- 1School of Psychology, University of Birmingham, Birmingham, UK
- 2West Midlands Regional Genetics Laboratory, Birmingham Women’s Hospital, NHS Foundation Trust, Birmingham, UK
- 3Centre for Research in Psychology, Behaviour and Achievement, Coventry University, Coventry, UK
- 4Cerebra Centre for Neurodevelopmental Disorders, School of Psychology, University of Birmingham, Birmingham, UK
- 5Department of Complex Genetics, NUTRIM School for Nutrition, Toxicology and Metabolism, Maastricht University, Maastricht, Netherlands
- 6Center for Autism Research, Children’s Hospital of Philadelphia, Philadelphia, PA, USA
Previous studies have documented both neuroplasticity-related BDNF Val66Met and emotion regulation-related 5-HTTLPR polymorphisms as genetic variants that contribute to the processing of emotions from faces. More specifically, research has shown the BDNF Met allele and the 5-HTTLPR Short allele to be associated with mechanisms of negative affectivity that relate to susceptibility for psychopathology. We examined visual scanning pathways in response to angry, happy, and neutral faces in relation to BDNF Val66Met and 5-HTTLPR genotyping in 49 children aged 4–7 years. Analyses revealed that variations in the visual processing of facial expressions of anger interacted with BDNF Val66Met genotype, such that children who carried at least one low neuroplasticity Met allele exhibited a vigilance–avoidance pattern of visual scanning compared to homozygotes for the high neuroplasticity Val allele. In a separate investigation of eye gaze towards the eye versus mouth regions of neutral faces, we observed that short allele 5-HTTLPR carriers exhibited reduced looking at the eye region compared with those with the higher serotonin uptake Long allele. Together, these findings suggest that genetic mechanisms early in life may influence the establishment of patterns of visual scanning of environmental stressors, which in conjunction with other factors such as negative life events, may lead to psychological difficulties and disorders in the later adolescent and adult years.
Introduction
Rapid responses to threatening stimuli have been proposed to have a strong evolutionary component, with threat stimuli requiring urgent facilitation of cognitive and behavioral responding (Öhman and Mineka, 2001; Holmes et al., 2009; Becker et al., 2011). Due to the importance of the human face as an explicit signal for possible aggression and, therefore, in the detection of risk for immediate social threat, the use of facial expressions of anger has come to be used as a reliable index of early fear-related social affectivity in this area of research. For example, an “anger superiority effect” has emerged to reflect a documented pattern of preferential processing of angry faces compared with facial expressions of other emotions (Hansen and Hansen, 1988; Holmes et al., 2009; Öhman et al., 2010). The majority of the studies that have examined visual scanning of angry versus other emotional faces have supported the existence of this superiority effect, reporting quicker speed of detection for angry faces compared with both happy and neutral facial expressions (Gilboa-Schechtman et al., 1999; Fox and Damjanovic, 2006; Horstmann and Bauland, 2006; Lipp et al., 2009; Pinkham et al., 2010; Öhman et al., 2010; Susa et al., 2012).
Recent accounts have suggested a dual-stage processing of emotional stimuli, with anxious individuals exhibiting increased attentional orientation toward negatively valenced stimuli during early stages of processing (e.g., 0–500 ms) compared with control participants, while simultaneously exhibiting an avoidant looking pattern for the same negative stimuli at a later stage of processing (e.g., 1000–1500; Koster et al., 2005). This pattern of results, known in the field as vigilance–avoidance, is believed to reflect automatic attentional orienting to threat-related information followed by strategic visual avoidance in an effort to suppress negative arousal resulting from continued exposure to the negative stimuli (Mogg et al., 2004).
In addition to investigations of visual scanning behavior toward negative emotional expressions in faces, a considerable body of research has focused on investigating eye gaze patterns toward specific facial features that have been suggested to be critical for successful social interaction. Most notably, attending to the eyes has been identified as critical for successful facial identification (Gold et al., 2008), as well as for the effective detection and classification of another individual’s facial emotions and intentions [Baron-Cohen et al., 1997; but see also Dailey and Cottrell (1999) and Blais et al. (2012)]. In addition, typically developing children have been found to focus quickly on the eye region of the human face from very early in life (Nation and Penny, 2008; Shepherd, 2010). Furthermore, healthy individuals have been observed to first fixate on the eyes, and to subsequently spend relatively more time looking at the eye region compared with the mouth region of the face [for a review, see Itier and Batty (2009)]. Affected young populations, such as children with autism, have been found to exhibit an atypical pattern of processing these facial features, spending more time looking at the mouth than the eyes during face scanning in some studies (Klin et al., 2002; Pelphrey et al., 2002; de Wit et al., 2008; Norbury et al., 2009; Kliemann et al., 2010). However, a recent review of eye-tracking studies examining social-specific visual attention in ASD has confirmed the evidence that suggest atypicalities on the visual scanning of the most significant social cues but also reported that this behavioral pattern is not generalized across various contexts, such as in the case of enhanced child-directed speech (Guillon et al., 2014). Emerging research has also highlighted atypical looking to the eye region of faces in individuals with Fragile X syndrome, a genetically defined neurodevelopmental disorder associated with social and communication impairments, as well as social anxiety and reduced amygdala volume [Crawford et al., 2014; see also Dalton et al. (2008), Holsen et al. (2008), Farzin et al. (2009), and Hazlett et al. (2012)]. Together, these findings suggest that reduced eye looking during static face scanning may be closely associated with individual differences in social anxiety and associated neural systems (Crawford et al., 2014).
Although recent evidence provides insight into the time-course of face scanning behavior of anxious adults [e.g., Rohner (2002), Calvo and Avero (2005), and Garner et al. (2006)], the ways in which neurobiological mechanisms may contribute to the early manifestation of these behaviors are currently poorly understood. According to the Differential Susceptibility Hypothesis, individuals are differentially affected by their experiences over the course of development, which is determined by pre-existing elevated biological sensitivity factors such as genetic vulnerabilities that are determined from normal variations in candidate genes (Belsky, 1997; Belsky et al., 2007).
For example, there has been increasing scientific consensus in recent years to support the involvement of BDNF Val66Met variants in modulating behavior, including stress reactivity and depressive symptomatology. Brain-derived neurotrophic factor (BDNF) is a secreted protein present in the human brain that is part of the neurotrophin growth factor family and has been observed to be involved in the regulation of survival and differentiation of neurons, as well as synaptic plasticity (Lu, 2003). Most notably, the BDNF single nucleotide polymorphism (SNP) Val66Met results in a change from Guanine (G) to Adenine (A) at nucleotide position 196 in the protein coding sequence of the gene, as well as subsequent change in amino acid from valine to methionine at position 66 (rs6265). This ultimately leads to decreased availability of BDNF in the brain due to decreased secretion of the variant form of BDNF (Egan et al., 2003). Relevant to emotion regulation, neuroimaging studies have found healthy adults carrying the Met allele to exhibit functional neural over-activation in response to emotional stimuli (Montag et al., 2008; Lau et al., 2010). The results of studies of children also suggest that the BDNF Met allele may act as a susceptibility factor for affective disorders [e.g., Beevers et al. (2009)], especially in combination with early life stressors (Gatt et al., 2009). Despite the consistency in the findings discussed here, however, there remains significant controversy in the literature regarding BDNF Val66Met [for a recent review, see Groves (2007)].
In addition to the putative role of BDNF and associated neuroplasticity in affective responses to emotional faces, associations between common genetic variation in serotonin transporter genes and individual differences in visual scanning of emotional faces have also been observed [e.g., Battaglia et al. (2005) and Lau et al. (2009)]. In particular, a common polymorphism in the 5-HT promoter region, 5-HTTLPR, involved in the transport of serotonin to the presynaptic neuron, has been identified as a reliable indicator of psychological maladjustment. This polymorphism is represented by two variants, a short (S) allele and a long (L) allele, with the short allele associated with significant decreases in serotonin reuptake (Lesch et al., 1996). These alleles produce three genotypes (short/short, short/long, long/long). In combination with exposure to life-threatening situations, individuals carrying at least one copy of the Short allele have been reported to be at increased susceptibility for negative cognitive, behavioral, and neurophysiological outcomes [Caspi et al., 2003; Mercer et al., 2012; Xie et al., 2012; Disner et al., 2013; see also Boll and Gamer (2014) and Christou et al. (2015)]. However, discrepancies still exist in the field regarding the particular role of variations in the serotonin gene in moderating responses to environmental events [for a meta-analysis, see also Karg et al. (2011) and Risch et al. (2009)]. From a developmental perspective, children as young as 9 years of age carrying the 5-HTTLPR Short allele have been found to exhibit greater neural activation in response to fearful and angry faces than children homozygous for the Long allele, in various brain regions previously linked to attentional control in adults (Thomason et al., 2010). In line with this neurophysiological evidence, a range of behavioral studies in both children and adults have measured behavioral reaction times, and reported that the presence of two copies of the high activity Long 5-HTTLPR allele is associated with positive affectivity (shorter reaction times) toward happy facial stimuli compared to neutral facial stimuli [for a review, see Homberg and Lesch (2010)], suggesting the existence of a protective factor against affective psychopathology in homozygotes for the Long allele.
