- Department of Pharmaceutics and Pharmaceutical Technology, Shree S. K. Patel College of Pharmacy Education and Research, Ganpat University, Mehsana, India
Self-assembled nanocarrier drug delivery has received profuse attention in the field of diagnosis and treatment of diseases. These carriers have proved that serious life-threatening diseases can be eliminated evidently by virtue of their characteristic design and features. This review is aimed at systematically presenting the research and advances in the field of self-assembled nanocarriers such as polymeric nanoparticles, dendrimers, liposomes, inorganic nanocarriers, solid lipid nanoparticles, polymerosomes, micellar systems, niosomes, and some other nanoparticles. The self-assembled delivery of nanocarriers has been developed in recent years for targeting diseases. Some of the innovative attempts with regard to prolonging drug action, improving bioavailability, avoiding drug resistance, enhancing cellular uptake, and so on have been discussed. The discussion about various delivery systems included the investigation conducted at the preliminary stage, i.e., preclinical trials and assessment of safety. The clinical studies of some of the recently developed self-assembled products are currently at the clinical trial phase or FDA approved.
Introduction
Since the last decade, particularly the fields of nanoscience and nanotechnology have received much attention. A wide gamut of industrial activities and research areas is now opened to be employed in applied sciences from fundamental sciences on the nanoscale. The application and production of nanocarriers have gained much importance in biological science. The production of a nanoparticle is possible in a variety of shapes and sizes along with a variety of materials that can enhance their ability to penetrate the cells (Liu, 2006). The biological system interacts with nanocarriers differently depending on its particle size, shape, aggregation state, type of cell, route of administration, and organelle targeted. A variety of physical and chemical properties is imparted by the nanocarriers as compared to other small-scale molecules (Elahi et al., 2018). The therapies which are already available can be optimized with the help of nanocarriers because they can penetrate many blockades of biological membranes. The degradation of the drug can also be avoided by nanosizing the drug according to the requirements of the administration site. The drug release can be controlled by optimizing the dose size. In addition, it also helps in increasing the economic life of the formulation (Costantino and Boraschi, 2012). Nano-sized formulation offers a larger surface area to volume ratio and helps the particle to travel in a region overcome by quantum effect. The dimensions of nanocarriers are underneath the critical wavelength of light which in turn helps them to be used for various packaging materials, coating materials, and cosmetics (Raj et al., 2012; Vasile, 2018). Nanocarriers are composed of a biodegradable polymer which is usually made up of lipids having the size in the range of 10–1000 nm. The drug particles can be entrapped inside the matrix of polymer or over the surface of the particle. The selective targeting of organelles can be done successfully with the help of nanocarriers (Kingsley et al., 2006).
Unlike bulk samples of material, nanocarriers can confer different properties. Similarly, an assembly of nanoparticles can impart a collection of properties that will be different from individual nanocarriers. Due to the ability to exploit different properties of ensembled nanocarriers, this area has started gaining tremendous interest (Figure 1). The mechanical properties of a material can be improved with the help of self-assembled nanocarriers. This type of technology confers a different type of electronic interaction, magnetic moments, and plasmons of surface of the individual nanocarriers. A variety of approaches is available for the formulation of self-assembled nanocarriers such as self-assembly in solution, self-assembly using templating methods, self-assembly at interfaces, and assisted self-assembly (Nie et al., 2010). The self-assembled nanocarriers can confer various biomedical as well as pharmaceutical applications. The structure of self-assembled nanoparticles is made up of a polycore of hydrophobic moiety with an outer shell made up of a hydrophilic group. To obtain an increased drug delivery at the site, the drug loaded nanocarriers are internalized which helps in gaining a high degree of selectivity (Na et al., 2003). The amphiphilic nature of self-assembled nanoparticles helps them to aggregate on their own in an aqueous solution (Kwon et al., 2003). As the name indicates, the self-assembled nanocarriers can adsorb on a thick layer of the substrate which can be used to form films (Fendler, 1996). The self-assembled particles are formed with the help of interaction between the particles and external forces. The forces from the interparticle act on the nanocrystal in the aqueous phase of the solution. Due to the van der Waals interaction, an electric dipole is induced and it acts along the connecting line between the atoms (Weddemann et al., 2010). To optimize the release of the drug, the distribution of the drug, therapeutic efficacy, and accumulation at the target site can be accessed with the aid of bioimaging techniques. The bioimaging probes are used to generate signals and contrast when they interact with targeted molecules. The sensitivity and biocompatibility are prerequisites to recognize the cells and tissues (Xing and Zhao, 2016).
Applications of Self-Assembled Nanoparticles
Polymeric Nanoparticles
A number of polymeric nanoparticles based self-assembled systems have been attempted since 1970 to improve the therapeutic potential of existing or novel therapeutic agents (Osorno et al., 2021). These systems are utilized in various scientific disciplines such as pharmaceutical, biomedical, and nanomaterial, due to their flexibility, stability, scalability, and cost-effectiveness (Pippa et al., 2016; Yadav et al., 2020). A self-assembled nano-betamethasone produced by chitosan cross-linking was developed by Hudan-Tsilo et al. (2021) for the treatment of contact dermatitis and comparing it with betamethasone ointments. The therapeutic efficiency of the nano-betamethasone was much better than betamethasone which was indicated by the reductions in severity of lesions, thickness of skinfold, erythrocyte sedimentation rate, WBC count, etc. Furthermore, the nano-betamethasone was also found to increase the levels of cytokines, interleukin 10, interleukin 4, and reducing the levels of tumor necrosis factor α and interleukin 1β 1 to furnish an anti-inflammatory effect. The self-assembled systems for targeting the inflammatory cells were prepared to resolve the faster clearance, low stability, and shorter half-life issues of superoxide dismutase. The group of novel polymers was utilized for their self-assembly into micellar nanoparticles to impart desirable hydrolytic characteristics, prolonged circulation, biocompatibility, and inflammation targetability. The pharmacodynamic outcomes showed excellent antioxidant and anti-inflammatory activities in adjuvant arthritis and peritonitis models (Sun et al., 2021).
The innovative pH-responsive polymeric nanoparticles for the delivery of anti-tumor drugs were fabricated by self-assembly of aminated conjugate of lignin-histidine and 10-hydroxycamptothecin. Owing to the pH sensitivity of the histidine, the pH triggered drug release was observed from the nanoparticles in the acidic environment of the tumor cells. This was confirmed from the in vitro release studies as the drug release was considerably higher at pH 4.5 (72.5%) as compared to pH 5.5 and pH 7.2 (39.1%). The treatment group in the in vivo studies in the animal models depicted about two fold increase tumor inhibition and lowered the therapy-induced side effects (Zhao et al., 2020). Desai et al. (2018) has proposed that ellipsoidal nanoparticles can facilitate the movement of the drug across the physiological barriers, cellular uptake, and biodistribution. A simplified strategy was put forward to induce the self-assembly of poly(glycerol sebacate)-co-poly(ethylene glycol) into ellipsoidal nanoparticles. Highly biocompatible nanoparticles were prepared which has the potential to exhibit delivery applications in a variety of fields. An innovative carboxymethylcellulose containing polymeric nanoparticles was fabricated by Liu et al. with a dual-responsive behavior (pH and redox reaction). High drug loading and exceptionally biocompatible nanocarriers loaded with 10-hydroxy camptothecin and pterostilbene were produced. These nanocarriers released the loaded drug quickly in the acidic environment inside the cancer cells without compromising their stability. The dual-responsive behavior was confirmed from the in vitro and in vivo studies which also indicates their potential for eliminating the cancer cells with significantly low adverse effects (Ke-Feng Liu et al., 2018).
Yang et al. (2016) linked the docetaxel to the semitelechelic N-(2-hydroxypropyl) methacrylamide copolymeric graft and incorporated the graft into polyhedral oligomeric silsesquioxane with the objective of controlling the drug release and improving therapeutic actions. These nanoparticles displayed drug release in response to stimuli such as acidic lysosomal and cytoplasmic environment, therefore considered to be particularly cytotoxic against prostate cancer cells. The in vivo studies showed that the nanocarriers were homogeneously distributed in the tumor cells and 78.9% of tumor growth inhibition was observed. Wang et al. (2019) developed an aggregation-induced emission active group of polymers to improve anticancer therapy of doxorubicin for producing the self-indicating cancer therapy by using fluorescence resonance energy transfer process. The self-assembled homogeneous nanoparticles were generated with no surface charge, high colloidal stability, discriminating drug release, and in vivo antitumor activity was similar to that of the plan doxorubicin. Additionally, these systems allow tracking of in vitro and in vivo release of drug which may confer them with the ability to perform real-time monitoring of drug release and targeting at a specific site. It was proposed that self-assembled nanoparticles of doxorubicin can be generated by freeze drying and incorporating into monomethoxy(polyethylene glcyol)-b-P(D,L-lactic-co-glycolic acid)-b-P(L-glutamic acid). Hydroxypropyl-beta-cyclodextrin was utilized as a stabilizer to protect the destruction of polymeric nanoparticles. The pharmacokinetic studies revealed that AUC and t1/2 were observed to increase several folds for the nanoparticles in comparison with the plain doxorubicin solution. Further, the distribution of drug in the tumor also increased two times with no signs of organ toxicity (Xu et al., 2015). Novel immunosensors were fabricated by following the principle of electrophoretic deposition of self-assembled polymeric for estimation of carcinoembryonic antigen. The deposition of chitosan and gold particles was undertaken to develop biocompatible and stable nanoparticles. The immunosensor exhibited extensive detection range with the lowest possible detection limit of the carcinoembryonic antigen and this flexible approach can be used for the detection of other tumors and biomolecules (Xu et al., 2017). Self-assembled vesicular polyion complexes of siRNAs and PEG block catiomers in molar ratios of 1:2 and 2:3 were reported. The observations from the transmission electron microscopy showed that the membrane thickness of 1:2 and 2:3 combinations to be 11.0 and 7.2 nm, respectively. The complexes were stabilized by cross-linking with glutaraldehyde and the resulted positively charged siRNAsome enhanced the siRNA uptake by cancer cells leading to considerable silencing of the gene and reduced cytotoxicity (Kim et al., 2019).
