- 1Department of Mechanical Engineering, SGT University, Gurugram, India
- 2B. K. Mody Government Pharmacy College, Gujarat Technological University, Rajkot, Gujarat, India
- 3Department of Chemistry, Faculty of Science, Chulalongkorn University, Bangkok, Thailand
- 4Department of Food Technology, SRM University, Sonipat, Haryana, India
- 5Faculty of Pharmaceutical Sciences, The ICFAI University, Himachal Pradesh, Baddi, India
- 6Shree S. K. Patel College of Pharmaceutical Education and Research, Ganpat University, Mehsana, Gujarat, India
- 7Department of Pharmaceutical Sciences, Faculty of Pharmacy, Chiang Mai University, Chiang Mai, Thailand
- 8Dr. Dayaram Patel Pharmacy College, Bardoli, India
Biogenic metallic nanoparticles (BMNPs) are nanostructure materials synthesized through biological processes that have gained significant attention due to their small size and high surface area-to-volume ratio. BMNPs have several advantages over chemically synthesized ones due to their eco-friendly synthesis regimen, sustainability, biocompatibility, and diverse multifarious biomedical applications. Moreover, the superior cytocompatibility and stability due to the capping layer over metallic nanoparticles (MNPs), reduces the like hood of toxicity and side effects, making them a safer alternative to traditional drug delivery methods. Among several promising applications of BMNPs, their antibacterial activity, analytical sensing of heavy metals, and their roles in food preservations have been widely explored. In addition, to drug delivery and imaging, BMNPs have also been investigated for therapeutic activity such as antimicrobial efficacy against the skin and soft tissue nosocomial pathogens and targeting cancer cells in cancer therapy. The present review bestows several characterization techniques involved with MNPs and compressive aspects of the biogenic synthesis of MNPs using agricultural and biological materials, which reduces the cost of synthesis and minimizes the use of hazardous chemicals. The review also focuses on the multifold applications of BMNPs including biomedical, analytical, preservation of food, and in other consumable goods with toxicological aspects.
Introduction
Green synthesis of MNPs involves the use of natural or renewable resources as reducing and stabilizing agents instead of traditional chemical methods that can be harmful to the environment. Several technological based biogenic syntheses of MNPs have been reported including plant extracts, microorganisms, solar radiation, and green chemistry (Nwabor et al., 2020). Plant extracts have been widely explored in reduction of metal ions due to the significant presence of secondary metabolites such as flavonoids, alkaloids, and phenolics that are effective in reducing and stabilization of MNPs in varied shapes and sizes (Nwabor et al., 2021a). The ability of microorganisms to produce enzymes and metabolites imbues them with versatile precursors in metal ions reduction. The MNPs synthesized using microorganisms are generally more stable due to the presence of proteins and polysaccharides on their surface (Eze and Nwabor, 2020). Recently solar radiation has been reported in effective reduction and stabilization of metal ions. Whereas green chemistry involves various technology such as microwave-assisted, sonochemical synthesis, and mechanochemical synthesis that are eco-friendly and less harmful to the environment (Seku et al., 2018).
MNPs are tiny particles of metals with dimensions on the nanometer scale, typically ranging from 1 to 100 nm. These NPs have unique physicochemical properties due to their small size, large surface area, and quantum confinement effects. Several BMNPs reported such as silver nanoparticles (AgNPs), gold nanoparticles (AuNPs), iron oxide nanoparticles (FeONPS), copper nanoparticles (CuNPs), zinc oxide nanoparticles (ZnNPs), titanium dioxide nanoparticles (TiO2NPs), etc., have found numerous applications in various fields such as medicine, electronics, catalysis, and energy, among others (Salem and Fouda, 2021; Al-Zahrani et al., 2022a; Salem, 2022a; Abdelghany et al., 2023; Shehabeldine et al., 2023; Soliman et al., 2023). AgNPs and CuNPs exhibit unique optical, electrical, catalytic, and antibacterial properties which make them useful for multiple applications (An and Somorjai, 2012). AuNPs exhibit excellent surface plasmon resonance, and are thus very useful in biomedical applications such as cancer diagnosis and treatment, drug delivery, and in cell imaging (Jayeoye et al., 2021a). Additionally, AuNPs have also been used in electronics as conductive materials, catalysts for various chemical reactions, and in sensing heavy metals. FeONPs exhibit unique magnetic properties that make them useful in biomedical applications such as magnetic resonance imaging (MRI), drug delivery, and hyperthermia (Desai et al., 2023). Moreover, FeONPs have found immense applications in environmental remediation as catalysts for the degradation of organic pollutants and in energy storage as electrode materials for batteries and supercapacitors (Hammad et al., 2022; Saied et al., 2022). While, TiO2NP and ZnNPs offer unique properties of ultraviolet absorption and fluorescence, which make them useful in biomedicine as imaging materials, fabrication of sunscreen UV-protective cosmetics, and in electronics as optoelectronic devices (Shahanaz and Shalini, 2023). The BMNPs offer a sustainable and eco-friendly alternative to traditional chemical methods, which produce toxic MNPs. Furthermore, these methods not only reduce the use of toxic chemicals but also utilize natural resources to synthesize MNPs of desired size and shapes with reduced toxic effects. A recent review detailed the in vitro and in vivo toxicology reports of biogenic synthesis of metal oxide to MNPs, indicating toxicity analysis play a key role in selecting non-toxic NPs with unique biological properties (Andra et al., 2019). The present review focuses on biogenic synthesis of metal ions and the applications of MNPs in biomedical, analytical sensing, food preservation, coating of surgical devices, and other consumable goods. Moreover, the review to briefly discussion on BMNPs for characterization, properties, and their toxicological aspects reported in various study.
Approaches in synthesis of MNPs
There are various approaches incorporated in the synthesis of MNPs including chemical reduction, electrochemical, physical, biosynthesize (Saied et al., 2021; Salem, 2022b), microwave assisted (Abdelmoneim et al., 2022), photochemical (Al-Rajhi et al., 2022; Saied et al., 2022), and green synthesis (Abdelaziz et al., 2022; Alsaiari et al., 2023). The chemical approach for synthesizing MNPs involves the use of chemical reactions to convert metal ions into NPs. The chemical approach in synthesizing MNPs typically involves three main steps. In the first step, a solution of metal ions is prepared, which can be obtained from a variety of sources, such as metal salts or metal complexes, which serve as the starting materials for the synthesis of the MNPs. Later, the solution of metal ions is mixed with a reducing agent, which may be a chemical compound of biological fluid that donate electrons to the metal ions. The reducing agent donates electrons to the metal ions, causing them to reduce and form MNPs. In the end, the reaction mixture is heated to a temperature that is appropriate for the synthesis of the MNPs. The temperature of the reaction mixture and duration of reduction time determines the size and shape of the MNPs, as well as their purity and stability. The reaction mixture is typically heated to a temperature of between 100ºC and 200°C, depending on the type of metal ions and the reducing agent used (Jayeoye et al., 2021b). Electrochemical synthesis involves the use of electric current to reduce the metal ions to form MNPs.
The physical approach for synthesizing MNPs involves the use of physical processes, such as thermal decomposition or laser ablation, to convert metal precursors into MNPs. In particular, the physical approach is well-suited for the synthesis of NPs for drug delivery, as it can produce NPs with well-defined sizes and shapes, which are critical to their performance in this application. In thermal decomposition, the metal precursor is heated to a temperature that is sufficient to break the bonds within the precursor molecule and release the metal ions. In laser ablation, a focused laser beam is used to vaporize the metal precursor and form a cloud of metal atoms, which then condense to form MNPs. At the end, MNPs are separated from the reaction mixture and collected after centrifugation at high speed. Microwave-assisted synthesis of MNPs involves the use of microwave radiation to promote the reduction of metal ions and the formation of MNPs (Zdenka et al., 2023). While photochemical synthesis involves the use of light to promote the reduction of metal ions from MNPs.
The chemical approach for synthesizing MNPs involves the use of chemical reactions to convert metal ions into NPs. This approach is commonly used in the laboratory and in industry, and it has several of advantages over other methods of nanoparticle synthesis. The chemical approach for synthesizing MNPs typically involves three main steps (Habibullah et al., 2021). In the first step, a solution of metal ions is prepared, which serve as the starting materials for the synthesis of the NPs. Subsequently the solution of metal ions is mixed with a reducing agent, which is a chemical compound that can donate electrons to the metal ions. The reducing agent is typically a molecule that contains a carbon-hydrogen bond, such as formaldehyde or glucose (Singh et al., 2018). Later the reducing agent is added to the solution of metal ions that donates electrons to the metal ions, causing them to reduce and form nanoparticles. Finally the reaction mixture is heated to a temperature that is appropriate for the synthesis of the nanoparticles. The temperature of the reaction mixture determines the size and shape of the nanoparticles, as well as their purity and stability. The reaction mixture is typically heated to a temperature of between 100 and 200°C, depending on the type of metal ions and the reducing agent used (Zhang et al., 2020).
The green synthesis approach for NPs is a method for synthesizing MNPs using eco-friendly and sustainable materials and processes. In this approach, MNPs are synthesized from natural or renewable materials, such as plant extracts or microorganisms, rather than synthetic chemicals. BMNPs are low or less toxic and produce less waste with improved sustainability. One key advantage of the green synthesis approach is that it uses natural materials or biocatalysts, such as enzymes (Fouda et al., 2020), fungies (Shaheen et al., 2021a) or microorganisms (Aref and Salem, 2020; Al-Zahrani et al., 2022b) with or without the use of high temperatures in reduction of metal ions. These biocatalysts help to control the size and shape of the NPs and to improve their stability in solution (11). Overall, the green synthesis approach for MNPs offers several benefits over traditional synthetic methods. It uses environmentally friendly and sustainable materials and processes, and it can produce MNPs with improved properties and enhanced performance in various applications. As a result, the green synthesis approach is an important area of research and development for the synthesis of metallic NPs.
Characterization of MNPs
The characterization of MNPs is an important step in the development and application of these materials. Characterization involves the use of a variety of techniques such as UV-vis absorption, transmission electron microscopy (TEM), field emission scan electron microscopy (FESEM), dynamic light scattering (DLS), X-ray diffraction (XRD), inductively coupled plasma mass spectrometry (ICP-MS), differential scanning calorimeter (Bundschuh et al., 2018), thermogravimetric analysis (TGA), and surface-enhanced Raman spectroscopy (SERS) to measure and analyze the properties of MNPs, including their size, shape, composition, and surface characteristics (Figures 1, 2). This information is critical for understanding the behavior of MNPs and for optimizing their performance in various applications.
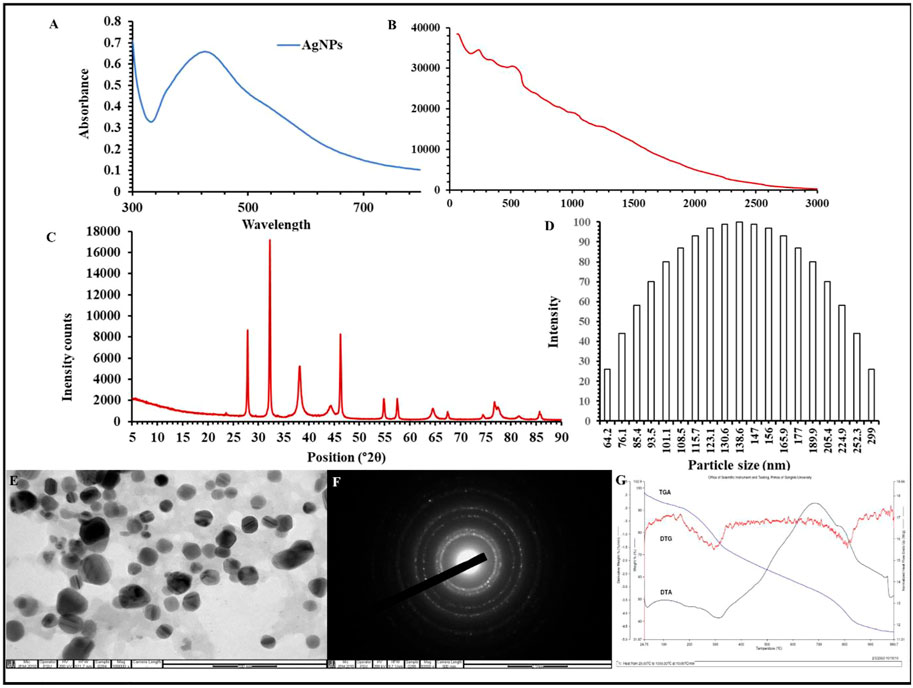
FIGURE 2. Different techniques are used to characterize metallic nanoparticle’s UV-vis spectra (A), Raman spectra (B), X-ray diffraction spectra (C), dynamic light scattering (D), transmission electron microscopy (E, F), and thermogravimetric analysis (G). Reproduced with permission (Singh et al., 2022a). Copyright 2022, Elsevier.
One common technique used to characterize MNPs is TEM. TEM uses a high-energy electron beam to produce images of NPs at high resolution. This allows researchers to visualize the size and shape of MNPs and to measure their dimensions with high accuracy. TEM can also be used to study the composition and structure of MNPs, including the arrangement of atoms within the NPs and the presence of any defects or impurities. TEM is widely used in research and development to study the properties of MNPs and to evaluate their potential for various applications (An and Somorjai, 2012). A recent study on NPs demonstrates different size and shape dependent synthesis with a wide ranging biomedical applications owing to their unique physicochemical properties, controlled size, and shape (An and Somorjai, 2012) (Figure 3).
