- 1Shobhaben Pratapbhai Patel School of Pharmacy and Technology Management, SVKM’S NMIMS, Vile-Parle (W), Mumbai, Maharashtra, India
- 2Department of Pharmaceutics, School of Pharmacy and Technology Management, SVKM’S NMIMS, Shirpur, Maharashtra, India
Skin cancer is one of the most prominent diseases, affecting all continents worldwide, and has shown a significant rise in mortality and prevalence. Conventional therapy, including chemotherapy and surgery, has a few drawbacks. The ethosomal systems would be thoroughly reviewed in this compilation, and they would be classified based on constituents: classical ethosomes, binary ethosomes, and transethosomes. Ethosomes systems are model lipid vesicular carriers with a substantial portion of ethanol. The impacts of ethosomal system components, preparation techniques, and their major roles in selecting the final characteristics of these nanocarriers are comprehensively reviewed in this chapter. The special techniques for ethosomes, including the cold approach, hot approach, injection method, mechanical dispersion method, and conventional method, are explained in this chapter. Various evaluation parameters of ethosomes were also explained. Furthermore, ethosomal gels, patches, and creams can be emphasised as innovative pharmaceutical drug formulations. Some hybrid ethosomal vesicles possessing combinatorial cancer therapy using nanomedicine could overcome the current drug resistance of specific cancer cells. Through the use of repurpose therapy, phytoconstituents may be delivered more effectively. A wide range of in vivo models are employed to assess their effectiveness. Ethosomes have provided numerous potential skin cancer therapeutic approaches in the future.
Introduction
Cancer is among the main causes of mortality globally, based on chronic tissue damage, host-environment interactions, etc. Repeated exposure to carcinogens like smoke, UV rays, and infections causes a variety of genetic (mutations), epigenetic (loss of heterozygosity), and global transcriptome alterations (through inflammatory pathways), all of which are associated with an increased risk of developing cancer. The previous 10 years have seen an upsurge in the incidence and prevalence of cancer, which has presented a significant challenge to medical experts. According to World Health Organization (WHO) data, there will be a worrying 45% increase in cancer deaths worldwide by 2030, with India contributing 70% of those fatalities. The most prevalent malignant condition, especially in Caucasians, is skin cancer (Garrett-mayer, 2019). Each year, more than a million new cases are recorded globally. The cells from whence they arise and their clinical characteristics are used to designate the various forms of skin cancer. The much more prevalent varieties are malignant melanoma, basal cell carcinoma (BCC), and squamous cell carcinoma (SCC), collectively known as non-melanocytic skin cancer (NMSC) (World Health Organization, 2022).
Topical medication delivery using a liposomal formulation has drawn a great deal of attention in the last 10 years. When administered in non-occluded environments, deformable liposomes and transferosomes the first generation of elastic vesicles—were shown to penetrate intact skin while delivering a therapeutic concentration of medicines (Honeywell-Nguyen and Bouwstra, 2003). They were initially presented by Ceve and Blume in 1992. The medication is known to be carried into and through the skin via lipid vesicles made of phospholipids and non-ionic surfactants. The lipids in the skin help to maintain the skin’s barrier functions and stop medications from being absorbed into the body. Lipid vesicles may act as non-toxic penetration enhancers due to their amphiphilic nature. Additionally, hydrophilic (Biju et al., 2006), lipophilic, high-and low-molecular-weight compounds can be enclosed in the vesicles (Essa et al., 2003). Furthermore, it is theorised that these lipid-rich vesicles transport a considerable amount of drug through the skin, improving the systemic absorption of medication. It has been discovered and shown that ethosomes, which are lipid vesicular networks that contain ethanol in some really high concentrations, are extremely effective at increasing the skin absorption of a wide range of medications (Touitou et al., 2000).
This review intends to assemble and explain major research employing these nanovesicular drug delivery for skin cancer. This review article discusses many forms of skin cancer and their incidence. Nanotechnology methods provide a chance to improve the therapies for different forms of cancer. Also, a discussion related to both traditional and non-conventional therapy. Then there’s skin cancer’s problems (Borgheti-Cardoso et al., 2020). The physiological hurdles that obstruct regional and national therapy will be highlighted. Multidrug resistance, as well as systemically distributed ethosomal vesicles with many types of anticancer formulation. Then, based on the route of administration, the designs and optimal properties of ethosomes for successful drug delivery in skin cancer therapy will be presented. The several ethosomal formulations that have been described for the management of basal cell carcinoma, squamous cell carcinoma, and melanoma are presented in the review that follows. These formulations have been utilized for systemic and transdermal drug administration in skin cancer. Characteristics of the skin barrier are also highlighted in respect on current clinical care for skin cancer, with major focus on non-surgical therapeutic treatments. This review also discusses the relationships of ethosomes with skin tissue, the many pathways for drug administration via the skin, and a comprehensive investigation of the ethosomes topical permeability. This paper describes the various methods of topical or dermal distribution and gives prospective researchers guidelines for topical formulation invention.
Skin as a barrier
The human body skin has more efficient delivery of drugs by barrier “our insides in and the outside out”. Skin has some barrier issues to selected drugs passing through for topically applied various types of formulations containing active pharmaceutical agents. The skin surrounds the body’s surface, integrates with the environment, and protects the inner organs against direct contact from the outside atmosphere (Williams and Barry, 2004) It has the property of altering the entry of chemical and biological agents because of the stratum corneum (subcutaneous) that functions to regulate heat through regulation such as blood flux, perspiration, and sweating (Harman et al., 1966). Lipid bilayers are composed of primary ceramides sequentially arranged on the flattened corneocyte layer of skin. The largest organ in the body is the skin, which covers almost 10 percent of the total body mass; it weighs an average of 4 kg and covers an area of 2 m2 (Mishra et al., 2013). Schematic structure of skin layer represented in Figure 1.
The drug penetration through the skin is mainly by the stratum corneum layer. It follows different pathways through penetration, which helps in delivering drugs through novel drug delivery systems. It has the ability to enter through the skin and trigger the necessary action.
Some permeation enhancers such as Piperine and also some vascular carriers facilitate transport across the skin (Jantarat et al., 2018). Pathway through drug absorption via skin represented in Figure 2.
The following stages are associated with drug penetration through the skin.
• Product sorption by the stratum corneum
• Drug penetration even by intact skin
• Drug absorption by the capillary network throughout the dermal papillary layer
The permeation level throughout the skin (dQ/dt) is provided by
This Equation helps to determine the permeation rate of a drug through delivery system.
Based on their content, ethosomes as a carrier can transfer topically administered abilities to different skin layers. Dissolution from the inside and release from the preparation are typically two-step processes by which a medicinal substance is released from a topical application and transferred to the blood circulation (Barua and Mitragotri, 2014), primarily via the lipidic intercellular route through the SC by partitioning into the viable aqueous epidermis. The healthy epidermis diffuses into the upper dermis, the papillary dermal layer, and is eventually absorbed by the vascular system.
Skin cancer
Skin cancer is a form of cancer that develops on the skin surface as a result of the proliferation of aberrant cells. Cancer cells have the ability to spread across the body and invade other organs. In recent years, non-melanoma and melanoma, the two most common kinds of skin cancer, the most common cause of skin cancer is sensitivity to ultraviolet (UV) radiation. Specific genes are inactivated by UV radiation, whereas oncogenes are activated. Although skin cancer is not the most lethal type of cancer, it is the most frequent in the United States and many other nations (Misak et al., 2013). Melanoma accounts for the great majority of skin cancer mortality despite accounting for just a small percentage of skin cancer rates. Early detection and treatment of skin cancer may be biopsied at a preliminary phase and has a 99 percent survival probability, but metastasized melanoma kills 80 percent of patients within 5 years of diagnosis (Jerant et al., 2000). Basal cell carcinoma and squamous cell carcinoma are the two most frequent kinds of skin cancer. For these restricted conditions, excision is the gold standard therapy. They can, however, spread to regional lymph nodes and distant sites in extremely rare circumstances. Melanoma is still a major cause of death and morbidity in the U.S. and across the globe. Greater UV radiation exposure and increased monitoring have both been blamed for the rise in melanoma cases. There has been no change in the behavior of young adults who use indoor tanning despite increased public knowledge of the hazards of extreme UV exposure in connection to melanoma formation Beds. The following are some of the current public health programs aimed at lowering melanoma rates: sunlight exposure should be reduced, sunscreen should be used more frequently, and indoor tanning should be avoided, particularly for kids. Lowering the rates of illness and death linked to melanoma will necessitate a combined public and healthcare professional effort (Carr et al., 2020).
Melanoma is a cancer that starts in the melanocytes and is classified as cutaneous or non-cutaneous depending on how it manifests (Garbe and Leiter, 2008) the most common type of melanoma mentioned below.
• Superficial spreading melanoma (70%) prevalent type of melanoma is Before vertical (invasive) growth, it passes through lateral (radial) growth.
• Nodular melanomas (15%–30%) are fast-expanding raised or polypoid lesions that are frequently blue or black in colour and have an early vertical development phase.
• Lentigo malignant melanoma (4%–10%) is more prevalent in older people who have had their skin exposed to the Sun for a long time. It usually starts as a tiny macule that looks like a freckle. It expands over time, becoming darker, more asymmetrical, and exhibiting a vertical development phase.
• Acral lentiginous (5%): tumours most usually appear on the palms, soles, subungual, and mucosal surfaces. Melanoma can develop in any non-cutaneous place with melanocytes, such as the ophthalmic, gastrointestinal, genitourinary, and nasopharyngeal areas. Type of carcinoma represented in Figure 3.
According to a study published in the journal Cancer, they are far less prevalent than cutaneous melanoma. The National Cancer Data Base has information for 84,836 people with cutaneous cancer. Melanoma of the skin and non-cutaneous melanoma (Carr et al., 2020). Intermittent Sun exposure and ultraviolet (UV) radiation have been linked to the development of melanoma, basal cell, and squamous cell carcinoma in several studies (Nagore et al., 2010; Bauer et al., 2011). UVA radiation stimulates the creation of reactive oxygen species (ROS), which leads to the degradation of deoxyribonucleic acid (DNA), lipids, and proteins during oxidative reactions (Viros et al., 2014). Indoor tanning also hastens the progression of melanoma, with non-exposed people having a 16–20 percent increased risk of developing the disease. Even in the absence of mutations, the major melanoma mutations (BRAF, MEK, NRAS, and MAPK) cause different melanogenic pathway functions. However, due to the restrictive strategy associated with several medications, a novel technique is particularly needed (Feoktistova and Panayotova-Dimitrova, 2017). Surgery, radiation treatment (Kalekhan et al., 2022), immunotherapy (Nanamori and Sawada, 2022), systematic therapy, and antibody therapy (Esnault et al., 2022) are among some of the therapeutic interventions for malignant melanoma. In addition, because of the specific delivery of therapeutic components, nanotechnology-based approaches have emerged as promising strategies in melanoma treatment (Portugal et al., 2021). Stages of Melanoma represented in Figure 4.
Non-melanoma skin cancer
Melanoma and non-melanoma skin cancer (NMSC) incidence have been rising in the U.S. for the past 2 decades, and this has been linked to increased ultraviolet radiation exposure (UVR). With these escalating rates, it has become much more crucial than ever to take preventative actions to lower the incidence and encourage timely diagnosis of these tumours (Trager et al., 2022). Patients with non-melanoma skin cancer are the most highly prevalent cancer form. Because the growth of non-melanoma skin cancer follows molecular pathways, it leads to effective therapy options for NMSC (Didona et al., 2018). NMSC is typically categorised into two categories: BCC and SCC.