In the current scenario therapeutics based on nucleic acid are being considered as one of the most promising approaches for disease treatment. The development of non-toxic and efficient gene carriers is a prerequisite for success in gene delivery. Coumarin-anchored dendrimers were self-assembled in an aqueous solution with the help of hydrophobic interactions. The DNA binding was significantly improved with a minimum amount of toxicity on the transfected cells. The self-assembly of dendrimers endowed a light-responsive behavior of the drug. They were able to display anticancer activity dependent on light. The drug moiety was delivered with a gene encoding for tumor necrosis factor-related apoptosis-inducing ligand (TRAIL) because it has less effect on normal cells along with 5-fluorouracil. This study has opened the path to developing an efficient method of gene delivery and light-responsive delivery of the drug (Wang et al., 2018). To overcome the oral protein delivery due to its degradability and instability, a benzoboroxole-containing amphiphilic dendrimer (APD) was developed sensitive to glucose and pH. The glucose molecule was self-assembled in the form of micelles in an aqueous solution. The formulation exhibited good encapsulation for insulin. Benzoboroxole and glucose were made to bind reversibly so that the disassembly of micelles can be regulated with the release rate of insulin. This helped to stabilize the fluctuations in blood sugar. A mouse model with type 1 diabetes was used to demonstrate that it was able to reduce the risk of hypoglycemia. The branched APD was able to self-assemble without the use of inorganic solvents. The shell made up of PEG and APD dendrimer was able to exhibit decent biocompatibility. This study can lead to several pathways for the development of carriers that can deliver proteins via the oral route (Zeng et al., 2019).
Dendrimer-based self-assembled nanocarriers are used to develop as a potential candidate for delivery of the drug. In this study, enzyme-sensitive amphiphilic scattering Pegylated dendron-GFLG-DOX with the help of a two-step click reaction. The self-assembled dendrimer nanoparticle was prepared with an interface having a negative charge. The self-assembled nanoparticles were able to retain their place within the tumor for a longer period. Toxicity induced by DOX was reduced and no side effects were presented toward the functioning of normal organs and tissues (Li et al., 2014). Human serum albumin was used to deliver the dendriplexes of PAMAM dendrimer with the help of electrostatic interactions for the formation of self-assembled HAS-dendriplexes. The complex was characterized with the help of MTT assay and analysis of zeta potential. The HAS-dendriplexes were able to exhibit decreased cytotoxicity. It was also able to increase the transfection efficiency and decrease hemolysis. Due to these properties, it can be used as a promising technique for delivering a gene to the targeted sites. Further in vivo studies can be performed to understand the mechanism and distribution pattern of these self-assembled complexes (Liu et al., 2012).
Liposomes
The term liposomes was put forward by Sessa and Weissmann in the 1960s and since then different kinds of lipids have been utilized for developing liposomes to serve wide applications in different fields (Ranson et al., 1996; Wang and Rempel, 2015). Liposomes may be classified on the basis of their typical composition, mechanism targeting, and applications, such as intracellular, conventional liposomes, long circulating, immunoliposomes, pH-sensitive, and cationic liposomes (Sharma and Sharma, 1997; Chen et al., 2016). The liposome offers several advantages as a drug delivery system, such as ability to self-assemble, high drug loading, site targeting, lowering side effects, etc. (Sercombe et al., 2015). The application of self-assembled liposomal nanoparticles in combination with nontoxic photosensitizers for providing photodynamic therapy may be one of the interesting future prospects (Sadasivam et al., 2013). Ngweniform et al. (2009) developed cationic liposomes containing zinc phthalocyanine (ZnPc) as a photodynamic therapy for providing the cancer treatment. The drugs can be accumulated over anionic protein nanotube by electrostatic attraction which confers the system with cancer cell targeting. Figure 2 illustrates the self-assembly of cationic ZnPc containing liposomes over a genetically engineered anionic phage.
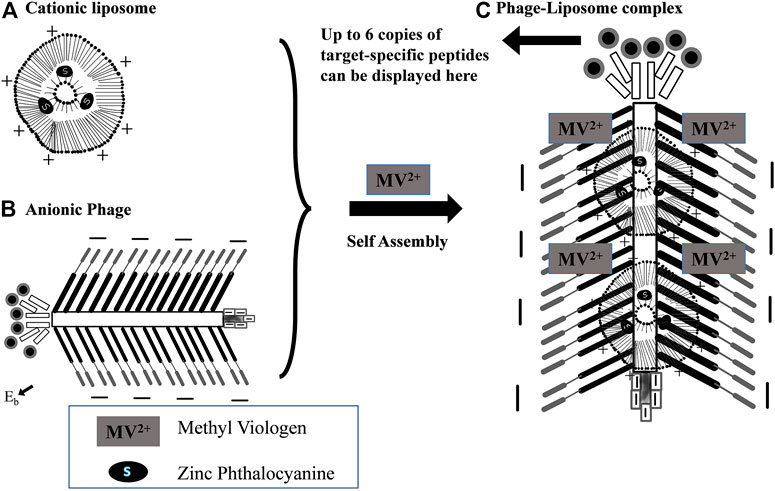
FIGURE 2. Diagrammatic illustration of the self-assembly of cationic ZnPc-liposomes (A) first over genetically engineered anionic phage, (B) then, formation of a rod-like complex between phage and liposomes, and (C) fluorescence quenching of the ZnPc. Reproduced from Soft Matter with permission from the Royal Society of Chemistry (Ngweniform et al., 2009).
Kailin Liu et al. (2018) developed pH responsive liposomes of ketoprofen due to self-assembly of microparticles containing Eudragit L100 and phosphatidyl choline by using the electrospraying method. Highly stable suspension of liposomes with about 200–300 nm particle size was generated and their shape and structure in various pH conditions altered with the concentrations of Eudragit. The pH dependent drug release was observed as around 80% of drug released after 12 h at pH 7.4, as opposed to 58% release at pH 4.5. A liposome containing therapeutic combination of a chemotherapeutic drug and a tumor microenvironment modulator were developed by Lv et al. (2018) This dual targeting system self-assembled into liposomes by incorporating hyaluronic acid-paclitaxel prodrug and Marimastat to produce a hybrid nanocarrier. The rapid release of drug from nanoparticles upon reaching the environment with mild hyperthermia, followed by quick entry into 4T1 cells. These nanoparticles depicted that 1.6-times more tumor accumulation, 10-times better tumor suppression, deeper penetration, and 10-fold improvement in angiogenesis. Figure 3 is included to display preparation of hybrid nanoparticles and mechanism of breast cancer treatment.
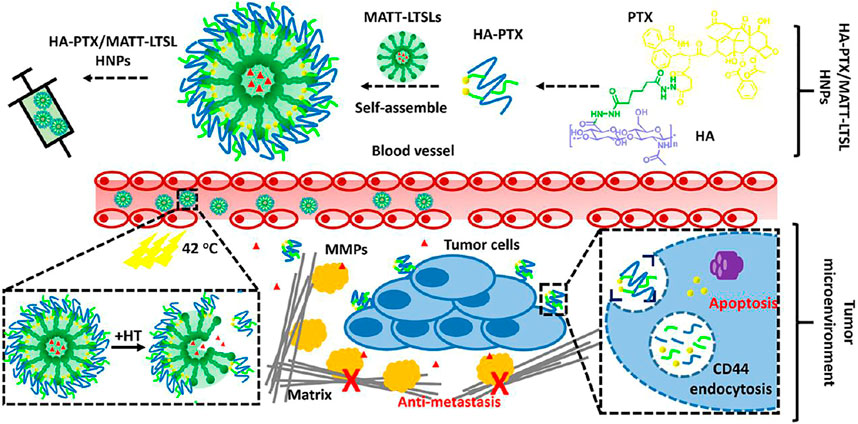
FIGURE 3. Pictorial representation dual targeting liposome-based nanoparticles for the treatment of breast cancer. These nanocarriers are comprised of hyaluronic acid-paclitaxel prodrug which gets self-assembled onto the surface of marimastat loaded liposomes. The nanoparticles when they reach the tumor tissue, it releases the drug rapidly due to hyperthermia at the tumor site. The marimastat binds with matrix metalloproteinases in the tumor microenvironment and stops the metastasis of tumor cells. On the other hand, paclitaxel enters the cell through CD44-mediated endocytosis and destroys the cancer cells. Reprinted with permission from (Lv et al., 2018). Copyright 2018 ACS Publications.
Carvedilol containing liposomes were prepared by self-assembly from fibrous mucoadhesive membranes to improve the bioavailability of the drug. The encapsulation efficiency of the drug is affected by the ratio of phospholipids to carvedilol and molecular weight of Polyvinylpyrrolidone. In vitro dialysis dissolution and permeation study revealed exceptional increase in permeability of carvedilol. Furthermore, the pharmacokinetic study showed 154% increase in relative bioavailability of the drug (Chen et al., 2018). Further, these scientists have prepared self-assembled liposomes individually by using carboxymethyl chitosan and sodium carboxymethyl cellulose. The linear release of carvedilol with almost 100% release after 2 h was observed from the dissolution study. The nanoparticles showed high permeability in the porcine TR146 cell culture and buccal mucosa and considerable safety from the MTT-assay (Chen et al., 2019). To achieve the tumor targeting self-assembled DNA, nanostructures were fabricated by incorporating doxorubicin. The nanostructures possess improved serum stability, better cellular and tissue permeability, and tumor specific drug delivery. Moreover, the anticancer activity of the formulation was much better as compared to PEGylated liposomes (Kim et al., 2016).
Inorganic Nanocarriers
The inorganic nanoparticles cover a wide arena of diagnostic and therapeutic applications due to outstanding features in contrast to their polymer and organic counterparts. They facilitate the polymers and ligands to be incorporated so that the biological function can be improved. They can be used to provide on-demand release of drugs (Anselmo and Mitragotri, 2015). The interactions via electrostatic and/or steric capabilities can provide colloidal stability of inorganic nanoparticles. Inorganic nanoparticles can form noncovalent bonds which are reversible and monomer units to organize themselves into a colloidal polymer (Henriksen-Lacey et al., 2017). Inorganic nanoparticles impart toxicity because of the alterations in their physiochemical properties when they are exposed to biological fluids. A change in their surface chemistry and size is observed under these situations. To quantify the cellular uptake for toxicity determination, various techniques such as fluorescence microscopy and flow cytometry are not able to differentiate between the inorganic nanoparticles which are absorbed and internalized (Yoon et al., 2017). Gold nanoparticles offer less stability and are less liable for targeted delivery. Superparamagnetic iron oxide nanoparticles are used as contrast agents in CT scans, but sometimes they are misleading because it is difficult to identify them with other dark areas. They possess a very high magnetic moment which can result in a blooming effect which can make the image of organs cloudy due to overestimation of the size of the labeled tissues (Moorcroft et al., 2018). Inorganic nanoparticles are being used as delivery vehicles for antibiotics. They can be easily incorporated for easy drug delivery and increased colloidal stability with better biocompatibility and offer high drug loading capacity. The inorganic nanoparticles which are optically active can efficiently convert light energy to heat energy which helps in the liberation of encapsulated antibiotics (Kim et al., 2018). They find extensive applications as probes in biomedical imaging in vivo. Inorganic nanoparticles are also used as a better alternative for organic dyes in optical imaging (Li et al., 2011).