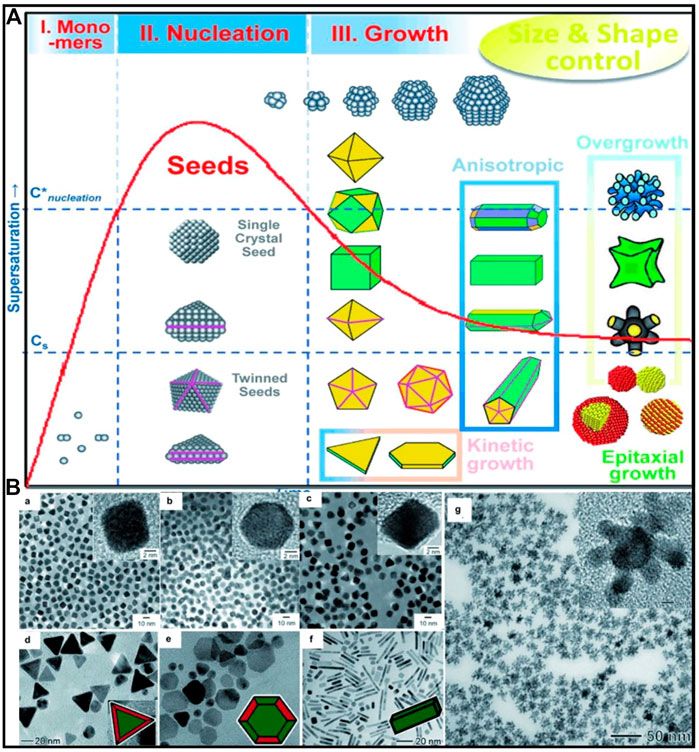
FIGURE 3. General synthesis strategies for size and shape controlled MNPs combined with LaMer representation (A). HRTEM images of shape controlled MNPs (B): cube NPs (b,a), cuboctahedra NPs (B), octahedra NPs (b,c), triangular plates NPs (b-d), hexagonal NPs (b-e), nano-bars NPs (b-f), nano-dendrites NPs (b-g). Reproduced with permission (An and Somorjai, 2012). Copyright 2022, John Wiley and Sons.
Another technique used to characterize MNPs is DLS. DLS measures the size of NPs by measuring the light scattering properties of MNPs in solution. This technique is particularly useful for characterizing the size distribution of NPs, which is an important factor that can affect their performance in various applications. DLS can also be used to study the stability of nanoparticle suspensions, which is another important factor that can influence their behavior (Jayeoye et al., 2021b).
Other techniques that are commonly used to characterize MNPs include XRD, ICP-MS, and SERS (Singh et al., 2022b). These techniques provide additional information about the composition, structure, and surface properties of MNPs, which is useful in understanding their behavior and optimizing their performance in various applications.
Properties of NPs
Several properties of MNPs have been reported and evaluated among them are electronic, optical magnetic, mechanical, thermal, and antimicrobial properties for various biomedical applications have been illustrated in the review. The electronic properties of MNPs depend on their size, surface area, chemical composition, and modification (Shin et al., 2015). A study indicated that modification of Al2O3 with organic ligands can control the size of the materials produced through the aggregation process (Henkel et al., 2020). The presence of organic compounds such as ligand significantly improve the surface characteristics of MNPs. While different types of ligands monomers showed effects on the structural characteristics of materials produced. This kind of modification collectively produce different electrical characteristics. Thus, the electrical properties, such as electrical conductivity and the dielectric constant, could be improved by the addition of barium titanate due to its perovskite nanostructures and piezoelectric properties.
The optical properties of MNPs, especially semiconductor materials, are important for several applications, such as in photocatalysts and photovoltaics. The optical properties can be determined by basic light principles and the Beer-Lambert law. The increased absorption of wavelength in semiconductor MNPs are influenced by several factors, including size distribution, shape, sizes, and the type of modifiers. Moreover, the optical properties influenced by the composition of the nanostructures, such as metal ion doping, surface modification, and especially the reflectance and scattering phenomena of MNPs. The reflectance depends on MNPs size, increases with increasing particle size and decreases on decreasing the size of MNPs (Mikhailov et al., 2018).
Magnetic NPs are commonly used for biomedical applications and environmental remediation. The magnetic properties are influenced by the particle size of NPs with the best performance showing a particle size <35 nm (Lamouri et al., 2020). The magnetization value of MNPs depends on surface metal oxide which changes with the particle size due to mismatches between the lattice parameters of the metal and metal oxide, which can cause interfacial stress on the surface. Moreover, the magnetic property of MNPs also depends on the composition of the nanostructure and the synthesis methods (Lakshmiprasanna et al., 2019).
Nanoparticles exhibit different mechanical properties compared with bulk materials or microparticles. MNPs provide a large surface area and are easy to modify, resulting in an increase in mechanical properties such as hardness, adhesion, stress and strain, and the elastic modulus. NPs from a group of inorganic compounds show mechanical properties, while organic compounds generally have low mechanical properties. The thermal properties of NPs are better than their fluid form because they have a large surface area and so heat transfer occur directly on the surface of the materials (An et al., 2019). Moreover, the mechanical properties of the materials gradually increased by increasing the metal oxide contents. In addition, nanofiller supplementation with high intrinsic thermal conductivity significantly influenced the thermal properties of MNPs (Jeon and Lee, 2019). Overall, the thermal properties of NPs depend on the large surface area, mass concentration, the ratio of energic atoms in MNPs, and the fraction of NP volume dispersed. NPs have been shown some other important properties such as hydrophilicity, hydrophobicity, suspension, diffusion, and thermal conductivity.
Current development in biomedical applications
MNPs are synthesized using several physical and chemical methods and reducing agents, however, generally these methods are energy and capital demanding, which employ toxic chemicals and organic solvents in the synthesis process. In addition, after synthesis requires incorporation of a capping agent for stabilization. Hence, biogenic synthesis of these MNPs using green-chemistry or plant-based extracts is considered safe and effective with minimum waste generation. Green synthesis MNPs such as gold and silver are widely used in biomedical applications including pharmacy, immunoassay, polynucleotide detection, and drug delivery. Silver in varied salt forms is documented as an antibacterial and anti-inflammatory agent; thereby reduced forms of silver to NPs are more effectively used as antimicrobial and in mitigation of dermal wound infections. Several studies reported that the incorporation of MNPs in different dosage forms such as gel (Ontong et al., 2020), transdermal patch (Singh et al., 2022a), cosmetics (Singh et al., 2022b), surgical sutures (Syukri et al., 2020; Syukri et al., 2021), dental products (Mohandoss et al., 2023), antitumor (Mata et al., 2023), etc., effectively inhibited both Gram-positive and Gram-negative bacteria.
Dutt et al. (2023), fabricated biogenic NPs using Azadirachta indica leaf extract, demonstrated high zeta potential (−55 mV), strong antibacterial efficacy against multi-drug resistant-pathogen and 62.47% of Chinese hamster lung fibroblast cells with insignificant DNA damage observed by mean fluorescence intensity using fluorescent dye. Though no significant improvement in cell proliferation was reported due to the tested concentrations, AgNPs inhibit the growth of wound pathogens (Figure 4). In another study, biogenic synthesized AgNPs fortified within a biodegradable polymeric matrix indicated dose-dependent antibacterial efficacy with a significant reduction in colony forming units of important wound pathogens including A. baumannii, Enterococcus coli, k. Pneumoniae, Pseudomonas aeruginosa, S. aureus, S. epidermidis, and C. albicans. Furthermore, in vivo study on pet animals visiting Veterinary hospitals under inclusion and exclusion criteria indicated healing of wounds on usage with biocompatibility (Figure 5) (An and Somorjai, 2012). Spent mycogenic medium assisted AgNPs synthesis showed antifungal activity against common hospital clinical strains of C. albicans and non-albicans species such as C. krusei, C. glabrata, C. parapsilosis, C. tropicalis, and C. guilliemondi and agriculture pathogens such as F. oxysporum, F. saccchari, F. subglutinas, F. verticillioides, and C. lunata (Ribeiro et al., 2023). Furthermore, the BMNPs fortified peel off gel formulations indicated excellent inhibitory effects property against microorganisms commonly found in skin including Staphylococcus aureus ATCC 25923, Staphylococcus epidermidis ATCC1228, and P. aeruginosa ATCC 27853 yielded >3 log and 2 to 3 log reductions, respectively after 3 h of treatment. The results indicated that the MNPs embedded facial gel formulations significantly decreased the growth of P. aeruginosa by 99% after 1 h, and 99.9% after 3 h (Figure 6).
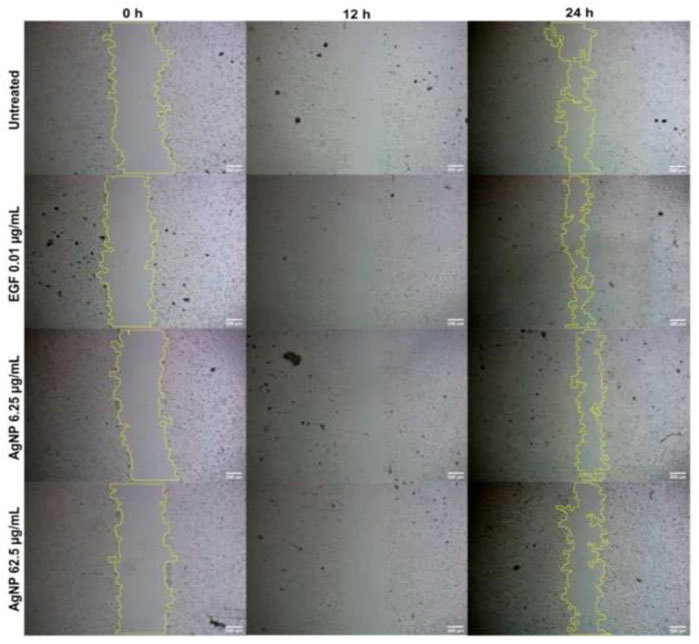
FIGURE 4. Untreated fibroblast cellular proliferation, presence of varied concentrations of MNPs, and standard EGF-mRNA. Reproduced with permission (Dutt et al., 2023) Copyright 2023, MDPI.
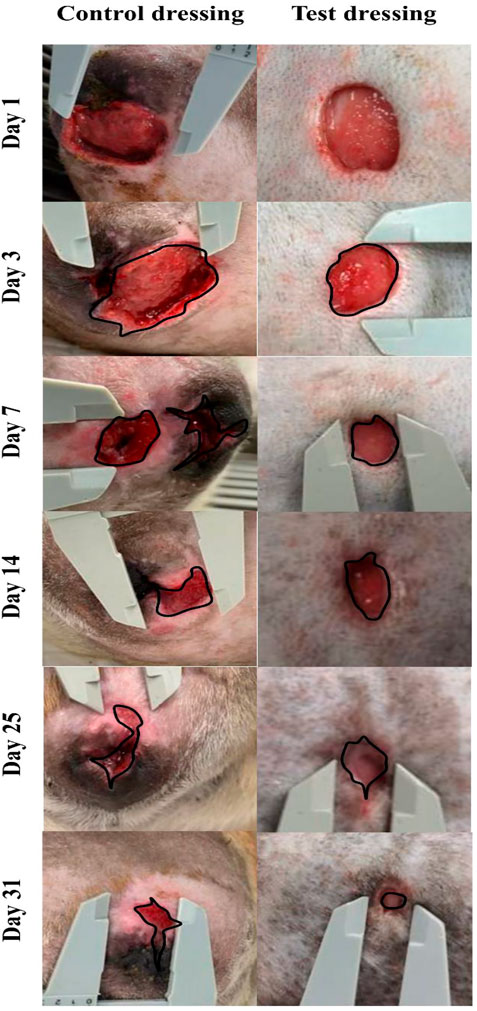
FIGURE 5. Wound healing efficacy of MNPs incorporated composite and control composite assessed on healthy dogs with similar natural wound conditions of any bread, sex, and age visiting at Veterinary Teaching Hospital, Prince of Songkla University, Thailand. Reproduced with permission (Singh et al., 2022a). Copyright 2022, Elsevier.
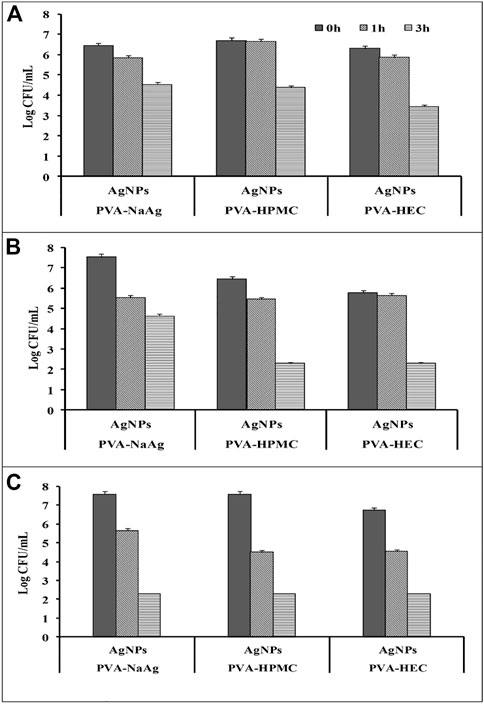
FIGURE 6. Antibacterial efficacy of BMNPs incorporated facial peel-off PVA-NaAlg, PVA-HPMC, and PVA-HEC mask gel against pathogenic bacteria Staphylococcus aureus ATCC 25923 (A), Staphylococcus epidermidis ATCC1228 (B), and Pseudomonas aeruginosa ATCC 27853 (C). Reproduced with permission (Singh et al., 2022b). Copyright 2022, Springe nature.
Zada et al. used the cyanobacterium leptolyngbya JSC-1cell free extract to extract AuNPs. The antibacterial activity of AuNP was evaluated against various bacterial species. S. aureus and Enterococcus coli strains were used to check the antibacterial activity of AuNPs. The inhibition zone of AuNPs against E. coli and S. aureus were 18 ± 2 mm and 14 ± 2 mm, respectively at different concentrations. In another study, AuNPs exhibited stronger antibacterial activity against gram-positive bacteria than that of gram-negative bacteria. It was observed that maximum inhibition was found in the order of Aeromonas liquefaciens > Enterococcus fecalis > Salmonela typhimurium (Mahdi and Parveen, 2019). Rajesh et al. derived the optical, electrical, and magnetic potential of CuNPs with strong antibacterial properties against gram-positive and negative. Moreover, CuNPs indicated strong antibacterial activity against Botrytis subtilis and E. coli. This study revealed that CuNPs can be effectively used for biomedical applications against fungal and bacterial infections (Jayarambabu et al., 2020).
Sundeep et al. (2017) synthesized AgNPs using Mangifera indica leave extract, and demonstrated excellent antimicrobial activity with improved stability. Glass ionomer cement microparticles were integrated with AgNPs indicating improved mechanical strength compared to microparticles without GIC. These AgNPs were tested for the restoration of minerals and colonial suppression of biofilm. This study revealed the dual dentistry application of the synthesized AgNPs. Ahanand et al. fabricated an adhesive containing AgNPs composite that demonstrated a low cariogenic feature against Streptococcus than the conventional orthodontic composites. This study revealed that AgNPs used in lower concentrations do not affect the mechanical properties (Ahn et al., 2009). Thomas et al. studied the use of poly (methyl methacrylate) (PMMA) for dentures and crowns. The polymer matrix of PMMA is fortified with many titanium dioxides and FeONPs to improve its efficacy. These materials specifically exhibit high molecular weight and low porosity, which can be utilized to reduce the adherence of C. albicans toward the resin materials (Thomas et al., 2014).
