- 1Lab No 202 Microbial Biotechnology, Department of Bio and Nano Technology, Guru Jambheshwar University of Science and Technology, Hisar, India
- 2Amity Institute of Biotechnology, Amity University Gurugram, Gurugram, India
Wastewater remediation is of paramount importance for the water-stressed modern civilization from the perspective of sustainable development and the management of limited freshwater resources. Contaminants such as heavy metal and metalloid ions, toxic dyes, and pathogenic microbes present in the aquatic ecosystem have adverse effects not only on the environment but also on human health. Traditional wastewater remediation processes are costly, energy-consuming, and have less efficiency in removing pollutants from wastewater. Multi-phasic nanomaterials or nanocomposites have enormous potential for removing contaminants from water bodies. These materials have an enormous tunable surface area for interacting with the target toxicants and are extremely stable in the aquatic system, but they readily agglomerate. Many research groups have exploited biosorption, bioleaching, and bioprecipitation capacities of fungal hyphae for wastewater remediation. Recently, researchers have attempted to explore the synergistic effect of the bionanocomposite of fungal hyphae and nanomaterials for removing pollutants from wastewater. This bionanocomposite has already shown promise as a superior material for wastewater treatment and can easily be separated from the polluted water for reuse over several cycles. The bionanocomposite-mediated decontamination of water can lead to a total paradigm shift in wastewater management strategies.
1 Introduction
Increasing anthropogenic activity and global climate change are pushing the already water-stressed human civilization into an era where access to fresh water will be limited for a majority of individuals (Anjum et al., 2019). In today’s world, one-third of the drinking water requirement is fulfilled from various surface water sources, such as rivers, lakes, dams, and canals (Edokpayi et al., 2017). Pollution in these water bodies is not only escalating the potable water crisis but also adversely affecting the environment (Häder et al., 2020). Impact on the aquatic ecosystem becomes more severe because of the bioaccumulation and biomagnification of contaminants in water bodies (Kaushal and Singh, 2017). Regarding the world’s water consumption, the industrial sector accounts for 22% on average. Nearly 80% of the wastewater produced was dumped into waterways, which damages human health, pollutes the environment, and harms aqueous lives (Dutta et al., 2021). Recycling of this water is of paramount importance for sustainable development, environment protection, and above all, healthy human life. Water contaminants can be pathogenic microbes, such as bacteria, fungi, and protozoa, which mostly come from domestic sewage. Industrial effluents, mining, unplanned use of agrochemicals, plastics, vehicular emission, etc., contaminate the water bodies with heavy metals and metalloids, such as lead (Pb), cadmium (Cd), mercury (Hg), chromium (Cr), cobalt (Co), nickel (Ni), arsenic (As), fluoride (F), uranium (U), copper (Cu), and toxic dyes. All these may cause health disorders such as diarrhea, anemia, renal damage, encephalopathy, hepatitis, miscarriage, cancer, kidney damage, respiratory problems, DNA damage, and carcinogenicity (Baby et al., 2019).
In general, conventional wastewater treatment involves chemical precipitation, ion exchange, redox reaction, reverse osmosis, ultrafiltration, adsorption, electrodialysis, and membrane separation processes (Fu and Wang, 2011). Each treatment process has its own limitations, including the high cost of design, construction, and maintenance; higher energy input; complex operational process; and high-cost chemical additives (Crini and Lichtfouse, 2019). In this context, scientists are looking for cost-effective and more efficient materials for wastewater remediation (Ahmad et al., 2021). Microbes act as a good template, and due to their rapid growth, easy availability, and fast reproducing capacity, they are widely used in practical applications (Cheng et al., 2015). Bioremediation is a process of degradation, transformation, and adsorption of unwanted materials via microbes such as bacteria, microalgae, and fungi. Fungi-based remediation is known as mycoremediation; fungi are excellent scavengers of nature, with a high capacity of metal uptake capacity, due to the presence of extracellular and intracellular enzymatic machinery for the removal of heavy metal ions via biosorption and degradation. Hence, fungi were acting as a potential bioremediating agent. Several filamentous fungi, such as Trichoderma, Penicillium, Aspergillus flavus, A. niger, and A. fumigatus, were used to remove heavy metal ions from contaminated water (Joshi et al., 2011; Kumar et al., 2020; Vaksmaa et al., 2023). Recent advances in nanoscience and technology have paved the way for the development of better wastewater treatment strategies. An enhanced surface area to volume ratio, increased reactivity, and tunable surface functionality make nanoparticles (NPs) suitable as novel materials for adsorbing heavy metal ions and dyes and removing pathogenic microbes from contaminated water (Vunain E et al., 2016). Despite having several advantages, a single nanomaterial-enabled wastewater management regime has several technical bottlenecks arising due to nanoparticle agglomeration, difficulty in separation, nanomaterial leakage into the contact water, etc. However, to overcome these problems, fungus-based bionanocomposites (NCs) can be used as superior materials for the removal of heavy metal ions from wastewater. With negligible aggregation, excellent enrichment, and high exposure of the surface area of the nanomaterials, the bionanocomposite can grow consistently across the entire surface of the microorganisms. The structure of the composite can be guided and controlled at the microscale using fungus as the template, which is low-cost and has great compatibility (Xu et al., 2013). Over the past decade, there has been a significant increase in research related to heavy metal ion removal from wastewater. This growing trend is driven by the growing awareness of environmental pollution and the adverse effects of heavy metals on human health. This increasing trend in research articles (Figure 1) reflects the ongoing efforts to find sustainable, cost-effective, and efficient solutions for heavy metal removal. Researchers are also exploring the integration of multiple techniques to achieve synergistic effects and optimize the overall performance of wastewater treatment systems.
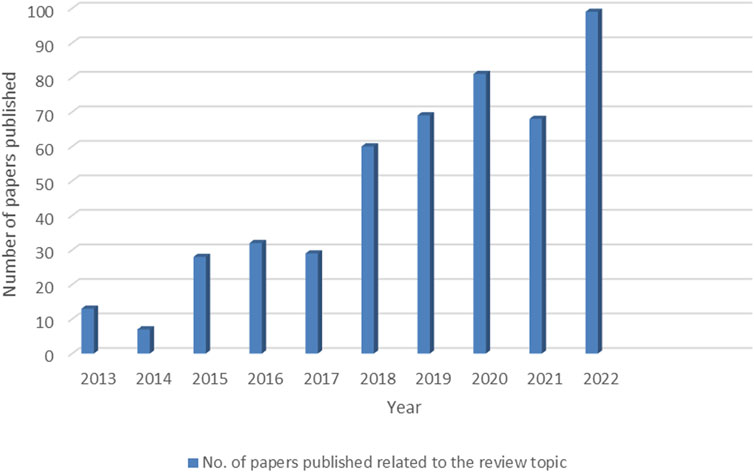
FIGURE 1. Dataset for the papers published in the last decade related to the removal of heavy metal ions using nanocomposites and bionanocomposites.
In this review article, the recent advancements in the use of different nanocomposites and fungal-based bionanocomposites for decontaminating wastewater pollutants such as heavy metals are discussed.
2 Nanocomposites
NCs are multi-phasic solid materials with at least one phase in the nanoscale (1–100 nm) domain (Dutta et al., 2020). The physico-chemical properties of NCs depend on the morphology and interfacial characteristics of the component materials, and these properties can be precisely tuned with different chemical species to increase their functionality toward specific targets. NCs have widespread applications in various sectors, such as the aerospace, automotive, electronics, and biotechnology industries; bio-/chemical sensing; drug delivery; microwave absorbing devices; tumor detection; orthopedic application; and wastewater remediation (Müller et al., 2017; Beyene and Ambaye, 2019; Said et al., 2020).
Based on the morphology of the component present in the individual phase, NCs can be classified into three principal types: 1) nanolayered composite, comprising alternating layers of nanoscale dimension; 2) nano filamentary composite comprising a matrix with embedded nanoscale diameter filaments; and 3) nanoparticulate composite consisting of a matrix with embedded nanoscale particles (Pandya et al., 2017). Based on structural materials, NCs can be classified into non-polymer NCs or NPNCs (such as metal/metal, metal/ceramic, and ceramic/ceramic NPNCs) and polymer NCs or PNCs (polymer/ceramic, inorganic/organic, inorganic/organic hybrid, polymeric/layered silicate, polymer/polymer PNCs, and biocomposites) (Hussain et al., 2006; Omanović-Mikličanin et al., 2020).
In the last decade, nanomaterials have been used for the development of novel high-tech materials such as membranes, adsorption materials, nanocatalysts, functionalized surfaces, coatings, and reagents for the efficient removal of water contaminants. However, the wide-scale use of NPs was restricted, mainly due to their instability, which arises because of their large surface area and strong interparticle attractive interaction leading to agglomeration. In NCs, the attractive forces between NPs causing agglomeration can be overcome without altering the nanoparticle structure by modulating the interphase properties. Uncontrolled dispersion of NPs is another challenge, which may result in the remobilization of contaminants in groundwater (Müller et al., 2017). Moreover, the long-term ecotoxicological effects of these dispersed NPs are yet to be fully evaluated, and they may have hazardous effects in living organisms in the future. Again, this disadvantage can be overcome by converting nanomaterials to nanocomposite (Pandey et al., 2017). Altogether, NCs have great potential to treat wastewater because of their high surface area, high chemical reactivity, excellent mechanical strength, and cost-effectiveness. They can remove bacteria, viruses, and inorganic and organic pollutants from contaminated water through multimodal interactions, such as chelation, absorption, and ion exchange (Beyene and Ambaye, 2019).
3 Nanocomposites for heavy metal ion removal from wastewater
Metal and metalloid ions such as Pb2+, Cd2+, Hg2+, Cr6+, Co2+, Ni2+, As3+, F−, U6+, and Cu2+ are the main pollutants present in wastewater (Wang et al., 2012). Because of high water solubility and non-biodegradability, heavy metal ions interact with biotic and abiotic components to be accumulated within living organisms. The adverse effects of heavy metal exposure on human health are shown in Figure 2, and the permissible limit of these toxicants in drinking water, according to the World Health Organization (WHO) and the Environmental Protection Agency (EPA) guidelines, is listed in Table 1. The most popular method for the removal of heavy metals from wastewater is adsorption. However, the major limitations of popular adsorbent materials are their low adsorption capacities, relatively weak interactions with metallic ions, and difficulties in the separation and reusability of wastewater. In this context, NCs are an excellent adsorbent material for heavy metal removal because their enhanced surface area provides more sites for chemical interaction, and surface functionalization increases their affinity for specific target ions. Nanocomposite-mediated heavy metal remediation strategies are summarized in Supplementary Table S1 and explained below.
3.1 Lead removal
Pb contamination in the environment is caused by industrial activities, mine tailings, and disposal of high-metal wastes, leaded fossil fuels, paints, the application of fertilizers, animal manures, sewage sludge, pesticides, untreated wastewater irrigation, coal combustion residues, spillage of petrochemicals into water, etc. According to the WHO, the permissible limit of Pb that may be present in drinking water is 3–10 μg L−1 (Shehu and Lamayi, 2019; Table 1). Pb toxicity causes damage to various organs, such as the liver, kidney, lungs, reproductive system, and circulatory system, and impairs brain functions. It also affects plant growth by interrupting the transport mechanism and even hindering photosynthesis.
Various NCs are currently undergoing experiments as potential materials for the removal of Pb2+ from wastewater (Lofrano et al., 2016). Polymer-layered silicate nanocomposites were used earlier because of their capacity to filter various-sized particles from industrial effluents (Pavlidou and Papaspyrides, 2008). Musico et al. (2013) blended poly(N-vinylcarbazole) (PVK) with graphene oxide (GO) to make a PVK–GO nanocomposite that could adsorb heavy metal ions from wastewater. The higher the amount of GO in the polymer nanocomposites, the more they adsorbed Pb2+ ions as the concentration of oxygen-containing functional groups resulted in an increase in adsorption capacity. The GO–montmorillonite nanocomposite (GMN) can be prepared by adding stearyl trimethyl ammonium chloride as a crosslinking agent in the suspension of montmorillonite and GO. This fabricated nanocomposite appeared to have a honeycomb texture, providing the chemical reaction site for the adsorption of Pb2+, although the efficiency of adsorption was dependent on pH, contact time, contact temperature, and GMN dosage during the adsorption process (Zhang et al., 2019). Chitosan/sulfydryl-functionalized GO composite (CS/GO-SH), a novel NC containing multifunctional groups, such as -OH, -COOH, -SH, and -NH2, was prepared by Li et al. (2015). –OH, –COOH, and -SH groups were effective for the removal of Pb2+ ions from an aqueous solution. A molybdenum sulfide (MoS2)/thiol-functionalized MWCNT (MoS2/SH- MWCNT) NC was synthesized and tested for the removal of Pb2+. This could efficiently remove Pb2+ from mine water effluents (Gusain et al., 2019). Porous metal-organic frameworks (MOFs) have shown great potential to remove hazardous compounds from water. They can adsorb pollutants by their coordinatively unsaturated site or by forming a hydrogen bond, electrostatic interaction, or π-complex formation (Khan et al., 2013). A magnetic NC based on aluminum MOF (MIL-53) was synthesized, and the composite exhibited great removal capacity as high as 492.4 mg g–1 for Pb2+ (Ricco et al., 2015).
A polyaniline polystyrene (PANI/PS) NC was studied for the removal of Pb2+ from wastewater, and its efficiency was as high as 95% (Bhadra et al., 2016). Its maximum adsorption efficiency was achieved at pH 5. Agricultural waste can also be converted into novel low-cost nanoadsorbents using various physical, biological, or chemical techniques. A nanoadsorbent made up of chemically modified orange peel has the potential to remove Pb2+ ions from polluted water. Silica coating on this orange peel (SiO2@OPW) made it a more efficient adsorbent. SiO2@OPW showed maximum Pb2+ ion removal capacity at pH 6 and a concentration of 0.02 g L−1 with a contact time of 60 min (Saini et al., 2020). Lingamdinne et al. (2020) prepared a mesoporous hexagonal crystalline iron NC from tangerine peels. This could help remove Pb2+ from wastewater with 95% efficiency. Another group made silica from rice husk and coupled it with GO in situ using (3-aminopropyl) triethoxysilane as a coupling agent. The obtained NC had an enhanced surface area and was used to remove Pb2+ from an aqueous solution (Thy et al., 2021). The hydroxyapatite nanoadsorbent prepared by microwave irradiation of egg shells also had high adsorption capacity for Pb2+ from wastewater samples (Safatian et al., 2019). Magnetic NPs are used as an effective adsorbent because of their simplicity of synthesis, large surface area, and high efficiency for the removal of heavy metal ions from wastewater. CaO/Fe3O4 was used as a magnetic adsorbent for the effective removal of Pb2+ from wastewater (Shakerian and Esmaeili, 2018). The magnetic properties of magnetite nanocrystals were unaffected in the magnetite–hydroxyapatite NC, prepared by in situ precipitation of the calcium phosphate phase in iron oxide colloidal suspension. This NC has a high affinity for aqueous Pb2+ ions (Yang et al., 2015). A novel magnetic NC comprising GO and magnetic chitosan-ionic liquids (GOMCS-ILs) showed excellent Pb2+ removal capacity. The incorporation of ionic liquid not only improved the dispersivity of the adsorbent but also increased the adsorption sites for Pb2+ ions (Sun et al., 2016). An exceptional nanocomposite was made via coating of micron-sized polystyrene beads (MPS) with MoS2 nanoflowers via the adhesive agent polydopamine (PDA) between the layers for the removal of Pb ions from wastewater with a maximum adsorption capacity of 371.7 mg g−1 (Hao et al., 2023).