Three hypotheses were tested as part of this study. Taking into account previous evidence suggesting a moderating role of the BDNF Val66Met for reactivity (Montag et al., 2008; Schofield et al., 2009; Lau et al., 2010), it was hypothesized that the low neuroplasticity Met BDNF allele would be significantly associated with vigilance–avoidance patterns in the time spent looking toward the facial expressions of anger, compared to the high neuroplasticity Val/Val genotype. Furthermore, considering evidence suggesting modulation of reactivity in response to facial emotions by 5-HTTLPR genotype (Thomason et al., 2010), we hypothesized that carriers of at least one low serotonin uptake 5-HTTLPR Short allele would similarly display a vigilance–avoidance pattern in response to angry facial expression. A third hypothesis was that carriers of at least one 5-HTTLPR Short allele would exhibit an avoidance pattern in overall time spent looking at the eyes versus the mouth region of neutral face pairs, compared with the high serotonin uptake Long/Long genotype. Finally, we tested the hypothesis that carriers of at least one BDNF Met allele would similarly exhibit an avoidance pattern in overall time spent looking at the eyes versus the mouth of neutral face pairs, compared with individuals homozygous for the Val allele.
Materials and Methods
Participants
Forty-nine children from Caucasian ancestry participated in the study (24 males; 25 females; M age in months = 60.26, SD = 11.80, age range: 4–7 years of age). Participants were recruited through a local community research participation advertisement/outreach program at the local University from a pool of 70 children that have originally genotyped for the needs of previous neuroimaging genetics study on our lab (Christou et al., 2015). Parents or guardians of all participants reported that the child had no history of a neurological or psychiatric disorder, with normal or corrected to normal vision. All participants scored above 75 on the British Ability Scales (BAS-II; Elliot et al., 1996), a standardized assessment of intelligence/developmental age and abilities, equivalent to IQ scores. Informed written consent was obtained from the parents/guardians of all participants prior to participation in the study. In addition, children aged 7 provided written assent to participate in the study. Families were provided with compensation of £10 toward their travel expenses. Ethical consent was gained from the local University Ethical Committee.
Emotion Regulation Measures
For the assessment of children’s emotional regulation abilities, the Child Behavioural Checklist was used (CBCL; Achenbach and Rescorla, 2001). The CBCL includes two different versions: the Early Years version (for children between 1 ½ and 5 years of age) and the School Age version (for children and adolescents aged 6–18 years). Both the Early Years and School Age versions were used here. Both versions of CBCL have two main subscales which are structurally independent from each other (Achenbach and Rescorla, 2001), which map externalizing (e.g., aggression, impulsivity, and hyperactivity) and internalizing symptoms (e.g., distressful and over controlled behaviors). Higher total scores in each subscale or in each symptom category suggest the existence of more problematic behaviors, with t-scores of more than 60 to be considered in the range of clinical significance. Therefore, participants who scored higher than the cut-off point were excluded from further analyses.
Eye-Tracking Assessment
Stimuli
A total of 80 trials of colored happy-neutral, angry-neutral, and neutral–neutral face pairs constructed the experiment. All the face stimuli were selected from the MacBrain Face Stimulus Set1 (Tottenham et al., 2009) and were matched in terms of gender, race, and age. Available validity data for the MacBrain Face Stimulus Set in both children and adults have been reported high inter-rater agreement for the emotion that is displayed in these facial expressions (Tottenham et al., 2009). Pairs of faces were presented simultaneously side-by-side, with emotional faces presented equally on the right and the left side of the screen. In order to determine whether increased or reduced fixation duration toward the emotional faces (critical trials) resulted from heightened orientation, difficulty in disengaging from emotional stimuli, or both, the experiment was constructed using baseline neutral–neutral face pair trials (baseline; N = 70) and critical trials of emotional-neutral face trails (i.e., five angry-neutral pairs; five happy-neutral pairs; N = 10). The experiment started with seven baseline trials (pairs of neutral–neutral faces), where at least four baseline trials were presented between the critical trials (pairs of emotional-neutral faces). Baseline and critical trials were pseudorandomly allocated across the experiment in line with previous behavioral studies [e.g., Mogg et al. (2004), Salemink et al. (2007), Arndt and Fujiwara (2012), and Crawford et al. (2014)]. The eye-tracking experiment was programed using Experiment Builder software for EyeLink (SR Research, ON, Canada). The facial stimuli consisted of 38 color photographs of male and female faces1 (1024 × 768 pixels) depicting one of three expressions (neutral, happy, and angry). Although some of the neutral face pairs were repeated across the experiment, the neutral face stimuli used during the critical trials were not used elsewhere during the experiment. Therefore, face familiarity did not affect face preferences during critical trials.
Each trial began with a fixation point (in the shape of an animated dolphin), measuring 2.81 × 2.08 ° of visual angle in the middle of the screen which was displayed for 1000 ms (except in the case of mini calibration; see Section “Procedure”). This was followed by a pair of faces presented side by side against a white background for 2500 ms. The inter trial interval was 1000 ms (see Figure 1). The gap between the two faces was 7.2° of visual angle. Each stimulus pair was presented with a visual angle of 14.3 × 18.6°.
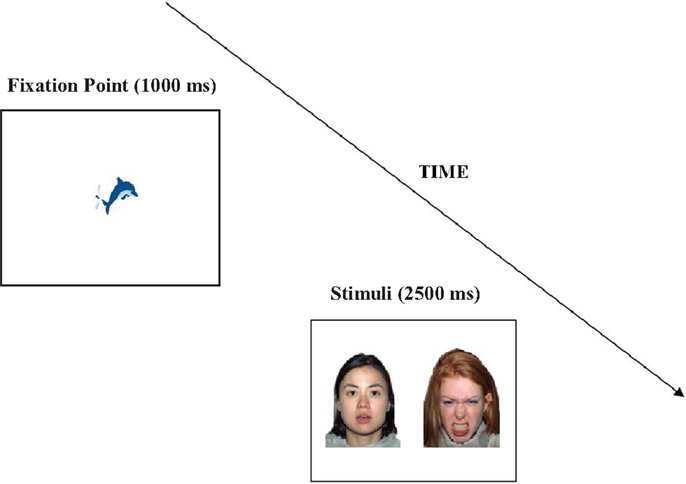
Figure 1. An example of the face stimuli pairs used in the eye-tracking experiment and an illustration of a trial structure.
Procedure
Participants’ eye movements were recorded using an Eyelink 1000 Tower Mount eye-tracking system and the stimuli were presented on a 19-inch CRT with a resolution of 1024 × 768 pixels. The eye-tracker sampled eye position at 500 Hz (i.e., every 2 ms). Average spatial accuracy is between 0.25° and 0.5° of visual angle. Participants were seated in a dimly lit room, 60 cm away from the display screen, and they had their head positioned against a head rest and their chin placed on a chinrest to minimize the possibility of movements. Viewing was binocular, but only data from the right eye were collected. During calibration, the EyeLink recorded the eye position at five target locations, providing the required reference data for computing gaze positions to ensure a point of fixation error rate of <0.5°. A mini calibration was repeated every five trials in order to ensure that eye movement data were adjusted for small-scale movement of the head. In the case of unsatisfactory eye-tracking, a 5-point calibration was repeated.
Data Collection Procedures
Children were told that they were going to see a range of interesting photos on a computer screen, while a special camera recorded their eye movements. The eye-tracking and behavioral assessments took place in one laboratory visit.
Analysis of Behavioral Data
All children in this study had t-scores of <60 (below subclinical range) at the CBCL. Therefore, no participants were excluded due to elevated clinical symptomatology. Raw scores from the two clusters of symptomatology (i.e., internalizing and externalizing symptoms) were used for statistical analysis from both CBCL versions following the authors’ guidelines (Achenbach and Rescorla, 2001, p. 89) and as previously reported in studies with children [e.g., Stanger et al. (2011)]. Higher total scores in each CBCL subscale suggest the existence of more problematic behaviors. For the measures of cognitive abilities (BAS II), mean standardized IQ scores were calculated.
Reduction of Eye-Tracking Data
Fixations were calculated via online detection analysis when eye movement met the following four criteria: (a) velocity threshold of 30°/s, b) a motion threshold of.1°, (c) a 8000°/s2 acceleration threshold, (d) and the pupil was not missing consecutively for three or more times from a sample. Trials were classified as “invalid” if a child did not look at all at the faces during the trial. In addition, if more than 40% invalid trials were evident, the participant’s data were excluded from further analyses. No participant met this exclusion criterion; therefore, all 49 participants provided valid eye-tracking data.
For analyses, each 2500 ms trial was divided into five 500 ms intervals. The relative mean proportions of viewing time for the angry and happy faces were then calculated for each 500 ms time interval of watching during the critical trials. This was done by subtracting the overall dwell time of the neutral stimuli (for each critical trial) from the overall dwell time looking at the emotional (happy or angry) face. This was done separately for each subject and for each happy and angry critical trial. Average dwell time of looking for each emotion type (i.e., angry, happy) was later calculated for each subject. Two additional regions of interest (RoIs) for the eyes and mouth region were identified. For this analysis, the neutral only/baseline pairs were used, where the coordinates of gaze for each eye as well as the mouth region were identified and extracted using the EyeLink Data viewer software. The overall amount of time spent (in ms) looking at the eye and mouth regions was divided by the amount of time spent (in ms) looking at the whole neutral face. This was done separately for each neutral baseline trial (for the overall 2500 ms), and then averaged across the baseline trials for each participant.
