- 1Department of Psychology, Arizona State University, Tempe, AZ, United States
- 2Department of Psychology, University of Kentucky, Lexington, KY, United States
In drug addiction, cues previously associated with drug use can produce craving and frequently trigger the resumption of drug taking in individuals vulnerable to relapse. Environmental stimuli associated with drugs or natural reinforcers can become reliably conditioned to increase behavior that was previously reinforced. In preclinical models of addiction, these cues enhance both drug self-administration and reinstatement of drug seeking. In this review, we will dissociate the roles of conditioned stimuli as reinforcers from their modulatory or discriminative functions in producing drug-seeking behavior. As well, we will examine possible differences in neurobiological encoding underlying these functional differences. Specifically, we will discuss how models of drug addiction and relapse should more systematically evaluate these different types of stimuli to better understand the neurobiology underlying craving and relapse. In this way, behavioral and pharmacotherapeutic interventions may be better tailored to promote drug use cessation outcomes and long-term abstinence.
Introduction
Relapse triggered by cues associated with drugs of abuse is a hallmark of addiction and is a primary contributor to impeding success in maintaining abstinence. Discrete and contextual stimuli associated with previous drug use can initiate craving in individuals with substance dependence, potentially leading to subsequent relapse (Childress et al., 1993). In fact, it has been hypothesized that compulsive drug use could be a form of automatized behavior that is stereotyped, challenging to regulate, occurs separate from awareness, and is bound to stimuli associated with the drug (Tiffany and Carter, 1998). Individuals become sensitive to environmental stimuli that acquire motivational salience through repeated pairings with primary reinforcers, such as the presentation of food or drug (Bouton et al., 1993; O'Brien, 2003; Kalivas and Volkow, 2005; Weiss, 2010). Animal studies have established that these cues enhance drug self-administration, illustrating the importance of cues in maintaining drug use (Caggiula et al., 2001; Schenk and Partridge, 2001). These environmental stimuli become conditioned with the primary reinforcing effects of abused drugs, as can discriminative stimuli that predict drug presentation, both of which consistently produce drug seeking in animal models of cue-induced relapse (See, 2002; Shaham et al., 2003; Crombag et al., 2008; Bossert et al., 2013). Interestingly, cocaine-related stimuli (presented non-contingently) can evoke drug conditioned responses in cocaine-dependent humans (Ehrman et al., 1992).
Contingent, discrete environmental cues associated with drugs of abuse can produce increases in behavior previously reinforced by the drug when presented to an animal under conditions of abstinence. Additionally, physical environments contain a constellation of stimuli that can become occasion setters (OSs) and modulate the response-eliciting efficacy of discrete Pavlovian conditioned stimuli paired with drug self-administration (Gerber and Stretch, 1975). Likewise, these cues can serve a discriminatory function that predicts the availability of a drug of abuse upon the completion of a particular emitted response (Weiss et al., 2001). In addition to these salient, motivating cues or contexts, reinstatement of drug seeking (typically defined as increased responding on a previously drug-paired operandum following extinction) can be produced following presentation of other stimuli, including footshock or pharmacological stressors such as yohimbine (Erb et al., 1996; Buczek et al., 1999; Shaham et al., 2000) or priming injections of the previously self-administered drug (Gerber and Stretch, 1975).
Although the above preclinical models are frequently used to examine the neurobiology of drug relapse, it is important to critically examine their predictive validity (Epstein et al., 2006). It has been proposed that the process involved in behavioral change is unstable, thus lapse and relapse are likely (Bouton, 2014). From a learning perspective, changing behavior (i.e., inhibiting relapse) can be difficult since learning a new behavior does not erase the old behavior that is dependent on the context in which it is learned (Bouton, 2014; Bouton and Todd, 2014). Thus, lapse and relapse are especially probable if extinction of the drug-related behavior does not occur in the context in which drugs were taken. With that caveat, the reinstatement model of drug relapse has been widely used to examine the neurobiology underpinning lapse and relapse, and has been used to characterize potential vulnerabilities in the propensity to relapse (Knackstedt and Kalivas, 2009; Robinson et al., 2014).
In this review, we will examine the neurobiology underlying the process of encoding drug-related cues and how these cues might be encoded differentially depending on how they modulate behavior. As well, we will explore the neurobehavioral mechanisms underlying differential stimulus control of cues in motivated behaviors. Finally, we will discuss the importance of systematically examining and describing different types of cues in the discovery of neurobiological underpinnings of motivated behavior.
Role of Cues in Drug-Seeking Behavior
Stimuli that are consistently paired with reward or reinforcers can come to influence reward-related behavior on their own, including drugs of abuse. These stimulus-reward relations are most obvious in Pavlovian paradigms, where a stimulus reliably predicts the occurrence of a food or drug reward. In addition to Pavlovian control, stimuli can also serve a discriminative or modulatory function, informing the organism as to the availability of a reward. Specifically, these stimuli inform an organism when a particular action will result in a specific reward (i.e., discriminative stimulus; SD; (Shahan, 2010), or when a specific stimulus will reliably predict a particular reward (occasion setter; OS; Holland, 1998); these modulatory relationships can also come to guide reward-related behavior on their own. Intriguingly, it appears that the neurobehavioral mechanisms engaged by a stimulus are dependent upon what function it serves (reinforcing or modulatory; Willuhn et al., 2010; Beckmann and Chow, 2015). Thus, understanding the role of stimulus-reward relationships in substance abuse and other reward-associated pathologies first requires an understanding of the neurobehavioral mechanisms involved in the different functional relationships between reward-associated stimuli and behavior. Above all, the dissociation of the neurobehavioral mechanisms underlying differential stimulus control might reveal novel, more-specific neurobehavioral targets to treat reward-associated pathologies, especially substance use disorders.
Control of Drug Seeking by Contingent Pavlovian Conditioned Stimuli
When an initially neutral stimulus is contiguously and consistently paired with a primary reward, like food or an abused drug, that stimulus can come to elicit responses on its own that are related to the primary reward it has been paired with, becoming a conditioned stimulus (CS). In preclinical models, one way experimenters utilize this process to study the role of stimulus control in drug-related behavior is to pair a neutral stimulus with an intravenous infusion of a drug of abuse; this is typically done by presenting the stimulus simultaneously with the drug infusion following an operant response (e.g., lever press). The repeated pairing with the primary (rewarding) effects of the drug transforms the stimulus into a drug-associated CS that can maintain the lever press alone.
Behavioral control via a CS is typically exhibited by response contingent CS-induced reinstatement of lever pressing following some extended period of extinction. Specifically, following the training period, the experimenter places the animal under extinction conditions, where lever presses no longer lead to the drug infusion or the associated CS. Over time, lever press behavior will decrease under extinction conditions. The experimenter will then present the CS alone following each lever press during reinstatement test sessions. The control of lever pressing via the CS is exhibited by the once extinguished operant response (lever press) being reinstated through the response contingent presentation of the drug-associated CS alone. It should also be noted that “incubation of craving” is another model in which contingent CSs maintain lever pressing alone. In this model, the animal undergoes forced abstinence for a period of time. When placed back into the drug-associated context (i.e., operant chamber), animals will emit lever presses under control of a contingent CS alone. Generally speaking, the amount of emitted lever pressing increases as more time elapses in forced abstinence (Conrad et al., 2008), hence the “incubation” of CS efficacy to maintain operant lever presses alone.
Importantly, the presentation of the CS alone during a reinstatement test session is contingent upon the previously trained lever-press behavior. Thus, from a behavioral perspective, the mechanism responsible for the reinstatement of the previously extinguished lever pressing is the conditioned reinforcing effects of the drug-associated CS. Through the repeated pairings between the CS and drug during self-administration, the CS comes to acquire reinforcing properties capable of maintaining lever pressing on its own. Although, it is important to note that these properties are short-lived without maintaining the drug-CS association. In typical contingent cue-induced reinstatement paradigms, lack of reconditioning to the CS results in an extinction of CS-related conditioned reinforcement with repeated reinstatement test sessions.
The conditioned reinforcing efficacy of drug-associated CSs is the most commonly used method for studying stimulus control of drug-seeking behavior (Bossert et al., 2013) in preclinical models and has demonstrated to be an effective method of study for many drug classes, including stimulants (Gipson et al., 2013a; Parsegian and See, 2014) and opiates (Bossert et al., 2005). Additionally, as stated above, the CS model has been extended to study long-term control of drug-associated stimuli following long periods of forced abstinence. Specifically, these studies have shown that the drug-associated CS can maintain greater rates of discrete cue-reinforced responding following longer periods of forced abstinence (Tran-Nguyen et al., 1998; Pickens et al., 2011). However, because of its contingent nature, it is not likely to model all types of stimulus control that individuals with substance use disorders are subjected to, as substance abusers are often exposed to drug-associated cues through non-contingent means.
Control of Drug Seeking by Non-contingent Stimuli
Non-contingent stimuli often take on a modulatory function, as opposed to the reinforcing function assumed by discrete Pavlovian CSs; they can serve to either inform the organism when a Pavlovian CS will effectively predict a future reward or when a particular action will result in a specific consequence. Despite the modulatory nature of these cues, they can have powerful control over drug seeking. First, it has been shown that context can serve as an OS, modulating the reinforcing efficacy of a drug-associated CS (Crombag and Shaham, 2002; Crombag et al., 2008). Following training of self-administration with a drug-CS relationship within a particular context (A), animals are then placed into a different context and responses then lead only to the CS alone (B). After responses for the CS alone have extinguished, animals are then placed back into the original training context (A), where responses again are followed only by the CS alone. This return to the original training context (A) after extinction in a different context (B) results in the renewal of responding for the CS.
Additionally it has been shown that environmental context can serve as a discriminative stimulus (SD). Specifically, the context in which an individual is placed can differentially signal the availability of drug following a particular operant response (Fuchs et al., 2007, 2008; Crombag et al., 2008; Lasseter et al., 2009, 2010, 2011, 2014; Ramirez et al., 2009). Following self-administration within a specific context (A), animals are then placed into a separate context (B) where responses result in no consequences. Following extinction of responding in the extinction context (B), animals are then returned to the original training context (A), where responding once again has no consequences. However, return to the original training context alone increases drug seeking responses. An SD can also signal duration of access to drug self-administration and intake patterns can be brought under discriminative control. Specifically, the extended access model of self-administration (Ahmed and Koob, 1999), which is used to investigate a potential dysregulation of drug intake relevant to addiction in humans, has recently been found to be subject to stimulus control (Beckmann et al., 2012).
In addition to context, discrete SDs have been used to study stimulus control of drug seeking, where specific stimuli can be used to signal the availability of drug, the non-availability of drug, or the availability of a non-drug reinforcer (e.g., food; Weissenborn et al., 1995; Weiss et al., 2000, 2001, 2003; Stairs et al., 2010; McCuddy et al., 2014), all upon a specific operant response. This approach relies on the use of a multiple schedule, where changes in response-outcome contingency are split into components that are differentially signaled by a specific stimulus change throughout training. For example, left lever presses may lead to cocaine, but only in components coupled with the presence of a particular visual stimulus (e.g., left lever light), while right lever presses during this component are without consequence. Conversely, in the presence of a different stimulus (e.g., right lever light) during alternate components, right lever presses lead to food, while left lever presses within the alternate component are without consequence. Components typically alternate for fixed periods of time either within or across each training session. Following extinction of responses to either lever in the absence of either SD, non-contingent re-exposure to the previously trained SDs alone will specifically increase the response associated with that SD.
As discussed above, both CSs used as conditioned reinforcers and non-contingent exposure to SDs can reinstate drug-seeking behavior, although it appears they do so through different neurobehavioral processes. For instance, while contingent presentation of a drug-paired CS can reinstate drug seeking and produce alterations in accumbens synaptic plasticity, non-contingent presentation of the same CS does not (Grimm et al., 2000; Gipson et al., 2013a). These data support that reinstatement of drug seeking via CSs is operant and reinforced by the drug-paired CS. Contingent access to cocaine-associated SDs is much less robust than CSs, thus illustrating an important mechanistic difference between control of drug seeking through CSs and SDs (Di Ciano and Everitt, 2003). Notably, there are individual differences among rats in incentive salience attributed to discrete conditioned cues vs. non-contingent discriminative cues (Saunders and Robinson, 2010; Saunders et al., 2014). Specifically, discrete cocaine-associated cues increase drug seeking in rats that attribute motivational value to a discrete food cue, an effect that is absent in rats that do not attribute incentive salience to the food cue (Saunders and Robinson, 2010). Conversely, non-contingent SDs are less robust in renewing extinguished drug seeking in rats that attribute motivational value to a discrete food cue (Saunders et al., 2014). Taken together, these findings highlight the mechanistic differences between these varying stimulus modalities. Below we provide a detailed discussion of literature that suggests different neural mechanisms may underlie these distinct behavioral processes.
Neurobiological Differences in Encoding Conditioned vs. Discriminative Cues Associated with Natural and Drug Rewards
Understanding the neural mechanisms underlying stimulus control over drug-seeking behavior is critical to our understanding of drug addiction and relapse as a pathological disease state and developing effective treatment strategies to promote drug abstinence. In this section, we will first describe neuroadaptations within dopaminergic (DAergic) and glutamatergic systems found to underlie different types of cue-induced motivated behavior (from the current literature when possible). We will then describe drug-induced perturbations to other cellular and molecular mechanisms that are differentially expressed between conditioned and discriminative stimuli. Finally, we will highlight what future research is needed to examine novel targets that may underlie different types of cue-triggered motivated behavior, which may prove to be potentially effective pharmacotherapeutic avenues to improve drug use cessation outcomes. Tables 1, 2 provide a summary of neurobiological mechanisms underlying CS- and SD-induced reinstatement (respectively) across drug classes.
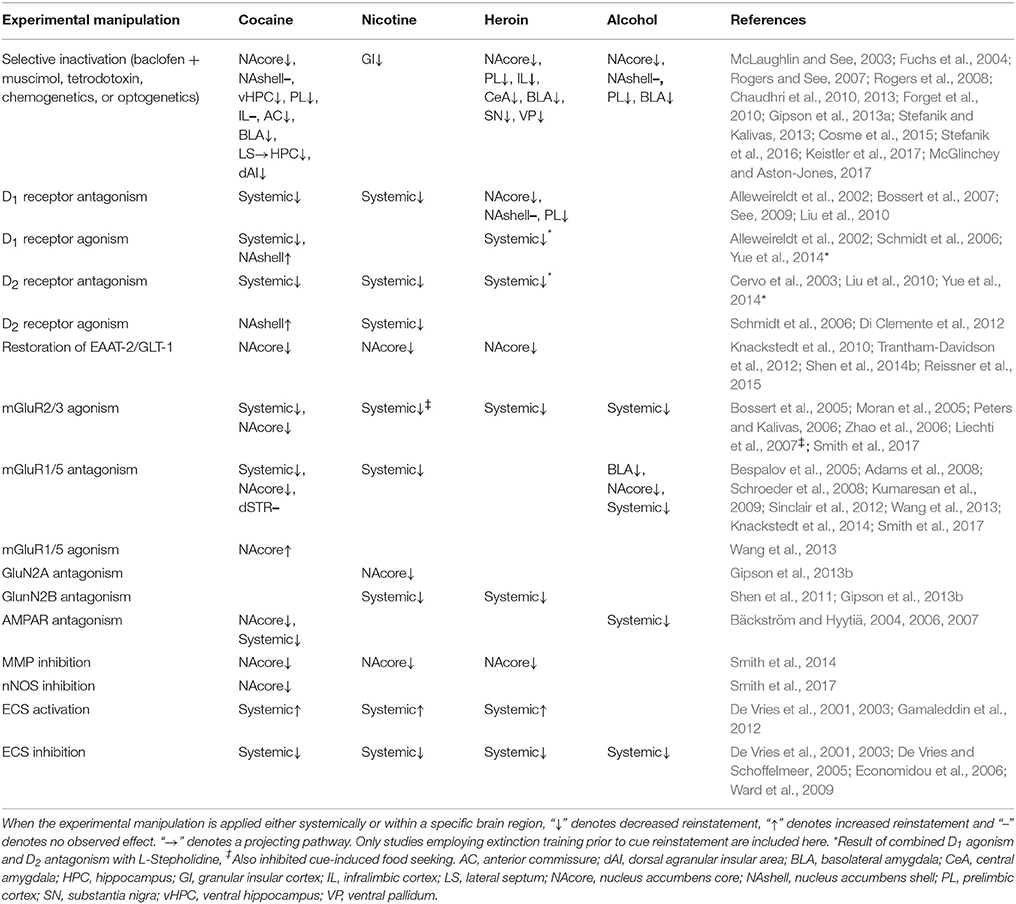
Table 1. Summary of neurobiological mechanisms underlying CS-induced reinstatement across drug classes.
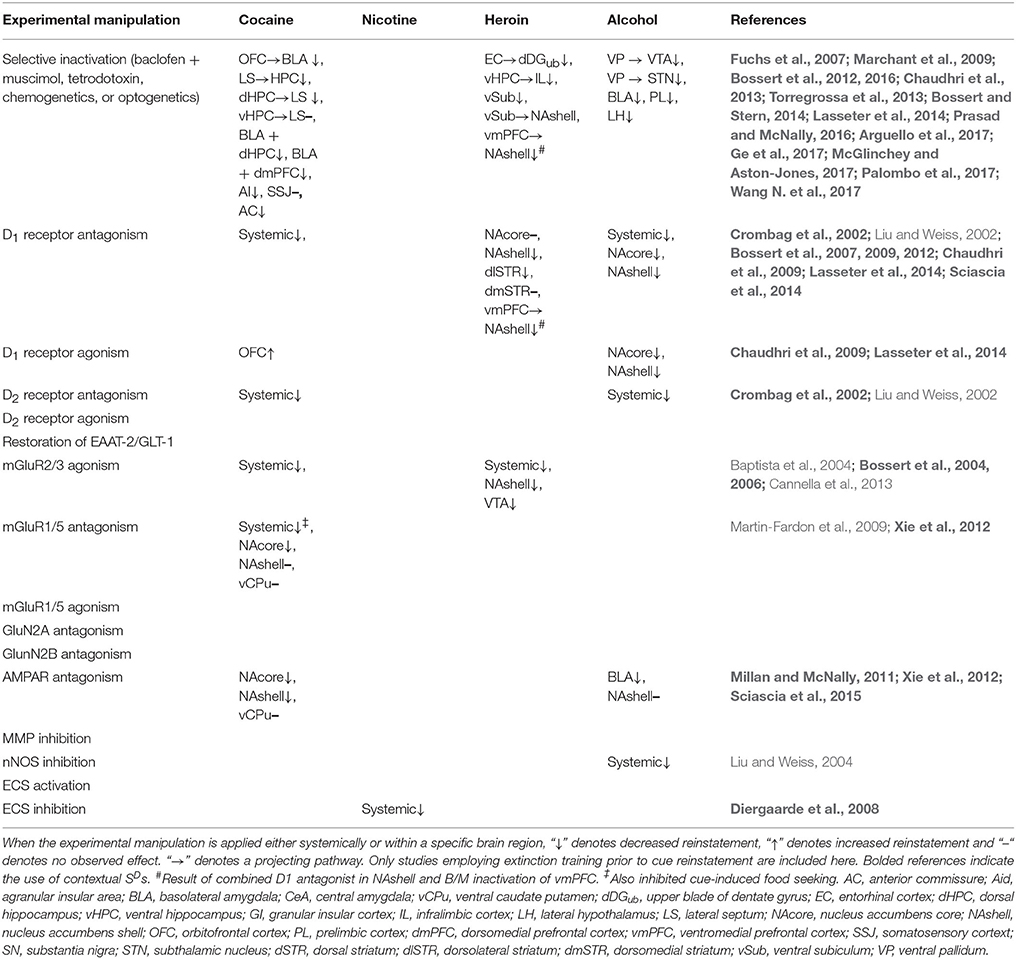
Table 2. Summary of neurobiological mechanisms underlying SD-induced reinstatement across drug classes.
