- 1Department of Microbiology, Obafemi Awolowo University, Ile-Ife, Nigeria
- 2Microbiology Unit, Department of Applied Sciences, Osun State College of Technology, Esa-Oke, Nigeria
- 3Department of Microbiology, University of Ilorin, Ilorin, Nigeria
- 4Department of Microbiology, Landmark University, Omu-Aran, Nigeria
- 5Department of Chemistry, Obafemi Awolowo University, Ile-Ife, Nigeria
Pathogenic bacteria and several biomolecules produced by cells and living organisms are common biological components posing a harmful threat to global health. Several studies have devised methods for the detection of varying pathogenic bacteria and biomolecules in different settings such as food, water, soil, among others. Some of the detection studies highlighting target pathogenic bacteria and biomolecules, mechanisms of detection, colorimetric outputs, and detection limits have been summarized in this review. In the last 2 decades, studies have harnessed various nanotechnology-based methods for the detection of pathogenic bacteria and biomolecules with much attention on functionalization techniques. This review considers the detection mechanisms, colorimetric prowess of bio-receptors and compares the reported detection efficiency for some bio-receptor functionalized nanoparticles. Some studies reported visual, rapid, and high-intensity colorimetric detection of pathogenic bacteria and biomolecules at a very low concentration of the analyte. Other studies reported slight colorimetric detection only with a large concentration of an analyte. The effectiveness of bio-receptor functionalized nanoparticles as detection component varies depending on their selectivity, specificity, and the binding interaction exhibited by nanoparticles, bio-receptor, and analytes to form a bio-sensing complex. It is however important to note that the colorimetric properties of some bio-receptor functionalized nanoparticles have shown strong and brilliant potential for real-time and visual-aided diagnostic results, not only to assess food and water quality but also for environmental monitoring of pathogenic bacteria and a wide array of biomolecules.
Introduction
The growing prevalence of pathogenic bacteria has become a serious challenge globally (Wang C. et al., 2018). Despite the strict implementation of good hygienic and manufacturing practices in many nations of the world, diseases outbreaks relating to food and water infections caused by pathogenic bacteria remain the leading cause of death. Examples of such include; Escherichia coli (Duan et al., 2020), Salmonella typhi (Yi et al., 2019), Salmonella enteritidis (Alamer et al., 2018), Staphylococcus aureus (Du et al., 2020), Vibrio cholera (Peng and Chen, 2019), Vibrio parahaemolyticus (Sun et al., 2019), Bacillus cereus (Li et al., 2018), Listeria monocytogenes (Zhang L. et al., 2016), Shigella flexneri (Feng et al., 2019), Pseudomonas aeruginosa (Das et al., 2019), Campylobacter jejuni (Alamer et al., 2018), and Group A Streptococcus pyogenes (Eryılmaz et al., 2020). A review by Daramola et al. (2020) reported traditional-based (plate culture), immunological-based (ELISA), nucleic acid-based (PCR, Sequencing), and protein-based (MALDI-TOF-MS) methods as veritable techniques for the detection of pathogenic bacteria. However, limitations which include longer detection time, limited trained personnel, and the use of expensive and sophisticated materials have constantly challenged the relevance of these widely used methods (Daramola et al., 2020; Ferone et al., 2020). In recent times, nanotechnology-biosensor-based techniques have received global attention with growing interest in optical biosensors namely; surface plasmon resonance (SPR), photographic and colorimetric detection (Yoo and Lee, 2016; Mocan et al., 2017; Huang et al., 2020).
To achieve reliable nanotechnology-biosensor-based techniques, bio-receptors (bio-capturing molecule/bio-recognition elements) and transducers play important roles (Figure 1) (Torimiro et al., 2021). Analytes-Bio-receptors complex triggers signals which are readable by these transducers. These bio-receptor surfaces are distinctly designed to identify and initiate attachment to identified analytes. Several bio-receptors which include antibodies, oligonucleotide aptamers, proteins, enzymes, nanozymes (chromogens), cells, antigens, microorganisms, bio-mimic substances, and other small molecules have been reported as promising functionalizing agents on nanomaterials (Nguyen and Kim, 2020). According to Daramola et al. (2020), bio-receptors attachment on nanomaterials surfaces can be achieved by a direct or indirect method which oftentimes, is dependent on the biochemical mechanisms of the bio-recognizing elements. The direct method which includes physical adsorption and covalent coupling is achieved through hydrophobic and electrostatic interactions of linkers such as Polyethylene glycol (PEG), Poly-L-lysine (PLL), Polyethylenimine (PEI), and Ethylenediaminetetraacetic acid (EDTA). On the other hand, the indirect method involves the formation of a bridge link between analytes and nanomaterials initiated by high-affinity linkers such as biotin, avidin, and streptavidin. To detect target analytes, the choice of suitable, appropriate, and corresponding bio-receptors on nanomaterials is important to ensure active biocompatibility, high selectivity sensitivity in the complex (Nguyen and Kim, 2020).
Aside from the general concept of direct detection of pathogenic bacteria by functionalized nanoparticles, biomolecules present in, and produced by microbes and other living organisms have been identified as another detectable component capable of inducing color transition. These biomolecules ranging from small molecules such as metabolites, antibiotics, antibodies, antigens, amino acids, hormones to large macromolecules such as proteins, carbohydrates, lipids, nucleic acids, enzymes, and toxins are important organic molecules required for their maintenance and metabolic activities, acting as essential molecules needed for proliferation and life’s sustenance, however, harmful to man. The accumulation of metabolites, toxins and antibiotics has increasingly endangered human and animal physiological health, posing huge risks to food safety with an increasing presence of antimicrobial-resistant bacterial pathogens in the food chain (Verraes et al., 2013; Founou et al., 2016). To further improve the applicability of functionalized nanoparticles for biosensing, colorimetric detection of bacterial pathogens and biomolecules have been adopted (Figure 2). Colorimetric-based biosensors compared with other optical biosensors support direct and visual detection of analytes by the naked eyes with visible color changes (Ziyaina et al., 2019). This simple, reliable, sensitive, and specific approach which is dependent on signal generation and amplification occur due to the presence of chromogenic substrate and the induction of specific enzyme giving off colorimetric output (Elghanian et al., 1997; Ng et al., 2016).
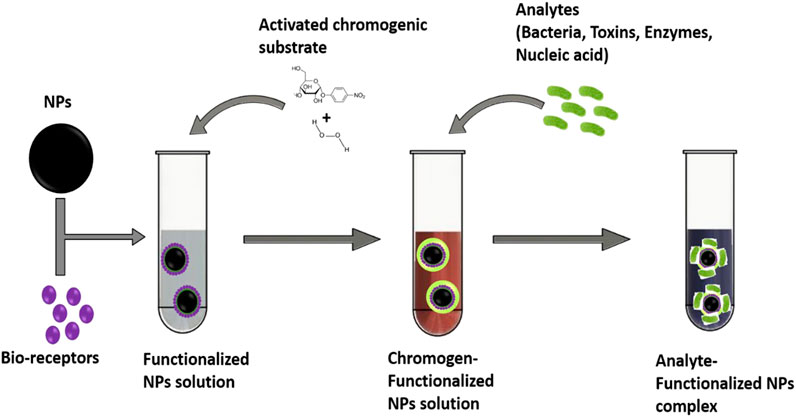
FIGURE 2. Descriptive image on the colorimetric detection of analytes using bio-receptors/chromogens–functionalized nanoparticles solution.
Increasing the relevance of nanoparticles are several chromogenic substrates which have been used as signal amplifiers (Wu et al., 2017). Interestingly, chromogenic substrates have shown excellent remark as bio-receptors and functionalizing agents, facilitating attachment and interaction of nanoparticles to analytes. Some studies have reported on the competence of chromogenic bio-materials as reliable indicators. For example, horseradish peroxidase (HRP), 3,3,5,5′-tetramethylbenzidine (TMB), and 2,2-azino-bis(3-ethylbenzothiazo-line-6-sulfonic acid) diammonium salt (ABTS) had established the potential of chromogenic materials for the detection of bacterial pathogens and biomolecules (Saunders and Bartlett, 1977; Sibanda et al., 1999). Similarly, Guven et al. (2011) reported the prospect of 5,5-dithiobis-2-nitrobenzoic acid-coated gold nanorods immobilized on polyclonal antibodies for the detection of Escherichia coli. A novel colorimetric aptasensor also reported the use of lanthanum ion-assisted gold nanoparticles for the detection of chloramphenicol (Wu et al., 2019). This review thus sought to compare the detection efficiencies of some commonly used nanoparticles functionalized with chromogenic substrates as colorimetric biosensors and electrochemical sensors in works of literature. Target analytes, detection parameters, color outputs as well as detection limits were tabulated and compared. This approach attempts to illustrate the discussion of reported detection strategies and in all, ease the identification of the most promising functionalized nanoparticles with much emphasis on chromogenic-modified nanoparticles.
Nucleic Acids
Nucleic acids which are the basic units of life have been widely recognized as the main materials that store, copy and transmit genetic information in living components. In microbial cells, they play significant roles in all their biological processes and function posing either positive or adverse effect in human (Lee et al., 2015; Tang et al., 2020). Nucleic acids existing as ribonucleic acid (RNA) and deoxyribonucleic acid (DNA) have been important biomarkers both in biological studies and medical diagnostic approaches (Amos and Patnaik, 2002; van Belkum, 2003). For over 3 decades, several methods have been developed to detect specific DNA/RNA sequences among which Polymerase chain reaction (PCR) was found as the most accurate nucleic acid amplification technology (Huang et al., 2019). Its wide practical applications and suitability for the detection of trace nucleic acid content have proved its advantages, however, the need for a precise cycling temperature, the use of expensive equipment, and a sophisticated design of primer has provided some difficulty in its use as a point-of-care test (POCT) (Huang et al., 2019).
