037/0735-7044.114.6.1156
El Rawas, R., Thiriet, N., Lardeux, V., Jaber, M., and Solinas, M. (2009). Environmental enrichment decreases the rewarding but not the activating effects of heroin. Psychopharmacology 203, 561–570. doi: 10.1007/s00213-008-1402-6
Epstein, D. H., Preston, K. L., Stewart, J., and Shaham, Y. (2006). Toward a model of drug relapse: an assessment of the validity of the reinstatement procedure. Psychopharmacology 189, 1–16. doi: 10.1007/s00213-006-0529-6
Ersche, K. D., Jones, P. S., Williams, G. B., Turton, A. J., Robbins, T. W., and Bullmore, E. T. (2012). Abnormal brain structure implicated in stimulant drug addiction. Science 335, 601–604. doi: 10.1126/science.1214463
Ettenberg, A. (2004). Opponent process properties of self-administered cocaine. Neurosci. Biobehav. Rev. 27, 721–728. doi: 10.1016/j.neubiorev.2003.11.009
Ettenberg, A. (2009). The runway model of drug self-administration. Pharmacol. Biochem. Behav. 91, 271–277. doi: 10.1016/j.pbb.2008.11.003
Everitt, B. J., and Robbins, T. W. (2016). Drug addiction: updating actions to habits to compulsions ten years on. Annu. Rev. Psychol. 67, 23–50. doi: 10.1146/annurev-psych-122414-033457
Fachin-Scheit, D. J., Ribeiro Frozino, A., Pigatto, G., Oliveira Goeldner, F., and Boerngen de Lacerda, R. (2006). Development of a mouse model of ethanol addiction: naltrexone efficacy in reducing consumption but not craving. J. Neural Transm. 113, 1305–1321. doi: 10.1007/s00702-005-0416-z
Famous, K. R., Kumaresan, V., Sadri-Vakili, G., Schmidt, H. D., Mierke, D. F., Cha, J. H., et al. (2008). Phosphorylation-dependent trafficking of GluR2-containing AMPA receptors in the nucleus accumbens plays a critical role in the reinstatement of cocaine seeking. J. Neurosci. 28, 11061–11070. doi: 10.1523/JNEUROSCI.1221-08.2008
Fantegrossi, W. E., Woods, J. H., and Winger, G. (2004). Transient reinforcing effects of phenylisopropylamine and indolealkylamine hallucinogens in rhesus monkeys. Behav. Pharmacol. 15, 149–157. doi: 10.1097/00008877-200403000-00007
Fellows, L. K., and Farah, M. J. (2005). Different underlying impairments in decision-making following ventromedial and dorsolateral frontal lobe damage in humans. Cereb. Cortex 15, 58–63. doi: 10.1093/cercor/bhh108
Ferris, M. J., Calipari, E. S., Melchior, J. R., Roberts, D. C., España, R. A., and Jones, S. R. (2013). Paradoxical tolerance to cocaine after initial supersensitivity in drug-use-prone animals. Eur. J. Neurosci. 38, 2628–2636. doi: 10.1111/ejn.12266
Fiorino, D. F., and Phillips, A. G. (1999a). Facilitation of sexual behavior and enhanced dopamine efflux in the nucleus accumbens of male rats after D-amphetamine-induced behavioral sensitization. J. Neurosci. 19, 456–463. doi: 10.1523/JNEUROSCI.19-01-00456.1999
Fiorino, D. F., and Phillips, A. G. (1999b). Facilitation of sexual behavior in male rats following d-amphetamine-induced behavioral sensitization. Psychopharmacology 142, 200–208. doi: 10.1007/s002130050880
Fitzpatrick, C. M., Runegaard, A. H., Christiansen, S. H., Hansen, N. W., Jørgensen, S. H., McGirr, J. C., et al. (2019). Differential effects of chemogenetic inhibition of dopamine and norepinephrine neurons in the mouse 5-choice serial reaction time task. Prog. Neuropsychopharmacol. Biol. Psychiatry 90, 264–276. doi: 10.1016/j.pnpbp.2018.12.004
Flagel, S. B., Cameron, C. M., Pickup, K. N., Watson, S. J., Akil, H., and Robinson, T. E. (2011a). A food predictive cue must be attributed with incentive salience for it to induce c-fos mRNA expression in cortico-striatal-thalamic brain regions. Neuroscience 196, 80–96. doi: 10.1016/j.neuroscience.2011.09.004
Flagel, S. B., Clark, J. J., Robinson, T. E., Mayo, L., Czuj, A., Willuhn, I., et al. (2011b). A selective role for dopamine in stimulus-reward learning. Nature 469, 53–57. doi: 10.1038/nature09588
Flagel, S. B., Chaudhury, S., Waselus, M., Kelly, R., Sewani, S., Clinton, S. M., et al. (2016). Genetic background and epigenetic modifications in the core of the nucleus accumbens predict addiction-like behavior in a rat model. Proc. Natl. Acad. Sci. U S A 113, E2861–E2870. doi: 10.1073/pnas.1520491113
Flagel, S. B., and Robinson, T. E. (2017). Neurobiological basis of individual variation in stimulus-reward learning. Curr. Opin. Behav. Sci. 13, 178–185. doi: 10.1016/j.cobeha.2016.12.004
Flagel, S. B., Robinson, T. E., Clark, J. J., Clinton, S. M., Watson, S. J., Seeman, P., et al. (2010). An animal model of genetic vulnerability to behavioral disinhibition and responsiveness to reward-related cues: implications for addiction. Neuropsychopharmacology 35, 388–400. doi: 10.1038/npp.2009.142
Flagel, S. B., Watson, S. J., Robinson, T. E., and Akil, H. (2007). Individual differences in the propensity to approach signals vs goals promote different adaptations in the dopamine system of rats. Psychopharmacology 191, 599–607. doi: 10.1007/s00213-006-0535-8
Freimuth, M., Moniz, S., and Kim, S. R. (2011). Clarifying exercise addiction: differential diagnosis, co-occurring disorders, and phases of addiction. Int. J. Environ. Res. Public Health 8, 4069–4081. doi: 10.3390/ijerph8104069
Fuchs, R. A., Evans, K. A., Ledford, C. C., Parker, M. P., Case, J. M., Mehta, R. H., et al. (2005). The role of the dorsomedial prefrontal cortex, basolateral amygdala, and dorsal hippocampus in contextual reinstatement of cocaine seeking in rats. Neuropsychopharmacology 30, 296–309. doi: 10.1038/sj.npp.1300579
Gaiardi, M., Bartoletti, M., Bacchi, A., Gubellini, C., Costa, M., and Babbini, M. (1991). Role of repeated exposure to morphine in determining its affective properties: place and taste conditioning studies in rats. Psychopharmacology 103, 183–186. doi: 10.1007/bf02244201
Galuska, C. M., Banna, K. M., Willse, L. V., Yahyavi-Firouz-Abadi, N., and See, R. E. (2011). A comparison of economic demand conditioned-cued reinstatement of methamphetamine-seeking or food-seeking in rats. Physiol. Behav. 22, 312–323. doi: 10.1097/fbp.0b013e3283473be4
García Pardo, M. P., Roger Sánchez, C., De la Rubia Ortí, J. E., and Aguilar Calpe, M. A. (2017). Animal models of drug addiction. Adicciones 29, 278–292. doi: 10.20882/adicciones.862
Garofalo, S., and di Pellegrino, G. (2015). Individual differences in the influence of task-irrelevant Pavlovian cues on human behavior. Front. Behav. Neurosci. 9:163. doi: 10.3389/fnbeh.2015.00163
Geist, T. D., and Ettenberg, A. (1990). A simple method for studying intravenous drug reinforcement in a runaway. Pharmacol. Biochem. Behav. 36, 703–706. doi: 10.1016/0091-3057(90)90278-p
Giuliano, C., Goodlett, C. R., Economidou, D., García-Pardo, M. P., Belin, D., Robbins, T. W., et al. (2015). The novel mu-opioid receptor antagonist GSK1521498 decreases both alcohol seeking and drinking: evidence from a new preclinical model of alcohol seeking. Neuropsychopharmacology 40, 2981–2992. doi: 10.1038/npp.2015.152
Goldman, D., Oroszi, G., and Ducci, F. (2005). The genetics of addictions: uncovering the genes. Nat. Rev. Genet. 6, 521–532. doi: 10.1038/nrg1635
Goldstein, R. Z., and Volkow, N. D. (2011). Dysfunction of the prefrontal cortex in addiction: neuroimaging findings and clinical implications. Nat. Rev. Neurosci. 12, 652–669. doi: 10.1038/nrn3119
Goltseker, K., Hopf, F. W., and Barak, S. (2019). Advances in behavioral animal models of alcohol use disorder. Alcohol 74, 73–82. doi: 10.1016/j.alcohol.2018.05.014
Griffin, W. C. III. (2014). Alcohol dependence and free-choice drinking in mice. Alcohol 48, 287–293. doi: 10.1016/j.alcohol.2013.11.006
Griffiths, R. R., and Ator, N. A. (1980). Benzodiazepine self-administration in animals and humans: a comprehensive literature review. NIDA Res. Monogr. 33, 22–36.
