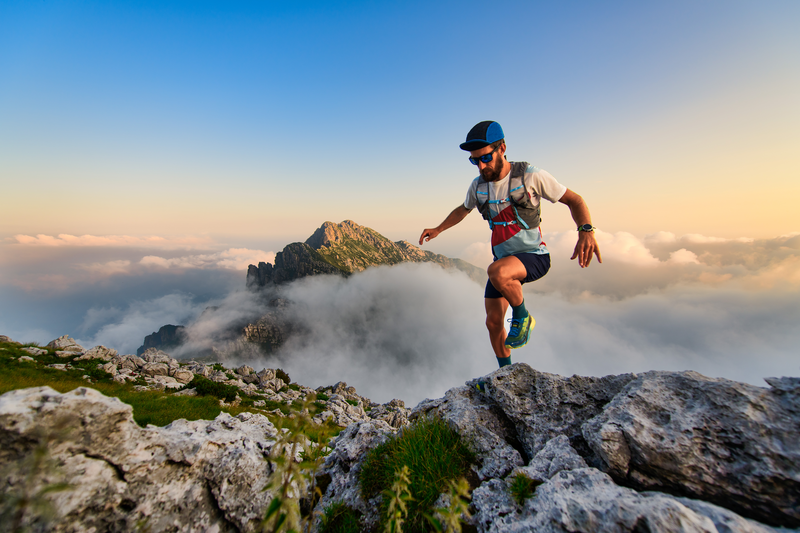
94% of researchers rate our articles as excellent or good
Learn more about the work of our research integrity team to safeguard the quality of each article we publish.
Find out more
REVIEW article
Front. Nanotechnol. , 12 February 2025
Sec. Biomedical Nanotechnology
Volume 7 - 2025 | https://doi.org/10.3389/fnano.2025.1521131
Cancer is a leading cause of death worldwide, with an estimated 20 million new cancer cases and 9.7 million cancer deaths worldwide in 2022. Immunotherapy provides innovative strategies among the most groundbreaking developments in cancer treatment. Cancer vaccines, as a form of immunotherapy, have a great prospect to help patients resistant to other standard-of-care immunotherapies. On the other hand, the various properties of nanomaterials play an essential role in the collecting, maturation, and activation of the immune system. Cancer vaccines based on nanomaterials (also called nanovaccines) can be specifically delivered to target tissues and cells through nanocarriers and nanoplatforms, thereby improving efficacy, extending the duration of antitumor immunity, and minimizing side effects. This paper reviewed the research progress of some nanovaccines in cancer immunotherapy, including polymer nanoparticle vaccine, liposome nanoparticle vaccine, cell-based nanoparticle vaccine, inorganic nanoparticle vaccine, adjuvant and auxiliary work. We believe that polymer nanoparticle-based nanovaccines have the most widespread applications currently, while liposome nanovaccines using mRNA are expected to see greater development in the future. We also think that nanovaccines can play a great role in cancer prevention and treatment, especially in prolonging the life span of patients.
In 2023, the global incidence of new cancer cases hit 18.1 million, with most cancer patients undergoing conventional treatments like surgery, chemotherapy, radiation therapy, immunotherapy, targeted therapy, or hormone therapy (Zhuang et al., 2022). Chemotherapy, while widely used, suffers from drawbacks such as its lack of specificity and cytotoxic effects (Yang et al., 2022). Nanomedicine has spurred the creation of innovative cancer treatment strategies, encompassing diagnosis and prevention (Gao, 2020). Immunotherapy represents one of these treatments, aiming to educate immune cells to specifically identify and eliminate cancer cells (Mao et al., 2021). Based on immune memory, the immune system can defend against tumor recurrence and metastasis for a long time. Recent studies and statistical data taken from the patients showed that immunotherapy responses raised concerns about the clinical success of cancer immunotherapy) (Bezbaruah et al., 2022).
Cancer vaccination represents a promising addition to conventional cancer treatment. These vaccines are engineered to instruct a patient’s immune system to identify and eradicate cancer cells. By doing so, they can elicit a targeted antitumor immune response that specifically destroys tumor cells, with minimal side effects, while also fostering immune memory, which offers prolonged protection against tumor recurrence (Liang et al., 2022). Nanovaccines offer numerous benefits, including the ability to concurrently deliver antigens and adjuvants, facilitate phagocytosis and processing by antigen-presenting cells (APCs), and enhance the adaptive immune response (Wu and Lv, 2024). NPs significantly improved the stability of nanovaccines, protected antigens from degradation by intracellular proteases, and maintained antigen release.
Tumor immunotherapy aims to stimulate the immune system to produce an antitumor immune response to prevent the appearance, growth and recurrence of tumors. Common immunotherapy techniques include monoclonal antibody technique and tumor vaccine technique. Tumor vaccines can induce local tumor regression by effectively delivering tumor-specific antigens to stimulate APCs and tumor-specific T lymphocytes.
Tumor cell vaccines harbor a multitude of antigens, yet their capacity to bolster tumor immunity necessitates substantial refinement (Lu et al., 2020). Immunotherapy has emerged as a novel benchmark in cancer treatment (Gurunathan et al., 2024). However, its benefits have been limited to a minority of patients, and it faces immunotoxicity challenges that constrain its broader therapeutic potential (Zhang J. et al., 2024). With the development of nanomedicine and immunotherapy, nanoengineering technology has become a new research field, aiming to give full play to the therapeutic potential of immunotherapy. It is believed that the defects of existing cancer immunotherapy can be effectively alleviated by vigorously developing nano-immunotherapy. Nano vaccines aim to meet the unmet needs in cancer immunotherapy, particularly for drug-resistant tumor types. Current treatments often face limitations due to tumor resistance. Nanovaccines, with the help of nanotechnology, present tumor antigens more effectively, activating a stronger immune response to target resistant cancer cells (Han et al., 2022).
Moreover, AI-driven neoantigen prediction is significant. It identifies neoantigens from genomic data. In nanovaccine design, it selects tumor-specific neoantigens, optimizes vaccine efficacy, tailors immune responses, and monitors and adapts the vaccine, enhancing its potential in cancer treatment.
Nanovaccines encompass various types, such as polymer nanoparticles (e.g., PLGA, PEG), liposomes, inorganic nanoparticles (e.g., silica, gold), cell-based nanoparticles (e.g., exosomes), and virus-like particles (VLPs), which provide diverse delivery mechanisms for antigens and adjuvants. Among them, polymer nanovaccines excel in controlled release, while liposome nanovaccines are proficient in mRNA delivery. Inorganic nanoparticles enable theranostic applications. Cell-based nanovaccines and VLPs leverage natural immune interactions to trigger personalized and highly immunogenic immune responses. Adjuvants (e.g., TLR agonists) and auxiliary systems can overcome immune suppression and further enhance vaccine efficacy. Each type of nanovaccine possesses unique advantages, such as biocompatibility and targeting. However, they also face challenges in terms of stability, toxicity, and scalability, highlighting the necessity for continuous innovation in cancer immunotherapy and infectious disease prevention.
In this paper, nanovaccines are classified as follows: polymer nanoparticle vaccine, inorganic nanoparticle vaccine, liposome nanoparticle vaccine, cell-based nanoparticle vaccine. Finally, auxiliary work and adjuvants of nanovaccines are also done. The specific classification diagram is shown in (Figure 1).
The cancer immune pathway is intricate. Cancer cell-derived neoantigens activate T cells. The STING pathway, initiated by cytosolic DNA, induces interferon production, amplifying the immune response. It activates dendritic cells and promotes cytokine release. However, tumors can evade through checkpoint proteins. Comprehending this pathway, especially STING’s role, is vital for devising effective nanovaccine strategies to counter cancer by augmenting the immune system’s anti-tumor activity. The cGAS-STING signaling pathway is shown in (Figure 2).
Figure 2. The cGAS-STING signaling pathway (Zhang M. et al., 2024).
A variety of polymers have been used to prepare nanoparticles, such as (Poly Lactate-glycolic Acid, PLGA), (Poly Lactic Acid, PLA), (poly 2-oxazoline, POx), (Polyethylene Glycol, PEG), and natural polysaccharides. Among them, PLGA nanoparticles have been the most widely studied because of their excellent safety and biodegradability, and have been approved by the FDA as vaccine carriers.
Firstly, the nanoparticles were prepared based on PLGA. In 2022, Gou prepared a nanocarrier (PLGA/STING@EPBM) with a particle size of 157.5 ± 1.9 nm and a potential of −21.1 ± 2.0 mV, showing an obvious core-shell structure that can be stable for 4 days in vitro (Gou, 2022). In vitro and in vivo phagocytosis experiments showed that PLGA/STING@EPBM could be efficiently absorbed by Clec9a + DC. Nanometer carrier with DC incubation find PLGA/STING @ EPBM rise significantly in DC interferon stimulation gene (ISG) expression level, enhance the antigen cross presented and antigen specific T cell activation. In 2023, Xiong used PLGA to carry CpG-ODN immune adjuvant and coated ovarian cancer cell membrane on the surface of PLGA-CPG nanospheres to construct PLGA-CpG@ID8-M nanotumor vaccine, and explored the regulation of macrophage polarization in tumor microenvironment and anti-tumor effects of nanotumor vaccine (Xiong, 2023). The mechanism of nano tumor vaccine regulating M1 polarization of macrophages was also discussed. In 2023, Liu et al. induced immunogenic cell death (ICD) in TC-1 tumor cells using a combination of mitoxantrone and curcumin. Subsequently, they coated chitosan (CS)-modified PLGA nanoparticles with the HPV16 E744-62 peptide on the prepared ICD tumor cell membrane (Liu et al., 2023a). These nanoparticles, along with adenosine triphosphate (ATP), were encapsulated within sodium alginate (ALG) hydrogel. The immune properties and therapeutic efficacy of this system were assessed both in vitro and in vivo. Sun et al. also designed a nanoscale cancer vaccine based on PLGA in 2023, which efficiently encapsulated engineered tumor cell membrane proteins and mRNA and acoustic sensitized agent chlorine e6 (Ce6) (Sun et al., 2023). After subcutaneous injection, nanovaccines can be effectively delivered to APCs in lymph nodes. In apc, the coated cell membrane and RNA from engineered cells, similar to metastatic cells, are interferentially spliced to provide neoantigens of metastatic cancer in advance, and the processing results are shown in (Figure 3) (Sun et al., 2023).