Basal cell carcinoma
Basal cell carcinoma, also known as Jacob’s ulcer, rodent ulcer, basal cell epithelioma, or basalioma, is a cancer that arises from the epidermis’s basal membrane (Sari et al., 2020). Out of all the skin cancers, BCC is a really limited malignancy that requires lineage-specific immunohistochemistry investigation for accurate identification and is recommended to be totally excised at an early stage (Bisceglia et al., 2020). It occurs in 80% of patients, primarily in the head and neck areas. BCC seldom metastasizes, although it does regularly invade and destroy tissue locally, resulting in high morbidity (Cameron et al., 2019). It has been discovered that genetic disposition increases skin ageing and shares molecular pathogenic characteristics in the establishment of BCC (van der Poort et al., 2020).
The Hedgehog (HH) pathway, which is normally suppressed in adults, plays a vital role in organogenesis, patterning, proliferation, survivability, and differentiation (Rimkus et al., 2016). It had been Drosophila was the source of the discovery. The HH is an abbreviation for “highly Three factors might trigger a signalling cascade. Various ligands involved in this pathway include: The Indian hedgehog (SHH), the Sonic hedgehog (SHH), and the Hedgehog of the Desert SHH, on the other hand, is very common. Research expressed in human tissues. These are the ligands. Binds to the Patched-1 (PTCH) receptor (Skoda et al., 2018). On the surface of the membrane, there is a receptor for target cells, which usually works by obstructing Another membrane is smoothened (SMO). The HH signalling cascade is then initiated (Pak and Segal, 2016; Doheny et al., 2020).
The inhibition of SMO is lifted, resulting in a cascade of stimulation that is abnormal. SMO plays a crucial function in this process; it works as a signalling molecule via triggering the HH signalling cascade, which results in the transcription regulators’ activation for homolog 1 of the glioma-associated oncogene (Gli1), which stimulates angiogenesis and the expression of target genes Bcl-2, Cyclin-D1, and Myc (Bcl-2, Cyclin-D1, and Myc) (Pak and Segal, 2016). When there is not any The SHH ligand is inert, the HH pathway is dormant, and PTCH is inactive. Gli1-targeting is prevented by inhibiting SMO activity. transcription of genes. The HH route is implicated in the process. In the control of cell growth and differentiation. It is active throughout embryogenesis but not in adulthood. tissues. The HH pathway’s abnormal activation Increased cell proliferation and survival are associated with the pathophysiology of several solid tumours, especially BCC (Luca et al., 2044).
BCCs were advised to be divided into two categories: “simple to treat” and “difficult to treat,” with distinct treatment choices for each. Treatment methods that have been licenced and are indicated for both local and systemic therapy, such as imiquimod and 5-fluorouracil. New medicinal medicines that have undergone clinical studies are explored. Targeted treatments, such as GLI antagonists or anti-VEGFR drugs, immunotherapies, such as checkpoint inhibitors, recombinant cytokines, or silencing RNA, and intralesional virotherapies using modified adeno-as well as herpes viruses (Cameron et al., 2019).
Squamous cell carcinoma
The second leading cutaneous cancer that requires rapid detection is squamous cell carcinoma. The definition of clinical characteristics, diagnostic procedures, grading, staging, surgery, and non-invasive therapy must all be updated. Topical therapy, cryosurgery, photodynamic therapy, and radiation are among the non-surgical current therapies (Dreyfuss et al., 2022).
Cemiplimab and pembrolizumab are two anti-PD1 medicines that have been authorised by the Drug Administration for the treatment of established CSCC and have shown therapeutic significance and long-lasting responses, as well as an acceptable safety profile. Immune system blockers are now the gold standard of therapy for both laCSCC and mCSCC. Anti-PD1 immunotherapy’s safety and efficacy in both lcscc and mcscc must be validated in real-world research (de Jong et al., 2022).
Immunotherapeutic drugs such as PD-1 inhibitors are utilised to treat advanced cutaneous squamous cell carcinoma (cSCC) (Aboul-Fettouh et al., 2022). The anticancer activity, increased lifespan, antiviral, and tolerability decrease of a cisplatin-and imiquimod-loaded pro-vesicular dual-drug delivery system were all excellent, demonstrating the benefit of localised drug administration and adjusted combination treatment (Gupta et al., 2014). Topical formulations encased in polymeric and lipid nanoparticles, nanoemulsions, dendrimers, and liposomes have higher skin penetration and stability, resulting in improved effectiveness (Ravikumar and Tatke, 2019). The skin deposition ability of nobiletin vesicular systems was effectively created and compared. Small particle size, high entrapment for nobiletin, and high skin deposition ability were exhibited in composite penetration booster vesicles made of phospholipids, transcutol P as a penetration enhancer, and chitosan (Bayoumi et al., 2021).
Conventional treatments and limitations of the conventional dosage form for skin cancer
The rising incidence of skin cancer throughout the world necessitates a variety of treatment choices (Simões et al., 2015). Chemotherapy, immunotherapy, surgical excision, radiation, Mohs micrographic surgery (MMS), and cryotherapy are among the well-known therapies for skin cancer.
Chemotherapy is administering cytotoxic medications to patients in order to extend their lives and enhance their quality of life. After oral delivery, only a tiny percentage of medications reach the therapeutic location, decreasing therapeutic effectiveness. Frequent doses are necessary to compensate for the loss of therapeutic potential and sustain the optimal drug concentration at the therapeutic site, which may lead to drug resistance and produce harmful adverse effects due to recurrent medication use (Mou et al., 2016). In a conventional chemotherapy procedure, cytotoxic medicines are frequently given in conjunction with two or more additional treatments to help patients tolerate the side effects of other adjuvants and obtain greater therapeutic efficacy. With so many pharmaceuticals being used in patients, the possibility of a drug–drug interaction is unavoidable. According to (Buajordet et al., 2001), drug–drug interactions are expected to be the cause of mortality in 4% of cancer patients. There were five incidences of anticancer agents being used in cancer treatment that resulted in fatal side effects (Marks et al., 2001). The graph depicts the total number of Research papers related ethosomes for skin cancer has reviewed from 2008 to until December 2022 mentioned in Figure 5. Also included summary of research articles work for treatment of skin cancer represented in Table 1.
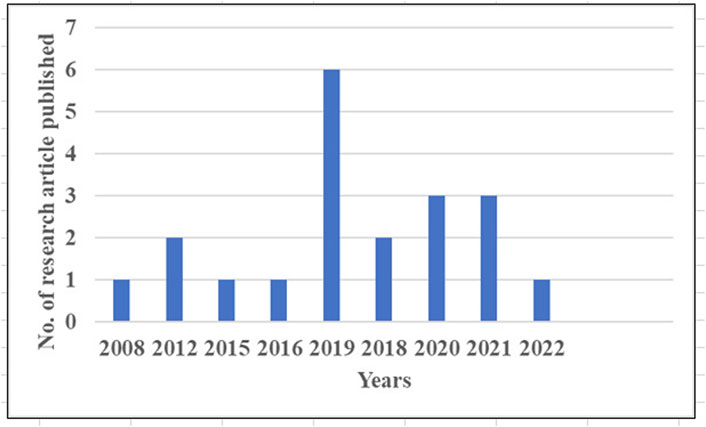
FIGURE 5. Number of research articles published on ethosomes used for treatment skin cancer from 2008 to 2022.
To study the current scenario of scientific investigations heading towards the development of a potential strategy for the diagnosis, management, and treatment of skin cancer, we have analysed all the relevant data or work through the Pub Med search engine.
We searched for the number of research papers done related to ethosomes drug delivery system. The number of articles searched with the set of keywords applied with available data is ethosomes for skin cancer.
Ethosomes, invented by Touitou in 1996 (Touitou, 1996), are non-invasive lipid vesicular agents that have been well for attaining effective skin administration and have nanometric dimensions (Touitou et al., 2000; Bendas and Tadros, 2007; Ibrahim et al., 2019). They include phospholipids in the range of 2%–5% and a high concentration of ethanol in the range of 20%–40% (Romero and Morilla, 2013). The combined potential of lower chain alcohol and soft pliable vesicles, the former essential for imparting fluidic characteristics (Blume et al., 1993) and membrane permeability, and the latter penetrating the deep dermal layers (Elsayed et al., 2007), results in a bigger penetration response in the lipid environment. Because of their structural shape and amount of lipid bilayers, ethosomes act as entrapment carriers for hydrophilic (buspirone), lipophilic (simvastatin), and large molecular weight compounds (Bayerl et al., 2002; Godin and Touitou, 2003; Fu et al., 2019). Numerous studies have supported conclusions that ethosomes improve encapsulation efficiency, permeability, and drug deposition. Structure of ethosomes represented in Figure 6.
Benefits of Ethosomal Drug Delivery (Godin and Touitou, 2003; Abdulbaqi et al., 2016; Pandey et al., 2017; Garg and Jain, 2022).
Ethosomes improve the drug’s transdermal and dermal absorption via the skin.
• Ethosomes serve as delivery systems for a wide range of medications.
• In terms of quantity and depth, ethosomal systems are significantly effective in delivering a fluorescent probe (quantum dots) to the skin.
• Low risk profile: Because the toxicity profiles of the ethosomes components have been thoroughly documented in the scientific literature, there is no risk associated with large-scale medication development using this technique.
• Excellent patient compliance—The semisolid form (gel or cream) in which the ethosomes medicines are administered results in high patient compliance. Iontophoresis and phonophoresis, on the other hand, are somewhat difficult to utilise, which will impact patient compliance (Ferrara et al., 2022).
• High market appeal for items utilising patented technologies ethosomes manufacturing is rather easy and does not require any expensive technical inputs.
• The ethosomes technology is passive and non-passive, and it is now commercialized.
Limitations
Ethosomal administration is intended to give a constant stream of drug over a period of time rather than a bolus of drug all at once (Laïb and Routh, 2008; Rakesh and Anoop, 2012; Patel et al., 2013).
• Adequate drug solubility allows drug penetration into the dermal microcirculation and circulatory system under both lipophilic and lipophobic conditions.
• The drug’s molecular weight should be such that it can be absorbed via the skin.
• Adhesive may or may not be suitable for all skin types.
• It will not be economically viable.
• The yield of interest is negligible.
• Excipients and enhancers may cause dermatitis or skin irritation in the vicinity of the pharmaceutical distribution structure.
• When ethosomes are submerged in water and the shell locking fails, the ethosomes cluster and disintegrate.
• There is a loss when transitioning from the organic to aqueous phase.
Mechanism
Ethosomal possibilities are eco-friendly, and a rise in ethanol content produces negatively charged moieties that promote stability, bioavailability, and penetrability. Going to follow ethosomal delivery, it has been noted that the stratum corneum’s lipidic component is disrupted, inter-cellular lipid is extracted and disintegrated, and the skin’s horny layer’s temperature dependence is changed (Bindu Madhavi et al., 2013). Extensive research has confirmed the findings about ethosomes' enhanced entrapment efficiency, penetrability, and drug-deposition. The interdigitated, flexible ethosome vesicles are capable of creating pathways through the unstructured SC and eventually delivering medication into the deep skin layers. The fusion of ethosomes might then lead to the transdermal absorption of medicines. Lipids from the skin. As a consequence, it is anticipated that drugs will be discharged at different sites along the penetration route (Godin et al., n.d.; Heeremans et al., 1995; Touitou et al., 2000; Touitou and Godin, 2007; Verma et al., n.d.). Permeation mechanism of drug from ethosomes represented in Figure 7.
According to research, ethanol and phospholipids work together to improve the skin absorption of drugs in ethosomal compositions. Ethanol changes the configuration and lowers the density of skin lipids by fluidizing the lipid bilayers of the stratum corneum and ethosomal vesicles at the same time. As a result, the altered stratum corneum structure will be penetrated by the very flexible and soft vesicles of an ethosomal system, providing a passage through the skin.
This would be followed by the “ethosomes effect,” which is the releasing of the medication into the deep layers of the skin through lipid penetration and permeation by the formation of new routes as a result of the flexibility and merging of ethosomes with skin lipids (Verma and Fahr, 2004). These vesicles merge with cell membranes in the deeper layers of the skin to deliver the therapeutic agent. Certain research suggests that transethosomes may offer better skin-permeation abilities than conventional ethosomes. This is due to the presence of ethanol in transethosomes as well as the edge activator or penetration enhancer, both of which work together to promote vesicular elasticity and skin-lipid alteration.
