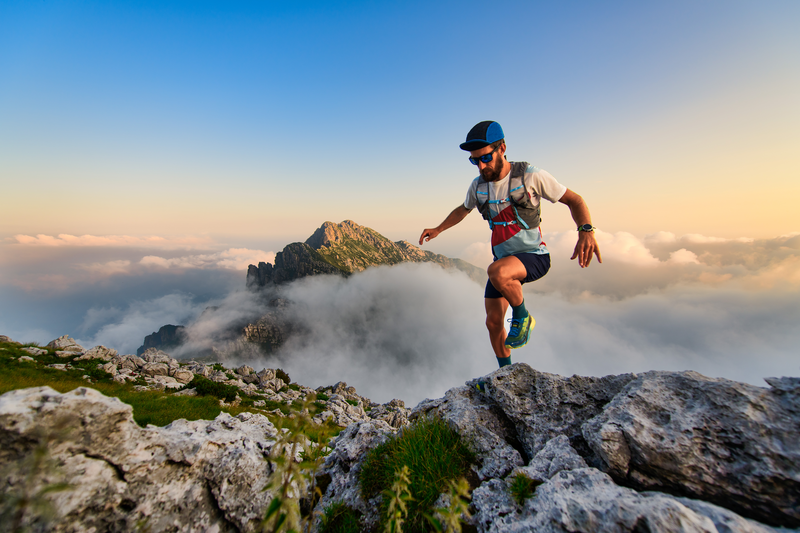
94% of researchers rate our articles as excellent or good
Learn more about the work of our research integrity team to safeguard the quality of each article we publish.
Find out more
PERSPECTIVE article
Front. Behav. Neurosci. , 21 July 2010
Sec. Individual and Social Behaviors
Volume 4 - 2010 | https://doi.org/10.3389/fnbeh.2010.00041
This article is part of the Research Topic Neuroethology View all 23 articles
How the brain mediates behavior is a question relevant to a broad range of disciplines including evolutionary biology, basic neuroscience, psychiatry, and population health. Experiments in animals have traditionally used two distinct approaches to explore brain–behavior relationships; one uses naturally existing behavioral models while the other focuses on the creation and investigation of medically oriented models using existing laboratory-amenable organisms. Scientists using the first approach are often referred to and self identify as “neuroethologists,” while the second category spans a variety of other sub-disciplines but is often referred to broadly as “behavioral neuroscience.” Despite an overall common scientific goal – the elucidation of the neural basis of behavior – members of these two groups often come from different scientific lineages, seek different sources of funding, and make their homes in different departments or colleges. The separation of these groups is also fostered by their attendance at different scientific conferences and publication records that reflect different journal preferences. Bridging this divide represents an opportunity to explore previously unanswerable questions and foster rapid scientific advances. This article explores the reasons for this divide and proposes measures that could help increase technology transfer and communication between these groups, potentially overcoming both physical and ideological gaps.
The traditional process of scientific training encourages exploration of a single or few concepts in depth. The focused passion required for this pursuit is simultaneously exalted and mocked in mass media, but the common view of the scientist with tunnel vision is not completely incorrect. What is not usually portrayed in mass media, though, is the extent to which such tunnel vision can lead to disciplinary and intellectual divides that hinder progress at multiple levels, even among closely related fields. So while scientists have been avidly trying to expand scientific viewpoints with respect to translating laboratory findings into clinical treatments, we have placed less emphasis on trying to translate techniques and findings across similar and seemingly compatible fields of study, such as the study of the brain and behavior.
I have spent most of the last decade of my life working in various lab groups in the United States that study the brain and behavior. In that time, I have realized that, with a few exceptions, scientists who are interested in the brain and behavior can be divided into two groups – neuroethologists who are interested in “natural” behavior and behavioral neuroscientists who investigate behavior from a more medically oriented perspective. Having worked in both of these areas, I am still surprised that, at least in the United States, there isn’t more collaboration across these groups or more people working at the intersection of these two fields.