Griffiths, R. R., Troisi, J. R., Silverman, K., and Mumford, G. K. (1993). Multiple-choice procedure: an efficient approach for investigating drug reinforcement in humans. Behav. Pharmacol. 4, 3–13. doi: 10.1097/00008877-199302000-00001
Griffiths, M. D., Urban, R., Demetrovics, Z., Lichtenstein, M. B., de la Vega, R., Kun, B., et al. (2015). A cross-cultural re-evaluation of the Exercise Addiction Inventory (EAI) in five countries. Sports Med. Open 1:5. doi: 10.1186/s40798-014-0005-5
Hagues, G., Costentin, J., and Duterte-Boucher, D. (2008). Locomotor effects of morphine or alcohol in mice after a repeated treatment with the cannabinoid agonist HU 210. Eur. J. Pharmacol. 586, 197–204. doi: 10.1016/j.ejphar.2008.02.062
Haight, J. L., Fuller, Z. L., Fraser, K. M., and Flagel, S. B. (2017). A food-predictive cue attributed with incentive salience engages subcortical afferents and efferents of the paraventricular nucleus of the thalamus. Neuroscience 340, 135–152. doi: 10.1016/j.neuroscience.2016.10.043
Haney, M., and Spealman, R. (2008). Controversies in translational research: drug self-administration. Psychopharmacology 199, 403–419. doi: 10.1007/s00213-008-1079-x
Hao, Y., Martin-Fardon, R., and Weiss, F. (2010). Behavioral and functional evidence of metabotropic glutamate receptor 2/3 and metabotropic glutamate receptor 5 dysregulation in cocaine-escalated rats: factor in the transition to dependence. Biol. Psychiatry 68, 240–248. doi: 10.1016/j.biopsych.2010.02.011
Harris, G. C., and Aston-Jones, G. (2003a). Altered motivation and learning following opiate withdrawal: evidence for prolonged dysregulation of reward processing. Neuropsychopharmacology 28, 865–871. doi: 10.1038/sj.npp.1300122
Harris, G. C., and Aston-Jones, G. (2003b). Critical role for ventral tegmental glutamate in preference for a cocaine-conditioned environment. Neuropsychopharmacology 28, 73–76. doi: 10.1038/sj.npp.1300011
Hausenblas, H. A., Schreiber, K., and Smoliga, J. M. (2017). Addiction to exercise. BMJ 357:j1745. doi: 10.1136/bmj.j1745
Havassy, B. E., Hall, S. M., and Wasserman, D. A. (1991). Social support and relapse: commonalities among alcoholics, opiate users, and cigarette smokers. Addict. Behav. 16, 235–246. doi: 10.1016/0306-4603(91)90016-b
Heal, D. J., Gosden, J., and Smith, S. L. (2018). Evaluating the abuse potential of psychedelic drugs as part of the safety pharmacology assessment for medical use in humans. Neuropharmacology 142, 89–115. doi: 10.1016/j.neuropharm.2018.01.049
Heilig, M., Epstein, D. H., Nader, M. A., and Shaham, Y. (2016). Time to connect: bringing social context into addiction neuroscience. Nat. Rev. Neurosci. 17, 592–599. doi: 10.1038/nrn.2016.67
Herman, J. P., Stinus, L., and Le Moal, M. (1984). Repeated stress increases locomotor response to amphetamine. Psychopharmacology 84, 431–435. doi: 10.1007/bf00555227
Higgins, G. A., and Silenieks, L. B. (2017). Rodent test of attention and impulsivity: the 5-choice serial reaction time task. Curr. Protoc. Pharmacol. 78, 5.49.1–5.49.34. doi: 10.1002/cpph.27
Hodos, W. (1961). Progressive ratio as a measure of reward strength. Science 134, 943–944. doi: 10.1126/science.134.3483.943
Hok, V., Poucet, B., Duvelle, É., Save, É., and Sargolini, F. (2016). Spatial cognition in mice and rats: similarities and differences in brain and behavior. Wiley Interdiscip. Rev. Cogn. Sci. 7, 406–421. doi: 10.1002/wcs.1411
Hooks, M. S., Jones, G. H., Liem, B. J., and Justice, J. B. Jr. (1992). Sensitization and individual differences to IP amphetamine, cocaine, or caffeine following repeated intracranial amphetamine infusions. Pharmacol. Biochem. Behav. 43, 815–823. doi: 10.1016/0091-3057(92)90413-a
Hopf, F. W., Chang, S. J., Sparta, D. R., Bowers, M. S., and Bonci, A. (2010). Motivation for alcohol becomes resistant to quinine adulteration after 3 to 4 months of intermittent alcohol self-administration. Alcohol. Clin. Exp. Res. 34, 1565–1573. doi: 10.1111/j.1530-0277.2010.01241.x
Hopf, F. W., and Lesscher, H. M. (2014). Rodent models for compulsive alcohol intake. Alcohol 48, 253–264. doi: 10.1016/j.alcohol.2014.03.001
Horger, B. A., Giles, M. K., and Schenk, S. (1992). Preexposure to amphetamine and nicotine predisposes rats to self-administer a low dose of cocaine. Psychopharmacology 107, 271–276. doi: 10.1007/bf02245147
Horger, B. A., Shelton, K., and Schenk, S. (1990). Preexposure sensitizes rats to the rewarding effects of cocaine. Pharmacol. Biochem. Behav. 37, 707–711. doi: 10.1016/0091-3057(90)90552-s
Hull, C. L. (1934). The rat’s speed-of-locomotion gradient in the approach to food. J. Comp. Psychol. 17, 393–422. doi: 10.1037/h0071299
Humby, T., Laird, F. M., Davies, W., and Wilkinson, L. S. (1999). Visuospatial attentional functioning in mice: interactions between cholinergic manipulations and genotype. Eur. J. Neurosci. 11, 2813–2823. doi: 10.1046/j.1460-9568.1999.00701.x
Hunt, P. S., Burk, J. A., and Barnet, R. C. (2016). Adolescent transitions in reflexive and non-reflexive behavior: review of fear conditioning and impulse control in rodent models. Neurosci. Biobehav. Rev. 70, 33–45. doi: 10.1016/j.neubiorev.2016.06.026
Hursh, S. R. (1980). Economic concepts for the analysis of behavior. J. Exp. Anal. Behav. 34, 219–238. doi: 10.1901/jeab.1980.34-219
Hursh, S. R. (1991). Behavioral economics of drug self-administration and drug abuse policy. J. Exp. Anal. Behav. 56, 377–393. doi: 10.1901/jeab.1991.56-377
Hursh, S. R., Raslear, T. G., Shurtleff, D., Bauman, R., and Simmons, L. (1988). A cost-benefit analysis of demand for food. J. Exp. Anal. Behav. 50, 419–440. doi: 10.1901/jeab.1988.50-419
Hursh, S. R., and Silberberg, A. (2008). Economic demand essential value. Psychol. Rev. 115, 186–198. doi: 10.1037/0033-295x.115.1.186
Hursh, S. R., and Winger, G. (1995). Normalized demand for drugs and other reinforcers. J. Exp. Anal. Behav. 64, 373–384. doi: 10.1901/jeab.1995.64-373
Ishii, H., Ohara, S., Tobler, P. N., Tsutsui, K., and Iijima, T. (2015). Dopaminergic and serotonergic modulation of anterior insular and orbitofrontal cortex function in risky decision making. Neurosci. Res. 92, 53–61. doi: 10.1016/j.neures.2014.11.009
Jaffe, J. H., Cascella, N. G., Kumor, K. M., and Sherer, M. A. (1989). Cocaine-induced cocaine craving. Psychopharmacology 97, 59–64. doi: 10.1007/bf00443414
Jhou, T. C., Good, C. H., Rowley, C. S., Xu, S. P., Wang, H., Burnham, N. W., et al. (2013). Cocaine drives aversive conditioning via delayed activation of dopamine-responsive habenular and midbrain pathways. J. Neurosci. 33, 7501–7512. doi: 10.1523/JNEUROSCI.3634-12.2013