The biochar fibril–magnesium oxide composite (BCF@MgO) was investigated for the removal of Cd and lead ions, with maximum removal capacities for Cd2+ and Pb2+ 2035.4 and 3410.1 mg g−1, respectively. If these two heavy metal ions were found in the same system, the removal efficiency could reach up to 100%, and furthermore, this composite will also help reduce secondary pollution (Zhao et al., 2023). An nMgO–bentonite nanocomposite was prepared and tested for the removal of Pb ions from aqueous solution, and the maximum adsorption efficiency was found to be 4.5 times that of bentonite alone; it was 75 mg g−1 at pH 9 and at a temperature of 307 K (Elkhatib et al., 2022). Ateia et al. (2020) found that citrate auto-combustion techniques have been used to develop magnetic NPs with graphene adsorbents (CoFe2O4/GR) for the removal of Pb ions. The outcomes indicated that CoFe2O4/0.02 GR effectively removed 99% of Pb2+ from water. The prepared samples’ distinctive advantages include ease of separation, high adsorption per unit area, low cost, and remarkable performance when recycled. The novel graphene oxide–polyether sulfone nanocomposite (GPN) membrane was examined for the removal of heavy metal ions. The removal efficiencies of heavy metal elimination in descending order of Pb2+, Al3+, Cu2+, and Fe2+ were 94%, 67%, 63%, and 43%, respectively. MSP-PANI was prepared using sporopollenin, magnetic NPs and polyaniline Pb2+ were better absorbed from wastewater samples using MSP-PANI, and at pH 6, adsorbent dosage 40 mg, and contact time 90 min, the maximum adsorption efficiency was found to be 95% (Mosleh et al., 2022). An eco-friendly NC was synthesized by coating cellulose nanocrystals (CNCs) with silver (Ag) and zinc oxide (ZnO) NPs separately and investigated for the removal of Pb ions. When compared to CNCs, the Ag/CNC nanocomposite improved Pb2+ uptake by approximately 22% and ZnO/CNC uptake by approximately 60% (Badawy et al., 2021). An iron nanoparticle-decorated adsorption material based on carboxymethylcellulose (CMC) and GO has been produced hydrothermally to purify heavy metal water. At pH 6 and contact time 30 min, the GO/CMC/FeNP nanocomposite showed a maximum removal capacity of 1850 mg g−1 for Pb ions (Neskoromnaya et al., 2022).
3.2 Cadmium removal
Cd is extremely toxic because of its non-biodegradability and its ability to accumulate in biotic and abiotic components. Cd is used in batteries (Ni–Cd batteries), pigments, alloys, fertilizers, and metal plating. According to WHO guidelines, the permissible Cd concentration in drinking water is 0.003 mg L−1 (Swaddiwudhipong et al., 2012), and the EPA considers the presence of Cd in less than 0.005 mg L−1 concentration to be safe (Gusain et al., 2019; Table 1). Several diseases, such as renal damage, diarrhea, emphysema, hypertension, testicular atrophy, and skeletal malformation in the fetus, are related to Cd toxicity (Fowler, 2009). In Japan, a deadly disease called itai–itai is reported due to Cd poisoning in the Jinzu River basin (Georgescu et al., 2019).
Biodegradable cellulose nanofibers obtained from sugarcane waste were doped using nano MgS to increase their adsorption capacity for Cd2+ ion removal from wastewater. Cellulose nanofibers acted as a template for the effective dispersion of MgS and also helped prevent the aggregation of nano MgS. MgS provided S2+ ions, which complexed with Cd2+ and caused their removal from wastewater (Sankararamakrishnan et al., 2019). Magnetic CaCO3 nano adsorbents were found to possess an outstanding capacity to adsorb Cd2+ ions (Wang et al., 2020). MoS2/SH-MWCNT NC, synthesized by Gusain et al. (2019), could also remove Cd2+ from contaminated water effluents. A magnetite/carbon NC was prepared by Andelescu et al. (2018) and was investigated as an adsorbent for the effective removal of Cd2+ from wastewater. A cobalt ferrite NC was prepared, and its efficacy in adsorbing Cd2+ from synthetic wastewater was studied. At pH 7, 100% of Cd2+ ions were removed from wastewater (Khoshkerdar and Esmaeili, 2019). The chitosan/sulfydryl-functionalized GO composite was found to be effective in adsorbing Cd2+ from wastewater because of the presence of -NH2 and –SH groups in the NC (Li et al., 2015). Fosso-Kankeu et al. (2017) reported the use of an NC made up of gelatine hydrogel and clinoptilolite for the adsorption of Cd2⁺ ions from mine effluents. Sharififard et al. (2018) prepared chitosan and activated carbon from shrimp shells and grape stalks, respectively. These materials were used to synthesize activated carbon/iron/chitosan NCs, which showed excellent Cd2+ removal capacity. In another experiment, acrylamide was in situ doped into titanium by a sol–gel reaction, and this NC was used for Cd2+ removal from an aqueous solution (Sharma and Lee, 2014). BCF@MgO was investigated for the removal of cadmium and lead ions, and the maximum removal capacities for Cd2+ and Pb2+ were 2035.4 and 3410.1 mg g−1, respectively. If these two heavy metal ions were found in the same system, the removal efficiency could reach up to 100%, and furthermore, this composite could reduce secondary pollution (Zhao et al., 2023). An Mg–Fe binary layered hydroxide/graphene oxide (Mg–Fe LDH/GO) NC was synthesized and investigated for the removal of Cd2+ from wastewater, and the highest uptake capacity was found to be 174.83 mg g−1. The Mg–Fe LDH/GO NC efficiently eliminated 99% of Cd2+ from the environment. It could be used more than four times before it began to diminish its stability and capacity (Narasimharao et al., 2022). Using a low-temperature plasma-induced process, polyaniline-functionalized magnetic graphene oxide (PANI-g-MGO) composites were successfully synthesized. The removal of Cd in the presence of phenol by the PANI-g-MGO composite was found to be 99.7%, and the removal of phenol in the presence of Cd by the PANI-g-MGO composite was found to be 99.3% (Zong et al., 2020).
3.3 Mercury removal
The removal of Hg from water resources is a serious environmental management endeavor due to its short-term or long-term toxic effects on human health and the aquatic ecosystem. The major problem with Hg toxicity arises from its nature of bio-accumulation in the ecosystem (Malar et al., 2015). Hg2+ ions are released into the ecosystem from different industries such as paper, plastic, paints, batteries, pharmaceuticals, and oil refineries. Hg toxicity can lead to pneumonia, cerebral palsy, kidney damage, etc. (Bernhoft, 2012). Many NCs are now being investigated for their potential to remove Hg2+ ions from wastewater. Rahmanzadeh et al. (2016) used a novel adsorbent comprising a magnetite–polyrhodanine core shell for the efficient removal of Hg2+ from wastewater. Its maximum adsorption capacity of 29.14 mg g−1 was achieved at pH 6.5° and 25°C with 10 g L−1 nanoadsorbent. A mesoporous magnetic zirconium phosphate (ZrP/Fe3O4) NC was found to have remarkable potential for the removal of Hg2+ from contaminated water (Ahamad et al., 2017). Although ZrP has a strong affinity for toxic metal ions and is stable in water, toxic metal-loaded ZrP cannot be readily separated from an aqueous medium. This problem can be overcome by making an NC of Fe3O4 and ZrP, as this NC can be separated magnetically. A natural quartz/silver NC was found to be a more effective adsorbent than nano Ag alone for the removal of Hg2+ from polluted water sources. The NC could remove Hg2+ ions with 96% efficiency at 60 min and at pH 6, with an adsorbent dosage of 0.5 g (El-Tawil et al., 2019). Chavan et al. (2015) prepared an elastomeric polymer NC made up of ZnSe nanocrystal-capped polydimethylsiloxane (PDMS), which could entrap heavy metal ions such as Hg2+ and successfully remove these toxicants by a cation exchange reaction. CdS/carbon NCs, synthesized from chestnut and cadmium nitrate, could successfully remove heavy metals such as Hg2+ from water (Ebadi et al., 2016). Although activated carbon is one of the most commonly used adsorbents for pollutant removal, it is expensive. Al-Ghouti et al. (2019) prepared silane- and sulfur-modified roasted date pits and applied these for Hg2+ removal from water. It was observed that Ag NPs uniformly doped in ordered mesoporous silica can reduce Hg2+ to Hg, which ultimately reacts with Ag present in the NC, followed by alloy formation (Ganzagh et al., 2016). A MnO2-coated magnetic NC (Fe3O4/MnO2) was prepared by the hydrothermal process, and the thiol-functionalized hierarchical zeolite NC showed good sorption ability for Hg2+ ions (Xia et al., 2019). The production and application of CoS2/GO NCs as high-efficiency adsorbents for Hg2+ removal in a water system was also addressed. Even when other interfering metal ions exist, CoS2/GO adsorbents exhibited excellent performance in capturing Hg2+ from wastewater (1,267 mg g−1), which was comparatively higher than that of other metal sulfide adsorbents. They also rapidly reduced Hg2+ contaminants below the critical level for drinking water (2 ppb) (Wang et al., 2020). By combining activated carbon (AC) produced by a waste rubber with a copolymer of diethylenetriamine (DETA) and trimesoyl chloride (TMC), a novel adsorbent was developed.
The AC/DETA-TMC NC was shown to have an adsorption capacity of 317.3 mg g−1 for inorganic mercury (Hg2+) and 263.6 mg g−1 for organic mercury (methyl mercury; [CH3Hg]+) (Tuzen et al., 2022). Malachite green (MG), an anionic dye known as reactive red 120 (RR120), and Hg2+ were effectively eliminated from an aqueous solution using low-cost epichlorohydrin crosslinked chitosan at magnetic Fe3O4/activated carbon NC, known as CH-EP@Fe3O4/AC. The maximum adsorption capacity is 166.6 mg g−1 (Kaveh and Bagherzadeh, 2022). To eliminate both inorganic pollutants, such as Hg2+ and Cu2+, and organic pollutants, such as methylene blue (MB) and crystal violet (CV), from wastewater, an EDTA-functionalized graphene oxide–chitosan nanocomposite (GO-EDTA-CS) was developed. For Hg2+, Cu2+, MB, and CV, the maximum adsorption efficiencies of the adsorbent were determined to be 324 ± 3.30, 130 ± 2.80, 141 ± 6.60, and 121 ± 3.50 mg g−1, respectively. The presence of a few active functional groups on the adsorbent, such as -COOH, -OH, and -NH2, was considered to be the cause of the outstanding adsorption capacity (Verma et al., 2022).
3.4 Chromium removal
Cr is one of the most common metallic contaminants present in water. The sources of Cr include different chemical dyes, mining, electroplating, metal processing, leather tanning, and automobile manufacturing industries (Rodríguez et al., 2018). Although Cr can be present in numerous oxidation states (from −2 to +6) depending on the medium’s chemical and physical properties (Malaviya and Singh, 2016), in an aqueous solution, Cr is majorly present as chromite (Cr3+) or chromate (Cr6+) ions. Cr6+ present in water is 1,000 times more toxic than Cr3+ because of its mobility. Overexposure to Cr6+ ions may lead to various diseases, such as liver damage, nephritis, stomach distress, and even cancer (Baby et al., 2019; Maitlo et al., 2019). Khoshkerdar and Esmaeili (2019) reported that a cobalt ferrite NC could remove 100% Cr3+ from wastewater at pH 7. This NC could be used as a sorbent and photo-catalyzer for the purification of contaminated water. Photocatalysis is an attractive treatment technique for the removal of hexavalent Cr from wastewater. In acidic water solutions, TiO2 is mostly used for the photocatalytic reduction of Cr6+ (Cheng et al., 2015). A mixture of TiO₂ and titanate nanotubes gained popularity as it could simultaneously remove Cr6+ and Cr3+ from wastewater by a photocatalytic reduction of Cr6+ followed by the subsequent adsorption of Cr3+ ions (Figure 3). Previously, the removal of Cr3+ and Cr6+ was a two-step process. This single-step process reduced the reaction time by 50%. The combination of photocatalysis and adsorption plays an important role in increasing the efficiency of the Cr removal process (Liu et al., 2014).
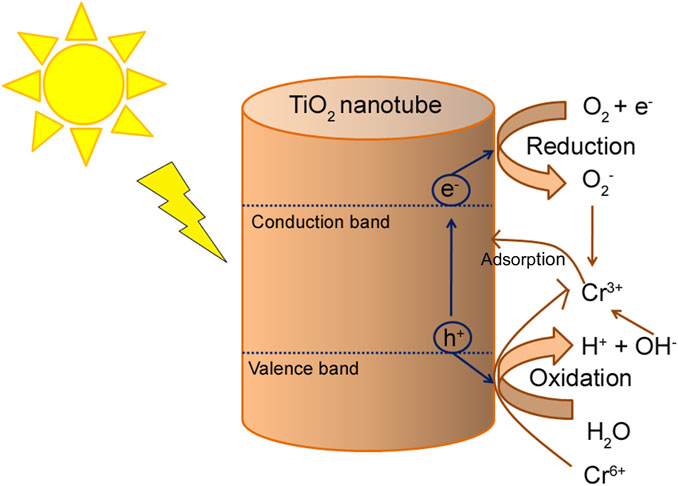
FIGURE 3. Photocatalytic reduction of Cr+6 into Cr+3 followed by subsequent adsorption of Cr+3 by the composite of TiO2 and titanate nanotubes.
Several carbon-based NCs were also explored as potential adsorbents of toxic Cr ions from wastewater. A carboxyl-rich carbon NC based on natural diatomite was an effective adsorbent for removing Cr6+ from an aqueous solution. It had optimum adsorption efficiency at pH 1 (Sun et al., 2020). Han et al. (2018) fabricated a novel NC of microspherical carbon loaded with a nanoscale zerovalent iron to remove Cr6+ from water with 100% efficiency. The resultant NC could be separated from water because of its magnetism. Similarly, the magnetic NC of GO and rGO is applied not only to enhance the adsorption characteristics but also to allow magnetic recovery (Abu -Nada et al., 2020). Liu et al. (2018) synthesized a sandwiched NC using GO, MnO2 nanowires, nano Fe3O4, and polypyrrole for the removal of hexavalent Cr ions from water via an adsorption–reduction process. In the sandwiched NCs, GO provided an enhanced surface area, functional groups, and hydrophilic surface for efficient absorption. Fe3O4 NPs made it easy to separate, purify, and recover the NC from water. Baby et al. (2019) reported that various MWCNT-based NC materials, such as MWCNT/Fe2O3, MWCNT/ZrO2, MWCNT/Fe3O4, MWCNT/Al2O3, and MWCNT/MnO2/Fe2O3, can be utilized for the adsorption of Cr6+ ions from water. Among several adsorbents, silica has some advantages over other materials because of its high porosity, large surface area, and several functionalities (Dinker and Kulkarni, 2015). Using a one-pot technique, a PEI-silica NC was synthesized by uniformly dispersing PEI polymers on silica NPs. The adsorption capacity of the NC for the removal of Cr ions was 183.7 mg g−1, and the result showed that in addition to the adsorption of Cr6+, this NC also reduced Cr6+ to Cr3+ (Choi et al., 2018). Another silica-based adsorbent made from a glutaraldehyde cross-linked silica gel/chitosan-g-poly (butyl acrylate) was prepared using the sol–gel method and found to be very efficient in the removal of toxic chromium ions from wastewater (Nithya et al., 2016). Zeolitic imidazolate frameworks ZIF-8 and their various composites with GO and Mg(OH)2/GO were synthesized using sonochemical and hydrothermal methods. Among these, ZIF-8/NH2/Mg(OH)2/GO composites have been demonstrated to be excellent adsorbents for Cr6+ removal (Begum et al., 2020). A halloysite–bentonite clay/magnetite NC was synthesized using ball milling and calcination and was investigated for the removal of chromium. At optimum conditions, with an adsorbent dosage of 0.5 g, and a contact time of 60 min, the maximum adsorption capacity was found to be 199 mg g−1 (Masindi et al., 2021). For the removal of Cr6+ ions from tannery effluent, Fe3O4@polyaniline/itaconic acid (Fe3O4@PANI/IA) NCs are a potential adsorbent material. The findings demonstrated that the PANI with IA nanocomposite’s percentage of Cr6+ elimination was increased (Parthiban et al., 2020). Singh et al. (2022) developed chitosan/polyvinyl alcohol (CS/PVA), PEI, and Fe3O4 impregnated beads for wastewater treatment that simultaneously removed hazardous azo dyes and Cr6+.
3.5 Cobalt removal
Co is an essential element for all organisms as it is a constituent of vitamin B12. However, overexposure to Co2+ may lead to several clinical syndromes, including neurological, cardiovascular, and endocrine deficits (Leyssens et al., 2017). It may also lead to genetic disorders and cell mutations in humans (Khoo and Esmaeili, 2018).