Neuroethology has a rich history of studying the neural underpinnings of diverse natural behaviors both in the lab and in the field (Hoyle, 1984; Bullock, 1999; Pflüger and Menzel, 1999). Much of the work of neuroethology adopts Krogh’s principle that “for such a large number of problems there will be some animal, or a few such animals, on which it can be most conveniently studied” (Krogh, 1929). Accordingly, neuroethology has made notable contributions to our understanding of the neural basis of many behaviors, including but not limited to sound localization in barn owls (Knudsen, 2002), bat echolocation (Smotherman, 2007), signal jamming in electric fish (Fortune, 2006), stereotyped courtship behaviors (Villella et al., 2008), and the role of central pattern generators in repetitive actions (Selverston, 2005). Historically, this research has not been medically motivated but it is worth noting that it has none-the-less impacted many aspects of health-related science. For instance, our understanding of the basic physiology of the spinal cord as well as insights into spinal cord injury owes much to researchers who have studied motor patterns in invertebrates (reviewed broadly in Pearson, 1993).
A variety of behavioral brain research is also carried out under the broad heading of behavioral neuroscience by scientists who are compelled to examine behavior in the context of the human condition, using animal models as proxies to elucidate mechanisms of human behavior, biology, and disease. Unlike neuroethologists and in contrast to Krogh’s principle, these scientists often use genetic manipulation and other means to create animal models using a limited number of laboratory-amenable organisms. These species have been studied in depth in a variety of biological fields and include mice, rats, fruit flies, zebrafish, and the roundworm, Caenorhabditis elegans. In most cases, these species are not particularly suited to the study of human behavior. Rather, this dependence seems to have resulted from the focus on a few animal models in other health-related fields of biology and the resulting availability of tools, resources, and extensive life-history data for these species.
My goal here is not to argue for or against either type of research as both fields have their notable successes. Rather, I would like to argue that these fields each have reciprocal strengths and that efforts at collaboration across fields have the potential to be extremely fruitful. To support this argument, I will provide a few examples of successes that have occurred via the integration of neuroethology and behavioral neuroscience perspectives. I will also draw on my personal experiences in both fields to highlight factors that limit interactions, and I will discuss a few ways we can foster future interactions. Finally, I will also discuss why I feel we should be tentatively hopeful that future work that draws on both fields of study is both technologically and idealistically realistic to the extent that it may garner more intellectual and, perhaps, monetary support than in the past.
Behavioral neuroscience, with the backing of the National Institutes of Health (NIH), has made tremendous progress in establishing powerful tools and techniques that have been used primarily in a small number of model organisms. The mouse and fly genomes have yielded to the tremendous power of genetic manipulation. The nervous system of C. elegans has been poked and prodded in ways previously unimaginable. Advances in neuronal tract tracing have elucidated primate and rodent brain circuitry. Reproducible and simple rodent behavioral assays have been used to dissect the regional contributions of the brain in behavior. As a result, we now have a tremendous depth of insight in a few, key model systems.
In contrast, we have invested relatively few resources toward understanding behavior in other organisms, which has led to two problems. First, in some instances, researchers have tried to force a square block through a round hole by attempting to study specific behaviors in inadequate or inappropriate models. The temptation to do so is understandable given the tremendous wealth of tools available to manipulate these few species. Second, many behaviors have been neglected for lack of appropriate model species. Fortunately, though, the diverse animal models of neuroethology provide the solutions to both of these problems. Likewise, newer, more broadly applicable technologies that have arisen in behavioral neuroscience will expand the types of questions that can be answered in neuroethological models. Adapting cutting edge technologies to dissect the behavioral diversity of lesser studied species represents a novel opportunity to shed new light on human-relevant behaviors and physiologies. For example, it has been proposed that we try to understand facets of human social behavior by applying such tools to “model social” species, such as songbirds, cichlid fish, social insects, and monogamous and eusocial mammals (Robinson et al., 2008; Holmes et al., 2009).
Neither the tool-rich but organismically limited approach of behavioral neuroscience nor the tool-limited but behaviorally diverse neuroethological approach is right or wrong. Rather, they are in many ways perfect complements of each other. A number of examples now exist that demonstrate the power of working at the intersection between neuroethology and behavioral neuroscience, integrating the strengths of both.