Jobes, M. L., Ghitza, U. E., Epstein, D. H., Phillips, K. A., Heishman, S. J., and Preston, K. L. (2011). Clonidine blocks stress-induced craving in cocaine users. Psychopharmacology 218, 83–88. doi: 10.1007/s00213-011-2230-7
Joyner, M. A., Gearhardt, A. N., and Flagel, S. B. (2018). A translational model to assess sign-tracking and goal-tracking behavior in children. Neuropsychopharmacology 43, 228–229. doi: 10.1038/npp.2017.196
Kabbaj, M., Morley-Fletcher, S., Le Moal, M., and Maccari, S. (2007). Individual differences in the effects of chronic prazosin hydrochloride treatment on hippocampal mineralocorticoid and glucocorticoid receptors. Eur. J. Neurosci. 25, 3312–3318. doi: 10.1111/j.1460-9568.2007.05585.x
Kaiser, A., Bonsu, J. A., Charnigo, R. J., Milich, R., and Lynam, D. R. (2016). Impulsive personality and alcohol use: bidirectional relations over one year. J. Stud. Alcohol Drugs 77, 473–482. doi: 10.15288/jsad.2016.77.473
Kalivas, P. W., and Stewart, J. (1991). Dopamine transmission in the initiation and expression of drug- and stress-induced sensitization of motor activity. Brain Res. Rev. 16, 223–244. doi: 10.1016/0165-0173(91)90007-u
Kalivas, P. W., and Volkow, N. D. (2005). The neural basis of addiction: a pathology of motivation and choice. Am. J. Psychiatry 162, 1403–1413. doi: 10.1176/appi.ajp.162.8.1403
Kasahara, M., Groenink, L., Bijlsma, E. Y., Olivier, B., and Sarnyai, Z. (2015). Lifelong, central corticotropin-releasing factor (CRF) overexpression is associated with individual differences in cocaine-induced conditioned place preference. Eur. J. Pharmacol. 753, 151–157. doi: 10.1016/j.ejphar.2014.07.050
Kasanetz, F., Deroche-Gamonet, V., Berson, N., Balado, E., Lafourcade, M., Manzoni, O., et al. (2010). Transition to addiction is associated with a persistent impairment in synaptic plasticity. Science 328, 1709–1712. doi: 10.1126/science.1187801
Kassinove, J. I., and Schare, M. L. (2001). Effects of the “near miss” and the ”big win” on persistence at slot machine gambling. Psychol. Addict. Behav. 15, 155–158. doi: 10.1037/0893-164x.15.2.155
Kawa, A. B., Bentzley, B. S., and Robinson, T. E. (2016). Less is more: prolonged intermittent access cocaine self-administration produces incentive-sensitization and addiction-like behavior. Psychopharmacology 233, 3587–3602. doi: 10.1007/s00213-016-4393-8
Kawa, A. B., Valenta, A. C., Kennedy, R. T., and Robinson, T. E. (2019). Incentive and dopamine sensitization produced by intermittent but not long access cocaine self-administration. Eur. J. Neurosci. 50, 2663’2682. doi: 10.1111/ejn.14418
Kelley, A. E., Baldo, B. A., Pratt, W. E., and Will, M. J. (2005). Corticostriatal-hypothalamic circuitry and food motivation: integration of energy, action and reward. Physiol. Behav. 86, 773–795. doi: 10.1016/j.physbeh.2005.08.066
Kentros, C. G., Agnihotri, N. T., Streater, S., Hawkins, R. D., and Kandel, E. R. (2004). Increased attention to spatial context increases both place field stability and spatial memory. Neuron 42, 283–295. doi: 10.1016/s0896-6273(04)00192-8
Klebaur, J. E., and Bardo, M. T. (1999). The effects of anxiolytic drugs on novelty-induced place preference. Behav. Brain Res. 101, 51–57. doi: 10.1016/s0166-4328(98)00145-4
Klebaur, J. E., Bevins, R. A., Segar, T. M., and Bardo, M. T. (2001). Individual differences in behavioral responses to novelty and amphetamine self-administration in male and female rats. Behav. Pharmacol. 12, 267–275. doi: 10.1097/00008877-200107000-00005
Knackstedt, L. A., and Kalivas, P. W. (2007). Extended access to cocaine self-administration enhances drug-primed reinstatement but not behavioral sensitization. J. Pharmacol. Exp. Ther. 322, 1103–1109. doi: 10.1124/jpet.107.122861
Ko, M. C., Terner, J., Hursh, S., Woods, J. H., and Winger, G. (2002). Relative reinforcing effects of three opioids with different durations of action. J. Pharmacol. Exp. Ther. 301, 698–704. doi: 10.1124/jpet.301.2.698
Koob, G. F., and Weiss, F. (1990). Pharmacology of drug self-administration. Alcohol 7, 193–197. doi: 10.1016/0741-8329(90)90004-v
Kowalczyk, W. J., Phillips, K. A., Jobes, M. L., Kennedy, A. P., Ghitza, U. E., Agage, D. A., et al. (2015). Clonidine maintenance prolongs opioid abstinence and decouples stress from craving in daily life: a randomized controlled trial with ecological momentary assessment. Am. J. Psychiatry 172, 760–767. doi: 10.1176/appi.ajp.2014.14081014
Kozak, K., Lucatch, A. M., Lowe, D. J. E., Balodis, I. M., MacKillop, J., and George, T. P. (2019). The neurobiology of impulsivity and substance use disorders: implications for treatment. Ann. N Y Acad. Sci. 1451, 71–91. doi: 10.1111/nyas.13977
Kranzler, H. R., and Soyka, M. (2018). Diagnosis and pharmacotherapy of alcohol use disorder: a review. JAMA 320, 815–824. doi: 10.1001/jama.2018.11406
Kruzich, P. J., Congleton, K. M., and See, R. E. (2001). Conditioned reinstatement of drug-seeking behavior with a discrete compound stimulus classically conditioned with intravenous cocaine. Behav. Neurosci. 115, 1086–1092. doi: 10.1037/0735-7044.115.5.1086
Kuhn, B. N., Campus, P., and Flagel, S. B. (2018a). “Neurobiological mechanisms underlying sign-tracking behavior,” in Sign-Tracking and Drug Addiction, eds A. Tomie and J. Morrow (Ann Arbor, MI: Maize Books). doi: 10.3998/mpub.10215070
Kuhn, B. N., Klumpner, M. S., Covelo, I. R., Campus, P., and Flagel, S. B. (2018b). Transient inactivation of the paraventricular nucleus of the thalamus enhances cue-induced reinstatement in goal-trackers, but not sign-trackers. Psychopharmacology 235, 999–1014. doi: 10.1007/s00213-017-4816-1
Lacaille, H., Duterte-Boucher, D., Liot, D., Vaudry, H., Naassila, M., and Vaudry, D. (2015). Comparison of the deleterious effects of binge drinking-like alcohol exposure in adolescent and adult mice. J. Neurochem. 132, 629–641. doi: 10.1111/jnc.13020
Lamarque, S., Taghzouti, K., and Simon, H. (2001). Chronic treatment with Δ9-tetrahydrocannabinol enhances the locomotor response to amphetamine and heroin. Implications for vulnerability to drug addiction. Neuropharmacology 41, 118–129. doi: 10.1016/s0028-3908(01)00039-9
Langdon, A. J., Hathaway, B. A., Zorowitz, S., Harris, C. B. W., and Winstanley, C. A. (2019). Relative insensitivity to time-out punishments induced by win-paired cues in a rat gambling task. Psychopharmacology 236, 2543–2556. doi: 10.1007/s00213-019-05308-x
Lawrence, N. S., Jollant, F., O’Daly, O., Zelaya, F., and Phillips, M. L. (2009). Distinct roles of prefrontal cortical subregions in the Iowa Gambling Task. Cereb. Cortex 19, 1134–1143. doi: 10.1093/cercor/bhn154