A novel adsorbent made up of bentonite embedded in a polyacrylamide gel acted as a good adsorbent for the removal of Co2+ from an aqueous solution. The rate of sorption was optimal at pH 7 (Zhao et al., 2010). Kang et al. (2016) reported a magnetic cobalt ion-imprinted polymer prepared by precipitation polymerization using 1-vinylimidazole as the adsorbent. This NC was reusable and stable and could remove Co2+ with an efficiency of 96%. Tizro and baseri (2017) prepared a magnetite–clinoptilolite nanoadsorbent using the co-precipitation method to remove Co2+ from wastewater. Moreno-Sader et al. (2019) prepared an NC comprising polyacrylamide and sodium montmorillonite (PAM/Na-MMT) by free-radical crosslinking polymerization and used it for Co2+ adsorption from an aqueous solution. Earlier CNTs had limited application as an adsorbent of heavy metal ions because of their intense agglomeration property. However, Hayati et al. (2016) prepared a poly-amidoamine dendrimer (PAMAM)-functionalized CNT. This PAMAM/CNT NC had a high absorption capacity for several heavy metals, including CO2+. A biosorbent made up of phosphorylated MWCNT-cyclodextrin/silver-doped titanium could remove Co2+ from the mine effluent sample (Taka et al., 2018). Mohammadnezhad and Mohammadnezhad (2020) reported the TiO2/poly (acrylamide–styrene sodium sulfonate) NC prepared by the in situ intercalative polymerization of polyacrylamide and styrene sodium sulfonate in the presence of TiO2 NPs as an inorganic filler. Although this NC could adsorb Co2+ ions from aqueous solutions, its efficiency was dependent on the concentration of TiO2 in the NC. Magnetite NPs were coated with tannic acid; Bagtash et al. (2016) synthesized a magnetite–tannic acid composite that proved to be a viable sorbent for the pre-concentration of Co2+. Similarly, a CaO-magnetic NC was found to be a low-cost adsorbent for the removal of CO2+ from wastewater (Khoo and Esmaeili, 2018). The magnetic chitosan/activated carbon bionanocomposite (MCS/AC) was modified with UiO-66 MOFs, and the resulting bionanocomposite was used as an effective adsorbent for the simultaneous removal of Co2+ ions, MG dye, and imidacloprid (IMI) pesticide from an aqueous solution with ultrasound assistance. For Co2+, MG, and IMI, the maximal adsorption capacity values were 44.5 mg g−1, 62.1 mg g−1, and 25.2 mg g−1, respectively (Motaghi et al., 2022).
Poly-ortho aminophenol and glycerol (P(oAP)/G), a novel NC, has garnered significant interest in this field because of the special characteristics of polymer-based composites and has been investigated for the removal of Co ions from wastewater. P(oAP)/G efficiency for the removal of Co2+ ions was 96%, and the adsorption capacity was 117.9 mg g−1, indicating a better capacity for the treatment of water (Abdelfatah et al., 2022). To remove Ni2+ and Co2+ ions from synthetic and industrial effluents, a nanocomposite ceramic containing clay, bovine bone nanopowder, and human hair was invented. From industrial wastewater, the Ni2+ and Co2+ adsorption efficiency was observed to be greater than 95%. Up to 60 cycles of renewal and reuse were possible for the adsorbents (Abdollahi et al., 2022). One-dimensional palygorskite (Pal) and two-dimensional material GO were crosslinked to produce NCs of GO-modified palygorskite (mPal-GO), which was used to remove Co2+ from wastewater. At pH 6.0 and T 298 K, the maximum adsorption capacity was achieved at 16.9 mg g−1 (Mou et al., 2021).
3.6 Nickel removal
Ni2+ is a toxic metal ion abundant in industrial effluents. The permissible limit of the presence of Ni2+ ions in potable water is 0.02 mg L−1 (Table 1). The presence of excess Ni2+ in water causes several diseases in human beings, such as anemia, diarrhea, encephalopathy, hepatitis, lung and kidney damage, gastrointestinal distress, pulmonary fibrosis, renal edema, skin dermatitis, and central nervous system dysfunction (Zhang and Wang, 2015). Various carbon-based nanomaterials, such as activated carbon, CNTs, and GO, have the potential to remove Ni2+ contaminants from wastewater. An Fe3O4/GO/chitosan NC (FGC) was prepared using the co-precipitation method for the effective removal of Ni2+ ions from wastewater via the adsorption process. Because of the presence of magnetic NPs, the FGC adsorbent could be recovered by an external magnet (Tran et al., 2019). The magnetic recovery of Ni2+-loaded FGC is shown in Figure 4. The NC of magnetic hydroxyapatite was synthesized by adding Fe3O4 NPs to hydroxyapatite nanorods at pH 11. This NC proved to be a good adsorbent for Ni2+ ions, and the fully adsorbed NC can easily be separated from aqueous media using an external magnetic field (Thanh et al., 2018).
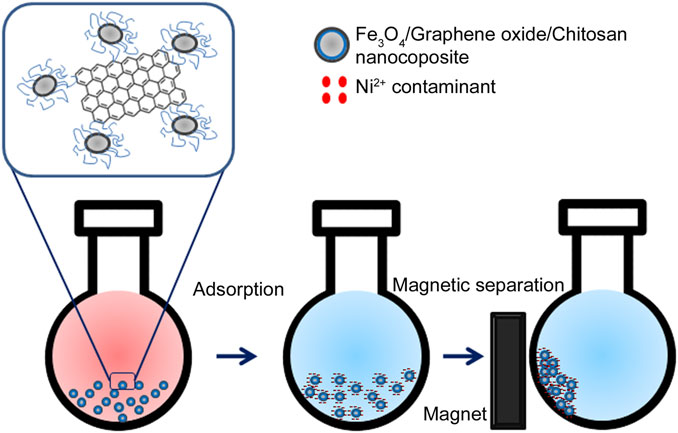
FIGURE 4. Fe3O4/graphene oxide/chitosan nanocomposite can adsorb Ni2+ from wastewater. Ni2+-loaded FGC adsorbent can easily be recovered by an external magnet.
A novel and inexpensive lignocellulose/montmorillonite (LNC/MMT) NC was prepared via a chemical intercalation of LNC with MMT and tested for the removal of Ni2+ ions via the adsorption and desorption processes in an aqueous solution. The highest adsorption capacity of the LNC/MMT NC was 94.86 mg g−1 when the initial Ni2+ concentration was 0.0032 mol L−1, at pH 6.8 and at adsorption temperature and time of 70 °C and 40 min, respectively. This NC acted as a potential adsorbent in the detoxification of Ni2+ from contaminated wastewater (Zhang and Wang, 2015). The PAM/Na-MMT NC prepared by Moreno-Sader et al. (2019) could also be used for the adsorption of Ni2+ ions from wastewater effluents. To remove Ni2+ and Co2+ ions from synthetic and industrial effluents, respectively, a nanocomposite ceramic containing clay, bovine bone nanopowder, and human hair was invented. From industrial wastewater, the Ni2+ and Co2+ adsorption efficiency was observed to be greater than 95%. Up to 60 cycles of renewal and reuse were possible for the adsorbents (Abdollahi et al., 2022). One-dimensional Pal and two-dimensional GO were crosslinked to produce an NC of mPal-GO, which was used to remove Co2+ from wastewater. At pH 6.0 and T 298 K, the maximum adsorption capacity was achieved at 16.9 mg g−1 (Mou et al., 2021).
For the first time, a new class of multifunctional material identified as PANI/NiO/MnO2 NC was developed by chemically polymerizing an aniline monomer in an acidic aqueous solution with metal oxides (NiO and MnO2), an oxidant (ammonium persulfate), and methyl orange (MO) dye. According to the results, PANI NCs doped with MnO2 and NiO NPs showed an adsorption efficiency of 97% compared to 53% for pure PANI. The PANI/NiO/MnO2 NC’s experimental adsorption capacity (248.4 mg g−1) was significantly greater than that of PANI (57.5 mg g−1) (Ali et al., 2021).
A new ZIF-8@SnO2@CoFe2O4 nanostructure was generated by the hydrothermal route, and its efficacy for Ni2+ sorption and microbial disinfection was tested. The results showed that ZIF8@SnO2@CoFe2O4 exhibited excellent performance for Ni2+ adsorption from an aqueous medium, such that mixing 40 mg of adsorbent with 50 mg L−1 of pollutant for 60 min at a pH of 6 is possible. The highest possible adsorption capacity, 21.43 mg g−1, was also attained (Roudbari et al., 2021). A polypyrrole-polyethyleneimine (PPy-PEI) polymer nanocomposite was prepared via the coupling of polyethyleneimine with polypyrrole and later tested for the elimination of nickel ions from the wastewater. In comparison with the PPy-PEI nanocomposite, which had a substantially greater nickel ion adsorption efficiency of 99.8%, pure polypyrrole was found to have 42% adsorption efficiency (Birniwa et al., 2021). Rajivgandhi et al. (2021) employed GO and reduced rGO NCs to investigate the removal of Ni ions from the electroplating effluent. For GO and rGO, the highest sorption effectiveness was determined to be 90.8% and 84.4%, respectively, at optimal pH 8, contact duration 180–1,440 m, RPM 250–300, and adsorbent dosage 0.2 mg L−1.
3.7 Arsenic (As) removal
As is a naturally occurring metalloid present in rocks, soil, water, air, plants, and animals. Volcanic eruptions, forest fires, and erosion of rocks are some natural causes of As release in the environment; mining, application of pesticides and herbicides, and disposal of waste containing As are the anthropogenic sources of arsenic (Nicomel et al., 2015). As can exist in four oxidation states: arsenite (As3+), arsenate (As5+), arsenic (As0), and arsine (As3-). Among these four As species, arsenite and arsenate are most commonly found in water, and these are, unfortunately, the most toxic forms of As (Pous et al., 2015). Drinking As-contaminated water can cause several disorders, such as cancer, melanosis, hyperkeratosis, restrictive lung disease, gangrene, hypertension, type 2 diabetes, and peripheral vascular disease (Ashraf et al., 2019).
Among various metal- and metal oxide-based NCs, TiO2-based NCs showed an excellent affinity for the removal of both arsenite and arsenate from water (Ashraf et al., 2019). A mesoporous TiO2/Fe2O3 bifunctional composite was synthesized by infusing Fe3+ into mesoporous TiO2. This bifunctional NC could not only convert more toxic As3+ into less toxic As5+ species but also adsorbed As5+ on its surface (Zhou et al., 2008). Similarly, a Ce/TiO2 nanoadsorbent could efficiently remove As5+ (Deng et al., 2010). Interestingly, Zr-doped TiO2 could adsorb both As3+ and As5+ from water (Andjelkovic et al., 2016). Another NC made up of nano-Ti loaded basic yttrium carbonate (BYC) showed an enhanced capacity for arsenate removal from water.
It could remove As5+ in a wide pH range and was a better adsorbent than BYC alone because of enhanced surface area and surface charge (Lee et al., 2015). Iron-doped TiO2 NPs also showed higher arsenic removal capability compared to nano TiO2 alone (Yang et al., 2019). Polyaniline is one of the most popular polymers used in water purification. The polyaniline hollow microsphere/Fe3O4 magnetic NC, synthesized by Dutta et al. (2020), could adsorb both arsenate and arsenite from water. Hao et al. (2018) reported the use of several iron NCs, such as iron-doped activated carbon, iron-doped polymer/biomass materials, iron-doped inorganic minerals, and iron-containing combined metal oxides, for the removal of As from wastewater. Su et al. (2017a) prepared an iron oxide/GO mesoporous NC synthesized by the co-precipitation of iron oxide on GO sheets. The amorphous morphology endowed the NC with a high surface area, which ultimately increased adsorption sites and enhanced arsenic adsorption capability. Another iron-based porous NC where the iron oxide nanoparticle was loaded onto the carbon nanosphere matrix had excellent adsorption capacity for both As3+ and As5+ (Su et al., 2017b). Singh et al. (2020) made biochar from rice and wheat husk by pyrolysis and then converted to iron oxide rice husk biochar by the co-pyrolysis of FeCl3 and the biochar. This nanohybrid adsorbs both As3+ and As5+ over a broad pH range. Saif et al. (2019) investigated the arsenate adsorption potential of polymer NCs of iron oxide. Polymers such as chitosan and polyvinyl alcohol (PVA) alginate were used to make the iron oxide/polymer NC. The iron oxide/PVA alginate NC was found to be a superior adsorbent material for As5+ from an aqueous medium compared to its other chitosan counterpart. Ansari et al. (2017) showed that CeO2/Fe2O3 NC can effectively remove As3+ from water.
Chowdhury et al. (2018) synthesized MOF/GO NCs using an aluminum-based MOF MIL-53(Al) (AlC8H5O5) and found it to be highly promising in the removal of arsenite from an aqueous solution. An MWCNT-loaded carbon foam was used for the removal of As5+ and was found to be more efficient than similar materials in this regard (Agrawal et al., 2018). The goethite/silica NC adsorbent showed high efficiency in removing arsenic from wastewater effluents (Attinti et al., 2015). The NC-functionalized membrane made up of surface-functionalized mesoporous silica NPs (MCM-NH2 or MCM-PEI) crosslinked to a modified polyacrylonitrile nanofibrous substrate and showed far superior efficacy in removing As5+ than the NC in a colloidal form. Modifying the NC membrane surface with Fe3+ could result in the membrane being more efficient in arsenic removal (Yohai et al., 2019). The nanocomposite material (ZnO–CuO/g–C3N4) has been synthesized using the solution approach for an efficient extraction of As3+. As3+ is absorbed from water on NC surfaces because of their large surface area and abundance of hydroxyl groups. At a medium pH of 3, As3+ had a maximum adsorption capacity of 98% (Khan et al., 2022). To eliminate the arsenate ion (AsO4-3) from water, CuO/TiO2 NCs were manufactured using the precipitation–deposition method, and this NC demonstrated a higher adsorption capacity for As5+ (Farooq et al., 2022).
3.8 Copper removal
The major sources of Cu contamination are mining and metallurgy, paints and dyes, electroplating, explosives, fertilizers and pesticides, microelectronics industry effluents, etc. Although Cu is an essential element, a higher dosage of Cu in wastewater causes several health issues in humans, such as liver and kidney damage, gastrointestinal problems, and diarrhea (Varma and Mishra, 2018). Even at relatively low concentrations, this heavy metal is an inhibitor of microbial activity (Ochoa-Herrera et al., 2011).
A mixture of different types of CNTs and graphene was synthesized, and its composite showed higher efficiency for the removal of Cu2+ via the adsorption process from wastewater. The maximum adsorption capacity of the CNT/GO NC was found to be higher than 250 mg g−1 (Dichiara et al., 2015). Magnetically aligned NCs were synthesized and tested for the removal of Cu ions. NCs were synthesized by embedding silica gel with co-precipitated nanomagnetite particles of Fe3O4. A magnetically aligned gel and a non-aligned gel had the potential for Cu2+ ion removal from wastewater. The efficiency of magnetically aligned NCs was found to be 34.4% with the highest adsorption capacity at 23 mg g−1 (Lo et al., 2018). Polystyrene-Schiff base (PS/SB) NCs were synthesized by encapsulating Schiff base molecules in sulfonate groups containing charged PS beads. PS/SB NCs have the potential to remove Cu2+ ions from wastewater (Zhou et al., 2018).
A novel and efficient NC polypyrrole/alumina-iron oxide was prepared by Lissy et al. (2019), and the NC showed 100% removal of Cu2+ from wastewater at pH 8. The magnetite/carbon NC synthesized by Andelescu et al. (2018) also proved to be an efficient adsorbent of Cu2+ ions. The GO/chitosan aerogel microspheres with honeycomb cobweb could efficiently remove Cu2+ contaminants from wastewater (Yu et al., 2017). Several other GO-based NCs, such as chitosan/GO, EDTA-functionalized chitosan GO, and 2,2′-dipyridylamine-modified GO, were also found to be suitable for the removal of Cu2+ contaminants from wastewater (Abu-Nada et al., 2020). A novel GPN membrane was examined for the removal of heavy metal ions. The removal efficiencies of heavy metal elimination in descending order of Pb2+, Al3+, Cu2+, and Fe2+ were 94%, 67%, 63%, and 43%, respectively. To eliminate both inorganic pollutants from wastewater, such as mercury (Hg2+) and copper (Cu2+), and organic pollutants, such as MB and CV, an EDTA-functionalized graphene oxide–chitosan nanocomposite (GO-EDTA-CS) was developed. For Hg2+, Cu2+, MB, and CV, the maximum adsorption efficiencies of the adsorbent were determined to be 324 ± 3.30, 130 ± 2.80, 141 ± 6.60, and 121 ± 3.50 mg g−1, respectively. The presence of a few active functional groups on the adsorbent, such as -COOH, -OH, and -NH2, was considered to be the cause of the outstanding adsorption capacity (Verma et al., 2022). Studies have been conducted on how well the nanocomposite of alumina and iron oxide behaves as an adsorbent for eliminating copper. An observation of 98% elimination and optimal adsorption on the uptake of copper ions was made (Lissy et al., 2019). A graphene oxide/chitosan (GO/CS) nanocomposite was produced using a simple method and used in batch tests to remove copper ions from industrial effluents. The maximum removal percentage was 99.4% with an initial copper ion concentration of 5 × 10−2 mol L-1, pH of 6, contact time of 75 min, temperature of 25 °C, and adsorbent dosage of 0.1 g/L (Kadhim and saleh, 2022).