Some works in recent times have undoubtedly advanced the diagnosis of many kinds of diseases using nanoparticles (NPs) for nucleic acid extraction (Kang et al., 2021; Zandieh and Liu, 2021) and applying electrochemical methods for the detection of specific nucleic acids in complex fluid (Jiang et al., 2021). Among these new methodologies, optical detection methods, which hinges on the hybridization between nucleic acid and substrate modified with radioactive, fluorescent, chemiluminescent, or nanoparticle tags have been of major interest. Gold nanoparticles which are more preferred than other metals have been marked as an excellent labeling tag due to their unique chemical, physical, and highly sensitive detection properties (Qin and Yung, 2007). Further quest on the need for a simple, convenient colorimetric approach for an instant and non-enzymatic detection of nucleic acid showed gold and other metals together coupled with chromogenic substrates exhibit unique optical properties which include visual color changes and varying absorption spectral which are subject to functionalized nanoparticles dispersion to aggregation state (Zhang et al., 2020). Some studies highlighting these visible color changes are discussed below.
Xia et al. (2010) in their experiment detected complementary DNA using probe DNA and AuNPs conjugated with polyelectrolytes (poly [(9,9-bis(6′-N, N, N-trimethylammonium) hexyl)fluorene-alt-1,4-phenylene] bromide). AuNPs-polyelectrolyte conjugate (red solution) which recognizes and sequesters most of the single-stranded DNA (probe DNA) produces a characteristic blue color, however, the presence of complementary strand (double-stranded DNA) initiate a weak bond with AuNPs-polyelectrolyte conjugates, alters the aggregation of the nanoparticles and thus retained the red color. This method was also found useful for the detection of small molecules, proteins, and inorganic ions. Gold nanoparticles modified with chitosan were explored for the detection of amplified Mycobacterium tuberculosis DNA (MTB DNA) (Tammam et al., 2017). The study reported a color change of the solution from red to blue. It was found that the free chitosan content in the cationic chitosan-coated AuNPs process was responsible for the direct interaction of functionalized NPs with the amplified MTB DNA. The amount of free chitosan as reported in their study suggests the accuracy of the positive indication of MTB DNA and the visual color change.
The application of peptide nucleic acid (PNA) as a bio-receptor and the potential chromogenic substrate has been reported, as it can induce immediate aggregation of NPs without the catalytic action of NaCl (Su and Kanjanawarut, 2009). Joshi et al. (2013) using PNA assessed the detection of Newcastle disease viral RNA, a single-stranded negative-sense RNA virus belonging to the family of Paramyxovirus causing highly contagious disease in poultry and almost all species of birds. It was observed that the agglomeration of AuNP-PNA produces an intense blue coloration, however, the presence of the viral DNA (complementary DNA strand) sufficiently prevents AuNPs agglomeration to PNA, hence, preventing the color change from red to blue. In addition to our examples, PNA-AgNPs have also been studied for the detection of mRNAs (proto-oncogene c-Myc mRNA) since they have an important role in the detection of oncogenes and tumor suppressors hence, emerging as potential biomarkers for cancer detection (Li et al., 2016). The study justifies the high affinity of PNA-AgNPs conjugate to only double-stranded helix structure than single-stranded DNA or RNA which protects AgNPs from salt-induced aggregation thus, producing a color change from brown to yellow. Interestingly, the study distinguished a full match from mismatch mRNA by producing a visual color change to red-brown with the presence of a single-base-mismatch mRNA.
Another related study exploited the fabrication of a paper-based device (PAD) using platinum nanoparticles (PtNPs) functionalized with a chromogenic substrate (3,3,5,5,-tetramethylbenzidine –(TMB)) for the detection and quantitation of DNA target (Chen et al., 2017). According to their study, TMB-PtNPs in the absence of DNA showed good catalytic efficiency, producing visible blue precipitates however, PtNPs peroxidase-like activities are rapidly blocked upon the addition of DNA with no color change to the solution. Summary of studies with colorimetric outputs and their limit of detection are summarized in Table 1.
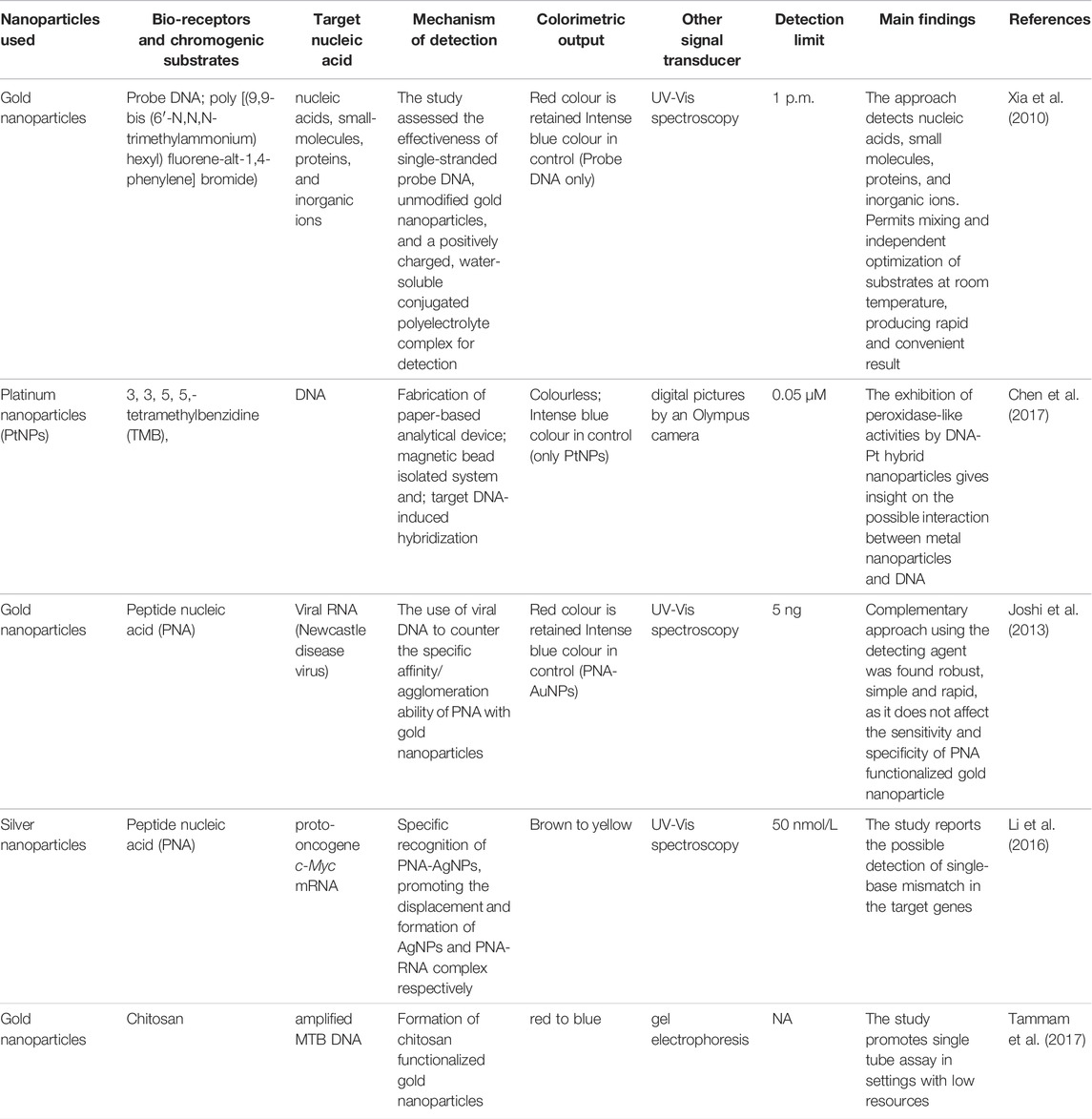
TABLE 1. Summary of studies that have applied bio-receptors and chromogenic substrates for the detection of nucleic acid.
