- 1Division of Genetics and Genomics, Boston Children’s Hospital, Boston, MA, United States
- 2Department of Immunology and Nano-Medicine, Herbert Wertheim College of Medicine, Florida International University, Miami, FL, United States
- 3NanoBioTech Laboratory, Department of Environmental Engineering, Florida Polytechnic University, Lakeland, FL, United States
Neurogenesis encompasses the formation and development of neurons in the mammalian brain, mainly occurring in hippocampus and the olfactory system. This process is rapid, accurate, and very sensitive to the external stressors including environment, diet, age, anxiety, stress, depression, diet, and hormones. The range of stressors is big and directly impacts the generation, maturation and migration, efficacy, and myelination of the neuronal cells. The field of regenerative medicine focuses on combating the direct or indirect effects of these stressors on the process of neurogenesis, and ensures increased general and neuronal communications and functioning. Understanding the deep secrets of brain signaling and devising ways to increase drug availability is tough, considering the complexity and intricate details of the neuronal networks and signaling in the CNS. It is imperative to understand this complexity and introduce potent and efficacious ways to combat diseases. This perspective offers an insight into how neurogenesis could be aided by nanotechnology and what plausible nanomaterials are available to culminate neurogenesis-related neurological disorders. The nanomaterials are promising as they are minute, robust, and effective and help in diagnostics and therapeutics such as drug delivery, maturation and neuroprotection, neurogenesis, imaging, and neurosurgery.
Introduction
Neurogenesis is defined as the process by which new neurons develop in the mammalian brain, and this process may be carried over throughout the human life in selective areas such as the hippocampus and the olfactory system, termed as neurogenic regions (Reid et al., 2020; Khan et al., 2021; Riddle and Lichtenwalner, 2011; Liaw et al., 2019) (Figure 1, Niklison-Chirou et al., 2020). Even though the phenomenon of neurogenesis happens throughout the life span, it is observed to be faster in young ages compared to aging subjects, where it is much slower. The most significant neurogenesis happens in the hippocampus region of the brain which is directly associated with memory formation and learning, and any defect in hippocampus neurogenesis has severe effects (Kempermann et al., 2015; Eriksson et al., 1998; Gage et al., 2019; Gonçalves et al., 2016; Ihunwo et al., 2016; Paredes et al., 2018). The newly formed neurons advance into the formation of new synaptic connections along with the old network of neurons, leading to the development of activity-dependent structural plasticity in the brain (Kolb and Gibb, 2011). The process of neurogenesis then advances into maturation and migration of the neural cells, synapse formation, and neuronal myelin formation to form mature healthy functioning neurons (Kolb and Gibb, 2011; Kolb et al., 2014). The process of initial neurogenesis is mostly achieved by 5 months, but the cells in hippocampus continue to produce neuronal cells for the entire lifetime. Adult neurogenesis is regulated by copious stimuli, which could be specific or non-specific stimuli and contribute toward acclimatization of the neuronal response in complex or novel situations (Kempermann et al., 2015).
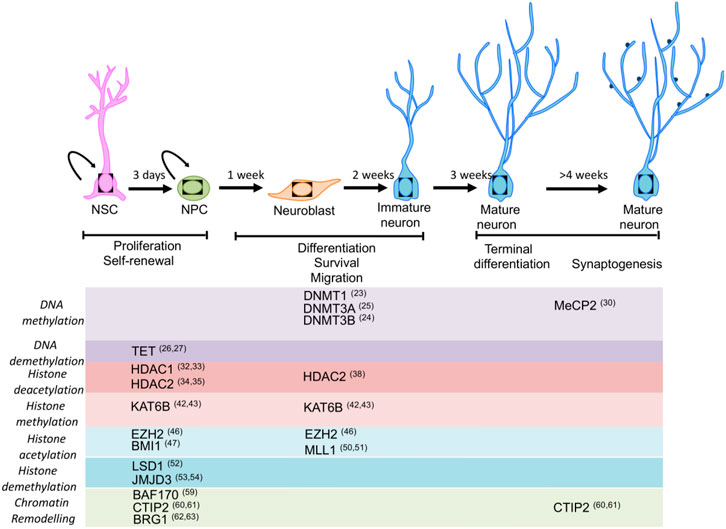
FIGURE 1. Adult mammalian neurogenesis and the associated factors. The important steps are cell proliferation, differentiation, and survival, which are rhythmic and very sensitive to external stressors and cellular environment (Niklison-Chirou et al., 2020); copyright permission from Frontiers 2020.
