- 1Laboratory of Comparative Neuropsychology, Psychology Department, Towson University, Towson, MD, United States
- 2Canadian Center for Behavioural Neuroscience, University of Lethbridge, Lethbridge, AB, Canada
Overview
Technological advances have made it possible to capture a specific assembly of neurons active during a learning event and manipulate the captured cells, demonstrating some form of relationship between brief retention events and the cell assembly. The reductionists' claim of localizing the engram has been met with considerable skepticism. The potential for off-target effects in “cell capture” techniques, such as optogenetics, reveal serious limitations when given the highly interconnected nature of brain networks beyond a selective assembly of cells in a restricted area of manipulation. Recent studies of subcortical-cortical interactions prompted us to review the historic search for the engram, revealing a parallel between the empirical attempts to localize the engram in cortical then subcortical systems. When brought together conceptually, the very extensive independent work of Karl Lashley and Robert Thompson suggest that each held a piece of the puzzle. Recent research implicates the interaction between subcortical memory systems and association cortex in the transformation of mnemonic information from a short-term process of plasticity to a long-term state of stability. We propose that one function of subcortical-cortical connectivity is to continuously update contextually retrieved long-term memory during reconsolidation of newly acquired information processing, creating a constantly evolving emergent engram.
The Early Engram
Researchers claim to now “capture” activated cells allocated during a learning experience to represent an enduring change among an assembly of neurons called an engram (Josselyn et al., 2015; Tonegawa et al., 2015). Semon (1921, p. 12) made up the term and defined it as “…the enduring though primarily latent modification in the irritable substance produced by a stimulus.” Semon favored his novel word creation to avoid undesirable connotations of everyday terms in favor of a precise scientific definition. However, he did not provide a precise definition due to “a lack of physiological knowledge at the time,” and claiming that “…such speculation was unwarranted” (Schacter et al., 1978, p. 726). The lack of a satisfactory definition has led to the “filling-in” of what's missing scientifically, and has become popular in mainstream culture (from scientology to popular video games).
Two Routes of Investigation
Research on the engram then took two routes of inquiry. The first route (Hebb, 1949), described cell assemblies and the “learning rule” as an enduring synaptic change later supported by the discovery of long-term potentiation (Bliss and Lomo, 1973; Bliss and Collingridge, 1993). The second route, taken by Hebb's mentor, Karl Lashley (Lashley, 1950; Bruce, 2001), set out to localize an engram empirically somewhere within association cortex, thought to be the repository of long-term memory (Battaglia et al., 2004; Dash et al., 2004; Rauchs et al., 2005; Girardeau et al., 2009; Wang and Morris, 2010; Insel and Takehara-Nishiuchi, 2013; Wiltgen and Tanaka, 2013; de Voogd et al., 2016; Sekeres et al., 2018).
Karl Lashley used various post-training lesion techniques to disrupt the retrieval of memory in rats and monkeys (Lashley, 1950). After decades of systematic empirical work, Lashley concluded that it was not possible to localize an engram in association cortex because memory was widely distributed throughout a network of neurons that all have an equal potential to contribute to the memory trace. Robert Thompson, continued Lashley's work in subcortical structures using pre- and post-training lesions (Thorne, 1995), and published two books (Thompson, 1978; Thompson et al., 1990) that summarized findings from over 120 published journal articles.
Though his work has not been widely recognized in recent reviews of the engram (Josselyn et al., 2015; Tonegawa et al., 2015), it has been pointed out that Thompson was friends with Karl Lashley at the Yerkes Primate Center where they discussed research and the lack of progress in localizing an engram (Thorne, 1995). Robert Thompson thought that Lashley may not be looking in the right place for engrams and, his interest in Penfield's Centrencephalic Integrating System (Penfield, 1958), as the general learning system (Thompson, 1993), resulted in his continuation of a systematic empirical search within subcortical structures, resulting in greater success than Lashley's work (Thorne, 1995).
The success of Thompson's work supports the more modern research of parallel and interactive memory systems in subcortical structures (McDonald et al., 2017). Thompson declared that a level of localization was possible with pre-training lesions among subcortical substrates, later borne out by findings as the understanding and sophistication of behavioral tests were designed for associative learning specificity (Packard et al., 1989; McDonald and White, 1993, 2013; White et al., 2013). More recently, studies of cooperative interactions between memory systems and memory subsystems using the water maze paradigm in rats (Devan et al., 1996, 1999; Devan and White, 1999) provide new models of cognitive-habit interactions (Devan et al., 2011, 2016; Sukumar et al., 2012).
