- 1Laboratorio de Estudios Farmacológicos y Toxicológicos -LEFyT, Facultad de Ciencias Veterinarias, Universidad Nacional de La Plata, Buenos Aires, Argentina
- 2Consejo Nacional de Investigaciones Científicas y Técnicas (CONICET), Buenos Aires, Argentina
- 3CINDEFI -Centro de Investigación y Desarrollo en Fermentaciones Industriales, Laboratorio de Nanobiomateriales (UNLP-CONICET LA PLATA), Facultad de Ciencias Exactas UNLP, La Plata, Argentina
Introduction: Bovine mastitis is a major infectious disease affecting dairy cattle, impacting public health and milk industry profitability. Staphylococcus aureus is a contagious pathogen responsible for causing bovine subclinical mastitis. Its pathogenesis and antimicrobial resistance highlight the need for alternative treatments, being the nanoencapsulation of essential oils (EO) very promising.
Methods: Nanostructured lipid carriers (NLCs) containing 5% of Melaleuca armillaris EO were synthesized and characterized. Their physicochemical characteristics, antimicrobial activity against methicillin-resistant and sensitive S. aureus (MRSA and MSSA, respectively), and protective activity against polymorphonuclear cells were evaluated.
Results: NLC-EO nanoparticles were morphologically spherical and the mean size was around 190 nm, Polydispersity index (PdI) was 0.21 (±0.01), Z potential was −18.4 (±0.4) and EO encapsulation efficiency was 71.5%. Of this parameters Z potential was the only which changed after 6 months of storage at four°C, turning into a more negative value of −31.6 (±1.9). NLC-EO showed a biphasic behavior with a fast initial release during the first 6 h, followed by a slow phase for at least 72 h. Free and nanoencapsulated EO had a minimum inhibitory concentration (MIC90) of 6.25 μL/mL; however, free EO had a minimum inhibitory concentrations of biofilm formation (MICB90) of 3.12 μL/mL and for EO nanoencapsulated was 6.25 μL/mL. Minimum inhibitory concentrations of biofilm formation and eradication (MECB90) were 12.5 μL/mL and 6.25 μL/mL for the nanoencapsulated EO and free EO, respectively. Empty NLC inhibited biofilm formation, but not planktonic growth or eradicated preformed biofilms.
Discussion: The EO was efficiently encapsulated and released from NLC, and its antimicrobial activity against MRSA and MSSA was high. Neutrophil viability was higher when EO was encapsulated, being an important result for future experiments evaluating intracellular EO activity, where Staphylococcus aureus survives and evades poorly penetrating antibiotics activity.
1 Introduction
Mastitis is considered the most relevant disease worldwide in bovine dairy industry due to its health and economic impact. Staphylococcus aureus is one of the main contagious pathogens responsible for causing bovine subclinical mastitis. It can adapt, survive and grow in the skin of the mammary gland and teat lesions, so the main reservoirs are infected udders (Monistero et al., 2018). Poor handling practices in dairy, lack of hygiene in the establishments, animals and staff contribute to the spread of this microorganism. Staphylococcus aureus is important for public health due to its virulence and the potential risk of antimicrobial resistance genetic determinants transferring between animals, humans and the environment (World Health Organization, 2021). Because of this, it has been included in surveillance and monitoring programs of resistance to antimicrobial agents as an emerging zoonotic pathogenic bacterium (OIE, chapter 6.7 of the Terrestrial Animal Health Code). The ability of S. aureus to invade epithelial cells, form biofilms and produce polysaccharides has been known for many years. These phenomena are responsible for its persistence in the udder, hindering the arrival of chemotherapeutics in adequate concentrations and for effective periods (Peyrusson et al., 2020). Bacteria in this state (within the biofilm structure) are more resistant to antibiotics, compared to planktonic bacteria.
The growing threat of antimicrobial resistance has drastically increased therapeutic failure and encourages the exploration of new alternatives to deal with it (World Health Organization, 2020). In the world, approximately 700,000 people die per year due to infections caused by bacteria resistant to multiple antimicrobials, it is estimated that this number will reach around 10 million deaths per year by 2050 (Antimicrobial Resistance Collaborators, 2022). Another important factor is the difficulty to develop new molecules and the high cost that this implies for pharmaceutical industry (Dutescu and Hillier, 2021). Methicillin-resistant S. aureus (MRSA), which was originally restricted to hospitals, has become a significant pathogen in the community, considerably increasing its importance to human and animal health (Vandenesch et al., 2003). Although S. aureus is a common microorganism in bovine mastitis, MRSA is not commonly associated with this pathology (Hendriksen et al., 2008). However, there are reports of its appearance in different parts of the world, including Brazil (Guimarães et al., 2017) and Argentina (Srednika et al., 2019).
Usage of antimicrobials in dry cow therapy is an important part of mastitis control programs, being effective in reducing the prevalence of intramammary infections and increasing milk production in subsequent lactation (Bradley and Green, 2004). However, despite being a good strategy in the dairy, the antimicrobial-based treatments used in drying are becoming increasingly ineffective due to growing bacterial resistance, making it important to have a bacteriological diagnosis and to know the sensitivity profile of the pathogenic microorganism involved (Rajala-Schultz et al., 2021). Nanotechnology and phytotherapy are fields in active growth, generating innovative products with promising results. The production of nanoparticles containing phototherapeutic products become strong candidates for the future treatment of mastitis caused by Staphylococcus spp. providing sustainability in its production, safety and quality in the product (Falcão et al., 2018).
Plant essential oils (EOs) are complex mixtures of secondary metabolites that have antimicrobial activity on their own and together with antibiotics can have synergistic effects (Yap et al., 2014). The different compounds present in plant extracts make it difficult for bacteria to develop simultaneous resistance mechanisms against each of them (Begun et al., 2005; Sifri et al., 2006). Melaleuca armillaris is a widely cultivated plant of the genus Melaleuca, whose EO is rich in 1,8-cineole (Buldain et al., 2021; Chabir et al., 2011). Inhibitory activity was found, in vitro, against several bacterial species, including S. aureus (Amri et al., 2012; Buldain et al., 2021).
The yield obtained from this plant species by our group (1.22%) (Buldain et al., 2018) and the fast regeneration of its biomass after harvest make its exploitation economically viable and sustainable with good management. To this we must add the high density of plants that can be cultivated per hectare, a very similar species of Melaleuca (Melaleuca alternifolia), is cultivated industrially with densities of 30,000 plants/Ha (Davis, 2003), so the amount of EO obtained is very important.
Another interesting approach to address the problem of intracellular persistence of S. aureus and antimicrobial resistance associated with subclinical and recurrent bovine mastitis infections is the development of nanostructures for drug delivery. Nanoparticles penetrate the cell membrane and subcellular organelles, reach the biophase where the pathogenic microorganism is located, remain intracellular for long periods, and are released from their cargo through membrane pores (Zhou et al., 2018). In this way, pharmacological activity is enhanced by a uniform dosage of the active agent, its bioavailability is improved, it allows for localization at the infected site, and decreases treatment time and side effects (Garg et al., 2015). Solid lipid nanoparticles (SLN) matrices present interesting characteristics from the biological point of view such as cytocompatibility, biodegradability and stability. This type of nanoparticles is a great option for encapsulating hydrophobic compounds such as EO compared to other emulsion systems (Shidhaye et al., 2008; Rodenak-Kladniew et al., 2023; Menossi et al., 2024). Nanostructured lipid carriers (NLC) present improvements in the physicochemical properties of SLN, including greater drug storage stability and encapsulation efficiency, as well as better control of the drug release kinetic profile (Islan et al., 2017). NLC has other interesting properties for biological applications like the absence of organic solvents in the synthesis and suitability for scaling-up production (Ribeiro et al., 2017). For all this, the encapsulation of EOs in nanoparticles is extremely promising for improving the efficacy of antimicrobial therapy, since they favor their stability, facilitate handling, improve their safety by reducing toxic side effects that may occur, allow a controlled release, improve the water solubility of hydrophobic compounds, improve bioavailability and therefore increase efficacy (Bilia et al., 2014).
This study aimed to evaluate the antimicrobial activity of M. armillaris EO free and nanoencapsulated in NLC against MSSA and MRSA grown in biofilms and planktonic cultures, and the evaluation of its cytotoxicity on polymorphonuclear leukocytes (PMNs).
