- 1Department of Psychiatry, Queen’s University, Kingston, ON, Canada
- 2Providence Care Hospital, Kingston, ON, Canada
A compulsive phenotype characterizes several neuropsychiatric illnesses – including but not limited to – schizophrenia and obsessive compulsive disorder. Because of its perceived etiological heterogeneity, it is challenging to disentangle the specific neurophysiology that precipitates compulsive behaving. Using polydipsia (or non-regulatory water drinking), we describe candidate neural substrates of compulsivity. We further postulate that aberrant neuroplasticity within cortically projecting structures [i.e., the bed nucleus of the stria terminalis (BNST)] and circuits that encode homeostatic emotions (thirst, hunger, satiety, etc.) underlie compulsive drinking. By transducing an inaccurate signal that fails to represent true homeostatic state, cortical structures cannot select appropriate and adaptive actions. Additionally, augmented dopamine (DA) reactivity in striatal projections to and from the frontal cortex contribute to aberrant homeostatic signal propagation that ultimately biases cortex-dependent behavioral selection. Responding becomes rigid and corresponds with both erroneous, inflexible encoding in both bottom-up structures and in top-down pathways. How aberrant neuroplasticity in circuits that encode homeostatic emotion result in the genesis and maintenance of compulsive behaviors needs further investigation.
Introduction
Compulsivity can be a dominant and debilitating clinical feature of several psychiatric conditions including obsessive-compulsive disorder (OCD), schizophrenia, substance abuse, and other obsessive-compulsive spectrum disorders (OCSD) (Hariprasad et al., 1980; Berman et al., 1995; Everitt and Robbins, 2005). Compulsions are repetitive, apparent, and purposeful behaviors that are performed according to certain rules or in a stereotyped fashion. When expressed, compulsions are time-consuming, cause significant distress, and interfere in both function and quality of daily life (American Psychiatric Association, 2013). Despite an emergent body of research, identifying the neurophysiology of compulsivity is challenging and the underlying neural mechanisms of compulsivity remain speculative.
Behavioral heterogeneity and symptom dimensionality observed across compulsive spectrum disorders complicates the search for a neurological trace of compulsivity. For instance, the “classical” compulsive behaviors seen in OCD are characterized by excessive repetition of intentional “normal” behaviors and/or mental acts in an attempt to soothe discomfort brought on by obsessions, the accompanying diagnostic feature of OCD. However, not all observed compulsions captured by this definition are associated with an OCD diagnosis. Primary polydipsia, or excessive, non-regulatory water drinking, is just one example of the clinical heterogeneity found in OCSD, a behavior that shares some of its core features with other psychiatric diagnoses including schizophrenia. By isolating and identifying common neurological substrates across diagnoses marked by compulsive behaving (including primary polydipsia associated with schizophrenia and OCD) we can highlight unique neurological features specific to compulsivity in psychiatric illness.
To further characterize the neuropathophysiology of psychiatric disease states, we need appropriate animal models. Some preclinical models of compulsivity have been enormously useful in distilling discrete mechanisms and neural representations of pathological behavior (Everitt and Robbins, 2005; Szechtman et al., 2017). Among the currently available animal models, schedule-induced polydipsia (SIP) is recognized as the most robust and replicable preclinical model of compulsivity (Woods et al., 1993; Moreno and Flores, 2012; Gardner Gregory, 2018). Excessive water drinking (polydipsia) occurs experimentally when hungry animals are exposed to intermittent/scheduled access to food and unlimited access to water (see Figure 1). In this protocol, some animals will drink themselves into a water intoxicated state mimicking primary polydipsia. SIP is an ideal animal model to study compulsivity specifically because the behavior is both ethological and ecological and can be induced across species, including humans (Schuster and Woods, 1966; Falk, 1969; Kachanoff et al., 1973; Dale, 1979).
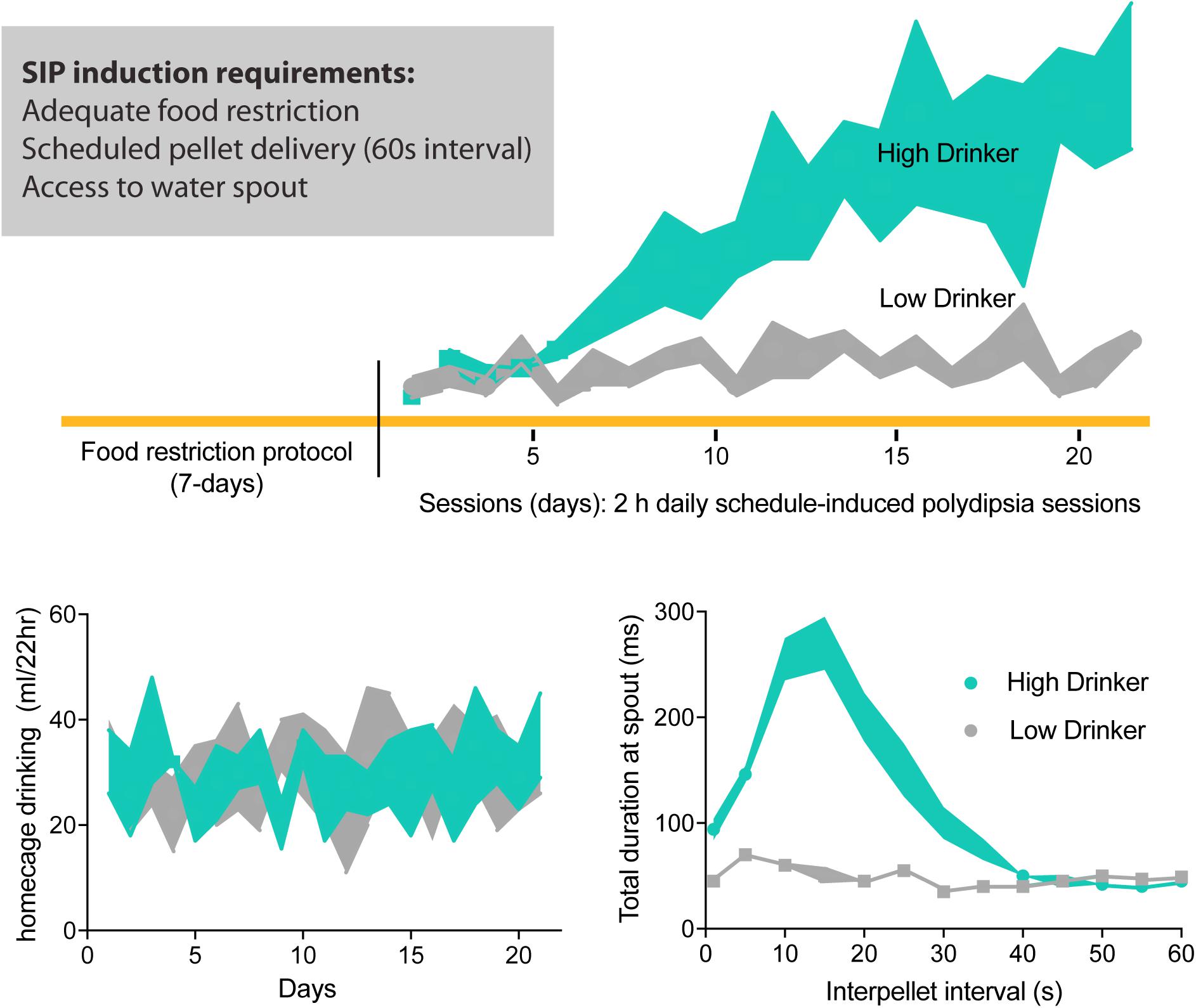
Figure 1. Schedule-induced polydipsia (SIP) protocol. Food restricted rodents are placed into an operant chamber with intermittent pellet delivery (recommended, 1 min interval) and access to water drinking spout. Over 2 h daily SIP sessions, some animals will develop SIP expressed as increased sessional drinking across days; low-drinking animals maintain low levels of water consumption. Daily 22 h homecage drinking during SIP protocol does not differ between high- and low-drinkers. Furthermore, high-drinkers show an adjunctive pattern of drinking during the interpellet interval 60 s, where more time is spent at the drinking spout post-prandial or adjacent to pellet consumption. Adapted with permission from Hawken et al. (2013b).
Decades of research using the SIP protocol enables a full exploration of the hypothesis presented here, that disordered neuroplasticity underlying essential homeostatic emotions within limbic and cortical structures contributes to the compulsive phenotype observed in polydipsia. Because compulsive drinking (expressed both as SIP in an OCD-like animal model and also as primary polydipsia associated with schizophrenia) can be induced in humans as well as rodents, we further speculate that identified neural substrates [including the bed nucleus of the stria terminalis (BNST)] can apply to psychiatric populations, i.e., those with OCSD. Thus, herein, we synthesize the current understanding of the neurophysiology of compulsivity through the lens of polydipsia.
Homeostatic Emotions Drive Appropriate Action Selection for Adaptive Behaving
Observationally, polydipsia is simply the overconsumption of fluid, and most often, water. Polydipsia can occur for several physiological reasons, for instance, in response to exaggerated thirst that results from disease states like diabetes insipidus. However, primary polydipsia or “psychogenic” polydipsia is non-physiologic and non-regulatory in nature, i.e., no underlying physiological condition can explain the individual’s need to overdrink. Polydipsia is maladaptive as over-drinking individuals seek and consume water despite the risk of severe life-threatening consequences (Hawken et al., 2009). Additionally, psychogenic polydipsia most commonly co-occurs with disordered thinking associated with a psychotic state. While some patients report drinking in response to excessive thirst, more report drinking excessively to feel better, to cleanse or purify themselves, or to appease/soothe other obsessions and/or delusions (Illowsky and Kirch, 1988; Millson et al., 1992). Observationally, in overdrinking patients normal thirst and satiety signals are no longer tied to homeostatic fluid regulation.
Maintaining adequate hydration is essential to an animal’s survival. Although most of our everyday drinking behavior is social, prandial, or habitual in nature and not in response to thirst, multiple fail-safe systems are in place to ensure that fluids lost are quickly replenished (McKinley et al., 2019). One such system is our brain’s ability to accurately recognize the feeling or emotion of “thirst” and behave appropriately (drink) to satiation. Most studies regulating thirst indicate that normal drinking behavior is generally preparatory, and to a lesser extent consummatory, in nature (Konorski, 1967; Egan et al., 2003; Saker et al., 2016). In other words, the brain quickly adjusts behavior in anticipation of falling dangerously far from homeostasis by initiating actions to maintain or restore fluid balance. Within the preparatory/consummatory framework, normal ingestive drinking behavior is therefore tightly regulated by a combination of incentive and homeostatic cues making the drive to drink both introceptively driven yet also perpetuated by anxiogenic situations that are extroceptively mediated. Drinking when thirsty is both pleasurable and rewarding, and extinction of thirst activates dopamine (DA) pathways in the brain (Ettenberg and Camp, 1986). Thus, many of the same brain regions that are activated in decision making, reward, stress, fear, and anxiety also mediate water seeking and intake (Egan et al., 2003; Shin and Liberzon, 2010). Such a coordinated response by multiple interconnected circuits across the brain ensures specific goal-directed behaviors are engaged to maintain fluid homeostasis to promote survival (Allen et al., 2019).
Being entirely subjective, thirst can be categorized along with the undeniable physiological need to eat, breath, and sleep as an essential homeostatic “emotion.” This primal and essential emotion arises from interoceptive cues compiled by sensory circumventricular organs that sense dramatic shifts in water and energy homeostasis (McKinley et al., 2019; Zimmerman et al., 2019). From these interfaces, interoceptive/homeostatic information is projected onto the cortex though polysynaptic relays including structures in the limbic system like the BNST (McKinley et al., 2019). Cortical structures decode the ascending homeostatic information to motivate appropriate behavior (to drink) and restore physiological thresholds, or quench one’s thirst (Craig, 2003). Interestingly, satiety or satiation of thirst is likely more than a lack of thirst or removal of a thirsty state, as distinct cortical regions are activated in each condition (McKinley et al., 2019). Allen et al. (2019) recently reported thirsty and sated states produce separate but proximal patterns of neuronal activation across several structures in the rodent brain. Such a brain-wide and independent representation of drinking behavior likely reflects the fact that thirst-satiety signals are evolutionarily necessary to prevent the threat of excessive hydration. Indeed, the satiety signal is so important that swallowing water is perceived first before any systemic absorption of water (McKinley, 2009). Thus satiety, like thirst, is itself an essential homeostatic emotion, with both playing pivotal roles in preventing the dangerous physiological consequences of unregulated non-homeostatic drinking.
While thirst and satiety are likely represented in various iterations across the brain, imaging studies in humans infused intravenously with hypertonic saline to induce thirst identify key structures involved in the conscious detection or “feeling” of thirst. Both positron emission tomography (PET) and functional magnetic resonance imaging (fMRI) show that the anterior cingulate (ACC) and insular cortex are activated in subjects made thirsty, and that satiation of thirst by drinking quickly extinguished that activity (Denton et al., 1999a,b; de Araujo et al., 2003; Egan et al., 2003). Upon wetting the mouth, activation of orbitofrontal cortex (OFC) occurs, a feature also extinguished with satiety. If subjects are asked to overdrink, a reactivation of insular cortex is seen, along with activation of the amygdala and periaqueductal gray regions – regions implicated in the “valence” networks and associated emotions including stress, fear, and anxiety (Saker et al., 2016). Despite widespread activity in several cortical and other brain regions correlating with thirst scores and subsequent satiety, conscious feelings of “thirst” and “satiety” and other homeostatic emotions arise from a yet to be identified aggregate or pattern of circuit/network activity (Allen et al., 2019).
Nevertheless, cortical regions including the ACC, insular, and OFC cortices have been implicated in many homeostatic emotions beyond thirst, including hunger and deep pain, reflecting an adverse condition within the body that requires immediate behavioral response (Craig, 2003). Generally, the ACC is activated by occurrences of adverse conditions that require decision making as to appropriate strategic responding (Gehring and Taylor, 2004). Accordingly, lesions of the ACC in humans, primates, and rats results in discernable apathy, or indifference and a lack of motivation to rectify an adverse condition (Eslinger and Damasio, 1985; Johansen et al., 2001). Conversely, a role for the insular cortex may be to identify the specific homeostatic perturbation and tag it with the appropriate emotional label – for instance, the insular cortex detects dehydration and translates it as thirst. Lastly the OFC, a cortical structure interconnected with ACC and insula, provides the hedonic component to the behavioral response of drinking to signal that water in the mouth is pleasant if thirsty but less so when not thirsty. Thus, sensory signals conveying the homeostatic state of the individual will reach the OFC, from the bottom-up, and be interpreted according to their biological significance to dictate appropriate action selection. In this proposed schema (McKinley, 2009), the cortical generation of the emotion of thirst would involve activations of neurons within the ACC for motivational intensity (urgency), the insula for homeostatic specificity (e.g., thirst, hunger, and satiety), and the orbito-frontal region for decision to initiate or maintain fluid seeking and consummatory behavior. However, as a clear neural “seat” of homeostatic emotions remains unknown, we can only speculate how homeostatic signals stop eliciting appropriate and adaptive behavioral repertoires in polydipsia.
Psychogenic Polydipsia in Schizophrenia
In primary or “psychogenic” polydipsia, homeostatic emotions somehow become disassociated from appropriate behavioral selection. A person with polydipsia may either feel thirsty but is unable to encode satiety to stop drinking behavior or is not drinking in response to thirst in the first place. Whether compulsive over-drinking results from an altered perception of thirst and/or satiety is unconfirmed (Goldman et al., 1996). However, in response to infusion of hypertonic saline, thirst ratings in compulsive water drinkers are higher than in normal drinkers, and remain elevated following drinking episodes. Conversely, drinking rapidly abolishes thirst emotion in non-compulsive drinkers (Thompson et al., 1991). To understand how or when a disconnect between homeostatic emotional drive-states and goal-directed behaviors occur, we must examine the context in which polydipsic behavior manifests.
The significant incidence of psychogenic polydipsia in patients with severe chronic psychiatric illness (de Leon et al., 1994) highlights central traits key to resolving the neurophysiology of compulsive overdrinking. While not exclusive to mental illness (Stuart et al., 1980), polydipsia is detected in over 20% of long-term institutionalized patients with a diagnosis of schizophrenia (Blum et al., 1983; Kirch et al., 1985; de Leon et al., 1996; Mercier-Guidez and Loas, 2000). Additionally, patients with polydipsia associated with schizophrenia are also more likely to be male and to compulsively smoke (de Leon et al., 1994; Mercier-Guidez and Loas, 2000). Furthermore, episodes of polydipsia-induced water intoxication in schizophrenia are correlated with a greater severity of psychotic illness, characterized by earlier onset of psychosis, severe symptom breakthrough in periods of illness stability, higher rates of alcohol abuse, lower levels of global functioning, and longer institutionalized living (Poirier et al., 2010). From a mechanistic perspective, severe polydipsia may represent an identifiable subgroup that exhibits more neurological impairments than those without compulsive drinking tendencies (Illowsky and Kirch, 1988).
