- 1NanoScience Technology Center, University of Central Florida, Orlando, FL, United States
- 2Department of Design, Indian Institute of Technology Kanpur, Kanpur, Uttar Pradesh, India
- 3Department of Biological Sciences and Bioengineering, Indian Institute of Technology Kanpur, Kanpur, Uttar Pradesh, India
Introduction: Agriculture predominantly follows three farming systems: natural, organic (organic manures for soil nutrition), and agrochemical intensive. Agrochemical-intensive farming uses synthetic fertilizers, insecticides, pesticides, and weedicides. It holds the most prominent place in the modern agriculture business. Humankind realizes that over-reliance on synthetic agrochemicals has resulted in the declining health of the ecosystem’s soil, water, and living species. It must explore strategies to reduce synthetic agrochemicals without compromising agricultural production to restore the ecological balance. Nano-formulations help lower dosages of agrochemicals, leading to the emergence of nano-agriculture. However, the critical challenge is how we could exploit nanomaterials’ power to selectively improve crop plants’ metabolic performance so that it has a better competitive edge in the field to withstand the nutrient-seeking pressure exerted by other plants.
Methods: Here we have achieved the same by root treatment of the onion crop and then growing them in the presence of organic goat manure.
Results: In a 2 years field trial with onion crops, we report an increase in yield through root treatment with nano-pyrite (FeS2) plus soil application of goat dropping (Test) as compared to the use of goat dropping alone (Control). In the first-year field trial (November 2018- April 2019), the total biomass (bulb + leaf) weight of the test sample was 4.75 kg (n = 86), while control samples weighed 3.5 kg (n = 83). The total bulb weight for the control and test was 2.6 and 3.6 kg, respectively. In the second-year field trial (December 2019- April 2020), the total biomass of the test sample was 2.65 kg (n = 64), while control samples weighed 2.30 kg (n = 64). We observed a yield-boosting effect of root treatment with nano-pyrite plus soil application of goat dropping in onion crops. As monitored by spectroscopic absorbance, there is a significant increase in the anthocyanin content in test bulbs (0.069) compared to control bulbs (0.02). Similarly, the relative flavanol content in test leaves (0.253) was significantly higher compared to the control samples (0.086). We have integrated nano-agriculture and organic farming, resulting in a hybrid form; nano-organic farming bolsters the metabolic fitness of the onion (Allium cepa) to achieve sustainable food production.
Introduction
Through evolution, humankind has developed various strategies for food production. When we as a race shifted from food gatherers to food producers, the first form of farming emerged as natural farming, relying on minimal interference to the ecosystem (Gopal De., 1989; Loukas et al., 2009; Douglas et al., 2018). Even today, many parts of the world follow natural agriculture. Wisdom-driven advancement of human skills led to the observation that organic matter in the soil helped improve crop productivity, resulting in the emergence of organic farming. One of the salient organic farming features is that essential plant nutrients are entrapped in a mass of carbon, helping sustain soil health and fertility (Rigby and Cáceres, 2001; Andreas et al., 2012; Yadav et al., 2013; Crowder and Reganold, 2015). Various studies conducted in the early 19th century by scientists, namely J B Boussingault, Justus Von Liebig, Jon Bennet Lawes, and JH Gilbert, explored soil, plant, and plant and organic matter influencing soil fertility. They discovered the major plant nutrients: nitrogen, phosphorus, potassium, calcium, magnesium, sulfur, and iron (Gopal De., 1989).
These discoveries marked the onset of agrochemical-intensive farming. One of the hallmark discoveries was the ammonia synthesis by the Haber-Bosch process at the beginning of the 20th century (Delwiche, 1970). There was a meteoric rise in agrochemicals in the agricultural production system following the green revolution around the second half of the 20th century (Gignoux et al., 2011; Pingali, 2012; Kravchenko et al., 2017).
The intensive use of agrochemicals in agriculture during the last five decades has resulted in soil health and water quality degradation, eventually affecting various life forms of the ecosystem. Reduction in chemical fertilizers is a significant challenge, with the global demand for fertilizer nutrients (N + P2O5 + K2O) being 198107 thousand tonnes in 2019 and forecasted to be 201663 thousand tonnes by 2020 (Food & Agriculture Organization FAO of the United Nations, 2017). There is a need to develop sustainable strategies to restore the ecological balance and maintain agricultural production. The current thrust is to reduce agrochemicals’ consumption and increase their efficacy.
Several sustainable solutions, such as the use of bio-fertilizers (Rao, 1982), organic manure (Gaur et al., 1984), nano-fertilizers (Tripathi et al., 2011; Sonkar et al., 2012; Tripathi and Sarkar, 2015; Bhati et al., 2018; Raliya et al., 2018; Boutchuen et al., 2019), and nano-encapsulations, are currently being explored to reduce the use of chemical fertilizers and prevent disease infestation (Ahuja et al., 2019; Ahuja et al., 2020; Shukla et al., 2019; Gahukar and Das, 2020; El-Moneim et al., 2021). All the strategies of fertilizer reduction target achieving healthy soil dynamics. The area of nano-agriculture holds the potential to achieve sustainable growth through smart farming.
On an optimistic note, if all these solutions mentioned above successfully improve soil health, ironically, the benefit will be enjoyed by all the existing flora and the crop. Thus, it is imperative that unless the crop plant has higher metabolic fitness to compete with the surroundings, eventually, it will fall short in the competition. Thus, the challenge is that we could develop a nano-strategy to bolster the metabolic fitness of the crop plant so that it can compete better with the biotic and abiotic stress of the environment. In the present work, we have exploited the role of nano iron pyrite in offering better metabolic fitness to the root system to offer an improved yield. We have experimented on onion (Allium cepa) as a case study since it has a shallow root system and needs nutrients close to the soil surface for healthy growth. Goat manure provides nutrients at the soil surface. So here, we have applied an iron-sulfur nanomaterial as a root treatment agent to boost the root metabolism and used organic manure to supply nutrients-thus following a nano-organic farming approach.
Philosophically, this work draws inspiration from hydrothermal vents’ extreme ecosystem, where anaerobic microbes receive their energy from nano pyrite and other metal sulfides. Earlier, Srivastava et al. discovered that a 12 h seed treatment of the chickpea in an aqueous nano pyrite suspension increased biomass (Srivastava et al., 2014a). In the follow-up field trials on chickpeas (Jangir, 2020a; Jangir et al., 2020c), spinach (Srivastava et al., 2014b), beetroot, carrot, fenugreek, alfalfa, mustard, and sesamum (Das et al., 2016a), we observed higher productivity following pyrite seed treatment. In pyrite-treated rice, an NPK equivalent yield was reported (Das et al., 2018). Jangir et al. recently showed an improved result in wheat crops following nano pyrite seed treatment (Jangir, 2020a; Jangir et al., 2020b). The nano pyrite seed treatment gets nullified by co-treatment with an antioxidant nanomaterial, cerium oxide (Das et al., 2016b). Recently Jangir et al. discovered that upon treating the roots of chili, marigold, cabbage, cauliflower, and tomato in an aqueous suspension of nano pyrite for 3 h, a significant increase in yield was observed (Jangir et al., 2019a; Jangir et al., 2019b; Jangir, 2020a; Jangir and Das, 2021). Further root and seed treatment with nano pyrite improves the plants’ root-foraging ability (Jangir et al., 2019a; Jangir et al., 2019b; Jangir, 2020a; Jangir et al., 2020b; Jangir et al., 2020c; Jangir and Das, 2021).
