- 1Telecommunication and Control Engineering Department, Polytechnic School of the University of São Paulo, São Paulo, Brazil
- 2Electronic Systems Engineering Department, Polytechnic School of the University of São Paulo, São Paulo, Brazil
- 3Institute for Technological Research (IPT)—Laboratory of Industrial Biotechnology, Group for Bionanomanufacturing (BIONANO), São Paulo, Brazil
In this study, we delved into cutting-edge strategies for the effective management of wastewater, a critical issue exacerbated by industrial pollution and urban expansion. We introduce the use of carbon-based nanomaterials (CBNs), either alone or functionalized with bacteria, as a novel nanobiotechnological solution for urgent nanobioremediation needs. This technique is notable for its exceptional ability to remove various industrial pollutants, including heavy metals, pesticides, textiles, and dyes, emphasizing the pivotal role of CBNs. The development of bionanocomposites through the integration of CBNs with bacteria represents a significant advancement in enhancing bioremediation efforts. In this study, we assessed the potential health and environmental risks associated with CBN usage while offering an in-depth evaluation of the adsorption mechanisms and factors influencing bioremediation effectiveness. Furthermore, the improved efficiency in treating industrial effluents facilitated by bionanocomposites was investigated, and their alignment with circular economy principles through recyclability is discussed. We aimed to provide, a detailed overview of recent advancements, challenges, and prospects for CBNs and bacterial application in sophisticated wastewater treatment, underscoring their vital importance in promoting the environment.
1 Introduction
1.1 Nanobioremediation and the challenges of industrial effluent in the era of sustainability
Efficiently managing industrial effluents is essential for preserving the environment and safeguarding public health. The UN World Water Development Report has emphasized the pressing need for clean water and sanitation, underscoring the importance of nanobioremediation in addressing these challenges (Ningombam et al., 2024b; Gwenzi et al., 2024). Enhancing bioremediation through bioaugmentation and biostimulation poses challenges such as competition from native microorganisms and the potential risks of eutrophication. The effectiveness of bioremediation can vary depending on environmental conditions and the types of pollutants present. It may also face challenges when dealing with resistant pollutants, which can impact its speed and responsiveness (Ashkanani et al., 2024; Tripathi et al., 2024).
Nanobioremediation combines the power of nanotechnology with bioremediation to tackle the obstacles faced by conventional wastewater treatment methods. This innovative approach improves the breakdown of pollutants and minimizes the impact of environmental factors. This method utilizes nanoparticles and carbon-based nanomaterials (CBNs) to effectively target both organic and inorganic pollutants. As a result, it greatly enhances the efficiency and cost-effectiveness of bioremediation practices, while also aligning with goals of environmental sustainability (Chauhan et al., 2023; Chauhan et al., 2023; Xiang et al., 2023; Dey et al., 2024a; Ningombam et al., 2024a; Singh et al., 2024a; Singh et al., 2024b; Ezeorba et al., 2024).
Significant improvements have been made in pollutant removal efficiency with carbon-based nanomaterials such as graphene and carbon nanotubes in nanobioremediation. The adaptability and interaction of these materials with microorganisms provide a sustainable solution to industrial pollution. They have proven to be effective in treating heavy metals, dye, pesticide, and complex pollutants, thereby promoting environmental sustainability (Chauhan et al., 2023; Xiang et al., 2023; Ningombam et al., 2024a; Ningombam et al. 2024a; Ningombam et al. 2024a; Chen, Liu, and Liu, 2024; Kaur et al., 2024; Ogunsola et al., 2024; Sornaly et al., 2024; Sornaly et al., 2024).
Our work highlights the importance of interdisciplinary collaboration in advancing global environmental sustainability. By introducing an innovative approach to wastewater treatment using carbon-based nanomaterials (CBNs) in conjunction with bacteria, we demonstrate remarkable efficacy in removing industrial pollutants, emphasizing the pivotal role of CBNs in nanobioremediation. The integration of CBNs with bacteria to form bionanocomposites represents a significant advancement, with research focusing on molecular mechanisms to enhance pollution remediation strategies. Additionally, our study addresses critical challenges such as sustainability, nanomaterial recovery, and circular economy, while advocating for collaborative efforts to propel future nanobioremediation research towards environmental sustainability and environmental preservation.
2 Materials and methods
2.1 Parameters considered in this review—identification of relevant studies
We systematically searched the following electronic databases to develop this review and meta-analysis: PubMed-NCBI, Google Scholar, and ScienceDirect. Only articles published in the last 2 years or articles that were fundamental to this research were included. The major scientific articles were selected using the following relevant keywords: “nanobioremediation” OR “carbon-based nanomaterials” OR “genetic engineer” OR “industrial effluents” OR “wastewater treatment” OR “bacteria” OR “bionanocomposite” OR “nanomaterials/functionalization OR “genetic engineer” OR “circular economy”. The following inclusion criteria were used to select the literature for this study: 1) An initial literature search of articles containing the exact keywords; 2) a subsequent search of selected articles containing related words; and 3) reviews published in English. We did not include articles that 1) were not written in English or 2) did not contain the exact and/or associated words cited in the title or abstract of the reference article. Two reviewers independently screened the articles. A total of 126 articles were included in this review based on the relevance of the results and the use of nanotechnologies, particularly CBNs and bacteria, for the bioremediation of industrial residues. Therefore, we considered research articles, review articles, and prospective studies focusing on the bioremediation of microorganisms associated with the use of CBNs as carrier effects and industrial residue degradability agents.
2.2 Outline the experimental setup
The manuscript critically examines the methodologies of selected studies on the use of bacteria and carbon nanomaterials for industrial waste cleanup, emphasizing replicable experimental and analytical methods, as delineated in Figure 1.
a) Identification required the collection of articles using specific keywords from databases.
b) The screening process involved carefully reviewing abstracts to determine their relevance to the topic, followed by thorough assessments of the full-text articles. Non-English works were not included in the evaluation.
c) The final selection comprised studies that met the criteria and had a specific focus on nanomaterials and bacterial bioremediation.

Figure 1. Presents a step-by-step process for conducting a thorough literature review. It involves searching various databases using specific keywords, followed by a careful evaluation of abstracts and full texts in English to determine relevance. Finally, studies that specifically address the use of carbon nanomaterials and bacteria for bioremediation of industrial waste are included.
3 Industrial effluent treatment: the new frontier has arrived
3.1 Innovations in bacterial genetics and molecular techniques that improve the bioremediation of industrial effluent
We present a notable breakthrough in bioremediation, specifically highlighting the use of genetically modified bacteria to address challenging contaminants found in industrial wastewater. Genetic modifications seek to enhance the capacity of bacteria to break down substances that are typically resistant in the environment. Notable advancements include the following:
1) Special Metabolic Pathway Modifications: Techniques enable the introduction of genes that encode enzymes with the remarkable ability to break down specific pollutants. For instance, the xylE gene from P. putida is utilized for xylene degradation. An intriguing case involves the genetic modification of P. putida, which incorporates the todC1 gene (Xue et al., 2022). This modification significantly enhances the capacity of bactéria to degrade toluene, resulting in a remarkable improvement in decontamination efficiency compared to that of nonmodified strains (Chunyan et al., 2023; Maurya et al., 2023).
2) Physiological Adaptations for Pollutant Resistance: Various techniques have been employed to enhance the resistance of bacteria to toxic conditions, such as high concentrations of heavy metals. For instance, the introduction of the acrB gene into Escherichia coli has proven effective in developing bacteria with enhanced resistance. This remarkable adaptation enables bacteria to not only survive but also thrive in extremely challenging environments. Genetic modifications enhance bacteria’s ability to withstand toxic compounds, enabling them to thrive in polluted environments. The task at hand is to modify the bacterial efflux system to eliminate detrimental substances (Li et al., 2024; Wang et al., 2024a).
3) Genetic Modification Techniques Utilizing the CRISPR-Cas9 Technology: Cutting-edge methods, such as CRISPR-Cas9, are utilized to manipulate the bacterial genome, enabling the manipulation of DNA sequences through insertion, deletion, or modification. This technique enables precise modifications in bacterial gene expression, enhancing the synthesis of crucial enzymes involved in the breakdown of pollutants. One example involves improving the capacity of P. putida to produce catechol 2,3-dioxygenase, which is essential for breaking down complex xenobiotics such as aromatic compounds (A. K. Verma et al., 2024; Zhao et al., 2024).
One fascinating aspect of this research involves the development of bacteria that can break down polycyclic aromatic hydrocarbons (PAHs) and process bisphenol A (BPA), a harmful organic pollutant that disrupts the endocrine system. These genetic engineering advancements showcase the immense potential for improving bioremediation techniques, providing a more efficient and environmentally conscious method for treating industrial effluent (Aryal, 2024; Wang et al., 2024a; Wang et al., 2024b; Nandy and Kapley, 2024; Venkatraman et al., 2024; Venkatraman et al., 2024). One of the fascinating aspects of this research is the exploration of previously unknown metabolic pathways and the identification of key genes involved in the breakdown of challenging pollutants.
The progress in bioremediation technologies highlights a notable shift toward more sustainable and eco-friendly approaches to handling industrial pollutants, laying the foundation for a cleaner and more sustainable future. Different genetically distinct bacteria are involved in the bioremediation of industrial pollutants as in Table 1. The examples provided cover a wide range of applications, showcasing the effectiveness and precision of different bacterial species and their genetic mechanisms in tackling pollutants from different industrial sectors (Chauhan et al., 2023; Dey et al., 2024a; Wang et al., 2024b).
The following is a summary of bacteria commonly used in bioremediation:• P. putida is highly skilled in enzymatic degradation and catabolic processes. It excels in breaking down aromatic hydrocarbons and phenols, which are commonly found in oil refineries and the chemical industry. With the help of the toluene/benzene degradation pathway (Tod genes) and the xylulose reductase enzyme (Xyl gene), this degradation process has achieved an impressive efficiency of 70%–90% (Gao, Guo, and Niu, 2024; Gutiérrez et al., 2024).• Bacillus subtilis utilizes biosorption and enzymatic reduction mechanisms to effectively reduce heavy metals and dyes commonly present in the mining and textile industries. This process is facilitated by the presence of arsenate reductase genes (arsC), resulting in an impressive degradation efficiency of 60%–80% (Wu et al., 2023a).• E. coli is vital in the chemical transformation and biosorption of volatile organic compounds and heavy metals, causing it to be critical in the chemical and metallurgical sectors. The cueO and cusA genes are crucial in maintaining a copper balance, exhibiting 50%–70% efficiency in copper degradation (Kojima et al., 2024).• Sphingomonas sp. is highly proficient in the enzymatic breakdown of polychlorinated biphenyls (PCBs) and dioxins. The bph and dxn genes are crucial in facilitating the degradation of biphenyl and toluene/xylene, respectively. This leads to an efficiency of 80%–95%, which is vital for industries such as paper, pulp, and plastics (Sorouri et al., 2023).• Deinococcus radiodurans is widely recognized for its remarkable resistance to radiation and unique ability to remove heavy metals from the environment. The drA and pprA genes have been identified as crucial factors in these exceptional traits. This bacterium has proven to be highly effective in addressing heavy metals and radioactive waste, especially in the fields of nuclear energy and waste treatment. It has demonstrated an impressive degradation efficiency ranging from 65% to 85% (Wang et al., 2024a; Li et al., 2024).