Dopamine Modulates Drug-Induced Plasticity and Drug Seeking in a Cue-Specific Manner
Cues that are conditionally paired with rewarding stimuli can alter patterns of neuronal activity, cell morphology, synaptic plasticity, and signal transduction. For instance, it is well known that natural reward-predicting CSs elicit responses in midbrain dopamine (DA) neurons such that neurons encode reward value and response pattern shifts from the occurrence of the reward itself to the CS associated with the reward (Waelti et al., 2001). Conditional and discriminative stimuli recruit distinct neurobiological mechanisms, where activation of certain neuronal subpopulations such as in the nucleus accumbens shell (NAshell) and core (NAcore) subcompartments, sustains motivated behavior that is dependent on stimulus function (Pezze et al., 2001; Ito et al., 2004; Kobayashi and Schultz, 2014).
The NAcore is composed mainly of GABAergic medium spiny neurons (MSNs), accounting for 90–95% of the neuronal cell types within the striatum (Hedreen, 1981; Bolam, 1984; Chang and Kitai, 1985; Meredith, 1999). MSNs are typically segregated into one of two distinguishable populations, as they express either DA D1- or D2-like receptors (Gerfen et al., 1990; Gerfen and Surmeier, 2011). DA released from ventral tegmental area (VTA) efferents into the NA binds to these G-protein coupled receptors (GPCRs) on MSNs (Gerfen et al., 1987; Jimenez-Castellanos and Graybiel, 1987; Davidson and Stamford, 1993; Sesack et al., 2003). Although historically it was thought that D1 and D2-expressing MSNs were exclusively part of the direct and indirect basal ganglia pathways (Gerfen et al., 1990) and that manipulation of D1 or D2 MSNs specifically illustrates pathway specificity (Bock et al., 2013; MacAskill et al., 2014), recent studies have shown that this subdivision of cell type is not synonymous with these distinct pathways in projections of the NA (Smith et al., 2013; Kupchik et al., 2015; Kupchik and Kalivas, 2017). DAergic neurons in the VTA respond during conditioned behavior (Ljungberg et al., 1992) and, as described in detail below, conditioned and discriminative cues incorporate distinct DAergic mechanisms to modulate drug-induced neurobehavioral plasticity. Specifically, a vast body of literature has evaluated dopaminergic neuromodulation of drug-seeking behavior. Figures 1, 2 provide a non-exhaustive depiction of differential and overlapping glutamatergic and DAergic circuits found to be important in the induction of motivated drug seeking behavior by both types of cues. It should be noted that some projections may be involved in both types of motivated behavior, however these studies may not have been conducted yet to show overlapping (or differential) involvement.
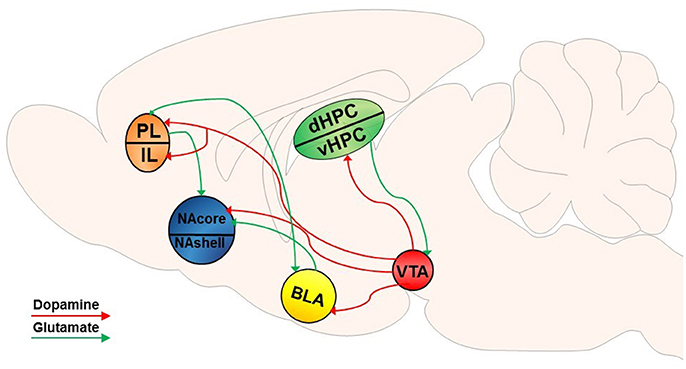
Figure 1. Dopamine-glutamate circuitry underlying CS-induced reinstatement of drug seeking. VTA dopamine (DA) input into the NAcore mediates CS-induced reinstatement. DA input into the PFC may also modulate rapid, transient glutamatergic plasticity in the NAcore in response to drug-associated CSs and mediates drug seeking behavior. DA input into the BLA may alter excitatory inputs into the NAcore and reciprocal BLA-PFC signaling. As well, DA input into the vHPC has been implicated in CS-induced reinstatement of drug seeking, and glutamatergic projections from the vHPC to the VTA may be an indirect pathway through which the HPC modulates dopaminergic input into other brain regions.
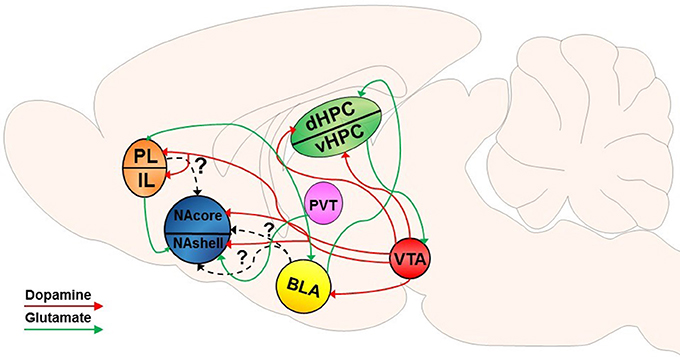
Figure 2. Dopamine-glutamate circuitry underlying SD-induced reinstatement of drug seeking. VTA dopamine (DA) input into the NAcore and NAshell mediates SD-induced reinstatement. DA input into the BLA and PFC have also been implicated in SD-induced reinstatement of drug seeking. Projections from the PFC to the NAshell have been implicated in SD-induced reinstatement; however, it is not known if PFC projections to the NAcore play a specific role in SD-induced reinstatement. Likewise, it is unclear if projections from the BLA directly to the NA play a role in SD-induced reinstatement akin to CS-induced reinstatement. The dHPC receives converging input from the BLA and VTA and has been implicated in SD-induced reinstatement. Similar to CS-induced reinstatement, the vHPC communicates bidirectionally with the VTA and may modulate dopaminergic input into the NA, PFC, and BLA. As well, glutamatergic projections from the PVT to the NAshell have also been shown to be involved in SD-induced drug seeking. Dotted lines indicate unknown effects of specific projecting pathways.
Dopaminergic signaling in CS-induced reinstatement of drug seeking
A rich body of literature has shown that after repeated pairings of a stimulus with an appetitive reward, DA neurons in the pars compacta of the substantia nigra and VTA respond phasically to CSs rather than to the reward itself and are dependent on event unpredictability (Schultz et al., 1997; Hollerman and Schultz, 1998; Schultz, 1998). GPCRs are modulatory and historically have been thought to deliver general neuromodulation with relatively slow time resolution. Given the possible dichotomous role of neuromodulatory DA signaling in delivering both fast and slow-timed information to other systems (Schultz et al., 1997), it is likely that extracellular DA release during relapse delivers precisely timed information to specific structures altering the neural environment in a modulatory manner and impacting behavioral output. For example, DA signaling could deliver precise information to impact rapid, transient plasticity associated with drug seeking (described in detail below).
Recent studies have begun to further elucidate the role of NAcore DA release in the reinstatement of drug seeking as well as in the neurobiological changes that occur within the NAcore during reinstatement. For instance, Spencer et al. (2016) reported that re-administration of cocaine, which increases extracellular DA (Willuhn et al., 2010), reversed the rapid, transient plasticity associated with CS-induced cocaine seeking (involvement of rapid, transient glutamatergic plasticity during drug seeking is described in more detail below). Additionally, antagonism of VTA D1 (Alleweireldt et al., 2002) and D2 receptors has been shown to inhibit both reinstated cocaine seeking as well as rapid synaptic plasticity in the NAcore (Shen et al., 2014a). Another recent study discovered a positive correlation between prelimbic (PL)-NAcore pathway activation and CS-induced cocaine reinstatement (McGlinchey et al., 2016). This projection was found to be DA-dependent, where pharmacological inhibition of DA signaling in the PL attenuated discrete CS-induced cocaine reinstatement but not sucrose seeking in drug-naïve animals. This DAergic neuromodulation of prefrontal glutamate release into the NA is also supported by a previous study that found that DA efflux was only increased in the dorsomedial prefrontal cortex (dmPFC) and not in the NA during CS-induced, methamphetamine-induced, and CS + methamphetamine-induced reinstatement (Parsegian and See, 2014). Taken together, these results highlight the possibility that extracellular DA release may suppress NAcore glutamatergic plasticity associated with discrete CS-induced drug seeking. As well, these data further support the role of DA in modulating glutamatergic signaling during motivated behavior.
DAergic signaling may also serve to encode prediction errors to adjust reward-seeking behavior in response to new environmental contingencies (Hollerman and Schultz, 1998; Schultz, 1998). Fast-scan cyclic voltammetry (FSCV) studies, which use electrodes that emit fast, alternating electrical currents that rapidly oxidize and reduce an electroactive molecule of interest to monitor rapid fluctuations in extracellular levels, have shown that DA release can change rapidly and transiently in response to distinct conditioned stimuli that predict changes in reward. For instance, it has been demonstrated that a discrete CS that is paired with delayed cocaine availability is associated with blunted DA levels, which are then rapidly elevated when a discrete cue paired with immediate cocaine delivery is presented (Wheeler et al., 2011). Another study utilizing FSCV found that DA was released in the NA transiently in response to a discrete auditory cue paired with a food reward during appetitive Pavlovian conditioning. Extinction training significantly reduced DA release in response to the cue but was reinstated after two non-contingent presentations of the reward (Sunsay and Rebec, 2014). There is also evidence to suggest that mesolimbic DA signals are transduced differentially following the presentation of a reward-paired cue and directly following a goal-directed behavior (e.g., a lever press). For instance, one study found that DA-mediated neuronal responses to conditioned cues associated with intracranial stimulation of the VTA specifically recruited D2-expressing neurons in the NA, whereas DA-mediated responses that were temporally proximal to lever presses involved both D1- and D2-expressing neurons (Owesson-White et al., 2016). These studies demonstrate that DA neurotransmission and signal transduction can change rapidly and transiently in response to drug-associated CSs and following goal-directed behavior to modulate future reward-seeking behavior.
Research investigating the dichotomous functional roles of the NAcore and NAshell indicates that DA input into these two sub-regions depends on behavioral and environmental conditions. For example, non-contingent presentation of a discrete conditioned stimulus (i.e., a light) that was previously paired with an infusion of cocaine selectively increased DA into the NAcore, while DA levels were elevated in both the NAcore and NAshell following intravenous cocaine self-administration in rats (Ito et al., 2000). As mentioned previously, non-contingent exposure to drug-paired CSs does not sufficiently reinstate drug seeking or produce changes in synaptic plasticity within the NA. As such, the neuromodulatory influence of DA on neurobehavioral plasticity in response to drug-paired cues may be dependent on the contingency between the operant response and the CS together, as opposed to the CS itself. Specifically, the CS-induced surge of DA may essentially reinforce the behavioral response. Similar to the disparate roles of the NAcore and NAshell in CS-induced reinstatement, D1- and D2-type MSNs also appear to have distinct functional roles in mediating drug seeking. For instance, one recent study found that loss of GABAergic plasticity in D2-type (but not D1-type) MSNs projecting to the ventral pallidum (VP) potentiates CS-induced reinstatement of cocaine seeking (Heinsbroek et al., 2017). As well, we recently discovered that D1(+) rather than D1(–) MSNs mediate rapid, transient synaptic plasticity during CS-induced cocaine seeking within the NAcore (Bobadilla et al., 2017). Together, these studies highlight the differential roles of D1- vs. D2-type MSN signaling in CS-induced drug seeking.
Dopaminergic signaling in SD-induced reinstatement of drug seeking
Although DA has been shown to be important in SD-induced drug seeking behavior (e.g., alcohol, Sciascia et al., 2014; Marchant and Kaganovsky, 2015; cocaine, Lasseter et al., 2014; heroin, Bossert et al., 2009), it remains unclear if DA impacts rapid synaptic plasticity associated with SD-induced drug seeking. The only study to date examining rapid plasticity in SD-induced drug seeking did not examine DAergic modulation of this neural event (Stankeviciute et al., 2014). Unlike CS-induced reinstatement, studies utilizing SD-induced reinstatement of drug seeking following extinction training demonstrate that both the NAcore and NAshell are involved in SD-induced drug seeking (Fuchs et al., 2004, 2008; Chaudhri et al., 2009). However, one study has demonstrated that antagonism of D1 receptors in the NAshell, but not the NAcore, is sufficient to attenuate SD-induced reinstatement of heroin seeking (Bossert et al., 2007). While research on this topic is limited, it appears SD-induced drug seeking may not employ the same degree of DA receptor specificity as CS-induced reinstatement. Nevertheless, antagonism of DA receptors in the dorsal prefrontal cortex (dPFC), which receives DA input from the VTA and sends excitatory glutamatergic projections to the NA, was shown to inhibit cocaine-primed reinstatement, while DA release into the NAcore and ventral pallidum (VP) were not causally associated with drug-primed reinstatement (McFarland and Kalivas, 2001). Considering prefrontal glutamate release into the NA is required for cocaine-primed reinstatement of drug seeking (McFarland et al., 2003), it may be that DAergic stimulation of Gs-coupled D1 receptors in the PFC is necessary for activation of glutamatergic projections to the NA, a mechanism which may extend to SD-induced drug seeking (Sciascia et al., 2014).
Dopaminergic projections to other neural structures involved in CS- and SD-induced drug seeking
Beyond the NA, the functional roles of the amygdala (AMY) and the hippocampus (HPC) in reward seeking are a subject of intense study, as both regions communicate either directly or indirectly with the VTA and project to the NA. Importantly, previous studies have revealed their role in mediating cue-triggered behavioral responses. For instance, it has been demonstrated that selective inactivation of the dorsal hippocampus (dHPC), the basolateral amygdala (BLA), and the dmPFC abolishes contextual SD-induced cocaine reinstatement, whereas inactivation of the dHPC did not alter discrete CS- or drug-induced reinstatement (Fuchs et al., 2005). However, context by nature is a complex spatial array of stimuli, and thus use of a punctate stimulus could uncover differential involvement of various sub-regions of the HPC. Other studies suggest that the ventral hippocampus (vHPC) may also be involved in SD-induced (Lasseter et al., 2010) as well as CS- and drug-induced reinstatement (Rogers and See, 2007). The HPC-VTA loop has been characterized by several studies (see Lisman and Grace, 2005, for review) and is thought to contribute to long-term potentiation (LTP) and changes in synaptic plasticity required for learning and memory in goal-directed tasks (Wise, 2004). One study found that stimulation of the vHPC increased VTA DA neuron activity and NA DA levels, suggesting a neuromodulatory role of the vHPC on DAergic input into the NA (Legault et al., 2000). Curiously, another study found that vHPC activation increased DA levels in the NAshell and that dHPC activation had an inhibitory effect on extracellular DA levels in the NAcore (Peleg-Raibstein and Feldon, 2006), which might suggest that the NAcore is not involved in HPC-mediated activation of VTA DA release in SD-induced reinstatement.
In addition to the HPC, the BLA receives significant DAergic input and appears to be especially involved in mediating CS-induced reinstatement (Weiss et al., 2000; See et al., 2001; Kantak et al., 2002). Specifically, both D1- and D2-like receptors in the BLA appear to be involved in the formation of cocaine-CS associations that are required for CS-induced reinstatement of cocaine seeking (Berglind et al., 2006). As well, the BLA and PFC form strong reciprocal connections that have been shown to regulate contextual SD-induced reinstatement of cocaine seeking (Lasseter et al., 2011). As it appears, the functional heterogeneity of brain regions such as the NA, AMY, and HPC may be demonstrated with distinct cues that recruit specific and potentially differential neural pathways to promote the acquisition and maintenance of reward seeking as well as relapse vulnerability.
Glutamatergic Plasticity Mediates Drug Seeking and Relapse Vulnerability
Dysregulations in glutamate neurotransmission between key brain regions along the mesocorticolimbic pathway have been found to underlie the chronic, compulsive, relapsing use of drugs that characterizes addiction. Several studies have elucidated key glutamatergic pathways along the reward circuit that are recruited differentially depending on the type of relapse model being utilized. Projections from the PFC (prelimbic and infralimbic cortex; PL and IL, respectively), AMY, HPC, and thalamus (THAL) innervate the NAshell (Groenewegen et al., 1999). Importantly, projections from the BLA to the PL as well as the BLA to dHPC have been implicated in the neural circuit underlying contextual SD-induced cocaine seeking (Fuchs et al., 2007). The paraventricular thalamus (PVT) is important in contextual SD-induced drug seeking (James et al., 2011), and its projection to the NAshell is important in contextual SD-induced alcohol seeking (using decarbonated beer) (Hamlin et al., 2009). Additionally, glutamatergic projections from the PL to NAcore have been found to be involved in CS-induced cocaine reinstatement (Gipson et al., 2013a; Stefanik et al., 2016), whereas ventromedial PFC (vmPFC, encompassing the IL; Peters et al., 2013) to NAshell has been implicated in contextual SD-induced heroin seeking (Bossert et al., 2012). Recently, glutamatergic projections from the BLA to the NAcore has been found to be important in CS-induced cocaine seeking (Stefanik and Kalivas, 2013).