Seopsi et al. studied the interaction of AuNPs and proteins. These AuNPs have a high interacting ability with proteins due to their electrostatic, hydrophobic, and hydrophilic capabilities that form a protein-NP complex known as protein corona. The resulting interactions were used for labelling techniques where the AuNPs were modified with the help of antibodies. This modification renders them with capacity to mark the cells and act as a probe for SEM or TEM analysis (Kalimuthu et al., 2020). Arpita et al. investigated the AgNPs for their potential in nanomedicine because of their biological and physiochemical properties like cancer cytotoxicity, antimicrobial activity, and improved surface-to-volume ratio. AgNPs are used for developing drug delivery systems to act on tumours. They act as cytotoxic agents and help in modulating transporting molecules. AgNPs attack the fluidity of the cell membrane and enter the cells reducing the accumulation of cancer cells by hindering their uncontrolled proliferation (Tripathy et al., 2020). Frederik et al. explored the potential of FeNPs for their utility in cancer care. FeNPs are approved by FDA for the diagnosis of cancer. The ferrofluid formulation was developed by MagForce AG to treat brain tumours. They are used as contrast agents in counting MRI and multimodal imaging. The FeNPs are equipped with strong targeting ability. They pass through the circulatory system to reach the site of action and release the drug in tumour cells. This allows less damage to neighbouring cells. The magnetic properties of FeNPs can convert the radiant energy into heat and adverse effects of cancer therapy can be kept in check (Soetaert et al., 2020).
Analytical applications
The application of biogenic MNPs has continued to wax increasingly, strong owing to their environmentally friendly synthesis strategy. Since they are constructed using nature-derived materials, their capacity to modulate or control the entire optical and biological properties of the resulting nanomaterials is highly probable. Kumar et al. (2020) demonstrated the role of tryptophan analogues based synthesis of biocompatible AuNPs. It was noted that the use of an amino acid-tryptophan conjugated with ionic liquids, as stabilizer of AuNPs, played significant roles in the in-vitro biocompatibility assays on red blood cells and hemolysis. Recently, a biocompatible AgNPs-based colorimetric assay for toxic Hg2+ ion in solution using Asystasia gangetica phenolic extract and biopolymer-konjac glucomannan as reducing and stabilizing agents respectively (Jayeoye et al., 2022). With humanity’s insatiable drive for energy generation and industrialization taking a huge toll on the environment, advocates of environmental sustainability have welcomed “green technology” with soothing relief. This concept entails everything green, such as green chemistry, green synthesis, etc. Heavy metals (HMs), and pollution of the major environmental compartments (air, soil, and water), are the results of anthropogenic imbalance in resources exploration beyond nature’s capacity for replacement or regeneration. HMs are metals/metalloids having a high density of around 5.0 g/cm-3, with potential toxicity at part per million (ppm) level (Ali and Khan, 2018). From the environment perspective, HMs of interest include Hg2+, Ag+, As3+, Cr3+, Cd2+, Pb2+, Fe2+, Fe3+, Cu2+, Zn2+, Co2+, Mn2+ etc. While some of these metals are important to plants and animals (Fe2+, Fe3+, Mn2+, Zn2+, and Cu2+), for the efficient biochemical performance of cells, others however are toxic even at low concentrations. The general concern for HMs pollution or contamination stems from their behavior. They are not easily degraded in the environmental compartment they are found, and more worrisome is the possibility of their bio-accumulation across the trophic levels (Chang et al., 2023). They enter the human system and induce deleterious health complications, resulting from their impact on the major human organs, including, liver, lungs, skin, etc.
Colorimetric detection/sensing of HMs based on MNPs as optical nanoprobe presents a balance between detection sensitivity, easy tunability, robustness and the avoidance of toxic materials for nanomaterials synthesis steps. Since MNPs exhibit surface plasmon resonance, defined as the excitation of surface electron clouds on metal (electron available on the conduction bands), resulting from the interaction with electromagnetic radiation (Jana et al., 2016). For colloidal MNPs, this is sometimes represented as localized surface plasmon resonance (LSPR). At this level, MNPs exhibit the highest light absorption and scattering phenomena, with characteristic naked eye observable color. Factors affecting the properties of colloidal MNPs at the LSPR state include particle size, shape, morphology, the environment in which the MNPs are embedded (dielectric property) and their refractive index (Ringe et al., 2012), etc. Stable and dispersed MNPs particularly, AgNPs (yellow color), AuNPs (Red or purple), CuNPs (orange), and PdNPs (dirty brown) are known to possess a sharp absorption maximum, except for PdNPs (Han et al., 2019). The color and absorption maximum are sensitive to the changes induced by the analyte injection into their solution and thus can be reliably applied as detection probes. With this strategy, facile and sensitive detection of various analytes can be realized, with sensitivities comparable to responses from the highly sophisticated analytical equipment.
Mechanism of MNPs based colorimetric assays
MNPs based colorimetric detections rely on wide ranging mechanisms to achieve sensitive, reproducible, and selective assay to the analyte of interest. Some of these mechanisms exploited in the literature include aggregation-based assay (which rely on particles inter-plasmon coupling), anti-aggregation-based strategy, method based on analyte reductive efficacy at a given pH, method based on analyte induced oxidation of MNPs probe and method based on MNP transformation to core shell or bimetallic nanostructures, etc.
Annadhasan et al. reported a greenly fabricated AgNPs and AuNPs for multi-metal ion detection in solution, based on sunlight assisted L-tyrosine synthesized AgNPs and AuNPs (Annadhasan et al., 2014). The synthesized NPs were applied for the detection of four metal ions, including, Pb2+, Mn2+, Ag+ and Hg2+. As depicted in Figure 7, multiple detection mechanisms was proposed for the (Ag and AuNPs) based detection of the various metal ions, including, Hg2+ mediated oxidation of AgNPs (detection mechanism A), Mn2+ based aggregation effect on AgNPs (detection mechanism B), Pb2+ induced plasmon coupling of AuNPs (detection mechanism C) and Hg2+ induced Au@Hg amalgam formation (detection mechanism D). These different highlighted mechanisms are marked by naked eye observable color changes and maximum absorption spectra perturbations.
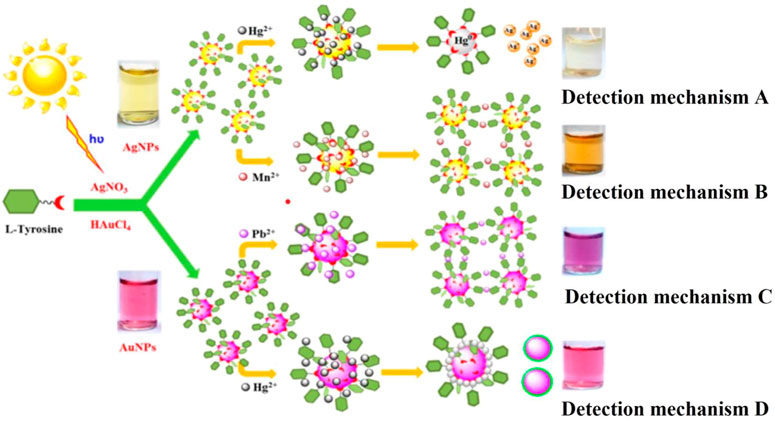
FIGURE 7. Schematic illustration of sunlight assisted L-tyrosine synthesized AgNPs and AuNPs for multiple metal ions detection. Reproduced with permission (Annadhasan et al., 2014). Copyright 2014, American Chemical Society.
Further, L-DOPA mediated mussel inspired chemistry was adopted for AgNPs@pDOPA (pDOPA = poly 3,4-dihydroxy-L-phenylalanine), towards sensitive colorimetric detection of Pb2+ and Cu2+ (Cheon and Park, 2016). In the presence of sodium hydroxide solution L-DOPA can polymerize to pDOPA, which is highly reductive. The pDOPA solution was further injected with AgNO3 to realize sparkling yellow color AgNPs@pDOPA colloidal nanomaterial. The material was tested for Pb2+ and Cu2+ sensing through naked eye color transition from yellow to orange, with calculated LOD of 9.4 × 10−5 and 8.1 × 10−5 μM, respectively. It was proved that the multiple hydroxyl group functionality stability the AgNPs@pDOPA nanoprobe can be brought together in proximity by the tested metal ions (Pb2+ and Cu2+), and thus induce particle aggregation.
Also, Samari et al. reported photo-synthesis of Capparis spinosa leaf extract assisted synthesis of CuONPs, at room temperature for colorimetric detection of ferric and ferrous ions in solution (Fe3+ and Fe2+) (Samari et al., 2019). The multitudinous phyto-compounds present in the plant extract, containing hydrophilic functional groups, including, -NH2, -SH2, and COOH provided perfect surface attachments for Fe3+ and Fe2+ binding with consequent particles aggregation. This is also followed by color changes from yellow to deep brown and particles sizes increment as obtained from the DLS (dynamic light scattering) system.
Moreover, the standard electrode potential or standard reduction potential (SRP), value of metals can provide detailed and informative relationships between metal’s redox potentials. Generally, metals with higher SEP/SRP will serve as oxidizing agent and can accordingly be reduced in the ensuing redox reaction. This relationship has been well exploited in several reports of greenly synthesized AgNPs for Hg2+ detection. The SEP of Ag+/Ag0 is 0.800 V while Hg2+/Hg0 is 0.850 V. Hence in AgNPs based probe for Hg2+ detection, the analyte would serve as the oxidizing agent, and it is thus reduced to Hg0 on AgNPs surfaces, which initiates the AgNPs oxidation, with concomitant color changes from yellow to colorless (Ghosh and Mondal, 2020; Jayeoye et al., 2021c; Jayeoye et al., 2022). Table 1 shows a summarized greenly realized MNPs-based optical probe for diverse metal ions detection.
Applications of MNPs as colorimetric sensors
The efficient working of the human biological system requires optimal aggregation of information from diverse organs. Biomolecules or biological macromolecules are metabolites covered under carbohydrates, amino acids (the building block of proteins), proteins, enzymes, hormones, neurotransmitters, nucleic acids, etc. The use of MNPs as optical probes for the detection and semi-quantitative estimation of biomolecules abounds in literature.
A sensitive colorimetric detection assay for L-cysteine in human simulated serum sample was investigated using corn cob aqueous extract derived AgNPs (Ragunathan et al., 2022). AgNPs were carried out at 80°C under stirring, to realize yellow color solution. L-cysteine detection was achieved through the injection of the amino acid into a pH 7.0 solution of the NPs, using phosphate buffer. Through Ag-S interaction and the changes in the side chains of L-cysteine, since the isoelectric point (pI = 5.0), then AgNPs aggregation was observed with color changes from yellow to red.
Ascorbic acid (AA) is an important antioxidant derived from food substances (fruits, vegetables, etc.). Humans cannot synthesize AA, owing to its lack of the key enzyme (gluconolactone oxidase) involved in the conversion of L-glucono-γ-lactone source to AA. Hence the detection of AA in food sources has been pursued with keen interest by scientists. Jayeoye et al. reported a reliable detection probe for AA in food samples based on biopolymer-sodium alginate supported AuNPs (Jayeoye et al., 2021a). As shown in Figure 8A, SA-AuNPs probe was synthesized at room temperature, by stirring gold salt and SA aqueous solution, which was further incubated for 12 h in the refrigerator. Faint purple color SA-AuNPs were realized afterward. The addition of AA to the probe led to color transition from faint purple to red, with absorption spectra enhancement (Figure 8B). This was explained to be due to AA, facilitating further reduction of un-reduced gold salt ion in the SA-AuNPs solution, through in situ seed mediated growth process. The sensor demonstrated linearity ranging from 12.5 to 150.0 µM (Figure 8C) and was applied for AA detection of common fruits.
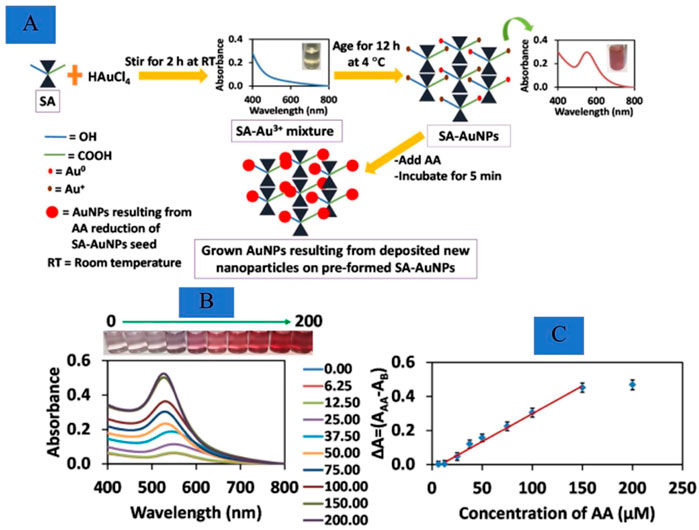
FIGURE 8. (A) Depiction of synthesis protocol of sodium reduced and stabilized AuNPs probe (SA-AuNPs), and its AA detection strategy (B) Photo images and absorption spectra enhancement after AA injection and (C) Linear range of the sensor within 12.5–150.0 µM. Reproduced with permission from Jayeoye et al. (2021b). Copyright 2021, Elsevier.
Further, glucose and hydrogen peroxide (H2O2) are two highly important metabolites of interest in the human system. Increased H2O2, an amount beyond a certain level in the human system could be a pre-disposing factor to radicals’ generations (reactive oxygen species, ROS), hence the detection of H2O2 is crucial in human biological fluids and the environment. Glucose on the other hand, is an important sugar needed for cells energy requirement. Excessive concentration of glucose could lead to diabetes, obesity, and other debilitating diseased conditions (Martemucci et al., 2022).