Le, A. D., Quan, B., Juzytch, W., Fletcher, P. J., Joharchi, N., and Shaham, Y. (1998). Reinstatement of alcohol-seeking by priming injections of alcohol and exposure to stress in rats. Psychopharmacology 135, 169–174. doi: 10.1007/s002130050498
LeCocq, M. R., Lahlou, S., Chahine, M., Padillo, L. N., and Chaudhri, N. (2018). Modeling relapse to pavlovian alcohol-seeking in rats using reinstatement and spontaneous recovery paradigms. Alcohol. Clin. Exp. Res. 42, 1795–1806. doi: 10.1111/acer.13825
Lenoir, M., and Ahmed, S. H. (2008). Supply of a nondrug substitute reduces escalated heroin consumption. Neuropsychopharmacology 33, 2272–2282. doi: 10.1038/sj.npp.1301602
Lenoir, M., Serre, F., Cantin, L., and Ahmed, S. H. (2007). Intense sweetness surpasses cocaine reward. PLoS One 2:e698. doi: 10.1371/journal.pone.0000698
Lett, B. T. (1989). Repeated exposures intensify rather than diminish the rewarding effects of amphetamine, morphine, and cocaine. Psychopharmacology 98, 357–362. doi: 10.1007/bf00451687
Li, T. K., Lumeng, L., McBride, W. J., and Waller, M. B. (1979). Progress toward a voluntary oral consumption model of alcoholism. Drug Alcohol Depend. 4, 45–60. doi: 10.1016/0376-8716(79)90040-1
Liu, Y., Le Foll, B., Liu, Y., Wang, X., and Lu, L. (2008). Conditioned place preference induced by licit drugs: establishment, extinction, and reinstatement. ScientificWorldJournal 8, 1228–1245. doi: 10.1100/tsw.2008.154
Liu, Y., Roberts, D. C., and Morgan, D. (2005). Sensitization of the reinforcing effects of self-administered cocaine in rats: effects of dose and intravenous injection speed. Eur. J. Neurosci. 22, 195–200. doi: 10.1111/j.1460-9568.2005.04195.x
Liu, X., and Weiss, F. (2002). Additive effect of stress and drug cues on reinstatement of ethanol seeking: exacerbation by history of dependence and role of concurrent activation of corticotropin-releasing factor and opioid mechanisms. J. Neurosci. 22, 7856–7861. doi: 10.1523/JNEUROSCI.22-18-07856.2002
Lopez, M. F., and Becker, H. C. (2005). Effect of pattern and number of chronic ethanol exposures on subsequent voluntary ethanol intake in C57BL/6J mice. Psychopharmacology 181, 688–696. doi: 10.1007/s00213-005-0026-3
Lorains, F. K., Cowlishaw, S., and Thomas, S. A. (2011). Prevalence of comorbid disorders in problem and pathological gambling: systematic review and meta-analysis of population surveys. Addiction 106, 490–498. doi: 10.1111/j.1360-0443.2010.03300.x
Lovic, V., Saunders, B. T., Yager, L. M., and Robinson, T. E. (2011). Rats prone to attribute incentive salience to reward cues are also prone to impulsive action. Behav. Brain Res. 223, 255–261. doi: 10.1016/j.bbr.2011.04.006
Lucantonio, F., Kambhampati, S., Haney, R. Z., Atalayer, D., Rowland, N. E., Shaham, Y., et al. (2015). Effects of prior cocaine versus morphine or heroin self-administration on extinction learning driven by overexpectation versus omission of reward. Biol. Psychiatry 77, 912–920. doi: 10.1016/j.biopsych.2014.11.017
Lukács, A., Sasvári, P., Varga, B., and Mayer, K. (2019). Exercise addiction and its related factors in amateur runners. J. Behav. Addict. 8, 343–349. doi: 10.1556/2006.8.2019.28
Lynch, W. J. (2018). Modeling the development of drug addiction in male and female animals. Pharmacol. Biochem. Behav. 164, 50–61. doi: 10.1016/j.pbb.2017.06.006
Mabrouk, O. S., Han, J. L., Wong, J. T., Akil, H., Kennedy, R. T., and Flagel, S. B. (2018). The in vivo neurochemical profile of selectively bred high-responder and low-responder rats reveals baseline, cocaine-evoked and novelty-evoked differences in monoaminergic systems. ACS Chem. Neurosci. 9, 715–724. doi: 10.1021/acschemneuro.7b00294
Macfarlane, L., Owens, G., and Cruz Bdel, P. (2016). Identifying the features of an exercise addiction: a delphi study. J. Behav. Addict. 5, 474–484. doi: 10.1556/2006.5.2016.060
MacKillop, J., and Murphy, J. G. (2007). A behavioral economic measure of demand for alcohol predicts brief intervention outcomes. Drug Alcohol Depend. 89, 227–233. doi: 10.1016/j.drugalcdep.2007.01.002
Magos, L. (1969). Persistence of the effect of amphetamine on stereotyped activity in rats. Eur. J. Pharmacol. 6, 200–201. doi: 10.1016/0014-2999(69)90220-9
Mandt, B. H., Copenhagen, L. I., Zahniser, N. R., and Allen, R. M. (2015). Escalation of cocaine consumption in short and long access self-administration procedures. Drug Alcohol Depend. 149, 166–172. doi: 10.1016/j.drugalcdep.2015.01.039
Mantsch, J. R., Baker, D. A., Funk, D., Le, A. D., and Shaham, Y. (2016). Stress-induced reinstatement of drug seeking: 20 years of progress. Neuropsychopharmacology 41, 335–356. doi: 10.1038/npp.2015.142
Mantsch, J. R., Ho, A., Schlussman, S. D., and Kreek, M. J. (2001). Predictable individual differences in the initiation of cocaine self-administration by rats under extended-access conditions are dose-dependent. Psychopharmacology 157, 31–39. doi: 10.1007/s002130100744
Mantsch, J. R., Yuferov, V., Mathieu-Kia, A. M., Ho, A., and Kreek, M. J. (2004). Effects of extended access to high versus low cocaine doses on self-administration, cocaine-induced reinstatement and brain mRNA levels in rats. Psychopharmacology 175, 26–36. doi: 10.1007/s00213-004-1778-x
Marchant, N. J., Khuc, T. N., Pickens, C. L., Bonci, A., and Shaham, Y. (2013). Context-induced relapse to alcohol seeking after punishment in a rat model. Biol. Psychiatry 73, 256–262. doi: 10.1016/j.biopsych.2012.07.007
McCutcheon, J. E., White, F. J., and Marinelli, M. (2009). Individual differences in dopamine cell neuroadaptations following cocaine self-administration. Biol. Psychiatry 66, 801–803. doi: 10.1016/j.biopsych.2009.04.018
Meil, W. M., and See, R. E. (1996). Conditioned cued recovery of responding following prolonged withdrawal from self-administered cocaine in rats: an animal model of relapse. Behav. Pharmacol. 7, 754–763.
Meririnne, E., Kankaanpaa, A., and Seppala, T. (2001). Rewarding properties of methylphenidate: sensitization by prior exposure to the drug and effects of dopamine D1- and D2-receptor antagonists. J. Pharmacol. Exp. Ther. 298, 539–550.
Meyer, P. J., Lovic, V., Saunders, B. T., Yager, L. M., Flagel, S. B., Morrow, J. D., et al. (2012). Quantifying individual variation in the propensity to attribute incentive salience to reward cues. PLoS One 7:e38987. doi: 10.1371/journal.pone.0038987
Miller, N. E. (1944). “Experimental studies of conflict,” in Personality and The Behavior Disorders, ed. J. McV. Hunt (New York, NY: Ronald Press), 431–465.