Neurodegenerative processes may underlie some of the illness progression observed in schizophrenia (Lieberman, 1999) and may contribute to the incidence of polydipsia in this population. In first episode schizophrenia, structural alterations are present indicating whole brain and hippocampal volume reductions (Steen et al., 2006; Vita et al., 2006). Importantly, some structural changes progress in a subgroup of patients during the course of illness suggesting that polydipsia could be a behavioral expression of neurodegeneration (DeLisi et al., 2004). In support of this hypothesis, primary polydipsia is significantly associated with a chronic course of psychotic illness (de Leon et al., 1996). Accordingly, when polydipsia develops it typically onsets 5–15 years following the original psychiatric diagnosis (Vieweg V. et al., 1984; Vieweg W.V. et al., 1984). With disease progression in schizophrenia, stereotypical behaviors also become increasing common and can include compulsive smoking, odd grooming patterns, pacing, and other repetitive motor actions (Arieti, 1974; Luchins et al., 1992; Alexander et al., 1993; Tracy et al., 1996; Morrens et al., 2006). Polydipsia is typically clustered into this stereotypical or “bizarre behaviors” category. Thus, some posit that stereotypies and other ritualistic mannerisms (including polydipsia) co-occurring in schizophrenia constitute a coherent group of symptoms that are mediated by common (across diagnoses) neurological abnormalities (Luchins, 1990).
Polydipsia, Schizophrenia, and OCD: Is There Neurobiological Overlap?
In part due to polydipsia’s temporal association with other repetitive behaviors (grooming, pacing, and ruminating), some suggest that polydipsia is related to the specific stereotypies and compulsions observed in OCSD or OCD itself (Deas-Nesmith and Brewerton, 1992; Shutty et al., 1995; Subramanian et al., 2017). However, the incidence of co-occurring polydipsia in OCD is far less evident than the association of polydipsia with schizophrenia. Nevertheless, rates of obsessive-compulsive symptoms in psychotic illness vary greatly and are estimated to range between 2.5 and 64% (de Haan et al., 2013; Grover et al., 2017). Differential diagnoses pose challenges for both clinicians and investigators to distinguish between compulsions and stereotypic behavior (Berrios, 1989). Incidentally, a substantial proportion (up to 37.5%) of those with schizophrenia also have a diagnosis of OCD (Lysaker et al., 2000; Nechmad et al., 2003; de Haan et al., 2013; Grover et al., 2017). The high incidence rate of schizophrenia with concurrent OCD symptomology resulted in the formulation of a new clinical entity for the dual diagnosis termed “schizo-obsessive disorder” (Hwang and Opler, 1994; Scotti-Muzzi and Saide, 2017). Therefore, comparing the neurophysiology of compulsions associated with schizophrenia with OCD could identify common disease-specific mechanisms of compulsive behavior.
Indirect lines of evidence from imaging studies support a loss of brain matter across brain structures common to polydipsia, schizophrenia, and OCD (Table 1). While limited, imaging studies of patients with polydipsia associated with schizophrenia show a global loss of brain matter suggested by the presence of significantly enlarged ventricles as compared to those without polydipsia (Emsley et al., 1995; Leadbetter et al., 1999). While schizophrenia itself is not associated with ventricular enlargement (Ebdrup et al., 2010), individuals with OCD and poor insight also exhibit bilateral ventricular enlargement (Luxenberg et al., 1988; Okasha et al., 2000). Gray and white matter loss throughout fronto-cortico-striatal and limbic regions known to be dysfunctional in both schizophrenia and OCD could account for the observed ventricular enlargement (Harrison et al., 2009; Fornito et al., 2013; Jung et al., 2013; Huang et al., 2018).
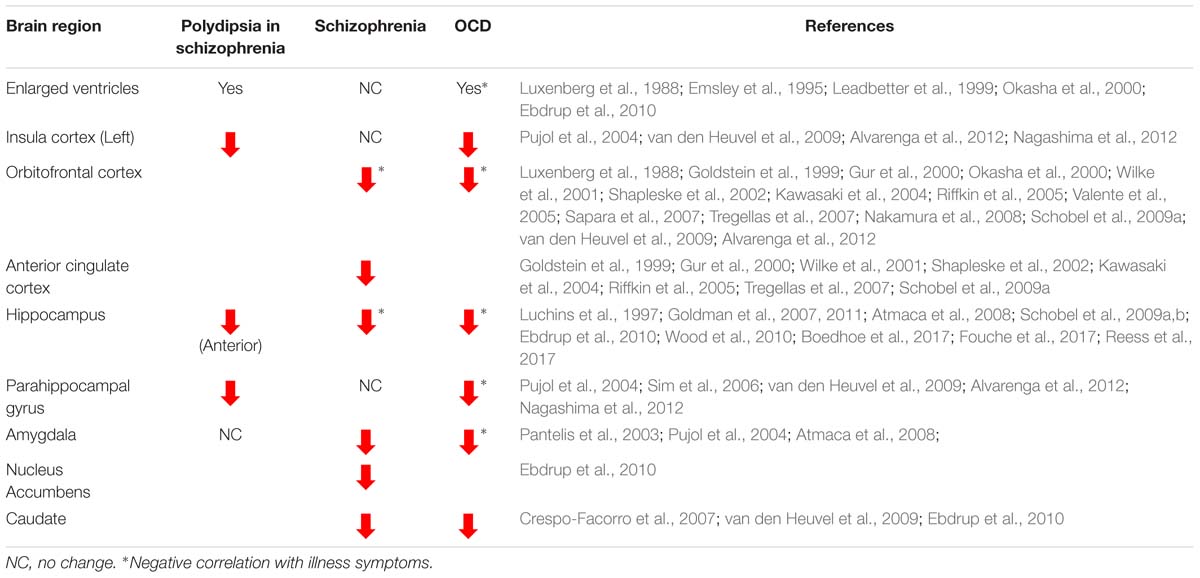
Table 1. Gray and white matter volume changes reported across the brain in polydipsia associated with schizophrenia, schizophrenia, and obsessive compulsive disorder (OCD).
In line with a view that reduced functional neuroplasticity in key networks contributes to OCSD, structural volume loss across fronto-cortico-striatal loops is consistently reported in both schizophrenia and OCD. In schizophrenia, reduced OFC and ACC volumes are linked with greater severity of formal thought disorder, low levels of insight, and a longer duration of the illness (Goldstein et al., 1999; Gur et al., 2000; Wilke et al., 2001; Shapleske et al., 2002; Kawasaki et al., 2004; Riffkin et al., 2005; Shad et al., 2006, Shad et al., 2007; Tregellas et al., 2007; Bellani and Brambilla, 2008; Nakamura et al., 2008; Schobel et al., 2009a). Resting state connectivity studies also correlate checking behavior in OCD with alterations in frontal regions, specifically the OFC and ACC (Harrison et al., 2013). Patients with polydipsia associated with schizophrenia additionally exhibit volume reductions in the left insular cortex (Nagashima et al., 2012) as do individuals with OCD and exaggerated checking compulsions (Pujol et al., 2004; van den Heuvel et al., 2009; Alvarenga et al., 2012). In striatal structures, reduced volumes are reported in the caudate nucleus in both treatment naïve, first-episode patients with schizophrenia and in OCD (Pujol et al., 2004; Crespo-Facorro et al., 2007; Atmaca et al., 2008; Rotge et al., 2009; van den Heuvel et al., 2009; Ebdrup et al., 2010). Additionally, in treatment refractory OCD, amygdalar volumes are negatively correlated with symptom “harm/checking” scores, illness duration, and symptom-severity (Pujol et al., 2004; Atmaca et al., 2008; Boedhoe et al., 2017; Fouche et al., 2017; Reess et al., 2018). Thus, reduced functional capacity of fronto-cortico-striatal and limbic structures likely contribute to the development of specific maladaptive behaviors associated with each disease state. However, how compulsive drinking (polydipsia) reflects deficits in neuroplasticity within pathologically common structures cannot be determined from available human imaging studies. Further insight into the neural plastic mechanisms regulating polydipsia can be gained from pre-clinical animal models of compulsive drinking.
Dopamine Drives Polydipsia in Humans and Rodents
Cumulative evidence suggests that schedule-induced polydipsia (SIP) is a valid animal model of compulsive water drinking (Woods et al., 1993; Moreno and Flores, 2012; Gardner Gregory, 2018). Good disease models show a similar pattern of symptoms to the disease being modeled, have measures that can be objectively quantified, and are both reproducible and robust (Geyer and Markou, 1994). In rodents, excessive drinking in the SIP protocol was first observed (Falk, 1961) when a hungry animal consumed excessive amounts of water during predictable but intermittent food access (i.e., scheduled; Figure 1). Like primary polydipsia, compulsive drinking induced by the SIP protocol is non-homeostatic in that animals engage in drinking behavior in the face of excessive overhydration (Falk, 1971). Like compulsions, SIP behavior is excessive, ritualistic, and maladaptive as animals can drink themselves into a dilutional water-toxic state that results in death (Hawken et al., 2013b). Furthermore, not all psychiatric patients nor all animals exposed to the SIP paradigm develop compulsive drinking. Such face validity suggests SIP is a powerful pre-clinical tool to help us understand how neurological, environmental, and genetic factors trigger or contribute to this compulsive phenotype (Rosenzweig-Lipson et al., 2007; Toscano et al., 2008; Moreno and Flores, 2012).
As the compulsion to overdrink in humans is postulated to be a behavioral manifestation of schizophrenia, some of the discrete neurobiology underlying the expression of polydipsia can be inferred from the neuropathology of schizophrenia itself. Currently, the etiology of schizophrenia continues to be debated, although it is generally conceptualized as a complex neurodevelopmental disorder with features of neurodegeneration and risk factors (both genetic and environmental) predicting its onset (Lieberman, 1999; Lewis and Lieberman, 2000; Tandon et al., 2008a,b). Historically, increased striatal DA function and impaired prefrontal cortical activity are the two most robust neuropathological features associated with schizophrenia’s core positive, negative, and cognitive symptoms (Lewis and Anderson, 1995; Laruelle and Abi-Dargham, 1999). Accordingly, DA is identified as one of the key neuromodulators dysregulated in schizophrenia.
In its original inception, the DA hypothesis proposed that schizophrenia results from DA overactivity within subcortical striatal structures (van Rossum, 1966). Evidence for hyperdopaminergia in schizophrenia was initially observed in chronic users of psychostimulants, where excessive use of DA-augmenting drugs induced psychotic states (Bell, 1965; Angrist and Gershon, 1970). Concurrently, antipsychotic drugs developed to alleviate psychosis did so through selectively blocking the DA D2 receptors (Seeman et al., 1975). Much later, increased dopaminergic activity in schizophrenia was confirmed by imaging studies. In patients with schizophrenia, displacement of DA at D2 receptors by amphetamine (AMPH)-induced DA release is increased in the striatum (Laruelle et al., 1996; Abi-Dargham et al., 1998; Laruelle and Abi-Dargham, 1999). Additionally, estimated striatal DA release following AMPH correlates with the severity of AMPH-exacerbated psychotic symptoms (Laruelle et al., 1999). However, striatal hyperdopaminergia fails to fully explain the negative and cognitive symptoms that also characterize schizophrenia. Thus, in a revised DA hypothesis, hyperdopaminergia, or increased reactivity of the mesolimbic DA system, is now primarily linked to the positive, psychotic features of schizophrenia, i.e., hallucinations and delusions (Carlsson, 1974) while a hypodopaminergia of the PFC may underlie observed cognitive dysfunction (Slifstein et al., 2015).
Psychogenic polydipsia in schizophrenia is temporally tied to psychosis, arguably a state of striatal hyperdopaminergia, where patients’ increased drinking behavior parallels psychotic exacerbation and is normalized when psychosis remits (Raskind et al., 1975; Zubenko et al., 1984). This suggests a role for elevated striatal DA function in primary polydipsia associated with schizophrenia. In animals, an ideally fluctuating amount of striatal DA is necessary for compulsive drinking in SIP to develop. Fixed, short intervals of food presentation to a food-restricted animal stimulates necessary DA activity (Cousins et al., 1999) with more DA released in the nucleus accumbens (NAc) following food consumption when animals are hungry versus when they are sated (Ahn and Phillips, 1999). In a hungry rat, DA levels increase in the NAc along-side elevated drinking over the course of several SIP trials (Hooks et al., 1994; Weissenborn et al., 1996; Moreno and Flores, 2012). This implies that an appropriately reactive DA system is necessary for SIP. Disrupting the integrity of the short-latency DA signal with acutely administered DA agonists and antagonists prior to each SIP session consistently prevents SIP development (see Table 2) (Mittleman et al., 1994; Flores and Pellón, 1995; Escher et al., 2006; López-Grancha et al., 2008). Furthermore, increased D2-like and decreased D1-like receptor binding throughout the NAc, medial prefrontal cortex, amygdala, and the ventral tagmental area (VTA) are associated with SIP expression (Pellón et al., 2011). Therefore, increased dopaminergic tone and/or reactivity throughout the mesolimbic and mesocortical networks may lead to aberrant reward-related learning (Banasikowski et al., 2012) that facilitates the compulsive water consumption as seen in primary polydipsia.
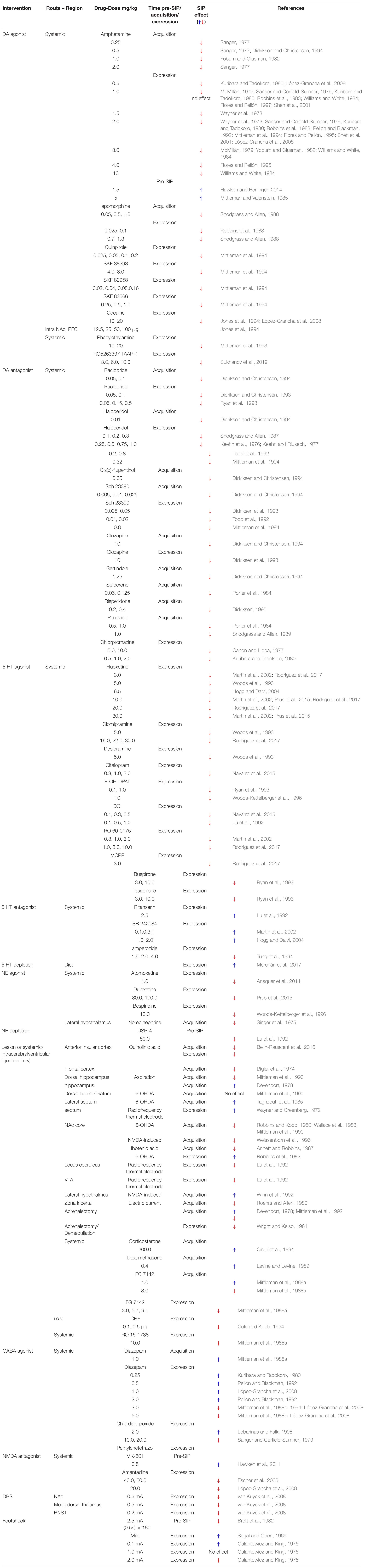
Table 2. Interventions and effects on compulsive behaving induced by the schedule-induced polydipsia (SIP) protocol.
By inducing subtle but functionally significant augmentation of the DA system, compulsive drinking can be enhanced. We assessed the ability of amphetamine sensitization (subchronic AMPH), NMDA hypofunction (subchronic MK-801), and social isolation (from weaning) models of schizophrenia-like symptoms to augment striatal DA and “mimic” a schizophrenia-like state in rodents. Evidence suggests that repeated AMPH and MK-801 permanently increases DA transmission along the rat VTA-NAc pathway (Hall et al., 1998; Jentsch et al., 1998) (for review see Svensson, 2000; Lodge and Grace, 2008, 2012; Beninger et al., 2009). Chronic exposure to NMDA induces a loss of GABAergic transmission to disinhibit DA neuron population activity of midbrain DA neurons (Floresco et al., 2003; Lodge and Grace, 2006). Cortical parvalbumin-positive GABA interneurons affected by chronic exposure to NMDA receptor antagonists are similarly affected by isolation rearing, a developmental model of schizophrenia (Jones et al., 1990; Wilkinson et al., 1994; Hall et al., 1998; Heidbreder et al., 2000; Miura et al., 2002; Fabricius et al., 2010, 2011; Han et al., 2011; Powell et al., 2012). As predicted, all models that increase striatal DA reactivity also significantly increased compulsive water drinking expressed as SIP (Hawken et al., 2011, 2013a; Hawken and Beninger, 2014).
Collectively, both animal and human data suggest that those with augmented, but intact, DA systems are prone to develop compulsive polydipsic behavior. In SIP, the temporal contiguity of the DA signal evoked by food stimuli precipitates a non-specific locomotor/approach behavior to an available drinking spout and facilitates acquisition of SIP (Jacobs and Farel, 1971; Vaccarino et al., 1989; Blackburn et al., 1992; Wise, 2004; Alcaro and Panksepp, 2011). Rats with a “compulsive phenotype” may have a more reactive DA system (e.g., a larger food-evoked DA signal and subsequent locomotor activation) and thus an increased sensitivity to the “activating” properties of food and other stimuli associated with schedules inside and outside the SIP-protocol. While overall homecage drinking may not differ between rats with SIP and those without, compulsive phenotypes may demonstrate a pattern of adjunctive drinking to any predictable (scheduled) environmental cues (Hawken et al., 2013b). In schizophrenia and particularly during psychotic episodes, individuals experience an exaggerated striatal DA response when presented with reinforcing stimuli, like meals, initiating generalized behavior and possibly drinking, if the appropriate stimuli (access to water) are available. Thus, the acutely psychotic and hospitalized individual could be prone to stereotypical/ritualistic behaviors due to augmented DA reactivity and the scheduled routines of institutionalized living. How DA specifically modulates circuits and systems central to OCSD to generate compulsive actions, however, must be studied through investigating SIP as an animal model of OCD.