Figure 3. (A) Representative confocal microscope images of the distribution of DiO labeled nanoparticles in the lymph nodes and other main organs 12 h after subcutaneous injection. (B) The preparation steps of cancer vaccine and schematic of vaccine delivery in lymph node and intercellular antigen presentation (Sun et al., 2023).
Then there are the natural polysaccharides. In 2024, Liang constructed a self-assembled nanovaccine that could co-encapsulate neoantigens Adpgk and CpG ODN (Liang, 2024). After that, a small molecule chitosan that is soluble and can activate cGAS-STING signaling pathway was screened, and then Carboxymethyl dextran (CMD) and CS “double adjuvant” nanovaccine system containing CpG ODN and Adpgk was constructed. In 2021, Pei modified chitosan with catechol groups to obtain CSC/CaP antigen delivery carrier with sheet structure. X-ray powder diffraction (XRD) results show that the nanosheets are composed of CaHPO4·2H2O crystals, which can be efficiently taken up by Dendritic cells (DCs) through macropinytosis (Pei, 2021). Intracellular lysosomes were labeled with Lysotracker Red and Lamp-1(CD107a), and the nanosheets were observed to promote lysosomal escape of antigens. The upregulation of co-stimulatory molecules on DCs surface and the secretion of Helper T cell 1 (Th1) cytokines suggested that the nanosheets could promote the activation and maturation of DCs. Kaveh-Samani et al. cloned DNA vaccine by chemical method in 2024 and verified it by PCR and restriction endonuclease (Kaveh-Samani et al., 2024). The immunogenicity and immunoprotection of the vaccine were evaluated in BALB/c mice. The study showed that prophylactic alginate/Pinot-Ureh nanovaccines injected directly into the stomach can effectively trigger a powerful immune response to prevent H. pylori infection in mice (Kaveh-Samani et al., 2024).
PEG is rarely used in the preparation of nanoparticles. In 2023, Liu et al. designed an experiment. Each neoantigen peptide was combined with amphiphilic lipid 1, 2-distearoyl-SN-glycerol-3-phosphate ethanolamine-N [hydroxy-succinyl (polyethylene glycol)] (DSPE-PEG2000-NHS) pairs to form nanoparticles, and the last nine nanoparticles were mixed to form PNVAC (Liu et al., 2023b). Molecular weight characterization using NMR and MALDI-TOF mass spectrometry validated the successful conjugation of the peptide. Dynamic light scattering analysis revealed that the particle size of PNVAC predominantly ranges between 20–30 nm and exhibits a slight negative charge. The amphiphilic DSPEPEG2000-polypeptide self-assembled with DSPE as the core and PEG2000-peptide as the shell. The size and polydispersity index of PNVAC remained stable for 96 h across temperatures of 4, 25, and 37°C. Using MFC-9-based nanoparticles as an example, over 80% of the antigenic peptides were released within 96 h at 25°C, with the specific effects illustrated in (Figure 4) (Liu et al., 2023a).
Figure 4. Computed tomography (CT) scans showed that after three doses of anti-PD-1 blockade immunotherapy, multiple metastatic lymph nodes shrank, but ascites appeared (Liu et al., 2023b).
POx is also used to prepare nanoparticles. In 2024, Chen et al. demonstrated in vivo that POx coupled with OVA257-264 peptide through matrix metalloproteinase 2 (MMP-2) sensitive junctions could efficiently and selectively modify tumor cells with OVA peptide (Chen et al., 2024). Then, a pox-based nanovaccine containing OVA257-264 peptide was designed to induce OVA-specific T cell responses.
Finally, the preparation of nanoparticles using polylactic acid is studied. Carina Peres et al. reported the preparation and characterization of a biodegradable multifunctional vaccine based PLA nanoparticles in (Peres et al., 2024). The vaccine is capable of co-delivering cancer-associated antigens, Toll-like receptor (TLR) ligands, and small interfering RNA (siRNA) targeting TGF-β1 (siTGF-β1) within the breast in vivo. They demonstrated the synergistic anti-tumor effects of alpha OX40 and this NP-based combination vaccine in two different mouse models of breast cancer (TNBC and luminal B-like breast cancer) to halt tumor growth and improve overall survival, com-position shown in (Figure 5) (Peres et al., 2024).
Figure 5. Multifunctional polymeric nanoparticles (NP) delivery of combinations of immune modulators promotes dendritic cell (DC) activation and maturation. (A) Schematic representation of the NP. (B) Atomic force microscopy (AFM) images of empty NP showing spherical shape. (C) Cell viability of DC and breast tumor cells after incubation of NP for 48 h. (D) Rho-labeled NP internalization by murine immature DC. (E) Confocal images (Z-stack and maximum projection) of murine immature DC (JAWSII) after 4, 12, and 24 h of incubation. (F) Percentage of DC internalizing Cy5-labeled NP, 17 h after C57BL/6J mice immunization with NP entrapping antigen and adjuvants. (G) MFI of activated migratory DC present in the lymph nodes recovered 17 h after mice immunization with PBS, a ntigen, and adjuvants in solution or Cy5-labeled NP entrapping antigen and adjuvants.
While significant advances in nanoparticle vaccine research continue, several challenges remain, including the difficulty of repeatedly synthesizing nanoparticles with consistency and desirability. The lack of a basic understanding of how the physical properties of nanoparticles interact with cells, tissues, and even entire biological systems. Therefore, reasonably designed nanoparticle vaccines with ideal performance and efficacy are becoming increasingly important. In the past 20 years, multifunctional inorganic nanomaterials have been rapidly developed in biomedical fields such as imaging, tumor therapy, and drug delivery. The recent studies have found that the existence of inorganic nanoparticle vaccines is conducive to the recruitment and maturation of immune cells, thereby activating the function of the immune system.
At present, inorganic nanoparticle vaccines are mainly composed of nanoparticles based on mesoporous silica, carbon point, manganese dioxide, and metal compounds. Among them, metal compound nanoparticles have been studied widely.
Firstly, the preparation of nanoparticles using mesoporous silica has been discussed. In 2023, Huang developed a diagnostic and treatment platform based on combining Mesoporous silica nanoparticles (MSNs) and liquid Perfluoro hexane (PFH) (Huang, 2023). PFH is encapsulated in the pores of MSNs by negative pressure method, and Polydopamine (PDA) is coated on the surface of MSNs while effectively embedding Indocyanine green using a near-infrared fluorescent dye approved by FDA. Finally, PEG-FA was chemically connected in the PDA layer to obtain a nano diagnosis and treatment system of PTT-PDT combination therapy guided by FL/US dual-mode imaging MSNs-PFH@PDA-ICG-PEG-FA. Both in vivo and in vitro experiments showed that the system could significantly improve the stability of ICG and the deficiency of ICG being easily photobleached. It could make ICG stay in the tumor longer and achieve an effective temperature increase after multiple rounds of 808 nm laser irradiation. The system can also effectively enhance the uptake efficiency of ICG by tumor cells and the production of intracellular (ROS), and effectively kill tumor cells under the dual action of hyperthermia and ROS.
Carbon points are also often used to construct nanoparticles. In 2024, Liu prepared chiral carbon points (L/DCDs) by using citric acid (CA) and L/D-glutamic acid (L/D-Glu) as raw materials, and took ovalbumin (OVA) as model antigen, through the co-assembly of L/D-CDs and OVA (Liu, 2024). Chiral nanovaccines (L/D-OVA) were prepared. L/D-OVA can be effectively taken up by mouse bone marrowderived dendritic cells (BMDCs), induce maturation of BMDCs, crosspresent antigens to T cells, and inhibit the growth of B16-OVA melanoma. The developed nanovaccine can be effectively absorbed by dendritic cells (DC2.4), promote the maturation of DC cells, cross-present antigens to T cells, and is specific for B16F10 melanoma and CT26 colon cancer, and significantly inhibit tumor growth. The work illustrates the prospect of CDs as a multifunctional vector to deliver antigens for optimal immunotherapy effects.
As for the study on the preparation of nanoparticles using manganese dioxide, in 2023, Gu synthesized antigenic biomimetic manganese dioxide nanoparticles MnO2@OVA using model antigen ovalbumin (OVA) as a template through simulated biomoneralization reaction (Gu, 2023). In order to realize the targeted regulation of dendritic cells and macrophages in immunosuppressive microenvironment, a targeted nano vaccine (Man-MnO2@OVA) was constructed with mannose (Man) functionalized MnO2@OVA. The composition, structure and properties were characterized by transmission electron microscopy, X-ray crystal diffraction, X-ray photoelectricity spectroscopy, and dynamic light scattering. Secondly, at the cellular level, the ability of Man-MnO2@OVA nano-vaccine to stimulate phenotypic polarization of macrophages, activates dendritic cell maturation, and X-ray sensitization. The activation of immune-related signaling pathways was investigated by cell imaging, cytometry, cytokine monitoring and Western Blot. At in vivo level, the efficacy of Man-MnO2@OVA nanovaccine assisted radioimmunotherapy was investigated by tumor growth curve and remote metastasis inhibition effect. Flow cytometry, cytokine monitoring, and proteomics of splenic, lymphoid, and tumor cell suspension were used to explore the radio immuno microenvironmental regulatory mechanism of Man-MnO2@OVA nanovaccines. Finally, it was proved that the bionic mineralized Man-MnO2@OVA nanovaccine can target and regulate the tumor inhibitory radioimmune microenvironment and enhance the antitumor effect of X-ray. To achieve combined radioimmune therapy and inhibit the remote metastasis of tumor.