2 Materials and methods
2.1 NLC loaded with Melaleuca armillaris EO preparation
Nanostructured lipid carriers (NLC) containing M. armillaris EO were prepared by ultrasonication. Briefly, 400 mg of myristyl myristate (lipid) was melted in a 60°C–70°C water bath and mixed with 500 μL of EO and 50 μL of coda oil. After 10 min, a hot aqueous solution (10 mL) with 3.0% (w/v) of Pluronic® F68 (PF-68) was added to the lipid phase. The mixture was immediately sonicated for 10 min (70% amplitude) using an ultrasonic processor equipped with a 6 mm titanium tip. The dispersion was then cooled to room temperature and stored at 4°C. Additionally, empty nanoparticles were prepared in the same way, but without the addition of EO. The final NLC concentration, referenced based on lipid content, was 40 mg lipid/mL (Medina-Montano et al., 2022). In the case of the NLC-EO, the lipid content was the same, containing 50 μL/mL of EO. The EO of M. armillaris used was obtained previously by steam distillation and characterized by GC-MS-FID (Buldain et al., 2021), and stored protected from light at −20°C.
2.2 Measurement of loading efficiency
The final volume of the NLC-EO suspension was determined after its synthesis, and the concentration of free EO was measured analytically. The EO was quantified by UV–VIS spectrophotometry (λmax = 230 nm) using a microplate reader (Eon Biomek, United States). The EO calibration curve was performed in concentrations between 0.04 and 10.00 μL/mL dissolved in ethanol.
An ultrafiltration centrifugal device (MWCO = 10,000 Da, Microcon, Millipore, Ma., United States) was filled with 500 µL of NLC-EO preparation, and the nanoparticles were separated by centrifugation at 10,000 rpm for 10 min. The filtrate solution was diluted ten-fold in ethanol and the nonencapsulated EO was measured by UV spectrophotometer. The encapsulation efficiency (EE) was calculated according to the following Equation 1:
where Q0 is the initial amount of EO added into the lipid phase, Cf is the EO concentration in the filtered solution, and V is the final volume of the NLC-EO (Rodenak-Kladniew et al., 2023).
2.3 Characterization of Melaleuca armillaris EO-loaded NLC by size, polydispersity index (PdI) and zeta potential
Dynamic Light Scattering (DLS) method using a Nano ZS Zetasizer Instrument (Malvern Instruments Corp., United Kingdom) was applied to evaluate the mean size, Z potential and the polydispersity index (PdI) of NLC and NLC-EO at 25°C as was previously reported (Rodenak-Kladniew et al., 2023). Briefly, NLC and NLC-EO samples were diluted in Milli-Q water at a 1:10 ratio and analyzed in polystyrene cuvettes with a 10 mm path length at 25°C and pH 7.0 for size and PdI measurements. The Zeta potential was determined in capillary cells, also with a 10 mm path length.
2.4 Transmission electron microscopy (TEM)
TEM images of NLC-EO were obtained in a Jeol-1200 EX II-TEM microscope (Jeol, MA, United States) following a previous stablish protocol from our laboratory (Rodenak-Kladniew et al., 2017). The samples were tenfold diluted with ultrapure water and deposited on a collodion-coated Cu grid (400 mesh) in presence of Phosphotungstic acid as contrast enhancer.
2.5 Physical stability
Mean particle size, Z potential, PdI and EE of freshly prepared nanoparticle dispersion, and after 6 months storage at 4°C and protected from light, were measured.
2.6 Release studies
The evaluation of EO was released using a dialysis membrane (MWCO 10 kDa, Spectra Pore, United States). The dialysis membrane was placed in a tube with distilled water for 24 h and then filled with 3.0 mL of each formulation (EO loaded NLC, EO dissolved in ethanol and EO in PBS) followed by incubation in 10 mL of 10 mM phosphate buffer (pH 7.4) at 37°C and constant stirring of 150 rpm (Rodenak-Kladniew et al., 2017). At different times (0.5, 1, 2, 3, 4, 5, 6, 24, 26, 48, 72 h), samples of 200 µL were withdrawn, and EO concentration was measured at 230 nm using a UV–VIS spectrophotometer (Eon Biotek, United States). The EO calibration curve was performed in concentrations between 0.02 and 6.25 μL/mL in PBS.
2.7 Antimicrobial activity of free and nanoencapsulated EO
Antimicrobial activities of the EO, NLC-EO and empty NLC were evaluated against six wild type S. aureus isolates (three MRSA and three MSSA) and a reference strain (S. aureus ATCC 29213).
The Minimum Inhibitory Concentration (MIC) of EO, NLC (empty) and NLC-EO was evaluated by microdilution method. The range of EO concentrations tested (half serial dilution) was 12.5–0.02 μL/mL. Each well was inoculated with a final bacterial concentration of 5 × 105 CFU/mL and the microplates were incubated at 35°C for 18–24 h. The MIC90 was established by adding the vital dye resazurin and considering the lowest concentration that inhibits bacterial growth. Each determination was performed in triplicate. The MBC90 was determined as was previously described (Buldain et al., 2021).
2.8 Antibiofilm activity of free and encapsulated Melaleuca armillaris EO
The evaluation of the antibiofilm activity of EO, NLC and NLC-EO was carried out, establishing the ability to inhibit the biofilms formation of planktonic microorganism, as well as their effectiveness in eradicating previously formed biofilms. Formerly to antibiofilm activity determinations, the ability of the MRSA and MSSA isolates to form biofilm was determined. Isolates were cultured in trypticase soy broth (TSB) supplemented with 1% (w/v) glucose for 24 h at 37°C. Subsequently, the inoculums were adjusted to a concentration of 5 × 105 CFU/mL from a 0.5 McFarland scale bacterial suspension (1 × 108 CFU/mL) in TSB supplemented with 1% (w/v) glucose. 200 μL of diluted culture broth were inoculated into flat-bottomed 96-well microtiter plates and incubated at 37°C for 24 h under static conditions. After incubation, the contents of the wells were discarded, washed with distilled water and dried for 30 min in an oven at 60°C. Then, 125 µL of 0.1% w/v crystal violet solution were added to each well and then incubated for 10 min at room temperature. The plate was emptied, washed twice with water to remove excess crystal violet. Finally, to elute the dye incorporated by the cells, 200 µL of 96° ethanol were added for 15 min. The content of each well was transferred to another microplate to determine the absorbance by spectrophotometry at 595 nm. Biofilm formation capacity was compared with the control. The OD cut-off value (ODc) is defined as the mean value of the negative controls plus 3 standard deviations. The strains were classified as non-producers of biofilm (OD ≤ ODc); weak (ODc < OD ≤ 2x ODc); moderate (2x ODc < OD < 4x ODc) and strong biofilm producers (4x ODc < OD).
2.8.1 Inhibition of biofilm formation and eradication of preformed biofilms
The ability to inhibit the formation of biofilms of free EO, NLC (empty) and NLC-EO was evaluated. 96-well flat-bottom microplates were loaded with TSB broth with 1% w/v glucose, with bacterial inoculum (5 × 105 CFU/mL) and the corresponding dilution of empty and EO-loaded NLC; they were incubated statically at 37°C for 24 h. Subsequently, the culture medium was removed, and each well was washed with PBS to remove planktonic cells. The biofilm formed was stained with 0.1% w/v crystal violet and solubilized with 96% ethanol to measure its absorbance at 595 nm. Untreated positive controls (bacterial inoculum and TSB culture medium with 1% w/v glucose) were used. The inhibition percentage for each treatment was calculated by the Equation 2:
The MBIC90 was defined as the minimum concentration that produces ≥90% inhibition of biofilm formation, concerning to untreated controls in 90% of the isolates (Provenzani et al., 2021).