In a bid to design a completely environmentally friendly synthesis protocol, 3-aminophenyl boronic acid (an aniline analogue), in conjunction with biopolymer (sodium alginate), have been combined to synthesis a conducting polymer impregnated and stabilized AgNPs (Jayeoye and Rujiralai, 2020). The fabricated colloidal nanocomposite was investigated as an optical probe for H2O2. The detection mechanism relied on the ability of H2O2, to induce etching/oxidation of the stable and dispersed AgNPs in solution. Color transition from yellow to colorless ensued, with associated UV-vis absorption spectra quenching. Similarly, Basiri et al., applied agar stabilized AgNPs for triple analyte sensing, including Fe2+, glucose and H2O2 (Basiri et al., 2018). Through tuning the reaction between Fe2+ and H2O2, (Fenton reaction), both Fe2+and H2O2, could be detected using AgNPs, while coupling enzyme glucose oxidase (GOx), could facilitate the detection of glucose. The adoption of MNPs based optical sensors for biomolecule detection should fulfill two major limiting factors. The issue of specificity, i.e., response to analytes of interest in the presence of diverse interfering species and real sample detection capacity without aggregation. More scientific works are currently geared in this direction to increase detection robustness and practical effectiveness.
Application of MNPs in the preservation of food product
Although nanoparticle use in the food industry is relatively new, its unique optical properties, diffusivity, mechanical resistance, and solubility have also contributed to their utilization at all stages of the food industry including production, processing, packaging, transport, and storage, with noticeable effects on food safety and quality. For the development of NPs-food packages polypropylene, polyethylene, and polyethylene terephthalate are generally used, to prevent the permeation of water and oxygen molecules. Packages are coated with antioxidants, antibacterial agents, and preservatives to ensure food safety and preserve food quality (Onyeaka et al., 2022). Additionally, it improves food stability, detects food spoilage organisms, prevents oxidation, and has antimicrobial and UV protective properties (Hamad et al., 2018). The protection, preservation, and extension of food’s shelf life are all possible by employing MNPs in the food sector. The utilization of MNPs has made it possible to improve the properties of food packages such as antibacterial activity, and mechanical properties, and reduced permeability with regards to water vapor (Nwabor et al., 2020). For example, Silica-NPs (SiNPs) inhibit gaseous exchange across the layers of the package and extend the product’s shelf life whereas Silver-NPs (AgNPs) have increased antibacterial capabilities of coated ceramics. With effective spoilage control, the addition of metallic NPs has dramatically extended the shelf life of preserved and canned foods (Primožič et al., 2021). Additionally, NPs with antimicrobial properties such as carbon nanotubes, nano-TiO2, nanoCe2O4, nanoZnO, and nano-Ag have been employed to enhance food quality. Thus, based on the available data, the present study describes the utilization of MNPs in food packaging. Additionally, an effort has been made to comprehend the hazards, toxicity, and potential applications of MNPs. The growth of L. Plantarum, Enterococcus coli, S. aureus, and yeasts may be suppressed by using MNPs in food packaging (An et al., 2008; Fernández et al., 2009). MNPs-based packaging act as a barrier for various gases such as carbon dioxide, and oxygen. It also acts as a barrier to moisture and ethanol. Moreover, biodegradable biopolymers, intelligent packaging, and active packaging are a few additional benefits of MNPs packaging.
AuNPs are utilized in the packaging of food goods and have shown potential as antibacterial agents. Such packaging enables controlling oxygen levels and microbiological growth while increasing the shelf life of the packaged goods. AgNPs from Digitalis purpurea extract were developed to test nanocomposites’ mechanical barrier, adhesion, and antibacterial qualities. It significantly increased the sensitivity of E. coli and S. aureus to these nanocomposites (Ramji and Vishnuvarthanan, 2022). Kim et al. developed a chitosan-based nanocomposite film, which was combined with Nigella sativa extract and Ag-NPs. These nanocomposite films were created with persistent Ag-NP release that was pH-dependent. Their effectiveness was evaluated against two gram-positive bacteria (Botrytis subtilis and S. aureus) and two gram-negative bacteria (E. coli and P. aeruginosa). As a result, the antibacterial activities that have been noted, rely on the Ag-NP concentration that prevents bacterial development (Kim et al., 2019). The chitosan-based composite fortified with AgNP was recently used for food packaging and has shown antibacterial action against pathogens. By directly damaging the membranes and cell walls of microorganisms, AgNPs provide a crucial mechanism for antimicrobial activities (Figure 9) (Nwabor et al., 2020). Moreover, in a similar study ceramic coated with AgNPs indicated damage to the bacterial cell membrane (Figure 10) (Nwabor et al., 2021a). A more thorough investigation into the antibacterial mechanism of nano-Ag materials was recently carried out. The results indicated diverse processes, including inhibiting metabolic pathways, membrane disruption, protein complexes, and DNA damage (Khan et al., 2019).
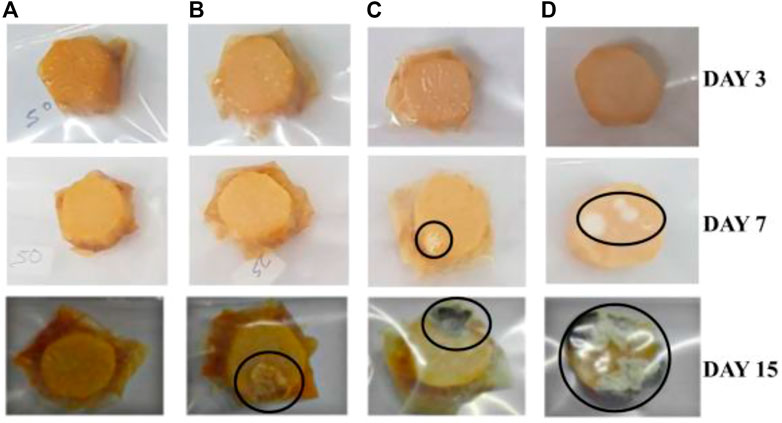
FIGURE 9. Shelf-life extension of packed food wrapped with chitosan-poly (vinyl) alcohol film fortified with MNPs. Where (A–D) represent the different concentrations of chitosan and poly (vinyl) alcohol used in the fabrication of the film. Reproduced with permission (Nwabor et al., 2020). Copyright 2020, Elsevier.
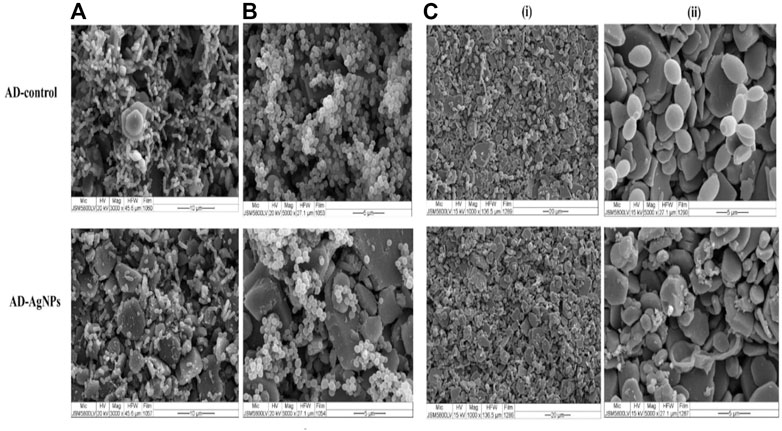
FIGURE 10. Scan electron microscopy image of biofilm formation of MNPs modified and control ceramic against E. coli (A), S. aureus (B), C. albicans (C). Microbial adhesion to MNPs modified and control ceramics after 4 h. Reproduced with permission from Nwabor et al. (2021a). Copyright 2021, Taylor and Francis.
Ag-NPs-containing packaging materials have significantly increased the storage life of goods such as potatoes and rice (Li et al., 2017). Due to Ag + ions, a PLA film was utilized in further research to preserve rice at high temperatures and humidity. This film has fungicidal effect on Aspergillus flavus and significantly slowed the aging of rice (Li et al., 2017). In another research, a unique tenary nanocomposite film based on tragacanth, hydroxy propyl methyl cellulose, and beeswax impregnated with AgNPs was fabricated, which demonstrated a dose-dependent inhibitory action against Botrytis cereus, S. aureus, S. pneumoniae, Listeria monocytogenes, Enterococcus coli, K. pneumoniae, P. aeruginosa, and S. typhimurium, indicating that it is appropriate for use in food packaging (Bahrami et al., 2019). According to Olmos et al., low-density polyethylene-Ag nanocomposites were efficient for both general-purpose containers and food preservation against biofilm-forming E. coli (Olmos et al., 2018).
Due to low cost and UV blocking activity than AgNPs, ZnONPs are used to develop food packaging films. The mechanical strength, blocking characteristics, and durability of films may be significantly improved by the absorption of ZnONPs (Kim et al., 2019). ZnONPs-based eco-friendly packaging materials have shown bactericidal action. Prakash et al. showed how BC-PPy-ZnO (polypyrrole-Zinc oxide) nanocomposite be used as active and intelligent food packaging (Prakash et al., 2019). By inhibiting the growth of pathogens in meat products, these packaging materials increase the shelf life of meat products. ZnONPs fabrication using Catharanthus roseus extract had considerable antibacterial activities against Pseudomonas aeruginosa, Staphylococcus aureus, Botrytis thuringiensis, Botrytis subtilis, E. coli, and C. jejuni. At a high concentration of more than 3 mmol/L, ZnNPs also showed an antifungal effect against Botrytis cinerea and Pseudomonas expansum (Prakash et al., 2019). Based on the findings, P. expansum is more sensitive than B. cinerea (Kim et al., 2019; Prakash et al., 2019). In micro-concentration, these NPs are effective antibacterial agents for food packaging against pathogens, such as P. aeruginosa, S. aureus, and E. coli (Pandey et al., 2020). Zhang et al. (2017) developed a ZnOPLA-coated paper-based packaging material, that showed cidal effect against E. coli and S. aureus. Moreover, waste paper fortified with AgNPs demonstrated significant inhibition of potential food pathogens (Figure 11) (Nwabor et al., 2021b). Arfat et al. developed a nanocomposite film with fish skin gelatin, fish protein isolate, and ZnO-NPs. This nanocomposite film showed potent antibacterial action (Arfat et al., 2016). A new biodegradable biopolymer nanocomposite was produced by blending ZnO-Ag with Thymus vulgaris leaf extract. This nanocomposite film had antibacterial activity and excellent mechanical properties, which extended the shelf life of meat and suggested a possible replacement for conventional petrochemical-based polymers (Zare et al., 2019).
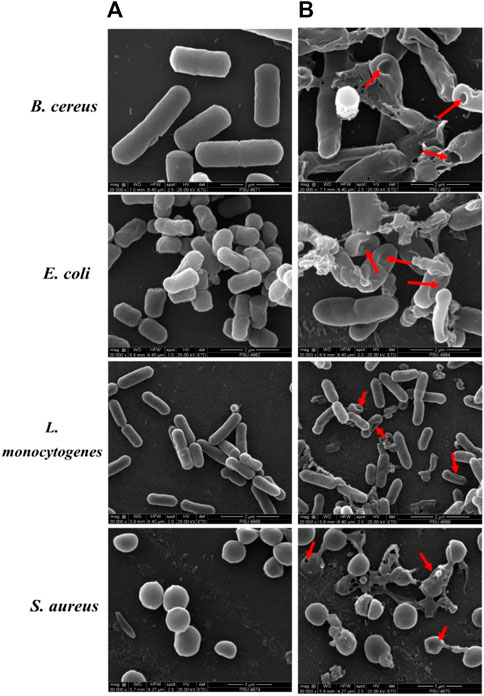
FIGURE 11. Scanning electron micrograph of MNPs treated foodborne bacterial isolates. Showing unruptured (A) and rupture with damage of bacterial cell membrane after treatment with a bactericidal concentration of MNPs for 4 h (B). Reproduced with permission from Nwabor et al. (2021a). Copyright 2021, Springer Nature.
Recently, the combination of TiO2-NP and chitosan has received keen attention. Youssef et al. (2015) developed a nanocomposite with chitosan/PVA/TiO2NP which enhanced the shelf life of soft white cheese. Another chitosan film was created using TiO2NPs and Cymbopogon citratus essential oil. Minced meat was then packed using the films after they had been formed and kept for 10 days at 4°C. TiO2-NPs were added, which boosted the tensile strength and water vapor permeability, while essential oil was added to prolong the meat’s shelf life (Hosseinzadeh et al., 2020). In a study lamb meat was stored at 4°C, the ability of edible nanocomposite films (whey protein isolate/cellulose nanofiber) containing TiO2 and a rosemary essential oil preserve the meat from microbial spoilage and maintain the sensory quality. The results revealed that gram-positive bacteria were more inhibited than Gram-negative bacteria (Sani et al., 2017).
CuNPs adhere to the bacterial cell surface through diffusion, adherent forces, or chemical interactions. Encapsulated NPs enter the cell and degrade the bacterial cell wall or membrane and the genetic makeup leads to bacterial death (Applerot et al., 2012). AgCuNPs-based nanocomposite has improved oxygen and UV light barrier properties, and mechanical strength. However, Salmonella typhimurium and L. monocytogenes were more resistant to the film-confined antimicrobial action than gram-positive and gram-negative bacteria (Ahmed et al., 2018). In chitosan films, the combination of AuNPs and Ag-NPs shows remarkable antibacterial capabilities that inhibit the development of microorganisms such as A. niger, S. aureus, P. aeruginosa, and C. albicans (Yaşa et al., 2012). A nanocomposite made of poly (vinyl) alcohol, titanium, chitosan, and TiO2NPs decreased the number of food-spoiling microorganisms and aided in cheese preservation after packing (Li et al., 2008).
Applications of MNPs in fabrics and textiles
Textiles can now serve several purposes primarily in the usage of nanotechnology. Due to the use of nanotechnology, it is clear that molecularly altered and improved textiles will eventually be able to outperform current textile goods in terms of performance and durability. Nanomaterials are frequently incorporated into textile materials without noticeably changing their texture or comfort. The main functional aspects of nano-finished textiles being researched right now are their antibacterial and UV resistance (Fouda et al., 2018; Eid et al., 2020; Salem et al., 2020; Shaheen et al., 2021b). Surface plasmons, which give MNPs their hues by changing their size and form, are an additional advantage of employing them. Sykuri et al. coated the surgical sutures with AgNPs and demonstrated excellent antibacterial efficacy against nosocomial pathogens (Figure 12) (Syukri et al., 2020; Syukri et al., 2021). Nanotechnology offers a way to enhance the value of the textile product and may, in the future, be a useful instrument for boosting revenue in the struggling textile sector.