3.9 Fluoride removal
Fluoride contamination in the environment is caused by uncontrolled discharge from industries, such as fertilizers, refining metals, rubber manufacturing, glass and ceramic production, and other sources, such as weathering and leaching of rocks and volcanic activities (Liu et al., 2016). According to the WHO, the permissible limit of F that may be present in drinking water is 1.5 mgL-1. At low concentrations, fluoride provides an antioxidant defense against tooth decay; however, excessive fluoride intake can result in dental and skeletal fluorosis, infertility, and neurological disorders (Kusrini et al., 2015; Chigonda et al., 2018).
Various NCs are currently being investigated as potential materials for the removal of fluoride ions from wastewater. A titanium-grafted β-cyclodextrin nanocomposite was synthesized via a nucleophilic reaction to remove fluoride ions from wastewater using a batch technique. It exhibited a great removal capacity, at 99.88% at an optimum pH of 5, at room temperature, and with a contact time of 40 min. An overall positive charge present on the surface of this nanocomposite resulted in an increase in the chemisorption of ionic charge between positive charge and negative charges present on fluoride ions (Fallah et al., 2020). Mondal and Purkait (2019) synthesized a green iron–aluminum nanocomposite and tested for its ability to remove fluoride ions from contaminated water. The highest adsorption capacity for fluoride removal was 42.95 mg g−1 for 0.25 g L−1 adsorbent dosage. The mechanism of fluoride adsorption was an ion exchange process due to the presence of hydroxyl groups on the surface. Another group made a chitosan-based magnetic nanocomposite and tested it for the removal of fluoride ions from wastewater. Its maximum adsorption capacity was found to be 15.38 mg g−1 at optimum pH 5 and temperature 318 K. Some modifications have been made to chitosan to make it a better adsorbent by its modification using chloroacylchloride and 2-(2-aminoethylamino)ethanol. Electrostatic and hydrogen bonding are the main elements for the sorption of fluoride (Abri et al., 2019). An exceptional nanocomposite (Fe3O4@LDH/poly) nanocomposite adsorbent for defluoridation from drinking water was synthesized using magnetic iron oxide nanoparticles and Zn–Ce double-layer hydroxide/cellulose. The ion exchange mechanism is the basic mechanism through which the removal of fluoride ions occurs. The (Fe3O4@LDH/poly) NC exhibited many positively charged ions, such as Zn2+, Fe2+, Fe3+, and Ce3+, and formed complexes with negatively charged fluoride ions through electrostatic interactions. It showed the highest adsorption capacity of 167.63 mg g−1 at optimum conditions, namely, pH 7, temperature 300 K, adsorbent dosage 20 mg, and contact time 40 min (Ammavasi and Mariappan, 2018). Using a simple co-precipitation deposition technique on pre-synthesized polyaniline nanofibers (PANI NFs), hydrous CeO2–Fe3O4 (HCeFe)-decorated polyaniline nanofibers (HCeFe NFs) were generated. Then, these NFs were assessed as adsorbents for the removal of fluoride from both synthetic and actual water samples. It showed a maximum adsorption capacity of 116.28 mg g−1 via electrostatic interaction and the ion exchange mechanism. Optimum conditions for maximum defluoridation were pH 3, temperature 25οC, and dose 0.03 g (Chigonda et al., 2018). A graphene oxide/alumina NC was synthesized using a hydrothermal method and was tested for the removal of fluoride ions. It showed a maximum adsorption capacity of 4.68 mg g−1 for fluoride ions (Xu et al., 2020). Chitosan-decorated iron nanoparticles were synthesized and tested for the removal of fluoride ions from wastewater. Due to their presence in surface charge, they act as an excellent defluoridating agent (Mohammadi et al., 2019). Mohseni-bandpi et al. (2015) reported a novel magnetite–chitosan composite for defluoridation. It showed a maximum adsorption capacity of 9.43 mg g−1 at optimum conditions, namely, pH 7, temperature 500οC, adsorbent dosage 0.5 g L−1, and contact time 60 min. It was an excellent adsorbent because it can be separated quickly and easily because of its magnetic properties. Sahoo and Hota (2018) effectively created a new magnetic MgO–MgFe2O4 nanocomposite, which was anchored onto a GO substrate (MgO–MgFe2O4/GO) and a MgO–Fe2O3 nanocomposite through hydrothermal techniques. These GO-based nanocomposites were used as effective adsorbents to remove fluoride ions from an aqueous solution. The maximum adsorption capacity was found to be 34 mg g−1 at pH 6, temperature 30°C, contact time 60 min, and adsorbent dose 0.05 g/100 mL.
3.10 Uranium removal
The US Environmental Protection Agency (USEPA) has identified uranium, an essential byproduct of the nuclear industry, as a hazardous and radioactive heavy metal. Uranium is accessible due to a variety of sources, including natural deposits, mine tailings, combustion byproducts, nuclear industry emissions, and corrosion of uranium and phosphate fertilizers containing uranium (Su et al., 2017). The WHO has set the provisional guideline value for uranium in drinking water at 30 µgL−1. Uranium toxicity causes damage to the kidney and affects other organs such as the bones, liver, and reproductive system. High exposure to radiation may lead to various kinds of cancer, such as lung, bone, and liver cancer (Bhalara et al., 2014).
Many NCs are now being investigated for their potential to remove uranium ions from wastewater. A simple microwave-assisted method was used for the synthesis of magnetically modified hydroxyapatite nanoparticles and was tested for the removal of uranium (VI) ions from wastewater (Zheng et al., 2020). It was found that the maximum adsorption efficiency was 310 mg g−1, at pH 5, with an equilibrium time of 120 min, and at a temperature of 25°C. The mechanism of adsorption was via complex formation between the phosphate group of the adsorbent and U(VI) ions (El-Maghrabiet et al., 2019). An excellent reusable nanocomposite was synthesized by conjugating BR with hydroxyapatite (HAP) and tested for the removal of uranium [U(VI)] from an aqueous solution. The maximum adsorption capacity of BR/HAP NC was found to be 428.25 mg g−1, while BR alone showed a max. adsorption capacity of 110.56 mg g−1. Optimum conditions for the adsorption were pH 5.5, temperature 298 K, and contact time 120 min. The primary mechanism of adsorption was the ion exchange mechanism between -OH and -COOH groups present on the surface of the adsorbent and UO22+ (Ahmed et al., 2021). An Fe3O4@TiO2 core shell magnetic composite was synthesized and investigated for the removal of uranium ions from water. It was acting as a fast and efficient sorbent with a maximum adsorption capacity of 118.8 mg g−1 at pH 6, temperature 25°C, and shaking time 240 min. A potent and promising sorbent for the effective extraction of uranium (VI) from aqueous solutions was the Fe3O4@TiO2 nanocomposite (Tan et al., 2015). Li et al. (2019) reported a novel low-cost and eco-friendly composite, the magnetic biochar composite, and investigated this composite for the removal of uranium ions from wastewater. Due to the porous structure and large surface area of the composite, it was acting as an excellent wastewater remediating agent. The highest adsorption capacity was found to be 52.63 mg g−1 at pH 4, temperature 318 K, and contact time 2–14 days. The β-cyclodextrin magnetic bentonite (βCD-FB) nanocomposite, a novel low-cost adsorbent, was synthesized and tested for the removal of uranium ions from wastewater. The maximum adsorption capacity was recorded as 305 mg g−1 in just 60 min at room temperature, and the adsorption efficiency was 61%. The low-cost nanoadsorbent that has been designed has a high degree of sustainability and holds potential for use in the industrial treatment of wastewater that contains radioactive materials that are harmful (Zahran et al., 2019). Nanocomposite-mediated heavy metal remediation strategies are summarized in Supplementary Table S1.
4 Fungi-based bionanocomposite-mediated removal of heavy metal ions from wastewater
Among other different strategies, many researchers are also adopting biosorption strategies for wastewater management, specifically for heavy metal removal. Although some microorganisms, such as bacteria, algae, and fungi, have been used in such biosorption processes, fungi are considered the best possible contenders because they are simple to handle, are mostly tolerant of heavy metals at low pH, produce a significant amount of biomass, and can be separated from aqueous solutions using simple techniques because of their hyphal network, and genetic and morphological manipulations in fungi can be performed very easily (Deshmukh et al., 2016; Lotlikar et al., 2018). To optimally use fungi for the biosorption of toxic materials from wastewater, a suitable matrix is required to immobilize the fungi. Two decades ago, various inorganic matrices were used for the immobilization of fungal biomass such as Ca-alginate beads for the white rot fungus Trametes versicolor and Phanerochaete chrysosporium, polyurethane for Aspergillus terrus, and polyethylene amine (PEI) for Penicillium chrysogenum (Arica et al., 2001; Dias et al., 2002; Kacara et al., 2002; Deng and Ting, 2005). The composites of fungi and nanomaterials can become an attractive choice for industrial wastewater management, and there is not much information on the long-term practical applications of merging nanotechnology with existing fungal-based biosorption strategies. The nanomaterials can not only provide an immobilization platform but also have a huge potential to show a synergistic effect on toxic material management. A suitable microorganism for immobilization should also be chosen through screening examinations, and the feasibility of scaling up these bionanocomposites must be considered. It should be noted that only fungi that can form hyphae or pellets are used in the synthesis of fungal-based bionanocomposites, as fungal morphology is important in the biosorption process (Xu et al., 2012).
Polyaromatic hydrocarbons (PAHs) are released from various human activities, such as the combustion of fossil fuel, petroleum leakage, and burning of waste, as well as from several natural activities, such as volcanic activity and the synthesis of microbial vegetation (Shakya and Esmaeili, 2018). The removal of PAHs is very important because they have carcinogenic and mutagenic effects on organisms and cause bioaccumulation at various levels in the food chain. A novel approach to assembling inorganic NPs with fungal mycelia was developed to enhance the adsorption and degradation of PAHs. Zhou et al. (2021) reported the coupling of CNTs with Penicillium oxalicum SYJ-1 fungal mycelium, which resulted in overall PAH degradation in adsorption by CNTs and biodegradation by fungal mycelium. Assembling CNTs with functional fungal mycelia may be an alternative to avoid the drawbacks of only adsorption and biodegradation by swiftly adsorbing PAHs onto CNTs to lower the aqueous PAH content and adsorb PAHs to enhance their bioavailability to fungi, thus accelerating PAH breakdown. CNTs alone had a capacity of approximately 80% for the removal of pyrene, and this fungal mycelium also exhibited a removal capacity of 72% in 72 h. The pyrene removal efficacy for the composites and mycelia was estimated to be 1.98 and 0.44 mg g−1 dry weight h−1 during the first 48 h, respectively, and to be 0.52 and 0.29 mg g− 1 dry weight h−1 at the end of 72 h, respectively. The composite of CNTs and Penicillium oxalicum SYJ-1 fungal mycelium promoted the efficiency of pyrene removal at 20 mgL-1 within 48 h. Conventionally, using a combined process of the amidation reaction, crosslinking polymerization, and the sol–gel method, an insoluble nanosponge biopolymer composite was synthesized to obtain phosphorylated multiwalled carbon nanotubes (MWCNT)-cyclodextrin/silver-doped titanium (pMWCNT-βCD/TiO2-Ag) for the removal of Pb2+ ions from wastewater effluents (Taka et al., 2018). Iron oxide NPs, a potential adsorbent, and Ca-alginate immobilized on the surface of Phanerochaete chrysosporium were used by Xu et al. (2012) to remove Pb2+ ions from polluted water, and the reusability of this bionanocomposite was monitored. The adsorption capacity was 176.33 mg g−1 at pH 5 and temperature 35 °C, and adsorption equilibrium was attained within 8 h. PVA and PVA/MnCl2 nanofibers immobilized on the surface of Mn2+-oxidizing fungi, Coprinellus sp. and Coniothyrium sp., were used for the adsorption of Mn2+, Cu2+, and Pb2+. Compared to the Mn oxide synthesized, biogenic Mn(III/IV)O has superior adsorption qualities. Due to the extensive amount of vacancies in Mn(III/IV)O, the compound is very adsorptive. With the goal of removing heavy metals from water, hybrids of Mn(III/IV)O-producing fungi and nanofibers have been suggested as environmentally friendly approaches. This innovative method immobilizes biosorbents on the surface of nanofibers. The outcomes of this cutting-edge strategy support the scalable application of nanotechnologies for the remediation of groundwater. Mn (III/IV)-O coated nanofibers and the Coniothyrium sp.-nanofiber composite were potentially effective not only in the removal of Mn2+ ions but also in enhancing the adsorption efficiency, leading to >90% removal of Mn2+ ions (Park et al., 2020).
Four fluoride-resistant fungal strains were gathered from the fluoride-contaminated area, Pencillium camemberti SIT CH-1, Aspergillus ficcum SIT CH-2, Aspergillus terreus SIT CH-3, and Aspergillus flavipes SIT CH-4, and a nanobiocomposite was developed via zirconium doping on these four biosorbent fungal strains. This novel and eco-friendly nanobiocomposite was very efficient and inexpensive for the removal of fluoride ions from groundwater not only at higher concentrations but also at low concentrations via adsorption, electrostatic attraction, ion exchange, etc. Greater sorption uptake, greater adsorption, or higher mechanical stability could arise from physical alterations of fungal biomass. Therefore, fungi were bound to tetravalent zirconium and used as an adsorbent for fluoride removal. Fungi are recognized to have strong heavy metal sorption ability. It was expected that fluoride removal occurred due to chemisorption, in which fluoride ions created covalent bonds with the surface of the biosorbent by electron sharing or exchange. The adsorption efficiencies of Pencillium camemberti SIT CH-1 + zirconium, Aspergillus ficcum SIT CH-2 + zirconium, Aspergillus terreus SIT CH-3 + zirconium, and Aspergillus flavipes SIT CH-4 + zirconium were 94.9%, 95.98%, 95.2%, and 96.35%, respectively (Hiremath and Theodore, 2016).
Discharges from industries, such as textiles, leather, paper, plastics, food, and rubber, are continuously polluting our water bodies with toxic dyes (Ashrafi et al., 2017). The amount of dye may vary from 10 to 200 mg L−1 in contaminated water (Muntean et al., 2018). Since many of the chemicals used in these dyes are poisonous to both aquatic animals and human health, it is a matter of paramount concern to remove these dyes from contaminated water bodies (Kandisa and Saibaba, 2016). In addition to conventional methods for the removal of dyes, the potential of the fungal hyphae/carbon nanotube (FH/CNT) composite prepared by embedding CNTs on the surface of fungal hyphae (Xylaria sp.) was investigated for the removal of anionic (Congo red) and cationic (methylene violet) dyes from polluted water. The maximum adsorption efficiencies of fungal hyphae alone for Congo red (CR) and methylene violet (MV) were 27.72 and 13.61 mg g−1, respectively, but due to the fixation of CNTs onto fungal hyphae, these adsorption capacities were observed to be enhanced to 43.99 and 20.89 mg g−1, respectively. The maximum absorption capacities were attained at pH 7 and at 30 °C, and the equilibrium reaction time was 120–160 min (Zhu et al., 2018).