Amikacin inorganic nanoparticles can be used in functional devices because there is a vast area open to exploit their collective properties. Doping of inorganic nanoparticles into the polymer matrix is used to engineer the flexible hybrid nanocomposites with outstanding magnetic, optical, and electric properties. Self-assembly of symmetric deblock copolymers was used in addition to gold nanoparticles into an amikacin emulsion formulation so that a neutral interface can be generated to form hybrid nanoparticles. A mixture of cetyltrimethylammonium bromide and polyvinyl alcohol was used to adapt the properties of emulsion droplets. This technique used the contributions of entropy and enthalpy to control the alignment and location of nanoparticles. Both the terminals of the nanoparticles can be well optimized with the help of this method which can give a universal route for the designing of well-defined nanomaterials (Yan et al., 2017). To control the properties of bulk at the molecular level the control in shape and size of inorganic nanocarriers is of utmost importance. Cyclophosphamide self-assembled organometallic spheres were used as endo-templates for the preparation of monodisperse nanoparticles of silica. Tetramethoxysilane was condensed to confirm the self-assembly of spheres and a DMSO-d solution was used to dilute it and kept for 4 days. The solution remained clear which indicated the condensation of spheres. The self-assembled silica nanoparticles were successfully developed with the help of this technique with a well-defined framework of the shell. Nanoparticles of size less than 10 nm can also be prepared with this technique. This can be used to exploit the new bulk properties (Suzuki et al., 2010).
The most common form of dementia is Alzheimer’s disease which affects more than 24 million people across the world. With the aid of hybrid technology of inorganic-organic compositions having specific properties, the conditions of Alzheimer’s disease are treated. This hybrid system was integrated with a combination of proteins and inorganic components. Polyoxometalates are a class of metal-oxygen-anion nanoclusters that can inhibit the aggregation of amyloid-β. These POM@P hybrids showed potent activity against the aggregation of A β1-40. These nanocarriers were also able to block cellular toxicity up to 46%. The cell survival rate was increased to 82%. The nanocarriers were nontoxic. The self-assembled POM-A β particles were used to target the peptide-CR (Congo red) hybrids having the ability to inhibit the target and real-time monitoring. These types of systems can be used for the development of targeted agents for the treatment of Alzheimer’s disease (Li et al., 2013). Nanotechnology has been expanded to exploit new avenues for the treatment, diagnosis, and prevention of cancers. In the process of self-assembly, the inorganic molecules are being developed into unforeseen structures. Carbon-based nanomaterials are used for controlled drug delivery. Fullerene, nanodiamonds, and graphene are produced on an industrial scale. Due to their outstanding chemical stability, they render themselves biologically inert. With the help of self-assembly, the bio-functional agents are being attached to the nanocarbon surface. They turn themselves into an effective adsorbent for the hydrophobic anticancer drugs. Methotrexate was co-delivered with a PEGylated self-assembled graphene nanocarrier that leads to hydrophobic and π−π stacking. This was a non-covalent drug-loading process. This type of mechanism can be further used to deliver anticancer drugs and their analogs (Li et al., 2018).
Micellar Systems
Self-assembled micelle nanoparticles comprised of thermo-sensitive polyion complex and chitosan-g-poly (N-isopropyl acrylamide) were developed to generate a sustained release dosage form. The micelle Np’s were formed as multilayer corona structures with the inner core being hydrophobic. The interaction between the drug and the micelle was modified with a change in temperature and pH for a sustained release formulation of DOX. The micelle prepared were having good stability with high drug loading capacity and an easy method of preparation. Due to the variable value of the degree of ionization of polyacrylic acid, the nanoparticles formed had a variable size in different pH conditions. The particle size was minimum at pH 4.5 to 6.0 and maximum or larger size in a more basic and acidic medium (Li et al., 2011). Nowadays amphiphilic copolymeric cations are being employed as an alternative for nucleic acid delivery. The PEGylated derivatives offer good gene expression to be used in various cancer therapies. A tri-copolymer having an amphiphilic moiety of ethylene glycol, polycaprolactone, and a cationic block was used to prepare self-assembled micelle nanoparticles in an aqueous solution to deliver the siRNA. The choice of excipients was determined to create a hydrophobic interaction and micelle core formation so that its stability can be increased. This unique combination allowed the loading of siRNA after the formation of nanoparticles so that uniformity can be maintained. The complexes were able to deliver good compatibility with cells at higher concentrations too. The incorporation of the hydrophobic core with PEG was able to improve the stability of nanoparticles (Sun et al., 2008).
In the development of drug delivery systems that can be used for tumor targeting the polymeric nanoparticles, which are responsive toward stimuli, can be used to get improved efficacy and lower the side effects. Micelle self-assembled nanoparticles that have been developed in recent years are proved to be more biocompatible and responsive toward multiple stimuli. The researcher has used a derivative of phenylalanine to study its drug delivery capabilities. The amphiphilic copolymer synthesized was more biocompatible and water-soluble. It was able to show its activity at slightly higher temperatures. The prepared nanoparticles were used to encapsulate the hydrophobic drug doxorubicin with a higher loading capacity. The drug was effective at a lower IC50 value for cancerous cells as compared to healthy cells (Biswas et al., 2021). The amphiphilic block copolymers can form a self-assembled nano-carrier system with a hydrophobic surrounding shell. In this study, PBLG-b-PEG block copolymer solution was used to show the effect on the self-assembly of the nanoparticles. Gold nanoparticles were incorporated with the micelle core. Standard NCA procedure was used. After the introduction of AuNPs, the cylindrical micelles were transformed into a spherical shape with a core surrounded by AuNPs. The morphological transformation was due to the breaking of ordered packing of PBLG rods (Cai et al., 2012). The group of scientists has reported the use of unimer polyion complexes for the delivery of siRNA into solid tumors. The developed micelles had greater potential of circulation in blood and higher localization at the tumor as compared to the control. The inhibition of tumor growth was also observed to be substantial due to higher internalization of siRNA upon intravenous administration in the murine model of a cervical tumor (Zheng et al., 2017). Further, a novel strategy for treating the peripheral vascular disease was proposed using polyion complex micelles. The scientists have found out that 100 nm micelles showed potentially better localization in the collateral arteries around macrophages. The results of the investigation illustrated that appropriately sized particles can be useful in improving targetability of micellar complexes for the management of angiogenic diseases (Suhara et al., 2018).
Niosomes
The drug delivery systems comprising cholesterol and non-ionic surfactants are called niosomes. They offer better encapsulation for drugs and they are biocompatible with low-immunogenicity. They also possess targeting and controlled release properties. Due to their ability to solubilize lipophilic compounds, they help in increasing the bioavailability of enzymes and natural products. In this study, non-ionic surfactants such as Tween 80 or Span 80 were used to develop the assemblies of niosomes by using purified water as the dispersion system. The incorporation of block copolymer alters the morphology of niosomes. In-vitro studies showed exceptionally low cytotoxicity. In-vivo studies showed no signs of hepatotoxicity (Pippa et al., 2019). The patients who are compromised immunologically are more prone to fungal infections. The study was aimed at developing a safer ophthalmic drug delivery system with increased retention time and permeability. The drug was incorporated into niosomal versicles and self-assembled NPs were prepared. Cyclodextrin and nanovesicles were combined to optimize the nanoformulation. To enhance the drug permeation in-situ gel was prepared so that its nasolacrimal drainage can be reduced and unwanted absorption in a system can be avoided. The particle size was maintained at 290 ± 35 nm. High entrapment efficiency was achieved, and corneal penetration was also enhanced. A high level of growth was inhibited for the Candida albicans (Elmotasem and Awad, 2020).
Metastatic breast cancer is a major cause of mortality and morbidity in women. A combination of treatments is used for the effective therapy of this type of cancer. Tamoxifen and doxorubicin are the anticancer categories of drugs that are used to treat cancer targeting the estrogen receptor selectively. Combination therapy helps to ensure long-term use of the drugs. Formulating the drug into niosomes helps to increase the entrapment efficiency and improve hydrophobic solubility. In this study, a single noisome was used to entrap both drugs. Box-Behnken experimental design was used to optimize the self-assembled niosomes. MCF-7 breast cancer cell line was also used to evaluate the in vitro characteristics. The drug-loaded noisome was able to exhibit good in vitro characteristics. The localization of the noisome was substantially improved as compared to the earlier studies. The distribution and external modification were found to be like previous studies (Kulkarni and Rawtani, 2019). Synthetic membrane vesicles are made with the help of nonionic surfactants by self-assembly. A combination of cholesterol, nonionic surfactant, and diacetyl phosphate is used for the preparation of a noisome. In this study, controlled self-assembly of niosomes was prepared as an alternative to liposomes. To reduce the size of niosomes, the flow rate ratio was increased which decreased the mixing time by diffusion. The microfluidic method was used to prepare nanoscale niosomes to improve their consistency and encapsulation efficiency. With the help of surfactants and using a shorter C12 alkyl chain more stable and smaller niosomes were prepared (Lo et al., 2010). Capecitabine is approved by the FDA to use in the treatment of various types of cancers. This study was aimed to develop drug-loaded niosomes so that their side effects and chemoresistance can be masked. The prepared self-assembled nanoniosomes were evaluated for their characteristics such as size analysis with help of SEM. Cytotoxicity was evaluated with the help of MCF7 and PANC1 cell lines. The formulation of drug-loaded self-assembled nanoniosomes increased the permeability and efficacy of the drug with reduced side effects. Reduced cytotoxicity was observed with formulated nanoniosomes than free drug (Nazari-Vanani et al., 2019).