Glial glutamate transport critically mediates CS-induced reinstatement of drug seeking
Research investigating dysregulations in the molecular mechanisms driving glutamate signaling after chronic exposure to drugs of abuse has revealed that these aberrant neuroadaptations are dependent upon behavioral and environmental conditions. The glial glutamate transporter (GLT-1/EAAT-2), which is responsible for regulating >90% of extracellular levels of glutamate, is one key neural substrate that has been implicated in drug relapse. In addition, the sodium-independent cystine-glutamate exchanger, system xc- (also referred to by its catalytic subunit xCT), works synergistically with GLT-1 to provide glutamatergic tone on presynaptic mGluR2/3 autoreceptors to limit presynaptic release of glutamate (Moran et al., 2005; Kalivas, 2009). Particularly, GLT-1 function and expression has been shown to modulate the expression of CS-induced reinstatement. For example, one study demonstrated that the β-lactam antibiotic ceftriaxone attenuated CS-induced cocaine reinstatement and increased GLT-1 in the NAcore, but not the NAshell (Fischer et al., 2013). Ceftriaxone has been found to up-regulate xCT in addition to GLT-1 (Knackstedt et al., 2009, 2010; Alhaddad et al., 2014), restore basal levels of extracellular glutamate following cocaine self-administration (Trantham-Davidson et al., 2012), and is considered a potential therapeutic target for attenuating relapse in humans (Reissner and Kalivas, 2010). Similar studies have also shown that up-regulation of GLT-1 in the NA is associated with decreased CS-induced reinstatement (Sari et al., 2009; Knackstedt et al., 2010; Sondheimer and Knackstedt, 2011).
Akin to ceftriaxone, the cysteine prodrug N-acetylcysteine (NAC) is associated with an up-regulation of both xCT and GLT-1 (Knackstedt et al., 2010) and has previously been shown to inhibit cocaine-primed reinstatement (Baker et al., 2003; Moran et al., 2005) as well as both CS- and heroin-induced drug seeking (Zhou and Kalivas, 2008), CS-induced cocaine seeking (Reissner et al., 2015), and CS-induced nicotine seeking (Ramirez-Niño et al., 2013). It was recently discovered that chronic NAC treatment inhibits CS-induced cocaine reinstatement through a GLT-1-dependent mechanism, where inhibition of xCT expression did not block NAC's attenuating effect on reinstatement (Reissner et al., 2015). While NAC is believed to drive system xc- and depotentiate glutamatergic afferents in the NA, the pharmacotherapeutic potential and clinical relevance of specifically driving system xc- remains somewhat unclear. Although one study found that NAC-mediated attenuation of cocaine primed-reinstatement was reversed when system xc- was pharmacologically inhibited (Kau et al., 2008), it is still unclear whether driving system xc- is a prerequisite for NAC-mediated attenuation of CS-induced relapse and if this effect is drug-specific. Likely, there are other mechanisms underlying NAC's therapeutic potential that have yet to be fully elucidated.
Glial glutamate transport in SD-induced reinstatement of drug seeking
As mentioned above, up-regulation of GLT-1 in the NAcore but not the NAshell is associated with a decrease in CS-induced reinstatement of cocaine seeking (Fischer et al., 2013). One recent study that examined GLT-1 expression and glutamate efflux after a period of forced abstinence found that although ceftriaxone up-regulated GLT-1 and attenuated drug seeking, glutamate efflux was not reduced during cocaine seeking induced by drug context (LaCrosse et al., 2016). Thus, despite showing similar ameliorations to dysregulated glutamatergic signaling and subsequent behavior as described in (Trantham-Davidson et al., 2012) (which utilized a cocaine-induced reinstatement model), failure of ceftriaxone to inhibit glutamate efflux in this study potentially highlights a differential role of glial glutamate transport between different models of reinstated/renewed drug seeking. The role of glial-glutamate transport in SD-induced reinstatement of drug seeking is poorly understood and more research is needed to fully characterize the functional differences in glutamate efflux during reinstatement between drug-paired CSs and SDs.
Dendritic morphology and physiology at glutamatergic synapses alters in response to drug-associated CSs
Acute and chronic exposure to drugs of abuse is associated with changes in synaptic structure and function that increase sensitivity to drug-associated cues. Early studies using Golgi-cox staining to examine dendritic morphology in the PFC and NA revealed that repeated exposure to psychostimulants (Robinson and Kolb, 1997; Brown and Kolb, 2001) as well as morphine (Robinson and Kolb, 1999) is associated with alterations in dendritic complexity and increases in spine density. More recently, it has been demonstrated that nicotine self-administration and extinction training is associated with enduring increases in basal spine head diameter, increases in AMPA to NMDA excitatory post-synaptic currents (EPSCs), an increase in AMPA (GluA1), and NMDA (GluN2A and GluN2B) receptor subunit expression, and a decrease in GLT-1 expression within the NA (Gipson et al., 2013b). In this study, CS-induced reinstatement was also associated with rapid, transient plasticity, such as increases in extracellular glutamate, dendritic spine diameter, and AMPA to NMDA ratios within 15 min of cue exposure. Similar changes in transient synaptic plasticity have also been observed following CS-induced cocaine seeking, which requires glutamatergic input from the PL (Gipson et al., 2013a). Interestingly, another study examining the role of GluA1 AMPA receptor subunits in cocaine seeking found that targeted deletion of GRIA1 (i.e., the gene that encodes GluA1 AMPAR subunits) in mice was not associated with changes in cocaine self-administration or discrete CS-induced reinstatement (Mead et al., 2006).
Both acute and chronic cocaine exposure has been linked to increases in GluA2-lacking, calcium permeable AMPA receptors (CP-AMPARs), which is thought to underlie “incubated” CS-induced reinstatement of drug seeking (Conrad et al., 2008; Wolf, 2010). Additionally, early withdrawal from cocaine is associated with the presence of “silent synapses” between the BLA and NAcore, which are often characterized by the expression of stable NMDA receptors (NMDARs) and labile AMPARs that are inserted into the membrane after prolonged withdrawal (Lee et al., 2013). Incubation of drug craving is generally characterized by time-dependent increases in drug seeking and corticostriatal activity following an extended period of withdrawal (Tran-Nguyen et al., 1998; Pickens et al., 2011). However, incubation of drug craving is often measured as an increase in drug seeking in response to discrete conditioned cues in the same context in which the drug was initially administered. Importantly, it is unknown how SDs impact incubation of drug seeking behavior. Thus, understanding the differential role of SD-induced alterations in synaptic structure and function is necessary to fully elucidate the neurobiological underpinnings of complex drug-associated stimulus interactions.
In addition to studies utilizing CS-models, a necessary consideration must be made regarding drug-induced reinstatement models, as some of the neuroadaptations described herein between CS- and SD-models have also been observed similarly or differentially in drug-induced reinstatement paradigms. Acute cocaine exposure is associated with an increase in dendritic spine density and synaptic plasticity in the VTA (Sarti et al., 2007). In addition to changes in spine density, it has been demonstrated that withdrawal from chronic, non-contingent cocaine exposure induces changes in spine head diameter in the NAcore, as well as rapid alterations in dendritic morphology in response to a cocaine challenge similar to those observed in CS-induced reinstatement models (Shen et al., 2009). Another study examining AMPA to NMDA ratio and spine head diameter in the NAcore following cocaine-primed reinstatement found that inhibition of the PL potentiated AMPA to NMDA currents and spine head diameter, while inhibition of the VTA or administration of D1/D2 antagonists inhibited such changes (Shen et al., 2014a). Intriguingly, inhibition of the PL was still associated with a decrease in cocaine-primed reinstatement of drug seeking. This poses an interesting contrast to what has been observed previously in a CS-induced reinstatement model of cocaine seeking (Gipson et al., 2013a).
SD-induced alterations in dendritic structure and function at glutamatergic synapses
While the role of CP-AMPARs in SD-induced reinstatement has not been fully characterized, recent evidence suggests that a reduction in GluA1 expression in the NA may be associated with a decrease in contextual SD-induced cocaine seeking (LaCrosse et al., 2016). Given these findings, it appears that changes in dendritic spine structure, AMPA to NMDA ratios, and AMPAR expression in the NA may not be unconditionally linked to all forms of reinstated drug seeking. Rather, it appears that reinstatement may depend on stimulus function-specific neural mechanisms. While these changes in dendritic spine morphology and physiology have been examined extensively in discrete conditioned cue- and drug-induced reinstatement, very few studies have attempted to elucidate whether discriminative cues elicit similar cellular responses. However, one recent study demonstrated that re-exposure to a cocaine-associated environment renewed cocaine seeking following extinction training in a separate environment and produced similar alterations in dendritic spine head diameter as in the previously mentioned studies that utilized non-contingent cocaine injections or conditioned cues (Stankeviciute et al., 2014). Taken together, certain alterations in synaptic potentiation and dendritic spine morphology may serve as a common neurobiological mechanism underlying drug seeking across drug types and environmental conditions (Scofield et al., 2016). However, more work will need to be conducted to more clearly define the underlying cellular and molecular mechanisms that drive alterations in dendritic morphology and physiology across different behavioral conditions and in response to different cue types.
Future Neurobiological Targets Underlying Motivated Behavior
Brain- and glial cell line-derived neurotrophic factors
In addition to DAergic and glutamatergic signaling, drug-induced alterations in synaptic plasticity and signal transduction along the mesocorticolimbic pathway are mediated by neurotrophic factors, neuropeptides, extracellular matrix substrates, and other signaling molecules. Brain-derived neurotrophic factor (BDNF) is one neurotrophic factor that has been extensively studied and is known to produce enduring neuroadaptations in response to drugs of abuse. For instance, BDNF increases progressively over time in response to cocaine (but not sucrose) withdrawal in the NA, VTA, and AMY, which is also thought to underlie incubation of drug craving as described previously (Grimm et al., 2003). The mPFC is the primary source of striatal BDNF and has been demonstrated to mediate cocaine seeking such that acute administration of BDNF into the mPFC produces time-dependent attenuation of CS-induced cocaine seeking following self-administration with no effect on food-seeking behavior (Berglind et al., 2007, 2009). As well, BDNF expression and its effects on drug seeking appears to be dependent on withdrawal, where elevated levels in the NAcore and NAshell following extended cocaine self-administration are detectable on withdrawal day (WD) 45 and WD90, respectively (Li et al., 2013). Similar to BDNF, glial cell line-derived neurotrophic factor (GDNF) expression in the VTA is associated with time-dependent increases in CS-induced cocaine seeking following a period of withdrawal (Ghitza et al., 2010). As well, heroin self-administration and withdrawal is associated with time-dependent increases in GDNF mRNA (but not protein levels) in the VTA and NA, and exogenous administration of GDNF into the NA is associated with increased CS-induced heroin seeking (Airavaara et al., 2011). Administration of GDNF into the VTA is also associated with an increase in extinction responding following cocaine self-administration (Lu et al., 2009). Considering the effects of BDNF and GDNF are drug-specific, brain region-specific, and time-dependent, it is unlikely that a systemic pharmacotherapeutic targeting some aspect of BDNF or GDNF signal transduction would be clinically efficacious (Ghitza et al., 2010). Regardless, understanding the heterogeneous profile of expression of neuromodulators such as BDNF and GDNF is necessary to more clearly elucidate differential neural mechanisms governing drug seeking.
Neuropeptides
Neuropeptides originating from the lateral hypothalamus (LH) such as orexin (i.e., hypocretin) and melanin-concentrating hormone (MCH) have long since been implicated in regulating feeding behavior (DiLeone et al., 2003) and may also drive drug seeking. For instance, MCH signaling from the LH to the NA may underlie the rewarding aspects of feeding (Saper et al., 2002) and MCH signaling has also been shown to sensitize animals to the rewarding effects of psychostimulants (Cabeza de Vaca et al., 2002). A recent study found that the LH has glutamatergic and GABAergic projections that innervate both dopaminergic and GABAergic neurons in the VTA (Nieh et al., 2015). The LH also sends glutamatergic projections to the lateral habenula (LHb), which projects to VTA/rostromedial tegmental nucleus (RMTg, or tail of the VTA, tVTA; Jhou et al., 2009; Kaufling et al., 2009) GABAergic neurons that are capable of inhibiting VTA dopaminergic neurons (Poller et al., 2013). Electrical stimulation of the LHb is associated with decreases in both cocaine self-administration and extinction responding (Friedman et al., 2010) and also mediates the aversive effects of nicotine (Fowler and Kenny, 2014). Conversely, exposure to discrete heroin CSs and reinstated heroin seeking is associated with an increase in c-Fos expression in the medial portion of the LHb (Zhang et al., 2005). Currently, there is little evidence that clearly distinguishes the role of the LHb in CS- vs. SD-induced reinstatement. Similar to MCH, orexin neurons in the LH project to multiple corticolimbic structures (Peyron et al., 1998) and have been implicated in mediating reward-seeking behavior (Aston-Jones et al., 2010). Antagonism of OxR1 (but not OxR2) inhibits CS-induced cocaine reinstatement (Smith et al., 2009) and also attenuates SD-induced cocaine seeking following both forced abstinence and extinction (Smith et al., 2010). Given such findings, it appears that orexin signaling may mediate both CS- and SD-driven reinstatement of drug seeking. In addition to MCH and orexin signaling, endogenous opioid peptides such as dynorphin have also been implicated in reward seeking (described in further detail below). It appears that neuropeptides may have a significant neuromodulatory role in controlling reward seeking and motivated behavior. Although, future studies will need to further elucidate whether the mechanisms driving these systems are conserved across drug classes and behavioral conditions.
Matrix metalloproteinases
Recently, neurobiological activity within the extracellular matrix (ECM), hypothesized to be the fourth component of the tetrapartite synapse (Smith et al., 2015), has become increasingly implicated in mediating structural plasticity that occurs in response to chronic exposure to drugs of abuse. Matrix metalloproteinases (MMPs), which are a family of zinc-containing endopeptidases, remodel the ECM and have been shown to mediate synaptogenesis, synaptic plasticity, and LTP (Ethell and Ethell, 2007). For example, studies have shown MMPs (particularly MMP-9) to be involved in dendritic remodeling of the dentate gyrus in response to kainate (KA)-induced excitotoxicity (Szklarczyk et al., 2002; Jourquin et al., 2003). In particular, systemic administration of KA is associated with increased expression of MMP-9 in the dentate gyrus of the HPC (Szklarczyk et al., 2002). Conversely, acute exposure to ethanol impairs spatial memory and is associated with decreased MMP-9 (but not MMP-2) activity in the HPC and PFC (Wright et al., 2003). Alterations in the expression and activity of MMPs within the mPFC as well as the NA have been implicated in drug seeking, where extinction of heroin self-administration is associated with a downregulation of MMPs in these regions. This neuroadaptation was partially restored following re-exposure to heroin-associated cues and acute pre-treatment with a broad-spectrum MMP inhibitor attenuated CS-induced reinstatement (Van den Oever et al., 2010). MMP activity has been shown to underlie changes in constitutive potentiation of glutamatergic synapses in the NAcore as well as changes in transient synaptic potentiation in response to conditioned stimuli paired with cocaine, nicotine, and heroin (Smith et al., 2014). Disruptions in MMP function and expression following both acute and chronic exposure to drugs appears to be conserved across drug classes and behavioral conditions. In fact, it has been suggested that these changes in MMPs might reflect a functional adaptation that resembles early developmental conditions in the brain (Smith et al., 2015), where the high expression of MMP-2 and MMP-9 during early development is significantly reduced over time (Ayoub et al., 2005). Given these findings, MMPs may be a potential therapeutic target for the treatment of substance use disorders. Nevertheless, the functional role of MMPs between CS- and SD-induced drug seeking is still unclear and more research is needed to elucidate whether MMP activity and its effects on drug seeking and synaptic plasticity is drug- and/or brain-region-specific between these two types of drug-associated stimuli.
Nitric oxide and endocannabinoids
Retrograde messengers such as nitric oxide (NO) and endocannabinoids (eCBs) are involved in critical signaling systems that mediate rapid changes in synaptic structure and function (see Regehr et al., 2009, for review). For example, inhibition of nitric oxide synthase (NOS) is associated with impairments in learning and memory, such as in spatial memory tasks (Estall et al., 1993; Yamada et al., 1995) as well as in olfactory memory tasks (Böhme et al., 1993). Recently, it has been suggested that long-term memories (such as drug-cue associations) can become labile upon retrieval and undergo re-consolidation processes that are susceptible to disruption (Hu and Schacher, 2015). In one study examining NO and the motivational properties of cocaine, inhibition of neuronal nitric oxide synthase (nNOS) activity was associated with decreased cocaine self-administration, extinction, and cocaine-primed reinstatement (although acquisition was unaffected) (Orsini et al., 2002). Smith, Scofield, Heinsbroek, Gipson and colleagues have recently shown that a small population of nNOS-expressing interneurons in the NAcore mediates glutamatergic plasticity of MSNs in response to cocaine-paired CSs and that this process is an mGluR5-dependent mechanism (Smith et al., 2017). In this study, pharmacological activation of mGluR5 as well as activation of designer Gq-coupled receptors on nNOS interneurons was associated with activation of nNOS in the absence of drug-associated CSs. Additionally, the degree of inactivation of nNOS interneurons was positively correlated with CS-induced reinstatement, and chemogenetic stimulation of nNOS interneurons was associated with an increase in MMP activity and AMPA currents in MSNs, both of which are known to drive CS-induced reinstatement (Smith et al., 2017). This study is the first to demonstrate that a small population of nNOS-expressing interneurons in the NA is capable of mediating transient plasticity induced by drug-associated cues. Considering nNOS activity is associated with increased drug seeking in CS-, SD-, and drug-prime models of reinstatement, NO signaling may constitute a neurobiological mechanism underlying relapse vulnerability.
Endocannabinoids comprise a family of endogenous retrograde messengers that are involved in a variety of physiological processes, such as pain-sensation, mood, hunger, learning, and memory (Aizpurua-Olaizola et al., 2017). The endocannabinoid system (ECS) also underlies the psychoactive effects of cannabis. The ECS mediates long-term depression (LTD) of synapses in regions such as the NA and HPC (Robbe et al., 2002; Chevaleyre and Castillo, 2003; Gerdeman and Lovinger, 2003) and dysregulations in this signaling system may underlie compulsive drug seeking. The cannabinoid receptor type 1 (CB1), which binds exogenous compounds such as Δ9-tetrahydrocannabinol (THC, the psychoactive constituent of cannabis) as well as endogenous cannabinoids such as anandamide and 2-arachidonoylglycerol (2-AG), has been shown to mediate both CS- and cocaine-induced reinstatement of drug seeking (De Vries et al., 2001). Similarly, CB1 receptors also mediate nicotine self-administration, where systemic antagonism of CB1 receptors following prolonged nicotine withdrawal decreased CS-induced nicotine seeking (Cohen et al., 2005). While these studies seem to suggest that blockade of CB1 receptors may suppress CS-induced drug seeking, one recent study found that inhibition of fatty-acid-amide-hydrolase (FAAH), the enzyme that degrades anandamide, decreased CS-induced nicotine reinstatement (which was reversed by administering a CB1 antagonist) (Forget et al., 2016). Therefore, induction and suppression of different aspects of the ECS may produce varying effects on drug-seeking behavior. It must be noted as well that alterations in the ECS have been shown to have long-lasting, transgenerational effects on cannabinoid, DA, and glutamate receptor genes along the mesolimbic pathway, where parental exposure to THC is associated with increased heroin seeking and decreased levels of GluN1 and GluN2B in the subsequent generation (Szutorisz et al., 2014). Whether this prenatal exposure can induce a heightened sensitivity to drug-associated cues later in life has yet to be fully elucidated. The ECS remains a putative target for treating substance abuse; however, our current understanding of the molecular mechanisms driving its effects on motivated behavior is still inadequate.