Finally, the most widely based research on metal compound nanoparticles is conducted. In 2024, Qiu introduced polyphenol Tannic acid (TA) into the model antigen OVA through a simple Schiff base reaction, utilizing the coordination properties of polyphenols and metal ions (Qiu, 2024). The FeIII-TA-OVA nanovaccine was prepared. Based on IDO inhibitor 1-methyltryptophan (1-MT), FeIII-TA-OVA@1-MT nanovaccine was obtained. The successful construction of FeIII-TA-OVA@1-MT nanovaccine was verified by infrared, Raman, and circular dichroism characterization methods. The photo-thermal properties and active oxygen generation capacity of FeIII-TA-OVA@1-MT nanovaccines were investigated. In addition, the cytotoxicity, tumor cell killing effect, promotion of macrophage polarization, and dendritic cell maturation of FeIII-TA-OVA@1-MT nanovaccine were investigated in vitro. Based on the good potential of photo heat-immunotherapy in vitro, the melanoma model was selected for further in vivo experiments. The inhibitory effect of FeIII-TA-OVA@1-MT nanovaccine combined photo heat-immunotherapy on tumor growth was investigated in a single tumor mouse model. At the same time, the polarization of macrophages and the immune effect of 1-MT were investigated. Based on the single tumor mouse experiment, a double tumor mouse experiment was carried out to investigate the inhibitory effect of FeIII-TA-OVA@1-MT nano vaccine combined photo heat-immunotherapy on the distal tumor, and to explore the immune activation in the distal tumor. A mouse lung metastasis model was established according to the characteristics of melanoma metastasis. The study investigated the ability of combination therapy to inhibit tumor metastasis and demonstrated that combination therapy can establish long term effective immune memory in vivo. Liu prepared Gold nanocages (AuNCs/SV) loaded with Simvastatin (SV) adjuvant in 2022 (Liu, 2022). The AuNCs/SV were coated with Folic acid (FA) -modified Temperature-sensitive liposomes (TSL), and a tumor-targeting nanodrug delivery system (FATSL/AuNCs/SV) was constructed. When the drug delivery system efficiently reaches the tumor site, Photothermal therapy (PTT) generated by AuNCs under 808 nm laser irradiation can cause the external FA-TSL to peel off and cause the tumor cell injury to release TDPAs. Then, the AuNCs/SV kernel of the drug delivery system can capture TDPAs through Au-S bond and other forces to form a personalized in-situ recombinant nanovaccine (AuNCs/SV/TDPAs). The recombinant vaccine has a particle size of about 50 nm and facilitates drainage to lymph nodes. It is noteworthy that the vasodilation caused by hyperthermia can promote the drainage of recombinant nanovaccines to the lymph nodes, which can further enhance the body’s immune response.
In 2024, Du et al. constructed an intelligent reactive bimetallic nanovaccine, which enhanced the efficacy of the cGAS-STING pathway by inducing immunogenic cell death through pyro death (Du et al., 2024). The nanovaccine was enhanced with sorafenib (SOR) and further modified with MIL-100 (Fe). In this system, Fe³⁺, SOR, and Mn2⁺ were released into the tumor, facilitated by the tumor microenvironment (TME). Subsequently, Fe³⁺ synergistically interacts with SOR-induced immunogenic pyroptosis (through both classical and non-classical signaling pathways), leading to the substantial release of immunogenic factors. These factors promote the maturation of dendritic cells (DCs) and expose double-stranded DNA (dsDNA). The exposed dsDNA, along with Mn2⁺, coactivates the cGAS-STING pathway, triggering the release of type I interferon and further enhancing DC maturation. Additionally, Mn2⁺, when associated with T1 magnetic resonance imaging (MRI), enables the visual assessment of the intelligent response function of the nanovaccine. This approach offers a promising model for the treatment of HCC, as illustrated in (Figure 6) (Du et al., 2024).
Figure 6. Schematic illustration of the synthesis (A) and mechanism (B) of the nanovaccine in liver cancer immunotherapy.
In the same year, Wang et al. designed an “in situ nanovaccine” Au/CuNDs-R848 for image-guided photothermal therapy (PTT)/chemo dynamic therapy (CDT) synergistic therapy to trigger a dual immunomodulatory effect on TNBC (Wang et al., 2024). On one hand, Au/CuNDs-R848, serving as both a photothermal agent and a nanoenzyme, is anticipated to achieve photothermal therapy (PTT) and photothermal-enhanced chemo dynamic therapy for the treatment of primary triple-negative breast cancer (TNBC) tumors. Simultaneously, the released antigens and damage-associated molecular patterns (DAMPs) stimulate the maturation of dendritic cells (DCs) and the infiltration of T lymphocytes. Consequently, Au/CuNDs-R848 functions as an “in situ nanovaccine,” enhancing the immunogenicity of TNBC through the induction of ICD. On the other hand, these nanovaccines inhibit myeloid-derived suppressor cells (MDSCs), thereby reversing the immunosuppressive microenvironment. By dual immune regulation, the “cold tumor” is transformed into a “hot tumor,” not only implementing the therapeutic strategy of “turning foes into friends” but also boosting the immunotherapy of metastatic TNBC. Furthermore, Au/CuNDs-R848 acts as an excellent nanoprobe, enabling high-resolution near-infrared fluorescence and computed tomography for the precise visualization of TNBC (Wang et al., 2024).
The study of liposomes in the immune system dates back to the early 1970s. Liposome nanoparticle vaccine mainly includes high-density lipoprotein (HDL), phospholipid derivatives, tetraether liposome, Choline 1, 2-dioleyl-SN-glycerol-3-ethyl phosphate (EDOPC), which can effectively improve the immunogenicity of tumor antigens. Liposomes can be modified with antigen-presenting cell-specific ligands for specific immune cells or subcellular organelles.
The first is the study of using EDOPC to prepare nanoparticles. In 2023, Chen found that mRNA nanovaccines effectively stimulated BMDC to produce TNF-α, IL-1β, IFN-β, CCL5, and other inflammation-promoting cytokines (Chen, 2023). It was found that Stimulator of Interferon Genes (STING) pathway was significantly correlated with IFN-β expression, while TNF-α expression was regulated by Mitochondrial antiviral signaling proteins (MAVS) pathway. The cationic lipid EDOPC plays an important role, but it loses its function after being replaced by DOTAP and DOPC. Western blot results showed obvious phosphorylation of P65 in the downstream IRF7NF-κB pathway of TLR7 pathway and TBK1 and IRF3 in the downstream STING pathway, which proved that mRNA nanovaccines could activate the corresponding pathways. In STING-KO mice, compared with WT mice, mRNA nanovaccine-related DC maturation level was significantly inhibited, and T cell activation level was also reduced. The number of antigen specific IFN-γ+ cells was also significantly reduced in STING-KO mice. In MAVS-KO mice, compared with WT mice, the proportion of OVA antigen-specific CD8+T cells and CD44+CD8+ memory T cells did not show significant changes, and the number of antigen-specific IFN-γ+ cells was similar to that of WT mice. In the MC38-OVA tumor model of STING-KO mice, the therapeutic effect of mRNA nanovaccine was significantly inhibited, and the proportion of tumor antigen-specific CD8+T cells was reduced. Therefore, the STING pathway found in study is of great significance for the therapeutic effect of mRNA nanovaccines. It is concluded that mRNA nanovaccines can efficiently transport target mRNA in DC, combined with self-adjuvant effect, produce specific tumor cell clearing effect, and effectively inhibit tumor growth in mouse MC38-OVA colon cancer model. It was also found that STING pathway has an important influence on the immunotherapy effect of mRNA nanovaccines by regulating the secretion of IFN-β. Cationic lipid EDOPC plays a decisive role in the activation of STING pathway.
The use of phospholipid derivatives to prepare nanoparticles is the most extensive research. In 2024, Baljon et al. used a scalable limited impact jet mixing method to reproducibly prepare vaccines, loading multiple physiochemically distinct peptide antigens and multiple vaccine adjuvants into ph-responsive monodisperse saclike nanoparticles with a diameter of less than 100 nm (Baljon et al., 2024). The synergistic adjuvants cGAMP and mono phosphoryl lipid A (MPLA) were incorporated into nanocarriers to elicit a robust and tailored innate immune response, thereby boosting the immunogenicity of the peptide antigen. By combining these two adjuvants within the nanovaccine, they aimed to synergistically augment the expression of dendritic cell costimulatory markers, the production of proinflammatory cytokines, and the cross presentation of peptide antigens. Furthermore, the nanoparticle delivery system enhanced the uptake of peptide antigens by dendritic cells, leading to increased accumulation in lymph nodes and draining lymph nodes. Consequently, in various mouse models, the codelivery of peptide antigen, cGAMP, and MPLA via nanoparticles significantly enhanced the response of antigen specific CD8+ T cells and delayed tumor growth, as illustrated in (Figure 7) (Baljon et al., 2024).
Figure 7. Schematic representation of coloaded nanoparticle vaccine platform promoting MPLA delivery to cell surface and endosome (Baljon et al., 2024).