One of the most well developed lines of research at this intersection focuses on the molecular and neurobiological underpinnings of social cognition and bonding. Much of the early work on rodent social affiliation and bonding was performed in rats. Among other findings, researchers demonstrated that oxytocin facilitates the onset of maternal behavior in this species (Fahrbach et al., 1984). However, polygamous laboratory rats and mice do not form selective sociosexual bonds with their mates, like humans do. As such, these species are not suitable for investigating the underpinnings of this behavior. Instead, the research groups of Sue Carter and, later, Tom Insel turned to monogamous prairie voles. Building off of the work in rats that suggested that oxytocin modulates maternal affiliation, they demonstrated that oxytocin is also crucial for the formation of selective partner preferences, a proxy for pair bonding, in female prairie voles (Carter et al., 1995). They also found that oxytocin’s partner molecule, vasopressin, which differs from oxytocin by only two of nine amino acids, modulates pair bonding in male prairie voles (Wang et al., 1999). Thus, findings in behavioral neuroscience inspired new avenues of research in neuroethology, but this was merely the beginning of the interplay between this two disciplines with respect to our understanding of social affiliation and bonding.
Oxytocin and vasopressin are exceptionally conserved across species (Donaldson and Young, 2008), and subsequent comparative work in monogamous and polygamous voles demonstrated that species differences in bonding behaviors are governed by variation in receptor patterns rather than differences in the peptide hormones themselves. Monogamous prairie voles have a vasopressin V1a receptor pattern that differs strikingly from that of polygamous meadow voles, and it was hypothesized that these differences underlie the disparate social behaviors of these species (Young et al., 1997). To demonstrate that alteration of receptor patterns can cause differences in social behavior, Young et al. (1999) isolated the prairie vole V1a receptor gene along with a few kilobases of upstream flanking DNA and inserted it into the mouse genome. The resulting transgenic mice had a V1a receptor pattern that was reminiscent of that of prairie voles, and when infused with vasopressin, the mice demonstrated higher levels of social affiliation. Then, in a complimentary experiment in voles, Lim et al. (2004) altered V1a receptor patterns within the meadow vole brain to make them more prairie-vole-like. Lim used a viral vector to increase V1a receptor levels within the ventral pallidum of polygamous meadow voles, a region with high levels of receptors in prairie voles but low levels in meadow voles. Subsequently, the meadow voles with the altered receptor distribution demonstrated a social preference for their mating partner, a trait typically exhibited by prairie but not meadow voles. These two experiments demonstrate the causal relationship between central V1a receptor patterns and differences in social behavior, and both merge aspects of behavioral neuroscience and neuroethology. In one instance, a hypothesis generated in a neuroethological model was tested using a model system and tools common to behavioral neuroscience. In the other, a tool developed in behavioral neuroscience research was applied to a neuroethological model. In both cases, these mergers resulted in powerful and informative experiments.
In the last few years, human geneticists, psychologists, and psychiatrists have also designed experiments in humans that were inspired by the advances made in rodents with respect to vasopressin, oxytocin, and social behavior (reviewed in Donaldson and Young, 2008; Ebstein et al., 2010). Genetic studies have suggested that variation in the 5′ flanking region of the human V1a receptor gene is associated with a variety of social traits, predictive of relationship quality, and correlates with differences in the neural processing of social cues. Intranasally applied oxytocin has been shown to stimulate trust and other prosocial tendencies, and groups have now begun to explore the possibility of using intranasal oxytocin as a treatment for autism and other neuropsychiatric disorders characterized by deficits in social behavior (Bartz et al., 2008; Guastella et al., 2010).
The trajectory of research on social bonding has continued to make major strides forward through the use of multiple model organisms, both common (mice and rats) and uncommon (prairie voles), and the adoption of the tools of behavioral neuroscience within a neuroethological model. Because of these efforts, we are closer to understanding natural variation in human behavior as well as what processes may be disregulated in the human social brain with respect to various psychiatric disorders.
Social behavior is not the only area in which this approach has proven valuable. Although I will not devote much time to other examples, there are currently a number of lines of research that are or could benefit from combining behavioral neuroscience and neuroethological approaches. For instance, the FoxP2 gene, which encodes a transcription factor, was found to be mutated in a family with a heritable language impairment (Lai et al., 2001). A FoxP2 knockout mouse has highlighted the developmental role of this transcription factor (Vernes and Fisher, 2009) while work with siRNAs targeting this gene in song birds is beginning to shed light on how it may be involved in vocal learning and behaviors (Haesler et al., 2007). These two complimentary approaches will likely each lead to a better understanding of the neurodevelopmental factors important for language-associated traits.
