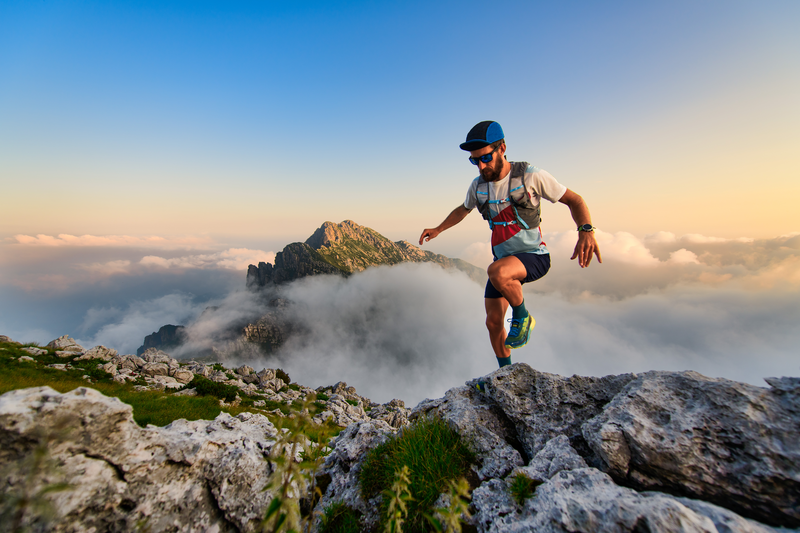
94% of researchers rate our articles as excellent or good
Learn more about the work of our research integrity team to safeguard the quality of each article we publish.
Find out more
ORIGINAL RESEARCH article
Front. Behav. Neurosci. , 12 March 2015
Sec. Individual and Social Behaviors
Volume 9 - 2015 | https://doi.org/10.3389/fnbeh.2015.00066
Environmental enrichment (EE) is a widely used paradigm for investigating the influence of complex stimulations on brain and behavior. Here we examined whether pre-reproductive exposure to EE of female rats may influence their maternal care and offspring cognitive performances. To this aim, from weaning to breeding age enriched females (EF) were reared in enriched environments. Females reared in standard conditions were used as controls. At 2.5 months of age all females were mated and reared in standard conditions with their offspring. Maternal care behaviors and nesting activity were assessed in lactating dams. Their male pups were also behaviorally evaluated at different post-natal days (pnd). Brain BDNF, reelin and adult hippocampal neurogenesis levels were measured as biochemical correlates of neuroplasticity. EF showed more complex maternal care than controls due to their higher levels of licking, crouching and nest building activities. Moreover, their offspring showed higher discriminative (maternal odor preference T-maze, pnd 10) and spatial (Morris Water Maze, pnd 45; Open Field with objects, pnd 55) performances, with no differences in social abilities (Sociability test, pnd 35), in comparison to controls. BDNF levels were increased in EF frontal cortex at pups' weaning and in their offspring hippocampus at pnd 21 and 55. No differences in offspring reelin and adult hippocampal neurogenesis levels were found. In conclusion, our study indicates that pre-reproductive maternal enrichment positively influences female rats' maternal care and cognitive development of their offspring, demonstrating thus a transgenerational transmission of EE benefits linked to enhanced BDNF-induced neuroplasticity.
Environmental enrichment (EE) is a widely used paradigm for investigating the influence of complex sensori-motor, cognitive and social stimulations on brain and behavior displayed during both development and adulthood in different experimental conditions (Rosenzweig et al., 1964; Nithianantharajah and Hannan, 2006). EE exerts beneficial effects on many behavioral (improved motor, cognitive and emotional performances, reduced stress reactivity), morphological (increase in brain weight, dendritic branching, number of spines, synaptic density, and neurogenesis), and molecular (changes in gene expression, modulation of neurotrophic and neurotransmitter systems) brain features (Nithianantharajah and Hannan, 2006, 2009; Petrosini et al., 2009; Baroncelli et al., 2010; Simpson and Kelly, 2011; Sale et al., 2014).
Despite the huge literature on the beneficial effects of EE applied immediately after weaning, in adulthood or even in aging, the transgenerational proactive effects of pre-reproductive EE have been only recently investigated (Arai et al., 2009; Arai and Feig, 2011; Leshem and Schulkin, 2012; Mashoodh et al., 2012; Caporali et al., 2014). The transfer of parents' phenotypic traits to the offspring is a debated process in biology since its promotion by Lamarck (Lamarck, 1809). This epigenetic (non-genetic) phenomenon of imprinting of parental environmental experiences on the offspring genome can lead to a different offspring phenotype that can persist over generations (Weaver, 2007).
The neurobehavioral consequences of the interactions between individual and environment take place as early as conception and continue to be important across the life. During embryonic and fetal development, activation of orchestrated chains of genes is the prime driving force directing central nervous system maturational processes. However, besides the overwhelming impact of the genetic control, even the environmental stimuli strongly influence the highly susceptible developing structures. Namely, the environment experienced by the pregnant mother exerts substantial effects on the intrauterine milieu and alters fetal organogenesis. During pregnancy, the maternal exposure to environmental toxins, pollutants, radiations, drugs, or alcohol, hormonal alterations (e.g., prenatal stress), food deprivation, or micronutrient deficiency have a deleterious impact on physical and behavioral offspring development (Rice and Barone, 2000; Meck and Williams, 2003; Van den Bergh et al., 2005; Weinstock, 2005; Mueller and Bale, 2008; Swanson et al., 2009; Thompson et al., 2009; Charil et al., 2010; Glover, 2011). On the other hand, even positive neurodevelopmental effects may occur, as in the case of the prenatal exposure to complex environments (McKim and Thompson, 1975; Kiyono et al., 1985; Dell and Rose, 1987; Welberg et al., 2006; Leshem and Schulkin, 2012; Mychasiuk et al., 2012; Rosenfeld and Weller, 2012). For example, voluntary exercise during pregnancy evokes positive and long-lasting effects on the offspring behavioral performances by increasing hippocampal neurogenesis and brain-derived neurotrophic factor (BDNF) expression (Parnpiansil et al., 2003; Bick-Sander et al., 2006; Lee et al., 2006; Sale et al., 2007; Herring et al., 2012).
Also after birth the newborn remains very sensitive to the (positive or negative) action of the environment. Given the primary source of environmental stimulation is represented by the mother, pup's post-natal development is mainly sculptured by maternal care (Francis and Meaney, 1999; Meaney, 2001; Macrì and Wurbel, 2006; Champagne and Meaney, 2007; Rosenfeld and Weller, 2012). Notably, maternal care may be in turn altered by the environmental factors experienced by the mothers. For example, stressed mothers display abusive behaviors on the pups that result in persisting changes in BDNF gene expression in the prefrontal cortex of the adult offspring (Roth et al., 2009). Also spontaneous variations in the quality or quantity of maternal care leave long-lasting epigenetic marks on offspring molecular factors crucial for plasticity, such as expression of BDNF, NMDA and glucocorticoid receptors (Weaver et al., 2004; Champagne and Meaney, 2006; Weaver, 2007; Champagne and Curley, 2009). Nevertheless, the relationship of prenatal exposure to EE, maternal care and offspring cognitive development has never been investigated. In fact, the few studies addressing the pre-reproductive and prenatal EE effects on offspring behavior neglect the issue of maternal care (Arai et al., 2009; Mychasiuk et al., 2012). One study addressing the EE exposure effects during pregnancy reports enhanced licking levels, but does not provide information on cognitive development of the offspring (Sale et al., 2004). Another study reports that early pre-reproductive EE transgenerationally influences offspring motor and cognitive behavior, but does not provide information on maternal care (Leshem and Schulkin, 2012; Caporali et al., 2014). Finally, modifications of maternal care are reported in standard reared females mated with enriched male, but no information on offspring cognitive abilities are provided (Mashoodh et al., 2012).
It is well known that neurotrophins, as the BDNF, influence brain development, neuronal plasticity (synapse formation, axonal outgrowth, and circuital remodeling), as well as plastic mechanisms involved in learning and memory, and in the response to stress or injury (Pham et al., 2002; Segal, 2003; Blum and Konnerth, 2005; Branchi et al., 2006; Angelucci et al., 2009; Gelfo et al., 2011). The expression of offspring neurotrophins is modified by early experiences, either negative (i.e., maternal maltreatment) or positive (i.e., environmental enrichment) (Sale et al., 2004; Roth et al., 2009; Caporali et al., 2014). Interestingly, early enrichment affects the BDNF epigenetic structure without altering its expression (Branchi et al., 2011). Moreover, it has been shown that the offspring of mothers that exhibit high levels of pup licking have an increased BDNF expression in the hippocampus (Liu et al., 2000). A recent study by our team (Caporali et al., 2014) has shown that the offspring of enriched mothers display anticipated development of complex motor behaviors associated with increased BDNF and nerve growth factor (NGF) levels in the principal motor areas, striatum and cerebellum.
On such a basis the present study was aimed at analyzing whether and how pre-reproductive exposure to EE of female rats may influence their maternal care as well as cognitive performances of their offspring. To this aim, from weaning to breeding age, the enriched female rats were reared in large groups in a highly stimulating environment with different objects frequently changed. Females used as controls were pair-housed in standard conditions. At 2.5 months of age all females were mated and reared in standard conditions with their offspring, which thus never experienced the environmental enrichment. Maternal care behaviors and nesting activity were assessed in lactating dams. At different post-natal days (pnd), their male pups were behaviorally evaluated for discriminative, social and cognitive abilities. Given adult hippocampal neurogenesis is a form of structural and functional plasticity particularly sensitive to environmental modifications (Masiulis et al., 2011; Kempermann, 2012), at the end of behavioral testing it was measured in the dentate gyrus of adult pups. Furthermore, to gain information on the functional modifications induced by pre-reproductive maternal EE exposure, BDNF expression in frontal cortex, hippocampus, and cerebellum was evaluated in dams at pups' weaning and in the offspring at birth, weaning and adulthood. Finally, due to its integral role in brain development and adult neurogenesis (Masiulis et al., 2011), hippocampal and cortical levels of the structural protein reelin were also measured in the pups at birth and in adulthood.
At weaning (pnd 21) 20 female Wistar rats (54.73 ± 1.99 g) were randomly assigned to enriched or standard rearing conditions.
From pnd 21 to 72 Enriched Females (EF) were housed in group of 10 in a large cage (100 × 50 × 80 cm) with an extra level constructed of galvanized wire mesh and connected by ramps to create two interconnected levels, according to the enrichment conditions previously described (Petrosini et al., 2009; Cutuli et al., 2011; Foti et al., 2011; Caporali et al., 2014). The cage contained wood sawdust, two running wheels, a shelter (a house-shaped toy with a concave opening in which the rat could enter), colored plastic toys (red or green small balls, little bells, jingle noise-maker playthings, and ropes), and small objects (transparent rat igloo, colored bricks, cubes, tunnels, a mirror, and platform). Throughout the enrichment period, the shelter and running wheels were kept in the cage and the toys and constructions were changed twice a week. Once a week, the feeding boxes and water bottles were moved to different cage areas to promote explorative behaviors. Furthermore, each enriched animal was handled daily for at least 10 min.
Standard Females (SF) were pair-housed in cages (40 × 26 × 18 cm) containing wood sawdust, a red plastic tube and no toys. Food and water were delivered ad libitum through dispensers kept always in the same position. SF received the usual care by the animal house staff without any extra-manipulations. This procedure avoided an impoverished rearing and allowed being accustomed to the human contact.
A 12/12 h dark/light cycle (light on between 07:00 and 19:00 h) was applied to both EF and SF groups. On the pnd 72, all females were weighted, and EF were pair-housed in standard cages. After a week, each EF and SF female in oestrus stage was caged for 5 days with a standard-reared male rat (≈300 g) to allow mating. Afterwards, the males were removed, and the females were maintained in the standard home cages throughout pregnancy, delivery and until offspring's weaning.
All efforts were made to minimize animal suffering and reduce the number of animals that were used, per the European Directive (2010/63/EU). All procedures were approved by the Italian Ministry of Health.