The antibiofilm effect of free and encapsulated M. armillaris EO in NLC was also determined by eradication analyses in preformed biofilms by assessing the Minimum Eradication Concentration of Biofilm (MECB90), defined as the minimum concentration that prevents visible growth after exposing a biofilm to an antimicrobial. Briefly, biofilm formation was carried out as above and washed with sterile PBS. Subsequently, the free and encapsulated EO dilutions (prepared in TSB with 1% w/v glucose) were added in concentrations corresponding to 0.25, 0.5, 1, two and 4xMIC of the nanoparticles loaded with EO and 0.5, 1, 2, four and 8xMIC of free EO. Wells loaded only with TSB supplemented with 1% (w/v) glucose were used as negative control, and biofilms performed with TSB with 1% w/v glucose were used as positive control. A control for empty nanoparticles was also added. After incubation at 37°C for 24 h under static conditions, the inhibitory effect with resazurin, crystal violet staining and CFU counts were measured. Each experiment was performed in quadruplicate.
2.9 Cytotoxicity of EO free and encapsulated
PMNs were isolated from the blood of healthy bovines that did not receive any antibiotic treatment along the last 3 months before the trial. The protocol was approved by the Institutional Committee for the Control and Use of Animal Laboratories of the Faculty of Veterinary Sciences, UNLP (47.3.15J). The isolated PMNs were resuspended in RPMI 1640 medium in a concentration of 5 × 106 cells/mL. Briefly, 3 mL of histopaque 1077 were placed in a sterile glass conical tube, then 3 mL of heparinized whole bovine blood were added, without mixing the blood with the reagent. They were centrifuged at 400 g for 30 min, so the cells of the granulocytic series are located between the histopaque 1077 and the red blood cells (located at the bottom of the tube), while the lymphocytes, other mononuclear cells and platelets are in the bottom of the tube. The upper layers were gently extracted with a sterile Pasteur pipette and then transferred to another conical glass tube to resuspend it in 5 volumes of sterile PBS pH 7.4. It was centrifuged for 15 min at 400 g, the supernatant was discarded, and the pellet was resuspended in 10 mL of ammonium chloride (0.85%), shaking slowly for 10 min to lyse the erythrocytes. Then, it was centrifuged at 400 g for 15 min, the supernatant was extracted, and the pellet was resuspended in 6 mL of PBS. Then, it was centrifuged again at 400 g for 10 min, and after discarding the supernatant, it was resuspended in 4 mL of RPMI 1640 medium.
Once the cells were isolated, the cell count was carried out and the viability of the PMNs was evaluated using a Neubauer chamber. The isolated cells were cultured in an oven at 37°C in microaerophilic, corroborating viability every 2 h along 6 h. For this, 2 mL of cell suspension (in RPMI 1640) were placed in 6-well plates at a concentration of 5 × 105 PMNs/mL.
Subsequently, cells were exposed to free EO and NLC-EO in different concentrations (empty nanoparticles were used as control). To achieve the dissolution of the free EO, 2.5% v/v of DMSO was added to the culture medium. In the case of nanoparticles, it was not necessary to add DMSO for solubilization. The EO concentrations evaluated were in the range of 10–0.05 μL/mL. Cell viability was evaluated at 0, 2, 4, and 6 h with vital exclusion technique, described above. For this, at each sampling time, a 10 μL aliquot was placed in the Neubauer chamber together with the trypan blue. In addition to evaluating the free and encapsulated EO, a positive control containing PMNs dissolved in RPMI 1640; and a control with the addition of DMSO 2.5% v/v for the case of EO alone.
2.10 Statistical analysis
The data obtained for inhibitory percentage of biofilm formation and eradication were graphed including ±SD grouping all isolates evaluated and analyzed by a Non-parametric ANOVA Kruskal–Wallis test with post hoc Bonferroni analysis was performed comparing the effect of the EO free and nanoencapsulated and the empty nanoparticles. Results of statistical analyses with a significance level of p < 0.05 were considered significant. Nanoparticles characteristics, release data and cytotoxicity results are shown as mean ± SD.
3 Results
3.1 Nanoparticles characterization and release
The nanoparticles size, Z potential and PdI results are presented in Table 1. Stables NLC were obtained with a narrow size distribution around 180 nm. Through direct observation, no phase separation or precipitation was observed, even after 6 months storage at 4°C. We found that the size of NLC-EO was slightly higher than empty NLC and did not change after the storage. However, the PdI had a very small decrease because of storage and for the Z potential we found an important increased in the value (in module) due the EO encapsulation.

Table 1. NLC formulations: Melaleuca armillaris EO encapsulation efficiency (EE) and Dynamic Light Scattering (DLS) results. A comparison in the properties of fresh and 6 month storaged (4°C) NLC was performed.
TEM image was obtained to confirm the presence of nanoparticles (Figure 1). Spherical NLC were observed in a narrow size distribution. Accordingly, to DLS measurements, the mean size of NLC-EO were around 200 nm.
The EO was efficiently encapsulated at 71.5% ± 5.2% and after 6-month storage did not show significant changes (p˃0.05) with a value of 68.3% ± 4.1% (Table 1). The evaluation of the EO release profile from NLC formulations in PBS buffer at 37°C is shown in Figure 2. The release profile showed a biphasic behavior, with a burst release along the first 6 h, followed by a lower release rate remaining for 72 h (Figure 2). A similar behavior was observed with the EO solubilized in EtOH suggesting an increased solubility of the EO due to vehiculation. On the other hand, the EO incorporated into PBS in the dialysis device did not diffuse through the membrane more than a minimal amount.
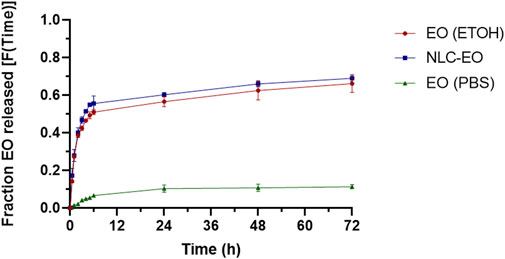
Figure 2. Release profile of the EO from NLC, EO dissolved in PBS and EO dissolved in ethanol (expressed as the fraction of EO released as a function of time). The experimental values are the mean ± SD, n = 3.
3.2 Evaluation of antimicrobial activity of free and nanoencapsulated EO
The pharmacodynamics parameters obtained for planktonic and biofilm cultures are listed in Table 2. The free and nanoencapsulated EO presented a MIC90 and MBC90 of 6.25 μL/mL (this concentration corresponds to 5 mg lipid/mL) against all the isolates evaluated, while empty NLC had no antimicrobial activity on planktonic cultures in the whole range of concentrations (10–0.019 mg lipid/mL). All isolates had a MICB90 of 3.1 μL/mL for the free EO, while this parameter for the NLC-EO was 6.25 μL/mL. The empty nanoparticles (NLC) used as control had an inhibitory effect on biofilm formation also diluting the NLC formulation 128-fold (0.31 mg lipid/mL), but not on bacterial growth inhibition or biofilm eradication even at high concentrations (10 mg lipid/mL). No differences in the inhibition of biofilm formation were observed for EO and NLC-EO in EO concentrations of 1.5, 3.1 and 6.2 μL/mL (p˃0.05) and NLC in the same concentrations contained in NLC-EO (Supplementary Figure S1). Only in a concentration of 0.3 μL/mL the biofilm formation inhibition for EO was higher compared to NLC-EO (0.31 mg lipid/mL) and NLC (0.31 mg lipid/mL) treatments (p < 0.05).

Table 2. Antimicrobial activity of free and encapsulated Melaleuca armillaris EO. MIC90, MBC90, MICB90 and MECB90, are expressed in μL/mL. NA: not active.
On the other hand, free and encapsulated EO demonstrated efficacy for eradicating mature biofilms (Table 2), evaluated by bacterial count and viability with resazurin. In contrast, empty NLCs were inactive in eradicating biofilms. Figure 3 shows the eradication evaluated with violet crystal stain, representing the biofilm mass eradicated independently of its viability. EO and NLC-EO produced high biofilm eradication without significant differences because of the encapsulation (p < 0.05) at concentrations of 6.2, 12.5 and 25 μL/mL. In concentrations of 1.5 and 3.1 μL/mL, eradication by NLC-EO was lower than for free EO but significantly higher than for NLC (p < 0.05).
