- 1Department of Morphology and Pathology (DMP), Federal University of São Carlos, São Carlos, Brazil
- 2Department of Chemistry, Federal University of São Carlos, São Carlos, Brazil
Nanoparticles (NPs) have gained great attention in recent years due to their extensive and innovative applications in the field of medicine. However, conventional physicochemical approaches for the synthesis of NPs may be limited and costly, and the reaction by-products are potentially toxic for human health and the environment. Bio-mediated synthesis of NPs exploiting microorganisms as nanofactories has emerged as an alternative to traditional methods, as it provides economic and environmental benefits. Tropical ecosystems harbor a high diversity of endophytes, which have a diverse array of metabolic pathways that confer habitat adaptation and survival and that can be used to produce novel bioactive compounds with a variety of biological properties. Endophytic bacteria and fungi cultivated under optimum conditions have potential for use in biogenic synthesis of NPs with different characteristics and desired activities for medical applications, such as antimicrobial, antitumoral, antioxidant and anti-inflammatory properties. The bio-mediated synthesis of metal-based NPs can be favored because endophytic microorganisms may tolerate and/or adsorb metals and produce enzymes used as reducing agents. To our knowledge, this is the first review that brings together exclusively current research highlighting on the potential of endophytic bacteria and fungi isolated from native plants or adapted to tropical ecosystems and tropical macroalgae as nanofactories for the synthesis of NPs of silver, gold, copper, iron, zinc and other most studied metals, in addition to showing their potential use in human health.
Introduction
Nanotechnology is an interdisciplinary field of science related to the manipulation and rearrangement of individual atoms and molecules to create materials at the nanometer scale (1 to 100 nm) (Katranidis and Choli-Papadopoulou, 2012). In recent years, the incorporation of nanostructured materials in human, animal and environmental health and biotechnological sectors has been contributing to the improvement of diagnosis and treatment of diseases (Raffa et al., 2010; Patel and Nanda, 2015; Panda et al., 2021).
Metal-based NPs synthetized by chemical and physical methods have a positive impact on human health care (Alaqad and Saleh, 2016; Xu et al., 2020), including the targeted delivery of anticancer agents (Desai et al., 2021), action against antibiotic-resistant Gram-negative and Gram-positive bacteria (Bankier et al., 2019), pathogenic fungi (Abdelnaby et al., 2021) and viruses (Tortella et al., 2021), and anti-inflammatory activity (Wong et al., 2009). Particular traits such as shape, composition, small size, high surface area and charge give NPs their multi-biomedical properties and applications (Navya and Daima, 2016). Nevertheless, the NPs can have cytotoxicity and genotoxicity effects at some concentrations and time of exposition, which cause concern about their clinical use (Jamuna and Ravishankar, 2014; Rezvani et al., 2019). In addition, studies have shown the potential risks of NPs for both aquatic and terrestrial environments (Bundschuh et al., 2018).
The green synthesis of metal-based NPs using microorganisms has emerged as an attractive and alternative method to provide a more clean and sustainable production, and consequently minimize toxic effects to health and the environment (Pal et al., 2019). Microorganisms can be easily cultivated on a large scale in tiny time, and the culture conditions (e.g. biomass quantity, culture time, temperature and pH) can be optimized to synthetize NPs with stability, size and desired shape (Barabadi et al., 2014; Taran et al., 2017).
The synthesis of metal-based NPs using microorganisms occurs by both intra- and extracellular mechanisms (Ovais et al., 2018). In general, in the intracellular synthesis there is an electrostatic interaction between positive charges of the metallic ions and negative charges of the microbial cell wall, followed by a reduction of the metal ion (M+) to its metallic form (Mo). This process is catalyzed by microbial reductases and reductases dependent on the cofactors nicotinamide adenine dinucleotide (NADH) and nicotinamide adenine dinucleotide phosphate (NADPH) that act as electron carriers in the oxidation-reduction reactions. As a result, the NPs are stabilized by capping proteins in the cytoplasm or periplasmic space. However, a disruption of cells is required to obtain purified NPs (Hulkoti and Taranath, 2014; Busi and Rajkumari, 2019). In contrast, in the extracellular synthesis the culture supernatant, biomass or cell-free extract is mixed with the metal solution, and the NPs are produced outside the microbial cell. This process is carried out by reductases produced and secreted to the culture medium by microbial cells and cofactors. After bio-reduction and nucleation, the synthetized NPs are also stabilized by capping agents (Hulkoti and Taranath, 2014). The NPs synthesized intra- or extracellularly can have different shapes, such as spherical, triangular, oval, cubic and square (Busi and Rajkumari, 2019).