Table 1 presents a thorough examination of different bacteria and their role in the purification of industrial effluents through bioremediation. We provide a comprehensive analysis of the physiological mechanisms employed by bacteria, the specific genes involved in these mechanisms, the types of contaminants they can target, the industries where these pollutants are frequently encountered, and the varying degrees of degradation efficiency exhibited by different bacterial species (Aguilar-Romero et al., 2024; Ezeorba et al., 2024; Huang et al., 2024; Ray and Pattnaik, 2024; Sajjad et al., 2024; Yang et al., 2024).
*Please be aware that the “Degradation Efficiencies (%)” provided are approximate values obtained from scientific literature and may differ depending on the specific research being conducted. Several variables can influence the effectiveness of degradation, such as the type of bacteria, the environment in which degradation occurs, and the presence of other microbes or chemicals. The relationship between pollutants and industries is intricate and influenced by a variety of factors, including processes, legislation, and environmental management methods. The table provided offers a simplified framework that can be used as a basis for conducting a more extensive study.
Further examples highlight the wide range of bacterial capabilities in environmental remediation. For instance, Alcaligenes faecalis can treat cadmium and nickel, Mycobacterium vanbaalenii can breakdown polycyclic aromatic hydrocarbons, and Lysinibacillus sphaericus can detoxify mercury. Thus, this manuscript highlights the significance of utilizing the genetic and physiological diversity of bacteria to create specific bioremediation approaches, which hold great potential for effectively removing industrial pollutants and detoxifying the environment (Ray and Pattnaik, 2024; Xu et al., 2024). The fusion of these biological insights with nanotechnology may revolutionize environmental protection and sustainability. Further research in this interdisciplinary field may greatly improve our capacity to address the effects of industrial pollutants, promoting a cleaner and more sustainable future (Chauhan et al., 2023; Ningombam et al., 2024a).
This summary emphasizes the pivotal role of genetically modified bacteria and their molecular pathways in bioremediating industrial effluents, illustrating the broad spectrum of contaminants they can address. This highlights the need for the integration of genetic and molecular innovations to improve bioremediation efficiency, as demonstrated by Wang et al., who showcased the effectiveness of genetically engineered bacteria in detoxifying wastewater (Zhang et al., 2022; Wang Xin et al., 2024; Sajjad et al., 2024). This breakthrough underlines the potential of genetic engineering to provide targeted, eco-friendly solutions for effluent treatment and promote sustainable environmental practices. Advancements in molecular engineering and synthetic biology are crucial for developing effective microbial systems capable of pinpointing and eliminating pollutants, thereby enhancing bioremediation methods, and supporting green decontamination techniques (Xue et al., 2022; Elmore et al., 2023; Hassanien et al., 2023; Wang et al., 2024a; Chen, Liu, and Liu, 2024).
3.2 How can industrial effluent treatment be enhanced through the functionalization and optimization of carbon-based nanomaterials?
CBNs, including CNTs, quantum dots (QDs), carbon fibers (CF), and graphene (G), are key for treating a variety of industrial wastes due to their superior adsorption capabilities. These nanomaterials excel in contaminant removal and wastewater purification by effectively adsorbing heavy metals such as lead, mercury, cadmium, and arsenic, thus mitigating water pollution and protecting human health and the environment (Ahmed et al., 2022). Furthermore, they are adept at handling effluents from the oil sector, which contain hydrocarbons and volatile organic compounds. Demonstrating versatility across sectors, CBNs are particularly effective in the chemical industry for detecting and decomposing pesticides and chemical residues, with efficiencies of up to 95% for pesticides (Singh and Saxena, 2022b). CNTs achieve 100% efficiency in removing heavy metals from acidic mine effluents and sediments. In the oil and gas sector, CFs are crucial in treating hydrocarbons and heavy metals, with up to 95% efficiency. Moreover, in agriculture, G and CDs effectively remove nitrates, phosphates, and pesticides, reaching 90% removal rates for nitrates and phosphates, demonstrating their broad applicability and significant contributions to environmental sustainability (Dhanapal et al., 2024a; Sajjad et al., 2024; Tripathi et al., 2024).
Each of these materials, presented in Table 2, has distinct properties that make them well suited for the removal of various pollutants, as outlined below:
1) Carbon Nanotubes (CNTs) with diameters ranging from 1 nm (single-walled) to 100 nm (multi-walled) and lengths up to micrometers, have a unique structure that provides a large specific surface area up to 1,320 m2/g. This attribute makes them highly effective at adsorbing heavy metals such as lead, mercury, cadmium, and arsenic from industrial effluents. Novel synthesis techniques, such as microwave radiation, have been introduced to enhance the production of CNTs, demonstrating their potential to remove toxic dyes from textile wastewater (Ceroni et al., 2024). Furthermore, the use of biomass-derived CNTs for heavy metal removal represents a significant step towards environmentally friendly remediation methods. Functionalizing CNTs with specific groups significantly improves their adsorption capacity, enabling selective interactions with metal ions and enhancing their effectiveness in water treatment (Dey et al., 2024a; Ningombam et al., 2024a). The adsorption of heavy metal ions in aqueous solutions is directly influenced by the functionalization of CNTs. The adsorption capacity of CNTs is significantly enhanced by the addition of specific functional groups, enabling more selective and strong interactions with specific metal ions. Thus, through a range of heavy metals, functionalization affects the affinity of CNTs. Some of the elements that fall into this category are arsenic (As), cadmium (Cd), and lead (Pb). This method of functionalization enhances the effectiveness of CNTs in eliminating metallic pollutants from industrial effluents. Additionally, it facilitates the creation of more efficient and tailored water treatment techniques, highlighting the significance of surface chemistry in optimizing adsorptive materials for environmental remediation (Lee, Lee, and Kim, 2023b; Dey et al., 2024a; Ogunsola et al., 2024). Furthermore, a novel microemulsion technique has been developed to functionalize carbon nanotubes (CNTs) with a hydrocarbon tail. The objective is to effectively eliminate various hydrocarbon contaminants from water polluted by oil spills. Several methods have been developed to modify surfaces, such as acid treatment, grafting functional groups, and impregnating with metals or metal oxides (Hu et al., 2024; Yahyaee and Mofidi, 2024). In general, the modifications of CNTs can be achieved through either covalent or non-covalent functionalization, which greatly improves their effectiveness in environmental remediation (Y. Singh and Saxena, 2022a).
2) Carbon Quantum Dot (CD) with sizes typically less than 10 nm, exhibit unique quantum properties, such as quantum confinement effects and a high surface area-to-mass ratio, causing them to be highly effective in photocatalytic pollutant degradation, with efficiencies up to 95%. Their remarkable ability to interact with a wide range of contaminants is enhanced through surface modifications with functional groups such as carboxyls, amines, and thiols, improving adsorption and selective binding with both metal and organic ions (Hu et al., 2024; Hu et al., 2024; Soni et al., 2024). The functionalization of CDs, as all CBNs enhances their selectivity and adsorption efficiency, enabling precise chemical recognition and increased binding affinity for specific pollutants through mechanisms such as electrostatic attraction and hydrogen bonding. This adaptability and efficiency cause CDs to be a promising tool for water purification because they can effectively eliminate contaminants and have the potential for adsorbent regeneration in cases of physical adsorption, thereby demonstrating their versatility in environmental cleanup efforts (Soni et al., 2024). Various mechanisms govern this interaction, such as electrostatic attraction, hydrophobic interactions, hydrogen bonding, and metal complexation (Cruz-Cruz et al., 2024). The process of adsorption may effectively eliminate contaminants from water. In certain cases, this process can be reversed for physical adsorptions, which enables CD regeneration. In contrast, chemical adsorption has a longer-lasting effect, demonstrating the remarkable adaptability and efficiency of CD in the field of water purification (Kausar and Ahmad, 2024).
3) Carbon fibers (CF) with diameters ranging between 5 and 10 μm, possess remarkable mechanical and thermal resistance. Additionally, they exhibit a remarkable absorption capacity, enabling the efficient removal of hydrocarbons and heavy metals from effluents with impressive efficiencies up to 95%. This groundbreaking technique significantly increases the adsorption capacity of the fibers by introducing a selective and reactive surface. Furthermore, it bestows an adsorbent material with antimicrobial properties. The presence of silver nanoparticles improves the interaction with radioactive iodine ions by utilizing mechanisms such as electrostatic attractions and ion exchange. This increases the effectiveness of eliminating these detrimental pollutants (Jia et al., 2023; Joseph and Vijayanandan, 2023). Optimizing water purification processes, particularly in the remediation of radioactive effluents, can be achieved through the modification of adsorbent materials with metallic nanoparticles. This may drive major progress in ensuring environmental safety and safeguarding public health. In addition, sustainable waste management includes techniques that enable the recovery and reuse of CF obtained from bioremediation procedures. This is accomplished through a creative method that enables the repurposing of CF and follows the principles of a circular economy while minimizing the environmental consequences of disposing composites. We suggest a notable change in waste management by advocating for the recycling of CF, encouraging the growth of closed-loop value chains, and reducing the need for new resource extraction. This is a noteworthy advancement towards adopting more environmentally friendly approaches (P. Zhang et al., 2024; Joseph and Vijayanandan, 2023).
4) Graphene (G) is a remarkable material with a single layer of carbon atoms that are only 0.34 nm thick. It has an impressive surface area of 2,630 m2/g, which enable it to efficiently adsorb a wide range of pollutants. This includes dyes and heavy metals, as G can interact effectively with different types of contaminant molecules. G, with its exceptional properties, holds immense promise for propelling progress in various scientific and technological domains. G has shown great potential in various fields, such as water filtration, advanced electronics, and the creation of composite materials. This is mostly because of its remarkable surface area, impressive mechanical strength, and excellent thermal and electrical conductivity (Obayomi et al., 2022; N; Verma et al., 2023). The remarkable versatility of G is showcased by its wide range of applications, which hold great potential for driving significant advancements in sustainable solutions and environmentally friendly technologies (Kausar and Ahmad, 2024). Additionally, the treatment of industrial effluents has been significantly transformed through the functionalization of G, enabling the development of highly efficient membranes that can selectively eliminate heavy metals and toxic organic substances. Through this procedure, the surface of G undergoes a chemical modification with specific functional groups, such as aminos groups, to effectively capture metallic ions while repelling organic compounds (Jatoi et al., 2024).
5) Nanodiamonds (ND) ranging in size from 2 to 8 nm, possess a highly adaptable surface that can be tailored to optimize interactions with targeted pollutants. With their impressive customization capability, exceptional chemical stability, and expansive surface area, these materials hold great potential as effective tools for eliminating a wide range of pollutants. The synthesis and functionalization of NDs enable them to effectively remove and break down a wide range of pollutants, including heavy metals and persistent organic compounds (Molavi et al., 2024b; Silah et al., 2024). These materials have superior performance compared to traditional materials due to their large surface area, excellent chemical stability, and impressive ability to specifically target pollutants. Utilizing nanocomposites composed of ND integrated into polyaniline/polyvinylidene fluoride (PANI/PVDF) microfiltration membranes to eliminate industrial pollutants results in a distinctive amalgamation that significantly amplifies the membranes’ ability to adsorb, select, and remain stable in complex aqueous environments (Yotinov et al., 2022; Molavi et al., 2024a). This groundbreaking technique not only increases the effectiveness of eliminating pollutants, such as heavy metals and volatile organic compounds, but also provides the membranes with exceptional mechanical properties and chemical resistance. This causes them to be perfect for use in industrial effluent treatment applications (Siddiqa et al., 2023a).