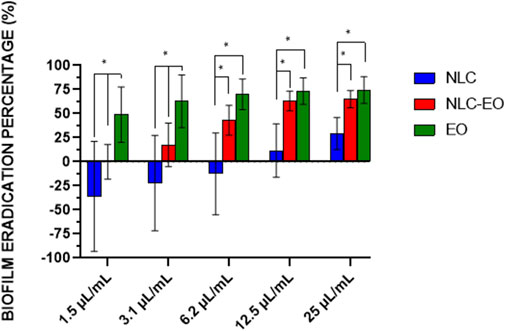
Figure 3. Eradication percentage of biofilm by the action of different concentrations of EO encapsulated in NLC and free EO compared to the untreated control (NLC empty). Error bars represent the standard deviation of all strains evaluated in quadruplicate experiments. The asterisks indicate significant differences (*p < 0.05). The concentration of lipid (mg lipid/mL) is the same in each dilution comparing NLC and NLC-EO, therefore the difference between both is the presence or absence of EO.
3.3 Evaluation of cytotoxicity of free and nanoencapsulated EO
The survival percentage of PMNs relative to the corresponding positive controls was plotted (Figure 4). Free EO was immediately cytotoxic for PMNs at all concentrations tested. Only a concentration of 0.05 μL/mL of EO allowed for maintaining viable PMNs after 6 h, but with a 90% decrease concerning the initial cell count (Figure 4). Encapsulation considerably decreased the cytotoxicity of EO, especially for NLC-EO, concentrations of 0.05 and 0.1 μL/mL of EO did not present significative differences with the NLC (considering the same amount of lipid, 0.04 and 0.08 mg lipid/mL, respectively) (p < 0.05). In higher concentrations NLC-EO was more cytotoxic on PMNs but significantly lower than free EO (p < 0.05).
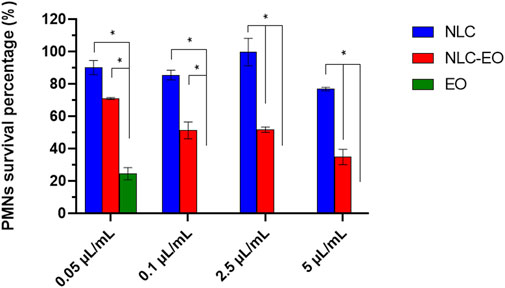
Figure 4. Percentage of survival of polymorphonuclear leukocytes (PMNs) exposed to Melaleuca armillaris EO (EO), EO-containing NLC (NLC-EO) and empty NLC. Error bars represent standard deviation of all strains evaluated in quadruplicate experiments. The asterisks indicate significant differences (*p < 0.05). The concentration of lipid (mg lipid/mL) is the same in each dilution comparing NLC and NLC-EO, therefore the difference between both is the presence or absence of EO.
4 Discussion
4.1 Characterization of NLC, encapsulation and release
According to the literature, nanoparticles with a mean size of less than 200 nm and a Polydispersity index (PdI) of less than 0.3 could be considered as optimal and stable formulations for biological purposes (Rahman et al., 2013; Izham et al., 2019). It was established that good colloidal suspension quality is represented by a PdI value range from 0 to 1, with lower values as indicative of homogeneity (Anton et al., 2007). Another important parameter to evaluate the characteristics of a nanoparticulate system is the Z potential, which provides insights of electrostatic repulsive forces present between nanoparticles that could affect the stability of the suspension. It has been established that this value must be in the order of 30 mV (in module) for preventing the nanoparticles from aggregation and precipitation but without cytotoxic effects (Thatipamula et al., 2011). However, in different publications, it has been shown that colloidal systems such as SLN and NLC are closer to neutrality, being stable even at low values of Z potential (Thatipamula et al., 2011; Izham et al., 2021). Nevertheless, in the case of non-ionic surfactants, the stabilization is produced by steric groups, which contribute to avoiding agglomeration of the nanoparticles (Lee et al., 2007; Honary and Zahir, 2013).
In our study, all the NLC, whether empty or loaded, exhibited low PdI values (see Table 1), indicating a uniform size distribution. It is complicated to compare parameters like size with other studies due to variations in components and synthesis techniques used for NLC production, which affect particle size (Mitrea et al., 2014). Empty NLC exhibited similar values of PdI, size, and Z potential even after 6 months of storage at 4°C. The NLC loaded with the EO presented similar size (185.1–189.5 nm) and PdI (0.18–0.21) after the same period of storage, but the Z potential shifted to more negative values. The addition of EO, which is a liquid oil, in the synthesis of nanoparticles could contribute to the efficiency of NLC structuring. Putranti et al. (2017) reported that higher concentrations of liquid lipids were associated with higher Z potential modulus values. This was observed by achieving higher stability of solid lipid nanoparticles containing terpenes (Rodenak-Kladniew et al., 2023). Over time the terpenes may accommodate themselves into the structure of the nanoparticles, stabilizing it and producing a nanostructuration of the matrix to a less crystalline architecture. Furthermore, it has been reported that higher concentrations of Q10 in ultra-small NLCs were associated with higher absolute Z potential values. According to the authors, this was related to the accumulation of the active ingredient on the surface of the particles rather than in their cores. The accumulation of neutral molecules on the surface of nanoparticles may affect the accumulation of counterions in the Stern layer, resulting in a higher Z potential (Keck et al., 2014). The colloidal suspension did not undergo phase separation or nanoparticle precipitation after the storage and the EO also remained efficiently encapsulated at around 70%. The unencapsulated EO did not affect the nanoparticles stability and probably remained in solution forming micelles with surfactant (Rodenak-Kladniew et al., 2017), keeping the one phase solution obtained initially.
The evaluation of the release profile demonstrated a fast initial EO release from NLC, which may be attributed to the presence of non-encapsulated EO, probably incorporated in surfactant micelles (Rodenak-Kladniew et al., 2017). Additionally, that effect could be also related to the EO superficially incorporated in NLCs. EOs are liquid lipids, could produce imperfect crystalline structure on nanoparticles, favoring the movement of the cargo molecules land improving the diffusion of the EO to the nanoparticle outside (Fang et al., 2013). Rodenak-Kladniew et al. (2017) synthesized SLN loaded with terpenes such as linalool, which exhibited a biphasic release behavior like what we observed, with a rapid initial release in the first 6 h, followed by a slow release continuing until 72 h. Something similar was observed for SLN encapsulating Clinopodium nepeta EO (Rodenak-Kladniew et al., 2023). We also observed a similar behavior when EO was solubilized in ethanol, possibly due to its solubility in PBS facilitated by miscibility in ethanol.
4.2 Antimicrobial activity of NLC nanoparticles loaded with EO from Melaleuca armillaris
Melaleuca armillaris EO exhibited strong antimicrobial activity against S. aureus, as previously reported in other publications (Amri et al., 2012; Ballarre et al., 2022; Buldain et al., 2018; 2020; 2022). Our study also confirmed anti-staphylococcal activity, including MRSA strongly biofilm producers.
The high content of 1,8 cineole may contribute to the antibacterial activity of M. armillaris EO. The permeabilization of membranes in microorganisms such as S. aureus has been attributed as an antimicrobial mechanism due to its great hydrophobicity (Carson et al., 2006; Oliveira et al., 2015; Rueda et al., 2012 found an important activity of this species against S. aureus ATCC 29213 (MIC of 12.4 μg/mL), in which its composition had a high content of 1,8 cineole (82.27%). Also, we found that the MBC90/MIC90 ratio was between 1 and 4, characteristic of bactericides (Pankey and Sabath, 2004), independently of pH. The specific mechanism of action of M. armillaris EO against S. aureus has not yet been investigated. Hayouni et al. (2008) studied the antimicrobial activity of this species against Lactobacillus. As 1,8 cineole was the main component found (68.92%), the authors hypothesized that this compound could destabilize the cytoplasmic membrane of these bacteria, as demonstrated by Li et al. (2014). Hayouni et al. (2008) also implicated the minor components found (α-pinene, terpinene-4-ol, and α-terpinene, among others) as responsible for the antimicrobial action. According to these authors, these molecules interact with the cell membrane, dissolving in the phospholipid bilayer and destabilizing it, increasing fluidity and therefore, passive permeability.