Endophytic bacteria and fungi have been considered as potent biofactories for the synthesis of metal-based NPs with medicinal properties (Rahman et al., 2019; Eid et al., 2020; Rana et al., 2020). These microorganisms colonize inter- and/or intracellular tissues of plants and stablish with them a symbiotic relationship without causing any apparent harm (Petrini, 1991; Hallmann et al., 1997). They may improve plant health and development by several mechanisms, including the production of antimicrobial compounds (Fadiji and Babalola, 2020).
Endophytes can tolerate and/or accumulate metals present in the environmental to alleviate host plant stress and toxicity, and favor its own adaptation and competitive edge over other microorganisms in this niche (Xu et al., 2015; Franco-Franklin et al., 2021). This metal detoxification ability of the endophytes can be explored to synthetize metal-based NPs via intra- and extracellular mechanisms. The supernatant of silver (Ag)-resistant Bacillus safensis TEN12 was used for the intracellular synthesis of silver (Ag) NPs with an average size from 22 to 45 nm and spherical shape (Ahmed et al., 2020). Stable and quasi-spherical zinc oxide (ZnO) NPs sized 2–9 nm were synthesized by the zinc-tolerant endophytic Cochliobolus geniculatus through the extracellular mechanism (Vijayanandan and Balakrishnan, 2018). Similarly, spherical cobalt oxide (CoO) NPs sized 20 nm were synthesized by the CoO-tolerant endophytic Aspergillus nidulans also via the extracellular mechanism (Vijayanandan and Balakrishnan, 2018).
Endophytic microorganisms can produce bioactive secondary metabolites with a high degree of biological activities and structural diversity to be explored in human health (Gupta and Shukla, 2020; Adeleke and Babalola, 2021). Thus, bacteria and fungi isolated from different parts of plants can be easily culturable in laboratory under optimum culture conditions to manufacture NPs with desired characteristics and activities (Zielonka and Klimek-Ochab, 2017; Messaoudi and Bendahou, 2020).
Tropical ecosystems are considered a hotspot of diversity of endophytic microorganisms that contains species not recovered from other areas (Arnold and Lutzoni, 2007; Roy and Banerjee, 2018). The great competition, limited resources and selection pressure in tropical environments favor not only the high diversity of endophytes, but also the production of novel biomolecules with medical properties (Rosa, 2011; Pereira et al., 2017; Sebastianes et al., 2017), opening up perspectives for the synthesis of new NPs for healthcare applications. In this way, several studies have demonstrated the potential of endophytes isolated from native plants or adapted to tropical ecosystems for the green synthesis of silver (Ag) (Balakumaran et al., 2015), gold (Au) (Munawer et al., 2020), copper (Cu) (Hassan et al., 2018), zinc (Zn) (Uddandarao and B, 2016) and other metal-based NPs. Marine macroalgae widespread across the tropics are also a promising source of endophytes for the biosynthesis of NPs with medicinal properties (Manjunath et al., 2017).
An overview of metal-based NPs synthesis from endophytes is shown in Figure 1. Our manuscript brings as novelty a literature review focused exclusively on the potential of endophytic bacteria and fungi of a range of host plants and macroalgae from tropical ecosystems for the green synthesis of metal-based NPs with different characteristics and their potential applications in the health sector.