The current study addresses the synergistic effect of nano pyrite root treatment and organic manure (goat droppings) on onion production (Allium cepa). It has tremendous commercial significance. It is a temperate crop, and India is the highest contributor to onion production after China. Globally it has multiple uses (spice, salad, and green vegetables). The allyl propyl disulfide contributes to onion pungency. For optimal onion production, the soil must be rich in organic matter. Recommended fertilizer dosage is 125:60:100 kg/ha of Nitrogen (N): Phosphorus (P): Potassium (K) (ICAR, 2018; Choudhury, 2020).
Experimental
Experimental layout: The overall field layout of the study is shown in Figure 1.
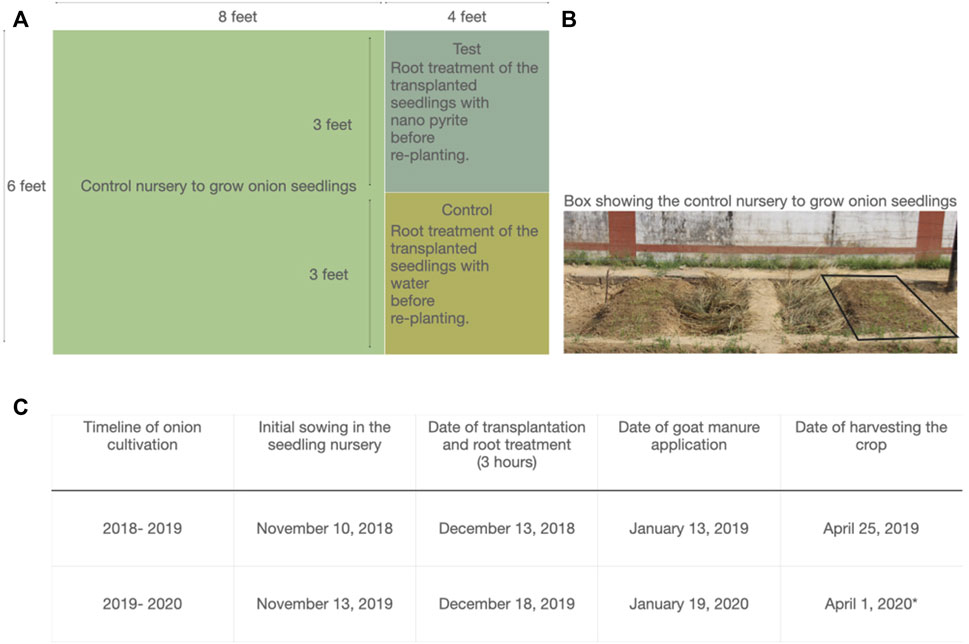
FIGURE 1. Onion cropping protocol. (A) Overall layout. (B) The seedling nursery in the institute. (C) A timeline of cropping followed during the first and second seasons. *Due to the pandemic, the harvesting was performed early.
Nano pyrite synthesis: Nano pyrite synthesis is a two-step reaction. In the first step, we synthesize fresh polysulfide. We take an aqueous sodium hydroxide solution (0.8 M, 100 ml) divided into two equal parts. In one part, we purged hydrogen sulfide gas until it turned yellow-green, and then the remaining half of sodium hydroxide (0.8 M, 100 ml) and 0.04 mol of sulfur were added. The solution was stirred in the presence of hydrogen sulfide for the next 2 h at room temperature to obtain yellow-orange polysulfide suspension.
The suspension was filtered and stored at 4°C. For the second step, fresh aqueous ferric chloride (0.04 M, 100 ml) was prepared in a round bottom flask and maintained at a pH of 5.6 using a buffer of 91 ml sodium acetate (0.2 M, 100 ml) and 9 ml acetic acid (0.2 M, 100 ml). The flask was placed in an oil bath with continuous argon purging and stirred continuously for 1 h at room temperature. To this solution, we slowly add 15 ml of polysulfide. The oil bath temperature remains at 120°C for 2 h, and a dark grey suspension will appear. Then, the oil bath temperature was switched to 180°C for 10 min to obtain a grey-colored suspension. The nano pyrite suspension settles at the bottom of the flask. We wash the rest with hydrochloric acid, toluene, and acetone. We store the nano pyrite particles in a moisture-free chamber (Srivastava et al., 2014a; Srivastava et al., 2014b; Das et al., 2016a; Das et al., 2016b; Das et al., 2018; Jangir et al., 2019a; Jangir et al., 2019b; Jangir, 2020a; Jangir et al., 2020b; Jangir et al., 2020c; Jangir and Das, 2021).
Nano pyrite characterization: Structural features of nano pyrite were observed using X-ray diffraction (XRD) and Scanning electron microscopy (SEM). XRD pattern was obtained to study the characteristic planes of nano pyrite crystal, for which a powdered sample was analyzed using X’Pert3 powder-PANalytical XRD (Malvern Panalytical). SEM and EDS samples were prepared by drop-casting a suspension of nano pyrite in methanol on a copper stub. The sample was gold coated and observed using FEI, Quanta 200 SEM (FEI Company). Fourier Transform Infrared Spectroscopy (FTIR) was performed to investigate the bonding characteristics of the nano pyrite particles. The sample was prepared by making a pellet of nano pyrite powdered with KBr. Perkin-Elmer FTIR spectrum BX spectrometer was employed to obtain the FTIR spectrum. X-ray Photoelectron Spectroscopy (XPS) was performed for powdered samples using PHI 5000 Versa Prob II, FEI (Physical Electronics). XPS was used to study the surface characteristics of nano pyrite particles (Srivastava et al., 2014a; Srivastava et al., 2014b; Das et al., 2016a; Das et al., 2016b; Das et al., 2018; Jangir et al., 2019a; Jangir et al., 2019b; Jangir, 2020a; Jangir et al., 2020b; Jangir et al., 2020c; Jangir and Das, 2021). Figures 2–5 documents the nanoparticle characterization results.
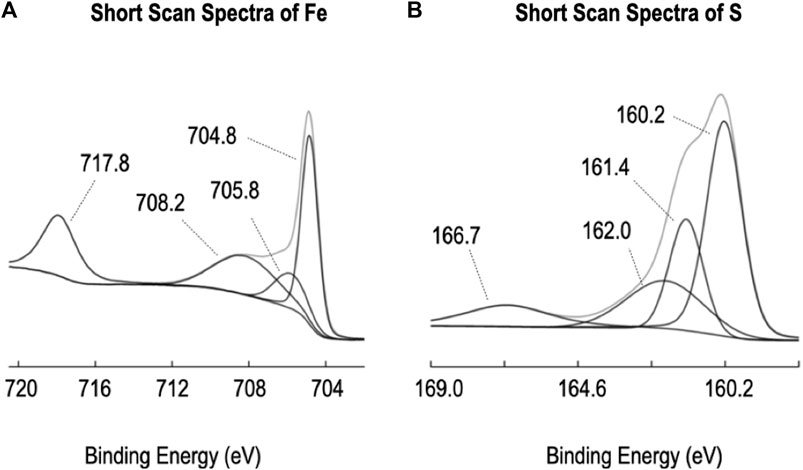
FIGURE 5. Representative XPS of nano pyrite. (A) Short scan XPS spectra of iron. (B) Short scan XPS spectra of sulfur.
Field trial: The field trial included the following steps: land preparation, soil nutrient profiling, Sowing, root treatment, transplantation, manure application, crop care, and harvesting.