Scientific advancement using the latest technology in localizing engrams depends on the systems-level circuit analysis of functional interactions. First proposed by Richard Hirsh (Hirsh, 1974; Hirsh and Krajden, 1982) and later exemplified by the meticulous, cell recording, lesion and chemical inactivation work by Richard F. Thompson and colleagues (The “R. Thompson” ambiguity may have contributed to the confusion and lack of recognition for Robert Thompson) identified “temporal neuronal models” of activation formed in hippocampus early in classical conditioning (Berger et al., 1976). Richard F. Thompson and colleagues then discovered the essential role of the cerebellar dentate-interpositus nuclei in learning and performance of classically conditioned skeletal responses (McCormick and Thompson, 1984). A combination of conceptual and methodological approaches continue to define memory substrates and circuits within cerebellum (Poulos and Thompson, 2015) and the general advancement of the neurobiology of learning and memory (Thompson, 1986). This kind of extraordinary scientific progress, or strong inference (Platt, 1964), depends on converging operations in eliminating alternative/competing explanations of scientific findings.
The Present State of the Engram
Decades of research to localize an engram using various techniques in the past (Lashley, 1950; Thompson, 1978, 2005, 2013; Thompson et al., 1990; Mayford, 2014; Eichenbaum, 2016) has provided a foundation for the current state of engram research, expressed in a recent Forum (Poo et al., 2016). Different levels of organization of an engram were considered, including processes from chemical, synaptic, and cell levels. However, systems-level interactions were largely missing from the discussion, except for proposed changes in the site of engrams from subcortical structures to association cortex. Robert Thompson and Lashley's independent work on these very locations warrant further consideration within an interactive memory systems perspective.
Two exciting papers recently published in Science (Khodagholy et al., 2017; Kitamura et al., 2017) begin to show how the neglected interactive systems-level is involved in the wide distribution of the engram over time and space, i.e., the succession of events in neuronal activity (Buzsáki and Llinás, 2017). As no approach is perfect, the weaknesses of one are offset by the strengths of another; such converging operations therefore strengthen the conclusions that could not be reached by either approach alone. Although the conceptual strength of optogenetics and other cell “capture techniques” is the precise localization of the sparse network of neurons that make up an isolated engram (see Figure 1), the physical representation of a complex episodic engram is much more likely the emergent property of interactive memory systems (Tulving, 1987; Nyberg et al., 1998; McDonald et al., 2004a,b, 2017). Other technical problems of optogenetics may be considered, such as off-target effects in downstream circuits, as well as other spatial and temporal factors (Otchy et al., 2015; Südhof, 2015; Yates, 2016; Hardt and Nadel, 2017; McDonald and Deibel, 2017). For example, the specificity of a few neurons active in one area seems to mimic an in vitro-like isolation approach, while the snapshot-like association specificity in learning limits the unfolding of an event over time. A densely interconnected brain with molecularly distinct subclasses of neurons, glia and vasculature cell types in different brain areas (Zeisel et al., 2015) may expand the simple redux engram.
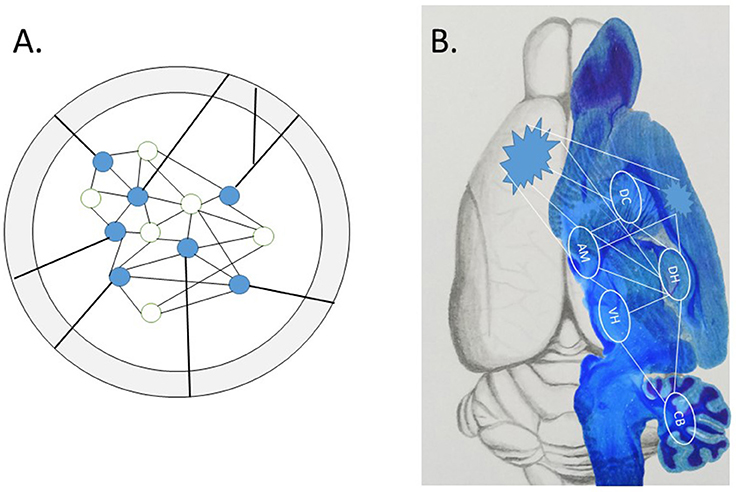
Figure 1. (A) A local engram identified by cell capture techniques within a defined brain structure. This engram is precise yet conceptually isolated from the rest of the brain. Evidence reviewed in text show “off-target” effects outside the in vitro-like conceptual environment, as projections from axon fibers interconnect the local engram with widespread subcortical and cortical targets. (B) The emergent engram with subcortical and cortical components. The wide distribution of connectivity balances the scope of local engrams (pointed clusters) and distributed components of the potential system-wide interactions among multiple subcortical memory systems, with routes to association cortex for (re)consolidation of stable long-term memory representations, thought to occur during sleep (Sara and Hars, 2006; Sara, 2017).
