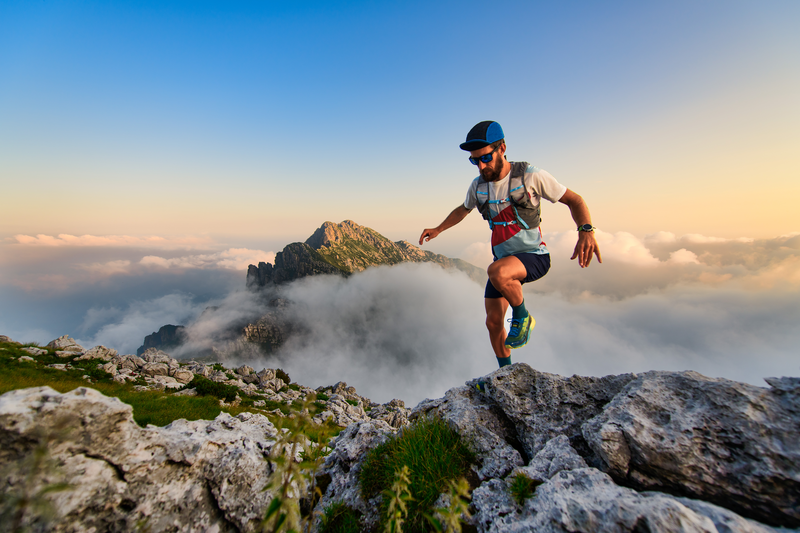
95% of researchers rate our articles as excellent or good
Learn more about the work of our research integrity team to safeguard the quality of each article we publish.
Find out more
HYPOTHESIS AND THEORY article
Front. Behav. Neurosci. , 30 July 2010
Sec. Learning and Memory
Volume 4 - 2010 | https://doi.org/10.3389/fnbeh.2010.00042
This article is part of the Research Topic Neuroethology View all 23 articles
Magnetoreception can play a substantial role in long distance navigation by animals. I hypothesize that locomotion guided by a magnetic compass sense could also play a role in short distance navigation. Animals identify mates, prey, or other short distance navigational goals using different sensory modalities (olfaction, vision, audition, etc.) to detect sensory cues associated with those goals. In conditions where these cues become unreliable for navigation (due to flow changes, obstructions, noise interference, etc.), switching to a magnetic compass sense to guide locomotion toward the navigational goals could be beneficial. Using simulations based on known locomotory and flow parameters, I show this strategy has strong theoretical benefits for the nudibranch mollusk Tritonia diomedea navigating toward odor sources in variable flow. A number of other animals may garner similar benefits, particularly slow-moving species in environments with rapidly changing cues relevant for navigation. Faster animals might also benefit from switching to a magnetic compass sense, provided the initial cues used for navigation (acoustic signals, odors, etc.) are intermittent or change rapidly enough that the entire navigation behavior cannot be guided by a continuously detectable cue. Examination of the relative durations of navigational tasks, the persistence of navigational cues, and the stability of both navigators and navigational targets will identify candidates with the appropriate combination of unreliable initial cues and relatively immobile navigational goals for which this hypothetical behavior could be beneficial. Magnetic manipulations can then test whether a switch to a magnetic compass sense occurs. This hypothesis thus provides an alternative when considering the behavioral significance of a magnetic compass sense in animals.
Animal navigation over short distances can depend on intermittent cues associated with navigational targets. For example, potential mates may only be visible for brief moments, as they are obscured by habitat features or other organisms. Alternatively, odor plumes emanating from potential prey can be swept away by changes in wind or flow direction. In such circumstances, successful navigation toward targets cannot rely solely on cues from the target. Several navigational strategies could overcome this problem. Continuing locomotion without guidance by any cue could succeed, provided the organism’s direction of movement is unlikely to be changed by external factors (gusts, eddies, substrate irregularities, etc.), a situation which may be rare for some animals in some habitats. Alternatively, many animals could continue navigation based on spatial representations of their environment generated through visual cues. Spatial cognition has been studied in both rodents and birds, and information from landmarks and geometric structure could be combined with information about the animal’s orientation to execute movements toward a navigational goal (Jeffery, 2007; Taube, 2007; Moser et al., 2008; Wilzeck et al., 2009). A third option could be based on the sun compass used by a number of taxa for navigation (e.g., von Frisch, 1950; Landreth and Ferguson, 1967; Wiltschko et al., 2000; Müller and Wehner, 2007). In this case, orientation based on the sun’s location could be used to follow a heading set when the navigational goal was originally detectable. Finally, a similar compass-based solution that does not rely on visual cues could be to switch to a magnetic compass bearing. This could allow movement to continue toward a goal that becomes impossible or difficult to detect directly. My goal here is a hypothetical analysis of this final option. Could animals that first locate nearby navigational targets using cues that are subsequently unreliable for navigation switch to a magnetic compass sense, and thereby navigate along a compass bearing toward the target using the more reliable magnetic cue?