In 2022, Chu et al. developed a neoantigen nanovaccine using liposomes with lymph node targeting properties, which consisted of neoantigens, amphiphilic liposomes, and adjuvant Montanide™ ISA 51 (Chu et al., 2022). A small animal imaging system and immunofluorescence staining technique were employed to determine the distribution of nanovaccines. To assess the antitumor effect, a mouse melanoma subcutaneous tumor resection model was established. Flow cytometry was utilized to analyze the immune response triggered by the nanovaccines. The research revealed that nanovaccines can infiltrate lymph nodes and be absorbed by CD11c+ dendritic cells (DCs), facilitating DC maturation. Following treatment with the neoantigen nanovaccine, the average recurrence time was prolonged from 11 days to 16 days, and the median survival time was extended by 7.5 days compared to the control group (NS group). The nanovaccine boosted neoantigen specific T cells to 10 times the level of the free vaccine and upregulated Th1 cytokines such as IFN-γ and TNF-α. The splenic lymphocyte antitumor activity in the nanovaccine group was notably stronger than in other groups. However, under the immune pressure exerted by the neoantigen nanovaccines, some immunosuppressive cells or molecules in the tumor microenvironment were found to be upregulated, including Tregs and PD-L1. The therapeutic efficacy of the neoantigen nanovaccine combined with anti-PD1 antibody or Treg inhibitory peptide P60 was superior to that of monotherapy. In 2020, Yu et al. dried the mixture of DMPC (3 μmol) and CO (0.2 μmol) in chloroform (300 μL, AR grade) under nitrogen to form a uniform lipid film (Yu et al., 2020). Subsequently, α-melittin (0.19 μmol, a hybrid polypeptide) and α-polypeptide (0.87 μmol, a simulated apoa1 polypeptide) were dissolved in 1 mL of PBS and double distilled water to create a nanovaccine. Alpha-melittin-NPS was observed to markedly enhance lymph node (LN) accumulation and APC activation compared to free melittin, leading to a 3.6-fold increase in the antigen-specific CD8+ T cell response. Furthermore, alpha-melittin-NPS significantly suppressed the growth of both primary and distant tumors in bilateral ventral B16F10 tumor models, with inhibition rates of 95% and 92%, respectively. Consequently, α-melittin-NPS can serve as an effective LN-targeted whole-cell nanovaccine to induce a systemic antitumor response (Yu et al., 2020).
Tetraether liposomes can also be used to prepare nanoparticles. In 2020, Karimi et al. found that human body is often exposed to archaea lipids, so archaea are safe and suitable for human body as a natural adjuvant (Karimi et al., 2020). One of the significant challenges faced by many new adjuvant technologies, particularly those designed to enhance an effective immune response, is their potential toxicity, especially at high doses. Archaea, however, are nontoxic and possess the ability to activate the immune system. The synthesis of tether liposomes incorporating HPV 16 genes L1, E6, and E7 in conjunction with paleosomes represents an exceptional strategy to stimulate an immune response against existing HPV-16-associated malignancies and holds considerable promise for the development of therapeutic vaccines. This treatment approach offers new avenues for enhancing and rationally designing effective and safe vaccines capable of eliciting T-cell immunity against cancer and intracellular infections. Paleosomes have been introduced as highly valuable vectors for vaccines. They not only appropriately present antigens to immunoactive cells and improve antigen stability but also possess the ability to overcome biological barriers, such as skin and mucous membranes. Additionally, they provide controlled and slow release of antigens. Beyond their ability to induce a strong immune response through adjuvant properties, archaeosome-based vaccines also exhibit features that are crucial for improving modern vaccine formulations, as illustrated in (Figure 8) (Karimi et al., 2020).
Figure 8. Fluorescence microscopic observation of pIRES-L1/E6/E7 expression. HEK293T cells were transfected with pIRES-L1/E6/E7 and Mock plasmids (Karimi et al., 2020). (A) PIRES-E6/E7/L1. (B) Mock plasmid.
As a substance composed of liposome and protein, HDL has unique physiological functions and can be used as a component of nanoparticles. Liu et al. developed a nanovaccine simulating HDL in 2024, which has good lymphatic targeting ability and can effectively trigger antitumor immunity (Liu et al., 2024). Lipid nanoparticles are used that are coloaded with specific cancer cell membranes containing tumor-associated antigen collections and immune adjuvants. They also explored the impact of nanoparticles on the uptake efficiency of lymphatic-targeting and dendritic cells. Optimized nanovaccines facilitate the codelivery of antigens and adjuvants to lymph nodes and maintain antigen presentation in dendritic cells, resulting in long-term immune surveillance. Their findings revealed that in a mouse model, the nanovaccine could suppress tumor formation and growth through immunosuppression and enhance the therapeutic effect of checkpoint inhibitors on high dry melanoma (Liu et al., 2024).
The integration of mRNA vaccine technology and nanovaccines is a significant development. mRNA vaccines’ COVID-19 success stems from encoding antigens. In nanovaccines, mRNA is encased in nanoparticles. These protect mRNA from degradation and enhance uptake into APCs. Like in COVID-19 vaccines, it may enable quick development and better targeting. Nanoparticles can also act as adjuvants, strengthening immunogenicity for broader disease protection. However, concerns about long-term safety and formulation optimization persist. Overall, this combination is a promising frontier in vaccinology, despite challenges (Jogdeo et al., 2024).
Cell-based nanoparticles, such as extracellular vesicles (EVs) and outer membrane vesicles (OMVs), are derived from natural sources like dendritic cells, tumor cells, or bacteria. These nanoparticles inherently carry antigens, immunomodulatory molecules, and genetic material, making them highly effective in stimulating immune responses. Recent advancements in EV-based cancer vaccines have highlighted their unique ability to deliver native cargo, including tumor-specific antigens, miRNAs, and proteins, directly to immune cells. This native cargo delivery enhances antigen presentation and promotes robust anti-tumor immune responses. EVs also exhibit low immunogenicity and high biocompatibility, reducing the risk of adverse effects. Additionally, EVs can be engineered to display specific antigens or immune-stimulating molecules, further enhancing their therapeutic potential. These features make EV-based vaccines a promising platform for personalized cancer immunotherapy, particularly in overcoming immune suppression in the tumor microenvironment.
Many researchers have used ovalbumin as an antigen for the preparation of nanovaccines. In 2023, Wang prepared a vaccine containing only neoantigen peptides derived from gastric cancer cells (DP-Ag-MFC-NEs) and a combined vaccine containing both neoantigen peptides and CD73 inhibitor nanoparticles (DP-Ag-MFC-NEs + antiCD73) using ovalbumin, respectively + CpG) to observe the therapeutic effect of vaccine on tumor-bearing mice (Wang, 2024). It was found that a 10-week-old 615 mouse could be isolated and purified to an average of about 1.5 × 106 NEs, the cell activity was above 95%, and the purity was above 90%. Compared with the control group, DP-Ag-MFC-NEs could significantly inhibit subcutaneous tumor growth in mice, and some mice were cured (CR). The proportion of CD4 and CD8 positive cells in mouse lymph nodes was significantly increased (P < 0.05). CR mice were inoculated with MFC gastric cancer cells again, and no mice had tumor recurrence. The combined vaccine was effective and had the highest survival rate in mice (75%, P = 0.038). Neutrophil vaccine loaded with neoantigen peptides derived from gastric cancer cells and CD73 inhibitors had no apparent toxicity to mice and could significantly inhibit tumor growth and even cure mice. In 2023, Zhang included CpG ODN and Ovalbumin as adjuvant in tumor vaccine, and used model antigen in tumor vaccines (Zhang, 2023). They modified G250 and Cy5.5 onto Neutravidin (nAvidin) and self-assembled Cy5.5-Navidin-G250 nanoparticles, possibly because the enhanced hydrophobicity of modified avidin led to its self-assembly into nanoparticles. According to the interaction of avidin and biotin, biotinylated OVA and CpG ODN were then loaded into Cy5.5-nAvidin-G250 nanoparticles to construct a new nano tumor vaccine Cy5.5-Navidin-G250-OVA-CPG. Coincidentally, in the same year, Zhang et al. developed an antigen-self-assembly nanovaccine, which induced self-assembly by simple acryloyl antigen modification (Zhang et al., 2023). Furthermore, the integration or absorption of dendritic cell-targeting mannose monomers and the mevalonate pathway inhibitor Zoledronic acid (Zol) onto the nanoparticle (designated as MEAO-Z) was employed to amplify the immune response. The synthesized nanovaccines, with a diameter of approximately 70 nm, are distinguished by their efficient lymph node delivery, robust dendritic cell uptake, enhancement of costimulatory molecule expression, and superior antigen cross-presentation. Owing to these benefits, MEAO-Z elicited significantly higher serum antibody titers, cytotoxic T lymphocyte immune responses, and IFN-γ secretion compared to free antigens and adjuvants. In vivo studies demonstrated that MEAO-Z effectively suppressed the growth of, EG7-OVA tumors and extended the survival time of tumor-bearing mice (Zhang et al., 2023).
Heat shock proteins can also be used to prepare nanovaccines. For example, in 2024, Wang successfully constructed mRNA nanoparticles that can target Hepa1-6 cells, which can translate the target protein GPC3127-136-HSP70 in Hepa1-6 cells and secrete it into the extracellular system (Wang, 2024). The target protein secreted into the extracellular can specifically target DC2.4 cells, which provides experimental and theoretical basis for designing mRNA vaccine.
Dendritic cell lysates can also be used as a component of nanovaccines. In 2023, Liu used genetic engineering technology and cell membrane surface display technology to build a recombinant adenovirus system with specific epitope cell membrane directed expression that is widely applicable to many types of tumors (Liu, 2023). By infecting immature DC with the constructed recombinant virus, the target antigen is overexpressed and anchored to the outer surface of cell membrane in the form of natural structure. Endogenous antigen introduction mediated by viral infection realized controllable regulation of epitope presentation based on MHCI-like pathways, and displayed the MHC1-Ag complex on DC cell membrane. During this process, DC under-went differentiation and maturation, and the expression of costimulatory molecules and chemokines on the membrane surface was upregulated. Based on the previous work, they carried out further nanocrystallization of the above engineered DC to prepare a nanovesicle vaccine with complete antigen presenting functional unit. The regular morphology, particle size and good stability through a variety of characterization methods were verified.