The above studies illustrate widespread connections and changes in plasticity that may form the neurochemical and electrophysiological substrates of a broader episodic engram representation. In the first study (Kitamura et al., 2017), functional reorganization of circuits occurred at the systems level from hippocampal and basolateral amygdala engrams, slowly strengthening the vertical pathways to prefrontal assemblies, while the hippocampal engrams became silent and amygdala engrams remained active. In the second study (Khodagholy et al., 2017), instead of optogenetics, a NeuroGrid and subcortical recording of local field potentials and spiking throughout dorsal cortex, revealed that not only does hippocampal activity transform prefrontal activity during sleep following learning, but also ripple-ripple coupling alters parietal and midline cortical activity, possible components promoting widespread network change throughout association neocortex (Buzsáki, 1996; Xu et al., 2016). Perhaps even long-term consolidation (McGaugh, 1966) over time may continuously alternate between malleable and rigid states of change in a never-ending cycle throughout life (Dudai and Eisenberg, 2004; Dudai, 2012).
The Emergent Engram
It is apparent that the isolated engram is now expanded to an interactive memory systems representation with widespread association cortex coupling (Khodagholy et al., 2017) and subcortical silencing (Kitamura et al., 2017) before recall (Figure 1). It took Lashley three decades to conclude that the cortical component was widely distributed, and several more years for Robert Thompson to begin to localize subcortical substrates. In our view, this extensive empirical and theoretical work has set the stage for important future scientific discovery. An integration among ascending vertical (subcortical-neocortical) with horizontal (cortico-cortical) connectivity (Qin et al., 1997) will further our understanding of the emerging engram. A balanced scientific approach may allow us to appreciate the complexities and vastness of the forest in relation to the details of individual trees.
Conclusion
Although the specific term “emergent engram” does not appear in major databases (e.g., PubMed and PsychINFO), its general idea is prevalent throughout contemporary memory research. Indeed, it is prominent throughout cognitive psychology, e.g., in the writings of Endel Tulving with the emergence of multiple memory systems (Tulving, 1987; Nyberg et al., 1998) among others in the Gestalt tradition (Kałamała et al., 2017; Allon et al., 2018). In addition, it has more contemporary relevance to other recent work in behavioral neuroscience (Sara and Hars, 2006; Sara, 2017). For example, Sara (2010) eloquently summarized the view as “…memory is a dynamic property of the nervous system, in constant flux as a result of being retrieved within current cognitive environments” (pg. 4).
The recent physiological findings described above are consistent with previous studies of coordinated activity within several interactive systems. For example, associative learning of a spatial discrimination was related to fast oscillations or pronounced ripple activity in the hippocampus (Ramadan et al., 2009). Neocortical slow oscillation synchrony increased with thalamocortical spindle activity and hippocampal ripple activity during non-REM sleep (Mölle et al., 2009). These findings may relate to hippocampal-cortical/subcortical coordination of reactivation events (Skelin et al., 2018) involving multiple subcortical memory systems with densely distributed networks of “hard” synaptic changes among cells in different neocortical assemblies (Milner, 1989, 1996).
The interactions among subcortical memory systems and the coordination of spatial processing within the hippocampus and the thalamocortical output of basal ganglia loops (Alexander et al., 1986, 1990; Méndez-Couz et al., 2015) may mediate a form of cognitive-habit integration (Devan et al., 2011, 2016). This hypothesis is consistent with fMRI studies that show cooperation between these systems in the flexible navigation of virtual spatial tasks (Brown et al., 2012; Woolley et al., 2015), in the demonstration of exceptional memory performance among competitive mnemonists (Muller et al., 2018) and disrupted functional connectivity between hippocampus and caudate nucleus in patients suffering from obstructive sleep apnea (Song et al., 2018). Further evidence suggests a potential role of glia in consolidation-related sleep processes (Hyden and Lange, 1965; Chen et al., 2015; Frank, 2018).
