- 1Department of Biomedical Sciences, University of Cagliari – Cittadella Universitaria di Monserrato, Monserrato, Italy
- 2CNR Institute of Neuroscience-Cagliari, National Research Council, Rome, Italy
Cannabis is the most commonly used illicit drug worldwide. Although its use is associated with multiple adverse health effects, including the risk of developing addiction, recreational and medical cannabis use is being increasing legalized. In addition, use of synthetic cannabinoid drugs is gaining considerable popularity and is associated with mass poisonings and occasional deaths. Delineating factors involved in cannabis use and addiction therefore becomes increasingly important. Similarly to other drugs of abuse, the prevalence of cannabis use and addiction differs remarkably between males and females, suggesting that sex plays a role in regulating cannabinoid sensitivity. Although it remains unclear how sex may affect the initiation and maintenance of cannabis use in humans, animal studies strongly suggest that endogenous sex hormones modulate cannabinoid sensitivity. In addition, synthetic anabolic-androgenic steroids alter substance use and further support the importance of sex steroids in controlling drug sensitivity. The recent discovery that pregnenolone, the precursor of all steroid hormones, controls cannabinoid receptor activation corroborates the link between steroid hormones and the endocannabinoid system. This article reviews the literature regarding the influence of endogenous and synthetic steroid hormones on the endocannabinoid system and cannabinoid action.
Introduction
Drug use causes considerable harm because of premature death and disability as well as other adverse health effects. The United Nations Office on Drugs and Crime estimated that around 0.6% of the world population suffers from substance use disorders (United Nations Office on Drugs and Crime [UNODC], 2017). Although opioids are considered the most harmful drugs for their addiction potential and negative consequences, cannabis use is a much larger problem when it comes to the number of users. Around 183 million “past-year” cannabis users were reported worldwide in 2015, which is 2.6 times higher than the cumulative number of “past-year” worldwide users of opioids, amphetamines and cocaine, making cannabis the most widely used illicit drug at a global level (United Nations Office on Drugs and Crime [UNODC], 2017). Although worldwide cannabis use has remained stable (3.4% in 1998 versus 3.8% in 2015), the absolute number of cannabis users has increased because of the growing world population, especially in Africa and Asia (United Nations Office on Drugs and Crime [UNODC], 2017). Legalization of marijuana for medical and recreational purposes might increase cannabis use even further (Hopfer, 2014). In addition to traditional marijuana use, the use of synthetic cannabinoids (i.e., designer drugs that mimic the physical and psychological effects of delta-9-tetrahydrocannabinol (THC), the primary active constituent in cannabis) is gaining considerable popularity. Since 2008, when the first synthetic cannabinoid (JWH-018) was detected in the market, at least 169 different synthetic cannabinoids have been discovered (Fattore and Fratta, 2011; European Monitoring Centre for Drugs and Drug Addiction [EMCDDA], 2017). The emergence of synthetic cannabinoids is becoming an increasing concern because of their undetermined addiction potential and adverse health effects (Fattore, 2016; Weinstein et al., 2017; De Luca and Fattore, 2018; Zanda and Fattore, 2018).
Acute toxicity of traditional cannabis use is considered low (Nahas, 1972); yet, long-term cannabis use is associated with serious adverse health effects which include lower birth weight of offspring (maternal cannabis smoking), diminished lifetime achievement, development of psychosis, depression or anxiety, symptoms of chronic bronchitis, motor vehicle accidents, and risk of cannabis addiction (Hall and Degenhardt, 2009; Volkow et al., 2014; United Nations Office on Drugs and Crime [UNODC], 2017). Although the existence of cannabis addiction was disputed in the 1990s, current evidence predicts that around 1 in 10 cannabis users will develop cannabis addiction or dependence (Lopez-Quintero et al., 2011), which is currently defined as cannabis use disorder (CUD) in the fifth edition of the Diagnostic and Statistical Manual for Mental Disorders (5th ed.; DSM-5; American Psychiatric and Association, 2013). CUD is characterized by high cannabis intake over longer periods of time, problems with controlling cannabis use, tolerance, withdrawal signs, craving and negative effects on personal, social and occupational activities (DSM-5).
The demand for CUD treatment is increasing dramatically. The European Monitoring Centre for Drugs and Drug Addiction reported a 50% increase in the number of first-time entrants for CUD treatment in 2011 (European Monitoring Centre for Drugs and Drug Addiction [EMCDDA], 2013). The increasing need for CUD treatment is thought to be driven by the increased availability of cannabis products containing higher concentrations of THC or synthetic cannabinoids (Freeman and Winstock, 2015). Regrettably, current CUD treatment protocols show modest effects only (Budney et al., 2007; Weinstein and Gorelick, 2011). Delineating risk factors involved in the initiation and maintenance of cannabis use therefore becomes increasingly important and critical for optimizing evidence-based prevention and treatment protocols.
Similarly to other drugs of abuse, cannabis use differs remarkably between males and females (European Monitoring Centre for Drugs and Drug Addiction [EMCDDA], 2005), indicating a different sensitivity to cannabinoid-induced effects in the two sexes (Davis and Fattore, 2015; Figure 1). Although it remains uncertain which specific biological (i.e., sex) and socio-cultural (i.e., gender) factors affect cannabis use in humans, animal studies strongly suggest the involvement of sex (Fattore and Fratta, 2010) and anabolic-androgenic steroids (AAS) hormones (Struik et al., 2017) as important modulators of cannabinoid sensitivity. This review aims to describe the role of sex differences in cannabis use with reference to the modulating role of sex and AAS hormones (Figure 2) in cannabinoid sensitivity.
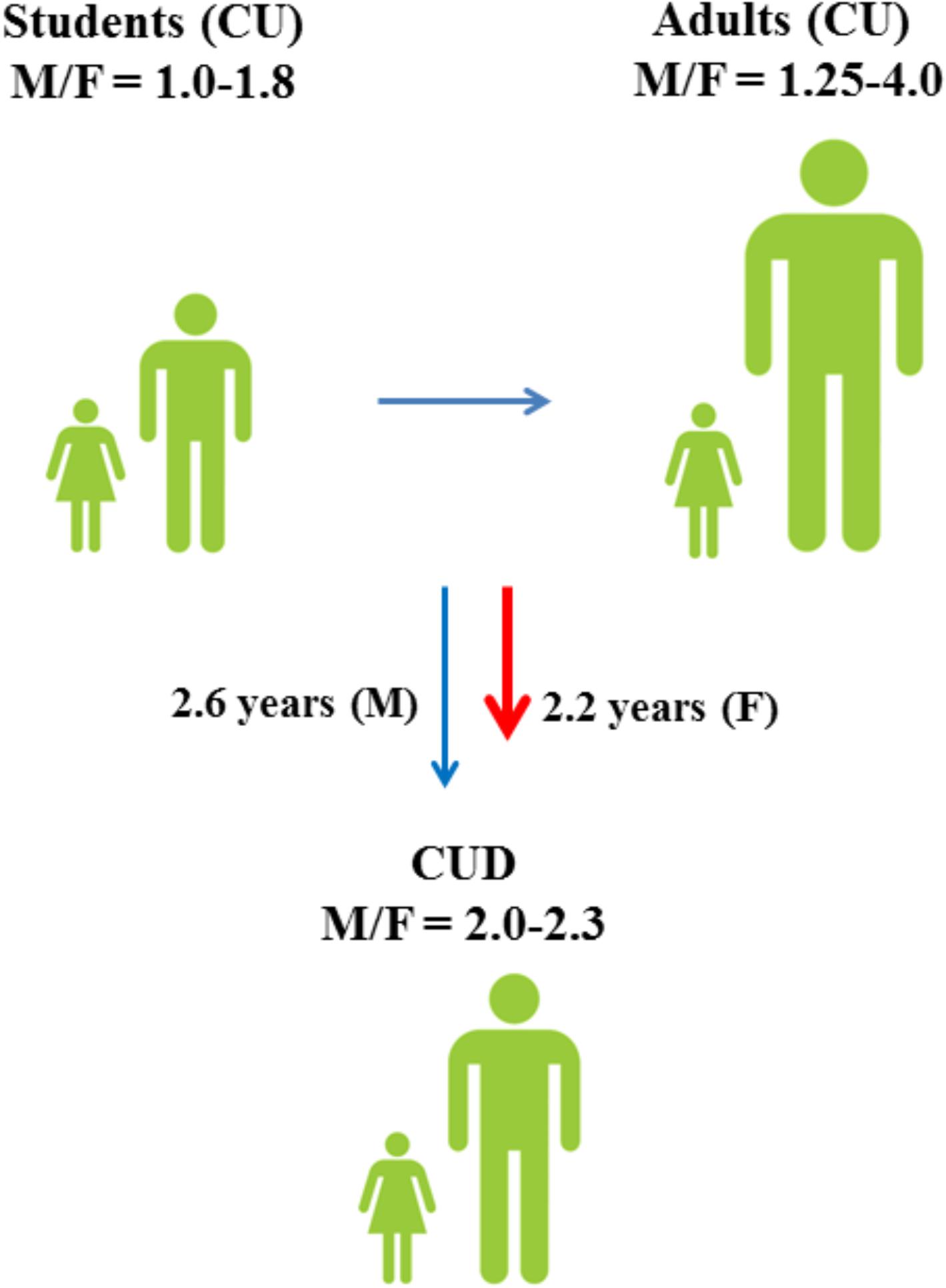
FIGURE 1. Male to female ratios of lifetime cannabis use (CU) and progression toward cannabis use disorder (CUD) among students (15–16 years) and adults (European Monitoring Centre for Drugs and Drug Addiction [EMCDDA], 2005). Although males have a higher risk of developing CUD (Zhu and Wu, 2017), progression toward CUD is faster in females (Khan et al., 2013).
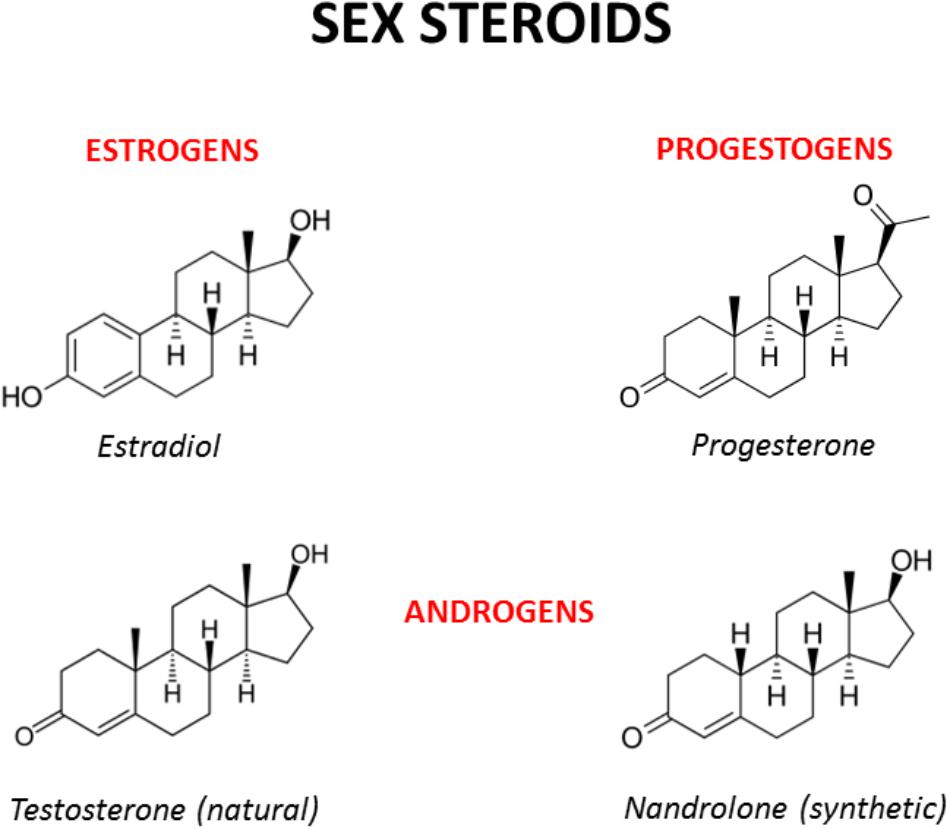
FIGURE 2. Chemical structures of the main male and female sex hormones and the anabolic-androgenic steroid nandrolone. The strict chemical homology with a common core cyclic structure among natural and synthetic steroids accounts for the relatively high cross-reactivity displayed by sex steroids and AAS at receptor level. The only difference between testosterone and nandrolone (19-nortestosterone) is the methyl group (CH3) of nandrolone in position C19 instead of the hydrogen (H) of testosterone, which increases the anabolic activity of nandrolone and is at the basis of its use as a doping drug (see Busardò et al., 2015 and references enclosed).