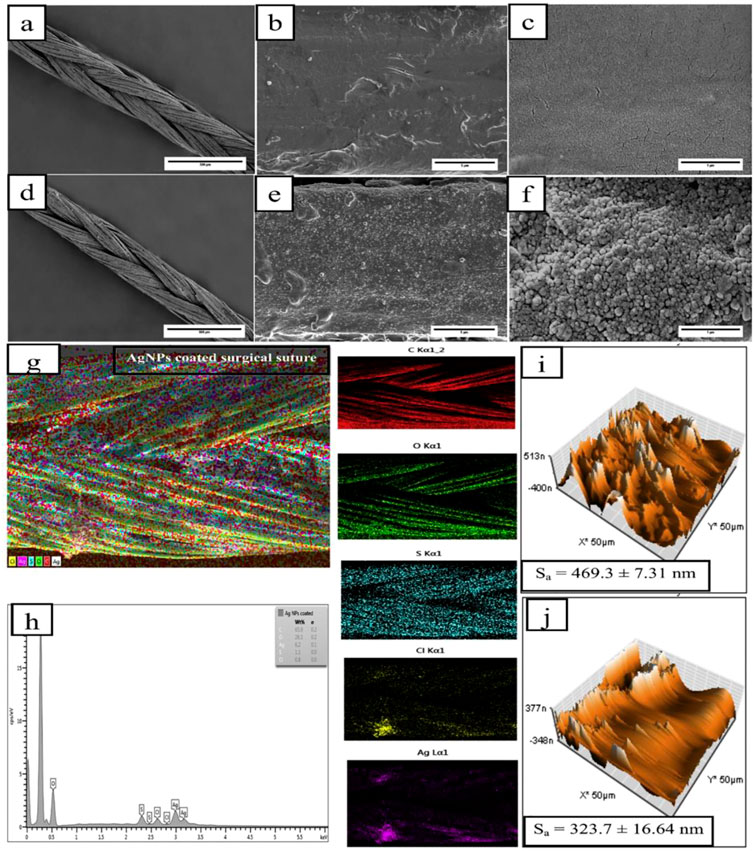
FIGURE 12. Field emission scanning electron microscopy image of braided (A–C) and uncoated (D–F) MNPs coated surgical sutures at different magnifications. Distribution of MNPs-coated surgical sutures using energy dispersive x-ray (G, H). Surface roughness of uncoated (I) and MNPs-coated (J) surgical sutures using atomic force microscopy. Reproduced with permission from Syukri et al. (2020). Copyright 2020, Elsevier.
Applications for MNPs in home appliance
Due to their numerous applications, NPs have come to draw attention in recent years. When metal or non-metal oxides are added to a base fluid, a new working fluid known as Nano Fluid is created. Both the fluid’s temperature and transport properties change as a result of the addition of NPs. NMs including carbon nanotubes, quantum dots, and AgNPs are some of the most often used ones for electrical and electronic devices (Parameshwaran and Kalaiselvam, 2014). A certain amount of lubricating oil will be carried away by the refrigerant in the compressor in a conventional air conditioning system. Thus, a particular amount of lubricating oil circulates through the air conditioning circuit along with the refrigerant. There is a risk of lubricating oil building up in the condenser if the lubricating oil’s solubility in the refrigerant is poor. If the lubricating oil in the refrigerant is very soluble, the refrigerant will wash away all the lubricating oil from the compressor, increasing the risk of abrasion. If NPs are not correctly disseminated in the oil, they may silt in the compressor. There is a risk of the NPs interfering with the environment if they are not distributed properly. Plant growth is discovered to be hampered by NPs. Since they cause cancer, caution should be used when handling them. Due to their high cost, economic considerations surrounding the use of NPs must also be made. R-410a is the refrigerant utilized in the testing air-conditioning setup. The fact that R-410a is azeotropic suggests that, unlike with conventional refrigerants, the temperature at which the phase shift occurs does not remain constant. During the phase shift, the temperature of the refrigerant (R-410a) falls. R-410a is the refrigerant utilized in the testing air-conditioning system.
Applications of MNPs for disinfection and microbial control of wastewater
Surface water in the majority of developing nations is severely contaminated by untreated or partially treated industrial wastewater containing a variety of contaminants, including microbiological pathogens. This problem is made worse by improperly constructed home waste disposal channels and poorly planned latrines that end up in river or canal systems. If they are flooded and connect with nearby surface water channels during the monsoon, improperly designed sanitary dump sites in nearby cities could be another possible source of pollutants (such as diseases, heavy metals, etc.) in the aquatic environment (Rikta et al., 2018). In addition to the pollution situation, there is an increase in water demand due to population growth, ongoing urbanization, industrialization, agricultural use, and home usage. The world’s water resources are under a lot of strain because of this whole situation. By 2025, over half of the world’s population will reside in water-stressed areas as a result (Chalchisa et al., 2018). To maintain good public health, safe and clean water is essential for drinking, residential use, food production, and other industrial uses. Drinking water is used for a variety of activities besides eating, including cooking, taking showers, washing dishes, and cleaning.
According to Wang et al., these uses greatly increase the channels by which pollutants and pathogens are exposed (Abdal Dayem et al., 2017). Growing food requires a lot of water through irrigation, and depending on the crop, a particular quality must be maintained. Water quality must be guaranteed during food processing because using water of poor quality can have a negative impact on people’s health. Various dangerous and toxic pollutants are contaminating water systems in most developing and industrialized nations. Heavy metals, micro pollutants, recalcitrant organic compounds, emerging contaminants, endocrine disrupting substances, and many more are examples of these contaminants. However, the need to rehabilitate this contaminated water is urgent given the current rise in water demand, the scarcity of potable water, and environmental concerns. Even though water quality has received considerable attention in many nations, microbiological pollution of water and wastewater remains one of the main issues due to the high mortality rate from waterborne diseases. Around the world, at least 2 billion people consume feces contaminated water. Most victims are children under the age of five, and waterborne diarrheal illnesses are responsible for almost 2 million annual deaths (Li et al., 2008). On the other hand, microbial pathogens in water spread diseases that are transmitted through the water, but they also cause crisis in the availability of potable water. The inability to use precious water sources due to pathogen contamination leads to a shortage of resources arising from increasing demand. A fundamental source of drinking water is unavailable to 844 million people worldwide. In areas where problems are prevalent, water disinfection is therefore an essential step for a safe water supply. Preventing and controlling the spread of waterborne disease requires effective microbial cleansing (Abbaszadegan et al., 1997). Disinfection is a technique used to inactivate microbiological pathogens (such as bacteria, viruses, and protozoa) in water. Chemical and physical disinfection processes are the two main categories of conventional disinfection techniques. Pathogens are inactivated using chemical disinfectants like chlorine and chlorine dioxide during chemical disinfection, whereas, during physical disinfection, microbial inactivation is carried out using physical methods like radiation, ultrasound, high temperatures, membrane filtration, and other physical means (Kraft, 2008). Fortunately, current research has identified numerous cutting-edge procedures to use NPs to get over the severe restrictions of conventional disinfection approaches. Because of their simplicity, economic viability, and disinfection effectiveness, these procedures are extremely promising.
Safety and toxicology of MNPs
Metal and metal oxide NPs demonstrate their suitability for a variety of uses. For disease diagnosis, drug delivery, food preservation, sensors, and water treatment; numerous researchers have used Ag NPs, Fe NPs, ZnO NPs, Au NPs, CuO NPs, TiO2 NPs, Co3O4 NPs, Al2O3 NPs (Parameshwaran and Kalaiselvam, 2014; Hassanpour et al., 2018; Eivazzadeh-Keihan et al., 2020; Murthy et al., 2020). The elements that hold the most significant potential for technological development also endanger people, animals, and the environment. Although there are strict laws against nano-intervention in biological sciences. Nanotechnology has significantly advanced from the bench to bedside in the last decade; increasing the exposure to a wide range of metallic nano-formulations. The development of NMs raises the risk of environmental harm by intentional discharge or unintentional spillages and raises the possibility of negative consequences. Numerous studies have looked at the toxicity of various MNPs (Mao et al., 2018). MNPs do not dissolve easily, so they are stable. Whereas, when metal oxide and metal alloy-based NPs are introduced to a biological environment they are less stable and more prone to disintegration and release ions, which increases the oxidative stress in cells via reactive oxygen species (ROS) production (Abdal Dayem et al., 2017). The particles’ micro size allows them to enter the circulatory and lymphatic systems. MNPs are exposed to a host by ingestion, inhalation, cutaneous absorption, or parenteral delivery of NPs-containing substances. The path of NPs entry within the body, the variety of NPs, and their quantity all substantially impact the health of various organs and tissues. Typical nanotoxicity processes include, but are not confined to, cell signalling modulation, oxidative stress and inflammation, genotoxicity, cytotoxicity, apoptosis, development of ROS, and cancer formation (Yang et al., 2020). When NPs are internalized, they activate cellular mechanisms via membrane-based receptors, which causes the production of ROS and oxidative stress (Yang et al., 2020). Lipid peroxidation, pathways of genotoxicity, and even downregulation of antioxidant enzymes or genes associated with such enzymes may all be part of an additional route of apoptosis or necrosis (Grijalva et al., 2018). Mitotic catastrophe, endoplasmic reticulum breakdown, and necrosis lead to cell cycle arrest and may cause cell death, apoptosis is a common cellular reaction in NP-based toxicity (Grijalva et al., 2018).
Many difficulties may be faced due to the adverse effects of metal/metal oxide NPs, these difficulties can be alleviated or minimized by appropriate nano/microstructure formulation and development. The appropriate encapsulation or entrapment of NPs into the matrix may be used to distribute metal/metal oxide NPs while preventing adverse consequences. The limits of toxicity, aggregation and instability may be avoided by the encapsulation of polymers during the manufacture of these polymeric MNPs. Coating NPs with discrete-sized polymers that deliver the substance with lesser toxicity but with increased efficiency when administered at smaller dosages is the most excellent strategy to promote biocompatibility and prevent particle aggregation (Balmuri et al., 2017). The most recent types of crystalline hybrid materials are metal-organic frames (MOFs), created via coordination bonding from seamless pairings of organic ligands and metal subunits. Additionally called porous coordination polymer (Balmuri et al., 2017). They are considered possible nanocarriers in the biomedical industry because of their high density, contact, broad surface area, non-toxicity, high pore volume, cohesiveness, and tiny size. MOFs can provide more effective treatments while reducing side effects. According to preliminary research, MOFs significantly lower the toxicity of Zn, Zr, Mg, and Fe (Balmuri et al., 2017). Because they are highly polar, less toxic, and easy to remove, organic ligands like polycarboxylic acid are a good choice (Shen et al., 2015). Computational techniques based on artificial intelligence-based mathematical/simulation models may be used to develop predictive software to forecast their behaviour in biological systems (Winkler, 2020). This enables high throughput screening before in vitro and in vivo studies. The potential dangers related to the biological interactions might be decreased by predicting the potential cytotoxicity of NPs based on their geometric and physicochemical features (Bai et al., 2017).
Regulatory aspects of MNPs
The top-down strategy and the bottom-up approach are employed to create MNPs. Three physical, chemical, and biological strategies can be used to synthesize these techniques. Coprecipitation, reduction, sonochemical, solvothermal, and other chemical processes are available, while discharge, milling, and ion implantation are examples of physical procedures. Plants and their extracts, agricultural byproducts, microbes, and seaweed are examples of biological processes. They are characterized using a variety of methods, including transmission electron microscopy, dynamic light scanning, and scanning electron microscopy. All MNPs have unique visual, electrical, magnetic, and chemical capabilities and are biocompatible. They are utilized in several sectors, including the pharmaceutical sector where they serve as biosensors, anticancer agents, antibacterial, antifungal, and antioxidants. The most popular MNPs utilized in the pharmaceutical sector include gold, silver, iron oxide, zinc oxide, platinum, copper oxide, and palladium. In general, monometallic, and multi-metallic NPs fall within this category. So, employing living organisms like fungi, bacteria, and plants to synthesize MNPs is an alternative method. Numerous studies have demonstrated that the experimental conditions, the stabilizing agent’s process of adsorption, and the kinetics of the interaction of metal ions with reducing agents have a significant impact on the characteristics of MNPs like size, stability, physical, and chemical properties, and morphology. Due to their distinct physical and chemical characteristics from bulk metals, MNPs have drawn attention in a variety of industrial applications with a variety of characteristics, including magnetic and optical capabilities, high surface area, low melting point, and mechanical strengths. MNPs catalysts are extremely active, selective, and have a long lifespan for a variety of chemical processes. Only the optical qualities of the randomly oriented gold nanorods placed on the disc make this possible (Badawy et al., 2021; Doghish et al., 2022; Hashem et al., 2022; Salem et al., 2022; Soliman et al., 2022).
Conclusion
Metallic nanoparticles synthesized using biogenic materials have gained significant attention due to their unique properties, eco-friendliness, and potential for various applications in medicines, sensing, food preservations, and other consumable products. The use of biological sources as reducing and capping agents for the synthesis of these MNPs have shown several advantages over conventional synthetic methods, including low cost, sustainability, and the absence of toxic chemicals. Moreover, BMNPs are significantly utilized for multifarious applications such as analytical sensing of toxic chemciacals, biomedical applications, food preservations, waste-water remediation, coating of surgical deveices, and in house appliance. Although BMNPs holds great potential, their commercial use is still in the early stages of development, and much research is required to fully understand their properties and potential applications. Nonetheless, the increasing interest in these MNPs indicates a promising future for their use in various fields, and it is expected that more discoveries and advancements will be made in near future.
Author contributions
BP and SS: conceptualization, reviewing, and editing. AiK, SRS, TJJ, AhK, AP, BP, SS, and DK writing-original draft. All authors have read and agreed to the published version of the manuscript.
Conflict of interest
The authors declare that the research was conducted in the absence of any commercial or financial relationships that could be construed as a potential conflict of interest.
Publisher’s note
All claims expressed in this article are solely those of the authors and do not necessarily represent those of their affiliated organizations, or those of the publisher, the editors and the reviewers. Any product that may be evaluated in this article, or claim that may be made by its manufacturer, is not guaranteed or endorsed by the publisher.
Abbreviations
AA, Ascorbic acid; BMNPs, Biogenic metallic nanoparticles; CuNPs, Copper nanoparticles; DSC, Differential scanning calorimeter; DLS, Dynamic light scattering; FESEM, Field emission scan electron microscopy; TEM, Transmission electron microscopy; UV-vis, UV-vis absorption; XRD, X-ray diffraction; SERS, Surface-enhanced Raman spectroscopy; TGA, Thermogravimetric analysis; AuNPs, Gold nanoparticles; ICP-MS, Inductively coupled plasma mass spectrometry; FeONPS, Iron oxide nanoparticles; LOD, Limit of detection; MNPs, Metallic nanoparticles; PdNPs, Palladium nanoparticles; PMMA, Poly (methyl methacrylate); RT, Room temperature; AgNPs, Silver nanoparticles; NaBH4, Sodium borohydride; SRP, Standard reduction potential; TiO2NPs, Titanium dioxide nanoparticles; ZnNPs, Zinc oxide nanoparticles; RT, Room temperature; SRP, Standard reduction potential; NaBH4, Sodium borohydride.