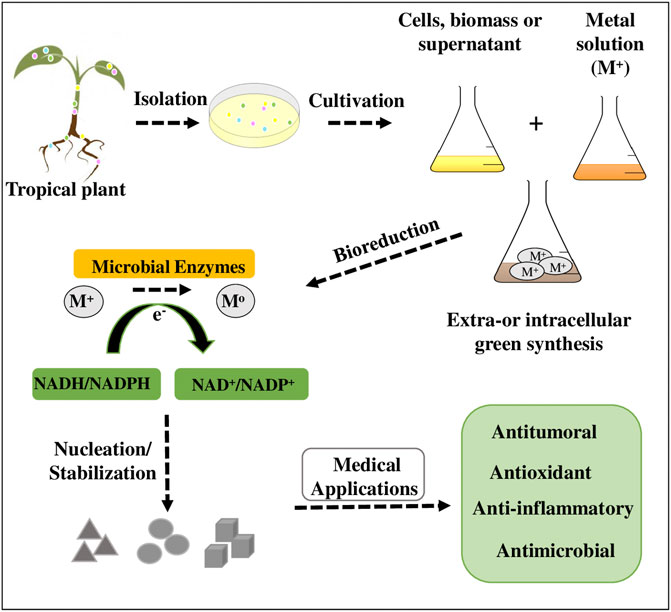
FIGURE 1. Schematic representation of the general steps for green synthesis of metal-based NPs using endophytic microorganisms isolated from tropical plant.
Biosynthesis of Nanostructural Materials
Nanoparticulate materials can have overwhelming advantages over micro structured materials. For these materials to be applied in the health and wellness sectors, several factors must be considered to define their efficiency, such as chemical composition, size and morphology. When using the green biosynthesis from endophytic microorganisms, the determining variables for the synthetic process include the microorganisms that will be used, how these microorganisms will be used in the biosynthesis and the normal synthesis conditions. These metal reduction processes normally occur through NADH and NADPH-dependent reductases present in different endophytic fungi and bacteria (Durán et al., 2005).
The first point to be considered for the synthesis of nanomaterials from endophytic microorganisms is the isolation of the target fungus/bacteria. Various parts of the target plants/algae such as seeds, roots, leaves and stems are cleaned. Pieces of these plant parts are placed under specific conditions for optimal growth of microorganisms (culture medium, supplements, temperature, time, CO2 rate, agitation, etc.) (Adeleke and Babalola, 2021). After growth, isolation of a target microorganism can occur, or use all microorganisms present. Once the strategy is defined, the total biomass of the microorganisms can be used, the cell extract (obtained through the filtration of the initial biomass), or the cell-free extract (which contains metabolites produced by the microorganisms) to produce NPs (Andleeb et al., 2021). It is particularly important to define which of the types of interaction mentioned above will be used, as these living systems have intrinsic factors (such as metabolites and enzymes) that will define the sort of nanoparticle obtained. Furthermore, it is worth remembering that each microorganism will produce different types of metabolites and enzymes in different proportions. These factors can act during the biosynthesis process as surfactants, stabilizers or even reducing agents (Patra and Baek, 2014).
As a general procedure for the synthesis of nanoparticulate materials, biomass/cell extract/cell-free extract is added to a solution of the NPs metal precursor salt. Usually, transition metal nitrates, chlorides, sulfates and acetates are used. Reactional conditions of time, pH, temperature, time, pressure and starting reactants are key elements to maximize the interactions necessary for the formation of the NPs. In this way it is possible to control the size, shape and even the composition of the formed NP. After defining all these parameters, a colloid can be obtained from the NPs, or they can be filtered and washed, obtaining a powder as the final product. Supplementary Table 1 show an overview of metals and metal oxides NPs, respectively, synthesized from endophytic microorganisms from tropical plants.
Silver Nanoparticles (Ag NPs)
Silver as a metal has been used since ancient times due to its microbicidal and/or anti-inflammatory properties (Assis et al., 2020; Alavi and Varma, 2021; Salesa et al., 2021). As a result, it has become a key NP of easy obtention through green biosynthesis by endophytic microorganisms. It is interesting to note that all Ag NPs biosynthesized by endophytic microorganisms derived from tropical plants have spheroidal morphology, using silver nitrate as a source of Ag+ ions.