Schedule-Induced Polydipsia as a Model of Obsessive Compulsive Disorder
Schedule-induced polydipsia is recognized as a valid model of compulsive behaving (i.e., OCD) in part due to the ability of serotonin reuptake inhibitors (SRIs) to disrupt SIP development and expression (Table 2). The ameliorative effects of SRIs on OCD symptoms were originally sufficient to assume serotonin (5-HT) dysfunction to be at the neurophysiological core of OCD symptoms (DeVeaugh-Geiss et al., 1989) for review see, (Barr et al., 1992). However, SRI monotherapy often fails (McDougle et al., 1994) and a subset of patients treatment-refractory to SRIs have benefited from adjunctive therapies that include DA antagonists (e.g., antipsychotics; for review see Koo et al., 2010).
Evidence to support a role for DA in OCD and other compulsive behaviors is reported. Notably, pharmacologically increasing DA neurotransmission with DA agonists exacerbates compulsivity traits and behaviors in both animal models (Szechtman et al., 1998) and susceptible humans (Frye and Arnold, 1981; Rosse et al., 1993; Kotsopoulos and Spivak, 2001). Evidence for modified synaptic DA activity (via dopamine transporter binding) in the striatum is also found in patients with OCD (van der Wee et al., 2004; Hesse et al., 2005). In SIP-prone rats, differences in the binding affinity of DA D1-like/D2-like receptors exist in limbic and cortical circuits (amygdala, VTA, NAc, and medial prefrontal cortex [mPFC]) (Pellón et al., 2011). Furthermore, pharmacological pretreatment with either DA agonists/antagonists, serotonergic or monoamine modulators, reduce SIP behaviors (i.e., drinking and licking; see Table 2) (Moreno and Flores, 2012; Navarro et al., 2015; Rodriguez et al., 2017; Sukhanov et al., 2019). High drinking rats also show elevated serotonergic activity in the amygdala (Moreno et al., 2012) and reduced 5-HT2A receptor binding in the mPFC (Mora et al., 2018) with additional evidence for changes in DA activity in the PFC, NAc, and amygdala (Moreno et al., 2012). Evidence supports a clear role for 5-HT, DA, and potentially 5-HT-DA interactions in the pathophysiology of compulsive phenotypes.
The DA hypothesis in OCSD is based on region-specific DA dysfunction within cortico-limbic-striato-thalamic-cortical (CLSTC) loops (Modell et al., 1989). For instance, enhanced or attenuated DA reactivity in some neurocircuits may change the weight of circuits to bias behavior toward habitual and compulsive responding (Nelson and Killcross, 2006; Ott and Nieder, 2019). Habits result from behavior performed frequently with an unchanging outcome and once established, are generally less flexible to future changes in predicted/expected outcomes (Balleine and Dickinson, 1998; Gillan et al., 2014). The medial parts of the striatum are necessary for goal-directed, outcome-sensitive behaviors but as responding becomes habitual, neuronal control gradually shifts to more lateral parts of the striatum critical for behavioral habits (Yin et al., 2004, 2006). In these regions, DA modulates the acquisition of stimulus-outcome, action-outcome and stimulus-response associations (Yin et al., 2004, 2006; Clarke et al., 2008; Xue et al., 2008; Belin et al., 2009). Flexible and appropriate behavioral responses or “cognitive control” via the frontal cortex is also heavily influenced by dopaminergic neuromodulation (Ott and Nieder, 2019). Thus, aberrant DA functioning in striatal and cortico-striatal loops that include the OFC and mPFC are believed to promote maladaptive or excessive habit formation like that observed in psychiatric disorders (Robbins et al., 2012; Gillan et al., 2016).
Accordingly, a dysfunctional balance between goal-directed and habit behavior with an over reliance on habitual circuitry may precipitate the compulsive phenotype (Gillan et al., 2011; Gremel et al., 2016). Technically, SIP is classified as an “adjunctive” behavior along with other behaviors reliably produced by schedules (for reviews see Flory, 1971; Roper, 1978; Singer et al., 1982). Adjunctive or displacement behaviors are considered a separate behavioral class outside of those produced by classical operant or Pavlovian learning paradigms, and are incentive in nature (Breland and Breland, 1961). Attempts to re-classify SIP as partially goal-directed in nature highlights the elements of the behavior that overlap with operant mechanisms (Killeen and Pellón, 2013). SIP expression is contingent on multiple pairings of salient stimuli in a defined context (Falk, 1966, 1967; Flory, 1971; Lashley and Rosellini, 1980) suggesting acquisition and expression of excessive drinking engages classical learning processes (Hawken and Beninger, 2014). Incidentally, initial SIP expression is likely the result of normal goal-directed learning that over time, can precede an over reliance on habitual responding observed in compulsivity (Hawken and Beninger, 2014) (for review see Gillan and Robbins, 2014).
The most commonly reported brain abnormality in OCD and OCSD is dysregulation of the neural feedback circuit that involves both goal-directed and habit circuits, including frontal, limbic, and striatal structures (Saxena et al., 1998; Graybiel and Rauch, 2000; Kopell and Greenberg, 2008). As in humans, compulsive-like animals may have an inherent predisposition to form habits and compulsive behavior due to imbalanced striatal circuits (Graybiel and Rauch, 2000; Gillan et al., 2011). In SIP, we demonstrated that animals who use predominately striatal-learning strategies (those that rely on the integrity of the striatum – specifically the DLS) (Packard and McGaugh, 1996) to learn the location of a food pellet in a Y-maze drink significantly more water when subsequently exposed to the SIP paradigm (Gregory et al., 2015). In addition, spine density in DLS neurons was found to increase following SIP demonstrating that plasticity in brain regions central to habit formation may contribute to SIP (Íbias et al., 2015). Furthermore, early in SIP training we found significant increases in neuronal activation in the mPFC and OFC regions. The increase in immediate early gene (IEG) FosB/ΔFosB was most pronounced in animals that demonstrated both SIP and striatal-learning tendencies. Repeated activation of OFC-ventral striatal pathway in mice produced grooming sensitization over days further supporting cortical-striatal involvement in compulsive behaving (Ahmari et al., 2013). Additionally, SIP-prone rats exhibited more c-Fos activity in the OFC and basolateral amygdala compared to non-compulsive drinking animals (Merchán et al., 2018). This is in line with human studies that show increased metabolic activity of the striatum and OFC in OCD patients and during symptom provocation (Breiter and Rauch, 1996; Saxena et al., 1999). However, the identity of the discrete mechanism(s) in the OFC/subcortical regions responsible for selecting appropriate behavior via goal-oriented or habit circuit recruitment in humans is not fully known.
The Bed Nucleus of the Stria Terminalis (BNST) in Homeostatic Emotion and Compulsive Behaving
Current effective treatments for OCDS may provide further insight into putative neurophysiology. Deep brain stimulation of the BNST has yielded sustained symptom relief in obsessions and compulsions in otherwise refractory OCD (Nuttin et al., 1999, 2013; Raymaekers et al., 2017; Winter et al., 2018). A collection of several sexually dimorphic interconnected nuclei, the BNST is part of the extended amygdala with extensive bi-directional connectivity with the CLSTC-loop and beyond (Prewitt and Herman, 1998; McDonald et al., 1999; Dong et al., 2001; Hasue and Shammah-Lagnado, 2002; Dong and Swanson, 2003, 2004b, 2006a, b, c; Rodaros et al., 2007; Li and Kirouac, 2008; Avery et al., 2014; Gregory et al., 2019). Functionally, the BNST filters and/or integrates multiple ascending modalities, mapping with adequate resolution, interoceptive information onto motivational systems for adaptive physiological and behavioral outcomes (Jennings et al., 2013; Kim et al., 2013; Lebow and Chen, 2016). Preclinical work compliments studies in humans: we have demonstrated a role for the oval subregion of the BNST (ovBNST) in compulsive behaviors including addiction, compulsive sucrose-seeking, and finally, in SIP (Krawczyk et al., 2013; Gardner Gregory, 2018; Maracle et al., 2019). Accordingly, electrical stimulation of the BNST is reported to suppress SIP in rodents (van Kuyck et al., 2008). Together, these findings highlight an emergent player, the BNST, in compulsive neurophysiology.
The BNST is fast becoming a relevant region of interest in stress-related psychiatric illness because of its role in emotion processing (Avery et al., 2016; Lebow and Chen, 2016). OCD is “stress responsive,” with stressful events precipitating OCD onset and symptoms (Toro et al., 1992). Nuclei within the BNST are bi-directionally connected to several nuclei in both the hypothalamus and the amygdala (Dong et al., 2001; Dong and Swanson, 2004a,b; Jennings et al., 2013), two structures implicated in stress and anxiety, respectively. A role for the cortico-amygdalar circuitry in OCD is emerging (Simon et al., 2014), however, how the hypothalamic-pituitary-adrenal (HPA)-axis, a key region involved in stress-reactivity, and stress itself impacts OCD pathophysiology is understudied. Along the HPA-axis in rodents, adrenalectomy hastens the emergence of SIP and exogenous corticosterone reverses the effect (Table 2; Devenport, 1978). In intact rats, corticosterone administration also inhibits SIP (Tang et al., 1984; Mittleman et al., 1988b). Furthermore, anxiogenic stimuli (e.g., foot shock) that increase corticosterone also suppress SIP acquisition (Brett et al., 1982). However, foot shock effects on SIP are dose-dependent, where low shock intensity augments SIP and high voltage prevents SIP (Galantowicz and King, 1975). Together, these findings indicate stress reactivity may modulate SIP and/or compulsivity. Given its role in processing classic emotional stimuli and events (e.g., stress and anxiety), we further postulate that the BNST encodes homeostatic emotions, specifically hunger and satiety, to guide appropriate adaptive responding.
Indeed, we recently identified a neural mechanism within the ovBNST that may represent the homeostatic emotion of hunger-satiety (Figure 2; Hawken et al., 2019). In order to establish compulsive drinking through the SIP protocol, animals must first be hungry, a drive-state typically induced through food restriction (Figure 1). In sated (fed ad libitum) male rats, low-frequency stimulation of ovBNST GABAergic synapses produces increases in inhibitory postsynaptic currents and promotes long-term potentiation (iLTP) in the majority of neurons recorded. Following acute food-restriction (24 h), however, GABAergic plasticity toggles to long-term depression (iLTD) via endocannabinoid dependent mechanisms (Figure 2; Hawken et al., 2019). In this framework, the novel endocannabinoid receptor GPR55 and its ligand, LPI, mediate a hunger-satiety signal (iLTP) while the classic endocannabinoid CB1 receptors and their ligand, 2-AG, promote a hunger-state (iLTD). This effect is plastic as after a brief refeeding window, iLTP is reinstated. In SIP-prone rats, bi-directional inhibitory plasticity in ovBNST neurons is lost, unable to toggle between iLTP and iLTD, as synapses become stuck transmitting a “hunger” signal well after being refed (Figure 2; Gardner Gregory, 2018). In this case, CB1 receptors and 2-AG drive reduced GABA transmission in the synapse and iLTD predominates. A loss of synaptic plasticity may be reflected in SIP studies that confirm SIP-prone rats continue to excessively drink despite correcting for their caloric deficit (Falk, 1971). Thus, synapses within the ovBNST driven by hunger and satiety cues lose the ability to correctly encode representative homeostatic emotion. Future research is needed to explore the consequences of inflexible GABAergic synapses in the ovBNST in the bottom-up development of complex behaviors like compulsivity.
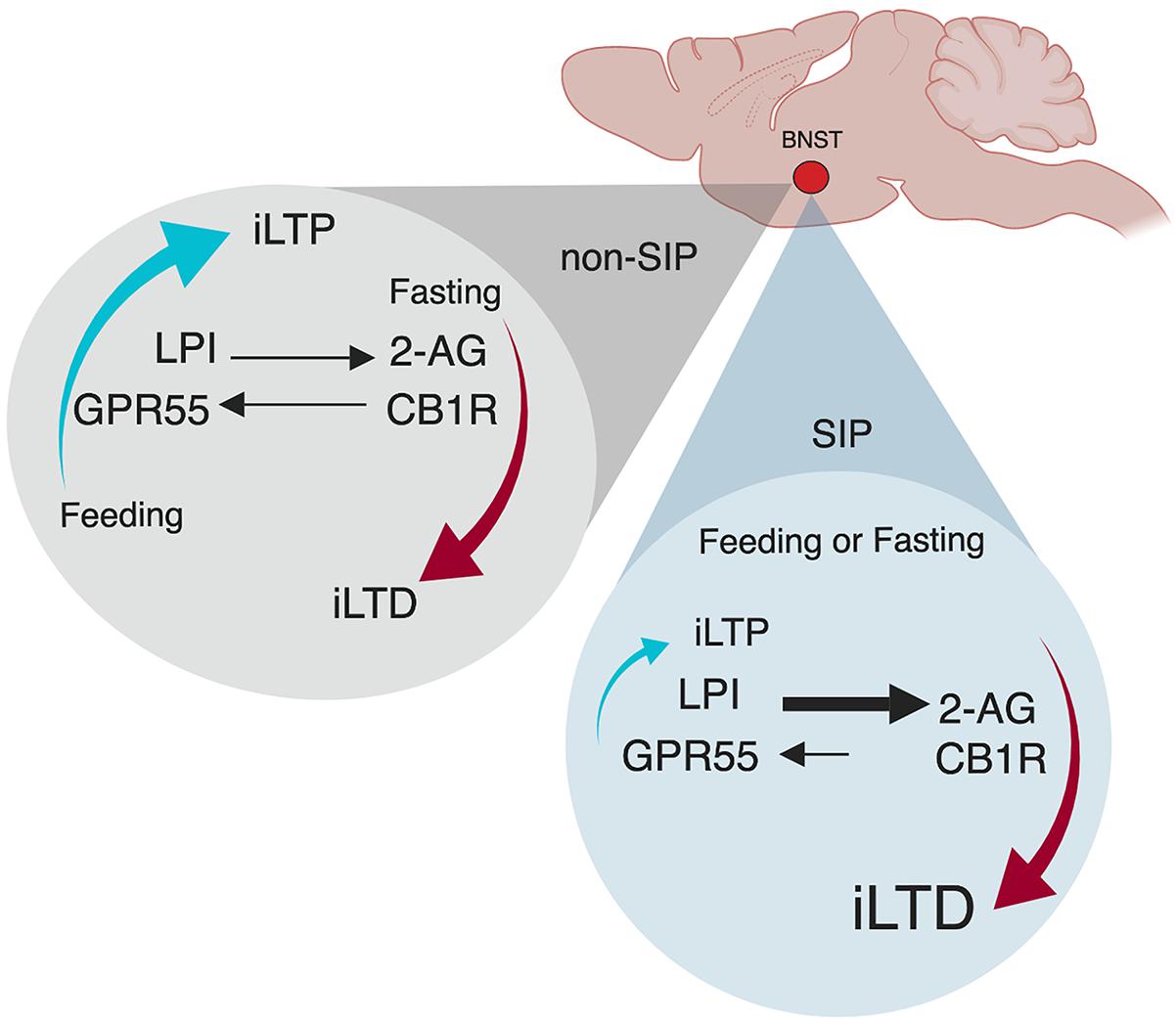
Figure 2. Oval bed nucleus of the stria terminalis (ovBNST) inhibitory plasticity is satiety-state independent in SIP. Adapted with permission from Hawken et al. (2019) and Gardner Gregory (2018). Plasticity at ovBNST GABAergic synapses is caloric-state dependent exhibiting bi-directional plasticity. In a sated state, synapses are biased toward iLTP but when food deprived, they can also express iLTD. When refed, synaptic plasticity quickly returns to iLTP. However, in SIP animals, refeeding does not reinstate iLTP, the mechanism becomes stuck as bidirectional plasticity is lost in animals that show compulsive behaving. iLTP, inhibitory long-term potentiation; iLTD, inhibitory long-term depression; LPI, L-α-lysophosphatidylinositol GPR55 ligand; GPR55, putative cannabinoid receptor; 2-AG, 2-arachidonoylglycerol CB1R ligand; CB1R, cannabinoid receptor.
A role for the BNST in compulsivity, however, is gleaned by the structural and functional connectivity of its nuclei within the CLSTC-loops implicated in compulsivity. Locally, the ovBNST subregion sends projections to the lateral hypothalamus with efferents and afferents to regions of the amygdala (Dong et al., 2001). The ovBNST also is bi-directionally connected to the fusiform (Dong et al., 2001), another nucleus within the BNST complex thought to receive projections from the frontal cortex (Lebow and Chen, 2016). Top-down, structural and functional projections from the frontal cortex (i.e., OFC/PFC/insula) to the BNST have been identified in humans, non-human primates, and rodents (Reynolds and Zahm, 2005; Fox et al., 2010; Motzkin et al., 2015). Bottom-up, pathways from the BNST to the frontal cortex are likely multi-synaptic and indirect via the amygdala, striatum, or other areas. For instance, the BNST in humans and rodents is both structurally and functionally connected to the NAc (Dong and Swanson, 2004b; Wood and Swann, 2005; Avery et al., 2014). Both the BNST and the NAc have prominent roles in compulsive drug use (Koob and Volkow, 2010, 2016; Krawczyk et al., 2013). Consistent with a distinct role in “valence surveillance” (Lebow and Chen, 2016), we postulate that the BNST, in part, contributes bottom-up afferent interoceptive information regarding homeostatic satiety via direct and indirect connections with the cortex and goal-directed and habit circuits to represent and convey homeostatic emotions of hunger and hunger-satiety.