Pathogenic Microbes
The application of gold nanoparticles in pathogen detection has been established in several studies due to their good stability and reproducibility (Mosier-Boss, 2017; Yilmaz and Yilmaz, 2020; Norouz Dizaji et al., 2021). Functionalized gold nanoparticles (AuNPs), as suggested in studies could involve the immobilization of one or more bio-receptors increasing the binding specificity of gold nanoparticles to target components (Pissuwan et al., 2020; Norouz Dizaji et al., 2021). As a resourceful strategy, the reaction of functionalized gold nanoparticles together with chromogenic substrate targeting pathogenic bacteria generates visual color change such as blue, red, purple (Kim et al., 2018; Feng et al., 2019; Sun et al., 2019). Studies have demonstrated the potential of several chromogenic-mediated and functionalized gold nanoparticles methods for the detection of pathogenic bacteria with different detection limits (Das et al., 2019; Du et al., 2020; Huang et al., 2020). Das et al. (2019) reported a detection limit of 60 CFU/ml for Pseudomonas aeruginosa using aptamer and 3,3′,5,5′-tetramethylbenzidine (TMB) as bio-receptor and color indicator on gold nanoparticles. Aptamer which suppresses the intrinsic peroxidase-like activity (NanoZyme activity) of AuNPs enhances the activities of TMB as a color indicator for the detection approach. The adaptation of the aptamer-Nanozyme colorimetric sensor (TMB) on a screen-printed electrode produced not only an electrochemical sensor output but also produces a visual blue coloration, marking the presence of P. aeruginosa. The use of both aptamers and ethanolamine as functionalizing components on gold nanoparticles was demonstrated for the detection of E. coli lipopolysaccharides (Zhu et al., 2019). Aptamer-ethanolamine interaction induces the aggregation of AuNPs, transitioning red to light purple and finally a colorless solution with a detection limit of 1 μg/ml. The use of 4-mercaptophenylboronic acid (4-MPBA) as a color indicator for the detection of several pathogens (Escherichia coli; Salmonella pullorum; Staphylococcus aureus; Enterococcus faecalis; Streptococcus mutans) was carried out by Huang et al. (2020). 4-MPBA which contains two functional groups: a thiol group and a boronic acid group facilitates strong binding with nanoparticles and peptidoglycan present in bacterial cell walls through the covalent bonds. The study established the importance of 1M NaCl concentration as a color inducer in the detection of bacterial pathogens. Thiramanas and Laocharoensuk (2016) conducted one of the foremost studies that demonstrated the efficiency of Chlorophenol red β-D-galactopyranoside (CPRG) for the detection of Staphylococcus aureus and Escherichia coli (ETEC). The competitive binding of positively charged polyethyleneimine-coated gold nanoparticles to the negatively charged bacterial enzymes (β-galactosidase) in the presence of CPRG transforms the red solution to yellow with a detection limit of 10 CFU/ml.
Silver nanoparticles (AgNPs) in comparison with AuNPs have also received attention in their single functionalized form but with lesser application due to their toxic properties although, minimal (Alabi et al., 2019; Ogunsuyi et al., 2019). Nevertheless, a study demonstrated the use of AgNPs coated with urease both as bio-receptor and chromogenic substrate for the detection of Salmonella typhimurium. The coated AgNPs were found to preferentially bind to bacterial cell surfaces, allowing the catalytic elevation of the solution pH by urease and inducing a color change from yellow to pink (Singh et al., 2019). Functionalized magnetic nanomaterials have been explored for colorimetric detection of pathogenic Salmonella typhimurium (Park et al., 2015; Srisa-Art et al., 2018). Srisa-Art et al. (2018) assessed the use of CPRG as a colorimetric paper-based analytical device (PAD) for detection and immunomagnetic separation of the pathogen. The development of the colorimetric PAD eliminates conventional pre-enrichment of samples before detection.
Some studies have reported the advantages of functionalized metal nanocomposite (bi-metallic) over the single functionalized metal nanoparticles in that, its enhances sensitivity, strong affinity with a broad group of Gram-positive and Gram-negative bacteria, and in most cases effectively capture bacterial cells when nanoparticles with magnetic (Fe3O4) and metal-organic framework (MOF) properties are involved (Wang K.-Y. et al., 2018; Duan et al., 2020; Xu et al., 2021). Dehghani et al. (2018) studied the potential of gold-palladium (Au-Pd) nanocomposites functionalized with aptamer and TMB as colorimetric aptasensor against Campylobacter jejuni. The increased aptamer-bacterial cells complex based on the strong peroxidase-like activity of Au-Pd in buffered saline intensifies the blue color of the solution. Similarly, pyrrolidonyl arylamidase (PYR) functionalized magnetic-gold (Fe-Au) nanocomposites for the detection of Group A Streptococcus pyogenes induces a red color with the addition of 4-(dimethylamino)-cinnamaldehyde (DMACA). Bacterial cells detection was due to its affinity to PYR (substrate) enhancing the production of β-naphthylamine, which upon reaction with DMACA, intensified color change from yellow to red (Eryılmaz et al., 2020). Other studies that have used bio-receptors and chromogenic substrates inducing visual color change for the detection of pathogenic bacteria are summarized in Table 2.
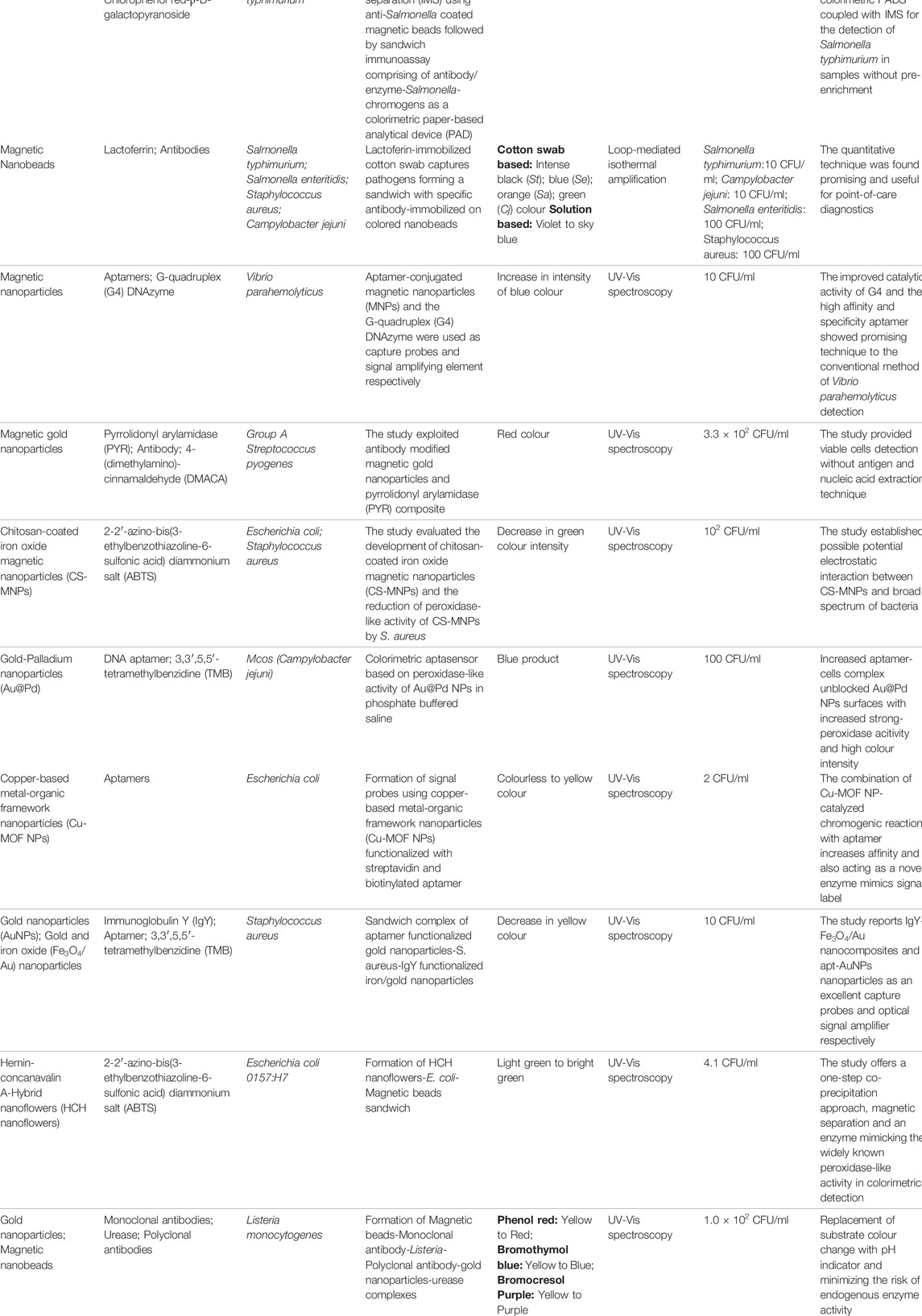
TABLE 2. Summary of studies that have applied bio-receptors and chromogenic substrates for the detection of pathogenic bacteria.
Amino Acids, Enzymes and Proteins
Enzymes and proteins act very crucial roles in facilitating diverse forms of activities that are pivotal to the survival of life in microbes, plants, animals, and humans. Enzymes are involved in the catalysis of various biochemical reactions, while proteins form structures that help in the catalysis of biochemical reactions and regulation of biological processes (Ayantunji et al., 2020). Amino acids, Enzymes and proteins include, but are not limited to cysteine, lysozyme, SARS-CoV-2 protein, C-reactive protein (CRP), Okadaic acid (OA), carcinoembryonic antigen, concanavalin A, lipase, transferrin (glycoprotein), Ebola virus secreted glycoprotein (sGP), carbonic anhydrase, alpha-amylase, catalase, and protease. For example, salivary alpha-amylase (sAA) (EC 3.2.1.1) aids in the breakdown of starch to simple sugars in the buccal cavity and has been applied as a biomarker to assess the changes relating to stress in the sympathetic nervous system (SNS) (Nater and Rohleder, 2009). The levels of diverse enzymes and proteins have been linked to the occurrence and development of myriads of diseases (Borrebaeck, 2017). Amylase has been applied in forensic medicine when investigating crime scenes to presumptively test for suspicious stains by searching for appropriate genetic material because saliva has a higher amount of amylase than sweat, semen, urine, or secretions from the nose (Wittstein et al., 2005). Therefore, selective and precise detection of enzymes and proteins is very important in proteomic research, early diagnosis, and treatment of various diseases (Xue et al., 2019). Enzyme-linked immunosorbent assay (ELISA) has been widely used for the detection of proteins due to its level of selectivity (Ambrosi et al., 2010), however, the antibodies used in this assay are less stable and very costly (Adhikary and Banerjee, 2021). Other methods used for the detection of enzymes and proteins usually consume time, require cumbersome sample preparation, and could be less discriminatory in mixtures containing complex proteins.