References
Abbaszadegan, M., Hasan, M. N., Gerba, C. P., Roessler, P. F., Wilson, B. R., Kuennen, R., et al. (1997). The disinfection efficacy of a point-of-use water treatment system against bacterial, viral and protozoan waterborne pathogens. Water Res. 31 (3), 574–582. doi:10.1016/s0043-1354(96)00263-1
Abdal Dayem, A., Hossain, M. K., Lee, S. B., Kim, K., Saha, S. K., Yang, G-M., et al. (2017). The role of reactive oxygen species (ROS) in the biological activities of metallic nanoparticles. Int. J. Mol. Sci. 18 (1), 120. doi:10.3390/ijms18010120
Abdelaziz, A. M., Salem, S. S., Khalil, A. M. A., El-Wakil, D. A., Fouda, H. M., and Hashem, A. H. (2022). Potential of biosynthesized zinc oxide nanoparticles to control Fusarium wilt disease in eggplant (Solanum melongena) and promote plant growth. BioMetals 35 (3), 601–616. doi:10.1007/s10534-022-00391-8
Abdelghany, T. M., Al-Rajhi, A. M. H., Yahya, R., Bakri, M. M., Al Abboud, M. A., Yahya, R., et al. (2023). Phytofabrication of zinc oxide nanoparticles with advanced characterization and its antioxidant, anticancer, and antimicrobial activity against pathogenic microorganisms. Biomass Convers. Biorefinery 13 (1), 417–430. doi:10.1007/s13399-022-03412-1
Abdelmoneim, H. E. M., Wassel, M. A., Elfeky, A. S., Bendary, S. H., Awad, M. A., Salem, S. S., et al. (2022). Multiple applications of CdS/TiO2 nanocomposites synthesized via microwave-assisted sol–gel. J. Clust. Sci. 33 (3), 1119–1128. doi:10.1007/s10876-021-02041-4
Ahmed, J., Arfat, Y. A., Bher, A., Mulla, M., Jacob, H., and Auras, R. (2018). Active chicken meat packaging based on polylactide films and bimetallic AgCu nanoparticles and essential oil. J. food Sci. 83 (5), 1299–1310. doi:10.1111/1750-3841.14121
Ahn, S-J., Lee, S-J., Kook, J-K., and Lim, B-S. (2009). Experimental antimicrobial orthodontic adhesives using nanofillers and silver nanoparticles. Dent. Mat. 25 (2), 206–213. doi:10.1016/j.dental.2008.06.002
Al-Rajhi, A. M. H., Salem, S. S., Alharbi, A. A., and Abdelghany, T. M. (2022). Ecofriendly synthesis of silver nanoparticles using Kei-apple (Dovyalis caffra) fruit and their efficacy against cancer cells and clinical pathogenic microorganisms. Arabian J. Chem. 15 (7), 103927. doi:10.1016/j.arabjc.2022.103927
Al-Zahrani, F. A. M., Al-Zahrani, N. A., Al-Ghamdi, S. N., Lin, L., Salem, S. S., and El-Shishtawy, R. M. (2022). Synthesis of Ag/Fe2O3 nanocomposite from essential oil of ginger via green method and its bactericidal activity. Biomass Conversion and Biorefinery.
Al-Zahrani, F. A. M., Salem, S. S., Al-Ghamdi, H. A., Nhari, L. M., Lin, L., and El-Shishtawy, R. M. (2022). Green synthesis and antibacterial activity of Ag/Fe2O3 nanocomposite using buddleja lindleyana extract. Bioengineering 9 (9), 452. doi:10.3390/bioengineering9090452
Ali, H., and Khan, E. (2018). What are heavy metals? Long-Standing controversy over the scientific use of the term ‘heavy metals’–proposal of a comprehensive definition. Toxicol. Environ. Chem. 100 (1), 6–19. doi:10.1080/02772248.2017.1413652
Alsaiari, N. S., Alzahrani, F. M., Amari, A., Osman, H., Harharah, H. N., Elboughdiri, N., et al. (2023). Plant and microbial approaches as green methods for the synthesis of nanomaterials: Synthesis, applications, and future perspectives. Molecules 28 (1), 463. doi:10.3390/molecules28010463
An, J., Zhang, M., Wang, S., and Tang, J. (2008). Physical, chemical and microbiological changes in stored green asparagus spears as affected by coating of silver nanoparticles-PVP. LWT-Food Sci. Technol. 41 (6), 1100–1107. doi:10.1016/j.lwt.2007.06.019
An, K., and Somorjai, G. A. (2012). Size and shape control of metal nanoparticles for reaction selectivity in catalysis. ChemCatChem 4 (10), 1512–1524. doi:10.1002/cctc.201200229
An, L., Zhang, D., Zhang, L., and Feng, G. (2019). Effect of nanoparticle size on the mechanical properties of nanoparticle assemblies. Nanoscale 11 (19), 9563–9573. doi:10.1039/c9nr01082c
Andra, S., Balu, S. K., Jeevanandham, J., Muthalagu, M., Vidyavathy, M., Chan, Y. S., et al. (2019). Phytosynthesized metal oxide nanoparticles for pharmaceutical applications. Naunyn-Schmiedeberg's Archives Pharmacol. 392 (7), 755–771. doi:10.1007/s00210-019-01666-7
Annadhasan, M., Kasthuri, J., and Rajendiran, N. (2015). Green synthesis of gold nanoparticles under sunlight irradiation and their colorimetric detection of Ni2+ and Co2+ ions. RSC Adv. 5 (15), 11458–11468. doi:10.1039/c4ra14034f
Annadhasan, M., Muthukumarasamyvel, T., Sankar Babu, V., and Rajendiran, N. (2014). Green synthesized silver and gold nanoparticles for colorimetric detection of Hg2+, Pb2+, and Mn2+ in aqueous medium. ACS Sustain. Chem. Eng. 2 (4), 887–896. doi:10.1021/sc400500z
Applerot, G., Lellouche, J., Lipovsky, A., Nitzan, Y., Lubart, R., Gedanken, A., et al. (2012). Understanding the antibacterial mechanism of CuO nanoparticles: Revealing the route of induced oxidative stress. Small 8 (21), 3326–3337. doi:10.1002/smll.201200772
Aref, M. S., and Salem, S. S. (2020). Bio-callus synthesis of silver nanoparticles, characterization, and antibacterial activities via Cinnamomum camphora callus culture. Biocatal. Agric. Biotechnol. 27, 101689. doi:10.1016/j.bcab.2020.101689
Arfat, Y. A., Benjakul, S., Prodpran, T., Sumpavapol, P., and Songtipya, P. (2016). Physico-mechanical characterization and antimicrobial properties of fish protein isolate/fish skin gelatin-zinc oxide (ZnO) nanocomposite films. Food Bioprocess Technol. 9, 101–112. doi:10.1007/s11947-015-1602-0
Badawy, A. A., Abdelfattah, N. A. H., Salem, S. S., Awad, M. F., and Fouda, A. (2021). Efficacy assessment of biosynthesized copper oxide nanoparticles (CuONPs) on stored grain insects and their impacts on morphological and physiological traits of wheat (Triticum aestivum L.). Plant. Biol. 10 (3), 233. doi:10.3390/biology10030233
Bahrami, A., Mokarram, R. R., Khiabani, M. S., Ghanbarzadeh, B., and Salehi, R. (2019). Physico-mechanical and antimicrobial properties of tragacanth/hydroxypropyl methylcellulose/beeswax edible films reinforced with silver nanoparticles. Int. J. Biol. Macromol. 129, 1103–1112. doi:10.1016/j.ijbiomac.2018.09.045
Bai, X., Liu, F., Liu, Y., Li, C., Wang, S., Zhou, H., et al. (2017). Toward a systematic exploration of nano-bio interactions. Toxicol. Appl. Pharmacol. 323, 66–73. doi:10.1016/j.taap.2017.03.011
Balmuri, S. R., Selvaraj, U., Kumar, V. V., Anthony, S. P., Tsatsakis, A. M., Golokhvast, K. S., et al. (2017). Effect of surfactant in mitigating cadmium oxide nanoparticle toxicity: Implications for mitigating cadmium toxicity in environment. Environ. Res. 152, 141–149. doi:10.1016/j.envres.2016.10.005
Basiri, S., Mehdinia, A., Jabbari, A. J. C., Physicochemical, S. A., and Aspects, E. (2018). A sensitive triple colorimetric sensor based on plasmonic response quenching of green synthesized silver nanoparticles for determination of Fe2+, hydrogen peroxide, and glucose. Colloids Surfaces A Physicochem. Eng. Aspects 545, 138–146. doi:10.1016/j.colsurfa.2018.02.053
Bundschuh, M., Filser, J., Lüderwald, S., McKee, M. S., Metreveli, G., Schaumann, G. E., et al. (2018). Nanoparticles in the environment: Where do we come from, where do we go to? Environ. Sci. Eur. 30 (1), 6–17. doi:10.1186/s12302-018-0132-6
Chalchisa, D., Megersa, M., and Beyene, A. (2018). Assessment of the quality of drinking water in storage tanks and its implication on the safety of urban water supply in developing countries. Environ. Syst. Res. 6 (1), 12–16. doi:10.1186/s40068-017-0089-2
Chang, Z., Qiu, J., Wang, K., Liu, X., Fan, L., Liu, X., et al. (2023). The relationship between Co-exposure to multiple heavy metals and liver damage. J. Trace Elem. Med. Biol. 77, 127128. doi:10.1016/j.jtemb.2023.127128
Chaudhary, J. P., Kumar, A., Paul, P., and Meena, R. (2015). Carboxymethylagarose-AuNPs generated through green route for selective detection of Hg2+ in aqueous medium with a blue shift. Carbohydr. Polym. 117, 537–542. doi:10.1016/j.carbpol.2014.10.016
Cheon, J. Y., and Park, W. H. (2016). Green synthesis of silver nanoparticles stabilized with mussel-inspired protein and colorimetric sensing of lead (II) and copper (II) ions. Int. J. Mol. Sci. 17 (12), 2006. doi:10.3390/ijms17122006
Desai, M. P., Paiva-Santos, A. C., Nimbalkar, M. S., Sonawane, K. D., Patil, P. S., and Pawar, K. D. (2023). Iron tolerant Bacillus badius mediated bimetallic magnetic iron oxide and gold nanoparticles as Doxorubicin carrier and for hyperthermia treatment. J. Drug Deliv. Sci. Technol. 81, 104214. doi:10.1016/j.jddst.2023.104214
Ding, N., Cao, Q., Zhao, H., Yang, Y., Zeng, L., He, Y., et al. (2010). Colorimetric assay for determination of lead (II) based on its incorporation into gold nanoparticles during their synthesis. sensors 10 (12), 11144–11155. doi:10.3390/s101211144
Doghish, A. S., Hashem, A. H., Shehabeldine, A. M., Sallam, A. A. M., El-Sayyad, G. S., and Salem, S. S. (2022). Nanocomposite based on gold nanoparticles and carboxymethyl cellulose: Synthesis, characterization, antimicrobial, and anticancer activities. J. Drug Deliv. Sci. Technol. 77, 103874. doi:10.1016/j.jddst.2022.103874
Dutt, Y., Pandey, R. P., Dutt, M., Gupta, A., Vibhuti, A., Raj, V. S., et al. (2023). Silver nanoparticles phytofabricated through Azadirachta indica: Anticancer, apoptotic, and wound-healing properties. Antibiotics 12 (1), 121. doi:10.3390/antibiotics12010121
Ebrahimi, A., Samari, F., Eftekhar, E., and Yousefinejad, S. (2022). Rapid and efficient colorimetric sensing of clindamycin and Fe3+ using controllable phyto-synthesized silver/silver chloride nanoparticles by Syzygium cumini fruit extract. J. Anal. Sci. Technol. 13 (1), 10–16. doi:10.1186/s40543-022-00318-5
Eid, A. M., Fouda, A., Niedbała, G., Hassan, S. E., Salem, S. S., Abdo, A. M., et al. (2020). Endophytic Streptomyces laurentii mediated green synthesis of ag-nps with antibacterial and anticancer properties for developing functional textile fabric properties. Antibiotics 9 (10), 641. doi:10.3390/antibiotics9100641
Eivazzadeh-Keihan, R., Bahojb Noruzi, E., Khanmohammadi Chenab, K., Jafari, A., Radinekiyan, F., Hashemi, S. M., et al. (2020). Metal-based nanoparticles for bone tissue engineering. J. Tissue Eng. Regen. Med. 14 (12), 1687–1714. doi:10.1002/term.3131
Eze, F. N., and Nwabor, O. F. (2020). Valorization of Pichia spent medium via one-pot synthesis of biocompatible silver nanoparticles with potent antioxidant, antimicrobial, tyrosinase inhibitory and reusable catalytic activities. Mater. Sci. Eng. C 115, 111104. doi:10.1016/j.msec.2020.111104
Fernández, A., Soriano, E., López-Carballo, G., Picouet, P., Lloret, E., Gavara, R., et al. (2009). Preservation of aseptic conditions in absorbent pads by using silver nanotechnology. Food Res. Int. 42 (8), 1105–1112. doi:10.1016/j.foodres.2009.05.009
Fouda, A., El-Din Hassan, S., Salem, S. S., and Shaheen, T. I. (2018). In-vitro cytotoxicity, antibacterial, and UV protection properties of the biosynthesized Zinc oxide nanoparticles for medical textile applications. Microb. Pathog. 125, 252–261. doi:10.1016/j.micpath.2018.09.030
Fouda, A., Salem, S. S., Wassel, A. R., Hamza, M. F., and Shaheen, T. I. (2020). Optimization of green biosynthesized visible light active CuO/ZnO nano-photocatalysts for the degradation of organic methylene blue dye. Heliyon 6 (9), e04896. doi:10.1016/j.heliyon.2020.e04896
Ghosh, S., and Mondal, A. (2020). Aggregation chemistry of green silver nanoparticles for sensing of Hg2+ and Cd2+ ions. Colloids Surfaces A Physicochem. Eng. Asp. 605, 125335. doi:10.1016/j.colsurfa.2020.125335
Grijalva, M., Vallejo-López, M. J., Salazar, L., Camacho, J., and Kumar, B. (2018). “Cytotoxic and antiproliferative effects of nanomaterials on cancer cell lines: A review,” in Unraveling the safety profile of nanoscale particles and materials from biomedical to environmental applications, 63–85.