Table 2 presents a summary of using different CBNs for treating industrial wastes. This shows their impressive ability to effectively remove various contaminants in different industrial sectors.
For successful remediation, it is crucial to carry out thorough preparation and functionalization procedures for CBNs. This includes synthesis, purification, functionalization, and characterization, all of which are specifically designed to optimize pollutant interaction. Various techniques, such as chemical vapor deposition and laser ablation, are used to generate a wide range of CBNs. These CBNs are then purified to enhance their effectiveness. Surface modification improves the compatibility of CBN with contaminants, while thorough characterization confirms the existence of functional groups and evaluates material properties, guaranteeing efficient removal of pollutants (Ningombam et al., 2024a; Cruz-Cruz et al., 2024; Hu et al., 2024).
3.3 How can the combined use of microbes and carbon nanomaterials enhance the removal of industrial effluents?
Recent advancements in nanotechnology have shown significant potential in environmental remediation, especially in treating industrial effluents. Integrating CBN with bacteria has emerged as an effective strategy for pollution control (Mekonnen, Aragaw, and Genet, 2024) (Y. Singh and Saxena, 2022c). Studies have highlighted that nanoparticles can enhance biohydrogen production from fermentative bacteria by improving enzyme efficiency. The use of CBNs such as G, CDs, CNTs, and CFs shows significant potential in industrial effluent remediation. The functionalization of these materials with groups such as carboxyl, amino, or hydroxyl groups enhances their interaction with bacteria, leading to more effective pollution treatment. These modifications facilitate bacterial attachment and growth and support electron transfer in bioelectrochemical systems, increasing the efficiency of the removal of complex organic pollutants. By incorporating antimicrobial agents or selecting specific microbial communities, the proliferation of harmful microorganisms can be minimized (Chen, Liu, and Liu, 2024; Cruz-Cruz et al., 2024). The development of advanced effluent treatment technologies, including microbial fuel cells and bioreactors, leverages these functionalization strategies to provide eco-friendly solutions to the challenges of industrial wastewater treatment (Hu et al., 2024; Jiang et al., 2024).
CBN and G have been developed into innovative photocatalysts in wastewater treatment. These catalysts facilitate charge and heat transfer at interfaces, effectively removing organic and inorganic pollutants from industrial wastewater. This represents a notable leap in water purification technology (Joseph and Vijayanandan, 2023). The combination of CBN with bacteria not only boosts degradation efficiency beyond traditional bioremediation methods but also accelerates the breakdown of complex organic materials otherwise resistant to conventional treatments (Figure 2). This methodology is effective against persistent pollutants such as heavy metals and polycyclic aromatic hydrocarbons, significantly reducing their toxicity and bioavailability (Abril et al., 2024; Sun et al., 2024).
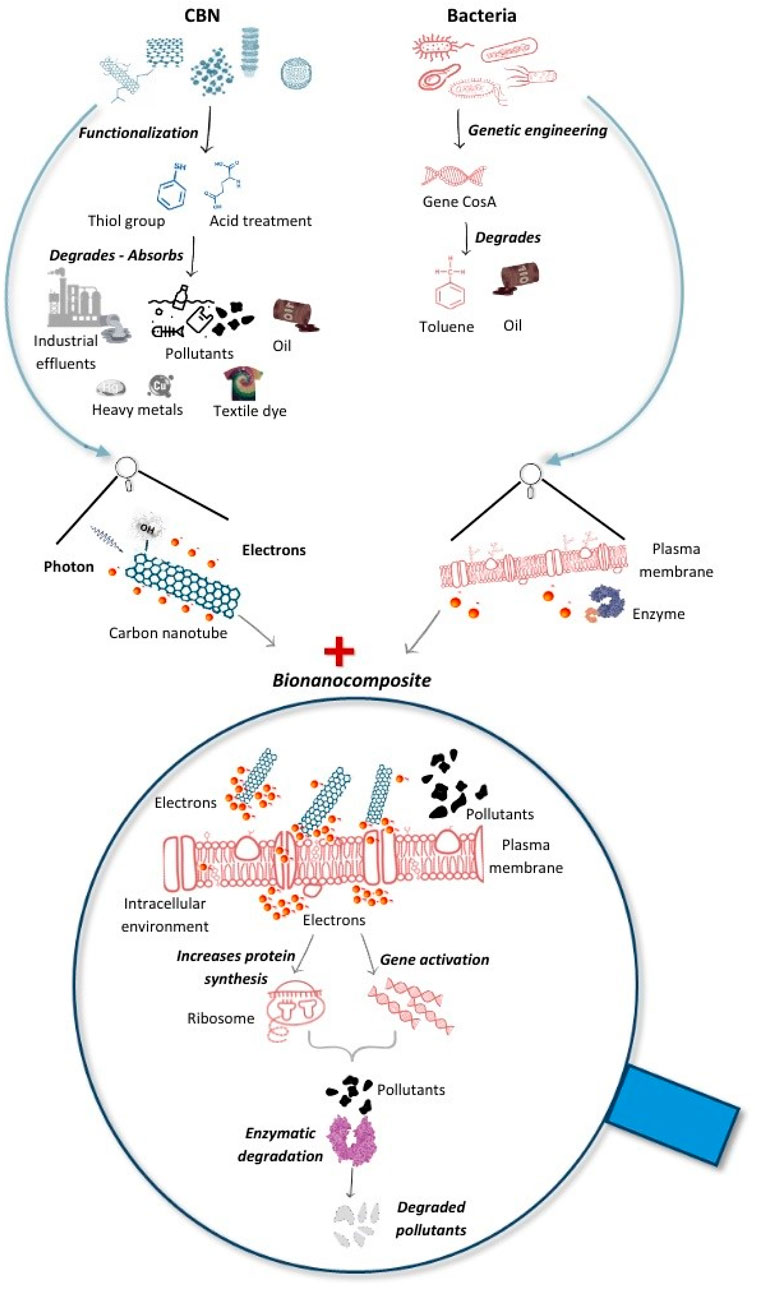
Figure 2. Comprehensively depicts the various bacterial species that play a crucial role in bioremediation endeavors. These bacteria play a vital role in bioaccumulation, biodegradation, and biosorption processes, which are essential for environmental purification. The figure illustrates the interplay between bacteria and carbon-based nanomaterials (CBNs) in the treatment of industrial effluents. These CBNs include carbon nanotubes (CNTs), carbon dots (CDs), graphene (G), carbon fibers (CFs), and nanodiamonds (NDs). The focus is on how these nanomaterials enhance the effectiveness of contaminant degradation and detection, highlighting their potential in advanced environmental remediation technologies (Singh and Saxena, 2022a; Mousa, Tulbure, and Fawaeer, 2023; Singh et al., 2023; Abril et al., 2024; Jatoi et al., 2024a; Balakumar et al., 2024; Ningombam et al., 2024b; Singh Simranjeet et al., 2024; Sajjad et al., 2024; Silah et al., 2024; Sun et al., 2024).
Moreover, the ability to recover and reuse nanomaterials after bioremediation underscores a move toward sustainability and a circular economy, aligning with efforts to minimize environmental risks. The fusion of biology and nanotechnology in remediation with nanocomposites represents a pioneering advancement. This nanocomposite approach promises more effective, environmentally friendly solutions for industrial effluent treatment, paving the way for future research to expand and enhance its applications (Dey et al., 2024a; Singh et al., 2024a).
The interaction between bacteria and CBNs, such as CNTs and G, improves bioremediation efficiency through the extensive surface area and reactivity of materials, the unique features of CBNs, including their high porosity, adsorption capacity, and electrical properties, support this synergistic interaction, facilitating pollutant degradation and adsorption. Surface modification of CBNs broadens their application, enabling them to adapt to various pollutants and environmental conditions, thus enabling treatment diversity (Hu et al., 2024; Sun et al., 2024). Notably, nanobioremediation offers a distinct advantage over traditional bioremediation by shortening the treatment time, resulting in swift and crucial remediation for cases of acute contamination.
This approach is characterized by its specificity, selectivity, and sustainability, reducing energy consumption and chemical use while enabling resource recovery. CBNs can recover valuable metals from effluents, underscoring the process’s adaptability and scalability for different contexts (Ningombam et al., 2024a; Dey et al., 2024b). Chemical functionalization of CBNs increases their hydrophilicity, thereby improving bacterial adhesion and activating essential metabolic pathways for pollutant breakdown (Ogunsola et al., 2024; Sam and Cao, 2024; Silah et al., 2024).
The integration of nanotechnology with microbial systems through nanocomposite approaches notably improves remediation outcomes by enabling more precise microbial interactions (Balakumar et al., 2024; Jagaba et al., 2024). The complementary roles of CBN adsorption capacity and bacterial biodegradation activities create a synergistic effect, while electron transfer facilitated by CBNs accelerates redox reactions critical for biodegradation. Recent advancements have highlighted how the interplay between bacteria and CBNs, including CNTs, G, and CDs, improves the removal of heavy metals, organic compounds, and other pollutants from wastewater (Dey et al., 2024a; Cruz-Cruz et al., 2024; Sam and Cao, 2024; Silah et al., 2024; Soni et al., 2024).
The use of nanocomposites in effluent treatment represents a breakthrough in environmental remediation because they can adsorb and decompose pollutants while supporting water recycling and material regeneration. This analysis highlights the potential of combining CBNs and microorganisms in nanobioremediation, offering sustainable solutions to environmental challenges (Singh et al., 2024a; Ezeorba et al., 2024). Case studies in industrial nanobioremediation have shown effective pollution removal, including hydrocarbon decomposition in oil waste, elimination of textile dyes, soil decontamination from heavy metals, and pesticide processing from agricultural waste, emphasizing the sustainability and effectiveness of these nanocomposite systems in environmental cleanup (Jatoi et al., 2024b; Yahyaee and Mofidi, 2024).
Electron conductivity facilitates electron transfer between bacteria and pollutants, which is crucial for biodegradation. Optimal CBN quantities enhance bacterial growth and metabolic activity, although excessive amounts may hinder it. Understanding these interactions is vital for developing effective and eco-conscious nanobioremediation methods. Despite its potential for effective effluent management and environmental preservation, challenges such as high development costs and technical difficulties in stabilizing CBNs in complex environments remain. This synergy between nanotechnology and microorganisms offers a sustainable approach to waste treatment, promoting renewable energy generation and resource utilization (Dey et al., 2024a; Chen, Liu, and Liu, 2024; Sun et al., 2024).
This innovative synergy between bacteria and CBNs represents a technical advancement in industrial effluent remediation, highlighting its potential for significant environmental progress (Chauhan et al., 2023; Ningombam et al., 2024a; Chen, Liu, and Liu, 2024). These challenges, along with potential environmental impacts, are the focus of ongoing research, highlighting the need for continued innovation in this promising field. Further research is needed to explore CBN-microbe-contaminant interactions for enhanced nanobioremediation, especially in challenging environments. Responsible use considers environmental, safety, and economic implications, ensuring sustainable nanobioremediation advancement (Chen, Liu, and Liu, 2024; Kaur et al., 2024).