Land preparation: We conducted the field trial in the institute nursery of the Indian Institute of Technology Kanpur, India. We selected a nutritionally deficient patch of land adjacent to the nursery’s greenhouse. We first plowed the ground and prepared three plots. Out of the three plots, we maintained the exact dimensions (3*4 ft2) for two plots, while for the third plot, we doubled the length and breadth of the plot (6*8 ft2). We use the third plot (6*8 ft2) as a nursery to grow the onion seedlings before we perform transplantation. For both seasons, the same protocol was used. In Figure 1, we have presented a flow chart of the cropping protocol followed during the 2 years of field trials.
The soil’s nutrient profile: At the beginning of the field trial, we selected a sizeable barren plot where no fertilizers or organic manure was added for many years. We divided the land into two parts for 2 years of field trials. One piece of the land was used for the first year of study, while the other was used for the second year. We perform a soil nutrient analysis for Nitrogen (N), Phosphorus (P), and Potassium (K) of the whole barren plot. During the first year of the trial, we left the other plot completely undisturbed. N was estimated by the Kjeldahl method, and P and K were calculated using ICPMS analysis (Thermo, iCAP Q) as described in our earlier work (Jangir et al., 2019a; Jangir et al., 2019b). In the present study, during the first year of study, a nutritionally deficient patch of land (total nitrogen (158.78 kg/ha), P2O5 (0.366 kg/ha), and K2O (0.380 kg/ha) was selected in the Institute Nursery of Indian Institute of Technology Kanpur, India.
Sowing, root treatment, transplantation, and manure application: The field trial was initiated on November 10, 2018, for season I. 50 mg 'Nasik Red’ variety of onion seeds were germinated in nursery beds (6*8 ft2). After 33 days (December 13, 2018), the germinated seedlings were harvested and were randomly divided into two sets of 100 seedlings each. Next, the root treatment was performed for 3 h before transplantation. In the control group, the roots of seedlings were soaked in water, while in the test group, the roots were soaked in 100 μg/ml aqueous suspension of nano pyrite. After 3 hours of root treatment, the control and test seedlings were transplanted in their respective assigned plots of individual dimensions of 3*4 ft2. The pyrite-treated roots were washed thoroughly under running water to remove any trace of iron pyrite particles. We followed a similar protocol for both seeds and roots to remove the trace amounts of pyrite from seed and root surfaces before introducing them into the soil (Srivastava et al., 2014a; Srivastava et al., 2014b; Das et al., 2016a; Das et al., 2016b; Das et al., 2018; Jangir et al., 2019a; Jangir et al., 2019b; Jangir, 2020a; Jangir et al., 2020b; Jangir et al., 2020c; Jangir and Das, 2021). Goat droppings were used for manuring both plots (1 kg/plot) on January 19, 2019. Goat droppings were collected from the local marginal farmers who rear goats for extra income. Based on the available literature, typically, goat manure comprises ∼22.15 g/kg of total nitrogen, 4.27 g/kg of total phosphorous, and 41.15 g/kg of total potassium (Azeez and Van Averbeke, 2010). Considering the chemical variability in the goat manure, we pooled all the goat manure, mixed them thoroughly, and applied them in both control and test plots from the same lot. The second (season II) cropping season follows a similar calendar, as shown in Figure 1.
Crop care: Recommended irrigations were given, and all other parameters were kept the same for both sets. All the other agronomic practices were followed per the recommendations (ICAR, 2018; Choudhury, 2020).
Harvest: Harvesting was done manually, and the mud was removed and cleaned before weighing the samples.
Crop Analysis and Growth Parameter (2018-2019): The crop was harvested and analyzed for various plant growth parameters such as fresh weight, dry weight, leaf area, specific leaf area, leaf area index, size/area of the bulb, onion bulb weight, etc. (Figures 6–9). The relative anthocyanin and flavonol content were also measured for control and test samples. The anthocyanin content of the bulb is an indicator of the colorations of the bulb and, thus, marketability (Metrani et al., 2020; Samota et al., 2022).
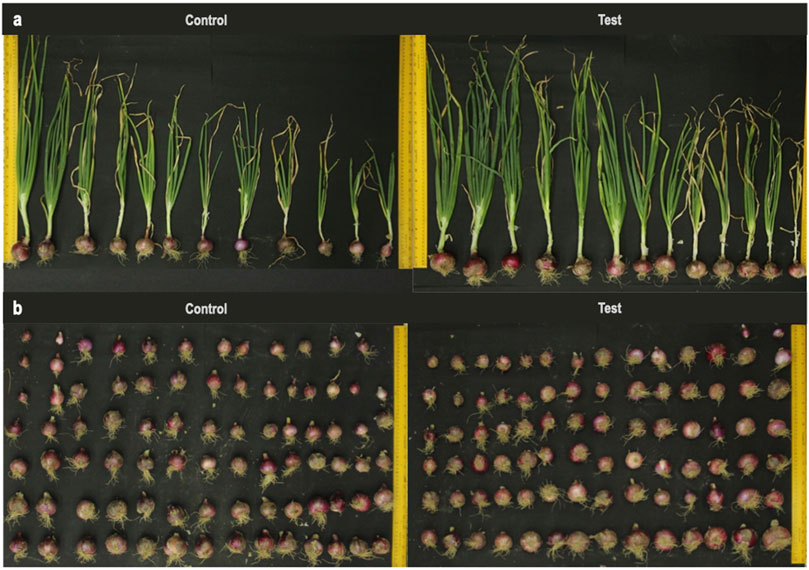
FIGURE 6. Representative image of the control and test sample of the onion harvest during the 2018-2019 season. (A) Representative image of the whole onion plant in the control and test group. (B) The total number of bulbs was obtained from the control and test.
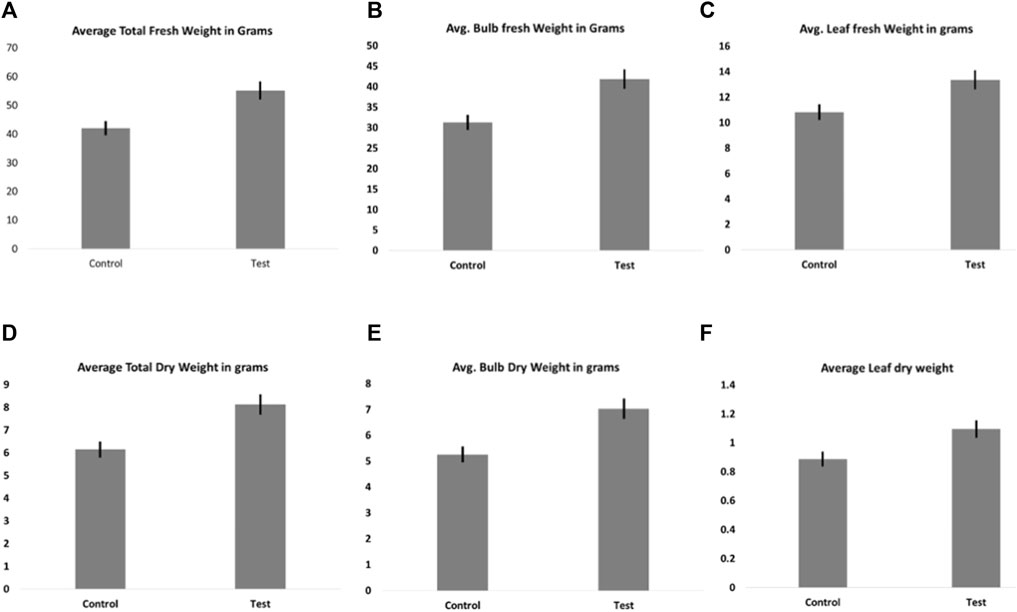
FIGURE 7. The figure shows the average crop yield in foliage and bulb production. Fresh and dry matter for the control (n = 83) and test (n = 86) crops was measured. (A) Average total fresh weight in g. (B) Average bulb fresh weight. (C) Average leaf fresh weight. (D) Average total dry weight in grams. (E) Average bulb dry weight. (F) Average leaf dry weight. Vertical bars denote 5% error bars.