Zhu et al. (2016) synthesized fungal hyphae/carbon nanotube (FH/CNT) composite spheres for the treatment of polluted water via the removal of heavy metal ions and dyes present inside it. The presence of O-containing groups on functionalized CNTs leads to an increase in their adsorption capacities for various pollutants. Hence, these CNTs were coupled with microbes such as FH to make them a more versatile and robust adsorbent. They used FH/CNT spheres for the removal of malachite green and Cu2+ ions from contaminated water. The maximum adsorption capacities for Cu2+ and malachite green were 154 and 50.2 mg g− 1, respectively. Pure FH had adsorption efficiencies of 35% and 42% for Cu2+ and malachite green, respectively, and this was obviously lesser than that of the composite. Lain et al. (2017) also prepared a novel three-layered sphere FH/N-TiO2/NG (fungal hyphae/N-doped titanium dioxide/N-doped graphene), with fungal hyphae as the innermost layer, fungal hyphae/Fe3O4 as the middle layer, and fungal hyphae/N-TiO2/NG as the outermost layer, and its adsorption and photocatalytic activity were tested on tannin removal. The addition of nano ferric oxide (Fe3O4) to FMT (fungal hyphae/Fe3O4/N-doped TiO2 and graphene) made it easy to recycle without any pollution. The following order of adsorption capacities was observed: Fe3O4 < N-TiO2/NG < FMT < FH. Polysaccharides, proteins, and phosphate groups present on the fungal cell wall interacted with tannin and made it an excellent tannin adsorbent. N-doping in NTG (N-doped TiO2 and graphene) was also responsible for tannin adsorption. After the adsorption of tannin on the surface of FMT, sunlight led to the breakdown of tannin by photocatalysis. FH-magnetic NPs can also be a promising tool for the elimination of Cu2+ from an aqueous solution. Saccharomyces cerevisiae immobilized on the surface of chitosan-coated magnetic NPs (CCM) were used. The high hydrophilicity of CCM due to the substantial number of -OH groups in glucose units and the presence of numerous functional groups, such as acetamido, primary amino, and hydroxyl groups, enabled it to absorb heavy metal ions in wastewater treatment, and these groups had high chemical reactivity, and the polymer chain’s versatility also contributed to the material’s excellent adsorption properties for heavy metals. At optimum pH 4.5, a maximum removal efficiency of 96.8% was attained, and the adsorption capacity increased as the initial Cu2+ concentration increased. Adsorption equilibrium could be obtained in 1 h using CCM, which was particularly effective for the quick adsorption of Cu2+ within the first 10 min. The maximum adsorption capacity of Saccharomyces-CCM for Cu2+ was found to be 144.9 mg g−1, which was considerably greater than that of many magnetic nanocomposites (Peng et al., 2010). A general scheme for the bionanocomposite-based wastewater strategy is shown in Figure 5. A bionanocomposite prepared by immobilizing alginate beads laden with the filamentous fungus Stereum hirsutum inside nanoporous silica hydrogels was found to be useful for the bioremediation of contaminated water. The fundamental premise behind this was that silica-alginate matrices exhibited good mechanical and chemical stability to produce simple-to-handle operative units with minimal ecological danger, slow passage of contaminants to microorganisms, preventing intoxication under high levels of pollutant, and leakage decreased the losses of enzymes involved in the dissolution of contaminants. Dye adsorption, managed transport, and the preservation of dye breakdown enzymes inside the hydrogel were the main challenges. Porosity was vital for the efficient interaction of dye-degrading enzymes. Three reference conditions were taken, and the embedded mycelium can be either an active reactor (AR) or a passive reactor (PR) depending on whether it is in an active form or inactivated by autoclaving. Bare support (BS) is a ca-alginate capsule containing active mycelium. After 20 h of contact with the dye, AR, PR, and BS eliminated 80%, 65%, and 35% of MG, respectively. The production of ligninolytic enzymes by S. hirsutum, their retention in the immediate vicinity of the fungus to increase the possibility of the dye–enzyme contact, and the fine supply of MG to the fungi contributed to the remarkable efficacy of AR (Perullini et al., 2010).
Titanium dioxide NPs (TiO2 NPs) were loaded with filamentous fungus from an oil plant biomass and utilized as a sorbent for Pb2+ enrichment and separation in on-line-flow injection. At pH 4.0, Pb2+ ions were adsorbed on a biosorbent mini-column and then removed using 288 µL of a 1.0-mol L−1 HCl solution. The detection threshold was 0.78 μg L−1 (Bakircioglu et al., 2010). Phanerochaete chrysosporium pellets immobilized with elemental selenium NPs (nSe0-pellets) were used as a novel hybrid biosorbent to remove zinc from an aqueous solution. At pH 4.5 and an initial Zn content of 10 mg L−1, fungal pellets containing nSe0 (nSe0 pellets) demonstrated superior biosorbancy by eliminating more Zn (88.1 % ± 5.3%) 700 than Se-free fungal pellets (56.2% ± 2.8%). The increased sorption capacity of nSe0-pellets was attributed to an increase in the number of sorption sites, which provided a denser distribution of negatively charged surfaces. The functional groups, including -OH and -COOH groups, involved in the sorption process were identifiable in fungal pellets prior to and following the adsorption of Zn (Espinosa et al., 2016). Based on the ultrasonic cavitation-assisted oxygen implosion strategy, a new bioadsorbent agent named the nanomagnetic/carboxyl Candida albicans biomass composite (MCC) was developed, and the adsorption capacity of the toxic metal ions (Pb2+, Cd2+, and Cu2+) was investigated. At pH 4, 6, and 6.5, the best removal efficiencies for Cu2+, Cd2+, and Pb2+ were observed, respectively. For Pb2+, Cd2+, and Cu2+, the adsorption capacities were calculated to be 1,009, 80, and 202 mg g−1, respectively. In treated wastewater with varying quantities of coexisting ions, the removal rates for Pb2+, Cd2+, and Cu2+ reached 99.8%, 99.4%, and 94.7%, respectively. The MCC nanocomposite bioadsorbent agent fulfilled an increasing demand for water resource purification and can be extensively used in industrial wastewater treatment (Azzam et al., 2021).
Phanerochaete chrysosporium was successfully immobilized using iron oxide magnetic NPs (MNPs) and Ca-alginate to generate a novel biosorbent. To capture P. chrysosporium to produce an efficient biosorbent for the removal of Pb2+, iron oxide NPs blended with Ca-alginate were used as an immobilization matrix. The highest Pb2+ biosorption efficiency, which was 96.03%, was achieved at pH 5.0 and 35 °C. The uptake of metal started extremely quickly and reached equilibrium after 8 h. In a sample containing 500 mg L−1 of Pb2+, the maximum biosorption capacity reached up to 185.25 mg g−1 dry biosorbent (Xu et al., 2013). Nano-hydroxyapatites (n-HAPs) have limited ability to immobilize heavy metals in contaminated soils due to self-aggregation. Low-cost, easily available, and eco-friendly filamentous fungi could be utilized for making a bionanocomposite-based matrix to potentially overcome the problem of n-HAPs. To construct the hybrid bionanocomposite, Aspergillus niger and Penicillium hyphae were immobilized with n-HAPs, which not only solved the problems of self-aggregation but also improved the efficiency of heavy metal (Cd2+ and Pb2+) adsorption. In contrast to n-HAPs or fungal hyphae alone, the bionanocomposites demonstrated immobilization efficiencies one to four times greater for diethylenetriaminepentaacetic acid (DTPA)-extractable Cd2+ and Pb2+ in polluted soils. The Aspergillus niger-based bionanocomposite (ANHP) also outperformed the Penicillium Chrysogenum F1-based bionanocomposite (PCHP) in immobilizing Cd2+ and Pb2+ in contaminated soils. The immobilization efficiency of DTPA-extractable Cd2+ by ANHP was 84.0%, which was 2.77, 3.86, 3.89, and 7.53 times greater than that of n-HAPs, PCHP, and the two fungi hyphae, respectively. Meanwhile, the immobilization efficiencies for DTPA-extractable Pb2+ by ANHP (42.6%) and PCHP (39.7%) were comparable and greater than those by the two fungus hyphae, n-HAPs, respectively. Aspergillus niger hyphae were immobilized more effectively than Penicillium chrysogenum F1 hyphae by the previously mentioned modifications. The efficiency of heavy metal removal from the soil was in the following order: ANHP > PCHP > n-HAP. The soils treated with ANHP obtained the immobilization equilibrium of DTPA-extractable Cd2+ and Pb2+ within 7 days, with the greatest efficiencies being 83.95% and 42.55%, respectively (Yang et al., 2018). A low-cost, novel fungus–Fe3O4 bionanocomposite was synthesized and tested for the removal of radionuclides such as U(VI). Fungus in this bionanocomposite acts as a template for nano-Fe3O4 and, hence, increases the dispersity and stability of nano-Fe3O4. Oxygen-containing groups, such as alcohol, acetyl, and carboxyl, were present in this bionanocomposite, which primarily form complexes with the inner spheres of radionuclides, enhancing their sorption. The maximum sorption capacity for U (VI) was found to be 280.8 mg g−1 at pH 5 and temperature 303 K (Ding et al., 2015). FH/Fe3O4/GO-layered core shell-structured nanocomposite spheres (FFGS) were effectively created by cultivating FH in media containing either GO or Fe3O4. This three-layered core shell FFGS composite was used for the removal of U (VI) ions from wastewater. The mechanism for the removal was chemical adsorption via chemical interaction between functional groups present on the surface of the FFGS composite and U (VI) hydroxy compounds. The highest adsorption efficiency was 219.71 mg g−1 at pH 5 and temperature 293 K (Zhu et al., 2019). A novel adsorbent of the fungus/attapulgite (F/ATP) composite was synthesized hydrothermally and is a promising material for the removal of uranium from wastewater. The maximum sorption capacity was 125 mg g−1 at pH 4 and temperature 303 K. Over a period of six cycles, the F/ATP composites showed a strong sorption of U (VI) (∼91%) (Cheng et al., 2015). As an efficient material for treating nuclear wastewater, a FH/GO composite was invented. It was found that at an initial pH of 6.0 (±0.1) at 20°C, the U (VI) adsorption capacity of the FH/GO composite increased by 60% compared to biomass (FH), and its maximum U(VI) adsorption efficiency was 199.37 mg g−1 (Li et al., 2018). CNTs were attached to FH using a biological assembly technique to create a sphere FH/CNT composite, which can be employed as a flexible adsorbent for mitigating water pollution. The maximum adsorption capacity of the FH/CNT composite for uranium ions was 187.26 mg g−1 (Zhu et al., 2018).
5 Conclusion
Nanomatrix-embedded bioaccumulating/biosorbing microbes were investigated for the removal of heavy metal ions from wastewater because this bio-nanocomposite can be bioremediation by adsorption. Conventional methods used for wastewater remediation, such as chemical precipitation, ultrafiltration, reverse osmosis, ultrafiltration, and ion exchange, have several drawbacks, such as dealing with low concentrations of metal ions, the generation of high sludge, and the aggregation of metal precipitates. To overcome these bottlenecks, nanocomposites with advantages such as high efficiency, cost-effectiveness, recycling, enhanced surface area, and increased reactivity were used. Adding fungal biomass to nanocomposites made them a superior adsorbent. Bionanocomposites are wonder materials with colossal potential to clear aquatic systems from the menace of harmful contaminants. These multi-phasic materials are stable in an aqueous solution and have enormous surface area to interact with the target toxicants. Moreover, easy separation processes and reusability of the initial composite materials make them suitable for wastewater remediation. Various bionanocomposites are already being investigated for their capability to adsorb or catalytically degrade heavy metal ions and toxic dye effluent from wastewater. Although all these fungal-based bionanocomposites are promising materials for the eradication of pollution in water, they also demand a deep insight into their potential side effects on human health. A large-scale application of bionanocomposites in water remediation may deliberately increase the nanomaterial exposure, but there is no comprehensive study on their toxicity, which may drastically differ depending on the size, shape, and surface coating of the material. Hence, a fungal-based bionanocomposite-enabled wastewater decontaminating infrastructure should be set up in such a way that there is minimal nanomaterial leakage in the water bodies.
6 Scope statement
To 4 October 2023 The Editor, Frontiers in nanotechnology-nanomaterials Dear Sir, We are submitting a review article entitled “Recent advancements in nanocomposite and bionanocomposite mediated wastewater remediation with special emphasis on heavy metal removal” for your kind consideration. In this review article, we have discussed the nanocomposite mediated wastewater decontamination strategies, which mostly came up in recent years. Moreover, we have highlighted the potential of nanomatrix embedded bioaccumulating/biosorbing fungi as a new-age wonder material with superior capability in pollutant removal from wastewater. We hereby declare that the manuscript has not and will not be submitted for publication elsewhere so long as it is under consideration by “Frontiers in Nanotechnology-Nanomaterials.” We, Namita Singh, Annu Yadav, Sumistha Das, and Nitai Debnath have participated in the study and concur with the submission and subsequent revisions submitted by the corresponding author. Yours sincerely, Prof. Namita Singh, Professor, Department of Bio and Nano Technology, GJUS&T, Hisar Haryana—125001, India.
Author contributions
NS: funding acquisition, conceptualization, investigation, project administration, supervision, and writing–review and editing. AY: writing–original draft. SD: supervision and writing–review and editing. ND: writing–review and editing and funding acquisition.
Funding
The authors declare that financial support was received for the research, authorship, and/or publication of this article.
The authors would like to thank DBT-HRD, DST/INT/UK R/P-14/2015, and SAP-DRS-II and the Department of Science and Technology, Govt. of India (DST-FIST), DST (Grant Nos. YSS/2015/000152 and DST/NM/NS/2018/27), and AICTE for their financial support in the form of Fellowship.
Conflict of interest
The authors declare that the research was conducted in the absence of any commercial or financial relationships that could be construed as a potential conflict of interest.
Publisher’s note
All claims expressed in this article are solely those of the authors and do not necessarily represent those of their affiliated organizations, or those of the publisher, the editors, and the reviewers. Any product that may be evaluated in this article, or claim that may be made by its manufacturer, is not guaranteed or endorsed by the publisher.
Supplementary material
The Supplementary Material for this article can be found online at: https://www.frontiersin.org/articles/10.3389/fnano.2024.1307353/full#supplementary-material
Supplementary Figure 1 | Bionanocomposite enabled decontamination of wastewater.