Neuronal ensembles and cue-induced reinstatement of drug seeking
Neuronal ensembles represent a subpopulation of functionally interconnected neurons that are collectively involved in specific computations. This concept was first developed by Donald Hebb in The Organization of Behavior (Hebb, 1949), where he postulated that neuronal cells could functionally assemble into “closed systems” and participate in various computations. Early work investigating neuronal ensembles in the NA found that cues associated with drug delivery produce activation of corticolimbic nuclei that converge onto and activate neuronal ensembles in the NA (Pennartz et al., 1994). More precisely, targeted inactivation of cocaine-activated neurons in the NA blocked locomotor sensitization specific to the cocaine context (Koya et al., 2009). Recent studies have examined this phenomenon in reinstatement paradigms, particularly in context-induced reinstatement of drug seeking. For example, Cruz et al. (2014) found that inactivation of NAshell but not NAcore neurons that were activated by a cocaine-associated context attenuated cocaine seeking behavior. Similarly, ensembles in the vmPFC are activated by a heroin-associated context and inhibition of these neurons attenuates context-induced (i.e., SD-induced) reinstatement of heroin seeking (Bossert et al., 2011). Neuronal ensembles in the OFC encoding heroin cues have also been shown to mediate cue-induced renewal of heroin seeking after a period of abstinence (Fanous et al., 2012). Interestingly, neurons encoding drug-cue associations are largely distinct from those encoding associations with natural rewards such as food (Carelli et al., 2000) and convergent lines of evidence seem to suggest that only a small proportion of cells (about 2–5%) encode cocaine memories (Mattson et al., 2008; Koya et al., 2009; Cameron and Carelli, 2012; Cruz et al., 2013). Recently, it has been suggested that rapid, transient synaptic potentiation of MSNs, which drives CS-induced drug-seeking behavior (Gipson et al., 2013a), may functionally expand the original ensemble due to glutamate spillover (Bobadilla et al., 2017). The underlying mechanism postulated here involves dysregulation of glutamate homeostasis following chronic drug exposure (Kalivas, 2009), which increases extrasynaptic levels of glutamate during reinstatement (Gipson et al., 2013a) and consequently activates NO and MMPs in an mGluR5-dependent manner. As described above, NO and MMP signaling mediate cue-triggered drug seeking and associated synaptic plasticity. As Bobadilla et al. (2017) describe, this transient “potentiation wave” may recruit additional neurons that reside adjacent to the neuronal ensemble as a consequence of this transient glutamate spillover, thus producing a robust behavioral response. In fact, 18% of the total recorded neurons in (Gipson et al., 2013a) expressed AMPA-to-NMDA (A/N) ratios that were at least two standard deviations above the mean A/N for cue-induced cocaine reinstatement. This contrasts with cue-induced sucrose seeking, where only 6% of the total recorded neurons expressed A/N ratios that were at least two standard deviations above the mean (Bobadilla et al., 2017). These findings potentially illustrate transient recruitment of additional neurons to the primary engram due to increased extracellular glutamate. We recommend that future studies examine this process further across drugs of abuse and between both CS- and SD-induced reinstatement paradigms to elucidate the cellular and molecular mechanisms that underlie the formation, persistence, and modulation of neuronal ensembles.
Other neurobiological targets involved in motivated behavior
Cue-type specificity is an important caveat underlying the neurobehavioral adaptations induced by drugs of abuse. Thus, future research investigating alternative neurobiological targets aimed at promoting drug abstinence and reducing relapse must take this into consideration. While not the primary focus of this review, the serotonin (5-HT) and norepinephrine (NE) neurotransmitter systems have also been extensively studied and are critically involved in both CS- and SD-induced drug seeking. For instance, the 5-HT2A antagonist M100907 has been demonstrated to attenuate CS-induced cocaine reinstatement following extinction training (Nic Dhonnchadha et al., 2009). Intra-vmPFC injections of M100907 have also been demonstrated to inhibit CS-induced cocaine reinstatement (Pockros et al., 2011). Conversely, stimulation of 5-HT2C with the agonist Ro60-0175 inhibits both CS- and SD-induced drug seeking (Burbassi and Cervo, 2008; Fletcher et al., 2008). In addition to 5-HT, NE receptors (particularly the α2 receptor) have also been demonstrated in rodents to mediate both drug- and CS-induced reinstatement, although these findings are mixed in nonhuman primates (for review, see Zaniewska et al., 2015).
One recent study examining the role of voltage-gated calcium channels (VGCC) in the NA found that selective antagonism of the α2δ-1 VGCC subunit with gabapentin attenuated cocaine-primed (but not CS-induced) reinstatement following cocaine self-administration, where cocaine infusions were paired with discrete light and tone cues (Spencer et al., 2014). As mentioned previously, certain neuropeptide systems may be potential neurobiological targets aimed at reducing drug seeking. For example, selective activation of the kappa opioid receptor (KOR) by dynorphin along the mesolimbic pathway inhibits VTA and NA DA release (Margolis et al., 2003). Recent evidence suggests that the dynorphin/KOR system maintains drug seeking since both ethanol (Walker and Koob, 2008) and nicotine self-administration (Galeote et al., 2009) depend on dynorphin/KOR signaling. Corticotropin-releasing factor (CRF) is another neuropeptide that has been implicated in drug seeking, particularly in stress-induced reinstatement of drug seeking, where a footshock can serve as discrete conditioned stimulus that reinstates drug seeking (Koob and Le Moal, 2005). While manipulating these neuropeptide systems may be efficacious in attenuating drug seeking, it is not entirely clear what specific role these substrates play in CS- vs. SD-induced drug seeking.
Another potential neurobiological target is the molecular clock. Many studies have implicated circadian rhythms as important mediators of drug seeking (Falcón and McClung, 2009). As such, manipulating transcriptional regulators of this system may be effective in suppressing drug seeking. Indeed, diurnal and circadian patterns of behavior are heavily reliant on environmental stimuli (e.g., zeitgebers), so careful consideration should be taken regarding how particular cue types impact this molecular system. Recently, sex differences in both clinical and pre-clinical settings have been observed in the training, maintenance, withdrawal, and relapse of drug use (Becker and Koob, 2016). Notably, gonadal hormones appear to play a significant role in motivated behavior and may be a potential neurobiological target in the treatment of substance abuse. For instance, one study found that cocaine-seeking following an extended period of withdrawal was enhanced during estrus in female rats relative to non-estrus females or males (Kerstetter et al., 2008). However, how these hormones specifically impact sensitivity to conditioned or discriminative drug-associated cues has yet to be determined.
Discussion
Much preclinical research has used contingent CSs as conditioned reinforcers to produce drug seeking behavior (See, 2002), and the construct validity of this reinstatement model must be taken as a caveat to interpreting the translational reach of the neurobehavioral mechanisms underlying this motivated behavior (Epstein et al., 2006). The contingent CS-induced reinstatement model, however, has led to pharmacotherapeutic developments that have shown some translational relevance in reducing drug relapse and craving in human clinical studies across different drug classes (Mardikian et al., 2007; Knackstedt et al., 2009; Gray et al., 2010; Berk et al., 2013; McClure et al., 2014, 2015). Preclinically, similar neuroadaptations in glutamatergic signaling within the mesocorticolimbic pathway have been reported with different drugs of abuse including nicotine, cocaine, and heroin (LaLumiere and Kalivas, 2008; Shen et al., 2011; Gipson et al., 2013a,b; Scofield et al., 2016). However, an appreciable amount of neurobiological heterogeneity exists even within contingent CS-induced drug seeking, where corticothalamic and corticostriatal neuronal projections from the PFC have opposing functional roles in controlling reward-seeking behavior (Otis et al., 2017).
Compared to the contingent CS-induced reinstatement model, the neurobehavioral mechanisms that underlie drug seeking induced by non-contingent SDs or OSs is sorely understudied. Additionally, what we do know about these stimuli has been derived from models using context as the SD. These non-contingent modulatory stimuli occur often in the lives of substance abusers and can lead to increased drug craving and relapse in humans (Childress et al., 1993). Clinical study regarding the effects of drug cues is almost exclusively done with models that utilize non-contingent presentation of drug-associated stimuli, including stimulus-induced craving and attentional bias (Sinha and Li, 2007; Field and Cox, 2008). Thus, using similar methods in preclinical models, like OS- and SD-induced drug seeking, may help to identify more clinically relevant neurobehavioral mechanisms underlying substance use disorders. Likewise, increased use of contingent CS models in human clinical studies may help to validate existing preclinical models. Together these approaches may aid in forging a better bridge between clinical and preclinical research on cue effects in substance abuse, helping to identify future therapeutics.
As mentioned above, a potential caveat of previous models of cue effects in drug seeking is the lack of specificity. Most prior research using either contingent CSs or non-contingent SDs to study cue effects on drug seeking has been done in isolation of other reinforcers. If an attempt to demonstrate specificity of either treatment effects or stimulus effects is made, it is typically done through a separate group of drug-naïve animals trained to respond solely for a natural reinforcer, like food. However, chronic exposure to drugs of abuse results in dramatic changes within the brain, affecting reward, learning, memory, and decision-making processes, and how a reward-associated stimulus interacts with a drug-naïve system is very different than in a drug-exposed system (Hyman et al., 2006). Thus, it is important for future research to dissociate drug-associated neurobehavioral processes from those associated with natural reinforcers within a system that has been chronically exposed to drugs of abuse to better aid in the future discovery of more specific behavioral and pharmacological therapies for substance use disorders. Toward this goal, there have been a few attempts to utilize preclinical models that allow for direct comparison of stimulus control of drug seeking by drug-associated cues vs. control of food seeking by food-associated cues within animals chronically exposed to drugs of abuse (Weiss et al., 2003; Kearns and Weiss, 2007; Weiss et al., 2007; Lombas et al., 2008; McCuddy et al., 2014). Although these models demonstrate clearly specific control of drug and food seeking by their associated stimuli, the neurobehavioral mechanisms that underlie this differential control are unknown. Only one study to date has evaluated multimodal reinstatement, illustrating specific stimulus control over food and drug taking within an animal (Batten and Beckmann, 2017). Future research into the neurobehavioral processes that govern differential stimulus control of seeking drug vs. natural reinforcers will help in identifying more specific neurobehavioral targets for future therapies to treat reward-related pathologies.
It is important to note that studies examining the neurobiological mechanisms underlying contextual SDs in comparison to CSs don't consider that contextual stimuli are spatially-variable, multimodal stimuli and may incorporate multiple sensory systems and themselves engage distinct neural circuits (e.g., hippocampal nuclei) that may not be specifically related to their function as SDs within the context-induced drug seeking procedure. Thus, future studies should employ a single stimulus (e.g., a light) to serve as both a contingent conditioned reinforcer as well as a non-contingent SD in order to dissociate the neurobehavioral processes underlying the functional differences between these two types of stimuli.
Conclusions
We propose that the models discussed herein are useful in determining the differential neurobiological substrates underlying various types of motivated behavior and that care should be taken to better dissociate the roles of different types of cues in models of relapse. As we have attempted to highlight in this review, there is a tendency in the substance use disorder literature (both clinical and preclinical) to posit the stimulus control of drug-associated behavior as the product of a single, unitary Pavlovian process. However, stimulus-induced drug seeking and associated craving, as highlighted herein, can manifest through various neurobehavioral mechanisms that recruit differential neural circuitry, including conditioned reinforcement and modulatory effects on both operant and Pavlovian relations via SDs and OSs, respectively. Thus, stimulus-induced drug seeking and relapse is not a unitary process and it is imperative to pay closer attention to the functional role of drug-associated stimuli during the development of behavioral and pharmaceutical interventions. Contingent CS models of reinstatement have yielded important and informative results that have led to translationally valuable advances in the development of pharmacotherapeutics to reduce relapse vulnerability. Regardless, our knowledge of their modulatory counterparts (e.g., SD and OS) is comparatively infinitesimal. Thus, there is much room for improvement as relapse rates remain high and pharmacotherapeutic advancements that have emerged from these preclinical models have somewhat checkered success in the clinic (Knackstedt et al., 2009; LaRowe et al., 2013; McClure et al., 2014). Future research should attempt to directly dissociate the neurobehavioral mechanisms underlying the stimulus control of drug seeking associated with CS, SD, and OS models, as behavioral and pharmacotherapeutic development for intervention of drug relapse may be improved by systematically examining these functionally distinct paths to drug seeking. As highlighted throughout this review, SD reinstatement models of drug relapse almost exclusively utilize contextual cues as the SD. Thus, future research should employ discrete (as opposed to contextual) discriminative cues to further elucidate the neurobehavioral mechanisms underlying these distinct stimuli. Moreover, polydrug abuse is a typical feature of substance use disorders, where abuse of multiple drugs can hinder successful treatment outcomes (Wang L. et al., 2017). Specifically, primary drug use, which is defined as the main substance reported at the time of admission into a treatment program (Mattson et al., 2017), is modulated by use of secondary substances. No studies to date have thoroughly examined how primary drug cues influence secondary drug seeking and vice versa. Such research would reveal novel insights into how complex drug-cue associations modulate drug seeking behavior in individuals who engage in polydrug use. Finally, future research should also incorporate stimulus control of alternative sources of reinforcement, such as in a multiple schedule, to better isolate drug-associated stimulus control in models of chronic drug exposure. There are numerous and varied roads to relapse. Therefore, both behavioral and pharmacotherapeutic interventions must be tailored to the individual to promote better drug cessation outcomes.
Author Contributions
MN, JB, and CG designed and wrote the paper. ST and MFO edited and contributed to sections of the paper.
Funding
This work was supported by Public Health Service grants R00 DA036569 and DA036569–S1 (CG), AA AA025590 and DA043172 (MFO), and R00 DA033373 (JB) from the National Institutes of Health.
Conflict of Interest Statement
The authors declare that the research was conducted in the absence of any commercial or financial relationships that could be construed as a potential conflict of interest.
References
Adams, C. L., Cowen, M. S., Short, J. L., and Lawrence, A. J. (2008). Combined antagonism of glutamate mGlu5 and adenosine A2A receptors interact to regulate alcohol-seeking in rats. Int. J. Neuropsychopharm. 11, 229–241. doi: 10.1017/S1461145707007845
Ahmed, S. H., and Koob, G. F. (1999). Long-lasting increase in the set point for cocaine self-administration after escalation in rats. Psychopharmacology 146, 303–312. doi: 10.1007/s002130051121
Airavaara, M., Pickens, C. L., Stern, A. L., Wihbey, K. A., Harvey, B. K., Bossert, J. M., et al. (2011). Endogenous GDNF in ventral tegmental area and nucleus accumbens does not play a role in the incubation of heroin craving. Addict. Biol. 16, 261–272. doi: 10.1111/j.1369-1600.2010.00281.x
Aizpurua-Olaizola, O., Elezgarai, I., Rico-Barrio, I., Zarandona, I., Etxebarria, N., and Usobiaga, A. (2017). Targeting the endocannabinoid system: future therapeutic strategies. Drug Discov. Today 22, 105–110. doi: 10.1016/j.drudis.2016.08.005
Alhaddad, H., Das, S. C., and Sari, Y. (2014). Effects of ceftriaxone on ethanol intake: a possible role for xCT and GLT-1 isoforms modulation of glutamate levels in P rats. Psychopharmacology (Berl). 231, 4049–4057. doi: 10.1007/s00213-014-3545-y
Alleweireldt, A. T., Weber, S. M., Kirschner, K. F., Bullock, B. L., and Neisewander, J. L. (2002). Blockade or stimulation of D1 dopamine receptors attenuates cue reinstatement of extinguished cocaine-seeking behavior in rats. Psychopharmacology (Berl). 159, 284–293. doi: 10.1007/s002130100904
Arguello, A. A., Richardson, B. D., Hall, J. L., Wang, R., Hodges, M. A., Mitchell, M. P., et al. (2017). Role of a lateral orbital frontal cortex-basolateral amygdala circuit in cue-induced cocaine-seeking behavior. Neuropsychopharmacology 42, 727–735. doi: 10.1038/npp.2016.157
Aston-Jones, G., Smith, R. J., Sartor, G. C., Moorman, D. E., Massi, L., Tahsili-Fahadan, P., et al. (2010). Lateral hypothalamic orexin/hypocretin neurons: a role in reward-seeking and addiction. Brain Res. 1314C, 74. doi: 10.1016/j.brainres.2009.09.106
Ayoub, A. E., Cai, T.-Q., Kaplan, R. A., and Luo, J. (2005). Developmental expression of matrix metalloproteinases 2 and 9 and their potential role in the histogenesis of the cerebellar cortex. J. Comp. Neurol. 481, 403–415. doi: 10.1002/cne.20375
Bäckström, P., and Hyytiä, P. (2004). Ionotropic glutamate receptor antagonists modulate cue-induced reinstatement of ethanol-seeking behavior. Alcohol Clin. Exp. Res. 28, 558–565. doi: 10.1097/01.ALC.0000122101.13164.21
Bäckström, P., and Hyytiä, P. (2006). Ionotropic and metabotropic glutamate receptor antagonism attenuates cue-induced cocaine seeking. Neuropsychopharmacology 31, 778–786. doi: 10.1038/sj.npp.1300845
Bäckström, P., and Hyytiä, P. (2007). Involvement of AMPA/kainate, NMDA, and mGlu5 receptors in the nucleus accumbens core in cue-induced reinstatement of cocaine seeking in rats. Psychopharmacology (Berl) 192, 571–580. doi: 10.1007/s00213-007-0753-8
Baker, D. A., McFarland, K., Lake, R. W., Shen, H., Tang, X. C., Toda, S., et al. (2003). Neuroadaptations in cystine-glutamate exchange underlie cocaine relapse. Nat. Neurosci. 6, 743–749. doi: 10.1038/nn1069
Baptista, M. A., Martin-Fardon, R., and Weiss, F. (2004). Preferential effects of the metabotropic glutamate 2/3 receptor agonist LY379268 on conditioned reinstatement versus primary reinforcement: comparison between cocaine and a potent conventional reinforcer. J. Neurosci. 24, 4723–4727. doi: 10.1523/JNEUROSCI.0176-04.2004
Batten, S. R., and Beckmann, J. S. (2017). Differential stimulus control of drug-seeking: multimodal reinstatement. Addict. Biol. doi: 10.1111/adb.12544. [Epub ahead of print].