Tumor cell lysates are also often used in the preparation of nanovaccines. In 2022, Shi et al. prepared a nano-encapsulated CPG adjuvant and combined it with the cell lysate of colon cancer cell line (MC38) to form nanovaccines (Shi et al., 2022). Mouse bone marrow-derived dendritic cells (BMDC) were cultured in vitro and BMDC activation experiments were performed in 4 groups, namely, PBS group, empty nanoparticle group, dissolved CPG group and CPG-loaded nanoparticle (CNP) group. The C57BL/6 colon cancer tumor bearing mouse model inoculated with MC38 tumor cells was established. When the tumor volume increased to 50 mm3, the mouse model was divided into four immune groups, namely, PBS group, MC38 lysate group, CNP group and vaccine group formed by MC38 lysate supplemented with CNP. Subcutaneous immunization was performed every 7 days for a total of 3 times. The proportion of T lymphocytes in peripheral blood, spleen and inguinal lymph nodes and the content of TNF⁃α and IFN⁃γ in serum were detected after the last immunization 3d. Results After CNP treatment, BMDC maturity ratio increased (P < 0.01) and secreted a large number of cytokines (P < 0.0001). The tumor volume of MC38 tumor lysate nanovaccine was significantly smaller than that of other groups (P < 0.0001), and the proportion of peripheral blood T lymphocytes, spleen T lymphocytes and lymph nodes T lymphocytes was significantly higher Increased (P < 0.05), serum cytokine content was also significantly increased (P < 0.05). It was found that MC38 tumor lysate nanovaccine can effectively activate dendritic cells (DC), induce tumor-specific cellular immune response, and play a significant anti-tumor effect. In 2021, Yang focused on the combined use of Endo alginate hydrogels and tumor cell lysate nanoparticle vaccines (Yang, 2021). In vitro cytotoxicity tests showed that no alginate hydrogel (AH), alginate hydrogel and polydopamine nanoparticles were empty materials (AH/PDA), or the combination of endo alginate hydrogel and tumor cell lysate nanoparticle vaccine (EH/TCLN) was toxic to mouse embryonic fibroblasts NIH3T3. ELISA results showed that EH42/TCLN treatment dramatically changed the level of intracellular cytokines, suggesting that it caused the change of tumor immunosuppressive microenvironment in mice. Flow cytometry detected the proportion of T cell subpopulations in spleen, lymph nodes and tumors. It was found that EH42/TCLN combined treatment significantly increased the proportion of CD4+ and CD8+T cells, promoted the maturation of DC cells, effectively promoted antigen presentation, and improved the killing activity of cytotoxic T lymphocytes (CTL) in spleen against MC-38 cells (76. 5%). Confocal microscopy showed that the tumor microvascular density (MVD) of EH42/TCLN group was the lowest (0.57), and the number of apoptotic cells was the highest (74.2%). In survival experiments of tumor-bearing mice, EH42/TCLN treatment resulted in complete regression of tumors in most tumor-bearing mice, with a survival rate of 62.5% after 62 days of tumor inoculation, compared with 0% in the other groups. The surviving mice continued to survive free of tumor after being reinoculated with tumor cells on the opposite side. All results showed that the combination therapy not only significantly inhibited tumor angiogenesis, but also significantly enhanced the anti-tumor immune response, showing a high degree of synergy and proving the inevitable link between active immunotherapy and anti-angiogenesis therapy.
Serum proteins can also be used as antigens to prepare nanovaccines. In 2024, Zheng et al. developed STING-activated peptide nanovaccines using human serum albumin (HSA) and biodegradable MnO2. These nanovaccines enhance the stability and immunogenicity of antigenic peptides, facilitating their uptake by dendritic cells (DCs) (Zheng et al., 2024). At the same time, Mn2+ degraded by nanovaccines can activate STING pathway and further promote dendritic cell maturation. The nanovaccine prepared by this method can effectively mediate the immune response of t cells, thus playing a role in tumor prevention and treatment, and its model is shown in (Figure 9) (Zheng et al., 2024).
Figure 9. Schematic illustration of the preparation of STING-activating peptide nanovaccine and its application in antitumor immunotherapy (Zheng et al., 2024).
However, Nanovaccines have some limitations. Potential cytotoxicity exists as nanomaterials may harm healthy cells. Immune evasion is a problem since tumors can avoid the immune response triggered by nanovaccines. Also, in certain tumor types like pancreatic cancer, their clinical efficacy is limited due to the harsh microenvironment and tumor heterogeneity. These issues require further research to overcome for better cancer treatment.
Adjuvants play a crucial role in eliciting a strong immune response in vaccinated individuals by activating the immune system, enhancing immunity, and directing immune responses. Also, adjuvants and auxiliary systems are vital in overcoming nanovaccine limitations. For cytotoxicity, they enhance stability and control release to reduce harm to healthy cells. Against immune evasion, they activate the innate immune system and can include checkpoint inhibitors. In tumors with limited efficacy, they can be tailored for better penetration and targeting, thus improving nanovaccine performance. This is particularly important for subunit antigens, which are typically weakly immunogenic. Adjuvants are commonly categorized based on their primary mechanism of action into vaccine delivery systems and immune-stimulating molecular adjuvants. The latter includes TLR agonists, STING agonists, costimulatory ligands, and cytokines that activate immune cells, such as APCs, to boost antigen-specific immune responses. When adjuvants and antigens are encapsulated within nanoparticles, they are protected from degradation by proteases, phosphatases, and nucleases. However, adjuvants can sometimes lead to systemic toxicity, resulting in symptoms such as fever, diarrhea, nausea, and fatigue in vaccinated individuals. The toxicity of NPs can be shielded by the encapsulation of NPS by adjuvants and antigens. The auxiliary roles of nanovaccines refers to the related work not in the nanovaccine microstructure or clinical trials. The auxiliary work aims to improve the availability, convenience, and success rate of nanovaccines.
In terms of adjuvants, in 2021, Zhang synthesized iodized oil nano emulsion adjuvant, prepared iodized oil nanovaccine, and conducted characterization and performance testing of the preparation (Zhang, 2021). An oil-in-water iodized oil nano emulsion was synthesized using the emulsification method, and an iodized oil nanovaccine was prepared by inserting a cholesterol-modified antigen on its surface. The particle size distribution of the nanovaccine was detected by particle size analyzer. The morphology of the nanoparticles in the emulsion were detected by transmission electron microscopy (TEM) to determine the stability and uniformity of the nanoparticles. Enzyme-linked immunosorbent assay (ELISA) was used to quantitatively detect the antigen release in iodized oil nanovaccines by calculating the antigen linking rate and the antigen content in iodized oil nanovaccines. The results provide a reference for the dose used in subsequent experiments. ICG was used to label the adjuvant in iodized oil, and Cy5.5 was used to label the antigen. The stability and targeting in vivo were tested using a small animal imaging system (IVIS) after double-fluorescent vaccine labeling. It was found that iodized oil nanovaccine could promote the maturation and differentiation of DCs, and upregulate the expression of T cell costimulatory molecules CD80 and CD86. In the same year, Guo used BCG protein as an adjuvant to stimulate immunity (Guo, 2021). The work is based on connecting BCG protein and tumor antigen peptide to linear heparin molecules through the amide bond to obtain an irregularly round assembled vaccine with a diameter of about 20 nm. The assembled vaccine has no apparent toxicity, and can be efficiently ingested by dendritic cells, activate dendritic cells, activate T cells, and cause specific cytotoxic T cell responses against prostate cancer cells. After repeated intervention with the assembled vaccine, the tumor growth of prostatic cancer bearing mice can be slowed down, and the survival time of mice can be prolonged.
In 2023, Su et al. synthesized two chiral polypeptide constructs: poly (L-phenylalanine) -block poly (L-lysine) (PL-K) and poly (L-phenylalanine) -block poly (D-lysine) (PD-K). These were employed as nano adjuvants within nanovaccines designed for the prevention and treatment of cancer (Su et al., 2023). Amphiphilic polypeptides spontaneously form nanoparticles with a diameter of approximately 30 nm when subjected to ultrasound-assisted dissolution in phosphate-buffered saline. The nanovaccines PL-K-OVA and PD-K-OVA were created by combining PL-K or PD-K with the model antigen chicken egg albumin (OVA), achieving nearly 100% loading efficiency. In comparison to PL-K-OVA, PD-K-OVA demonstrated superior efficacy in promoting dendritic cell maturation, antigen cross-presentation, and adaptive immune responses. Notably, it effectively prevented and treated the B16-OVA melanoma model expressed in ova. When combined with PD-K-OVA and anti-PD-1 antibody, the tumor inhibition rate could reach 94.9% or even 97.0%. Consequently, chiral polypeptide nanoparticles emerge as simple, efficient, and broadly applicable nano-adjuvants for various nanovaccines (Su et al., 2023).
In 2020, Ni et al. engineered a dual-adjuvant neoantigen nanovaccine (banNV) designed to simultaneously deliver a peptide neoantigen Adpgk along with two adjuvants: the Toll-like receptor (TLR) 7/8 agonist R848 and the TLR9 agonist CpG. This innovative approach was aimed at enhancing cancer immunotherapy (Ni et al., 2020). Specifically, banNVs are fabricated through a process involving the synthesis of tandem CpG via nanotemplates, nanopolycondensation with cationic polypeptides, and subsequent physical loading with hydrophobic R848 and Adpgk. This method significantly enhances the immunogenicity of neoantigens by effectively co-delivering neoantigens and double synergistic adjuvants, while also reducing acute systemic toxicity. BanNVs were found to sensitize the immune checkpoint (Immune checkpoints are regulatory mechanisms in the immune system, like “brakes.” CTLA-4 and PD-1/PD-L1 are important ones. They control immune responses; dysregulation can lead to autoimmune diseases or cancer evasion. Immunotherapies target these checkpoints but may cause side effects.) programmed death receptor 1 (PD-1) on T cells. When combined with anti-PD-1 (aPD-1), BanNVs elicited a robust therapeutic response, resulting in the complete regression of 70% of neoantigenspecific tumors without recurrence, as illustrated in (Figure 10) (Ni et al., 2020).