Finally, since neuroethology owes much to the study of invertebrates, I will outline one last example of interplay between neuroethology and behavioral neuroscience within insects. One of the long-standing neuroethological questions with regard to honeybee social behavior centers around the biological basis of the division of labor within the hive (Smith et al., 2008). How does an individual “know” what job it is supposed to perform within the hive, and likewise, what is the underlying biology of individual job transitions, e.g., from hive-bound nurse early in life to nectar/pollen forager later in life? Within D. melanogaster, regulatory variants in the foraging (for) gene lead to individual differences in foraging behavior; flies with higher levels of for forage more actively for food than flies with lower transcript levels (Sokolowski, 2001). By drawing on this work, Ben-Shahar et al. (2002) asked whether the same gene could be involved in different life stages in bees. They demonstrated that upregulation of the foraging (for) gene is associated with the transition from nurse to forager in honey bees and that activation of the pathway regulated by for drives precocious foraging behavior. Thus a gene that modulates differences in feeding behavior in a commonly studied model organism yielded insight into the genetic control of social activity in a “model social” organism (Robinson and Ben-Shahar, 2002). In this instance, behavioral neuroscience in fruit flies inspired a new look at an old neuroethological question in honey bees. One can also imagine that, in the future, hypotheses generated in honey bees will be tested in Drosophila. For the time being, genetic manipulation in honeybees is limited, but candidate genes identified in bees may be manipulated within Drosophila to better understand their role in behavior.
In sum, both neuroethology and behavioral neuroscience have much to gain by capitalizing on each other’s advances. On the one hand, many questions simply cannot be answered using the model systems typically employed by behavioral neuroscientists. On the other hand, neuroethologists will be able to explore natural models in new ways by using techniques and approaches pioneered by behavioral neuroscientists. In moving forward, we must seek to identify ways in which the barriers between these groups of scientists can be broken down.
Pubmed1 is a database of Medline-indexed and other life science articles that plays a dominant role in many neuroscientists’ literature search habits – “pubmedding,” like googling, has become a verb among scientists. Due to its emphasis on biomedical literature and the historical division between biomedical and ecological research, Pubmed has had a limited listing of animal behavioral journals. However, a growing interest in behavioral models of human mental health disorders would suggest that animal behavior findings do deserve a seat that the Pubmed table. And the overseers of Pubmed seem to agree – to an extent. For instance, papers in Animal Behaviour and The American Naturalist are assigned a Pubmed ID, but those in Behavioral Ecology and Sociobiology and Ethology are not (with the exception of articles deemed to be related to population demographics/reproductive health, which are indexed by MEDLINE). This seemingly arbitrary inclusion and exclusion of various behavioral journals does a disservice to the neuroscience community as a whole and limits potential expansion into new animal models.
Inclusion of more behavioral journals in Pubmed will serve to bridge the gap between neuroethology and psychobiology in a number of ways. First, addition of behavioral journals means that anyone searching Pubmed for information on a given behavior will have, within their results, relevant references from both neuroethological and behavioral neuroscience perspectives. Second, by including journals that routinely publish studies performed in less commonly studied animal models, we will increase the chances that NIH reviewers will view such organisms as legitimate biomedical models. Finally, scientists will not have to think twice about whether they will get credit for their work, as they do now when they publish in a journal not currently in the Pubmed database. A quick Pubmed search is often used to identify publications authored by a specific scientist, and when an author publishes in journals outside of Pubmed’s current scope, they risk being unfairly seen as less productive than other scientists. They also risk reduced readership of their articles. Thus, simply expanding the number of behavioral journals available in Pubmed is a relatively easy first step toward expanding the productive interaction between neuroethology and psychobiology. It also seems to be in line with Pubmed’s goal of disseminating life sciences and biomedically relevant findings.
The term “you are what you eat” could just as easily be applied to science. The ideas and approaches we “consume” during training and at conferences largely dictate the types of experiments that we pursue. Thus, attempts to form collaborations and merge neuroethology and behavioral neuroscience will benefit from diverse training opportunities and cross-field conference exposure.