Becker, J. B., and Koob, G. F. (2016). Sex differences in animal models: focus on addiction. Pharmacol. Rev. 68, 242–263. doi: 10.1124/pr.115.011163
Beckmann, J. S., and Chow, J. J. (2015). Isolating the incentive salience of reward-associated stimuli: value, choice, and persistence. Learn. Mem. 22, 116–127. doi: 10.1101/lm.037382.114
Beckmann, J. S., Gipson, C. D., Marusich, J. A., and Bardo, M. T. (2012). Escalation of cocaine intake with extended access in rats: dysregulated addiction or regulated acquisition? Psychopharmacology (Berl) 222, 257–267. doi: 10.1007/s00213-012-2641-0
Berglind, W. J., Case, J. M., Parker, M. P., Fuchs, R. A., and See, R. E. (2006). Dopamine D1 or D2 receptor antagonism within the basolateral amygdala differentially alters the acquisition of cocaine-cue associations necessary for cue-induced reinstatement of cocaine-seeking. Neuroscience 137, 699–706. doi: 10.1016/j.neuroscience.2005.08.064
Berglind, W. J., See, R. E., Fuchs, R. A., Ghee, S. M., Whitfield, T. W., Miller, S. W., et al. (2007). A BDNF infusion into the medial prefrontal cortex suppresses cocaine seeking in rats. Eur. J. Neurosci. 26, 757–766. doi: 10.1111/j.1460-9568.2007.05692.x
Berglind, W. J., Whitfield, T. W., LaLumiere, R. T., Kalivas, P. W., and McGinty, J. F. (2009). A single intra-PFC infusion of BDNF prevents cocaine-induced alterations in extracellular glutamate within the nucleus accumbens. J. Neurosci. 29, 3715–3719. doi: 10.1523/JNEUROSCI.5457-08.2009
Berk, M., Malhi, G. S., Gray, L. J., and Dean, O. M. (2013). The promise of N-acetylcysteine in neuropsychiatry. Trends Pharmacol. Sci. 34, 167–177. doi: 10.1016/j.tips.2013.01.001
Bespalov, A. Y., Dravolina, O. A., Sukhanov, I., Zakharova, E., Blokhina, E., Zvartau, E., et al. (2005). Metabotropic glutamate receptor (mGluR5) antagonist MPEP attenuated cue- and schedule-induced reinstatement of nicotine self-administration behavior in rats. Neuropharmacology 49, 167–178. doi: 10.1016/j.neuropharm.2005.06.007
Bobadilla, A. C., Heinsbroek, J. A., Gipson, C. D., Griffin, W. C., Fowler, C. D., Kenny, P. J., et al. (2017). Corticostriatal plasticity, neuronal ensembles, and regulation of drug-seeking behavior. Prog. Brain Res. 235, 93–112. doi: 10.1016/bs.pbr.2017.07.013
Bock, R., Shin, J. H., Kaplan, A. R., Dobi, A., Markey, E., Kramer, P. F., et al. (2013). Strengthening the accumbal indirect pathway promotes resilience to compulsive cocaine use. Nat. Neurosci. 16, 632–638. doi: 10.1038/nn.3369
Böhme, G. A., Bon, C., Lemaire, M., Reibaud, M., Piot, O., Stutzmann, J. M., et al. (1993). Altered synaptic plasticity and memory formation in nitric oxide synthase inhibitor-treated rats. Proc. Natl. Acad. Sci. U.S.A. 90, 9191–9194. doi: 10.1073/pnas.90.19.9191
Bolam, J. P. (1984). Synapses of identified neurons in the neostriatum. Ciba Found. Symp. 107, 30–47. doi: 10.1002/9780470720882.ch3
Bossert, J. M., Adhikary, S., St Laurent, R., Marchant, N. J., Wang, H. L., Morales, M., et al. (2016). Role of projections from ventral subiculum to nucleus accumbens shell in context-induced reinstatement of heroin seeking in rats. Psychopharmacology (Berl) 233, 1991–2004. doi: 10.1007/s00213-015-4060-5
Bossert, J. M., Busch, R. F., and Gray, S. M. (2005). The novel mGluR2/3 agonist LY379268 attenuates cue-induced reinstatement of heroin seeking. Neuroreport 16, 1013–1016. doi: 10.1097/00001756-200506210-00026
Bossert, J. M., Gray, S. M., Lu, L., and Shaham, Y. (2006). Activation of group II metabotropic glutamate receptors in the nucleus accumbens shell attenuates context-induced relapse to heroin seeking. Neuropsychopharm. 31, 2197–2209. doi: 10.1038/sj.npp.1300977
Bossert, J. M., Liu, S. Y., Lu, L., and Shaham, Y. (2004). A role of ventral tegmental area glutamate in contextual cue-induced relapse to heroin seeking. J. Neurosci. 24, 10726–10730. doi: 10.1523/JNEUROSCI.3207-04.2004
Bossert, J. M., Marchant, N. J., Calu, D. J., and Shaham, Y. (2013). The reinstatement model of drug relapse: recent neurobiological findings, emerging research topics, and translational research. Psychopharmacology 229, 453–476. doi: 10.1007/s00213-013-3120-y
Bossert, J. M., Poles, G. C., Wihbey, K. A., Koya, E., and Shaham, Y. (2007). Differential effects of blockade of dopamine D1-family receptors in nucleus accumbens core or shell on reinstatement of heroin seeking induced by contextual and discrete cues. J. Neurosci. 27, 12655–12663. doi: 10.1523/JNEUROSCI.3926-07.2007
Bossert, J. M., and Stern, A. L. (2014). Role of ventral subiculum in context-induced reinstatement of heroin seeking in rats. Addict. Biol. 19, 338–342. doi: 10.1111/adb.12015
Bossert, J. M., Stern, A. L., Theberge, F. R., Cifani, C., Koya, E., Hope, B. T., et al. (2011). Ventral medial prefrontal cortex neuronal ensembles mediate context-induced relapse to heroin. Nat. Neurosci. 14, 420–422. doi: 10.1038/nn.2758
Bossert, J. M., Stern, A. L., Theberge, F. R., Marchant, N. J., Wang, H. L., Morales, M., et al. (2012). Role of projections from ventral medial prefrontal cortex to nucleus accumbens shell in context-induced reinstatement of heroin seeking. J. Neurosci. 32, 4982–4991. doi: 10.1523/JNEUROSCI.0005-12.2012
Bossert, J. M., Wihbey, K. A., Pickens, C. L., Nair, S. G., and Shaham, Y. (2009). Role of dopamine D(1)-family receptors in dorsolateral striatum in context-induced reinstatement of heroin seeking in rats. Psychopharmacology (Berl). 206, 51–60. doi: 10.1007/s00213-009-1580-x
Bouton, M. E. (2014). Why behavior change is difficult to sustain. Prev. Med. 68, 29–36. doi: 10.1016/j.ypmed.2014.06.010
Bouton, M. E., Rosengard, C., Achenbach, G. G., Peck, C. A., and Brooks, D. C. (1993). Effects of contextual conditioning and unconditional stimulus presentation on performance in appetitive conditioning. Q. J. Exp. Psychol. B Comp. Physiol. Psychol. 46, 63–95.
Bouton, M. E., and Todd, T. P. (2014). A fundamental role for context in instrumental learning and extinction. Behav. Process. 104, 13–19. doi: 10.1016/j.beproc.2014.02.012
Brown, R. W., and Kolb, B. (2001). Nicotine sensitization increases dendritic length and spine density in the nucleus accumbens and cingulate cortex. Brain Res. 899, 94–100. doi: 10.1016/S0006-8993(01)02201-6
Buczek, Y., Le, A. D., Wang, A., Stewart, J., and Shaham, Y. (1999). Stress reinstates nicotine seeking but not sucrose solution seeking in rats. Psychopharmacology (Berl) 144, 183–188. doi: 10.1007/s002130050992
Burbassi, S., and Cervo, L. (2008). Stimulation of serotonin2C receptors influences cocaine-seeking behavior in response to drug-associated stimuli in rats. Psychopharmacology (Berl) 196, 15–27. doi: 10.1007/s00213-007-0916-7
Cabeza de Vaca, S., Kim, G.-Y., and Carr, K. D. (2002). The melanocortin receptor agonist MTII augments the rewarding effect of amphetamine in ad-libitum-fed and food-restricted rats. Psychopharmacology (Berl). 161, 77–85. doi: 10.1007/s00213-002-0998-1
Caggiula, A. R., Donny, E. C., White, A. R., Chaudhri, N., Booth, S., Gharib, M. A., et al. (2001). Cue dependency of nicotine self-administration and smoking. Pharmacol. Biochem. Behav. 70, 515–530. doi: 10.1016/S0091-3057(01)00676-1
Cameron, C. M., and Carelli, R. M. (2012). Cocaine abstinence alters nucleus accumbens firing dynamics during goal-directed behaviors for cocaine and sucrose. Eur. J. Neurosci. 35, 940–951. doi: 10.1111/j.1460-9568.2012.08024.x
Cannella, N., Halbout, B., Uhrig, S., Evrard, L., Corsi, M., Corti, C., et al. (2013). The mGluR2/3 gonist LY379268 induced anti-reinstatement effects in rats exhibiting addiction-like behavior. Neuropsychopharm. 38, 2048–2056. doi: 10.1038/npp.2013.106
Carelli, R. M., Ijames, S. G., and Crumling, A. J. (2000). Evidence that separate neural circuits in the nucleus accumbens encode cocaine versus “natural” (water and food) reward. J. Neurosci. 20, 4255–4266.
Cervo, L., Carnovali, F., Stark, J. A., and Mennini, T. (2003). Cocaine-seeking behavior in response to drug-associated stimuli in rats: involvement of D3 and D2 dopamine receptors. Neuropsychopharmaclogy 28, 1150–1159. doi: 10.1038/sj.npp.1300169
Chang, H. T., and Kitai, S. T. (1985). Projection neurons of the nucleus accumbens: an intracellular labeling study. Brain Res. 347, 112–116. doi: 10.1016/0006-8993(85)90894-7
Chaudhri, N., Sahuque, L. L., and Janak, P. H. (2009). Ethanol seeking triggered by environmental context is attenuated by blocking dopamine D1 receptors in the nucleus accumbens core and shell in rats. Psychopharmacology (Berl). 207, 303–314. doi: 10.1007/s00213-009-1657-6
Chaudhri, N., Sahuque, L. L., Schairer, W. W., and Janak, P. H. (2010). Separable roles of the nucleus accumbens core and shell in context- and cue-induced alcohol-seeking. Neuropsychopharm. 35, 783–791. doi: 10.1038/npp.2009.187
Chaudhri, N., Woods, C. A., Sahuque, L. L., Gill, T. M., and Janak, P. H. (2013). Unilateral inactivation of the basolateral amygdala attenuates context-induced renewal of Pavlovian-conditioned alcohol-seeking. Eur. J. Neurosci. 38, 2751–2761. doi: 10.1111/ejn.12278
Chevaleyre, V., and Castillo, P. E. (2003). Heterosynaptic LTD of hippocampal GABAergic synapses: a novel role of endocannabinoids in regulating excitability. Neuron 38, 461–472. doi: 10.1016/S0896-6273(03)00235-6
Childress, A. R., Hole, A. V., Ehrman, R. N., Robbins, S. J., McLellan, A. T., and O'Brien, C. P. (1993). Cue reactivity and cue reactivity interventions in drug dependence. NIDA Res. Monogr. 137, 73–95.
Cohen, C., Kodas, E., and Griebel, G. (2005). CB1 receptor antagonists for the treatment of nicotine addiction. Pharmacol. Biochem. Behav. 81, 387–395. doi: 10.1016/j.pbb.2005.01.024
Conrad, K. L., Tseng, K. Y., Uejima, J. L., Reimers, J. M., Heng, L. J., Shaham, Y., et al. (2008). Formation of accumbens GluR2-lacking AMPA receptors mediates incubation of cocaine craving. Nature 454, 118–121. doi: 10.1038/nature06995
Cosme, C. V., Gutman, A. L., and LaLumiere, R. T. (2015). The dorsal agranular insular cortex regulates the cued reinstatement of cocaine-seeking, but not food-seeking, behavior in rats. Neuropsychopharmacology 40, 2425–2433. doi: 10.1038/npp.2015.92
Crombag, H. S., Bossert, J. M., Koya, E., and Shaham, Y. (2008). Review. Context-induced relapse to drug seeking: a review. Philos. Trans. Royal Soc. Lond. B Biol. Sci. 363, 3233–3243. doi: 10.1098/rstb.2008.0090
Crombag, H. S., Grimm, J. W., and Shaham, Y. (2002). Effect of dopamine receptor antagonists on renewal of cocaine seeking by reexposure to drug-associated contextual cues. Neuropsychopharmacology 27, 1006–1015. doi: 10.1016/S0893-133X(02)00356-1
Crombag, H. S., and Shaham, Y. (2002). Renewal of drug seeking by contextual cues after prolonged extinction in rats. Behav. Neurosci. 116, 169–173. doi: 10.1037/0735-7044.116.1.169
Cruz, F. C., Babin, K. R., Leao, R. M., Goldart, E. M., Bossert, J. M., Shaham, Y., et al. (2014). Role of nucleus accumbens shell neuronal ensembles in context-induced reinstatement of cocaine-seeking. J. Neurosci. 34, 7437–7446. doi: 10.1523/JNEUROSCI.0238-14.2014
Cruz, F. C., Koya, E., Guez-Barber, D. H., Bossert, J. M., Lupica, C. R., Shaham, Y., et al. (2013). New technologies for examining the role of neuronal ensembles in drug addiction and fear. Nat. Rev. Neurosci. 14, 743–754. doi: 10.1038/nrn3597
Davidson, C., and Stamford, J. A. (1993). Neurochemical evidence of functional A10 dopamine terminals innervating the ventromedial axis of the neostriatum: in vitro voltammetric data in rat brain slices. Brain Res. 615, 229–239. doi: 10.1016/0006-8993(93)90032-I
De Vries, T. J., Homberg, J. R., Binnekade, R., Raasø, H., and Schoffelmeer, A. N. M. (2003). Cannabinoid modulation of the reinforcing and motivational properties of heroin and heroin-associated cues in rats. Psychopharmacology (Berl) 168, 164–169. doi: 10.1007/s00213-003-1422-1
De Vries, T. J., and Schoffelmeer, A. N. (2005). Cannabinoid CB1 receptors control conditioned drug seeking. Trends. Pharmacol. Sci. 26, 420–426. doi: 10.1016/j.tips.2005.06.002
De Vries, T. J., Shaham, Y., Homberg, J. R., Crombag, H., Schuurman, K., Dieben, J., et al. (2001). A cannabinoid mechanism in relapse to cocaine seeking. Nat. Med. 7, 1151–1154. doi: 10.1038/nm1001-1151
Di Ciano, P., and Everitt, B. J. (2003). Differential control over drug-seeking behavior by drug-associated conditioned reinforcers and discriminative stimuli predictive of drug availability. Behav. Neurosci. 117, 952–960. doi: 10.1037/0735-7044.117.5.952
Di Clemente, A., Franchi, C., Orrù, A., Arnt, J., and Cervo, L. (2012). Bifeprunox: a partial agonist at dopamine D2 and serotonin 1A receptors, influences nicotine-seeking behaviour in response to drug-associated stimuli in rats. Addict. Biol. 17, 274–286. doi: 10.1111/j.1369-1600.2011.00319.x
Diergaarde, L., de Vries, W., Raasø, H., Schoffelmeer, A. N., and De Vries, T. J. (2008). Contextual renewal of nicotine seeking in rats and its suppression by the cannabinoid-1 receptor antagonist Rimonabant (SR141716A). Neuropharmacology 55, 712–716. doi: 10.1016/j.neuropharm.2008.06.003
DiLeone, R. J., Georgescu, D., and Nestler, E. J. (2003). Lateral hypothalamic neuropeptides in reward and drug addiction. Life Sci. 73, 759–768. doi: 10.1016/S0024-3205(03)00408-9
Economidou, D., Mattioli, L., Cifani, C., Perfumi, M., Massi, M., Cuomo, V., et al. (2006). Effect of the cannabinoid CB1 receptor antagonist SR-141716A on ethanol self-administration and ethanol-seeking behaviour in rats. Psychopharmacology (Berl) 183, 394–403. doi: 10.1007/s00213-005-0199-9
Ehrman, R. N., Robbins, S. J., Childress, A. R., and O'Brien, C. P. (1992). Conditioned responses to cocaine-related stimuli in cocaine abuse patients. Psychopharmacology (Berl) 107, 523–529. doi: 10.1007/BF02245266
Epstein, D. H., Preston, K. L., Stewart, J., and Shaham, Y. (2006). Toward a model of drug relapse: an assessment of the validity of the reinstatement procedure. Psychopharmacology (Berl) 189, 1–16. doi: 10.1007/s00213-006-0529-6
Erb, S., Shaham, Y., and Stewart, J. (1996). Stress reinstates cocaine-seeking behavior after prolonged extinction and a drug-free period. Psychopharmacology (Berl) 128, 408–412. doi: 10.1007/s002130050150
Estall, L. B., Grant, S. J., and Cicala, G. A. (1993). Inhibition of nitric oxide (NO) production selectively impairs learning and memory in the rat. Pharmacol.Biochem. Behav. 46, 959–962. doi: 10.1016/0091-3057(93)90228-L
Ethell, I. M., and Ethell, D. W. (2007). Matrix metalloproteinases in brain development and remodeling: synaptic functions and targets. J. Neurosci. Res. 85, 2813–2823. doi: 10.1002/jnr.21273
Falcón, E., and McClung, C. A. (2009). A role for the circadian genes in drug addiction. Neuropharmacology 56, 91–96. doi: 10.1016/j.neuropharm.2008.06.054
Fanous, S., Goldart, E. M., Theberge, F. R., Bossert, J. M., Shaham, Y., and Hope, B. T. (2012). Role of orbitofrontal cortex neuronal ensembles in the expression of incubation of heroin craving. J. Neurosci. 32, 11600–11609. doi: 10.1523/JNEUROSCI.1914-12.2012
Field, M., and Cox, W. M. (2008). Attentional bias in addictive behaviors: a review of its development, causes, and consequences. Drug Alcohol Depend. 97, 1–20. doi: 10.1016/j.drugalcdep.2008.03.030
Fischer, K. D., Houston, A. C., and Rebec, G. V. (2013). Role of the major glutamate transporter GLT1 in nucleus accumbens core versus shell in cue-induced cocaine-seeking behavior. J. Neurosci. 33, 9319–9327. doi: 10.1523/JNEUROSCI.3278-12.2013
Fletcher, P. J., Rizos, Z., Sinyard, J., Tampakeras, M., and Higgens, G. A. (2008). The 5-HT2C receptor agonist Ro60-0175 reduces cocaine self-administration and reinstatement induced by the stressor yohimbine, and contextual cues. Neuropsychopharmacology 33, 1402–1412. doi: 10.1038/sj.npp.1301509
Forget, B., Guranda, M., Gamaleddin, I., Goldberg, S. R., and Le Foll, B. (2016). Attenuation of cue-induced reinstatement of nicotine seeking by URB597 through cannabinoid CB1 receptor in rats. Psychopharmacology (Berl). 233, 1823–1828. doi: 10.1007/s00213-016-4232-y
Forget, B., Pushparaj, A., and Le Foll, B. (2010). Granular insular cortex inactivation as a novel therapeutic strategy for nicotine addiction. Biol. Psychiatry 68, 265–271. doi: 10.1016/j.biopsych.2010.01.029
Fowler, C. D., and Kenny, P. J. (2014). Nicotine aversion: neurobiological mechanisms and relevance to tobacco dependence vulnerability. Neuropharmacology 76(Pt B) 533–544 doi: 10.1016/j.neuropharm.2013.09.008
Friedman, A., Lax, E., Dikshtein, Y., Abraham, L., Flaumenhaft, Y., Sudai, E., et al. (2010). Electrical stimulation of the lateral habenula produces enduring inhibitory effect on cocaine seeking behavior. Neuropharmacology 59, 452–459. doi: 10.1016/j.neuropharm.2010.06.008
Fuchs, R. A., Eaddy, J. L., Su, Z. I., and Bell, G. H. (2007). Interactions of the basolateral amygdala with the dorsal hippocampus and dorsomedial prefrontal cortex regulate drug context-induced reinstatement of cocaine-seeking in rats. Eur. J. Neurosci. 26, 487–498. doi: 10.1111/j.1460-9568.2007.05674.x
Fuchs, R. A., Evans, K. A., Ledford, C. C., Parker, M. P., Case, J. M., Mehta, R. H., et al. (2005). The role of the dorsomedial prefrontal cortex, basolateral amygdala, and dorsal hippocampus in contextual reinstatement of cocaine seeking in rats. Neuropsychopharmacology 30, 296–309. doi: 10.1038/sj.npp.1300579
Fuchs, R. A., Evans, K. A., Parker, M. C., and See, R. E. (2004). Differential involvement of the core and shell subregions of the nucleus accumbens in conditioned cue-induced reinstatement of cocaine seeking in rats. Psychopharmacology (Berl). 176, 459–465. doi: 10.1007/s00213-004-1895-6
Fuchs, R. A., Ramirez, D. R., and Bell, G. H. (2008). Nucleus accumbens shell and core involvement in drug context-induced reinstatement of cocaine seeking in rats. Psychopharmacology (Berl) 200, 545–556. doi: 10.1007/s00213-008-1234-4
Galeote, L., Berrendero, F., Bura, S. A., Zimmer, A., and Maldonado, R. (2009). Prodynorphin gene disruption increases the sensitivity to nicotine self-administration in mice. Int. J. Neuropsychopharmacol. 12, 615–625. doi: 10.1017/S1461145708009450
Gamaleddin, I., Wertheim, C., Zhu, A. Z., Coen, K. M., Vemuri, K., Makryannis, A., et al. (2012). Cannabinoid receptor stimulation increases motivation for nicotine and nicotine seeking. Addict. Biol. 17. 47–61. doi: 10.1111/j.1369-1600.2011.00314.x
Ge, F., Wang, N., Cui, C., Li, Y., Liu, Y., Ma, Y., et al. (2017). Glutamatergic projections from the entorhinal cortex to dorsal dentate gyrus mediate context-induced reinstatement of heroin seeking. Neuropsychopharm. 42, 1860–1870. doi: 10.1038/npp.2017.14
Gerber, G. J., and Stretch, R. (1975). Drug-induced reinstatement of extinguished self-administration behavior in monkeys. Pharmacol. Biochem. Behav. 3, 1055–1061. doi: 10.1016/0091-3057(75)90016-7
Gerdeman, G. L., and Lovinger, D. M. (2003). Emerging roles for endocannabinoids in long-term synaptic plasticity. Br. J. Pharmacol. 140, 781–789. doi: 10.1038/sj.bjp.0705466
Gerfen, C. R., Engber, T. M., Mahan, L. C., Susel, Z., Chase, T. N., Monsma, F. J., et al. (1990). D1 and D2 dopamine receptor-regulated gene expression of striatonigral and striatopallidal neurons. Science 250, 1429–1432. doi: 10.1126/science.2147780
Gerfen, C. R., Herkenham, M., and Thibault, J. (1987). The neostriatal mosaic: II. Patch- and matrix-directed mesostriatal dopaminergic and non-dopaminergic systems. J. Neurosci. 7, 3915–3934.