At birth (pnd 0), litter size and sex ratio were noted. Then, culling of the litter was quickly performed (all procedures taking max 5 min) reducing the litters to six males and two females. Litters not compliant with this condition were excluded from biochemical and behavioral analyses. Thus, litters from six EF and SF dams were used. Depending on the maternal rearing conditions, two groups of male pups were obtained: one group encompassed the pups of EF (group name: EF-p; n = 24); the other group encompassed the pups of SF (group name: SF-p; n = 24). Note that the difference in rearing conditions concerned the mothers in their pre-reproductive phase and not the pups that were all reared in standard conditions.
Two pups/litter (n = 12/group) were behaviorally evaluated for discriminative (maternal odor preference T-maze test on pnd 10), social (Sociability test on pnd 35) and cognitive abilities (Morris Water Maze, MWM, on pnd 45, and Open Field with objects, OF, on pnd 55). A sub-group of pups randomly selected among the behaviorally tested ones was used to perform BrdU analyses at the end of behavioral procedures (pnd 55; n = 6/group).
Other two pups/litter (n = 12/group) not previously behaviorally tested were submitted to neurochemical analyses to measure levels of BDNF (at three time-points: pnd 0, 21, 55; n = 4/group/time-point) and reelin (at two time-points: pnd 0, 55; n = 4/group/time-point).
BDNF determination was also performed on dams (n = 4/group) at post-partum day (ppd) 21.
Pup weight was collected at pnd 10, 35, and 55. Siblings of the behaviorally or neurochemically analyzed pups were devoted to different researches. Global timing of experimental procedures is reported in Figure 1.
Figure 1. Timeline of the experimental procedure. Experimental groups of female rats according to different pre-reproductive rearing conditions (EF, Enriched Females; SF, Standard Females. Duration: ≈7 weeks). Experimental groups of male pups (EF-p, Enriched Females' pups; SF-p, Standard Females' pups), behavioral testing (maternal odor preference; Sociability test; Morris Water Maze, MWM; Open Field with objects, OF) and biochemical analyses (■, neurotrophin determination; Δ, reelin quantification; •, hippocampal neurogenesis analyses).
To test the nest-building ability and proneness, each day from the day of delivery (ppd 0) till ppd 13 dams were given the opportunity to build a nest providing them 6 g of sterile surgical cotton placed on the lid of each cage, as described in previous studies (Schneider and Lynch, 1984; Laviola et al., 1990; Petruzzi et al., 1995; Venerosi et al., 2008). Quantitative index of nest building activity was the weight of the residual cotton. To analyze nest building propensity, the built nests were removed on ppd 1, 2, 4, 6, 9, 12. After nest removal new cotton was provided and latency to manipulate it was assessed. Nest building started when the female pulled down the cotton from the cage lid with forelimbs and mouth. The cut-off time was 10 min.
The nest quality was assessed daily by two independent observers blind to the pre-reproductive rearing condition of the dams (inter-rate reliability >0.9), using the following 4-point scale, modified from Kalueff et al. (2006) and Venerosi et al. (2008) (Supplementary Figure 1):
(1) No nest (the dam did not build the nest or scattered the cotton and sawdust throughout the home cage with no clear nest shape);
(2) Primitive flat nest (the dam used the cotton to build a plane nest);
(3) Complex cup-shaped nest (the dam used the cotton to build an open nest with walls);
(4) Complex hooded nest (the dam built a round and well-shaped nest with walls forming a ceiling). We recorded further qualitative nest features, as position (under or opposite the water bottle), height (lower, at the same level or higher than the middle height of the cage), texture (low, intermediate, high work out of the nest surface), and presence of additional structures (little agglomerates of cotton, sawdust or excrements put on the top of the cage).
Maternal behavior in rats consists of several behaviors toward the litter that ensure the pups' survival and promote offspring development. To obtain information about the effects of pre-reproductive environmental enrichment exposure of female rats on their maternal care at early stages, mother-pups interactions were recorded on ppd 1 (modified from Calamandrei and Keverne, 1994; Venerosi et al., 2008). All 30 min-observation sessions were made under dim light in a testing room out of the animal facility. The observations were made between 10.00 and 16.00 h and performed by a trained observer blind to pre-reproductive rearing condition of the dam. Animals were habituated to the testing room for 10 min. Thirty minutes before the start of each observation session, the pups were removed (isolation period), the nest eliminated, and the dam remained alone in her home cage. During the isolation period, the pups were weighted and then maintained altogether in a small box at 32° ± 1°C. This procedure allows to study maternal behavior in a condition eliciting it, not in basal level condition.
At the beginning of each observation, the cage lid was gently replaced by a transparent perforated Plexiglas top, the pups were re-placed in their home cage in the side opposite to the previously removed nest, and dam's behavior was video-recorded for 30 min. Latency, frequency and duration of the following behaviors (Fleming and Rosenblatt, 1974; Petruzzi et al., 1995; Venerosi et al., 2008) were collected:
- Pup-directed Behaviors:
- Retrieving: the dam was picking up any pup in her mouth and carrying it to the nest;
- Licking: the dam was licking or grooming any part of the pup's body, primarily the ano-genital region;
- Sniffing: the dam was sniffing one or more pups;
- Nursing: part of the litter was attached to dam's nipples while the dam did not show obvious back-arching;
- Crouching (or arched-back nursing): the dam was domed over all pups with the body arched, hind-limbs splayed and no apparent movement;
- Nest Building: the dam was pushing and pulling the sawdust toward the pups to form a nest.
- Non pup-directed behaviors:
- Digging: the dam was nuzzling in the sawdust out of nest area, pushing and kicking it around using the snout and/or both fore- and hind-paws;
- Grooming: the dam was wiping, licking, combing or scratching any part of its own body;
- Wall Rearing: the dam was rearing on hindlimbs, while leaning (or not) with the forelimbs on the cage walls, often sniffing the air;
- Exploring: the dam was moving around the cage and sniffing the substrate, but not carrying pups or nesting material;
- Resting: the dam was lying down alone, out of the nest.
- Other behaviors: all behaviors different from the ones classified in the previous categories.
Manual scoring was performed by a researcher blind to pre-reproductive rearing condition of the dams by using Ethovision XT (Noldus, The Netherlands). Data analysis was performed on three 10 min-blocks.
To assess early discriminative performances and maternal preference behavior, pups were tested in an odor discrimination test at pnd 10 (eyelids still closed). The apparatus consisted in a black Plexiglas T-shaped maze (start arm: 25 × 9 cm; choice arms: 12.5 × 9 cm; height: 8.5 cm). Each pup was gently placed in the start arm and allowed to freely explore the maze for 5 min. Two glass cups (diameter: 2.5 cm; height: 2 cm) containing sawdust of the home cage with mother's excrements (maternal olfactory stimulus) or clean sawdust (neutral olfactory stimulus) were placed at the end of each choice arms. Time spent in the two arms and time spent in direct contact with the olfactory stimuli were recorded. After each testing session the apparatus was cleaned and the position of the olfactory stimulus was alternated among pups.
To quantitate sociability tendencies in rat adolescent pups we used the Sociability test (Nadler et al., 2004; Cutuli et al., 2013). The apparatus consisted of a rectangular three-chamber wooden box (150 × 40 × 40 cm) with black floor and white lateral walls. The central chamber was 30 cm-long and the two lateral chambers were 60 cm-long. The three chambers were divided by two transparent Plexiglas walls with an opened middle door (height: 10 cm; width: 8 cm), which allowed free access to each lateral chamber. Each lateral chamber contained a small plastic cage (diameter: 18 cm) with mesh-like holes in which a stranger rat was confined during the Sociability test. The experimental procedure consisted of two sessions, Habituation and Sociability test, with an inter-session interval of 3 min (Cutuli et al., 2013). During Habituation each rat was allowed to freely move for 10 min in the entire apparatus with the small cages maintained empty. During Sociability test an unfamiliar conspecific male rat of the same age (about 35–38 pnd) was placed inside one of the two small wire cages in the lateral chambers (randomly selected and counterbalanced for each group). The to-be-tested rat was placed in the central chamber and it was allowed to freely explore for 10 min the entire apparatus and contact the small wire cages. Rat behavior was recorded by a video camera mounted on the ceiling. The resulting video signal was relayed to a monitor and to an image analyzer (Ethovision XT, Noldus, The Netherlands).
The parameters analyzed were: total distance traveled, and time (s) spent in the three compartments; frequency of entries in the lateral compartments. In addition, time spent in a 9 cm-square (target) around the small wire cages (containing the social stimulus or empty) was calculated as direct contact.
To assess spatial and procedural abilities, late adolescent rats were submitted to MWM (Cutuli et al., 2009). The rats were placed in a circular white pool (diameter 140 cm) filled with 24°C water made opaque by the addition of atoxic acrylic color (Giotto, Italy). An escape platform (diameter 10 cm) was submerged 2 cm below the water level in the NE quadrant. Each rat was submitted to a 10-trial Place phase followed by an 1-trial Probe phase with an inter-phase interval of 3 min. During Place trials, the rat was released into the water from randomly varied starting points and allowed to find the hidden platform for a maximum of 60 s with an inter-trial interval of 30 s. When the rat reached the platform, it was allowed to remain there for 30 s. If the rat failed to reach the hidden platform within 60 s, it was gently guided there by the experimenter. During Probe trial, the platform was removed and rat was allowed to swim for 30 s in searching for it. Navigational trajectories were recorded by a video camera whose signal was relayed to a monitor and to the previously described image analyzer.
The following MWM parameters were considered: latencies to find the platform; total and peripheral distances swum in the pool; mean swimming velocity; percentage of time spent in the previous rewarded quadrant (presence of platform) during Probe phase; navigational strategies put into action in reaching the platform. The navigational strategies were classified in four main categories (Cutuli et al., 2009), regardless the platform was reached or not: Circling (C), swimming in a 20-cm peripheral annulus, with inversion of swimming direction and counterclockwise and clockwise turnings in the peripheral sector of the pool; Extended Searching (ES), swimming around the pool in all quadrants, visiting the same areas more than once; Restricted Searching (RS), swimming not more than two pool quadrants, not visiting some tank areas at all; direct Finding (F), swimming toward the platform without any foraging around the pool. Two researchers who were unaware of the individual specimen's group categorized the swimming trajectories drawn by the image analyzer. They attributed the dominant behavior in each trial to a specific category. Categorization was considered reliable only when their judgments were consistent.
To assess spatial discrimination and novelty recognition abilities, young adult rats were submitted to the OF test (Cutuli et al., 2009, 2013). The apparatus consisted of a circular arena (diameter 140 cm) delimited by a 30-cm-high wall. During session 1 (S1), each rat was allowed to freely move in the empty open field and its baseline activity level was measured. During the habituation phase (S2–S4), four objects were placed in a square arrangement in the middle annulus of the arena and a fifth one was placed in the central area. The five objects were: (1) a metal bar with a conical base; (2) a plunger; (3) a long steel rod; (4) a yellow rubber plug; and (5) a black cylinder with a plastic cup turned upside down on top of it. During the spatial change (S5 and S6), the spatial configuration was changed by moving objects 2 and 5 so that the initial square arrangement was modified to a polygon-shaped configuration, without any central object. During the novelty session (S7), the configuration was modified by substituting object 4 with a green plastic object shaped like a half moon. All sessions lasted 6 min with inter-session intervals of 3 min (Cutuli et al., 2009, 2013). Rats' behavior was recorded by a video camera whose signal was relayed to a monitor and to the previously described image analyzer.
In S1 the following motor and emotional parameters were analyzed: total distance (in cm) traveled in the arena; peripheral distance, percentage of the total distance traveled in a 20-cm peripheral annulus; number of rearings; number of central crossings; motionless time; number of defecation boluses. In S2–S4, the total contact time spent in contacting objects was analyzed. Contact was considered to have taken place when the rat's snout actually touched an object or when it sniffed the object for at least 1 s, but not when the rat leaned against, stood, or sat on the object. In S5–S7, the time spent contacting objects was expressed as discrimination index (d index), that is time exploring displaced (or novel) objects/total exploration time.
To correlate hippocampus-dependent behavioral performances (namely, in MWM and OF) with neuroanatomical changes in the hippocampal neurogenenic niche, the subgranular zone of the dentate gyrus, the hippocampal adult neurogenesis was examined. Twelve behaviorally tested animals (3 rats/litter/group) received three i.p. injections of 50 mg/kg BrdU dissolved in saline (0.9% NaCl adjusted to pH 7.2 with NaOH) during 1 day (inter-injection interval: 2 h). One day after the final injection the animals were sacrificed and transcardially perfused with 4% paraformaldehyde (PFA) in 0.1 M phosphate buffer (PBS), under deep anesthesia with chloral hydrate. The brains were removed and kept overnight in 4% PFA. Afterwards, brains were equilibrated in 30% sucrose and cryopreserved at −80°C.