Millan, E. Z., Ong, Z., and McNally, G. P. (2017). Paraventricular thalamus: gateway to feeding, appetitive motivation and drug addiction. Prog. Brain Res. 235, 113–137. doi: 10.1016/bs.pbr.2017.07.006
Moazen, P., Azizi, H., Salmanzadeh, H., and Semnanian, S. (2018). Adolescent morphine exposure induces immediate and long-term increases in impulsive behavior. Psychopharmacology 235, 3423–3434. doi: 10.1007/s00213-018-5051-0
Monleón, S., Duque, A., Mesa-Gresa, P., Redolat, R., and Vinader-Caerols, C. (2019). An animal model of alcohol binge drinking: chronic-intermittent ethanol administration in rodents. Methods Mol. Biol. 2011, 281–293. doi: 10.1007/978-1-4939-9554-7_16
Morgan, D., Brebner, K., Lynch, W. J., and Roberts, D. C. (2002). Increases in the reinforcing efficacy of cocaine after particular histories of reinforcement. Behav. Pharmacol. 13, 389–396. doi: 10.1097/00008877-200209000-00012
Morgan, D., Smith, M. A., and Roberts, D. C. (2005). Binge self-administration and deprivation produces sensitization to the reinforcing effects of cocaine in rats. Psychopharmacology 178, 309–316. doi: 10.1007/s00213-004-1992-6
Mueller, D., and Stewart, J. (2000). Cocaine-induced conditioned place preference: reinstatement by priming injections of cocaine after extinction. Behav. Brain Res. 115, 39–47. doi: 10.1016/s0166-4328(00)00239-4
Müller, C. P. (2018). Animal models of psychoactive drug use and addiction-present problems and future needs for translational approaches. Behav. Brain Res. 352, 109–115. doi: 10.1016/j.bbr.2017.06.028
Nocjar, C., Middaugh, L. D., and Tavernetti, M. (1999). Ethanol consumption and place-preference conditioning in the alcohol-preferring C57BL/6 mouse: relationship with motor activity patterns. Alcohol. Clin. Exp. Res. 23, 683–692. doi: 10.1097/00000374-199904000-00015
Nocjar, C., and Panksepp, J. (2007). Prior morphine experience induces long-term increases in social interest and in appetitive behavior for natural reward. Behav. Brain Res. 181, 191–199. doi: 10.1016/j.bbr.2007.04.004
Nogales, F., Rua, R. M., Ojeda, M. L., Murillo, M. L., and Carreras, O. (2014). Oral or intraperitoneal binge drinking and oxidative balance in adolescent rats. Chem. Res. Toxicol. 27, 1926–1933. doi: 10.1021/tx5002628
O’Brien, C. P., Childress, A. R., McLellan, A. T., and Ehrman, R. (1992). Classical conditioning in drug-dependent humans. Ann. N Y Acad. Sci. 654, 400–415. doi: 10.1111/j.1749-6632.1992.tb25984.x
Oleson, E. B., Richardson, J. M., and Roberts, D. C. (2011). A novel IV cocaine self-administration procedure in rats: differential effects of dopamine, serotonin and GABA drug pre-treatments on cocaine consumption and maximal price paid. Psychopharmacology 214, 567–577. doi: 10.1007/s00213-010-2058-6
Oleson, E. B., and Roberts, D. C. (2009). Behavioral economic assessment of price and cocaine consumption following self-administration histories that produce escalation of either final ratios or intake. Neuropsychopharmacology 34, 796–804. doi: 10.1038/npp.2008.195
Paredes, R. G. (2009). Evaluating the neurobiology of sexual reward. ILAR J. 50, 15–27. doi: 10.1093/ilar.50.1.15
Paterson, N. E., and Markou, A. (2003). Increased motivation for self-administered cocaine after escalated cocaine intake. Neuroreport 14, 2229–2232. doi: 10.1097/00001756-200312020-00019
Paulson, P. E., Camp, D. M., and Robinson, T. E. (1991). Time course of transient behavioral depression and persistent behavioral sensitization in relation to regional brain monoamine concentrations during amphetamine withdrawal in rats. Psychopharmacology 103, 480–492. doi: 10.1007/bf02244248
Pavlov, I. (1927). Conditioned Reflexes: An Investigation of the Physiological Activity of the Cerebral Cortex. London: Oxford University Press.
Peters, H., Hunt, M., and Harper, D. (2010). An animal model of slot machine gambling: the effect of structural characteristics on response latency and persistence. J. Gambl. Stud. 26, 521–531. doi: 10.1007/s10899-010-9183-3
Phillips, T. J., Roberts, A. J., and Lessov, C. N. (1997). Behavioral sensitization to ethanol: genetics and the effects of stress. Pharmacol. Biochem. Behav. 57, 487–493. doi: 10.1016/s0091-3057(96)00448-0
Piazza, P. V., Deminiere, J. M., Le Moal, M., and Simon, H. (1989). Factors that predict individual vulnerability to amphetamine self-administration. Science 245, 1511–1513. doi: 10.1126/science.2781295
Piazza, P. V., Deminiere, J. M., Le Moal, M., and Simon, H. (1990). Stress- and pharmacologically-induced behavioral sensitization increases vulnerability to acquisition of amphetamine self-administration. Brain Res. 514, 22–26. doi: 10.1016/0006-8993(90)90431-a
Piazza, P. V., Deroche, V., Rouge-Pont, F., and Le Moal, M. (1998). Behavioral and biological factors associated with individual vulnerability to psychostimulant abuse. NIDA Res. Monogr. 169, 105–133.
Piazza, P. V., Deroche-Gamonent, V., Rouge-Pont, F., and Le Moal, M. (2000). Vertical shifts in self-administration dose-response functions predict a drug-vulnerable phenotype predisposed to addiction. J. Neurosci. 20, 4226–4232. doi: 10.1523/jneurosci.20-11-04226.2000
Piazza, P. V., Maccari, S., Deminiere, J. M., Le Moal, M., Mormede, P., and Simon, H. (1991). Corticosterone levels determine individual vulnerability to amphetamine self-administration. Proc. Natl. Acad. Sci. U S A 88, 2088–2092. doi: 10.1073/pnas.88.6.2088
Pierce, R. C., Bell, K., Duffy, P., and Kalivas, P. W. (1996). Repeated cocaine augments excitatory amino acid transmission in the nucleus accumbens only in rats having developed behavioral sensitization. J. Neurosci. 16, 1550–1560. doi: 10.1523/jneurosci.16-04-01550.1996
Pierre, P. J., and Vezina, P. (1998). D1 dopamine receptor blockade prevents the facilitation of amphetamine self-administration induced by prior exposure to the drug. Psychopharmacology 138, 159–166. doi: 10.1007/s002130050658
Pitchers, K. K., Phillips, K. B., Jones, J. L., Robinson, T. E., and Sarter, M. (2017a). Diverse roads to relapse: a discriminative cue signaling cocaine availability is more effective in renewing cocaine seeking in goal trackers than sign trackers and depends on basal forebrain cholinergic activity. J. Neurosci. 37, 7198–7208. doi: 10.1523/JNEUROSCI.0990-17.2017
Pitchers, K. K., Kane, L. F., Kim, Y., Robinson, T. E., and Sarter, M. (2017b). ‘Hot’ vs. ‘cold’ behavioural-cognitive styles: motivational-dopaminergic vs. cognitive-cholinergic processing of a Pavlovian cocaine cue in sign- and goal-tracking rats. Eur. J. Neurosci. 46, 2768–2781. doi: 10.1111/ejn.13741
Pitchers, K. K., Wood, T. R., Skrzynski, C. J., Robinson, T. E., and Sarter, M. (2017c). The ability for cocaine and cocaine-associated cues to compete for attention. Behav. Brain Res. 320, 302–315. doi: 10.1016/j.bbr.2016.11.024
Post, R. M., and Rose, H. (1976). Increasing effects of repetitive cocaine administration in the rat. Nature 260, 731–732. doi: 10.1038/260731a0
Power, Y., Goodyear, B., and Crockford, D. (2012). Neural correlates of pathological gamblers preference for immediate rewards during the iowa gambling task: an fMRI study. J. Gambl. Stud. 28, 623–636. doi: 10.1007/s10899-011-9278-5
Purgianto, A., Scheyer, A. F., Loweth, J. A., Ford, K. A., Tseng, K. Y., and Wolf, M. E. (2013). Different adaptations in AMPA receptor transmission in the nucleus accumbens after short vs. long access cocaine self-administration regimens. Neuropsychopharmacology 38, 1789–1797. doi: 10.1038/npp.2013.78
Pushparaj, A., Kim, A. S., Musiol, M., Zangen, A., Daskalakis, Z. J., Zack, M., et al. (2015). Differential involvement of the agranular vs. granular insular cortex in the acquisition and performance of choice behavior in a rodent gambling task. Neuropsychopharmacology 40, 2832–2842. doi: 10.1038/npp.2015.133
Rademacher, D. J., Anders, K. A., Thompson, K. J., and Steinpreis, R. E. (2000). The failure of some rats to acquire intravenous cocaine self-administration is attributable to conditioned place aversion. Behav. Brain Res. 117, 13–19. doi: 10.1016/s0166-4328(00)00277-1
Rash, C. J., Weinstock, J., and Van Patten, R. (2016). A review of gambling disorder and substance use disorders. Subst. Abuse Rehabil. 7, 3–13. doi: 10.2147/SAR.S83460
Rhodes, J. S., Best, K., Belknap, J. K., Finn, D. A., and Crabbe, J. C. (2005). Evaluation of a simple model of ethanol drinking to intoxication in C57BL/6J mice. Physiol. Behav. 84, 53–63. doi: 10.1016/j.physbeh.2004.10.007
Richardson, N. R., and Roberts, D. C. (1996). Progressive ratio schedules in drug self-administration studies in rats: a method to evaluate reinforcing efficacy. J. Neurosci. Methods 66, 1–11. doi: 10.1016/0165-0270(95)00153-0
Richter, S. H., Gass, P., and Fuss, J. (2014). Resting is rusting: a critical view on rodent wheel-running behavior. Neuroscientist 20, 313–325. doi: 10.1177/1073858413516798
Roberts, D. C., Loh, E. A., and Vickers, G. (1989). Self-administration of cocaine on a progressive ratio schedule in rats: dose-response relationship and effect of haloperidol pretreatment. Psychopharmacology 97, 535–538. doi: 10.1007/bf00439560
Roberts, D. C., Morgan, D., and Liu, Y. (2007). How to make a rat addicted to cocaine. Prog. Neuropsychopharmacol. Biol. Psychiatry 31, 1614–1624. doi: 10.1016/j.pnpbp.2007.08.028
Roberts, D. C. S., and Richardson, N. R. (1992). “Self-administration of psychomotor stimulants using progressive ratio schedules of reinforcement,” in Neuromethods (Vol. 24), eds A. Boulton, G. Baker and P. H. Wu (Totawa, NJ: Humana), 223–269.