4 Risks of nanomaterials’ biopersistence in environmental remediation and contributions to the circular economy
4.1 Assessment and monitoring of nanomaterials’ biopersistence risks in nanobioremediation
Progress in nanobioremediation technology relies on the development of enhanced nanomaterials that merge nanotechnology, molecular biology, and genetic engineering to offer more efficient pollution cleanup methods. While nanotechnology presents significant prospects for environmental rehabilitation, questions about its long-term ecological implications remain. A detailed understanding of nanomaterial biopersistence is crucial for accurately identifying hazards and enabling the responsible development of nanobioremediation technology. Maintaining the highest safety standards is crucial for the responsible use of nanomaterials and the advancement of sustainable practices (Rajput et al., 2022a; Hidangmayum et al., 2023a; Dey et al., 2024b; Dey et al., 2024b; Yang et al., 2024).
Therefore, it is imperative to explore its use in challenging areas and understand its long-term environmental and toxicological implications. The introduction of universal regulatory standards, sophisticated characterization techniques, and economic evaluations will aid in the sustainable expansion of nanobioremediation. The evolution of nanobioremediation hinges on creating new nanomaterials with enhanced features and biocompatibility, emphasizing the need for safety standards and responsible use. Understanding the biopersistence of nanomaterials is key to addressing environmental concerns and supporting the safe use of nanotechnology (Devasena et al., 2022; Vineeth Kumar et al., 2022; Rahman et al., 2023; Chávez-Hernández et al., 2024a). Despite its potential, addressing ecological issues remains a priority to ensure the responsible progression of technology. This thorough analysis highlights the need for ongoing research, multidisciplinary collaboration, and global cooperation to address the challenges and opportunities of nanobioremediation, ensuring that its development favorably contributes to environmental sustainability and pollution remediation (Kim et al., 2023; Chávez-Hernández et al., 2024a).
Advanced biodegradation studies employing sophisticated methodologies, such as metagenomic techniques, are crucial for understanding how microorganisms and enzymes degrade nanomaterials and the microbiota involved in these processes. High-resolution mass spectrometry is essential for accurately identifying and quantifying nanoparticles in environmental samples, providing precise traceability at very low levels. Investigations into biodegradation, including advanced approaches, such as metagenomics, enhance our knowledge of nanomaterial degradation processes and related microbial populations (Wang et al., 2024a; Cai et al., 2024). Modeling and observation methodologies can predict the accumulation of nanomaterials in the environment, which is vital for assessing long-term sustainability.
In situ transmission electron microscopy (TEM) and atomic force microscopy (AFM) are instrumental in examining the interactions between nanomaterials and organisms, as well as nanoscale changes, providing insights into the biopersistence and impact of these materials. Real-time monitoring systems, leveraging advancements in nanotechnology and molecular biology, offer swift insights into bioremediation progress, enabling adaptive management in response to environmental changes (Rather et al., 2023b; Chaudhary et al., 2023; Salazar et al., 2023). Evaluating the long-term environmental consequences and toxicity of nanomaterials and bacteria used in bioremediation is essential. Also, strategies for implementing nanobioremediation on a large scale in industry and assessing its economic and environmental viability compared to traditional remediation methods require thorough investigation (Sornaly et al., 2024; Sun et al., 2024; Tripathi et al., 2024).
This underscores the need for multidisciplinary research, ongoing progress, and global collaboration in this expanding field. The integration of degrading bacteria with CBNs in nanobioremediation systems offers a viable technique for removing environmental pollution. However, the potential toxicity of these nanocomposite systems to the food chain has raised considerable concerns. CBNs, such as non-functionalized CNTs and G, are toxic to aquatic and terrestrial animals and impact biodiversity and ecosystem functionality (Audira et al., 2024; Sandra de et al., 2024). Furthermore, the interaction between degrading bacteria and CBNs may lead to the formation of secondary metabolites or degradation intermediates, whose implications for organism health and food safety are not well characterized. The bioaccumulation of these compounds in base trophic organisms, such as phytoplankton and zooplankton, can adversely affect not only these organisms but also secondary and tertiary consumers, highlighting the need for detailed environmental risk assessments for nanobioremediation systems (Mousa, Tulbure, and Fawaeer, 2023; Petersen et al., 2023; Audira et al., 2024; Sandra de et al., 2024).
Thus, it is crucial to develop risk mitigation techniques and special restrictions for the use of nanocomposite systems combining degrading bacteria and CBNs in nanobioremediation. Establishing stringent criteria for the synthesis, release, and disposal of these systems can help minimize potential impacts on the food chain and ensure ecological safety. Additionally, promoting research on environmental detection and monitoring methods for these nanomaterials, as well as on exposure pathways and toxicity mechanisms, is key to protecting ecosystems and human health. These approaches will ensure that the benefits of nanobioremediation can be responsibly harnessed without compromising ecosystem integrity or food chain safety (Kim et al., 2023; Sandra de et al., 2024).
4.2 Enhancing the circular economy: the role of nanocomposite nanoremediation systems with carbon nanomaterials and bacteria in the reuse of industrial effluent waste
The use of nanocomposites in wastewater treatment exemplifies the concepts of circular economy by highlighting material recovery and reuse. This is crucial for the circular bioeconomy, which is built upon the principles of recycling, reusing, disposing, and reducing (Figure 3). Nanocomposites in effluent treatment exemplify CE concepts by emphasizing material recovery and regeneration (Chen, Liu, and Liu, 2024; Kaur et al., 2024). The water treated using nanobioremediation has the potential to be reused in different sectors, especially in agriculture where the environment is often polluted by pesticides and other harmful substances. This method of treatment contributes to the preservation of water resources. Postremediation recovery of biomass and nanomaterials facilitates the creation of sustainable materials and energy sources, reducing operational costs and reliance on new resources. Moreover, the conversion of biological waste into biogas offers a sustainable energy source. Nanocomposite systems capitalize on CBN features to enhance bacterial activity, pollutant adsorption, and enzymatic catalysis. This integrated approach supports efficient waste reuse within the circular economy, promoting sustainability across sectors (Awogbemi and Von Kallon, 2024; Liaquat and Muddasar, 2024; Sravan, Matsakas, and Sarkar, 2024).
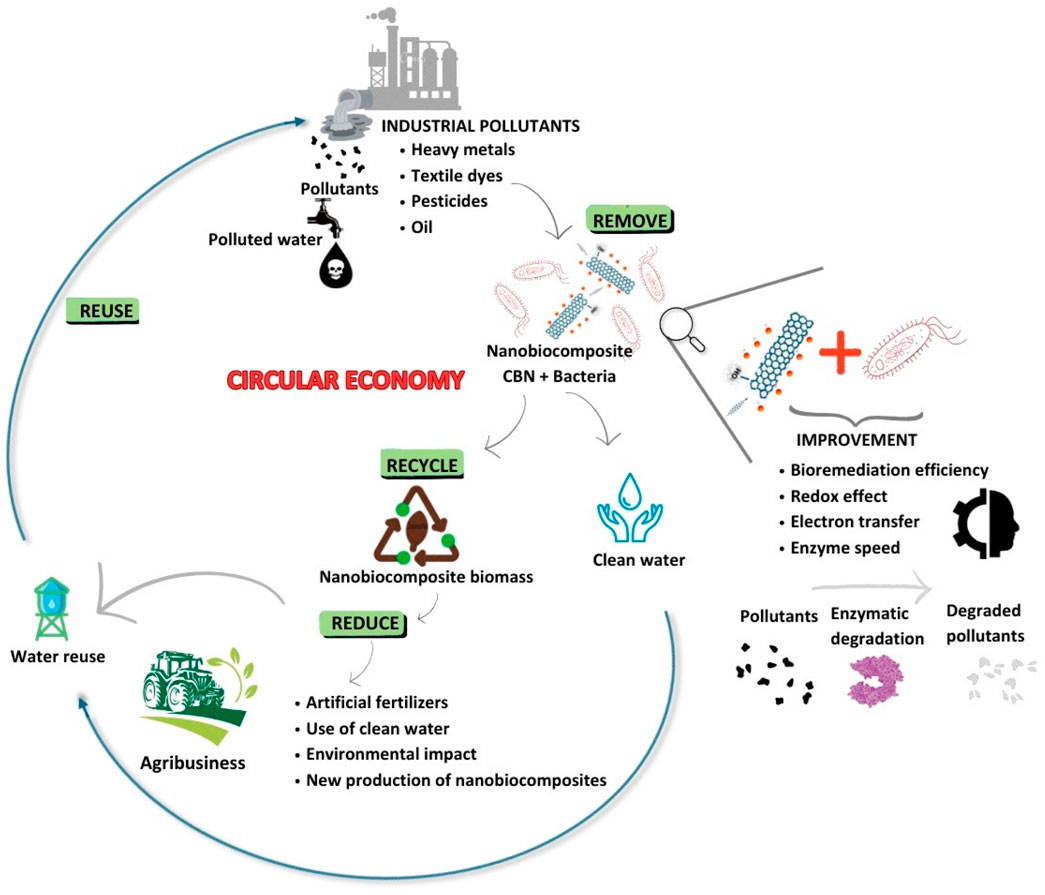
Figure 3. Shows a cyclical method for treating industrial wastewater using bacteria and carbon nanomaterials. This approach promotes the circular economy by eliminating pollutants, converting waste into biomass, and minimizing environmental damage (Blažina et al. 2022; Salehi and Wang 2022; Kumar et al. 2023; Zhang et al. 2023; Chen et al., 2024; Ezeorba et al. 2024; Kaur et al. 2024)
The use of nanocomposites in effluent treatment promotes sustainability by minimizing pollution at their source, improving water quality, and aiding in decentralized water treatment emphasizing sustainable design and material recovery, which agree with the circular economy (CE). Their adsorption and degradation capabilities, coupled with easy regeneration, offer a sustainable solution prioritizing sustainable production methods and materials to ensure efficient recovery and reduce costs and waste. The synergistic interactions observed in nanobioremediation processes, as demonstrated by Chen et al. (2024), lead to efficient pollutant elimination. Reusing waste from these technologies is crucial for CE sustainability, (Salehi and Wang, 2022; Chen, Liu, and Liu, 2024).
Recycling nutrient-rich effluents enhances agricultural sustainability by reducing reliance on artificial fertilizers. These innovative approaches underscore the potential of nanobioremediation waste in fostering a CE, minimizing environmental impacts, and improving waste management economics. Nanobioremediation waste has significant potential for integrating into the CE, offering solutions to environmental challenges while promoting sustainability. Advanced techniques enable efficient retrieval of nanomaterials from bioremediation residues, facilitating their reuse in subsequent cycles or other industrial applications (Pereira et al., 2024; Poonia et al., 2024; Trivedi et al., 2024). Additionally, technologies such as anaerobic digestion can convert microbial biomass into renewable energy and chemicals, further minimizing landfill waste. Case studies demonstrate the successful recovery of G from water contaminated with heavy metals, demonstrating its reusability within the CE. Moreover, repurposing microbial biomass into biofertilizers enriches the soil and enhances plant growth, contributing to sustainable agriculture. These practices align with global sustainability goals, emphasizing the transformative potential of nanobioremediation waste in converting environmental liabilities into sustainable assets.