Types of Ethosomes
When compared to traditional liposomes, those vesicle nanocarriers have already shown superior delivery of drugs due to their smaller particle size, negative zeta-potential, greater encapsulation efficiency, and enhanced stability. Nevertheless, a new generation of ethosomes—binary ethosomes and transethosomes—was created in search of a more effective skin permeation mechanism. Types of ethosomes represented in Figure 8.
Conventional or classical ethosomes are a version of traditional liposomes and are made up of phospholipids, water, and ethanol in high concentrations (up to 45% w/w). For topically applied drug administration, conventional ethosomes were reportedly better than conventional liposomes because they had a smaller size, negative potential, and higher entrapment efficiency. In contrast, traditional ethosomes are up to a high standard liposome in terms of skin penetration and stability characteristics (Zhang Z et al., 2012; Sarwa et al., 2013; Jain et al., 2015; Tiwari et al., 2016). Drugs bound in traditional ethosomes have had molecular weights ranging from 130.077 Da to 24 kDa (Mishra et al., 2010; Zhou et al., 2010; Li et al., 2012).
Binary ethosomes were first discovered in 2010 by Zhou et al. Binary ethosomes include two alcohols in addition to ethanol, usually propylene glycol (PG) and isopropyl alcohol (Dave et al., 2010; Akhtar and Pathak, 2012; Zhang J. P. et al., 2012; Shen et al., 2014). PG is a well-known penetration enhancer, and it has greater viscosity and hygroscopicity in comparison to ethanol, as well as non-toxicity, decreased skin inflammation, and stability.
Transethosomes
Introduced a new generation of ethosomes with the goal of Because they included both ethanol and edge activators, the transethosomes offered the benefits of both ethosomes and deformable liposomes (Song et al., 2012). Transethosomes comprise an edge activator or a penetration enhancer in addition to the identical ethosome makeup by using surfactant (Song et al., 2012). In addition to phospholipid and water, edge activators make deformable liposomes more flexible by disrupting the lipid bilayers. Utilizing this technique, drugs in the low to high molecular weight range may be easily captured. The bioactive agent releases its payload slowly and gradually since it is preserved by encapsulation. They have a highly effective trapping ability since they are biodegradable and biocompatible. The formulation does not need any additional adjuvant and no complicated method of preparation for it (Honeywell-Nguyen and Bouwstra, 2003; Trotta et al., 2004; Song et al., 2012).Because ethanol has a natural tendency to fluidize the cellular membranes, its contact with the lipids of both skin and vesicles has rendered the latter more flexible. Because of this ethanol property, medicines bound in ethosomes were able to penetrate through stratum corneum barriers relatively rapidly.
Impacts of ethosomal components, preparation techniques, and their major roles in shaping the final properties
Composition of ethosomes
Phospholipid
The composition of the ethosomal system has made use of phospholipids from various sources. When creating an ethosomal system, selecting the appropriate phospholipid type and concentration is crucial since it affects the vesicle’s size, entrapment efficiency, Zeta-potential, stability, and penetrating abilities (Abdulbaqi et al., 2016).
In order to enhance the safety and efficiency of anthralin, liposomes and anthralin-loaded ethosomal formulations were examined. The drug encapsulation efficiency was substantially enhanced from 97.2 .4% to 99.2 .2% when the drug/lipid ratio was raised from 10% to 25%. As compared to the 10% ratio, the drug encapsulation efficiency significantly increased with further increases in the ratio to 50% and 75%. However, there was no statistically relevant difference in the drug encapsulation efficiency between 25%, 50%, and 75% (Paliwal et al., 2019).
Phospholipids can be derived from a variety of sources, including natural, semisynthetic, and synthetic materials, and can be included in concentrations ranging from 5% to 10% of the final ethosome content (Ascenso et al., 2013). Several phospholipids have been employed in prior ethosomal formulations, including PC, dipalmitoylphosphatidylcholine (DPPC), phosphatidylethanolamine (PE), Phospholipon® 90, and Lipoid S 100 (Abdulbaqi et al., 2016). Skin permeation also depends on the selected phospholipid, as it will combine with the skin lipid bilayer and enable the vesicle to produce tiny openings inside the SC (Arora and Nanda, 2019). In an ethosomal formulation, phospholipid concentrations generally range from .5% to 5%. Vesicular size will increase slightly or considerably when phospholipid concentration rises, while entrapment efficiency will rise significantly. (Chen et al., 2011; Prasanthi and Lakshmi, 2012).The relationship is valid up to a specific concentration, beyond which an increase in phospholipid concentration has no further impact on the effectiveness of entrapment (Naeff, 1996; Vanic, 2015).
Ethanol
Ethanol effectively increases penetration. Despite giving the vesicles distinctive qualities in regards to size, potential, stability, and other factors, they play a crucial role in ethosomal systems. Improved skin permeability and effective trapping (Prasanthi and Lakshmi, 2012). It has been observed that ethosomal systems contain ethanol concentrations of 10%–50% Several studies have found that increasing the ethanol content reduces the size of the ethosomes.
The concentration of ethanol in the vesicle membrane was presumably the cause of the greater entrapment effectiveness of lamivudine in ethosomes compared to liposomal formulation. Due to an increase in membrane fluidity, the entrapment efficiency as the ethanol concentration was raised from 15% to 45% (Paolino et al., 2012). However, subsequent ethanol ingestion would likely have rendered the vesicle membrane more permeable, decreasing the effectiveness of trapping. With a rise in ethanol concentration from 15% to 60%, the size of the vesicles shrank. The solution with 15% ethanol included the biggest vesicles, measuring 207 nm in size, whereas the preparation with 60% ethanol contained the smallest vesicles, measuring 72 nm in size. Since different quantities of ethanol are present, there is a substantial variability in the size of ethosomal compositions. Ethanol most likely modifies the system’s net charge and gives it some stearic stabilisation, which may induce the average vesicle size to decrease (Dubey et al., 2007)
During the process of formulation, the concentration of ethanol should be optimised because even at small concentrations, the effectiveness of entrapment will be relatively low, and at very high concentrations, the ethosomal membrane will be more permeable even though phospholipids can quickly dissolve in ethanol, which results in a significant decrease in entrapment efficacy. Composition of ethosomes represented in Table 2.
Cholesterol
Since cholesterol is a solid steroid molecule, adding it to ethosomal systems improves the stability and effectiveness of drug entrapment. In addition to preventing loss, it decreases vesicular fusion as well as permeability. It is typically applied at a concentration of 3%. At a fixed drug/lipid ratio of 10%, the impact of the cholesterol/PL-90G ratio on the drug encapsulation efficiency showed that the drug encapsulation efficacy was considerably reduced as the cholesterol concentration of the vesicles increased. As a result, when the CH/PL-90G ratio rose from .3:0.7 to .4:0.6, drug encapsulation efficiency dropped substantially from 97.2% to 94.8% (Fathalla et al., 2020). Cholesterol (30 mol%) was added into the ethosomes to convey an ordering arrangement of the phospholipids’ acyl chains and cause a condensing effect. Stearylamine (0–5 mol%) was also incorporated in order to assess the effect of cationic lipids in the formulation (Arora and Nanda, 2019).
Other alcohols
Propylene glycol (PG)
A common penetration enhancer is PG. It influences the ethosomal features of size, entrapment effectiveness, permeability, and stability when utilised in the formation of binary ethosomes at concentrations between 5% and 20%. When PG is added to ethosomal solutions, particle size is reduced much more, in contrast to ethosomal systems lacking PG.
Other additives in ethanol
To avoid vesicle aggregation and improve the stability of the formulation, diacetyl phosphate is frequently used. In the ethosomal, it is employed in quantities ranging from 8% to 20% of the overall phospholipid content. It was recently discovered that diacetyl phosphate-containing ethosomal compositions all resulted in vesicles with strongly negative potential.
In different experiments, the positive-charge agent stearyl amine was utilised in ethosomal preparations. The first research used an ethosomal system that contained mycophenolic acid and a 2:1:1 mass ratio of phosphatidylcholine, cholesterol, and stearyl amine. When Stearyl amine was added to the ethosomal formulation, it resulted in a significant rise in vesicular size, a decrease in entrapment effectiveness, a change from a negative to a positive-potential charge, and aggregation of both the vesicles within 1 week.
Methods of preparation
Different ethosomes kinds may be prepared using straightforward procedures that are simple to significantly increase without the use of expensive machinery. There are two common ways to create ethosomes (the cold technique and the hot method), although alternative approaches, such as the traditional mechanical-dispersion method and the transmembrane pH-gradient approach, have additionally been described. (Dubey et al., 2007).
Classic method
The medication and phospholipid are dissolved in ethanol and heated in a steam bath to 30°C + 1°C. In a sealed container, the lipid mixture is poured with double-distilled water in a fine mist while being constantly stirred at a speed of 700 rpm. Over three repetitions of passing through a polycarbonate membrane using a hand extruder, the final vesicle solution is homogenised (Dubey et al., 2007)
Traditional mechanical dispersion technique
In a round-bottom flask, soy phosphatidylcholine is dissolved in a solution of chloroform: methanol (3:1). A thin lipid layer is formed on the flask wall when the organic solvents are evaporated using a rotating vacuum evaporator just above the lipid annealing temperature. The deposited lipid film is then cleaned of any remaining solvent combination by placing the container’s components under a vacuum for the night. By revolving the flask at the appropriate temperature, hydration is accomplished with various concentrations of a hydroethanolic medium containing the medication (Dubey et al., 2007).
Hot method
This process requires heating the phospholipids in a water bath at 40°C until they generate a colloidal solution, and then dispersing them in water. In a different vessel, ethanol and propylene glycol are mixed and heated to 40°C. After both solutions reach 400°C, the organic component is added to the aqueous phase. Depending on how hydrophilic or hydrophobic the medication is, it is dissolved in ethanol or water. An ethosomal formulation’s vesicle size can be decreased to the required size via exploratory sonication or even the extrusion method. (United States Patent, 2022).
Cold method
This technique for ethosomal preparation represents the most popular and commonly utilised one. The medication, phospholipids, and other lipid components are mixed in ethanol with continuous stirring at room temperature. In a water bath, the mixture is heated to 30°C. In another vessel, the water has been heated to 30°C before being added, combined, and swirled for 5 minutes. If desired, the ethosomal formulation’s vesicle size can be reduced by sonication or extrusion in order to increase its extensibility. Furthermore, the mixture needs to be better refrigerated. (Verma and Pathak, 2010).
Transmembrane pH-Gradient method
The creation of empty binary ethosomes and the active loading of the medication are two distinct processes in this procedure. First, an alcoholic phase made up of PG and ethanol dissolves the phospholipid (for instance, PC). With continual 700 rpm stirring, a citrate buffer solution is progressively added to the initial solution. Throughout this procedure, the system is maintained at a temperature of around 30.1°C before being cooled to ambient temperature. The generation of blank binary ethosomes is now complete. In order to efficiently scatter and dissolve the drug, it is then actively injected into the ethosomes and the system is continually agitated at 700 rpm. This process involves two distinct steps: preparing an empty the ethosomal system’s internal phase (acid) and exterior portion (alkaline) can be separated by a pH gradient that can be generated by adjusting by introducing a .5 M sodium hydroxide (NaOH) solution to the pH outside. The system is then incubated under an opportune moment and climate, enabling the union. Drugs actively pass through the lipid bilayer of ethosomes and get imprisoned in the vesicles (Abdulbaqi et al., 2016; Zhang et al., 2016).
Evaluation parameters of ethosomes
The development of ethosomes can make use of a wide range of compounds. Phospholipids, whether with or without cholesterol, are frequently the chief constituent. The composition of the vesicle is a crucial element that directly affects the physical and chemical characteristics of the ethosome, affecting a number of aspects, including the bilayer’s flexibility, size, charges, and thermodynamic state. The following is an assessment of some of these characteristics.