Risk Factors for Cannabis Use
As for other drugs of abuse, both genetic and environmental factors play a role in cannabis use and addiction (Agrawal and Lynskey, 2006; Verweij et al., 2010). Twin studies estimate that the genetic contribution to cannabis use is between 17 and 67%, while the genetic contribution to cannabis addiction is much higher and ranges from 45 to 78% (Verweij et al., 2010; Vink et al., 2010; Distel et al., 2011; Lynskey et al., 2012). Interestingly, the genetic contribution to the initiation of cannabis use increases with age (Distel et al., 2011) and is higher in males than in females (van den Bree et al., 1998). Although it is clear that genetics is an important risk factor in cannabis use and abuse, it has so far proved difficult to identify specific gene variants that alter cannabis sensitivity. At present, most genome-wide association studies (GWAS) failed to detect significant associations between cannabis use and genetic variants (Agrawal et al., 2011; Verweij et al., 2013; Stringer et al., 2016). However, using gene-based testing, four genes that are significantly associated with lifetime cannabis use have been recently identified, which include the neural cell adhesion molecule 1 (NCAM1), the cell adhesion molecule 2 (CADM2), the Short Coiled-Coil Protein (SCOC) and the potassium sodium-activated channel subfamily T member 2 (KCNT2) (Stringer et al., 2016). Interestingly, NCAM1 has been associated with substance abuse (Gelernter et al., 2006) and is part of the NTAD gene cluster (NCAM1-TTC12-ANKK1-DRD2) which is linked to neurogenesis and dopaminergic signaling (Yang et al., 2008). In the most recent GWAS, single-nucleotide polymorphisms (SNPs) in novel antisense transcript RP11-206M11.7, solute carrier family 35 member G1, and the CUB and Sushi multiple domains 1 gene were significantly associated with cannabis dependence (Sherva et al., 2016). However, whether or not these genes contribute to altered cannabinoid action remains unclear. Next to genetic variation, epigenetic-dependent changes in gene expression might contribute to altered cannabinoid sensitivity. Interestingly, a recent study reported increased DNA methylation of the NCAM1 gene in cannabis users compared to control subjects (Gerra et al., 2018).
The vulnerability to initiation of cannabis use and CUD development appears heritable. Yet, numerous social and environmental factors (e.g., age of cannabis use initiation, peer drug use, availability of drugs, low socio-economic status, experience of childhood sexual abuse, cigarette smoking or alcohol drinking during early adolescence) and the presence of pre/comorbid psychopathology (e.g., mood disorders, ADHD, psychosis) are thought to enhance the risk of transitioning from initiation of cannabis use to CUD (reviewed in Courtney et al., 2017). Personality/biological traits, such as impulsivity, schizotypy and sensation-seeking, are also positively correlated with the initiation of cannabis use in adolescents and young adults (Haug et al., 2014; Muro and Rodríguez, 2015).
As for other drugs of abuse, the prevalence of cannabis use differs remarkably between males and females (Figure 1; European Monitoring Centre for Drugs and Drug Addiction [EMCDDA], 2005) and sex is considered an important risk factor for cannabis use (Cooper and Craft, 2017). Among 15–16-years-old students, lifetime cannabis use is higher in males than in females and the male to female ratio (M/F) of lifetime cannabis use increases even further among all adults (M/F: 1.25–4.0) (European Monitoring Centre for Drugs and Drug Addiction [EMCDDA], 2005). Although males have a higher risk of developing CUD (Zhu and Wu, 2017), progression toward CUD is slightly faster in females than in males (Khan et al., 2013; European Monitoring Centre for Drugs and Drug Addiction [EMCDDA], 2017). Males also show different cannabis use patterns as compared to females and appear to use cannabis more frequently and at higher amounts (Cuttler et al., 2016). However, a faster progression to problematic cannabis use (Cooper and Haney, 2014) and more severe withdrawal symptoms (Levin et al., 2010) could explain why women typically show greater propensity to relapse to drug use than men (Becker and Hu, 2008; Fattore et al., 2008).
The fact that differences in cannabis use between males and females vary across countries suggests an influence of environmental (i.e., socio-cultural) factors. However, animal studies clearly indicate that biological factors, such as sex hormones and chromosomes, are significant modulators of drug sensitivity (Quinn et al., 2007; Marusich et al., 2015). In keeping with this, gender-tailored detoxification treatments and relapse prevention strategies for patients with CUD are increasingly requested (Fattore, 2013).
Sex Steroid Hormones
Sex differences arise because of differences in sex chromosomes. The presence of the sex-determining region of Y (Sry) gene on the Y chromosome induces testicular development and consequently the production of testosterone (Polanco and Koopman, 2007). Testosterone and its derivative dihydrotestosterone (DHT) are responsible for the development of the male phenotype. Absence of the Sry gene leads to the development of ovaries that produce estradiol and progesterone. Estrogens, progesterone and testosterone have a strong impact on sexual differentiation, maturation and adult sexual behavior (Arnold and Breedlove, 1985; McEwen et al., 1987; Wallen, 1990; Meisel and Sachs, 1994; Hull et al., 1999; Morris et al., 2004; Becker, 2009; Argiolas and Melis, 2013; Motta-Mena and Puts, 2017). The presence of sex hormones during development gives rise to various organizational differences in the male and female brain, which ultimately affect reproductive and non-reproductive behavior (Beatty, 1979).
Sex hormones are synthesized by conversion of cholesterol into pregnenolone, which is the precursor of all steroid hormones (Hanukoglu, 1992). Interestingly, pregnenolone protects the brain from cannabinoid type-1 receptor (CB1R) overactivation, by acting as a potent endogenous allosteric inhibitor of CB1Rs (Vallée et al., 2014), and prevents cannabinoid-induced psychosis in mice (Busquets-Garcia et al., 2017). Sex hormones can be divided into three main subtypes with distinct molecular functions and sexually dimorphic expression and distribution: androgens (e.g., testosterone, dehydroepiandrosterone, androstenedione), estrogens (e.g., 17-alpha and 17-beta estradiol, estrone, estriol) and progestogens (e.g., progesterone, allopregnanolone, pregnenolone) (Figure 2). Sex hormones are produced by the gonads in response to the stimulating activity of the pituitary gonadotropins whose release is, in turn, under the control of the hypothalamic gonadotropin releasing hormone (GnRH). At the central level, several neurotransmitters are able to modify the release of GnRH, including norepinephrine, dopamine, serotonin, gamma-aminobutyric acid (GABA) and glutamate (Sagrillo et al., 1996). Cannabinoids were found to significantly modulate the activity of the hypothalamic-pituitary-gonadal (HPG) and -adrenal (HPA) axes (Brown and Dobs, 2002) and their interactions (Karamikheirabad et al., 2013). Interestingly, sex hormones influence the action of cannabinoids on these axes (López, 2010) suggesting bidirectional interactions between sex hormones and the endocannabinoid system (Table 1).
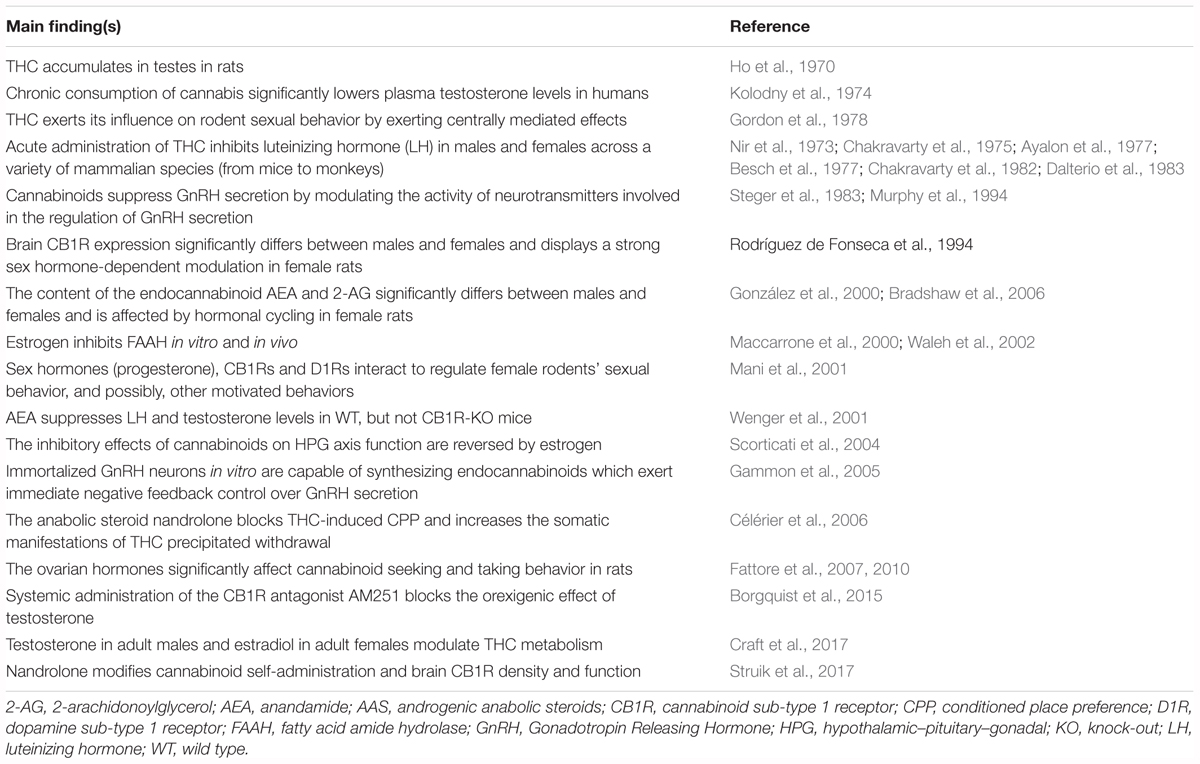
TABLE 1. Main findings from representative studies investigating the interaction between the endocannabinoid system and the sex or ASS hormones.
The main molecular targets of sex hormones are members of the nuclear hormone receptor family, which areligand-activated transcription factors involved in the regulation of gene expression (Mangelsdorf et al., 1995). Testosterone, estrogen and progesterone target the androgen receptors (ARα and ARβ), the estrogen receptors (ERα and ERβ) and the progesterone receptor, respectively, although considerable receptor “promiscuity” might exist in each case. Nuclear receptors are ubiquitously expressed in the central nervous system (CNS), including areas associated with reward and addiction (Bookout et al., 2006). Besides transcriptional effects, sex hormones are also reported to have fast non-genomic actions by modulating the activity of G protein-coupled receptors (GPRCs), ion channels and signaling proteins (Simoncini and Genazzani, 2003).
Sex hormones cause permanent organizational sex differences that are fixed during early development but they also maintain certain sex differences during the adult phase as long as these hormones are present, i.e., induce activational effects (McCarthy et al., 2012). For example, gonadectomy in adulthood completely suppresses sexual behavior in males and receptive and proceptive behaviors in females, all effects being reverted by exogenous hormonal replacement (Micheal and Wilson, 1974; Mitchell and Stewart, 1989; Jones et al., 2017). When released, sex hormones are also able to deeply influence the organization and activity of one of the most important target organs of hormonal action, which is the brain (Arnold and Breedlove, 1985; McEwen and Milner, 2017). Gonadal hormones thus provide a biological basis for sex differences in endocannabinoid-related behaviors and are expected to contribute to the sexual dimorphic actions of cannabinoids (Craft and Leitl, 2008; Craft et al., 2013).
Sex Differences in the Endocannabinoid System
The endocannabinoid system is an evolutionary conserved signaling system that modulates multiple functions and consists of cannabinoid receptors, endogenous ligands (i.e., endocannabinoids) and several enzymes involved in the synthesis and degradation of endocannabinoids. The receptors and endogenous ligands of the endocannabinoid system were discovered in the late ‘80s and early ’90s, respectively. CB1Rs are highly expressed in the brain (Tsou et al., 1998; Freund et al., 2003; Howlett et al., 2004) and are considered the main type of receptor mediating cannabinoid signaling in response to exposure to THC (Moldrich and Wenger, 2000). CB1Rs are also highly expressed in fat tissue which might explain their role in energy homeostasis regulation, while cannabinoid type-2 receptors (CB2Rs) are predominantly expressed in cells of the immune system (van der Stelt and Di Marzo, 2005). CB1Rs and CB2Rs are GPCR and can be activated by THC or endogenous cannabinoid ligands like anandamide (AEA) and 2-arachidonylglycerol (2-AG) (McPartland et al., 2007). Activation of cannabinoid receptors results in the modulation of several signals, including inhibition of adenylate cyclase, activation of the MAPK pathway, stimulation of inwardly rectifying K+ channels, and inhibition of voltage-activated Ca2+ channels. Ultimately, cannabinoid receptor activation modulates the activity of most neurotransmitter systems, including GABA, glutamate, dopamine, and serotonin (van der Stelt and Di Marzo, 2003). The tonic 2-AG signaling at inhibitory inputs onto dopamine neurons has been shown to differ between sexes (Melis et al., 2013), supporting the notion that there are quantitative differences in the endocannabinoid system in males and females which likely contribute to altered cannabinoid sensitivity. Noteworthy, several sex differences in the endocannabinoid system are related to changes in steroid hormone levels and activity.
Sex hormones can affect the activity of several neurotransmitters in the CNS, including the endocannabinoid functioning (Nguyen et al., 2017; Moraga-Amaro et al., 2018), and significant sex-dependent differences in CB1R density and function have been described (reviewed in Antinori and Fattore, 2017). In a pioneering work, Rodríguez de Fonseca et al. (1994) investigated the expression of brain CB1Rs in male and female rats under different hormonal conditions and reported higher CB1R binding in males than females in almost all the brain areas investigated (i.e., striatum, limbic forebrain, and mesencephalon). Notably, CB1R binding in males was not affected by gonadectomy and/or testosterone replacement, while in females a strong sex hormone-dependent modulation of CB1R expression was observed, with ovariectomy increasing CB1R affinity in the striatum and decreasing CB1R density in the limbic forebrain (Rodríguez de Fonseca et al., 1994). González et al. (2000) found that males have higher levels of CB1R-mRNA transcripts than females in the anterior pituitary gland but that, in females, CB1R-mRNA transcripts fluctuate during the different phases of the ovarian cycle with the highest expression on the second day of diestrus and the lowest expression on estrus. Based on these findings it was suggested that higher levels of estrogen in the anterior pituitary gland could serve to inhibit CB1R expression, reducing the inhibitory endocannabinoid tone within the HPG axis around the time of ovulation (López, 2010). More recently, Castelli et al. (2014) found that CB1R density was significantly lower in the prefrontal cortex (PFC) and amygdala of cycling females compared to males and ovariectomized (OVX) females, and that administration of estradiol to OVX markedly reduced the density of CB1Rs to the levels observed in cycling females. In addition, OVX females displayed higher CB1R function in the cingulate cortex compared to intact and OVX + estradiol females. Interestingly, sex and estradiol also affected motor activity, social behavior and sensorimotor gating (Castelli et al., 2014), which are behaviors sensitive to the effects of different classes of drugs of abuse, in line with the idea that females can represent a more vulnerable phenotype (at neurochemical and behavioral level) than male rats in developing addiction-like behaviors. In addition, estradiol time-dependently modulates CB1R binding in brain structures that mediate nociception and locomotor activity (Wakley et al., 2014).