In summary, the historical search for the engram identified ascending vertical subcortical-cortical networks supporting consolidation processes in studies using the latest technology (Khodagholy et al., 2017; Kitamura et al., 2017). Further, support for multiple memory systems provided horizontal integration among subcortical systems (Devan et al., 2011, 2016). The combination of connections enable soft or labile interactions between local subcortical cell assemblies and a dense or hard (Milner, 1989) network of vastly rich cortical associations that are constantly responsive to smaller units of reconsolidation through the different stages of sleep (Sara and Hars, 2006; Sara, 2017). As we learn more about glial cells and sleep physiology (Panatier et al., 2006; Fields, 2011; Fields et al., 2014; Cousins et al., 2016; Frank, 2018) we may gain valuable insight into the complexities of yet unknown processes supporting (re)consolidation, hence continually remolding the emerging engram to express both stability and change.
Author Contributions
BD conceived of idea talking with RM regarding a previous paper. Worked on a novel conceptualization of the engram with the other authors. KB worked on historical account, and the novel conceptualizations with other authors. RB wrote a critical commentary on a high profile journal article using the term engram and helped BD to conceptualize the new emergent engram framework.
Funding
This work was supported by NSERC Discovery (Grant #06347).
Conflict of Interest Statement
The authors declare that the research was conducted in the absence of any commercial or financial relationships that could be construed as a potential conflict of interest.
References
Alexander, G. E., Crutcher, M. D., and DeLong, M. R. (1990). Basal ganglia-thalamocortical circuits: parallel substrates for motor, oculomotor, “prefrontal” and “limbic” functions. Prog. Brain Res. 85, 119–146. doi: 10.1016/S0079-6123(08)62678-3
Alexander, G. E., DeLong, M. R., and Strick, P. L. (1986). Parallel organization of functionally segregated circuits linking basal ganglia and cortex. Annu. Rev. Neurosci. 9, 357–381. doi: 10.1146/annurev.ne.09.030186.002041
Allon, A. S., Vixman, G., and Luria, R. (2018). Gestalt grouping cues can improve filtering performance in visual working memory. Psychol. Res. doi: 10.1007/s00426-018-1032-5. [Epub ahead of print].
Battaglia, F. P., Sutherland, G. R., and McNaughton, B. L. (2004). Hippocampal sharp wave bursts coincide with neocortical “up-state” transitions. Learn. Mem. 11, 697–704. doi: 10.1101/lm.73504
Berger, T. W., Alger, B., and Thompson, R. F. (1976). Neuronal substrate of classical conditioning in the hippocampus. Science 192, 483–485. doi: 10.1126/science.1257783
Bliss, T. V., and Collingridge, G. L. (1993). A synaptic model of memory: long-term potentiation in the hippocampus. Nature 361, 31–39. doi: 10.1038/361031a0
Bliss, T. V., and Lomo, T. (1973). Long-lasting potentiation of synaptic transmission in the dentate area of the anaesthetized rabbit following stimulation of the perforant path. J. Physiol. 232, 331–356. doi: 10.1113/jphysiol.1973.sp010273
Brown, T. I., Ross, R. S., Tobyne, S. M., and Stern, C. E. (2012). Cooperative interactions between hippocampal and striatal systems support flexible navigation. Neuroimage 60, 1316–1330. doi: 10.1016/j.neuroimage.2012.01.046
Bruce, D. (2001). Fifty years since Lashley's In search of the Engram: refutations and conjectures. J. Hist. Neurosci. 10, 308–318. doi: 10.1076/jhin.10.3.308.9086
Buzsáki, G. (1996). The hippocampo-neocortical dialogue. Cereb. Cortex, 6, 81–92. doi: 10.1093/cercor/6.2.81
Buzsáki, G., and Llinás, R. (2017). Space and time in the brain. Science 358, 482–485. doi: 10.1126/science.aan8869
Chen, W. F., Maguire, S., Sowcik, M., Luo, W., Koh, K., and Sehgal, A. (2015). A neuron-glia interaction involving GABA transaminase contributes to sleep loss in sleepless mutants. Mol. Psychiat. 20, 240–251. doi: 10.1038/mp.2014.11
Cousins, J. N., El-Deredy, W., Parkes, L. M., Hennies, N., and Lewis, P. A. (2016). Cued reactivation of motor learning during sleep leads to overnight changes in functional brain activity and connectivity. PLoS Biol. 14:e1002451. doi: 10.1371/journal.pbio.1002451
Dash, P. K., Hebert, A. E., and Runyan, J. D. (2004). A unified theory for systems and cellular memory consolidation. Brain Res. Brain Res. Rev. 45, 30–37. doi: 10.1016/j.brainresrev.2004.02.001
Devan, B. D., Chaban, N., Piscopello, J., Deibel, S. H., and McDonald, R. J. (2016). “Cognitive and stimulus–response habit functions of the Neo- (Dorsal) striatum,” in The Basal Ganglia, Innovations in Cognitive Neuroscience, ed J. Soghomonian (Springer International Publishing), 413–456.