The M. armillaris EO was also active against biofilms produced by S. aureus, favoring both the eradication of preformed biofilms and the inhibition of biofilm formation. Antibiotic treatment of bacterial infections with biofilm development is often ineffective, making it difficult to eradicate such infections, leading to persistent and recurrent infections. This is a serious problem in the control of intramammary infections in cattle (Pedersen et al., 2021). The biofilm eradication test evaluates the effectiveness of a treatment in removing an established biofilm, while the inhibition of biofilm formation test assesses the ability of a treatment to prevent biofilm development from the outset. Both approaches are important because of S. aureus can live in the milk within the mammary gland, and it can also form biofilms turning difficulty the treatment (Vargová et al., 2023). The antibiofilm activity of EOs is widely reported, but not for M. armillaris EO. It is expected that the antimicrobial resistance of S. aureus will increase because of the formation of biofilms with respect to suspension cultures (Vázquez-Sánchez et al., 2015). In our work the MBC90 was 6.25 μL/mL, similar to the MECB90.1,8 Cineole has been reported as an inhibitor of the transcription of genes involved into the formation of S. aureus biofilms (Schürmann et al., 2019).
According to literature, this is the first report of encapsulation of M. armillaris EO into nanoparticles like NLC. Antimicrobial activity against Escherichia coli and S. aureus of M. armillaris EO encapsulated in another drug delivery system such as Bioactive Glass Nanoparticles (BGNs) has been reported (Ballarre et al., 2022; Buldain et al., 2024). Mesoporous glass nanoparticles can offer controlled and sustained release of EOs due to their porosity and modifiable surface. However, lipid carriers can improve the solubility and stability of EOs, facilitating their absorption in biological applications. These systems consist of a mixture of lipid and solid phases that create a disordered liquid lipid matrix, allowing for the incorporation of active substances (Viegas et al., 2023). Lipid systems tend to be more biocompatible and less toxic, which is crucial for healthcare applications. The primary use of BGNs is in the treatment of hard tissues, owing to their inorganic composition and mechanical strength. These properties make them similar to calcified tissues, such as bone, which is typical of bioactive ceramics and glasses (Hooshmand et al., 2021). Mesoporous glass nanoparticles with M. armillaris essential oil have demonstrated great antimicrobial capacity for coating titanium implants, contributing to bone regeneration (Buldain et al., 2024).
In this work, we found that MIC90 of the EO encapsulated in the NLC nanoparticles was the same as that obtained when using the free EO, so the encapsulation does not impair its antimicrobial activity in a suspension culture against S. aureus. Saporito et al., 2017 obtained a similar effect when encapsulating EO from Eucalyptus globulus. Empty NLCs were able to prevent the formation of S. aureus biofilms although they did not have antimicrobial activity on bacteria in suspension or in the eradication of preformed biofilms. One of the components used in the synthesis of NLCs in this study was Pluronic F-68. Bridgett et al. (1992) observed that PF-68 had a strong capacity to inhibit the adhesion and formation of Staphylococcus epidermidis biofilms, while the bacteria remained viable during treatments. This finding may be related to our results, where NLCs did not inhibit the growth of S. aureus but did affect the inhibition of biofilm formation. On the other hand, EO-loaded NLCs turned out to have significant activity both against S. aureus in suspension and in the formation and eradication of biofilms, even for MRSA strains. Therefore, the antimicrobial activity of free and encapsulated EO was similar for planktonic cultures, the difference is observed against biofilm already formed (MICB90 and MECB90 of free EO are slightly lower). This may be because biofilms are structures with higher resistance to antimicrobial action, and the proportion of free EO when working with NLC-EO is a little lower, as can be seen in the figure of the release curve. Furthermore, in microbiological tests, free EO is added directly to the culture medium using Tween 80 to promote its dissolution; therefore, it does not have to cross a physical barrier such as in the dialysis utilizing a diffusion process into an aqueous medium. In this way, the bacteria are in contact with all the EO from the beginning of the assay.
4.3 Cytotoxicity of free and nanoencapsulated Melaleuca armillaris EO
The determination of the intracellular activity of the M. armillaris EO against PMNs has not yet been reported in the literature. The EO turned out to be cytotoxic in a wide range of concentrations (10–0.05 μL/mL). Caldefie-Chézet et al. (2006) found that PMNs, isolated from humans, exposed to 1% M. alternifolia EO lost 50% of their viability after 2 h, while there was no loss of viability at 0.1% EO concentrations, therefore least for 2 hours. On the other hand, using peripheral human mononuclear cells against 0.1% of EO, viability drops to 20% at 30 min and to zero at 3 h, but viability is maintained even for 24 h against a concentration of 0.01%. In another study, Tullio et al. (2012), evaluated the intracellular activity of Thymus vulgaris EO against Candida albicans internalized in human PMNs. The concentrations of EO used were 0.25% and 0.5%, reporting intracellular activity against C. albicans, however, the authors do not mention carrying out viability tests because of EO on PMNs. The reduction in the count of microorganisms could be the result of intracellular killing by the antimicrobial, lysis of the phagocytic cells with the release and subsequent destruction of the microorganisms by the extracellular antimicrobial, elimination of microorganisms by residual antimicrobial after rupture of the cells by sonication at the end of the experiment and the stimulation of the intracellular destruction mechanisms by the action of the antimicrobial (Easmon, 1979). In another work carried out by Tullio et al. (2019) the toxicity of M. alternifolia EO and “Mentha de Pancalieri” on PMNs was evaluated, finding that viability drastically decreased with 0.25% of EOs 30 min after being exposed.
A possible solution to this problem of toxicity of EOs on PMNs is nanoencapsulation as a “delivery” of molecules to the intracellular medium. The nanoparticles penetrate the cell membrane and subcellular organelles, depositing themselves in the infected site, remaining intracellular for a long time and releasing their cargo through the pores that appeared in the nanoparticle membranes (Zhou et al., 2018). There are nanomaterials capable of incorporating one or more molecules without influencing their charge and thus increasing their pharmacological activity. They allow a uniform dosage of the drug, increase its bioavailability, localize it in the infected site, reducing treatment time and side effects (Garg et al., 2015). In this work we managed to reduce the cytotoxicity of EO for PMN cells by incorporating them into NLC nanoparticles. These empty nanoparticles maintained 90% viability compared to the untreated control. In the case of NLC-EO, survival was 70% at the lowest concentration evaluated (0.05 μL/mL) and 30% at the highest concentration evaluated (10 μL/mL). The effect of NLC nanoparticles on the viability of PMN cells has not been reported before. The encapsulation in NLC of EO decreased the amount of this in contact with the eucaryotic cells favoring greater PMN viability. Additionally, Pluronic F-68, which has been reported as a protector of eukaryotic cells (Palomares et al., 2000), may be contributing to the decreased cytotoxicity observed for neutrophils in this study against EO. This effect is likely enhanced by the encapsulation, which reduces the EO concentration to which the cells are exposed. The effects of Pluronic on eukaryotic cell cultures are consistent with a mechanism in which the polymer forms a protective layer on the cell membrane, significantly reducing its hydrophobicity (Tharmalingam et al., 2008).
It is crucial to keep viable PMNs in the mammary gland to fight infections, as they play a vital role in the immune system (Srithanasuwan et al., 2023). Therefore, it is very useful to have antimicrobial formulations that are not only capable of inhibiting S. aureus (growing in suspension and biofilms) in the udder, but also allow the viability of immune cells to be maintained.
5 Conclusion
Our results indicate that M. armillaris essential oil (EO) exhibits strong antimicrobial activity against biofilm-forming MRSA and MSSA strains. Nanostructured lipid carriers (NLCs) show great potential as nanocarriers for hydrophobic compounds like EOs, enhancing their stability and bioavailability. Encapsulating this EO in NLCs not only preserves its antimicrobial activity but also reduces the mortality of polymorphonuclear neutrophils (PMNs) caused by the plant extract. This is crucial for future experiments evaluating the intracellular activity of EO in PMNs, where S. aureus can survive and evade poorly penetrating antibiotics. Additionally, the potential to administer this in an intramammary (IMM) form in the future suggests it will not adversely affect the animal’s immune system.
Data availability statement
The raw data supporting the conclusions of this article will be made available by the authors, without undue reservation.
Ethics statement
The animal study was approved by Institutional Committee for the Control and Use of Animal Laboratories of the Faculty of Veterinary Sciences, UNLP (47.3.15J). The study was conducted in accordance with the local legislation and institutional requirements.