Besides impacting on CB1R expression, sex hormones regulate the levels of endocannabinoids. Bradshaw et al. (2006), for example, measured the levels of AEA and 2-AG in several brain areas (i.e., pituitary gland, hypothalamus, thalamus, striatum, midbrain, hippocampus, and cerebellum) in male rats and in females at five different time points along the estrous cycle. They found that AEA content was higher in females than males in both the anterior pituitary gland and hypothalamus (Bradshaw et al., 2006). With the exception of the cerebellum, all brain regions examined revealed significant differences along the estrous cycle in the level of at least one endocannabinoid, with changes occurring predominantly within the 36-h time period surrounding ovulation and behavioral estrus. In general, studies on the regulatory role of sex hormones on the endocannabinoid system failed to provide a clear and linear relationship between the two, and rather showed that these relations are quite complex and depend largely on the specific aspect considered (i.e., receptor affinity or density), the specific endocannabinoid (i.e., AEA or 2-AG) or the brain area investigated (Gorzalka et al., 2010; Gorzalka and Dang, 2012). The interpretation of these findings is further complicated by the fact that (i) all studies performed behavioral testing and/or tissue and serum collection at different time points after gonadectomy, (ii) animals were of different strains and tested at different ages (although they were adult in all studies), and that (iii) animals were kept under hormonal replacement regimen for different periods of time (1 day–3 weeks) before testing. However, the following findings are consistent among studies: (i) higher density of CB1Rs in male hypothalamus and limbic areas coupled, in general, with lower levels of endocannabinoids; (ii) there are significant differences along the hormonal cycle of females, with major changes occurring in the expression of CB1Rs in pituitary gland, hypothalamus and midbrain limbic structures when passing from diestrus to proestrus and behavioral estrus.
Sex steroids, like estrogens, can also regulate the activity of the endocannabinoid metabolizing enzymes. Fatty Acid Amide Hydrolase (FAAH) is the main enzyme involved in the degradation of AEA (Patel et al., 2017). The promoter region of the FAAH gene contains an estrogen binding response element, and translocation of the estrogen receptor to the nucleus results in repression of FAAH transcription in vitro (Waleh et al., 2002) and in vivo (Maccarrone et al., 2000). Ovariectomy prevents the estrogen-induced down-regulation of FAAH expression, and both progesterone and estrogen reduce basal levels of FAAH (Maccarrone et al., 2000). The impact of estrogen-mediated regulation of FAAH activity at behavioral and neurochemical level is still under investigation (Hill et al., 2007).
In humans, plasma AEA levels fluctuate across the menstrual cycle, with a peak at ovulation and the lowest plasma AEA levels observed during the late luteal phase (El-Talatini et al., 2010). In addition, significant positive correlations exist between plasma levels of AEA and plasma levels of estradiol, luteinizing (LH) and follicle-stimulating hormone (FSH) levels (El-Talatini et al., 2010). More recently, brain imaging studies revealed sex differences in the endocannabinoid system. By using positron emission tomography (PET) and the CB1R-selective radioligand [(11)C]OMAR it was shown that CB1R availability is higher in healthy females than in males (Neumeister et al., 2013; Normandin et al., 2015). In addition, it was reported that anandamide levels are lower in females than males (Neumeister et al., 2013). Another study combined PET with the CB1R-selective radioligand [18F]MK-9470 to examine CB1R binding in healthy men and women (van Laere et al., 2008). In this study, CB1R binding was higher in males than in females in all the brain areas investigated and strongly increased with aging in females, suggesting that age-dependent changes in the levels of sex hormones can control CB1R binding in females (van Laere et al., 2008).
While some of the sexual dimorphisms in the brain endocannabinoid system might be permanent, cannabinoid sensitivity is not fixed and can be acutely modulated by hormone-dependent fluctuations of CB1R density, levels of endocannabinoids and of endocannabinoid metabolizing enzymes. Collectively, the hormone-driven sexual dimorphic endocannabinoid system provides a biological basis for sex differences in endocannabinoid-related behaviors, including reward-related behavior (Fattore and Fratta, 2010; Fratta and Fattore, 2013).
Sex Differences in Cannabinoid Action
Numerous studies show sex differences in functions in which the endocannabinoid system is involved, which span from regulation of motivated behaviors, like sex activity (Gorzalka et al., 2010; López, 2010; Androvicova et al., 2017) and food intake (Farhang et al., 2009), to locomotor and exploratory activity (Craft and Leitl, 2008; Craft et al., 2017), nociception (Tseng and Craft, 2001; Craft et al., 2017), working memory (Crane et al., 2013), anxiety (Viveros et al., 2011; Bowers and Ressler, 2016) and vulnerability to develop addictive disorders (Fattore et al., 2014; Marusich et al., 2014; Becker, 2016). Endocannabinoids are also directly involved in the anxiolytic effects of estrogen; in turn, estrogen may elicit changes in emotional behavior through an endocannabinoid mechanism (Hill et al., 2007).
Sexual maturation takes place under hormonal control during puberty and adolescence. Due to the deep changes occurring during these periods of life, individuals of both sexes are particularly (although differentially) sensitive to many stimuli, vulnerable toward the development of psychopathological conditions and more prone to abuse drugs, including cannabis (Wiley and Burston, 2014; Silva et al., 2016; Wagner, 2016). Exposure to cannabinoids during critical developmental periods alters several functions in adult animals (Schneider, 2008; Rubino and Parolaro, 2016), including working (Schneider and Koch, 2003; O’Shea et al., 2004) and spatial memory (Rubino et al., 2009), sensorimotor gating (Schneider and Koch, 2003), anxiety and anxiolytic-like responses (Biscaia et al., 2003; O’Shea et al., 2004; Viveros et al., 2005b), anhedonia, depressive-like states (Schneider and Koch, 2003; Rubino et al., 2008) and sexual behavior (Chadwick et al., 2011). Long-term alterations induced by cannabinoids in the developing organism are well known (Gupta and Elbracht, 1983; Navarro et al., 1994; Pistis et al., 2004; Viveros et al., 2005a; Spano et al., 2006; Ellgren et al., 2007; Renard et al., 2014; Rubino and Parolaro, 2016; Melas et al., 2018) and recent reports are pointing out epigenetic mechanisms underlying cannabis action (Szutorisz and Hurd, 2016, 2018; Prini et al., 2017). Yet, researchers started only recently to unravel sexually dimorphic long-term effects of early cannabinoid exposure on behavior, cognition and emotional states (Viveros et al., 2011; Lee et al., 2014; Keeley et al., 2015a,b).
For instance, the ability of sex hormones to affect cannabinoid self-administration was established only recently. Such a delay is probably due to the fact that human cannabis use is extremely difficult to model in laboratory animals (Panlilio et al., 2010) and that the development of reliable protocols of cannabinoid self-administration in mice (Martellotta et al., 1998), rats (Fattore et al., 2001) and monkeys (Justinova et al., 2003) has taken long time and efforts. Importantly, these models made it possible to investigate factors that modulate spontaneous cannabinoid intake in animals, including strain (Deiana et al., 2007) and sex (Fattore et al., 2007, 2010). Notably, female rats are able to discriminate THC from vehicle at a lower dose and faster rate than male rats (Wiley et al., 2017), although no significant sex differences were observed in the cannabinoid place preference test (Hempel et al., 2017). Moreover, ovarian hormones were identified as important modulators of cannabinoid self-administration, since bilateral ovariectomy significantly reduced drug-taking and drug-seeking in female rats (Fattore et al., 2007, 2010). Unfortunately, which specific sex hormone is able to finely modulate cannabinoid intake is still uncertain, highlighting the need for studies that combine gonadectomy with hormone replacement.
The effects of hormonal fluctuation during the menstrual cycle on the responses to drugs of abuse have been consistently investigated (Terner and de Wit, 2006; Carroll et al., 2015; Weinberger et al., 2015). Yet, the influence of sex hormones and menstrual cycle on the subjective and objective effects of marijuana has only been occasionally studied in female smokers. For example, Griffin et al. (1986) found no effect of the specific phase of the menstrual cycle on marijuana intake, a finding consistent with the negative results reported by Lex et al. (1984) which monitored cannabis-induced changes in pulse rate and mood in women during the follicular, ovulatory and luteal phases of the cycle. These earlier studies, however, failed to detect strong hormonal-dependent effects of marijuana intake along the menstrual cycle, and more controlled studies are needed before reaching any definitive conclusion on hormonal influences on cannabinoid use and sensitivity.
(Endo)Cannabinoids, Sex Hormones and Dopamine
Sex hormones have been found to be important modulators of several drugs of abuse (Lynch et al., 2000; Carroll et al., 2004; Fattore et al., 2007, 2008; Lynch, 2008; Carroll and Lynch, 2016; Swalve et al., 2016). Estradiol and progesterone rapidly induce changes in dopaminergic signaling within the dorsal striatum and nucleus accumbens of female rats (Becker, 1999), effects that are important for the regulation of normal physiological states and relevant reproductive behaviors (Yoest et al., 2018). While the enhancing effect of ovarian hormones on drug craving has been traditionally attributed to estrogens (even in view of their ability to elicit direct dopamine release in the brain), it was suggested that progesterone, rather than estradiol, is responsible for the reducing effect on drug-seeking behavior (Feltenstein and See, 2007; Feltenstein et al., 2009; Carroll and Lynch, 2016).
As discussed above, brain CB1R distribution, synthesis of endogenous cannabinoids and activity of enzymes involved in cannabinoid metabolism (turnover) are significantly affected by sex hormones. At systems level, hormone-dependent differences and fluctuations in cannabinoid function may directly affect the activity of brain neurotransmitters and structures involved in cognitive and emotional aspects of motivated behaviors (Schultz, 1997; Berridge and Robinson, 1998; Ikemoto and Panksepp, 1999; Salamone and Correa, 2002; Goto and Grace, 2005; Cheng and Feenstra, 2006; Di Chiara and Bassareo, 2007; Berridge et al., 2009), like feeding (Melis et al., 2007; Bassareo et al., 2015; Fois et al., 2016; Coccurello and Maccarrone, 2018; Contini et al., 2018) and sexual behavior (Pfaus et al., 1990; Pfaus and Everitt, 1995; Sanna et al., 2015, 2017) as well as psychopathological states (Dunlop and Nemeroff, 2007; Maia and Frank, 2017) and addiction-like behaviors (Everitt and Robbins, 2005, 2016). Such a modulation can happen (i) by a direct interaction of the cannabinoid system with the mesolimbic dopaminergic system, the core component of the neurobiological substrates at the basis of motivated behavior (Gardner, 2005; Fadda et al., 2006; Lecca et al., 2006; Zangen et al., 2006; Melis and Pistis, 2007; Panagis et al., 2014; Bloomfield et al., 2016; Maldonado et al., 2006), or (ii) by indirect actions in limbic areas (e.g., hippocampus, amygdala, PFC) strictly interconnected with mesolimbic dopaminergic neurons through (mainly) glutamatergic projections to the ventral tegmental area and nucleus accumbens (Laviolette and Grace, 2006; Laviolette, 2017). Sex hormones can modulate cannabinoid influence on motivated behaviors and stress responses by acting also at the level of several hypothalamic nuclei (Cota, 2008).
The leading hypothesis that sex steroids and (endo)cannabinoid actions can converge on the dopaminergic mesolimbic system to regulate important motivational aspects in a sexually dimorphic manner deserves further confirmation. To date, it explains interactions of cannabinoids and sex hormones only at the level of specific brain systems, while most of the information at molecular and genetic/epigenetic level are still missing, although initial efforts in this direction have begun to fill the gap (see for example Mani et al., 2001; Gammon et al., 2005; Szutorisz and Hurd, 2016, 2018; Prini et al., 2017; Rosas et al., 2018). Furthermore, this hypothesis takes into account almost exclusively the cannabinoid effects mediated by central CB1Rs, but CB2Rs may also play a part through their actions on brain dopamine systems (Liu et al., 2017). The importance of sex hormones in modulating drug sensitivity is further supported by studies that have shown a clear association between exposure to synthetic male steroids and drug sensitivity.
Anabolic-Androgenic Steroids and Cannabinoid Action
Anabolic-androgenic steroids are synthetic derivatives of the male hormone testosterone and are used therapeutically for the treatment of various diseases including hypogonadism, angioedema, anemia, osteoporosis, and muscle wasting (Basaria et al., 2001). Non-medical use of AAS is often observed among professional and non-professional athletes in order to improve physical appearance and enhance performance (Sagoe et al., 2014). Global lifetime prevalence rate of non-medical AAS use is estimated to be 3.3% (Sagoe et al., 2014). AAS doses used for non-medical purposes are typically much higher (10–100×) than doses for medical use and are associated with several physical and psychological side effects (Hartgens and Kuipers, 2004). Physical side effects that have been observed after use of AAS include infertility, baldness, breast development, severe acne, high blood pressure, blood clots, heart attack, and stroke (Hartgens and Kuipers, 2004). Possible psychological consequences of AAS use are increased aggression, anxiety, and depression (Hartgens and Kuipers, 2004). Clinical and epidemiological data show that AAS are often co-abused with addictive substances, including cannabis (DuRant et al., 1995; Arvary and Pope, 2000; Kanayama et al., 2003). Several reasons might explain why polypharmacy occurs in more than 95% AAS users (Parkinson and Evans, 2006). First, AAS users are known to take other drugs to counteract adverse side but they might also have a higher sensitivity toward substance abuse. Alternatively, AAS might have direct effects on various components of the brain reward system which alters the sensitivity of users toward other drugs of abuse.