As a candidate for encoding homeostatic emotions, the extended amygdala (i.e., the BNST) acts as an integrative hub to detect and signal exteroceptive and interoceptive shifts in the body. Subsequently, the BNST transduces homeostatic information onto circuits that assess contexts and invigorate behaviors to correct any physiological imbalance (eating when hungry, drinking when thirsty or escape/avoidance of threat stimulus). Thus, inaccurate neural representation of interoceptive information within the BNST could have drastic behavioral impact. For instance, the reinstatement of bi-directional GABA plasticity (i.e., iLTP) in the ovBNST following hunger-satiety could signal to the cortical structures a need to shift responding strategies to stop food-seeking behaviors. When iLTD persists despite homeostatic correction (feeding), cortical structures receive a “hunger” signal and continue to engage circuits that promote preparatory and consummatory behaviors, including locomotion and drinking. Convergent findings from animal and human research postulate that together with the mPFC (Killcross and Coutureau, 2003), activity of the OFC manages the activity of subcortical pathways including the striatum (Gremel and Costa, 2013; Pauls et al., 2014; Gremel et al., 2016). Perhaps based on the firing frequency of glutamatergic inputs to the OFC, the OFC selects the appropriate circuit to activate (either goal-oriented or habit) downstream from the cortex (Gremel et al., 2016). In the presence of increased striatal DA reactivity, circuits may be weighted for preferential activation by cortical structures, resulting in a loss of striatal circuit plasticity. In this way, inadequate resolution of homeostatic emotion by subcortical structures through aberrant neural plasticity may promote maladaptive behavioral responding ultimately dictated by the OFC (Hardung et al., 2017).
Conclusion
Using compulsive drinking in humans (primary polydipsia) and rodents (schedule-induced polydipsia) we suggest a putative neurobiological framework for the etiology of compulsive behaviors. Thirst, hunger, and satiety constitute essential homeostatic emotions, or subjective feelings that drive motivated, goal-directed behavioral selection needed for survival. In polydipsia, homeostatic emotions fail to illicit appropriate adaptive behaviors. How the brain might switch from a state responsive to shifts in homeostasis to one that is unresponsive was surmised by identifying key over-lapping brain changes between polydipsia associated with schizophrenia, schizophrenia without polydipia, and obsessive compulsive disorder. Composite data suggest that DA-regulated neural plasticity within the striatum and regions of the cortex underlie compulsive phenotypes. Ecological and validated animal models of compulsive drinking, or SIP, confirm a role for increased striatal DA activity in compulsive behaving. Additionally, literature points toward aberrant (non-exclusive) monoamine (e.g., DA) modulation of goal-directed and habitual behavior within cortico-limbic-striato-thalamic-cortical loops. Exciting new evidence suggests that non-representative encoding of homeostatic emotions (hunger/satiety) by a cluster of nuclei in the extended amygdala, promote the development of compulsions observed in SIP. The BNST’s role in valence attribution or “valence surveillance” and its extensive web of connectivity within the cognitive, affective, and reward-related circuits highlights the importance of accurate encoding and bottom-up (to the cortex) transduction of homeostatic emotions. Aberrant neuroplasticity within bottom-up and top-down circuits that support homeostatic emotion signaling likely contribute to maladaptive behavioral selection and inappropriate responding. Future research can explore our postulated mechanism of compulsive behaving.
Author Contributions
TB and EH equally contributed to the design, writing, and editing of the manuscript.
Conflict of Interest Statement
The authors declare that the research was conducted in the absence of any commercial or financial relationships that could be construed as a potential conflict of interest.
References
Abi-Dargham, A., Gil, R., Krystal, J., Baldwin, R. M., Seibyl, J. P., Bowers, M. et al. (1998). Increased striatal dopamine transmission in schizophrenia: confirmation in a second cohort. Am. J. Psychiatry 155, 761–767. doi: 10.1176/ajp.155.6.761
Ahmari, S. E., Spellman, T., Douglass, N. L., Kheirbek, M. A., Simpson, H. B., Deisseroth, K. et al. (2013). Repeated cortico-striatal stimulation generates persistent OCD-like behavior. Science 340, 1234–1239. doi: 10.1126/science.1234733
Ahn, S., and Phillips, A. G. (1999). Dopaminergic correlates of sensory-specific satiety in the medial prefrontal cortex and nucleus accumbens of the rat. J. Neurosci. 19:RC29.
Alcaro, A., and Panksepp, J. (2011). The SEEKING mind: primal neuro-affective substrates for appetitive incentive states and their pathological dynamics in addictions and depression. Neurosci. Biobehav. Rev. 35, 1805–1820. doi: 10.1016/j.neubiorev.2011.03.002
Alexander, R. C., Lowenkopf, T., Certa, K. M., and Luchins, D. J. (1993). Repetitive behaviors in schizophrenic patients admitted to an acute-care unit: a replicative study. J. Nerv. Ment. Dis. 181, 763–764.
Allen, W. E., Chen, M. Z., Pichamoorthy, N., Tien, R. H., Pachitariu, M., Luo, L. et al. (2019). Thirst regulates motivated behavior through modulation of brainwide neural population dynamics. Science 364:253. doi: 10.1126/science.aav3932
Alvarenga, P. G., do Rosário, M. C., Batistuzzo, M. C., Diniz, J. B., Shavitt, R. G., Duran, F. L. S. et al. (2012). Obsessive-compulsive symptom dimensions correlate to specific gray matter volumes in treatment-naïve patients. J. Psychiatr. Res. 46, 1635–1642. doi: 10.1016/j.jpsychires.2012.09.002
American Psychiatric Association (2013). Diagnostic and Statistical Manual of Mental Disorders, 5th Edn. Washington, DC: American Psychiatric Association.
Angrist, B. M., and Gershon, S. (1970). The phenomenology of experimentally induced amphetamine psychosis–preliminary observations. Biol. Psychiatry 2, 95–107.
Annett, L., and Robbins, T. (1987). Ibotenic acid lesions of the nucleus accumbens block the acquisition of adjunctive drinking. Behav. Brain Res. 26:203. doi: 10.1016/0166-4328(87)90170-7
Ansquer, S., Belin-Rauscent, A., Dugast, E., Duran, T., Benatru, I., Mar, A. C. et al. (2014). Atomoxetine decreases vulnerability to develop compulsivity in high impulsive rats. Biol. Psychiatry 75, 825–832. doi: 10.1016/j.biopsych.2013.09.031
Arieti, S. (1974). Acting out and unusual behavior in schizophrenia. Am. J. Psychother. 28, 333–342. doi: 10.1176/appi.psychotherapy.1974.28.3.333
Atmaca, M., Yildirim, H., Ozdemir, H., Ozler, S., Kara, B., Ozler, Z. et al. (2008). Hippocampus and amygdalar volumes in patients with refractory obsessive-compulsive disorder. Prog. Neuropsychopharmacol. Biol. Psychiatry 32, 1283–1286. doi: 10.1016/j.pnpbp.2008.04.002
Avery, S. N., Clauss, J. A., and Blackford, J. U. (2016). The human BNST: functional role in anxiety and addiction. Neuropsychopharmacology 41, 126–141. doi: 10.1038/npp.2015.185
Avery, S. N., Clauss, J. A., Winder, D. G., Woodward, N., Heckers, S., and Blackford, J. U. (2014). BNST neurocircuitry in humans. Neuroimage 91, 311–323. doi: 10.1016/j.neuroimage.2014.01.017
Balleine, B. W., and Dickinson, A. (1998). Goal-directed instrumental action: contingency and incentive learning and their cortical substrates. Neuropharmacology 37, 407–419. doi: 10.1016/s0028-3908(98)00033-1
Banasikowski, T. J., MacLeod, L. S., and Beninger, R. J. (2012). Comparison of nafadotride, CNQX, and haloperidol on acquisition versus expression of amphetamine-conditioned place preference in rats. Behav. Pharmacol. 23, 89–97. doi: 10.1097/FBP.0b013e32834ecb32
Barr, L. C., Goodman, W. K., Price, L. H., McDougle, C. J., and Charney, D. S. (1992). The serotonin hypothesis of obsessive compulsive disorder: implications of pharmacologic challenge studies. J. Clin. Psychiatry 53(Suppl.), 17–28.
Belin, D., Jonkman, S., Dickinson, A., Robbins, T. W., and Everitt, B. J. (2009). Parallel and interactive learning processes within the basal ganglia: relevance for the understanding of addiction. Behav. Brain Res. 199, 89–102. doi: 10.1016/j.bbr.2008.09.027
Belin-Rauscent, A., Daniel, M.-L., Puaud, M., Jupp, B., Sawiak, S., Howett, D. et al. (2016). From impulses to maladaptive actions: the insula is a neurobiological gate for the development of compulsive behavior. Mol. Psychiatry 21, 491–499. doi: 10.1038/mp.2015.140
Bell, D. S. (1965). Comparison of amphetamine psychosis and schizophrenia. Br. J. Psychiatry J. Ment. Sci. 111, 701–707. doi: 10.1192/bjp.111.477.701
Bellani, M., and Brambilla, P. (2008). Is there a neurobiological basis of insight in schizophrenia? Epidemiol. Psichiatr. Soc. 17, 28–30. doi: 10.1017/s1121189x00002657
Beninger, R. J., Forsyth, J. K., Van Adel, M., Reynolds, J. N., Boegman, R. J., and Jhamandas, K. (2009). Subchronic MK-801 behavioural deficits in rats: partial reversal by the novel nitrate GT 1061. Pharmacol. Biochem. Behav. 91, 495–502. doi: 10.1016/j.pbb.2008.09.003
Berman, I., Kalinowski, A., Berman, S. M., Lengua, J., and Green, A. I. (1995). Obsessive and compulsive symptoms in chronic schizophrenia. Compr. Psychiatry 36, 6–10.
Berrios, G. E. (1989). Obsessive-compulsive disorder: its conceptual history in France during the 19th century. Compr. Psychiatry 30, 283–295. doi: 10.1016/0010-440x(89)90052-7
Bigler, E. D., Fleming, D. E., and Shearer, D. E. (1974). Schedule-induced polydipsia and neocortical lesions in the rat. Psychol. Rep. 35, 935–941. doi: 10.2466/pr0.1974.35.2.935
Blackburn, J. R., Pfaus, J. G., and Phillips, A. G. (1992). Dopamine functions in appetitive and defensive behaviours. Prog. Neurobiol. 39, 247–279. doi: 10.1016/0301-0082(92)90018-a
Blum, A., Tempey, F. W., and Lynch, W. J. (1983). Somatic findings in patients with psychogenic polydipsia. J. Clin. Psychiatry 44, 55–56.
Boedhoe, P. S. W., Schmaal, L., Abe, Y., Ameis, S. H., Arnold, P. D., Batistuzzo, M. C. et al. (2017). Distinct subcortical volume alterations in pediatric and adult OCD: a worldwide meta- and mega-analysis. Am. J. Psychiatry 174, 60–69. doi: 10.1176/appi.ajp.2016.16020201
Breiter, H. C., and Rauch, S. L. (1996). Functional MRI and the study of OCD: from symptom provocation to cognitive-behavioral probes of cortico-striatal systems and the amygdala. Neuroimage 4, S127–S138. doi: 10.1006/nimg.1996.0063
Breland, K., and Breland, M. (1961). The misbehavior of organisms. Am. Psychol. 16, 681–684. doi: 10.1037/h0040090
Brett, L. P., Patterson, J., and Levine, S. (1982). Adjunctive drinking and the pituitary-adrenal response: effects of prior aversive stimulation (preshock). Physiol. Behav. 29, 219–223. doi: 10.1016/0031-9384(82)90007-5
Canon, J. G., and Lippa, A. S. (1977). Effects of clozapine, chlorpromazine and diazepam upon adjunctive and schedule controlled behaviors. Pharmacol. Biochem. Behav. 6, 581–587. doi: 10.1016/0091-3057(77)90120-4
Carlsson, A. (1974). Antipsychotic drugs and catecholamine synapses. J. Psychiatr. Res. 11, 57–64. doi: 10.1016/b978-0-08-018242-1.50014-3
Cirulli, F., van Oers, H., De Kloet, E. R., and Levine, S. (1994). Differential influence of corticosterone and dexamethasone on schedule-induced polydipsia in adrenalectomized rats. Behav. Brain Res. 65, 33–39. doi: 10.1016/0166-4328(94)90070-1
Clarke, H. F., Robbins, T. W., and Roberts, A. C. (2008). Lesions of the medial striatum in monkeys produce perseverative impairments during reversal learning similar to those produced by lesions of the orbitofrontal cortex. J. Neurosci. 28, 10972–10982. doi: 10.1523/JNEUROSCI.1521-08.2008
Cole, B. J., and Koob, G. F. (1994). Corticotropin-releasing factor and schedule-induced polydipsia. Pharmacol. Biochem. Behav. 47, 393–398. doi: 10.1016/0091-3057(94)90134-1
Cousins, M. S., Trevitt, J., Atherton, A., and Salamone, J. D. (1999). Different behavioral functions of dopamine in the nucleus accumbens and ventrolateral striatum: a microdialysis and behavioral investigation. Neuroscience 91, 925–934. doi: 10.1016/s0306-4522(98)00617-4
Craig, A. D. (2003). Interoception: the sense of the physiological condition of the body. Curr. Opin. Neurobiol. 13, 500–505. doi: 10.1016/s0959-4388(03)00090-4
Crespo-Facorro, B., Roiz-Santiáñez, R., Pelayo-Terán, J. M., González-Blanch, C., Pérez-Iglesias, R., Gutiérrez, A. et al. (2007). Caudate nucleus volume and its clinical and cognitive correlations in first episode schizophrenia. Schizophr. Res. 91, 87–96. doi: 10.1016/j.schres.2006.12.015
Dale, R. H. (1979). Concurrent drinking by pigeons on fixed-interval reinforcement schedules. Physiol. Behav. 23, 977–980. doi: 10.1016/0031-9384(79)90212-9
de Araujo, I. E. T., Kringelbach, M. L., Rolls, E. T., and McGlone, F. (2003). Human cortical responses to water in the mouth, and the effects of thirst. J. Neurophysiol. 90, 1865–1876. doi: 10.1152/jn.00297.2003
de Haan, L., Sterk, B., Wouters, L., and Linszen, D. H. (2013). The 5-year course of obsessive-compulsive symptoms and obsessive-compulsive disorder in first-episode schizophrenia and related disorders. Schizophr. Bull. 39, 151–160. doi: 10.1093/schbul/sbr077
de Leon, J., Dadvand, M., Canuso, C., Odom-White, A., Stanilla, J., and Simpson, G. M. (1996). Polydipsia and water intoxication in a long-term psychiatric hospital. Biol. Psychiatry 40, 28–34. doi: 10.1016/0006-3223(95)00353-3
de Leon, J., Verghese, C., Tracy, J. I., Josiassen, R. C., and Simpson, G. M. (1994). Polydipsia and water intoxication in psychiatric patients: a review of the epidemiological literature. Biol. Psychiatry 35, 408–419. doi: 10.1016/0006-3223(94)90008-6
Deas-Nesmith, D., and Brewerton, T. D. (1992). A case of fluoxetine-responsive psychogenic polydipsia: a variant of obsessive-compulsive disorder? J. Nerv. Ment. Dis. 180, 338–339. doi: 10.1097/00005053-199205000-00009
DeLisi, L. E., Sakuma, M., Maurizio, A. M., Relja, M., and Hoff, A. L. (2004). Cerebral ventricular change over the first 10 years after the onset of schizophrenia. Psychiatry Res. 130, 57–70. doi: 10.1016/j.pscychresns.2003.08.004
Denton, D., Shade, R., Zamarippa, F., Egan, G., Blair-West, J., McKinley, M. et al. (1999a). Correlation of regional cerebral blood flow and change of plasma sodium concentration during genesis and satiation of thirst. Proc. Natl. Acad. Sci. U.S.A. 96, 2532–2537. doi: 10.1073/pnas.96.5.2532
Denton, D., Shade, R., Zamarippa, F., Egan, G., Blair-West, J., McKinley, M. et al. (1999b). Neuroimaging of genesis and satiation of thirst and an interoceptor-driven theory of origins of primary consciousness. Proc. Natl. Acad. Sci. U.S.A. 96, 5304–5309. doi: 10.1073/pnas.96.9.5304
DeVeaugh-Geiss, J., Landau, P., and Katz, R. (1989). Preliminary results from a multicenter trial of clomipramine in obsessive-compulsive disorder. Psychopharmacol. Bull. 25, 36–40.
Devenport, L. D. (1978). Schedule-induced polydipsia in rats: adrenocortical and hippocampal modulation. J. Comp. Physiol. Psychol. 92, 651–660. doi: 10.1037/h0077499
Didriksen, M. (1995). The effect of risperidone on schedule-induced polydipsia. Behav. Pharmacol. 6, 290–292.