Vesicle size and shape
A photomicrograph, transmission electron microscopy (TEM), or scanning electron microscopy (SEM) micrograph may all be used to see the vesicle form clearly. The transmission electron microscope photograph of the sample verifies the three-dimensional shape or structure of the phospholipid vesicles to be unilamellar and multilamellar. Utilizing Zetasizer may be used to determine whether phospholipids and ethanol concentration influence the size and dispersion of ethosome vesicles. The formulation’s ingredients can affect the ethosomes' size, which can range from hundreds of nanometers to microns. The ethosomes' size and zeta potential are influenced by a number of parameters. Although ethanol alters the system’s net charge and gives it a little more degree of stearic stability, which may eventually induce a drop in the average vesicle size, the mean vesicle diameter is diminished (Pathak and Kumari, 2013; Hallan et al., 2021).
Transition temperature
Differential scanning calorimetry (DSC), a technique that also measures ethanol-skin phospholipid interaction, which is a characteristic related to the fluidizing impact of ethanol on the phospholipid bilayers, may be used to measure the glass transition of the vesicular lipid structures (Mbah et al., 2021).
Ethosomes-skin interaction study
Ethosomes skin interaction can be analyze using differential scanning calorimeter (DSC) and FTIR. A 12-h penetration test was carried out. The Untreated was another skin sample placed on Franz’s cell. Afterward, skin samples were taken from both Franz cells. Taken and wet, dried, and chopped into small pieces and analysed using DSC and FTIR.
Entrapment efficiency
After extracting the drug that’s not trapped inside the ethosomes, the drug that was may be estimated. The dispersion can be centrifuged in a cooled centrifuge to release the unentrapped medicine from the ethosomes (Raghuvanshi et al., 2022). The samples will be centrifuged in a centrifuge machine for 3 hours at a temperature of four degrees. The silt that had formed a layer of vesicles was ruptured, and the sediment was then filtered to determine the concentration of drug inside the ethosomes vesicles using HPLC or UV spectroscopy. After a reasonable dilution using the proper medium, the supernatant will also be collected, and the drug concentration will be calculated using the formula below.
Vesicle elasticity measurement
The extrusion method was used to gauge the ethosome vesicles' flexibility (Mbah et al., 2021). A stainless-steel filter device with a 50 mm diameter was used to extrude the ethosomal formulation through a filter membrane with a pore size of 100 nm while applying a pressure of 2.5 bar. It was calculated how many vesicle suspensions were extruded in 5 min.
E is the elastic property of the vesicle, J is the volume of suspension extruded in 5 minutes, and r and r are the size of the vesicle following v are extrusion and the pore diameter of the filter membrane, respectively.
Stability study
While keeping the vesicles at 4°C and .5°C, the stability of the vesicles was evaluated. During 180 days, the vesicles' size, zeta potential, and entrapment effectiveness can be analysed.
Other parameters
Confocal laser scanning microscopy can be used to assess the ethosomal preparation’s penetration ability into the epidermal layers (Niu et al., 2019). Investigations into the capacity of the ethosomal formulation to increase the penetration of both hydrophilic and hydrophobic compounds through the skin in both in vitro and in vivo settings have shown that it is superior to traditional liposomes. According to numerous researchers, ethosomes-formulated medications penetrate the skin 5–10 times better than traditional liposomal therapies.
Ethosomes incorporated in suitable dosage form
The great majority of research that has been published has examined ethosomal systems while they were initially suspended. Even though ethosomal suspension has a strong alcohol content, further incorporating the system into an appropriate vehicle for dermal or transdermal delivery has some benefits, including trying to prevent ethanol evaporation; extending the skin’s time in interaction with the drug; increasing the drug’s therapeutic effectiveness; improving the stability and shelf life of the final form of administration; and improving patient compliance. Innovative pharmaceutical compositions have been created using ethosomal systems in a variety of vehicles, including ethosomal gels, transdermal patches, and creams.
Ethosomes in gel form
The pH, viscosity, spreadability, and extrudability of ethosomal gels are their unique features. For integrating ethosomal systems, carbopol and hydroxypropyl methylcellulose in all of their associated categories are the two most frequently employed gel-forming agents. These polymers provide the necessary viscosity and bioadhesive characteristics, and it has been proven that they are acceptable with ethosomal systems. In comparison to conventional or commercially available gels or creams, researchers have looked into how effectively medications from ethosomal gels penetrate the skin and the way they are released effectively. One of the research targets reach that ethosomes nanocarrier system for the transdermal administration of complex molecular protein drugs has superior clinical potential application and gives a potentially useful design for ethosomal gels with a variety of transdermal delivery drugs (such as Chinese medicine, vaccines, and interleukins) for further investigation (Fu et al., 2019).
Brucine based ethosomal formulation was well designed in the proposed investigation utilising a response surface approach and included in an HPMC gel foundation. The produced BRU-loaded ethosomal gel showed sufficient nanoscale vesicular size, adequate physical properties, adequate encapsulation efficiency, and optimal flux. Whenever BRU was incorporated into ethosomal gel, the in-vitro release of BRU was significantly impacted (Ismail et al., 2021).
In vivo studies were based on mice exhibiting chemically induced skin cancer responded significantly to topical treatment of metformin ethosomal gel (Mousa et al., 2022).
One of the research works has proposed on ethosomal gel with itraconazole in it offered improved transdermal flow, a shorter lag time, excellent entrapment efficiency, and a minimal chance for skin irritation. The drug’s ethosomal formulation kills cytotoxic cells more rapidly and thoroughly. Drugs incorporated into ethosomal gels have a longer half-life and a more potent cytotoxic action than free drugs (Saraf and Kumar Gupta, 2018).
Ethosomes in patch form
Although moulds are required to prepare ethosomal patches, their manufacture and assessment are more difficult than those for ethosomal gels. In vitro and in vivo, examined the transdermal delivery capabilities of Testoderm’s ethosomal patches with non-ethosomal patches of testosterone. Both patches had the same size and pharmaceutical composition. The drug deposition just on skin was obtained from the ethosomal patch and was seven times more powerful than the patch of testoderm. Since they allow the administration of ethosomes in occlusive circumstances, ethosomal patches have several benefits over ethosomal gels and creams in respect of expected penetration.
Ethosomes in cream form
Based on all of the previous research, adding ethosomal systems to appropriate delivery methods such as gels, patches, and creams enhances the medication or agent delivered via the ethosomal systems’ skin penetration. The incorporation of ethosomal systems is best suited for the vehicles discussed—gels—while creams may be favoured for cosmetic preparations.
Developing and evaluating naringin-loaded ethosomes has proposed work that have been enable the antioxidant potential of naringin to absorb free radicals. These ethosomes was then be included in sunscreen lotions that comprise zinc oxide (ZnO) and titanium dioxide (TiO2) to ensure desired skin penetration as well as skin retention (Gollavilli et al., 2020).
Modification of ethosomes for transdermal delivery
Transcutaneous immunization patches have achieved impressive transdermal medication release. Also had an inhibitory impact on tumour development in mice having melanoma and significantly increased the antitumor effect of aPD-1 without inducing systemic toxicity. This method provides a simple and non-intrusive method for enhancing the effectiveness of immune checkpoint inhibitor treatment (Song et al., 2022). Co-administration of the protein 1 monoclonal antibody (aPD1) and transcutaneous immunisation (TCI) patch can successfully slow the growth of tumours by raising levels of interleukin-12, TNF-, and IFN-, as well as encouraging the infiltration of cytotoxic T lymphocytes into cancerous tissue. After changing the mannose molecule on the exterior of the ethosome via electrostatic adsorption and layer-by-layer self-assembly techniques, a unique mannosylated chitosan (MC)-modified ethosome was developed throughout this work that has the capability to target dendritic cells. The plasmid (pIL-12@Eth-MC) encoding the IL-12 gene was demonstrated to induce the maturation of DCs in vitro. The TCI patch was created by electro spraying nanoparticles loaded with pIL-12@Eth-MC onto the surface of SF-PVA nanofibers.
New investigation on transcutaneous immunisation delivery method was created with electro-spraying microspheres carrying ethanol-mannosylated polyethyleneimine onto the electro spun SF-PVA nanofibrous patch (Hong et al., 2021). The transcutaneous medication administration demonstrated by the composite patches was effective. The use of the transcutaneous tumour vaccination combined with the anti-programmed death-1 monoclonal antibody greatly inhibited the proliferation of melanoma. Additionally, the combination of therapy led to a synergistic antitumor effect, which may be explained by the therapies’ enhanced infiltration of CD4+ and CD8+ T cells.
Research has investigated based on synergistic effect of 5-fluorouracil (5-FU) ethosomes with microwave (Khan and Wong, 2016). 5-FU retention in skin from ethosomes was enhanced in vitro and in vivo by which was before skin by microwave energy at 2450 MHz for 2.5 min. Nevertheless, compared to single ethosomal administration in vivo, the transdermal penetration flux and total drug absorbed into the systemic circulation were significantly decreased. The human melanoma cell line SKMEL-28 was pre-treated with microwave energy at 2450 MHz for 2.5 min alone, in addition to in conjunction with ethosomes, which significantly reduced the viability of the cells compared to those receiving plain 5-FU (Khan and Wong, 2016).
Repurpose therapy for the treatment of skin cancer
After just a few weeks of receiving traditional anti-cancer medications, patients build up a high tolerance, which facilitates metastatic spread and recurrence. Therefore, it is crucial to find new anti-skin cancer medications and combinations.
Mice with chemically induced skin cancer respond significantly to topical treatment of metformin ethosomal gel. This was demonstrated by presenting a high percentage of the EE%, lowest vesicle size, maximum ZP, and maximum DR% utilising the Box-Behnken design of “a three-level three-factor.” When isopropyl alcohol was combined with ethanol to create ethosomes, ethanol’s capacity to solubilize lecithin was enhanced, which improved the stability and efficiency of the ethosomes vesicles (Mousa et al., 2022).
One of research were met to the physicochemical and technical evaluation of the stable sulforaphane-loaded ethosomes® and transfersomes®, always one ethosomal formulation demonstrated a satisfactory ability to hold and release the medication as well as effectively penetrate the skin. Furthermore, the disparities in the ways that ethosomes® and transfersomes® work seem to indicate that they are less effective than one another as melanoma therapy agents (Cristiano et al., 2019). In contrast to transferosomes and drug solutions, ethosomes® increased the in vitro percutaneous penetration of sulforaphane. This result is most likely attributable to the inclusion of ethanol in the ethosomes® constitution, which may interact among carriers and stratum corneum lipids.
Therapeutic potential of herbal ethosomes
Most phytochemical constituents fail to attain bioavailability due to low absorption due to low absorption, even after showing potential therapeutic benefits. High molecular sizes with low lipid solubilities are the primary causes of phytoconstituents poor absorption and hence limited bioavailability. The bioavailability of such plant active ingredients or extracts is significantly increased when they are incorporated into vesicular carriers like ethosomes (Natsheh et al., 2019). Ethosomes are specifically crafted and constructed vesicles that contain a significant amount of ethanol. This gives them extra flexibility and competence enough to respond to peripheral stress by fluidizing and upsetting the lipids inside the stratum corneum. Ultimately, it results in the effective deep skin penetration of medicinal substances. (Sultana et al., 2018).
New innovation that successful in producing ethosomes that were co-loaded with evodiamine (EVO) and berberine chloride (BBR). The targeted ethosomes were at least 90% entrapment efficient for both BBR and EVO, with a mean size of 171 nm. Incorporating various in vitro applications to human skin, it was established that these ethosomes could carry medication to the basal layers of human skin. Furthermore, when BBR and EVO were co-loaded in ethosomes, B16 cells in cultured cells showed better anti-melanoma activities. The enhanced drug bioavailability and synergistic interactions between BBR and EVO may be responsible for this elevation in anticancer activity. This finding showed the ethosomes’ usefulness as possible dermal carriers for the management of melanoma (Lin et al., 2020).