Ph.D. programs in neuroscience and psychology within the U.S. typically provide one to two years of classroom-based education. These programs tend to encompass an excellent and many-faceted examination of the brain, but the extent to which neuroethology is included in the curriculum varies across institutions. Not all schools provide elective courses in neuroethology. We should strive to increase the ubiquitousness of the elective courses in neuroethology, and ensure representation of neuroethological findings in core curricula. It would be considered neglectful to exclude behavioral lessons we have learned from gene knockouts in a graduate level core curriculum and likewise it should be considered ridiculous to exclude key neuroethological findings. A seemless blending of neuroethology and behavioral neuroscience in coursework will stimulate cross-disciplinary approaches, just as new musical genres arise from the fusion of old concepts. In addition, as long as NIH relies on the dual inputs of scientists and medical professionals, it would be advantageous to include lectures on diverse animal models in medical schools. Medical doctors, and especially clinical scientists, may benefit from thinking outside of the confines of traditional behavioral neuroscience.
A few years of graduate training, however, make up only a small portion of most scientific careers. Thus, in order to foster communication and the application of new approaches in neuroethology and behavioral neuroscience, we should examine the segregation that occurs at conferences. While it is a tribute to neuroethology’s success that it is recognized as an independent section at the annual Society for Neuroscience meeting, this partitioning physically separates neuroethologists from behavioral neuroscientists, who typically present their work in other sections of the meeting. Simply by serving as a gathering place for both sub-disciplines simultaneously, however, the Society for Neuroscience meeting serves as a model, for neuroethologists and behavioral neuroscientists often attend different conferences. Neuroethologists typically attend the International Congress of Neuroethology (2010)2 or the Gordon Conference on Neuroethology (2011)3, while behavioral neuroscientists attend a smattering of other conferences, including but not limited to the Genes, Brain, and Behavior Annual Meeting (2010)4, and the International Behavioral Neuroscience Society (IBNS) Meeting (2010)5.
One way to begin bridging this conference divide is to invite speakers from the lesser-represented field to each meeting. At this year’s IBNS meeting in June, the presidential symposium on the search for effective animal models in behavioral neuroscience will attempt to do this (2010b). However, the symposium’s focus on zebrafish, rats and mice does not delve very deeply beyond the traditional confines of behavioral neuroscience (though one talk about hamsters will be included). Likewise, this year’s upcoming International Congress on Neuroethology (2010c) does a particularly good job of integrating human research into the schedule of presentations but fails to attract speakers from the realm of behavioral neuroscience. Providing informative and (hopefully) entertaining cross-field viewpoints at future conferences has the potential to open lines of communication, spur new techniques, and encourage creative scientific avenues.
Finally, in what may prove to be my most-difficult-to-accomplish suggestion, we must identify ways to bridge the funding divide in the United States. In this sense, America may be able to glean something from other funding models – like those of various European countries where a single agency oversees biological research. In the U.S. neuroethological research tends to receive more of its funding from the National Science Foundation (NSF) than NIH. As of February 2010, a grants database search 6 for “neuroethology” at NSF yields 49 awards with this word in the title or abstract while the same search of the NIH RePORT database yields only a single result. Conversely, the underlying medical motivation of behavioral neuroscience makes it more amenable to NIH funding.
This divide in the funding of neuroethological and behavioral neuroscience research has more important implications than which governmental organization is represented on one’s curriculum vitae. Grants are peer reviewed, and, with some exceptions, NIH and NSF often draw on different groups of scientists for their review panels. Because the critical review of a grant is limited by the approaches the reviewers are familiar with, such a system fails to encourage the submission of proposals detailing innovative ways to bridge these fields. One way to overcome this difficulty would be to generate a call for proposals specifically geared toward the development of new animal models at NIH. Another could solicit proposals for technology transfer grants aimed at introducing behavioral neuroscience technologies into less commonly used model species at NSF. Ideally, the review panels for such grants would include a mixture of scientists from neuroethological and behavioral neuroscience backgrounds.
The path of science has been, and will continue to be, influenced by the divergent cultures of different sub-disciplines and by disparate funding sources. Within the past few decades, especially at NIH, there has been a narrowing of focus that emphasizes study of only a few model organisms. As a result, we have been endowed with tremendous depth of understanding and creative techniques for manipulating these organisms, but at the cost of exploring the great diversity of biology. Despite this history, there are reasons to believe that the culture of science generally, including, to an extent, the zeitgeist at its major funding sources, may be moving toward the embrace of more integrated and diverse approaches.