Robinson, T. E., Angus, A. L., and Becker, J. B. (1985). Sensitization to stress: the enduring effects of prior stress on amphetamine-induced rotational behavior. Life Sci. 37, 1039–1042. doi: 10.1016/0024-3205(85)90594-6
Robinson, T. E., Becker, J. B., and Presty, S. K. (1982). Long-term facilitation of amphetamine-induced rotational behavior and striatal dopamine release produced by a single exposure to amphetamine: sex differences. Brain Res. 253, 231–241. doi: 10.1016/0006-8993(82)90690-4
Robinson, T. E., and Berridge, K. C. (1993). The neural basis of drug craving: an incentive-sensitization theory of addiction. Brain Res. Rev. 18, 247–291. doi: 10.1016/0165-0173(93)90013-p
Robinson, T. E., and Flagel, S. B. (2009). Dissociating the predictive and incentive motivational properties of reward-related cues through the study of individual differences. Biol. Psychiatry 65, 869–873. doi: 10.1016/j.biopsych.2008.09.006
Robison, A. J., and Nestler, E. J. (2011). Transcriptional and epigenetic mechanisms of addiction. Nat. Rev. Neurosci. 12, 623–637. doi: 10.1038/nrn3111
Rodefer, J. S., and Carroll, M. E. (1997). A comparison of progressive ratio schedules versus behavioral economic measures: effect of an alternative reinforcer on the reinforcing efficacy of phencyclidine. Psychopharmacology 132, 95–103. doi: 10.1007/s002130050325
Rogers, R. D., Wong, A., McKinnon, C., and Winstanley, C. A. (2013). Systemic administration of 8-OH-DPAT and eticlopride, but not SCH23390, alters loss-chasing behavior in the rat. Neuropsychopharmacology 38, 1094–1104. doi: 10.1038/npp.2013.8
Rømer Thomsen, K., Callesen, M. B., Hesse, M., Kvamme, T. L., Pedersen, M. M., Pedersen, M. U., et al. (2018). Impulsivity traits and addiction-related behaviors in youth. J. Behav. Addict. 7, 317–330. doi: 10.1556/2006.7.2018.22
Rougé-Pont, F., Piazza, P. V., Kharouby, M., Le Moal, M., and Simon, H. (1993). Higher and longer stress-induced increase in dopamine concentrations in the nucleus accumbens of animals predisposed to amphetamine self-administration. A microdialysis study. Brain Res. 602, 169–174. doi: 10.1016/0006-8993(93)90260-t
Sanchez, C. J., and Sorg, B. A. (2001). Conditioned fear stimuli reinstate cocaine-induced conditioned place preference. Brain Res. 908, 86–92. doi: 10.1016/s0006-8993(01)02638-5
Sanchez-Roige, S., Peña-Oliver, Y., Ripley, T. L., and Stephens, D. N. (2014). Repeated ethanol exposure during early and late adolescence: double dissociation of effects on waiting and choice impulsivity. Alcohol. Clin. Exp. Res. 38, 2579–2589. doi: 10.1111/acer.12535
Sanchez-Roige, S., Peña-Oliver, Y., and Stephens, D. N. (2012). Measuring impulsivity in mice: the five-choice serial reaction time task. Psychopharmacology 219, 253–270. doi: 10.1007/s00213-011-2560-5
Sarter, M., and Lustig, C. (2019). Cholinergic double duty: cue detection and attentional control. Curr. Opin. Psychol. 29, 102–107. doi: 10.1016/j.copsyc.2018.12.026
Sarter, M., and Phillips, K. B. (2018). The neuroscience of cognitive-motivational styles: sign- and goal-trackers as animal models. Behav. Neurosci. 132, 1–12. doi: 10.1037/bne0000226
Saunders, B. T., O’Donnell, E. G., Aurbach, E. L., and Robinson, T. E. (2014). A cocaine context renews drug seeking preferentially in a subset of individuals. Neuropsychopharmacology 39, 2816–2823. doi: 10.1038/npp.2014.131
Saunders, B. T., and Robinson, T. E. (2010). A cocaine cue acts as an incentive stimulus in some but not others: implications for addiction. Biol. Psychiatry 67, 730–736. doi: 10.1016/j.biopsych.2009.11.015
Saunders, B. T., and Robinson, T. E. (2011). Individual variation in the motivational properties of cocaine. Neuropsychopharmacology 36, 1668–1676. doi: 10.1038/npp.2011.48
Saunders, B. T., and Robinson, T. E. (2012). The role of dopamine in the accumbens core in the expression of Pavlovian-conditioned responses. Eur. J. Neurosci. 36, 2521–2532. doi: 10.1111/j.1460-9568.2012.08217.x
Saunders, B. T., Yager, L. M., and Robinson, T. E. (2013). Cue-evoked cocaine “craving”: role of dopamine in the accumbens core. J. Neurosci. 33, 13989–14000. doi: 10.1523/jneurosci.0450-13.2013
Schad, D. J., Rapp, M. A., Garbusow, M., Nebe, S., Sebold, M., Obst, E., et al. (2019). Dissociating neural learning signals in human sign- and goal-trackers. Nat. Hum. Behav. doi: 10.1038/s41562-019-0765-5 [Epub ahead of print].
Schuster, C. R., and Thompson, T. (1969). Self administration of and behavioral dependence on drugs. Annu. Rev. Pharmacol. 9, 483–502. doi: 10.1146/annurev.pa.09.040169.002411
Segal, D. S., and Mandell, A. J. (1974). Long-term administration of d-amphetamine: progressive augmentation of motor activity and stereotypy. Pharmacol. Biochem. Behav. 2, 249–255. doi: 10.1016/0091-3057(74)90060-4
Seif, T., Chang, S. J., Simms, J. A., Gibb, S. L., Dadgar, J., Chen, B. T., et al. (2013). Cortical activation of accumbens hyperpolarization-active NMDARs mediates aversion-resistant alcohol intake. Nat. Neurosci. 16, 1094–1100. doi: 10.1038/nn.3445
Shaham, Y., Shalev, U., Lu, L., De Wit, H., and Stewart, J. (2003). The reinstatement model of drug relapse: history, methodology and major findings. Psychopharmacology 168, 3–20. doi: 10.1007/s00213-002-1224-x
Shaham, Y., and Stewart, J. (1995). Stress reinstates heroin-seeking in drug-free animals: an effect mimicking heroin, not withdrawal. Psychopharmacology 119, 334–341. doi: 10.1007/bf02246300
Shalev, U., Highfield, D., Yap, J., and Shaham, Y. (2000). Stress and relapse to drug seeking in rats: studies on the generality of the effect. Psychopharmacology 150, 337–346. doi: 10.1007/s002130000441
Shepard, J. D., Bossert, J. M., Liu, S. Y., and Shaham, Y. (2004). The anxiogenic drug yohimbine reinstates methamphetamine seeking in a rat model of drug relapse. Biol. Psychiatry 55, 1082–1089. doi: 10.1016/j.biopsych.2004.02.032
Shippenberg, T. S., and Heidbreder, C. (1995). Sensitization to the conditioned rewarding effects of cocaine: pharmacological and temporal characteristics. J. Pharmacol. Exp. Ther. 273, 808–815.