A controlled release of the lipophilic chemical curcumin was accomplished by loading it into the vesicles and then introducing it into the ethosomal gel. The ethosomal gel had good skin deposition, which allowed curcumin to stay in the skin’s deeper layers for a longer duration of time, aiding in the full destruction of melanoma cells without inflicting significant discomfort to the skin (Krishna Kollipara et al., 2019).
Also, with the use of Carbopol-934 and sodium CMC as the polymer, camptothecin extracted from Nothapodytes nimmoniana may be administered by transdermal route in the form of a herbal gel formulation. These herbal gel preparations can efficiently deliver camptothecin in the treatment of dermatological cancer as evidenced by anticancer activity mostly on epidermoid carcinoma cell line-A431, which has an IC50 value of 48.03 g/mL and a percent apoptosis of 54.67 4.58, respectively. This is due to the controlled release that can be achieved by utilising these herbal gel formulations for a longer duration of time (Galatage et al., 2020).
Stability of ethosomes
The compositions long-term stability was evaluated in terms of their particle size and entrapment capability. Fundamentally, choosing the right lipid composition seems to be crucial for producing stable ethosome dispersions with the best possible pharmacological and medicinal properties. When it comes to liposomes, a variety of alterations could take place after storage. When stored, liposomes tend to merge and expand into larger vesicles, which creates a significant problem with medication leakage from the vesicles. The predisposition of the neutral liposome to aggregate is likely due to the absence of electrostatic repulsion, but with ethosomes, ethanol modifies the system’s net charge and confers some degree of steric stabilisation, increasing the dispersion’s stability against agglomeration and possibly reducing the mean vesicle size.
Since the membranes are more flexible, increasing the ethanol content from 15% to 45% improves the entrapment efficiency. However, ethanol concentrations above 45% may cause the vesicle membrane to become more permeable, decreasing the effectiveness of entrapment. Thus, the ethosomes become unstable as a result. The natural and/or chemically synthesised phospholipid sources are used to make the ethosomes lipid component. Unsaturated fatty acid-containing phospholipids are understood to go through oxidative processes. Then Changes in permeability can be caused by reactive species. Bilayers in the ethosomes. Lipids can undergo oxidative breakdown that can be reduced by safeguarding the lipid formulation by including antioxidants like light tocopherol Additionally, lipid hydrolysis results in lyso-PC formation. Lyso-PC addition increases the permeability of ethosomes, making it imperative to maintain a minimum in a particular preparation (Patel, 1990; Touitou et al., 2000; Rao, 2019).
IN-VIVO studies
Several researchers have evaluated ethosomal systems including skin permeability, pharmacokinetics, pharmacodynamics, safety, and skin irritation studies utilising highly variable in vivo studies and models using humans, rabbits, rats, mice, and guinea pigs. Several in vivo investigations have revealed that ethosomal systems may penetrate intact skin and transport compounds with various physicochemical properties in therapeutic levels to the blood circulation. Some research based on In- vivo studies mentioned in table 1.
In this study, scientist has investigated the ethosomal system filled with Sonidegib (SNG) and its pharmacokinetic and pharmacodynamic impacts in mice. Sonidegib Loaded Ethosomes (SNE) gel had a substantially higher AUC (210.80 7.83 g/h/mL) than oral SNG (66.14 4.53 g/h/mL), which was a difference of 3.18 folds. The AUC for SLE gel was 2.34 times (significantly) higher than that of the AUC for SLL gel (90.04 vs 5.52 g/mL). In comparison to oral SNG (.132 .07 h), the elimination rate constant (K) with SLE gel (.0547 .05 h1) was much lower. The skin deposition concentration of the gel developed had a much greater half-life (t0.5) compared to that of the SLE gel (12.54 h) (Gamal et al., 2021).
The increased bioavailability and longer plasma profile of the revised nimodipine loaded (NIM-ETH) gel formulation indicated a decrease in the dose and frequency of administration, which would affect patient tolerance, price, and dose-dependent negative impacts. Although the NIM-ETH gel formulation had maintained plasma concentration for roughly 36 h as seen by a drop in Cmax and delayed tmax of 3.61 ng/mL and 12 h, respectively, oral preparation’s pharmacokinetic parameters following oral administration culminated in Cmax of 8.1 ng/mL and Tmax of 5 h. When contrasted to the oral formulation (Nymalize oral preparation), the mean AUC0 to AUC0-1 of NiM-Eth gel rose from 4.1 to 5.9 folds, which also was determined to be the outcome of first pass effect (Moideen Muthu Mohamed et al., 2022).
The safety of conventional ethosomes has been established in both human and animal in vivo safety investigations, and they have great skin tolerability (Dubey et al., 2007; Ascenso et al., 2013; Zhang et al., 2014). In specific, for the other two classifications of ethosomal systems (binary ethosomes and transethosomes), which encompass penetration enhancers and/or edge activators in their preparation and may therefore increase the probability of developing harmful skin reactions, such as discomforts or erythema, more than that in vivo safety studies are needed to assess the short- and long-term impacts of repeated trials of these nanocarriers mostly on skin.
Ethosomes safety issues and their clinical applications in the treatment of skin cancer
According to in vitro and preliminary investigations, the majority of the vesicular drugs approved for the management of skin cancer appear to be safe, with minimal systemic risk. It was discovered that the treatment of skin cancer greatly benefits from the use of ethosomes. Ethosomes can lessen the negative side effects of chemotherapy while enhancing the efficiency of anticancer activity (Yingchoncharoen et al., 2016). However, the use of lipid nanoparticles has resulted in instances of complement activation-related pseudo allergy (CARPA). The complementing system is activated upon initial exposure to lipid additives, resulting in the pseudo allergy taking place when there has been no previous sensitization. Despite a large number of in vivo and preclinical studies that support their therapeutic application in the management of skin cancer, there is presently less information available on the topical application of vesicular drug delivery systems in the clinical-trial phases. In dermatological illnesses, such as skin cancer, topical medication may reduce local drug concentration and adverse effects when compared to systemic administration. The stratum corneum of the skin, however, functions as an efficient barrier, and its effectiveness is influenced by a particular compound’s importance in relation to a chemical property’s molecular size (Jain et al., 2017). The European Medicines Agency (EMA) and the US Food and Drug Administration (FDA) respectively, authorised Vismodegib, a top-notch Hedgehog Signalling Activation Inhibitor, for the treatment of BCC in 2012 and 2013 (Calienni et al., 2019). The clinical potential, safety, and efficacy of vesicular delivery of drugs against skin cancer should always be assessed by clinical research.
Future prospects
Since the discovery of ethosomes, vesicular research on transdermal medication administration has entered a new phase. Numerous studies indicate that ethosomes have a bright future. It is efficient in improving the transdermal administration of several substances. Additionally, this research will enable improved control over medication release in real time, enabling doctors to render the treatment more efficient. Ethosomes provide the opportunity to distribute modest, non-invasive packages. Since drug molecules have a medium to large size, it may be inferred logically that ethosomal compounds have a bright future in efficient dermal/transdermal transporting bioactive agents.
Conclusion
Transethosomes are a new generation of ethosomal systems that have been developed as a result of substantial and ongoing study. These transethosomes have been proven to have superior vesicular characteristics and skin-permeation capabilities compared to traditional ethosomes. Transethosomes give the formulator the most flexibility to alter the ethosomal characteristics in accordance with the necessary study requirements by altering the edge activators and/or penetration enhancers. To improve skin-permeation and therapeutic results, ethosomal systems must be included in appropriate vehicles such as gels, patches, and creams. Additional research is needed to improve the ethosomal system’s stability. The potential of ethosomal systems in cutaneous and transdermal distribution is being demonstrated by the outcomes of in vivo experiments and clinical trials.
Author contributions
PS, AP, and SB were involved in the conception and design, or analysis and interpretation of the data; drafting of the paper, revising it critically for intellectual content; and SB did final approval of the version to be published. PS, AP, and SB agree to be accountable for all aspects of the work.
Acknowledgments
This initiative would not have been possible without the help and support of R.S. Gaud, Director of SVKM’s NMIMS Deemed-to-be University, Shirpur.
Conflict of interest
The authors declare that the research was conducted in the absence of any commercial or financial relationships that could be construed as a potential conflict of interest.
Publisher’s note
All claims expressed in this article are solely those of the authors and do not necessarily represent those of their affiliated organizations, or those of the publisher, the editors and the reviewers. Any product that may be evaluated in this article, or claim that may be made by its manufacturer, is not guaranteed or endorsed by the publisher.
Abbreviations
WHO, World Health Organization; BCC, Basal Cell Carcinoma; SCC, Squamous Cell Carcinoma; NMSC, Non-melanocytic Skin Cancer; UV, Ultraviolet; ROS, Reactive Oxygen Species; CSCC, Cutaneous Squamous Cell Carcinoma; MMS, Mohs Micrographic Surgery; PG, Propylene Glycol; TEM, Transmission Electron Microscopy; SEM, Scanning Electron Microscopy Micrograph; DSC, Differential Scanning Calorimetry; aPD1, Protein 1 Monoclonal Antibody; TCI, Transcutaneous Immunisation; EVO, Evodiamine; BBR, Berberine Chloride; AUC, Area under Curve; CARPA, Complement Activation-Related Pseudo Allergy; EMA, European Medicines Agency; US FDA, US Food and Drug Administration.
References
Abdulbaqi, I. M., Darwis, Y., Abdul, N., Khan, K., Abou, R., Arshad, A., et al. (2016). Ethosomal nanocarriers: the impact of constituents and formulation techniques on ethosomal properties, in vivo studies, and clinical trials. Int. J. Nanomedicine Dovepress 11, 2279–2304. doi:10.2147/IJN.S105016
Aboul-Fettouh, N., Chen, L., Ma, J., Patel, J., Silapunt, S., and Migden, M. (2022). PD-1 inhibitors for cutaneous squamous cell carcinoma: A meta-analysis. Australas. J. Dermatology 63 (1), 36–42. doi:10.1111/AJD.13733
Akhtar, N., and Pathak, K. (2012). Cavamax W7 composite ethosomal gel of clotrimazole for improved topical delivery: Development and comparison with ethosomal gel. AAPS PharmSciTech 13 (1), 344–355. doi:10.1208/S12249-012-9754-Y
Arora, D., and Nanda, S. (2019). Quality by design driven development of resveratrol loaded ethosomal hydrogel for improved dermatological benefits via enhanced skin permeation and retention. Int. J. Pharm. 567, 118448. doi:10.1016/J.IJPHARM.2019.118448
Ascenso, A., Pinho, S., Eleutério, C., Praça, F. G., Bentley, M. V. L. B., Oliveira, H., et al. (2013). Lycopene from tomatoes: Vesicular nanocarrier formulations for dermal delivery. J. Agric. Food Chem. 61 (30), 7284–7293. doi:10.1021/JF401368W
Barua, S., and Mitragotri, S. (2014). Challenges associated with penetration of nanoparticles across cell and tissue barriers: A review of current status and future prospects. Nano Today 9 (2), 223–243. doi:10.1016/J.NANTOD.2014.04.008
Bauer, A., Diepgen, T. L., and Schmitt, J. (2011). Is occupational solar ultraviolet irradiation a relevant risk factor for basal cell carcinoma? A systematic review and meta-analysis of the epidemiological literature. Br. J. Dermatology 165 (3), 612–625. doi:10.1111/J.1365-2133.2011.10425.X
Bayerl, T. M., Schmidt, C. F., and Sackmann, E. (2002). Kinetics of symmetric and asymmetric phospholipid transfer between small sonicated vesicles studied by high-sensitivity differential scanning calorimetry, NMR, electron microscopy, and dynamic light scattering. Biochemistry 27 (16), 6078–6085. doi:10.1021/BI00416A037
Bayoumi, M., Arafa, M. G., Nasr, M., and Sammour, O. A. (2021). Nobiletin-loaded composite penetration enhancer vesicles restore the normal miRNA expression and the chief defence antioxidant levels in skin cancer. Sci. Rep. 11 (1), 20197. doi:10.1038/S41598-021-99756-1
Bendas, E. R., and Tadros, M. I. (2007). Enhanced transdermal delivery of salbutamol sulfate via ethosomes. AAPS PharmSciTech 8 (4), 213. doi:10.1208/PT0804107
Biju, S., Talegaonkar, S., Mishra, P., and Khar, R. (2006). Vesicular systems: An overview. Indian J. Pharm. Sci. 68 (2), 141–153. doi:10.4103/0250-474X.25707
Bindu Madhavi, B., Siri Vennela, K., Masana, P., and Madipoju, B. (2013). Enhanced transdermal drug penetration of curcumin via ethosomes. Malays. J. Pharm. Sci. 11 (1), 49–58.