The hippocampus from brains embedded in Tissue-Tek OCT (Sakura, USA) was cut by cryostat at −25°C in 40 μm coronal serial free-floating sections. To perform BrdU detection, DNA was denaturated with 2N HCl for 40 min at 37°C to facilitate antibody access, followed by 0.1 M borate buffer pH 8.5 for 20 min. Sections were incubated overnight at 4°C with a primary antibody rat anti-BrdU (AbD Serotec Cat# MCA2060 RRID:AB_323427) diluted 1:300 in TBS containing 0.1% Triton, 0.1% Tween 20 and 3% normal donkey serum (blocking solution). For immunofluorescence analysis, sections were stained for multiple labeling by using fluorescent methods. After permeabilization with 0.3% Triton X-100 in PBS, the sections were incubated with 3% normal donkey serum in PBS for 16–18 h with the following primary antibodies: 1:200 goat polyclonal antibodies against Doublecortin (DCX) (Santa Cruz Biotechnology, Inc. Cat# sc-8066). Secondary antibodies used to visualize the antigen were 1:200 donkey anti-rat Cy3-conjugated (Jackson ImmunoResearch; BrdU) 1:100, donkey anti-goat Cy2-conjugated (Jackson ImmunoResearch; Dcx). Images of the immunostained sections were obtained by laser scanning confocal microscopy by using a TCS SP5 microscope (Leica Microsystem; Germany).
Quantitative analysis of hippocampal cell populations was performed by means of design-based (assumption-free, unbiased) stereology as previously described (Cutuli et al., 2014; Farioli-Vecchioli et al., 2014).
For BDNF and reelin determination, 4 animals were selected from each group of dams (EF and SF, at ppd 21) and not behaviorally tested pups (EF-p and SF-p, at pnd 0, 21, 55; 2 pups/dam). The animals were decapitated; the brains were quickly removed and dissected on ice by using a binocular dissection microscope. The following brain regions were collected according to method Glowinski and Iversen's, 1966: cortex and hippocampus (pups at pnd 0); frontal cortex, hippocampus and cerebellum (dams; pups at pnd 21, 55). Cerebellar neurotrophin expression was not analyzed at pnd 0, because of the very weak BDNF expression in this extremely immature area in the very early postnatal days, as previously described (Katoh-Semba et al., 1997; Sherrard and Bower, 2002). Brain regions were extracted in 1 ml extraction buffer/100 mg tissue. Brain tissue samples were homogenized in an ice-cold lysis buffer containing 137 mM NaCl, 20 mM Tris–HCl (pH 8.0), 1% NP40, 10% glycerol, 1 mM phenylmethanesulfonylfluoride (PMSF) 10 mg/ml aprotinin, 1 mg/ml leupetin, and 0.5 mM sodium vanadate. The tissue homogenate solutions were centrifuged at 14000 g for 25 min at 4°C. The supernatants were collected and stored at −80°C until analyses.
As an index of the functional modifications induced by pre-reproductive maternal EE exposure, we evaluated in several brain regions the expression of BDNF, a neurotrophin specifically involved in synaptic plasticity changes supporting mnesic functions. BDNF concentrations were assessed using a two-site enzyme immunoassay kit (Promega, Madison, WI, USA). In brief, 96-well immunoplates (NUNC) were coated with 50 μl/well with the corresponding capture antibody which binds the neurotrophin, and stored overnight at 4°C. The next day serial dilutions of known amounts of BDNF ranging from 0 to 500 pg/ml were performed in duplicate to generate a standard curve. Then, the plates were washed three times with wash buffer and the standard curves and supernatants of brain tissue homogenates were incubated in the coated wells (100 μl each) for 2 h at room temperature (RT) with shaking. After additional washes, the antigen was incubated with second specific antibody for 2 h at RT, as specified in the protocol. The plates were washed again with wash buffer and then incubated with an anti-IgY HRP for 1 h at RT. After another wash, the plates were incubated with a TMB/Peroxidase substrate solution for 15 min and phosphoric acid 1M (100 μl/well) was added to the wells. The colorimetric reaction product was measured at 450 nm using a microplate reader (Dynatech MR 5000, Germany). BDNF concentrations were determined from the regression line for the BDNF standard (ranging from 7.8 to 500 pg/ml-purified mouse BDNF) incubated under similar conditions in each assay. Cross-reactivity with other related neurotrophic factors, for example, NT-3 and NT-4 was less than 3%. BDNF concentration was expressed as pg/g wet weight and all assays were performed in triplicate.
As further correlate of neuroplasticity reelin levels were assessed in both experimental pups groups. Protein content was quantified by Bradford's colorimetric assay (Bio-Rad, Milan, Italy). Each protein sample was separated by SDS-polyacrylamide gel electrophoresis and transferred to a nitrocellulose membrane. Membranes were saturated with 5% dried non-fat milk and incubated overnight with specific primary antibodies, such as mouse anti-Reelin (1:1000; Millipore, USA) and mouse anti-β-actin (1:5000; Sigma) (Biamonte et al., 2014). Membranes were then incubated with the appropriate horseradish peroxidase-conjugated secondary antibodies. Immunoreactive bands were detected by using an enhanced chemiluminescence kit (ECL; Amersham Biosciences).
Statistical analyses were performed by using STATISTICA 8.0 (StatSoft). Data were firstly tested for normality (Wilk-Shapiro's test) and homoscedasticity (Levene's test). Then, behavioral data were analyzed by one-way and Two-Way ANOVAs for independent (group) and repeated measures (compartment, target, trial, strategy, session, object). Data on BDNF levels were analyzed by One-Way ANOVAs followed by Tukey's HSD test, separately for dams and pups. Reelin levels were analyzed using Student's T-test. When parametric assumptions were not fully met, data transformations (angular transformation for percentages) or non-parametric analyses of variance (Mann-Whitney's U, Friedman's test, Wilcoxon's test) were used. Differences were considered significant at the p = 0.05 level.
At end of the EE exposure (pnd 72), EF (212.30 ± 6.90 g) weighted significantly less than SF (231.52 ± 3.23 g) [One-Way ANOVA: F(1, 18) = 7.16, p = 0.01]. Pre-reproductive maternal enrichment did not affect litter size [EF: 11.0 ± 0.5 pups; SF: 11.6 ± 0.6 pups; One-Way ANOVA: F(1, 18) = 0.54, p = 0.47] and male/female ratio [percentage of male pups: EF: 60.70 ± 2.17%; SF: 59.77 ± 4.12%; One-Way ANOVA: F(1, 18) = 0.04, p = 0.84]. At pnd 1 EF male (6.64 ± 0.07 g) and female (6.61 ± 0.05 g) pups' weight was lower than SF male (7.25 ± 0.08 g) and female (7.05 ± 0.09 g) pups' one [One-Way ANOVA: male pups, F(1, 70) = 12.02, p = 0.0009; female pups, F(1, 22) = 7.59, p = 0.01]. No differences were found in the weight of male pups that underwent the behavioral testing during the successive time-points [pnd 10: EF-p: 22.80 ± 0.74 g; SF-p: 23.80 ± 1.08 g; One-Way ANOVA: F(1, 22) = 0.64, p = 0.43; pnd 35: EF-p: 134.72 ± 2.19 g; SF-p: 130.36 ± 3.12 g; One-Way ANOVA: F(1, 22) = 1.30, p = 0.27; pnd 55: EF-p: 205.61 ± 4.92 g; SF-p: 210.89 ± 3.45 g; One-Way ANOVA: F(1, 22) = 0.77, p = 0.39].
Non parametric analyses (Mann-Whitney's U) performed on cotton weight used by dams in their nest building activity (Supplementary Table 1) as well as on the latency to manipulate cotton (Supplementary Table 1) revealed that the EF and SF did not significantly differ (Figures 2A,B). Friedman's test performed on cotton weight used during the six days of observation revealed a similar inverted U-shaped trend in both groups (EF, p = 0.04; SF, p = 0.02). Namely, both EF and SF groups used more cotton during the intermediate days of observation (ppd 2–9) than in the first (ppd 1) and last (ppd 12) days. Friedman's test performed on latency to manipulate cotton revealed a significant trend to increase the latencies as observation days went only in SF (p = 0.004), while EF showed latencies not significantly different among days (p = 0.78).
Figure 2. Nest building activity. Results of pre-reproductive maternal rearing condition on nest building activity are depicted. Quantitative (cotton weight, A; latency, B) and qualitative indexes (nest quality, C; position, D; position change, E; height, F; texture, G; additional structures, H) of nest building activity analyzed in EF and SF from the day of delivery (ppd 0) to ppd 13 (*p < 0.05; **p < 0.01). Results are reported as mean ± SEM.
As for nest quality score, EF obtained higher scores than SF in the final observation days (Supplementary Table 1) because still built complex cup-shaped nests on ppd 11 (p = 0.03) and 12 (p = 0.007), instead of primitive flat nest, as SF did (Figure 2C). Friedman's test revealed that in both EF and SF groups nest quality scores increased from ppd 1 onwards and decreased during the last observation days (EF, p = 0.0008; SF, p = 0.002).
No effect of pre-reproductive rearing condition was evident on the further qualitative nest features (Supplementary Table 1), as position (Figures 2D,E), height (Figure 2F), texture (Figure 2G), additional structures (Figure 2H).
In summary, in the 2nd post-natal week EF dams appeared to be longer engaged in building nests of higher shape complexity than SF.
Non parametric analyses (Mann-Whitney's U) performed on the sum of pup-directed behaviors at ppd 1 revealed significant differences between EF and SF groups in the final phase (3rd 10 min-block) of the 30 min-observations, being EF longer engaged in pup-directed behaviors than SF (Figure 3; Supplementary Table 2A). Detailed analyses performed on single pup-directed behaviors demonstrated that during just the 3rd block of observation the EF showed higher Crouching duration (Figure 4A) and Licking frequency (Figure 4B), and lower Nursing duration and frequency than SF (Figure 4C; Supplementary Table 2A). Also during the 1st block of observation EF showed higher duration and frequency of Nest Building (Figure 4D; Supplementary Table 2A). Symmetrically, EF emitted less non pup-directed behaviors than SF during the 3rd block of observation (Supplementary Table 2B). Namely, EF engaged shorter and less frequent Grooming (Figure 4E) and Exploring (Figure 4F; Supplementary Table 2B). No differences were observed in the remaining behaviors (Supplementary Tables 2A,B).
Figure 3. Maternal behavior observation. Results of pre-reproductive maternal rearing condition on maternal behavior are depicted. Sum of duration of the different kind of maternal behaviors (pup-directed, non pup-directed and other behaviors) in each of the three 10 min-blocks of observation. (*p < 0.05). Results are reported as mean ± SEM.
Figure 4. Maternal behaviors. Results of pre-reproductive maternal rearing condition on specific maternal behaviors are depicted. Line graphs represent duration and frequency of single pup-directed (A–D) and non pup-directed (E–F) maternal behaviors analyzed in EF and SF on ppd 1 (*p < 0.05; **p < 0.01). Results are reported as mean ± SEM.
In summary, the day after delivery in response to a brief separation the EF dams were engaged in behaviors directed to pup care longer than SF. Interestingly, the main differences in maternal behavior between EF and SF dams were found in those behaviors that indicate a more and functional complex maternal care style, as Crouching, Licking and Nest Building.
At pnd 10, pups were submitted to a discriminative test of maternal odor. Although all pups spent more time in the “maternal” arm (EF-p: Wilcoxon's test, Z = 2.80, p = 0.005; SF-p: Wilcoxon's test, Z = 2.71, p = 0.007) and in direct contact with the maternal stimulus (EF-p: Wilcoxon's test, Z = 2.80, p = 0.005; SF-p: Wilcoxon's test, Z = 2.59, p = 0.009), EF-p contacted the maternal stimulus longer then SF-p did (Mann-Whitney's U, Z = 2.11, p = 0.03) (Figures 5A,B).
Figure 5. Maternal odor preference T-maze test. Results of pre-reproductive maternal rearing condition on pup's discriminative performances are depicted. Histograms show time spent in maternal and neutral arm (A), and time spent in direct contact with the maternal and neutral olfactory stimulus (B) analyzed in EF-p and SF-p on pnd 10 (*p < 0.05; **p < 0.01). Results are reported as mean ± SEM.