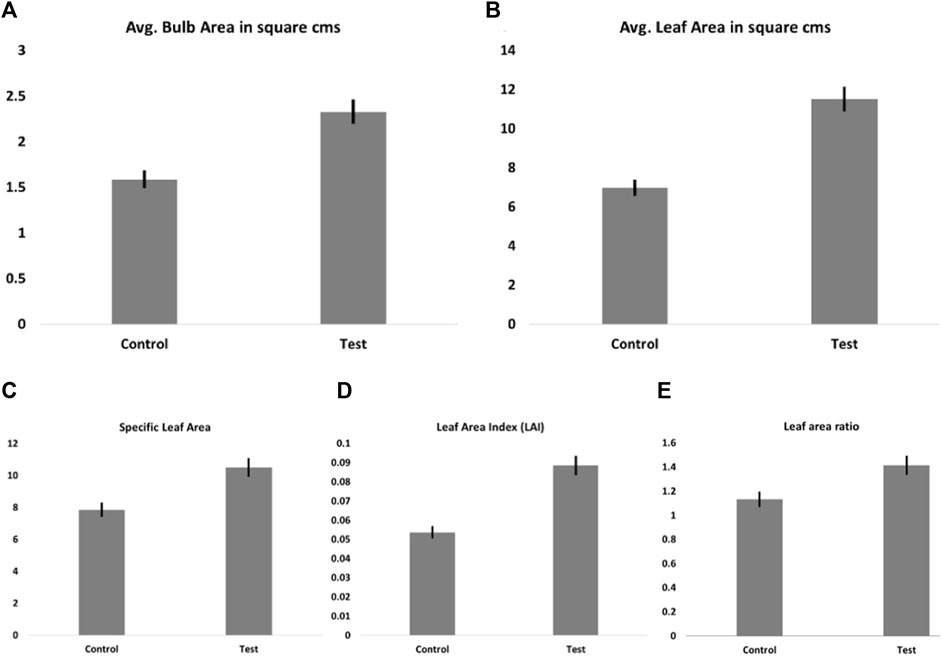
FIGURE 8. The figure shows the leaf area and indicative onion bulb size. Specific leaf area and leaf area index are also offered, which are essential parameters regarding foliage, photosynthetic activity area, and transpiration area available. Vertical bars indicate 5% error bars. Control (n = 12) and test (n = 13). (A) Average bulb area in cm2. (B) Average leaf area in cm2. (C) Specific leaf area. (D) Leaf area index. (E) Leaf area ratio.
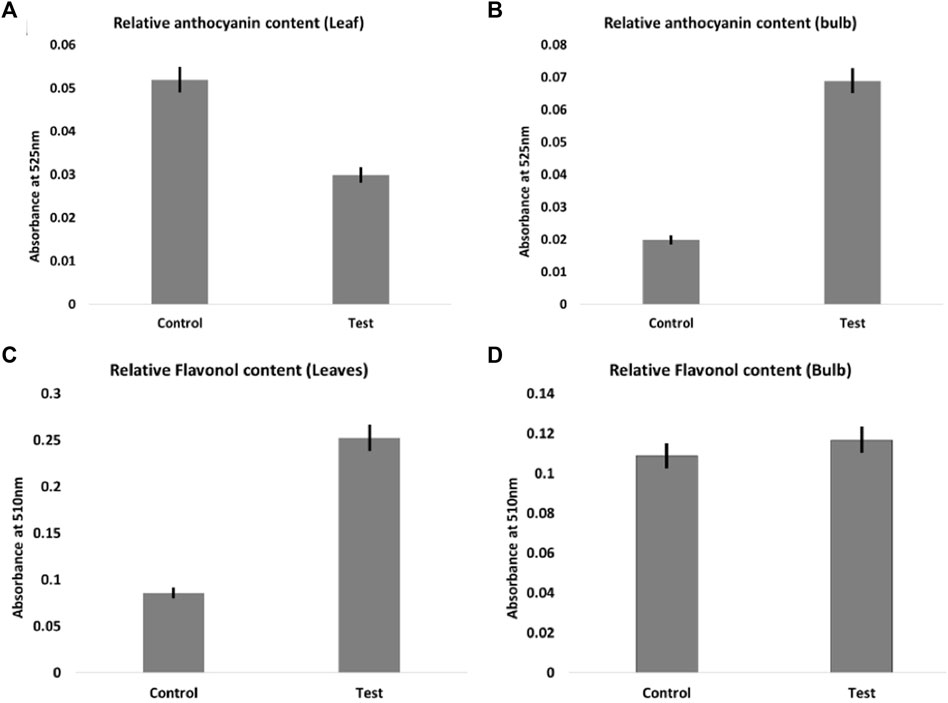
FIGURE 9. The figure shows relative anthocyanin contents in leaves and bulbs. Vertical bars indicate 5% error bars, n = 3. (A) Relative anthocyanin content in leaves. (B) Relative anthocyanin content in onion bulbs. (C) Relative flavonol content in leaves. (D) Relative flavonol content in onion bulbs.
The onion crop yield obtained at harvest was weighed for both control and test groups. Fresh weight was measured for the whole plant (leaves and bulb) and the bulb. The average yield obtained is reported in grams for bulbs and leaves. The weight of the leaves was calculated by subtracting the bulbs’ weight from the whole plant’s weight. Total onion bulbs obtained from control and test groups were counted. The control and test groups chose twelve and thirteen representative plants, respectively. These plants were placed alongside a ruler, and a snapshot was taken. The picture is shown in Figure 6. It was used to calculate the area of leaves and bulbs using ImageJ. The leaf area is a direct measurement of photosynthetic activity and transpiration. Standard errors have been reported for image analysis. The student’s t-test was performed to calculate the significance of the results. Leaves and bulbs were kept at 70°C for 6 h to measure the dry weight of the samples. Once we had primary data, other plant growth parameters were calculated as per standard procedure using the following:
Leaf Area Ratio: It measures the relative size of the assimilatory apparatus. In simpler terms gives an idea of the portion of the plant involved in the photosynthetic activity.
Leaf Area Ratio = Total Leaf Area/Total Dry Weight.
Specific Leaf Area (SLA): SLA signifies the leaf density of the plant. It tells us about the leaf area used to produce unit biomass.
Specific Leaf Area = Total Leaf Area/Leaf Dry Mass.
Leaf Area Index (LAI): A plant’s leaf area index (LAI) is defined as its leaf area per unit of ground area. The leaf area index (LAI) is one of the most critical parameters in plant ecology. It signifies how much foliage (leaf) is present on the plant. It measures the active photosynthetic area and the area available for transpiration. More photosynthetic area means a higher rate of photosynthesis and food production and accumulation. In comparison, higher transpiration rates ensure higher uptake of water and minerals. These two factors provide better nutrition accumulation in edible parts of the plant.