Bisceglia, M., Panniello, G., Nirchio, V., Sanguedolce, F., Centola, M., and Ben-Dor, D. J. (2020). Metastatic cutaneous basal cell carcinoma: Report of 2 cases preceding the hedgehog pathway antagonists era. Adv. Anatomic Pathology 27 (2), 98–111. doi:10.1097/PAP.0000000000000259
Blume, A., Jansen, M., Ghyczy, M., and Gareiss, J. (1993). Interaction of phospholipid liposomes with lipid model mixtures for stratum corneum lipids. Int. J. Pharm. 99 (2–3), 219–228. doi:10.1016/0378-5173(93)90364-L
Borgheti-Cardoso, L. N., Viegas, J. S. R., Silvestrini, A. V. P., Caron, A. L., Praça, F. G., Kravicz, M., et al. (2020). Nanotechnology approaches in the current therapy of skin cancer. Adv. Drug Deliv. Rev. 153, 109–136. doi:10.1016/J.ADDR.2020.02.005
Buajordet, I., Ebbesen, J., Erikssen, J., Brørs, O., and Hilberg, T. (2001). Fatal adverse drug events: The paradox of drug treatment. J. Intern. Med. 250 (4), 327–341. doi:10.1046/J.1365-2796.2001.00892.X
Calienni, M. N., Febres-Molina, C., Llovera, R. E., Zevallos-Delgado, C., Tuttolomondo, M. E., Paolino, D., et al. (2019). Nanoformulation for potential topical delivery of Vismodegib in skin cancer treatment. Int. J. Pharm. 565, 108–122. doi:10.1016/J.IJPHARM.2019.05.002
Cameron, M. C., Lee, E., Hibler, B. P., Barker, C. A., Mori, S., Cordova, M., et al. (2019). Basal cell carcinoma: Epidemiology; pathophysiology; clinical and histological subtypes; and disease associations. J. Am. Acad. Dermatology 80 (2), 303–317. doi:10.1016/J.JAAD.2018.03.060
Carr, S., Smith, C., and Wernberg, J. (2020). Epidemiology and risk factors of melanoma. Surg. Clin. N. Am. 100 (1), 1–12. doi:10.1016/J.SUC.2019.09.005
Chen, M., Liu, X., and Fahr, A. (2011). Skin penetration and deposition of carboxyfluorescein and temoporfin from different lipid vesicular systems: In vitro study with finite and infinite dosage application. Int. J. Pharm. 408 (1–2), 223–234. doi:10.1016/J.IJPHARM.2011.02.006
Cristiano, M. C., Froiio, F., Spaccapelo, R., Mancuso, A., Nisticò, S. P., Udongo, B. P., et al. (2019). Sulforaphane-loaded ultradeformable vesicles as A potential natural nanomedicine for the treatment of skin cancer diseases. Pharmaceutics 12 (1), 6. doi:10.3390/PHARMACEUTICS12010006
Dave, V., Kumar, D., Lewis, S., and Paliwal, S. (2010). Ethosome for enhanced transdermal drug delivery of aceclofenac. Int. J. Drug Deliv. 2 (1), 81–92. doi:10.5138/IJDD.2010.0975.0215.02016
de Jong, E., Lammerts, M. U. P. A., Genders, R. E., and Bouwes Bavinck, J. N. (2022). Update of advanced cutaneous squamous cell carcinoma. J. Eur. Acad. Dermatology Venereol. 36 (S1), 6–10. doi:10.1111/JDV.17728
Didona, D., Paolino, G., Bottoni, U., and Cantisani, C. (2018). Non-melanoma skin cancer pathogenesis overview. Biomedicines 6 (1), 6. doi:10.3390/BIOMEDICINES6010006
Doheny, D., Manore, S. G., Wong, G. L., and Lo, H. W. (2020). Hedgehog signaling and truncated GLI1 in cancer. Cells 9 (9), 2114. doi:10.3390/cells9092114
Dreyfuss, I., Kamath, P., Frech, F., Hernandez, L., and Nouri, K. (2022). Squamous cell carcinoma: 2021 updated review of treatment. Dermatol. Ther. 35, e15308. doi:10.1111/DTH.15308
Dubey, V., Mishra, D., and Jain, N. K. (2007). Melatonin loaded ethanolic liposomes: Physicochemical characterization and enhanced transdermal delivery. Eur. J. Pharm. Biopharm. Official J. Arbeitsgemeinschaft Fur Pharmazeutische Verfahrenstechnik e.V 67 (2), 398–405. doi:10.1016/J.EJPB.2007.03.007
El-Kayal, M., Nasr, M., Elkheshen, S., and Mortada, N. (2019). Colloidal (-)-epigallocatechin-3-gallate vesicular systems for prevention and treatment of skin cancer: A comprehensive experimental study with preclinical investigation. Eur. J. Pharm. Sci. Official J. Eur. Fed. Pharm. Sci. 137, 104972. doi:10.1016/J.EJPS.2019.104972
Elsayed, M. M. A., Abdallah, O. Y., Naggar, V. F., and Khalafallah, N. M. (2007). Lipid vesicles for skin delivery of drugs: Reviewing three decades of research. Int. J. Pharm. 332 (1–2), 1–16. doi:10.1016/J.IJPHARM.2006.12.005
Esnault, C., Schrama, D., Houben, R., Guyétant, S., Desgranges, A., Martin, C., et al. (2022). Antibody-drug conjugates as an emerging therapy in oncodermatology. Cancers 14 (3), 778. doi:10.3390/CANCERS14030778
Essa, E. A., Bonner, M. C., and Barry, B. W. (2003). Electroporation and ultradeformable liposomes; human skin barrier repair by phospholipid. J. Control. Release 92 (1–2), 163–172. doi:10.1016/S0168-3659(03)00326-2
Fadel, M., Kassab, K., Abdel Fadeel, D. A., and Farag, M. A. (2020). Topical photodynamic therapy of tumor bearing mice with meso-tetrakis (N-methyl-4-pyridyl) porphyrin loaded in ethosomes. Photodiagnosis Photodyn. Ther. 30, 101789. doi:10.1016/J.PDPDT.2020.101789
Fang, Y. P., Tsai, Y. H., Wu, P. C., and Huang, Y. B. (2008). Comparison of 5-aminolevulinic acid-encapsulated liposome versus ethosome for skin delivery for photodynamic therapy. International Journal of Pharmaceutics 356 (1-2), 144–152. doi:10.1016/J.IJPHARM.2008.01.020
Fathalla, D., Youssef, E. M. K., and Soliman, G. M. (2020). Liposomal and ethosomal gels for the topical delivery of anthralin: Preparation, comparative evaluation and clinical assessment in psoriatic patients. Pharmaceutics 12 (5), 446. doi:10.3390/PHARMACEUTICS12050446
Feoktistova, M., and Panayotova-Dimitrova, D. (2017). Overcoming cell death resistance in skin cancer therapy: Novel translational perspectives. Exp. Dermatol. 26 (10), 854–857. doi:10.1111/EXD.13309
Ferrara, F., Benedusi, M., Sguizzato, M., Cortesi, R., Baldisserotto, A., Buzzi, R., et al. (2022). Ethosomes and transethosomes as cutaneous delivery systems for quercetin: A preliminary study on melanoma cells. Pharmaceutics 14 (5), 1038. doi:10.3390/pharmaceutics14051038
Fu, X., Shi, Y., Wang, H., Zhao, X., Sun, Q., Huang, Y., et al. (2019). Ethosomal gel for improving transdermal delivery of thymosin β-4. Int. J. Nanomedicine 14, 9275–9284. doi:10.2147/IJN.S228863
Galatage, S. T., Hebalkar, A. S., Gote, R. v., Mali, O. R., Killedar, S. G., Bhagwat, D. A., et al. (2020). Design and characterization of camptothecin gel for treatment of epidermoid carcinoma. Future J. Pharm. Sci. 6 (1), 50–11. doi:10.1186/S43094-020-00066-6
Gamal, A., Saeed, H., Abo El-Ela, F. I., and Salem, H. F. (2021). Improving the antitumor activity and bioavailability of Sonidegib for the treatment of skin cancer. Pharmaceutics 13 (10), 1560. doi:10.3390/PHARMACEUTICS13101560
Garbe, C., and Leiter, U. (2008). Epidemiology of melanoma and nonmelanoma skin cancer--the role of sunlight. Adv. Exp. Med. Biol. 624, 89–103. doi:10.1007/978-0-387-77574-6_8
Garg, U., and Jain, K. (2022). Dermal and transdermal drug delivery through vesicles and particles: Preparation and applications. Adv. Pharm. Bull. 12 (1), 45–57. doi:10.34172/apb.2022.006
Garrett-mayer, E. (2019). Chapter 23 NCI-sponsored clinical trials. Clin. Pharm. Educ. Pract. Res. 2019, 323–344. doi:10.1016/B978-0-12-814276-9.00023-4
Godin, B., and Touitou, E. (2003). Ethosomes: New prospects in transdermal delivery. Crit. Rev. Ther. Drug Carr. Syst. 20 (1), 63–102. doi:10.1615/CRITREVTHERDRUGCARRIERSYST.V20.I1.20
Gollavilli, H., Hegde, A. R., Managuli, R. S., Bhaskar, K. V., Dengale, S. J., Reddy, M. S., et al. (2020). Naringin nano-ethosomal novel sunscreen creams: Development and performance evaluation. Colloids Surfaces. B, Biointerfaces 193, 111122. doi:10.1016/J.COLSURFB.2020.111122
Gupta, V., Dhote, V., Paul, B. N., and Trivedi, P. (2014). Development of novel topical drug delivery system containing cisplatin and imiquimod for dual therapy in cutaneous epithelial malignancy. J. Liposome Res. 24 (2), 150–162. doi:10.3109/08982104.2013.865216
Hallan, S. S., Sguizzato, M., Esposito, E., and Cortesi, R. (2021). Challenges in the physical characterization of lipid nanoparticles. Pharmaceutics 13 (4), 549. doi:10.3390/PHARMACEUTICS13040549
Harman, W. W., Mckim, R. H., Mogar’, R. E., Fadiman, ’ A. N. D, J., and Stolaroff, M. J. (1966). Psychedelic agents in creative problem-solving: A pilot study. Psychol. Rep. 19 (1), 211–227. Southern Universities Press. doi:10.2466/pr0.1966.19.1.211
Heeremans, J. L. M., Gerrttsen, H. R., Meusen, S. P., Mijnheer, F. W., Gangaram Panday, R. S., Prevost, R., et al. (1995). The preparation of tissue-type plasminogen activator (t-PA) containing liposomes: Entrapment efficiency and ultracentrifugation damage. J. Drug Target. 3 (4), 301–310. doi:10.3109/10611869509015959
Honeywell-Nguyen, P. L., and Bouwstra, J. A. (2003). The in vitro transport of pergolide from surfactant-based elastic vesicles through human skin: A suggested mechanism of action. J. Control. Release 86 (1), 145–156. doi:10.1016/S0168-3659(02)00415-7
Hong, H., Wang, X., Song, X., El Fawal, G., Wang, K., Jiang, D., et al. (2021). Transdermal delivery of interleukin-12 gene targeting dendritic cells enhances the anti-tumour effect of programmed cell death protein 1 monoclonal antibody. Biomater. Transl. 2 (2), 151–164. doi:10.12336/BIOMATERTRANSL.2021.02.005
Ibrahim, T. M., Abdallah, M. H., El-Megrab, N. A., and El-Nahas, H. M. (2019). Transdermal ethosomal gel nanocarriers; a promising strategy for enhancement of anti-hypertensive effect of carvedilol. J. Liposome Res. 29 (3), 215–228. doi:10.1080/08982104.2018.1529793
Ismail, T. A., Shehata, T. M., Mohamed, D. I., Elsewedy, H. S., and Soliman, W. E. (2021). Quality by design for development, optimization and characterization of brucine ethosomal gel for skin cancer delivery. Molecules 26 (11), 3454. doi:10.3390/MOLECULES26113454
Jain, S., Patel, N., Madan, P., and Lin, S. (2015). Quality by design approach for formulation, evaluation and statistical optimization of diclofenac-loaded ethosomes via transdermal route. Pharm. Dev. Technol. 20 (4), 473–489. doi:10.3109/10837450.2014.882939
Jain, S., Patel, N., Shah, M. K., Khatri, P., and Vora, N. (2017). Recent advances in lipid-based vesicles and particulate carriers for topical and transdermal application. J. Pharm. Sci. 106 (2), 423–445. doi:10.1016/J.XPHS.2016.10.001
Jantarat, C., Sirathanarun, P., Boonmee, S., Meechoosin, W., and Wangpittaya, H. (2018). Effect of piperine on skin permeation of curcumin from a bacterially derived cellulose-composite double-layer membrane for transdermal curcumin delivery. Sci. Pharm. 86 (3), 39. doi:10.3390/scipharm86030039
Jerant, A. F., Johnson, J. T., Sheridan, C. D., and Caffrey, T. J. (2000). Early detection and treatment of skin cancer. Am. Fam. Physician 62 (2), 357–368.