The use of endophytic microorganisms located in the roots of tropical plants can be an important pathway for the biosynthesis of Ag NPs. Some works using cell-free extracts (metabolites and proteins generated in culture) of endophytic bacteria SYSU 333150 Isoptericola sp. (Dong et al., 2017) and Streptomyces laurentii (Eid et al., 2020) reported the obtention of spheroidal Ag NPs with diameter smaller than 40 nm. Other studies investigated cell-free extracts of fungi Alternaria sp., Aspergillus sp., Chaetomium sp., Cladosporium sp., Colletotrichum sp., Curvularia sp., Guignardia sp., Penicillium sp., Pestalotia sp., Pestalotiopsis sp., and Phomopsis sp. obtained from leaves of Raphanus sativus (Singh et al., 2017) and Azadirachta indica (Balakumaran et al., 2015), which gave rise to Ag NPs sized 30 nm.
The cell extract of these endophytic microorganisms also becomes a differentiated platform for synthesis, as it allows their interaction specifically with Ag+ ions and the possibility of bio-reduction to metallic Ag NPs. Some studies demonstrated that cell extracts of Penicillium sp., Alternaria sp., Aspergillus sp. and Cladosporium sp. isolated from leaves extract of Calotropis procera (Chowdhury et al., 2016; Mohamed et al., 2019), Aspergillus tubingensis and Bionectria ochroleuca from Rhizophora magle and Laguncularia racemose (Rodrigues et al., 2013) and Aspergillus niger from Stypandra glauca (Hemashekhar et al., 2017) can form Ag NPs sized up to 45 nm. Ag NPs smaller than 15 nm can be synthesized in the same way using fungi cell extracts of Exserohilum rostrata from Ocimum tenuiflorum (Bagur et al., 2020b) and Penicillium sp. from Tinospora cordifolia (Bagur et al., 2020a).
The total biomass of these microorganisms can also be used to obtain these Ag NPs. The fungi biomass of Colletotrichum incarnatum, Alternaria solani and Penicillium funiculosum (from leaves of Datura metel and Gloriosa superba) (Devi et al., 2014; Chandankere et al., 2020) and Trichoderma spp. (from seeds of B. excelsa) (Ramos et al., 2020) was used, resulting in particles smaller than 40 nm. Biomass of endophytic fungi from tropical seaweeds can also be used for the biosynthesis of Ag NPs. Hulikere et al. removed this biomass of Cladosporium cladosporioides from the seaweed Sargassum wightii, yielding Ag NPs with size of 60 nm (Manjunath and Joshi, 2019). In another work, Neethu et al. obtained Ag NPs with size of 15 nm using cell extract of Penicillium polonicum from the seaweed Chaetomorpha antennina (Neethu et al., 2018).
Gold Nanoparticles (Au NPs)
Like Ag NPs, the antimicrobial and anti-inflammatory properties of Au NPs are already extensively known. However, since gold is a noble metal with high added value, its use may be compromised. Regarding the synthesis of such metal, the most used Au-based starting reagent is HAuCl4. Endophytic fungi from tropical marine environments are widely used for the biosynthesis of Au NPs. It was found that the biomass of the fungus Cladosporium cladosporioides extracted from the seaweed Sargassum wightii has the ability to synthesize Au NPs spheres with diameter of up to 60 nm (Manjunath et al., 2017). Au NPs spheres sized approximately 10 nm were obtained from the cell extract of the fungus Cladosporium sp. located in the leaves of Commiphora wightii (Munawer et al., 2020). Regarding Au NPs with triangular morphology, they were formed by cell extracts of fungi of the genera Aspergillus sp. and Alternaria sp. found in the stem of Azadirachta indica and in the roots of Rauvolfia tetraphylla, respectively (Verma et al., 2010; Hemashekhar et al., 2019). Au NPs with needle-like morphology and size of 45 nm were also synthesized, but using the biomass of the fungus Fusarium solani present in the roots of Cleistes fragrans (Clarance et al., 2020).