Figure 10. Schematic depiction of the development of banNVs to potentiate neoantigen immunogenicity for personalized cancer immunotherapy. (A) BanNVs were self-assembled in three steps. (B) BanNVs enable efficient codelivery of adjuvants and antigen peptides to LNs, inducing DC mutation by adjuvants, facilitating antigen cross presentation, and profoundly expanding the repertoires of neoantigen-specific CD8+ effector T cells.
In 2023, Xie et al. developed a new peptide-based tumor nanovaccine that can enhance antigen presentation and induce effective antitumor immunity (Xie C. et al., 2023). The adjuvant capabilities of FK-13, a core peptide derived from antimicrobial peptides, were investigated, leading to the creation of FK-33, a fusion peptide incorporating a tumor antigen epitope. L-phenylalanine poly (ester amide) (PH-PEA), specifically 8p4, has been identified as an effective delivery vector for the fusion peptide FK-33. Intriguingly, in vivo administration of 8p4 + FK-33 nanoparticles (8FNs) stimulated dendritic cell activation in lymph nodes and elicited a robust tumor antigen-specific CD8+ T cell response. The nanovaccine 8FNs demonstrated significant therapeutic and preventive effects against tumor growth in situ, effectively curbing tumor metastasis, and markedly extending the survival time of tumor-bearing mice. Furthermore, 8FNs can bind to various tumor antigens and exhibit a synergistic therapeutic effect when combined with antiprogrammed cell death protein 1 (PD-1) treatment (Xie Y.-J. et al., 2023).As for the auxiliary work on nanovaccines, in 2023, Xin et al. introduced a novel neoantigen prediction model called NUCC, aimed at filtering out a greater number of neoantigens to optimize the PNVAC platform (Xin et al., 2024). The predictive tool showcased superior neoantigen prediction performance compared to other tools, not only on two independent epitope datasets but also on a completely new epitope dataset that we constructed from scratch. This dataset comprised 25 patients with advanced gastric cancer and 150 candidate mutant peptides, 13 of which were confirmed as neoantigens through in vitro immunogenicity tests. In 2024, Cao et al. used the Web of Science Core Collection (WOSCC) to search relevant literature on antitumor nanoimmunotherapy since its establishment (Cao et al., 2024). Statistical analysis and visualization were conducted using Bibliometrix, VOSviewer, CiteSpace, GraphPad Prism, and Excel. The evaluation covered the annual output, active institutions, core journals, main authors, keywords, major countries, key literature, and impact factors of selected journals. A total of 443 relevant studies were included from 2004 to 2022, reflecting an annual growth rate of 16.85%. The countries with the highest number of published papers are China and the United States. Core journals such as Journal of Controlled Release, Biomaterials, Acta Biomaterialia, Theranostics, Advanced Materials, and ACS Nano have published high-quality literature on the latest advancements in the field. Articles focusing on dendritic cells and drug delivery constitute a significant portion of this domain. Keywords like regulatory T cells, tumor microenvironment, immune checkpoint blocking, drug delivery, photodynamic therapy, photothermal therapy, and tumor-associated macrophages are prominent topics in current research. Dendritic cells, vaccines, and T cells are anticipated to be popular and emerging research areas in the future. The combination therapy of nanomaterials with antitumor immunotherapy, known as antitumor nano-immunotherapy, has garnered increasing attention. This field is evolving from simple to complex, and from phenotype to mechanism-based research.
In 2024, Sun et al. used a multifunctional dendrite nanoplatform to coordinate ICDs and immunoagonists to develop a minimalist “in situ” tumor vaccine for enhanced chemical immunotherapy (Sun et al., 2024). The ICD inducer Adriamycin and the immune antagonist unmethylated CpG were combined with the cationic dendritic polyamine (PAMAM). DOX was wrapped in the hydrophobic core and CpG was adsorbed on the PAMAM positive surface. The constructed DOX@PAMAM/CpG nanoparticles (DPC NP) have suitable physical properties and can be used as a minimal “in situ” tumor vaccine nanoplatform. Through convenient intratumoral injection, tumor cells can be endowed with good accumulation and internalization ability with minimal systemic toxicity. With the assistance of CpG immunoagonists, DPC NP further triggers tumor’s strong ICD and stimulates comprehensive antitumor immunity.
The studies listed in this paper cover the treatment of a variety of tumors, of which breast cancer related nanovaccines are the main, because breast cancer treatment is currently a hot direction. However, it seems that treating digestive tract cancer will have a good prospect in the future. The incidence of digestive tract cancer is on the rise in all populations, and drugs targeting digestive tract cancer will be further developed in the future, so this paper believes that nanovaccines will also be further studied. Currently, there are four vaccines approved by the FDA that can assist in preventing cancer, include Cervarix®, Gardasil®, Gardasil-9®, Hepatitis B (HBV) vaccine (HEPLISAV-B®). In addition, there are two FDA-approved vaccines for the treatment of cancer, include Bacillus Calmette-Guérin (BCG) and Sipuleucel-T (Provenge®). Nanovaccines use nanoparticles to carry tumor antigens, accurately present and activate immune cells. They have strong specificity and great potential for personalization. However, the safety of nanomaterials needs to be studied. Traditional cancer vaccines such as BCG are mostly composed of attenuated or inactivated pathogens. They non-specifically activate immune cells to indirectly fight tumors. They have weak precision, limited applicable cancer types, and there is a risk of infection when used by people with low immunity. However, a lot of nanovaccines have not yet been approved, as shown in (Figure 11).
Cancer vaccines have the potential (Guo et al., 2021) to significantly enhance immunosuppression and stimulate cancer-specific immune responses (Zhang et al., 2022). These attributes may aid in addressing the limitations of current immunotherapies (Lin et al., 2024), such as low response rates and immune-related side effects (Xie C. et al., 2023). The potential of nanovaccines lies in their ability to improve the efficacy of cancer treatment vaccines (Bezbaruah et al., 2022). By effectively codelivering polyvalent molecular antigens and adjuvants into lymphoid tissue and immune cells (Koyande et al., 2022), nanovaccines can substantially enhance the immunogenicity of molecular vaccines (Thangam et al., 2021), thereby boosting antigen specific adaptive immune responses during cancer treatment (Singh, 2021). Various nanovaccines are developed and evaluated through the integration (Liu et al., 2020) of various nanoengineering techniques in preclinical models (Bhardwaj et al., 2020) and, occasionally, in human cancer immunotherapy (Nooraei et al., 2021). This paper reviews the latest advancements in nanovaccines, encompassing polymer nanoparticles, liposome nanoparticles, virus-like particles, and inorganic nanoparticles. The advantages and challenges of each nanovaccine type are shown in (Figure 12).
Nanovaccines outshine conventional immunotherapy as they precisely target APCs, protect and sustain antigen release, carry adjuvants for enhanced immunogenicity, enable personalization, and reduce systemic toxicity, being a promising cancer immunotherapy option (Sun et al., 2022). The literature shows that the polymer-based preparation of nanoparticles is currently the most extensive research, and using PLGA to prepare nanovaccines is the most promising. Firstly, such research has the deepest foundation and the most comprehensive field. Secondly, the preparation process of this type of nanovaccine is relatively simple, and the preparation difficulty is smaller than that of other kinds of nanovaccines. However, in the future, this paper believes that liposome vaccines based on mRNA vaccines can also be greatly developed, the reason is that with the development of genetic engineering, the efficacy of liposome nanovaccines using mRNA will be further improved. We produced mRNA-based nanovaccine summary tables containing various details of it, just in (Table 1).
Despite the promising potential of nanovaccines in cancer immunotherapy, researchers still face challenges in enhancing the immunogenicity of antigens, countering the immune escape (Immune escape is the phenomenon where pathogens or cancer cells avoid the host’s immune system. It happens through mechanisms like antigenic changes in pathogens and immunosuppressive actions in cancer cells. This is significant as it causes chronic infections and hampers disease treatment.)mechanisms of tumors, and achieving effective delivery of tumor vaccines. Scaling up nanoparticle synthesis for nanovaccines is also tough. Larger production volumes make it harder to control nanoparticle features precisely, ensure reproducibility and consistency. Regulatory approval is complex as new guidelines for nanoparticle assessment are needed. Lack of standardized characterization methods and underdeveloped costly manufacturing tech with strict quality control impede consistency in synthesis. To overcome these challenges, in production, automated and standardized processes like continuous flow synthesis are key, with academia-industry collaboration for GMP. Regulators engage experts, e.g., FDA’s initiatives, using clinical trial data. For consistency, advanced characterization, reference materials, and standard procedures are being developed via international consortiums. Future vaccinations are anticipated to hinge on the development of stable, safe, and personalized nanovaccines to ensure a robust and enduring antitumor immune response.
In the Web of Science database, we searched with the keyword nanovaccines cancer and retrieved a total of 485 papers. Through the analysis of the results of papers published in the past 5 years, we can see that the research papers on nanovaccines in cancer diagnosis and treatment have increased by more than 15% year by year, and the research directions are mainly concentrated in Biomaterials, Immune System, Chemical Coordination and Homeostasis, etc., showing that nanovaccines have good prospects in the research field. At the same time, research directions such as Human Medicine Medical Sciences, Biochemistry and Molecular Biophysics, and Infection also showed a good growth trend, which may be an important research direction in the future. In addition, through the investigation of the current clinical trial progress of drugs, we found that there are still few drugs approved by the FDA, mainly concentrated in solid tumors, such as breast cancer, colon cancer, etc. The progress of drugs in existing clinical trials is not very smooth, so there is still a long way to go for large-scale clinical application of nanovaccines.