References
Abdelfatah, A., Abdel-Gawad Of, , Elzanaty, A. M., Rabie, A. M., and Mohamed, F. (2022). Fabrication and optimization of poly(ortho-aminophenol) doped glycerol for efficient removal of cobalt ion from wastewater. J. Mol. Liq. 345, 117034. doi:10.1016/j.molliq.2021.117034
Abdollahi, S. A., Mokhtariyan, N., and Ameri, E. (2022). Design, synthesis and application of a sponge-like nanocomposite ceramic for the treatment of Ni(II) and Co(II) wastewater in the zinc ingot industry. Arabian J. Chem. 15 (1), 103477. doi:10.1016/j.arabjc.2021.103477
Abri, A., Tajbakhsh, M., and Sadeghi, A. (2019). Adsorption of fluoride on a chitosan-based magnetic nanocomposite: equilibrium and kinetics studies. Water Supply 19 (1), 40–51. doi:10.2166/ws.2018.050
Abu-Nada, A., McKay, G., and Abdala, A. (2020). Recent advances in applications of hybrid graphene materials for metals removal from wastewater. Nanomaterials 10 (3), 595. doi:10.3390/nano10030595
Agrawal, P. R., Singh, N., Kumari, S., and Dhakate, S. R. (2018). Multiwall carbon nanotube embedded phenolic resin-based carbon foam for the removal of as (V) from contaminated water. Mater Res. Express 5 (3), 035601. doi:10.1088/2053-1591/aaaf7c
Ahamad, T., Mu, N., Al-Maswari, B. M., Ahmed, J., Alothman, Z. A., Alshehri, S. M., et al. (2017). Synthesis of a recyclable mesoporous nanocomposite for efficient removal of toxic Hg 2+ from aqueous medium. J. Industrial Eng. Chem. 53, 268–275. doi:10.1016/j.jiec.2017.04.035
Ahmed, W., Núñez-Delgado, A., Mehmood, S., Ali, S., Qaswar, M., Shakoor, A., et al. (2021). Highly efficient uranium (VI) capture from aqueous solution by means of a hydroxyapatite-biochar nanocomposite: adsorption behavior and mechanism. Environ. Res. 201, 111518. doi:10.1016/j.envres.2021.111518
Al-Ghouti, M. A., Da’ana, D., Abu-Dieyeh, M., and Khraisheh, M. (2019). Adsorptive removal of mercury from water by adsorbents derived from date pits. Sci. Rep. 9 (1), 15327. doi:10.1038/s41598-019-51594-y
Ali, L. I. A., Ismail, H. K., Alesary, H. F., and Aboul-Enein, H. Y. (2021). A nanocomposite based on polyaniline, nickel and manganese oxides for dye removal from aqueous solutions. Int. J. Environ. Sci. Technol. 18 (7), 2031–2050. doi:10.1007/s13762-020-02961-0
Ammavasi, N., and Mariappan, R. (2018). Enhanced removal of hazardous fluoride from drinking water by using a smart material: magnetic iron oxide fabricated layered double hydroxide/cellulose composite. J. Environ. Chem. Eng. 6 (4), 5645–5654. doi:10.1016/j.jece.2018.08.071
Andelescu, A., Nistor, M. A., Muntean, S. G., and Rădulescu-Grad, M. E. (2018). Adsorption studies on copper, cadmium, and zinc ion removal from aqueous solution using magnetite/carbon nanocomposites. Sep. Sci. Technol. 53 (15), 2352–2364. doi:10.1080/01496395.2018.1457696
Andjelkovic, I., Jovic, B., Jovic, M., Markovic, M., Stankovic, D., Manojlovic, D., et al. (2016). Microwave-hydrothermal method for the synthesis of composite materials for removal of arsenic from water. Environ. Sci. Pollut. Res. 23 (1), 469–476. doi:10.1007/s11356-015-5283-z
Anjum, M., Miandad, R., Waqas, M., Gehany, F., and Barakat, M. A. (2019). Remediation of wastewater using various nano-materials. Arabian J. Chem. 12 (8), 4897–4919. doi:10.1016/j.arabjc.2016.10.004
Arıca, M. Y., Kaçar, Y., and Genç, Ö. (2001). Entrapment of white-rot fungus Trametes versicolor in Ca-alginate beads: preparation and biosorption kinetic analysis for cadmium removal from an aqueous solution. Bioresour. Technol. 80 (2), 121–129. doi:10.1016/s0960-8524(01)00084-0
Ashraf, S., Siddiqa, A., Shahida, S., and Qaisar, S. (2019). Titanium-based nanocomposite materials for arsenic removal from water: a review. Heliyon 5 (5), e01577. doi:10.1016/j.heliyon.2019.e01577
Ashrafi, M., Arab Chamjangali, M., Bagherian, G., and Goudarzi, N. (2017). Application of linear and non-linear methods for modeling removal efficiency of textile dyes from aqueous solutions using magnetic Fe 3 O 4 impregnated onto walnut shell. Spectrochimica Acta Part A Mol. Biomol. Spectrosc. 171, 268–279. doi:10.1016/j.saa.2016.07.049
Ateia, E. E., Ramadan, R., and Shafaay, A. S. (2020). Efficient treatment of lead-containing wastewater by CoFe2O4/graphene nanocomposites. Appl. Phys. A 126 (3), 222. doi:10.1007/s00339-020-3401-3
Attinti, R., Sarkar, D., Barrett, K. R., and Datta, R. (2015). Adsorption of arsenic(V) from aqueous solutions by goethite/silica nanocomposite. Int. J. Environ. Sci. Technol. 12 (12), 3905–3914. doi:10.1007/s13762-015-0902-2
Azzam, A. M., El-Wakeel, S. T., and Mostafa, B. B. (2021). Magnetic nanocomposite based on carboxyl-functionalized Candida albicans for removal of heavy metals ions from wastewater. ChemistrySelect 6 (47), 13564–13571. doi:10.1002/slct.202102852
Baby, R., Hussein, M. Z., Abdullah, A. H., and Zainal, Z. (2022). Nanomaterials for the treatment of heavy metal contaminated water. Polymers 14 (3), 583. doi:10.3390/polym14030583
Baby, R., Saifullah, B., and Hussein, M. Z. (2019). Carbon nanomaterials for the treatment of heavy metal-contaminated water and environmental remediation. Nanoscale Res. Lett. 14 (1), 341. doi:10.1186/s11671-019-3167-8
Badawy, A. A., Ghanem, A. F., Yassin, M. A., Youssef, A. M., and Abdel Rehim, M. H. (2021). Utilization and characterization of cellulose nanocrystals decorated with silver and zinc oxide nanoparticles for removal of lead ion from wastewater. Environ. Nanotechnol. Monit. Manag. 16, 100501. doi:10.1016/j.enmm.2021.100501
Bagtash, M., Yamini, Y., Tahmasebi, E., Zolgharnein, J., and Dalirnasab, Z. (2016). Magnetite nanoparticles coated with tannic acid as a viable sorbent for solid-phase extraction of Cd2+, Co2+ and Cr3+. Microchim. Acta 183 (1), 449–456. doi:10.1007/s00604-015-1667-5
Bakircioglu, Y., Bakircioglu, D., and Akman, S. (2010). Biosorption of lead by filamentous fungal biomass-loaded TiO2 nanoparticles. J. Hazard. Mater. 178 (1–3), 1015–1020. doi:10.1016/j.jhazmat.2010.02.040
Begum, J., Hussain, Z., and Noor, T. (2020). Adsorption and kinetic study of Cr(VI) on ZIF-8 based composites. Mater Res. Express 7 (1), 015083. doi:10.1088/2053-1591/ab6b66
Bernhoft, R. A. (2012). Mercury toxicity and treatment: a review of the literature. J. Environ. Public Health 2012, 1–10. doi:10.1155/2012/460508
Bhalara, P. D., Punetha, D., and Balasubramanian, K. (2014). A review of potential remediation techniques for uranium(VI) ion retrieval from contaminated aqueous environment. J. Environ. Chem. Eng. 2 (3), 1621–1634. doi:10.1016/j.jece.2014.06.007
Birniwa, A. H., Abubakar, A. S., Huq, A. K. O., and Mahmud, HNME (2021). Polypyrrole-polyethyleneimine (PPy-PEI) nanocomposite: an effective adsorbent for nickel ion adsorption from aqueous solution. J. Macromol. Sci. Part A. 58 (3), 206–217. doi:10.1080/10601325.2020.1840921
Chen, L., Zhang, K., He, J., Cai, X. G., Xu, W., and Liu, J. H. (2016). Performance and mechanism of hierarchically porous Ce–Zr oxide nanospheres encapsulated calcium alginate beads for fluoride removal from water. RSC Adv. 6 (43), 36296–36306. doi:10.1039/c6ra01337f
Cheng, Q., Wang, C., Doudrick, K., and Chan, C. K. (2015). Hexavalent chromium removal using metal oxide photocatalysts. Appl. Catal. B Environ. 176–177, 740–748. doi:10.1016/j.apcatb.2015.04.047
Cheng, W., Ding, C., Sun, Y., and Wang, X. (2015). Fabrication of fungus/attapulgite composites and their removal of U(VI) from aqueous solution. Chem. Eng. J. 269, 1–8. doi:10.1016/j.cej.2015.01.096
Chigondo, M., Kamdem Paumo, H., Bhaumik, M., Pillay, K., and Maity, A. (2018). Hydrous CeO2-Fe3O4 decorated polyaniline fibers nanocomposite for effective defluoridation of drinking water. J. Colloid Interface Sci. 532, 500–516. doi:10.1016/j.jcis.2018.07.134
Choi, K., Lee, S., Park, J. O., Park, J. A., Cho, S. H., Lee, S. Y., et al. (2018). Chromium removal from aqueous solution by a PEI-silica nanocomposite. Sci. Rep. 8 (1), 1438. doi:10.1038/s41598-018-20017-9
Chowdhury, T., Zhang, L., Zhang, J., and Aggarwal, S. (2018). Removal of arsenic(III) from aqueous solution using metal organic framework-graphene oxide nanocomposite. Nanomaterials 8 (12), 1062. doi:10.3390/nano8121062
Crini, G., and Lichtfouse, E. (2019). Advantages and disadvantages of techniques used for wastewater treatment. Environ. Chem. Lett. 17 (1), 145–155. doi:10.1007/s10311-018-0785-9
Deng, S., Li, Z., Huang, J., and Yu, G. (2010). Preparation, characterization and application of a Ce–Ti oxide adsorbent for enhanced removal of arsenate from water. J. Hazard. Mater. 179 (1–3), 1014–1021. doi:10.1016/j.jhazmat.2010.03.106
Deng, S., and Ting, Y. P. (2005). Characterization of PEI-modified biomass and biosorption of Cu(II), Pb(II) and Ni(II). Water Res. 39 (10), 2167–2177. doi:10.1016/j.watres.2005.03.033
Deshmukh, R., Khardenavis, A. A., and Purohit, H. J. (2016). Diverse metabolic capacities of fungi for bioremediation. Indian J. Microbiol. 56 (3), 247–264. doi:10.1007/s12088-016-0584-6
Dias, M. A., Lacerda, I. C. A., Pimentel, P. F., De Castro, H. F., and Rosa, C. A. (2002). Removal of heavy metals by an Aspergillus terreus strain immobilized in a polyurethane matrix. Lett. Appl. Microbiol. 34 (1), 46–50. doi:10.1046/j.1472-765x.2002.01040.x
Ding, C., Cheng, W., Sun, Y., and Wang, X. (2015). Novel fungus-Fe3O4 bio-nanocomposites as high performance adsorbents for the removal of radionuclides. J. Hazard. Mater. 295, 127–137. doi:10.1016/j.jhazmat.2015.04.032
Dinker, M. K., and Kulkarni, P. S. (2015). Recent advances in silica-based materials for the removal of hexavalent chromium: a review. J. Chem. Eng. Data 60 (9), 2521–2540. doi:10.1021/acs.jced.5b00292
Dutta, D., Arya, S., and Kumar, S. (2021). Industrial wastewater treatment: current trends, bottlenecks, and best practices. Chemosphere 285, 131245. doi:10.1016/j.chemosphere.2021.131245
Dutta, S., Manna, K., Srivastava, S. K., Gupta, A. K., and Yadav, M. K. (2020). Hollow polyaniline microsphere/Fe3O4 nanocomposite as an effective adsorbent for removal of arsenic from water. Sci. Rep. 10 (1), 4982. doi:10.1038/s41598-020-61763-z
Elkhatib, E. A., Moharem, M. L., Saad, A. F., and Attia, F. A. (2022). Novel metal based nanocomposite for rapid and efficient removal of lead from contaminated wastewater sorption kinetics, thermodynamics and mechanisms. Sci. Rep. 12 (1), 8412. doi:10.1038/s41598-022-12485-x
El-Maghrabi, H. H., Younes, A. A., Salem, A. R., Rabie, K., and El-shereafy, E. sayed (2019). Magnetically modified hydroxyapatite nanoparticles for the removal of uranium (VI): preparation, characterization and adsorption optimization. J. Hazard. Mater. 378, 120703. doi:10.1016/j.jhazmat.2019.05.096
El-Tawil, R. S., El-Wakeel, S. T., Abdel-Ghany, A. E., Abuzeid, H. A. M., Selim, K. A., and Hashem, A. M. (2019). Silver/quartz nanocomposite as an adsorbent for removal of mercury (II) ions from aqueous solutions. Heliyon 5 (9), e02415. doi:10.1016/j.heliyon.2019.e02415
Espinosa-Ortiz, E. J., Shakya, M., Jain, R., Rene, E. R., Van Hullebusch, E. D., and Lens, P. N. L. (2016). Sorption of zinc onto elemental selenium nanoparticles immobilized in Phanerochaete chrysosporium pellets. Environ. Sci. Pollut. Res. 23 (21), 21619–21630. doi:10.1007/s11356-016-7333-6
Fallah, Z., Isfahani, H. N., and Tajbakhsh, M. (2020). Removal of fluoride ion from aqueous solutions by titania-grafted β-cyclodextrin nanocomposite. Environ. Sci. Pollut. Res. 27 (3), 3281–3294. doi:10.1007/s11356-019-06948-4
Farooq, S., Siddiqa, A., Ashraf, S., Haider, S., Imran, S., Shahida, S., et al. (2022). Effective removal of arsenic (V) from aqueous solutions using efficient CuO/TiO2 nanocomposite adsorbent. Eur. J. Chem. 13 (3), 284–292. doi:10.5155/eurjchem.13.3.284-292.2283
Fosso-Kankeu, E., Mittal, H., Waanders, F., and Ray, S. S. (2017). Thermodynamic properties and adsorption behaviour of hydrogel nanocomposites for cadmium removal from mine effluents. J. Industrial Eng. Chem. 48, 151–161. doi:10.1016/j.jiec.2016.12.033
Fowler, B. A. (2002). Monitoring of human populations for early markers of cadmium toxicity: a review. Toxicol. Appl. Pharmacol. 238 (3), 294–300. doi:10.1016/j.taap.2009.05.004
Fu, F., and Wang, Q. (2011). Removal of heavy metal ions from wastewaters: a review. J. Environ. Manag. 92 (3), 407–418. doi:10.1016/j.jenvman.2010.11.011
Ganzagh, M. A. A., Yousefpour, M., and Taherian, Z. (2016). The removal of mercury (II) from water by Ag supported on nanomesoporous silica. J. Chem. Biol. 9 (4), 127–142. doi:10.1007/s12154-016-0157-5
Georgescu, A. A., Eliescu, A., Nicolescu, C. M., Bumbac, M., Cioateră, N., Mureșeanu, M., et al. (2019). Performance of Pleurotus ostreatus mushrooms and spent substrate for the biosorption of Cd(II) from aqueous solution. Anal. Lett. 52 (13), 2007–2027. doi:10.1080/00032719.2019.1590380
Gusain, R., Kumar, N., Fosso-Kankeu, E., and Ray, S. S. (2019). Efficient removal of Pb(II) and Cd(II) from industrial mine water by a hierarchical MoS 2/SH-MWCNT nanocomposite. ACS Omega 4 (9), 13922–13935. doi:10.1021/acsomega.9b01603
Häder, D. P., Banaszak, A. T., Villafañe, V. E., Narvarte, M. A., González, R. A., and Helbling, E. W. (2020). Anthropogenic pollution of aquatic ecosystems: emerging problems with global implications. Sci. Total Environ. 713, 136586. doi:10.1016/j.scitotenv.2020.136586
Han, J., Zhang, G., Zhou, L., Zhan, F., Cai, D., and Wu, Z. (2018). Waste carton-derived nanocomposites for efficient removal of hexavalent chromium. Langmuir 34 (21), 5955–5963. doi:10.1021/acs.langmuir.8b00225
Hao, L., Liu, M., Wang, N., and Li, G. (2018). A critical review on arsenic removal from water using iron-based adsorbents. RSC Adv. 8 (69), 39545–39560. doi:10.1039/c8ra08512a
Hao, Z., Nie, D., Zhang, M., Wang, W., Zou, D., and Nie, G. (2023). Polymer-microsphere coated with MoS2 nanoflowers for the removal of Pb(II) from water: polydopamine-mediated MoS2 assembly and efficient Pb(II) adsorption. Chem. Eng. J. 465, 142721. doi:10.1016/j.cej.2023.142721