The possibility of switching to a magnetic compass sense for short distance navigation builds on ideas about long distance animal navigation. Animals can navigate both over short distances, when the navigational target is directly detectable by the animal’s sensory systems, and also over long distances, when the target itself is beyond detection and other cues are used to guide the animal toward the target. The magnetic sense has largely been studied in the context of long distance navigation (reviewed in Wiltschko and Wiltschko, 2005). Numerous taxa have been shown to respond to magnetic fields, with evidence for both magnetic compass and magnetic map senses (Lohmann et al., 2007). Many animals with a magnetic compass sense show more than just an innate preference for a particular magnetic heading. For example, hatchling turtles adjust their magnetic heading preference based on wave directions they experience (Lohmann and Lohmann, 1996; Goff et al., 1998). Geomagnetic imprinting by fish as the swim away from natal areas has been proposed to generate magnetic preferences used in subsequent return migrations (Lohmann et al., 2008). Experiments in birds have shown that other sensory cues can calibrate a magnetic compass for long distance navigation behaviors (Cochran et al., 2004; reviewed in Muheim et al., 2006), and indeed that magnetic compass preferences can be learned in the laboratory (Wilzeck et al., 2010). Newts can learn a shoreward magnetic compass heading (Phillips, 1986; Deutschlander et al., 1999), and fruit fly larvae can also establish persistent magnetic preferences in the laboratory in the opposite direction to ultraviolet illumination (Dommer et al., 2008). Thus, many animals have changeable magnetic preferences that help guide long distance navigation. I propose that calibration of a magnetic compass guidance cue based on some other sensory cue could also occur for short distance navigation, particularly for slow moving animals in rapidly changing environments.
Magnetoreception has received little attention in the context of short distance navigation, yet a compass sense could play a role in maintaining headings over shorter distances as it does for longer distances. Olfactory, auditory, or visual cues often identify prey, mates, or other nearby navigational goals, yet these cues can be unreliable for reaching those goals: they are impermanent and can be obstructed. In contrast, magnetic cues are reliable for short distance navigation, just as they are for long distance navigation: they are permanent and rarely obstructed (Johnsen and Lohmann, 2005). Thus, if a navigational target identified using an unreliable cue can be correlated to a direction identifiable with a reliable magnetic cue, then an animal may improve its chances of reaching that target. The benefits of this hypothetical behavior depend critically on the relative durations of a stable primary cue and the navigation behavior. If the duration of the behavior is often longer than the stability of the primary cue, then switching to a magnetic compass could be beneficial. Hence the suggestion the behavior may be more common in slower-moving animals. However, if the navigation duration is likely to be similar or shorter than the duration of a stable primary cue, then the potential errors introduced by switching cues (the target moves, navigator drift, errors in compass calibration, etc.) suggest the behavior is unlikely to improve navigation success. Thus, contingent on the potential improvements to navigation success that depend on the relative stability of the primary cue and the locomotory capabilities of the navigation animals, natural selection could favor the sensory systems and neural circuits necessary to switch to a magnetic compass sense for short distance navigation.
I hypothesize that animals using a primary cue to locate or move toward a local navigational target will switch to a compass sense that uses magnetic fields as a more reliable secondary guidance cue if the primary cue is variable or ephemeral. At least four situations make cue-switching more probable: (1) the likelihood of using the primary cue to find the target is low; (2) the target is unlikely to move; (3) the probability of drift is low (i.e., undetectable navigator movement relative to the target is unlikely), and (4) other possible spatial representations of the environment (particularly visual) are limited either by the environment or the animal’s sensory or cognitive abilities. If any or all of these occur, switching to a magnetic compass sense might be favored by natural selection.
I use a theoretical case study of the nudibranch Tritonia diomedea to test whether this hypothetical strategy could have benefits when both an animals’ locomotory abilities and sensory environment are considered in detail. The slugs use odor-gated rheotaxis (OGR) to find both mates and prey, moving upstream when attractive odors are encountered (Wyeth and Willows, 2006c). They can detect the earth’s magnetic field, although how this cue is used in nature is unclear (Lohmann and Willows, 1987; Willows, 1999; Murray et al., 2006; Wyeth et al., 2006). The slugs’ navigation matches the criteria for switching cues. First, the primary cues used to identify the location of prey and mates are unreliable: the flow that transports the odors can often change direction before a slug is able to reach the target. Flow changes large enough to sweep an odor plume away from animal occur every 5 min in their natural habitat, while the slow-moving slugs navigation behaviors last an average of 8 min (Figure 1; Wyeth and Willows, 2006b). Second, neither navigational target is likely to move substantially as an animal navigates toward them. Prey are sedentary (Birkeland, 1974; Wyeth and Willows, 2006b), and mates, although mobile, are usually stationary as another animal approaches (Wyeth and Willows, 2006b). Third, although strong currents can move the slugs over the substratum, the slugs rarely navigate under such conditions (Wyeth and Willows, 2006b) and thus the probability of drift while navigating is low. Finally, the slugs are effectively blind (Chase, 1974), and therefore cannot construct a spatial representation of their environment based on visual cues. Thus a magnetic compass sense could form the basis of a secondary guidance system for continuous movement toward upstream targets that can no longer be detected by odors carried in the flow (Figure 2).