Didriksen, M., and Christensen, A. V. (1993). Differences in performance in three strains of rats in a 5-choice serial reaction time task. Pharmacol. Toxicol. 72, 66–68. doi: 10.1111/j.1600-0773.1993.tb01341.x
Didriksen, M., and Christensen, A. V. (1994). The effects of amphetamine, phencyclidine, dopaminergic antagonists and atypical neuroleptics on schedule-induced polydipsia (SIP) are distinguishable. Behav. Pharmacol. 5, 32–41. doi: 10.1097/00008877-199402000-00004
Didriksen, M., Olsen, G. M., and Christensen, A. V. (1993). Effect of clozapine upon schedule-induced polydipsia (SIP) resembles neither the actions of dopamine D1 nor D2 blockade. Psychopharmacology 113, 250–256. doi: 10.1007/bf02245706
Dong, H.-W., Petrovich, G. D., Watts, A. G., and Swanson, L. W. (2001). Basic organization of projections from the oval and fusiform nuclei of the bed nuclei of the stria terminalis in adult rat brain. J. Comp. Neurol. 436, 430–455. doi: 10.1002/cne.1079
Dong, H.-W., and Swanson, L. W. (2003). Projections from the rhomboid nucleus of the bed nuclei of the stria terminalis: implications for cerebral hemisphere regulation of ingestive behaviors. J. Comp. Neurol. 463, 434–472. doi: 10.1002/cne.10758
Dong, H.-W., and Swanson, L. W. (2004a). Organization of axonal projections from the anterolateral area of the bed nuclei of the stria terminalis. J. Comp. Neurol. 468, 277–298. doi: 10.1002/cne.10949
Dong, H.-W., and Swanson, L. W. (2004b). Projections from bed nuclei of the stria terminalis, posterior division: implications for cerebral hemisphere regulation of defensive and reproductive behaviors. J. Comp. Neurol. 471, 396–433. doi: 10.1002/cne.20002
Dong, H.-W., and Swanson, L. W. (2006a). Projections from bed nuclei of the stria terminalis, anteromedial area: cerebral hemisphere integration of neuroendocrine, autonomic, and behavioral aspects of energy balance. J. Comp. Neurol. 494, 142–178. doi: 10.1002/cne.20788
Dong, H.-W., and Swanson, L. W. (2006b). Projections from bed nuclei of the stria terminalis, dorsomedial nucleus: implications for cerebral hemisphere integration of neuroendocrine, autonomic, and drinking responses. J. Comp. Neurol. 494, 75–107. doi: 10.1002/cne.20790
Dong, H.-W., and Swanson, L. W. (2006c). Projections from bed nuclei of the stria terminalis, magnocellular nucleus: implications for cerebral hemisphere regulation of micturition, defecation, and penile erection. J. Comp. Neurol. 494, 108–141. doi: 10.1002/cne.20789
Ebdrup, B. H., Glenthøj, B., Rasmussen, H., Aggernaes, B., Langkilde, A. R., Paulson, O. B. et al. (2010). Hippocampal and caudate volume reductions in antipsychotic-naive first-episode schizophrenia. J. Psychiatry Neurosci. 35, 95–104. doi: 10.1503/jpn.090049
Egan, G., Silk, T., Zamarripa, F., Williams, J., Federico, P., Cunnington, R. et al. (2003). Neural correlates of the emergence of consciousness of thirst. Proc. Natl. Acad. Sci. U.S.A. 100, 15241–15246. doi: 10.1073/pnas.2136650100
Emsley, R., Roberts, M., Smith, R., Spangenberg, J., and Chalton, D. (1995). Disordered water homeostasis in schizophrenia and cerebral ventricular size. Br. J. Psychiatry J. Ment. Sci. 166, 501–506. doi: 10.1192/bjp.166.4.501
Escher, T., Call, S. B., Blaha, C. D., and Mittleman, G. (2006). Behavioral effects of aminoadamantane class NMDA receptor antagonists on schedule-induced alcohol and self-administration of water in mice. Psychopharmacology 187, 424–434. doi: 10.1007/s00213-006-0465-5
Eslinger, P. J., and Damasio, A. R. (1985). Severe disturbance of higher cognition after bilateral frontal lobe ablation: patient EVR. Neurology 35, 1731–1741.
Ettenberg, A., and Camp, C. H. (1986). A partial reinforcement extinction effect in water-reinforced rats intermittently treated with haloperidol. Pharmacol. Biochem. Behav. 25, 1231–1235. doi: 10.1016/0091-3057(86)90117-6
Everitt, B. J., and Robbins, T. W. (2005). Neural systems of reinforcement for drug addiction: from actions to habits to compulsion. Nat. Neurosci. 8, 1481–1489. doi: 10.1038/nn1579
Fabricius, K., Helboe, L., Fink-Jensen, A., Wörtwein, G., Steiniger-Brach, B., and Sotty, F. (2010). Increased dopaminergic activity in socially isolated rats: an electrophysiological study. Neurosci. Lett. 482, 117–122. doi: 10.1016/j.neulet.2010.07.014
Fabricius, K., Steiniger-Brach, B., Helboe, L., Fink-Jensen, A., and Wörtwein, G. (2011). Socially isolated rats exhibit changes in dopamine homeostasis pertinent to schizophrenia. Int. J. Dev. Neurosci. 29, 347–350. doi: 10.1016/j.ijdevneu.2010.09.003
Falk, J. L. (1961). Production of polydipsia in normal rats by an intermittent food schedule. Science 133, 195–196. doi: 10.1126/science.133.3447.195
Falk, J. L. (1966). Schedule-induced polydipsia as a function of fixed interval length. J. Exp. Anal. Behav. 9, 37–39. doi: 10.1901/jeab.1966.9-37
Falk, J. L. (1967). Control of schedule-induced polydipsia: type, size, and spacing of meals. J. Exp. Anal. Behav. 10, 199–206. doi: 10.1901/jeab.1967.10-199
Falk, J. L. (1969). Conditions producing psychogenic polydipsia in animals. Ann. N. Y. Acad. Sci. 157, 569–593. doi: 10.1111/j.1749-6632.1969.tb12908.x
Falk, J. L. (1971). The nature and determinants of adjunctive behavior. Physiol. Behav. 6, 577–588. doi: 10.1016/0031-9384(71)90209-5
Flores, P., and Pellón, R. (1995). Rate-dependency hypothesis and the rate-decreasing effects of d-amphetamine on schedule-induced drinking. Behav. Pharmacol. 6, 16–23.
Flores, P., and Pellón, R. (1997). Effects of d-amphetamine on temporal distributions of schedule-induced polydipsia. Pharmacol. Biochem. Behav. 57, 81–87. doi: 10.1016/s0091-3057(96)00131-1
Floresco, S. B., West, A. R., Ash, B., Moore, H., and Grace, A. A. (2003). Afferent modulation of dopamine neuron firing differentially regulates tonic and phasic dopamine transmission. Nat. Neurosci. 6, 968–973. doi: 10.1038/nn1103
Flory, R. K. (1971). The control of schedule-induced polydipsia: frequency and magnitude of reinforcement. Learn. Motiv. 2, 215–227. doi: 10.1016/0023-9690(71)90022-1
Fornito, A., Harrison, B. J., Goodby, E., Dean, A., Ooi, C., Nathan, P. J. et al. (2013). Functional dysconnectivity of corticostriatal circuitry as a risk phenotype for psychosis. JAMA Psychiatry 70, 1143–1151. doi: 10.1001/jamapsychiatry.2013.1976
Fouche, J.-P., du Plessis, S., Hattingh, C., Roos, A., Lochner, C., Soriano-Mas, C. et al. (2017). Cortical thickness in obsessive-compulsive disorder: multisite mega-analysis of 780 brain scans from six centres. Br. J. Psychiatry J. Ment. Sci. 210, 67–74. doi: 10.1192/bjp.bp.115.164020
Fox, A. S., Shelton, S. E., Oakes, T. R., Converse, A. K., Davidson, R. J., and Kalin, N. H. (2010). Orbitofrontal cortex lesions alter anxiety-related activity in the primate bed nucleus of stria terminalis. J. Neurosci. 30, 7023–7027. doi: 10.1523/JNEUROSCI.5952-09.2010
Frye, P. E., and Arnold, L. E. (1981). Persistent amphetamine-induced compulsive rituals: response to pyridoxine(B6). Biol. Psychiatry 16, 583–587.
Galantowicz, E. P., and King, G. D. (1975). The effects of three levels of lick-contingent footshock on schedule-induced polydipsia. Bull. Psychon. Soc. 5, 113–116. doi: 10.3758/BF03333217
Gardner Gregory, J. (2018). Estrogens in the Bed Nucleus of the Stria terminalis: State-Dependent Modulation of Inhibitory Synaptic Transmission in Rats. Ph.D. thesis, Queen’s University, Kingston.
Gehring, W. J., and Taylor, S. F. (2004). When the going gets tough, the cingulate gets going. Nat. Neurosci. 7, 1285–1287. doi: 10.1038/nn1204-1285
Geyer, M., and Markou, A. (1994). “Animal models of psychiatric disorders,” in Psychopharmacology, eds F. Bloom and D. Kupfer (New York, NY: Raven Press), 787–798.
Gillan, C. M., Morein-Zamir, S., Urcelay, G. P., Sule, A., Voon, V., Apergis-Schoute, A. M. et al. (2014). Enhanced avoidance habits in obsessive-compulsive disorder. Biol. Psychiatry 75, 631–638. doi: 10.1016/j.biopsych.2013.02.002
Gillan, C. M., Papmeyer, M., Morein-Zamir, S., Sahakian, B. J., Fineberg, N. A., Robbins, T. W. et al. (2011). Disruption in the balance between goal-directed behavior and habit learning in obsessive-compulsive disorder. Am. J. Psychiatry 168, 718–726. doi: 10.1176/appi.ajp.2011.10071062
Gillan, C. M., and Robbins, T. W. (2014). Goal-directed learning and obsessive-compulsive disorder. Philos. Trans. R. Soc. Lond. B. Biol. Sci. 369:20130475. doi: 10.1098/rstb.2013.0475
Gillan, C. M., Robbins, T. W., Sahakian, B. J., van den Heuvel, O. A., and van Wingen, G. (2016). The role of habit in compulsivity. Eur. Neuropsychopharmacol. 26, 828–840. doi: 10.1016/j.euroneuro.2015.12.033
Goldman, M. B., Robertson, G. L., Luchins, D. J., and Hedeker, D. (1996). The influence of polydipsia on water excretion in hyponatremic, polydipsic, schizophrenic patients. J. Clin. Endocrinol. Metab. 81, 1465–1470. doi: 10.1210/jcem.81.4.8636352
Goldman, M. B., Wang, L., Wachi, C., Daudi, S., Csernansky, J., Marlow-O’Connor, M. et al. (2011). Structural pathology underlying neuroendocrine dysfunction in schizophrenia. Behav. Brain Res. 218, 106–113. doi: 10.1016/j.bbr.2010.11.025
Goldman, M. B., Wood, G., Goldman, M. B., Gavin, M., Paul, S., Zaheer, S. et al. (2007). Diminished glucocorticoid negative feedback in polydipsic hyponatremic schizophrenic patients. J. Clin. Endocrinol. Metab. 92, 698–704. doi: 10.1210/jc.2006-1131
Goldstein, J. M., Goodman, J. M., Seidman, L. J., Kennedy, D. N., Makris, N., Lee, H. et al. (1999). Cortical abnormalities in schizophrenia identified by structural magnetic resonance imaging. Arch. Gen. Psychiatry 56, 537–547. doi: 10.1001/archpsyc.56.6.537
Graybiel, A. M., and Rauch, S. L. (2000). Toward a neurobiology of obsessive-compulsive disorder. Neuron 28, 343–347. doi: 10.1016/s0896-6273(00)00113-6
Gregory, J. G., Hawken, E. R., Banasikowski, T. J., Dumont, E. C., and Beninger, R. J. (2015). A response strategy predicts acquisition of schedule-induced polydipsia in rats. Prog. Neuropsychopharmacol. Biol. Psychiatry 61, 37–43. doi: 10.1016/j.pnpbp.2015.03.012
Gregory, J. G., Hawken, E. R., Angelis, S., Bouchard, J.-F., and Dumont, É. C. (2019). Estradiol potentiates inhibitory synaptic transmission in the oval bed nucleus of the striaterminalis of male and female rats. Psychoneuroendocrinology 106, 102–110. doi: 10.1016/j.psyneuen.2019.03.030
Gremel, C. M., Chancey, J., Atwood, B., Luo, G., Neve, R., Ramakrishnan, C. et al. (2016). Endocannabinoid modulation of orbitostriatal circuits gates habit formation. Neuron 90, 1312–1324. doi: 10.1016/j.neuron.2016.04.043
Gremel, C. M., and Costa, R. M. (2013). Orbitofrontal and striatal circuits dynamically encode the shift between goal-directed and habitual actions. Nat. Commun. 4:2264. doi: 10.1038/ncomms3264
Grover, S., Dua, D., Chakrabarti, S., and Avasthi, A. (2017). Obsessive compulsive symptoms/disorder in patients with schizophrenia: prevalence, relationship with other symptom dimensions and impact on functioning. Psychiatry Res. 250, 277–284. doi: 10.1016/j.psychres.2017.01.067
Gur, R. E., Cowell, P. E., Latshaw, A., Turetsky, B. I., Grossman, R. I., Arnold, S. E. et al. (2000). Reduced dorsal and orbital prefrontal gray matter volumes in schizophrenia. Arch. Gen. Psychiatry 57, 761–768.