Use of high doses of AAS can lead to addiction, which makes it conceivable that AAS are able to modulate the brain reward system (Kanayama et al., 2009). Although part of the rewarding effects of AAS might be derived from their effects on physical appearance, animal studies have shown that testosterone and other AAS can induce conditioned place preference in a dopamine receptor-dependent manner (Arnedo et al., 2000; Schroeder and Packard, 2000; Parrilla-Carrero et al., 2009) and increase self-administration behavior (Clark et al., 1996; Wood, 2004). In addition to their effects on reward-related behavior, AAS cause molecular and neurochemical changes in the dopaminergic, serotonergic and opioid neurotransmitter systems (Johansson et al., 1997; Kindlundh et al., 2001; Zotti et al., 2014) and alter the behavioral effects of different types of drugs (Kurling, 2008; Kurling-Kailanto, 2010; Kailanto, 2011).
Studies investigating the effects of AAS exposure on cannabinoid sensitivity are scarce at present. It was shown that testosterone significantly reduces THC-induced locomotor suppression or catalepsy in gonadectomized males (Craft and Leitl, 2008; Craft et al., 2017) and that chronic exposure to nandrolone, a derivative of testosterone also known as 19-nortestosterone (Figure 2), blocked THC-induced conditioned place preference in rats (Célérier et al., 2006). Further, we recently reported that chronic treatment of rats with nandrolone does not alter CB1R levels or function in several reward-related brain areas. However, when chronic nandrolone treatment is followed by cannabinoid self-administration, we observed a strong decrease in CB1R function in the hippocampus and a significant increase in cannabinoid intake (Struik et al., 2017). Given the profound effects that AAS have on various aspects of the molecular machinery of the brain reward system, it might come as no surprise that AAS also interfere with the rewarding properties of drugs of abuse, including cannabinoids.
Altogether, studies available so far suggest that AAS can have a repressing effect on the brain reward system, a notion that is strengthened by the observation that AAS reduce drug-induced neurochemical and behavioral effects of amphetamine, MDMA, THC, and cocaine, and increase voluntary alcohol and cannabinoid drug intake (Mhillaj et al., 2015). The hypothesized AAS-induced suppression of the reward system might result in the use of higher doses of drugs, which is associated with a higher addiction risk. It would be intriguing to find out to what extent blockade of steroid hormone activity contributes to prevent the repressive effect of these hormones in the reward system. Further studies are needed also to assess whether or not AAS can act as gateway drugs and lead to CUD and to better understand how they can impinge upon the endocannabinoid signaling within the brain.
Conclusion
Cannabis is the most commonly used illicit drug worldwide and its use is associated with multiple adverse health effect including the risk of addiction. Identifying factors involved in cannabis use and abuse is critical for optimizing evidence-based prevention and treatment protocols. Similarly to other drugs of abuse, the prevalence of cannabis use and addiction differs between males and females, suggesting that sex is an important modulator of cannabinoid sensitivity. Accumulating evidence shows that the endocannabinoid system is sexually dimorphic and that sex hormones play a key role. Hormone-driven differentiation of the endocannabinoid system seems to provide a biological basis for sex differences in endocannabinoid-related behaviors, including reward-related behaviors. While sex differences in cannabinoid action are being increasingly studied in animals, controlled human studies are still limited. The endocannabinoid system is, for its intrinsic characteristics, a privileged target of the actions of both sex and anabolic-androgenic steroid hormones at different levels and, in turn, it can modulate the activity of sex hormones (Table 1). The observation that exposure to AAS causes dysfunction of the brain reward pathway in rats points to a potential risk factor for initiation of cannabis use, maintenance of regular use and development of CUD. The cross talk between endocannabinoid signaling and steroid hormones can occur differently in males and females, and many questions about underlying mechanisms remain unanswered, demanding further research in the field in an attempt to elucidate the basis of the sex differences often observed in cannabinoid sensitivity.
Author Contributions
DS has developed the original idea and wrote the Introduction and the parts related to the risk factors for cannabis use and anabolic-androgenic steroids. FS wrote the parts related to sexual behavior, gonadal hormones, and dopamine-cannabinoid interactions. LF has developed the original idea, wrote the parts related to brain sexual dimorphisms and sex/gender differences and coordinated the work structuring of the different parts. All authors have approved the final version of the review.
Conflict of Interest Statement
The authors declare that the research was conducted in the absence of any commercial or financial relationships that could be construed as a potential conflict of interest.
References
Agrawal, A., and Lynskey, M. T. (2006). The genetic epidemiology of cannabis use, abuse and dependence. Addiction 101, 801–812. doi: 10.1111/j.1360-0443.2006.01399.x
Agrawal, A., Lynskey, M. T., Hinrichs, A., Grucza, R., Saccone, S. F., Krueger, R., et al. (2011). A genome-wide association study of DSM-IV cannabis dependence. Addict. Biol. 16, 514–518. doi: 10.1111/j.1369-1600.2010.00255.x
American Psychiatric and Association (2013). Diagnostic and Statistical Manual of Mental Disorders, 5th Edn. Washington, DC: American Psychiatric and Association.
Androvicova, R., Horacek, J., Stark, T., Drago, F., and Micale, V. (2017). Endocannabinoid system in sexual motivational processes: Is it a novel therapeutic horizon? Pharmacol. Res. 115, 200–208. doi: 10.1016/j.phrs.2016.11.021
Antinori, S., and Fattore, L. (2017). “How CB1 receptor activity and distribution contribute to make the male and female brain different toward cannabinoid-induced effects,” in Endocannabinoids and Lipid Mediators in Brain Functions, ed. M. Melis (Berlin: Springer), 27–51.
Argiolas, A., and Melis, M. R. (2013). Neuropeptides and central control of sexual behavior from the past to the present: a review. Prog. Neurobiol. 108, 80–107. doi: 10.1016/j.pneurobio.2013.06.006
Arnedo, M. T., Salvador, A., Martinez-Sanchis, S., and Gonzalez-Bono, E. (2000). Rewarding properties of testosterone in intact male mice: a pilot study. Pharmacol. Biochem. Behav. 65, 327–332. doi: 10.1016/S0091-3057(99)00189-6
Arnold, A. P., and Breedlove, S. M. (1985). Organizational and activational effects of sex steroids on brain and behavior: a reanalysis. Horm. Behav. 19, 469–498. doi: 10.1016/0018-506X(85)90042-X
Arvary, D., and Pope, H. G. Jr. (2000). Anabolic-androgenic steroids as a gateway to opioid dependence. N. Engl. J. Med. 342:1532. doi: 10.1056/NEJM200005183422018
Ayalon, D., Nir, I., Cordova, T., Bauminger, S., Puder, M., Naor, Z., et al. (1977). Acute effect of delta1-tetrahydrocannabinol on the hypothalamo-pituitary-ovarian axis in the rat. Neuroendocrinology 23, 31–42. doi: 10.1159/000122652
Basaria, S., Wahlstrom, J. T., and Dobs, A. S. (2001). Clinical review 138: anabolic-androgenic steroid therapy in the treatment of chronic diseases. J. Clin. Endocrinol. Metab. 86, 5108–5117. doi: 10.1210/jcem.86.11.7983
Bassareo, V., Cucca, F., Frau, R., and Di Chiara, G. (2015). Differential activation of accumbens shell and core dopamine by sucrose reinforcement with nose poking and with lever pressing. Behav. Brain. Res. 294, 215–223. doi: 10.1016/j.bbr.2015.08.006
Beatty, W. W. (1979). Gonadal hormones and sex differences in non-reproductive behaviors in rodents: organizational and activational influences. Horm. Behav. 12, 112–163. doi: 10.1016/0018-506X(79)90017-5
Becker, J. B. (1999). Gender differences in dopaminergic function in striatum and nucleus accumbens. Pharmacol. Biochem. Behav. 64, 803–812. doi: 10.1016/S0091-3057(99)00168-9
Becker, J. B. (2009). Sexual differentiation of motivation: a novel mechanism? Horm. Behav. 55, 646–654. doi: 10.1016/j.yhbeh.2009.03.014
Becker, J. B., and Hu, M. (2008). Sex differences in drug abuse. Front. Neuroendocrinol. 29, 36–47. doi: 10.1016/j.yfrne.2007.07.003
Berridge, K. C., and Robinson, T. E. (1998). What is the role of dopamine in reward: hedonic impact, reward learning, or incentive salience? Brain Res. Rev. 28, 309–369. doi: 10.1016/S0165-0173(98)00019-8
Berridge, K. C., Robinson, T. E., and Aldridge, J. W. (2009). Dissecting components of reward: ’liking’, ’wanting’, and learning. Curr. Opin. Pharmacol. 9, 65–73. doi: 10.1016/j.coph.2008.12.014
Besch, N. F., Smith, C. G., Besch, P. K., and Kaufman, R. H. (1977). The effect of marihuana (delta- 9-tetrahydrocannabinol) on the secretion of luteinizing hormone in the ovariectomized rhesus monkey. Am. J. Obstet. Gynecol. 128, 635–642. doi: 10.1016/0002-9378(77)90209-5
Biscaia, M., Marín, S., Fernández, B., Marco, E. M., Rubio, M., Guaza, C., et al. (2003). Chronic treatment with CP 55,940 during the peri-adolescent period differentially affects the behavioural responses of male and female rats in adulthood. Psychopharmacology 170, 301–308. doi: 10.1007/s00213-003-1550-7
Bloomfield, M. A., Ashok, A. H., Volkow, N. D., and Howes, O. D. (2016). The effects of Δ9-tetrahydrocannabinol on the dopamine system. Nature 539, 369–377. doi: 10.1038/nature20153
Bookout, A. L., Jeong, Y., Downes, M., Yu, R. T., Evans, R. M., and Mangelsdorf, D. J. (2006). Anatomical profiling of nuclear receptor expression reveals a hierarchical transcriptional network. Cell 126, 789–799. doi: 10.1016/j.cell.2006.06.049
Borgquist, A., Meza, C., and Wagner, E. J. (2015). The role of AMP-activated protein kinase in the androgenic potentiation of cannabinoid-induced changes in energy homeostasis. Am. J. Physiol. Endocrinol. Metab. 308, E482–E495. doi: 10.1152/ajpendo.00421.2014
Bowers, M. E., and Ressler, K. J. (2016). Sex-dependence of anxiety-like behavior in cannabinoid receptor 1 (Cnr1) knockout mice. Behav. Brain Res. 300, 65–69. doi: 10.1016/j.bbr.2015.12.005
Bradshaw, H. B., Rimmerman, N., Krey, J. F., and Walker, J. M. (2006). Sex and hormonal cycle differences in rat brain levels of pain-related cannabimimetic lipid mediators. Am. J. Physiol. Regul. Integr. Comp. Physiol. 291, R349–R358. doi: 10.1152/ajpregu.00933.2005
Brown, T. T., and Dobs, A. S. (2002). Endocrine effects of marijuana. J. Clin. Pharmacol 42, 90S–96S. doi: 10.1002/j.1552-4604.2002.tb06008.x
Budney, A. J., Roffman, R., Stephens, R. S., and Walker, D. (2007). Marijuana dependence and its treatment. Addict. Sci. Clin. Pract. 4, 4–16. doi: 10.1151/ASCP07414
Busardò, F. P., Frati, P., Sanzo, M. D., Napoletano, S., Pinchi, E., Zaami, S., et al. (2015). The impact of nandrolone decanoate on the central nervous system. Curr. Neuropharmacol. 13, 122–131. doi: 10.2174/1570159X13666141210225822
Busquets-Garcia, A., Soria-Gómez, E., Redon, B., Mackenbach, Y., Vallée, M., Chaouloff, F., et al. (2017). Pregnenolone blocks cannabinoid-induced acute psychotic-like states in mice. Mol. Psychiatry 22, 1594–1603. doi: 10.1038/mp.2017.4
Carroll, H. A., Lustyk, M. K., and Larimer, M. E. (2015). The relationship between alcohol consumption and menstrual cycle: a review of the literature. Arch. Womens Ment. Health 18, 773–781. doi: 10.1007/s00737-015-0568-2
Carroll, M. E., and Lynch, W. J. (2016). How to study sex differences in addiction using animal models. Addict. Biol. 21, 1007–1029. doi: 10.1111/adb.12400
Carroll, M. E., Lynch, W. J., Roth, M. E., Morgan, A. D., and Cosgrove, K. P. (2004). Sex and estrogen influence drug abuse. Trends Pharmacol. Sci. 25, 273–279. doi: 10.1016/j.tips.2004.03.011
Castelli, M. P., Fadda, P., Casu, A., Spano, M. S., Casti, A., Fratta, W., et al. (2014). Male and female rats differ in brain cannabinoid CB1 receptor density and function and in behavioural traits predisposing to drug addiction: effect of ovarian hormones. Curr. Pharm. Des. 20, 2100–2113. doi: 10.2174/13816128113199990430