Diversely functionalized nanoparticles have been reported by employing colorimetric and UV-vis spectrometric methods for the detection of proteins including the report by Lee et al. (2008). These researchers conjugated gold nanoparticles with a thiol-modified oligonucleotide sequence that has been labeled with a fluorophore and incubated with different concentrations of cysteine, the conjugate provided a colorimetric change from red to purple with a detection limit of 100 nM, monitored by UV-vis spectrometry. A similar colorimetric change from red to blue with a detection limit of 100 nM was observed in a study by Zhang et al. (2010) where gold was conjugated with EDTA and complexed with lead ions (Pb2+) to induce the aggregation of AuNPs for quick qualitative and quantitative analysis. These reports showed a highly selective and sensitive assay but limited by the inability to carry out point-of-care-testing, that is free of cumbersome procedure in the laboratory. In a recent study, Adhikary and Banerjee (2021) demonstrated the use of core-shell nanoparticles made by coating chitosan-tripolyphosphate (produced by ionic gelation) with a starch-iodine shell to detect salivary alpha-amylase. These functionalized nanoparticles gave a colorimetric change from blue to red and were fabricated into paper-based diagnostic material with a detection limit of 140 units/mL (70 mg/ml) and a coated swab with a lower detection limit of 2.5 units/mL (1.25 mg/ml). This has made possible room for accessible, affordable, and instrument-free visual biosensors for point-of-care testing in diagnostic and forensic medicine by color-switching (Adhikary and Banerjee, 2021).
To improve on the limitations of the conventional ELISA, Tang et al. (2021) functionalized magnetic nanoparticles (MNPs) with maleimide and thiol-modified antibodies to detect carcinoembryonic antigen. MNPs-based ELISA for improved antigen detection was established, with an experimental sensitivity of about 100 times greater than the traditional microplate ELISA giving a detection limit of 0.02 ng/ml. Despite, the high level of sensitivity of the biomaterial design, the expensive cost, and lesser stability of antibodies involved are still major concerns. However, aptamers are being used to cater to the cost and stability concerns of antibodies. In a study by Xie et al. (2020), gold nanoparticles were conjugated with aptamer and citicoline bovine serum albumin (citicoline-BSA) to detect C-reactive protein. A very low detection limit of 8 pg/ml and a color change from colorless to blue was reported. Since the materials utilized were easy to prepare and the conjugate design has good stability between batches with high specificity, low limit of detection, low-cost and easy operation with simple instruments, the focus should be on fabricating a simple device for point-of-care-testing that would be a major predictor for the risks of cardiovascular activities (Xie et al., 2020).
Electrochemical sensors are gaining much more attention in the detection of enzymes and proteins, particularly with the emergence of SARS-CoV-2. Chest computed tomography (CT) was used for early diagnosis of COVID-19, but to detect the SARS-CoV-2 antigen, real-time reverse-transcriptase polymerase chain reaction (RT-PCR) is the gold test (Roberts et al., 2021). Though effective, RT-PCR is time-consuming, gives false-negative results for low titer value at times, is labor-intensive, and is limited by the inability for rapid use where there is a large outbreak and even for asymptomatic patients in a bid to curtail the virus spread. Chen et al. (2022) functionalized gold nanoparticles with streptavidin and biotinylated nanobodies to detect SARS-CoV-2 spike protein using spectrophotometry or portable electronic circuitry to give a detection limit of ∼1.3 p.m. An all-solid-state protein biosensor by Zhao et al. (2022) gave a quantitative analysis of SARS-CoV-2 antibody with a detection limit of 7.73 ng/ml monitored by differential pulse voltammetry (DPV) and correlation coefficient of 93.8% compared to enzyme-linked immunosorbent assay (ELISA) results. These researchers manufactured colloidal quantum dots (CQDs) and functionalized them with lead sulfide (PbS) and gold electrodes which also discriminated patient and normal samples with 90% accuracy and 1 min reading of results by handheld testing system prototype. This is a very good breakthrough if the detection limit can be further optimized. However, an earlier report by Roberts et al. (2021) accounted that gold nanoparticles functionalized with fluorine-doped tin oxide (FTO) electrodes detected SARS-CoV-2 Spike S1 antigen with a very low limit of 0.63 fM in standard buffer and 120 fM in spiked saliva samples using differential pulse voltammetry and cyclic voltammetry. No cross-reactivity was reported with other viral antigens, the response was rapidly provided within 10 s, the manufactured electrode can be stored for about 4 weeks and there is a possibility of using it directly to detect non-invasive SARS-CoV-2 from saliva samples of patients. A detailed summary of functionalized nanoparticles used for the detection of various enzymes and proteins is presented in (Supplementary Table S1). Though the overall cost of fabricating the electrode is low, there is a need for a potentiostat to sense the electrode which may need to be done in a laboratory setting and a trained handler. Yet, this could be further fabricated into a hand-held device without the need of a technician, possible usage outside of the laboratory environment, and the sensor could be manipulated to detect diverse diseases aside from SARS-CoV-2 spike S1 protein (Roberts et al., 2021).
Toxins
Toxins are biomolecules produced by living organisms (like animals, plants, and microbes) primarily for defensive purposes (Dorner and Rummel, 2015). Toxins could either be the products of metabolism secreted directly by living organisms or the materials that become toxic through microbial metabolic activity and at times, they could be the degradation products of non-living organisms (Torimiro et al., 2022). These heterogeneous groups of molecules could exert toxic properties on other organisms through ingestion, inhalation, injection, or absorption (Janik et al., 2019). Toxins include ciguatoxin, tetrodotoxin, ricin, abrin, mycotoxins, tetanus toxin, botulinum toxins, staphylococcal enterotoxins, and so on (Janik et al., 2019). The effect of these toxins on health could be acute, prolonged, or permanent, but it is to be noted that most of these biomolecules are very toxic in small amounts (Pitschmann and Hon, 2016). Toxins easily spread via contaminated food and water, and are such, are critical agents of bioterrorism (Labib et al., 2009). Previously, the detection of toxic biomolecules requires labor-intensive methods of culturing tissues and testing animals but was later replaced with laboratory methods of detection like the polymerase chain reaction (PCR), latex agglutination, and enzyme-linked immunosorbent assay (ELISA). However, these methods are difficult for field detection, as the equipment needed for such analysis is not designed for use outside of the laboratory and could take some time to confirm the presence of toxin (Patel et al., 2017). Hence, simpler, quicker, and highly selective detection methods are now evolving with better advantages over conventional methods. Nanomaterials are being used to manufacture nanosensors to assist with the possibility of onsite detection of toxic biomolecules (Zeng et al., 2016). Gold, silver and magnetic nanoparticles are the main types of nanoparticles being used because of their ability to be surface-modified and functionalized with multiple compounds, not only that, they bind specifically and selectively to target toxins (Morsi et al., 2017).
Cholera toxin has a hexametric structure with an A-subunit that is enzymatically active and linked non-covalently to the pentameric core of five B-subunits that are similar. Cholera is caused by when the A-subunit and B-subunits synergistically induce serious intestinal infection (Kuramitz et al., 2011). To detect cholera toxin, Plasmonic gold nanoparticles coated with phospholipid and embedded Raman indicators were functionalized with CT-binding ligands of ganglioside (GM1) by Zhang C.-H. et al. (2016). Surface-Enhanced Raman Scattering (SERS) was used as the signal transducer that resulted in a detection limit of 0.3 pg/ml of cholera toxin. This is useful for point of care testing and diagnostic monitoring of cholera because the nanobeacon designed is simple, prompt, and reproducible in a dynamic range (Zhang C.-H. et al., 2016). Though there was no chemical immobilization, the disadvantage with this method of using SERS is that analytes with less binding affinity to the SERS substrate will be difficult to detect. The homogeneous dispersion of silver nanoparticles was self-assembled on a floating 2D platform of mesoporous silica modified reduced graphene oxide nanosheets by Juang et al. (2020), where the advantage of the highly porous structure of mesoporous silica (MPS) and the benefit of Raman enhancement of reduced graphene oxide nanosheets were used as templates to detect uric acid which is a uremic toxin. Here, the SERS intensity increased resulting in a detection limit below 10–6 M and the signal-to-background (S/B) ratio was enhanced 6.9 times. However, electrochemical sensors which provide multiplexed analyte detection, require small amounts of analytes, and label-free sensors are now being adopted (Brahmkhatri et al., 2021).