XY: Data curation, Formal Analysis, Resources, Visualization, Writing–original draft. TX: Data curation, Formal Analysis, Visualization, Writing–original draft, Investigation. MH: Validation, Writing–review and editing. BL: Validation, Writing–review and editing, Conceptualization, Funding acquisition, Methodology, Software. JZ: Conceptualization, Methodology, Supervision, Validation, Writing–review and editing.
The author(s) declare that financial support was received for the research, authorship, and/or publication of this article. This work was supported by NSFC (62075098, 61401217, and W2433193), the Key Research and Development Program of Jiangsu (BE2022160), Nanjing Medical University Changzhou Medical Centre Post-doctoral Program (CZKYCMCP202201), Jiangsu Province Excel-lent Postdoctoral Program (2023ZB880), and the Science and Technology Research Plan of Rugao (SRGS (23)049).
The authors declare that the research was conducted in the absence of any commercial or financial relationships that could be construed as a potential conflict of interest.
The author(s) declare that no Generative AI was used in the creation of this manuscript.
All claims expressed in this article are solely those of the authors and do not necessarily represent those of their affiliated organizations, or those of the publisher, the editors and the reviewers. Any product that may be evaluated in this article, or claim that may be made by its manufacturer, is not guaranteed or endorsed by the publisher.
Baljon, J. J., Kwiatkowski, A. J., Pagendarm, H. M., Stone, P. T., Kumar, A., Bharti, V., et al. (2024). A cancer nanovaccine for Co-delivery of peptide neoantigens and optimized combinations of STING and TLR4 agonists. ACS NANO 18, 6845–6862. doi:10.1021/acsnano.3c04471
Bezbaruah, R., Chavda, V. P., Nongrang, L., Alom, S., Deka, K., Kalita, T., et al. (2022). Nanoparticle-based delivery systems for vaccines. Vaccines 10, 1946. doi:10.3390/vaccines10111946
Bhardwaj, P., Bhatia, E., Sharma, S., Ahamad, N., and Banerjee, R. (2020). Advancements in prophylactic and therapeutic nanovaccines. Acta Biomater. 108, 1–21. doi:10.1016/j.actbio.2020.03.020
Cao, W., Jin, M., Zhou, W., Yang, K., Cheng, Y., Chen, J., et al. (2024). Forefronts and hotspots evolution of the nanomaterial application in anti-tumor immunotherapy: a scientometrics analysis. J. Nanobiotechnology 22, 30. doi:10.1186/s12951-023-02278-3
Chen, H., Huang, Z., Li, J., Dong, S., Xu, Y., Ma, S., et al. (2024). Hit-and-run vaccine system that overcomes limited neoantigen epitopes for efficient broad antitumor response. Sci. Bull. 69, 922–932. doi:10.1016/j.scib.2024.01.039
Chen, Z. (2023). “Preparation of lipid-based mRNA nanovaccine and its application in colon cancer immunotherapy. dissertation,”. Central South University. doi:10.27661/d.cnki.gzhnu.2023.000026
Chu, Y., Qian, L., Ke, Y., Feng, X., Chen, X., Liu, F., et al. (2022). Lymph node-targeted neoantigen nanovaccines potentiate anti-tumor immune responses of post-surgical melanoma. J. Nanobiotechnology 20, 190. doi:10.1186/s12951-022-01397-7
Du, Q., Luo, Y., Xu, L., Du, C., Zhang, W., Xu, J., et al. (2024). Smart responsive Fe/Mn nanovaccine triggers liver cancer immunotherapy via pyroptosis and pyroptosis-boosted cGAS-STING activation. J. Nanobiotechnology 22, 95. doi:10.1186/s12951-024-02354-2
Gao, J. (2020). Research progress of nanoparticle vaccine in tumor immunotherapy. Chem. and Bioeng. 37, 1–4+12. doi:10.3969/j.issn.1672-5425.2020.08.001
Gou, S. (2022). The construction and antitumor immunological activity of adjuvant-free peptide vaccine and biomimetic nanovaccine by targeting Clec9a+ dendritic cell. Zhengzhou, China: Zhengzhou University. dissertation. doi:10.27466/d.cnki.gzzdu.2022.000367
Gu, Y. (2023). Antigen-biomimetic MnO2 nanovaccines for radioimmunotherapy of tumor. Suzhou: Soochow University. master’s thesis. doi:10.27351/d.cnki.gszhu.2021.003530
Guo, L. (2021). Construction and anti-tumor efficiency of multivalent assembly nano-vaccine for prostate cancer. Tianjin: Tianjin Medical University. dissertation. doi:10.27366/d.cnki.gtyku.2021.000290
Guo, Z., Liu, Y., He, N., Deng, Y., and Jin, L. (2021). Discussion of the protein characterization techniques used in the identification of membrane protein targets corresponding to tumor cell aptamers. Chin. Chem. Lett. 32, 40–47. doi:10.1016/j.cclet.2020.11.061
Gurunathan, S., Thangaraj, P., Wang, L., Cao, Q., and Kim, J.-H. (2024). Nanovaccines: an effective therapeutic approach for cancer therapy. Biomed. and Pharmacother. 170, 115992. doi:10.1016/j.biopha.2023.115992
Han, Y., Wen, P., Li, J., and Kataoka, K. (2022). Targeted nanomedicine in cisplatin-based cancer therapeutics. J. Control. Release 345, 709–720. doi:10.1016/j.jconrel.2022.03.049
Huang, C. (2023). Construction and antitumor study of multifunctional mesoporous silica nanomedicine/nanovaccine. dissertation. Beijing. Peking Union Medical College. doi:10.27648/d.cnki.gzxhu.2022.000068
Jogdeo, C., Siddhanta, K., Das, A., Ding, L., Panja, S., Kumari, N., et al. (2024). Beyond lipids: exploring advances in polymeric gene delivery in the lipid nanoparticles era. Adv. Mater. 36, e2404608. doi:10.1002/adma.202404608
Karimi, H., Soleimanjahi, H., Abdoli, A., and Banijamali, R. S. (2020). Combination therapy using human papillomavirus L1/E6/E7 genes and archaeosome: a nanovaccine confer immuneadjuvanting effects to fight cervical cancer. Sci. Rep. 10, 5787. doi:10.1038/s41598-020-62448-3
Kaveh-Samani, A., Dalali, S., Kaviani, F., Piri-Gharaghie, T., and Doosti, A. (2024). Oral administration of DNA alginate nanovaccine induced immune-protection against Helicobacter pylori in Balb/C mice. BMC Immunol. 25, 11. doi:10.1186/s12865-024-00602-6
Koyande, N. P., Srivastava, R., Padmakumar, A., and Rengan, A. K. (2022). Advances in nanotechnology for cancer immunoprevention and immunotherapy: a review. Vaccines 10, 1727. doi:10.3390/vaccines10101727
Liang, C. (2024). Antitumor immune effect of chitosan-mediated neoantigen nanovaccine. Beijing: Beijing University of Technology. dissertation. doi:10.26935/d.cnki.gbjgu.2022.000020
Liang, X., Cheng, H., Liu, C., and Liu, G. (2022). Antigen self-presenting nanovaccine for cancer immunotherapy. Sci. Bull. 67, 1611–1613. doi:10.1016/j.scib.2022.07.018
Lin, Y., Chen, X., Wang, K., Liang, L., and Zhang, H. (2024). An overview of nanoparticle-based delivery platforms for mRNA vaccines for treating cancer. Vaccines 12, 727. doi:10.3390/vaccines12070727
Liu, C. (2023). The research and application of functionalized dendritic cell vesicular nanovaccine for anti-tumor immunotherapy. Xiamen: Xiamen University. dissertation. doi:10.27424/d.cnki.gxmdu.2020.003122
Liu, H. (2024). Carbon-dots-based nanovaccines for enhanced cancer immunotherapy. Changchun: Changchun University of Technology. master’s thesis. doi:10.27805/d.cnki.gccgy.2023.000685
Liu, M., Feng, Y., Lu, Y., Huang, R., Zhang, Y., Zhao, Y., et al. (2024). Lymph-targeted high-density lipoprotein-mimetic nanovaccine for multi-antigenic personalized cancer immunotherapy. Sci. Adv. 10, eadk2444. doi:10.1126/sciadv.adk2444
Liu, Q., Chu, Y., Shao, J., Qian, H., Yang, J., Sha, H., et al. (2023a). Benefits of an immunogenic personalized neoantigen nanovaccine in patients with high-risk gastric/gastroesophageal junction cancer. Adv. Sci. 10, 2203298. doi:10.1002/advs.202203298
Liu, Q., Hu, Y., Zheng, P., Yang, Y., Fu, Y., Yang, Y., et al. (2023b). Exploiting immunostimulatory mechanisms of immunogenic cell death to develop membrane-encapsulated nanoparticles as a potent tumor vaccine. J. Nanobiotechnology 21, 326. doi:10.1186/s12951-023-02031-w
Liu, X. (2022). Investigation on the construction of an in vivo individualized recombinant nanovaccine for enhancing tumor immunotherapy. Zhengzhou: Zhengzhou University. master’s thesis. doi:10.27466/d.cnki.gzzdu.2021.000606
Liu, Y., Guo, J., and Huang, L. (2020). Modulation of tumor microenvironment for immunotherapy: focus on nanomaterial-based strategies. Theranostics 10, 3099–3117. doi:10.7150/thno.42998
Lu, Z., Peng, Z., Liu, C., Wang, Z., Wang, Y., Jiao, X., et al. (2020). Current status and future perspective of immunotherapy in gastrointestinal cancers. Innovation 1, 100041. doi:10.1016/j.xinn.2020.100041