Hall, F. S., Wilkinson, L. S., Humby, T., Inglis, W., Kendall, D. A., Marsden, C. A. et al. (1998). Isolation rearing in rats: pre- and postsynaptic changes in striatal dopaminergic systems. Pharmacol. Biochem. Behav. 59, 859–872. doi: 10.1016/s0091-3057(97)00510-8
Han, X., Wang, W., Shao, F., and Li, N. (2011). Isolation rearing alters social behaviors and monoamine neurotransmission in the medial prefrontal cortex and nucleus accumbens of adult rats. Brain Res. 1385, 175–181. doi: 10.1016/j.brainres.2011.02.035
Hardung, S., Epple, R., Jäckel, Z., Eriksson, D., Uran, C., Senn, V. et al. (2017). A functional gradient in the rodent prefrontal cortex supports behavioral inhibition. Curr. Biol. 27, 549–555. doi: 10.1016/j.cub.2016.12.052
Hariprasad, M. K., Eisinger, R. P., Nadler, I. M., Padmanabhan, C. S., and Nidus, B. D. (1980). Hyponatremia in psychogenic polydipsia. Arch. Intern. Med. 140, 1639–1642. doi: 10.1001/archinte.140.12.1639
Harrison, B. J., Pujol, J., Cardoner, N., Deus, J., Alonso, P., López-Solà, M. et al. (2013). Brain corticostriatal systems and the major clinical symptom dimensions of obsessive-compulsive disorder. Biol. Psychiatry 73, 321–328. doi: 10.1016/j.biopsych.2012.10.006
Harrison, B. J., Soriano-Mas, C., Pujol, J., Ortiz, H., López-Solà, M., Hernández-Ribas, R. et al. (2009). Altered corticostriatal functional connectivity in obsessive-compulsive disorder. Arch. Gen. Psychiatry 66, 1189–1200. doi: 10.1001/archgenpsychiatry.2009.152
Hasue, R. H., and Shammah-Lagnado, S. J. (2002). Origin of the dopaminergic innervation of the central extended amygdala and accumbens shell: a combined retrograde tracing and immunohistochemical study in the rat. J. Comp. Neurol. 454, 15–33. doi: 10.1002/cne.10420
Hawken, E. R., and Beninger, R. J. (2014). The amphetamine sensitization model of schizophrenia symptoms and its effect on schedule-induced polydipsia in the rat. Psychopharmacology 231, 2001–2008. doi: 10.1007/s00213-013-3345-9
Hawken, E. R., Crookall, J. M., Reddick, D., Millson, R. C., Milev, R., and Delva, N. (2009). Mortality over a 20-year period in patients with primary polydipsia associated with schizophrenia: a retrospective study. Schizophr. Res. 107, 128–133. doi: 10.1016/j.schres.2008.09.029
Hawken, E. R., Delva, N. J., and Beninger, R. J. (2013a). Increased drinking following social isolation rearing: implications for polydipsia associated with schizophrenia. PLoS One 8:e56105. doi: 10.1371/journal.pone.0056105
Hawken, E. R., Lister, J., Winterborn, A. N., and Beninger, R. J. (2013b). Spontaneous polydipsia in animals treated subchronically with MK-801. Schizophr. Res. 143, 228–230. doi: 10.1016/j.schres.2012.11.013
Hawken, E. R., Delva, N. J., Reynolds, J. N., and Beninger, R. J. (2011). Increased schedule-induced polydipsia in the rat following subchronic treatment with MK-801. Schizophr. Res. 125, 93–98. doi: 10.1016/j.schres.2010.07.022
Hawken, E. R., Normandeau, C. P., Gregory, J. G., Cécyre, B., Bouchard, J.-F., Mackie, K. et al. (2019). A novel GPR55-mediated satiety signal in the oval bed nucleus of the stria terminalis. Neuropsychopharmacology 44, 1274–1283. doi: 10.1038/s41386-018-0309-0
Heidbreder, C. A., Weiss, I. C., Domeney, A. M., Pryce, C., Homberg, J., Hedou, G. et al. (2000). Behavioral, neurochemical and endocrinological characterization of the early social isolation syndrome. Neuroscience 100, 749–768. doi: 10.1016/s0306-4522(00)00336-5
Hesse, S., Müller, U., Lincke, T., Barthel, H., Villmann, T., Angermeyer, M. C. et al. (2005). Serotonin and dopamine transporter imaging in patients with obsessive-compulsive disorder. Psychiatry Res. 140, 63–72. doi: 10.1016/j.pscychresns.2005.07.002
Hogg, S., and Dalvi, A. (2004). Acceleration of onset of action in schedule-induced polydipsia: combinations of SSRI and 5-HT1A and 5-HT1B receptor antagonists. Pharmacol. Biochem. Behav. 77, 69–75. doi: 10.1016/j.pbb.2003.09.020
Hooks, M. S., Jones, G. H., Juncos, J. L., Neill, D. B., and Justice, J. B. (1994). Individual differences in schedule-induced and conditioned behaviors. Behav. Brain Res. 60, 199–209. doi: 10.1016/0166-4328(94)90148-1
Huang, H., Shu, C., Chen, J., Zou, J., Chen, C., Wu, S. et al. (2018). Altered corticostriatal pathway in first-episode paranoid schizophrenia: resting-state functional and causal connectivity analyses. Psychiatry Res. Neuroimaging 272, 38–45. doi: 10.1016/j.pscychresns.2017.08.003
Hwang, M. Y., and Opler, L. A. (1994). Schizophrenia with obsessive-compulsive features: assessment and treatment. Psychiatr. Ann. 24, 468–472. doi: 10.3928/0048-5713-19940901-08
Íbias, J., Soria-Molinillo, E., Kastanauskaite, A., Orgaz, C., DeFelipe, J., Pellón, R. et al. (2015). Schedule-induced polydipsia is associated with increased spine density in dorsolateral striatum neurons. Neuroscience 300, 238–245. doi: 10.1016/j.neuroscience.2015.05.026
Illowsky, B. P., and Kirch, D. G. (1988). Polydipsia and hyponatremia in psychiatric patients. Am. J. Psychiatry 145, 675–683. doi: 10.1176/ajp.145.6.675
Jacobs, B. L., and Farel, P. B. (1971). Motivated behaviors produced by increased arousal in the presence of goal objects. Physiol. Behav. 6, 473–476. doi: 10.1016/0031-9384(71)90191-0
Jennings, J. H., Sparta, D. R., Stamatakis, A. M., Ung, R. L., Pleil, K. E., Kash, T. L. et al. (2013). Distinct extended amygdala circuits for divergent motivational states. Nature 496, 224–228. doi: 10.1038/nature12041
Jentsch, J. D., Taylor, J. R., and Roth, R. H. (1998). Subchronic phencyclidine administration increases mesolimbic dopaminergic system responsivity and augments stress- and psychostimulant-induced hyperlocomotion. Neuropsychopharmacology 19, 105–113. doi: 10.1016/S0893-133X(98)00004-9
Johansen, J. P., Fields, H. L., and Manning, B. H. (2001). The affective component of pain in rodents: direct evidence for a contribution of the anterior cingulate cortex. Proc. Natl. Acad. Sci. U.S.A. 98, 8077–8082. doi: 10.1073/pnas.141218998
Jones, G. H., Hooks, M. S., Juncos, J. L., and Justice, J. B. (1994). Effects of cocaine microinjections into the nucleus accumbens and medial prefrontal cortex on schedule-induced behaviour: comparison with systemic cocaine administration. Psychopharmacology 115, 375–382. doi: 10.1007/bf02245080
Jones, G. H., Marsden, C. A., and Robbins, T. W. (1990). Increased sensitivity to amphetamine and reward-related stimuli following social isolation in rats: possible disruption of dopamine-dependent mechanisms of the nucleus accumbens. Psychopharmacology 102, 364–372. doi: 10.1007/bf02244105
Jung, W. H., Kang, D.-H., Kim, E., Shin, K. S., Jang, J. H., and Kwon, J. S. (2013). Abnormal corticostriatal-limbic functional connectivity in obsessive-compulsive disorder during reward processing and resting-state. Neuroimage Clin. 3, 27–38. doi: 10.1016/j.nicl.2013.06.013
Kachanoff, R., Leveille, R., McLelland, J. P., and Wayner, M. J. (1973). Schedule induced behavior in humans. Physiol. Behav. 11, 395–398. doi: 10.1016/0031-9384(73)90018-8
Kawasaki, Y., Suzuki, M., Nohara, S., Hagino, H., Takahashi, T., Matsui, M. et al. (2004). Structural brain differences in patients with schizophrenia and schizotypal disorder demonstrated by voxel-based morphometry. Eur. Arch. Psychiatry Clin. Neurosci. 254, 406–414. doi: 10.1007/s00406-004-0522-1
Keehn, J. D., Coulson, G. E., and Klieb, J. (1976). Effects of haloperidol on schedule-induced polydipsia. J. Exp. Anal. Behav. 25, 105–112. doi: 10.1901/jeab.1976.25-105
Keehn, J. D., and Riusech, R. (1977). Schedule-induced water and saccharin polydipsia under haloperidol. Bull. Psychon. Soc. 9, 413–415. doi: 10.3758/BF03337041
Killcross, S., and Coutureau, E. (2003). Coordination of actions and habits in the medial prefrontal cortex of rats. Cereb. Cortex 1991, 400–408. doi: 10.1093/cercor/13.4.400
Killeen, P. R., and Pellón, R. (2013). Adjunctive behaviors are operants. Learn. Behav. 41, 1–24. doi: 10.3758/s13420-012-0095-1
Kim, S.-Y., Adhikari, A., Lee, S. Y., Marshel, J. H., Kim, C. K., Mallory, C. S. et al. (2013). Diverging neural pathways assemble a behavioural state from separable features in anxiety. Nature 496, 219–223. doi: 10.1038/nature12018
Kirch, D. G., Bigelow, L. B., Weinberger, D. R., Lawson, W. B., and Wyatt, R. J. (1985). Polydipsia and chronic hyponatremia in schizophrenic inpatients. J. Clin. Psychiatry 46, 179–181.
Konorski, J. (1967). Integrative Activity of the Brain. Second Printing edition. Chicago, IL: University of Chicago Press.
Koo, M.-S., Kim, E.-J., Roh, D., and Kim, C.-H. (2010). Role of dopamine in the pathophysiology and treatment of obsessive-compulsive disorder. Expert Rev. Neurother. 10, 275–290. doi: 10.1586/ern.09.148
Koob, G. F., and Volkow, N. D. (2010). Neurocircuitry of addiction. Neuropsychopharmacology 35, 217–238. doi: 10.1038/npp.2009.110
Koob, G. F., and Volkow, N. D. (2016). Neurobiology of addiction: a neurocircuitry analysis. Lancet Psychiatry 3, 760–773. doi: 10.1016/S2215-0366(16)00104-8
Kopell, B. H., and Greenberg, B. D. (2008). Anatomy and physiology of the basal ganglia: implications for DBS in psychiatry. Neurosci. Biobehav. Rev. 32, 408–422. doi: 10.1016/j.neubiorev.2007.07.004
Kotsopoulos, S., and Spivak, M. (2001). Obsessive-compulsive symptoms secondary to methylphenidate treatment. Can. J. Psychiatry Rev. Can. Psychiatr. 46:89.
Krawczyk, M., Mason, X., DeBacker, J., Sharma, R., Normandeau, C. P., Hawken, E. R. et al. (2013). D1 dopamine receptor-mediated LTP at GABA synapses encodes motivation to self-administer cocaine in rats. J. Neurosci. 33, 11960–11971. doi: 10.1523/JNEUROSCI.1784-13.2013
Kuribara, H., and Tadokoro, S. (1980). Effects of psychotropic drugs on FI responding and adjunctive drinking in rats. Pharmacol. Biochem. Behav. 13, 657–662. doi: 10.1016/0091-3057(80)90009-x
Laruelle, M., and Abi-Dargham, A. (1999). Dopamine as the wind of the psychotic fire: new evidence from brain imaging studies. J. Psychopharmacol. 13, 358–371. doi: 10.1177/026988119901300405
Laruelle, M., Abi-Dargham, A., Gil, R., Kegeles, L., and Innis, R. (1999). Increased dopamine transmission in schizophrenia: relationship to illness phases. Biol. Psychiatry 46, 56–72. doi: 10.1016/s0006-3223(99)00067-0
Laruelle, M., Abi-Dargham, A., van Dyck, C. H., Gil, R., D’Souza, C. D., Erdos, J. et al. (1996). Single photon emission computerized tomography imaging of amphetamine-induced dopamine release in drug-free schizophrenic subjects. Proc. Natl. Acad. Sci. U.S.A. 93, 9235–9240. doi: 10.1073/pnas.93.17.9235
Lashley, R. L., and Rosellini, R. A. (1980). Modulation of schedule-induced polydipsia by Pavlovian conditioned states. Physiol. Behav. 24, 411–414. doi: 10.1016/0031-9384(80)90108-0
Leadbetter, R. A., Shutty, M. S., Elkashef, A. M., Kirch, D. G., Spraggins, T., Cail, W. S. et al. (1999). MRI changes during water loading in patients with polydipsia and intermittent hyponatremia. Am. J. Psychiatry 156, 958–960. doi: 10.1176/ajp.156.6.958
Lebow, M. A., and Chen, A. (2016). Overshadowed by the amygdala: the bed nucleus of the stria terminalis emerges as key to psychiatric disorders. Mol. Psychiatry 21, 450–463. doi: 10.1038/mp.2016.1
Levine, R., and Levine, S. (1989). Role of the pituitary-adrenal hormones in the acquisition of schedule-induced polydipsia. Behav. Neurosci. 103, 621–637. doi: 10.1037//0735-7044.103.3.621
Lewis, D. A., and Anderson, S. A. (1995). The functional architecture of the prefrontal cortex and schizophrenia. Psychol. Med. 25, 887–894. doi: 10.1017/s0033291700037375
Lewis, D. A., and Lieberman, J. A. (2000). Catching up on schizophrenia: natural history and neurobiology. Neuron 28, 325–334. doi: 10.1016/s0896-6273(00)00111-2
Li, S., and Kirouac, G. J. (2008). Projections from the paraventricular nucleus of the thalamus to the forebrain, with special emphasis on the extended amygdala. J. Comp. Neurol. 506, 263–287. doi: 10.1002/cne.21502
Lieberman, J. A. (1999). Is schizophrenia a neurodegenerative disorder? A clinical and neurobiological perspective. Biol. Psychiatry 46, 729–739. doi: 10.1016/s0006-3223(99)00147-x
Lobarinas, E., and Falk, J. L. (1998). Schedule-induced polydipsic consumption of hypertonic NaCl solutions: effects of chlordiazepoxide. Physiol. Behav. 63, 419–423. doi: 10.1016/s0031-9384(97)00461-7
Lodge, D. J., and Grace, A. A. (2006). The hippocampus modulates dopamine neuron responsivity by regulating the intensity of phasic neuron activation. Neuropsychopharmacology 31, 1356–1361. doi: 10.1038/sj.npp.1300963
Lodge, D. J., and Grace, A. A. (2008). Amphetamine activation of hippocampal drive of mesolimbic dopamine neurons: a mechanism of behavioral sensitization. J. Neurosci. 28, 7876–7882. doi: 10.1523/JNEUROSCI.1582-08.2008
Lodge, D. J., and Grace, A. A. (2012). Divergent activation of ventromedial and ventrolateral dopamine systems in animal models of amphetamine sensitization and schizophrenia. Int. J. Neuropsychopharmacol. 15, 69–76. doi: 10.1017/S1461145711000113
López-Grancha, M., Lopez-Crespo, G., Sanchez-Amate, M. C., and Flores, P. (2008). Individual differences in schedule-induced polydipsia and the role of gabaergic and dopaminergic systems. Psychopharmacology 197, 487–498. doi: 10.1007/s00213-007-1059-6
Lu, C. C., Tseng, C. J., Wan, F. J., Yin, T. H., and Tung, C. S. (1992). Role of locus coeruleus and serotonergic drug actions on schedule-induced polydipsia. Pharmacol. Biochem. Behav. 43, 255–261. doi: 10.1016/0091-3057(92)90666-4
Luchins, D. J. (1990). A possible role of hippocampal dysfunction in schizophrenic symptomatology. Biol. Psychiatry 28, 87–91. doi: 10.1016/0006-3223(90)90625-c
Luchins, D. J., Goldman, M. B., Lieb, M., and Hanrahan, P. (1992). Repetitive behaviors in chronically institutionalized schizophrenic patients. Schizophr. Res. 8, 119–123. doi: 10.1016/0920-9964(92)90027-3
Luchins, D. J., Nettles, K. W., and Goldman, M. B. (1997). Anterior medial temporal lobe volumes in polydipsic schizophrenic patients with and without hypo-osmolemia: a pilot study. Biol. Psychiatry 42, 767–770. doi: 10.1016/s0006-3223(96)00491-x
Luxenberg, J. S., Swedo, S. E., Flament, M. F., Friedland, R. P., Rapoport, J., and Rapoport, S. I. (1988). Neuroanatomical abnormalities in obsessive-compulsive disorder detected with quantitative X-ray computed tomography. Am. J. Psychiatry 145, 1089–1093. doi: 10.1176/ajp.145.9.1089
Lysaker, P. H., Marks, K. A., Picone, J. B., Rollins, A. L., Fastenau, P. S., and Bond, G. R. (2000). Obsessive and compulsive symptoms in schizophrenia: clinical and neurocognitive correlates. J. Nerv. Ment. Dis. 188, 78–83.
Maracle, A. C., Normandeau, C. P., Dumont, É. C., and Olmstead, M. C. (2019). Dopamine in the oval bed nucleus of the stria terminalis contributes to compulsive responding for sucrose in rats. Neuropsychopharmacology 44, 381–389. doi: 10.1038/s41386-018-0149-y
Martin, J. R., Ballard, T. M., and Higgins, G. A. (2002). Influence of the 5-HT2C receptor antagonist, SB-242084, in tests of anxiety. Pharmacol. Biochem. Behav. 71, 615–625. doi: 10.1016/s0091-3057(01)00713-4
McDonald, A. J., Shammah-Lagnado, S. J., Shi, C., and Davis, M. (1999). Cortical afferents to the extended amygdala. Ann. N. Y. Acad. Sci. 877, 309–338. doi: 10.1111/j.1749-6632.1999.tb09275.x
McDougle, C. J., Goodman, W. K., Leckman, J. F., Lee, N. C., Heninger, G. R., and Price, L. H. (1994). Haloperidol addition in fluvoxamine-refractory obsessive-compulsive disorder. A double-blind, placebo-controlled study in patients with and without tics. Arch. Gen. Psychiatry 51, 302–308.
McKinley, M. (2009). “Thirst,” in Handbook of Neuroscience for the Behavioral Sciences, eds J. T. Cacioppo and G. G. Berntson (Chichester: John Wiley & Sons, Inc.), 680–709.
McKinley, M. J., Denton, D. A., Ryan, P. J., Yao, S. T., Stefanidis, A., and Oldfield, B. J. (2019). From sensory circumventricular organs to cerebral cortex: neural pathways controlling thirst and hunger. J. Neuroendocrinol. 31:e12689. doi: 10.1111/jne.12689
McMillan, D. E. (1979). Effects of d-amphetamine and caffeine on schedule-controlled and schedule-induced responding. J. Exp. Anal. Behav. 32, 445–456. doi: 10.1901/jeab.1979.32-445
Merchán, A., Mora, S., Gago, B., Rodriguez-Ortega, E., Fernández-Teruel, A., Puga, J. L. et al. (2018). Excessive habit formation in schedule-induced polydipsia: microstructural analysis of licking among rat strains and involvement of the orbitofrontal cortex. Genes Brain Behav. 18:e12489. doi: 10.1111/gbb.12489
Merchán, A., Navarro, S. V., Klein, A. B., Aznar, S., Campa, L., Suñol, C. et al. (2017). Tryptophan depletion affects compulsive behaviour in rats: strain dependent effects and associated neuromechanisms. Psychopharmacology 234, 1223–1236. doi: 10.1007/s00213-017-4561-5
Mercier-Guidez, E., and Loas, G. (2000). Polydipsia and water intoxication in 353 psychiatric inpatients: an epidemiological and psychopathological study. Eur. Psychiatry J. Assoc. Eur. Psychiatr. 15, 306–311. doi: 10.1016/s0924-9338(00)00399-0
Millson, R. C., Koczapski, A. B., Cook, M. I., and Daszkiewicz, M. (1992). A survey of patient attitudes toward self-induced water intoxication. Can. J. Psychiatry Rev. Can. Psychiatr. 37, 46–47. doi: 10.1177/070674379203700110
Mittleman, G., Blaha, C. D., and Phillips, A. G. (1992). Pituitary-adrenal and dopaminergic modulation of schedule-induced polydipsia: behavioral and neurochemical evidence. Behav. Neurosci. 106, 408–420. doi: 10.1037/0735-7044.106.2.408
Mittleman, G., Brener, J., and Robbins, T. W. (1990). Physiological correlates of schedule-induced activities in rats. Am. J. Physiol. 259, R485–R491. doi: 10.1152/ajpregu.1990.259.3.R485
Mittleman, G., Jones, G. H., and Robbins, T. W. (1988a). Effects of diazepam, FG 7142, and RO 15-1788 on schedule-induced polydipsia and the temporal control of behavior. Psychopharmacology 94, 103–109. doi: 10.1007/bf00735889
Mittleman, G., Jones, G. H., and Robbins, T. W. (1988b). The relationship between schedule-induced polydipsia and pituitary-adrenal activity: pharmacological and behavioral manipulations. Behav. Brain Res. 28, 315–324. doi: 10.1016/0166-4328(88)90134-9
Mittleman, G., LeDuc, P. A., and Whishaw, I. Q. (1993). The role of D1 and D2 receptors in the heightened locomotion induced by direct and indirect dopamine agonists in rats with hippocampal damage: an animal analogue of schizophrenia. Behav. Brain Res. 55, 253–267. doi: 10.1016/0166-4328(93)90121-6
Mittleman, G., Rosner, A. L., and Schaub, C. L. (1994). Polydipsia and dopamine: behavioral effects of dopamine D1 and D2 receptor agonists and antagonists. J. Pharmacol. Exp. Ther. 271, 638–650.