Author contributions
DB: Conceptualization, Data curation, Formal Analysis, Investigation, Methodology, Supervision, Writing–original draft, Writing–review and editing. LG: Methodology, Writing–review and editing. LM: Methodology, Writing–review and editing. AB: Methodology, Writing–review and editing. FH: Methodology, Writing–review and editing. GI: Conceptualization, Supervision, Writing–review and editing, Methodology. NM: Conceptualization, Supervision, Writing–review and editing, Data curation, Funding acquisition, Methodology, Project administration, Resources.
Funding
The author(s) declare that financial support was received for the research, authorship, and/or publication of this article. This work was partially financed by the Laboratory of Pharmacological and Toxicological Studies (LEFyT) and the National Agency for Scientific and Technical Promotion (ANPCyT) (PICT 2020-01429). This work was also supported by UNLP (PPID X077 2022-2023 to GI) and CONICET (PIBAA 1078, PIP 2051, PUE 0010).
Acknowledgments
The authors thank to National Scientific and Technical Research Council (CONICET) for their collaboration in granting Ph.D Scholarships. We want to thank the technical support from Dr. Yamil Chain and Dr. Sebastián Cisneros for the DLS measurements.
Conflict of interest
The authors declare that the research was conducted in the absence of any commercial or financial relationships that could be construed as a potential conflict of interest.
Publisher’s note
All claims expressed in this article are solely those of the authors and do not necessarily represent those of their affiliated organizations, or those of the publisher, the editors and the reviewers. Any product that may be evaluated in this article, or claim that may be made by its manufacturer, is not guaranteed or endorsed by the publisher.
Supplementary material
The Supplementary Material for this article can be found online at: https://www.frontiersin.org/articles/10.3389/fnano.2024.1476423/full#supplementary-material
References
Amri, I., Mancini, E., De Martino, L., Marandino, A., Hamrouni, L., Mohsen, H., et al. (2012). Chemical composition and biological activities of the essential oils from three Melaleuca species grown in Tunisia. Int. J. Mol. Sci. 13 (12), 16580–16591. doi:10.3390/ijms131216580
Antimicrobial Resistance Collaborators. Murray, C. J. L., Ikuta, K. S., Sharara, F., Swetschinski, L., Robles Aguilar, G., et al. (2022). Global burden of bacterial antimicrobial resistance in 2019: a systematic analysis. Lancet 399 (10325), 629–655. doi:10.1016/S0140-6736(21)02724-0
Anton, N., Gayet, P., Benoit, J. P., and Saulnier, P. (2007). Nano-emulsions and nanocapsules by the PIT method: an investigation on the role of the temperature cycling on the emulsion phase inversion. Int. J. Pharm. 344, 44–52. doi:10.1016/j.ijpharm.2007.04.027
Ballarre, J., Buldain, D., Unalan, I., Pastore, J. I., Mestorino, N., and Boccaccini, A. R. (2022). Melaleuca armillaris essential oil as an antibacterial agent: the use of mesoporous bioactive glass nanoparticles as drug carrier. Nanomater. (Basel) 21 (1), 34. doi:10.3390/nano13010034
Begun, J., Sifri, C. D., Goldman, S., Calderwood, S. B., and Ausubel, F. M. (2005). Sthapylococcus aureus virulence factors identified by using a high troughput Caenorhabditis elegans-killing model. Infect. Immun. 73 (2), 872–877. doi:10.1128/IAI.73.2.872-877.2005
Bilia, A. R., Guccione, C., Isacchi, B., Righeschi, C., Firenzuoli, F., and Bergonzi, M. C. (2014). Essential oils loaded in nanosystems: a developing strategy for a successful therapeutic approach. Evid. Based Complement. Altern. Med. 2014, 651593. doi:10.1155/2014/651593
Bradley, A. J., and Green, M. J. (2004). The importance of the nonlactating period in the epidemiology of intramammary infection and strategies for prevention. Vet. Clin. North Am. Food Anim. Pract. 20 (3), 547–568. doi:10.1016/j.cvfa.2004.06.010
Bridgett, M. J., Davies, M. C., and Denyer, S. P. (1992). Control of staphylococcal adhesion to polystyrene surfaces by polymer surface modification with surfactants. Biomaterials 13 (7), 411–416. doi:10.1016/0142-9612(92)90159-l
Buldain, D., Buchamer, A. V., Marchetti, M. L., Aliverti, F., Bandoni, A., and Mestorino, N. (2018). Combination of cloxacillin and essential oil of Melaleuca armillaris as an alternative against Staphylococcus aureus. Front. Vet. Sci. 2 (5), 177. doi:10.3389/fvets.2018.00177
Buldain, D., Diaz, F., Unalan, I., Mestorino, N., Boccaccini, A. R., and Ballarre, J. (2024). Regional chitosan and Melaleuca armillaris essential oil with mesoporous glass particles for enhancing bioactive and antibacterial behaviour of Ti6Al4V implants. Arab. J. Sci. Eng. doi:10.1007/s13369-024-09414-7
Buldain, D., Gortari Castillo, L., Buchamer, A. V., Aliverti, F., Bandoni, A., Marchetti, L., et al. (2020). Melaleuca armillaris essential oil in combination with rifaximin against Staphylococcus aureus isolated of dairy cows. Front. Vet. Sci. 15 (7), 344. doi:10.3389/fvets.2020.00344
Buldain, D., Gortari Castillo, L., Buchamer, A. V., Bandoni, A., Marchetti, L., and Mestorino, N. (2022). In vitro synergistic interaction between Melaleuca armillaris essential oil and erythromycin against Staphylococcus aureus isolated from dairy cows. Front. Vet. Sci. 15 (9), 1005616. doi:10.3389/fvets.2022.1005616
Buldain, D., Gortari Castillo, L., Marchetti, M. L., Julca Lozano, K., Bandoni, A., and Mestorino, N. (2021). Modeling the growth and death of Staphylococcus aureus against Melaleuca armillaris essential oil at different pH conditions. Antibiot. (Basel) 10 (2), 222. doi:10.3390/antibiotics10020222
Caldefie-Chézet, F., Fusillier, C., Jarde, T., Laroye, H., Damez, M., Vasson, M. P., et al. (2006). Potential anti-inflammatory effects of Melaleuca alternifolia essential oil on human peripheral blood leukocytes. Phytother. Res. 20, 364–370. doi:10.1002/ptr.1862
Carson, C. F., Hammer, K. A., and Riley, T. V. (2006). Melaleuca alternifolia (tea tree) oil: a review of antimicrobial and other medicinal properties. Clin. Microbiol. Rev. 19 (1), 50–62. doi:10.1128/CMR.19.1.50-62.2006
Chabir, N., Romdhane, M., Valentin, A., Moukarzel, B., Marzoug, H. N., Brahim, N. B., et al. (2011). Chemical study and antimalarial, antioxidant, and anticancer activities of Melaleuca armillaris (Sol Ex Gateau) Sm essential oil. J. Med. Food. 14 (11), 1383–1388. doi:10.1089/jmf.2010.0168
Davis, R. L. (2003). The Australian tea tree oil industry. Paper presented at the IFEAT International Conference ‘Australia and New Zealand: Essential Oils and Aroma Chemicals-Production and Markets’; Sydney, 2-6 Nov. 2003. 29–40.
Dutescu, I. A., and Hillier, S. A. (2021). Encouraging the development of new antibiotics: are financial incentives the right way forward? A systematic review and case study. Infect. Drug Resist. 14, 415–434. doi:10.2147/IDR.S287792
Easmon, C. S. (1979). The effect of antibiotics on the intracellular survival of Staphylococcus aureus in vitro. Br. J. Exp. Pathol. 60 (1), 24–28.
Falcão, D. Q., Oliveira, A. P., Lima, B. G., Cardoso, A. C., Almeida, K. B., Santos, T. C., et al. (2018). “Chapter 3 Nanotechnology in phytotherapy: current challenges of lipid-based nanocarriers for the delivery of natural products,”. Lipid nanocarriers for drug targeting. Editor A. M. Grumezescu (Norwich, NY, USA: William Andrew Publishing), 2018, 139–174.