The formation and development of neurons is very sensitive to external stressors such as environmental stress, hormones, diet, psychoactive drug abuse, psychedelic drugs, aging, anxiety and depression, and lifestyle (Begdache et al., 2020). This process of neuronal developmental is fast and accurate; therefore, it is more sensitive to the brain perturbations and stressors. In addition, adult neurogenesis process is carried out in the dentate gyrus as well along with the hippocampus, which is easily affected by physical and environmental stressors (Fares et al., 2019; Ming and Song, 2011). Many factors have an effect on the efficacy and speed of adult neurogenesis, which could be positive such as exercise, diet, mindfulness-based meditation, and good environment (Shaffer, 2016; Phillips, 2017, Jackson et al., 2016, Mora, 2013). Neurogenesis is important for the regeneration of adult CNS neurons after any disorder or injury. The failure of adult neurogenesis in hippocampus results in complexity and various disorders such as depression, dementia, epilepsy, and stress (Kempermann et al., 2015).
Regenerative Medicine and Neurogenesis
Understanding of neurogenesis in various tissues especially in the CNS opens an important task toward damage control of human cells and tissues, and this field devoted to studying genesis is called regenerative medicine (Liaw et al., 2019). Therefore, regenerative medicine is currently an urgent need for CNS-related disorders and injuries. Regenerative medicine is a research front which studies human cell regeneration into heathy and functional cells to ensure recovery and efficacy after damage of tissues due to diseases or trauma. It comprises studying of various tools such as biological delivery, cellular delivery and function, and therapeutics intervention to the target tissues (Tian et al., 2018). It is a promising tool in aiding a lot of tissue damage and disorders but faces a big challenge in treating CNS-related degenerative disorders due to limiting access of the pharmaceuticals in the CNS pertaining to the semipermeable nature of the blood–brain barrier and increases risk of causing damage to the healthy tissue while targeting the nervous system injuries and degeneration as neurogenesis comprises both cellular genesis and replacement of old cells as well as functional efficacy such as restoration and synaptic plasticity. The neuronal cells and associated immune factors play an important role and need external aid during regeneration after coping with neuronal or CNS-associated trauma and injury.
Existing Challenges in Neurogenesis
It is imperative to understand that after an external CNS damage or impact, the stimulation and start of neurogenesis is important along with the suppression of the growth-inhibiting processes of the biological response to injury (Urbán and Guillemot, 2014; Zamproni et al., 2021). This process is convoluted and involves various cellular pathways and the bioavailability of the regenerative medicine without extreme invasion is highly required as well as difficult to achieve. That is why the clinical win of the regenerative medicine with CNS injury–related recovery has been thin. There is a pressing need for an intervention and introduction of alternate ways to account for more bioavailability of the drugs in the brain without extreme invasive approach in patients. Alternate strategies including bio scaffolds (Zamproni et al., 2021), bioengineering (Oliveira et al., 2018), and nanotechnology (Liaw et al., 2019) have been reported to increase the availability of therapeutics in the CNS and aid neuro repair. Current mediations lack direct stimulation of neurogenesis as they tend to target the stabilization, maturation, and alleviation of neuronal death. Therefore, there is more inclination toward nanotechnology as it has been aiding and introducing innovative therapeutic advances in several fields of research such as infectious diseases (Kaushik et al., 2017; Kaushik et al., 2018), cancer, pulmonary, and several more including the field of regenerative medicine and neurological disorders (Kaushik et al., 2016; Tiwari et al., 2019). The nanomedicine focuses on the pairing of the available drugs with a wide range of available nanoparticles to enhance drug delivery, overcome the shortcoming of less bioavailability of the drugs at the target, and stimulate signaling and cellular processes.