Devan, B. D., Goad, E. H., and Petri, H. L. (1996). Dissociation of hippocampal and striatal contributions to spatial navigation in the water maze. Neurobiol. Learn. Mem. 66, 305–323. doi: 10.1006/nlme.1996.0072
Devan, B. D., Hong, N. S., and McDonald, R. J. (2011). Parallel associative processing in the dorsal striatum: segregation of stimulus-response and cognitive control subregions. Neurobiol. Learn. Mem. 96, 95–120. doi: 10.1016/j.nlm.2011.06.002
Devan, B. D., McDonald, R. J., and White, N. M. (1999). Effects of medial and lateral caudate-putamen lesions on place- and cue-guided behaviors in the water maze: relation to thigmotaxis. Behav. Brain Res. 100, 5–14. doi: 10.1016/S0166-4328(98)00107-7
Devan, B. D., and White, N. M. (1999). Parallel information processing in the dorsal striatum: relation to hippocampal function. J. Neurosci. 19, 2789–2798. doi: 10.1523/JNEUROSCI.19-07-02789.1999
de Voogd, L. D., Fernández, G., and Hermans, E. J. (2016). Awake reactivation of emotional memory traces through hippocampal-neocortical interactions. Neuroimage 134, 563–572. doi: 10.1016/j.neuroimage.2016.04.026
Dudai, Y. (2012). The restless engram: consolidations never end. Annu. Rev. Neurosci. 35, 227–247. doi: 10.1146/annurev-neuro-062111-150500
Dudai, Y., and Eisenberg, M. (2004). Rites of passage of the engram: reconsolidation and the lingering consolidation hypothesis. Neuron 44, 93–100. doi: 10.1016/j.neuron.2004.09.003
Eichenbaum, H. (2016). Still searching for the engram. Learn. Behav. 44, 209–222. doi: 10.3758/s13420-016-0218-1
Fields, R. D. (2011). Imaging learning: the search for a memory trace. Neuroscientist 17, 185–196. doi: 10.1177/1073858410383696
Fields, R. D., Araque, A., Johansen-Berg, H., Lim, S. S., Lynch, G., Nave, K. A., et al. (2014). Glial biology in learning and cognition. Neuroscientist 20, 426–431. doi: 10.1177/1073858413504465
Frank, M. G. (2018). The role of glia in sleep regulation and function. Handb. Exp. Pharmacol. doi: 10.1007/164_2017_87. [Epub ahead of print].
Girardeau, G., Benchenane, K., Wiener, S. I., Buzsáki, G., and Zugaro, M. B. (2009). Selective suppression of hippocampal ripples impairs spatial memory. Nat. Neurosci. 12, 1222–1223. doi: 10.1038/nn.2384
Hardt, O., and Nadel, L. (2017). Systems consolidation revisited, but not revised: the promise and limits of optogenetics in the study of memory. Neurosci. Lett. doi: 10.1016/j.neulet.2017.11.062. [Epub ahead of print].
Hebb, D. O. (1949). The Organization of Behavior: A Neuropsychological Approach. New York, NY: John Wiley & Sons.
Hirsh, R. (1974). The hippocampus and contextual retrieval of information from memory: a theory. Behav. Biol. 12, 421–444. doi: 10.1016/S0091-6773(74)92231-7
Hirsh, R., and Krajden, J. (1982). “The hippocampus and the expression of knowledge,” in The Expression of Knowledge: Neurobehavioral Transformations of Information into Action, eds R. L. Isaacson and N. E. Spear (New York, NY: Plenum Press), 213–241.