Célérier, E., Ahdepil, T., Wikander, H., Berrendero, F., Nyberg, F., and Maldonado, R. (2006). Influence of the anabolic-androgenic steroid nandrolone on cannabinoid dependence. Neuropharmacology 50, 788–806. doi: 10.1016/j.neuropharm.2005.11.017
Chadwick, B., Saylor, A. J., and López, H. H. (2011). Adolescent cannabinoid exposure attenuates adult female sexual motivation but does not alter adulthood CB1R expression or estrous cyclicity. Pharmacol. Biochem. Behav. 100, 157–164. doi: 10.1016/j.pbb.2011.07.006
Chakravarty, I., Sheth, A. R., and Ghosh, J. J. (1975). Effect of acute delta9-tetrahydrocannabinol treatment on serum luteinizing hormone and prolactin levels in adult female rats. Fertil. Steril. 26, 947–948. doi: 10.1016/S0015-0282(16)41364-6
Chakravarty, I., Sheth, P. R., Sheth, A. R., and Ghosh, J. J. (1982). Delta-9-tetrahydrocannabinol: its effect on hypothalamo-pituitary system in male rats. Arch. Androl. 8, 25–27. doi: 10.3109/01485018208987014
Cheng, J. J., and Feenstra, G. P. (2006). Individual differences in dopamine efflux in nucleus accumbens shell and core during instrumental learning. Learn. Mem. 13, 168–177. doi: 10.1101/lm.1806
Clark, A. S., Lindenfeld, R. C., and Gibbons, C. H. (1996). Anabolic-androgenic steroids andbrain reward. Pharmacol. Biochem. Behav. 53, 741–745. doi: 10.1016/0091-3057(95)02082-9
Coccurello, R., and Maccarrone, M. (2018). Hedonic eating and the “Delicious Circle”: from lipid-derived mediators to brain dopamine and back. Front. Neurosci. 12:271. doi: 10.3389/fnins.2018.00271
Contini, A., Sanna, F., Maccioni, P., Colombo, G., and Argiolas, A. (2018). Comparison between male and female rats in a model of self-administration of a chocolate-flavored beverage: Behavioral and neurochemical studies. Behav. Brain Res. 344, 28–41. doi: 10.1016/j.bbr.2018.02.004
Cooper, Z. D., and Craft, R. M. (2017). Sex-dependent effects of cannabis and cannabinoids: a translational perspective. Neuropsychopharmacology 43, 34–51. doi: 10.1038/npp.2017.140
Cooper, Z. D., and Haney, M. (2014). Investigation of sex-dependent effects of cannabis in daily cannabis smokers. Drug Alcohol Depend. 136, 85–91. doi: 10.1016/j.drugalcdep.2013.12.013
Cota, D. (2008). The role of the endocannabinoid system in the regulation of hypothalamic-pituitary-adrenal axis activity. J. Neuroendocrinol. 20, 35–38. doi: 10.1111/j.1365-2826.2008.01673.x
Courtney, K. E., Mejia, M. H., and Jacobus, J. (2017). Longitudinal studies on the etiology of cannabis use disorder: a review. Curr. Addict. Rep. 4, 43–52. doi: 10.1007/s40429-017-0133-3
Craft, R. M., Haas, A. E., Wiley, J. L., Yu, Z., and Clowers, B. H. (2017). Gonadal hormone modulation of Δ9-tetrahydrocannabinol-induced antinociception and metabolism in female versus male rats. Pharmacol. Biochem. Behav. 152, 36–43. doi: 10.1016/j.pbb.2016.09.006
Craft, R. M., and Leitl, M. D. (2008). Gonadal hormone modulation of the behavioral effects of Delta9-tetrahydrocannabinol in male and female rats. Eur. J. Pharmacol. 578, 37–42. doi: 10.1016/j.ejphar.2007.09.004
Craft, R. M., Marusich, J. A., and Wiley, J. L. (2013). Sex differences in cannabinoid pharmacology: a reflection of differences in the endocannabinoid system? Life Sci. 92, 476–481. doi: 10.1016/j.lfs.2012.06.009
Crane, N. A., Schuster, R. M., Fusar-Poli, P., and Gonzalez, R. (2013). Effects of cannabis on neurocognitive functioning: recent advances, neurodevelopmental influences, and sex differences. Neuropsychol. Rev. 23, 117–137. doi: 10.1007/s11065-012-9222-1
Cuttler, C., Mischley, L. K., and Sexton, M. (2016). Sex differences in cannabis use and effects: a cross-sectional survey of cannabis users. Cannabis Cannabinoid. Res. 1, 166–175. doi: 10.1089/can.2016.0010
Dalterio, S., Mayfield, D. L., and Bartke, A. (1983). Effects of delta-9-THC on plasma hormone levels in female mice. Subst. Alcohol Actions Misuse 4, 339–345.
Davis, C., and Fattore, L. (2015). “Gender differences in cannabinoid addiction and dependence,” in Cannabinoid Modulation of Emotion, Memory, and Motivation, eds P. Campolongo, and L. Fattore (Berlin: Springer), 283–325. doi: 10.1007/978-1-4939-2294-9_12
De Luca, M. A., and Fattore, L. (2018). Therapeutic use of synthetic cannabinoids: still an open issue? Clin. Ther. doi: 10.1016/j.clinthera.2018.08.002 [Epub ahead of print].
Deiana, S., Fattore, L., Spano, M. S., Cossu, G., Porcu, E., Fadda, P., et al. (2007). Strain and schedule-dependent differences in the acquisition, maintenance and extinction of intravenous cannabinoid self-administration in rats. Neuropharmacology 52, 646–654. doi: 10.1016/j.neuropharm.2006.09.007
Di Chiara, G., and Bassareo, V. (2007). Reward system and addiction: what dopamine does and doesn’t do. Curr. Opin. Pharmacol. 7, 69–76. doi: 10.1016/j.coph.2006.11.003
Distel, M. A., Vink, J. M., Bartels, M., van Beijsterveldt, C. E., Neale, M. C., and Boomsma, D. I. (2011). Age moderates non-genetic influences on the initiation of cannabis use: a twin-sibling study in Dutch adolescents and young adults. Addiction 106, 1658–1666. doi: 10.1111/j.1360-0443.2011.03465.x
Dunlop, B. W., and Nemeroff, C. B. (2007). The role of dopamine in the pathophysiology of depression. Arch. Gen. Psychiatry 64, 327–337. doi: 10.1001/archpsyc.64.3.327
DuRant, R. H., Escobedo, L. G., and Heath, G. W. (1995). Anabolic-steroid use, strength training, and multiple drug use among adolescents in the United States. Pediatrics 96, 23–28.
Ellgren, M., Spano, S. M., and Hurd, Y. L. (2007). Adolescent cannabis exposure alters opiate intake and opioid limbic neuronal populations in adult rats. Neuropsychopharmacology 32, 607–615. doi: 10.1038/sj.npp.1301127
El-Talatini, M. R., Taylor, A. H., and Konje, J. C. (2010). The relationship between plasma levels of the endocannabinoid, anandamide, sex steroids, and gonadotrophins during the menstrual cycle. Fertil. Steril. 93, 1989–1996. doi: 10.1016/j.fertnstert.2008.12.033
European Monitoring Centre for Drugs and Drug Addiction [EMCDDA] (2005). Differences in Patterns of Drug Use. (between) Women and Men 2005. Lisbon: EMCDDA.
European Monitoring Centre for Drugs and Drug Addiction [EMCDDA] (2013). Perspectives on Drugs: Synthetic Cannabinoids. (in) Europe. Luxembourg: Publications Office of the European Union.
European Monitoring Centre for Drugs and Drug Addiction [EMCDDA] (2017). European Drug Report 2017: Trends. (and) Developments. Luxembourg: Publications Office of the European Union.
Everitt, B. J., and Robbins, T. W. (2005). Neural systems of reinforcement for drug addiction: from actions to habits to compulsion. Nat. Neurosci. 8, 1481–1489. doi: 10.1038/nn1579
Everitt, B. J., and Robbins, T. W. (2016). Drug addiction: updating actions to habits to compulsions ten years on. Annu. Rev. Psychol. 67, 23–50. doi: 10.1146/annurev-psych-122414-033457
Fadda, P., Scherma, M., Spano, M. S., Salis, P., Melis, V., Fattore, L., et al. (2006). Cannabinoid self-administration increases dopamine release in the nucleus accumbens. Neuroreport 17, 1629–1632. doi: 10.1097/01.wnr.0000236853.40221.8e
Farhang, B., Diaz, S., Tang, S. L., and Wagner, E. J. (2009). Sex differences in the cannabinoid regulation of energy homeostasis. Psychoneuroendocrinology 34, S237–S246. doi: 10.1016/j.psyneuen.2009.04.007
Fattore, L. (2013). Considering gender in cannabinoid research: a step towards personalized treatment of marijuana addicts. Drug Test Anal. 5, 57–61. doi: 10.1002/dta.1401
Fattore, L. (2016). Synthetic cannabinoids-further evidence supporting the relationship between cannabinoids and psychosis. Biol. Psychiatry 79, 539–548. doi: 10.1016/j.biopsych.2016.02.001
Fattore, L., Altea, S., and Fratta, W. (2008). Sex differences in drug addiction: a review of animal and human studies. Womens Health 4, 51–65. doi: 10.2217/17455057.4.1.51
Fattore, L., Cossu, G., Martellotta, M. C., and Fratta, W. (2001). Intravenous self-administration of the cannabinoid CB1 receptor agonist WIN 55,212-2 in rats. Psychopharmacology 156, 410–416. doi: 10.1007/s002130100734
Fattore, L., and Fratta, W. (2010). How important are sex differences in cannabinoid action? Br. J. Pharmacol. 160, 544–548. doi: 10.1111/j.1476-5381.2010.00776.x
Fattore, L., and Fratta, W. (2011). Beyond THC: the new generation of cannabinoid designer drugs. Front. Behav. Neurosci. 5:60. doi: 10.3389/fnbeh.2011.00060
Fattore, L., Melis, M., Fadda, P., and Fratta, W. (2014). Sex differences in addictive disorders. Front. Neuroendocrinol. 35, 272–284. doi: 10.1016/j.yfrne.2014.04.003
Fattore, L., Spano, M. S., Altea, S., Angius, F., Fadda, P., and Fratta, W. (2007). Cannabinoid self-administration in rats: sex differences and the influence of ovarian function. Br. J. Pharmacol. 152, 795–804. doi: 10.1038/sj.bjp.0707465
Fattore, L., Spano, M. S., Altea, S., Fadda, P., and Fratta, W. (2010). Drug- and cue-induced reinstatement of cannabinoid-seeking behaviour in male and female rats: influence of ovarian hormones. Br. J. Pharmacol. 160, 724–735. doi: 10.1111/j.1476-5381.2010.00734.x
Feltenstein, M. W., Byrd, E. A., Henderson, A. R., and See, R. E. (2009). Attenuation of cocaine-seeking by progesterone treatment in female rats. Psychoneuroendocrinology 34, 343–352. doi: 10.1016/j.psyneuen.2008.09.014
Feltenstein, M. W., and See, R. E. (2007). Plasma progesterone levels and cocaine-seeking in freely cycling female rats across the estrous cycle. Drug Alcohol Depend. 89, 183–189. doi: 10.1016/j.drugalcdep.2006.12.017
Fois, G. R., Fattore, L., Murineddu, G., Salis, A., Pintore, G., Asproni, B., et al. (2016). The novel cannabinoid antagonist SM-11 reduces hedonic aspect of food intake through a dopamine-dependent mechanism. Pharmacol. Res. 113, 108–115. doi: 10.1016/j.phrs.2016.08.012
Fratta, W., and Fattore, L. (2013). Molecular mechanisms of cannabinoid addiction. Curr. Opin. Neurobiol. 23, 487–492. doi: 10.1016/j.conb.2013.02.002
Freeman, T. P., and Winstock, A. R. (2015). Examining the profile of high-potency cannabis and its association with severity of cannabis dependence. Psychol. Med. 45, 3181–3189. doi: 10.1017/S0033291715001178
Freund, T. F., Katona, I., and Piomelli, D. (2003). Role of endogenous cannabinoids in synaptic signaling. Physiol. Rev. 83, 1017–1066. doi: 10.1152/physrev.00004.2003
Gammon, C. M., Freeman, G. M. Jr., Xie, W., Petersen, S. L., and Wetsel, W. C. (2005). Regulation of gonadotropin-releasing hormone secretion by cannabinoids. Endocrinology 146, 4491–4499. doi: 10.1210/en.2004-1672
Gardner, E. L. (2005). Endocannabinoid signaling system and brain reward: emphasis on dopamine. Pharmacol. Biochem. Behav. 81, 263–284. doi: 10.1016/j.pbb.2005.01.032
Gelernter, J., Panhuysen, C., Wilcox, M., Hesselbrock, V., Rounsaville, B., Poling, J., et al. (2006). Genomewide linkage scan for opioid dependence and related traits. Am. J. Hum. Genet. 78, 759–769. doi: 10.1086/503631
Gerra, M. C., Jayanthi, S., Manfredini, M., Walther, D., Schroeder, J., Phillips, K. A., et al. (2018). Gene variants and educational attainment in cannabis use: mediating role of DNA methylation. Transl. Psychiatry 8:23. doi: 10.1038/s41398-017-0087-1
González, S., Bisogno, T., Wenger, T., Manzanares, J., Milone, A., Berrendero, F., et al. (2000). Sex steroid influence on cannabinoid CB(1) receptor mRNA and endocannabinoid levels in the anterior pituitary gland. Biochem. Biophys. Res. Commun. 270, 260–266. doi: 10.1006/bbrc.2000.2406
Gordon, J. H., Bromley, B. L., Gorski, R. A., and Zimmermann, E. (1978). Delta9-tetrahydrocannahinol enhancement of lordosis behavior in estrogen treated female rats. Pharmacol. Biochem. Behav. 8, 603–608. doi: 10.1016/0091-3057(78)90395-7
Gorzalka, B. B., and Dang, S. S. (2012). Minireview: endocannabinoids and gonadal hormones: bidirectional interactions in physiology and behavior. Endocrinology 153, 1016–1024. doi: 10.1210/en.2011-1643
Gorzalka, B. B., Hill, M. N., and Chang, S. C. (2010). Male-female differences in the effects of cannabinoids on sexual behavior and gonadal hormone function. Horm. Behav. 58, 91–99. doi: 10.1016/j.yhbeh.2009.08.009