Assistance of Nanotechnology for Neurogenesis
Neurogenesis happens in the CNS and is extremely vulnerable to innate and environmental factors, affecting the overall process of neuron regeneration in the brain. It is only fit to address the overview of nanotechnology and its applications in CNS regeneration, its targeting efficacy of cellular and extracellular targets. Unraveling the functioning of the CNS milieu and understanding the functioning of various molecules and pathways is not robust due to the severe complexity and intricacy of CNS neuronal network, and it poses a big challenge (Das et al., 2016; Kumar et al., 2017; Tan et al., 2017). Where the current available interventions lag in providing direct stimulation of neurogenesis, neuro-nanotechnology in medicine stands as one of the potent and promising tools to provide faster, more efficacious, and targeted aid in neurogenesis to contribute toward successful clinical trials of various available and potent therapeutics and pharmaceuticals.
The nanotechnology has evolved and incorporates sophisticated nanomaterials to increase accessibility to the brain, in efforts toward ensuring the regenerative medicine reaches the brain and aids synaptic and functional repair of the neurons and plasticity. Nanotechnology provides innovative materials to aid nervous system regeneration by direct stimulation with the least invasion (Kumar et al., 2017). Nanotechnological maneuvers enhance the crucial cellular and tissue recovery and function and repair. Some of the important nanotechnological front comprises the delivery of nanotechnological devices for targeted cellular stimulation of neuronal cells, delivery of therapeutics, and targeted gene delivery to aid neurogenesis (Singh et al., 2021; Asghari et al., 2021; Krishnan, 2021; Carradori et al., 2017). It also comprises drugs loaded within or on the surface of the nanoparticle to ensure increased bioavailability, less toxicity, accurate targeting, and increased potential (Rakotoarisoa and Angelova, 2018; Pampaloni et al., 2018). Some of the promising tools for efficacious delivery in the field of infectious diseases and cancer, against the semipermeable blood–brain barrier, include organic nanoparticles, non-toxic hydrogels, polymers, carbon nanotubes, nano-scaffolds, liposomes, and magnetic nanoparticles (Xu et al., 2021; Kaushik, 2019; Bhardwaj et al., 2019) (Figure 2).
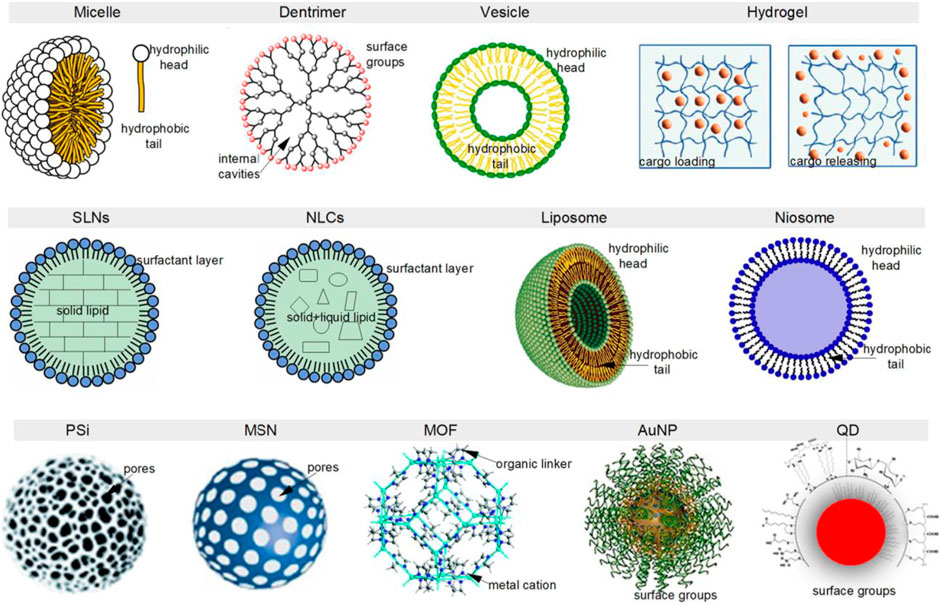
FIGURE 2. Nanotechnology introduces various types of nanoparticles to be employed in the non-invasive and effective CNS drug delivery which aids in neurogenesis (Figure from article Dubey et al., 2022).