Figure 1. Four examples of flow patterns from the habitat of Tritonia diomedea with frequent flow direction changes that could render strict odor-gated rheotaxis unusable as an odor source search strategy. Note the compass headings are circular data, and thus the y-axis maxima and minima both indicate magnetic North (0°). Chemical plumes spread ∼30° in the habitat, and thus any flow direction change greater than 30° (the distance between y-axis tick marks) will likely sweep an odor plume away from an animal, forcing it to either stop or use a different strategy to find the odor plume source. On average, the flow remains within a 30° sector for only 5 min in this habitat, less than the 8 min average slugs spend navigating in a single direction (Wyeth and Willows, 2006a; Wyeth et al., 2006).
Figure 2. An example of how switching to a magnetic compass sense can improve navigation. Three simulated Tritonia diomedea start at the same location, 2 m distant from an odor source (X), and then use three strategies for to find the source. (1) Strict odor-gated rheotaxis (OGR), stopping once the odor plume is no longer detected (red); (2) OGR followed by rheotaxis for 10 min after odors are no longer detected (blue); (3) OGR, followed by a switch to a magnetic compass sense to follow last known heading for 10 min after odors are no longer detected (black). As the simulation progresses (A–H, time indicated in lower right for each frame), the slug using strict OGR moves only a short distance toward the target since it is only intermittently in contact with the odor plume (A,D). The slug that continues rheotaxis crawls much further, but is lead away from the source by the variable currents (E–G). These problems are overcome by the slug that switches to a magnetic compass, following the last heading from which odor was detected, as it makes continuous progress toward the odor source despite the intermittent odor plume (A–G), and makes a final approach to the source while in the odor plume (H). (I) The movement tracks for the three strategies demonstrate that for this particular flow pattern, switching to a magnetic compass sense was the best strategy to find the odor source. Movie versions of this figure using both simulation methods are available in the Supplementary Material.
Switching cues has not been incorporated into previous theoretical treatments of odor based navigation. Most studies have concerned optimal searches to find an odor plume (Sabelis and Schippers, 1984; Dusenbery, 1989, 1990; Balkovsky and Shraiman, 2002; Pasternak et al., 2009) or movements inside a plume to find its source (Li et al., 2001; Lytridis et al., 2001; Balkovsky and Shraiman, 2002; Pang and Farrell, 2006; Harvey et al., 2008a). Weissburg and Dusenbery (2002) explored the efficacy of OGR for finding an odor plume source without variations in bulk flow, and Harvey et al. (2008b) considered strategies for relocating an odor plume but not the odor source after a flow change. My goal here is to test the potential benefits of cue-switching for finding an odor source in variable flow. Since the relative performance of different strategies will depend critically on both the locomotory capabilities of the navigating animals and the patterns of flow variation they encounter, I use a simulation approach that allows incorporation of realistic locomotion and flow variations. I show that for the slugs, switching to magnetically guided crawling does indeed have strong hypothetical benefits for find odor sources, suggesting this navigational strategy may be present in these and other animals in similar situations.
I used biased random walk simulations with movement parameters and flow patterns gathered from field observations of the slugs to explore the success rates of different navigational strategies. A two-dimensional continuous space was used to track the locations of simulated odors and slugs over discrete time steps. Flow was simulated from 30 min current direction data sets (n = 88; Figure 1) gathered from the slugs’ natural habitat (Wyeth and Willows, 2006a; Wyeth et al., 2006). Flow speed changes were not simulated as these do not appear to be important for the slugs (the slugs navigate similarly at all but the highest velocities, when they simply stop moving). Attractive odor plumes were simulated by designating regions of the two-dimensional space as either with or without detectable levels of the odor. Two types of odor plume were simulated (Figure 3). In one set of simulations, odors were simulated as being released from a source, spreading through turbulent diffusion, and moving by advection according to flow directions. This was accomplished by creating circles of detectable odor at the origin (the odor source) at each time step, which then both expanded in diameter over time and whose center moved according to the flow direction at each time step. In the second set, used to increase computational speeds, an odor plume shape was oriented according to flow. A trapezoid approximating the shape of odor plumes was placed with its apex at the origin (the odor source) and then rotated so that its long axis was parallel to flow direction for that time step. In both cases, parameters (circle expansion rates and movement speeds or polygon vertices) were selected to best approximate dye plume shapes and movements recorded in the natural habitat (Table 1; Wyeth and Willows, 2006b). A comparison of the two model types with similarly optimized odor shape parameters (to create 30° plumes, matching dye plumes in the field) showed no significant differences in the relative success rates of different slug movement strategy-duration combinations (three factor ANOVA of arcsin transformed normalized minimum distances; full factorial model with odor simulation type, strategy, and duration as factors; three-way interaction factor: F16, 3915 = 0.067, P = 1.00).