For both of these analyses, after the subtraction, positive values represented a visual preference for the emotionally expressive face (versus neutral) or facial feature, and negative values represented visual patterns that relate to avoidance behavior for the emotionally expressive face (versus neutral) or facial feature.
At this stage, it is worth underlining that eye-tracking studies have examined additional patterns of eye movements over time, such as mean proportion of fixations [e.g., Garner et al. (2006) and Gamble and Rapee (2010)], where vigilance–avoidance patterns of attention to angry faces have been reported for individuals with social phobia. However, the absence of continuous eye gaze data recorded from overall dwell time in these studies has resulted in significant criticism [e.g., Schofield et al. (2012)], suggesting that longer periods of looking time, as opposed to shorter (e.g., 500 ms; Schofield et al., 2012), provide the most reliable evidence for vigilance–avoidance patterns of visual scanning. To this end, the present study, in line with the procedures of other eye tracking studies with young populations [e.g., de Wit et al. (2008), Farzin et al. (2009), and Crawford et al. (2014)], reports the overall time spent looking at emotional faces as well as on the additional RoIs identified.
Analysis of Genetic Material
Genomic DNA was extracted from saliva samples using the Oragene OG-500 self-collection kit (Oragene, DNA Genotek Inc., Canada), according to the manufacturer’s recommendations. DNA concentrations ranged from 65 to 962 ng/ul and the 260/280 ratio was between 1.8 and 2 for all samples. Genotyping results were successfully obtained for all 49 subjects.
BDNF Val66Met Genotyping
Direct bidirectional sequencing was used to genotype the SNP within the BDNF gene (rs6265). PCR primers were designed to flank the polymorphism producing a 249 bp amplification product. Sequences of the primers are as follows: forward AAACATCCGAGGACAAGGTG and reverse AGAAGAGGAGGCTCCAAAGG. PCR was performed using Megamix PCR solution (supplied by Microzone UK Ltd.) in a total volume of 25 ul containing 25 pmol of each primer. An initial denaturation step at 95°C for 5 min was followed by 30 cycles of PCR (95°C 1 min, 58°C 1 min, 72°C 1 min) and then a final extension at 72°C for 10 min. PCR products were purified using Exonuclease I and Shrimp Alkaline Phosphatase (according to manufacturer’s instructions). Ten microliters sequencing reactions were generated, containing 0.25 ul BigDye Terminator (v3.1, Applied Biosystems), 1.9 ul molecular grade water, 3 pmol of forward or reverse primer, and 1 ul purified 5-HTTLPR PCR amplicon (diluted 1 in 2). Cycle conditions for sequencing included an initial denaturation step at 95°C for 5 min followed by 30 cycles of PCR (95°C 30 s, 50°C 10 s, 60°C 4 min) and reaction products were purified using CleanSEQ® beads (Agencourt) in a 1:1 ratio as described by the manufacturer. Products were resuspended in 70 ul molecular grade water and analyzed on a 3730 Genetic Analyzer (Applied Biosystems).
Allele frequencies for the BDNF Val66Met was n = 24 (25.5%) for Mel alleles and n = 74 (75.5%) for Met alleles, respectively. To this end, three genotype groups were generated, one with Met allele homozygotes (i.e., M/M; N = 3), heterozygotes V/M (N = 18), as well as homozygotes for the Val allele (i.e., V/V; N = 28). However, taken the small sample of participants homozygous for the low activity Met allele (N = 3) and the previous evidence associating the presence of at least one Met activity with behavioral outcomes [e.g., Wichers et al. (2008)], here, carriers of at least one Met allele [i.e., heterozygotes (Met/Val) and homozygotes for the Met allele (Met/Met)] were grouped in one “Met allele carriers” group (i.e., M/−; N = 21).
5-HTTLPR Genotyping
Direct bidirectional sequencing was used to genotype the 5-HTTLPR polymorphism. The region containing the 43 bp insertion polymorphism was amplified using primers described in Hu et al. (2006) producing a 528 bp amplification product from the L allele and a 485 bp product from the S allele. PCR was performed using Megamix PCR solution (supplied by Microzone UK Ltd.) in a total volume of 25 ul, containing 25 pmol of each primer and 3 ul of betaine. An initial denaturation step at 95°C for 5 min was followed by 30 cycles of PCR (95°C 1 min, 58°C 1 min, 72°C 1 min) and then a final extension at 72°C for 10 min. PCR products and the remaining sequencing procedure were the same as described for the BDNF Val66Met genotyping.
Allele frequencies across participants for 5-HTTLPR were n = 59 (42.1%) for Short allele and n = 81 (57.9%) for Long Allele. We classify three groups of participants: one with homozygous for the Short allele (S/S; N = 10), one with heterozygotes (S/L; N = 22), and one with participants homozygous for the Long allele (L/L; N = 17). Similar to the BDNF Val66Met genotype, carriers of at least one Short allele [i.e., Heterozygotes (S/L), and homozygotes for the Short allele (S/S) were grouped in one “Short allele carriers” group (i.e., S/−; N = 32) and compared with the remaining homozygous participants for the high serotonin uptake Long allele (L/L; N = 17). Hardy–Weinberg Equilibrium (HWE) for both 5-HTTLPR and BDNF Val66Met genotype was calculated using a tool available online (http://www.tufts.edu/~mcourt01/Documents/Court%20lab%20-%20HW%20calculator.xls).
Statistical Analyses
Preliminary Analyses
Descriptive statistics were conducted in order to describe the sample’s demographic characteristics such as, gender, age, and distribution of cognitive abilities. Raw data from the behavioral and cognitive scales were examined for normality using Kolmogorov–Smirnov tests. The CBCL subscales did not meet criteria for normal distributions (Kolmogorov–Smirnov, p < 0.05). Therefore, to further examine possible correlations between age, gender, IQ, and scores on the behavioral measures, Spearman’s Rho non-parametric correlations coefficients tests were also performed. Moreover, Pearson correlation analyses were conducted to determine if a correlation among demographic characteristics or cognitive performance and genotype group was evident, and Spearman correlation analyses were conducted to investigate possible correlations between BDNF Val66Met and 5-HTTLPR Genotypes and demographic, cognitive, and affective symptomatology in the sample.
Behavioral Ratings and Eye Gaze Patterns
The primary behavioral analysis examined whether children’s behavioral scores were correlated with fixation duration toward particular emotional faces and fixation duration toward facial features. For the cognitive abilities (BAS II) measures, mean standardized IQ-scores were assessed. Furthermore, correlation analyses were conducted to investigate possible correlation between dwell time looking at the emotional faces and participants’ demographic characteristics for each emotion and face feature separately, which showed no effect of age, developmental age, or gender in predicting overall looking time.
Genetics and Visual Scanning
To assess looking preferences toward and away from the emotional faces, overall dwell time spent fixating on the emotional face minus the overall dwell time spent fixating on the accompanying neutral face was computed for five time intervals: 0–500 ms (T1), 501–1000 ms (T2), 1001–1500 ms (T3), 1501–2000 ms (T4), and 2001–2500 ms (T5). A 2 (Emotion: positive versus negative) × 5 (Time: 0–500 versus 501–1000 versus 1001–1500 versus 1501–2000 versus 2001–2500 ms) mixed analysis of variance (ANOVA) with gender (female, male) and genotype (BDNF V/V versus M/−; 5-HTTLPR L/L versus S/−) as between-groups variables was conducted. All within subjects effects that violated the assumption of sphericity were adjusted using the Greenhouse–Geisser correction. To further evaluate the time course of attention, independent samples t-tests were conducted to determine whether there was a looking preference toward or away from the emotional images of a specific genotype group at any of the 500 ms time intervals. This was done for each SNP (BDNF Val66Met and 5-HTTLPR) and each facial expression (happy and angry), separately, after the initial ANOVA. When the data did not satisfy Kolmogorov–Smirnov tests for normality, Mann–Whitney U tests were performed instead of t-tests.
To investigate looking preferences toward the eye and mouth regions, a separate two-way mixed ANOVA with the repeated factor RoI (eyes, mouth) and genotype group (i.e., S/− versus L/L; M/− versus V/V) and gender as independent factor was conducted to examine gaze behavior for each face region for the baseline trials only (neutral–neutral face pairs). After the omnibus ANOVA, and because eye gaze data were non-normally distributed, a Mann–Whitney U test was conducted to investigate the 5-HTTLPR genotype effects on overall viewing time for the eye and mouth regions, respectively. As a secondary analysis, the original ANOVA was repeated using with additional RoIs as repeated factor (eyes, mouth, forehead, nose, cheeks, chin) and genotype group (i.e., S/− versus L/L; M/− versus V/V) and gender as independent factor (see also Figure S1 in Supplementary Material for an example of RoIs selection).