Rakotoariosa et al. demonstrated the development of soft lipid– and polymer-based delivery carriers of curcumin, with focus on amphiphilic liquid crystalline nanocarriers for encapsulating curcumin, for ease in delivery to minimize the advanced neuronal loss in neurological disorders (Rakotoarisoa and Angelova, 2018). Furthermore, nanomaterials aid in developing novel and effective tissue engineering and therapeutics for CNS repair. Nanotube scaffolds are promising candidates for increasing conductivity to enhance regenerative potential of the implant site by increasing electrochemical activity (Kumar et al., 2015). Moreover, hydrogels which have promising potential (Buwalda et al., 2017), carbon-based materials, such as carbon nanotubes and graphene (Rauti et al., 2016), nano-scaffolds (Bosi et al., 2015), carbon nanotubes as substrates for neuronal growth (Mattson et al., 2000; Lovat et al., 2005), polymers (Cui et al., 2016) have been widely studied in clinical and applied neuroscience research. Nanobiotechnology brings in a platform to aid early-stage neurogenesis and provide therapy of specific neurodegeneration-related disorders (Nehra et al., 2021; Kumar et al., 2021; Dubey et al., 2022).
Conclusion
There is an urgency to unravel the various novel nanotechnological devices, therapeutics, and strategies to assure targeted and efficacious delivery of pharmaceuticals and therapies to target especially CNS injuries and aid in neurogenesis. It is important to advocate technologies which can aid in targeting and activating immune cells with nanotechnology, boost activation of immune system during tissue injury with nanotechnology, target injured tissues, and activate neurogenesis and repair with the aid of nanotechnology, nanomedicine to increase bioavailability of pharmaceuticals in the CNS, nanomedicine to facilitate delivery across blood–brain barrier, nano biosensors to sense tissue damage and activate repair, nanomedicine to target CNS cells and stimulate immune cells, and nano-enabled efficiency increase in neurogenesis in the CNS. This will promote the understanding of the neurogenesis strategies and how nanotechnology will bring development in this field, promising potential growth and efficacy in this field leading to more successful clinical trials, to combat neuronal disorders, as illustrated in Figure 3.
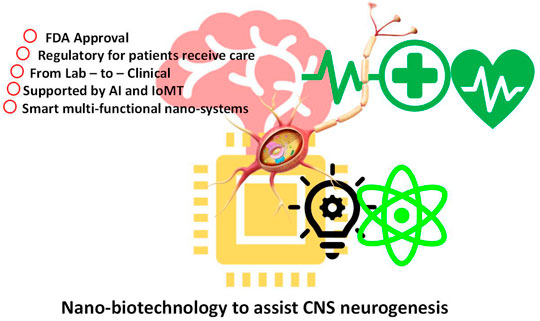
FIGURE 3. Schematic presentation of strategies and steps by which nanobiotechnology assists CNS neurogenesis.
Data Availability Statement
The original contributions presented in the study are included in the article/Supplementary Material; further inquiries can be directed to the corresponding authors.
Author Contributions
All authors listed have made a substantial, direct, and intellectual contribution to the work and approved it for publication.
Funding
Please be noted that this article is invited, and a complete waiver has been approved with reference to the code as DSC-12032329620PRD.