Goto, Y., and Grace, A. A. (2005). Dopaminergic modulation of limbic and cortical drive of nucleus accumbens of goal-directed behavior. Nat. Neurosci. 8, 805–812. doi: 10.1038/nn1471
Griffin, M. L., Mendelson, J. H., Mello, N. K., and Lex, B. W. (1986). Marihuana use across the menstrual cycle. Drug Alcohol Depend. 18, 213–224. doi: 10.1016/0376-8716(86)90053-0
Gupta, D., and Elbracht, C. (1983). Effect of tetrahydrocannabinols on pubertal body weight spurt and sex hormones in developing male rats. Res. Exp. Med. 182, 95–104. doi: 10.1007/BF01851115
Hall, W., and Degenhardt, L. (2009). Adverse health effects of non-medical cannabis use. Lancet 374, 1383–1391. doi: 10.1016/S0140-6736(09)61037-0
Hanukoglu, I. (1992). Steroidogenic enzymes: structure, function, and role in regulation of steroid hormone biosynthesis. J. Steroid. Biochem. Mol. Biol. 43, 779–804. doi: 10.1016/0960-0760(92)90307-5
Hartgens, F., and Kuipers, H. (2004). Effects of androgenic-anabolic steroids in athletes. Sports Med. 34, 513–554. doi: 10.2165/00007256-200434080-00003
Haug, S., Núñez, C. L., Becker, J., Gmel, G., and Schaub, M. P. (2014). Predictors of onset of cannabis and other drug use in male young adults: results from a longitudinal study. BMC Public Health 14:1202. doi: 10.1186/1471-2458-14-1202
Hempel, B. J., Wakeford, A. G., Nelson, K. H., Clasen, M. M., Woloshchuk, C. J., and Riley, A. L. (2017). An assessment of sex differences in Δ9-tetrahydrocannabinol (THC) taste and place conditioning. Pharmacol. Biochem. Behav. 153, 69–75. doi: 10.1016/j.pbb.2016.11.006
Hill, M. N., Karacabeyli, E. S., and Gorzalka, B. B. (2007). Estrogen recruits the endocannabinoid system to modulate emotionality. Psychoneuroendocrinology 32, 350–357. doi: 10.1016/j.psyneuen.2007.02.003
Ho, B. T., Fritchie, G. E., Kralik, P. M., Englert, L. F., Mclsaac, W. M., and Idanpaan-Heikkila, J. (1970). Distribution of tritiated l-delta-9-tetrahydrocannahinol in rat tissues after inhalation. J. Pharm. Pharmacol. 22, 538–539. doi: 10.1111/j.2042-7158.1970.tb10563.x
Hopfer, C. (2014). Implications of marijuana legalization for adolescent substance use. Subst. Abus. 35, 331–335. doi: 10.1080/08897077.2014.943386
Howlett, A. C., Breivogel, C. S., Childers, S. R., Deadwyler, S. A., Hampson, R. E., and Porrino, L. J. (2004). Cannabinoid physiology and pharmacology: 30 years of progress. Neuropharmacology 47, 345–358. doi: 10.1016/j.neuropharm.2004.07.030
Hull, E. M., Lorrain, D. S., Du, J., Matuszewich, L., Lumley, L. A., Putnam, S. K., et al. (1999). Hormone-neurotransmitter interactions in the control of sexual behavior. Behav. Brain Res. 105, 105–116. doi: 10.1016/S0166-4328(99)00086-8
Ikemoto, S., and Panksepp, J. (1999). The role of nucleus accumbens dopamine in motivated behavior: a unifying interpretation with special reference to reward-seeking. Brain Res. Brain Res. Rev. 31, 6–41. doi: 10.1016/S0165-0173(99)00023-5
Johansson, P., Ray, A., Zhou, Q., Huang, W., Karlsson, K., and Nyberg, F. (1997). Anabolic androgenic steroids increase beta-endorphin levels in the ventral tegmental area in the male rat brain. Neurosci. Res. 2, 185–189. doi: 10.1016/S0168-0102(96)01141-8
Jones, S. L., Ismail, N., and Pfaus, J. G. (2017). Facilitation of sexual behavior in ovariectomized rats by estradiol and testosterone: a preclinical model of androgen effects on female sexual desire. Psychoneuroendocrinology 79, 122–133. doi: 10.1016/j.psyneuen.2017.02.018
Justinova, Z., Tanda, G., Redhi, G. H., and Goldberg, S. R. (2003). Self-administration of delta9-tetrahydrocannabinol (THC) by drug naive squirrel monkeys. Psychopharmacology 169, 135–140. doi: 10.1007/s00213-003-1484-0
Kailanto, S. I., Kankaanpää, A., and Seppälä, T. (2011). Subchronic steroid administration induces long lasting changes in neurochemical and behavioral response to cocaine in rats. Steroids 76, 1310–1316. doi: 10.1016/j.steroids.2011.06.011
Kanayama, G., Brower, K. J., Wood, R. I., Hudson, J. I., and Pope, H. G. Jr. (2009). Anabolic-androgenic steroid dependence: an emerging disorder. Addiction 104, 1966–1978. doi: 10.1111/j.1360-0443.2009.02734.x
Kanayama, G., Cohane, G. H., Weiss, R. D., and Pope, H. G. (2003). Past anabolic-androgenic steroid use among men admitted for substance abuse treatment: an underrecognized problem? J. Clin. Psychiatry 64, 156–160.
Karamikheirabad, M., Behzadi, G., Faghihi, M., Raoofian, R., Ejtemaei Mehr, S., Zuure, W. A., et al. (2013). A role for endocannabinoids in acute stress-induced suppression of the hypothalamic-pituitary-gonadal axis in male rats. Clin. Exp. Reprod. Med. 40, 155–162. doi: 10.5653/cerm.2013.40.4.155
Keeley, R. J., Trow, J., and McDonald, R. J. (2015b). Strain and sex differences in puberty onset and the effects of THC administration on weight gain and brain volumes. Neuroscience 305, 328–342. doi: 10.1016/j.neuroscience.2015.07.024
Keeley, R. J., Trow, J., Bye, C., and McDonald, R. J. (2015a). Part II: Strain- and sex-specific effects of adolescent exposure to THC on adult brain and behaviour: variants of learning, anxiety and volumetric estimates. Behav. Brain Res. 288, 132–152. doi: 10.1016/j.bbr.2015.01.001
Khan, S. S., Secades-Villa, R., Okuda, M., Wang, S., Pérez-Fuentes, G., Kerridge, B. T., et al. (2013). Gender differences in cannabis use disorders: results from the national epidemiologic survey of alcohol and related conditions. Drug Alcohol Depend. 130, 101–108. doi: 10.1016/j.drugalcdep.2012.10.015
Kindlundh, A. M. S., Lindblom, J., Bergström, L., Wikberg, J. E., and Nyberg, F. (2001). The anabolic-androgenic steroid nandrolone decanoate affects the density of dopamine receptors in the male rat brain. Eur. J. Neurosci. 13, 291–296. doi: 10.1046/j.0953-816X.2000.01402.x
Kolodny, R. C., Masters, W. H., Kolodner, R. M., and Toro, G. (1974). Depression of plasma testosterone levels after chronic intensive marihuana use. N. Engl. J. Med. 290, 872–874. doi: 10.1056/NEJM197404182901602
Kurling, S., Kankaanpää, A., and Seppälä, T. (2008). Sub-chronic nandrolone treatment modifies neurochemical and behavioral effects of amphetamine and 3,4-methylenedioxymethamphetamine (MDMA) in rats. Behav. Brain Res. 189, 191–201. doi: 10.1016/j.bbr.2007.12.021
Kurling-Kailanto, S., Kankaanpää, A., and Seppälä, T. (2010). Subchronic nandrolone administration reduces cocaine-induced dopamine and 5-hydroxytryptamine outflow in the rat nucleus accumbens. Psychopharmacology 209, 271–281. doi: 10.1007/s00213-010-1796-9
Laviolette, S. R. (2017). Cannabinoid regulation of opiate motivational processing in the mesolimbic system: the integrative roles of amygdala, prefrontal cortical and ventral hippocampal input pathways. Curr. Opin. Behav. Sci. 13, 46–54. doi: 10.1016/j.cobeha.2016.10.004
Laviolette, S. R., and Grace, A. A. (2006). The roles of cannabinoid and dopamine receptor systems in neural emotional learning circuits: implications for schizophrenia and addiction. Cell Mol. Life Sci. 63, 1597–1613. doi: 10.1007/s00018-006-6027-5
Lecca, D., Cacciapaglia, F., Valentini, V., and Di Chiara, G. (2006). Monitoring extracellular dopamine in the rat nucleus accumbens shell and core during acquisition and maintenance of intravenous WIN 55,212-2 self-administration. Psychopharmacology 188, 63–74. doi: 10.1007/s00213-006-0475-3
Lee, T. T., Wainwright, S. R., Hill, M. N., Galea, L. A., and Gorzalka, B. B. (2014). Sex, drugs, and adult neurogenesis: sex-dependent effects of escalating adolescent cannabinoid exposure on adult hippocampal neurogenesis, stress reactivity, and amphetamine sensitization. Hippocampus 24, 280–292. doi: 10.1002/hipo.22221
Levin, K. H., Copersino, M. L., Heishman, S. J., Liu, F., Kelly, D. L., Boggs, D. L., et al. (2010). Cannabis withdrawal symptoms in non-treatment-seeking adult cannabis smokers. Drug Alcohol Depend. 111, 120–127. doi: 10.1016/j.drugalcdep.2010.04.010
Lex, B. W., Mendelson, J. H., Bavli, S., Harvey, K., and Mello, N. K. (1984). Effects of acute marijuana smoking on pulse rate and mood states in women. Psychopharmacology 84, 178–187. doi: 10.1007/BF00427443
Liu, Q. R., Canseco-Alba, A., Zhang, H. Y., Tagliaferro, P., Chung, M., Dennis, E., et al. (2017). Cannabinoid type 2 receptors in dopamine neurons inhibits psychomotor behaviors, alters anxiety, depression and alcohol preference. Sci. Rep. 7:17410. doi: 10.1038/s41598-017-17796-y
López, H. H. (2010). Cannabinoid-hormone interactions in the regulation of motivational processes. Horm. Behav. 58, 100–110. doi: 10.1016/j.yhbeh.2009.10.005
Lopez-Quintero, C., Pérez de los Cobos, J., Hasin, D. S., Okuda, M., Wang, S., Grant, B. F., et al. (2011). Probability and predictors of transition from first use to dependence on nicotine, alcohol, cannabis, and cocaine: results of the National Epidemiologic Survey on Alcohol and Related Conditions (NESARC). Drug Alcohol Depend. 115, 120–130. doi: 10.1016/j.drugalcdep.2010.11.004
Lynch, W. J. (2008). Acquisition and maintenance of cocaine self-administration in adolescent rats: effects of sex and gonadal hormones. Psychopharmacology 197, 237–246. doi: 10.1007/s00213-007-1028-0
Lynch, W. J., Arizzi, M. N., and Carroll, M. E. (2000). Effects of sex and estrous cycle on regulation of intravenously self-administered cocaine in rats. Psychopharmacology 152, 132–139. doi: 10.1007/s002130000488
Lynskey, M. T., Agrawal, A., Henders, A., Nelson, E. C., Madden, P. A., and Martin, N. G. (2012). An Australian twin study of cannabis and other illicit drug use and misuse, and other psychopathology. Twin Res. Hum. Genet. 15, 631–641. doi: 10.1017/thg.2012.41
Maccarrone, M., De Felici, M., Bari, M., Klinger, F., Siracusa, G., and Finazzi-Agrò, A. (2000). Down-regulation of anandamide hydrolase in mouse uterus by sex hormones. Eur. J. Biochem. 267, 2991–2997. doi: 10.1046/j.1432-1033.2000.01316.x
Maia, T. V., and Frank, M. J. (2017). An Integrative perspective on the role of dopamine in Schizophrenia. Biol. Psychiatry 81, 52–66. doi: 10.1016/j.biopsych.2016.05.021
Maldonado, R., Valverde, O., and Berrendero, F. (2006). Involvement of the endocannabinoid system in drug addiction. Trends Neurosci. 29, 225–232. doi: 10.1016/j.tins.2006.01.008
Mangelsdorf, D. J., Thummel, C., Beato, M., Herrlich, P., Schütz, G., Umesono, K., et al. (1995). The nuclear receptor superfamily: the second decade. Cell 83, 835–839. doi: 10.1016/0092-8674(95)90199-X
Mani, S. K., Mitchell, A., and O’Malley, B. W. (2001). Progesterone receptor and dopamine receptors are required in Delta 9-tetrahydrocannabinol modulation of sexual receptivity in female rats. Proc. Natl. Acad. Sci. U.S.A. 98, 1249–1254. doi: 10.1073/pnas.031563998
Martellotta, M. C., Cossu, G., Fattore, L., Gessa, G. L., and Fratta, W. (1998). Self-administration of the cannabinoid receptor agonist WIN 55,212-2 in drug-naive mice. Neuroscience 85, 327–330. doi: 10.1016/S0306-4522(98)00052-9
Marusich, J. A., Craft, R. M., Lefever, T. W., and Wiley, J. L. (2015). The impact of gonadal hormones on cannabinoid dependence. Exp. Clin. Psychopharmacol. 23, 206–216. doi: 10.1037/pha0000027
Marusich, J. A., Lefever, T. W., Antonazzo, K. R., Craft, R. M., and Wiley, J. L. (2014). Evaluation of sex differences in cannabinoid dependence. Drug Alcohol Depend. 137, 20–28. doi: 10.1016/j.drugalcdep.2014.01.019
McCarthy, M. M., Arnold, A. P., Ball, G. F., Blaustein, J. D., and De Vries, G. J. (2012). Sex differences in the brain: the not so inconvenient truth. J. Neurosci. 32, 2241–2247. doi: 10.1523/JNEUROSCI.5372-11.2012
McEwen, B. S., Jones, K. J., and Pfaff, D. W. (1987). Hormonal control of sexual behavior in the female rat: molecular, cellular and neurochemical studies. Biol. Reprod. 36, 37–45. doi: 10.1095/biolreprod36.1.37
McEwen, B. S., and Milner, T. A. (2017). Understanding the broad influence of sex hormones and sex differences in the brain. J. Neurosci. Res. 95, 24–39. doi: 10.1002/jnr.23809
McPartland, J. M., Glass, M., and Pertwee, R. G. (2007). Meta-analysis of cannabinoid ligand binding affinity and receptor distribution: interspecies differences. Br. J. Pharmacol. 152, 583–593. doi: 10.1038/sj.bjp.0707399
Meisel, R. L., and Sachs, B. D. (1994). “The physiology of male sexual behaviour,” in The Physiology of Reproduction, 2nd Edn, Vol. 2, eds E. Knobil and J. Neil (New York, NY: Raven Press), 3–96.