Results
Demographic Characteristics
Tables 1 and 2 present participants’ main demographic characteristics, including gender, age, and cognitive abilities. Correlation analyses did not reveal any significant correlation between demographic characteristics and behavioral measures, or correlations between demographics, early symptomatology, and genotype. Moreover, t-tests showed that the two 5-HTTLPR genotype groups did not differ in terms of age [t(47) = −0.264, p = 0.793], gender [t(47) = 0.994, p = 0.325], IQ [t(47) = −1.17, p = 0.245], developmental age [t(47) = −0.245, p = 0.808], or other behavioral measures. Similarly, the two BDNF genotype groups did not differ in terms of age [t(47) = 0.107, p = 0.915], gender [t(47) = 0.162, p = 0.872], IQ [t(47) = −0.427, p = 0.671], or developmental age [t(47) = −0.223, p = 0.824]. BDNF Val66Met [x2(1) = 0.002, p = 0.962] and 5-HTTLPR genotype frequencies [x2(1) = 0.340, p = 0.559] were in Hardy–Weinberg equilibrium.
Behavioral Effects in Fixation Duration
Pearson correlation analyses revealed a negative correlation between externalizing symptomatology and children’s age (r = −0.362, p = 0.011). No further relationships of participants’ demographic characteristics in cognitive development or early affective symptoms were observed. Furthermore, Spearman’s Rho correlations showed a significant positive correlation between internalizing and externalizing symptoms (r = 0.524, p < 0.001). In addition, since the eye movement data varied in terms of normality across different time points of processing (i.e., Happy T2, T3, T4 and Angry T2, T5 were p > 0.005; Happy T1, T5 and Angry T1, T3, T4 were p < 0.05 in Kolmogorov–Smirnov test of normality), both parametric and non-parametric correlation analyses were conducted with CBCL subscales and looking dwell time spent for each time point and each type of emotion separately. These analyses revealed no significant correlations.
Genotype Effects in Fixation Duration for Emotional Expressions
A 2 (Emotion: positive versus negative) × 5 (Time: 0–500 versus 501–1000 versus 1001–1500 versus 1501–2000 versus 2001–2500 ms) mixed ANOVA with Gender (female, male) and Genotype (BDNF M/− versus V/V) as between-groups factors revealed a main effect of Emotion, [F(1, 45) = 7.10, , p = 0.011], a main effect of Time [F(4, 180) = 46.89, , p < 0.001], and a two-way Emotion by Time interaction [F(4, 180) = 13.07, , p < 0.001]. In terms of genotype effects, a two-way Time by BDNF Genotype [F(4, 180) = 4.01, , p = 0.004], as well as a three-way Emotion by Time by BDNF genotype interaction [F(4, 45) = 3.52, , p = 0.009] were evident. No further interaction effects were observed.
To further delineate the observed Time by BDNF genotype effect, the dwell time at each of the five time points was averaged across the two emotions. A Kolmogorov–Smirnov test of normality showed that the averaged data at each time point were normally distributed (p > 0.005); therefore, t-tests were conducted at each time point of visual scanning averaged across the two emotions. This analysis revealed a significant difference between the two genotype groups (i.e., M/− versus V/V) on the time spent looking at emotional stimuli during T4 [t(47) = −0.205, p < 0.05)]. Moreover to delineate the three-way Emotion by Time by BDNF interaction, follow up analyses were conducted to determine whether there was a preference toward or away each emotion at each of the time intervals. Because a Kolmogorov–Smirnov test revealed that the relative viewing time between stimuli in specific time points were normally distributed (e.g., Happy T2, T3, T4 and Angry T2, T5 where p > 0.005; where Happy T1, T5 and Angry T1, T3, T4 where p < 0.05 in Kolmogorov–Smirnov test of normality), this analysis was followed up with complementary parametric and non-parametric analyses at each Time Point separately.
For the time points with not-normally distributed data, a Mann–Whitney U-test revealed a significant difference between the two BDNF genotypes in the dwell time toward the facial expressions of Anger at T4 (U = 157.00, p = 0.010; see Figure 2; Table S3 in Supplementary Material). Moreover for the normally distributed time points, a t-test for T5 was shown that the carriers of the low neuroplasticity Met allele spent significantly less time looking at the angry faces [t(47) = −2.10, p = 0.041], which was absent for the happy faces. By contrast, carriers of two copies of the Val allele exhibited an increase in time looking to the angry faces.
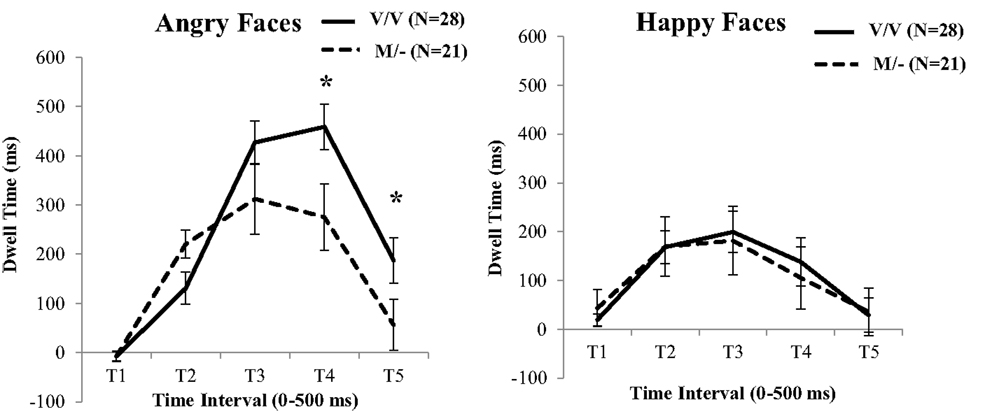
Figure 2. BDNF genotype differences for fixation durations to facial expressions of Anger versus Neutral (left) and Happiness versus Neutral (right). Carriers of at least one Met allele fixated angry face more at the early stages, but later exhibited an avoidance pattern of attention to these faces. Error bars denotes 1 SEM. *p < 0.05.
In continuing, the above ANOVA analysis was repeated with the 5-HTTLPR genotype (i.e., L/L versus S/−) as a between factor. Contrary to the BDNF genotype effects, this analysis did not show a significant Time by 5-HTTLPR [F(1, 45) = 1.35, , p = 0.857], or an Emotion by Time by 5-HTTLPR interaction [F(1, 45) = 0.33, , p = 0.011; see Figure 3]. No further effects were detected from this analysis.
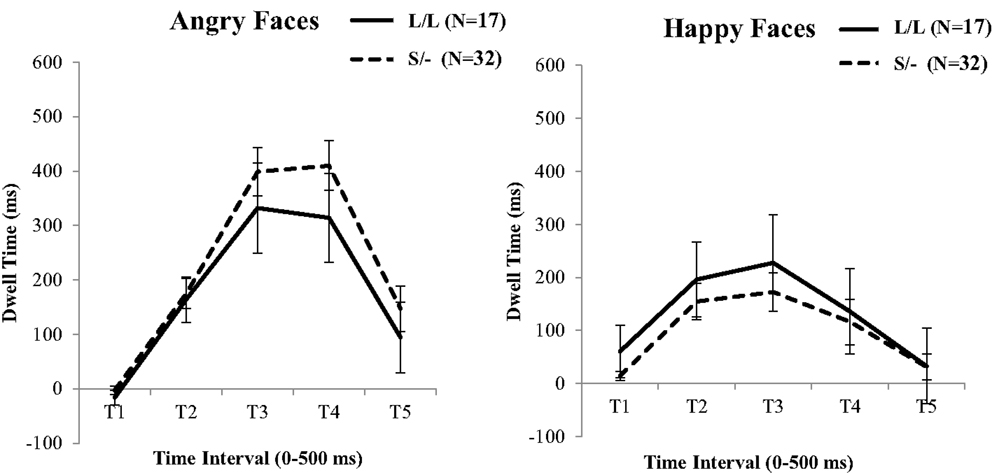
Figure 3. 5-HTTLPR genotype differences in fixation duration to facial expressions of Anger versus Neutral (left) and Happiness versus Neutral (right). Genotype groups do not differ at any time point across the two types of emotional faces. Error bars denotes 1 SEM.
Genotype Effects on Gaze Patterns
A two-way mixed ANOVA, with the repeated factor RoI (eyes, mouth) and genotype group (V/V, M/−; L/L, S/−) and gender as independent factors, examined gaze behavior for each face region on the baseline trials. There was a significant effect of RoI for the face areas of interest [F(1,45) = 126.11, p < 0.001], whereby children spent more time looking at the eyes region of the neutral faces (see also Table S4 in Supplementary Material). However, a significant interaction between RoI and BDNF genotype group was not evident [F(1,45) = 0.74, , p = 0.393]. By contrast, the 5-HTTLPR genotype shown a significant interaction between RoI and genotype group [F(1,45) = 7.25, , p = 0.010]. To further examine the interaction effects observed in the ANOVA, and given that the data for the eyes region met normal distribution criteria (Kolmogorov–Smirnov test p > 0.05) complementary parametric tests were conducted. Therefore, an independent samples t-test was performed to further investigate the association between viewing time of the eyes region and the Genotype (L/L, S/−). This analysis revealed a significant effect of the 5-HTTLPR Genotype group on viewing time for the eye region [t(47) = 27.15, p = 0.008], providing evidence that Short allele carriers spent relatively less time viewing the eye region compared to participants homozygous for the Long allele. This evidence provides support for the statistical interaction observed in the initial ANOVA (see Figure 4; Table S4 in Supplementary Material).