Figure 3. Two simulation methods for odor plumes. (A) In one set of simulations, the region with detectable odors (gray) was defined by the location of an odor source (x) and expanding circles (outlined) moved by the current (arrow). (B) In the second set of simulations, the region with detectable odors (gray) was defined by a trapezoidal region (outlined, cut off at right) oriented around the odor source (x) by the current (arrow). Inset: trapezoid schematic indicating segments for which dimensions are given in Table 1. Movies in Supplementary Material demonstrate the differences between the two simulation methods.
Slug movements were biased random walks with different navigation strategies (biases) contingent on flow directions, the presence or absence of odor, and past experience of odor. All slugs used OGR in the presence of a detectable odor plume. To test the benefits of the cue-switching hypothesis, I compared three navigational strategies that differed in their post-OGR navigation: (1) continued rheotaxis, (2) switching to unguided crawling initiated along the upstream heading measured when odors were last detected, and (3) switching cues to a magnetic compass sense to actively follow the last upstream direction measured while odors were present. Each post-OGR strategy was tested at five durations, 0 (controls), 1, 2.5, 5, and 10 min, after which slugs stopped until they next detected an odor plume. Initial positions for slugs were arrayed symmetrically around the central odor source on three concentric rings (1, 2, and 3 m) each with eight locations (every 45°around the rings) and four initial headings (0°, 90°, 180°, 270°), totaling 96 slugs. To compare strategies, the minimum distance to the source each slug reached, normalized to its initial distance was averaged across all 96 slugs for each strategy in each current data set. Strategies were compared across all current data sets using arcsine transformed data in an ANOVA (two factors: strategy and duration, full factorial model) followed by Tukey’s post hoc comparisons (α = 0.05) of the marginal strategy means.
Most simulation parameters (Table 1) were either gathered directly from field data or estimated based on field observations of slug movements and dye plumes. The single exception was the odor detection threshold for the slugs. In nature, this parameter will depend on the initial concentration of an odor, the reduction in concentration through turbulent diffusion (molecular diffusion is likely negligible at these scales; Vogel, 1994), and the physiological detection threshold. In the model, these can all be captured in the spread of the detectable odor plume: either the expansion rate of the circles or the angle of the trapezoid used to determine the region containing detectable odor levels. In the second set of simulations, I explored three detectable plume spread angles (Table 1), and found no significant difference in the relative success rates of the different navigation strategy-duration combinations (three factor ANOVA of arcsin transformed normalized minimum distances; full factorial model with plume spread, strategy, and duration; three-way interaction factor: F16, 3915 = 0.289, P = 0.997).
Switching to magnetically guided crawling was consistently the best navigational strategy in the simulations (Figure 4). Slugs that switched to magnetoreception to crawl along the last heading from which odor was detected moved consistently and significantly closer to a navigational target than slugs that stopped navigation, that continued rheotaxis, or that used unguided crawling initiated along the last heading from which odor was detected (ANOVA F14, 1305 = 29.4, P < 0.001 followed by Tukey’s post hoc comparisons of the three strategies).. Moreover, the longer the post-OGR strategies continued after a plume was lost, the greater the relative benefits of switching to a magnetic compass sense. Thus, magnetoreception for 10 min was the best strategy for slugs navigating toward an odor source, and this strategy held the greatest advantage over the other comparable 10 min duration strategies (either continued rheotaxis or unguided crawling). Although crawling without any guidance cue was almost as successful as magnetically guided crawling, post hoc comparisons indicated that, over all post-OGR durations, switching to magnetoreception moved the slugs significantly closer to the odor source. Thus, the best overall strategy for moving as close as possible to an odor source was based on an initial identification and movement toward the source using odor and flow cues, and then, if necessary, switching to a magnetic compass sense to continue locomotion in the direction from which odors were last detected.
Figure 4. Switching to a magnetic compass sense is consistently the best strategy for simulated T. diomedea that lose contact with an odor plume due to flow heading changes. Shown are minimum distances that simulated slugs reached relative to an odor source for each of three strategies invoked over five different durations after an odor plume was no longer detected. Values are means normalized to the initial distance from the source, with standard errors. Strategies: continued rheotaxis (gray), switching to a magnetic compass to crawl along the last heading from which odor was detected (black), and unguided crawling initiated along the last heading from which odor was detected (white). Over all non-zero durations, switching to magnetically guided crawling was consistently the best strategy. The zero duration strategies (which are the same for all three strategies types since, in all three cases, the strategy is strict odor-gated rheotaxis followed by no subsequent strategy) were the worst strategies for finding the odor source.
These simulations indicate that for T. diomedea the probability of successful navigation is theoretically enhanced by switching cues (Figure 4). The slow-moving slugs may be able to circumvent the problem of rapidly changing flows by continuing along the last heading from which odor was detected. This behavior could be guided using a magnetic compass sense, or simply involve unguided crawling initiated along the last heading from which odor was detected. A third option, using an internal reference system to maintain a heading is unlikely due to the reduction in performance resulting from cumulative errors (Cheung et al., 2007). Unguided crawling is theoretically viable only while outside perturbations or internal instabilities in locomotion control do not veer the animals off course. Increasing the random variation in simulated slug headings significantly decreased the performance of unguided crawling relative to magnetically guided crawling (data not shown). In their natural habitat, topography, obstructions (algae, shells, etc.), and buffeting by flow could all reduce the success rate of unguided crawling (Wyeth and Willows, 2006b). The slug heading variation used in the simulations likely underestimates heading variation during unguided crawling since measurements were made during at least some guided behaviors. Thus, the benefits of magnetically guided crawling over unguided crawling are likely greater than the simulation data indicate.