In our group research, recent work (Sousa et al., 2021) was conducted highlighting the green synthesis. Through the nanoparticles biosynthesis using Paenibacillus polymyxa and P. terrae isolated from Brazilian tropical savannah plants Prunus spp. (Ratti et al., 2008) and Tabebuia spp. (Romano, 2014) respectively, the process was optimized considering the synthesis time, the extract concentration, the wavelength and the particle size. The experiment was divided into phases where the best focus (1, 1:2, 1:4, 1:8 and 1:16) was analyzed, observing through UV-Vis spectroscopy at 540 nm the best absorption of light in relation to time and over 72 h in a 12-h interval. As the best indicators were 1:2 and 1:4, for both bacterial extracts and 12–24 h as the best periods. The next phase was analyzed hourly for 24 h, with the values 1:2 and 1:4, for light absorption at 540 nm and in the spectral range of 300 to 800 nm, demonstrating a statistic of gold nanoparticle for 1:2 dilutions, whose peak at around 540 nm, and as of 7 a.m. are already described. Based on the results of the characterization by DLS and absorbance, such as Au NPs synthesized through crude extractions P. polymyxa 1:2 and P. terrae 1:2 was as obtaining better results, obtaining the average level of 262 and 372 nm, respectively, in monodispersed systems, with the following occurrences after 7 h of biosynthesis reaction, obtaining greater results between 12 and 24 h. The main absorption was detected at the red spectral range.
Copper-Based Nanoparticles (Cu NPs)
A cheaper alternative to both Ag NPs and Au NPs are Cu NPs. It was found that the fungus Aspergillus terreus isolated from the plants Aegle marmelosa and Origanum majorana is capable of producing spheroidal CuO NPs from CuSO4 (Mani et al., 2021; Mousa et al., 2021). In this case, the use of biomass and cell extract of the fungus led to the formation of CuO NPs with different sizes. It was reported that Cu NPs sized less than 100 nm can also be obtained from marine actinomycetes taken from tropical seaweeds (Rasool and Hemalatha, 2017), and from Streptomyces capillispiralis extracted from leaves of Calendula arvensis (Hassan et al., 2018). According to the literature, by changing the source of Cu2+ ions to Cu(NO3)2 and using the fungus Phaeoacremonium sp. it is possible to modulate the morphology of Cu NPs, obtaining nanorods with size of approximately 97 nm (Morais et al., 2021).
Zinc-Based Nanoparticles (Zn NPs)
Zn-based nanoparticles have attracted great commercial interest, as they are an alternative for the design of new antimicrobial materials with low cellular toxicity. From the biomass of the fungus Aspergillus flavus it was possible to obtain ZnS NPs using ZnSO4 as a starting reagent (Uddandarao, 2016). This fungus was isolated from Nothapodytes foetida leaves, generating ZnS spheres with diameter of 18 nm. In another study, Uddandarao et al. (2019) were able to perform Gd doping of ZnS NPs by adding Gd(NO3)3 to the biomass. Furthermore, it was demonstrated that ZnO NPs can also obtained from the cell extract of Periconium sp. (Ganesan et al., 2020). ZnO spheres (15 nm) and rods (50 nm), for instance, can be formed using ZnSO4 and Zn(NO3)2 as starting reagents, respectively (Ganesan et al., 2020; Mousa et al., 2021).
Cobalt-, Titanium-, Iron- and Nickel-Based Nanoparticles (Co, Ti, Fe and Ni NPs)
The fungus Aspergillus terreus can be used to perform the reduction of Co, Fe and Ni salts, giving rise to spherical Co3O4, Fe3O4 and NiO NPs sized 15, 40 and 60 nm, respectively (Mousa et al., 2021). It was reported that spheroidal CoO NPs can also be obtained from the fungus Aspergillus nidulans isolated from N. foetida leaves (Vijayanandan and Balakrishnan, 2018). Micro- and nanostructured TiO2 particles of different shapes, such as rod-like, triangular, pentagonal and spherical, can be formed using the biomass of the fungus Trichoderma citrinoviride isolated from the roots of Sorghum bicolor (Arya et al., 2021).