Leaf Area Index = Total Leaf Area/Total Land Area.
The harvested plant materials were further tested for their anthocyanin and flavonol content in both leaves and bulbs. Flavonol is a class of flavonoids that participate in stress responses. These are the most primitive and widespread flavonoids with various plant physiological functions. Anthocyanin is a specific class of plant pigments that belong to flavonoids. These pigments provide bright color to the onion bulb and thus increase the marketability and visual acceptability of the crop produced. The higher anthocyanin content in onion bulbs also signifies earlier maturity, thus helping the farmers get a reasonable price for their early produce. Anthocyanin is also a well-known antioxidant; therefore, a higher anthocyanin content ensures better health (Slimestad et al., 2007; Lin et al., 2016; Martín et al., 2017; Metrani et al., 2020; Samota et al., 2022).
Preparation of plant samples: Plant samples were finely cut into small pieces and homogenized using a pestle and mortar. 2 gm of this sample was mixed with 7 ml of 80% ethanol (0.1% HCl) and further homogenized. The extract was filtered to obtain an alcoholic extract (Lin et al., 2016). The experiments were performed in triplicates.
Flavonol measurement: Relative flavonol content was measured for control and test samples (leaves and bulb) by taking absorbance at 510 nm (Lin et al., 2016).
Anthocyanin measurement: Relative anthocyanin content was measured using the ethanol extract, mixing in equal quantity with buffer (pH 4.5), and taking absorbance at 525 nm (Lin et al., 2016).
Results
Nano pyrite synthesis: Iron pyrite nanoparticles were synthesized as described in our previous work via a low-temperature nucleophilic reaction (Srivastava et al., 2014a; Srivastava et al., 2014b; Das et al., 2016a; Das et al., 2016b; Das et al., 2018; Jangir et al., 2019a; Jangir et al., 2019b; Jangir, 2020a; Jangir et al., 2020b; Jangir et al., 2020c). The reaction involves iron (III) chloride and sodium polysulfide, the chief intermediaries being FeS and FeSH+. These intermediates are further attacked by polysulfide nucleophiles, which leads to the formation of FeS2. The reaction was carried out in an inert environment to prevent interaction with oxygen.
Nano pyrite characterization: In Figure 2, the nano FeS2 characterization has been shown. XRD peaks at 2θ = 28.8, 33.1, 37.2, 40.7, 47.5, 56.3, 61.5, 64.2, 79.0 correspond to characteristic crystal planes of iron pyrite with indices (111), (200), (210), (211), (220), (311), (302), (321), and (420), respectively (Figure 2A). Nanosized iron pyrite flakes can be seen in the SEM image. These flakes assemble to form large pitcher-shaped structures ranging from 70 nm to more than 500 nm (Figure 2B).
EDS was also performed along with the SEM analysis. Figures 3A, B show the EDS analysis. Figure 3A shows the EDS overlay with the SEM image, and Figure 3B shows the EDS spectrum for nano iron pyrite.
FTIR peaks at 400-800 cm−1 marked pyrite bonding characteristics, the peak at 1076.5 cm−1 showed S-O bonding characteristics marking surface oxidation, and the presence of moisture was marked by peaks at 1623.8 cm−1 and 3407.6 cm−1 (Figure 4). The specific peak at 3407.6 cm−1 highlights the O–H vibration (Khataee et al., 2016).
Fe2p narrow XPS spectrum marked the presence of Fe2p3/2 and Fe2p1/2 spin-orbital coupling at 704.8 and 717.8 eV, respectively, along with signatures of stoichiometric defects and FeSO4 at 705.8 and 708.2 eV, respectively (Figure 5A). In the S2p narrow XPS spectrum, peaks at 160.2 and 161.4 eV depicted S2p3/2 and S2p1/2 spin-orbit coupling, respectively. XPS peaks at 162.0 and 166.7 eV marked the presence of surface defects and oxidation causing the formation of FeSO4, respectively (Figure 5B). Our earlier work found similar results (Srivastava et al., 2014a; Srivastava et al., 2014b; Das et al., 2016a; Das et al., 2016b; Das et al., 2018; Jangir et al., 2019a; Jangir et al., 2019b; Jangir, 2020a; Jangir et al., 2020b; Jangir et al., 2020c; Jangir and Das, 2021).
Field trial: Nutrient profile of the soil: In the present study, a nutritionally deficient patch of land was selected in the Institute Nursery of Indian Institute of Technology Kanpur, India. The NPK analysis is shown in the method section. In earlier work, it has been shown that the nitrogen, phosphorous, and potassium levels mentioned above are considered nutritionally deficient (Jangir et al., 2020b). As mentioned earlier in the methods section, the selected plot was divided into two equal parts, and randomly, one plot was assigned for the test. In contrast, the other was assigned for the control experiment. The detailed plot sizes are documented in the methods section.
Crop Analysis (2018-2019). In Figure 6A, representative pictures of the control (n = 12) and the test (n = 13) bulbs obtained at the time of harvest are shown (2018-2019 season). Visual observations show comparatively denser foliage in test plants compared to the control. Figure 6B shows the total bulb harvested from the control (n = 83) and the test (n = 86) crops. Initially, 100 seedlings were sown for both the control and test groups.
In the first-year field trial (November 2018- April 2019), the total biomass (bulb + leaf) weight of the test sample was 4.75 kg (n = 86), while control samples weighed 3.5 kg (n = 83). The total bulb weight for the control and test was 2.6 and 3.6 kg, respectively. In the second-year field trial (December 2019- April 2020), the total biomass of the test sample was 2.65 kg (n = 64), while control samples weighed 2.30 kg (n = 64).
A detailed crop analysis (2018-2019) is shown in Figures 7A–F, Figures 8A–E, Figures 9A–D. The test samples resulted in higher productivity in terms of fresh weight in comparison to untreated plants. The average fresh weight of the whole plant (leaves and bulb) for control samples was 42.16 g, while the test group had an average fresh weight of 55.23 g (Figure 7A). The leaves and bulbs were weighed separately to investigate the weight distribution between foliage and the economic yield. The average bulb weight for test samples was significantly higher (41.86 gm) as compared to the control (31.32 gm) (Figure 7B). Similarly, the average fresh weight of test leaves (13.37 gm) was higher in comparison to control leaves (10.84 gm) (Figure 7C).
Average water accumulation in both control and test plants was approximately equal. Since the fresh weight of the test samples was high, the average dry weight of the test plants (8.13 gm) was also more as compared to the control (6.158) untreated plants (Figure 7D). Approximately equal moisture content was found in both test and control sample bulbs and leaves. The average dry weight of test bulbs (7.04 gm) was higher as compared to the control onion bulb samples (5.26 gm) (Figure 7E). The average dry weight of the control leaves was 0.889 gm, while the test leaves had an average dry weight of 1.096 gm (Figure 7F). Like the leaves, the bulbs also accumulated approximately the same amount of water for both control and test samples.
The average bulb area for test samples (2.33 cm2) was significantly higher as compared to control samples (1.59 cm2) (Figure 8A). Notably, denser leaf foliage was observed in test samples with an average leaf area of (11.53 cm2) as compared to the control (6.99 cm2) (Figure 8B). The increase in leaf area resulted in significantly higher specific leaf area (10.51) (Figure 8C), leaf area index (0.08) (Figure 8D), and leaf area ratio (1.4) (Figure 8E) in test samples as compared to control (7.86, 0.05, and 1.1 respectively).