Kalekhan, F., Kudva, A. K., Raghu, S. v., Rao, S., Hegde, S. K., Simon, P., et al. (2022). Traditionally used natural products in preventing ionizing radiation-induced. Anti-Cancer Agents Med. Chem. 22 (1), 64–82. doi:10.2174/1871520621666210405093236
Khan, N. R., and Wong, T. W. (2016). Microwave-aided skin drug penetration and retention of 5-fluorouracil-loaded ethosomes. Expert Opin. Drug Deliv. 13 (9), 1209–1219. doi:10.1080/17425247.2016.1193152
Krishna Kollipara, R., Tallapaneni, V., Kumar Reddy Sanapalli, B., Kumar, V. G., and Venkata Satyanarayana Reddy Karri, V. (2019). Curcumin loaded ethosomal vesicular drug delivery system for the treatment of melanoma skin cancer. Res. J. Pharm. Tech 12 (4), 1783. doi:10.5958/0974-360X.2019.00298.1
Laïb, S., and Routh, A. F. (2008). Fabrication of colloidosomes at low temperature for the encapsulation of thermally sensitive compounds. Available at: https://www.sciencedirect.com/science/article/pii/S0021979707013306 (Accessed October 31, 2022).
Li, G., Fan, Y., Fan, C., Li, X., Wang, X., Li, M., et al. (2012). Tacrolimus-loaded ethosomes: Physicochemical characterization and in vivo evaluation. Eur. J. Pharm. Biopharm. Official J. Arbeitsgemeinschaft Fur Pharmazeutische Verfahrenstechnik e.V 82 (1), 49–57. doi:10.1016/J.EJPB.2012.05.011
Lin, H., Lin, L., Choi, Y., and Michniak-Kohn, B. (2020). Development and in-vitro evaluation of co-loaded berberine chloride and evodiamine ethosomes for treatment of melanoma. Int. J. Pharm. 581, 119278. doi:10.1016/J.IJPHARM.2020.119278
Luca, A. V., Gabriella, P., and Scalvenzi, F. M. (2022). New emerging treatment options for advanced basal cell carcinoma and squamous cell carcinoma. Adv. Ther. 39 (3), 1164–1178. doi:10.1007/s12325-022-02044-1
Marks, R., Gebauer, K., Shumack, S., Amies, M., Bryden, J., Fox, T. L., et al. (2001). Imiquimod 5% cream in the treatment of superficial basal cell carcinoma: Results of a multicenter 6-week dose-response trial. J. Am. Acad. Dermatology 44 (5), 807–813. doi:10.1067/MJD.2001.113689
Mbah, C., Ogbonna, J., Nzekwe, I., Ugwu, G., Ezeh, R., Builders, P., et al. (2021). Nanovesicle formulation enhances anti-inflammatory property and safe use of piroxicam. Pharm. Nanotechnol. 9 (3), 177–190. doi:10.2174/2211738509666210129151844
Misak, H., Zacharias, N., Song, Z., Hwang, S., Man, K. P., Asmatulu, R., et al. (2013). Skin cancer treatment by albumin/5-Fu loaded magnetic nanocomposite spheres in a mouse model. J. Biotechnol. 164 (1), 130–136. doi:10.1016/J.JBIOTEC.2013.01.003
Mishra, D. K., Dhote, V., and Mishra, P. K. (2013). Transdermal immunization: Biological framework and translational perspectives. Expert Opin. Drug Deliv. 10 (2), 183–200. doi:10.1517/17425247.2013.746660
Mishra, D., Mishra, P. K., Dabadghao, S., Dubey, V., Nahar, M., and Jain, N. K. (2010). Comparative evaluation of Hepatitis B surface antigen-loaded elastic liposomes and ethosomes for human dendritic cell uptake and immune response. Nanomedicine Nanotechnol. Biol. Med. 6 (1), 110–118. doi:10.1016/J.NANO.2009.04.003
Moideen Muthu Mohamed, J., Khan, B. A., Rajendran, V., El-Sherbiny, M., Othman, G., Bashir Ahmed Hussamuldin, A., et al. (2022). Polymeric ethosomal gel loaded with nimodipine: Optimisation, pharmacokinetic and histopathological analysis. Saudi Pharm. J. 30, 1603–1611. doi:10.1016/J.JSPS.2022.09.003
Moolakkadath, T., Aqil, M., Ahad, A., Imam, S. S., Praveen, A., Sultana, Y., et al. (2019). Fisetin loaded binary ethosomes for management of skin cancer by dermal application on UV exposed mice. Int. J. Pharm. 560, 78–91. doi:10.1016/j.ijpharm.2019.01.067
Mou, Q., Ma, Y., Zhu, X., and Yan, D. (2016). A small molecule nanodrug consisting of amphiphilic targeting ligand-chemotherapy drug conjugate for targeted cancer therapy. J. Control. Release Official J. Control. Release Soc. 230, 34–44. doi:10.1016/J.JCONREL.2016.03.037
Mousa, I. A., Hammady, T. M., Gad, S., Zaitone, S. A., El-Sherbiny, M., and Sayed, O. M. (2022). Formulation and characterization of metformin-loaded ethosomes for topical application to experimentally induced skin cancer in mice. Pharmaceuticals 15 (6), 657. doi:10.3390/PH15060657
Naeff, R. (1996). Feasibility of topical liposome drugs produced on an industrial scale. Adv. Drug Deliv. Rev. 18 (3), 343–347. doi:10.1016/0169-409X(95)00080-Q
Nagore, E., Hueso, L., Botella-Estrada, R., Alfaro-Rubio, A., Serna, I., Guallar, J., et al. (2010). Smoking, sun exposure, number of nevi and previous neoplasias are risk factors for melanoma in older patients (60 years and over). J. Eur. Acad. Dermatology Venereol. 24 (1), 50–57. doi:10.1111/J.1468-3083.2009.03353.X
Nair, R. S., Billa, N., Leong, C. O., and Morris, A. P. (2021). An evaluation of tocotrienol ethosomes for transdermal delivery using Strat-M® membrane and excised human skin. Pharm. Dev. Technol. 26 (2), 243–251. doi:10.1080/10837450.2020.1860087
Nanamori, H., and Sawada, Y. (2022). Epigenetic modification of PD-1/PD-L1-mediated cancer immunotherapy against melanoma. Int. J. Mol. Sci. 23 (3), 1119. doi:10.3390/IJMS23031119
Nasr, S., Rady, M., Gomaa, I., Syrovet, T., Simmet, T., Fayad, W., et al. (2019). Ethosomes and lipid-coated chitosan nanocarriers for skin delivery of a chlorophyll derivative: A potential treatment of squamous cell carcinoma by photodynamic therapy. Int. J. Pharm. 568, 118528. doi:10.1016/J.IJPHARM.2019.118528
Natsheh, H., Vettorato, E., and Touitou, E. (2019). Ethosomes for dermal administration of natural active molecules. Curr. Pharm. Des. 25 (21), 2338–2348. doi:10.2174/1381612825666190716095826
Nayak, D., Thathapudi, N. C., Ashe, S., and Nayak, B. (2021). Bioengineered ethosomes encapsulating AgNPs and Tasar silk sericin proteins for non melanoma skin carcinoma (NMSC) as an alternative therapeutics. Int. J. Pharm. 596, 120265. doi:10.1016/J.IJPHARM.2021.120265
Nazari Vishkaei, M., Khadeer Ahamed Basheer, M., and Malik Shah Abdul Majid, A. (2018). In vivo topical delivery of ethosomal formulation composed of Orthosiphon stamineus extract complexed with sophorolipid against melanoma. Front. Pharmacol. 9, 00034. doi:10.3389/CONF.FPHAR.2018.63.00034
Niu, X. Q., Zhang, D. P., Bian, Q., Feng, X. F., Li, H., Rao, Y. F., et al. (2019). Mechanism investigation of ethosomes transdermal permeation. Int. J. Pharm. X, 100027. doi:10.1016/J.IJPX.2019.100027
Pak, E., and Segal, R. A. (2016). Hedgehog signal transduction: Key players, oncogenic drivers, and cancer therapy. Dev. Cell 38 (4), 333–344. doi:10.1016/J.DEVCEL.2016.07.026
Paliwal, S., Tilak, A., Sharma, J., Dave, V., Sharma, S., Yadav, R., et al. (2019). Flurbiprofen loaded ethosomes - transdermal delivery of anti-inflammatory effect in rat model. Lipids Health Dis. 18 (1), 133–215. doi:10.1186/s12944-019-1064-x
Pandey, S., Misra, S. K., and Sharma, N. (2017). Ethosomes- A novelize vesicular drug delivery system. Res. J. Pharm. Technol. 10 (9), 3223–3232. doi:10.5958/0974-360X.2017.00572.8
Paolino, D., Celia, C., Trapasso, E., Cilurzo, F., and Fresta, M. (2012). Paclitaxel-loaded ethosomes®: Potential treatment of squamous cell carcinoma, a malignant transformation of actinic keratoses. Eur. J. Pharm. Biopharm. Official J. Arbeitsgemeinschaft Fur Pharmazeutische Verfahrenstechnik e.V 81 (1), 102–112. doi:10.1016/J.EJPB.2012.02.008
Patel, A., Sharma, R., Trivedi, M., and Panicker, A. (2013). Ethosomes: A novel tool for transdermal drug delivery. Res. J. Pharm. Tech 6 (8), 838–841.