Habibullah, G., Viktorova, J., and Ruml, T. (2021). Current strategies for noble metal nanoparticle synthesis. Nanoscale Res. Lett. 16 (1), 47–12. doi:10.1186/s11671-021-03480-8
Hamad, A. F., Han, J-H., Kim, B-C., and Rather, I. A. (2018). The intertwine of nanotechnology with the food industry. Saudi J. Biol. Sci. 25 (1), 27–30. doi:10.1016/j.sjbs.2017.09.004
Hammad, E. N., Salem, S. S., Mohamed, A. A., and El-Dougdoug, W. (2022). Environmental impacts of ecofriendly iron oxide nanoparticles on dyes removal and antibacterial activity. Appl. Biochem. Biotechnol. 194 (12), 6053–6067. doi:10.1007/s12010-022-04105-1
Han, Z., Dong, L., Zhang, J., Cui, T., Chen, S., Ma, G., et al. (2019). Green synthesis of palladium nanoparticles using lentinan for catalytic activity and biological applications. RSC Adv. 9 (65), 38265–38270. doi:10.1039/c9ra08051a
Hashem, A. H., Selim, T. A., Alruhaili, M. H., Selim, S., Alkhalifah, D. H. M., Al Jaouni, S. K., et al. (2022). Unveiling antimicrobial and insecticidal activities of biosynthesized selenium nanoparticles using prickly pear peel waste. J. Funct. Biomaterials 13 (3), 112. doi:10.3390/jfb13030112
Hassanpour, P., Panahi, Y., Ebrahimi Kalan, A., Akbarzadeh, A., Davaran, S., Nasibova, A. N., et al. (2018). Biomedical applications of aluminium oxide nanoparticles. Micro Nano Lett. 13 (9), 1227–1231. doi:10.1049/mnl.2018.5070
Henkel, C., Wittmann, J. E., Träg, J., Will, J., Stiegler, L. M., Strohriegl, P., et al. (2020). Mixed organic ligand shells: Controlling the nanoparticle surface morphology toward tuning the optoelectronic properties. Small 16 (2), 1903729. doi:10.1002/smll.201903729
Hosseinzadeh, S., Partovi, R., Talebi, F., and Babaei, A. (2020). Chitosan/TiO2 nanoparticle/Cymbopogon citratus essential oil film as food packaging material: Physico-mechanical properties and its effects on microbial, chemical, and organoleptic quality of minced meat during refrigeration. J. Food Process. Preserv. 44 (7), e14536. doi:10.1111/jfpp.14536
Jabariyan, S., and Zanjanchi, M. A. (2019). Colorimetric detection of cadmium ions using modified silver nanoparticles. Appl. Phys. A 125 (12), 872–910. doi:10.1007/s00339-019-3167-7
Jana, J., Ganguly, M., and Pal, T. (2016). Enlightening surface plasmon resonance effect of metal nanoparticles for practical spectroscopic application. RSC Adv. 6 (89), 86174–86211. doi:10.1039/c6ra14173k
Jayarambabu, N., Akshaykranth, A., Rao, T. V., Rao, K. V., and Kumar, R. R. (2020). Green synthesis of Cu nanoparticles using Curcuma longa extract and their application in antimicrobial activity. Mat. Lett. 259, 126813. doi:10.1016/j.matlet.2019.126813
Jayeoye, T. J., Eze, F. N., Olatunde, O. O., Singh, S., Zuo, J., and Olatunji, O. J. (2021). Multifarious biological applications and toxic Hg2+ sensing potentiality of biogenic silver nanoparticles based on securidaca inappendiculata hassk stem extract. Int. J. Nanomedicine 16, 7557–7574. doi:10.2147/ijn.s325996
Jayeoye, T. J., Eze, F. N., Olatunji, O. J., and Tyopine, A. A. (2022). Synthesis of biocompatible Konjac glucomannan stabilized silver nanoparticles, with Asystasia gangetica phenolic extract for colorimetric detection of mercury (II) ion. Sci. Rep. 12 (1), 9176–9215. doi:10.1038/s41598-022-13384-x
Jayeoye, T. J., Eze, F. N., Singh, S., Olatunde, O. O., Benjakul, S., and Rujiralai, T. (2021). Synthesis of gold nanoparticles/polyaniline boronic acid/sodium alginate aqueous nanocomposite based on chemical oxidative polymerization for biological applications. Int. J. Biol. Macromol. 179, 196–205. doi:10.1016/j.ijbiomac.2021.02.199
Jayeoye, T. J., and Rujiralai, T. (2020). Green, in situ fabrication of silver/poly (3-aminophenyl boronic acid)/sodium alginate nanogel and hydrogen peroxide sensing capacity. Carbohydr. Polym. 246, 116657. doi:10.1016/j.carbpol.2020.116657
Jayeoye, T. J., Sirimahachai, U., and Rujiralai, T. (2021). Sensitive colorimetric detection of ascorbic acid based on seed mediated growth of sodium alginate reduced/stabilized gold nanoparticles. Carbohydr. Polym. 255, 117376. doi:10.1016/j.carbpol.2020.117376
Jeon, H., and Lee, K. (2019). Effect of gold nanoparticle morphology on thermal properties of polyimide nanocomposite films. Colloids Surfaces A Physicochem. Eng. Aspects 579, 123651. doi:10.1016/j.colsurfa.2019.123651
Kalimuthu, K., Cha, B. S., Kim, S., and Park, K. S. (2020). Eco-friendly synthesis and biomedical applications of gold nanoparticles: A review. A Rev. 152, 104296. doi:10.1016/j.microc.2019.104296
Khan, N. A., Khan, S. U., Ahmed, S., Farooqi, I. H., Dhingra, A., Hussain, A., et al. (2019). Applications of nanotechnology in water and wastewater treatment: A review. Asian J. Water, Environ. Pollut. 16 (4), 81–86. doi:10.3233/ajw190051
Kim, M., Jee, S-C., Shinde, S. K., Mistry, B. M., Saratale, R. G., Saratale, G. D., et al. (2019). Green-synthesis of anisotropic peptone-silver nanoparticles and its potential application as anti-bacterial agent. Polymers 11 (2), 271. doi:10.3390/polym11020271
Kraft, A. (2008). Electrochemical water disinfection: A short review. Platin. Met. Rev. 52 (3), 177–185. doi:10.1595/147106708x329273
Kumar, S., Jha, I., Mogha, N. K., and Venkatesu, P. (2020). Biocompatibility of surface-modified gold nanoparticles towards red blood cells and haemoglobin. Appl. Surf. Sci. 512, 145573. doi:10.1016/j.apsusc.2020.145573
Lakshmiprasanna, H. R., Jagadeesha Angadi, V., Rajesh Babu, B., Pasha, M., Manjunatha, K., and Matteppanavar, S. (2019). Effect of Pr3+-doping on the structural, elastic and magnetic properties of Mn–Zn ferrite nanoparticles prepared by solution combustion synthesis method. Chem. Data Collect. 24, 100273. doi:10.1016/j.cdc.2019.100273
Lamouri, R., Mounkachi, O., Salmani, E., Hamedoun, M., Benyoussef, A., and Ez-Zahraouy, H. (2020). Size effect on the magnetic properties of CoFe2O4 nanoparticles: A Monte Carlo study. Ceram. Int. 46 (6), 8092–8096. doi:10.1016/j.ceramint.2019.12.035
Li, L., Zhao, C., Zhang, Y., Yao, J., Yang, W., Hu, Q., et al. (2017). Effect of stable antimicrobial nano-silver packaging on inhibiting mildew and in storage of rice. Food Chem. 215, 477–482. doi:10.1016/j.foodchem.2016.08.013
Li, Q., Mahendra, S., Lyon, D. Y., Brunet, L., Liga, M. V., Li, D., et al. (2008). Antimicrobial nanomaterials for water disinfection and microbial control: Potential applications and implications. Water Res. 42 (18), 4591–4602. doi:10.1016/j.watres.2008.08.015
Mahajan, P. G., Dige, N. C., Vanjare, B. D., Hong, S-K., and Lee, K. H. (2019). Gallotannin functionalized gold nanoparticles for rapid, selective and sensitive colorimetric detection of Ag+ in aqueous medium: Approach to eco-friendly water analysis. Sensors Actuators B Chem. 281, 720–729. doi:10.1016/j.snb.2018.10.116
Mahdi, H. S., and Parveen, A. (2019). Biosynthesis, Characterization and antibacterial activity of gold nanoparticles (Au-NPs) using black lemon extract. Mater. Today Proc. 18, 5164–5169. doi:10.1016/j.matpr.2019.07.514
Mao, B-H., Chen, Z-Y., Wang, Y-J., and Yan, S-J. (2018). Silver nanoparticles have lethal and sublethal adverse effects on development and longevity by inducing ROS-mediated stress responses. Sci. Rep. 8 (1), 2445–2516. doi:10.1038/s41598-018-20728-z
Martemucci, G., Costagliola, C., Mariano, M., D’andrea, L., Napolitano, P., and D’Alessandro, A. G. (2022). Free radical properties, source and targets, antioxidant consumption and health. Oxygen 2 (2), 48–78. doi:10.3390/oxygen2020006
Mata, R., Nakkala, J. R., and Sadras, S. R. (2023). Therapeutic role of biogenic silver and gold nanoparticles against a DMH-induced colon cancer model. Biomater. Adv. 146, 213279. doi:10.1016/j.bioadv.2023.213279
Memon, R., Memon, A. A., Sherazi, S. T. H., Uddin, S., Balouch, A., Shah, M. R., et al. (2020). Application of synthesized copper nanoparticles using aqueous extract ofZiziphus mauritiana L. leaves as a colorimetric sensor for the detection of Ag+. Turkish J. Chem. 44 (5), 1376–1385. doi:10.3906/kim-2001-51
Mikhailov, M. M., Yuryev, S. A., and Lovitskiy, A. A. (2018). On the correlation between diffuse reflectance spectra and particle size of BaSO4 powder under heating and modifying with SiO2 nanoparticles. Opt. Mater. 85, 226–229. doi:10.1016/j.optmat.2018.08.059
Mohandoss, S., Murugaboopathy, V., Haricharan, P. B., Hebbal, M. I., Saadaldin, S., Soliman, M., et al. (2023). Ulvan as a reducing agent for the green synthesis of silver nanoparticles: A novel mouthwash. Inorganics 11 (1), 5. doi:10.3390/inorganics11010005
Murthy, S., Effiong, P., and Fei, C. C. (2020). “Metal oxide nanoparticles in biomedical applications,” in Metal oxide powder technologies (Elsevier), 233–251.