The relative anthocyanin content in control leaves (0.052) was considerably higher as compared to test leaves (0.03) (Figure 9A). However, there is a highly significant increase in the anthocyanin content in test bulbs (0.069) as compared to control bulbs (0.02) (Figure 9B). The results indicate a selective partitioning of the pigment in leaves and bulbs. The test plants tend to accumulate significantly higher amounts of pigment in the food storage/edible parts. The relative flavonol content in test leaves (0.253) (Figure 9C) was found to be significantly higher concerning control samples (0.086). On the other hand, the flavonol content in the bulb (Figure 9D) was approximately equal in both control and test samples.
Discussion
As mentioned earlier that philosophically, this work is inspired by an extreme ecosystem, viz., hydrothermal vents in the deep ocean rifts of the Pacific, where microbes like archaebacteria and thiobacillus receive their energy from nano pyrite and other metal sulfides. The fundamental hypothesis for using nano pyrite pivots around an exothermic process, whereby metal sulfides are converted to sulfates to liberate 200 kcal/mol of energy (Tributsch, 1984). This kinetics is further accelerated when metal sulfides are covered with a thin film of water, approximately 4% of their weight, in the presence of oxygen (Tributsch, 1984). Subsequent reduction of oxygen leads to the release of trace amounts of H2O2, which further attacks additional sulfide molecules resulting in the generation of Fe2+, Fe3+, SO42−, and S0. So, when plant roots are treated in aqueous pyrite suspension, they are briefly exposed to iron, sulfur, pyrite, peroxide, and a proton-rich environment. These species orchestrate metabolic changes that enhance plant metabolism. In other words, this treatment results in metabolically more competent plants (Jangir et al., 2019a; Jangir et al., 2019b; Jangir, 2020a; Jangir and Das, 2021). Here, we tested the feasibility of this root treatment in plants with shallow root systems to draw nutrients efficiently from organic matter. The overall premise of the work is summarised in Figure 10.
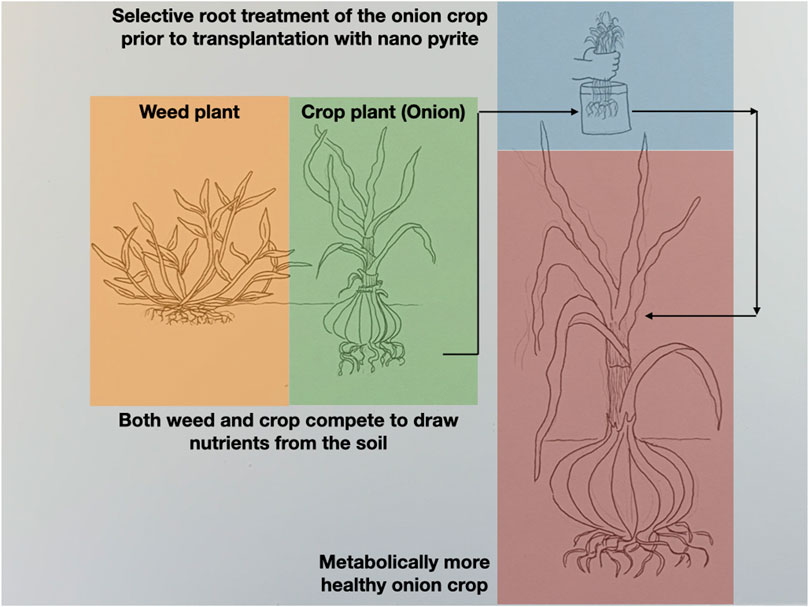
FIGURE 10. The figure depicts the overall outline of the work and the basic philosophy of root treatment with nano pyrite before transplantation. The nano pyrite root-treated plants are metabolically more active.
The experiment was purposefully conducted in a nutritionally deficient patch of land to study the dual effect of root treatment and organic manure, thus, closely simulating a sustainable organic farm environment with a nano intervention. We chose goat dropping as an organic supplement as it has high organic matter, nitrogen, and phosphorus contents and indirectly impacts fertilizer mobilization and biological cycling. Also, the total nitrogen mineralization over one-twenty days is the maximum for goat droppings compared to poultry and cattle (Gichangi et al., 2009; Gichangi et al., 2010; Azeez and Van Averbeke, 2010; Rizk et al., 2012). Further, goat manure is commonly used as a liquid fertilizer source (Sunaryo et al., 2018).
It was observed that organic manure solely could not suffice for maximum onion productivity. This could be due to the shallow root system of onion crops with lesser root penetrability. Onion crop requires nutrient application close to the root system, so we applied goat manure on the soil surface (Gichangi et al., 2009; Azeez and Van Averbeke, 2010; Gichangi et al., 2010; Rizk et al., 2012). Here, by pre-treating onion seedlings with nano pyrite before manure application, we demonstrated that boosting the metabolism of onion seedlings in the test group led to better nutrient acquisition and higher yield than the control group. Higher leaf area, specific leaf area, leaf area ratio, and leaf area index indicate a higher photosynthetic rate and higher transpiration. All these factors may have contributed to an increase in the size of the onion bulbs of test plants. More giant bulbs ensure a better market and price for the farmer.
An increase in anthocyanin content in bulbs indicates that the economic yield is brighter colored and has matured earlier. It also shows that the test yield shall have better marketability and may sell at a higher price. Higher flavonol content in test leaves ensures better stress response, particularly to UV-B radiation. Flavonols play a vital role in pollen germination and, thus, plant fertility (Falcone Ferreyra et al., 2012). The results indicate that the test plants have significantly higher flavonols and therefore are less prone to UV stress; the plants may have higher fertility and, therefore, higher seed production capability (Slimestad et al., 2007; Lin et al., 2016; Martín et al., 2017; Metrani et al., 2020; Samota et al., 2022).
In summary, on treatment with an aqueous suspension of nano pyrite, the root experiences a brief energy-rich microenvironment, which shapes its subsequent metabolism and growth. These metabolically boosted plants acquire nutrients efficiently from organically supplemented soil and hence have higher competitive fitness. This approach is feasible to open up avenues for nano-organic sustainable farming and further boost the economy of goat farmers of Asian and African continents.
Conclusion
While sustainable farming aims at reducing chemical fertilizers by using organic manure, bio-fertilizers, and nano fertilizers, a crop-specific increase in metabolic fitness could positively influence the yield. Here we show that a nano pyrite root treatment during transplantation could boost the metabolic fitness of the plant so that it can capitalize on the maximum benefits derived from organic farming, thus leading to a novel nano-organic farming strategy.
Data availability statement
The original contributions presented in the study are included in the article/supplementary material, further inquiries can be directed to the corresponding author.
Author contributions
HJ: Conceptualization, Data-curation, Formal Analysis, Investigation, Methodology, Validation, Writing–original draft, Writing–review and editing. BK: Investigation, Writing–review and editing. GS: Data-curation, Investigation, Writing–review and editing. MD: Conceptualization, Data-curation, Funding acquisition, Investigation, Methodology, Project administration, Resources, Supervision, Validation, Visualization, Writing–original draft, Writing–review and editing.
Funding
The author(s) declare financial support was received for the research, authorship, and/or publication of this article. MHRD, GOI, funds HJ. Part of the funding for the project is obtained from STARS MHRD, GOI project of MD, STARS1/626.