Clostridium botulinum produces a neurotoxin that blocks the neurotransmitter to induce paralysis, even in small quantities, hence prompt detection is highly needed. To detect botulinum neurotoxin A (BoNT/A), Afkhami et al. (2017) produced gold nanoparticles functionalized with a composite of graphene and chitosan, which was used to modify a glassy carbon electrode for signal amplification. The BoNT/A antibody was immobilized on this glassy electrode resulting in a detection limit of 0.11 pg/ml of the BoNT/A and a detection range of 0.27–268 pg/ml (Afkhami et al., 2017). The measurements observed in this study were greatly target-specific and linear with logarithmic BoNT/A concentrations in human serum and milk. Staphylococcal enterotoxin B (SEB) was detected by Mousavi Nodoushan et al. (2019) using a highly specific electrochemical aptasensor that gave a detection range of 5.0–500.0 fM with a detection limit of 0.21 fM. In their report, gold nano-urchins (AuNUs) and reduced graphene oxide (rGO) were incorporated into screen-printed electrode that was later modified with a single-stranded DNA probe and a precise aptamer. The basis of this study is the ability of the aptamer to detach from the surface of the modified electrode due to the affinity of the SEB toxin molecule to move in the direction of its precise aptamer leading to an electrochemical signal change monitored by Differential Pulse Voltammetry (DPV), Cyclic Voltammetry (CV) and Electrochemical Impedance Spectroscopy (EIS). The recovery percentages observed were better and the standard deviation of this aptasensor was lower when compared with the conventional ELISA kit of SEB detection (Mousavi Nodoushan et al., 2019). For the specific detection of Aflatoxin B1 (AfB1), Nirbhaya et al. (2021) functionalized graphitic carbon nitride nanosheets with thionine and immobilized same on an indium tin oxide (ITO) coated glass electrode (Thn/g-C3N4/ITO). The setup was harnessed to covalently bind 1-ethyl-3-(3-dimethylaminopropyl)carbodiimide-N-hydroxysuccinimide (EDC-NHS) with anti-aflatoxin B1 (anti-AfB1) which conveniently blocks the non-specific sites of Aflatoxin B1 (AfB1) through the bovine serum albumin molecules. Atomic force microscopy and cyclic voltammetry were used to monitor the signal change resulting in a detection limit of 0.328 fg/ml which indicated that the fabricated biosensing electrode can detect Aflatoxin (AfB1). Several other microbial toxins detection via colorimetric approach have been highlighted by Nguyen and Kim (2021). A comprehensive list of nanotechnology-based methods where nanoparticles are functionalized with other substrates to detect the presence of toxins is explained in Supplementary Table S2.
Conclusion and Prospects
Inadequate detection of pathogenic bacteria and other harmful biomolecules in food, water, and the environment pose a huge threat to global health and safety. Studies have recorded the huge presence of harmful biological agents in a wide range of human daily needs and activities across the globe causing deleterious effects constantly. Some studies considered in this review have adequately harnessed the importance of bio-receptor and chromogen functionalized nanoparticles as bio-sensing and detection tools on harmful biological agents in processed foods and water. Unfortunately, pathogenic bacteria and biomolecules detection via bio-receptor and chromogen functionalized nanoparticles have little or no documented study from Africa, an environment with high cases of food and water contamination. There is a need to utilize this promising approach for adequate exploration and proper monitoring of these biological agents in African countries.
From the studies, aptamers play a critical role in the detection and effectiveness of pathogen and chromogenic substrates respectively. Aptamers showcase their stronger affinity/complementary properties to specific biological agent oligonucleotides causing the displacement of nanoparticles and the release of its peroxidase-like activities which induces a color change. Similarly, chromogenic substrates not limited to the widely known chromogens reviewed herein have shown an excellent remark from several authors, identifying their promising properties as a point of care diagnostic and monitoring. Careful development and its wide acceptance would completely prevent the spread of diseases in the environment, mostly antimicrobial resistance species.
For almost 2 decades, the promising nanotechnology-biosensor-based technique is yet to receive commercial acceptance/usage as an on-site and point-of-care method instead, have been restricted as a laboratory experimental technique. In recent times, the use of inexpensive materials such as solution-based approach, paper-based strip, and immuno-chromogenic substances is gradually gaining acceptance. These automated hand-held devices with visual-aided approach present easy, rapid, portable sensing and colorimetric detection that could function primarily as an indicator test both within and outside laboratory though, there is still a need to further improve on the optical and colorimetric detection approach using highly specific bio-receptors that would adequately address the detection of bacterial pathogens and their biomolecules in food and water. It is also worthy of note that bio-receptor and chromogens functionalized nanoparticles will be fully maximized in the detection of pathogenic bacteria and other harmful biological molecules if actual hand-held devices that can concurrently give a real-time and quantitative estimation are manufactured without requiring special or additional training on how to handle instrumentation.
Author Contributions
OD: Conceptualization, literature search, wrote the first draft, reviewed, and edited the manuscript. RO: literature search, wrote the first draft, reviewed, and edited the manuscript. IA: literature search. FO: literature search. BA: literature search. TF: reviewed and edited the manuscript. RG: reviewed and edited the manuscript. NT: reviewed and edited the manuscript.
Funding
This review received support from the West African Research Association and MasterCard Foundation (Ideas Matter Fellowship award 2021, granted to OBD). We are grateful for the Open Access Publication Fee Support 2022 provided by Frontiers.
Conflict of Interest
The authors declare that the research was conducted in the absence of any commercial or financial relationships that could be construed as a potential conflict of interest.
Publisher’s Note
All claims expressed in this article are solely those of the authors and do not necessarily represent those of their affiliated organizations, or those of the publisher, the editors and the reviewers. Any product that may be evaluated in this article, or claim that may be made by its manufacturer, is not guaranteed or endorsed by the publisher.
Supplementary Material
The Supplementary Material for this article can be found online at: https://www.frontiersin.org/articles/10.3389/fnano.2022.885803/full#supplementary-material
References
Adhikary, R. R., and Banerjee, R. (2021). Development of Smart Core-Shell Nanoparticle-Based Sensors for the point-of-care Detection of Alpha Amylase in Diagnostics and Forensics. Biosens. Bioelectron. 184, 113244. doi:10.1016/j.bios.2021.113244
Afkhami, A., Hashemi, P., Bagheri, H., Salimian, J., Ahmadi, A., and Madrakian, T. (2017). Impedimetric Immunosensor for the Label-free and Direct Detection of Botulinum Neurotoxin Serotype A Using Au Nanoparticles/graphene-Chitosan Composite. Biosens. Bioelectron. 93, 124–131. doi:10.1016/j.bios.2016.09.059
Alabi, O. A., Silva, A. H., Purnhagen, L. R. P., Souza, G. R. R., de Mello Júnior, L. J., Filippin-Monteiro, F. B., et al. (2019). Genetic, Reproductive and Oxidative Damage in Mice Triggered by Co-exposure of Nanoparticles: From a Hypothetical Scenario to a Real Concern. Sci. Total Environ. 660, 1264–1273. doi:10.1016/j.scitotenv.2019.01.036