Efficient nanobioremediation of industrial pollutants requires balancing competitiveness with environmental sustainability (Bhatt et al., 2022a; Rajput and Tatiana, 2023a; Chávez-Hernández et al., 2024b). This entails evaluating nanomaterial manufacturing costs, prioritizing life cycle assessments to minimize environmental impact, and fostering collaboration among stakeholders. Continued research and development are crucial for enhancing efficiency and reducing environmental footprint. Ultimately, nanobioremediation serves as an advanced, economically viable, and sustainable industrial practice, exemplifying its potential as a transformative remediation technology.
4.3 Bottlenecks in nanoremediation for the treatment of industrial wastewater: what are they?
Through the combination of CBNs and bacteria, nanobioremediation has emerged as a highly advanced method for purifying industrial effluents. This innovative approach holds great potential for tackling intricate environmental issues. Nevertheless, the wider implementation of this technology encounters challenges such as scalability, affordability, and integration with infrastructures. These obstacles go beyond technical considerations and involve economic and regulatory aspects. To address these challenges, innovative strategies and significant support from public policy are necessary (Sati et al., 2022; Pratap and Krishnan, 2024a; Roy et al., 2024). Addressing the challenges of scaling up nanobioremediation systems, ensuring consistent performance, optimizing production costs, and minimizing environmental risks linked to nanomaterials present considerable hurdles. A deep understanding of how nanomaterials and microbes interact in the environment is crucial for improving remediation efforts and safeguarding beneficial species from any negative effects (Ningombam et al., 2024b; Chen, Liu, and Liu, 2024; Kaur et al., 2024).
Adhering to regulations set by esteemed authorities such as the U.S. Environmental Protection Agency (EPA) and the European Chemicals Agency (ECHA) is essential. The registration process requires thorough presentations on safety and efficacy (Kumari et al., 2023; Chávez-Hernández et al., 2024a). In addition, it is crucial to ensure that nanoparticles are properly labeled, accounting for all possible risks, following safety regulations, and undergoing thorough assessments of their environmental impact. Efficient management of nanomaterial waste requires careful planning and a strong commitment to ethical and social responsibility (Ayilara and Babalola, 2023; Gulati et al., 2023; Olawore, Ogunmola, and Desai, 2024). Beside this, successfully implementing and gaining recognition for nanobioremediation requires navigating bureaucratic challenges, adhering to international regulations, and actively involving stakeholders. Incorporating these technologies into a CE presents a chance for creative thinking and collaboration across different fields, promoting environmental Sustainability (Bhatt et al., 2022b; Gong et al., 2023; Dhanapal et al., 2024b). This integration promotes the adoption of sustainable practices, which agree with the principles of resource efficiency and waste reduction. Notable progress and promising potential in nanobioremediation highlight its effectiveness in addressing sustainability and CE issues (Rajput et al., 2022b; Rajput and Tatiana, 2023b; Rather et al., 2023d).
There are various obstacles to the implementation of this technology, including the technical complexities of dealing with contaminants, the economic challenges associated with system costs and benefits, and the varying regulatory restrictions across different countries. However, it is crucial to highlight the significance of collaboration across sectors, the support from policies, financial incentives, and engagement with stakeholders. These factors are essential for addressing the challenges and fully harnessing the potential of CE in sustainable resource management (Pandey et al., 2022; Hidangmayum et al., 2023b; Rather et al., 2023c; Pratap and Krishnan, 2024b). The goal is to achieve both environmental and economic advantages while maintaining strict adherence to environmental regulations. In this context, it is crucial to emphasize the importance of ongoing research and development, as well as the implementation of effective public policies, to harness these technologies for a more sustainable future.
5 Conclusion
This study represents a notable advancement in the field of nanobioremediation, providing valuable insights into the collaborative efforts of bacteria and CBNs, including CNTs and G, for the treatment of industrial effluents. We investigated the molecular mechanisms underlying this collaboration, revealing remarkably effective approaches to combating pollution. We emphasize the importance of the advanced functionalization of nanomaterials, which improves their ability to absorb and break down contaminants. Additionally, we focus on the selection of specialized bacterial strains that are more efficient at eliminating pollutants. This approach not only enhances decontamination efforts but also enhances our understanding of the underlying processes. The combination of bacteria and CBN leads to a fascinating interplay that enhances remediation, as demonstrated by compelling case studies. Although highly effective, several challenges need to be addressed in terms of sustainability, nanomaterial recovery, public acceptance, and regulatory issues concerning the biosafety of these nanocomposite systems. In this study, we highlight the latest progress in this promising area of research addressing industrial pollution and sets the stage for future investigations in nanobioremediation. We also highlight the significance of fostering innovation and continuous advancement to thoroughly unlock the possibilities of this growing field, with a focus on promoting environmental sustainability and safeguarding ecosystems. Collaboration across disciplines is essential for addressing environmental challenges, as it enables the integration of nanotechnology and biotechnology to offer promising solutions for sustainable industry and a cleaner future for generations.
Author contributions
EC: Writing–review and editing, Writing–original draft, Visualization, Validation, Supervision, Resources, Project administration, Methodology, Investigation, Funding acquisition, Formal Analysis, Conceptualization. LS: Writing–original draft, Investigation. EA: Writing–original draft, Investigation. AO: Writing–original draft, Investigation. JJ: Writing–review and editing, Visualization, Validation, Supervision, Conceptualization. JP: Writing–original draft, Visualization, Validation, Supervision, Resources, Project administration, Funding acquisition, Conceptualization.
Funding
The author(s) declare that financial support was received for the research, authorship, and/or publication of this article. This work was financially supported by FDTE Foundation for the Technological Development of Engineering, process number#2015/2022, and by the BiotecBrazil TM Company No. 2024/001. The funders had no role in the study design, data collection or analysis, decision to publish, or preparation of the manuscript.
Conflict of interest
The authors declare that the research was conducted in the absence of any commercial or financial relationships that could be construed as a potential conflict of interest.
Publisher’s note
All claims expressed in this article are solely those of the authors and do not necessarily represent those of their affiliated organizations, or those of the publisher, the editors and the reviewers. Any product that may be evaluated in this article, or claim that may be made by its manufacturer, is not guaranteed or endorsed by the publisher.
References
Abril, A. O., Biełło, K., Caballero, G. R., Cabello, P., Sáez, L. P., Moreno-Vivián, C., et al. (2024). Bacterial tolerance and detoxification of cyanide, arsenic and heavy metals: holistic approaches applied to bioremediation of industrial complex wastes. Microb. Biotechnol. 17 (1), e14399. doi:10.1111/1751-7915.14399
Aguilar-Romero, I., Madrid, F., Villaverde, J., and Morillo, E. (2024). Ibuprofen-enhanced biodegradation in solution and sewage sludge by a mineralizing microbial consortium. Shift in associated bacterial communities. J. Hazard. Mater. 464, 132970. doi:10.1016/j.jhazmat.2023.132970
Ahmed, M., Mavukkandy, M. O., Giwa, A., Elektorowicz, M., Katsou, E., Khelifi, O., et al. (2022). Recent developments in hazardous pollutants removal from wastewater and water reuse within a circular economy. Npj Clean. Water 5 (1), 12. doi:10.1038/s41545-022-00154-5
Aryal, M. (2024). Rhizomicrobiome dynamics: a promising path towards environmental contaminant mitigation through bioremediation. J. Environ. Chem. Eng. 12 (2), 112221. doi:10.1016/J.JECE.2024.112221
Ashkanani, Z., Mohtar, R., Al-Enezi, S., Smith, P. K., Calabrese, S., Ma, X., et al. (2024). AI-assisted systematic review on remediation of contaminated soils with PAHs and heavy metals. J. Hazard. Mater. 468, 133813. doi:10.1016/j.jhazmat.2024.133813
Audira, G., Lee, J. S., Vasquez, R. D., Roldan, M. J. M., Lai, Yu H., and Hsiao, C. D. (2024). Assessments of carbon nanotubes toxicities in zebrafish larvae using multiple physiological and molecular endpoints. Chemico-Biological Interact. 392, 110925. doi:10.1016/J.CBI.2024.110925
Awogbemi, O., and Von Kallon, D. V. (2024). Recent advances in the application of nanomaterials for improved biodiesel, biogas, biohydrogen, and bioethanol production. Fuel 358, 130261. doi:10.1016/J.FUEL.2023.130261
Ayilara, M. S., and Babalola, O. O. (2023). Bioremediation of environmental wastes: the role of microorganisms. Front. Agron. 5. doi:10.3389/fagro.2023.1183691
Balakumar, S., Mahesh, N., Kamaraj, M., Saranya, T., Suresh Babu, P., Aravind, J., et al. (2024). Customized carbon composite nanomaterials for the mitigation of emerging contaminants: a review of recent trends. Carbon Lett., doi:10.1007/s42823-024-00715-3