Conflict of Interest
The authors declare that the research was conducted in the absence of any commercial or financial relationships that could be construed as a potential conflict of interest.
Publisher’s Note
All claims expressed in this article are solely those of the authors and do not necessarily represent those of their affiliated organizations, or those of the publisher, the editors, and the reviewers. Any product that may be evaluated in this article, or claim that may be made by its manufacturer, is not guaranteed or endorsed by the publisher.
Acknowledgments
Authors acknowledge their respective department and institutions for providing support and facilities.
References
Asghari Niari, S., Rahbarghazi, R., Salehi, S., Kazemi, L., Fathi Karkan, S., Karimipour, M., et al. (2021). Fabrication, Characterization and Evaluation of the Effect of PLGA and PLGA-PEG Biomaterials on the Proliferation and Neurogenesis Potential of Human Neural SH-Sy5y Cells. Microsc. Res Tech. 85, 1433–1443. doi:10.1002/jemt.24006
Begdache, L., Sadeghzadeh, S., Derose, G., Abrams, C., Derose, G., and Abrams, C. (2020). Diet, Exercise, Lifestyle, and Mental Distress Among Young and Mature Men and Women: A Repeated Cross-Sectional Study. Nutrients 13, 24. doi:10.3390/nu13010024
Bhardwaj, V., Kaushik, A., Khatib, Z. M., Nair, M., McGoron, A. J., Nair, M., et al. (2019). Recalcitrant Issues and New Frontiers in Nano-Pharmacology. Front. Pharmacol. 10, 1369. doi:10.3389/fphar.2019.01369
Bosi, S., Rauti, R., Laishram, J., Turco, A., Lonardoni, D., Nieus, T., et al. (2015). From 2D to 3D: Novel Nanostructured Scaffolds to Investigate Signalling in Reconstructed Neuronal Networks. Sci. Rep. 5, 9562. doi:10.1038/srep09562
Buwalda, S. J., Vermonden, T., Hennink, W. E., Vermonden, T., and Hennink, W. E. (2017). Hydrogels for Therapeutic Delivery: Current Developments and Future Directions. Biomacromolecules 18, 316–330. doi:10.1021/acs.biomac.6b01604
Carradori, D., Eyer, J., Saulnier, P., Préat, V., des Rieux, A., Préat, V., et al. (2017). The Therapeutic Contribution of Nanomedicine to Treat Neurodegenerative Diseases via Neural Stem Cell Differentiation. Biomaterials 123, 77–91. doi:10.1016/j.biomaterials.2017.01.032
Cui, J., Richardson, J. J., Björnmalm, M., Faria, M., Caruso, F., Faria, M., et al. (2016). Nanoengineered Templated Polymer Particles: Navigating the Biological Realm. Acc. Chem. Res. 49, 1139–1148. doi:10.1021/acs.accounts.6b00088
Das, S., Carnicer-Lombarte, A., Fawcett, J. W., Bora, U., Fawcett, J. W., and Bora, U. (2016). Bio-inspired Nano Tools for Neuroscience. Prog. Neurobiol. 142, 1–22. doi:10.1016/j.pneurobio.2016.04.008
Dubey, A. K., Kumar Gupta, V., Kujawska, M., Orive, G., Kim, N.-Y., Li, C.-Z., et al. (2022). Exploring Nano-Enabled CRISPR-Cas-Powered Strategies for Efficient Diagnostics and Treatment of Infectious Diseases. J. Nanostructure Chem., 1–32. doi:10.1007/s40097-022-00472-7
Eriksson, P. S., Perfilieva, E., Björk-Eriksson, T., Alborn, A. M., Nordborg, C., Peterson, D. A., et al. (1998). Neurogenesis in the Adult Human hippocampus. Nat. Med. 4, 1313–1317. doi:10.1038/3305
Fares, J., Bou Diab, Z., Nabha, S., Fares, Y., Nabha, S., and Fares, Y. (2019). Neurogenesis in the Adult hippocampus: History, Regulation, and Prospective Roles. Int. J. Neurosci. 129, 598–611. doi:10.1080/00207454.2018.1545771
Gage, F. H. (2019). Adult Neurogenesis in Mammals. Science 364, 827–828. doi:10.1126/science.aav6885
Gonçalves, J. T., Schafer, S. T., and Gage, F. H. (2016). Adult Neurogenesis in the Hippocampus: From Stem Cells to Behavior. Cell 167, 897–914.