Melas, P. A., Qvist, J. S., Deidda, M., Upreti, C., Wei, Y. B., Sanna, F., et al. (2018). Cannabinoid modulation of eukaryotic initiation factors (eIF2α and eIF2B1) and behavioral cross-sensitization to cocaine in adolescent rats. Cell. Rep. 22, 2909–2923. doi: 10.1016/j.celrep.2018.02.065
Melis, M., De Felice, M., Lecca, S., Fattore, L., and Pistis, M. (2013). Sex-specific tonic 2-arachidonoylglycerol signaling at inhibitory inputs onto dopamine neurons of Lister Hooded rats. Front. Integr. Neurosci. 7:93. doi: 10.3389/fnint.2013.00093
Melis, M., and Pistis, M. (2007). Endocannabinoid signaling in midbrain dopamine neurons: more than physiology? Curr. Neuropharmacol. 5, 268–277. doi: 10.2174/157015907782793612
Melis, T., Succu, S., Sanna, F., Boi, A., Argiolas, A., and Melis, M. R. (2007). The cannabinoid antagonist SR 141716A (Rimonabant) reduces the increase of extra-cellular dopamine release in the rat nucleus accumbens induced by a novel high palatable food. Neurosci. Lett. 419, 231–235. doi: 10.1016/j.neulet.2007.04.012
Mhillaj, E., Morgese, M. G., Tucci, P., Bove, M., Schiavone, S., and Trabace, L. (2015). Effects of anabolic-androgens on brain reward function. Front. Neurosci. 9:295. doi: 10.3389/fnins.2015.00295
Micheal, R. P., and Wilson, M. (1974). Effects of castration and hormone replacement in fully adult male rhesus monkeys (Macaca mulatta). Endocrinology 95, 150–159. doi: 10.1210/endo-95-1-150
Mitchell, J. B., and Stewart, J. (1989). Effects of castration, steroid replacement, and sexual experience on mesolimbic dopamine and sexual behaviors in the male rat. Brain Res. 491, 116–127. doi: 10.1016/0006-8993(89)90093-0
Moldrich, G., and Wenger, T. (2000). Localization of the CB1 cannabinoid receptor in the rat brain. An immunohistochemical study. Peptides 21, 1735–1742. doi: 10.1016/S0196-9781(00)00324-7
Moraga-Amaro, R., van Waarde, A., Doorduin, J., and de Vries, E. F. J. (2018). Sex steroid hormones and brain function: PET imaging as a tool for research. J. Neuroendocrinol. 30:e12565. doi: 10.1111/jne.12565
Morris, J. A., Jordan, C. L., and Breedlove, S. M. (2004). Sexual differentiation of the vertebrate nervous system. Nat. Neurosci. 7, 1034–1039. doi: 10.1038/nn1325
Motta-Mena, N. V., and Puts, D. A. (2017). Endocrinology of human female sexuality, mating, and reproductive behavior. Horm. Behav. 91, 19–35. doi: 10.1016/j.yhbeh.2016.11.012
Muro, I., and Rodríguez, A. (2015). Age, sex and personality in early cannabis use. Eur. Psychiatry 30, 469–473. doi: 10.1016/j.eurpsy.2015.02.008
Murphy, L. L., Chandrashekar, V., and Bartke, A. (1994). Delta-9-tetrahydrocannabinol inhibits pulsatile luteinizing hormone secretion in the male rat: effect of intracerebroventricular norepinephrine infusion. Neuroendocrinol. Lett. 16, 1–7.
Navarro, M., Rubio, P., Rodríguez, and de Fonseca, F. (1994). Sex-dimorphic psychomotor activation after perinatal exposure to (-)-delta 9-tetrahydrocannabinol. An ontogenic study in Wistar rats. Psychopharmacology 116, 414–422. doi: 10.1007/BF02247471
Neumeister, A., Normandin, M. D., Pietrzak, R. H., Piomelli, D., Zheng, M. Q., Gujarro-Anton, A., et al. (2013). Elevated brain cannabinoid CB1 receptor availability in post-traumatic stress disorder: a positron emission tomography study. Mol. Psychiatry 18, 1034–1040. doi: 10.1038/mp.2013.61
Nguyen, T. V., Ducharme, S., and Karama, S. (2017). Effects of sex steroids in the human brain. Mol. Neurobiol. 54, 7507–7519. doi: 10.1007/s12035-016-0198-3
Nir, I., Ayalon, D., Tsafriri, A., Cordova, T., and Lindner, H. R. (1973). Suppression of the cyclic surge of luteinizing hormone secretion and of ovulation in the rat by delta 1- tetrahydrocannabinol. Nature 243, 470–471. doi: 10.1038/243470a0
Normandin, M. D., Zheng, M. Q., Lin, K. S., Mason, N. S., Lin, S. F., Ropchan, J., et al. (2015). Imaging the cannabinoid CB1 receptor in humans with [11C]OMAR: assessment of kinetic analysis methods, test-retest reproducibility, and gender differences. J. Cereb. Blood Flow Metab. 35, 1313–1322. doi: 10.1038/jcbfm.2015.46
O’Shea, M., Singh, M. E., McGregor, I. S., and Mallet, P. E. (2004). Chronic cannabinoid exposure produces lasting memory impairment and increased anxiety in adolescent but not adult rats. J. Psychopharmacol. 18, 502–508. doi: 10.1177/026988110401800407
Panagis, G., Mackey, B., and Vlachou, S. (2014). Cannabinoid regulation of brain reward processing with an emphasis on the role of CB1 receptors: a step back into the future. Front. Psychiatry 5:92. doi: 10.3389/fpsyt.2014.00092
Panlilio, L. V., Justinova, Z., and Goldberg, S. R. (2010). Animal models of cannabinoid reward. Br. J. Pharmacol. 160, 499–510. doi: 10.1111/j.1476-5381.2010.00775.x
Parkinson, A. B., and Evans, N. A. (2006). Anabolic androgenic steroids: a survey of 500 users. Med. Sci. Sports Exerc. 38, 644–651. doi: 10.1249/01.mss.0000210194.56834.5d
Parrilla-Carrero, J., Figueroa, O., Lugo, A., García-Sosa, R., Brito-Vargas, P., Cruz, B., et al. (2009). The anabolic steroids testosterone propionate and nandrolone, but not 17alpha-methyltestosterone, induce conditioned place preference in adult mice. Drug Alcohol Depend. 100, 122–127. doi: 10.1016/j.drugalcdep.2008.09.014
Patel, S., Hill, M. N., Cheer, J. F., Wotjak, C. T., and Holmes, A. (2017). The endocannabinoid system as a target for novel anxiolytic drugs. Neurosci. Biobehav. Rev. 76, 56–66. doi: 10.1016/j.neubiorev.2016.12.033
Pfaus, G. J., Dansma, G., Nomikos, G. G., Wenkstern, D. G., Blaha, C. D., Phillips, A. G., et al. (1990). Sexual Behavior enhances central dopamine transmission in the male rats. Brain Res. 530, 345–348. doi: 10.1016/0006-8993(90)91309-5
Pfaus, J. G., and Everitt, B. J. (1995). “The psychopharmacology of sexual behavior,” in Psychopharmacology: The Fourth Generation of Progress, eds F. E. Knobil and D. J. Kupfer (New York, NY: Raven Press), 742–758.
Pistis, M., Perra, S., Pillolla, G., Melis, M., Muntoni, A. L., and Gessa, G. L. (2004). Adolescent exposure to cannabinoids induces long-lasting changes in the response to drugs of abuse of rat midbrain dopamine neurons. Biol. Psychiatry 56, 86–94. doi: 10.1016/j.biopsych.2004.05.006
Polanco, J. C., and Koopman, P. (2007). Sry and the hesitant beginnings of male development. Dev. Biol. 302, 13–24. doi: 10.1016/j.ydbio.2006.08.049
Prini, P., Penna, F., Sciuccati, E., Alberio, T., and Rubino, T. (2017). Chronic Δ8-THC exposure differently affects histone modifications in the adolescent and adult rat brain. Int. J. Mol. Sci. 18:E2094. doi: 10.3390/ijms18102094
Quinn, J. J., Hitchcott, P. K., Umeda, E. A., Arnold, A. P., and Taylor, J. R. (2007). Sex chromosome complement regulates habit formation. Nat. Neurosci. 10, 1398–1400. doi: 10.1038/nn1994
Renard, J., Krebs, M. O., Le Pen, G., and Jay, T. M. (2014). Long-term consequences of adolescent cannabinoid exposure in adult psychopathology. Front. Neurosci. 8:361. doi: 10.3389/fnins.2014.00361
Rodríguez, de Fonseca, F., Cebeira, M., Ramos, J. A., Martín, M., and Fernández-Ruiz, J. J. (1994). Cannabinoid receptors in rat brain areas: sexual differences, fluctuations during estrous cycle and changes after gonadectomy and sex steroid replacement. Life Sci. 54, 159–170. doi: 10.1016/0024-3205(94)00585-0
Rosas, M., Porru, S., Giugliano, V., Antinori, S., Scheggi, S., Fadda, P., et al. (2018). Sex-specific differences in cannabinoid-induced extracellular-signal-regulated kinase phosphorylation in the cingulate cortex, prefrontal cortex, and nucleus accumbens of Lister Hooded rats. Behav. Pharmacol. 29, 473–481. doi: 10.1097/FBP.0000000000000395
Rubino, T., and Parolaro, D. (2016). The impact of exposure to cannabinoids in adolescence: insights from animal models. Biol. Psychiatry 79, 578–585. doi: 10.1016/j.biopsych.2015.07.024
Rubino, T., Realini, N., Braida, D., Guidi, S., Capurro, V., Viganò, D., et al. (2009). Changes in hippocampal morphology and neuroplasticity induced by adolescent THC treatment are associated with cognitive impairment in adulthood. Hippocampus 19, 763–772. doi: 10.1002/hipo.20554
Rubino, T., Vigano’, D., Realini, N., Guidali, C., Braida, D., Capurro, V., et al. (2008). Chronic delta 9-tetrahydrocannabinol during adolescence provokes sex-dependent changes in the emotional profile in adult rats: behavioral and biochemical correlates. Neuropsychopharmacology 33, 2760–2771. doi: 10.1038/sj.npp.1301664
Sagoe, D., Molde, H., Andreassen, C. S., Torsheim, T., and Pallesen, S. (2014). The global epidemiology of anabolic-androgenic steroid use: a meta-analysis and meta-regression analysis. Ann. Epidemiol. 24, 383–398. doi: 10.1016/j.annepidem.2014.01.009
Sagrillo, C. A., Grattan, D. R., McCarthy, M. M., and Selmanoff, M. (1996). Hormonal and neurotransmitter regulation of GnRH gene expression and related reproductive behaviors. Behav. Genet. 26, 241–277. doi: 10.1007/BF02359383
Salamone, J. D., and Correa, M. (2002). Motivational views of reinforcement: implications for understanding the behavioral functions of nucleus accumbens dopamine. Behav. Brain Res. 137, 3–25. doi: 10.1016/S0166-4328(02)00282-6
Sanna, F., Bratzu, J., Piludu, M. A., Corda, M. G., Melis, M. R., Giorgi, O., et al. (2017). Dopamine, noradrenaline and differences in sexual behavior between roman high and low avoidance male rats: a microdialysis study in the medial prefrontal cortex. Front. Behav. Neurosci. 11:108. doi: 10.3389/fnbeh.2017.00108
Sanna, F., Piludu, M. A., Corda, M. G., Melis, M. R., Giorgi, O., and Argiolas, A. (2015). Involvement of dopamine in the differences in sexual behavior between Roman high and low avoidance rats: an intracerebral microdialysis study. Behav. Brain Res. 281, 177–186. doi: 10.1016/j.bbr.2014.12.009
Schneider, M. (2008). Puberty as a highly vulnerable developmental period for the consequences of cannabis exposure. Addict. Biol. 13, 253–263. doi: 10.1111/j.1369-1600.2008.00110.x
Schneider, M., and Koch, M. (2003). Chronic pubertal, but not adult chronic cannabinoid treatment impairs sensorimotor gating, recognition memory, and the performance in a progressive ratio task in adult rats. Neuropsychopharmacology 28, 1760–1769. doi: 10.1038/sj.npp.1300225
Schroeder, J. P., and Packard, M. G. (2000). Role of dopamine receptor subtypes in the acquisition of a testosterone conditioned place preference in rats. Neurosci. Lett. 282, 17–20. doi: 10.1016/S0304-3940(00)00839-9
Schultz, W. (1997). Dopamine neurons and their role in reward mechanisms. Curr. Opin. Neurobiol. 7, 191–197. doi: 10.1016/S0959-4388(97)80007-4
Scorticati, C., Fernandez-Solari, J., De Laurentiis, A., Mohn, C., Prestifilippo, J. P., Lasaga, M., et al. (2004). The inhibitory effect of anandamide on luteinizing hormone-releasing hormone secretion is reversed by estrogen. Proc. Natl. Acad. Sci. U.S.A. 101, 11891–11896. doi: 10.1073/pnas.0404366101