Overall, the test demonstrated the stronger attractiveness of maternal odor for EF-p than for SF-p in the early developmental phases.
During adolescence (pnd 35), both EF-p and SF-p displayed the expected preference for the social stimulus, as revealed by Two-Way ANOVAs (group × compartment) on total distance [group: F(1, 22) = 0.03, p = 0.87; compartment: F(2, 44) = 22.22, p < 0.000001; interaction: F(2, 44) = 0.21, p = 0.82] and time spent in the compartment with the social stimulus [group: F(1, 22) = 1.51, p = 0.23; compartment: F(2, 44) = 18.92, p = 0.00001; interaction: F(2, 44) = 0.21, p = 0.81] (Figure 6A). Similarly, when the direct contact was analyzed, all animals spent more time in the target of the social stimulus [group: F(1, 22) = 0.07, p = 0.79; target: F(1, 22) = 6.84, p = 0.01; interaction: F(1, 22) = 0.01, p = 0.95] rather than the empty one (Figure 6B). No differences between groups were evident also in frequency of entries in the lateral compartments [group: F(1, 22) = 2.42, p = 0.13; compartment: F(1, 22) = 1.98, p = 0.17; interaction: F(1, 22) = 0.19, p = 0.66].
Figure 6. Sociability test. Results of pre-reproductive maternal rearing condition on pup's sociability are depicted. Histograms show time spent in the three compartment (A), and in the stranger and empty targets (B) analyzed in EF-p and SF-p on pnd 35 (*p < 0.05; **p = 0.01; ***p < 0.001). Results are reported as mean ± SEM.
In summary, all adolescent pups developed similar sociability performances.
The EF-p employed less time and traveled shorter distances to reach the hidden platform in comparison to the SF-p, as revealed by Two-Way ANOVAs (group × trial) on latency [group: F(1, 22) = 7.03, p = 0.01; trial: F(9, 198) = 2.63, p = 0.007; interaction: F(9, 198) = 0.59, p = 0.81] and total distance [group: F(1, 22) = 13.16, p = 0.001; trial: F(9, 198) = 1.53, p = 0.14; interaction: F(9, 198) = 0.60, p = 0.79] (Figures 7A,B). Furthermore, Two-Way ANOVAs (group × trial) on peripheral distance [group: F(1, 22) = 6.18, p = 0.02; trial: F(9, 198) = 6.13, p < 0.000001; interaction: F(9, 198) = 1.68, p = 0.10] and mean velocity [group: F(1, 22) = 13.02, p = 0.002; trial: F(9, 198) = 5.95, p < 0.000001; interaction: F(9, 198) = 1.62, p = 0.11] indicated that EF-p swam less in peripheral sectors and more slowly than SF-p (Figures 7C,D). Significant differences were found in the navigational strategies put into action [group: F(1, 22) = 1.69, p = 0.21; strategy: F(3, 66) = 1.88, p = 0.14; interaction: F(3, 66) = 7.12, p = 0.0003], with EF-p showing higher percentages of Finding (p = 0.03) and lower percentages of Circling (p = 0.05) than SF-p (Figure 7E). Finally, Two-Way ANOVA on time spent in searching the platform during the Probe phase failed to show any significant difference between groups [group: F(1, 22) = 0.27, p = 0.61; quadrant: F(3, 66) = 3.72, p = 0.02; interaction: F(3, 66) = 0.23, p = 0.88] (Figure 7F).
Figure 7. Morris Water Maze test. Results of pre-reproductive maternal rearing condition on pup's spatial and procedural performances are depicted. Latency (A), total and peripheral distances (B,C), velocity (D), and navigational strategies put into action (E) throughout MWM test. Percentage of time spent in the four quadrants during Probe phase (F) analyzed in EF-p and SF-p on pnd 45 (*p = 0.05; **p = 0.01; ***p < 0.001). Results are reported as mean ± SEM. C, Circling; ES, Extended Searching; RS, Restricted Searching; F, Finding; NE, North-East (previously rewarded) quadrant; NW, North-West quadrant; SE, South-East quadrant; SW, South-West quadrant.
In summary, at pnd 45 EF-p displayed better spatial learning performances than SF-p in the MWM.
In S1, no significant difference between groups was evident on motor parameters [total distance: F(1, 22) = 1.47, p = 0.24; number of rearing: F(1, 22) = 0.13, p = 0.72]. All animals exhibited comparable levels of anxiety, as indicated by the absence of significant differences between groups on emotional parameters [peripheral distance: F(1, 22) = 1.83, p = 0.19; number of central crossing: F(1, 22) = 0.07, p = 0.80; motionless time: F(1, 22) = 0.01, p = 0.91; number of defecation boluses: F(1, 22) = 2.63, p = 0.12].
In S2–S4, all animals showed habituation and progressively decreased object contact time, as revealed by a Two-Way ANOVA (group × session) [group: F(1, 22) = 2.76, p = 0.11; session: F(2, 44) = 45.29, p < 0.000001; interaction: F(2, 44) = 2.51, p = 0.09]. In S5 (spatial change), EF-p showed a value of discrimination index significantly greater than SF-p [One-Way ANOVA: F(1, 22) = 4.53, p = 0.04], indicating their marked preference to explore the displaced objects (Figure 8A). In S6, no difference between groups was observed [One-Way ANOVA: F(1, 22) = 0.29, p = 0.60]. When a new object was inserted into the arena (S7), all rats recognized the novelty and preferred to explore the new object more than the familiar ones [One-Way ANOVA: F(1, 22) = 0.94, p = 0.34] (Figure 8B).
Figure 8. Open Field with Objects. Results of pre-reproductive maternal rearing condition on pup's spatial and novelty recognition performances are depicted. Histograms represent discrimination index of spatial change (A) and novelty (B) analyzed in EF-p and SF-p on pnd 55 (*p < 0.05). Results are reported as mean ± SEM.
In summary, at adulthood EF-p showed a more pronounced recognition of spatial rearrangement in comparison to SF-p.
In order to evaluate the effect of pre-reproductive exposure to EE of female rats on the adult neurogenesis of their offspring, the proliferation and differentiation parameters in newly generated neurons of the dentate gyrus were analyzed at pnd 55. No significant differences in the absolute number of proliferating BrdU+ newborn neurons were found between EF-p and SF-p groups (T = 0.32, p = 0.75) (Supplementary Figure 2A). Moreover, no differences were found in the total number of early differentiating progenitors DCX+ (T = −0.49, p = 0.63), of early differentiating newborn neurons BrdU+DCX+ (T = 0.79, p = 0.43) (Supplementary Figures 2B–C).
In frontal cortex EF had significantly higher BDNF levels than SF, as revealed by one-ANOVA [F(1, 6) = 8.59, p = 0.03], while no significant differences in the BDNF levels were found in hippocampus [F(1, 6) = 0.34, p = 0.58] and cerebellum [F(1, 6) = 0.36, p = 0.57] between groups (Figure 9A).
Figure 9. BDNF protein levels. Results of pre-reproductive maternal rearing condition on BDNF expression are depicted. Histograms show: BDNF expression levels in frontal cortex, hippocampus and cerebellum of EF and SF at ppd 21 (A); BDNF expression levels in cortex and hippocampus of EF-p and SF-p at birth (pnd 0, B1); BDNF expression levels in frontal cortex, hippocampus and cerebellum of EF-p and SF-p at weaning (pnd 21, B2) and early adulthood (pnd 55, B3) (*p = 0.05). Results are reported as mean ± SEM. FC, Frontal Cortex; Hp, Hippocampus; Cb, Cerebellum; C, Cortex.
At pnd 0, no significant differences between SF-p and EF-p were found in cortical [F(1, 6) = 0.71, p = 0.43] and hippocampal [F(1, 6) = 1.21, p = 0.31] BDNF levels (Figure 9B1).
At pnd 21, EF-p had BDNF levels significantly higher than SF-p in hippocampus [F(1, 6) = 6.92, p = 0.04]. No significant differences in the BDNF levels were found in frontal cortex [F(1, 6) = 0.20, p = 0.67] and cerebellum [F(1, 6) = 1.18, p = 0.32] between groups (Figure 9B2).
Similarly, at pnd 55 EF-p had BDNF levels significantly higher than SF-p in hippocampus [F(1, 6) = 10.78, p = 0.02], while no significant differences in the BDNF levels were found in frontal cortex [F(1, 6) = 0.16, p = 0.70] and cerebellum [F(1, 6) = 1.89, p = 0.22] between groups (Figure 9B3).
No significant differences in reelin levels were found at pnd 0 (hippocampus: T = 1.48, p = 0.20; cortex: T = 1.40, p = 0.25) as well as at pnd 55 (hippocampus: T = 1.91, p = 0.13; frontal cortex: T = 1.93, p = 0.13) between groups (Supplementary Figures 3A,B).
This study aimed at evaluating whether and how positive pre-reproductive maternal experience influences maternal behavior and offspring phenotype. The pre-reproductive maternal enrichment did influence both maternal care and pups' discriminative and mnesic performances across life. In fact, in comparison to standard females enriched dams showed a more complex maternal care repertoire, as indicated by their higher levels of pup-directed behaviors and nest building activities. The offspring of enriched females showed better discriminative performances in the maternal odor preference T-maze as pups, and higher spatial performances in the MWM at late adolescence and in the OF when adults. Conversely, during adolescence no differences between enriched and standard females' offspring were found in the social abilities.
The superior behavioral performances of enriched females and their offspring were accompanied by profound rearrangement of the neurotrophin pattern. While enriched dams expressed higher BDNF levels in the frontal cortex, their offspring expressed higher BDNF levels in the hippocampus from weaning to adulthood. Conversely, offspring reelin levels and adult neurogenesis were not influenced by the maternal rearing conditions.
In the rat, maternal care influences hippocampal development and thus the functions of spatial learning and memory (Bredy et al., 2004). Namely, the offspring of mothers that exhibit increased levels of pup licking/grooming and arched-back nursing (high LG-ABN mothers) show increased hippocampal N-methyl-d-aspartate (NMDA) subunit mRNA expression, enhanced synaptogenesis and improved hippocampal-dependent spatial learning in comparison to animals reared by low LG-ABN mothers (Francis and Meaney, 1999; Bredy et al., 2004). Moreover, cross-fostering studies provide evidence for the direct effect of maternal care on hippocampal development and cognitive and emotional functions of the offspring (Francis et al., 1999; Liu et al., 2000). Interestingly, in the present study at ppd 1 the enriched females showed increased Licking frequencies and Crouching duration. These behaviors were associated to high levels of nest building activities either at ppd 1 and later at ppd 11–12. Thus, pre-reproductive EE exposure seems to have qualitatively shifted maternal care features to more complex patterns, namely increasing early pup-directed behaviors, similarly to the ones more consistently taken into account in the studies by Meaney's group (i.e., LG and ABN; Caldji et al., 1998), and maintaining high pup care proneness also during later stages of lactation (i.e., nest building).
Overall, these differences in the maternal care may have contributed in changing the developmental trajectories of the pups. In fact, enriched females' offspring resulted more competent in the hippocampal-dependent MWM and OF tests. Interestingly, these better offspring performances were accompanied by increased hippocampal BDNF levels, a well-known modulator of neuronal neuroplasticity, especially in the hippocampus, and thus key regulator for synaptic circuits underlying memory functions (Lu et al., 2005).
Furthermore, in the maternal odor preference T-maze enriched females' offspring were more attracted by dam's olfactory stimuli. In this task performances are mainly related to the early and rapid development of an imprinting process ensuring that the infant forms the caregiver attachment necessary for altricial species survival (Moriceau and Sullivan, 2004). In fact, pups' survival is dependent on learning the maternal odor preference/approach. Besides being attractive for the pups, the maternal odor also organizes their social behavior and ensures that they will nipple attach and receive necessary care and warmth. The unique sensory and mnesic network of maternal odor learning by the infant requires the release of copious amounts of norepinephrine by locus coeruleus into the olfactory bulb until pnd 10 (Moriceau and Sullivan, 2004). This process occurs in the absence of the involvement of brain areas that during infancy are still developing and only partially functional and will become important for learning and memory functions in adulthood, such as the amygdala, hippocampus and frontal cortex (Raineki et al., 2010). The strengthening of the maternal odor learning in enriched females' pups may thus be interpreted as a plastic potentiation of the sensory-mnesic circuit involved in neural processes of attachment, even if we cannot exclude that the differences observed between the two groups of pups could be also due to differences in olfactory perception.