Ihunwo, A. O., Tembo, L. H., Dzamalala, C., Tembo, L. H., and Dzamalala, C. (2016). The Dynamics of Adult Neurogenesis in Human hippocampus. Neural Regen. Res. 11, 1869–1883. doi:10.4103/1673-5374.195278
Jackson, P. A., Pialoux, V., Corbett, D., Drogos, L., Erickson, K. I., Eskes, G. A., et al. (2016). Promoting Brain Health through Exercise and Diet in Older Adults: a Physiological Perspective. J. Physiol. 594, 4485–4498. doi:10.1113/JP271270
Kaushik, A., Jayant, R. D., Tiwari, S., Vashist, A., Nair, M., Vashist, A., et al. (2016). Nano-biosensors to Detect Beta-Amyloid for Alzheimer's Disease Management. Biosens. Bioelectron. 80, 273–287. doi:10.1016/j.bios.2016.01.065
Kaushik, A., Tiwari, S., Jayant, R. D., Vashist, A., Nikkhah-Moshaie, R., El-Hage, N., et al. (2017). Electrochemical Biosensors for Early Stage Zika Diagnostics. Trends Biotechnol. 35, 308–317. doi:10.1016/j.tibtech.2016.10.001
Kaushik, A., Yndart, A., Kumar, S., Jayant, R. D., Vashist, A., Brown, A. N., et al. (2018). A Sensitive Electrochemical Immunosensor for Label-free Detection of Zika-Virus Protein. Sci. Rep. 8, 9700. doi:10.1038/s41598-018-28035-3
Kaushik, A. (2019). Biomedical Nanotechnology Related Grand Challenges and Perspectives. Front. Nanotechnol. 1, 2673–3013.
Kempermann, G., Song, H., Gage, F. H., Song, H., and Gage, F. H. (2015). Neurogenesis in the Adult Hippocampus. Cold Spring Harb. Perspect. Biol. 7, a018812. doi:10.1101/cshperspect.a018812
Khan, S. M., Carter, G. T., Aggarwal, S. K., and Holland, J. (2021). Psychedelics for Brain Injury: A Mini-Review. Front. Neurol. 12, 685085.
Kolb, B., Gibb, R., Kolb, B., and Gibb, R. (2011). Brain Plasticity and Behaviour in the Developing Brain. J. Can. Acad. Child. Adolesc. Psychiatry 20, 265–276.