Hayati, B., Maleki, A., Najafi, F., Daraei, H., Gharibi, F., and McKay, G. (2016). Synthesis and characterization of PAMAM/CNT nanocomposite as a super-capacity adsorbent for heavy metal (Ni2+, Zn2+, As3+, Co2+) removal from wastewater. J. Mol. Liq. 224, 1032–1040. doi:10.1016/j.molliq.2016.10.053
Hiremath, P. G., and Theodore, T. (2016). Zirconium-doped fungal sorbents: preparation, characterization, adsorption isotherm, and kinetic and mathematical modelling study for removal of fluoride. Adv. Chem. 2016, 1–14. doi:10.1155/2016/6848693
Hussain, F., Hojjati, M., Okamoto, M., and Gorga, R. E. (2006). Review article: polymer-matrix nanocomposites, processing, manufacturing, and application: an overview. J. Compos. Mater. 40 (17), 1511–1575. doi:10.1177/0021998306067321
Joshi, P. K., Swarup, A., Maheshwari, S., Kumar, R., and Singh, N. (2011). Bioremediation of heavy metals in liquid media through fungi isolated from contaminated sources. Indian J. Microbiol. 51 (4), 482–487. doi:10.1007/s12088-011-0110-9
Kaçar, Y., Arpa, Ç., Tan, S., Denizli, A., Genç, Ö., and Arıca, M. Y. (2002). Biosorption of Hg(II) and Cd(II) from aqueous solutions: comparison of biosorptive capacity of alginate and immobilized live and heat inactivated Phanerochaete chrysosporium. Process Biochem. 37 (6), 601–610. doi:10.1016/s0032-9592(01)00248-5
Kadhim, H. H., and Saleh, K. A. (2022). Removing of copper ions from industrial wastewater using graphene oxide/chitosan nanocomposite. eijs, 1894–1908. doi:10.24996/ijs.2022.63.5.4
Kandisa, R. V., and Saibaba Kv, N. (2016). Dye removal by adsorption: a review. J. Bioremediat Biodegr. 07 (06). doi:10.4172/2155-6199.1000371
Kang, R., Qiu, L., Fang, L., Yu, R., Chen, Y., Lu, X., et al. (2016). A novel magnetic and hydrophilic ion-imprinted polymer as a selective sorbent for the removal of cobalt ions from industrial wastewater. J. Environ. Chem. Eng. 4 (2), 2268–2277. doi:10.1016/j.jece.2016.04.010
Kaveh, R., and Bagherzadeh, M. (2022). Simultaneous removal of mercury ions and cationic and anionic dyes from aqueous solution using epichlorohydrin cross-linked chitosan @ magnetic Fe3O4/activated carbon nanocomposite as an adsorbent. Diam. Relat. Mater. 124, 108923. doi:10.1016/j.diamond.2022.108923
Khan, N. A., Hasan, Z., and Jhung, S. H. (2013). Adsorptive removal of hazardous materials using metal-organic frameworks (MOFs): a review. J. Hazard. Mater. 244–245, 444–456. doi:10.1016/j.jhazmat.2012.11.011
Khan, Q. U., Begum, N., Khan, A. U., Nazir, S., Ali, A., Tahir, K., et al. (2022). Nanocomposite based adsorbent for enhanced arsenic removal: determination of adsorption mechanism, kinetic study, and factors affecting the adsorption capability of nanomaterials. SSRN J. doi:10.2139/ssrn.4202055
Khoshkerdar, I., and Esmaeili, H. (2019). Adsorption of Cr (III) and Cd (II) ions using mesoporous cobalt-ferrite nanocomposite from synthetic wastewater. ACSi 66, 208–216. doi:10.17344/acsi.2018.4795
Kumar, R., Sharma, P., Umar, A., Kumar, R., Singh, N., Joshi, P. K., et al. (2020). In vitro bioadsorption of Cd2+ ions: adsorption isotherms, mechanism, and an insight to mycoremediation. Processes 8 (9), 1085. doi:10.3390/pr8091085
Kusrini, E., Sofyan, N., Suwartha, N., Yesya, G., and Priadi, C. R. (2015). Chitosan-praseodymium complex for adsorption of fluoride ions from water. J. Rare Earths 33 (10), 1104–1113. doi:10.1016/s1002-0721(14)60533-0
Lee, S. H., Kim, K. W., Lee, B. T., Bang, S., Kim, H., Kang, H., et al. (2015). Enhanced arsenate removal performance in aqueous solution by yttrium-based adsorbents. IJERPH 12 (10), 13523–13541. doi:10.3390/ijerph121013523
Leyssens, L., Vinck, B., Van Der Straeten, C., Wuyts, F., and Maes, L. (2017). Cobalt toxicity in humans—a review of the potential sources and systemic health effects. Toxicology 387, 43–56. doi:10.1016/j.tox.2017.05.015
Li, M., Liu, H., Chen, T., Dong, C., and Sun, Y. (2019). Synthesis of magnetic biochar composites for enhanced uranium(VI) adsorption. Sci. Total Environ. 651, 1020–1028. doi:10.1016/j.scitotenv.2018.09.259
Li, X., Zhou, H., Wu, W., Wei, S., Xu, Y., and Kuang, Y. (2015). Studies of heavy metal ion adsorption on Chitosan/Sulfydryl-functionalized graphene oxide composites. J. Colloid Interface Sci. 448, 389–397. doi:10.1016/j.jcis.2015.02.039
Li, Y., Zou, G., Yang, S., Shi, P., Chen, T., Lian, Y., et al. (2018). Bioassembly of fungal hyphae/graphene oxide composite as high performance adsorbents for U(VI) removal. Appl. Surf. Sci. 458, 226–235. doi:10.1016/j.apsusc.2018.07.081
Lian, Y., Bai, X., Li, X., Gao, Z., Hu, Z., and Hu, G. (2017). Novel fungal hyphae/Fe 3 O 4 and N-TiO 2/NG composite for adsorption and photocatalysis. RSC Adv. 7 (12), 6842–6848. doi:10.1039/c6ra25964b
Lingamdinne, L. P., Vemula, K. R., Chang, Y. Y., Yang, J. K., Karri, R. R., and Koduru, J. R. (2020). Process optimization and modeling of lead removal using iron oxide nanocomposites generated from bio-waste mass. Chemosphere 243, 125257. doi:10.1016/j.chemosphere.2019.125257
Lissy, P. N. M., Madhu, G., and Thomas, R. M. (2019). Polypyrrole/alumina-iron oxide nanocomposite for removal of copper ions from aqueous solutions. MSF 969, 367–372. doi:10.4028/www.scientific.net/msf.969.367
Liu, L., Cui, Z., Ma, Q., Cui, W., and Zhang, X. (2016). One-step synthesis of magnetic iron–aluminum oxide/graphene oxide nanoparticles as a selective adsorbent for fluoride removal from aqueous solution. RSC Adv. 6 (13), 10783–10791. doi:10.1039/c5ra23676b
Liu, W., Ni, J., and Yin, X. (2014). Synergy of photocatalysis and adsorption for simultaneous removal of Cr(VI) and Cr(III) with TiO2 and titanate nanotubes. Water Res. 53, 12–25. doi:10.1016/j.watres.2013.12.043
Liu, W., Yang, L., Xu, S., Chen, Y., Liu, B., Li, Z., et al. (2018). Efficient removal of hexavalent chromium from water by an adsorption–reduction mechanism with sandwiched nanocomposites. RSC Adv. 8 (27), 15087–15093. doi:10.1039/c8ra01805g
Lotlikar, N. P., Damare, S. R., Meena, R. M., Linsy, P., and Mascarenhas, B. (2018). Potential of marine-derived fungi to remove hexavalent chromium pollutant from culture broth. Indian J. Microbiol. 58 (2), 182–192. doi:10.1007/s12088-018-0719-z
Maitlo, H. A., Kim, K. H., Kumar, V., Kim, S., and Park, J. W. (2019). Nanomaterials-based treatment options for chromium in aqueous environments. Environ. Int. 130, 104748. doi:10.1016/j.envint.2019.04.020
Malar, S., Sahi, S. V., Favas, P. J. C., and Venkatachalam, P. (2015). Mercury heavy-metal-induced physiochemical changes and genotoxic alterations in water hyacinths [Eichhornia crassipes (Mart.)]. Environ. Sci. Pollut. Res. 22 (6), 4597–4608. doi:10.1007/s11356-014-3576-2
Malaviya, P., and Singh, A. (2016). Bioremediation of chromium solutions and chromium containing wastewaters. Crit. Rev. Microbiol. 42 (4), 607–633. doi:10.3109/1040841x.2014.974501
Masindi, V., Foteinis, S., Tekere, M., and Ramakokovhu, M. M. (2021). Facile synthesis of halloysite-bentonite clay/magnesite nanocomposite and its application for the removal of chromium ions: adsorption and precipitation process. Mater. Today Proc. 38, 1088–1101. doi:10.1016/j.matpr.2020.06.084
Mohammadi, E., Daraei, H., Ghanbari, R., Dehestani Athar, S., Zandsalimi, Y., Ziaee, A., et al. (2019). Synthesis of carboxylated chitosan modified with ferromagnetic nanoparticles for adsorptive removal of fluoride, nitrate, and phosphate anions from aqueous solutions. J. Mol. Liq. 273, 116–124. doi:10.1016/j.molliq.2018.10.019
Mohammadnezhad, G., and Keikavousi Behbahan, A. (2020). Polymer matrix nanocomposites for heavy metal adsorption: a review. J. Iran. Chem. Soc. 17 (6), 1259–1281. doi:10.1007/s13738-020-01864-8
Mohseni-Bandpi, A., Kakavandi, B., Kalantary, R. R., Azari, A., and Keramati, A. (2015). Development of a novel magnetite–chitosan composite for the removal of fluoride from drinking water: adsorption modeling and optimization. RSC Adv. 5 (89), 73279–73289. doi:10.1039/c5ra11294j
Mondal, P., and Purkait, M. K. (2019). Preparation and characterization of novel green synthesized iron–aluminum nanocomposite and studying its efficiency in fluoride removal. Chemosphere 235, 391–402. doi:10.1016/j.chemosphere.2019.06.189
Moreno-Sader, K., García-Padilla, A., Realpe, A., Acevedo-Morantes, M., and Soares, J. B. P. (2019). Removal of heavy metal water pollutants (Co 2+ and Ni 2+) using polyacrylamide/sodium montmorillonite (PAM/Na-MMT) nanocomposites. ACS Omega 4 (6), 10834–10844. doi:10.1021/acsomega.9b00981
Mosleh, N., Najmi, M., Parandi, E., Rashidi Nodeh, H., Vasseghian, Y., and Rezania, S. (2022). Magnetic sporopollenin supported polyaniline developed for removal of lead ions from wastewater: kinetic, isotherm and thermodynamic studies. Chemosphere 300, 134461. doi:10.1016/j.chemosphere.2022.134461
Motaghi, H., Arabkhani, P., Parvinnia, M., and Asfaram, A. (2022). Simultaneous adsorption of cobalt ions, azo dye, and imidacloprid pesticide on the magnetic chitosan/activated carbon@UiO-66 bio-nanocomposite: optimization, mechanisms, regeneration, and application. Sep. Purif. Technol. 284, 120258. doi:10.1016/j.seppur.2021.120258
Mou, H., Huang, X., Deng, Q., Lei, Q., Luo, H., Liang, J., et al. (2021). Preparation of graphene oxide-modified palygorskite nanocomposites for high-efficient removal of Co(II) from wastewater. Environ. Sci. Pollut. Res. 28 (2), 1919–1932. doi:10.1007/s11356-020-07890-6
Müller, K., Bugnicourt, E., Latorre, M., Jorda, M., Echegoyen Sanz, Y., Lagaron, J., et al. (2017). Review on the processing and properties of polymer nanocomposites and nanocoatings and their applications in the packaging, automotive and solar energy fields. Nanomaterials 7 (4), 74. doi:10.3390/nano7040074
Muntean, S. G., Nistor, M. A., Muntean, E., Todea, A., Ianoş, R., and Păcurariu, C. (2018). Removal of colored organic pollutants from wastewaters by magnetite/carbon nanocomposites: single and binary systems. J. Chem. 2018, 1–16. doi:10.1155/2018/6249821
Musico, Y. L. F., Santos, C. M., Dalida, M. L. P., and Rodrigues, D. F. (2013). Improved removal of lead(ii) from water using a polymer-based graphene oxide nanocomposite. J. Mater Chem. A 1 (11), 3789. doi:10.1039/c3ta01616a
Narasimharao, K., Lingamdinne, L. P., Al-Thabaiti, S., Mokhtar, M., Alsheshri, A., Alfaifi, S. Y., et al. (2022). Synthesis and characterization of hexagonal Mg Fe layered double hydroxide/grapheme oxide nanocomposite for efficient adsorptive removal of cadmium ion from aqueous solutions: isotherm, kinetic, thermodynamic and mechanism. J. Water Process Eng. 47, 102746. doi:10.1016/j.jwpe.2022.102746
Neskoromnaya, E. A., Khamizov, R. K., Melezhyk, A. V., Memetova, A. E., Mkrtchan, E. S., and Babkin, A. V. (2022). Adsorption of lead ions (Pb2+) from wastewater using effective nanocomposite GO/CMC/FeNPs: kinetic, isotherm, and desorption studies. Colloids Surfaces A Physicochem. Eng. Aspects 655, 130224. doi:10.1016/j.colsurfa.2022.130224
Nithya, R., Gomathi, T., Sudha, P. N., Venkatesan, J., Anil, S., and Kim, S. K. (2016). Removal of Cr(VI) from aqueous solution using chitosan-g-poly(butyl acrylate)/silica gel nanocomposite. Int. J. Biol. Macromol. 87, 545–554. doi:10.1016/j.ijbiomac.2016.02.076
Ochoa-Herrera, V., León, G., Banihani, Q., Field, J. A., and Sierra-Alvarez, R. (2011). Toxicity of copper(II) ions to microorganisms in biological wastewater treatment systems. Sci. Total Environ. 412–413, 380–385. doi:10.1016/j.scitotenv.2011.09.072
Omanović-Mikličanin, E., Badnjević, A., Kazlagić, A., and Hajlovac, M. (2020). Nanocomposites: a brief review. Health Technol. 10 (1), 51–59. doi:10.1007/s12553-019-00380-x
Pandey, N., Shukla, S. K., and Singh, N. B. (2017). Water purification by polymer nanocomposites: an overview. Nanocomposites 3 (2), 47–66. doi:10.1080/20550324.2017.1329983
Park, Y., Liu, S., Gardner, T., Johnson, D., Keeler, A., Ortiz, N., et al. (2020). Biohybrid nanofibers containing manganese oxide–forming fungi for heavy metal removal from water. J. Eng. Fibers Fabr. 15, 155892501989895. doi:10.1177/1558925019898954
Parthiban, E., Kalaivasan, N., and Sudarsan, S. (2020). Dual responsive (pH and magnetic) nanocomposites based on Fe3O4@Polyaniline/itaconic acid: synthesis, characterization and removal of toxic hexavalent chromium from tannery wastewater. J. Inorg. Organomet. Polym. 30 (11), 4677–4690. doi:10.1007/s10904-020-01602-w
Pavlidou, S., and Papaspyrides, C. D. (2008). A review on polymer–layered silicate nanocomposites. Prog. Polym. Sci. 33 (12), 1119–1198. doi:10.1016/j.progpolymsci.2008.07.008
Peng, Q., Liu, Y., Zeng, G., Xu, W., Yang, C., and Zhang, J. (2010). Biosorption of copper(II) by immobilizing Saccharomyces cerevisiae on the surface of chitosan-coated magnetic nanoparticles from aqueous solution. J. Hazard. Mater. 177 (1–3), 676–682. doi:10.1016/j.jhazmat.2009.12.084
Perullini, M., Jobbágy, M., Mouso, N., Forchiassin, F., and Bilmes, S. A. (2010). Silica-alginate-fungi biocomposites for remediation of polluted water. J. Mater Chem. 20 (31), 6479. doi:10.1039/c0jm01144d
Pous, N., Casentini, B., Rossetti, S., Fazi, S., Puig, S., and Aulenta, F. (2015). Anaerobic arsenite oxidation with an electrode serving as the sole electron acceptor: a novel approach to the bioremediation of arsenic-polluted groundwater. J. Hazard. Mater. 283, 617–622. doi:10.1016/j.jhazmat.2014.10.014
Rajivgandhi, G., Rtv, V., Nandhakumar, R., Murugan, S., Alharbi, N. S., Kadaikunnan, S., et al. (2021). Adsorption of nickel ions from electroplating effluent by graphene oxide and reduced graphene oxide. Environ. Res. 199, 111322. doi:10.1016/j.envres.2021.111322
Ricco, R., Konstas, K., Styles, M. J., Richardson, J. J., Babarao, R., Suzuki, K., et al. (2015). Lead(ii) uptake by aluminium based magnetic framework composites (MFCs) in water. J. Mater Chem. A 3 (39), 19822–19831. doi:10.1039/c5ta04154f
Rodríguez, R., Espada, J. J., Gallardo, M., Molina, R., and López-Muñoz, M. J. (2018). Life cycle assessment and techno-economic evaluation of alternatives for the treatment of wastewater in a chrome-plating industry. J. Clean. Prod. 172, 2351–2362. doi:10.1016/j.jclepro.2017.11.175