Hyden, H., and Lange, P. W. (1965). Rhythmic enzyme changes in neurons and glia during sleep. Science 149, 654–656. doi: 10.1126/science.149.3684.654
Insel, N., and Takehara-Nishiuchi, K. (2013). The cortical structure of consolidated memory: a hypothesis on the role of the cingulate-entorhinal cortical connection. Neurobiol. Learn. Mem. 106, 343–350. doi: 10.1016/j.nlm.2013.07.019
Josselyn, S. A., Köhler, S., and Frankland, P. W. (2015). Finding the engram. Nat. Rev. Neurosci. 16, 521–534. doi: 10.1038/nrn4000
Kałamała, P., Sadowska, A., Ordziniak, W., and Chuderski, A. (2017). Gestalt effects in visual working memory. Exp. Psychol. 64, 5–13. doi: 10.1027/1618-3169/a000346
Khodagholy, D., Gelinas, J. N., and Buzsáki, G. (2017). Learning-enhanced coupling between ripple oscillations in association cortices and hippocampus. Science 358, 369–372. doi: 10.1126/science.aan6203
Kitamura, T., Ogawa, S. K., Roy, D. S., Okuyama, T., Morrissey, M. D., Smith, L. M., et al. (2017). Engrams and circuits crucial for systems consolidation of a memory. Science 356, 73–78. doi: 10.1126/science.aam6808
Mayford, M. (2014). The search for a hippocampal engram. Philos. Trans. R. Soc. Lond. B Biol. Sci. 369:20130161. doi: 10.1098/rstb.2013.0161
McCormick, D. A., and Thompson, R. F. (1984). Cerebellum: essential involvement in the classically conditioned eyelid response. Science 223, 296–299. doi: 10.1126/science.6701513
McDonald, R. J., and Deibel, S. H. (2017). Boosting weakened synapses to treat Alzheimer's disease. Learn. Behav. 45, 105–106. doi: 10.3758/s13420-016-0253-y
McDonald, R. J., Devan, B. D., and Hong, N. S. (2004a). Multiple memory systems: the power of interactions. Neurobiol. Learn. Mem. 82, 333–346. doi: 10.1016/j.nlm.2004.05.009
McDonald, R. J., Hong, N. S., and Devan, B. D. (2004b). The challenges of understanding mammalian cognition and memory-based behaviours: an interactive learning and memory systems approach. Neurosci. Biobehav. Rev. 28, 719–745. doi: 10.1016/j.neubiorev.2004.09.014
McDonald, R. J., Hong, N. S., and Devan, B. D. (2017). “Interactions among multiple parallel learning and memory systems in the mammalian brain,” in Reference Module in Neuroscience and Biobehavioral Psychology eds J. H. Byrne and H. Eichenbaum (Oxford: Academic Press; Elsevier Ltd), 1–33.
McDonald, R. J., and White, N. M. (1993). A triple dissociation of memory systems: hippocampus, amygdala, and dorsal striatum. Behav. Neurosci. 107, 3–22. doi: 10.1037/0735-7044.107.1.3
McDonald, R. J., and White, N. M. (2013). A triple dissociation of memory systems: hippocampus, amygdala, and dorsal striatum. Behav. Neurosci. 127, 835–853. doi: 10.1037/a0034883
McGaugh, J. L. (1966). Time-dependent processes in memory storage. Science 153, 1351–1358. doi: 10.1126/science.153.3742.1351
Méndez-Couz, M., Conejo, N. M., González-Pardo, H., and Arias, J. L. (2015). Functional interactions between dentate gyrus, striatum and anterior thalamic nuclei on spatial memory retrieval. Brain Res. 1605, 59–69. doi: 10.1016/j.brainres.2015.02.005
Milner, P. M. (1989). A cell assembly theory of hippocampal amnesia. Neuropsychologia 27, 23–30. doi: 10.1016/0028-3932(89)90087-0
Milner, P. M. (1996). Neural representations: some old problems revisited. J. Cogn. Neurosci. 8, 69–77. doi: 10.1162/jocn.1996.8.1.69
Mölle, M., Eschenko, O., Gais, S., Sara, S. J., and Born, J. (2009). The influence of learning on sleep slow oscillations and associated spindles and ripples in humans and rats. Eur. J. Neurosci. 29, 1071–1081. doi: 10.1111/j.1460-9568.2009.06654.x
Muller, N. C. J., Konrad, B. N., Kohn, N., Muñoz-López, M., Czisch, M., Fernández, G., et al. (2018). Hippocampal-caudate nucleus interactions support exceptional memory performance. Brain Struct. Funct. 223, 1379–1389. doi: 10.1007/s00429-017-1556-2
Nyberg, L., McIntosh, A. R., and Tulving, E. (1998). Functional brain imaging of episodic and semantic memory with positron emission tomography. J. Mol. Med. 76, 48–53.