From a technological perspective, advances in genetic tools have opened up the possibility of performing experiments in a wide range of organisms for the first time. For instance, advances with viral vectors provide a highly efficient means to introduce foreign genes into organisms other than mice or flies. Viral-vector mediated gene transfer can be used to modify the properties of a particular neural region or introduce transgenes into the early embryo in order to generate transgenic animals with mutant germlines. To date, scientists have used this and other technologies to generate transgenic prairie voles (Donaldson et al., 2009), zebra finches (Agate et al., 2009), rhesus macaques (Chan et al., 2001), marmosets (Sasaki et al., 2009), cats (Yin et al., 2008), dogs (So Gun et al., 2009), and a variety of agricultural animals (Park, 2007; Whitelaw et al., 2008). Genetic tools are not restricted to answering genetic questions. They can also be used to label specific cell types with a fluorescent marker for use in electrophysiology. Or they can be used to selectively modulate the firing properties of groups of neurons through alteration of neurotransmitter receptors or to introduce foreign receptors that can be modulated by pharmacological agents or even light (Miesenbock, 2009). In short, new technologies now make sophisticated cross-disciplinary studies feasible in less commonly studied organisms.
The recent emergence of comparative genomics as enthusiastically embracing Krogh’s principle serves as a model for how different fields and groups of scientists are congregating around shared resources and questions. Investigation of genomic diversity in humans and animals has applications for studying both human-relevant questions and also exploring hypotheses with respect to evolutionary theory more broadly. As such, genome sequences are the substrate that has brought together groups of scientists that would not normally interact or collaborate. For instance, both human and animal researchers can focus on genomic resources to shed light on mechanisms that generate risk or resilience to cancer as we age. Human association studies may identify gene variants associated with predisposition to cancer while investigation of long-living species with low incidence of tumors, such as naked mole rats, can identify novel genetic mechanisms for avoiding cancer (Seluanov et al., 2009). With a little luck and effort, this model of shared resources may inspire collaboration across other fields, such as neuroethology and behavioral neuroscience.
Finally, there is reason to believe that the dramatic way in which genomicists are embracing diversity is not the only example of government-funded health science branching out to explore new model organisms. The Department of Health and Human Services Interagency Autism Coordinating Committee 2009 Strategic Plan calls for $75 million to be allocated to identifying new models (i.e., animal/cellular) relevant to the study and treatment of autism spectrum disorder (2009). Hopefully, diverse behavioral models will stimulate new ways to approach the study of complex neurological disorders in humans and, as such, will stimulate a renewed respect and interest in the diverse field of neuroethology. In sum, there are now a number of indicators suggesting that researchers in various groups are recognizing the limits of approaches they are familiar with and are ready to listen to insights from other fields.
My intention is not to convince neuroethologists to take on the cause of human health; nor am I seeking to convince behavioral neuroscientists to take up field studies or abandon the study of mice and rats. I would argue, however, that approaching a question from more than one viewpoint is always useful. Neuroethologists and behavioral neuroscientists look at behavioral brain research differently. Both approaches have a rich history and a number of strengths. The question now is how we can best combine those strengths to facilitate the advancement science, and I hope that this article has provided at least a starting point for discussion.
Theodore H. Bullock, one of the founding fathers of neuroethology (Zupanc and Zupanc, 2008), has said, “With its particularly high demands for expertise in several disciplines, neuroethology is typically a tough challenge, but nothing beats it for satisfaction per square idea! (Bullock, 1999)”. I couldn’t agree more that neuroethology has tremendous promise. After all “it is time we knew what neuronal systems are used for! (pg 383, ref Hoyle, 1984)”. Understanding how brain function leads to behavior is going to require a creativity that will employ both behavioral neuroscience and neuroethological approaches. The sooner we can realize ways to bridge the ideological and social divides between these scientific groups, the faster this will happen.
The author declares that the research was conducted in the absence of any commercial or financial relationships that could be construed as a potential conflict of interest.
I would like to thank K. Harper and S. Donaldson for thoughtful review of previous versions of this perspective. Financial assistance was provided by the Robert Wood Johnson Foundation.
The Interagency Autism Coordinating Committee, Department of Health and Human Resources. (2009). Strategic Plan. NIH Publication No. 09-7465. Washington, DC: U.S. Department of Health and Human Services.