Nwabor, O. F., Singh, S., Ontong, J. C., Vongkamjan, K., and Voravuthikunchai, S. P. (2021b). Valorization of wastepaper through antimicrobial functionalization with biogenic silver nanoparticles, a sustainable packaging composite. Waste Biomass Valorization 12 (6), 3287–3301. doi:10.1007/s12649-020-01237-5
Nwabor, O. F., Singh, S., Paosen, S., Vongkamjan, K., and Voravuthikunchai, S. P. (2020). Enhancement of food shelf life with polyvinyl alcohol-chitosan nanocomposite films from bioactive Eucalyptus leaf extracts. Food Biosci. 36, 100609. doi:10.1016/j.fbio.2020.100609
Nwabor, O. F., Singh, S., Wunnoo, S., Lerwittayanon, K., and Voravuthikunchai, S. P. (2021a). Facile deposition of biogenic silver nanoparticles on porous alumina discs, an efficient antimicrobial, antibiofilm, and antifouling strategy for functional contact surfaces. Biofouling 37 (5), 538–554. doi:10.1080/08927014.2021.1934457
Olmos, D., Pontes-Quero, G. M., Corral, A., González-Gaitano, G., and González-Benito, J. (2018). Preparation and characterization of antimicrobial films based on LDPE/Ag nanoparticles with potential uses in food and health industries. Nanomaterials 8 (2), 60. doi:10.3390/nano8020060
Ontong, J. C., Singh, S., Nwabor, O. F., Chusri, S., and Voravuthikunchai, S. P. (2020). Potential of antimicrobial topical gel with synthesized biogenic silver nanoparticle using Rhodomyrtus tomentosa leaf extract and silk sericin. Biotechnol. Lett. 42 (12), 2653–2664. doi:10.1007/s10529-020-02971-5
Onyeaka, H., Passaretti, P., Miri, T., and Al-Sharify, Z. T. (2022). The safety of nanomaterials in food production and packaging. Curr. Res. Food Sci. 5, 763–774. doi:10.1016/j.crfs.2022.04.005
Pandey, V. K., Upadhyay, S. N., Niranjan, K., and Mishra, P. K. (2020). Antimicrobial biodegradable chitosan-based composite Nano-layers for food packaging. Int. J. Biol. Macromol. 157, 212–219. doi:10.1016/j.ijbiomac.2020.04.149
Parameshwaran, R., and Kalaiselvam, S. (2014). Energy conservative air conditioning system using silver nano-based PCM thermal storage for modern buildings. Energy Build. 69, 202–212. doi:10.1016/j.enbuild.2013.09.052
Prakash, J., Vignesh, K., Anusuya, T., Kalaivani, T., Ramachandran, C., Sudha, R. R., et al. (2019). Application of nanoparticles in food preservation and food processing. J. Food Hyg. Saf. 34 (4), 317–324. doi:10.13103/jfhs.2019.34.4.317
Primožič, M., Knez, Ž., and Leitgeb, M. (2021). (Bio) Nanotechnology in food science food packaging. Nanomaterials 11 (2), 292. doi:10.3390/nano11020292
Ragunathan, S. C. B., Rejeeth, C., Muthusamy, G., Abdulhaniff, P., and Palvannan, T. (2022). Green synthesis of silver nanoparticles from corn cob aqueous extract for colorimetric cysteine detection in serum simulated with cysteine samples. Optik 264, 169381. doi:10.1016/j.ijleo.2022.169381
Ramji, V., and Vishnuvarthanan, M. (2022). Influence of NiO supported silica nanoparticles on mechanical, barrier, optical and antibacterial properties of polylactic acid (PLA) bio nanocomposite films for food packaging applications. Silicon 14 (2), 531–538. doi:10.1007/s12633-020-00839-x
Rastogi, L., Dash, K., and Ballal, A. (2017). Selective colorimetric/visual detection of Al3+ in ground water using ascorbic acid capped gold nanoparticles. Sensors Actuators B Chem. 248, 124–132. doi:10.1016/j.snb.2017.03.138
Ribeiro, L. G., Roque, G. S. C., Conrado, R., and De Souza, A. O. (2023). Antifungal activity of mycogenic silver nanoparticles on clinical yeasts and phytopathogens. Antibiotics 12 (1), 91. doi:10.3390/antibiotics12010091
Rikta, S., Tareq, S. M., and Uddin, M. K. (2018). Toxic metals (Ni 2+, Pb 2+, Hg 2+) binding affinity of dissolved organic matter (DOM) derived from different ages municipal landfill leachate. Appl. water Sci. 8, 5–8. doi:10.1007/s13201-018-0642-9
Ringe, E., Langille, M. R., Sohn, K., Zhang, J., Huang, J., Mirkin, C. A., et al. (2012). Plasmon length: A universal parameter to describe size effects in gold nanoparticles. J. Phys. Chem. Lett. 3 (11), 1479–1483. doi:10.1021/jz300426p
Roy, K., Ghosh, C. K., and Sarkar, C. K. (2017). Degradation of toxic textile dyes and detection of hazardous Hg2+ by low-cost bioengineered copper nanoparticles synthesized using Impatiens balsamina leaf extract. Mater. Res. Bull. 94, 257–262. doi:10.1016/j.materresbull.2017.06.016
Saied, E., Eid, A. M., Hassan, S. E., Salem, S. S., Radwan, A. A., Halawa, M., et al. (2021). The catalytic activity of biosynthesized magnesium oxide nanoparticles (MgO-NPs) for inhibiting the growth of pathogenic microbes, tanning effluent treatment, and chromium ion removal. Catalysts 11 (7), 821. doi:10.3390/catal11070821
Saied, E., Salem, S. S., Al-Askar, A. A., Elkady, F. M., Arishi, A. A., and Hashem, A. H. (2022). Mycosynthesis of hematite (alpha;-Fe2O3) nanoparticles using Aspergillus Niger and their antimicrobial and photocatalytic activities. Bioengineering 9 (8), 397. doi:10.3390/bioengineering9080397
Salem, S. S., Ali, O. M., Reyad, A. M., Abd-Elsalam, K. A., and Hashem, A. H. (2022). Pseudomonas indica-mediated silver nanoparticles: Antifungal and antioxidant biogenic tool for suppressing mucormycosis fungi. J. Fungi 8 (2), 126. doi:10.3390/jof8020126
Salem, S. S. (2022). Baker’s yeast-mediated silver nanoparticles: Characterisation and antimicrobial biogenic tool for suppressing pathogenic microbes. BioNanoScience 12 (4), 1220–1229. doi:10.1007/s12668-022-01026-5
Salem, S. S. (2022). Bio-fabrication of selenium nanoparticles using baker’s yeast extract and its antimicrobial efficacy on food borne pathogens. Appl. Biochem. Biotechnol. 194 (5), 1898–1910. doi:10.1007/s12010-022-03809-8
Salem, S. S., El-Belely, E. F., Niedbała, G., Alnoman, M. M., Hassan, S. E., Eid, A. M., et al. (2020). Bactericidal and in-vitro cytotoxic efficacy of silver nanoparticles (Ag-NPs) fabricated by endophytic actinomycetes and their use as coating for the textile fabrics. Nanomaterials 10 (10), 2082. doi:10.3390/nano10102082
Salem, S. S., and Fouda, A. (2021). Green synthesis of metallic nanoparticles and their prospective biotechnological applications: An overview. Biol. Trace Elem. Res. 199 (1), 344–370. doi:10.1007/s12011-020-02138-3
Samari, F., Baluchi, L., Salehipoor, H., and Yousefinejad, S. (2019). Controllable phyto-synthesis of cupric oxide nanoparticles by aqueous extract of Capparis spinosa (caper) leaves and application in iron sensing. Microchem. J. 150, 104158. doi:10.1016/j.microc.2019.104158
Sani, M. A., Ehsani, A., and Hashemi, M. (2017). Whey protein isolate/cellulose nanofibre/TiO2 nanoparticle/rosemary essential oil nanocomposite film: Its effect on microbial and sensory quality of lamb meat and growth of common foodborne pathogenic bacteria during refrigeration. Int. J. food Microbiol. 251, 8–14. doi:10.1016/j.ijfoodmicro.2017.03.018
Sapyen, W., Toonchue, S., Praphairaksit, N., and Imyim, A. (2022). Selective colorimetric detection of Cr (VI) using starch-stabilized silver nanoparticles and application for chromium speciation. Spectrochim. Acta Part A Mol. Biomol. Spectrosc. 274, 121094. doi:10.1016/j.saa.2022.121094
Seku, K., Gangapuram, B. R., Pejjai, B., Kadimpati, K. K., and Golla, N. (2018). Microwave-assisted synthesis of silver nanoparticles and their application in catalytic, antibacterial and antioxidant activities. J. Nanostructure Chem. 8 (2), 179–188. doi:10.1007/s40097-018-0264-7
Shahanaz, L., and Shalini, A. S. (2023). Zinc oxide nanoparticles synthesized using Oldenlandia Umbellata leaf extract and their photocatalytic and biological characteristics. J. Indian Chem. Soc. 100 (2), 100917. doi:10.1016/j.jics.2023.100917
Shaheen, T. I., Fouda, A., and Salem, S. S. (2021). Integration of cotton fabrics with biosynthesized cuo nanoparticles for bactericidal activity in the terms of their cytotoxicity assessment. Industrial Eng. Chem. Res. 60 (4), 1553–1563. doi:10.1021/acs.iecr.0c04880
Shaheen, T. I., Salem, S. S., and Fouda, A. (2021). “Current advances in fungal nanobiotechnology: Mycofabrication and applications,” in Microbial nanobiotechnology: Principles and applications. Editors A. Lateef, E. B. Gueguim-Kana, N. Dasgupta, and S. Ranjan (Singapore: Springer Singapore), 113–143.
Shehabeldine, A. M., Amin, B. H., Hagras, F. A., Ramadan, A. A., Kamel, M. R., Ahmed, M. A., et al. (2023). Potential antimicrobial and antibiofilm properties of copper oxide nanoparticles: Time-kill kinetic essay and ultrastructure of pathogenic bacterial cells. Appl. Biochem. Biotechnol. 195 (1), 467–485. doi:10.1007/s12010-022-04120-2
Shen, L., Xu, H., and Yang, Y. (2015). Quantitative correlation between cross-linking degrees and mechanical properties of protein films modified with polycarboxylic acids. Macromol. Mater. Eng. 300 (11), 1133–1140. doi:10.1002/mame.201500145
Shin, S. W., Song, I. H., and Um, S. H. (2015). Role of physicochemical properties in nanoparticle toxicity. Nanomaterials 5 (3), 1351–1365. doi:10.3390/nano5031351
Singh, J., Dutta, T., Kim, K-H., Rawat, M., Samddar, P., and Kumar, P. (2018). ‘Green’synthesis of metals and their oxide nanoparticles: Applications for environmental remediation. J. nanobiotechnology 16 (1), 84–24. doi:10.1186/s12951-018-0408-4
Singh, S., Chunglok, W., Nwabor, O. F., Ushir, Y. V., Singh, S., and Panpipat, W. (2022). Hydrophilic biopolymer matrix antibacterial peel-off facial mask functionalized with biogenic nanostructured material for cosmeceutical applications. J. Polym. Environ. 30 (3), 938–953. doi:10.1007/s10924-021-02249-5
Singh, S., Nwabor, O. F., Sukri, D. M., Wunnoo, S., Dumjun, K., Lethongkam, S., et al. (2022). Poly (vinyl alcohol) copolymerized with xanthan gum/hypromellose/sodium carboxymethyl cellulose dermal dressings functionalized with biogenic nanostructured materials for antibacterial and wound healing application. Int. J. Biol. Macromol. 216, 235–250. doi:10.1016/j.ijbiomac.2022.06.172
Soetaert, F., Korangath, P., Serantes, D., Fiering, S., and Ivkov, R. (2020). Cancer therapy with iron oxide nanoparticles: Agents of thermal and immune therapies. Adv. Drug Deliv. Rev. 163, 65–83. doi:10.1016/j.addr.2020.06.025
Soliman, M. K. Y., Abu-Elghait, M., Salem, S. S., and Azab, M. S. (2022). Multifunctional properties of silver and gold nanoparticles synthesis by Fusarium pseudonygamai. Biomass Conversion and Biorefinery.
Soliman, M. K. Y., Salem, S. S., Abu-Elghait, M., and Azab, M. S. (2023). Biosynthesis of silver and gold nanoparticles and their efficacy towards antibacterial, antibiofilm, cytotoxicity, and antioxidant activities. Appl. Biochem. Biotechnol. 195 (2), 1158–1183. doi:10.1007/s12010-022-04199-7
Soomro, R. A., Nafady, A., Memon, N., Sherazi, T. H., and Kalwar, N. H. (2014). L-cysteine protected copper nanoparticles as colorimetric sensor for mercuric ions. Talanta 130, 415–422. doi:10.1016/j.talanta.2014.07.023
Sundeep, D., Vijaya Kumar, T., Rao, P. S., Ravikumar, R., and Krishna, G. A. (2017). Green synthesis and characterization of Ag nanoparticles from Mangifera indica leaves for dental restoration and antibacterial applications. Prog. Biomater. 6, 57–66. doi:10.1007/s40204-017-0067-9
Syukri, D. M., Nwabor, O. F., Singh, S., Ontong, J. C., Wunnoo, S., Paosen, S., et al. (2020). Antibacterial-coated silk surgical sutures by ex situ deposition of silver nanoparticles synthesized with Eucalyptus camaldulensis eradicates infections. J. Microbiol. Methods 174, 105955. doi:10.1016/j.mimet.2020.105955
Syukri, D. M., Nwabor, O. F., Singh, S., and Voravuthikunchai, S. P. (2021). Antibacterial functionalization of nylon monofilament surgical sutures through in situ deposition of biogenic silver nanoparticles. Surf. Coatings Technol. 413, 127090. doi:10.1016/j.surfcoat.2021.127090
Thomas, B., Mathew, C., and Muthuvignesh, J. (2014). Nanotechnology—applications in prosthodontics: A literature review, 103–110.
Tripathy, A., Behera, M., Rout, A. S., Biswal, S. K., and Phule, A. D. (2020). Optical, structural, and antimicrobial study of gold nanoparticles synthesized using an aqueous extract of mimusops elengi raw fruits. Biointerface Res. Appl. Chem. 10 (6), 7085–7096.
Winkler, D. A. (2020). Role of artificial intelligence and machine learning in nanosafety. Small 16 (36), 2001883. doi:10.1002/smll.202001883
Wu, X., Xu, Y., Dong, Y., Jiang, X., and Zhu, N. (2013). Colorimetric determination of hexavalent chromium with ascorbic acid capped silver nanoparticles. Anal. Methods 5 (2), 560–565. doi:10.1039/c2ay25989c
Yang, J-W., Shen, Y-C., Lin, K-C., Cheng, S-J., Chen, S-L., Chen, C-Y., et al. (2020). Organ-on-a-chip: Opportunities for assessing the toxicity of particulate matter. Front. Bioeng. Biotechnol. 8, 519. doi:10.3389/fbioe.2020.00519
Yaşa, İ., Lkhagvajav, N., Koizhaiganova, M., Çelik, E., and Sarı, Ö. (2012). Assessment of antimicrobial activity of nanosized Ag doped TiO2 colloids. World J. Microbiol. Biotechnol. 28, 2531–2539. doi:10.1007/s11274-012-1061-y
Youssef, A. M., El-Sayed, S. M., Salama, H. H., El-Sayed, H. S., and Dufresne, A. (2015). Evaluation of bionanocomposites as packaging material on properties of soft white cheese during storage period. Carbohydr. Polym. 132, 274–285. doi:10.1016/j.carbpol.2015.06.075
Zare, M., Namratha, K., Ilyas, S., Hezam, A., Mathur, S., and Byrappa, K. (2019). Smart fortified PHBV-CS biopolymer with ZnO–Ag nanocomposites for enhanced shelf life of food packaging. ACS Appl. Mater. interfaces 11 (51), 48309–48320. doi:10.1021/acsami.9b15724
Zdenka, B., Matej, B., Aneta, S., and Michal, G. (2023). “Synthesis of metal nanoparticles using lichens and their biological applications,” in Fungal cell factories for sustainable nanomaterials productions and agricultural applications (Elsevier), 163–203.
Zhang, D., Xl, M., Gu, Y., Huang, H., and Zhang, G. W. (2020). Green synthesis of metallic nanoparticles and their potential applications to treat cancer. Front. Chem. 8, 799. doi:10.3389/fchem.2020.00799
Zhang, H., Hortal, M., Jordá-Beneyto, M., Rosa, E., Lara-Lledo, M., and Lorente, I. (2017). ZnO-PLA nanocomposite coated paper for antimicrobial packaging application. Lwt 78, 250–257. doi:10.1016/j.lwt.2016.12.024
Keywords: nanoparticles, green synthesis, antibacterial, analytical sensing, food preservations, consumable products
Citation: Kumar A, Shah SR, Jayeoye TJ, Kumar A, Parihar A, Prajapati B, Singh S and Kapoor DU (2023) Biogenic metallic nanoparticles: biomedical, analytical, food preservation, and applications in other consumable products. Front. Nanotechnol. 5:1175149. doi: 10.3389/fnano.2023.1175149
Received: 27 February 2023; Accepted: 17 April 2023;
Published: 02 May 2023.
Edited by:
Elias Emeka Elemike, Federal University of Petroleum Resource Effurun, NigeriaReviewed by:
Salem S. Salem, Al-Azhar University, EgyptSatheesh kumar Balu, Saveetha Dental College and Hospitals, India
Copyright © 2023 Kumar, Shah, Jayeoye, Kumar, Parihar, Prajapati, Singh and Kapoor. This is an open-access article distributed under the terms of the Creative Commons Attribution License (CC BY). The use, distribution or reproduction in other forums is permitted, provided the original author(s) and the copyright owner(s) are credited and that the original publication in this journal is cited, in accordance with accepted academic practice. No use, distribution or reproduction is permitted which does not comply with these terms.
*Correspondence: Bhupendra Prajapati, bhupendra.prajapati@guni.ac.in; Sudarshan Singh, sudarshansingh83@hotmail.com