Otchy, T. M., Wolff, S. B., Rhee, J. Y., Pehlevan, C., Kawai, R., Kempf, A., et al. (2015). Acute off-target effects of neural circuit manipulations. Nature 528, 358–363. doi: 10.1038/nature16442
Packard, M. G., Hirsh, R., and White, N. M. (1989). Differential effects of fornix and caudate nucleus lesions on two radial maze tasks: evidence for multiple memory systems. J. Neurosci. 9, 1465–1472. doi: 10.1523/JNEUROSCI.09-05-01465.1989
Panatier, A., Theodosis, D. T., Mothet, J. P., Touquet, B., Pollegioni, L., Poulain, D. A., et al. (2006). Glia-derived D-serine controls NMDA receptor activity and synaptic memory. Cell 125, 775–784. doi: 10.1016/j.cell.2006.02.051
Penfield, W. (1958). Centrencephalic integrating system. Brain 81, 231–234. doi: 10.1093/brain/81.2.231
Platt, J. R. (1964). Strong Inference: certain systematic methods of scientific thinking may produce much more rapid progress than others. Science 146, 347–353. doi: 10.1126/science.146.3642.347
Poo, M. M., Pignatelli, M., Ryan, T. J., Tonegawa, S., Bonhoeffer, T., Martin, K. C., et al. (2016). What is memory? The present state of the engram. BMC Biol. 14:40. doi: 10.1186/s12915-016-0261-6
Poulos, A. M., and Thompson, R. F. (2015). Localization and characterization of an essential associative memory trace in the mammalian brain. Brain Res. 1621, 252–259. doi: 10.1016/j.brainres.2014.10.068
Qin, Y. L., McNaughton, B. L., Skaggs, W. E., and Barnes, C. A. (1997). Memory reprocessing in corticocortical and hippocampocortical neuronal ensembles. Philos. Trans. R. Soc. Lond. B Biol. Sci. 352, 1525–1533. doi: 10.1098/rstb.1997.0139
Ramadan, W., Eschenko, O., and Sara, S. J. (2009). Hippocampal sharp wave/ripples during sleep for consolidation of associative memory. PLoS ONE 4:e6697. doi: 10.1371/journal.pone.0006697
Rauchs, G., Desgranges, B., Foret, J., and Eustache, F. (2005). The relationships between memory systems and sleep stages. J. Sleep Res. 14, 123–140. doi: 10.1111/j.1365-2869.2005.00450.x
Sara, S. J. (2010). Reactivation, retrieval, replay and reconsolidation in and out of sleep: connecting the dots. Front. Behav. Neurosci. 4:185. doi: 10.3389/fnbeh.2010.00185
Sara, S. J. (2017). Sleep to Remember. J. Neurosci. 37, 457–463. doi: 10.1523/JNEUROSCI.0297-16.2017
Sara, S. J., and Hars, B. (2006). In memory of consolidation. Learn. Mem. 13, 515–521. doi: 10.1101/lm.338406
Schacter, D. L., Eich, J. E., and Tulving, E. (1978). Richard Semon's theory of memory. J. Verbal Learning Verbal Behav. 17, 721–743. doi: 10.1016/S0022-5371(78)90443-7
Sekeres, M. J., Winocur, G., and Moscovitch, M. (2018). The hippocampus and related neocortical structures in memory transformation. Neurosci. Lett. doi: 10.1016/j.neulet.2018.05.006. [Epub ahead of print].
Skelin, I., Kilianski, S., and McNaughton, B. L. (2018). Hippocampal coupling with cortical and subcortical structures in the context of memory consolidation. Neurobiol. Learn. Mem. doi: 10.1016/j.nlm.2018.04.004. [Epub ahead of print].