Interestingly, the two regions exhibiting neurotrophin levels influenced by pre-reproductive maternal rearing conditions were the frontal cortex (in the dams) and hippocampus (in the pups). Such areas are not by chance highly sensitive to parental experiences (Arai et al., 2009; Roth et al., 2009; Mychasiuk et al., 2012) and environmental enrichment (Pham et al., 2002; Gelfo et al., 2011; Chourbaji et al., 2012). Frontal cortex plays an active role in modulating maternal care, so that manipulations, such as cocaine treatment or lesions, may significantly impair maternal goal-directed behavior (Slotnick and Nigrosh, 1975; Ferris et al., 2005; Afonso et al., 2007; Febo and Ferris, 2007; Febo et al., 2010). Also human functional MRI studies evidence that mothers responding to infant sensory cues show metabolic activation in anterior cingulate cortex, insula, orbital and ventromedial frontal regions (Lorberbaum et al., 2002; Leibenluft et al., 2004; Nitschke et al., 2004; Ranote et al., 2004; Noriuchi et al., 2008; Strathearn et al., 2008; Swain, 2008). The increased BDNF levels found in the frontal cortex of the enriched dams can be considered an index of increased neuroplasticity mediated by EE, as we previously demonstrated. In fact, enriched male rats exhibit BDNF and NGF up-regulated frontal levels (Gelfo et al., 2011) as well as increased dendritic branching and spinogenesis in the frontal cortex (Gelfo et al., 2009). In the present study, dams' BDNF levels were measured at pups' weaning (≈6 weeks after the end of EE exposure). Thus, if in the enriched females the frontal neurotrophin levels were stably increased, then these levels might represent a sort of “neuroplastic reserve” (Petrosini et al., 2009) to be spent in the later demanding stages of life, as mothering. On the other hand, the mirroring hypothesis is also plausible: the more complex maternal care of enriched dams increased BDNF levels in the frontal cortex. However, we do not exclude that in the enriched dams the enhanced neuroplasticity induced by the previous EE exposure interacts with the subsequent increase in the maternal activity, resulting thus in higher frontal BDNF levels.
In any case, the enhanced neuroplasticity of the enriched mothers went along with the increased neuroplasticity of their pups. In fact, in the offspring the increase in hippocampal neurotrophin expression contributed in developing their better discriminative and mnesic performances. It has been demonstrated that BDNF regulates multiple aspects of hippocampal development and function (Hall et al., 2000). In fact, elevated hippocampal BDNF levels promote neurite outgrowth and synaptic plasticity through regulation of activity-dependent immediate early genes c-fos and early-growth-response-gene 1 and 2 (Egr-1 and Egr-2) (Xu et al., 2000; Zagrebelsky et al., 2005). Furthermore, the induction of long-term potentiation (LTP) increases BDNF transcript levels in the hippocampus (Kovalchuk et al., 2002). Whereas the inhibition of BDNF expression impairs learning and memory functions (Korte et al., 1995; Heldt et al., 2007; Tran et al., 2009), hippocampal BDNF injections improve performances in spatial memory and anxiety tests (Cirulli et al., 2004). Intriguingly, offspring of high LG-ABN dams exhibit enhanced performance in spatial learning and memory tests and increased hippocampal BDNF mRNA levels (Liu et al., 2000). Thus, given the mother represents the primary link between environment and pup, and even subtle variations in maternal care may have a profound impact on offspring development, the pre-reproductive maternal enrichment could have modified the features of the early mothering that probably contributed in turn to build a “neuroplastic reserve” in the pups through the enhanced hippocampal BDNF levels, thus ameliorating their mnesic performances.
While at pnd 0 no difference was found in the cortical and hippocampal BDNF levels, the enriched females' offspring displayed increased hippocampal BDNF levels both at weaning and at adulthood. The lack of neurotrophin modifications at birth does not allow ruling out that eventual latent genetic (germ-line) and epigenetic (intrauterine environment) differences in BDNF signaling required the subsequent interaction with the mother to become manifest in term of BDNF expression. However, once the hippocampal BDNF increase (intrauterine environment, maternal care) due to the pre-reproductive maternal enrichment occurred, it was stable from pnd 21 to pnd 55 and not activity-dependent in relation to behavioral testing. The latter point is particularly important given that in our previous study by Caporali et al. (2014) the accelerated acquisition of complex motor performances exhibited by pre-reproductively enriched dams' pups was associated with increased expression of BDNF and NGF in cerebellum and striatum, with no differences in the hippocampus and frontal cortex that were attributed to the prolonged handling of the pups required by the motor experimental protocol. In that case it was hard to determine whether the neurotrophin differences at cerebellar and striatal level caused the differences in motor behavior or vice versa whether the different motor behavior caused the neurotrophin differences. Notably, a strength point of the present research is that the effects induced by pre-reproductive maternal enrichment on the offspring were not influenced by the manipulations linked to behavioral testing, given that the BDNF increase was found in the not-tested siblings of behaviorally tested pups.
Unexpectedly, the pre-reproductive maternal rearing conditions did not influence reelin levels at birth and adulthood as well as adult neurogenesis. During neurodevelopment the glycoprotein reelin serves as a powerful stop signal for radially migrating principial neurons of the cerebral cortex and hippocampus, is crucial for migration of cortical interneurons, and is necessary in regulating cerebellar Purkinje cell migration (Lakatosova and Ostatnikova, 2012). In adulthood the reelin is expressed by GABAergic interneurons and has an important role as signaling protein in dendritic and axonal growth, neurotransmission and adult neurogenesis (Masiulis et al., 2011; Lakatosova and Ostatnikova, 2012). In particular, being expressed by the basket cells in the hilus of the dentate gyrus, reelin is involved in signaling pathways regulating the generation of new granule cells. It has been demonstrated that reelin alters the cell cycle properties of the transiently amplifying progenitors (probably Type-2 cells) and increases the survival of DCX+ immature neurons (Kuhn et al., 1996; Pujadas et al., 2010). It has been also demonstrated that EE modulates reelin expression (Li et al., 2007; Komitova et al., 2013) and adult hippocampal neurogenesis (Mora et al., 2007; Covic et al., 2010). Since we found no effect on both neurogenesis and reelin levels of the offspring, it is possible to advance that the transgenerational effects of pre-reproductive maternal enrichment critically affect the functional neuroplasticity linked to BDNF rather than the structural features linked to reelin and neurogenesis. Namely, we hypothesize that the BDNF modifications were associated to fine plastic changes regarding mature neuronal structure (such as branching and dendritic spine modifications) and changes in neurotransmission, LTP, and memory consolidation.
Knowing the mechanisms by which gene-environment interplay is achieved allows clarifying the dynamic nature of gene regulation and biological link between experiences of an organism and individual differences in neurodevelopment and behavior (Franklin and Mansuy, 2010). Multiple mechanisms, such as germ-line and somatic transmission, fetal development, and maternal nurturing, influence the transgenerational inheritance of qualities acquired through parental experience (Weaver et al., 2004; Champagne and Curley, 2009; Ho and Burggren, 2010; Meaney, 2010). In the present study environmental manipulation was applied to mothers and not to fathers, thus transgenerational effects could occur only through maternal germ-line, excluding any paternal influence. The beneficial effects of pre-reproductive EE maternal exposure could have been transmitted to the offspring before the birth in utero and after the birth by lactation. In fact, heritable epigenetic changes may have occurred through factors (e.g., hormones, antibodies, antioxidants) transmitted from mother to offspring via placenta or milk (Ho and Burggren, 2010). Also the mother-infant interaction during the early life stages has a remarkable impact on offspring phenotype, as indicated by Meaney's group studies (Weaver et al., 2004; Weaver, 2007; Champagne and Curley, 2009). Only more detailed maternal behavior observations, cross-fostering studies, and epigenetic analyses may determine if the transgenerational inheritance is a product of prenatal factors, post-natal experience or both.
Overall, the present results demonstrating that pre-reproductive maternal environmental enrichment shapes the offspring phenotype indicate that females that experience a particular environment transgenerationally prepare their progeny to cope with that environment (Debiec and Sullivan, 2014). Intriguingly, the transmission of maternal experience could have broad implications for progeny, improving their adaptive competencies and sculpting their behaviors (Petrosini et al., 2009).
LP, DC, and FA designed the research; DC, PC, DL, and FF performed behavioral evaluation; FG, PD, SF, EB, and FA performed biochemical analyses; DC, PC, FG, SF, EB, MM, and LP analyzed data; all authors discussed data; DC, LP, FG, SV, and EB wrote the paper.
The authors declare that the research was conducted in the absence of any commercial or financial relationships that could be construed as a potential conflict of interest.
This work was supported by University “Sapienza” of Rome funds to LP.
The Supplementary Material for this article can be found online at: http://www.frontiersin.org/journal/10.3389/fnbeh.2015.00066/abstract
Supplementary Figure 1. Nest quality photos. Illustrative photographs representing the different nest quality scores on the basis of the 4-point scale used (0, no nest; 1, flat nest; 3, cup-shaped nest; 4, complex hooded nest).
Supplementary Figure 2. Adult neurogenesis. Results of pre-reproductive maternal rearing condition on adult neurogenesis (pnd 55) are depicted. Histograms show: number of proliferating BrdU+ newborn neurons (A), number of early differentiating progenitors Dcx+ (B), differentiating newborn neurons (C). Representative images show dentate gyrus BrdU+DCX+ cells of EF-p and SF-p. Scale bar: 25 μm. Results are reported as mean ± SEM. DG: dentate gyrus.
Supplementary Figure 3. Levels of Reelin protein. Results of pre-reproductive maternal rearing condition on reelin protein expression are depicted. Representative immunoblots and densitometric graphs of reelin protein levels in hippocampus and cortex at birth (pnd 0) and in hippocampus and frontal cortex at adulthood (pnd 55). Results are reported as mean ± SEM. Hp, Hippocampus; C, Cortex; FC, Frontal Cortex.