The hypothesis that in certain circumstances animals navigating over short distances can switch to magnetically guided locomotion to improve navigation success rates has theoretical support. In particular, if the primary cue used to locate the navigation target becomes unreliable for movement toward that target, then switching to a magnetic compass sense allows continued movement in the direction of the target without ongoing detection of the target. This idea is intuitively sensible, and simulation results for T. diomedea show that, in at least one case, the relative time courses of primary cue variability and locomotion create a situation where the strategy should be beneficial. Other animals could similarly increase navigation success with this hypothetical strategy, particularly slow-moving animals like the slugs that encounter rapidly changing primary navigational cues. Thus, this strategy should be an option when considering the role of magnetoreception in a species’ navigation behaviors.
The existence of this navigational strategy now needs to be tested in T. diomedea. First, simultaneous measurements of slug crawling headings and the flow they experience while moving toward an odor source need to be compared to determine if the slugs abandon rheotaxis when large flow variations occur. If they do, then the cue-switching hypothesis can be tested by manipulating magnetic fields during such behaviors to determine if slugs are using magnetic guidance to follow a previously established heading. Given the difficulty in recreating the complicated changes in bulk flow that occur in their natural habitat (Wyeth and Willows, 2006b), these experiments are likely best conducted in the field.
The neural circuits for cue-switching could be constructed in two ways: (1) an initial period of navigation using the cue that identifies the target could calibrate navigation with the second cue, requiring no ongoing integration of the two sensory systems, or (2) direct integration of the two sensory systems could correlate the guidance information provided by the different cues. Thus, in the case of T. diomedea, the slugs could either use OGR to move toward prey or mates and at the same time detect crawling direction relative to the earth’s magnetic field, or alternatively they could directly measure the magnetic bearing of flow containing attractive odors. To partially test this, a pulse of odor in flow can be applied to slugs, ensuring the pulse ends before the slugs complete a turn response. If during subsequent crawling a magnetic field manipulation causes a corresponding offset in crawling direction, then the direct integration hypothesis is supported: slugs measure the magnetic headings of odor pulses. However, if the slugs are unaffected by the magnetic change after the odor pulse, then either the slugs measure the magnetic heading while using rheotactic crawling or their magnetic measurement of odor pulse heading takes longer than the animals take to turn into the pulse. Thus, distinguishing between these possibilities is not assured using behavioral experiments, and analysis of the neural circuitry underlying the navigational strategies may be required.
Switching cues to a magnetic compass sense might be beneficial for a number of animals navigating over shorter distances. It seems particularly advantageous for either slow-moving animals in rapidly changing environments (like T. diomedea) or those animals with either poor vision or homogenous visual surroundings, such that visual cues cannot be used to build a spatial representation of the environment. For example, many other slow-moving mollusks and echinoderms track odor plumes (Bousfield, 1978; Cook, 1980; Croll, 1983; Chelazzi et al., 1988; Swenson and McClintock, 1998; Drolet and Himmelman, 2004; Ferner and Weissburg, 2005) and many do so with little available information from vision (due to poor visual ability, low light, or few landmarks) suggesting a magnetic compass sense could be useful in habitats with variable flow directions. Invertebrates with magnetic compass senses implicated in other orientation behaviors (e.g., Arendse, 1978; Ugolini and Pezzani, 1995; Vacha and Soukopova, 2004; Dommer et al., 2008) could also use the magnetic compass in short distance navigation. Finally, switching to a magnetic compass sense could also benefit faster animals, provided the primary navigational cues change even more rapidly. Birds, salmonids, and hymenopteran insects all have known magnetoreceptive abilities (Wiltschko and Wiltschko, 2005; Lohmann et al., 2008; Wajnberg et al., 2010), and alongside any role in long distance navigation, these animals could also switch to a magnetic compass during short distance navigation if their primary navigational cues are especially variable or ephemeral. For example, marine birds tracking odor plumes to find prey (Nevitt et al., 2008), burrows (Bonadonna et al., 2001), or islands (Grubb Jr., 1979) in conditions with variable wind direction could potentially switch to a magnetic compass as the next best sensory option, when visual cues for orientation are limited. Alternatively, many faster animals (insects, frogs, birds etc.) track intermittent acoustic signals (Searcy and Andersson, 1986), and these might also improve navigational success by temporarily switching to a magnetic compass during silent periods. Regardless of how quickly the animals move, the common theme amongst all these speculative possibilities is a potentially unreliable primary cue that changes quickly enough that it cannot be used for the entire short distance navigation behavior.