Alamer, S., Eissa, S., Chinnappan, R., Herron, P., and Zourob, M. (2018). Rapid Colorimetric Lactoferrin-Based sandwich Immunoassay on Cotton Swabs for the Detection of Foodborne Pathogenic Bacteria. Talanta 185, 275–280. doi:10.1016/j.talanta.2018.03.072
Ambrosi, A., Airò, F., and Merkoçi, A. (2010). Enhanced Gold Nanoparticle Based ELISA for a Breast Cancer Biomarker. Anal. Chem. 82, 1151–1156. doi:10.1021/ac902492c
Amos, J., and Patnaik, M. (2002). Commercial Molecular Diagnostics in the U.S.: The Human Genome Project to the Clinical Laboratory. Hum. Mutat. 19, 324–333. doi:10.1002/humu.10061
Ayantunji, Y. J., Omole, R. K., Olojo, F. O., and Awojobi, K. O. (2020). Optimization of Alkaline Protease Production in Submerged Fermentation Using Bacillus Cereus Isolated from an Abattoir Wastewater in Ile-Ife, Nigeria. Jabb 23, 1–15. doi:10.9734/jabb/2020/v23i330143
Borrebaeck, C. A. K. (2017). Precision Diagnostics: Moving towards Protein Biomarker Signatures of Clinical Utility in Cancer. Nat. Rev. Cancer 17, 199–204. doi:10.1038/nrc.2016.153
Brahmkhatri, V., Pandit, P., Rananaware, P., D’Souza, A., and Kurkuri, M. D. (2021). Recent Progress in Detection of Chemical and Biological Toxins in Water Using Plasmonic Nanosensors. Trends Environ. Anal. Chem. 30, e00117. doi:10.1016/j.teac.2021.e00117
Chen, Q., Huang, F., Cai, G., Wang, M., and Lin, J. (2018). An Optical Biosensor Using Immunomagnetic Separation, Urease Catalysis and pH Indication for Rapid and Sensitive Detection of Listeria Monocytogenes. Sensors Actuators B: Chem. 258, 447–453. doi:10.1016/j.snb.2017.11.087
Chen, W., Fang, X., Li, H., Cao, H., and Kong, J. (2017). DNA-mediated Inhibition of Peroxidase-like Activities on Platinum Nanoparticles for Simple and Rapid Colorimetric Detection of Nucleic Acids. Biosens. Bioelectron. 94, 169–175. doi:10.1016/j.bios.2017.02.025
Chen, X., Kang, S., Ikbal, M. A., Zhao, Z., Pan, Y., Zuo, J., et al. (2022). Synthetic Nanobody-Functionalized Nanoparticles for Accelerated Development of Rapid, Accessible Detection of Viral Antigens. Biosens. Bioelectron. 202, 113971. doi:10.1016/j.bios.2022.113971
Daramola, O. B., Torimiro, N., Fadare, T. O., and Omole, R. K. (2020). Functionalized Inorganic Nanoparticles for the Detection of Food and Waterborne Bacterial Pathogens. Nnr 6, 1–14. doi:10.12691/nnr-6-1-1
Das, R., Dhiman, A., Kapil, A., Bansal, V., and Sharma, T. K. (2019). Aptamer-mediated Colorimetric and Electrochemical Detection of Pseudomonas aeruginosa Utilizing Peroxidase-Mimic Activity of Gold NanoZyme. Anal. Bioanal. Chem. 411, 1229–1238. doi:10.1007/s00216-018-1555-z
Dehghani, Z., Hosseini, M., Mohammadnejad, J., Bakhshi, B., and Rezayan, A. H. (2018). Colorimetric Aptasensor for Campylobacter Jejuni Cells by Exploiting the Peroxidase like Activity of Au@Pd Nanoparticles. Microchim Acta 185, 448. doi:10.1007/s00604-018-2976-2
Dorner, B., and Rummel, A. (2015). Preface Biological Toxins-Ancient Molecules Posing a Current Threat. Toxins 7, 5320–5321. doi:10.3390/toxins7124888
Du, J., Wu, S., Niu, L., Li, J., Zhao, D., and Bai, Y. (2020). A Gold Nanoparticles-Assisted Multiplex PCR Assay for Simultaneous Detection of Salmonella typhimurium, Listeria Monocytogenes and Escherichia coli O157:H7. Anal. Methods 12, 212–217. doi:10.1039/C9AY02282A
Duan, N., Yang, W., Wu, S., Zou, Y., and Wang, Z. (2020). A Visual and Sensitive Detection of Escherichia coli Based on Aptamer and Peroxidase-like Mimics of Copper-Metal Organic Framework Nanoparticles. Food Anal. Methods 13, 1433–1441. doi:10.1007/s12161-020-01765-9
Elghanian, R., Storhoff, J. J., Mucic, R. C., Letsinger, R. L., and Mirkin, C. A. (1997). Selective Colorimetric Detection of Polynucleotides Based on the Distance-dependent Optical Properties of Gold Nanoparticles. Science 277, 1078–1081. doi:10.1126/science.277.5329.1078
Eryılmaz, M., Tamer, U., and Boyacı, İ. H. (2020). Nanoparticle-assisted Pyrrolidonyl Arylamidase Assay for a Culture-free Group A Streptococcus Pyogenes Detection with Image Analysis. Talanta 212, 120781. doi:10.1016/j.talanta.2020.120781
Feng, J., Shen, Q., Wu, J., Dai, Z., and Wang, Y. (2019). Naked-eyes Detection of Shigella Flexneri in Food Samples Based on a Novel Gold Nanoparticle-Based Colorimetric Aptasensor. Food Control 98, 333–341. doi:10.1016/j.foodcont.2018.11.048
Ferone, M., Gowen, A., Fanning, S., and Scannell, A. G. M. (2020). Microbial Detection and Identification Methods: Bench Top Assays to Omics Approaches. Compr. Rev. Food Sci. Food Saf. 19, 3106–3129. doi:10.1111/1541-4337.12618
Founou, L. L., Founou, R. C., and Essack, S. Y. (2016). Antibiotic Resistance in the Food Chain: A Developing Country-Perspective. Front. Microbiol. 7, 1881. doi:10.3389/fmicb.2016.01881
Guven, B., Basaran-Akgul, N., Temur, E., Tamer, U., and Boyacı, İ. H. (2011). SERS-based sandwich Immunoassay Using Antibody Coated Magnetic Nanoparticles for Escherichia coli Enumeration. Analyst 136, 740–748. doi:10.1039/C0AN00473A
Huang, J., Sun, J., Warden, A. R., and Ding, X. (2020). Colorimetric and Photographic Detection of Bacteria in Drinking Water by Using 4-mercaptophenylboronic Acid Functionalized AuNPs. Food Control 108, 106885. doi:10.1016/j.foodcont.2019.106885
Huang, J., Zhang, Y., Lin, Z., Liu, W., Chen, X., Liu, Y., et al. (2019). Femtomolar Detection of Nucleic Acid Based on Functionalized Gold Nanoparticles. Nanophotonics 8, 1495–1503. doi:10.1515/nanoph-2019-0050
Janik, E., Ceremuga, M., Saluk-Bijak, J., and Bijak, M. (2019). Biological Toxins as the Potential Tools for Bioterrorism. Ijms 20, 1181. doi:10.3390/ijms20051181
Jiang, X., Zhu, Q., Zhu, H., Zhu, Z., and Miao, X. (2021). Antifouling Lipid Membrane Coupled with Silver Nanoparticles for Electrochemical Detection of Nucleic Acids in Biological Fluids. Analytica Chim. Acta 1177, 338751. doi:10.1016/j.aca.2021.338751
Joshi, V. G., Chindera, K., Singh, A. K., Sahoo, A. P., Dighe, V. D., Thakuria, D., et al. (2013). Rapid Label-free Visual Assay for the Detection and Quantification of Viral RNA Using Peptide Nucleic Acid (PNA) and Gold Nanoparticles (AuNPs). Analytica Chim. Acta 795, 1–7. doi:10.1016/j.aca.2013.06.037
Juang, R.-S., Cheng, Y.-W., Chen, W.-T., Wang, K.-S., Fu, C.-C., Liu, S.-H., et al. (2020). Silver Nanoparticles Embedded on Mesoporous-Silica Modified Reduced Graphene-Oxide Nanosheets for SERS Detection of Uremic Toxins and Parathyroid Hormone. Appl. Surf. Sci. 521, 146372. doi:10.1016/j.apsusc.2020.146372
Kang, J., Li, Y., Zhao, Y., Wang, Y., Ma, C., and Shi, C. (2021). Nucleic Acid Extraction without Electrical Equipment via Magnetic Nanoparticles in Pasteur Pipettes for Pathogen Detection. Anal. Biochem. 635, 114445. doi:10.1016/j.ab.2021.114445
Kim, Y.-J., Kim, H.-S., Chon, J.-W., Kim, D.-H., Hyeon, J.-Y., and Seo, K.-H. (2018). New Colorimetric Aptasensor for Rapid On-Site Detection of Campylobacter Jejuni and Campylobacter Coli in Chicken Carcass Samples. Analytica Chim. Acta 1029, 78–85. doi:10.1016/j.aca.2018.04.059
Kuramitz, H., Miyagaki, S., Ueno, E., Hata, N., Taguchi, S., and Sugawara, K. (2011). Binding Assay for Cholera Toxin Based on Sequestration Electrochemistry Using Lactose Labeled with an Electroactive Compound. Analyst 136, 2373. doi:10.1039/c1an15100b
Labib, M., Hedström, M., Amin, M., and Mattiasson, B. (2009). A Capacitive Immunosensor for Detection of Cholera Toxin. Analytica Chim. Acta 634, 255–261. doi:10.1016/j.aca.2008.12.035
Le, T. N., Tran, T. D., and Kim, M. I. (2020). A Convenient Colorimetric Bacteria Detection Method Utilizing Chitosan-Coated Magnetic Nanoparticles. Nanomaterials 10, 92. doi:10.3390/nano10010092
Lee, D., Gorkin, D. U., Baker, M., Strober, B. J., Asoni, A. L., McCallion, A. S., et al. (2015). A Method to Predict the Impact of Regulatory Variants from DNA Sequence. Nat. Genet. 47, 955–961. doi:10.1038/ng.3331
Lee, J.-S., Ulmann, P. A., Han, M. S., and Mirkin, C. A. (2008). A DNA−Gold Nanoparticle-Based Colorimetric Competition Assay for the Detection of Cysteine. Nano Lett. 8, 529–533. doi:10.1021/nl0727563