Afonso, V. M., Sison, M., Lovic, V., and Fleming, A. S. (2007). Medial prefrontal cortex lesions in the female rat affect sexual and maternal behavior and their sequential organization. Behav. Neurosci. 121, 515–526. doi: 10.1037/0735-7044.121.3.515
PubMed Abstract | Full Text | CrossRef Full Text | Google Scholar
Angelucci, F., De Bartolo, P., Gelfo, F., Foti, F., Cutuli, D., Bossù, P., et al. (2009). Increased concentrations of nerve growth factor and brain-derived neurotrophic factor in the rat cerebellum after exposure to environmental enrichment. Cerebellum 8, 499–506. doi: 10.1007/s12311-009-0129-1
PubMed Abstract | Full Text | CrossRef Full Text | Google Scholar
Arai, J. A., and Feig, L. A. (2011). Long-lasting and transgenerational effects of an environmental enrichment on memory formation. Brain Res. Bull. 85, 30–35. doi: 10.1016/j.brainresbull.2010.11.003
PubMed Abstract | Full Text | CrossRef Full Text | Google Scholar
Arai, J. A., Li, S., Hartley, D. M., and Feig, L. A. (2009). Transgenerational rescue of a genetic defect in long-term potentiation and memory formation by juvenile enrichment. J. Neurosci. 29, 1496–1502. doi: 10.1523/JNEUROSCI.5057-08.2009
PubMed Abstract | Full Text | CrossRef Full Text | Google Scholar
Baroncelli, L., Braschi, C., Spolidoro, M., Begenisic, T., Sale, A., and Maffei, L. (2010). Nurturing brain plasticity: impact of environmental enrichment. Cell Death Differ. 17, 1092–1103. doi: 10.1038/cdd.2009.193
PubMed Abstract | Full Text | CrossRef Full Text | Google Scholar
Biamonte, F., Latini, L., Giorgi, F. S., Zingariello, M., Marino, R., De Luca, R., et al. (2014). Associations among exposure to methylmercury, reduced reelin expression, and gender in the cerebellum of developing mice. Neurotoxicology 45, 67–80. doi: 10.1016/j.neuro.2014.09.006
PubMed Abstract | Full Text | CrossRef Full Text | Google Scholar
Bick-Sander, A., Steiner, B., Wolf, S. A., Babu, H., and Kempermann, G. (2006). Running in pregnancy transiently increases postnatal hippocampal neurogenesis in the offspring. Proc. Natl. Acad. Sci. U.S.A. 103, 3852–3857. doi: 10.1073/pnas.0502644103
PubMed Abstract | Full Text | CrossRef Full Text | Google Scholar
Blum, R., and Konnerth, A. (2005). Neurotrophin-mediated rapid signaling in the central nervous system: mechanisms and functions. Physiology. 20, 70–78. doi: 10.1152/physiol.00042.2004
PubMed Abstract | Full Text | CrossRef Full Text | Google Scholar
Branchi, I., D'Andrea, I., Fiore, M., Di Fausto, V., Aloe, L., and Alleva, E. (2006). Early social enrichment shapes social behavior and nerve growth factor and brain-derived neurotrophic factor levels in the adult mouse brain. Biol. Psychiatry. 60, 690–696. doi: 10.1016/j.biopsych.2006.01.005
PubMed Abstract | Full Text | CrossRef Full Text | Google Scholar
Branchi, I., Karpova, N. N., D'Andrea, I., Castrén, E., and Alleva, E. (2011). Epigenetic modifications induced by early enrichment are associated with changes in timing of induction of BDNF expression. Neurosci. Lett. 495, 168–172. doi: 10.1016/j.neulet.2011.03.038
PubMed Abstract | Full Text | CrossRef Full Text | Google Scholar
Bredy, T. W., Zhang, T. Y., Grant, R. J., Diorio, J., and Meaney, M. J. (2004). Peripubertal environmental enrichment reverses the effects of maternal care on hippocampal development and glutamate receptor subunit expression. Eur. J. Neurosci. 20, 1355–1362. doi: 10.1111/j.1460-9568.2004.03599.x
Calamandrei, G., and Keverne, E. B. (1994). Differential expression of Fos protein in the brain of female mice dependent on pup sensory cues and maternal experience. Behav. Neurosci. 108, 113–120. doi: 10.1037/0735-7044.108.1.113
PubMed Abstract | Full Text | CrossRef Full Text | Google Scholar
Caldji, C., Tannenbaum, B., Sharma, S., Francis, D., Plotsky, P. M., and Meaney, M. J. (1998). Maternal care during infancy regulates the development of neural systems mediating the expression of fearfulness in the rat. Proc. Natl. Acad. Sci. U.S.A. 95, 5335–5340. doi: 10.1073/pnas.95.9.5335
PubMed Abstract | Full Text | CrossRef Full Text | Google Scholar
Caporali, P., Cutuli, D., Gelfo, F., Laricchiuta, D., Foti, F., De Bartolo, P., et al. (2014). Pre-reproductive maternal enrichment influences offspring developmental trajectories: motor behavior and neurotrophin expression. Front. Behav. Neurosci. 8:195. doi: 10.3389/fnbeh.2014.00195
Champagne, F. A., and Curley, J. P. (2009). Epigenetic mechanisms mediating the long-term effects of maternal care on development. Neurosci. Biobehav. Rev. 33, 593–600. doi: 10.1016/j.neubiorev.2007.10.009
PubMed Abstract | Full Text | CrossRef Full Text | Google Scholar
Champagne, F. A., and Meaney, M. J. (2006). Stress during gestation alters postpartum maternal care and the development of the offspring in a rodent model. Biol. Psychiatry 59, 1227–1235. doi: 10.1016/j.biopsych.2005.10.016
PubMed Abstract | Full Text | CrossRef Full Text | Google Scholar
Champagne, F. A., and Meaney, M. J. (2007). Transgenerational effects of social environment on variations in maternal care and behavioral response to novelty. Behav. Neurosci. 121, 1353–1363. doi: 10.1037/0735-7044.121.6.1353
PubMed Abstract | Full Text | CrossRef Full Text | Google Scholar
Charil, A., Laplante, D. P., Vaillancourt, C., and King, S. (2010). Prenatal stress and brain development. Brain Res. Rev. 65, 56–79. doi: 10.1016/j.brainresrev.2010.06.002
PubMed Abstract | Full Text | CrossRef Full Text | Google Scholar
Chourbaji, S., Hörtnagl, H., Molteni, R., Riva, M. A., Gass, P., and Hellweg, R. (2012). The impact of environmental enrichment on sex-specific neurochemical circuitries - effects on brain-derived neurotrophic factor and the serotonergic system. Neuroscience 220, 267–276. doi: 10.1016/j.neuroscience.2012.06.016
PubMed Abstract | Full Text | CrossRef Full Text | Google Scholar
Cirulli, F., Berry, A., Chiarotti, F., and Alleva, E. (2004). Intrahippocampal administration of BDNF in adult rats affects short-term behavioral plasticity in the Morris water maze and performance in the elevated plus-maze. Hippocampus 14, 802–807. doi: 10.1002/hipo.10220
PubMed Abstract | Full Text | CrossRef Full Text | Google Scholar
Covic, M., Karaca, E., and Lie, D. C. (2010). Epigenetic regulation of neurogenesis in the adult hippocampus. Heredity 105, 122–134. doi: 10.1038/hdy.2010.27
PubMed Abstract | Full Text | CrossRef Full Text | Google Scholar
Cutuli, D., De Bartolo, P., Caporali, P., Laricchiuta, D., Foti, F., Ronci, M., et al. (2014). n-3 polyunsaturated fatty acids supplementation enhances hippocampal functionality in aged mice. Front. Aging Neurosci. 6:220. doi: 10.3389/fnagi.2014.00220
PubMed Abstract | Full Text | CrossRef Full Text | Google Scholar
Cutuli, D., De Bartolo, P., Caporali, P., Tartaglione, A. M., Oddi, D., D'Amato, F. R., et al. (2013). Neuroprotective effects of donepezil against cholinergic depletion. Alzheimers Res. Ther. 5, 50. doi: 10.1186/alzrt215
Cutuli, D., Foti, F., Mandolesi, L., De Bartolo, P., Gelfo, F., Federico, F., et al. (2009). Cognitive performances of cholinergically depleted rats following chronic donepezil administration. J. Alzheimers Dis. 17, 161–176. doi: 10.3233/JAD-2009-1040
PubMed Abstract | Full Text | CrossRef Full Text | Google Scholar
Cutuli, D., Rossi, S., Burello, L., Laricchiuta, D., De Chiara, V., Foti, F., et al. (2011). Before or after does it matter? Different protocols of environmental enrichment differently influence motor, synaptic and structural deficits of cerebellar origin. Neurobiol. Dis. 42, 9–20. doi: 10.1016/j.nbd.2010.12.007
PubMed Abstract | Full Text | CrossRef Full Text | Google Scholar
Debiec, J., and Sullivan, R. M. (2014). Intergenerational transmission of emotional trauma through amygdala-dependent mother-to-infant transfer of specific fear. Proc. Natl. Acad. Sci. U.S.A. 111, 12222–12227. doi: 10.1073/pnas.1316740111
PubMed Abstract | Full Text | CrossRef Full Text | Google Scholar
Dell, P. A., and Rose, F. D. (1987). Transfer of effects from environmentally enriched and impoverished female rats to future offspring. Physiol. Behav. 39, 187–190. doi: 10.1016/0031-9384(87)90008-4
PubMed Abstract | Full Text | CrossRef Full Text | Google Scholar
Farioli-Vecchioli, S., Ceccarelli, M., Saraulli, D., Micheli, L., Cannas, S., D'Alessandro, F., et al. (2014). Tis21 is required for adult neurogenesis in the subventricular zone and for olfactory behavior regulating cyclins, BMP4, Hes1/5 and Ids. Front. Cell. Neurosci. 8:98. doi: 10.3389/fncel.2014.00098
PubMed Abstract | Full Text | CrossRef Full Text | Google Scholar
Febo, M., Felix-Ortiz, A. C., and Johnson, T. R. (2010). Inactivation or inhibition of neuronal activity in the medial prefrontal cortex largely reduces pup retrieval and grouping in maternal rats. Brain Res. 1325, 77–88. doi: 10.1016/j.brainres.2010.02.027
PubMed Abstract | Full Text | CrossRef Full Text | Google Scholar
Febo, M., and Ferris, C. F. (2007). Development of cocaine sensitization before pregnancy affects subsequent maternal retrieval of pups and prefrontal cortical activity during nursing. Neuroscience 148, 400–412. doi: 10.1016/j.neuroscience.2007.05.026
PubMed Abstract | Full Text | CrossRef Full Text | Google Scholar
Ferris, C. F., Kulkarni, P., Sullivan, J. M. Jr., Harder, J. A., Messenger, T. L., and Febo, M. (2005). Pup suckling is more rewarding than cocaine: evidence from functional magnetic resonance imaging and three-dimensional computational analysis. J. Neurosci. 25, 149–156. doi: 10.1523/JNEUROSCI.3156-04.2005
PubMed Abstract | Full Text | CrossRef Full Text | Google Scholar
Fleming, A. S., and Rosenblatt, J. S. (1974). Maternal behavior in the virgin and lactating rat. J. Comp. Physiol. Psychol. 86, 957–972. doi: 10.1037/h0036414
PubMed Abstract | Full Text | CrossRef Full Text | Google Scholar
Foti, F., Laricchiuta, D., Cutuli, D., De Bartolo, P., Gelfo, F., Angelucci, F., et al. (2011). Exposure to an enriched environment accelerates recovery from cerebellar lesion. Cerebellum 10, 104–119. doi: 10.1007/s12311-010-0236-z
PubMed Abstract | Full Text | CrossRef Full Text | Google Scholar
Francis, D. D., Champagne, F. A., Liu, D., and Meaney, M. J. (1999). Maternal care, gene expression, and the development of individual differences in stress reactivity. Ann. N.Y. Acad. Sci. 896, 66–84. doi: 10.1111/j.1749-6632.1999.tb08106.x
PubMed Abstract | Full Text | CrossRef Full Text | Google Scholar
Francis, D. D., and Meaney, M. J. (1999). Maternal care and the development of stress responses. Curr. Opin. Neurobiol. 9, 128–134. doi: 10.1016/S0959-4388(99)80016-6
PubMed Abstract | Full Text | CrossRef Full Text | Google Scholar
Franklin, T. B., and Mansuy, I. M. (2010). Epigenetic inheritance in mammals: evidence for the impact of adverse environmental effects. Neurobiol. Dis. 39, 61–65. doi: 10.1016/j.nbd.2009.11.012
PubMed Abstract | Full Text | CrossRef Full Text | Google Scholar
Gelfo, F., Cutuli, D., Foti, F., Laricchiuta, D., De Bartolo, P., Caltagirone, C., et al. (2011). Enriched environment improves motor function and increases neurotrophins in hemicerebellar lesioned rats. Neurorehabil. Neural Repair. 25, 243–252. doi: 10.1177/1545968310380926
PubMed Abstract | Full Text | CrossRef Full Text | Google Scholar
Gelfo, F., De Bartolo, P., Giovine, A., Petrosini, L., and Leggio, M. G. (2009). Layer and regional effects of environmental enrichment on the pyramidal neuron morphology of the rat. Neurobiol. Learn. Mem. 91, 353–365. doi: 10.1016/j.nlm.2009.01.010
PubMed Abstract | Full Text | CrossRef Full Text | Google Scholar
Glover, V. (2011). Annual research review: prenatal stress and the origins of psychopathology: an evolutionary perspective. J. Child Psychol. Psychiatry 52, 356–367. doi: 10.1111/j.1469-7610.2011.02371.x
PubMed Abstract | Full Text | CrossRef Full Text | Google Scholar
Glowinski, J., and Iversen, L. L. (1966). Regional studies of catecholamines in the rat brain. I. The disposition of [3H]norepinephrine, [3H]dopamine and [3H]dopa in various regions of the brain. J. Neurochem. 13, 655–669. doi: 10.1111/j.1471-4159.1966.tb09873.x
PubMed Abstract | Full Text | CrossRef Full Text | Google Scholar
Hall, J., Thomas, K. L., and Everitt, B. J. (2000). Rapid and selective induction of BDNF expression in the hippocampus during contextual learning. Nat. Neurosci. 3, 533–535. doi: 10.1038/75698
PubMed Abstract | Full Text | CrossRef Full Text | Google Scholar
Heldt, S. A., Stanek, L., Chhatwal, J. P., and Ressler, K. J. (2007). Hippocampus-specific deletion of BDNF in adult mice impairs spatial memory and extinction of aversive memories. Mol. Psychiatry 12, 656–670. doi: 10.1038/sj.mp.4001957
PubMed Abstract | Full Text | CrossRef Full Text | Google Scholar
Herring, A., Donath, A., Yarmolenko, M., Uslar, E., Conzen, C., Kanakis, D., et al. (2012). Exercise during pregnancy mitigates Alzheimer-like pathology in mouse offspring. FASEB J. 26, 117–128. doi: 10.1096/fj.11-193193
PubMed Abstract | Full Text | CrossRef Full Text | Google Scholar
Ho, D. H., and Burggren, W. W. (2010). Epigenetics and transgenerational transfer: a physiological perspective. J. Exp. Biol. 213, 3–16. doi: 10.1242/jeb.019752
PubMed Abstract | Full Text | CrossRef Full Text | Google Scholar
Kalueff, A. V., Keisala, T., Minasyan, A., Kuuslahti, M., Miettinen, S., and Tuohimaa, P. (2006). Behavioural anomalies in mice evoked by “Tokyo” disruption of the Vitamin D receptor gene. Neurosci. Res. 54, 254–260. doi: 10.1016/j.neures.2005.12.008
PubMed Abstract | Full Text | CrossRef Full Text | Google Scholar
Katoh-Semba, R., Takeuchi, I. K., Semba, R., and Kato, K. (1997). Distribution of brain-derived neurotrophic factor in rats and its changes with development in the brain. J. Neurochem. 69, 34–42. doi: 10.1046/j.1471-4159.1997.69010034.x
PubMed Abstract | Full Text | CrossRef Full Text | Google Scholar
Kempermann, G. (2012). New neurons for ‘survival of the fittest’. Nat. Rev. Neurosci. 13, 727–736. doi: 10.1038/nrn3319
PubMed Abstract | Full Text | CrossRef Full Text | Google Scholar
Kiyono, S., Seo, M. L., Shibagaki, M., and Inouye, M. (1985). Facilitative effects of maternal environmental enrichment on maze learning in rat offspring. Physiol. Behav. 34, 431–435. doi: 10.1016/0031-9384(85)90207-0
PubMed Abstract | Full Text | CrossRef Full Text | Google Scholar
Komitova, M., Xenos, D., Salmaso, N., Tran, K. M., Brand, T., Schwartz, M. L., et al. (2013). Hypoxia-induced developmental delays of inhibitory interneurons are reversed by environmental enrichment in the postnatal mouse forebrain. J. Neurosci. 33, 13375–13387. doi: 10.1523/JNEUROSCI.5286-12.2013
Korte, M., Carroll, P., Wolf, E., Brem, G., Thoenen, H., and Bonhoeffer, T. (1995). Hippocampal long-term potentiation is impaired in mice lacking brain-derived neurotrophic factor. Proc. Natl. Acad. Sci. U.S.A. 92, 8856–8860. doi: 10.1073/pnas.92.19.8856
PubMed Abstract | Full Text | CrossRef Full Text | Google Scholar
Kovalchuk, Y., Hanse, E., Kafitz, K. W., and Konnerth, A. (2002). Postsynaptic Induction of BDNF-Mediated Long-Term Potentiation. Science. 295, 1729–1734. doi: 10.1126/science.1067766
PubMed Abstract | Full Text | CrossRef Full Text | Google Scholar
Kuhn, H. G., Dickinson-Anson, H., and Gage, F. H. (1996). Neurogenesis in the dentate gyrus of the adult rat: age-related decrease of neuronal progenitor proliferation. J. Neurosci. 16, 2027–2033.