Gerfen, C. R., and Surmeier, D. J. (2011). Modulation of striatal projection systems by dopamine. Annu. Rev. Neurosci. 34, 441–466. doi: 10.1146/annurev-neuro-061010-113641
Ghitza, U. E., Zhai, H., Wu, P., Airavaara, M., Shaham, Y., and Lu, L. (2010). Role of BDNF and GDNF in drug reward and relapse: a review. Neurosci. Biobehav. Rev. 35, 157–171. doi: 10.1016/j.neubiorev.2009.11.009
Gipson, C. D., Kupchik, Y. M., Shen, H., Reissner, K. J., Thomas, C. A., and Kalivas, P. W. (2013a). Relapse induced by cues predicting cocaine depends on rapid, transient synaptic potentiation. Neuron 77, 867–872. doi: 10.1016/j.neuron.2013.01.005
Gipson, C. D., Reissner, K. J., Kupchik, Y. M., Smith, A. C., Stankeviciute, N., Hensley-Simon, M. E., et al. (2013b). Reinstatement of nicotine seeking is mediated by glutamatergic plasticity. Proc. Natl. Acad. Sci. U.S.A. 110, 9124–9129. doi: 10.1073/pnas.1220591110
Gray, K. M., Watson, N. L., Carpenter, M. J., and Larowe, S. D. (2010). N-acetylcysteine (NAC) in young marijuana users: an open-label pilot study. Am. J. Addict. 19, 187–189. doi: 10.1111/j.1521-0391.2009.00027.x
Grimm, J. W., Kruzich, P. J., and See, R. E. (2000). Contingent access to stimuli associated with cocaine self-administration is required for reinstatement of drug-seeking behavior. Psychobiology 28, 383–386. doi: 10.3758/BF03331995
Grimm, J. W., Lu, L., Hayashi, T., Hope, B. T., Su, T.-P., and Shaham, Y. (2003). Time-dependent increases in brain-derived neurotrophic factor protein levels within the mesolimbic dopamine system after withdrawal from cocaine: implications for incubation of cocaine craving. J. Neurosci. 23, 742 LP-747.
Groenewegen, H. J., Wright, C. I., Beijer, A. V., and Voorn, P. (1999). Convergence and segregation of ventral striatal inputs and outputs. Ann. N.Y. Acad. Sci. 877, 49–63. doi: 10.1111/j.1749-6632.1999.tb09260.x
Hamlin, A. S., Clemens, K. J., Choi, E. A., and McNally, G. P. (2009). Paraventricular thalamus mediates context-induced reinstatement (renewal) of extinguished reward seeking. Eur. J. Neurosci. 29, 802–812. doi: 10.1111/j.1460-9568.2009.06623.x
Hedreen, J. C. (1981). “Neurons of the nucleus accumbens and other striatal regions in rats,” in Neurobiology of the Nucleus Accumbens, ed R. B. DeFrance (Brunswick, ME: The Haer Institute for Electrophysiologic Research), 82–96.
Heinsbroek, J. A., Neuhofer, D. N., Griffin, W. C., Siegel, G. S., Bobadilla, A.-C., Kupchik, Y. M., et al. (2017). Loss of plasticity in the D2-accumbens pallidal pathway promotes cocaine seeking. J. Neurosci. 37, 757–767. doi: 10.1523/JNEUROSCI.2659-16.2016
Holland, P. C. (1998). Temporal control in Pavlovian occasion setting. Behav. Process. 44, 225–236. doi: 10.1016/S0376-6357(98)00051-5
Hollerman, J. R., and Schultz, W. (1998). Dopamine neurons report an error in the temporal prediction of reward during learning. Nat. Neurosci. 1, 304–309. doi: 10.1038/1124
Hu, J., and Schacher, S. (2015). Persistent associative plasticity at an identified synapse underlying classical conditioning becomes labile with short-term homosynaptic activation. J. Neurosci. 35, 16159–16170. doi: 10.1523/JNEUROSCI.2034-15.2015
Hyman, S. E., Malenka, R. C., and Nestler, E. J. (2006). Neural mechanisms of addiction: the role of reward-related learning and memory. Annu. Rev. Neurosci. 29, 565–598. doi: 10.1146/annurev.neuro.29.051605.113009
Ito, R., Dalley, J. W., Howes, S. R., Robbins, T. W., and Everitt, B. J. (2000). Dissociation in conditioned dopamine release in the nucleus accumbens core and shell in response to cocaine cues and during cocaine-seeking behavior in rats. J. Neurosci. 20, 7489–7495.
Ito, R., Robbins, T. W., and Everitt, B. J. (2004). Differential control over cocaine-seeking behavior by nucleus accumbens core and shell. Nat. Neurosci. 7, 389–397. doi: 10.1038/nn1217
James, M. H., Charnley, J. L., Flynn, J. R., Smith, D. W., and Dayas, C. V. (2011). Propensity to “relapse” following exposure to cocaine cues is associated with the recruitment of specific thalamic and epithalamic nuclei. Neuroscience 199, 235–242. doi: 10.1016/j.neuroscience.2011.09.047
Jhou, T. C., Fields, H. L., Baxter, M. G., Saper, C. B., and Holland, P. C. (2009). The rostromedial tegmental nucleus (RMTg), a major GABAergic afferent to midbrain dopamine neurons, selectively encodes aversive stimuli and promotes behavioral inhibition. Neuron 61, 786–800. doi: 10.1016/j.neuron.2009.02.001
Jimenez-Castellanos, J., and Graybiel, A. M. (1987). Subdivisions of the dopamine-containing A8-A9-A10 complex identified by their differential mesostriatal innervation of striosomes and extrastriosomal matrix. Neuroscience 23, 223–242. doi: 10.1016/0306-4522(87)90285-5
Jourquin, J., Tremblay, E., Décanis, N., Charton, G., Hanessian, S., Chollet, A. M., et al. (2003). Neuronal activity-dependent increase of net matrix metalloproteinase activity is associated with MMP-9 neurotoxicity after kainate. Eur. J. Neurosci. 18, 1507–1517. doi: 10.1046/j.1460-9568.2003.02876.x
Kalivas, P. W. (2009). The glutamate homeostasis hypothesis of addiction. Nat. Rev. Neurosci. 10, 561–572. doi: 10.1038/nrn2515
Kalivas, P. W., and Volkow, N. D. (2005). The neural basis of addiction: a pathology of motivation and choice. Am. J. Psychiatry 162, 1403–1413. doi: 10.1176/appi.ajp.162.8.1403
Kantak, K. M., Black, Y., Valencia, E., Green-Jordan, K., and Eichenbaum, H. B. (2002). Dissociable effects of lidocaine inactivation of the rostral and caudal basolateral amygdala on the maintenance and reinstatement of cocaine-seeking behavior in rats. J. Neurosci. 22, 1126–1136.
Kau, K. S., Madayag, A., Mantsch, J. R., Grier, M. D., Abdulhameed, O., and Baker, D. A. (2008). Blunted cystine-glutamate antiporter function in the nucleus accumbens promotes cocaine-induced drug seeking. Neuroscience 155, 530–537. doi: 10.1016/j.neuroscience.2008.06.010
Kaufling, J., Veinante, P., Pawlowski, S. A., Freund-Mercier, M.-J., and Barrot, M. (2009). Afferents to the GABAergic tail of the ventral tegmental area in the rat. J. Comp. Neurol. 513, 597–621. doi: 10.1002/cne.21983
Kearns, D. N., and Weiss, S. J. (2007). Contextual renewal of cocaine seeking in rats and its attenuation by the conditioned effects of an alternative reinforcer. Drug Alcohol Depend. 90, 193–202. doi: 10.1016/j.drugalcdep.2007.03.006
Keistler, C. R., Hammarlund, E., Barker, J. M., Bond, C. W., DiLeone, R. J., Pittenger, C., et al. (2017). Regulation of alcohol extinction and cue-induced reinstatement by specific projections among medial prefrontal cortex, nucleus accumbens, and basolateral amygdala. J. Neurosci. 37, 4462–4471. doi: 10.1523/JNEUROSCI.3383-16.2017
Kerstetter, K. A., Aguilar, V. R., Parrish, A. B., and Kippin, T. E. (2008). Protracted time-dependent increases in cocaine-seeking behavior during cocaine withdrawal in female relative to male rats. Psychopharmacology (Berl) 198, 63–75. doi: 10.1007/s00213-008-1089-8
Knackstedt, L. A., and Kalivas, P. W. (2009). Glutamate and reinstatement. Curr. Opin. Pharmacol. 9, 59–64. doi: 10.1016/j.coph.2008.12.003
Knackstedt, L. A., LaRowe, S., Mardikian, P., Malcolm, R., Upadhyaya, H., Hedden, S., et al. (2009). The role of cystine-glutamate exchange in nicotine dependence in rats and humans. Biol. Psychiatry 65, 841–845. doi: 10.1016/j.biopsych.2008.10.040
Knackstedt, L. A., Melendez, R. I., and Kalivas, P. W. (2010). Ceftriaxone restores glutamate homeostasis and prevents relapse to cocaine seeking. Biol. Psychiatry 67, 81–84. doi: 10.1016/j.biopsych.2009.07.018
Knackstedt, L. A., Trantham-Davidson, H. L., and Schwendt, M. (2014). The role of ventral and dorsal striatum mGluR5 in relapse to cocaine-seeking and extinction learning. Addict. Biol. 19, 87–101. doi: 10.1111/adb.12061
Kobayashi, S., and Schultz, W. (2014). Reward contexts extend dopamine signals to unrewarded stimuli. Curr. Biol. 24, 56–62. doi: 10.1016/j.cub.2013.10.061
Koob, G. F., and Le Moal, M. (2005). Plasticity of reward neurocircuitry and the “dark side” of drug addiction. Nat. Neurosci. 8, 1442–1444. doi: 10.1038/nn1105-1442
Koya, E., Golden, S. A., Harvey, B. K., Guez-Barber, D. H., Berkow, A., Simmons, D. E., et al. (2009). Targeted disruption of cocaine-activated nucleus accumbens neurons prevents context-specific sensitization. Nat. Neurosci. 12, 1069–1073. doi: 10.1038/nn.2364
Kumaresan, V., Yuan, M., Yee, J., Famous, K. R., Anderson, S. M., Schmidt, H. D., et al. (2009). Metabotropic glutamate receptor 5 (mGluR5) antagonists attenuate cocaine priming- and cue-induced reinstatement of cocaine seeking. Behav. Brain. Res. 202, 238–244. doi: 10.1016/j.bbr.2009.03.039
Kupchik, Y. M., Brown, R. M., Heinsbroek, J. A., Lobo, M. K., Schwartz, D. J., and Kalivas, P. W. (2015). Coding the direct/indirect pathways by D1 and D2 receptors is not valid for accumbens projections. Nat. Neurosci. 18, 1230–1232. doi: 10.1038/nn.4068
Kupchik, Y. M., and Kalivas, P. W. (2017). The direct and indirect pathways of the nucleus accumbens are not what you think. Neuropsychopharmacology 42, 369–370. doi: 10.1038/npp.2016.160
LaCrosse, A. L., Hill, K., and Knackstedt, L. A. (2016). Ceftriaxone attenuates cocaine relapse after abstinence through modulation of nucleus accumbens AMPA subunit expression. Eur. Neuropsychopharmacol. 26, 186–194. doi: 10.1016/j.euroneuro.2015.12.022
LaLumiere, R. T., and Kalivas, P. W. (2008). Glutamate release in the nucleus accumbens core is necessary for heroin seeking. J. Neurosci. 28, 3170–3177. doi: 10.1523/JNEUROSCI.5129-07.2008
LaRowe, S. D., Kalivas, P. W., Nicholas, J. S., Randall, P. K., Mardikian, P. N., and Malcolm, R. J. (2013). A double-blind placebo-controlled trial of N-acetylcysteine in the treatment of cocaine dependence. Am. J. Addict. 22, 443–452. doi: 10.1111/j.1521-0391.2013.12034.x
Lasseter, H. C., Ramirez, D. R., Xie, X., and Fuchs, R. A. (2009). Involvement of the lateral orbitofrontal cortex in drug context-induced reinstatement of cocaine-seeking behavior in rats. Eur. J. Neurosci. 30, 1370–1381. doi: 10.1111/j.1460-9568.2009.06906.x
Lasseter, H. C., Wells, A. M., Xie, X., and Fuchs, R. A. (2011). Interaction of the basolateral amygdala and orbitofrontal cortex is critical for drug context-induced reinstatement of cocaine-seeking behavior in rats. Neuropsychopharmacology 36, 711–720. doi: 10.1038/npp.2010.209
Lasseter, H. C., Xie, X., Arguello, A. A., Wells, A. M., Hodges, M. A., and Fuchs, R. A. (2014). Contribution of a mesocorticolimbic subcircuit to drug context-induced reinstatement of cocaine-seeking behavior in rats. Neuropsychopharmacology 39, 660–669. doi: 10.1038/npp.2013.249
Lasseter, H. C., Xie, X., Ramirez, D. R., and Fuchs, R. A. (2010). Sub-region specific contribution of the ventral hippocampus to drug context-induced reinstatement of cocaine-seeking behavior in rats. Neuroscience 171, 830–839. doi: 10.1016/j.neuroscience.2010.09.032
Lee, B. R., Ma, Y. Y., Huang, Y. H., Wang, X., Otaka, M., Ishikawa, M., et al. (2013). Maturation of silent synapses in amygdala-accumbens projection contributes to incubation of cocaine craving. Nat. Neurosci. 16, 1644–1651. doi: 10.1038/nn.3533
Legault, M., Rompré, P.-P., and Wise, R. A. (2000). Chemical stimulation of the ventral hippocampus elevates nucleus accumbens dopamine by activating dopaminergic neurons of the ventral tegmental area. J. Neurosci. 20, 1635–1642.