Kolb, B., Mychasiuk, R., Gibb, R., Mychasiuk, R., and Gibb, R. (2014). Brain Development, Experience, and Behavior. Pediatr. Blood Cancer 61, 1720–1723. doi:10.1002/pbc.24908
Krishnan, U. M. (2021). Biomaterials in the Treatment of Parkinson's Disease. Neurochem. Int. 145, 105003. doi:10.1016/j.neuint.2021.105003
Kumar, A., Tan, A., Wong, J., Spagnoli, J. C., Lam, J., Blevins, B. D., et al. (2017). Nanotechnology for Neuroscience: Promising Approaches for Diagnostics, Therapeutics and Brain Activity Mapping. Adv. Funct. Mat. 27, 1700489. doi:10.1002/adfm.201700489
Kumar, R., Aadil, K. R., Mondal, K., Mishra, Y. K., Oupicky, D., Ramakrishna, S., et al. (2021). Neurodegenerative Disorders Management: State-Of-Art and Prospects of Nano-Biotechnology. Crit. Rev. Biotechnol., 1–33. doi:10.1080/07388551.2021.1993126
Kumar, S., Ahlawat, W., Kumar, R., Dilbaghi, N., Kumar, R., and Dilbaghi, N. (2015). Graphene, Carbon Nanotubes, Zinc Oxide and Gold as Elite Nanomaterials for Fabrication of Biosensors for Healthcare. Biosens. Bioelectron. 70, 498–503. doi:10.1016/j.bios.2015.03.062
Liaw, K., Zhang, Z., Kannan, S., Zhang, Z., and Kannan, S. (2019). Neuronanotechnology for Brain Regeneration. Adv. Drug Deliv. Rev. 148, 3–18. doi:10.1016/j.addr.2019.04.004
Lovat, V., Pantarotto, D., Lagostena, L., Cacciari, B., Grandolfo, M., Righi, M., et al. (2005). Carbon Nanotube Substrates Boost Neuronal Electrical Signaling. Nano Lett. 5, 1107–1110. doi:10.1021/nl050637m
Mattson, M. P., Haddon, R. C., Rao, A. M., Haddon, R. C., and Rao, A. M. (2000). Molecular Functionalization of Carbon Nanotubes and Use as Substrates for Neuronal Growth. J. Mol. Neurosci. 14, 175–182. doi:10.1385/JMN:14:3:175
Ming, G. L., Song, H., Ming, G.-L., and Song, H. (2011). Adult Neurogenesis in the Mammalian Brain: Significant Answers and Significant Questions. Neuron 70, 687–702. doi:10.1016/j.neuron.2011.05.001
Mora, F. (2013). Successful Brain Aging: Plasticity, Environmental Enrichment, and Lifestyle. Dialogues Clin. Neurosci. 15, 45–52.
Nehra, M., Uthappa, U. T., Kumar, V., Uthappa, R., Dixit, C., Dilbaghi, N., et al. (2021). Nanobiotechnology-assisted Therapies to Manage Brain Cancer in Personalized Manner. J. Control. Release 338, 224–243. doi:10.1016/j.jconrel.2021.08.027
Niklison-Chirou, M. V., Massimiliano, A., Ivano, A., and Gerry, M. (2020). Regulation of Adult Neurogenesis in Mammalian Brain. Int. J. Mol. Sci. 21, 4869.
Oliveira, E. P., Silva-Correia, J., Reis, R. L., Oliveira, J. M., Reis, R. L., and Oliveira, J. M. (2018). Biomaterials Developments for Brain Tissue Engineering. Adv. Exp. Med. Biol. 1078, 323–346. doi:10.1007/978-981-13-0950-2_17
Pampaloni, N. P., Giugliano, M., Scaini, D., Ballerini, L., Rauti, R., Ballerini, L., et al. (2018). Advances in Nano Neuroscience: From Nanomaterials to Nanotools. Front. Neurosci. 12, 953. doi:10.3389/fnins.2018.00953
Paredes, M. F., Sorrells, S. F., Cebrian-Silla, A., Sandoval, K., Qi, D., Kelley, K. W., et al. (2018). Does Adult Neurogenesis Persist in the Human Hippocampus? Cell Stem Cell 23, 780–781. doi:10.1016/j.stem.2018.11.006
Phillips, C. (2017). Lifestyle Modulators of Neuroplasticity: How Physical Activity, Mental Engagement, and Diet Promote Cognitive Health during Aging. Neural Plast. 2017, 3589271. doi:10.1155/2017/3589271
Rakotoarisoa, M., Angelova, A., Rakotoarisoa, M., and Angelova, A. (2018). Amphiphilic Nanocarrier Systems for Curcumin Delivery in Neurodegenerative Disorders. Medicines 5, 126. doi:10.3390/medicines5040126
Rauti, R., Lozano, N., León, V., Scaini, D., Musto, M., Rago, I., et al. (2016). Graphene Oxide Nanosheets Reshape Synaptic Function in Cultured Brain Networks. ACS Nano 10, 4459–4471. doi:10.1021/acsnano.6b00130
Reid, H. M. O., Lysenko-Martin, M. R., Snowden, T. M., Thomas, J. D., Christie, B. R., Thomas, J. D., et al. (2020). A Systematic Review of the Effects of Perinatal Alcohol Exposure and Perinatal Marijuana Exposure on Adult Neurogenesis in the Dentate Gyrus. Alcohol. Clin. Exp. Res. 44, 1164–1174. doi:10.1111/acer.14332
Riddle, D. R., and Lichtenwalner, R. J. (2011). “Neurogenesis in the Adult and Aging Brain,” in Brain Aging: Models, Methods, and Mechanisms. Editor D. R. Riddle (Boca Raton (FL): CRC Press/Taylor & Francis).