Lakatosova, S., and Ostatnikova, D. (2012). Reelin and its complex involvement in brain development and function. Int. J. Biochem. Cell Biol. 44, 1501–1504. doi: 10.1016/j.biocel.2012.06.002
PubMed Abstract | Full Text | CrossRef Full Text | Google Scholar
Lamarck, J. B. (1809). Philosophie Zoologique: ou Exposition des Considérations Relatives à L'histoire Naturelle des Animaux. Paris: Dentu.
Laviola, G., Sedowofia, K., Innes, J., Clayton, R., and Manning, A. (1990). Genetic differences in maternal behaviour patterns in mice administered phenobarbital during pregnancy. Psychopharmacology 102, 383–390. doi: 10.1007/BF02244108
PubMed Abstract | Full Text | CrossRef Full Text | Google Scholar
Lee, H. H., Kim, H., Lee, J. W., Kim, Y. S., Yang, H. Y., Chang, H. K., et al. (2006). Maternal swimming during pregnancy enhances short-term memory and neurogenesis in the hippocampus of rat pups. Brain Dev. 28, 147–154. doi: 10.1016/j.braindev.2005.05.007
PubMed Abstract | Full Text | CrossRef Full Text | Google Scholar
Leibenluft, E., Gobbini, M. I., Harrison, T., and Haxby, J. V. (2004). Mothers' neural activation in response to pictures of their children and other children. Biol. Psychiatry 56, 225–232. doi: 10.1016/j.biopsych.2004.05.017
PubMed Abstract | Full Text | CrossRef Full Text | Google Scholar
Leshem, M., and Schulkin, J. (2012). Transgenerational effects of infantile adversity and enrichment in male and female rats. Dev. Psychobiol. 54, 169–186. doi: 10.1002/dev.20592
PubMed Abstract | Full Text | CrossRef Full Text | Google Scholar
Li, C., Niu, W., Jiang, C. H., and Hu, Y. (2007). Effects of enriched environment on gene expression and signal pathways in cortex of hippocampal CA1 specific NMDAR1 knockout mice. Brain Res. Bull. 71, 568–577. doi: 10.1016/j.brainresbull.2006.11.011
PubMed Abstract | Full Text | CrossRef Full Text | Google Scholar
Liu, D., Diorio, J., Day, J. C., Francis, D. D., and Meaney, M. J. (2000). Maternal care, hippocampal synaptogenesis and cognitive development in rats. Nat. Neurosci. 3, 799–806. doi: 10.1038/77702
PubMed Abstract | Full Text | CrossRef Full Text | Google Scholar
Lorberbaum, J. P., Newman, J. D., Horwitz, A. R., Dubno, J. R., Lydiard, R. B., Hamner, M. B., et al. (2002). A potential role for thalamocingulate circuitry in human maternal behavior. Biol. Psychiatry 51, 431–445. doi: 10.1016/S0006-3223(01)01284-7
PubMed Abstract | Full Text | CrossRef Full Text | Google Scholar
Lu, B., Pang, P. T., and Woo, N. H. (2005). The yin and yang of neurotrophin action. Nat. Rev. Neurosci. 6, 603–614. doi: 10.1038/nrn1726
PubMed Abstract | Full Text | CrossRef Full Text | Google Scholar
Macrì, S., and Wurbel, H. (2006). Developmental plasticity of HPA and fear responses in rats: a critical review of the maternal mediation hypothesis. Horm. Behav. 50, 667–680. doi: 10.1016/j.yhbeh.2006.06.015
PubMed Abstract | Full Text | CrossRef Full Text | Google Scholar
Mashoodh, R., Franks, B., Curley, J. P., and Champagne, F. A. (2012). Paternal social enrichment effects on maternal behavior and offspring growth. Proc. Natl. Acad. Sci. U.S.A. 109, 17232–17238. doi: 10.1073/pnas.1121083109
PubMed Abstract | Full Text | CrossRef Full Text | Google Scholar
Masiulis, I., Yun, S., and Eisch, A. J. (2011). The interesting interplay between interneurons and adult hippocampal neurogenesis. Mol. Neurobiol. 44, 287–302. doi: 10.1007/s12035-011-8207-z
PubMed Abstract | Full Text | CrossRef Full Text | Google Scholar
McKim, M., and Thompson, W. R. (1975). Prenatal maternal enrichment and restriction in rats: effects on biological and foster offspring. Bull. Psychon. Soc. 5, 259–260. doi: 10.3758/BF03337626
Meaney, M. J. (2001). Maternal care, gene expression, and the transmission of individual differences in stress reactivity across generations. Annu. Rev. Neurosci. 24, 1161–1192. doi: 10.1146/annurev.neuro.24.1.1161
PubMed Abstract | Full Text | CrossRef Full Text | Google Scholar
Meaney, M. J. (2010). Epigenetics and the biological definition of gene x environment interactions. Child Dev. 81, 41–79. doi: 10.1111/j.1467-8624.2009.01381.x
PubMed Abstract | Full Text | CrossRef Full Text | Google Scholar
Meck, W. H., and Williams, C. L. (2003). Metabolic imprinting of choline by its availability during gestation: implications for memory and attentional processing across the lifespan. Neurosci. Biobehav. Rev. 27, 385–399. doi: 10.1016/S0149-7634(03)00069-1
PubMed Abstract | Full Text | CrossRef Full Text | Google Scholar
Mora, F., Segovia, G., and del Arco, A. (2007). Aging, plasticity and environmental enrichment: structural changes and neurotransmitter dynamics in several areas of the brain. Brain Res. Rev. 55, 78–88. doi: 10.1016/j.brainresrev.2007.03.011
PubMed Abstract | Full Text | CrossRef Full Text | Google Scholar
Moriceau, S., and Sullivan, R. M. (2004). Unique neural circuitry for neonatal olfactory learning. J. Neurosci. 24, 1182–1189. doi: 10.1523/JNEUROSCI.4578-03.2004
PubMed Abstract | Full Text | CrossRef Full Text | Google Scholar
Mueller, B. R., and Bale, T. L. (2008). Sex-specific programming of offspring emotionality after stress early in pregnancy. J. Neurosci. 28, 9055–9065. doi: 10.1523/JNEUROSCI.1424-08.2008
PubMed Abstract | Full Text | CrossRef Full Text | Google Scholar
Mychasiuk, R., Zahir, S., Schmold, N., Ilnytskyy, S., Kovalchuk, O., and Gibb, R. (2012). Parental enrichment and offspring development: modifications to brain, behavior and the epigenome. Behav. Brain Res. 228, 294–298. doi: 10.1016/j.bbr.2011.11.036
PubMed Abstract | Full Text | CrossRef Full Text | Google Scholar
Nadler, J. J., Moy, S. S., Dold, G., Trang, D., Simmons, N., Perez, A., et al. (2004). Automated apparatus for quantitation of social approach behaviors in mice. Genes Brain Behav. 3, 303–314. doi: 10.1111/j.1601-183X.2004.00071.x
PubMed Abstract | Full Text | CrossRef Full Text | Google Scholar
Nithianantharajah, J., and Hannan, A. J. (2006). Enriched environments, experience-dependent plasticity and disorders of the nervous system. Nat. Rev. Neurosci. 7, 697–709. doi: 10.1038/nrn1970
PubMed Abstract | Full Text | CrossRef Full Text | Google Scholar
Nithianantharajah, J., and Hannan, A. J. (2009). The neurobiology of brain and cognitive reserve: mental and physical activity as modulators of brain disorders. Prog. Neurobiol. 89, 369–382. doi: 10.1016/j.pneurobio.2009.10.001
PubMed Abstract | Full Text | CrossRef Full Text | Google Scholar
Nitschke, J. B., Nelson, E. E., Rusch, B. D., Fox, A. S., Oakes, T. R., and Davidson, R. J. (2004). Orbitofrontal cortex tracks positive mood in mothers viewing pictures of their newborn infants. Neuroimage 21, 583–592. doi: 10.1016/j.neuroimage.2003.10.005
PubMed Abstract | Full Text | CrossRef Full Text | Google Scholar
Noriuchi, M., Kikuchi, Y., and Senoo, A. (2008). The functional neuroanatomy of maternal love: mother's response to infant's attachment behaviors. Biol. Psychiatry 63, 415–423. doi: 10.1016/j.biopsych.2007.05.018
PubMed Abstract | Full Text | CrossRef Full Text | Google Scholar
Parnpiansil, P., Jutapakdeegul, N., Chentanez, T., and Kotchabhakdi, N. (2003). Exercise during pregnancy increases hippocampal brain-derived neurotrophic factor mRNA expression and spatial learning in neonatal rat pup. Neurosci. Lett 352, 45–48. doi: 10.1016/j.neulet.2003.08.023
PubMed Abstract | Full Text | CrossRef Full Text | Google Scholar
Petrosini, L., De Bartolo, P., Foti, F., Gelfo, F., Cutuli, D., Leggio, M. G., et al. (2009). On whether the environmental enrichment may provide cognitive and brain reserves. Brain Res. Rev. 61, 221–239. doi: 10.1016/j.brainresrev.2009.07.002
PubMed Abstract | Full Text | CrossRef Full Text | Google Scholar
Petruzzi, S., Chiarotti, F., Alleva, E., and Laviola, G. (1995). Limited changes of mouse maternal care after prenatal oxazepam: dissociation from pup-related stimulus perception. Psychopharmacology 122, 58–65. doi: 10.1007/BF02246442
PubMed Abstract | Full Text | CrossRef Full Text | Google Scholar
Pham, T. M., Winblad, B., Granholm, A. C., and Mohammed, A. H. (2002). Environmental influences on brain neurotrophins in rats. Pharmacol. Biochem. Behav. 73, 167–175. doi: 10.1016/S0091-3057(02)00783-9
PubMed Abstract | Full Text | CrossRef Full Text | Google Scholar
Pujadas, L., Gruart, A., Bosch, C., Delgado, L., Teixeira, C. M., Rossi, D., et al. (2010). Reelin regulates postnatal neurogenesis and enhances spine hypertrophy and long-term potentiation. J. Neurosci. 30, 4636–4649. doi: 10.1523/JNEUROSCI.5284-09.2010
PubMed Abstract | Full Text | CrossRef Full Text | Google Scholar
Raineki, C., Pickenhagen, A., Roth, T. L., Babstock, D. M., McLean, J. H., Harley, C. W., et al. (2010). The neurobiology of infant maternal odor learning. Braz. J. Med. Biol. Res. 43, 914–919. doi: 10.1590/S0100-879X2010007500090
PubMed Abstract | Full Text | CrossRef Full Text | Google Scholar
Ranote, S., Elliott, R., Abel, K. M., Mitchell, R., Deakin, J. F., and Appleby, L. (2004). The neural basis of maternal responsiveness to infants: an fMRI study. Neuroreport 15, 1825–1829. doi: 10.1097/01.wnr.0000137078.64128.6a