Li, X., DeJoseph, M. R., Urban, J. H., Bahi, A., Dreyer, J.-L., Meredith, G. E., et al. (2013). Different roles of BDNF in nucleus accumbens core versus shell during the incubation of cue-induced cocaine craving and its long-term maintenance. J. Neurosci. 33, 1130–1142. doi: 10.1523/JNEUROSCI.3082-12.2013
Liechti, M. E., Lhuillier, L., Kaupmann, K., and Markou, A. (2007). Metabotropic glutamate 2/3 receptors in the ventral tegmental area and the nucleus accumbens shell are involved in behaviors relating to nicotine dependence. J. Neurosci. 27, 9077–9085. doi: 10.1523/JNEUROSCI.1766-07.2007
Lisman, J. E., and Grace, A. A. (2005). The hippocampal-VTA loop: controlling the entry of information into long-term memory. Neuron 46, 703–713. doi: 10.1016/j.neuron.2005.05.002
Liu, X., Jernigen, C., Gharib, M., Booth, S., Caggiula, A. R., and Sved, A. F. (2010). Effects of dopamine antagonists on drug cue-induced reinstatement of nicotine-seeking behavior in rats. Behav. Pharmacol. 21, 153–160. doi: 10.1097/FBP.0b013e328337be95
Liu, X., and Weiss, F. (2002). Reversal of ethanol-seeking behavior by D1 and D2 antagonists in an animal model of relapse: differences in antagonist potency in previously ethanol-dependent versus nondependent rats. J. Pharmacol. Exp. Ther. 300, 882–889. doi: 10.1124/jpet.300.3.882
Liu, X., and Weiss, F. (2004). Nitric oxide synthesis inhibition attenuates conditioned reinstatement of ethanol-seeking, but not the primary reinforcing effects of ethanol. Alcohol. Clin. Exp. Res. 28, 1194–1199. doi: 10.1097/01.ALC.0000134219.93192.00
Ljungberg, T., Apicella, P., and Schultz, W. (1992). Responses of monkey dopamine neurons during learning of behavioral reactions. J. Neurophysiol. 67, 145–163. doi: 10.1152/jn.1992.67.1.145
Lombas, A. S., Kearns, D. N., and Weiss, S. J. (2008). Differential effects of a food-based conditioned inhibitor on food- or cocaine-seeking behavior. Learn. Motiv. 39, 323–333. doi: 10.1016/j.lmot.2008.06.001
Lu, L., Wang, X., Wu, P., Xu, C., Zhao, M., Morales, M., et al. (2009). Role of ventral tegmental area glial cell line–derived neurotrophic factor in incubation of cocaine craving. Biol. Psychiatry 66, 137–145. doi: 10.1016/j.biopsych.2009.02.009
MacAskill, A. F., Cassel, J. M., and Carter, A. G. (2014). Cocaine exposure reorganizes cell type– and input-specific connectivity in the nucleus accumbens. Nat. Neurosci. 17, 1198–1207. doi: 10.1038/nn.3783
Marchant, N. J., Hamlin, A. S., and McNally, G. P. (2009). Lateral hypothalamus is required for context-induced reinstatement of extinguished reward seeking. J. Neurosci. 29, 1331–1342. doi: 10.1523/JNEUROSCI.5194-08.2009
Marchant, N. J., and Kaganovsky, K. (2015). A critical role of nucleus accumbens dopamine D1-family receptors in renewal of alcohol seeking after punishment-imposed abstinence. Behav. Neurosci. 129, 281–291. doi: 10.1037/bne0000050
Mardikian, P. N., LaRowe, S. D., Hedden, S., Kalivas, P. W., and Malcolm, R. J. (2007). An open-label trial of N-acetylcysteine for the treatment of cocaine dependence: a pilot study. Prog. Neuropsychopharmacol. Biol. Psychiatry 31, 389–394. doi: 10.1016/j.pnpbp.2006.10.001
Margolis, E. B., Hjelmstad, G. O., Bonci, A., and Fields, H. L. (2003). κ-Opioid agonists directly inhibit midbrain dopaminergic neurons. J. Neurosci. 23, 9981–9986.
Martin-Fardon, R., Baptista, M. A., Dayas, C. V., and Weiss, F. (2009). Dissociation of the effects of MTEP [3-[(2-methyl-1,3-thiazol-4-yl)ethynyl]piperidine] on conditioned reinstatement and reinforcement: comparison between cocaine and a conventional reinforcer. J. Pharmacol. Exp. Ther. 329, 1084–1090. doi: 10.1124/jpet.109.151357
Mattson, B. J., Koya, E., Simmons, D. E., Mitchell, T. B., Berkow, A., Crombag, H. S., et al. (2008). Context-specific sensitization of cocaine-induced locomotor activity and associated neuronal ensembles in rat nucleus accumbens. Eur. J. Neurosci. 27, 202–212. doi: 10.1111/j.1460-9568.2007.05984.x
Mattson, M., Lipari, R. N., Hays, C., and Van Horn, S. L. (2017). A day in the Life of Older Adults: Substance Use Facts. The CBHSQ Report: May 11, 2017. Rockville, MD: Center for Behavioral Health Statistics and Quality, Substance Abuse and Mental Health Services Administration.
McClure, E. A., Baker, N. L., Gipson, C. D., Carpenter, M. J., Roper, A. P., Froeliger, B. E., et al. (2015). An open-label pilot trial of N-acetylcysteine and varenicline in adult cigarette smokers. Am. J. Drug Alcohol Abuse 41, 52–56. doi: 10.3109/00952990.2014.933839
McClure, E. A., Gipson, C. D., Malcolm, R. J., Kalivas, P. W., and Gray, K. M. (2014). Potential role of N-acetylcysteine in the management of substance use disorders. CNS Drugs 28, 95–106. doi: 10.1007/s40263-014-0142-x
McCuddy, W. T., Beckmann, J. S., and Bardo, M. T. (2014). Multiple schedules as a model to test cue effects of drug and natural reinforcers. Drug. Alcohol. Depend. 140, e140. doi: 10.1016/j.drugalcdep.2014.02.397
McFarland, K., and Kalivas, P. W. (2001). The circuitry mediating cocaine-induced reinstatement of drug-seeking behavior. J. Neurosci. 21, 8655–8663.
McFarland, K., Lapish, C. C., and Kalivas, P. W. (2003). Prefrontal glutamate release into the core of the nucleus accumbens mediates cocaine-induced reinstatement of drug-seeking behavior. J. Neurosci. 23, 3531–3537.
McGlinchey, E. M., and Aston-Jones, G. (2017). Dorsal hippocampus drives context-induced cocaine seeking via inputs to lateral septum. Neuropsychopharmacology. doi: 10.1038/npp.2017.144. [Epub ahead of print].
McGlinchey, E. M., James, M. H., Mahler, S. V., Pantazis, C., and Aston-Jones, G. (2016). Prelimbic to accumbens core pathway is recruited in a dopamine-dependent manner to drive cued reinstatement of cocaine seeking. J. Neurosci. 36, 8700–8711. doi: 10.1523/JNEUROSCI.1291-15.2016
McLaughlin, J., and See, R. E. (2003). Selective inactivation of the dorsomedial prefrontal cortex and the basolateral amygdala attenuates conditioned-cued reinstatement of extinguished cocaine-seeking behavior in rats. Psychopharmacology 168, 57–65. doi: 10.1007/s00213-002-1196-x
Mead, A. N., Zamanillo, D., Becker, N., and Stephens, D. N. (2006). AMPA-receptor GluR1 subunits are involved in the control over behavior by cocaine-paired cues. Neuropsychopharmacology 32, 343–353. doi: 10.1038/sj.npp.1301045
Meredith, G. E. (1999). The synaptic framework for chemical signaling in nucleus accumbens. Ann. N.Y. Acad. Sci. 877, 140–156. doi: 10.1111/j.1749-6632.1999.tb09266.x
Millan, E. Z., and McNally, G. P. (2011). Accumbens shell AMPA receptors mediate expression of extinguished reward seeking through interactions with basolateral amygdala. Learn. Mem. 18, 414–421. doi: 10.1101/lm.2144411
Moran, M. M., Mcfarland, K., Melendez, R. I., Kalivas, P. W., and Seamans, J. K. (2005). Cystine/glutamate exchange regulates metabotropic glutamate receptor presynaptic inhibition of excitatory transmission and vulnerability to cocaine seeking. J. Neurosci. 25, 6389–6393. doi: 10.1523/JNEUROSCI.1007-05.2005
Nic Dhonnchadha, B. A., Fox, R. G., Stutz, S. J., Rice, K. C., and Cunningham, K. A. (2009). Blockade of the serotonin 5-ht2a receptor suppresses cue-evoked reinstatement of cocaine-seeking behavior in a rat self-administration model. Behav. Neurosci. 123, 382–396. doi: 10.1037/a0014592
Nieh, E. H., Matthews, G. A., Allsop, S. A., Presbrey, K. N., Leppla, C. A., Wichmann, R., et al. (2015). Decoding neural circuits that control compulsive sucrose-seeking. Cell 160, 528–541. doi: 10.1016/j.cell.2015.01.003
O'Brien, C. P. (2003). Research advances in the understanding and treatment of addiction. Am. J. Addict. 12(Suppl. 2), S36–S47. doi: 10.1111/j.1521-0391.2003.tb00555.x
Orsini, C., Izzo, E., Koob, G. F., and Pulvirenti, L. (2002). Blockade of nitric oxide synthesis reduces responding for cocaine self-administration during extinction and reinstatement. Brain Res. 925, 133–140. doi: 10.1016/S0006-8993(01)03267-X
Otis, J. M., Namboodiri, V. M. K., Matan, A. M., Voets, E. S., Mohorn, E. P., Kosyk, O., et al. (2017). Prefrontal cortex output circuits guide reward seeking through divergent cue encoding. Nature. 543, 103–107. doi: 10.1038/nature21376
Owesson-White, C., Belle, A. M., Herr, N. R., Peele, J. L., Gowrishankar, P., Carelli, R. M., et al. (2016). Cue-evoked dopamine release rapidly modulates D2 neurons in the nucleus accumbens during motivated behavior. J. Neurosci. 36, 6011–6021. doi: 10.1523/JNEUROSCI.0393-16.2016
Palombo, P., Leao, R. M., Bianchi, P. C., de Oliveira, P. E. C., Planeta, C. D. S., and Cruz, F. C. (2017). Inactivation of the prelimbic cortex impairs the context-induced reinstatement of ethanol seeking. Front. Pharmacol. 8:725. doi: 10.3389/fphar.2017.00725
Parsegian, A., and See, R. E. (2014). Dysregulation of dopamine and glutamate release in the prefrontal cortex and nucleus accumbens following methamphetamine self-administration and during reinstatement in rats. Neuropsychopharmacology 39, 811–822. doi: 10.1038/npp.2013.231
Peleg-Raibstein, D., and Feldon, J. (2006). Effects of dorsal and ventral hippocampal NMDA stimulation on nucleus accumbens core and shell dopamine release. Neuropharmacology 51, 947–957. doi: 10.1016/j.neuropharm.2006.06.002
Pennartz, C. M., Groenewegen, H. J., and Lopes da Silva, F. H. (1994). The nucleus accumbens as a complex of functionally distinct neuronal ensembles: an integration of behavioural, electrophysiological and anatomical data. Prog. Neurobiol. 42, 719–761. doi: 10.1016/0301-0082(94)90025-6
Peters, J., and Kalivas, P. W. (2006). The group II metabotropic glutamate receptor agonist, LY379268, inhibits both cocaine- and food-seeking behavior in rats. Psychopharmacology (Berl) 186, 143–149. doi: 10.1007/s00213-006-0372-9
Peters, J., Pattij, T., and De Vries, T. J. (2013). Targeting cocaine versus heroin memories: divergent roles within ventromedial prefrontal cortex. Trends. Pharmacol. Sci. 34, 689–695, doi: 10.1016/j.tips.2013.10.004
Peyron, C., Tighe, D. K., van den Pol, A. N., de Lecea, L., Heller, H. C., Sutcliffe, J. G., et al. (1998). Neurons containing hypocretin (orexin) project to multiple neuronal systems. J. Neurosci. 18, 9996–10015.
Pezze, M. A., Heidbreder, C. A., Feldon, J., and Murphy, C. A. (2001). Selective responding of nucleus accumbens core and shell dopamine to aversively conditioned contextual and discrete stimuli. Neuroscience 108, 91–102. doi: 10.1016/S0306-4522(01)00403-1
Pickens, C. L., Airavaara, M., Theberge, F., Fanous, S., Hope, B. T., and Shaham, Y. (2011). Neurobiology of the incubation of drug craving. Trends Neurosci. 34, 411–420. doi: 10.1016/j.tins.2011.06.001
Pockros, L. A., Pentkowski, N. S., Swinford, S. E., and Neisewander, J. L. (2011). Blockade of 5-HT2A receptors in the medial prefrontal cortex attenuates reinstatement of cue-elicited cocaine-seeking behavior in rats. Psychopharmacology (Berl) 213, 307–320. doi: 10.1007/s00213-010-2071-9
Poller, W. C., Madai, V. I., Bernard, R., Laube, G., and Veh, R. W. (2013). A glutamatergic projection from the lateral hypothalamus targets VTA-projecting neurons in the lateral habenula of the rat. Brain Res. 1507, 45–60. doi: 10.1016/j.brainres.2013.01.029
Prasad, A. A., and McNally, G. P. (2016). Ventral pallidum output pathways in context-induced reinstatement of alcohol seeking. J. Neurosci. 36. 11716–11726. doi: 10.1523/JNEUROSCI.2580-16.2016
Ramirez, D. R., Bell, G. H., Lasseter, H. C., Xie, X., Traina, S. A., and Fuchs, R. A. (2009). Dorsal hippocampal regulation of memory reconsolidation processes that facilitate drug context-induced cocaine-seeking behavior in rats. Eur. J. Neurosci. 30, 901–912. doi: 10.1111/j.1460-9568.2009.06889.x
Ramirez-Niño, A. M., D'Souza, M. S., and Markou, A. (2013). N-acetylcysteine decreased nicotine self-administration and cue-induced reinstatement of nicotine seeking in rats: comparison with the effects of N-acetylcysteine on food responding and food seeking. Psychopharmacology (Berl). 225, 473–482. doi: 10.1007/s00213-012-2837-3
Regehr, W. G., Carey, M. R., and Best, A. R. (2009). Activity-dependent regulation of synapses by retrograde messengers. Neuron 63, 154–170. doi: 10.1016/j.neuron.2009.06.021
Reissner, K. J., Gipson, C. D., Tran, P. K., Knackstedt, L. A., Scofield, M. D., and Kalivas, P. W. (2015). Glutamate transporter GLT-1 mediates N-acetylcysteine inhibition of cocaine reinstatement. Addict. Biol. 20, 316–323. doi: 10.1111/adb.12127
Reissner, K. J., and Kalivas, P. W. (2010). Using glutamate homeostasis as a target for treating addictive disorders. Behav. Pharmacol. 21, 514–522. doi: 10.1097/FBP.0b013e32833d41b2
Robbe, D., Kopf, M., Remaury, A., Bockaert, J., and Manzoni, O. J. (2002). Endogenous cannabinoids mediate long-term synaptic depression in the nucleus accumbens. Proc. Natl. Acad. Sci. U.S.A. 99, 8384–8388. doi: 10.1073/pnas.122149199
Robinson, T. E., and Kolb, B. (1997). Persistent structural modifications in nucleus accumbens and prefrontal cortex neurons produced by previous experience with amphetamine. J. Neurosci. 17, 8491–8497.
Robinson, T. E., and Kolb, B. (1999). Morphine alters the structure of neurons in the nucleus accumbens and neocortex of rats. Synapse 33, 160–162.