By contrast, a number of factors reduce the likelihood that animals might switch to a magnetic compass over short distances. If the navigational target is likely to move, then switching cues is unlikely to be beneficial as only the primary cue can identify new target locations. The strategy is also less likely to succeed if the navigator is unable to detect changes in orientation or position relative to the target position (i.e., navigator drift). Note, however, that a magnetic compass sense could be used to detect orientation drift (and a magnetic map sense could detect positional drift), thus allowing the animals to maintain a preferred course. Finally, if an animal can construct a spatial representation of the environment using visual cues (or indeed any other cue) with a higher probability of navigational success, then switching to a magnetic compass sense for short distance navigation will be less likely. Overall, the likelihood that an animal might switch to a magnetic compass will depend on both a magnetic sense as well as the relative benefits of this strategy and all other alternative strategies for short distance navigation.
Importantly, detecting this navigational strategy depends on testing the animals in a situation with unreliable primary cue(s) that will invoke the switch to a magnetic compass sense. Thus, for example, although previous tests have not supported a role for magnetoreception in the wandering albatross’ navigation (Bonadonna et al., 2005), without focusing observations during periods of wind heading variation (if they occur), any differences in navigational behaviors affected by the magnetic manipulations may have been overwhelmed by data collected in periods of steady wind, when a magnetic compass sense may not be used in navigation. Consideration of this hypothesis for any particular animal should begin with measurements of locomotory patterns, the location of navigational targets, and the sensory cues animals use to detect those targets. If situations arise where the animals appear to be abandoning the primary cues and switching to another cue prior to reaching a stationary navigational goal, then magnetic and control manipulations in those situations can test whether a magnetic compass sense plays a role in short distance navigation.
Regardless of whether or not magnetoreception is involved in finding odor sources in T. diomedea, my results indicate that any additional strategy will be an improvement over strict OGR for these animals (Figure 4). The simulations suggest these relatively slow-moving animals encountering relatively rapid flow changes will be hindered in finding odor plume sources because the animals will often lose contact with the plume. Thus, additional strategies for finding an odor source (including switching to a magnetic compass sense) should be considered for these and other animals that encounter flow changes before they can reach an odor source using OGR.
Do the slugs or other animals actually switch to a magnetic compass sense for short periods when other cues are unreliable? In certain cases, particularly for slow-moving animals in rapidly changing environments, the behavior has plausible logical benefits for successful navigation, and the simulation results for T. diomedea navigating in variable flow bear this out. I propose that natural selection is most likely to support sensory integration such that animals are able switch back and forth between all available cues, as well as calibrate different modalities with respect to each other. Animals could then use whatever navigational cues are most reliable at any given moment for movement toward their goal. Thus, I suggest that animals may indeed use a magnetic compass to guide locomotion based on orientation information from other cues over short distances in addition to its role in any long distance navigation behaviors. Testing this hypothesis will require analysis of both the primary navigational cues and animal locomotion relative to those cues to determine if switching cues has potential benefits, as well as subsequent magnetic manipulations to test the role of a magnetic compass sense in the behaviors.
The author declares that the research was conducted in the absence of any commercial or financial relationships that could be construed as a potential conflict of interest.
I thank the National Sciences and Engineering Research Council of Canada and Nova Scotia Health Research Foundation for support, as well as A. O. D. Willows, S. D. Cain, J. A. Murray, O. M. Woodward, C. P. Holmes, and the three reviewers for help that contributed to this manuscript.
The Supplementary Material for this article can be found online at http://www.frontiersin.org/behavioralneuroscience/paper/10.3389/fnbeh.2010.00042/
Arendse, M. C. (1978). Magnetic field detection is distinct from light detection in the invertebrates Tenebrio and Talitrus. Nature 274, 358–362.
Balkovsky, E., and Shraiman, B. I. (2002). Olfactory search at high Reynolds number. Proc. Natl. Acad. Sci. U.S.A. 99, 12589–12593.
Birkeland, C. (1974). Interactions between a sea pen and seven of its predators. Ecol. Monogr. 44, 211–232.
Bonadonna, F., Bajzak, C., Benhamou, S., Igloi, K., Jouventin, P., Lipp, H. P., and Dell’Omo, G. (2005). Orientation in the wandering albatross: interfering with magnetic perception does not affect orientation performance. Proc. R. Soc. Lond., B, Biol. Sci. 272, 489–495.
Bonadonna, F., Spaggiari, J., and Weimerskirch, H. (2001). Could osmotaxis explain the ability of blue petrels to return to their burrows at night? J. Exp. Biol. 204, 1485–1489.
Bousfield, J. D. (1978). Rheotaxis and chemoreception in the freshwater snail Biomphalaria glabrata (Say): estimation of molecular-weights of active factors. Biol. Bull. 154, 361–373.
Chase, R. (1974). The electrophysiology of photoreceptors in the nudibranch mollusc, Tritonia diomedea. J. Exp. Biol. 60, 707–719.
Chelazzi, G., Le Voci, G., and Parpagnoli, D. (1988). Relative importance of airborne odours and trails in the group homing of Limacus flavus (Linnaeus) (Gastropoda, Pulmonata). J. Molluscan Stud. 54, 173–180.