Patel, H. M. (1990). Liposomes: A practical approach. FEBS Lett. 275 (1–2), 242–243. doi:10.1016/0014-5793(90)81487-9
Pathak, K., and Kumari, S. (2013). Cavamax W7 composite psoralen ethosomal gel versus cavamax W7 psoralen solid complex gel for topical delivery: A comparative evaluation. Int. J. Pharm. Investigation 3 (4), 171. doi:10.4103/2230-973X.121284
Portugal, I., Jain, S., Severino, P., and Priefer, R. (2021). Micro- and nano-based transdermal delivery systems of photosensitizing drugs for the treatment of cutaneous malignancies. Pharm. (Basel, Switz. 14 (8), 772. doi:10.3390/PH14080772
Prasanthi, D., and Lakshmi, P. K. (2012). Development of ethosomes with taguchi robust design-based studies for transdermal delivery of alfuzosin hydrochloride. Int. Curr. Pharm. J. 1 (11), 370–375. doi:10.3329/ICPJ.V1I11.12063
Puri, R., and Jain, S. (2012). Ethogel topical formulation for increasing the local bioavailability of 5-fluorouracil: A mechanistic study. Anti-Cancer Drugs 23 (9), 923–934. doi:10.1097/CAD.0B013E3283534051
Raghuvanshi, A., Shah, K., and Dewangan, H. K. (2022). Ethosome as antigen delivery carrier: Optimisation, evaluation and induction of immunological response via nasal route against Hepatitis B. J. Microencapsul. 39 (4), 352–363. doi:10.1080/02652048.2022.2084169
Rahim Khan, N., and Wong, T. W. (2018). Artificial Cells, Nanomedicine, and Biotechnology 5-Fluorouracil ethosomes-skin deposition and melanoma permeation synergism with microwaveFluorouracil ethosomes-skin deposition and melanoma permeation synergism with microwave 5-Fluorouracil ethosomes-skin deposition and melanoma permeation synergism with microwave. Nanomedicine, Biotechnol. 46, 568–577. doi:10.1080/21691401.2018.1431650
Rakesh, R., and Anoop, K. R. (2012). Formulation and optimization of nano-sized ethosomes for enhanced transdermal delivery of cromolyn sodium. Int. J. Pharm. Pharm. Sci. 4, 333. doi:10.4103/0975-7406.103274
Rao, L. S. (2019). Preparation of liposomes on the industrial scale: Problems and perspectives. Liposome Technol. 14, 247–257. doi:10.1201/9781351074100-18
Ravikumar, P., and Tatke, P. (2019). Advances in encapsulated dermal formulations in chemoprevention of melanoma: An overview. J. Cosmet. Dermatology 18 (6), 1606–1612. doi:10.1111/JOCD.13105
Rimkus, T. K., Carpenter, R. L., Qasem, S., Chan, M., and Lo, H. W. (2016). Targeting the sonic hedgehog signaling pathway: Review of smoothened and GLI inhibitors. Cancers 8 (2), 22. doi:10.3390/CANCERS8020022
Romero, E. L., and Morilla, M. J. (2013). Highly deformable and highly fluid vesicles as potential drug delivery systems: Theoretical and practical considerations. Int. J. Nanomedicine 8, 3171–3186. doi:10.2147/IJN.S33048
Saraf, S., and Kumar Gupta, M. (2018). Itraconazole loaded ethosomal gel system for efficient treatment of skin cancer. Int. J. Drug Deliv. 10 (1), 12–19. doi:10.5138/09750215.2246
Sari, Z. A. L., Yahya, Y. F., and Toruan, T. L. (2020). The applicability of Sonic hedgehog in mixed type basal cell carcinoma. J. General - Proced. Dermatology Venereol. Indonesia 4 (2), 86–90. doi:10.19100/JDVI.V4I2.128
Sarwa, K., Suresh, P., Debnath, M., and Ahmad, M. (2013). Tamoxifen citrate loaded ethosomes for transdermal drug delivery system: Preparation and characterization. Curr. Drug Deliv. 10 (4), 466–476. doi:10.2174/1567201811310040011
Shen, L. N., Zhang, Y. T., Wang, Q., Xu, L., and Feng, N. P. (2014). Enhanced in vitro and in vivo skin deposition of apigenin delivered using ethosomes. Int. J. Pharm. 460 (1–2), 280–288. doi:10.1016/J.IJPHARM.2013.11.017
Simões, M. C. F., Sousa, J. J. S., and Pais, A. A. C. C. (2015). Skin cancer and new treatment perspectives: A review. Cancer Lett. 357 (1), 8–42. doi:10.1016/J.CANLET.2014.11.001
Skoda, A. M., Simovic, D., Karin, V., Kardum, V., Vranic, S., and Serman, L. (2018). The role of the hedgehog signaling pathway in cancer: A comprehensive review. Bosnian J. Basic Med. Sci. 18 (1), 8–20. doi:10.17305/BJBMS.2018.2756
Song, C. K., Balakrishnan, P., Shim, C. K., Chung, S. J., Chong, S., and Kim, D. D. (2012). A novel vesicular carrier, transethosome, for enhanced skin delivery of voriconazole: Characterization and in vitro/in vivo evaluation. Colloids Surfaces. B, Biointerfaces 92, 299–304. doi:10.1016/J.COLSURFB.2011.12.004
Song, X., Jiang, Y., Zhang, W., Elfawal, G., Wang, K., Jiang, D., et al. (2022). Transcutaneous tumor vaccination combined with anti-programmed death-1 monoclonal antibody treatment produces a synergistic antitumor effect. Acta Biomater. 140, 247–260. doi:10.1016/J.ACTBIO.2021.11.033
Soni, K., Mujtaba, A., Akhter, M. H., Zafar, A., and Kohli, K. (2020). Optimisation of ethosomal nanogel for topical nano-CUR and sulphoraphane delivery in effective skin cancer therapy. J. Microencapsul. 37 (2), 91–108. doi:10.1080/02652048.2019.1701114
Sultana, B., Rajak, P., Bhuyan, B., Patra, E., Baruah, A., and Paul, D. (2018). Therapeutic potential of herbal ethosome in applied nanotechnology. Saudi J. Med. Pharm. Sci. 4 (4), 443–454. doi:10.21276/sjmps.2018.4.4.11
Tiwari, A., Mishra, K., Nayak, K., Yadav, K., and Shukla, A. (2016). Ethosomes:A novel vesicular carrier system for therapeutic applications. IOSR J. Pharm. 6 (9), 25–33.
Touitou, E., Dayan, N., Bergelson, L., Godin, B., and Eliaz, M. (2000). Ethosomes - novel vesicular carriers for enhanced delivery: Characterization and skin penetration properties. J. Control. Release 65 (3), 403–418. doi:10.1016/S0168-3659(99)00222-9
Touitou, E., and Godin, B. (2007). Ethosomes for skin delivery. J. Drug Deliv. Sci. Technol. 17 (5), 303–308. doi:10.1016/S1773-2247(07)50046-8
Touitou, Elka (1996). US5540934A - compositions for applying active substances to or through the skin - google Patents. Available at: https://patents.google.com/patent/US5540934A/en.
Trager, M. H., Geskin, L. J., Samie, F. H., and Liu, L. (2022). Biomarkers in melanoma and non-melanoma skin cancer prevention and risk stratification. Exp. Dermatol. 31 (1), 4–12. doi:10.1111/EXD.14114
Trotta, M., Peira, E., Carlotti, M. E., and Gallarate, M. (2004). Deformable liposomes for dermal administration of methotrexate. Int. J. Pharm. 270 (1–2), 119–125. doi:10.1016/J.IJPHARM.2003.10.006
United States Patent (2022). US5716638A - composition for applying active substances to or through the skin - google Patents. (n.d.). Available at: https://patents.google.com/patent/US5716638A/en (Accessed July 29, 2022).
van der Poort, E. K. J., Gunn, D. A., Beekman, M., Griffiths, C. E. M., Slagboom, P. E., van Heemst, D., et al. (2020). Basal cell carcinoma genetic susceptibility increases the rate of skin ageing: A mendelian randomization study. J. Eur. Acad. Dermatology Venereol. JEADV 34 (1), 97–100. doi:10.1111/JDV.15880
Vanic, Željka (2015). Phospholipid vesicles for enhanced drug delivery in dermatology. J. Drug Discov. Dev. Deliv. 1 (2), 8.
Verma, D. D., and Fahr, A. (2004). Synergistic penetration enhancement effect of ethanol and phospholipids on the topical delivery of cyclosporin A. J. Control. Release 97 (1), 55–66. doi:10.1016/J.JCONREL.2004.02.028
Verma, P., and Pathak, K. (2010). Therapeutic and cosmeceutical potential of ethosomes: An overview. J. Adv. Pharm. Technol. Res. 1 (3), 274–282. doi:10.4103/0110-5558.72415
Viros, A., Sanchez-Laorden, B., Pedersen, M., Furney, S. J., Rae, J., Hogan, K., et al. (2014). Ultraviolet radiation accelerates BRAF-driven melanomagenesis by targeting TP53. Nature 511 (7510), 478–482. doi:10.1038/nature13298
Williams, A. C., and Barry, B. W. (2004). Penetration enhancers. Adv. Drug Deliv. Rev. 56 (5), 603–618. doi:10.1016/j.addr.2003.10.025
World Health Organization (2022). Cancer. (n.d.). Available at: https://www.who.int/news-room/fact-sheets/detail/cancer (Accessed October 31, 2022).
Yingchoncharoen, P., Kalinowski, D. S., and Richardson, D. R. (2016). Lipid-based drug delivery systems in cancer therapy: What is available and what is yet to come. Pharmacol. Rev. 68 (3), 701–787. doi:10.1124/PR.115.012070
Yu, X., Du, L., Li, Y., Fu, G., and Jin, Y. (2015). Improved anti-melanoma effect of a transdermal mitoxantrone ethosome gel. Biomed. Pharmacother. = Biomedecine Pharmacother. 73, 6–11. doi:10.1016/J.BIOPHA.2015.05.002
Zhang, J. P., Wei, Y. H., Zhou, Y., Li, Y. Q., and Wu, X. A. (2012). Ethosomes, binary ethosomes and transfersomes of terbinafine hydrochloride: A comparative study. Archives Pharmacal Res. 35 (1), 109–117. doi:10.1007/S12272-012-0112-0
Zhang, Y. T., Shen, L. N., Wu, Z. H., Zhao, J. H., and Feng, N. P. (2014). Comparison of ethosomes and liposomes for skin delivery of psoralen for psoriasis therapy. Int. J. Pharm. 471 (1–2), 449–452. doi:10.1016/J.IJPHARM.2014.06.001
Zhang, Z., Wo, Y., Zhang, Y., Wang, D., He, R., Chen, H., et al. (2012). In vitro study of ethosome penetration in human skin and hypertrophic scar tissue. Nanomedicine Nanotechnol. Biol. Med. 8 (6), 1026–1033. doi:10.1016/J.NANO.2011.10.006
Zhang, Z., Zhang, Z., Chen, Y., Xu, H., Wo, Y., Liu, Y., et al. (2016). 5-Aminolevulinic acid loaded ethosomal vesicles with high entrapment efficiency for in vitro topical transdermal delivery and photodynamic therapy of hypertrophic scars. Nanoscale 8 (46), 19270–19279. doi:10.1039/C6NR06872C
Keywords: skin cancer, ethosomes, drug delivery, ethanol, characterization, phospholipid
Citation: Shinde P, Page A and Bhattacharya S (2023) Ethosomes and their monotonous effects on Skin cancer disruption. Front. Nanotechnol. 5:1087413. doi: 10.3389/fnano.2023.1087413
Received: 02 November 2022; Accepted: 02 January 2023;
Published: 12 January 2023.
Edited by:
Rovshan Khalilov, Baku State University, AzerbaijanReviewed by:
Dilpreet Singh, Indo-Soviet Friendship College of Pharmacy, IndiaVan-An Duong, Gachon University, South Korea
Copyright © 2023 Shinde, Page and Bhattacharya. This is an open-access article distributed under the terms of the Creative Commons Attribution License (CC BY). The use, distribution or reproduction in other forums is permitted, provided the original author(s) and the copyright owner(s) are credited and that the original publication in this journal is cited, in accordance with accepted academic practice. No use, distribution or reproduction is permitted which does not comply with these terms.
*Correspondence: Sankha Bhattacharya, sankhabhatt@gmail.com
†ORCID: Sankha Bhattacharya, orcid.org/0000-0002-0771-9582