Agate, R. J., Scott, B. B., Haripal, B., Lois, C., and Nottebohm, F. (2009). Transgenic songbirds offer an opportunity to develop a genetic model for vocal learning. Proc. Natl. Acad. Sci. U.S.A. 106, 17963–17967.
Bartz, J. A, Hollander, E., Inga DNaRL. (2008). Oxytocin and experimental therapeutics in autism spectrum disorders. Prog. Brain Res. 170, 451–462.
Ben-Shahar, Y., Robichon, A., Sokolowski, M. B., and Robinson, G. E. (2002). Influence of gene action across different time scales on behavior. Science 296, 741–744.
Bullock, T. H. (1999). Neuroethology has pregnant agendas. J. Comp. Physiol. A Neuroethol. Sens. Neural Behav Physiol 185, 291–295.
Carter, C. S., Devries, A. C., and Getz, L. L. (1995). Physiological substrates of mammalian monogamy: the prairie vole model. Neurosci. Biobehav. Rev. 19, 303–314.
Chan, A. W. S., Chong, K. Y., Martinovich, C., Simerly, C., and Schatten, G. (2001). Transgenic monkeys produced by retroviral gene transfer into mature oocytes. Science 291, 309–312.
Donaldson, Z. R., Yang, S.-H., Chan, A. W. S., and Young, L. J. (2009). Production of germline transgenic prairie voles (Microtus ochrogaster) using lentiviral vectors. Biol. Reprod. 81, 1189–1195.
Donaldson, Z. R., and Young, L. J. (2008). Oxytocin, vasopressin, and the neurogenetics of sociality. Science 322, 900–904.
Ebstein, R.P., Israel, S., Chew, S. H., Zhong, S., and Knafo, A. (2010). Genetics of human social behavior. Neuron 65, 831–844.
Fahrbach, S. E., Morrell, J. I., and Pfaff, D. W. (1984). Oxytocin induction of short-latency maternal behavior in nulliparous, estrogen-primed female rats. Horm Behav 18, 267–286.
Guastella, A. J., Einfeld, S. L., Gray, K. M., Rinehart, N. J., Tonge, B. J., Lambert, T. J., and Hickie, I. B. (2010). Intranasal oxytocin improves emotion recognition for youth with autism spectrum disorders. Biol. Psychiatry 67, 692–694.
Haesler, S., Rochefort, C., Georgi, B., Licznerski, P., Osten, P., and Scharff, C. (2007). Incomplete and inaccurate vocal imitation after knockdown of FoxP2 in songbird basal ganglia nucleus area X. PLoS Biol. 5, e321. doi:10.1371/journal.pbio.0050321.
Holmes, M. M., Goldman, B. D., Goldman, S. L., Seney, M. L., and Forger, N. G. (2009). Neuroendocrinology and sexual differentiation in eusocial mammals. Front. Neuroendocrinol. 30, 519–533.
Hong, S. G., Kim, M. K., Jang, G., Oh, H. J., Park, J. E., Kang, J. T., Koo, O. J., Kim, T., Kwon, M. S., Koo, B. C., Ra, J. C., Kim, D. Y., Ko, C., and Lee, B. C. (2009). Generation of red fluorescent protein transgenic dogs. Genesis 47, 314–322.
Knudsen, E. I. (2002). Instructed learning in the auditory localization pathway of the barn owl. Nature 417, 322–328.
Lai, C. S. L., Fisher, S. E., Hurst, J. A., Vargha-Khadem, F., and Monaco, A. P. (2001). A forkhead-domain gene is mutated in a severe speech and language disorder. Nature 413, 519–523.
Lim, M. M., Wang, Z., Olazabal, D. E., Ren, X., Terwilliger, E. F., and Young, L. J. (2004). Enhanced partner preference in a promiscuous species by manipulating the expression of a single gene. Nature 429, 754–757.
Park, F. (2007). Lentiviral vectors: are they the future of animal transgenesis? Physiol. Genomics 31, 159–173.
Pearson, K. G. (1993). Common principles of motor control in vertebrates and invertebrates. Annu. Rev. Neurosci. 16, 265–297.
Pflüger, H. J., and Menzel, R. (1999). Neuroethology, its roots and future. J. Comp. Physiol. A Neuroethol. Sens. Neural Behav Physiol 185, 389–392.