Mittleman, G., and Valenstein, E. S. (1985). Individual differences in non-regulatory ingestive behavior and catecholamine systems. Brain Res. 348, 112–117. doi: 10.1016/0006-8993(85)90366-x
Miura, H., Qiao, H., and Ohta, T. (2002). Influence of aging and social isolation on changes in brain monoamine turnover and biosynthesis of rats elicited by novelty stress. Synapse 46, 116–124. doi: 10.1002/syn.10133
Modell, J. G., Mountz, J. M., Curtis, G. C., and Greden, J. F. (1989). Neurophysiologic dysfunction in basal ganglia/limbic striatal and thalamocortical circuits as a pathogenetic mechanism of obsessive-compulsive disorder. J. Neuropsychiatry Clin. Neurosci. 1, 27–36. doi: 10.1176/jnp.1.1.27
Mora, S., Merchán, A., Vilchez, O., Aznar, S., Klein, A. B., Ultved, L. et al. (2018). Reduced cortical serotonin 5-HT2A receptor binding and glutamate activity in high compulsive drinker rats. Neuropharmacology 143, 10–19. doi: 10.1016/j.neuropharm.2018.09.004
Moreno, M., and Flores, P. (2012). Schedule-induced polydipsia as a model of compulsive behavior: neuropharmacological and neuroendocrine bases. Psychopharmacology 219, 647–659. doi: 10.1007/s00213-011-2570-3
Moreno, M., Gutiérrez-Ferre, V. E., Ruedas, L., Campa, L., Suñol, C., and Flores, P. (2012). Poor inhibitory control and neurochemical differences in high compulsive drinker rats selected by schedule-induced polydipsia. Psychopharmacology 219, 661–672. doi: 10.1007/s00213-011-2575-y
Morrens, M., Hulstijn, W., Lewi, P. J., De Hert, M., and Sabbe, B. G. C. (2006). Stereotypy in schizophrenia. Schizophr. Res. 84, 397–404. doi: 10.1016/j.schres.2006.01.024
Motzkin, J. C., Philippi, C. L., Oler, J. A., Kalin, N. H., Baskaya, M. K., and Koenigs, M. (2015). Ventromedial prefrontal cortex damage alters resting blood flow to the bed nucleus of stria terminalis. Cortex 64, 281–288. doi: 10.1016/j.cortex.2014.11.013
Nagashima, T., Inoue, M., Kitamura, S., Kiuchi, K., Kosaka, J., Okada, K. et al. (2012). Brain structural changes and neuropsychological impairments in male polydipsic schizophrenia. BMC Psychiatry 12:210. doi: 10.1186/1471-244X-12-210
Nakamura, M., Nestor, P. G., Levitt, J. J., Cohen, A. S., Kawashima, T., Shenton, M. E. et al. (2008). Orbitofrontal volume deficit in schizophrenia and thought disorder. Brain J. Neurol. 131, 180–195. doi: 10.1093/brain/awm265
Navarro, S. V., Gutiérrez-Ferre, V., Flores, P., and Moreno, M. (2015). Activation of serotonin 5-HT2A receptors inhibits high compulsive drinking on schedule-induced polydipsia. Psychopharmacology 232, 683–697. doi: 10.1007/s00213-014-3699-7
Nechmad, A., Ratzoni, G., Poyurovsky, M., Meged, S., Avidan, G., Fuchs, C. et al. (2003). Obsessive-compulsive disorder in adolescent schizophrenia patients. Am. J. Psychiatry 160, 1002–1004. doi: 10.1176/appi.ajp.160.5.1002
Nelson, A., and Killcross, S. (2006). Amphetamine exposure enhances habit formation. J. Neurosci. 26, 3805–3812. doi: 10.1523/JNEUROSCI.4305-05.2006
Nuttin, B., Cosyns, P., Demeulemeester, H., Gybels, J., and Meyerson, B. (1999). Electrical stimulation in anterior limbs of internal capsules in patients with obsessive-compulsive disorder. Lancet Lond. Engl. 354:1526. doi: 10.1016/S0140-6736(99)02376-4
Nuttin, B., Gielen, F., van Kuyck, K., Wu, H., Luyten, L., Welkenhuysen, M. et al. (2013). Targeting bed nucleus of the stria terminalis for severe obsessive-compulsive disorder: more unexpected lead placement in obsessive-compulsive disorder than in surgery for movement disorders. World Neurosurg. 80, S30.e11–S30.e16. doi: 10.1016/j.wneu.2012.12.029
Okasha, A., Rafaat, M., Mahallawy, N., El Nahas, G., El Dawla, A. S., Sayed, M., et al. (2000). Cognitive dysfunction in obsessive-compulsive disorder. Acta Psychiatr. Scand. 101, 281–285.
Ott, T., and Nieder, A. (2019). Dopamine and cognitive control in prefrontal cortex. Trends Cogn. Sci. 23, 213–234. doi: 10.1016/j.tics.2018.12.006
Packard, M. G., McGaugh, J. L. (1996). Inactivation of hippocampus or caudate nucleus with lidocaine differentially affects expression of place and response learning. Neurobiol. Learn. Mem. 65, 65–72. doi: 10.1006/nlme.1996.0007
Pantelis, C., Velakoulis, D., McGorry, P. D., Wood, S. J., Suckling, J., Phillips, L. J. et al. (2003). Neuroanatomical abnormalities before and after onset of psychosis: a cross-sectional and longitudinal MRI comparison. Lancet Lond. Engl. 361, 281–288. doi: 10.1016/S0140-6736(03)12323-9
Pauls, D. L., Abramovitch, A., Rauch, S. L., and Geller, D. A. (2014). Obsessive-compulsive disorder: an integrative genetic and neurobiological perspective. Nat. Rev. Neurosci. 15, 410–424. doi: 10.1038/nrn3746
Pellon, R., and Blackman, D. E. (1992). Effects of drugs on the temporal distribution of schedule-induced polydipsia in rats. Pharmacol. Biochem. Behav. 43, 689–695. doi: 10.1016/0091-3057(92)90397-x
Pellón, R., Ruíz, A., Moreno, M., Claro, F., Ambrosio, E., and Flores, P. (2011). Individual differences in schedule-induced polydipsia: neuroanatomical dopamine divergences. Behav. Brain Res. 217, 195–201. doi: 10.1016/j.bbr.2010.10.010
Poirier, S., Legris, G., Tremblay, P., Michea, R., Viau-Guay, L., Mérette, C. et al. (2010). Schizophrenia patients with polydipsia and water intoxication are characterized by greater severity of psychotic illness and a more frequent history of alcohol abuse. Schizophr. Res. 118, 285–291. doi: 10.1016/j.schres.2009.12.036
Porter, J. H., Goldsmith, P. A., McDonough, J. J., Heath, G. F., and Johnson, D. N. (1984). Differential effects of dopamine blockers on the acquisition of schedule-induced drinking and deprivation-induced drinking. Physiol. Psychol. 12, 302–306. doi: 10.3758/BF03327203
Powell, S. B., Sejnowski, T. J., and Behrens, M. M. (2012). Behavioral and neurochemical consequences of cortical oxidative stress on parvalbumin-interneuron maturation in rodent models of schizophrenia. Neuropharmacology 62, 1322–1331. doi: 10.1016/j.neuropharm.2011.01.049
Prewitt, C. M., and Herman, J. P. (1998). Anatomical interactions between the central amygdaloid nucleus and the hypothalamic paraventricular nucleus of the rat: a dual tract-tracing analysis. J. Chem. Neuroanat. 15, 173–185.
Prus, A. J., Mooney-Leber, S. M., Berquist, M. D., Pehrson, A. L., Porter, N. P., and Porter, J. H. (2015). The antidepressant drugs fluoxetine and duloxetine produce anxiolytic-like effects in a schedule-induced polydipsia paradigm in rats: enhancement of fluoxetine’s effects by the α2 adrenoceptor antagonist yohimbine. Behav. Pharmacol. 26, 489–494. doi: 10.1097/FBP.0000000000000159
Pujol, J., Soriano-Mas, C., Alonso, P., Cardoner, N., Menchón, J. M., Deus, J. et al. (2004). Mapping structural brain alterations in obsessive-compulsive disorder. Arch. Gen. Psychiatry 61, 720–730. doi: 10.1001/archpsyc.61.7.720
Raskind, M. A., Orenstein, H., and Christopher, T. G. (1975). Acute psychosis, increased water ingestion, and inappropriate antidiuretic hormone secretion. Am. J. Psychiatry 132, 907–910. doi: 10.1176/ajp.132.9.907
Raymaekers, S., Vansteelandt, K., Luyten, L., Bervoets, C., Demyttenaere, K., Gabriëls, L. et al. (2017). Long-term electrical stimulation of bed nucleus of stria terminalis for obsessive-compulsive disorder. Mol. Psychiatry 22, 931–934. doi: 10.1038/mp.2016.124
Reess, T. J., Rus, O. G., Gürsel, D. A., Schmitz-Koep, B., Wagner, G., Berberich, G. et al. (2017). Association between hippocampus volume and symptom profiles in obsessive–compulsive disorder. Neuroimage Clin. 17, 474–480. doi: 10.1016/j.nicl.2017.11.006
Reess, T. J., Rus, O. G., Gürsel, D. A., Schmitz-Koep, B., Wagner, G., Berberich, G. et al. (2018). Association between hippocampus volume and symptom profiles in obsessive-compulsive disorder. Neuroimage Clin. 17, 474–480. doi: 10.1016/j.nicl.2017.11.006
Reynolds, S. M., and Zahm, D. S. (2005). Specificity in the projections of prefrontal and insular cortex to ventral striatopallidum and the extended amygdala. J. Neurosci. 25, 11757–11767. doi: 10.1523/JNEUROSCI.3432-05.2005
Riffkin, J., Yücel, M., Maruff, P., Wood, S. J., Soulsby, B., Olver, J. et al. (2005). A manual and automated MRI study of anterior cingulate and orbito-frontal cortices, and caudate nucleus in obsessive-compulsive disorder: comparison with healthy controls and patients with schizophrenia. Psychiatry Res. 138, 99–113. doi: 10.1016/j.pscychresns.2004.11.007
Robbins, T. W., Gillan, C. M., Smith, D. G., de Wit, S., and Ersche, K. D. (2012). Neurocognitive endophenotypes of impulsivity and compulsivity: towards dimensional psychiatry. Trends Cogn. Sci. 16, 81–91. doi: 10.1016/j.tics.2011.11.009
Robbins, T. W., and Koob, G. F. (1980). Selective disruption of displacement behaviour by lesions of the mesolimbic dopamine system. Nature 285, 409–412. doi: 10.1038/285409a0
Robbins, T. W., Roberts, D. C., and Koob, G. F. (1983). Effects of d-amphetamine and apomorphine upon operant behavior and schedule-induced licking in rats with 6-hydroxydopamine-induced lesions of the nucleus accumbens. J. Pharmacol. Exp. Ther. 224, 662–673.
Rodaros, D., Caruana, D. A., Amir, S., and Stewart, J. (2007). Corticotropin-releasing factor projections from limbic forebrain and paraventricular nucleus of the hypothalamus to the region of the ventral tegmental area. Neuroscience 150, 8–13. doi: 10.1016/j.neuroscience.2007.09.043
Rodriguez, M. M., Overshiner, C., Leander, J. D., Li, X., Morrow, D., Conway, R. G. et al. (2017). Behavioral effects of a novel benzofuranyl-piperazine serotonin-2C receptor agonist suggest a potential therapeutic application in the treatment of obsessive-compulsive disorder. Front. Psychiatry 8:89. doi: 10.3389/fpsyt.2017.00089
Roehrs, T. A., and Allen, J. D. (1980). Delayed acquisition of schedule-induced polydipsia in rats with zona incerta lesions. Physiol. Behav. 24, 895–899. doi: 10.1016/0031-9384(80)90147-x
Roper, T. J. (1978). Diversity and substitutability of adjunctive activities under fixed-interval schedules of food reinforcement. J. Exp. Anal. Behav. 30, 83–96. doi: 10.1901/jeab.1978.30-83
Rosenzweig-Lipson, S., Sabb, A., Stack, G., Mitchell, P., Lucki, I., Malberg, J. E. et al. (2007). Antidepressant-like effects of the novel, selective, 5-HT2C receptor agonist WAY-163909 in rodents. Psychopharmacology 192, 159–170. doi: 10.1007/s00213-007-0710-6
Rosse, R. B., Fay-McCarthy, M., Collins, J. P., Risher-Flowers, D., Alim, T. N., and Deutsch, S. I. (1993). Transient compulsive foraging behavior associated with crack cocaine use. Am. J. Psychiatry 150, 155–156. doi: 10.1176/ajp.150.1.155
Rotge, J.-Y., Guehl, D., Dilharreguy, B., Tignol, J., Bioulac, B., Allard, M. et al. (2009). Meta-analysis of brain volume changes in obsessive-compulsive disorder. Biol. Psychiatry 65, 75–83. doi: 10.1016/j.biopsych.2008.06.019
Ryan, C. N., Evenden, J. L., and Petterson, M. (1993). Effects of buspirone and ipsapirone on schedule induced polydipsia: comparison with 8-hydroxy-2-(di-n-propylamino)tetralin (8-OH-DPAT) and raclopride. Psychopharmacology 112, 34–44. doi: 10.1007/bf02247361
Saker, P., Farrell, M. J., Egan, G. F., McKinley, M. J., and Denton, D. A. (2016). Overdrinking, swallowing inhibition, and regional brain responses prior to swallowing. Proc. Natl. Acad. Sci. U.S.A. 113, 12274–12279. doi: 10.1073/pnas.1613929113
Sanger, D. J. (1977). Schedule-induced drinking of chlordiazepoxide solutions by rats. Pharmacol. Biochem. Behav. 7, 1–6. doi: 10.1016/0091-3057(77)90003-X
Sanger, D. J., and Corfield-Sumner, P. K. (1979). Schedule-induced drinking and thirst: a pharmacological analysis. Pharmacol. Biochem. Behav. 10, 471–474. doi: 10.1016/0091-3057(79)90219-3
Sapara, A., Cooke, M., Fannon, D., Francis, A., Buchanan, R. W., Anilkumar, A. P. P. et al. (2007). Prefrontal cortex and insight in schizophrenia: a volumetric MRI study. Schizophr. Res. 89, 22–34. doi: 10.1016/j.schres.2006.09.016
Saxena, S., Brody, A. L., Maidment, K. M., Dunkin, J. J., Colgan, M., Alborzian, S. et al. (1999). Localized orbitofrontal and subcortical metabolic changes and predictors of response to paroxetine treatment in obsessive-compulsive disorder. Neuropsychopharmacology 21, 683–693. doi: 10.1016/S0893-133X(99)00082-2
Saxena, S., Brody, A. L., Schwartz, J. M., and Baxter, L. R. (1998). Neuroimaging and frontal-subcortical circuitry in obsessive-compulsive disorder. Br. J. Psychiatry Suppl. 35, 26–37. doi: 10.1192/s0007125000297870
Schobel, S. A., Kelly, M. A., Corcoran, C. M., Van Heertum, K., Seckinger, R., Goetz, R. et al. (2009a). Anterior hippocampal and orbitofrontal cortical structural brain abnormalities in association with cognitive deficits in schizophrenia. Schizophr. Res. 114, 110–118. doi: 10.1016/j.schres.2009.07.016
Schobel, S. A., Lewandowski, N. M., Corcoran, C. M., Moore, H., Brown, T., Malaspina, D. et al. (2009b). Differential targeting of the CA1 subfield of the hippocampal formation by schizophrenia and related psychotic disorders. Arch. Gen. Psychiatry 66, 938–946. doi: 10.1001/archgenpsychiatry.2009.115
Schuster, C. R., and Woods, J. H. (1966). Schedule-induced polydipsia in the rhesus monkey. Psychol. Rep. 19, 823–828. doi: 10.2466/pr0.1966.19.3.823
Scotti-Muzzi, E., and Saide, O. L. (2017). Schizo-obsessive spectrum disorders: an update. CNS Spectr. 22, 258–272. doi: 10.1017/S1092852916000390
Seeman, P., Chau-Wong, M., Tedesco, J., and Wong, K. (1975). Brain receptors for antipsychotic drugs and dopamine: direct binding assays. Proc. Natl. Acad. Sci. U.S.A. 72, 4376–4380. doi: 10.1073/pnas.72.11.4376
Segal, E. F., and Oden, D. L. (1969). Effects of drinkometer current and of foot shock on psychogenic polydipsia. Psychon. Sci. 14, 13–14. doi: 10.3758/BF03336399
Shad, M. U., Tamminga, C. A., Cullum, M., Haas, G. L., and Keshavan, M. S. (2006). Insight and frontal cortical function in schizophrenia: a review. Schizophr. Res. 86, 54–70. doi: 10.1016/j.schres.2006.06.006
Shad, M. U., Keshavan, M. S., Tamminga, C. A., Cullum, C. M., and David, A. (2007). Neurobiological underpinnings of insight deficits in schizophrenia. Int. Rev. Psychiatry 19, 437–446. doi: 10.1080/09540260701486324
Shapleske, J., Rossell, S. L., Chitnis, X. A., Suckling, J., Simmons, A., Bullmore, E. T. et al. (2002). A computational morphometric MRI study of schizophrenia: effects of hallucinations. Cereb. Cortex 12, 1331–1341. doi: 10.1093/cercor/12.12.1331
Shen, T. F., Wang, H. C., Wan, F. J., and Tung, C. S. (2001). Changes in the performance of schedule-induced polydipsia (SIP) in rats after arecoline and amphetamine treatments. Proc. Natl. Sci. Counc. Repub. China B 25, 214–222.