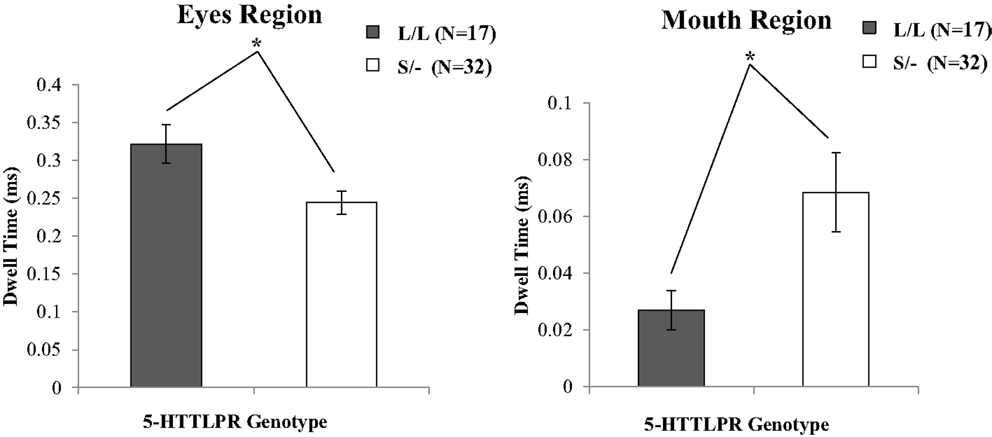
Figure 4. The proportion of time spent among 5-HTTLPR genotype groups looking at the eye and mouth regions relative to the rest of the face during baseline trials. Carriers of at least one Short allele fixated the eye region less, but spent more time looking the mouth region of neutral faces. Error bars denote 1 SEM. *p < 0.01.
Because a Kolmogorov–Smirnov test revealed that the data for the mouth region data were not normally distributed (p > 0.05), this analysis was followed up with complementary non-parametric tests using a Mann–Whitney U in order to further investigate the way in which 5-HTTLPR genotype groups (i.e., L/L versus S/−) differ in the time spent looking the mouth region.
This analysis revealed a significant effect of the 5-HTTLPR Genotype group on the viewing time for the mouth region (U = 139.0, p = 0.005), indicating that Short allele carriers spent relatively more time viewing the mouth region compared to participants homozygous for the Long allele. Moreover, a significant effect of genotype on looking the eye region was evident, where short allele carriers spent significant less dwell time fixating the eye region (U = 168.0, p = 0.029), when compared to carriers of two copies of the long allele. This evidence provides support for the statistical interaction (see Figure 4; Table S4 in Supplementary Material) observed in the initial ANOVA.
As a complementary analysis, we followed up the original effects of 5-HTTLPR genotype on the viewing time of the eyes versus mouth region to examine within genotype differences for looking at the eye versus mouth regions for each genotype. Therefore, we conducted additional Wilcoxon matched pairs tests which show that 5-HTTLPR S/− carriers spent significantly more time looking the mouth [t(31) = 16.15, p < 0.001] versus the eyes region [Z = −4.31, p < 0.001]. Conversely, 5-HTTLPR L/L carriers spent significantly more time looking at the eyes versus the mouth region [Z = −3.62, p < 0.001].
Repetition of the original ANOVA with additional RoIs as repeated factor (eyes, mouth, forehead, nose, cheeks, chin) confirmed the main effect of region [F(5,225) = 127.48, , p < 0.001] and a significant two-way interaction effect between Region and 5-HTTLPR genotype [F(5, 225) = 3.79, , p = 0.017]. To further examine the interaction effects observed in the ANOVA, and given that the data for the nose region met normal distribution criteria (Kolmogorov–Smirnov test p > 0.05), complementary parametric tests were conducted. Therefore, an independent samples t-test was performed to further investigate the association between viewing time of the nose region and the Genotype (L/L, S/−). This analysis did not revealed a significant effect of the 5-HTTLPR Genotype group on viewing time for the eye region [t(47) = –0.51, p = 0.610]. Similarly, Mann–Whitney U-tests for the rest of the RoIs (Kolmogorov-Smirnov test p > 0.05) did not show any effect of the 5-HTTLPR genotype for the viewing time spend looking the forehead (U = 237.5, p = 0.468), cheeks (U = 249.5, p = 0.636), and chin RoI (U = 218.5, p = 0.249).
Discussion
The present study was designed to examine relationships between normal variations in genetic SNPs involved in both neural plasticity (BDNF Val66Met) and serotonin availability (5-HTTLPR) and visual scanning of faces in typically developing young children. It is well-established that carriers of the BDNF Met allele exhibit increased reactivity in response to emotional stimuli (Montag et al., 2008; Schofield et al., 2009; Lau et al., 2010). In a separate line of research, 5-HTTLPR Short allele carriers have been reported to exhibit increased susceptibility for psychological maladjustment and affective disorders, such as anxiety (Munafo et al., 2008; Carver et al., 2009). In the present study, young children carrying the BDNF Met allele and children carrying the 5-HTTLPR Short allele exhibited potentially reactive patterns of visual scanning of faces. Specifically, it was shown that children carrying the low activity BDNF Met allele exhibited a robust vigilance–avoidance pattern of visual scanning when processing angry but not happy faces, when compared to Val allele homozygotes. Moreover, the carriers of the low activity 5-HTTLPR Short allele spent significantly less time looking at the eye region of the face relative to the whole face, and also spent more time looking at the mouth region, compared with participants homozygous for the high serotonin activity Long allele. Although participants were not followed into adulthood, both of these findings may be relevant to later psychological difficulties and disorders.
There is increasing evidence from studies of children to suggest heightened neurophysiological sensitivity of Met allele carriers in response to negative environmental stressors (Montag et al., 2008; Schofield et al., 2009; Lau et al., 2010; Scharinger et al., 2010; Gerritsen et al., 2011). Consistent with this notion, and in line with the current study’s hypothesis, Met allele carriers spent more time fixating angry versus neutral facial expressions during early stages of processing (501–1000 ms), which decreased during later stages of processing (1501–2000 ms; 2001–2500 ms). On the other hand, participants homozygous for the high activity Val allele did not show similar patterns of avoidance. Instead, they spent significantly more time looking at the angry faces after 1500 ms, suggesting the existence of a resilience mechanism expressed through the vigilant exploration of the negative facial expression. This is consistent with previous evidence suggesting the existence of a protective mechanism against forms of psychopathology in carriers of two copies of the high neuroplasticity Val allele [e.g., Zhang et al. (2014)]. To this end, the current study provides support for the hypothesis that normal variations that modulate low neuroplasticity are associated with visual scanning pathways of emotional stimuli, perhaps due to critical influences of the BDNF Val66Met on the connectivity between the amygdala and the PFC (Carlson et al., 2013). However, the analyses did not reveal a similar effect of 5-HTTLPR genotype on the processing of emotional faces. While this finding may be considered inconsistent with some previous neurophysiological and behavioral studies of children and adults that have suggested 5-HTTLPR effects related to responses to emotional faces (Homberg and Lesch, 2010; Thomason et al., 2010), it is possible that developmental effects of the sample, or differences in the material used, may have contributed to these inconsistencies.
In addition to the effects of the BDNF Val66Met genotype in predicting preferential looking, a separate analysis suggested a role of the serotonin transporter 5-HTTLPR polymorphism in associating with gaze direction toward the eye and the mouth regions of faces posed in neutral expressions in the current study. Consistent with a plethora of studies suggesting the existence of neurobiological susceptibility for negative affectivity, such as stress reactivity, in carriers of the Short 5-HTTLPR allele [e.g., Caspi et al. (2003), Thomason et al. (2010), Mercer et al. (2012), and Disner et al. (2013)], the pattern of results of the present study showed for the first time that, early in life, the presence of the Short 5-HTTLPR may be related to face scanning behavior that has previously been associated with pervasive anxiety and/or shyness [e.g., Horley et al. (2004)]. More specifically, carriers of the low activity Short allele spent significantly less time looking at the eye region relative to the rest of the face, compared to the participants homozygous for the high serotonin activity Long allele. These individuals also spent significantly more time looking at the mouth region. Interestingly, atypicalities in allocating attention to facial features have been widely evident in various atypically developing populations, including Fragile X Syndrome, which is a disorder of both social and intellectual functioning that has been shown to be related to pervasive social anxiety and shyness (Farzin et al., 2009; Crawford et al., 2014).