Polymerosomes
Polymersomes are robust vesicle-shaped developed from amphiphilic block co-polymers (Jang et al., 2016) which can be employed for the delivery of both hydrophilic and hydrophobic molecules. In the last 2 decades, the polymerosomes have been exploited in the research field for performing their role in stabilization and sustained delivery of biological macromolecules (Dai et al., 2006; Jaganathan et al., 2014), flexibility for effective delivery of topical products (Rastogi et al., 2009), delivery viral of capsid mimics (Yang et al., 2009), response mediated drug delivery (Jang et al., 2016), targeting cancer therapeutics (Anajafi and Mallik, 2015), cosmeceuticals (Kaul et al., 2018), diagnostic probes (Leong et al., 2018), etc. Although, the formulations possess exceptional biocompatibility, they are also associated with limitations such as difficulty in achieving small sized particles (below 100 nm) (Cheng et al., 2009), low membrane permeability (Rideau et al., 2018), and tedious and lengthy time-consuming process to manufacture the functionalized initializing materials (Li et al., 2010).
Self-assembled polymersomes were reported to be formulated by solvent evaporation using poly(caprolactone)-poly (ethylene glycol)-poly(caprolactone) co-polymer to target melanomas and basal cell carcinomas. The size of vesicles was of 122 ± 20 nm and double-walled deformable constructions were confirmed by TEM depicting the wall thickness of 25 nm (Rastogi et al., 2009). A group of scientists has developed pH-sensitive degradable polymersomes encapsulated with paclitaxel and doxorubicin hydrochloride formulated using diblock copolymer of PEG and polycarbonates. Polymersomes of PEG(1.9k)-PTMBPEC(6k) were obtained to produce the vesicle sizes of 100–200 nm. The acetal hydrolysis resulted in the substantial rise in polymersomes size to larger than 1000 nm within a day at pH 4.0 (Chen et al., 2010). The self-immolative polymersomes (SIPsomes) can be used for delivery of macromolecules and modulating the design of terminal capping moieties. Visible light, UV light, and reductive milieu can be exploited to trigger SIPsomes for breakdown into water-soluble minor molecules and hydrophilic blocks. Stimuli-triggered disassembly of block copolymer vesicles or polymersomes has been conventionally achieved via solubility switching of the bilayer-forming block, requiring cooperative changes of most of the repeating units (Liu et al., 2014).
Solid Lipid Nanoparticles
The hot homogenization process and warm microemulsions were used to create solid lipid nanoparticles for the first time in the early 1990s. Since then, a lot of researchers working in this field have steadily increased and along with that the increase in patients was also observed on various techniques such as solvent emulsification as well as diffusion procedures were developed and filed (Manjunath et al., 2005; Gasco, 2007). Besides SLNs, nanostructured lipid carriers (NCLs) are a lipid mixture of solid with lipids and of liquid with lipids at 37°C temperature (Müller et al., 2002). Pharmaceutical uses of lipid nanocarriers include transport of a wide range of therapeutic active molecules along with distribution, from biotechnological drugs to tiny medication particles (Scioli Montoto et al., 2020). Gradually, the lipid-built nano assemblies have made substantial progress in the transport of DNA/RNA and drugs due to their low toxicity especially for in vivo targeting (Puri et al., 2009). As per Cullis and Hope (2017), the cationic lipids are amphiphilic molecules that self-assemble into higher-order aggregates in an aqueous solution and have a positively charged polar head group and a hydrophobic tail domain. They bind electrostatically with mRNA molecules’ negatively charged phosphate groups, allowing them to be entrapped in a lipid-based nanoparticle. Under the PEG-lipid coating, self-assemble lipid nanoparticles can be stabilized. They concluded that it will be a dominating non-viral method to enable the enormous potential of gene therapy due to robust and fast formulation procedures, as well as benefits in potency, payload, and design flexibility. Zhai et al. (2018) developed self-assembled lipid nanoparticles (SALNs) with paclitaxel to targeted aggressive ovarian cancer and improved the therapeutic effect by functionalizing lipid particles through epidermal growth factor receptor (EGFR) antibody fragments. Pluronic F127 and a lipid poly(ethylene glycol) stabilizer were used to stabilize the produced biocontinuous cubic- and sponge-phase lipid nanoparticles. The formulation demonstrated greater cytotoxicity in vitro against HEY ovarian cancer cell lines than a free drug formulation as well as in an ovarian cancer mouse xenograft model, aside from EGFR targeting, it lowers tumor growth by 50% compared to pure drug or nondrug control. SALNs averaging size of 180 nm was developed to enclose and stabilize with iron oxide particles non-covalently coated with heparin (SALNs-Fe@hepa) by Truzzi et al. that are highly stable and demonstrated more than 90% encapsulation efficiency for oral delivery. The product stabilized the heparin and did not show any cytotoxicity (Truzzi et al., 2017). SALNs were formulated by Gindy et al. (2014) using a rapid precipitation process and studied the SALNs for siRNA delivery. They identify essential physiochemical and kinetic factors influencing the formation of lipid nanoparticulate three-dimensional macromolecular structure as a biorelevant SAR feature by a mechanistic analysis of SALNs via a fast antisolvent precipitation method. The manufacturing method can be used for common production of fine-tuned SALNs. Pham et al. (2016) studied in situ self-assembly lipid nanoparticles of lopinavir and ritonavir loaded in self-assembly nanocapsules for pediatric drug delivery. Li et al. (2019) studied the SALNs method for delivering a cisplatin prodrug and siRNA targeting the xeroderma pigmentosum endonuclease group to fight against lung cancer drug resistance. The findings showed that using a multi-targeted lipid nanoparticle technology to silence an NER-related gene to increase cisplatin-induced apoptosis, especially in cisplatin-refractory cancers, is a viable option. Sagnella et al. (2011) concluded that the oral administration of SALNs containing 5-fluorouracil was found to be highly effective as a target-activated chemotherapeutic agent with significantly higher efficacy compared to the marketed non-self-assembling alternative.
Other Nanoparticles
To provide a high drug loading capacity this platform provides a particularly good alternative with the specificity of targeting tissues and cells. The structure is composed of three layers, the outermost being the lipid layer, the middle being the hydrophobic layer, and the inner core is made up of a hollow shell (Shi et al., 2011). The outer layer can be modified with PEG so that immunological recognition can be avoided, and it also adds to its stability in systemic circulation while controlling polymer degradation. The therapeutics which are hydrophobic can be incorporated in the middle layer. They are formulated by employing a double-emulsion solvent evaporation technique (Tambuwala et al., 2018). Peptide-based drug delivery system provides various advantages such as chemical diversity, high drug loading, and site-specific targeting for several diseases. Proteins possess α-helices and β sheets that act as naturally occurring self-assembling motifs to form nanostructures of customizable shapes. The functionalization of peptides can be easily operated by introducing the compounds into the structure of peptides. They can also be used to facilitate intracellular uptake and enhance biological functions. The self-assembled peptides can be formulated into hydrogels, vesicles, and nanotubes for facilitating drug delivery to specific cancerous cells (Fan et al., 2017). Calcium phosphate is found in tricalcium phosphates and hydroxyapatite having chemical similarity and are biocompatible. They also possess a variable density of surface charge and can facilitate the controlled release of medicaments. Calcium phosphate nanoparticles have good penetration ability through the cell membranes, so they are used for DNA transfection and protection of DNA from the lysosomal enzymes which are ultimately retained in the cell’s cytoplasm. Our bone and teeth are composed of calcium; this also adds to the biocompatibility of calcium phosphate nanoparticles. The structure is composed of a core with calcium phosphate which is followed by a layer of DNA and an additional shell of calcium phosphate. This tri-layer structure helps in protecting the DNA from the nuclease enzymes. They can be prepared by sol-gel method, co-precipitation, and microemulsion (Banik and Basu, 2014).
In the field of sensors, storage devices and catalysis graphene hold major potential. Due to its honeycomb structure, it has a wider surface area along with better chemical and mechanical stability which helps in using it for drug delivery and biosensing. They offer low cytotoxicity and good biocompatibility (Shen et al., 2012). Graphene has oxygen as functional groups due to which they can be easily processed, and they are suitable for research. The efficacy of graphene is more than other carbon nanoparticles and they are lipophilic, they form conjugates in aqueous solvent due to van der Waals interaction. They can be formulated with PEG to render their aqueous solubility (Gaur et al., 2021). Fullerenes are nanomolecular carbon cages that are used as a delivery agent for therapeutics and imaging agents. Its structure can be a hollow sphere and tubes. They are used as a vector where the bio-active moiety can be linked with the structure of fullerene. The self-assembled structure of fullerene can be synthesized in the range of 100–200 nm (Li et al., 2015). Nucleic acid holds amino moiety and carbonyl on the surface of its nucleoside chain due to which they are used in nano-formulation as a biomaterial. This property also helps in its coordination with the metal ions. They can be used to form more stable and adaptable metal nanoparticles. They are employed in cellular imaging and drug delivery. With the help of electrostatic interaction, nucleic acid can retard the coagulation of nanoparticles because they gather around the metal ion (Hayes et al., 2006). Table 1 summarizes the application of various self-assembled nanocarriers as a drug delivery system.
Clinical Applications of Self-Assembled Nanoparticles
The development of self-assembled nanoparticles has been focused to improve upon the limitations of the traditional drug delivery systems. In order to demonstrate the clinical viability, these systems are generally used to impart controlled release, enhancing systemic availability, longer duration of action, localized delivery, etc., should be biocompatible, biodegradable, and non-toxic (Verma and Garg, 2001; Myrick et al., 2014; López-Sagaseta et al., 2016). The polymers used for the development of self-assembled systems can be used for sustained drug release owing to their reversible non-covalent bonds and polymerization-depolymerization nature (Davis et al., 2010). Table 2 illustrates some of the self-assembled products under clinical trial or FDA approval. NK105, a self-assembled micelle carrier of paclitaxel for the treatment of metastatic or recurrent breast cancer was reported in a phase III trial. The formulation was examined in murine model which showed passive targeting by accumulation in the tumor. The efficacy with safety of NK105 was comparable to pure paclitaxel, while slightly better performance with regard to avoidance of peripheral sensory neuropathy (Saeki et al., 2017). Matsumura et al. (2004) reported phase I trial of NK911 polymeric micelle nanocarrier incorporated with doxorubicin for treatment of pancreatic cancer. The major hematological toxicity was neutropenia, and this trial estimated the recommended dose and maximum tolerable dose as 50 mg/m2 and 67 mg/m2, respectively. NK911 is currently under phase II trial at the National Cancer Centre Hospital, Japan.