Song, X., Roy, B., Kang, D. W., Aysola, R. S., Macey, P. M., Woo, M. A., et al. (2018). Altered resting-state hippocampal and caudate functional networks in patients with obstructive sleep apnea. Brain Behav. 8:e00994. doi: 10.1002/brb3.994
Südhof, T. C. (2015). Reproducibility: experimental mismatch in neural circuits. Nature 528, 338–339. doi: 10.1038/nature16323
Sukumar, D., Rengaswamy, M., and Chakravarthy, V. S. (2012). Modeling the contributions of basal ganglia and hippocampus to spatial navigation using reinforcement learning. PLoS ONE 7:e47467. doi: 10.1371/journal.pone.0047467
Thompson, R. (1993). Centrencephalic theory, the general learning system, and subcortical dementia. Ann. N. Y. Acad. Sci. 702, 197–223. doi: 10.1111/j.1749-6632.1993.tb17249.x
Thompson, R., Crinella, F. M., and Yu, J. (1990). Brain Mechanisms in Problem Solving and Intelligence: A Lesion Survey of the Rat Brain. New York, NY: Springer Science and Business Media.
Thompson, R. F. (1986). The neurobiology of learning and memory. Science 233, 941–947. doi: 10.1126/science.3738519
Thompson, R. F. (2005). In search of memory traces. Annu. Rev. Psychol. 56, 1–23. doi: 10.1146/annurev.psych.56.091103.070239
Thompson, R. F. (2013). An essential memory trace found. Behav. Neurosci. 127, 669–675. doi: 10.1037/a0033978
Thorne, M. (1995). Robert thompson: Karl Lashley's heir? J. Hist. Behav. Sci. 31, 129–136. doi: 10.1002/1520-6696(199504)31:2<129::AID-JHBS2300310203>3.0.CO;2-4
Tonegawa, S., Liu, X., Ramirez, S., and Redondo, R. (2015). Memory engram cells have come of age. Neuron 87, 918–931. doi: 10.1016/j.neuron.2015.08.002
Wang, S. H., and Morris, R. G. (2010). Hippocampal-neocortical interactions in memory formation, consolidation, and reconsolidation. Annu. Rev. Psychol. 61, 49–79, C41–44. doi: 10.1146/annurev.psych.093008.100523
White, N. M., Packard, M. G., and McDonald, R. J. (2013). Dissociation of memory systems: the story unfolds. Behav. Neurosci. 127, 813–834. doi: 10.1037/a0034859
Wiltgen, B. J., and Tanaka, K. Z. (2013). Systems consolidation and the content of memory. Neurobiol. Learn. Mem. 106, 365–371. doi: 10.1016/j.nlm.2013.06.001
Woolley, D. G., Mantini, D., Coxon, J. P., D'Hooge, R., Swinnen, S. P., and Wenderoth, N. (2015). Virtual water maze learning in human increases functional connectivity between posterior hippocampus and dorsal caudate. Hum. Brain Mapp. 36, 1265–1277. doi: 10.1002/hbm.22700
Xu, J., Potenza, M. N., Calhoun, V. D., Zhang, R., Yip, S. W., Wall, J. T., et al. (2016). Large-scale functional network overlap is a general property of brain functional organization: reconciling inconsistent fMRI findings from general-linear-model-based analyses. Neurosci. Biobehav. Rev. 71, 83–100. doi: 10.1016/j.neubiorev.2016.08.035
Keywords: memory, memory trace, history, technology, cell assembly, functional circuits, engram, subcortical-cortical connectivity
Citation: Devan BD, Berger K and McDonald RJ (2018) The Emergent Engram: A Historical Legacy and Contemporary Discovery. Front. Behav. Neurosci. 12:168. doi: 10.3389/fnbeh.2018.00168
Received: 22 March 2018; Accepted: 16 July 2018;
Published: 07 August 2018.
Edited by:
Nadine Ravel, INSERM U1028 Centre de Recherche en Neurosciences de Lyon, FranceReviewed by:
Susan J. Sara, Collège de France, FranceCopyright © 2018 Devan, Berger and McDonald. This is an open-access article distributed under the terms of the Creative Commons Attribution License (CC BY). The use, distribution or reproduction in other forums is permitted, provided the original author(s) and the copyright owner(s) are credited and that the original publication in this journal is cited, in accordance with accepted academic practice. No use, distribution or reproduction is permitted which does not comply with these terms.
*Correspondence: Bryan D. Devan, bdevan@towson.edu