Mao, J., Qian, Y., Shi, G., Wang, J., and Chen, Y. (2021). Application of nanovaccines in cancer immunotherapy. Prog. Biochem. Biophysics 48, 1130–1136. doi:10.16476/j.pibb.2021.0007
Ni, Q., Zhang, F., Liu, Y., Wang, Z., Yu, G., Liang, B., et al. (2020). A bi-adjuvant nanovaccine that potentiates immunogenicity of neoantigen for combination immunotherapy of colorectal cancer. Sci. Adv. 6, eaaw6071. doi:10.1126/sciadv.aaw6071
Nooraei, S., Bahrulolum, H., Hoseini, Z. S., Katalani, C., Hajizade, A., Easton, A. J., et al. (2021). Virus-like particles: preparation, immunogenicity and their roles as nanovaccines and drug nanocarriers. J. Nanobiotechnol 19, 59. doi:10.1186/s12951-021-00806-7
Pei, M. (2021). Preparation of natural polysaccharides nano-vaccine and study of its application on tumor immunotherapy. Beijing: Peking Union Medical College. master’s thesis. doi:10.27648/d.cnki.gzxhu.2020.000588
Peres, C., Matos, A. I., Carreira, B., Moura, L. I. F., Kleiner, R., Vaskovich-Koubi, D., et al. (2024). Multifunctional nanovaccine sensitizes breast cancer to immune checkpoint therapy. Adv. Funct. Mater. 34, 2401749. doi:10.1002/adfm.202401749
Qiu, H. (2024). Bidirectional immunomodulatory nanovaccine: photothermal-immunotherapy via remodel of tumor immune microenvironment. Guilin: Guangxi Normal University. master’s thesis. doi:10.27036/d.cnki.ggxsu.2023.001331
Shi, S., Jia, R., Han, L., Deng, X., Liang, Z., Zhang, X., et al. (2022). Anti-tumor effect of colon cancer cell lysate nano vaccine. Pract. Pharm. Clin. Remedies 25, 289–294. doi:10.14053/j.cnki.ppcr.202204001
Singh, A. (2021). Eliciting B cell immunity against infectious diseases using nanovaccines. Nat. Nanotechnol. 16, 16–24. doi:10.1038/s41565-020-00790-3
Su, Y., Xu, W., Wei, Q., Ma, Y., Ding, J., and Chen, X. (2023). Chiral polypeptide nanoparticles as nanoadjuvants of nanovaccines for efficient cancer prevention and therapy. Sci. Bull. 68, 284–294. doi:10.1016/j.scib.2023.01.024
Sun, L., Lv, H., Feng, J., Guselnikova, O., Wang, Y., Yamauchi, Y., et al. (2022). Noble-metal-based hollow mesoporous nanoparticles: synthesis strategies and applications. Adv. Mater. 34, e2201954. doi:10.1002/adma.202201954
Sun, W., Ji, P., Zhou, T., Li, Z., Xing, C., Zhang, L., et al. (2023). Ultrasound responsive nanovaccine armed with engineered cancer cell membrane and RNA to prevent foreseeable metastasis. Adv. Sci. 10, 2301107. doi:10.1002/advs.202301107
Sun, Y., Chen, W., Sun, S., Shang, H., Guan, X., and Zhang, W. (2024). Minimalist “in situ” tumor vaccine leveraging versatile dendrimer nanoplatform coordinated ICD and immunoagonist for boosted chemoimmunotherapy. Sci. China Mater. 67, 2700–2708. doi:10.1007/s40843-024-2940-6
Thangam, R., Patel, K. D., Kang, H., and Paulmurugan, R. (2021). Advances in engineered polymer nanoparticle tracking platforms towards cancer immunotherapy—current status and future perspectives. Vaccines 9, 935. doi:10.3390/vaccines9080935
Wang, Y. (2024). Construction and functional verification of GPC3127-136-HSP70 mRNA nanoparticle vaccine for hepatocellular carcinoma in vitro. Chongqing: Southwest University. master’s thesis. doi:10.27684/d.cnki.gxndx.2023.003291
Wang, Z., Sha, T., Li, J., Luo, H., Liu, A., Liang, H., et al. (2024). Turning foes to friends: advanced “in situ nanovaccine” with dual immunoregulation for enhanced immunotherapy of metastatic triple-negative breast cancer. Bioact. Mater. 39, 612–629. doi:10.1016/j.bioactmat.2024.04.023
Wu, Q.-J., and Lv, W.-L. (2024). Cancer vaccines designed based the nanoparticle and tumor cells for the treatment of tumors: a perspective. IET Nanobiotechnol 2024, 1–8. doi:10.1049/2024/5593879
Xie, C., You, X., Zhang, H., Li, J., Wang, L., Liu, Y., et al. (2023a). A nanovaccine based on adjuvant peptide FK-13 and L-phenylalanine poly(ester amide) enhances CD8+ T cell-mediated antitumor immunity. Adv. Sci. 10, 2300418. doi:10.1002/advs.202300418
Xie, Y.-J., Liu, W.-Q., Li, D., Hou, J.-C., Coghi, P. S., and Fan, X. X. (2023b). Overcoming suppressive tumor microenvironment by vaccines in solid tumor. Vaccines 11, 394. doi:10.3390/vaccines11020394
Xin, K., Wei, X., Shao, J., Chen, F., Liu, Q., and Liu, B. (2024). Establishment of a novel tumor neoantigen prediction tool for personalized vaccine design. Hum. Vaccines and Immunother. 20, 2300881. doi:10.1080/21645515.2023.2300881
Xiong, J. (2023). The treatment and mechanism of macrophage M1 polarization mediated by nano-tumor vaccine in ovarian cancer. Wuhan: Wuhan University. dissertation. doi:10.27379/d.cnki.gwhdu.2023.000362
Yang, Y. (2021). “Peking union medical college,” in Study on the treatment of colon cancer by the combination of Endo-alginate hydrogel and tumor cell lysate nanoparticle vaccine. Beijing. master’s thesis. doi:10.27648/d.cnki.gzxhu.2020.000760
Yang, Y., Wang, H., Sun, Y., Sun, T., and Jiang, C. (2022). Advances in the study of nano-biomimetic tumor vaccines. Acta Pharm. Sin. 57, 963–975. doi:10.16438/j.0513-4870.2021-1069
Yu, X., Dai, Y., Zhao, Y., Qi, S., Liu, L., Lu, L., et al. (2020). Melittin-lipid nanoparticles target to lymph nodes and elicit a systemic anti-tumor immune response. Nat. Commun. 11, 1110. doi:10.1038/s41467-020-14906-9
Zhang, J., Yu, S., Peng, Q., Wang, P., and Fang, L. (2024a). Emerging mechanisms and implications of cGAS-STING signaling in cancer immunotherapy strategies. Cancer Biol. and Med. 21, 45–64. doi:10.20892/j.issn.2095-3941.2023.0440
Zhang, L. (2021). Application and mechanism of iodinaed oil nanovaccine mediated anti-tumor immunotherapy. Nanning: Guangxi Medical University. master’s thesis. doi:10.27038/d.cnki.ggxyu.2021.000436
Zhang, L., Xu, L., Wang, Y., Liu, J., Tan, G., Huang, F., et al. (2022). A novel therapeutic vaccine based on graphene oxide nanocomposite for tumor immunotherapy. Chin. Chem. Lett. 33, 4089–4095. doi:10.1016/j.cclet.2022.01.071
Zhang, M., Wang, Y., Song, Z., Lu, Y., Zhao, H., Wang, Y., et al. (2024b). Recent progress of bioinspired cell membrane in cancer immunotherapy. Clin. Med. Insights-oncology 18. doi:10.1177/11795549241236896
Zhang, Y. (2023). A biotin-avidin-system-based nanovaccine for tumor immunotherapy. Haikou: Hainan Medical College. master’s thesis. doi:10.27952/d.cnki.ghnyx.2022.000113
Zhang, Y., Jiang, M., Du, G., Zhong, X., He, C., Qin, M., et al. (2023). An antigen self-assembled and dendritic cell-targeted nanovaccine for enhanced immunity against cancer. ACTA Pharm. Sin. B 13, 3518–3534. doi:10.1016/j.apsb.2022.03.017
Zheng, A., Ning, Z., Wang, X., Li, Z., Sun, Y., Wu, M., et al. (2024). Human serum albumin as the carrier to fabricate STING-activating peptide nanovaccine for antitumor immunotherapy. Mater. Today Bio 25, 100955. doi:10.1016/j.mtbio.2024.100955
Keywords: nanoparticles, nanovaccines, immunotherapy, cancer, theranostics
Citation: Yuan X, Xu T, Hussain M, Liu B and Zhu J (2025) Recent advances of nanovaccines on cancer theranostics. Front. Nanotechnol. 7:1521131. doi: 10.3389/fnano.2025.1521131
Received: 01 November 2024; Accepted: 20 January 2025;
Published: 12 February 2025.
Edited by:
Chao Wang, Johns Hopkins University, United StatesReviewed by:
Shivani R. Pandya, Narnarayan Shastri Institute of Technology, IndiaCopyright © 2025 Yuan, Xu, Hussain, Liu and Zhu. This is an open-access article distributed under the terms of the Creative Commons Attribution License (CC BY). The use, distribution or reproduction in other forums is permitted, provided the original author(s) and the copyright owner(s) are credited and that the original publication in this journal is cited, in accordance with accepted academic practice. No use, distribution or reproduction is permitted which does not comply with these terms.
*Correspondence: Jianwei Zhu, and6aHVtZEBhbGl5dW4uY29t; Bin Liu, bGl1YmluQG5qbXUuZWR1LmNu
†These authors have contributed equally to this work and share first authorship
Disclaimer: All claims expressed in this article are solely those of the authors and do not necessarily represent those of their affiliated organizations, or those of the publisher, the editors and the reviewers. Any product that may be evaluated in this article or claim that may be made by its manufacturer is not guaranteed or endorsed by the publisher.
Research integrity at Frontiers
Learn more about the work of our research integrity team to safeguard the quality of each article we publish.