CPX-351 is a self-assembled liposomal carrier incorporated with a 5:1 ratio of cytarabine and daunorubicin for the treatment of acute myeloid leukemia. The product received FDA approval in 2017 due to significant improvements in therapeutic outcomes in the older individuals. The phase III trial showed higher overall survival and remission rate in the patients; however, the safety profile of the product was similar to conventional therapy (Lancet et al., 2018). Arikayce™ is another FDA approved liposomal formulation containing amikacin which was designed for the management of patients with severe cystic fibrosis infected with Pseudomonas aeruginosa for a long duration. It showed good tolerability, safety, and efficacy during the clinical studies in phase III (Clancy et al., 2013; Beltrán-Gracia et al., 2019; Khan and Chaudary, 2020). Adynovate®, a PEG-based micellar carrier system containing recombinant factor VIII was approved by the FDA in 2015 to be used in adult patients with hemophilia A. The product showed high pre-, intra-, and post-operative efficacy in previously treated patients with severe hemophilia A (Brand et al., 2016). Adynovate® has received approval from the U.S. FDA in June 2021 for the treatment and control of bleeding episodes in adolescents.
A spacer implantation (SpaceOAR®) to increase the perirectal spacing for lowering the need of high radiation therapy for prostate cancer patients is used. The safety of the spacer and its impact of rectal radiation therapy was evaluated by employing CT and MRI scan imaging controlled chemotherapeutic irradiation. The spacer was well tolerated by the patients with the decreased rectal toxicity and reduced number of patients facing problems with bowel quality (Mariados et al., 2015) Radiesse® was reported to be effective in reduction of facial folds and wrinkles by enhancing the production of collagen. It is prepared as a self-assembled hydrogel formulation incorporated with calcium hydroxylapatite filler (Gubanova and Starovatova, 2015; Mandal et al., 2020). ImDendrim is a self-assembled poly-L-lysine dendrimer complexed with 188Rhenium-ligand (nitro-imidazole-methyl-1,2,3-triazol-methyl-di-[2-pycolyl] amine) which was developed for effective treatment of untreatable solid tumors. The product displayed no complications after administration and patients responded well with the therapy. Overall, the initial outcomes indicated toward safety and effectiveness of InDendrim, which will be further assessed in undergoing clinical studies (Belhadj-Tahar et al., 2018). Starpharma developed two new PEGylated dendrimers of docetaxel (DEP) and cabazitaxel for improving or at least maintaining cytotoxic action of the drugs, along with avoiding chances of serious adverse effects of these drugs. DEP® docetaxel and DEP® cabazitaxel were tolerated well in the patients with breast, pancreatic, and prostate cancer with high survival rate in comparison with conventional anticancer therapies using taxane drugs. DEP® docetaxel is currently in the phase II clinical trial, while DEP® cabazitaxel is in a phase I/II adaptive trial (Kelly et al., 2020).
Conclusion and Future Perspectives
In recent times, self-assembled nanocarriers have emerged as a potential tool to resolve the drug delivery issues and to facilitate targeting of drug to particular cells or tissues. The objective of the review is to present the concise outlook over applications of various self-assembled nanoparticles as a drug delivery system in the treatment of some of the serious diseases. Recent investigations based on self-assembled polymeric nanoparticles were shown to increase anti-inflammatory activity, antitumor effect, biocompatibility, reducing side effects, etc. of some of the potent therapeutic agents. The preparation of liposomes also contributed to achieving high drug entrapment, stimuli-responsive release, dual targeting, bioavailability enhancement, improvement in drug uptake, etc. Further, inorganic nanocarriers, solid lipid nanoparticles, polymerosomes, micellar systems, and other nanoparticles were also summarized in the review to show augmentation of therapeutic benefits of the drugs. At the end, the self-assembled products under clinical trial and FDA-approved were added to attest to the clinical benefits of these systems for the treatment of several disorders.
The outcomes of the various studies showed potential of self-assembled systems in improving the delivery characteristics of drugs, biologicals, diagnostics, etc. The localization and targetability of these systems can be further verified at the clinical stages. These novel systems may be used individually or in hybrid mode for the management of rare diseases. The biocompatibility, biodegradability, and flexibility of self-assembled systems can also be exploited for the safe and effective delivery of challenging drug molecules. There has been a significant increase in the number of biological license applications for the treatment of chronic and serious diseases which can be incorporated in self-assembled carriers. Several research investigations which are currently at the preclinical stage have shown a lot of promise, but more pertinent explorations are still needed to establish clinical benefits of these systems.
Author Contributions
HP, AP, and BP were involved in the writing of this review. All the authors made significant contributions and approve the submitted version.
Conflict of Interest
The authors declare that the research was conducted in the absence of any commercial or financial relationships that could be construed as a potential conflict of interest.
Publisher’s Note
All claims expressed in this article are solely those of the authors and do not necessarily represent those of their affiliated organizations, or those of the publisher, the editors, and the reviewers. Any product that may be evaluated in this article, or claim that may be made by its manufacturer, is not guaranteed or endorsed by the publisher.
References
Anajafi, T., and Mallik, S. (2015). Polymersome-based Drug-Delivery Strategies for Cancer Therapeutics. Ther. Deliv. 6 (4), 521–534. doi:10.4155/tde.14.125
Anselmo, A. C., and Mitragotri, S. (2015). A Review of Clinical Translation of Inorganic Nanoparticles. Aaps J. 17 (5), 1041–1054. doi:10.1208/s12248-015-9780-2
Banik, M., and Basu, T. (2014). Calcium Phosphate Nanoparticles: a Study of Their Synthesis, Characterization and Mode of Interaction with salmon Testis DNA. Dalton Trans. 43 (8), 3244–3259. doi:10.1039/C3DT52522H
Belhadj-Tahar, H., Chen, A., Jia, Y., Wu, S., Sadeg, N., Zhao, H., et al. (2018). In Situ Anti-Cancer Agent Derived from [188re]rhenium nitro-imidazole Ligand Loaded Poly-L-Lysine Dendrimer Administrated by Direct CT Guided Stereotactic Intrahepatic Injection. Jco 36 (15_Suppl. l), e15569. doi:10.1200/JCO.2018.36.15_suppl.e15569
Beltrán-Gracia, E., López-Camacho, A., Higuera-Ciapara, I., Velázquez-Fernández, J. B., and Vallejo-Cardona, A. A. (2019). Nanomedicine Review: Clinical Developments in Liposomal Applications. Cancer Nano 10 (1), 11. doi:10.1186/s12645-019-0055-y
Biswas, G., Jena, B. C., Samanta, P., Mandal, M., Dhara, D., and Part, A. (2021). Synthesis, Self-Assembly and Drug Release Study of a New Dual-Responsive Biocompatible Block Copolymer Containing Phenylalanine Derivative. J. Macromol. Sci. A 58 (11), 792–803. doi:10.1080/10601325.2021.1947748
Brand, B., Gruppo, R., Wynn, T. T., Griskevicius, L., Lopez Fernandez, M. F., Chapman, M., et al. (2016). Efficacy and Safety of Pegylated Full‐length Recombinant Factor VIII with Extended Half‐life for Perioperative Haemostasis in Haemophilia A Patients. Haemophilia 22 (4), e251–258. doi:10.1111/hae.12963
Cai, C., Wang, L., Lin, J., and Zhang, X. (2012). Morphology Transformation of Hybrid Micelles Self-Assembled from Rod-Coil Block Copolymer and Nanoparticles. Langmuir 28 (9), 4515–4524. doi:10.1021/la204941w
Chen, W., Meng, F., Cheng, R., and Zhong, Z. (2010). pH-Sensitive Degradable Polymersomes for Triggered Release of Anticancer Drugs: a Comparative Study with Micelles. J. Controlled Release 142 (1), 40–46. doi:10.1016/j.jconrel.2009.09.023
Chen, H., Pan, H., Li, P., Wang, H., Wang, X., Pan, W., et al. (2016). The Potential Use of Novel Chitosan-Coated Deformable Liposomes in an Ocular Drug Delivery System. Colloids Surf. B: Biointerfaces 143, 455–462. doi:10.1016/j.colsurfb.2016.03.061
Chen, J., Pan, H., Yang, Y., Xiong, S., Duan, H., Yang, X., et al. (2018). Self-assembled Liposome from Multi-Layered Fibrous Mucoadhesive Membrane for Buccal Delivery of Drugs Having High First-Pass Metabolism. Int. J. Pharmaceutics 547 (1), 303–314. doi:10.1016/j.ijpharm.2018.05.062
Chen, J., Duan, H., Pan, H., Yang, X., and Pan, W. (2019). Two Types of Core/shell Fibers Based on Carboxymethyl Chitosan and Sodium Carboxymethyl Cellulose with Self-Assembled Liposome for Buccal Delivery of Carvedilol across TR146 Cell Culture and Porcine Buccal Mucosa. Int. J. Biol. Macromol. 128, 700–709. doi:10.1016/j.ijbiomac.2019.01.143
Cheng, Z., Thorek, D. L. J., and Tsourkas, A. (2009). Porous Polymersomes with Encapsulated Gd-Labeled Dendrimers as Highly Efficient MRI Contrast Agents. Adv. Funct. Mater. 19 (23), 3753–3759. doi:10.1002/adfm.200901253