Bhatt, P., Pandey, S. C., Joshi, S., Chaudhary, P., Pathak, V. M., Huang, Y., et al. (2022a). Nanobioremediation: a sustainable approach for the removal of toxic pollutants from the environment. J. Hazard. Mater. 427, 128033. doi:10.1016/j.jhazmat.2021.128033
Bhatt, P., Satish, C. P., Samiksha, J., Chaudhary, P., Pathak, V. M., Huang, Y., et al. (2022b). Nanobioremediation: a sustainable approach for the removal of toxic pollutants from the environment. J. Hazard. Mater. 427, 128033. doi:10.1016/j.jhazmat.2021.128033
Blažina, M., Fafanđel, M., Geček, S., Haberle, I., Klanjšček, J., Hrustić, E., et al. (2022). Characterization of pseudochloris wilhelmii potential for oil refinery wastewater remediation and valuable biomass cogeneration. Front. Mar. Sci. 9. doi:10.3389/fmars.2022.983395
Cai, Y., Chen, Xu, Qi, H., Bu, F., Shaaban, M., and Peng, Q.-An (2024). Genome analysis of shewanella putrefaciens 4H revealing the potential mechanisms for the chromium remediation. BMC Genomics 25 (1), 136. doi:10.1186/s12864-024-10031-9
Ceroni, L., Benazzato, S., Pressi, S., Calvillo, L., Marotta, E., and Menna, E. (2024). Enhanced adsorption of methylene blue dye on functionalized multi-walled carbon nanotubes. Nanomaterials 14 (6), 522. doi:10.3390/nano14060522
Chaudhary, P., Ahamad, L., Chaudhary, A., Kumar, G., Chen, W.-J., and Chen, S. (2023). Nanoparticle-mediated bioremediation as a powerful weapon in the removal of environmental pollutants. J. Environ. Chem. Eng. 11 (2), 109591. doi:10.1016/j.jece.2023.109591
Chauhan, P., Imam, A., Kumar Kanaujia, P., and Suman, S. K. (2023). Nano-bioremediation: an eco-friendly and effective step towards petroleum hydrocarbon removal from environment. Environ. Res. 231, 116224. doi:10.1016/j.envres.2023.116224
Chávez-Hernández, J. A., Velarde-Salcedo, A. J., Navarro-Tovar, G., and Gonzalez, C. (2024a). Safe nanomaterials: from their use, application, and disposal to regulations. Nanoscale Adv. 6 (6), 1583–1610. doi:10.1039/D3NA01097J
Chen, J., Liu, K., and Liu, Y. (2024). Synergistic molecular mechanism of degradation in dye wastewater by rhodopseudomonas palustris intimately coupled carbon nanotube - silver modified titanium dioxide photocatalytic composite with sodium alginate. J. Environ. Manag. 351, 119913. doi:10.1016/J.JENVMAN.2023.119913
Chunyan, Xu, Qaria, M. A., Qi, Xu, and Zhu, D. (2023). The role of microorganisms in petroleum degradation: current development and prospects. Sci. Total Environ. 865, 161112. doi:10.1016/J.SCITOTENV.2022.161112
Cruz-Cruz, A., Rivas-Sanchez, A., González-González, R. B., and Parra-Saldívar, R. (2024). Tuning catalytic attributes of enzymes by conjugation with functionalized carbon dots. Top. Catal., doi:10.1007/s11244-024-01911-1
Devasena, T., Iffath, B., Renjith Kumar, R., Muninathan, N., Baskaran, K., Srinivasan, T., et al. (2022). Insights on the dynamics and toxicity of nanoparticles in environmental matrices. Bioinorganic Chem. Appl. 2022, 1–21. doi:10.1155/2022/4348149
Dey, S., Narayan Parida, S., Kumar Rout, A., Dei, J., Maharana, J., Priyambada Pradhan, S., et al. (2024a). “Nanobioremediation: a sustainable approach for environmental monitoring with special reference to the restoration of heavy metal contaminated soil and wastewater treatment,” in Role of green chemistry in ecosystem restoration to achieve environmental sustainability (Amsterdam, Netherlands: Elsevier), 41–49. doi:10.1016/B978-0-443-15291-7.00012-2
Dey, S., Satya, N. P., Ajaya, K. R., Jyotsna, D., Maharana, J., Pradhan, S. P., et al. (2024b). Nanobioremediation: a sustainable approach for environmental monitoring with special reference to the restoration of heavy metal contaminated soil and wastewater treatment. Role Green Chem. Ecosyst. Restor. Achieve Environ. Sustain., 41–49. doi:10.1016/B978-0-443-15291-7.00012-2
Dhanapal, A. R., Thiruvengadam, M., Vairavanathan, J., Venkidasamy, B., Easwaran, M., and Ghorbanpour, M. (2024a). Nanotechnology approaches for the remediation of agricultural polluted soils. ACS Omega 9 (12), 13522–13533. doi:10.1021/acsomega.3c09776
Dhanapal, A. R., Thiruvengadam, M., Vairavanathan, J., Venkidasamy, B., Easwaran, M., and Ghorbanpour, M. (2024b). Nanotechnology approaches for the remediation of agricultural polluted soils. ACS Omega 9 (12), 13522–13533. doi:10.1021/acsomega.3c09776
Elmore, J. R., Dexter, G. N., Baldino, H., Huenemann, J. D., Francis, R., Peabody, G. L., et al. (2023). High-throughput genetic engineering of nonmodel and undomesticated bacteria via iterative site-specific genome integration. Sci. Adv. 9 (10), eade1285. doi:10.1126/sciadv.ade1285
Ezeorba, T. P. C., Sunday Okeke, E., Habila Mayel, M., Nwuche, C. O., and Ezike, T. C. (2024). Recent advances in biotechnological valorization of agro-food wastes (AFW): optimizing integrated approaches for sustainable biorefinery and circular bioeconomy. Bioresour. Technol. Rep. 26, 101823. doi:10.1016/j.biteb.2024.101823
Gao, T., Guo, J., and Niu, W. (2024). Genetic code expansion in Pseudomonas putida KT2440. ACS Synth. Biol. 11, 209–217. doi:10.1007/978-1-0716-3658-9_13
Gong, J., Du, H., and Sun, Y. (2023). Collaboration among governments, pesticide operators, and farmers in regulating pesticide operations for agricultural product safety. Agriculture 13 (12), 2288. doi:10.3390/agriculture13122288
Gulati, S., Kumar, S., Mongia, A., Diwan, A., and Singh, P. (2023). “Regulatory and toxicological perspectives of carbon nanomaterials,” in Functionalized carbon nanomaterials for theranostic applications (Elsevier), 483–503. doi:10.1016/B978-0-12-824366-4.00005-4
Gutiérrez, Y., Alberto, M., Wong, A. Y.C.-H., Ibarra-Muñoz, L. A., Figueroa Chávez, A. C., Sosa-Martínez, J. D., et al. (2024). Perspectives and progress in bioethanol processing and social economic impacts. Sustainability 16 (2), 608. doi:10.3390/su16020608
Gwenzi, W., Bashir, A., Kumar, P., Odedishemi Ajibade, F., Silva, L. F. O., Choi, K. S., et al. (2024). Human viral pathogens in the wastewater-source water-drinking water continuum: evidence, health risks, and lessons for future outbreaks in low-income settings. Sci. Total Environ. 918, 170214. doi:10.1016/j.scitotenv.2024.170214
Hassanien, A., Saadaoui, I., Schipper, K., Al-Marri, S., Dalgamouni, T., Aouida, M., et al. (2023). Genetic engineering to enhance microalgal-based produced water treatment with emphasis on CRISPR/cas9: a review. Front. Bioeng. Biotechnol. 10, 1104914. doi:10.3389/fbioe.2022.1104914
Hidangmayum, A., Debnath, A., Guru, A., Singh, B. N., Upadhyay, S. K., and Dwivedi, P. (2023a). Mechanistic and recent updates in nano-bioremediation for developing green technology to alleviate agricultural contaminants. Int. J. Environ. Sci. Technol. 20 (10), 11693–11718. doi:10.1007/s13762-022-04560-7
Hidangmayum, A., Debnath, A., Guru, A., Singh, B. N., Upadhyay, S. K., and Dwivedi, P. (2023b). Mechanistic and recent updates in nano-bioremediation for developing green technology to alleviate agricultural contaminants. Int. J. Environ. Sci. Technol. 20 (10), 11693–11718. doi:10.1007/s13762-022-04560-7
Hu, G., Pei, Z., Shen, B., Li, Y., Wei, W., Zhang, J., et al. (2024). Correlation between surface structure of carbon dots and selective detection of heavy metal ions. Appl. Phys. A 130 (2), 122. doi:10.1007/s00339-023-07265-x
Huang, W., Chen, Z., Liu, H., Wang, H., and Zaishan, W. (2024). Microbial induced carbonate precipitation for cadmium removal in flue gas from sludge incineration. J. Environ. Chem. Eng. 12, 112573. doi:10.1016/J.JECE.2024.112573
Jagaba, A. H., Kutty, S. R. M., Isa, M. H., Lawal, I. M., Usman, A. K., Al-dhawi, B. N. S., et al. (2024). Toxicity of carbon nanotubes in wastewater treatment. Water Treat. Using Eng. Carbon Nanotub. January, 365–411. doi:10.1016/B978-0-443-18524-3.00004-0
Jatoi, A. H., Ali, A., Ahmed, N., Phulpoto, S. N., Iqbal, M., Ali Memon, A., et al. (2024). High-performance asparagine-modified graphene oxide membranes for organic dyes and heavy metal ion separation. New J. Chem. 48 (4), 1715–1723. doi:10.1039/D3NJ04552H
Jatoi, A. S., Hashmi, Z., Usman, T., Mujawar Mubarak, N., Ali Mazari, S., Rao Karri, R., et al. (2024a). “Role of carbon nanomaterials for wastewater treatment—a brief review,” in Water treatment using engineered carbon nanotubes (Amsterdam, Netherlands: Elsevier), 29–62. doi:10.1016/B978-0-443-18524-3.00016-7
Jatoi, A. S., Hashmi, Z., Usman, T., Mujawar Mubarak, N., Ali Mazari, S., Rao Karri, R., et al. (2024b). Role of carbon nanomaterials for wastewater treatment—a brief review. Water Treat. Using Eng. Carbon Nanotub., 29–62. doi:10.1016/B978-0-443-18524-3.00016-7
Jia, G., Tendo Innocent, M., Yu, Y., Hu, Z., Wang, X., Xiang, H., et al. (2023). Lignin-based carbon fibers: insight into structural evolution from lignin pretreatment, fiber forming, to pre-oxidation and carbonization. Int. J. Biol. Macromol. 226, 646–659. doi:10.1016/J.IJBIOMAC.2022.12.053
Jiang, J., Sohn, W., Almuntashiri, A., Phuntsho, S., Wang, Q., Freguia, S., et al. (2024). Feasibility study of powdered activated carbon membrane bioreactor (PAC-MBR) for source-separated urine treatment: a comparison with mbr. Desalination 580, 117544. doi:10.1016/J.DESAL.2024.117544
Joseph, A., and Vijayanandan, A. (2023). Review on support materials used for immobilization of nano-photocatalysts for water treatment applications. Inorganica Chim. Acta 545, 121284. doi:10.1016/J.ICA.2022.121284
Kaur, P., Kumar, S., Rani, J., Singh, J., Kaushal, S., Hussain, K., et al. (2024). Rationally tailored synergy between adsorption efficiency of cotton shell activated carbon and PMS activation via biogenic Fe0 or Cu0 for effective mitigation of triphenylmethane dyes. Sep. Purif. Technol. 342, 127010. doi:10.1016/j.seppur.2024.127010
Kausar, A., and Ahmad, I. (2024). Graphene quantum dots—nascent adsorbent nanomaterials for water treatment. Environ. Nanotechnol. Monit. Manag. 21, 100943. doi:10.1016/J.ENMM.2024.100943
Kim, M., Goerzen, D., Jena, P. V., Zeng, E., Pasquali, M., Meidl, R. A., et al. (2023). Human and environmental safety of carbon nanotubes across their life cycle. Nat. Rev. Mater. 9 (1), 63–81. doi:10.1038/s41578-023-00611-8