Cheung, A., Zhang, S., Stricker, C., and Srinivasan, M. (2007). Animal navigation: the difficulty of moving in a straight line. Biol. Cybern. 97, 47–61.
Cochran, W. W., Mouritsen, H., and Wikelski, M. (2004). Migrating songbirds recalibrate their magnetic compass daily from twilight cues. Science 304, 405–408.
Cook, A. (1980). Field studies of homing in the pulmonate slug Limax pseudoflavus. J. Molluscan Stud. 46, 100–105.
Deutschlander, M. E., Borland, S. C., and Phillips, J. B. (1999). Extraocular magnetic compass in newts. Nature 400, 324–325.
Dommer, D. H., Gazzolo, P. J., Painter, M. S., and Phillips, J. B. (2008). Magnetic compass orientation by larval Drosophila melanogaster. J. Insect Physiol. 54, 719–726.
Drolet, D., and Himmelman, J. H. (2004). Role of current and prey odour in the displacement behaviour of the sea star Asterias vulgaris. Can. J. Zool. 82, 1547–1553.
Dusenbery, D. B. (1989). Optimal search direction for an animal flying or swimming in a wind or current. J. Chem. Ecol. 15, 2511–2519.
Dusenbery, D. B. (1990). Upwind searching for an odor plume is sometimes optimal. J. Chem. Ecol. 16, 1971–1976.
Ferner, M. C., and Weissburg, M. J. (2005). Slow-moving predatory gastropods track prey odors in fast and turbulent flow. J. Exp. Biol. 208, 809–819.
Goff, M., Salmon, M., and Lohmann, K. J. (1998). Hatchling sea turtles use surface waves to establish a magnetic compass direction. Anim. Behav. 55, 69–77.
Grubb, T. C. Jr. (1979). Olfactory guidance of Leach’s storm petrel to the breeding island. Wilson Bull. 91, 141–143.
Harvey, D. J., Lu, T. F., and Keller, M. A. (2008a). Comparing insect-inspired chemical plume tracking algorithms using a mobile robot. IEEE Trans. Robot. 24, 307–317.
Harvey, D. J., Lu, T. F., and Keller, M. A. (2008b). Effectiveness of insect-inspired chemical plume-tracking algorithms in a shifting wind field. IEEE Trans. Robot. 24, 196–201.
Jeffery, K. J. (2007). Self-localization and the entorhinal–hippocampal system. Curr. Opin. Neurobiol. 17, 684–691.
Johnsen, S., and Lohmann, K. J. (2005). The physics and neurobiology of magnetoreception. Nat. Rev. Neurosci. 6, 703–712.
Landreth, H. F., and Ferguson, D. E. (1967). Newts: sun-compass orientation. Science 158, 1459–1461.
Li, W., Farrell, J. A., and Carde, R. T. (2001). Tracking of fluid-advected odor plumes: strategies inspired by insect orientation to pheromone. Adapt. Behav. 9, 143–170.
Lohmann, K., and Lohmann, C. (1996). Orientation and open-sea navigation in sea turtles. J. Exp. Biol. 199, 73–81.
Lohmann, K. J., Lohmann, C. M. F., and Putman, N. F. (2007). Magnetic maps in animals: nature’s GPS. J. Exp. Biol. 210, 3697–3705.
Lohmann, K. J., Putman, N. F., and Lohmann, C. M. F. (2008). Geomagnetic imprinting: a unifying hypothesis of long-distance natal homing in salmon and sea turtles. Proc. Natl. Acad. Sci. U.S.A. 105, 19096–19101.
Lohmann, K. J., and Willows, A. O. D. (1987). Lunar-modulated geomagnetic orientation by a marine mollusk. Science 235, 331–334.
Lytridis, C., Virk, G. S., Rebour, Y., and Kadar, E. (2001). Odor-based navigational strategies for mobile agent. Adapt. Behav. 9, 171–187.
Moser, E. I., Kropff, E., and Moser, M. B. (2008). Place cells, grid cells, and the brain’s spatial representation system. Annu. Rev. Neurosci. 31, 69–89.
Muheim, R., Moore, F. R., and Phillips, J. B. (2006). Calibration of magnetic and celestial compass cues in migratory birds – a review of cue-conflict experiments. J. Exp. Biol. 209, 2–17.
Müller, M., and Wehner, R. (2007). Wind and sky as compass cues in desert ant navigation. Naturwissenschaften 94, 589–594.
Murray, J. A., Estepp, J., and Cain, S. D. (2006). Advances in the neural bases of orientation and navigation. Integr. Comp. Biol. 46, 871–879.
Nevitt, G. A., Losekoot, M., and Weimerskirch, H. (2008). Evidence for olfactory search in wandering albatross, Diomedea exulans. Proc. Natl. Acad. Sci. U.S.A. 105, 4576–4581.
Pang, S., and Farrell, J. A. (2006). Chemical plume source localization. IEEE Trans. Syst. Man Cybern. B Cybern. 36, 1068–1080.