PubMed Abstract | Full Text | CrossRef Full Text | Google Scholar
Rice, D., and Barone, S. Jr. (2000). Critical periods of vulnerability for the developing nervous system: evidence from humans and animal models. Environ. Health Perspect. 108, 511–533. doi: 10.1289/ehp.00108s3511
PubMed Abstract | Full Text | CrossRef Full Text | Google Scholar
Rosenfeld, A., and Weller, A. (2012). Behavioral effects of environmental enrichment during gestation in WKY and Wistar rats. Behav. Brain Res. 233, 245–255. doi: 10.1016/j.bbr.2012.05.006
PubMed Abstract | Full Text | CrossRef Full Text | Google Scholar
Rosenzweig, M. R., Bennett, E. L., and Krech, D. (1964). Cerebral Effects of Environmental Complexity and Training among Adult Rats. J. Comp. Physiol. Psychol. 57, 438–439. doi: 10.1037/h0046387
PubMed Abstract | Full Text | CrossRef Full Text | Google Scholar
Roth, T. L., Lubin, F. D., Funk, A. J., and Sweatt, J. D. (2009). Lasting epigenetic influence of earlylife adversity on the BDNF gene. Biol. Psychiatry 65, 760–769. doi: 10.1016/j.biopsych.2008.11.028
PubMed Abstract | Full Text | CrossRef Full Text | Google Scholar
Sale, A., Berardi, N., and Maffei, L. (2014). Environment and brain plasticity: towards an endogenous pharmacotherapy. Physiol. Rev. 94, 189–234. doi: 10.1152/physrev.00036.2012
PubMed Abstract | Full Text | CrossRef Full Text | Google Scholar
Sale, A., Cenni, M. C., Ciucci, F., Putignano, E., Chierzi, S., and Maffei, L. (2007). Maternal enrichment during pregnancy accelerates retinal development of the fetus. PLoS ONE 2:e1160. doi: 10.1371/journal.pone.0001160
PubMed Abstract | Full Text | CrossRef Full Text | Google Scholar
Sale, A., Putignano, E., Cancedda, L., Landi, S., Cirulli, F., Berardi, N., et al. (2004). Enriched environment and acceleration of visual system development. Neuropharmacology 47, 649–660. doi: 10.1016/j.neuropharm.2004.07.008
PubMed Abstract | Full Text | CrossRef Full Text | Google Scholar
Schneider, J. E., and Lynch, C. B. (1984). Investigation of a common physiological mechanism underlying progesterone-induced and maternal nesting in mice, Mus musculus. J. Comp. Psychol. 98, 165–176. doi: 10.1037/0735-7036.98.2.165
PubMed Abstract | Full Text | CrossRef Full Text | Google Scholar
Segal, R. A. (2003). Selectivity in neurotrophin signaling: theme and variations. Annu. Rev. Neurosci. 26, 299–330. doi: 10.1146/annurev.neuro.26.041002.131421
PubMed Abstract | Full Text | CrossRef Full Text | Google Scholar
Sherrard, R. M., and Bower, A. J. (2002). Climbing fiber development: do neurotrophins have a part to play? Cerebellum 1, 265–275. doi: 10.1080/147342202320883579
PubMed Abstract | Full Text | CrossRef Full Text | Google Scholar
Simpson, J., and Kelly, J. P. (2011). The impact of environmental enrichment in laboratory rats: behavioural and neurochemical aspects. Behav. Brain Res. 222, 246–264. doi: 10.1016/j.bbr.2011.04.002
PubMed Abstract | Full Text | CrossRef Full Text | Google Scholar
Slotnick, B. M., and Nigrosh, B. J. (1975). Maternal behavior of mice with cingulate cortical, amygdala, or septal lesions. J. Comp. Physiol. Psychol. 88, 118–127. doi: 10.1037/h0076200
PubMed Abstract | Full Text | CrossRef Full Text | Google Scholar
Strathearn, L., Li, J., Fonagy, P., and Montague, P. R. (2008). What's in a smile? Maternal brain responses to infant facial cues. Pediatrics 122, 40–51. doi: 10.1542/peds.2007-1566
PubMed Abstract | Full Text | CrossRef Full Text | Google Scholar
Swain, J. E. (2008). Baby stimuli and the parent brain: functional neuroimaging of the neural substrates of parent-infant attachment. Psychiatry 5, 28–36.
Swanson, J. M., Entringer, S., Buss, C., and Wadhwa, P. D. (2009). Developmental origins of health and disease: environmental exposures. Semin. Reprod. Med. 27, 391–402. doi: 10.1055/s-0029-1237427
PubMed Abstract | Full Text | CrossRef Full Text | Google Scholar
Thompson, B. L., Levitt, P., and Stanwood, G. D. (2009). Prenatal exposure to drugs: effects on brain development and implications for policy and education. Nat. Rev. Neurosci. 10, 303–312. doi: 10.1038/nrn2598
PubMed Abstract | Full Text | CrossRef Full Text | Google Scholar
Tran, P. V., Fretham, S. J., Carlson, E. S., and Georgieff, M. K. (2009). Long-term reduction of hippocampal brain-derived neurotrophic factor activity after fetal-neonatal iron deficiency in adult rats. Pediatr. Res. 65, 493–498. doi: 10.1203/PDR.0b013e31819d90a1
PubMed Abstract | Full Text | CrossRef Full Text | Google Scholar
Van den Bergh, B. R. H., Mulder, E. J. H., Mennesa, M., and Glover, V. (2005). Antenatal maternal anxiety and stress and the neurobehavioural development of the fetus and child: links and possible mechanisms: a review. Neurosci. Biobehav. Rev. 29, 237–258. doi: 10.1016/j.neubiorev.2004.10.007
PubMed Abstract | Full Text | CrossRef Full Text | Google Scholar
Venerosi, A., Cutuli, D., Colonnello, V., Cardona, D., Ricceri, L., and Calamandrei, G. (2008). Neonatal exposure to chlorpyrifos affects maternal responses and maternal aggression of female mice in adulthood. Neurotoxicol. Teratol. 30, 468–474. doi: 10.1016/j.ntt.2008.07.002
PubMed Abstract | Full Text | CrossRef Full Text | Google Scholar
Weaver, I. C. (2007). Epigenetic programming by maternal behavior and pharmacological intervention. Nature versus nurture: let's call the whole thing off. Epigenetics 2, 22–28. doi: 10.4161/epi.2.1.3881
PubMed Abstract | Full Text | CrossRef Full Text | Google Scholar
Weaver, I. C., Diorio, J., Seckl, J. R., Dymov, S., Szyf, M., and Meaney, M. J. (2004). Epigenetic programming by maternal behavior. Nat. Neurosci. 7, 847–854. doi: 10.1038/nn1276
PubMed Abstract | Full Text | CrossRef Full Text | Google Scholar
Weinstock, M. (2005). The potential influence of maternal stress hormones on development and mental health of the offspring. Brain Behav. Immun. 19, 296–308. doi: 10.1016/j.bbi.2004.09.006
PubMed Abstract | Full Text | CrossRef Full Text | Google Scholar
Welberg, L., Thrivikraman, K. V., and Plotsky, P. M. (2006). Combined pre- and postnatal environmental enrichment programs the HPA axis differentially in male and female rats. Psychoneuroendocrinology 31, 553–564. doi: 10.1016/j.psyneuen.2005.11.011
PubMed Abstract | Full Text | CrossRef Full Text | Google Scholar
Xu, B., Gottschalk, W., Chow, A., Wilson, R. I., Schnell, E., Zang, K., et al. (2000). The role of brain-derived neurotrophic factor receptors in the mature hippocampus: modulation of long-term potentiation through a presynaptic mechanism involving TrkB. J. Neurosci. 20, 6888–6897.
Zagrebelsky, M., Holz, A., Dechant, G., Barde, Y. A., Bonhoeffer, T., and Korte, M. (2005). The p75 neurotrophin receptor negatively modulates dendrite complexity and spine density in hippocampal neurons. J. Neurosci. 25, 9989–9999. doi: 10.1523/JNEUROSCI.2492-05.2005
PubMed Abstract | Full Text | CrossRef Full Text | Google Scholar
Keywords: environmental enrichment, BDNF, reelin, neurogenesis, cognition, maternal behavior
Citation: Cutuli D, Caporali P, Gelfo F, Angelucci F, Laricchiuta D, Foti F, De Bartolo P, Bisicchia E, Molinari M, Farioli Vecchioli S and Petrosini L (2015) Pre-reproductive maternal enrichment influences rat maternal care and offspring developmental trajectories: behavioral performances and neuroplasticity correlates. Front. Behav. Neurosci. 9:66. doi: 10.3389/fnbeh.2015.00066
Received: 31 October 2014; Accepted: 25 February 2015;
Published: 12 March 2015.
Edited by:
Serge Laroche, Centre National de la Recherche Scientifique and Université Paris-Sud, FranceReviewed by:
Sara Morley-Fletcher, Centre National de la Recherche Scientifique-Université Lille 1, FranceCopyright © 2015 Cutuli, Caporali, Gelfo, Angelucci, Laricchiuta, Foti, De Bartolo, Bisicchia, Molinari, Farioli Vecchioli and Petrosini. This is an open-access article distributed under the terms of the Creative Commons Attribution License (CC BY). The use, distribution or reproduction in other forums is permitted, provided the original author(s) or licensor are credited and that the original publication in this journal is cited, in accordance with accepted academic practice. No use, distribution or reproduction is permitted which does not comply with these terms.
*Correspondence: Debora Cutuli, Department of Psychology, University “Sapienza” of Rome, Via dei Marsi 78, Rome 00185, Italy; Santa Lucia Foundation, Laboratory of Experimental and Behavioral Neurophisiology, Via del Fosso di Fiorano 64, Rome 00143, ItalyZGVib3JhLmN1dHVsaUB1bmlyb21hMS5pdA==
Disclaimer: All claims expressed in this article are solely those of the authors and do not necessarily represent those of their affiliated organizations, or those of the publisher, the editors and the reviewers. Any product that may be evaluated in this article or claim that may be made by its manufacturer is not guaranteed or endorsed by the publisher.
Research integrity at Frontiers
Learn more about the work of our research integrity team to safeguard the quality of each article we publish.