Shippenberg, T. S., Heidbreder, C., and Lefevour, A. (1996). Sensitization to the conditioned rewarding effects of morphine: pharmacology and temporal characteristics. Eur. J. Pharmacol. 299, 33–39. doi: 10.1016/0014-2999(95)00852-7
Siemian, J. N., Xue, Z., Blough, B. E., and Li, J. X. (2017). Comparison of some behavioral effects of d- and l-methamphetamine in adult male rats. Psychopharmacology 234, 2167–2176. doi: 10.1007/s00213-017-4623-8
Sikora, M., Nicolas, C., Istin, M., Jaafari, N., Thiriet, N., and Solinas, M. (2018). Generalization of effects of environmental enrichment on seeking for different classes of drugs of abuse. Behav. Brain Res. 341, 109–113. doi: 10.1016/j.bbr.2017.12.027
Solberg Woods, L. C. (2014). QTL mapping in outbred populations: successes and challenges. Physiol. Genomics 46, 81–90. doi: 10.1152/physiolgenomics.00127.2013
Solinas, M., Chauvet, C., Thiriet, N., El Rawas, R., and Jaber, M. (2008). Reversal of cocaine addiction by environmental enrichment. Proc. Natl. Acad. Sci. U S A 105, 17145–17150. doi: 10.1073/pnas.0806889105
Solinas, M., Thiriet, N., Rawas El, R., Lardeux, V., and Jaber, M. (2009). Environmental enrichment during early stages of life reduces the behavioral, neurochemical, and molecular effects of cocaine. Neuropsychopharmacology 34, 1102–1111. doi: 10.1038/npp.2008.51
Sorg, B. A. (1992). Mesocorticolimbic dopamine systems: cross-sensitization between stress and cocaine. Ann. N Y Acad. Sci. 654, 136–144. doi: 10.1111/j.1749-6632.1992.tb25962.x
Spanagel, R., Holter, S. M., Allingham, K., Landgraf, R., and Zieglgansberger, W. (1996). Acamprosate and alcohol: I. Effects on alcohol intake following alcohol deprivation in the rat. Eur. J. Pharmacol. 305, 39–44. doi: 10.1016/0014-2999(96)00174-4
Staw, B. J. (1981). The escalation of commitment to a course of action. Acad. Manag. Rev. 6, 577–587. doi: 10.5465/amr.1981.4285694
Stewart, R. B., and Li, T. K. (1997). The neurobiology of alcoholism in genetically selected rat models. Alcohol Health Res. World 21, 169–176.
Strakowski, S. M., and Sax, K. W. (1998). Progressive behavioral response to repeated d-amphetamine challenge: further evidence for sensitization in humans. Biol. Psychiatry 44, 1171–1177. doi: 10.1016/s0006-3223(97)00454-x
Strakowski, S. M., Sax, K. W., Setters, M. J., and Keck, P. E. Jr. (1996). Enhanced response to repeated d-amphetamine challenge: evidence for behavioral sensitization in humans. Biol. Psychiatry 40, 872–880. doi: 10.1016/0006-3223(95)00497-1
Strong, D. R., and Kahler, C. W. (2007). Evaluation of the continuum of gambling problems using the DSM-IV. Addiction 102, 713–721. doi: 10.1111/j.1360-0443.2007.01789.x
Suto, N., Austin, J. D., and Vezina, P. (2001). Locomotor response to novelty predicts a rat’s propensity to self-administer nicotine. Psychopharmacology 158, 175–180. doi: 10.1007/s002130100867
Takahashi, K., Toyoshima, M., Ichitani, Y., and Yamada, K. (2019). Enhanced methamphetamine-induced conditioned place preference in risk-taking rats. Behav. Brain Res. doi: 10.1016/j.bbr.2019.112299 [Epub ahead of print].
Takeuchi, H., Tsurumi, K., Murao, T., Mizuta, H., Murai, T., and Takahashi, H. (2019). Amygdala volume is associated with risky probability cognition in gambling disorder. Addict. Biol. 24, 802–810. doi: 10.1111/adb.12640
Tanabe, J., Thompson, L., Claus, E., Dalwani, M., Hutchison, K., and Banich, M. T. (2007). Prefrontal cortex activity is reduced in gambling and nongambling substance users during decision-making. Hum. Brain Mapp. 28, 1276–1286. doi: 10.1002/hbm.20344
Tassin, J. P. (2008). Uncoupling between noradrenergic and serotonergic neurons as a molecular basis of stable changes in behavior induced by repeated drugs of abuse. Biochem. Pharmacol. 75, 85–97. doi: 10.1016/j.bcp.2007.06.038
Tatum, A. L., and Seevers, M. H. (1929). Experimental cocaine addiction. J. Pharmacol. Exp. Ther. 36, 401–410.
Thiele, T. E., and Navarro, M. (2014). “Drinking in the dark” (DID) procedures: a model of binge-like ethanol drinking in non-dependent mice. Alcohol 48, 235–241. doi: 10.1016/j.alcohol.2013.08.005
Toce-Gerstein, M., Gerstein, D. R., and Volberg, R. A. (2003). A hierarchy of gambling disorders in the community. Addiction 98, 1661–1672. doi: 10.1111/j.1360-0443.2003.00545.x
Torquet, N., Marti, F., Campart, C., Tolu, S., Nguyen, C., Oberto, V., et al. (2018). Social interactions impact on the dopaminergic system and drive individuality. Nat. Commun. 9:3081. doi: 10.1038/s41467-018-05526-5
Trask, S., Thrailkill, E. A., and Bouton, M. E. (2017). Occasion setting, inhibition, and the contextual control of extinction in Pavlovian and instrumental (operant) learning. Behav. Processes 137, 64–72. doi: 10.1016/j.beproc.2016.10.003
Tremblay, M., Cocker, P. J., Hosking, J. G., Zeeb, F. D., Rogers, R. D., and Winstanley, C. A. (2014). Dissociable effects of basolateral amygdala lesions on decision making biases in rats when loss or gain is emphasized. Cogn. Affect. Behav. Neurosci. 14, 1184–1195. doi: 10.3758/s13415-014-0271-1
Tunstall, B. J., and Kearns, D. N. (2014). Reinstatement in a cocaine versus food choice situation: reversal of preference between drug and non-drug rewards. Addict. Biol. 19, 838–848. doi: 10.1111/adb.12054
Tunstall, B. J., and Kearns, D. N. (2016). Cocaine can generate a stronger conditioned reinforcer than food despite being a weaker primary reinforcer. Addict. Biol. 21, 282–293. doi: 10.1111/adb.12195
Tzschentke, T. M. (2007). Measuring reward with the conditioned place preference (CPP) paradigm: update of the last decade. Addict. Biol. 12, 227–462. doi: 10.1111/j.1369-1600.2007.00070.x
U.S. Department of Health and Human Services, Food and Drug Administration, and Center for Drug Evaluation and Research (CDER). (2017). Assessment of Abuse Potential of Drugs Guidance for Industry. Silver Spring, MD: U.S. Department of Health and Human Services.