Pasternak, Z., Bartumeus, F., and Grasso, F. W. (2009). Levy-taxis search strategy. J. Phys. A Math. Theor. 42, 434010.
Phillips, J. B. (1986). Magnetic compass orientation in the eastern red-spotted newt (Notophthalmus viridescens). J. Comp. Physiol. A 158, 103–109.
Sabelis, M. W., and Schippers, P. (1984). Variable wind directions and anemotactic strategies of searching for an odour plume. Oecologia 63, 225–228.
Searcy, W. A., and Andersson, M. (1986). Sexual selection and the evolution of song. Annu. Rev. Ecol. Syst. 17, 507–533.
Swenson, D. P., and McClintock, J. B. (1998). A quantitative assessment of chemically-mediated rheotaxis in the asteroid Coscinasterias tenuispina. Mar. Freshw. Behav. Physiol. 31, 63–80.
Taube, J. S. (2007). The head direction signal: origins and sensory-motor integration. Annu. Rev. Neurosci. 30, 181–207.
Ugolini, A. L. B. E., and Pezzani, A. N. G. E. (1995). Magnetic compass and learning of the Y-axis (sea-land) direction in the marine isopod Idotea baltica basteri. Anim. Behav. 50, 295–300.
Vacha, M., and Soukopova, H. (2004). Magnetic orientation in the mealworm beetle Tenebrio and the effect of light. J. Exp. Biol. 207, 1241–1248.
Vogel, S. (1994). Life in Moving Fluids: The Physical Biology of Flow. Princeton, NJ: Princeton University Press.
Wajnberg, E., Acosta-Avalos, D., Alves, O. C., de Oliveira, J. F., Srygley, R. B., and Esquivel, D. M. S. (2010). Magnetoreception in eusocial insects: an update. J. R. Soc. Interface 7, S207–S225.
Weissburg, M. J., and Dusenbery, D. B. (2002). Behavioral observations and computer simulations of blue crab movement to a chemical source in a controlled turbulent flow. J. Exp. Biol. 205, 3387–3398.
Willows, A. O. D. (1999). Shoreward orientation involving geomagnetic cues in the nudibranch mollusc Tritonia diomedea. Mar. Freshw. Behav. Physiol. 32, 181–192.
Wiltschko, R., Walker, M., and Wiltschko, W. (2000). Sun-compass orientation in homing pigeons: compensation for different rates of change in azimuth? J. Exp. Biol. 203, 889–894.
Wiltschko, W., and Wiltschko, R. (2005). Magnetic orientation and magnetoreception in birds and other animals. J. Comp. Physiol. A 191, 675–693.
Wilzeck, C., Prior, H., and Kelly, D. M. (2009). Geometry and landmark representation by pigeons: evidence for species-differences in the hemispheric organization of spatial information processing? Eur. J. Neurosci. 29, 813–822.
Wilzeck, C., Wiltschko, W., Güntürkün, O., Buschmann, J. U., Wiltschko, R., and Prior, H. (2010). Learning of magnetic compass directions in pigeons. Anim. Cogn. 13, 443–451.
Wyeth, R. C., and Willows, A. O. D. (2006a). Adaptation of underwater video for near-substratum current measurement. Biol. Bull. 211, 101–105.
Wyeth, R. C., and Willows, A. O. D. (2006b). Field behavior of the nudibranch mollusc Tritonia diomedea. Biol. Bull. 210, 81–96.
Wyeth, R. C., and Willows, A. O. D. (2006c). Odours detected by rhinophores mediate orientation to flow in the nudibranch mollusc, Tritonia diomedea. J. Exp. Biol. 209, 1441–1453.
Keywords: navigation, magnetoreception, magnetic compass sense, short distance, simulation, odor-gated rheotaxis, acoustic signaling, Tritonia
Citation: Wyeth RC (2010) Should animals navigating over short distances switch to a magnetic compass sense?. Front. Behav. Neurosci. 4:42. doi: 10.3389/fnbeh.2010.00042
Received: 08 March 2010;
Paper pending published: 22 March 2010;
Accepted: 21 June 2010;
Published online: 30 July 2010
Edited by:
Paul S. Katz, Georgia State University, USAReviewed by:
Ken Lohmann, University of North Carolina at Chapel Hill, USACopyright: © 2010 Wyeth. This is an open-access article subject to an exclusive license agreement between the authors and the Frontiers Research Foundation, which permits unrestricted use, distribution, and reproduction in any medium, provided the original authors and source are credited.
*Correspondence: Russell C. Wyeth, Department of Biology, St. Francis Xavier University, P.O. Box 5000, Antigonish, NS B2G 2W5, Canada. e-mail:cnd5ZXRoQHN0ZnguY2E=
Disclaimer: All claims expressed in this article are solely those of the authors and do not necessarily represent those of their affiliated organizations, or those of the publisher, the editors and the reviewers. Any product that may be evaluated in this article or claim that may be made by its manufacturer is not guaranteed or endorsed by the publisher.
Research integrity at Frontiers
Learn more about the work of our research integrity team to safeguard the quality of each article we publish.