Clancy, J. P., Dupont, L., Konstan, M. W., Billings, J., Fustik, S., Goss, C. H., et al. (2013). Phase II Studies of Nebulised Arikace in CF Patients withPseudomonas Aeruginosainfection. Thorax 68 (9), 818–825. doi:10.1136/thoraxjnl-2012-202230
Costantino, L., and Boraschi, D. (2012). Is There a Clinical Future for Polymeric Nanoparticles as Brain-Targeting Drug Delivery Agents? Drug Discov. Today 17 (7-8), 367–378. doi:10.1016/j.drudis.2011.10.028
Cullis, P. R., and Hope, M. J. (2017). Lipid Nanoparticle Systems for Enabling Gene Therapies. Mol. Ther. 25 (7), 1467–1475. doi:10.1016/j.ymthe.2017.03.013
Dai, C., Wang, B., Zhao, H., Li, B., and Wang, J. (2006). Preparation and Characterization of Liposomes-In-Alginate (LIA) for Protein Delivery System. Colloids Surf. B: Biointerfaces 47 (2), 205–210. doi:10.1016/j.colsurfb.2005.07.013
Davis, M. E., Zuckerman, J. E., Choi, C. H. J., Seligson, D., Tolcher, A., Alabi, C. A., et al. (2010). Evidence of RNAi in Humans from Systemically Administered siRNA via Targeted Nanoparticles. Nature 464 (7291), 1067–1070. doi:10.1038/nature08956
Desai, P., Venkataramanan, A., Schneider, R., Jaiswal, M. K., Carrow, J. K., Purwada, A., et al. (2018). Self‐assembled, Ellipsoidal Polymeric Nanoparticles for Intracellular Delivery of Therapeutics. J. Biomed. Mater. Res. 106 (7), 2048–2058. doi:10.1002/jbm.a.36400
Elahi, N., Kamali, M., and Baghersad, M. H. (2018). Recent Biomedical Applications of Gold Nanoparticles: A Review. Talanta 184, 537–556. doi:10.1016/j.talanta.2018.02.088
Elmotasem, H., and Awad, G. E. A. (2020). A Stepwise Optimization Strategy to Formulate In Situ Gelling Formulations Comprising Fluconazole-Hydroxypropyl-Beta-Cyclodextrin Complex Loaded Niosomal Vesicles and Eudragit Nanoparticles for Enhanced Antifungal Activity and Prolonged Ocular Delivery. Asian J. Pharm. Sci. 15 (5), 617–636. doi:10.1016/j.ajps.2019.09.003
Fan, T., Yu, X., Shen, B., and Sun, L. (2017). Peptide Self-Assembled Nanostructures for Drug Delivery Applications. J. Nanomater. 2017, 1–16. doi:10.1155/2017/4562474
Fendler, J. H. (1996). Self-Assembled Nanostructured Materials. Chem. Mater. 8 (8), 1616–1624. doi:10.1021/cm960116n
Gasco, M. (2007). Lipid Nanoparticles: Perspectives and Challenges☆. Adv. Drug Deliv. Rev. 59 (6), 377–378. doi:10.1016/j.addr.2007.05.004
Gaur, M., Misra, C., Yadav, A. B., Swaroop, S., Maolmhuaidh, F. Ó., Bechelany, M., et al. (2021). Biomedical Applications of Carbon Nanomaterials: Fullerenes, Quantum Dots, Nanotubes, Nanofibers, and Graphene. Materials 14 (20), 5978. doi:10.3390/ma14205978
Gindy, M. E., DiFelice, K., Kumar, V., Prud’homme, R. K., Celano, R., Haas, R. M., et al. (2014). Mechanism of Macromolecular Structure Evolution in Self-Assembled Lipid Nanoparticles for siRNA Delivery. Langmuir 30 (16), 4613–4622. doi:10.1021/la500630h
Gubanova, E. I., Starovatova, P. A., and Rodina, M. Y. (2015). 12-Month Effects of Stabilized Hyaluronic Acid Gel Compared With Saline for Rejuvenation of Aging Hands. J. Drugs Dermatol. 14 (3), 288–298.
Hayes, M. E., Drummond, D. C., Kirpotin, D. B., Zheng, W. W., Noble, C. O., Park, J. W., et al. (2006). Genospheres: Self-Assembling Nucleic Acid-Lipid Nanoparticles Suitable for Targeted Gene Delivery. Gene Ther. 13 (7), 646–651. doi:10.1038/sj.gt.3302699
Henriksen-Lacey, M., Carregal-Romero, S., and Liz-Marzán, L. M. (2017). Current Challenges toward In Vitro Cellular Validation of Inorganic Nanoparticles. Bioconjug. Chem. 28 (1), 212–221. doi:10.1021/acs.bioconjchem.6b00514
Hudan-Tsilo, I., Tokarskyy, O., Shevchuk, O., and Korda, M. (2021). Chitosan Self-Assembled Polymeric Nanoparticles for Percutaneous Delivery of Betamethasone in Contact Dermatitis. Drug Dev. Ind. Pharm., 1–8. doi:10.1080/03639045.2021.1989457
Jaganathan, M., Madhumitha, D., and Dhathathreyan, A. (2014). Protein Microcapsules: Preparation and Applications. Adv. Colloid Interf. Sci. 209, 1–7. doi:10.1016/j.cis.2013.12.004
Jang, W.-S., Park, S. C., Reed, E. H., Dooley, K. P., Wheeler, S. F., Lee, D., et al. (2016). Enzymatically Triggered Rupture of Polymersomes. Soft matter 12 (4), 1014–1020. doi:10.1039/c5sm01881a
Liu, K., Li, H., Williams, G. R., Wu, J., and Zhu, L.-M. (2018). pH-Responsive Liposomes Self-Assembled from Electrosprayed Microparticles, and Their Drug Release Properties. Colloids Surf. A: Physicochem. Eng. Aspects 537, 20–27. doi:10.1016/j.colsurfa.2017.09.046
Kaul, S., Gulati, N., Verma, D., Mukherjee, S., and Nagaich, U. (2018). Role of Nanotechnology in Cosmeceuticals: A Review of Recent Advances. J. pharmaceutics 2018, 1–19. doi:10.1155/2018/3420204
Liu, K.-F., Liu, Y.-X., Li, C.-X., Wang, L.-Y., Liu, J., and Lei, J.-D. (2018). Self-Assembled pH and Redox Dual Responsive Carboxymethylcellulose-Based Polymeric Nanoparticles for Efficient Anticancer Drug Codelivery. ACS Biomater. Sci. Eng. 4 (12), 4200–4207. doi:10.1021/acsbiomaterials.8b00920
Kelly, B. D., McLeod, V., Walker, R., Schreuders, J., Jackson, S., Giannis, M., et al. (2020). Abstract 1716: Anticancer Activity of the Taxane Nanoparticles, DEP®docetaxel and DEP®cabazitaxel. Cancer Res. 80, 1716. doi:10.1158/1538-7445.AM2020-1716
Khan, O., and Chaudary, N. (2020). The Use of Amikacin Liposome Inhalation Suspension (Arikayce) in the Treatment of Refractory Nontuberculous Mycobacterial Lung Disease in Adults. Dddt 14, 2287–2294. doi:10.2147/dddt.S146111
Kim, K.-R., Kim, H. Y., Lee, Y.-D., Ha, J. S., Kang, J. H., Jeong, H., et al. (2016). Self-assembled Mirror DNA Nanostructures for Tumor-specific Delivery of Anticancer Drugs. J. Controlled Release 243, 121–131. doi:10.1016/j.jconrel.2016.10.015
Kim, D., Kim, J., Park, Y. I., Lee, N., and Hyeon, T. (2018). Recent Development of Inorganic Nanoparticles for Biomedical Imaging. ACS Cent. Sci. 4 (3), 324–336. doi:10.1021/acscentsci.7b00574
Kim, B. S., Chuanoi, S., Suma, T., Anraku, Y., Hayashi, K., Naito, M., et al. (2019). Self-Assembly of siRNA/PEG-B-Catiomer at Integer Molar Ratio into 100 Nm-Sized Vesicular Polyion Complexes (siRNAsomes) for RNAi and Codelivery of Cargo Macromolecules. J. Am. Chem. Soc. 141 (8), 3699–3709. doi:10.1021/jacs.8b13641
Kingsley, J. D., Dou, H., Morehead, J., Rabinow, B., Gendelman, H. E., and Destache, C. J. (2006). Nanotechnology: a Focus on Nanoparticles as a Drug Delivery System. Jrnl Neuroimm. Pharm. 1 (3), 340–350. doi:10.1007/s11481-006-9032-4
Kulkarni, P., and Rawtani, D. (2019). Application of Box-Behnken Design in the Preparation, Optimization, and In Vitro Evaluation of Self-Assembly-Based Tamoxifen- and Doxorubicin-Loaded and Dual Drug-Loaded Niosomes for Combinatorial Breast Cancer Treatment. J. Pharm. Sci. 108 (8), 2643–2653. doi:10.1016/j.xphs.2019.03.020
Kwon, S., Park, J. H., Chung, H., Kwon, I. C., Jeong, S. Y., and Kim, I.-S. (2003). Physicochemical Characteristics of Self-Assembled Nanoparticles Based on Glycol Chitosan Bearing 5β-Cholanic Acid. Langmuir 19 (24), 10188–10193. doi:10.1021/la0350608
Lancet, J. E., Uy, G. L., Cortes, J. E., Newell, L. F., Lin, T. L., Ritchie, E. K., et al. (2018). CPX-351 (Cytarabine and Daunorubicin) Liposome for Injection versus Conventional Cytarabine Plus Daunorubicin in Older Patients with Newly Diagnosed Secondary Acute Myeloid Leukemia. Jco 36 (26), 2684–2692. doi:10.1200/jco.2017.77.6112
Leong, J., Teo, J. Y., Aakalu, V. K., Yang, Y. Y., and Kong, H. (2018). Engineering Polymersomes for Diagnostics and Therapy. Adv. Healthc. Mater. 7 (8), 1701276. doi:10.1002/adhm.201701276
Li, F., de Wolf, F. A., Marcelis, A. T. M., Sudhölter, E. J. R., Cohen Stuart, M. A., and Leermakers, F. A. M. (2010). Triggered Templated Assembly of Protein Polymersomes. Angew. Chem. Int. Ed. 49 (51), 9947–9950. doi:10.1002/anie.201004003
Li, G., Guo, L., Meng, Y., and Zhang, T. (2011). Self-assembled Nanoparticles from Thermo-Sensitive Polyion Complex Micelles for Controlled Drug Release. Chem. Eng. J. 174 (1), 199–205. doi:10.1016/j.cej.2011.08.079
Li, M., Xu, C., Wu, L., Ren, J., Wang, E., and Qu, X. (2013). Self-Assembled Peptide-Polyoxometalate Hybrid Nanospheres: Two in One Enhances Targeted Inhibition of Amyloid β-Peptide Aggregation Associated with Alzheimer's Disease. Small 9 (20), 3455–3461. doi:10.1002/smll.201202612
Li, N., Li, N., Yi, Q., Luo, K., Guo, C., Pan, D., et al. (2014). Amphiphilic Peptide Dendritic Copolymer-Doxorubicin Nanoscale Conjugate Self-Assembled to Enzyme-Responsive Anti-cancer Agent. Biomaterials 35 (35), 9529–9545. doi:10.1016/j.biomaterials.2014.07.059
Li, F., Yager, K. G., Dawson, N. M., Jiang, Y.-B., Malloy, K. J., and Qin, Y. (2015). Nano-structuring Polymer/fullerene Composites through the Interplay of Conjugated Polymer Crystallization, Block Copolymer Self-Assembly and Complementary Hydrogen Bonding Interactions. Polym. Chem. 6 (5), 721–731. doi:10.1039/C4PY00934G
Li, M., Luo, Z., and Zhao, Y. (2018). Self-assembled Hybrid Nanostructures: Versatile Multifunctional Nanoplatforms for Cancer Diagnosis and Therapy. Chem. Mater. 30 (1), 25–53. doi:10.1021/acs.chemmater.7b03924
Li, C., Li, T., Huang, L., Yang, M., and Zhu, G. (2019). Self‐assembled Lipid Nanoparticles for Ratiometric Codelivery of Cisplatin and siRNA Targeting XPF to Combat Drug Resistance in Lung Cancer. Chem. Asian J. 14 (9), 1570–1576. doi:10.1002/asia.201900005
Liu, N., Hao, Y., Yin, Z., Ma, M., Wang, L., and Zhang, X. (2012). Self-assembled Human Serum Albumin-Coated Complexes for Gene Delivery with Improved Transfection. Pharmazie 67 (2), 174–181.