One possible explanation for the observed pattern of looking behaviors is that 5-HTTLPR Short allele carriers switched their attention away from the eye region of neutral faces, engaging in a type of vigilance pattern of scanning the mouth region as a compensatory mechanism to down-regulate heightened reactivity when processing the eye region. Attention to the eyes is considered necessary for effective emotion recognition, with healthy individuals being reported to first fixate on the eyes and subsequently spend relatively more time looking at the eye region compared to the mouth region of the face (Itier and Batty, 2009). This possibility that 5-HTTLPR Short allele carriers, known to experience higher susceptibility for poor reactivity to distressing negative emotional cues, exhibit such a pattern may help to link with the literature that suggests that reduced looking to the eye region is evident in individuals with social anxiety (Horley et al., 2004; Crawford et al., 2014). The current results further suggest that the neurobiology of 5-HTTLPR Short allele carriers may contribute to this pattern of early eye gaze and, in conjunction with other factors such as negative life events, may relate to later affect-related difficulties or psychopathology.
An additional aim of the present study was to examine the relationship between processing of emotional faces and facial features with early affective symptomatology, as indexed by parent questionnaires. Contrary to the study’s hypotheses, the present results did not uncover associations between parent reports of early affective symptomatology and overall fixation duration toward emotional faces. One potential explanation for this finding may be related to the study’s sample age, which consisted of young and unaffected children compared to previous observations with older children [see Battaglia et al. (2004)] or adolescents (Gamble and Rapee, 2009). Therefore, it is plausible that as individuals are exposed to a broad range and more variable rates of stress-induced situations (e.g., school transitions), they may be more behaviorally reactive in response to environmental stressors, for example. Another possibility in this respect may be related to differences in the stimulus materials or the experimental design used in the present study. For instance, previous studies have used various negative emotional faces (Gamble and Rapee, 2009), as opposed to only angry negative emotional faces, or longer periods of angry-neutral face pair presentations (Gamble and Rapee, 2010). As Bons et al. (2013) indicate, both of these variables may be critical in shaping the patterns of findings in studies examining individual differences and may contribute to the discrepancy among studies in typical and atypical development (Bons et al., 2013).
The allocation of attention is a critical ability, which has been suggested to reflect the development and functioning of fronto-cortical circuits that ultimately reach development during the late adolescent years (Hare et al., 2005; Pessoa, 2010). Consistent with this notion, it is now widely accepted that children undergo critical periods in the formation of neural circuits that are involved in effective emotion regulation. It is critical to identify the genetic and neurobiological mechanisms contributing to behaviors that may be indicative of later psychological maladjustment early in life. In line with the findings of the current study, cognitive models of child anxiety suggest that threat avoidance may maintain anxiety in children, since children are not developing critical evaluation abilities for the formation of effective emotion regulation (Rapee, 2002; Hudson and Rapee, 2004). The present findings fill an existing gap in the literature, contributing to our understanding of the potential effect of variations in BDNF Val66Met and 5-HTTLPR genotypes as moderators of preferential looking toward facial expressions of threat/anger and facial features, which may suggest the existence of behavioral patterns that may link with heightened sensitivity in response to environmental stressors and the presence of psychological problems and behaviors later in life.
In summary, the current study provides evidence to suggest that carriers of the Met BDNF allele exhibited a vigilance–avoidance pattern on the dwell time looking at angry versus neutral facial expressions, compared with a high neuroplasticity homozygous Val genotype group, early in childhood. Moreover, Short allele carriers of the 5-HTTLPR polymorphism spent significantly less overall time looking at the eyes, compared with Long allele homozygotes. Taken together, these results suggest that normal variation in genetic single-nucleotide polymorphisms may contribute to the establishment of particular patterns of visual scanning toward faces and face features early in life. Overall, the outcomes of the study are consistent with existing evidence from the adult, adolescent, and child psychopathology research literatures suggesting a contribution of both BDNF Val66Met and 5-HTTLPR genotypes to variations in affective and emotional regulation that may be relevant to later psychological difficulties and disorders. The current findings further offer insights into particular relationships between genetic, neural, and cognitive/behavioral functions that may be related to one another in this context.
Conflict of Interest Statement
The authors declare that the research was conducted in the absence of any commercial or financial relationships that could be construed as a potential conflict of interest.
Supplementary Material
The Supplementary Material for this article can be found online at http://journal.frontiersin.org/article/10.3389/fnbeh.2015.00175
Footnote
- ^The MacBrain Face stimuli used here are as follows: Angry Faces: 01F, 05F, 06F, 20M, 22M; Happy Faces: 08F, 11F, 12F, 24M, 26M; Neutral Faces: 02F, 03F, 07F, 09F, 10F,13F, 14F, 15F, 16F, 17F, 18F, 19F, 21M, 23M, 25M, 28M, 29M, 30M, 31M, 32M, 33M, 34M, 35M, 36M, 37M, 38M.
References
Achenbach, T., and Rescorla, L. (2001). The Manual for the ASEBA School-Age Forms & Profiles. Burlington, VT: University of Vermont, Research Center for Children, Youth, and Families.
Arndt, J. E., and Fujiwara, E. (2012). Attentional bias towards angry faces in trait-reappraisal. Pers. Individ. Dif. 52, 61–66. doi: 10.1016/j.paid.2011.08.030
Baron-Cohen, S., Wheelwright, S., and Jolliffe, T. (1997). Is there a language of the eyes’? Evidence from normal adults and adults with autism or asperger syndrome. Vis. cogn. 4, 311–331. doi:10.1080/713756761
Battaglia, M., Ogliari, A., Zanoni, A., Citterio, A., Pozzoli, U., Giorda, R., et al. (2005). Influence of the serotonin transporter promoter gene and shyness on children’s cerebral responses to facial expressions. Arch. Gen. Psychiatry 62, 85–94. doi:10.1001/archpsyc.62.1.85
Battaglia, M., Ogliari, A., Zanoni, A., Villa, F., Citterio, A., Binaghi, F., et al. (2004). Children’s discrimination of expressions of emotions: relationship with indices of social anxiety and shyness. J. Am. Acad. Child Adolesc. Psychiatry 43, 358–365. doi:10.1097/00004583-200403000-00019
Becker, S. I., Horstmann, G., and Remington, R. W. (2011). Perceptual grouping, not emotion, accounts for search asymmetries with schematic faces. J. Exp. Psychol. 37, 1739–1757. doi:10.1037/a0024665
Beevers, C. G., Wells, T. T., and McGeary, J. E. (2009). The BDNF Val66Met polymorphism is associated with rumination in healthy adults. Emotion 9, 579–584. doi:10.1037/a0016189
Belsky, J. (1997). Theory testing, effect-size evaluation, and differential susceptibility to rearing influence: the case of mothering and attachment. Child Dev. 68, 598–600. doi:10.2307/1132110
Belsky, J., Bakermans-Kranenbur, M. J., and van IJzendoorn, M. H. (2007). For better and for worse: differential susceptibility to environmental influences. Curr. Dir. Psychol. Sci. 16, 300–304. doi:10.1111/j.1467-8721.2007.00525.x
Blais, C., Roy, C., Fiset, D., Arguin, M., and Gosselin, F. (2012). The eyes are not the window to basic emotions. Neuropsychologia 50, 2830–2838. doi:10.1016/j.neuropsychologia.2012.08.010
Boll, S., and Gamer, M. (2014). 5-HTTLPR modulates the recognition accuracy and exploration of emotional facial expressions. Front. Behav. Neurosci. 8:255. doi:10.3389/fnbeh.2014.00255
Bons, D., van den Broek, E., Scheepers, F., Herpers, P., Rommelse, N., and Buitelaaar, J. K. (2013). Motor, emotional, and cognitive empathy in children and adolescents with autism spectrum disorder and conduct disorder. J. Abnorm. Child Psychol. 41, 425–443. doi:10.1007/s10802-012-9689-5
Calvo, M. G., and Avero, P. (2005). Time course of attentional bias to emotional scenes in anxiety: gaze direction and duration. Cogn. Emot. 19, 433–451. doi:10.1080/02699930441000157
Carlson, J. M., Mujica-Parodi, L. R., Harmon-Jones, E., and Hajcak, G. (2013). The orienting of spatial attention to backward masked fearful faces is associated with variation in the serotonin transporter gene. Emotion 12, 203–207. doi:10.1037/a0025170
Carver, C. S., Johnson, S. L., and Joormann, J. (2009). Two-mode models of self-regulation as a tool for conceptualizing effects of the serotonin system in normal behavior and diverse disorders. Curr. Dir. Psychol. Sci. 18, 195–199. doi:10.1111/j.1467-8721.2009.01635.x
Caspi, A., Sugden, K., Moffitt, T. E., Taylor, A., Craig, I. W., Harrington, H. L., et al. (2003). Influence of life stress on depression: moderation by a polymorphism in the 5-HHTgene. Science 301, 291–293. doi:10.1126/science.1083968
Christou, A., Endo, S., Wallis, Y., Bair, H., Zeegers, M., and McCleery, J. P. (2015). Variation in serotonin transporter linked polymorphic region (5-HTTLPR) short/long genotype modulates resting frontal electroencephalograhy asymmetries in children. Dev. Psychopathol. doi:10.1017/S0954579415000413
Crawford, H., Moss, J., Anderson, G., Oliver, C., and McCleery, J. P. (2014). Implicit discrimination of basic facial expressions of positive/negative emotion in Fragile X syndrome and autism spectrum disorder. Am. J. Intellect. Dev. Disabil. (in press).