Li, F., Li, F., Yang, G., Aguilar, Z. P., Lai, W., and Xu, H. (2018). Asymmetric Polymerase Chain Assay Combined with Propidium Monoazide Treatment and Unmodified Gold Nanoparticles for Colorimetric Detection of Viable Emetic Bacillus Cereus in Milk. Sensors Actuators B: Chem. 255, 1455–1461. doi:10.1016/j.snb.2017.08.154
Li, X., Song, J., Chen, B.-L., Wang, B., Li, R., Jiang, H.-M., et al. (2016). A Label-free Colorimetric Assay for Detection of C-Myc mRNA Based on Peptide Nucleic Acid and Silver Nanoparticles. Sci. Bull. 61, 276–281. doi:10.1007/s11434-016-1004-3
Ma, X., Song, L., Zhou, N., Xia, Y., and Wang, Z. (2017). A Novel Aptasensor for the Colorimetric Detection of S. typhimurium Based on Gold Nanoparticles. Int. J. Food Microbiol. 245, 1–5. doi:10.1016/j.ijfoodmicro.2016.12.024
Mocan, T., Matea, C. T., Pop, T., Mosteanu, O., Buzoianu, A. D., Puia, C., et al. (2017). Development of Nanoparticle-Based Optical Sensors for Pathogenic Bacterial Detection. J. Nanobiotechnol 15, 25. doi:10.1186/s12951-017-0260-y
Morsi, R. E., Alsabagh, A. M., Nasr, S. A., and Zaki, M. M. (2017). Multifunctional Nanocomposites of Chitosan, Silver Nanoparticles, Copper Nanoparticles and Carbon Nanotubes for Water Treatment: Antimicrobial Characteristics. Int. J. Biol. Macromolecules 97, 264–269. doi:10.1016/j.ijbiomac.2017.01.032
Mousavi Nodoushan, S., Nasirizadeh, N., Amani, J., Halabian, R., and Imani Fooladi, A. A. (2019). An Electrochemical Aptasensor for Staphylococcal Enterotoxin B Detection Based on Reduced Graphene Oxide and Gold Nano-Urchins. Biosens. Bioelectron. 127, 221–228. doi:10.1016/j.bios.2018.12.021
Nater, U. M., and Rohleder, N. (2009). Salivary Alpha-Amylase as a Non-invasive Biomarker for the Sympathetic Nervous System: Current State of Research. Psychoneuroendocrinology 34, 486–496. doi:10.1016/j.psyneuen.2009.01.014
Ng, B. Y. C., Wee, E. J. H., West, N. P., and Trau, M. (2016). Naked-Eye Colorimetric and Electrochemical Detection of Mycobacterium Tuberculosis-Toward Rapid Screening for Active Case Finding. ACS Sens. 1, 173–178. doi:10.1021/acssensors.5b00171
Nguyen, Q. H., and Kim, M. I. (2020). Nanomaterial-mediated Paper-Based Biosensors for Colorimetric Pathogen Detection. Trac Trends Anal. Chem. 132, 116038. doi:10.1016/j.trac.2020.116038
Nguyen, Q. H., and Kim, M. I. (2021). Using Nanomaterials in Colorimetric Toxin Detection. Biochip J. 15, 123–134. doi:10.1007/s13206-021-00013-4
Nirbhaya, V., Chauhan, D., Jain, R., Chandra, R., and Kumar, S. (2021). Nanostructured Graphitic Carbon Nitride Based Ultrasensing Electrochemical Biosensor for Food Toxin Detection. Bioelectrochemistry 139, 107738. doi:10.1016/j.bioelechem.2021.107738
Norouz Dizaji, A., Simsek Ozek, N., Aysin, F., Calis, A., Yilmaz, A., and Yilmaz, M. (2021). Combining Vancomycin-Modified Gold Nanorod Arrays and Colloidal Nanoparticles as a sandwich Model for the Discrimination of Gram-Positive Bacteria and Their Detection via Surface-Enhanced Raman Spectroscopy (SERS). Analyst 146, 3642–3653. doi:10.1039/D1AN00321F
Ogunsuyi, O. I., Fadoju, O. M., Akanni, O. O., Alabi, O. A., Alimba, C. G., Cambier, S., et al. (2019). Genetic and Systemic Toxicity Induced by Silver and Copper Oxide Nanoparticles, and Their Mixture in Clarias gariepinus (Burchell, 1822). Environ. Sci. Pollut. Res. 26, 27470–27481. doi:10.1007/s11356-019-05958-6
Park, J. Y., Jeong, H. Y., Kim, M. I., and Park, T. J. (2015). Colorimetric Detection System forSalmonella typhimuriumBased on Peroxidase-like Activity of Magnetic Nanoparticles with DNA Aptamers. J. Nanomater. 2015, 1–9. doi:10.1155/2015/527126
Patel, K., Halevi, S., Melman, P., Schwartz, J., Cai, S., and Singh, B. (2017). A Novel Surface Plasmon Resonance Biosensor for the Rapid Detection of Botulinum Neurotoxins. Biosensors 7, 32. doi:10.3390/bios7030032
Peng, H., and Chen, I. A. (2019). Rapid Colorimetric Detection of Bacterial Species through the Capture of Gold Nanoparticles by Chimeric Phages. ACS Nano 13 (2), 1244–1252. doi:10.1021/acsnano.8b06395
Pissuwan, D., Gazzana, C., Mongkolsuk, S., and Cortie, M. B. (2020). Single and Multiple Detections of Foodborne Pathogens by Gold Nanoparticle Assays. WIREs Nanomed Nanobiotechnol 12. doi:10.1002/wnan.1584
Pitschmann, V., and Hon, Z. (2016). Military Importance of Natural Toxins and Their Analogs. Molecules 21, 556. doi:10.3390/molecules21050556
Preechakasedkit, P., Pinwattana, K., Dungchai, W., Siangproh, W., Chaicumpa, W., Tongtawe, P., et al. (2012). Development of a One-step Immunochromatographic Strip Test Using Gold Nanoparticles for the Rapid Detection of Salmonella typhi in Human Serum. Biosens. Bioelectron. 31, 562–566. doi:10.1016/j.bios.2011.10.031
Qin, W. J., and Yung, L. Y. L. (2007). Nanoparticle-based Detection and Quantification of DNA with Single Nucleotide Polymorphism (SNP) Discrimination Selectivity. Nucleic Acids Res. 35, e111. doi:10.1093/nar/gkm602
Quintela, I. A., de los Reyes, B. G., Lin, C.-S., and Wu, V. C. H. (2019). Simultaneous Colorimetric Detection of a Variety of Salmonella Spp. In Food and Environmental Samples by Optical Biosensing Using Oligonucleotide-Gold Nanoparticles. Front. Microbiol. 10, 1138. doi:10.3389/fmicb.2019.01138
Roberts, A., Mahari, S., Shahdeo, D., and Gandhi, S. (2021). Label-free Detection of SARS-CoV-2 Spike S1 Antigen Triggered by Electroactive Gold Nanoparticles on Antibody Coated Fluorine-Doped Tin Oxide (FTO) Electrode. Analytica Chim. Acta 1188, 339207. doi:10.1016/j.aca.2021.339207
Saunders, G. C., and Bartlett, M. L. (1977). Double-antibody Solid-phase Enzyme Immunoassay for the Detection of Staphylococcal Enterotoxin A. Appl. Environ. Microbiol. 34, 518–522. doi:10.1128/aem.34.5.518-522.1977
Shim, W.-B., Song, J.-E., Mun, H., Chung, D.-H., and Kim, M.-G. (2014). Rapid Colorimetric Detection of Salmonella Typhimuriumusing a Selective Filtration Technique Combined with Antibody-Magnetic Nanoparticle Nanocomposites. Anal. Bioanal. Chem. 406, 859–866. doi:10.1007/s00216-013-7497-6
Sibanda, L., De Saeger, S., and Van Peteghem, C. (1999). Development of a Portable Field Immunoassay for the Detection of Aflatoxin M1 in Milk. Int. J. Food Microbiol. 48, 203–209. doi:10.1016/S0168-1605(99)00045-8
Singh, P., Kakkar, S., Bharti, B., Kumar, R., and Bhalla, V. (2019). Rapid and Sensitive Colorimetric Detection of Pathogens Based on Silver-Urease Interactions. Chem. Commun. 55, 4765–4768. doi:10.1039/C9CC00225A
Srisa-Art, M., Boehle, K. E., Geiss, B. J., and Henry, C. S. (2018). Highly Sensitive Detection ofSalmonella typhimuriumUsing a Colorimetric Paper-Based Analytical Device Coupled with Immunomagnetic Separation. Anal. Chem. 90, 1035–1043. doi:10.1021/acs.analchem.7b04628
Su, X., and Kanjanawarut, R. (2009). Control of Metal Nanoparticles Aggregation and Dispersion by PNA and PNA−DNA Complexes, and its Application for Colorimetric DNA Detection. ACS Nano 3, 2751–2759. doi:10.1021/nn9005768
Sun, Y., Duan, N., Ma, P., Liang, Y., Zhu, X., and Wang, Z. (2019). Colorimetric Aptasensor Based on Truncated Aptamer and Trivalent DNAzyme for Vibrio Parahemolyticus Determination. J. Agric. Food Chem. 67, 2313–2320. doi:10.1021/acs.jafc.8b06893
Tammam, S. N., Khalil, M. A. F., Abdul Gawad, E., Althani, A., Zaghloul, H., and Azzazy, H. M. E. (2017). Chitosan Gold Nanoparticles for Detection of Amplified Nucleic Acids Isolated from Sputum. Carbohydr. Polym. 164, 57–63. doi:10.1016/j.carbpol.2017.01.051
Tang, C., He, Z., Liu, H., Xu, Y., Huang, H., Yang, G., et al. (2020). Application of Magnetic Nanoparticles in Nucleic Acid Detection. J. Nanobiotechnol 18, 62. doi:10.1186/s12951-020-00613-6
Tang, J.-B., Yang, H.-M., Gao, X.-Y., Zeng, X.-Z., and Wang, F.-S. (2021). Directional Immobilization of Antibody onto Magnetic Nanoparticles by Fc-Binding Protein-Assisted Photo-Conjugation for High Sensitivity Detection of Antigen. Analytica Chim. Acta 1184, 339054. doi:10.1016/j.aca.2021.339054
Thiramanas, R., and Laocharoensuk, R. (2016). Competitive Binding of Polyethyleneimine-Coated Gold Nanoparticles to Enzymes and Bacteria: a Key Mechanism for Low-Level Colorimetric Detection of Gram-Positive and Gram-Negative Bacteria. Microchim. Acta 183, 389–396. doi:10.1007/s00604-015-1657-7