Kojima, D., Tanaka, S., Kurosaki, A., Zhiyu, X., and Ito, M. (2024). Isolation and Cs+ resistance mechanism of Escherichia coli strain ZX-1. Front. Microbiol. 14, 1340033. doi:10.3389/fmicb.2023.1340033
Kumar, K., Kumar, R., Kaushal, S., Thakur, N., Umar, A., Akbar, S., et al. (2023). Biomass waste-derived carbon materials for sustainable remediation of polluted environment: a comprehensive review. Chemosphere 345, 140419. doi:10.1016/j.chemosphere.2023.140419
Kumari, R., Suman, K., Karmakar, S., Mishra, V., Lakra, S. G., Saurav, G. K., et al. (2023). Regulation and safety measures for nanotechnology-based agri-products. Front. Genome Ed. 5, 1200987. doi:10.3389/fgeed.2023.1200987
Lee, Y., Lee, J., and Kim, S. (2023a). Direct extracellular electron transfer from Escherichia coli through modified carbon nanoparticles. Electrochimica Acta 437, 141497. doi:10.1016/j.electacta.2022.141497
Lee, Y., Lee, J., and Kim, S. (2023b). Direct extracellular electron transfer from Escherichia coli through modified carbon nanoparticles. Electrochimica Acta 437, 141497. doi:10.1016/j.electacta.2022.141497
Li, J., Guo, Yi, Li, J., Zhang, H., Wang, Li, and Liang, C. (2024). Improved stress tolerance of recombinant Escherichia coli strain expressing nitrile hydratase by combining global regulator IrrE. Mol. Catal. 557, 113904. doi:10.1016/J.MCAT.2024.113904
Liaquat, R., and Muddasar, M. (2024). Nanomaterial-assisted anaerobic digestion of biomass for bioenergy and biofuel production. Nanomater. Biomass Convers. January, 235–266. doi:10.1016/B978-0-443-13500-2.00007-9
Maurya, A., Sharma, D., Partap, M., Kumar, R., and Bhargava, B. (2023). Microbially-assisted phytoremediation toward air pollutants: current trends and future directions. Environ. Technol. Innovation 31, 103140. doi:10.1016/J.ETI.2023.103140
Mekonnen, B. A., Aragaw, T. A., and Genet, M. B. (2024). Bioremediation of petroleum hydrocarbon contaminated soil: a review on principles, degradation mechanisms, and advancements. Front. Environ. Sci. 12. doi:10.3389/fenvs.2024.1354422
Molavi, H., Mirzaei, K., Jafarpour, E., Mohammadi, A., Sepehr Salimi, M., Rezakazemi, M., et al. (2024a). Wastewater treatment using nanodiamond and related materials. J. Environ. Manag. 349, 119349. doi:10.1016/j.jenvman.2023.119349
Molavi, H., Mirzaei, K., Jafarpour, E., Mohammadi, A., Sepehr Salimi, M., Rezakazemi, M., et al. (2024b). Wastewater treatment using nanodiamond and related materials. J. Environ. Manag. 349, 119349. doi:10.1016/J.JENVMAN.2023.119349
Mousa, M. S., Tulbure, I., and Fawaeer, S. (2023). Environmental monitoring odds by carbon nanotubes: a sustainable approach. Adv. Challenges Sci. Technol. 9, 67–81. doi:10.9734/bpi/acst/v9/6620C
Nandy, S., and Kapley, A. (2024). Unraveling the potential of microbial communities for lake bioremediation via the metagenomics tool: a review. AQUA — Water Infrastructure, Ecosyst. Soc. 73 (1), 11–33. doi:10.2166/aqua.2024.154
Ningombam, L., Mana, T., Apum, G., Ningthoujam, R., and Singh, Y. D. (2024a). Nano-bioremediation: a prospective approach for environmental decontamination in focus to soil, water and heavy metals. Environ. Nanotechnol. Monit. Manag. 21, 100931. doi:10.1016/j.enmm.2024.100931
Ningombam, L., Mana, T., Apum, G., Ningthoujam, R., and Singh, Y. D. (2024b). Nano-bioremediation: a prospective approach for environmental decontamination in focus to soil, water and heavy metals. Environ. Nanotechnol. Monit. Manag. 21, 100931. doi:10.1016/J.ENMM.2024.100931
Obayomi, K. S., Yon Lau, S., Danquah, M., Chiong, T., and Takeo, M. (2022). Advances in graphene oxide based nanobiocatalytic technology for wastewater treatment. Environ. Nanotechnol. Monit. Manag. 17, 100647. doi:10.1016/j.enmm.2022.100647
Ogunsola, S. S., Ezekiel Oladipo, M., Olusakin Oladoye, P., and Kadhom, M. (2024). Carbon nanotubes for sustainable environmental remediation: a critical and comprehensive review. Nano-Structures Nano-Objects 37, 101099. doi:10.1016/J.NANOSO.2024.101099
Olawore, O., Ogunmola, M., and Desai, S. (2024). Engineered nanomaterial coatings for food packaging: design, manufacturing, regulatory, and sustainability implications. Micromachines 15 (2), 245. doi:10.3390/mi15020245
Pandey, G., Bajpai, S., Tripathi, S., Chauhan, R., and Sharma, P. (2022). Approaches, challenges, and prospects of life cycle assessment applied in nano bioremediation of contaminated sites. RASAYAN J. Chem. 15 (02), 1108–1125. doi:10.31788/RJC.2022.1526904
Pardhe, B. D., Lee, M.Ju, Lee, J. H., Do, H., and Oh, T.-J. (2023). Biochemical and structural basis of mercuric reductase, GbsMerA, from gelidibacter salicanalis PAMC21136. Sci. Rep. 13 (1), 17854. doi:10.1038/s41598-023-44968-w
Pereira, L., Castillo, V., Calero, M., González-Egido, S., Ángeles Martín-Lara, M., and Solís, R. R. (2024). Promoting the circular economy: valorization of a residue from industrial char to activated carbon with potential environmental applications as adsorbents. J. Environ. Manag. 356, 120753. doi:10.1016/J.JENVMAN.2024.120753
Petersen, E., Barrios, A. C., Bjorkland, R., Goodwin, D. G., Li, J., Waissi, G., et al. (2023). Evaluation of bioaccumulation of nanoplastics, carbon nanotubes, fullerenes, and graphene family materials. Environ. Int. 173, 107650. doi:10.1016/J.ENVINT.2022.107650
Poonia, K., Singh, P., Ahamad, T., Van Le, Q., Quang, H. H. P., Thakur, S., et al. (2024). Sustainability, performance, and production perspectives of waste-derived functional carbon nanomaterials towards a sustainable environment: a review. Chemosphere 352, 141419. doi:10.1016/J.CHEMOSPHERE.2024.141419
Pratap, J. K., and Krishnan, K. (2024a). “Microbial nanobioremediation of micro-nanoplastics: current strategies, challenges, and future prospects,” in Management of micro and nano-plastics in soil and biosolids (Cham: Springer Nature Switzerland), 419–445. doi:10.1007/978-3-031-51967-3_17
Pratap, J. K., and Krishnan, K. (2024b). “Microbial nanobioremediation of micro-nanoplastics: current strategies, challenges, and future prospects,” in Management of micro and nano-plastics in soil and biosolids (Cham: Springer Nature Switzerland), 419–445. doi:10.1007/978-3-031-51967-3_17
Rahman, Md. M., Ahmed, L., Anika, F., Akter Riya, A., Khatun Kali, S., Rauf, A., et al. (2023). Bioinorganic nanoparticles for the remediation of environmental pollution: critical appraisal and potential avenues. Bioinorganic Chem. Appl. 2023, 1–26. doi:10.1155/2023/2409642
Rajput, V. D., Minkina, T., Upadhyay, S. K., Kumari, A., Ranjan, A., Mandzhieva, S., et al. (2022a). Nanotechnology in the restoration of polluted soil. Nanomaterials 12 (5), 769. doi:10.3390/nano12050769
Rajput, V. D., Minkina, T., Upadhyay, S. K., Kumari, A., Ranjan, A., Mandzhieva, S., et al. (2022b). Nanotechnology in the restoration of polluted soil. Nanomaterials 12 (5), 769. doi:10.3390/nano12050769
Rajput, V. D., and Tatiana, M. (2023a). Editorial for special issue ‘nano-bioremediation approaches for degraded soils and sustainable crop production. Nanomaterials 13 (7), 1138. doi:10.3390/nano13071138
Rajput, V. D., and Tatiana, M. (2023b). Editorial for special issue ‘nano-bioremediation approaches for degraded soils and sustainable crop production. Nanomaterials 13 (7), 1138. doi:10.3390/nano13071138
Rather, M. A., Bhuyan, S., Chowdhury, R., Sarma, R., Roy, S., and Neog, P. R. (2023a). Nanoremediation strategies to address environmental problems. Sci. Total Environ. 886, 163998. doi:10.1016/j.scitotenv.2023.163998
Rather, M. A., Bhuyan, S., Chowdhury, R., Sarma, R., Roy, S., and Neog, P. R. (2023b). Nanoremediation strategies to address environmental problems. Sci. Total Environ. 886, 163998. doi:10.1016/j.scitotenv.2023.163998
Rather, M. A., Bhuyan, S., Chowdhury, R., Sarma, R., Roy, S., and Neog, P. R. (2023c). Nanoremediation strategies to address environmental problems. Sci. Total Environ. 886, 163998. doi:10.1016/j.scitotenv.2023.163998
Rather, M. A., Bhuyan, S., Chowdhury, R., Sarma, R., Roy, S., and Neog, P. R. (2023d). Nanoremediation strategies to address environmental problems. Sci. Total Environ. 886, 163998. doi:10.1016/J.SCITOTENV.2023.163998
Ray, R. R., and Pattnaik, S. (2024). Alcaligenes faecalis: a bacterium for sustainable management of environment. Environ. Qual. Manag., doi:10.1002/tqem.22189
Roy, R., Samanta, S., Pandit, S., Naaz, T., Banerjee, S., Mishra Rawat, J., et al. (2024). An overview of bacteria-mediated heavy metal bioremediation strategies. Appl. Biochem. Biotechnol. 196 (3), 1712–1751. doi:10.1007/s12010-023-04614-7
Sajjad, W., Ilahi, N., Haq, A., Shang, Z., Nabi, G., Rafiq, M., et al. (2024). Bacteria populating freshly appeared supraglacial lake possess metals and antibiotic-resistant genes. Environ. Res. 247, 118288. doi:10.1016/J.ENVRES.2024.118288
Salazar, S., Sebastián, T. B., Maldonado-Bravo, F., Paul, J., Nelson, C., Rojas-Romo, C., et al. (2023). Nanomaterials for potential detection and remediation: a review of their analytical and environmental applications. Coatings 13 (12), 2085. doi:10.3390/coatings13122085
Salehi, B., and Wang, L. (2022). Critical review on nanomaterials for enhancing bioconversion and bioremediation of agricultural wastes and wastewater. Energies 15 (15), 5387. doi:10.3390/en15155387
Sam, D. K., and Cao, Y. (2024). Porous carbon materials for adsorption: a mini-review. Fullerenes, Nanotub. Carbon Nanostructures, March, 1–12. doi:10.1080/1536383X.2024.2325524
Sandra de, la P., Fernández-Pampín, N., Garroni, S., Poddighe, M., de la Fuente-Vivas, D., Barros, R., et al. (2024). Comparative toxicological analysis of two pristine carbon nanomaterials (graphene oxide and aminated graphene oxide) and their corresponding degraded forms using human in vitro models. Toxicology 504, 153783. doi:10.1016/J.TOX.2024.153783
Sati, M., Sharma, V., Goswami, A. J., Giri, K., and Mishra, G. (2022). “Prospects and challenges of bio-nanomaterials for wastewater treatment,” in Water science and technology library (Berlin, Germany: Springer), 381–397. doi:10.1007/978-3-031-00812-2_18