Robinson, T. E., Yager, L. M., Cogan, E. S., and Saunders, B. T. (2014). On the motivational properties of reward cues: individual differences. Neuropharmacology 76(Pt B), 450–459. doi: 10.1016/j.neuropharm.2013.05.040
Rogers, J. L., Ghee, S., and See, R. E. (2008). The neural circuitry underlying reinstatement of heroin-seeking behavior in an animal model of relapse. Neuroscience 151, 579–588. doi: 10.1016/j.neuroscience.2007.10.012
Rogers, J. L., and See, R. E. (2007). Selective inactivation of the ventral hippocampus attenuates cue-induced and cocaine-primed reinstatement of drug-seeking in rats. Neurobiol. Learn. Mem. 87, 688–692. doi: 10.1016/j.nlm.2007.01.003
Saper, C. B., Chou, T. C., and Elmquist, J. K. (2002). The need to feed: homeostatic and hedonic control of eating. Neuron 36, 199–211. doi: 10.1016/S0896-6273(02)00969-8
Sari, Y., Smith, K. D., Ali, P. K., and Rebec, G. V. (2009). Upregulation of Glt1 attenuates cue-induced reinstatement of cocaine-seeking behavior in rats. J. Neurosci. 29, 9239–9243. doi: 10.1523/JNEUROSCI.1746-09.2009
Sarti, F., Borgland, S. L., Kharazia, V. N., and Bonci, A. (2007). Acute cocaine exposure alters spine density and long-term potentiation in the ventral tegmental area. Eur. J. Neurosci. 26, 749–756. doi: 10.1111/j.1460-9568.2007.05689.x
Saunders, B. T., O'Donnell, E. G., Aurbach, E. L., and Robinson, T. E. (2014). A cocaine context renews drug seeking preferentially in a subset of individuals. Neuropsychopharmacology 39, 2816–2823. doi: 10.1038/npp.2014.131
Saunders, B. T., and Robinson, T. E. (2010). A cocaine cue acts as an incentive stimulus in some, but not others: implications for addiction. Biol. Psychiatry 67, 730–736. doi: 10.1016/j.biopsych.2009.11.015
Schenk, S., and Partridge, B. (2001). Influence of a conditioned light stimulus on cocaine self-administration in rats. Psychopharmacology (Berl) 154, 390–396. doi: 10.1007/s002130000608
Schmidt, H. D., Anderson, S. M., and Pierce, R. C. (2006). Stimulation of D1-like or D2 dopamine receptors in the shell, but not the core, of the nucleus accumbens reinstates cocaine-seeking behavior in the rat. Eur. J. Neurosci. 23, 219–228. doi: 10.1111/j.1460-9568.2005.04524.x
Schroeder, J. P., Spanos, M., Stevenson, J. R., Besheer, J., Salling, M., and Hodge, C. W. (2008). Cue-induced reinstatement of alcohol-seeking behavior is associated with increased ERK1/2 phosphorylation in specific limbic brain regions: blockade by the mGluR5 antagonist MPEP. Neuropharmacology 55, 546–554. doi: 10.1016/j.neuropharm.2008.06.057
Schultz, W. (1998). Predictive reward signal of dopamine neurons. J. Neurophysiol. 80, 1–27. doi: 10.1152/jn.1998.80.1.1
Schultz, W., Dayan, P., and Montague, P. R. (1997). A neural substrate of prediction and reward. Science 275, 1593–1599 doi: 10.1126/science.275.5306.1593
Sciascia, J. M., Mendoza, J., and Chaudhri, N. (2014). Blocking dopamine D1-like receptors attenuates context-induced renewal of pavlovian-conditioned alcohol-seeking in rats. Alcohol. Clin. Exp. Res. 38, 418–427. doi: 10.1111/acer.12262
Sciascia, J. M., Reese, R. M., Janak, P. H., and Chaudhri, N. (2015). Alcohol-seeking triggered by discrete pavlovian cues is invigorated by alcohol contexts and mediated by glutamate signaling in the basolateral amygdala. Neuropsychopharmacology 40, 2801–2812. doi: 10.1038/npp.2015.130
Scofield, M. D., Heinsbroek, J. A., Gipson, C. D., Kupchik, Y. M., Spencer, S., Smith, A. C., et al. (2016). The nucleus accumbens: mechanisms of addiction across drug classes reflect the importance of glutamate homeostasis. Pharmacol. Rev. 68, 816–871. doi: 10.1124/pr.116.012484
See, R. E. (2002). Neural substrates of conditioned-cued relapse to drug-seeking behavior. Pharmacol. Biochem. Behav. 71, 517–529. doi: 10.1016/S0091-3057(01)00682-7
See, R. E. (2009). Dopamine D1 receptor antagonism in the prelimbic cortex blocks the reinstatement of heroin-seeking in an animal model of relapse. Int. J. Neuropsychopharmacol. 12, 431–436. doi: 10.1017/S1461145709000054
See, R. E., Kruzich, P. J., and Grimm, J. W. (2001). Dopamine, but not glutamate, receptor blockade in the basolateral amygdala attenuates conditioned reward in a rat model of relapse to cocaine-seeking behavior. Psychopharmacology (Berl). 154, 301–310. doi: 10.1007/s002130000636
Sesack, S. R., Carr, D. B., Omelchenko, N., and Pinto, A. (2003). Anatomical substrates for glutamate-dopamine interactions: evidence for specificity of connections and extrasynaptic actions. Ann. N.Y. Acad. Sci. 1003, 36–52. doi: 10.1196/annals.1300.066
Shaham, Y., Erb, S., and Stewart, J. (2000). Stress-induced relapse to heroin and cocaine seeking in rats: a review. Brain Res. Rev. 33, 13–33. doi: 10.1016/S0165-0173(00)00024-2
Shaham, Y., Shalev, U., Lu, L., de Wit, H., and Stewart, J. (2003). The reinstatement model of drug relapse: history, methodology and major findings. Psychopharmacology (Berl) 168, 3–20. doi: 10.1007/s00213-002-1224-x
Shahan, T. A. (2010). Conditioned reinforcement and response strength. J. Exp. Anal. Behav. 93, 269–289. doi: 10.1901/jeab.2010.93-269
Shen, H., Moussawi, K., Zhou, W., Toda, S., and Kalivas, P. W. (2011). Heroin relapse requires long-term potentiation-like plasticity mediated by NMDA2B-containing receptors. Proc. Natl. Acad. Sci. U.S.A. 108, 19407–19412. doi: 10.1073/pnas.1112052108
Shen, H. W., Gipson, C. D., Huits, M., and Kalivas, P. W. (2014a). Prelimbic cortex and ventral tegmental area modulate synaptic plasticity differentially in nucleus accumbens during cocaine-reinstated drug seeking. Neuropsychopharmacology 39, 1169–1177. doi: 10.1038/npp.2013.318
Shen, H. W., Scofield, M. D., Boger, H., Hensley, M., and Kalivas, P. W. (2014b). Synaptic glutamate spillover due to impaired glutamate uptake mediates heroin relapse. J. Neurosci. 34, 5649–5657. doi: 10.1523/JNEUROSCI.4564-13.2014
Shen, H. W., Toda, S., Moussawi, K., Bouknight, A., Zahm, D. S., and Kalivas, P. W. (2009). Altered dendritic spine plasticity in cocaine withdrawn rats. J. Neurosci. 29, 2876–2884. doi: 10.1523/JNEUROSCI.5638-08.2009
Sinclair, C. M., Cleva, R. M., Hood, L. E., Olive, M. F., and Gass, J. T. (2012). mGluR5 receptors in the basolateral amygdala and nucleus accumbens regulate cue-induced reinstatement of ethanol-seeking behavior. Pharmacol. Biochem. Behav. 101, 329–335. doi: 10.1016/j.pbb.2012.01.014
Sinha, R., and Li, C. S. (2007). Imaging stress- and cue-induced drug and alcohol craving: association with relapse and clinical implications. Drug. Alcohol. Rev. 26, 25–31. doi: 10.1080/09595230601036960
Smith, A. C. W., Kupchik, Y. M., Scofield, M. D., Gipson, C. D., Wiggins, A., Thomas, C. A., et al. (2014). Synaptic plasticity mediating cocaine relapse requires matrix metalloproteinases. Nat. Neurosci. 17, 1655–1657. doi: 10.1038/nn.3846
Smith, A. C. W., Scofield, M. D., Heinsbroek, J. A., Gipson, C. D., Neuhofer, D., Roberts-Wolfe, D. J., et al. (2017). Accumbens nNOS interneurons regulate cocaine relapse. J. Neurosci. 2673–16. doi: 10.1523/JNEUROSCI.2673-16.2016
Smith, A. C., Scofield, M. D., and Kalivas, P. W. (2015). The tetrapartite synapse: extracellular matrix remodeling contributes to corticoaccumbens plasticity underlying drug addiction. Brain Res. 1628, 29–39. doi: 10.1016/j.brainres.2015.03.027
Smith, R. J., Lobo, M. K., Spencer, S., and Kalivas, P. W. (2013). Cocaine-induced adaptations in D1 and D2 accumbens projection neurons (a dichotomy not necessarily synonymous with direct and indirect pathways). Curr. Opin. Neurobiol. 23, 546–552. doi: 10.1016/j.conb.2013.01.026
Smith, R. J., See, R. E., and Aston-Jones, G. (2009). Orexin / hypocretin signaling at the OX1 receptor regulates cue-elicited cocaine-seeking. Eur. J. Neurosci. 30, 493–503. doi: 10.1111/j.1460-9568.2009.06844.x
Smith, R. J., Tahsili-Fahadan, P., and Aston-Jones, G. (2010). Orexin/hypocretin is necessary for context-driven cocaine-seeking. Neuropharmacology 58, 179–184. doi: 10.1016/j.neuropharm.2009.06.042
Sondheimer, I., and Knackstedt, L. A. (2011). Ceftriaxone prevents the induction of cocaine sensitization and produces enduring attenuation of cue- and cocaine-primed reinstatement of cocaine-seeking. Behav. Brain Res. 225, 252–258. doi: 10.1016/j.bbr.2011.07.041
Spencer, S., Brown, R. M., Quintero, G. C., Kupchik, Y. M., Thomas, C. A., Reissner, K. J., et al. (2014). α2δ-1 signaling in nucleus accumbens is necessary for cocaine-induced relapse. J. Neurosci. 34, 8605–8611. doi: 10.1523/JNEUROSCI.1204-13.2014
Spencer, S., Garcia-Keller, C., Roberts-Wolfe, D., Heinsbroek, J. A., Mulvaney, M., Sorrell, A., et al. (2016). Cocaine use reverses striatal plasticity produced during cocaine seeking. Biol. Psychiatry 81, 616–624. doi: 10.1016/j.biopsych.2016.08.033
Stairs, D. J., Neugebauer, N. M., and Bardo, M. T. (2010). Nicotine and cocaine self-administration using a multiple schedule of intravenous drug and sucrose reinforcement in rats. Behav. Pharmacol. 21, 182–193. doi: 10.1097/FBP.0b013e32833a5c9e
Stankeviciute, N. M., Scofield, M. D., Kalivas, P. W., and Gipson, C. D. (2014). Rapid, transient potentiation of dendritic spines in context-induced relapse to cocaine seeking. Addict. Biol. 19, 972–974. doi: 10.1111/adb.12064
Stefanik, M. T., and Kalivas, P. W. (2013). Optogenetic dissection of basolateral amygdala projections during cue-induced reinstatement of cocaine seeking. Front. Behav. Neurosci. 7:213. doi: 10.3389/fnbeh.2013.00213
Stefanik, M. T., Kupchik, Y. M., and Kalivas, P. W. (2016). Optogenetic inhibition of cortical afferents in the nucleus accumbens simultaneously prevents cue-induced transient synaptic potentiation and cocaine-seeking behavior. Brain Struct. Funct. 221, 1681–1689. doi: 10.1007/s00429-015-0997-8
Sunsay, C., and Rebec, G. V. (2014). Extinction and reinstatement of phasic dopamine signals in the nucleus accumbens core during pavlovian conditioning. Behav. Neurosci. 128, 579–587. doi: 10.1037/bne0000012
Szklarczyk, A., Lapinska, J., Rylski, M., McKay, R. D. G., and Kaczmarek, L. (2002). Matrix metalloproteinase-9 undergoes expression and activation during dendritic remodeling in adult hippocampus. J. Neurosci. 22, 920–930.
Szutorisz, H., DiNieri, J. A., Sweet, E., Egervari, G., Michaelides, M., Carter, J. M., et al. (2014). Parental THC exposure leads to compulsive heroin-seeking and altered striatal synaptic plasticity in the subsequent generation. Neuropsychopharmacology 39, 1315–1323. doi: 10.1038/npp.2013.352
Tiffany, S. T., and Carter, B. L. (1998). Is craving the source of compulsive drug use? J. Psychopharmacol. 12, 23–30. doi: 10.1177/026988119801200104
Torregrossa, M. M., Gordon, J., and Taylor, J. R. (2013). Double dissociation between the anterior cingulate cortex and nucleus accumbens core in encoding the context versus the content of pavlovian cocaine cue extinction. J. Neurosci. 33, 8370–8377. doi: 10.1523/JNEUROSCI.0489-13.2013
Tran-Nguyen, L. T., Fuchs, R. A., Coffey, G. P., Baker, D. A., O'Dell, L. E., and Neisewander, J. L. (1998). Time-dependent changes in cocaine-seeking behavior and extracellular dopamine levels in the amygdala during cocaine withdrawal. Neuropsychopharmacology 19, 48–59.
Trantham-Davidson, H., LaLumiere, R. T., Reissner, K. J., Kalivas, P. W., and Knackstedt, L. A. (2012). Ceftriaxone normalizes nucleus accumbens synaptic transmission, glutamate transport, and export following cocaine self-administration and extinction training. J. Neurosci. 32, 12406–12410. doi: 10.1523/JNEUROSCI.1976-12.2012
Van den Oever, M. C., Lubbers, B. R., Goriounova, N. A., Li, K. W., Van der Schors, R. C., Loos, M., et al. (2010). Extracellular matrix plasticity and GABAergic inhibition of prefrontal cortex pyramidal cells facilitates relapse to heroin seeking. Neuropsychopharmacology 35, 2120–2133. doi: 10.1038/npp.2010.90
Waelti, P., Dickinson, A., and Schultz, W. (2001). Dopamine responses comply with basic assumptions of formal learning theory. Nature 412, 43–48. doi: 10.1038/35083500
Walker, B. M., and Koob, G. F. (2008). Pharmacological evidence for a motivational role of κ-opioid systems in ethanol dependence. Neuropsychopharmacology 33, 643–652. doi: 10.1038/sj.npp.1301438
Wang, L., Min, J. E., Krebs, E., Evans, E., Huang, D., Liu, L., et al. (2017). Polydrug use and its association with drug treatment outcomes among primary heroin, methamphetamine, and cocaine users. Int. J. Drug Policy 49, 32–40. doi: 10.1016/j.drugpo.2017.07.009
Wang, N., Ge, F., Cui, C., Li, Y., Sun, X., Sun, L., et al. (2017). Role of glutamatergic projections from the ventral CA1 to infralimbic cortex in context-induced reinstatement of heroin seeking. Neuropsychopharm. doi: 10.1038/npp.2017.279. [Epub ahead of print].
Wang, X., Moussawi, K., Knackstedt, L., Shen, H., and Kalivas, P. W. (2013). Role of mGluR5 neurotransmission in reinstated cocaine-seeking. Addict. Biol. 18, 40–49. doi: 10.1111/j.1369-1600.2011.00432.x
Ward, S. J., Rosenberg, M., Dykstra, L. A., and Walker, E. A. (2009). The CB1 antagonist rimonabant (SR141716) blocks cue-induced reinstatement of cocaine seeking and other context and extinction phenomena predictive of relapse. Drug. Alcohol. Depend. 105, 248–255. doi: 10.1016/j.drugalcdep.2009.07.002
Weiss, F. (2010). “Advances in animal models of relapse for addiction research,” in Advances in the Neuroscience of Addiction, 2nd Edn., eds C. M. Kuhn and G. F. Koob (Boca Raton, FL: CRC Press/Taylor & Francis).
Weiss, F., Maldonado-Vlaar, C. S., Parsons, L. H., Kerr, T. M., Smith, D. L., and Ben-Shahar, O. (2000). Control of cocaine-seeking behavior by drug-associated stimuli in rats: effects on recovery of extinguished operant-responding and extracellular dopamine levels in amygdala and nucleus accumbens. Proc. Natl. Acad. Sci. U.S.A. 97, 4321–4326. doi: 10.1073/pnas.97.8.4321
Weiss, F., Martin-Fardon, R., Ciccocioppo, R., Kerr, T. M., Smith, D. L., and Ben-Shahar, O. (2001). Enduring resistance to extinction of cocaine-seeking behavior induced by drug-related cues. Neuropsychopharmacology 25, 361–372. doi: 10.1016/S0893-133X(01)00238-X
Weiss, S. J., Kearns, D. N., Christensen, C. J., Huntsberry, M. E., Schindler, C. W., and Panlilio, L. V. (2007). Reduction of cocaine seeking by a food-based inhibitor in rats. Exp. Clin. Psychopharmacol. 15, 359–367. doi: 10.1037/1064-1297.15.4.359
Weiss, S. J., Kearns, D. N., Cohn, S. I., Schindler, C. W., and Panlilio, L. V. (2003). Stimulus control of cocaine self-administration. J. Exp. Anal. Behav. 79, 111–135. doi: 10.1901/jeab.2003.79-111
Weissenborn, R., Yackey, M., Koob, G. F., and Weiss, F. (1995). Measures of cocaine-seeking behavior using a multiple schedule of food and drug self-administration in rats. Drug Alcohol. Depend. 38, 237–246. doi: 10.1016/0376-8716(95)01107-A
Wheeler, R. A., Aragona, B. J., Fuhrmann, K. A., Jones, J. L., Day, J. J., Cacciapaglia, F., et al. (2011). Cocaine cues drive opposing context-dependent shifts in reward processing and emotional state. Biol. Psychiatry 69, 1067–1074. doi: 10.1016/j.biopsych.2011.02.014
Willuhn, I., Wanat, M. J., Clark, J. J., and Phillips, P. E. (2010). Dopamine signaling in the nucleus accumbens of animals self-administering drugs of abuse. Curr. Top. Behav. Neurosci. 3, 29–71. doi: 10.1007/7854_2009_27
Wise, R. A. (2004). Dopamine, learning and motivation. Nat. Rev. Neurosci. 5, 483–494. doi: 10.1038/nrn1406
Wolf, M. E. (2010). The Bermuda Triangle of cocaine-induced neuroadaptations. Trends Neurosci. 33, 391–398. doi: 10.1016/j.tins.2010.06.003
Wright, J. W., Masino, A. J., Reichert, J. R., Turner, G. D., Meighan, S. E., Meighan, P. C., et al. (2003). Ethanol-induced impairment of spatial memory and brain matrix metalloproteinases. Brain Res. 963, 252–261. doi: 10.1016/S0006-8993(02)04036-2
Xie, X., Lasseter, H. C., Ramirez, D. R., Ponds, K. L., Wells, A. M., and Fuchs, R. A. (2012). Subregion-specific role of glutamate receptors in the nucleus accumbens on drug context-induced reinstatement of cocaine-seeking behavior in rats. Addict. Biol. 17, 287–299. doi: 10.1111/j.1369-1600.2011.00325.x
Yamada, K., Noda, Y., Nakayama, S., Komori, Y., Sugihara, H., Hasegawa, T., et al. (1995). Role of nitric oxide in learning and memory and in monoamine metabolism in the rat brain. Br. J. Pharmacol. 115, 852–858. doi: 10.1111/j.1476-5381.1995.tb15011.x
Yue, K., Ma, B., Chen, L., Tian, X., Ru, Q., Gan, Y., et al. (2014). L-Stepholidine, a naturally occurring dopamine D1 receptor agonist and D2 receptor antagonist, attenuates heroin self-administration and cue-induced reinstatement in rats. Neuroreport 25, 7–11. doi: 10.1097/WNR.0000000000000012
Zaniewska, M., Filip, M., and Przegalinski, E. (2015). The involvement of norepinephrine in behaviors related to psychostimulant addiction. Curr. Neuropharmacol. 13, 407–418. doi: 10.2174/1570159X13666150121225659
Zhang, F., Zhou, W., Liu, H., Zhu, H., Tang, S., Lai, M., et al. (2005). Increased c-Fos expression in the medial part of the lateral habenula during cue-evoked heroin-seeking in rats. Neurosci. Lett. 386, 133–137. doi: 10.1016/j.neulet.2005.06.008
Zhao, Y., Dayas, C. V., Aujla, H., Baptista, M. A. S., Martin-Fardon, R., and Weiss, F. (2006). Activation of group II metabotropic glutamate receptors attenuates both stress and cue-induced ethanol-seeking and modulates c-fos expression in the hippocampus and amygdala. J. Neurosci. 26, 9967–9974. doi: 10.1523/JNEUROSCI.2384-06.2006
Keywords: discriminative stimulus, conditioned stimulus, relapse, neurobiology, reinstatement, addiction
Citation: Namba MD, Tomek SE, Olive MF, Beckmann JS and Gipson CD (2018) The Winding Road to Relapse: Forging a New Understanding of Cue-Induced Reinstatement Models and Their Associated Neural Mechanisms. Front. Behav. Neurosci. 12:17. doi: 10.3389/fnbeh.2018.00017
Received: 09 October 2017; Accepted: 22 January 2018;
Published: 09 February 2018.
Edited by:
Nuno Sousa, Instituto de Pesquisa em Ciências da Vida e da Saúde (ICVS), PortugalReviewed by:
Fabio Cardoso Cruz, Federal University of São Paulo, BrazilSelena Bartlett, Translational Research Institute, Australia
Copyright © 2018 Namba, Tomek, Olive, Beckmann and Gipson. This is an open-access article distributed under the terms of the Creative Commons Attribution License (CC BY). The use, distribution or reproduction in other forums is permitted, provided the original author(s) and the copyright owner are credited and that the original publication in this journal is cited, in accordance with accepted academic practice. No use, distribution or reproduction is permitted which does not comply with these terms.
*Correspondence: Joshua S. Beckmann, am9zaHVhLmJlY2ttYW5uQHVreS5lZHU=
Cassandra D. Gipson, Y2dpcHNvbnJAYXN1LmVkdQ==