Torimiro, N., Daramola, O. B., Omole, R. K., and Adesina, I. Z. (2022). Detection of Enterotoxin Genes in Bacillus Species Isolated from Cassava Processing Environment in Nigeria. Vat 02. doi:10.2174/2666121702666220112101938
Torimiro, N., Daramola, O. B., Oshibanjo, O. D., Otuyelu, F. O., Akinsanola, B. A., Yusuf, O. O., et al. (2021). Ecorestoration of Heavy Metals and Toxic Chemicals in Polluted Environment Using Microbe-Mediated Nanomaterials. Int. J. Environ. Bioremediation Biodegrad. 9, 8–21. doi:10.12691/ijebb-9-1-2
van Belkum, A. (2003). Molecular Diagnostics in Medical Microbiology: Yesterday, Today and Tomorrow. Curr. Opin. Pharmacol. 3, 497–501. doi:10.1016/s1471-4892(03)00108-5
Verraes, C., Van Boxstael, S., Van Meervenne, E., Van Coillie, E., Butaye, P., Catry, B., et al. (2013). Antimicrobial Resistance in the Food Chain: A Review. Ijerph 10, 2643–2669. doi:10.3390/ijerph10072643
Wang, C., Gu, B., Liu, Q., Pang, Y., Xiao, R., and Wang, S. (2018a). Combined Use of Vancomycin-Modified Ag-Coated Magnetic Nanoparticles and Secondary Enhanced Nanoparticles for Rapid Surface-Enhanced Raman Scattering Detection of Bacteria. Ijn 13, 1159–1178. doi:10.2147/IJN.S150336
Wang, K.-Y., Bu, S.-J., Ju, C.-J., Li, C.-T., Li, Z.-Y., Han, Y., et al. (2018b). Hemin-incorporated Nanoflowers as Enzyme Mimics for Colorimetric Detection of Foodborne Pathogenic Bacteria. Bioorg. Med. Chem. Lett. 28, 3802–3807. doi:10.1016/j.bmcl.2018.07.017
Wittstein, I. S., Thiemann, D. R., Lima, J. A. C., Baughman, K. L., Schulman, S. P., Gerstenblith, G., et al. (2005). Neurohumoral Features of Myocardial Stunning Due to Sudden Emotional Stress. N. Engl. J. Med. 352, 539–548. doi:10.1056/NEJMoa043046
Wu, S., Duan, N., He, C., Yu, Q., Dai, S., and Wang, Z. (2020). Surface-enhanced Raman Spectroscopic-Based Aptasensor for Shigella Sonnei Using a Dual-Functional Metal Complex-Ligated Gold Nanoparticles Dimer. Colloids Surf. B: Biointerfaces 190, 110940. doi:10.1016/j.colsurfb.2020.110940
Wu, S., Duan, N., Qiu, Y., Li, J., and Wang, Z. (2017). Colorimetric Aptasensor for the Detection of Salmonella enterica Serovar Typhimurium Using ZnFe 2 O 4 -reduced Graphene Oxide Nanostructures as an Effective Peroxidase Mimetics. Int. J. Food Microbiol. 261, 42–48. doi:10.1016/j.ijfoodmicro.2017.09.002
Wu, Y.-y., Liu, B.-w., Huang, P., and Wu, F.-Y. (2019). A Novel Colorimetric Aptasensor for Detection of Chloramphenicol Based on Lanthanum Ion-Assisted Gold Nanoparticle Aggregation and Smartphone Imaging. Anal. Bioanal. Chem. 411, 7511–7518. doi:10.1007/s00216-019-02149-7
Xia, F., Zuo, X., Yang, R., Xiao, Y., Kang, D., Vallée-Bélisle, A., et al. (2010). Colorimetric Detection of DNA, Small Molecules, Proteins, and Ions Using Unmodified Gold Nanoparticles and Conjugated Polyelectrolytes. Proc. Natl. Acad. Sci. U.S.A. 107, 10837–10841. doi:10.1073/pnas.1005632107
Xie, J., Tang, M.-Q., Chen, J., Zhu, Y.-H., Lei, C.-B., He, H.-W., et al. (2020). A sandwich ELISA-like Detection of C-Reactive Protein in Blood by Citicoline-Bovine Serum Albumin Conjugate and Aptamer-Functionalized Gold Nanoparticles Nanozyme. Talanta 217, 121070. doi:10.1016/j.talanta.2020.121070
Xu, Y., Hassan, M. M., Zhu, A., Li, H., and Chen, Q. (2021). Dual-mode of Magnetic Assisted Au@Ag SERS Tags and Cationic Conjugated UCNPs for Qualitative and Quantitative Analysis of Multiple Foodborne Pathogens. Sensors Actuators B: Chem. 344, 130305. doi:10.1016/j.snb.2021.130305
Xu, Z., Bi, X., Huang, Y., Che, Z., Chen, X., Fu, M., et al. (2018). Sensitive Colorimetric Detection of Salmonella Enteric Serovar Typhimurium Based on a Gold Nanoparticle Conjugated Bifunctional Oligonucleotide Probe and Aptamer. J. Food Saf. 38, e12482. doi:10.1111/jfs.12482
Xue, J., Yang, L., Jia, Y., Wang, H., Zhang, N., Ren, X., et al. (2019). Electrochemiluminescence Double Quenching System Based on Novel Emitter GdPO4:Eu with Low-Excited Positive Potential for Ultrasensitive Procalcitonin Detection. ACS Sens. 4, 2825–2831. doi:10.1021/acssensors.9b01552
Yao, S., Li, J., Pang, B., Wang, X., Shi, Y., Song, X., et al. (2020). Colorimetric Immunoassay for Rapid Detection of Staphylococcus aureus Based on Etching-Enhanced Peroxidase-like Catalytic Activity of Gold Nanoparticles. Microchim. Acta 187, 504. doi:10.1007/s00604-020-04473-7
Yi, J., Wu, P., Li, G., Xiao, W., Li, L., He, Y., et al. (2019). A Composite Prepared from Carboxymethyl Chitosan and Aptamer-Modified Gold Nanoparticles for the Colorimetric Determination of Salmonella typhimurium. Microchim. Acta 186, 711. doi:10.1007/s00604-019-3827-5
Yilmaz, A., and Yilmaz, M. (2020). Bimetallic Core-Shell Nanoparticles of Gold and Silver via Bioinspired Polydopamine Layer as Surface-Enhanced Raman Spectroscopy (SERS) Platform. Nanomaterials 10, 688. doi:10.3390/nano10040688
Yoo, S. M., and Lee, S. Y. (2016). Optical Biosensors for the Detection of Pathogenic Microorganisms. Trends Biotechnol. 34, 7–25. doi:10.1016/j.tibtech.2015.09.012
Zandieh, M., and Liu, J. (2021). Spherical Nucleic Acid Mediated Functionalization of Polydopamine-Coated Nanoparticles for Selective DNA Extraction and Detection. Bioconjug. Chem. 32, 801–809. doi:10.1021/acs.bioconjchem.1c00078
Zeng, Y., Ren, J., Shen, A., and Hu, J. (2016). Field and Pretreatment-free Detection of Heavy-Metal Ions in Organic Polluted Water through an Alkyne-Coded SERS Test Kit. ACS Appl. Mater. Inter. 8, 27772–27778. doi:10.1021/acsami.6b09722
Zhang, C.-H., Liu, L.-W., Liang, P., Tang, L.-J., Yu, R.-Q., and Jiang, J.-H. (2016a). Plasmon Coupling Enhanced Raman Scattering Nanobeacon for Single-step, Ultrasensitive Detection of Cholera Toxin. Anal. Chem. 88, 7447–7452. doi:10.1021/acs.analchem.6b00944
Zhang, L., Huang, R., Liu, W., Liu, H., Zhou, X., and Xing, D. (2016b). Rapid and Visual Detection of Listeria Monocytogenes Based on Nanoparticle Cluster Catalyzed Signal Amplification. Biosens. Bioelectron. 86, 1–7. doi:10.1016/j.bios.2016.05.100
Zhang, Y.-P., Chen, J., Bai, L.-Y., Zhou, X.-M., and Wang, L.-M. (2010). Gold Nanoparticle-Based Optical Probe for Quick Colorimetric Visualization of Cysteine. Jnl Chin. Chem. Soc 57, 972–975. doi:10.1002/jccs.201000135
Zhang, Z., Ye, X., Liu, Q., Hu, C., Yun, J., Liu, R., et al. (2020). Colorimetric Nucleic Acid Detection Based on Gold Nanoparticles with Branched DNA. Nano 15, 2050110. doi:10.1142/S1793292020501106
Zhao, Y., Chen, J., Hu, Z., Chen, Y., Tao, Y., Wang, L., et al. (2022). All-solid-state SARS-CoV-2 Protein Biosensor Employing Colloidal Quantum Dots-Modified Electrode. Biosens. Bioelectron. 202, 113974. doi:10.1016/j.bios.2022.113974
Zhu, L., Li, S., Shao, X., Feng, Y., Xie, P., Luo, Y., et al. (2019). Colorimetric Detection and Typing of E. coli Lipopolysaccharides Based on a Dual Aptamer-Functionalized Gold Nanoparticle Probe. Microchim Acta 186, 111. doi:10.1007/s00604-018-3212-9
Keywords: bio-receptor, functionalized nanoparticles, colorimetric detection, chromogenic substrate, bio-sensing, pathogenic bacteria, biomolecules
Citation: Daramola OB, Omole RK, Akinwale IV, Otuyelu FO, Akinsanola BA, Fadare TO, George RC and Torimiro N (2022) Bio-Receptors Functionalized Nanoparticles: A Resourceful Sensing and Colorimetric Detection Tool for Pathogenic Bacteria and Microbial Biomolecules. Front. Nanotechnol. 4:885803. doi: 10.3389/fnano.2022.885803
Received: 28 February 2022; Accepted: 21 March 2022;
Published: 13 April 2022.
Edited by:
Pradipta Ranjan Rauta, Asian Institute of Public Health, IndiaCopyright © 2022 Daramola, Omole, Akinwale, Otuyelu, Akinsanola, Fadare, George and Torimiro. This is an open-access article distributed under the terms of the Creative Commons Attribution License (CC BY). The use, distribution or reproduction in other forums is permitted, provided the original author(s) and the copyright owner(s) are credited and that the original publication in this journal is cited, in accordance with accepted academic practice. No use, distribution or reproduction is permitted which does not comply with these terms.
*Correspondence: Oluwafemi B. Daramola, b2x1d2FmZW1pZGFyYW1vbGE4QGdtYWlsLmNvbQ==