Fang, C. L., Al-Suwayeh, S. A., and Fang, J. Y. (2013). Nanostructured lipid carriers (NLCs) for drug delivery and targeting. Rec. Pat. Nanotechnol. 7, 41–55. doi:10.2174/1872210511307010041
Garg, T., Rath, G., Murthy, R. R., Gupta, U. D., Vatsala, P. G., and Goyal, A. K. (2015). Current nanotechnological approaches for an effective delivery of bioactive drug molecules to overcome drug resistance tuberculosis. Curr. Pharm. Des. 21 (22), 3076–3089. doi:10.2174/1381612821666150531163254
Guimarães, F. F., Manzi, M. P., Joaquim, S. F., Richini-Pereira, V. B., and Langoni, H. (2017). Short communication: outbreak of methicillin-resistant Staphylococcus aureus (MRSA)-associated mastitis in a closed dairy herd. J. Dairy Sci. 100 (1), 726–730. doi:10.3168/jds.2016-11700
Hayouni, E. A., Bouix, M., Abedrabba, M., Leveau, J. Y., and Hamdi, M. (2008). Mechanism of action of Melaleuca armillaris (Sol. Ex Gaertu) Sm. essential oil on six LAB strains as assessed by multiparametric flow cytometry and automated microtiter-based assay. Food Chem. 111 (3), 707–718. doi:10.1016/j.foodchem.2008.04.044
Hendriksen, R. S., Mevius, D. J., Schroeter, A., Teale, C., Meunier, D., Butaye, P., et al. (2008). Prevalence of antimicrobial resistance among bacterial pathogens isolated from cattle in different European countries: 2002-2004. Acta Vet. scan. 50 (1), 28. doi:10.1186/1751-0147-50-28
Honary, S., and Zahir, F. (2013). Effect of zeta potential on the properties of nano-drug delivery systems—a review (part 1). Trop. J. Pharm. Res. 12 (2), 255–264. doi:10.4314/tjpr.v12i2.19
Hooshmand, S., Mollazadeh, S., Akrami, N., Ghanad, M., El-Fiqi, A., Baino, F., et al. (2021). Mesoporous silica nanoparticles and mesoporous bioactive glasses for wound management: from skin regeneration to cancer therapy. Materials 14 (12), 3337. doi:10.3390/ma14123337
Islan, G. A., Cacicedo, M. L., Rodenak-Kladniew, B., Duran, N., and Castro, G. R. (2017). Development and tailoring of hybrid lipid nanocarriers. Curr. Pharm. Des. 23, 6643–6658. doi:10.2174/1381612823666171115110639
Izham, M. N. M., Hussin, Y., Aziz, M. N. M., Yeap, S. K., Rahman, H. S., Masarudin, M. J., et al. (2019). Preparation and characterization of self nano-emulsifying drug delivery system loaded with citraland its antiproliferative effect on colorectal cells in vitro. Nanomater. Basel, Switz. 9 (7), 1028. doi:10.3390/nano9071028
Izham, M. N. M., Hussin, Y., Rahim, N. F. C., Aziz, M. N. M., Yeap, S. K., Rahman, H. S., et al. (2021). Physicochemical characterization, cytotoxic effect and toxicity evaluation of nanostructured lipid carrier loaded with eucalyptol. BMC Complement. Med. Ther. 7 (1), 254. doi:10.1186/s12906-021-03422-y
Keck, C. M., Baisaeng, N., Durand, P., Prost, M., Meinke, M. C., and Müller, R. H. (2014). Oilenriched, ultra-small nanostructured lipid carriers (usNLC): a novel delivery system based on flip–flop structure. Int. J. Pharm. 477 (1–2), 227–235. doi:10.1016/j.ijpharm.2014.10.029
Lee, M.-K., Lim, S.-J., and Kim, C.-K. (2007). Preparation, characterization and in vitro cyto-toxicity of paclitaxel-loaded sterically stabilized solid lipid nanoparticles. Biomaterials 28 (12), 2137–2146. doi:10.1016/j.biomaterials.2007.01.014
Li, L., Li, Z. W., Yin, Z. Q., Wei, Q., Jia, R. Y., Zhou, L. J., et al. (2014). Antibacterial activity of leaf essential oil and its constituents from Cinnamomum longepaniculatum. Int. J. Clin. Exp. Med. 7 (7), 1721–1727.
Medina-Montano, C., Rivero Berti, I., Gambaro, R. C., Limeres, M. J., Svensson, M., Padula, G., et al. (2022). Nanostructured lipid carriers loaded with dexamethasone prevent inflammatory responses in primary non-parenchymal liver cells. Pharmaceutics 14 (8), 1611. doi:10.3390/pharmaceutics14081611
Menossi, M., Tejada, G., Colman, S. L., Nercessian, D., Mendieta, J. R., Islan, G. A., et al. (2024). Cannabis extract-loaded lipid and chitosan-coated lipid nanoparticles with antifungal activity. Colloids Surf. A Physicochem. Eng. Asp. 685, 133207. doi:10.1016/j.colsurfa.2024.133207
Mitrea, E., Ott, C., and Meghea, A. (2014). New approaches on the synthesis of effective nanostructured lipid carriers. Rev. Chim. 65 (1), 50–55.
Monistero, V., Graber, H. U., Pollera, C., Cremonesi, P., Castiglioni, B., Bottini, E., et al. (2018). Staphylococcus aureus isolates from bovine mastitis in eight countries: genotypes, detection of genes encoding different toxins and other virulence genes. Toxins 10 (6), 247. doi:10.3390/toxins10060247
Oliveira, K. A. R., Sousa, J. P., Medeiros, J. A. C., Figueiredo, R. C. B. Q., Maganni, M., Leite de Souza, E., et al. (2015). Synergistic inhibition of bacteria associated with minimally processed vegetables in mixed culture by carvacrol and 1,8-cineole. Food control. 47, 334–339. doi:10.1016/j.foodcont.2014.07.014
Palomares, L. A., González, M., and Ramírez, O. T. (2000). Evidence of pluronic F-68 direct interaction with insect cells: impact on shear protection, recombinant protein, and baculovirus production. Enzym. Microb. Technol. 26, 324–331. doi:10.1016/s0141-0229(99)00176-3
Pankey, G. A., and Sabath, L. D. (2004). Clinical relevance of bacteriostatic versus bactericidal mechanisms of action in the treatment of gram-positive bacterial infections. Clin. Infect. Dis. 38 (6), 864–870. doi:10.1086/381972
Pedersen, R. R., Krömker, V., Bjarnsholt, T., Dahl-Pedersen, K., Buhl, R., and Jørgensen, E. (2021). Biofilm research in bovine mastitis. Front. Vet. 8, 656810. doi:10.3389/fvets.2021.656810
Peyrusson, F., Varet, H., Nguyen, T. K., Legendre, R., Sismeiro, O., Coppée, J. Y., et al. (2020). Intracellular Staphylococcus aureus persisters upon antibiotic exposure. Nat. Commun. 11 (1), 2200. doi:10.1038/s41467-020-15966-7
Provenzani, R., San-Martin-Galindo, P., Hassan, G., Legehar, A., Kallio, A., Xhaard, H., et al. (2021). Multisubstituted pyrimidines effectively inhibit bacterial growth and biofilm formation of Staphylococcus aureus. Sci. Rep. 11 (1), 7931. doi:10.1038/s41598-021-86852-5
Putranti, A. R., Primaharinastiti, R., and Hendradi, E. (2017). Effectivity and physicochemical stability of nanostructured lipid carrier coenzyme Q10 in different ratio of lipid alfa cetyl palmitate and alpha tocopheryl acetate as carrier. Asian J. Pharm. Clin. Res. 10 (2), 146–152. doi:10.22159/ajpcr.2017.v10i2.14835
Rahman, H. S., Rasedee, A., How, C. W., Abdul, A. B., Zeenathul, N. A., Othman, H. H., et al. (2013). Zerumbone-loaded nanostructured lipid carriers: preparation, characterization, and antileukemic effect. Int. J. Nanomed. 8, 2769–2781. doi:10.2147/IJN.S45313
Rajala-Schultz, P., Nødtvedt, A., Halasa, T., and Persson Waller, K. (2021). Prudent use of antibiotics in dairy cows: the nordic approach to udder health. Front. Vet. Sci. 8, 623998. doi:10.3389/fvets.2021.623998
Ribeiro, L. N., Breitkreitz, M. C., Guilherme, V. A., da Silva, G. H., Couto, V. M., Castro, S. R., et al. (2017). Natural lipids-based NLC containing lidocaine: from pre-formulation to in vivo studies. Eur. J. Pharm. Sci. 106, 102–112. doi:10.1016/j.ejps.2017.05.060
Rodenak-Kladniew, B., Castro, M. A., Gambaro, R. C., Girotti, J., Cisneros, J. S., Viña, S., et al. (2023). Cytotoxic screening and enhanced anticancer activity of Lippia alba and Clinopodium nepeta essential oils-loaded biocompatible lipid nanoparticles against lung and colon cancer cells. Pharmaceutics 15 (8), 2045. doi:10.3390/pharmaceutics15082045
Rodenak-Kladniew, B., Islan, G. A., de Bravo, M. G., Durán, N., and Castro, G. R. (2017). Design, characterization and in vitro evaluation of linalool-loaded solid lipid nanoparticles as potent tool in cancer therapy. Colloids Surf. B Biointerfaces 154, 123–132. doi:10.1016/j.colsurfb.2017.03.021
Rueda, Y., Cuadro, X., and Mogollón, O. F. (2012). Composición química y actividad antibacteriana del AE de las especies Eucalyptus globulus y E. camaldulensis de tres zonas de Pamplona (Colombia). Bistua Rev. Fac. Ciencias Básicas. 10 (1), 52–61.