Robinson, G. E., and Ben-Shahar, Y. (2002). Social behavior and comparative genomics: new genes or new gene regulation? Genes Brain Behav 1, 197–203.
Robinson, G. E., Fernald, R. D., Clayton, D. F. (2008). Genes and social behavior. Science 322, 896–900.
Sasaki, E., Suemizu, H., Shimada, A., Hanazawa, K., Oiwa, R., Kamioka, M., et al. (2009). Generation of transgenic non-human primates with germline transmission. Nature 459, 523–527.
Seluanov, A., Hine, C., Azpurua, J., Feigenson, M., Bozzella, M., Mao, Z., et al. (2009). Hypersensitivity to contact inhibition provides a clue to cancer resistance of naked mole-rat. Proc. Natl. Acad. Sci. U.S.A. 106, 19352–19357.
Selverston, A. I. (2005). A neural infrastructure for rhythmic motor patterns. Cell. Mol. Neurobiol. 25, 223–244.
Smith, C. R., Toth, A. L., Suarez, A. V., Robinson, G. E. (2008). Genetic and genomic analyses of the division of labour in insect societies. Nat. Rev. Genet. 9, 735–748.
Smotherman, M. S. (2007). Sensory feedback control of mammalian vocalizations. Behav. Brain Res. 182, 315–326.
Vernes, S. C., and Fisher, S. E. (2009). Unravelling neurogenetic networks implicated in developmental language disorders. Biochem. Soc. Trans. 37, 1263–1269.
Villella, A., Hall, J. C., and Jeffrey, C. H. (2008). “Neurogenetics of courtship and mating in Drosophila, Chapter 3,” in Advances in Genetics, Vol. 62, ed. M. B. Sokolowski (Burlington, MA: Academic Press), 67–184.
Wang, Z., Young, L. J., De Vries, G. Jr., and Insel, T. R. (1999). Voles and vasopressin: a review of molecular, cellular, and behavioral studies of pair bonding and paternal behaviors. Prog. Brain Res., 119, 483–499.
Whitelaw, C. B., Lillico, S. G., King, T. (2008). Production of transgenic farm animals by viral vector-mediated gene transfer. Reprod. Domest. Anim. 43(Suppl. 2), 355–358.
Yin, X. J., Lee, H. S., Yu, X. F., Choi, E., Koo, B. C., Kwon, M. S., et al. (2008). Generation of cloned transgenic cats expressing red fluorescence protein. Biol. Reprod. 78, 425–431.
Young, L., Winslow, J., Nilsen, R., and Insel, T. (1997). Species differences in V1a receptor gene expression in monogamous and nonmonogamous voles: behavioral consequences. Behav. Neurosci. 111, 599–605.
Young, L. J., Nilsen, R., Waymire, K. G., MacGregor, G. R., and Insel, T. R. (1999). Increased affiliative response to vasopressin in mice expressing the V1a receptor from a monogamous vole. Nature 400, 766–768.
Keywords: neuroethology, behavioral neuroscience, academic divides
Citation: Donaldson ZR (2010) We’re the same…but different: addressing academic divides in the study of brain and behavior. Front. Behav. Neurosci. 4:41. doi: 10.3389/fnbeh.2010.00041
Received: 03 March 2010;
Paper pending published: 15 April 2010;
Accepted: 20 June 2010;
Published online: 21 July 2010
Edited by:
William Kristan, University of California San Diego, USAReviewed by:
Ronald M. Harris-Warrick, Cornell University, USACopyright: © 2010 Donaldson. This is an open-access article subject to an exclusive license agreement between the authors and the Frontiers Research Foundation, which permits unrestricted use, distribution, and reproduction in any medium, provided the original authors and source are credited.
*Correspondence: Zoe R. Donaldson, 406 Schermerhorn Extn, 1190 Amsterdam Avenue, New York, NY 10027, USA. e-mail:em9lLmRvbmFsZHNvbkBnbWFpbC5jb20=
Disclaimer: All claims expressed in this article are solely those of the authors and do not necessarily represent those of their affiliated organizations, or those of the publisher, the editors and the reviewers. Any product that may be evaluated in this article or claim that may be made by its manufacturer is not guaranteed or endorsed by the publisher.
Research integrity at Frontiers
Learn more about the work of our research integrity team to safeguard the quality of each article we publish.