Roudbari, R., Keramati, N., and Ghorbani, M. (2021). Porous nanocomposite based on metal-organic framework: antibacterial activity and efficient removal of Ni(II) heavy metal ion. J. Mol. Liq. 322, 114524. doi:10.1016/j.molliq.2020.114524
Safatian, F., Doago, Z., Torabbeigi, M., Rahmani Shams, H., and Ahadi, N. (2019). Lead ion removal from water by hydroxyapatite nanostructures synthesized from egg sells with microwave irradiation. Appl. Water Sci. 9 (4), 108. doi:10.1007/s13201-019-0979-8
Sahoo, S. K., and Hota, G. (2018). Surface functionalization of GO with MgO/MgFe2O4 binary oxides: a novel magnetic nanoadsorbent for removal of fluoride ions. J. Environ. Chem. Eng. 6 (2), 2918–2931. doi:10.1016/j.jece.2018.04.054
Said, R. A. M., Hasan, M. A., Abdelzaher, A. M., and Abdel-Raoof, A. M. (2020). Review—insights into the developments of nanocomposites for its processing and application as sensing materials. J. Electrochem Soc. 167 (3), 037549. doi:10.1149/1945-7111/ab697b
Saif, S., Tahir, A., Asim, T., Chen, Y., and Adil, S. (2019). Polymeric nanocomposites of iron–oxide nanoparticles (IONPs) synthesized using Terminalia chebula leaf extract for enhanced adsorption of arsenic(V) from water. Colloids Interfaces 3 (1), 17. doi:10.3390/colloids3010017
Saini, J., Garg, V. K., and Gupta, R. K. (2020). Green synthesized SiO2@OPW nanocomposites for enhanced Lead (II) removal from water. Arabian J. Chem. 13 (1), 2496–2507. doi:10.1016/j.arabjc.2018.06.003
Sankararamakrishnan, N., Singh, R., and Srivastava, I. (2019). Performance of novel MgS doped cellulose nanofibres for Cd(II) removal from industrial effluent – mechanism and optimization. Sci. Rep. 9 (1), 12639. doi:10.1038/s41598-019-49076-2
Shakerian, K., and Esmaeili, H. (2018). Synthesis of CaO/Fe3O4 magnetic composite for the removal of Pb(II) and Co(II) from synthetic wastewater. J. Serb Chem. Soc. 83 (2), 237–249. doi:10.2298/jsc170704098s
Sharififard, H., Shahraki, Z. H., Rezvanpanah, E., and Rad, S. H. (2018). A novel natural chitosan/activated carbon/iron bio-nanocomposite: sonochemical synthesis, characterization, and application for cadmium removal in batch and continuous adsorption process. Bioresour. Technol. 270, 562–569. doi:10.1016/j.biortech.2018.09.094
Sharma, A., and Lee, B. K. (2014). Cd(II) removal and recovery enhancement by using acrylamide–titanium nanocomposite as an adsorbent. Appl. Surf. Sci. 313, 624–632. doi:10.1016/j.apsusc.2014.06.034
Shehu, Z., and Lamayi, D. W. (2019). Recent advances and developments in nanoparticles/nanocomposites as nanoadsorbent for adsorptive removal of lead in wastewater: a review. NNOA 4 (3). doi:10.23880/nnoa-16000165
Singh, P., Sarswat, A., Pittman, C. U., Mlsna, T., and Mohan, D. (2020). Sustainable low-concentration arsenite [as(III)] removal in single and multicomponent systems using hybrid iron oxide–biochar nanocomposite adsorbents—a mechanistic study. ACS Omega 5 (6), 2575–2593. doi:10.1021/acsomega.9b02842
Singh, S., Arputharaj, E., Dahms, H. U., Patel, A. K., and Huang, Y. L. (2022). Chitosan-based nanocomposites for removal of Cr(VI) and synthetic food colorants from wastewater. Bioresour. Technol. 351, 127018. doi:10.1016/j.biortech.2022.127018
Su, H., Ye, Z., and Hmidi, N. (2017). High-performance iron oxide–graphene oxide nanocomposite adsorbents for arsenic removal. Colloids Surfaces A Physicochem. Eng. Aspects 522, 161–172. doi:10.1016/j.colsurfa.2017.02.065
Su, H., Ye, Z., Hmidi, N., and Subramanian, R. (2017). Carbon nanosphere–iron oxide nanocomposites as high-capacity adsorbents for arsenic removal. RSC Adv. 7 (57), 36138–36148. doi:10.1039/c7ra06187k
Sun, W., Li, L., Luo, C., and Fan, L. (2016). Synthesis of magnetic graphene nanocomposites decorated with ionic liquids for fast lead ion removal. Int. J. Biol. Macromol. 85, 246–251. doi:10.1016/j.ijbiomac.2015.09.061
Sun, Z., Liu, B., Li, M., Li, C., and Zheng, S. (2020). Carboxyl-rich carbon nanocomposite based on natural diatomite as adsorbent for efficient removal of Cr (VI). J. Mater. Res. Technol. 9 (1), 948–959. doi:10.1016/j.jmrt.2019.11.034
Swaddiwudhipong, W., Limpatanachote, P., Mahasakpan, P., Krintratun, S., Punta, B., and Funkhiew, T. (2012). Progress in cadmium-related health effects in persons with high environmental exposure in northwestern Thailand: a five-year follow-up. Environ. Res. 112, 194–198. doi:10.1016/j.envres.2011.10.004
Taka, A. L., Fosso-Kankeu, E., Pillay, K., and Mbianda, X. Y. (2018). Removal of cobalt and lead ions from wastewater samples using an insoluble nanosponge biopolymer composite: adsorption isotherm, kinetic, thermodynamic, and regeneration studies. Environ. Sci. Pollut. Res. 25 (22), 21752–21767. doi:10.1007/s11356-018-2055-6
Tan, L., Zhang, X., Liu, Q., Jing, X., Liu, J., Song, D., et al. (2015). Synthesis of Fe3O4@TiO2 core–shell magnetic composites for highly efficient sorption of uranium (VI). Colloids Surfaces A Physicochem. Eng. Aspects 469, 279–286. doi:10.1016/j.colsurfa.2015.01.040
Thanh, D. N., Novák, P., Vejpravova, J., Vu, H. N., Lederer, J., and Munshi, T. (2018). Removal of copper and nickel from water using nanocomposite of magnetic hydroxyapatite nanorods. J. Magnetism Magnetic Mater. 456, 451–460. doi:10.1016/j.jmmm.2017.11.064
Thy, L. T. M., Hau, D. T., Lien, L. T. K., Hung, N. D. H., Tu, T. H., Nam, H. M., et al. (2021). Fabrication of RHA-silica/graphene oxide nanocomposites for removal of lead ions from water. Environ. Technol. 42 (14), 2202–2210. doi:10.1080/09593330.2019.1696412
Tizro, S., and Baseri, H. (2017). Removal of cobalt ions from contaminated water using magnetite based nanocomposites: effects of various parameters on the removal efficiency. J. Water Environ. Nanotechnol. 2 (3). doi:10.22090/jwent.2017.03.005
Tran, L. T., Tran, H. V., Le, T. D., Bach, G. L., and Tran, L. D. (2019). Studying Ni(II) adsorption of magnetite/graphene oxide/chitosan nanocomposite. Adv. Polym. Technol. 2019, 1–9. doi:10.1155/2019/8124351
Tuzen, M., Sarı, A., Afshar Mogaddam, M. R., Kaya, S., Katin, K. P., and Altunay, N. (2022). Synthesis of carbon modified with polymer of diethylenetriamine and trimesoyl chloride for the dual removal of Hg (II) and methyl mercury ([CH3Hg]+) from wastewater: theoretical and experimental analyses. Mater. Chem. Phys. 277, 125501. doi:10.1016/j.matchemphys.2021.125501
Vaksmaa, A., Guerrero-Cruz, S., Ghosh, P., Zeghal, E., Hernando-Morales, V., and Niemann, H. (2023). Role of fungi in bioremediation of emerging pollutants. Front. Mar. Sci. 10, 1070905. doi:10.3389/fmars.2023.1070905
Varma, V. G., and Misra, A. K. (2018). Copper contaminated wastewater – an evaluation of bioremedial options. Indoor Built Environ. 27 (1), 84–95. doi:10.1177/1420326x16669397
Verma, M., Lee, I., Oh, J., Kumar, V., and Kim, H. (2022). Synthesis of EDTA-functionalized graphene oxide-chitosan nanocomposite for simultaneous removal of inorganic and organic pollutants from complex wastewater. Chemosphere 287, 132385. doi:10.1016/j.chemosphere.2021.132385
Vunain, E., Mishra, A., and Mamba, B. (2016). Dendrimers, mesoporous silicas and chitosan-based nanosorbents for the removal of heavy-metal ions: a review. Int. J. Biol. Macromol. 86, 570–586. doi:10.1016/j.ijbiomac.2016.02.005
Wang, P., Shen, T., Li, X., Tang, Y., and Li, Y. (2020). Magnetic mesoporous calcium carbonate-based nanocomposites for the removal of toxic Pb(II) and Cd(II) ions from water 3 (2), 1272–1281. doi:10.1021/acsanm.9b02036
Wang, X. (2012). Nanomaterials as sorbents to remove heavy metal ions in wastewater treatment. J. Environ. Anal. Toxicol. 02 (07). doi:10.4172/2161-0525.1000154
Xia, M., Chen, Z., Li, Y., Li, C., Ahmad, N. M., Cheema, W. A., et al. (2019). Removal of Hg(ii) in aqueous solutions through physical and chemical adsorption principles. RSC Adv. 9 (36), 20941–20953. doi:10.1039/c9ra01924c
Xu, N., Li, S., Li, W., and Liu, Z. (2020). Removal of fluoride by graphene oxide/alumina nanocomposite: adsorbent preparation, characterization, adsorption performance and mechanisms. ChemistrySelect 5 (6), 1818–1828. doi:10.1002/slct.201904867
Xu, P., Zeng, G., Huang, D., Hu, S., Feng, C., Lai, C., et al. (2013). Synthesis of iron oxide nanoparticles and their application in Phanerochaete chrysosporium immobilization for Pb(II) removal. Colloids Surfaces A Physicochem. Eng. Aspects 419, 147–155. doi:10.1016/j.colsurfa.2012.10.061
Xu, P., Zeng, G. M., Huang, D. L., Lai, C., Zhao, M. H., Wei, Z., et al. (2012). Adsorption of Pb(II) by iron oxide nanoparticles immobilized Phanerochaete chrysosporium: equilibrium, kinetic, thermodynamic and mechanisms analysis. Chem. Eng. J. 203, 423–431. doi:10.1016/j.cej.2012.07.048
Yang, H., Masse, S., Rouelle, M., Aubry, E., Li, Y., Roux, C., et al. (2015). Magnetically recoverable iron oxide–hydroxyapatite nanocomposites for lead removal. Int. J. Environ. Sci. Technol. 12 (4), 1173–1182. doi:10.1007/s13762-014-0514-2
Yang, J., Hou, B., Wang, J., Tian, B., Bi, J., Wang, N., et al. (2019). Nanomaterials for the removal of heavy metals from wastewater. Nanomaterials 9 (3), 424. doi:10.3390/nano9030424
Yang, Z., Liang, L., Yang, W., Shi, W., Tong, Y., Chai, L., et al. (2018). Simultaneous immobilization of cadmium and lead in contaminated soils by hybrid bio-nanocomposites of fungal hyphae and nano-hydroxyapatites. Environ. Sci. Pollut. Res. 25 (12), 11970–11980. doi:10.1007/s11356-018-1492-6
Yohai, L., Giraldo Mejía, H., Procaccini, R., Pellice, S., Laxman Kunjali, K., Dutta, J., et al. (2019). Nanocomposite functionalized membranes based on silica nanoparticles cross-linked to electrospun nanofibrous support for arsenic(v) adsorption from contaminated underground water. RSC Adv. 9 (15), 8280–8289. doi:10.1039/c8ra09866b
Zahran, F., El-Maghrabi, H. H., Hussein, G., and Abdelmaged, S. M. (2019). Fabrication of bentonite based nanocomposite as a novel low cost adsorbent for uranium ion removal. Environ. Nanotechnol. Monit. Manag. 11, 100205. doi:10.1016/j.enmm.2018.100205
Zhang, C., Luan, J., Chen, W., Ke, X., and Zhang, H. (2019). Preparation of graphene oxide–montmorillonite nanocomposite and its application in multiple-pollutants removal from aqueous solutions. Water Sci. Technol. 79 (2), 323–333. doi:10.2166/wst.2019.046
Zhang, X., and Wang, X. (2015). Adsorption and desorption of nickel(II) ions from aqueous solution by a lignocellulose/montmorillonite nanocomposite. PLoS ONE 10 (2), e0117077. doi:10.1371/journal.pone.0117077
Zhao, G., Zhang, H., Fan, Q., Ren, X., Li, J., Chen, Y., et al. (2010). Sorption of copper(II) onto super-adsorbent of bentonite–polyacrylamide composites. J. Hazard. Mater. 173 (1–3), 661–668. doi:10.1016/j.jhazmat.2009.08.135
Zhao, N. D., Wang, Y., Hou, Y. C., Guo, Y. R., and Pan, Q. J. (2023). High efficiency filter: cellulose-derived biochar fibril and magnesium oxide composite for boosting performance of heavy metal ions capture. Appl. Surf. Sci. 609, 155267. doi:10.1016/j.apsusc.2022.155267
Zheng, N., Yin, L., Su, M., Liu, Z., Tsang, D. C. W., and Chen, D. (2020). Synthesis of shape and structure-dependent hydroxyapatite nanostructures as a superior adsorbent for removal of U(VI). Chem. Eng. J. 384, 123262. doi:10.1016/j.cej.2019.123262
Zhou, H., Li, X., Hu, B., Wu, M., Zhang, Y., Yi, X., et al. (2021). Assembly of fungal mycelium-carbon nanotube composites and their application in pyrene removal. J. Hazard. Mater. 415, 125743. doi:10.1016/j.jhazmat.2021.125743
Zhou, J., Gao, F., Jiao, T., Xing, R., Zhang, L., Zhang, Q., et al. (2018). Selective Cu(II) ion removal from wastewater via surface charged self-assembled polystyrene-Schiff base nanocomposites. Colloids Surfaces A Physicochem. Eng. Aspects 545, 60–67. doi:10.1016/j.colsurfa.2018.02.048
Zhou, W., Fu, H., Pan, K., Tian, C., Qu, Y., Lu, P., et al. (2008). Mesoporous TiO 2/α-Fe 2 O 3: bifunctional composites for effective elimination of arsenite contamination through simultaneous photocatalytic oxidation and adsorption. J. Phys. Chem. C 112 (49), 19584–19589. doi:10.1021/jp806594m
Zhu, W., Lei, J., Li, Y., Dai, L., Chen, T., Bai, X., et al. (2019). Procedural growth of fungal hyphae/Fe3O4/graphene oxide as ordered-structure composites for water purification. Chem. Eng. J. 355, 777–783. doi:10.1016/j.cej.2018.08.215
Zhu, W., Li, Y., Dai, L., Li, J., Li, X., Li, W., et al. (2018). Bioassembly of fungal hyphae/carbon nanotubes composite as a versatile adsorbent for water pollution control. Chem. Eng. J. 339, 214–222. doi:10.1016/j.cej.2018.01.134
Zhu, W. K., Cong, H. P., Guan, Q. F., Yao, W. T., Liang, H. W., Wang, W., et al. (2016). Coupling microbial growth with nanoparticles: a universal strategy to produce functional fungal hyphae macrospheres. ACS Appl. Mater Interfaces 8 (20), 12693–12701. doi:10.1021/acsami.6b03399
Keywords: water pollution, sustainable development, potable water, biosorption, fungal hyphae, heavy metal, metalloid
Citation: Singh N, Yadav A, Das S and Debnath N (2024) Recent advances in heavy metal/metalloid ion treatment from wastewater using nanocomposites and bionanocomposites. Front. Nanotechnol. 6:1307353. doi: 10.3389/fnano.2024.1307353
Received: 04 October 2023; Accepted: 15 January 2024;
Published: 19 February 2024.
Edited by:
Dilfuza Jabborova, Academy of Sciences Republic of Uzbekistan (UzAS), UzbekistanCopyright © 2024 Singh, Yadav, Das and Debnath. This is an open-access article distributed under the terms of the Creative Commons Attribution License (CC BY). The use, distribution or reproduction in other forums is permitted, provided the original author(s) and the copyright owner(s) are credited and that the original publication in this journal is cited, in accordance with accepted academic practice. No use, distribution or reproduction is permitted which does not comply with these terms.
*Correspondence: Namita Singh, namitasingh71@gmail.com
†These authors have contributed equally to this work