Liu, G., Wang, X., Hu, J., Zhang, G., and Liu, S. (2014). Self-Immolative Polymersomes for High-Efficiency Triggered Release and Programmed Enzymatic Reactions. J. Am. Chem. Soc. 136 (20), 7492–7497. doi:10.1021/ja5030832
Liu, W.-T. (2006). Nanoparticles and Their Biological and Environmental Applications. J. Biosci. Bioeng. 102 (1), 1–7. doi:10.1263/jbb.102.1
Lo, C. T., Jahn, A., Locascio, L. E., and Vreeland, W. N. (2010). Controlled Self-Assembly of Monodisperse Niosomes by Microfluidic Hydrodynamic Focusing. Langmuir 26 (11), 8559–8566. doi:10.1021/la904616s
López-Sagaseta, J., Malito, E., Rappuoli, R., and Bottomley, M. J. (2016). Self-assembling Protein Nanoparticles in the Design of Vaccines. Comput. Struct. Biotechnol. J. 14, 58–68. doi:10.1016/j.csbj.2015.11.001
Lv, Y., Xu, C., Zhao, X., Lin, C., Yang, X., Xin, X., et al. (2018). Nanoplatform Assembled from a CD44-Targeted Prodrug and Smart Liposomes for Dual Targeting of Tumor Microenvironment and Cancer Cells. ACS Nano 12 (2), 1519–1536. doi:10.1021/acsnano.7b08051
Mandal, A., Clegg, J. R., Anselmo, A. C., and Mitragotri, S. (2020). Hydrogels in the clinic. Bioeng. Transl. Med. 5, e10158. doi:10.1002/btm2.10158
Manjunath, K., Reddy, J. S., and Venkateswarlu, V. (2005). Solid Lipid Nanoparticles as Drug Delivery Systems. Methods Find Exp. Clin. Pharmacol. 27 (2), 127–144. doi:10.1358/mf.2005.27.2.876286
Mariados, N., Sylvester, J., Shah, D., Karsh, L., Hudes, R., Beyer, D., et al. (2015). Hydrogel Spacer Prospective Multicenter Randomized Controlled Pivotal Trial: Dosimetric and Clinical Effects of Perirectal Spacer Application in Men Undergoing Prostate Image Guided Intensity Modulated Radiation Therapy. Int. J. Radiat. Oncol. Biol. Phys. 92 (5), 971–977. doi:10.1016/j.ijrobp.2015.04.030
Matsumura, Y., Hamaguchi, T., Ura, T., Muro, K., Yamada, Y., Shimada, Y., et al. (2004). Phase I Clinical Trial and Pharmacokinetic Evaluation of NK911, a Micelle-Encapsulated Doxorubicin. Br. J. Cancer 91 (10), 1775–1781. doi:10.1038/sj.bjc.6602204
Moorcroft, S. C. T., Jayne, D. G., Evans, S. D., and Ong, Z. Y. (2018). Stimuli‐Responsive Release of Antimicrobials Using Hybrid Inorganic Nanoparticle‐Associated Drug‐Delivery Systems. Macromol. Biosci. 18 (12), 1800207. doi:10.1002/mabi.201800207
Müller, R. H., Radtke, M., and Wissing, S. A. (2002). Solid Lipid Nanoparticles (SLN) and Nanostructured Lipid Carriers (NLC) in Cosmetic and Dermatological Preparations. Adv. Drug Deliv. Rev. 54 (Suppl. 1), S131–S155. doi:10.1016/s0169-409x(02)00118-7
Myrick, J. M., Vendra, V. K., and Krishnan, S. (2014). Self-assembled Polysaccharide Nanostructures for Controlled-Release Applications. J. Nanotechnol. Rev. 3 (4), 319–346. doi:10.1515/ntrev-2012-0050
Na, K., Bum Lee, T., Park, K.-H., Shin, E.-K., Lee, Y.-B., and Choi, H.-K. (2003). Self-assembled Nanoparticles of Hydrophobically-Modified Polysaccharide Bearing Vitamin H as a Targeted Anti-cancer Drug Delivery System. Eur. J. Pharm. Sci. 18 (2), 165–173. doi:10.1016/s0928-0987(02)00257-9
Nazari-Vanani, R., Karimian, K., Azarpira, N., and Heli, H. (2019). Capecitabine-loaded Nanoniosomes and Evaluation of Anticancer Efficacy. Artif. Cell Nanomed. Biotechnol. 47 (1), 420–426. doi:10.1080/21691401.2018.1559179
Ngweniform, P., Li, D., and Mao, C. (2009). Self-Assembly of Drug-Loaded Liposomes on Genetically Engineered Protein Nanotubes: a Potential Anti-Cancer Drug Delivery Vector. Soft Matter 5 (5), 954–956. doi:10.1039/B817863A
Nie, Z., Petukhova, A., and Kumacheva, E. (2010). Properties and Emerging Applications of Self-Assembled Structures Made from Inorganic Nanoparticles. Nat. Nanotech 5 (1), 15–25. doi:10.1038/nnano.2009.453
Osorno, L., Brandley, A., Maldonado, D., Yiantsos, A., Mosley, R., and Byrne, M. (2021). Review of Contemporary Self-Assembled Systems for the Controlled Delivery of Therapeutics in Medicine. Nanomaterials 11 (2), 278. doi:10.3390/nano11020278
Pham, K., Li, D., Guo, S., Penzak, S., and Dong, X. (2016). Development and In Vivo Evaluation of Child-Friendly Lopinavir/ritonavir Pediatric Granules Utilizing Novel In Situ Self-Assembly Nanoparticles. J. Controlled Release 226, 88–97. doi:10.1016/j.jconrel.2016.02.001
Pippa, N., Pispas, S., and Demetzos, C. (2016). Polymer Self-Assembled Nanostructures as Innovative Drug Nanocarrier Platforms. Cpd 22 (19), 2788–2795. doi:10.2174/1381612822666160217141232
Pippa, N., Naziris, N., Stellas, D., Massala, C., Zouliati, K., Pispas, S., et al. (2019). PEO-b-PCL Grafted Niosomes: The Cooperativilty of Amphiphilic Components and Their Properties In Vitro and In Vivo. Colloids Surf. B: Biointerfaces 177, 338–345. doi:10.1016/j.colsurfb.2019.01.036
Puri, A., Loomis, K., Smith, B., Lee, J.-H., Yavlovich, A., Heldman, E., et al. (2009). Lipid-based Nanoparticles as Pharmaceutical Drug Carriers: from Concepts to Clinic. Crit. Rev. Ther. Drug Carrier Syst. 26 (6), 523–580. doi:10.1615/critrevtherdrugcarriersyst.v26.i6.10
Raj, S., Sumod, U., Jose, S., and Sabitha, M. (2012). Nanotechnology in Cosmetics: Opportunities and Challenges. J. Pharm. Bioall Sci. 4 (3), 186–193. doi:10.4103/0975-7406.99016
Ranson, M., Howell, A., Cheeseman, S., and Margison, J. (1996). Liposomal Drug Delivery. Cancer Treat. Rev. 22 (5), 365–379. doi:10.1016/s0305-7372(96)90009-2
Rastogi, R., Anand, S., and Koul, V. (2009). Flexible Polymerosomes-An Alternative Vehicle for Topical Delivery. Colloids Surf. B: Biointerfaces 72 (1), 161–166. doi:10.1016/j.colsurfb.2009.03.022
Rideau, E., Dimova, R., Schwille, P., Wurm, F. R., and Landfester, K. (2018). Liposomes and Polymersomes: a Comparative Review towards Cell Mimicking. Chem. Soc. Rev. 47 (23), 8572–8610. doi:10.1039/C8CS00162F
Sadasivam, M., Avci, P., Gupta, G. K., Lakshmanan, S., Chandran, R., Huang, Y.-Y., et al. (2013). Self-assembled Liposomal Nanoparticles in Photodynamic Therapy. Eur. J. Nanomed. 5 (3), 115–129. doi:10.1515/ejnm-2013-001010.1515/ejnm-2013-0010
Saeki, T., Mukai, H., Ro, J., Lin, Y.-C., Fujiwara, Y., Nagai, S., et al. (2017). A Global Phase III Clinical Study Comparing NK105 and Paclitaxel in Metastatic or Recurrent Breast Cancer Patients. Ann. Oncol. 28, v80–v81. doi:10.1093/annonc/mdx365.013
Sagnella, S. M., Gong, X., Moghaddam, M. J., Conn, C. E., Kimpton, K., Waddington, L. J., et al. (2011). Nanostructured Nanoparticles of Self-Assembled Lipid Pro-drugs as a Route to Improved Chemotherapeutic Agents. Nanoscale 3 (3), 919–924. doi:10.1039/c0nr00781a
Scioli Montoto, S., Muraca, G., and Ruiz, M. E. (2020). Solid Lipid Nanoparticles for Drug Delivery: Pharmacological and Biopharmaceutical Aspects. Front. Mol. Biosci. 7, 587997. doi:10.3389/fmolb.2020.587997
Sercombe, L., Veerati, T., Moheimani, F., Wu, S. Y., Sood, A. K., and Hua, S. (2015). Advances and Challenges of Liposome Assisted Drug Delivery. Front. Pharmacol. 6, 286. doi:10.3389/fphar.2015.00286
Sharma, A., and Sharma, U. S. (1997). Liposomes in Drug Delivery: Progress and Limitations. Int. J. Pharmaceutics 154 (2), 123–140. doi:10.1016/S0378-5173(97)00135-X
Shen, H., Zhang, L., Liu, M., and Zhang, Z. (2012). Biomedical Applications of Graphene. Theranostics 2 (3), 283–294. doi:10.7150/thno.3642