Acknowledgments
MHRD GOI funds HJ, and the work is integral to HJ doctoral thesis. The authors are thankful to Mr. D. D. Pal for XPS, Mr. Mitesh for FESEM, Mr. A. Tiwari for the XRD facility, Ms. V. Singh for FTIR at Advance Centre for Material Science, IIT Kanpur, A. Singh for providing the ICPMS facility; Mr. Satish Singh for providing Institute Nursery facility.
Conflict of interest
The authors declare that the research was conducted in the absence of any commercial or financial relationships that could be construed as a potential conflict of interest.
Publisher’s note
All claims expressed in this article are solely those of the authors and do not necessarily represent those of their affiliated organizations, or those of the publisher, the editors and the reviewers. Any product that may be evaluated in this article, or claim that may be made by its manufacturer, is not guaranteed or endorsed by the publisher.
References
Ahuja, R., Sidhu, A., and Bala, A. (2020). Manganese sulfide nanospheres as mycocidal material and priming agent for fungi-infested rice seeds. J. Phytopathology 168, 678–687. doi:10.1111/jph.12948
Ahuja, R., Sidhu, A., and Bala, A. (2019). Synthesis and evaluation of iron (ii) sulfide aqua nanoparticles (FeS-NPs) against Fusarium verticillioides causing sheath rot and seed discoloration of rice. Eur. J. Plant Pathol. 155, 163–171. doi:10.1007/s10658-019-01758-3
Andreas, G., Adrian, M., Matthias, H., Colin, S., Andreas, F., Nina, B., et al. (2012). Enhanced top soil carbon stocks under organic farming. Proc. Natl. Acad. Sci. 109, 18226–18231. doi:10.1073/pnas.1209429109
Azeez, J. O., and Van Averbeke, W. (2010). Nitrogen mineralization potential of three animal manures applied on a sandy clay loam soil. Bioresour. Technol. 101, 5645–5651. doi:10.1016/j.biortech.2010.01.119
Bhati, A., Tripathi, K. M., Singh, A., Sarkar, S., and Sonkar, S. K. (2018). Exploration of nano carbons in relevance to plant systems. New J. Chem. 42, 16411–16427. doi:10.1039/C8NJ03642J
Boutchuen, A., Zimmerman, D., Aich, N., Masud, A. M., Arabshahi, A., and Palchoudhury, S. (2019). Increased plant growth with hematite nanoparticle fertilizer drop and determining nanoparticle uptake in plants using multimodal approach. J. Nanomater 2019, 1, 11. doi:10.1155/2019/6890572
Crowder, D. W., and Reganold, J. P. (2015) Financial competitiveness of organic agriculture on a global scale. Proc. Natl. Acad. Sci. U. S. A;112(24):7611–7616. doi:10.1073/pnas.1423674112
Das, C. K., Jangir, H., Kumar, J., Verma, S., Mahapatra, S. S., Philip, D., et al. (2018). Nano-pyrite seed dressing: A sustainable design for NPK equivalent rice production. Nanotechnol. Environ. Eng. 3, 14. doi:10.1007/s41204-018-0043-1
Das, C. K., Srivastava, G., Dubey, A., Roy, M., Jain, S., Sethy, N. K., et al. (2016a). Nano-iron pyrite seed dressing: A sustainable intervention to reduce fertilizer consumption in vegetable (beetroot, carrot), spice (fenugreek), fodder (alfalfa), and oilseed (mustard, sesamum) crops. Nanotechnol. Environ. Eng. 1, 2. doi:10.1007/s41204-016-0002-7
Das, C. K., Srivastava, G., Dubey, A., Verma, S., Saxena, M., Roy, M., et al. (2016b). The seed stimulant effect of nano iron pyrite is compromised by nano cerium oxide: regulation by the trace ionic species generated in the aqueous suspension of iron pyrite. RSC Adv. 6, 67029–67038. doi:10.1039/c6ra15584g
Delwiche, C. C. (1970). The nitrogen cycle. Sci. Am. 223, 136–146. doi:10.1038/scientificamerican0970-136
Douglas, B., Andrew, F., Emma, J., Louise, M., Caroline, M., Jessica, P., et al. (2018). Agricultural origins on the Anatolian plateau. Proc. Natl. Acad. Sci. 115, E3077–E3086. doi:10.1073/pnas.1800163115
El-Moneim, D. A., Dawood, M. F. A., Moursi, Y. S., Farghaly, A. A., Afifi, M., and Sallam, A. (2021). Positive and negative effects of nanoparticles on agricultural crops. Nanotechnol. Environ. Eng. 6, 21–11. doi:10.1007/s41204-021-00117-0
Falcone Ferreyra, M. L., Rius, S., and Casati, P. (2012). Flavonoids: biosynthesis, biological functions, and biotechnological applications. Front. Plant Sci. 3, 1. . doi:10.3389/fpls.2012.00222
Food & Agriculture Organization (FAO) of the United Nations (2017). World fertilizer trends and outlook to 2020. Available at: https://www.fao.org/3/i6895e/i6895e.pdf.
Gahukar, R. T., and Das, R. K. (2020). Plant-derived nanopesticides for agricultural pest control: challenges and prospects. Nanotechnol. Environ. Eng. 5, 3–9. doi:10.1007/s41204-020-0066-2
Gichangi, E. M., Mnkeni, P. N. S., and Brookes, P. C. (2009). Effects of goat manure and inorganic phosphate addition on soil inorganic and microbial biomass phosphorus fractions under laboratory incubation conditions. Soil Sci. Plant Nutr. 55, 764–771. doi:10.1111/j.1747-0765.2009.00415.x
Gichangi, E. M., Mnkeni, P. N. S., and Brookes, P. C. (2010). Goat manure application improves phosphate fertilizer effectiveness through enhanced biological cycling of phosphorus. Soil Sci. Plant Nutr. 56, 853–860. doi:10.1111/j.1747-0765.2010.00515.x
Gignoux, C. R., Henn, B. M., and Mountain, J. L. (2011). Rapid, global demographic expansions after the origins of agriculture. Proc. Natl. Acad. Sci. 108, 6044–6049. doi:10.1073/pnas.0914274108
ICAR (2018). Area, production and productivity of onion in India. Available at: http://dogr.res.in/index.php/en/download/20-downloads/69-statistic.
Jangir, H., Bharadwaj, A., Srivastava, G., and Das, M. (2020b). Fertilizer-free cultivation of wheat in nutrient-deficient soil by treating the seeds with nanopyrite. Nanotechnol. Environ. Eng. 5, 9. doi:10.1007/s41204-020-00072-2
Jangir, H., Bhardwaj, A., and Das, M. (2020c). Larger root nodules increased Fe, Mo, Mg, P, Ca, Mn, K in the roots and higher yield in chickpea grown from nano FeS2 pre-treated seeds: emulating nitrogenase. Appl. Nanosci. 10, 445–454. doi:10.1007/s13204-019-01238-4
Jangir, H., Bhardwaj, A., Srivastava, G., and Das, M. (2019a). Nano pyrite driven root foraging increases production of the heavy feeders, viz., cauliflower, cabbage and tomato in nutrient deficient soil with no fertiliser application. Adv. Nat. Sci. Nanosci. Nanotechnol. 10, 035007. doi:10.1088/2043-6254/ab38b4
Jangir, H., Das, C. K., Kumar, J., Mahapatra, S. S., Srivastava, G., Bhardwaj, A., et al. (2019b). Nano pyrite (FeS2) root priming enhances chilli and marigold production in nutrients-deficient soil: A nano strategy for fertilizer tuning. Appl. Nanosci. 9, 327–340. doi:10.1007/s13204-018-00943-w
Jangir, H., and Das, M. (2021). “A journey of nano iron pyrite from the chemosynthetic world of hydrothermal vents to the photosynthetic world of agricultural fields: A new class of seed and root bio-stimulant,” in Nanotechnology in sustainable agriculture (China: CRC Press), 249–270. eBook ISBN: 9780429352003.