Uslaner, J. M., Acerbo, M. J., Jones, S. A., and Robinson, T. E. (2006). The attribution of incentive salience to a stimulus that signals an intravenous injection of cocaine. Behav. Brain Res. 169, 320–324. doi: 10.1016/j.bbr.2006.02.001
Valadez, A., and Schenk, S. (1994). Persistence of the ability of amphetamine preexposure to facilitate acquisition of cocaine self-administration. Pharmacol. Biochem. Behav. 47, 203–205. doi: 10.1016/0091-3057(94)90132-5
Valjent, E., Bertran-Gonzalez, J., Aubier, B., Greengard, P., Herve, D., and Girault, J. A. (2010). Mechanisms of locomotor sensitization to drugs of abuse in a two-injection protocol. Neuropsychopharmacology 35, 401–415. doi: 10.1038/npp.2009.143
Vanderschuren, L. J., and Pierce, R. C. (2010). Sensitization processes in drug addiction. Curr. Top. Behav. Neurosci. 3, 179–195. doi: 10.1007/7854_2009_21
Vanderschuren, L. J., Schmidt, E. D., De Vries, T. J., Van Moorsel, C. A., Tilders, F. J., and Schoffelmeer, A. N. (1999a). A single exposure to amphetamine is sufficient to induce long-term behavioral, neuroendocrine, and neurochemical sensitization in rats. J. Neurosci. 19, 9579–9586. doi: 10.1523/jneurosci.19-21-09579.1999
Vanderschuren, L. J., Schoffelmeer, A. N., Mulder, A. H., and De Vries, T. J. (1999b). Lack of cross-sensitization of the locomotor effects of morphine in amphetamine-treated rats. Neuropsychopharmacology 21, 550–559. doi: 10.1016/s0893-133x(99)00051-2
Vanderschuren, L. J., Tjon, G. H., Nestby, P., Mulder, A. H., Schoffelmeer, A. N., and De Vries, T. J. (1997). Morphine-induced long-term sensitization to the locomotor effects of morphine and amphetamine depends on the temporal pattern of the pretreatment regimen. Psychopharmacology 131, 115–122. doi: 10.1007/s002130050273
Varvel, S. A., Martin, B. R., and Lichtman, A. H. (2007). Lack of behavioral sensitization after repeated exposure to THC in mice and comparison to methamphetamine. Psychopharmacology 193, 511–519. doi: 10.1007/s00213-007-0811-2
Vendruscolo, L. F., Barbier, E., Schlosburg, J. E., Misra, K. K., Whitfield, T. W. Jr., Logrip, M. L., et al. (2012). Corticosteroid-dependent plasticity mediates compulsive alcohol drinking in rats. J. Neurosci. 32, 7563–7571. doi: 10.1523/jneurosci.0069-12.2012
Vendruscolo, L. F., Estey, D., Goodell, V., Macshane, L. G., Logrip, M. L., Schlosburg, J. E., et al. (2015). Glucocorticoid receptor antagonism decreases alcohol seeking in alcohol-dependent individuals. J. Clin. Invest. 125, 3193–3197. doi: 10.1172/JCI79828
Venniro, M., Zhang, M., Caprioli, D., Hoots, J. K., Golden, S. A., Heins, C., et al. (2018). Volitional social interaction prevents drug addiction in rat models. Nat. Neurosci. 21, 1520–1529. doi: 10.1038/s41593-018-0246-6
Vezina, P. (1996). D1 dopamine receptor activation is necessary for the induction of sensitization by amphetamine in the ventral tegmental area. J. Neurosci. 16, 2411–2420. doi: 10.1523/jneurosci.16-07-02411.1996
Vezina, P., and Stewart, J. (1990). Amphetamine administered to the ventral tegmental area but not to the nucleus accumbens sensitizes rats to systemic morphine: lack of conditioned effects. Brain Res. 516, 99–106. doi: 10.1016/0006-8993(90)90902-n
Voon, V., and Dalley, J. W. (2016). Translatable and back-translatable measurement of impulsivity and compulsivity: convergent and divergent processes. Curr. Top. Behav. Neurosci. 28, 53–91. doi: 10.1007/7854_2015_5013
Wade-Galuska, T., Winger, G., and Woods, J. H. (2007). A behavioral economic analysis of cocaine and remifentanil self-administration in rhesus monkeys. Psychopharmacology 194, 563–572. doi: 10.1007/s00213-007-0858-0
Waselus, M., Flagel, S. B., Jedynak, J. P., Akil, H., Robinson, T. E., and Watson, S. J. Jr. (2013). Long-term effects of cocaine experience on neuroplasticity in the nucleus accumbens core of addiction-prone rats. Neuroscience 248, 571–584. doi: 10.1016/j.neuroscience.2013.06.042
Weeks, J. R. (1962). Experimental morphine addiction: method for automatic intravenous injections in unrestrained rats. Science 138, 143–144. doi: 10.1126/science.138.3537.143
Weiss, F. (2005). Neurobiology of craving, conditioned reward and relapse. Curr. Opin. Pharmacol. 5, 9–19. doi: 10.1016/j.coph.2004.11.001
Weiss, F., Maldonado-Vlaar, C. S., Parsons, L. H., Kerr, T. M., Smith, D. L., and Ben-Shahar, O. (2000). Control of cocaine-seeking behavior by drug-associated stimuli in rats: effects on recovery of extinguished operant-responding and extracellular dopamine levels in amygdala and nucleus accumbens. Proc. Natl. Acad. Sci. U S A 97, 4321–4326. doi: 10.1073/pnas.97.8.4321
Wikler, A. (1973). Dynamics of drug dependence. Implications of a conditioning theory for research and treatment. Arch. Gen. Psychiatry 28, 611–616. doi: 10.1001/archpsyc.1973.01750350005001
Winger, G., Galuska, C. M., Hursh, S. R., and Woods, J. H. (2006). Relative reinforcing effects of cocaine, remifentanil, and their combination in rhesus monkeys. J. Pharmacol. Exp. Ther. 318, 223–229. doi: 10.1124/jpet.105.100461
Winstanley, C. A., and Clark, L. (2016). Translational models of gambling-related decision-making. Curr. Top. Behav. Neurosci. 28, 93–120. doi: 10.1007/7854_2015_5014
Winstanley, C. A., Cocker, P. J., and Rogers, R. D. (2011). Dopamine modulates reward expectancy during performance of a slot machine task in rats: evidence for a ‘near-miss’ effect. Neuropsychopharmacology 36, 913–925. doi: 10.1038/npp.2010.230
Wise, R. A. (1973). Voluntary ethanol intake in rats following exposure to ethanol on various schedules. Psychopharmacologia 29, 203–210. doi: 10.1007/bf00414034
Woods, L. C., and Mott, R. (2017). Heterogeneous stock populations for analysis of complex traits. Methods Mol. Biol. 1488, 31–44. doi: 10.1007/978-1-4939-6427-7_2
Woolverton, W. L., and Wang, Z. (2004). Relationship between injection duration, transporter occupancy and reinforcing strength of cocaine. Eur. J. Pharmacol. 486, 251–257. doi: 10.1016/j.ejphar.2004.01.003
Xue, Z., Siemian, J. N., Johnson, B. N., Zhang, Y., and Li, J. X. (2018). Methamphetamine-induced impulsivity during chronic methamphetamine treatment in rats: effects of the TAAR 1 agonist RO5263397. Neuropharmacology 129, 36–46. doi: 10.1016/j.neuropharm.2017.11.012
Yager, L. M., Pitchers, K. K., Flagel, S. B., and Robinson, T. E. (2015). Individual variation in the motivational and neurobiological effects of an opioid cue. Neuropsychopharmacology 40, 1269–1277. doi: 10.1038/npp.2014.314
Yager, L. M., and Robinson, T. E. (2013). A classically conditioned cocaine cue acquires greater control over motivated behavior in rats prone to attribute incentive salience to a food cue. Psychopharmacology 226, 217–228. doi: 10.1007/s00213-012-2890-y
Yanagita, T. (1986). Intravenous self-administration of (-)-cathinone and 2-amino-1–(2,5-dimethoxy-4-methyl)phenylpropane in rhesus monkeys. Drug Alcohol Depend. 17, 135–141. doi: 10.1016/0376-8716(86)90004-9
Zeeb, F. D., Baarendse, P. J., Vanderschuren, L. J., and Winstanley, C. A. (2015). Inactivation of the prelimbic or infralimbic cortex impairs decision-making in the rat gambling task. Psychopharmacology 232, 4481–4491. doi: 10.1007/s00213-015-4075-y
Zeeb, F. D., Robbins, T. W., and Winstanley, C. A. (2009). Serotonergic and dopaminergic modulation of gambling behavior as assessed using a novel rat gambling task. Neuropsychopharmacology 34, 2329–2343. doi: 10.1038/npp.2009.62
Zeeb, F. D., and Winstanley, C. A. (2011). Lesions of the basolateral amygdala and orbitofrontal cortex differentially affect acquisition and performance of a rodent gambling task. J. Neurosci. 31, 2197–2204. doi: 10.1523/jneurosci.5597-10.2011
Zeeb, F. D., and Winstanley, C. A. (2013). Functional disconnection of the orbitofrontal cortex and basolateral amygdala impairs acquisition of a rat gambling task and disrupts animals’ ability to alter decision-making behavior after reinforcer devaluation. J. Neurosci. 33, 6434–6443. doi: 10.1523/jneurosci.3971-12.2013
Zimmer, B. A., Dobrin, C. V., and Roberts, D. C. (2011). Brain-cocaine concentrations determine the dose self-administered by rats on a novel behaviorally dependent dosing schedule. Neuropsychopharmacology 36, 2741–2749. doi: 10.1038/npp.2011.165
Keywords: addiction models, preclinical studies, DSM-V, drug seeking behavior, relapse activity
Citation: Kuhn BN, Kalivas PW and Bobadilla A-C (2019) Understanding Addiction Using Animal Models. Front. Behav. Neurosci. 13:262. doi: 10.3389/fnbeh.2019.00262
Received: 30 August 2019; Accepted: 15 November 2019;
Published: 29 November 2019.
Edited by:
Maria Asuncion Aguilar, University of Valencia, SpainReviewed by:
Marco Venniro, National Institute on Drug Abuse (NIDA), United StatesSantiago Monleón, University of Valencia, Spain
Copyright © 2019 Kuhn, Kalivas and Bobadilla. This is an open-access article distributed under the terms of the Creative Commons Attribution License (CC BY). The use, distribution or reproduction in other forums is permitted, provided the original author(s) and the copyright owner(s) are credited and that the original publication in this journal is cited, in accordance with accepted academic practice. No use, distribution or reproduction is permitted which does not comply with these terms.
*Correspondence: Peter W. Kalivas, kalivasp@musc.edu; Ana-Clara Bobadilla, bobadilla@musc.edu