Shin, L. M., and Liberzon, I. (2010). The neurocircuitry of fear, stress, and anxiety disorders. Neuropsychopharmacology 35, 169–191. doi: 10.1038/npp.2009.83
Shutty, M. S., McCulley, K., and Pigott, B. (1995). Association between stereotypic behavior and polydipsia in chronic schizophrenic patients. J. Behav. Ther. Exp. Psychiatry 26, 339–343. doi: 10.1016/0005-7916(95)00049-6
Sim, K., DeWitt, I., Ditman, T., Zalesak, M., Greenhouse, I., Goff, D. et al. (2006). Hippocampal and parahippocampal volumes in schizophrenia: a structural MRI study. Schizophr. Bull. 32, 332–340. doi: 10.1093/schbul/sbj030
Simon, D., Adler, N., Kaufmann, C., and Kathmann, N. (2014). Amygdala hyperactivation during symptom provocation in obsessive–compulsive disorder and its modulation by distraction. Neuroimage Clin. 4, 549–557. doi: 10.1016/j.nicl.2014.03.011
Singer, G., Armstrong, S., and Wayner, M. J. (1975). Effects of norepinephrine applied to the lateral hypothalamus on schedule induced polydipsia. Pharmacol. Biochem. Behav. 3, 869–872. doi: 10.1016/0091-3057(75)90119-7
Singer, G., Oei, T. P., and Wallace, M. (1982). Schedule-induced self-injection of drugs. Neurosci. Biobehav. Rev. 6, 77–83. doi: 10.1016/0149-7634(82)90008-2
Slifstein, M., van de Giessen, E., Van Snellenberg, J., Thompson, J. L., Narendran, R., Gil, R. et al. (2015). Deficits in prefrontal cortical and extrastriatal dopamine release in schizophrenia: a positron emission tomographic functional magnetic resonance imaging study. JAMA Psychiatry 72, 316–324. doi: 10.1001/jamapsychiatry.2014.2414
Snodgrass, S. H., and Allen, J. D. (1987). Effect of dopamine agents on schedule- and deprivation-induced drinking in rats. Pharmacol. Biochem. Behav. 27, 463–475. doi: 10.1016/0091-3057(87)90350-9
Snodgrass, S. H., and Allen, J. D. (1988). The effects of apomorphine on the acquisition of schedule-induced polydipsia in rats. Pharmacol. Biochem. Behav. 29, 483–488. doi: 10.1016/0091-3057(88)90008-1
Snodgrass, S. H., and Allen, J. D. (1989). Time-response effects of pimozide on operant behavior and schedule-induced polydipsia. Pharmacol. Biochem. Behav. 32, 949–955. doi: 10.1016/0091-3057(89)90064-6
Steen, R. G., Mull, C., McClure, R., Hamer, R. M., and Lieberman, J. A. (2006). Brain volume in first-episode schizophrenia: systematic review and meta-analysis of magnetic resonance imaging studies. Br. J. Psychiatry J. Ment. Sci. 188, 510–518. doi: 10.1192/bjp.188.6.510
Stuart, C. A., Neelon, F. A., and Lebovitz, H. E. (1980). Disordered control of thirst in hypothalamic-pituitary sarcoidosis. N. Engl. J. Med. 303, 1078–1082. doi: 10.1056/NEJM198011063031902
Subramanian, K., Rajan, T. M., Menon, V., and Rajkumar, R. P. (2017). Converging neurobiological evidence in primary polydipsia resembling obsessive-compulsive disorder. Indian J. Psychol. Med. 39, 369–372. doi: 10.4103/0253-7176.207327
Sukhanov, I., Dorotenko, A., Dolgorukova, A., Hoener, M. C., Gainetdinov, R. R., and Bespalov, A. Y. (2019). Activation of trace amine-associated receptor 1 attenuates schedule-induced polydipsia in rats. Neuropharmacology 144, 184–192. doi: 10.1016/j.neuropharm.2018.10.034
Svensson, T. H. (2000). Dysfunctional brain dopamine systems induced by psychotomimetic NMDA-receptor antagonists and the effects of antipsychotic drugs. Brain Res. Brain Res. Rev. 31, 320–329. doi: 10.1016/s0165-0173(99)00048-x
Szechtman, H., Ahmari, S. E., Beninger, R. J., Eilam, D., Harvey, B. H., Edemann-Callesen, H. et al. (2017). Obsessive-compulsive disorder: insights from animal models. Neurosci. Biobehav. Rev. 76, 254–279. doi: 10.1016/j.neubiorev.2016.04.019
Szechtman, H., Sulis, W., and Eilam, D. (1998). Quinpirole induces compulsive checking behavior in rats: a potential animal model of obsessive-compulsive disorder (OCD). Behav. Neurosci. 112, 1475–1485. doi: 10.1037//0735-7044.112.6.1475
Taghzouti, K., Simon, H., Tazi, A., Dantzer, R., and Le Moal, M. (1985). The effect of 6-OHDA lesions of the lateral septum on schedule-induced polydipsia. Behav. Brain Res. 15, 1–8. doi: 10.1016/0166-4328(85)90012-9
Tandon, R., Keshavan, M. S., and Nasrallah, H. A. (2008a). Schizophrenia, “just the facts” what we know in 2008. 2. Epidemiology and etiology. Schizophr. Res. 102, 1–18. doi: 10.1016/j.schres.2008.04.011
Tandon, R., Keshavan, M. S., and Nasrallah, H. A. (2008b). Schizophrenia, “Just the Facts”: what we know in 2008 part 1: overview. Schizophr. Res. 100, 4–19. doi: 10.1016/j.schres.2008.01.022
Tang, C., Wallace, M., Singer, G., and Mackenzie, L. (1984). Resistance of schedule-induced behaviours to hippocampal lesions. Pharmacol. Biochem. Behav. 20, 537–541. doi: 10.1016/0091-3057(84)90301-0
Thompson, C. J., Edwards, C. R., and Baylis, P. H. (1991). Osmotic and non-osmotic regulation of thirst and vasopressin secretion in patients with compulsive water drinking. Clin. Endocrinol. 35, 221–228. doi: 10.1111/j.1365-2265.1991.tb03526.x
Todd, K. G., Beck, C. H., and Martin-Iverson, M. T. (1992). Effects of D1 and D2 dopamine antagonists on behavior of polydipsic rats. Pharmacol. Biochem. Behav. 42, 381–388. doi: 10.1016/0091-3057(92)90130-8
Toro, J., Cervera, M., Osejo, E., and Salamero, M. (1992). Obsessive-compulsive disorder in childhood and adolescence: a clinical study. J. Child Psychol. Psychiatry 33, 1025–1037.
Toscano, C. A., Kameyama, M., Garcia-Mijares, M., Silva, M. T. A., and Santarem, E. M. M. (2008). Relationship between ethanol and sucrose self-administration and schedule-induced polydipsia. Pharmacol. Biochem. Behav. 90, 586–589. doi: 10.1016/j.pbb.2008.04.019
Tracy, J. I., de Leon, J., Qureshi, G., McCann, E. M., McGrory, A., and Josiassen, R. C. (1996). Repetitive behaviors in schizophrenia: a single disturbance or discrete symptoms? Schizophr. Res. 20, 221–229. doi: 10.1016/0920-9964(95)00104-2
Tregellas, J. R., Shatti, S., Tanabe, J. L., Martin, L. F., Gibson, L., Wylie, K. et al. (2007). Gray matter volume differences and the effects of smoking on gray matter in schizophrenia. Schizophr. Res. 97, 242–249. doi: 10.1016/j.schres.2007.08.019
Tung, C. S., Wu, W. H., Tseng, C. J., and Yin, T. H. (1994). Effects of amperozide on schedule-induced polydipsia in rats. Eur. J. Pharmacol. 256, 193–200. doi: 10.1016/0014-2999(94)90245-3
Vaccarino, F. J., Schiff, B. B., and Glickman, S. E. (1989). “Biological view of reinforcement,” in Contemporary Learning Theories: Instrumental Conditioning Theory and the Impact of Biological Constraints on Learning, eds S. B. Klein and R. R. Mowrer (Hillsdale, NJ: Lawrence Erlbaum Associates, Inc.), 111–142. doi: 10.4324/9781315788982-7
Valente, A. A., Miguel, E. C., Castro, C. C., Amaro, E., Duran, F. L. S., Buchpiguel, C. A. et al. (2005). Regional gray matter abnormalities in obsessive-compulsive disorder: a voxel-based morphometry study. Biol. Psychiatry 58, 479–487. doi: 10.1016/j.biopsych.2005.04.021
van den Heuvel, O. A., Remijnse, P. L., Mataix-Cols, D., Vrenken, H., Groenewegen, H. J., Uylings, H. B. M. et al. (2009). The major symptom dimensions of obsessive-compulsive disorder are mediated by partially distinct neural systems. Brain J. Neurol. 132, 853–868. doi: 10.1093/brain/awn267
van der Wee, N. J., Stevens, H., Hardeman, J. A., Mandl, R. C., Denys, D. A., and van Megen, H. J. et al. (2004). Enhanced dopamine transporter density in psychotropic-naive patients with obsessive-compulsive disorder shown by [123I]{beta}-CIT SPECT. Am. J. Psychiatry 161, 2201–2206. doi: 10.1176/appi.ajp.161.12.2201
van Kuyck, K., Brak, K., Das, J., Rizopoulos, D., and Nuttin, B. (2008). Comparative study of the effects of electrical stimulation in the nucleus accumbens, the mediodorsal thalamic nucleus and the bed nucleus of the stria terminalis in rats with schedule-induced polydipsia. Brain Res. 1201, 93–99. doi: 10.1016/j.brainres.2008.01.043
van Rossum, J. M. (1966). The significance of dopamine-receptor blockade for the mechanism of action of neuroleptic drugs. Arch. Int. Pharmacodyn. Ther. 160, 492–494.
Vieweg, V., Rowe, W., David, J., and Spradlin, W. (1984). Hyposthenuria as a marker for self-induced water intoxication and schizophrenic disorders. Am. J. Psychiatry 141, 1258–1260. doi: 10.1176/ajp.141.10.1258
Vieweg, W. V., Rowe, W. T., David, J. J., Sutker, L. H., and Spradlin, W. W. (1984). Evaluation of patients with self-induced water intoxication and schizophrenic disorders (SIWIS). J. Nerv. Ment. Dis. 172, 552–555. doi: 10.1097/00005053-198409000-00008
Vita, A., De Peri, L., Silenzi, C., and Dieci, M. (2006). Brain morphology in first-episode schizophrenia: a meta-analysis of quantitative magnetic resonance imaging studies. Schizophr. Res. 82, 75–88. doi: 10.1016/j.schres.2005.11.004
Wallace, M., Singer, G., Finlay, J., and Gibson, S. (1983). The effect of 6-OHDA lesions of the nucleus accumbens septum on schedule-induced drinking, wheelrunning and corticosterone levels in the rat. Pharmacol. Biochem. Behav. 18, 129–136. doi: 10.1016/0091-3057(83)90262-9
Wayner, M. J., and Greenberg, I. (1972). Effects of septal lesions on palatability modulation of schedule-induced polydipsia. Physiol. Behav. 9, 663–665. doi: 10.1016/0031-9384(72)90028-5
Wayner, M. J., Greenberg, I., and Trowbridge, J. (1973). Effects of d-amphetamine on schedule induced polydipsia. Pharmacol. Biochem. Behav. 1, 109–111. doi: 10.1016/0091-3057(73)90063-4
Weissenborn, R., Blaha, C. D., Winn, P., and Phillips, A. G. (1996). Schedule-induced polydipsia and the nucleus accumbens: electrochemical measurements of dopamine efflux and effects of excitotoxic lesions in the core. Behav. Brain Res. 75, 147–158. doi: 10.1016/0166-4328(95)00202-2
Wilke, M., Kaufmann, C., Grabner, A., Pütz, B., Wetter, T. C., and Auer, D. P. (2001). Gray matter-changes and correlates of disease severity in schizophrenia: a statistical parametric mapping study. Neuroimage 13, 814–824. doi: 10.1006/nimg.2001.0751
Wilkinson, L. S., Killcross, S. S., Humby, T., Hall, F. S., Geyer, M. A., and Robbins, T. W. (1994). Social isolation in the rat produces developmentally specific deficits in prepulse inhibition of the acoustic startle response without disrupting latent inhibition. Neuropsychopharmacology 10, 61–72. doi: 10.1038/npp.1994.8
Williams, J. L., and White, J. M. (1984). The effects of amphetamine and scopolamine on adjunctive drinking and wheel-running in rats. Psychopharmacology 82, 360–367. doi: 10.1007/bf00427686
Winn, P., Clark, J. M., Clark, A. J., and Parker, G. C. (1992). NMDA lesions of lateral hypothalamus enhance the acquisition of schedule-induced polydipsia. Physiol. Behav. 52, 1069–1075. doi: 10.1016/0031-9384(92)90461-a
Winter, L., Heitland, I., Saryyeva, A., Lütjens, G., Schwabe, K., Heissler, H. E. et al. (2018). Acute effects of electrical stimulation of the bed nucleus of the stria terminalis/internal capsule in obsessive-compulsive disorder. World Neurosurg. 111, e471–e477. doi: 10.1016/j.wneu.2017.12.084
Wise, R. A. (2004). Drive, incentive, and reinforcement: the antecedents and consequences of motivation. Nebr. Symp. Motiv. 50, 159–195.
Wood, R. I., and Swann, J. M. (2005). The bed nucleus of the stria terminalis in the Syrian hamster: subnuclei and connections of the posterior division. Neuroscience 135, 155–179. doi: 10.1016/j.neuroscience.2005.05.029
Wood, S. J., Kennedy, D., Phillips, L. J., Seal, M. L., Yücel, M., Nelson, B. et al. (2010). Hippocampal pathology in individuals at ultra-high risk for psychosis: a multi-modal magnetic resonance study. Neuroimage 52, 62–68. doi: 10.1016/j.neuroimage.2010.04.012
Woods, A., Smith, C., Szewczak, M., Dunn, R. W., Cornfeldt, M., and Corbett, R. (1993). Selective serotonin re-uptake inhibitors decrease schedule-induced polydipsia in rats: a potential model for obsessive compulsive disorder. Psychopharmacology 112, 195–198. doi: 10.1007/bf02244910
Woods-Kettelberger, A. T., Smith, C. P., Corbett, R., Szewczak, M. R., Roehr, J. E., Bores, G. M. et al. (1996). Besipirdine (HP 749) reduces schedule-induced polydipsia in rats. Brain Res. Bull. 41, 125–130. doi: 10.1016/s0361-9230(96)00163-3
Wright, J. W., and Kelso, S. C. (1981). Adrenal demedullation suppresses schedule-induced polydipsia in rats. Physiol. Behav. 26, 1–5. doi: 10.1016/0031-9384(81)90070-6
Xue, G., Ghahremani, D. G., and Poldrack, R. A. (2008). Neural substrates for reversing stimulus-outcome and stimulus-response associations. J. Neurosci. 28, 11196–11204. doi: 10.1523/JNEUROSCI.4001-08.2008
Yin, H. H., Knowlton, B. J., and Balleine, B. W. (2004). Lesions of dorsolateral striatum preserve outcome expectancy but disrupt habit formation in instrumental learning. Eur. J. Neurosci. 19, 181–189. doi: 10.1111/j.1460-9568.2004.03095.x
Yin, H. H., Knowlton, B. J., and Balleine, B. W. (2006). Inactivation of dorsolateral striatum enhances sensitivity to changes in the action-outcome contingency in instrumental conditioning. Behav. Brain Res. 166, 189–196. doi: 10.1016/j.bbr.2005.07.012
Yoburn, B. C., and Glusman, M. (1982). Effects of chronic d-amphetamine on the maintenance and acquisition of schedule-induced polydipsia in rats. Physiol. Behav. 28, 807–818. doi: 10.1016/0031-9384(82)90197-4
Zimmerman, C. A., Huey, E. L., Ahn, J. S., Beutler, L. R., Tan, C. L., Kosar, S. et al. (2019). A gut-to-brain signal of fluid osmolarity controls thirst satiation. Nature 568, 98–102. doi: 10.1038/s41586-019-1066-x
Keywords: obsessive compulsive disorder, schizophrenia, dopamine, BNST, orbitofrontal cortex
Citation: Banasikowski TJ and Hawken ER (2019) The Bed Nucleus of the Stria Terminalis, Homeostatic Satiety, and Compulsions: What Can We Learn From Polydipsia? Front. Behav. Neurosci. 13:170. doi: 10.3389/fnbeh.2019.00170
Received: 06 March 2019; Accepted: 12 July 2019;
Published: 01 August 2019.
Edited by:
Trevor W. Robbins, University of Cambridge, United KingdomReviewed by:
Ingo Willuhn, Netherlands Institute for Neuroscience (KNAW), NetherlandsTara Arbab, Netherlands Institute for Neuroscience, Netherlands, in collaboration with reviewer IW
Marco Venniro, National Institute on Drug Abuse (NIDA), United States
Copyright © 2019 Banasikowski and Hawken. This is an open-access article distributed under the terms of the Creative Commons Attribution License (CC BY). The use, distribution or reproduction in other forums is permitted, provided the original author(s) and the copyright owner(s) are credited and that the original publication in this journal is cited, in accordance with accepted academic practice. No use, distribution or reproduction is permitted which does not comply with these terms.
*Correspondence: Emily R. Hawken, aGF3a2VuQHF1ZWVuc3UuY2E=; ZXJoYXdrZW5AZ21haWwuY29t