Jangir, H. (2020a). Exploring sulfides to design strategies for sustainable agriculture and green energy. Available at: http://etd.iitk.ac.in:8080/jspui/handle/123456789/18922.
Khataee, A., Gholami, P., Sheydaei, M., Khorrram, S., and Joo, S. W. (2016) Preparation of nanostructured pyrite with N2 glow discharge plasma and the study of its catalytic performance in the heterogeneous Fenton process. New J. Chem., 40, 5221–5230. doi:10.1039/C5NJ03594E
Kravchenko, A. N., Snapp, S. S., and Robertson, G. P. (2017). Field-scale experiments reveal persistent yield gaps in low-input and organic cropping systems. Proc. Natl. Acad. Sci. 114, 926–931. doi:10.1073/pnas.1612311114
Lin, S., Zhang, P., Deng, Y., Xu, A., Lu, S., Wu, C., et al. (2016) Quantification and analysis of anthocyanin and flavonoids compositions, and antioxidant activities in onions with three different colors. Int. J. Integr. Agric. 9:2175–2181. doi:10.1016/S2095-3119(16)61385-0
Loukas, B., Fa-Hu, C., Hui, W., Thomas, P. G., and Robert, L. B. (2009). Agricultural origins and the isotopic identity of domestication in northern China. Proc. Natl. Acad. Sci. 106, 5523–5528. doi:10.1073/pnas.0809960106
Metrani, R., Singh, J., Acharya, P. K., Jayaprakasha, G., and Patil, S. B. (2020). Comparative metabolomics profiling of polyphenols, nutrients and antioxidant activities of two red onion (Allium cepa L). Cultiv. Plants 9, 1077. doi:10.3390/plants9091077
Martín, J., Navas, M. J., Asuero, A. M. J.-M., and Asuero, G. (2017). “Anthocyanin pigments: importance, sample preparation and extraction,” in Phenolic compounds. Editors M. Soto-Hernandez, M. Palma-Tenango, and M. del R. Garcia-Mateos (New York: Rijeka: IntechOpen). Ch. 5. doi:10.5772/66892
Pingali, P. L. (2012). Green revolution: impacts, limits, and the path ahead. Proc. Natl. Acad. Sci. 109, 12302–12308. doi:10.1073/pnas.0912953109
Raliya, R., Saharan, V., Dimkpa, C., and Biswas, P. (2018). Nanofertilizer for precision and sustainable agriculture: current state and future perspectives. J. Agric. Food Chem. 66, 6487–6503. doi:10.1021/acs.jafc.7b02178
Rigby, D., and Cáceres, D. (2001). Organic farming and the sustainability of agricultural systems. Agric. Syst. 68, 21–40. doi:10.1016/S0308-521X(00)00060-3
Rizk, F. A., Shaheen, A. M., El-Samad, E. H. A., and Sawan, O. M. (2012). Effect of different nitrogen plus phosphorus and sulphur fertilizer levels on growth, yield and quality of onion (AIlium cepa L). J. Appl. Sci. Res., 8(7): 3353–3361. 1819-544X.
Samota, M. K., Sharma, M., Kaur, K., Sarita, L., Yadav, D. K., Pandey, A. K., et al. (2022) Onion anthocyanins: extraction, stability, bioavailability, dietary effect, and health implications. Front. Nutr. 9:917617. doi:10.3389/fnut.2022.917617
Shukla, P., Chaurasia, P., Younis, K., Qadri, O. S., Faridi, S. A., and Srivastava, G. (2019). Nanotechnology in sustainable agriculture: studies from seed priming to post-harvest management. Nanotechnol. Environ. Eng. 4, 11. doi:10.1007/s41204-019-0058-2
Slimestad, R., Fossen, T., and Vagen, I. M. (2007) Onions: A source of unique dietary flavonoids. J. Agric. Food Chem. 55:10067–10080. doi:10.1021/jf0712503
Sonkar, S. K., Roy, M., Babar, D. G., and Sarkar, S. (2012). Water soluble carbon nano-onions from wood wool as growth promoters for gram plants. Nanoscale 4, 7670–7675. doi:10.1039/C2NR32408C
Srivastava, G., Das, A., Kusurkar, T. S., Roy, M., Airan, S., Sharma, R. K., et al. (2014a). Iron pyrite, a potential photovoltaic material, increases plant biomass upon seed pretreatment. Mater. Express 4, 23–31. doi:10.1166/mex.2014.1139
Srivastava, G., Das, C. K., Das, A., Singh, S. K., Roy, M., Kim, H., et al. (2014b). Seed treatment with iron pyrite (FeS2) nanoparticles increases the production of spinach. RSC Adv. 4, 58495–58504. doi:10.1039/c4ra06861k
Sunaryo, Y., Purnomo, D., Darini, M. T., and Cahyani, V. R. (2018). “Nutrients content and quality of liquid fertilizer made from goat manure,” in Journal of physics: Conference series (Germany: IOP Publishing). doi:10.1088/1742-6596/1022/1/01205312053
Tributsch, H. (1984). “Metal sulfides in photovoltaic, photoelectrochemical and solar biological energy conversion,” in Studies in inorganic chemistry (Germany: Elsevier), 277–310. doi:10.1016/B978-0-444-42355-9.50019-0
Tripathi, S., and Sarkar, S. (2015). Influence of water soluble carbon dots on the growth of wheat plant. Appl. Nanosci. 5, 609–616. doi:10.1007/s13204-014-0355-9
Tripathi, S., Sonkar, S. K., and Sarkar, S. (2011). Growth stimulation of gram (Cicer arietinum) plant by water soluble carbon nanotubes. Nanoscale 3, 1176–1181. doi:10.1039/c0nr00722f
Keywords: nano agriculture, iron pyrite, onion (Allium cepa L), organic farming, goat manure, nano-organic farming, root treatment, anthocyanin
Citation: Jangir H, Kaler B, Srivastava G and Das M (2023) Sustainable nano-interventions to enhance crop yield, anthocyanin content, and marketability of onion (Allium cepa). Front. Nanotechnol. 5:1256439. doi: 10.3389/fnano.2023.1256439
Received: 10 July 2023; Accepted: 01 August 2023;
Published: 14 August 2023.
Edited by:
Kaiser Younis, Integral University, IndiaReviewed by:
Suresh Kaushik, Indian Agricultural Research Institute (ICAR), IndiaSumit Sonkar, Malaviya National Institute of Technology, Jaipur, India
Copyright © 2023 Jangir, Kaler, Srivastava and Das. This is an open-access article distributed under the terms of the Creative Commons Attribution License (CC BY). The use, distribution or reproduction in other forums is permitted, provided the original author(s) and the copyright owner(s) are credited and that the original publication in this journal is cited, in accordance with accepted academic practice. No use, distribution or reproduction is permitted which does not comply with these terms.
*Correspondence: Mainak Das, mainakd@iitk.ac.in