Sherva, R., Wang, Q., Kranzler, H., Zhao, H., Koesterer, R., Herman, A., et al. (2016). Genome-wide association study of cannabis dependence severity. Novel risk variants, and shared genetic risks. JAMA Psychiatry 73, 472–480. doi: 10.1001/jamapsychiatry.2016.0036
Silva, L., Black, R., Michaelides, M., Hurd, Y. L., and Dow-Edwards, D. (2016). Sex and age specific effects of delta-9-tetrahydrocannabinol during the periadolescent period in the rat: The unique susceptibility of the prepubescent animal. Neurotoxicol. Teratol. 58, 88–100. doi: 10.1016/j.ntt.2016.02.005
Simoncini, T., and Genazzani, A. R. (2003). Non-genomic actions of sex steroid hormones. Eur. J. Endocrinol. 148, 281–292. doi: 10.1530/eje.0.1480281
Spano, M. S., Ellgren, M., Wang, X., and Hurd, Y. L. (2006). Prenatal cannabis exposure increases heroin seeking with allostatic changes in limbic enkephalin systems in adulthood. Biol. Psychiatry 61, 554–563. doi: 10.1016/j.biopsych.2006.03.073
Steger, R. W., DePaolo, L., Asch, R. H., and Silverman, A. Y. (1983). Interactions of delta 9- tetrahydrocannabinol (THC) with hypothalamic neurotransmitters controlling luteinizing hormone and prolactin release. Neuroendocrinology 37, 361–370. doi: 10.1159/000123576
Stringer, S., Minicã, C. C., Verweij, K. J., Mbarek, H., Bernard, M., Derringer, J., et al. (2016). Genome-wide association study of lifetime cannabis use based on a large meta-analytic sample of 32 330 subjects from the International Cannabis Consortium. Transl. Psychiatry 6:e769. doi: 10.1038/tp.2016.36
Struik, D., Fadda, P., Zara, T., Zamberletti, E., Rubino, T., Parolaro, D., et al. (2017). The anabolic steroid nandrolone alters cannabinoid self-administration and brain CB1 receptor density and function. Pharmacol. Res. 115, 209–217. doi: 10.1016/j.phrs.2016.11.031
Swalve, N., Smethells, J. R., and Carroll, M. E. (2016). Sex differences in the acquisition and maintenance of cocaine and nicotine self-administration in rats. Psychopharmacology 233, 1005–1013. doi: 10.1007/s00213-015-4183-8
Szutorisz, H., and Hurd, Y. L. (2016). Epigenetic effects of cannabis exposure. Biol. Psychiatry 79, 586–594. doi: 10.1016/j.biopsych.2015.09.014
Szutorisz, H., and Hurd, Y. L. (2018). High times for cannabis: epigenetic imprint and its legacy on brain and behavior. Neurosci. Biobehav. Rev. 85, 93–101. doi: 10.1016/j.neubiorev.2017.05.011
Terner, J. M., and de Wit, H. (2006). Menstrual cycle phase and responses to drugs of abuse in humans. Drug Alcohol Depend. 84, 1–13. doi: 10.1016/j.drugalcdep.2005.12.007
Tseng, A. H., and Craft, R. M. (2001). Sex differences in antinociceptive and motoric effects of cannabinoids. Eur. J. Pharmacol. 430, 41–47. doi: 10.1016/S0014-2999(01)01267-5
Tsou, K., Brown, S., Sañudo-Peña, M. C., Mackie, K., and Walker, J. M. (1998). Immunohistochemical distribution of cannabinoid CB1 receptors in the rat central nervous system. Neuroscience 83, 393–411. doi: 10.1016/S0306-4522(97)00436-3
United Nations Office on Drugs and Crime [UNODC] (2017). World Drug Report 2017 (United Nations publication, Sales No. E. (.)17.XI.6). Vienna: The United Nations Office on Drugs and Crime (UNODC). doi: 10.18356/c595e10f-en
Vallée, M., Vitiello, S., Bellocchio, L., Hébert-Chatelain, E., Monlezun, S., Martin-Garcia, E., et al. (2014). Pregnenolone can protect the brain from cannabis intoxication. Science 343, 94–98. doi: 10.1126/science.1243985
van den Bree, M. B., Johnson, E. O., Neale, M. C., and Pickens, R. W. (1998). Genetic and environmental influences on drug use and abuse/dependence in male and female twins. Drug Alcohol Depend. 52, 231–241. doi: 10.1016/S0376-8716(98)00101-X
van der Stelt, M., and Di Marzo, V. (2003). The endocannabinoid system in the basal ganglia and in the mesolimbic reward system: implications for neurological and psychiatric disorders. Eur. J. Pharmacol. 480, 133–150. doi: 10.1016/j.ejphar.2003.08.101
van der Stelt, M., and Di Marzo, V. (2005). Cannabinoid receptors and their role in neuroprotection. Neuromolecular Med. 7, 37–50. doi: 10.1385/NMM:7:1-2:037
van Laere, K., Goffin, K., Casteels, C., Dupont, P., Mortelmans, L., de Hoon, J., et al. (2008). Gender-dependent increases with healthy aging of the human cerebral cannabinoid-type 1 receptor binding using [(18)F]MK-9470 PET. Neuroimage 39, 1533–1541. doi: 10.1016/j.neuroimage.2007.10.053
Verweij, K. J., Vinkhuyzen, A. A., Benyamin, B., Lynskey, M. T., Quaye, L., Agrawal, A., et al. (2013). The genetic aetiology of cannabis use initiation: a meta-analysis of genome-wide association studies and a SNP-based heritability estimation. Addict. Biol. 18, 846–850. doi: 10.1111/j.1369-1600.2012.00478.x
Verweij, K. J., Zietsch, B. P., Lynskey, M. T., Medland, S. E., Neale, M. C., Martin, N. G., et al. (2010). Genetic and environmental influences on cannabis use initiation and problematic use: a meta-analysis of twin studies. Addiction 105, 417–430. doi: 10.1111/j.1360-0443.2009.02831.x
Vink, J. M., Wolters, L. M., Neale, M. C., and Boomsma, D. I. (2010). Heritability of cannabis initiation in Dutch adult twins. Addict. Behav. 35, 172–174. doi: 10.1016/j.addbeh.2009.09.015
Viveros, M. P., Llorente, R., Moreno, E., and Marco, E. M. (2005a). Behavioural and neuroendocrine effects of cannabinoids in critical developmental periods. Behav. Pharmacol. 16, 353–362.
Viveros, M. P., Marco, E. M., and File, S. E. (2005b). Endocannabinoid system and stress and anxiety responses. Pharmacol. Biochem. Behav. 81, 331–342.
Viveros, M. P., Marco, E. M., López-Gallardo, M., Garcia-Segura, L. M., and Wagner, E. J. (2011). Framework for sex differences in adolescent neurobiology: a focus on cannabinoids. Neurosci. Biobehav. Rev. 35, 1740–1751. doi: 10.1016/j.neubiorev.2010.09.005
Volkow, N. D., Baler, R. D., Compton, W. M., and Weiss, S. R. (2014). Adverse health effects of marijuana use. N. Engl. J. Med. 370, 2219–2227. doi: 10.1056/NEJMra1402309
Wagner, E. J. (2016). Sex differences in cannabinoid-regulated biology: a focus on energy homeostasis. Front. Neuroendocrinol. 40, 101–109. doi: 10.1016/j.yfrne.2016.01.003
Wakley, A. A., McBride, A. A., Vaughn, L. K., and Craft, R. M. (2014). Cyclic ovarian hormone modulation of supraspinal Δ9-tetrahydrocannabinol-induced antinociception and cannabinoid receptor binding in the female rat. Pharmacol. Biochem. Behav. 124, 269–277. doi: 10.1016/j.pbb.2014.06.007
Waleh, N. S., Cravatt, B. F., Apte-Deshpande, A., Terao, A., and Kilduff, T. S. (2002). Transcriptional regulation of the mouse fatty acid amide hydrolase gene. Gene 291, 203–210. doi: 10.1016/S0378-1119(02)00598-X
Wallen, K. (1990). Desire and ability: hormones and the regulation of female sexual behavior. Neurosci. Biobehav. Rev. 14, 233–241. doi: 10.1016/S0149-7634(05)80223-4
Weinberger, A. H., Smith, P. H., Allen, S. S., Cosgrove, K. P., Saladin, M. E., Gray, K. M., et al. (2015). Systematic and meta-analytic review of research examining the impact of menstrual cycle phase and ovarian hormones on smoking and cessation. Nicotine Tob. Res. 17, 407–421. doi: 10.1093/ntr/ntu249
Weinstein, A. M., and Gorelick, D. A. (2011). Pharmacological treatment of cannabis dependence. Curr. Pharm. Des. 17, 1351–1358. doi: 10.2174/138161211796150846
Weinstein, A. M., Rosca, P., Fattore, L., and London, E. D. (2017). Synthetic cathinone and cannabinoid designer drugs pose a major risk for public health. Front. Psychiatry 8:156. doi: 10.3389/fpsyt.2017.00156
Wenger, T., Ledent, C., Csernus, V., and Gerendai, I. (2001). The central cannabinoid receptor inactivation suppresses endocrine reproductive functions. Biochem. Biophys. Res. Commun. 284, 363–368. doi: 10.1006/bbrc.2001.4977
Wiley, J. L., and Burston, J. J. (2014). Sex differences in Δ(9)-tetrahydrocannabinol metabolism and in vivo pharmacology following acute and repeated dosing in adolescent rats. Neurosci. Lett. 576, 51–55. doi: 10.1016/j.neulet.2014.05.057
Wiley, J. L., Lefever, T. W., Marusich, J. A., and Craft, R. M. (2017). Comparison of the discriminative stimulus and response rate effects of Δ9-tetrahydrocannabinol and synthetic cannabinoids in female and male rats. Drug Alcohol Depend. 172, 51–59. doi: 10.1016/j.drugalcdep.2016.11.035
Wood, R. I. (2004). Reinforcing aspects of androgens. Physiol. Behav. 83, 279–289. doi: 10.1016/j.physbeh.2004.08.012
Yang, B. Z., Kranzler, H. R., Zhao, H., Gruen, J. R., Luo, X., and Gelernter, J. (2008). Haplotypic variants in DRD2, ANKK1, TTC12, and NCAM1 are associated with comorbid alcohol and drug dependence. Alcohol Clin. Exp. Res. 32, 2117–2127. doi: 10.1111/j.1530-0277.2008.00800.x
Yoest, K. E., Quigley, J. A., and Becker, J. B. (2018). Rapid effects of ovarian hormones in dorsal striatum and nucleus accumbens. Horm. Behav doi: 10.1016/j.yhbeh.2018.04.002 [Epub ahead of print].
Zanda, M. T., and Fattore, L. (2018). Old and new synthetic cannabinoids: lessons from animal models. Drug Metab. Rev. 50, 54–64. doi: 10.1080/03602532.2018.1430824
Zangen, A., Solinas, M., Ikemoto, S., Goldberg, S. R., and Wise, R. A. (2006). Two brain sites for cannabinoid reward. J. Neurosci. 26, 4901–4907. doi: 10.1523/JNEUROSCI.3554-05.2006
Zhu, H., and Wu, L. T. (2017). Sex differences in cannabis use disorder diagnosis involved hospitalizations in the United States. J. Addict. Med. 11, 357–367. doi: 10.1097/ADM.0000000000000330
Keywords: gonadal hormones, anabolic-androgenic steroids, cannabinoids, dependence, sex, dopamine
Citation: Struik D, Sanna F and Fattore L (2018) The Modulating Role of Sex and Anabolic-Androgenic Steroid Hormones in Cannabinoid Sensitivity. Front. Behav. Neurosci. 12:249. doi: 10.3389/fnbeh.2018.00249
Received: 25 June 2018; Accepted: 05 October 2018;
Published: 26 October 2018.
Edited by:
Nuno Sousa, Instituto de Pesquisa em Ciências da Vida e da Saúde (ICVS), PortugalReviewed by:
Styliani Vlachou, Dublin City University, IrelandJeffrey Tasker, Tulane University, United States
Elizabeth McCone Byrnes, Tufts University, United States
Copyright © 2018 Struik, Sanna and Fattore. This is an open-access article distributed under the terms of the Creative Commons Attribution License (CC BY). The use, distribution or reproduction in other forums is permitted, provided the original author(s) and the copyright owner(s) are credited and that the original publication in this journal is cited, in accordance with accepted academic practice. No use, distribution or reproduction is permitted which does not comply with these terms.
*Correspondence: Liana Fattore, bGZhdHRvcmVAaW4uY25yLml0
†Present address: Dicky Struik, Section of Molecular Metabolism and Nutrition, Department of Pediatrics, University Medical Center Groningen, University of Groningen, Groningen, Netherlands