Shaffer, J. (2016). Neuroplasticity and Clinical Practice: Building Brain Power for Health. Front. Psychol. 7, 1118. doi:10.3389/fpsyg.2016.01118
Singh, S., Mishra, S., Juha, S., Pramanik, M., Padmanabhan, P., Gulyás, B., et al. (2021). Nanotechnology Facilitated Cultured Neuronal Network and its Applications. Ijms 22, 5552. doi:10.3390/ijms22115552
Tan, A., Jeyaraj, R., Ashkan, K., Jeyaraj, R., and Ashkan, K. (2017). Nanotechnology in Neurosurgery: Thinking Small, Dreaming Big. Br. J. Neurosurg. 31, 538–550. doi:10.1080/02688697.2017.1327017
Tian, Z., Zhao, Q., Biswas, S., Deng, W., Biswas, S., and Deng, W. (2018). Methods of Reactivation and Reprogramming of Neural Stem Cells for Neural Repair. Methods 133, 3–20. doi:10.1016/j.ymeth.2017.08.014
Tiwari, S., Atluri, V., Kaushik, A., Yndart, A., Nair, M., Yndart, A., et al. (2019). Alzheimer's Disease: Pathogenesis, Diagnostics, and Therapeutics. Int. J. Nanomedicine 14, 5541–5554. doi:10.2147/IJN.S200490
Urbán, N., and Guillemot, F. (2014). Neurogenesis in the Embryonic and Adult Brain: Same Regulators, Different Roles. Front. Cell. Neurosci. 8, 396.
Xu, X., Liu, C., Wang, Y., Koivisto, O., Zhou, J., Shu, Y., et al. (2021). Nanotechnology-based Delivery of CRISPR/Cas9 for Cancer Treatment. Adv. Drug Deliv. Rev. 176, 113891. doi:10.1016/j.addr.2021.113891
Keywords: neurogenesis, brain diseases, dementia, nanomedicine, nanobiotechnology
Citation: Tiwari S and Kaushik A (2022) Nano-Neurogenesis for CNS Diseases and Disorders. Front. Nanotechnol. 4:931259. doi: 10.3389/fnano.2022.931259
Received: 28 April 2022; Accepted: 19 May 2022;
Published: 15 July 2022.
Edited by:
Chetna Dhand, Advanced Materials and Processes Research Institute (CSIR), IndiaReviewed by:
Jay Singh, Banaras Hindu University, IndiaPratima R. Solanki, Jawaharlal Nehru University, India
Copyright © 2022 Tiwari and Kaushik. This is an open-access article distributed under the terms of the Creative Commons Attribution License (CC BY). The use, distribution or reproduction in other forums is permitted, provided the original author(s) and the copyright owner(s) are credited and that the original publication in this journal is cited, in accordance with accepted academic practice. No use, distribution or reproduction is permitted which does not comply with these terms.
*Correspondence: Sneham Tiwari, c25laGFtLnRpd2FyaUBnbWFpbC5jb20=; Ajeet Kaushik, YWthdXNoaWtAZmxvcmlkYXBvbHkuZWR1