Siddiqa, A., Majid, A., Saira, F., Farooq, S., Qureshi, R., and Qaisar, S. (2023a). Nanodiamond embedded polyaniline/polyvinylidene fluoride nanocomposites as microfiltration membranes for removal of industrial pollution. RSC Adv. 13 (42), 29206–29214. doi:10.1039/D3RA05351B
Siddiqa, A., Majid, A., Saira, F., Farooq, S., Qureshi, R., and Qaisar, S. (2023b). Nanodiamond embedded polyaniline/polyvinylidene fluoride nanocomposites as microfiltration membranes for removal of industrial pollution. RSC Adv. 13 (42), 29206–29214. doi:10.1039/D3RA05351B
Silah, H., Unal, D. N., Selcuk, O., and Uslu, B. (2024). Applications of zero-dimensional carbon nanomaterials in water treatment. Zero-Dimensional Carbon Nanomater. January, 577–609. doi:10.1016/B978-0-323-99535-1.00018-4
Singh, A., Dhau, J., Kumar, R., Badru, R., Singh, P., Mishra, Y. K., et al. (2024a). Tailored carbon materials (TCM) for enhancing photocatalytic degradation of polyaromatic hydrocarbons. Prog. Mater. Sci. 144, 101289. doi:10.1016/J.PMATSCI.2024.101289
Singh, R., Samuel, M. S., Ravikumar, M., Ethiraj, S., Savunthari Kirankumar, V., Kumar, M., et al. (2023). Processing of carbon-based nanomaterials for the removal of pollutants from water/wastewater application. Water 15 (16), 3003. doi:10.3390/w15163003
Singh, S., Khan, N. A., Ramadan, R., Shehata, N., Kapoor, D., Singh Dhanjal, D., et al. (2024b). Environmental fate, toxicological impact, and advanced treatment approaches: atrazine degradation and emphasises on circular economy strategy. Desalination Water Treat. 317, 100201. doi:10.1016/j.dwt.2024.100201
Singh, Y., and Saxena, M. K. (2022a). Insights into the recent advances in nano-bioremediation of pesticides from the contaminated soil. Front. Microbiol. 13, 982611. doi:10.3389/fmicb.2022.982611
Singh, Y., and Saxena, M. K. (2022b). Insights into the recent advances in nano-bioremediation of pesticides from the contaminated soil. Front. Microbiol. 13, 982611. doi:10.3389/fmicb.2022.982611
Singh, Y., and Saxena, M. K. (2022c). Insights into the recent advances in nano-bioremediation of pesticides from the contaminated soil. Front. Microbiol. 13, 982611. doi:10.3389/fmicb.2022.982611
Skinner, J., Delgado, A. G., Hyman, M., and Chu, M.-Y. J. (2024). Implementation of in situ aerobic cometabolism for groundwater treatment: state of the knowledge and important factors for field operation. Sci. Total Environ. 925, 171667. doi:10.1016/J.SCITOTENV.2024.171667
Soni, H., Bhattu, M., Priya, S. D., Kaur, M., Verma, M., and Singh, J. (2024). Recent advances in waste-derived carbon dots and their nanocomposites for environmental remediation and biological applications. Environ. Res. 251, 118560. doi:10.1016/J.ENVRES.2024.118560
Sornaly, H. H., Ahmed, S., Titin, K. F., Islam, Md N., Parvin, A., Islam, Md A., et al. (2024). The utility of bioremediation approach over physicochemical methods to detoxify dyes discharges from textile effluents: a comprehensive review study. Sustain. Chem. Pharm. 39, 101538. doi:10.1016/J.SCP.2024.101538
Sorouri, B., Rodriguez, C. I., Gaut, B. S., and Allison, S. D. (2023). Variation in Sphingomonas traits across habitats and phylogenetic clades. Front. Microbiol. 14, 1146165. doi:10.3389/fmicb.2023.1146165
Sravan, J. S., Matsakas, L., and Sarkar, O. (2024). Advances in biological wastewater treatment processes: focus on low-carbon energy and resource recovery in biorefinery context. Bioengineering 11 (3), 281. doi:10.3390/bioengineering11030281
Sun, J., Xing, He, Yilin, L. E., Al-Tohamy, R., and Ali, S. S. (2024). Potential applications of extremophilic bacteria in the bioremediation of extreme environments contaminated with heavy metals. J. Environ. Manag. 352, 120081. doi:10.1016/j.jenvman.2024.120081
Tripathi, V., Kumar Gaur, V., Kaur, I., Srivastava, P. K., and Manickam, N. (2024). Unlocking bioremediation potential for site restoration: a comprehensive approach for crude oil degradation in agricultural soil and phytotoxicity assessment. J. Environ. Manag. 355, 120508. doi:10.1016/j.jenvman.2024.120508
Trivedi, R., Upadhyay, T. K., Khan, F., Pandey, P., Kaushal, R. S., Sonkar, M., et al. (2024). Innovative strategies to manage polluted aquatic ecosystem and agri-food waste for circular economy. Environ. Nanotechnol. Monit. Manag. 21, 100928. doi:10.1016/J.ENMM.2024.100928
Venkatraman, G., Giribabu, N., Mohan, P. S., Muttiah, B., Kumar Govindarajan, V., Alagiri, M., et al. (2024). Environmental impact and human health effects of polycyclic aromatic hydrocarbons and remedial strategies: a detailed review. Chemosphere 351, 141227. doi:10.1016/J.CHEMOSPHERE.2024.141227
Verma, A. K., Chettri, D., Verma, A. K., Selvaraj, M., and Assiri, M. A. (2024). Bioprospecting lignin for biorefinery: emerging innovations and strategies in microbial technology. Biomass Bioenergy 181, 107052. doi:10.1016/J.BIOMBIOE.2024.107052
Verma, N., Jujjavarapu, S. E., Mahapatra, C., and Mutra, J. K. R. (2023). Contemporary updates on bioremediation applications of graphene and its composites. Environ. Sci. Pollut. Res. 30 (17), 48854–48867. doi:10.1007/s11356-023-26225-9
Vineeth Kumar, C. M., Karthick, V., Ganesh Kumar, V., Inbakandan, D., Rene, E. R., Uma Suganya, K. S., et al. (2022). The impact of engineered nanomaterials on the environment: release mechanism, toxicity, transformation, and remediation. Environ. Res. 212, 113202. doi:10.1016/j.envres.2022.113202
Wang, Li, Tan, Y.-S., Chen, K., Ntakirutimana, S., Liu, Z.-H., Li, B.-Z., et al. (2024a). Global regulator IrrE on stress tolerance: a review. Crit. Rev. Biotechnol., 1–21. doi:10.1080/07388551.2023.2299766
Wang, X., Lyu, H., Hu, Z., and Shen, B. (2024b). Application of molecular imprinting for targeted removal of organic contaminants and resistance genes from water: a review. J. Environ. Chem. Eng. 12 (2), 112068. doi:10.1016/J.JECE.2024.112068
Wu, Bo, Xiu, J., Yu, Li, Huang, L., Yi, L., and Ma, Y. (2023a). Degradation of crude oil in a Co-culture system of Bacillus subtilis and Pseudomonas aeruginosa. Front. Microbiol. 14, 1132831. doi:10.3389/fmicb.2023.1132831
Wu, Bo, Xiu, J., Yu, Li, Huang, L., Yi, L., and Ma, Y. (2023b). Degradation of crude oil in a Co-culture system of Bacillus subtilis and Pseudomonas aeruginosa. Front. Microbiol. 14, 1132831. doi:10.3389/fmicb.2023.1132831
Xiang, X., Bai, J., Gu, W., Peng, S., and Shih, K. (2023). Mechanism and application of modified bioelectrochemical system anodes made of carbon nanomaterial for the removal of heavy metals from soil. Chemosphere 345, 140431. doi:10.1016/j.chemosphere.2023.140431
Xu, Q., Zhang, Y., Yang, R., Li, J., Chen, J., Wang, J., et al. (2024). The utilization of Lysinibacillus bacterial powder to induce Fe plaque formation mitigates cadmium and chromium levels in rice. J. Hazard. Mater. 463, 132825. doi:10.1016/J.JHAZMAT.2023.132825
Xue, Y., Qiu, T., Sun, Z., Liu, F., and Yu, Bo (2022). Mercury bioremediation by engineered Pseudomonas putida KT2440 with adaptationally optimized biosecurity circuit. Environ. Microbiol. 24 (7), 3022–3036. doi:10.1111/1462-2920.16038
Yahyaee, T., and Mofidi, S. R. (2024). The impact of multi-walled carbon nanotubes on the mechanical and environmental properties of porous concrete in removing pollutants for use in surface runoffs. Case Stud. Constr. Mater. 20, e02697. doi:10.1016/J.CSCM.2023.E02697
Yang, H., Qi, F., Zhu, J., Liu, G., Dai, Y., Zhou, Q., et al. (2024). Towards sustainable futures: a review of sediment remediation and resource valorization techniques. J. Clean. Prod. 435, 140529. doi:10.1016/j.jclepro.2023.140529
Yotinov, I., Belouhova, M., Foteva, A., Dinova, N., Todorova, Y., Schneider, I., et al. (2022). Application of nanodiamonds in modelled bioremediation of phenol pollution in river sediments. Processes 10 (3), 602. doi:10.3390/pr10030602
Zhang, P., Zhang, L., Yang, X., Chi, M., Han, Y., Zhang, Z., et al. (2024). Cotton-derived three-dimensional carbon fiber aerogel with hollow nanocapsules and ultrahigh adsorption efficiency in dynamic sewage treatment system. Bioresour. Technol. 399, 130563. doi:10.1016/J.BIORTECH.2024.130563
Zhang, R., Li, J., Zhou, L., Zhuang, H., Shen, S., and Wang, Y. (2022). Effect of graphene and graphene oxide on antibiotic resistance genes during copper-contained swine manure anaerobic digestion. Environ. Sci. Pollut. Res. 30 (10), 27863–27874. doi:10.1007/s11356-022-23741-y
Zhang, W., Zhang, H., Xu, R., Qin, H., Liu, H., and Zhao, K. (2023). Heavy metal bioremediation using microbially induced carbonate precipitation: key factors and enhancement strategies. Front. Microbiol. 14, 1116970. doi:10.3389/fmicb.2023.1116970
Keywords: nanobioremediation, carbon-based nanomaterials, bacteria, genetic engineering, industrial effluents, bionanocomposite, circular economy
Citation: de Carvalho Lima EN, Souza LHM, Aguiar EM, Octaviano ALM, Justo JF and Piqueira JRC (2024) Can bacteria and carbon-based nanomaterials revolutionize nanoremediation strategies for industrial effluents?. Front. Nanotechnol. 6:1389107. doi: 10.3389/fnano.2024.1389107
Received: 29 February 2024; Accepted: 22 April 2024;
Published: 05 June 2024.
Edited by:
Baljeet Singh Saharan, Washington State University, United StatesReviewed by:
Azim Uddin, Zhejiang University, ChinaNitai Debnath, Amity University Gurgaon, India
Anita Rani Santal, Maharshi Dayanand University, India
Joginder Singh Duhan, Chaudhary Devi Lal University, India
Copyright © 2024 de Carvalho Lima, Souza, Aguiar, Octaviano, Justo and Piqueira. This is an open-access article distributed under the terms of the Creative Commons Attribution License (CC BY). The use, distribution or reproduction in other forums is permitted, provided the original author(s) and the copyright owner(s) are credited and that the original publication in this journal is cited, in accordance with accepted academic practice. No use, distribution or reproduction is permitted which does not comply with these terms.
*Correspondence: Elidamar Nunes de Carvalho Lima, ZWxpZGFtYXJudW5lc0B1c3AuYnI=