Saporito, F., Sandri, G., Bonferoni, M. C., Rossi, S., Boselli, C., Icaro Cornaglia, A., et al. (2017). Essential oil-loaded lipid nanoparticles for wound healing. Int. J. Nanomedicine. 13, 175–186. doi:10.2147/IJN.S152529
Schürmann, M., Oppel, F., Gottschalk, M., Büker, B., Jantos, C. A., Knabbe, C., et al. (2019). The therapeutic effect of 1,8-cineol on pathogenic bacteria species present in chronic rhinosinusitis. Front. Microbiol. 22 (10), 2325. doi:10.3389/fmicb.2019.02325
Shidhaye, S. S., Vaidya, R., Sutar, S., Patwardhan, A., and Kadam, V. J. (2008). Solid lipid nanoparticles and nanostructured lipid carriers--innovative generations of solid lipid carriers. Curr. drug Deliv. 5 (4), 324–331. doi:10.2174/156720108785915087
Sifri, C. D., Barech-Bernal, A., Calderwood, S. B., and von Eiff, C. (2006). Virulence of Staphylococcus aureus small colony variants in the Caenorhabditis elegans infection model. Infect. Immun. 74 (2), 1091–1096. doi:10.1128/IAI.74.2.1091-1096.2006
Srednika, M. E., Crespia, E., Testorellia, M. F., Puigdevalla, T., Pereyra, A. M. D., Rumi, M. V., et al. (2019). First isolation of a methicillin-resistant Staphylococcus aureus from bovine mastitis in Argentina. Vet. Anim. Sci. 7, 100043. doi:10.1016/j.vas.2018.11.004
Srithanasuwan, A., Tata, L., Tananupak, W., Jaraja, W., Suriyasathaporn, W., and Chuammitri, P. (2023). Exploring the distinct immunological reactions of bovine neutrophils towards major and minor pathogens responsible for mastitis. Int. J. Vet. Sci. Med. 11 (1), 106–120. doi:10.1080/23144599.2023.2262250
Tharmalingam, T., Ghebeh, H., Wuerz, T., and Butler, M. (2008). Pluronic enhances the robustness and reduces the cell attachment of mammalian cells. Mol. Biotechnol. 39 (2), 167–177. doi:10.1007/s12033-008-9045-8
Thatipamula, R., Palem, C., Gannu, R., Mudragada, S., and Yamsani, M. (2011). Formulation and in vitro characterization of domperidone loaded solid lipid nanoparticles and nanostructured lipid carriers. Daru 19 (1), 23–32.
Tullio, V., Mandras, N., Allizond, V., Nostro, A., Roana, J., Merlino, C., et al. (2012). Positive interaction of thyme (red) essential oil with human polymorphonuclear granulocytes in eradicating intracellular Candida albicans. Planta medica. 78 (15), 1633–1635. doi:10.1055/s-0032-1315153
Tullio, V., Roana, J., Scalas, D., and Mandras, N. (2019). Enhanced killing of Candida krusei by polymorphonuclear leucocytes in the presence of subinhibitory concentrations of Melaleuca alternifolia and “mentha of Pancalieri” essential oils. Molecules 24 (21), 3824. doi:10.3390/molecules24213824
Vandenesch, F., Naimi, T., Enright, M. C., Lina, G., Nimmo, G. R., Heffernan, H., et al. (2003). Community-acquired methicillin-resistant Staphylococcus aureus carrying Panton-Valentine leukocidin genes: worldwide emergence. Emerg. Infect. Dis. 9 (8), 978–984. doi:10.3201/eid0908.030089
Vargová, M., Zigo, F., Výrostková, J., Farkašová, Z., and Rehan, I. F. (2023). Biofilm-producing ability of Staphylococcus aureus obtained from surfaces and milk of mastitic cows. Vet. Sci. 10, 386. doi:10.3390/vetsci10060386
Vázquez-Sánchez, D., Cabo, M. L., and Rodríguez-Herrera, J. J. (2015). Antimicrobial activity of essential oils against Staphylococcus aureus biofilms. Food. Sci. Technol. Int. 21 (8), 559–570. doi:10.1177/1082013214553996
Viegas, C., Patrício, A. B., Prata, J. M., Nadhman, A., Chintamaneni, P. K., and Fonte, P. (2023). Solid lipid nanoparticles vs. nanostructured lipid carriers: a comparative review. Pharmaceutics 15 (6), 1593. doi:10.3390/pharmaceutics15061593
World Health Organization (2020). La falta de nuevos antibióticos pone en peligro los esfuerzos mundiales por contener las infecciones farmacorresistentes. Press release. Available at: https://www.who.int/es/news/item/17-01-2020-lack-of-new-antibiotics-threatens-global-efforts-to-contain-drug-resistant-infections (Accessed July 10, 2024).
World Health Organization (2021). Resistencia a los antimicrobianos. Available at: https://www.who.int/es/news-room/fact-sheets/detail/antimicrobial-resistance (Accessed July 10, 2024).
Yap, P. S., Yiap, B. C., Ping, H. C., and Lim, S. H. E. (2014). Essential oils, A new horizon in combating bacterial antibiotic resistance. Open Microbiol. J. 8, 6–14. doi:10.2174/1874285801408010006
Keywords: Melaleuca armillaris, essential oil, nanostructured lipid carriers, Staphylococcus aureus, MSSA, MRSA
Citation: Buldain D, Gortari Castillo L, Marchetti L, Buchamer A, Honor Irala F, Islan G and Mestorino N (2024) Antimicrobial activity of nanostructured lipid carriers loaded with Melaleuca armillaris essential oil against Staphylococcus aureus sensitive and resistant to methicillin. Front. Nanotechnol. 6:1476423. doi: 10.3389/fnano.2024.1476423
Received: 05 August 2024; Accepted: 10 December 2024;
Published: 23 December 2024.
Edited by:
Themis Prodromakis, University of Edinburgh, United KingdomReviewed by:
Ayesha Sadaf, Nottingham Trent University, United KingdomRuixiang Li, Shanghai University of Traditional Chinese Medicine, China
Yuwei He, Cedars Sinai Medical Center, United States
Arvind P. Singh, Jawaharlal Nehru University, India
Copyright © 2024 Buldain, Gortari Castillo, Marchetti, Buchamer, Honor Irala, Islan and Mestorino. This is an open-access article distributed under the terms of the Creative Commons Attribution License (CC BY). The use, distribution or reproduction in other forums is permitted, provided the original author(s) and the copyright owner(s) are credited and that the original publication in this journal is cited, in accordance with accepted academic practice. No use, distribution or reproduction is permitted which does not comply with these terms.
*Correspondence: Daniel Buldain, ZGJ1bGRhaW5AZmN2LnVubHAuZWR1LmFy