Dailey, M. N., and Cottrell, G. W. (1999). PCA = Gabor for Expression Recognition. Technical Report CS-629. San Diego, CA: University of California.
Dalton, K. M., Holsen, L., Abbeduto, L., and Davidson, R. J. (2008). Brain function and gaze fixation during facial-emotion processing in fragile X and autism. Autism Res. 1, 231–239. doi:10.1002/aur.32
de Wit, T., Falck-Ytter, T., and Von Hofsten, C. (2008). Young children with autism spectrum disorder look differently at positive versus negative emotional faces. Res. Autism Spectr. Disord. 2, 651–659. doi:10.1016/j.rasd.2008.01.004
Disner, S. G., Beevers, C. G., Lee, H.-J., Ferrell, R. E., Hariri, A. H., and Telch, M. J. (2013). War zone stress interacts with the 5-HTTLPR polymorphism to predict the development of sustained attention for negative emotion stimuli in soldiers returning from Iraq. Clin. Psychol. Sci. 1, 413–425. doi:10.1177/2167702613485564
Egan, M. F., Kojima, M., Callicott, J. H., Goldberg, T. E., Kolachana, B. S., Bertolino, A., et al. (2003). The BDNF val66met polymorphism affects activity-dependent secretion of BDNF and human memory and hippocampal function. Cell 112, 257–269. doi:10.1016/S0092-8674(03)00035-7
Elliot, C. D., Smith, P., and McCulloch, K. (1996). British Ability Scales. Windsor: NFER-Nelson. Diagnostic and Statistical Manual of Mental Disorders (4th ed., text revision) Washington: American Psychiatric Association; 2000.
Farzin, F., Rivera, S. M., and Hessl, D. L. (2009). Visual processing of faces in individuals with fragile X syndrome: an eye tracking study. J. Autism Dev. Disord. 39, 946–952. doi:10.1007/s10803-009-0744-1
Fox, E., and Damjanovic, L. (2006). The eyes are sufficient to produce a threat superiority effect. Emotion 6, 534–539. doi:10.1037/1528-3542.6.3.534
Gamble, A. L., and Rapee, R. M. (2009). The time-course of attentional bias in anxious children and adolescents. J. Anxiety Disord. 23, 841–847. doi:10.1016/j.janxdis.2009.04.001
Gamble, A. L., and Rapee, R. M. (2010). The time-course of attention to emotional faces in social phobia. J. Behav. Ther. Exp. Psychiatry 41, 39–44. doi:10.1016/j.jbtep.2009.08.008
Garner, M., Mogg, K., and Bradley, B. P. (2006). Orienting and maintenance of gaze to facial expressions in social anxiety. J. Abnorm. Psychol. 115, 760–770. doi:10.1037/0021-843X.115.4.760
Gatt, J. M., Nemeroff, C. B., Dobson-Stone, C., Paul, R. H., Bryant, R. A., Schofield, P. R., et al. (2009). Interactions between BDNF Val66Met polymorphism and early life stress predict brain and arousal pathways to syndromal depression and anxiety. Mol. Psychiatry 14, 681–695. doi:10.1038/mp.2008.143
Gerritsen, L., Tendolkar, I., Franke, B., Vasquez, A. A., Kooijman, S., Buitelaar, J., et al. (2011). BDNF Val66Met genotype modulates the effect of childhood adversity on subgenual anterior cingulate cortex volume in healthy subjects. Mol. Psychiatry 17, 597–603. doi:10.1038/mp.2011.51
Gilboa-Schechtman, E., Foa, E. B., and Amir, N. (1999). Attentional biases for facial expressions in social phobia: the face-in-the-crowd paradigm. Cogn. Emot. 13, 305–318. doi:10.1080/026999399379294
Gold, J. M., Tadin, D., Cook, S. C., and Blake, R. (2008). The efficiency of biological motion perception. Percept. Psychophys. 70, 88–95. doi:10.3758/PP.70.1.88
Groves, J. O. (2007). Is it time to reassess the BDNF hypothesis of depression? Mol. Psychiatry 12, 1079–1088. doi:10.1038/sj.mp.4002075
Guillon, Q., Hadjikhani, N., Baduel, S., and Rogé, B. (2014). Visual social attention in autism spectrum disorder: insights from eye tracking studies. Neurosci. Biobehav. Rev. 42, 279–297. doi:10.1016/j.neubiorev.2014.03.013
Hansen, C., and Hansen, R. (1988). Finding the face in the crowd: an anger superiority effect. J. Pers. Soc. Psychol. 54, 917–924. doi:10.1037/0022-3514.54.6.917
Hare, T. A., Tottenham, N., Davidson, M. C., Glover, G. H., and Casey, B. J. (2005). Contributions of amygdala and striatal activity in emotion regulation. Biol. Psychiatry 57, 624–632. doi:10.1016/j.biopsych.2004.12.038
Hazlett, H. C., Poe, M. D., Lightbody, A. A., Styner, M., MacFall, J. R., Reiss, A. L., et al. (2012). Trajectories of early brain volume development in fragile X syndrome and autism. J. Am. Acad. Child Adolesc. Psychiatry 51, 921–933. doi:10.1016/j.jaac.2012.07.003
Holmes, A., Bradley, B. P., Nielsen, M., and Mogg, K. (2009). Attentional selectivity for emotional faces: evidence from human electrophysiology. Psychophysiology 46, 62–68. doi:10.1111/j.1469-8986.2008.00750.x
Holsen, L. M., Dalton, K. M., Johnstone, T., and Davidson, R. J. (2008). Prefrontal social cognition network dysfunction underlying face encoding and social anxiety in fragile X syndrome. Neuroimage 43, 592–604. doi:10.1016/j.neuroimage.2008.08.009
Homberg, J. R., and Lesch, P. (2010). Looking on the brightside of serotonin transporter gene variation. Biol. Psychiatry 69, 513–519. doi:10.1016/j.biopsych.2010.09.024
Horley, K., Williams, L., Gonsalvez, C., and Gordon, E. (2004). Face to face: visual scan path evidence for abnormal processing of facial expressions in social phobia. Psychiatry Res. 127, 45–53. doi:10.1016/j.psychres.2004.02.016
Horstmann, G., and Bauland, A. (2006). Search asymmetries with real faces: testing the anger-superiority effect. Emotion 6, 193–207. doi:10.1037/1528-3542.6.2.193
Hu, X., Lipsky, R. H., Zhu, G., Akhtar, L. A., Taubman, J., and Greenberg, B. D. (2006). Serotonin transporter promoter gain-of-function genotypes are linked to obsessive-compulsive disorder. Am. J. Hum. Genet. 78, 815–826. doi:10.1086/503850
Hudson, J. L., and Rapee, R. M. (2004). “From anxious temperament to disorder: an etiological model of generalized anxiety disorder,” in Generalized Anxiety Disorder: Advances in Research and Practice, eds R. G. Heimberg, C. L. Turk, and D. S. Mennin (New York, NY: Guilford), 51–74.