Shi, J., Xiao, Z., Votruba, A. R., Vilos, C., and Farokhzad, O. C. (2011). Differentially Charged Hollow Core/Shell Lipid-Polymer-Lipid Hybrid Nanoparticles for Small Interfering RNA Delivery. Angew. Chem. Int. Ed. 50 (31), 7027–7031. doi:10.1002/anie.201101554
Suhara, M., Miura, Y., Cabral, H., Akagi, D., Anraku, Y., Kishimura, A., et al. (2018). Targeting Ability of Self-Assembled Nanomedicines in Rat Acute Limb Ischemia Model Is Affected by Size. J. Controlled Release 286, 394–401. doi:10.1016/j.jconrel.2018.07.049
Sun, T.-M., Du, J.-Z., Yan, L.-F., Mao, H.-Q., and Wang, J. (2008). Self-assembled Biodegradable Micellar Nanoparticles of Amphiphilic and Cationic Block Copolymer for siRNA Delivery. Biomaterials 29 (32), 4348–4355. doi:10.1016/j.biomaterials.2008.07.036
Sun, X., Yu, K., Zhou, Y., Dong, S., Hu, W., Sun, Y., et al. (2021). Self-Assembled pH-Sensitive Polymeric Nanoparticles for the Inflammation-Targeted Delivery of Cu/Zn-Superoxide Dismutase. ACS Appl. Mater. Inter. 13 (15), 18152–18164. doi:10.1021/acsami.1c03589
Suzuki, K., Sato, S., and Fujita, M. (2010). Template Synthesis of Precisely Monodisperse Silica Nanoparticles within Self-Assembled Organometallic Spheres. Nat. Chem 2 (1), 25–29. doi:10.1038/nchem.446
Tambuwala, M. M., Kesharwani, P., Shukla, R., Thompson, P. D., and McCarron, P. A. (2018). Caffeic Acid Phenethyl Ester (CAPE) Reverses Fibrosis Caused by Chronic colon Inflammation in Murine Model of Colitis. Pathol. - Res. Pract. 214 (11), 1909–1911. doi:10.1016/j.prp.2018.08.020
Truzzi, E., Bongio, C., Sacchetti, F., Maretti, E., Montanari, M., Iannuccelli, V., et al. (2017). Self-Assembled Lipid Nanoparticles for Oral Delivery of Heparin-Coated Iron Oxide Nanoparticles for Theranostic Purposes. Molecules 22 (6), 963. doi:10.3390/molecules22060963
Vasile, C. (2018). Polymeric Nanocomposites and Nanocoatings for Food Packaging: A Review. Materials 11 (10), 1834. doi:10.3390/ma11101834
Verma, R. K., and Garg, S. J. P. T. (2001). Drug Delivery Technologies and Future Directions. Pharm. Technol. 25 (2), 1–14.
Wang, H., and Rempel, G. J. J. N. N. N. (2015). Introduction of Polymer Nanoparticles for Drug Delivery Applications. Herndon, USA: Herald Scholarly Open Access.
Wang, H., Miao, W., Wang, F., and Cheng, Y. (2018). A Self-Assembled Coumarin-Anchored Dendrimer for Efficient Gene Delivery and Light-Responsive Drug Delivery. Biomacromolecules 19 (6), 2194–2201. doi:10.1021/acs.biomac.8b00246
Wang, C., Wang, Z., Zhao, X., Yu, F., Quan, Y., Cheng, Y., et al. (2019). DOX Loaded Aggregation-Induced Emission Active Polymeric Nanoparticles as a Fluorescence Resonance Energy Transfer Traceable Drug Delivery System for Self-Indicating Cancer Therapy. Acta Biomater. 85, 218–228. doi:10.1016/j.actbio.2018.12.020
Weddemann, A., Ennen, I., Regtmeier, A., Albon, C., Wolff, A., Eckstädt, K., et al. (2010). Review and Outlook: from Single Nanoparticles to Self-Assembled Monolayers and Granular GMR Sensors. Beilstein J. Nanotechnol. 1 (1), 75–93. doi:10.3762/bjnano.1.10
Xing, P., and Zhao, Y. (2016). Multifunctional Nanoparticles Self-Assembled from Small Organic Building Blocks for Biomedicine. Adv. Mater. 28 (34), 7304–7339. doi:10.1002/adma.201600906
Xu, H., Fang, G., Gou, J., Wang, S., Yao, Q., Zhang, Y., et al. (2015). Lyophilization of Self-Assembled Polymeric Nanoparticles without Compromising Their Microstructure and Their In Vivo Evaluation: Pharmacokinetics, Tissue Distribution and Toxicity. J Biomater. Tissue Eng. 5 (12), 919–929. doi:10.1166/jbt.2015.1405
Xu, S., Zhang, R., Zhao, W., Zhu, Y., Wei, W., Liu, X., et al. (2017). Self-assembled Polymeric Nanoparticles Film Stabilizing Gold Nanoparticles as a Versatile Platform for Ultrasensitive Detection of Carcino-Embryonic Antigen. Biosens. Bioelectron. 92, 570–576. doi:10.1016/j.bios.2016.10.058
Yadav, S., Sharma, A. K., and Kumar, P. (2020). Nanoscale Self-Assembly for Therapeutic Delivery. Front. Bioeng. Biotechnol. 8, 127. doi:10.3389/fbioe.2020.00127
Yan, N., Zhang, Y., He, Y., Zhu, Y., and Jiang, W. (2017). Controllable Location of Inorganic Nanoparticles on Block Copolymer Self-Assembled Scaffolds by Tailoring the Entropy and Enthalpy Contributions. Macromolecules 50 (17), 6771–6778. doi:10.1021/acs.macromol.7b01076
Yang, Y., Hua, C., and Dong, C.-M. (2009). Synthesis, Self-Assembly, and In Vitro Doxorubicin Release Behavior of Dendron-like/Linear/Dendron-like Poly(ε-Caprolactone)-B-Poly(ethylene Glycol)-B-Poly(ε-Caprolactone) Triblock Copolymers. Biomacromolecules 10 (8), 2310–2318. doi:10.1021/bm900497z
Yang, Q., Li, L., Sun, W., Zhou, Z., and Huang, Y. (2016). Dual Stimuli-Responsive Hybrid Polymeric Nanoparticles Self-Assembled from POSS-Based Starlike Copolymer-Drug Conjugates for Efficient Intracellular Delivery of Hydrophobic Drugs. ACS Appl. Mater. Inter. 8 (21), 13251–13261. doi:10.1021/acsami.6b02403
Yoon, H. Y., Jeon, S., You, D. G., Park, J. H., Kwon, I. C., Koo, H., et al. (2017). Inorganic Nanoparticles for Image-Guided Therapy. Bioconjug. Chem. 28 (1), 124–134. doi:10.1021/acs.bioconjchem.6b00512
Zeng, Z., Qi, D., Yang, L., Liu, J., Tang, Y., Chen, H., et al. (2019). Stimuli-responsive Self-Assembled Dendrimers for Oral Protein Delivery. J. Controlled Release 315, 206–213. doi:10.1016/j.jconrel.2019.10.049
Zhai, J., Luwor, R. B., Ahmed, N., Escalona, R., Tan, F. H., Fong, C., et al. (2018). Paclitaxel-Loaded Self-Assembled Lipid Nanoparticles as Targeted Drug Delivery Systems for the Treatment of Aggressive Ovarian Cancer. ACS Appl. Mater. Inter. 10 (30), 25174–25185. doi:10.1021/acsami.8b08125
Zhao, J., Zheng, D., Tao, Y., Li, Y., Wang, L., Liu, J., et al. (2020). Self-assembled pH-Responsive Polymeric Nanoparticles Based on Lignin-Histidine Conjugate with Small Particle Size for Efficient Delivery of Anti-tumor Drugs. Biochem. Eng. J. 156, 107526. doi:10.1016/j.bej.2020.107526
Keywords: self-assembly, polymeric nanoparticles (NPs), liposomes, dendrimers, bioavailability, solid lipid nanoparticles (SLNs), polymerosomes, micellar systems
Citation: Paliwal H, Parihar A and Prajapati BG (2022) Current State-of-the-Art and New Trends in Self-Assembled Nanocarriers as Drug Delivery Systems. Front. Nanotechnol. 4:836674. doi: 10.3389/fnano.2022.836674
Received: 15 December 2021; Accepted: 09 February 2022;
Published: 11 March 2022.
Edited by:
Prajesh Prajapati, National Forensic Sciences University, IndiaReviewed by:
Natassa Pippa, National and Kapodistrian University of Athens, GreeceStergios Pispas, National Hellenic Research Foundation, Greece
Copyright © 2022 Paliwal, Parihar and Prajapati. This is an open-access article distributed under the terms of the Creative Commons Attribution License (CC BY). The use, distribution or reproduction in other forums is permitted, provided the original author(s) and the copyright owner(s) are credited and that the original publication in this journal is cited, in accordance with accepted academic practice. No use, distribution or reproduction is permitted which does not comply with these terms.
*Correspondence: Bhupendra G. Prajapati, bhupen27@gmail.com