Itier, R. J., and Batty, M. (2009). Neural bases of eye and gaze processing: the core of social cognition. Neurosci. Biobehav. Rev. 33, 843–863. doi:10.1016/j.neubiorev.2009.02.004
Karg, K., Burmeister, M., Shedden, K., and Sen, S. (2011). The serotonin transporter promoter variant (5-HTTLPR), stress, and depression meta-analysis revisited: evidence of genetic moderation. Arch. Gen. Psychiatry 68, 444–454. doi:10.1001/archgenpsychiatry.2010.189
Kliemann, D., Dziobek, I., Hatri, A., Steimke, R., and Heekeren, H. R. (2010). Atypical reflexive gaze patterns on emotional faces in autism spectrum disorders. J. Neurosci. 30, 12281–12287. doi:10.1523/JNEUROSCI.0688-10.2010
Klin, A., Jones, W., Schultz, R., Volkmar, F. R., and Cohen, D. J. (2002). Visual fixation patterns during viewing of naturalistic social situations as predictors of social competence in individuals with autism. Arch. Gen. Psychiatry 59, 809–816. doi:10.1001/archpsyc.59.9.809
Koster, E. H. W., De Raedt, R., Goeleven, E., Franck, E., and Crombez, G. (2005). Mood-congruent attentional biases in dysphoria: maintained attention to and impaired attentional disengagement from negative information. Emotion 5, 446–455. doi:10.1037/1528-3542.5.4.446
Lau, A. G., Irier, H. A., Gu, J., Tian, D., Ku, L., Liu, G., et al. (2010). Distinct 3 UTRs differentially regulate activity-dependent translation of brain-derived neurotrophic factor (BDNF). Proc. Natl. Acad. Sci. U.S.A. 107, 15945–15950. doi:10.1073/pnas.1002929107
Lau, J. Y., Goldman, D., Buzas, B., Fromm, S. J., Guyer, A. E., Hodgkinson, C., et al. (2009). Amygdala function and 5-HTT gene variants in adolescent anxiety and major depressive disorder. Biol. Psychiatry 65, 349–355. doi:10.1016/j.biopsych.2008.08.037
Lesch, K.-P., Bengel, D., Heils, A., Sabol, S. Z., Greenberg, B. D., Petri, S., et al. (1996). Association of anxiety-related traits with a polymorphism in the serotonin transporter gene regulatory region. Science 274, 1527–1531. doi:10.1126/science.274.5292.1527
Lipp, O. V., Price, S. M., and Tellegen, C. L. (2009). No effect of inversion on attentional and affective processing of facial expressions. Emotion 9, 248–259. doi:10.1037/a0014715
Lu, B. (2003). BDNF and activity-dependent synaptic modulation. Learn. Mem. 10, 86–98. doi:10.1101/lm.54603
Mercer, K. B., Orcutt, H. K., Quinn, J. F., Fitzgerald, C. A., Conneely, K. N., Barfield, R. T., et al. (2012). Acute and posttraumatic stress symptoms in a prospective gene × environment study of a university campus shooting. Arch. Gen. Psychiatry 69, 89–97. doi:10.1001/archgenpsychiatry.2011.109
Mogg, K., Philipot, P., and Bradley, B. P. (2004). Selective attention to angry faces in clinical social phobia. J. Abnorm. Psychol. 113, 160–165. doi:10.1037/0021-843X.113.1.160
Montag, C., Basten, U., Stelzel, C., Fiebach, C. J., and Reuter, M. (2008). The BDNF Val66Met polymorphism and smoking. Neurosci. Lett. 442, 30–38. doi:10.1016/j.neulet.2008.06.064
Munafo, M. R., Brown, S. M., and Hariri, A. R. (2008). Serotonin transporter (5-HTTLPR) genotype and amygdala activation: a meta-analysis. Biol. Psychiatry 63, 852–857. doi:10.1016/j.biopsych.2007.08.016
Nation, K., and Penny, S. (2008). Sensitivity to eye gaze in autism: is it normal? is it automatic? is it social? Dev. Psychopathol. 20, 79–97. doi:10.1017/S0954579408000047
Norbury, C. F., Brock, J., Cragg, L., Einav, S., Griffiths, H., and Nation, K. (2009). Eye-movement patterns are associated with communicative competence in autistic spectrum disorders. J. Child Psychol. Psychiatry 50, 834–842. doi:10.1111/j.1469-7610.2009.02073.x
Öhman, A., Juth, P., and Lundqvist, D. (2010). Finding the face in a crowd: relationships between distractor redundancy, target emotion, and target gender. Cogn. Emot. 24, 1216–1228. doi:10.1080/02699930903166882
Öhman, A., and Mineka, S. (2001). Fear, phobias and preparedness: toward an evolved module of fear and fear learning. Psychol. Rev. 108, 483–522. doi:10.1037/0033-295X.108.3.483
Pelphrey, K. A., Sasson, N. J., Reznick, J. S., Paul, G., Goldman, B. D., and Piven, J. (2002). Visual scanning of faces in autism. J. Autism Dev. Disord. 32, 249–261. doi:10.1023/A:1016374617369
Pessoa, L. (2010). Emotion and cognition and the amygdala: from “what is it?” to “what’s to be done?”. Neuropsychologia 48, 3416–3429. doi:10.1016/j.neuropsychologia.2010.06.038
Pinkham, A. E., Griffin, M., Baron, R., Sasson, N. J., and Gur, R. C. (2010). The face in the crowd effect: anger superiority when using real faces and multiple identities. Emotion 10, 141–146. doi:10.1037/a0017387
Rapee, R. M. (2002). The development and modification of temperamental risk for anxiety disorders: prevention of a lifetime of anxiety? Biol. Psychiatry 52, 947–957. doi:10.1016/S0006-3223(02)01572-X
Risch, N., Herrell, R., Lehner, T., Liang, K. Y., Eaves, L., Hoh, J., et al. (2009). Interaction between the serotonin transporter gene (5-HTTLPR), stressful life events, and risk of depression: a meta-analysis. JAMA 301, 2462–2471. doi:10.1001/jama.2009.878
Rohner, J. C. (2002). The time-course of visual threat processing: high trait anxious individuals eventually avert their gaze from angry faces. Cogn. Emot. 16, 837–844. doi:10.1080/02699930143000572
Salemink, E., van den Hout, M. A., and Kindt, M. (2007). Selective attention and threat: quick orienting versus slow disengagement and two versions of the dot probe task. Behav. Res. Ther. 45, 607–615. doi:10.1016/j.brat.2006.04.004
Scharinger, C., Rabl, U., Sitte, H. H., and Pezawas, L. (2010). Imaging genetics of mood disorders. Neuroimage 53, 810–821. doi:10.1016/j.neuroimage.2010.02.019
Schofield, C. A., Johnson, A. L., Inhoff, A. W., and Coles, M. E. (2012). Social anxiety and difficulty disengaging threat: evidence from eye-tracking. Cogn. Emot. 26, 300–311. doi:10.1080/02699931.2011.602050
Schofield, P. R., Williams, L. M., Paul, R. H., Gatt, J. M., Brown, K., Luty, A., et al. (2009). Disturbances in selective information processing associated with the BDNF Val66Met polymorphism: evidence from cognition, the P300 and fronto-hippocampal systems. Biol. Psychol. 80, 176–188. doi:10.1016/j.biopsycho.2008.09.001
Shepherd, S. V. (2010). Following gaze: gaze-following behavior as a window into social cognition. Front. Integr. Neurosci. 4:5. doi:10.3389/fnint.2010.00005
Stanger, C., Ryan, S. R., Hongyun, F., and Budney, A. J. (2011). Parent training plus contingency management for substance abusing families: a complier average causal effects (CACE) analysis. Drug Alcohol Depend. 118, 119–126. doi:10.1016/j.drugalcdep.2011.03.007
Susa, G., Pitică, I., Benga, O., and Miclea, M. (2012). The self regulatory effect of attentional control in modulating the relationship between attentional biases toward threat and anxiety symptoms in children. Cogn. Emot. 26, 1069–1083. doi:10.1080/02699931.2011.638910
Thomason, M. E., Henry, M. L., Paul, H. J., Joormann, J., Pine, D. S., Ernst, M., et al. (2010). Neural and behavioural responses to threatening emotion faces in children as a function of the short allele of the serotonin transporter gene. Biol. Psychol. 85, 38–44. doi:10.1016/j.biopsycho.2010.04.009
Tottenham, N., Tanaka, J. W., Leon, A. C., McCarry, T., Nurse, M., Hare, T. A., et al. (2009). The NimStim set of facial expressions: judgments from untrained research participants. Psychiatry Res. 168, 242–249. doi:10.1016/j.psychres.2008.05.006
Wichers, M., Kenis, G., Jacobs, N., Mengelers, R., Derom, C., Vlietinck, R., et al. (2008). The BDNF Val66Met x 5-HTTLR x child adversity interaction and depressive symptoms: an attempt at replication. Am. J. Med. Genet. B Neuropsychiatr. Genet. 141, 120–123. doi:10.1002/ajmg.b.30576
Xie, P., Kranzler, H. R., Farrer, L., and Gelernter, J. (2012). Serotonin transporter 5-HTTLPR genotype moderates the effects of childhood adversity on posttraumatic stress disorder risk: a replication study. Am. J. Med. Genet. B Neuropsychiatr. Genet. 159, 644–652. doi:10.1002/ajmg.b.32068
Keywords: BDNF Val66Met, 5-HTTLPR, eye movement, emotional face, facial features, affective neuroscience, early childhood
Citation: Christou AI, Wallis Y, Bair H, Crawford H, Frisson S, Zeegers MP and McCleery JP (2015) BDNF Val66Met and 5-HTTLPR genotype are each associated with visual scanning patterns of faces in young children. Front. Behav. Neurosci. 9:175. doi: 10.3389/fnbeh.2015.00175
Received: 27 March 2015; Accepted: 22 June 2015;
Published: 13 July 2015
Edited by:
Francesca Cirulli, Istituto Superiore di Sanità, ItalyReviewed by:
Alessandra Berry, Istituto Superiore di Sanità, ItalyDavid Meary, University Pierre Mendès France, France
Copyright: © 2015 Christou, Wallis, Bair, Crawford, Frisson, Zeegers and McCleery. This is an open-access article distributed under the terms of the Creative Commons Attribution License (CC BY). The use, distribution or reproduction in other forums is permitted, provided the original author(s) or licensor are credited and that the original publication in this journal is cited, in accordance with accepted academic practice. No use, distribution or reproduction is permitted which does not comply with these terms.
*Correspondence: Antonios I. Christou, Behavioural Brain Sciences Centre, School of Psychology, University of Birmingham, Edgbaston, Birmingham B15 2TT, UK, a.christou87@gmail.com;
Joseph P. McCleery, Center for Autism Research, Children’s Hospital of Philadelphia, 3535 Market Street, #860, Philadelphia, PA 19104, USA, joe.mccleery@gmail.com