Potential Applications of Metal-Based NPs in Medicine
Metal-based NPs have been successfully synthesized using a diversity of endophytic bacteria and fungi associated with plants and macroalgae found in both tropical terrestrial and marine ecosystems. Some studies related to their characteristics and medical applications are summarized in Supplementary Table 1 and will be further discussed in detail below.
Antimicrobial and Biofilm Activities
The use of NPs in the clinical area is very important in public health, especially when considering the increasingly worrying resistance of human pathogens to available drugs. Differently from conventional antimicrobials, nanoparticles do not necessarily need to enter microbial cells to start acting, as their action is normally related to the cell wall (Knetsch and Koole, 2011; Wang et al., 2017). In this way, a microorganism could hardly become resistant to them since successive mutations in the same cell would have to occur for it to develop resistance (Pandey et al., 2021). Thus, NPs have the potential to overcome the problems of resistance and multi-resistance to antimicrobials.
The antimicrobial mechanisms of different metal based-NPs synthetized intra or extracellularly by microorganisms are not yet well understood. However, many studies have been demonstrated that these NPs are able to physically interact with the cell wall and membrane causing disintegration and change in its permeability. The penetration of NPs into the microbial cell can lead to production of reactive oxygen species (ROS) and free radicals, besides alterations in mitochondrial respiratory chain proteins, inhibition of DNA replication and its denaturation, ribosomal subunits destabilization and inhibition of translation and protein synthesis, denaturation of proteins and enzymes, and subsequent general metabolic dysfunction and cell death (Wang et al., 2017; Busi and Rajkumari, 2019; Shaikh et al., 2019) (Figure 2).
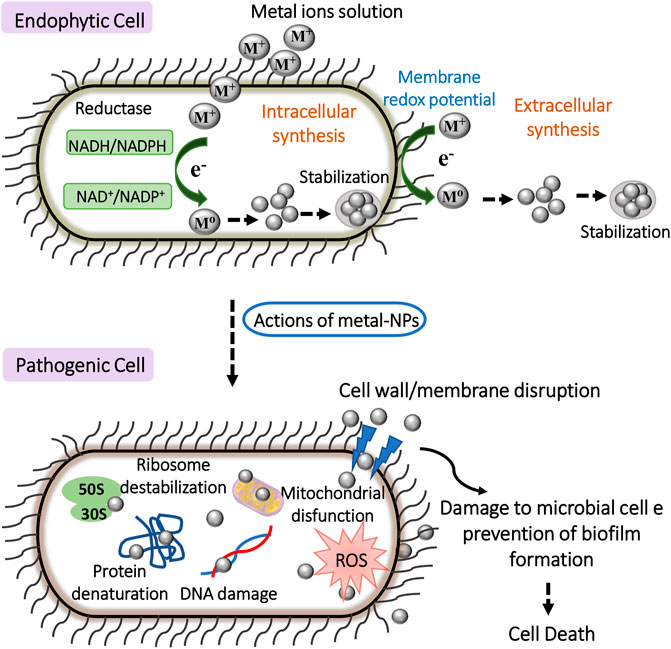
FIGURE 2. Schematic representation of general antimicrobial mechanisms of metal-based NPs synthetized by intra-or extracellular mechanisms using endophytic cells. The adherence of NPs to pathogen surface cause multiple damage to cellular machinery, inhibit bacterial growth and biofilm formation, and promote cell death.
According to Shariq Ahmed et al. (2019), Ag NPs synthesized by an endophytic bacterium isolated from the tropical plant Pennisetum setaceum inhibited the growth of Escherichia coli (ATCC 25922), Klebsiella pneumoniae (ATCC 35657), Acinetobacter baumannii (ATCC 19606), Pseudomonas aeruginosa (ATCC 27853), Salmonella typhimurium (ATCC 14028) and Staphylococcus aureus (MTCC 1430). Furthermore, these nanoparticles were also able to reduce biofilm formation, being the inhibition of pathogens attributed to the action of NPs on the cell walls of the indicators. It is noteworthy that studies on the antimicrobial activity against microorganisms of the “ESKAPE” group, which include the bacteria Enterococcus faecium, S. aureus, K. pneumoniae, A. baumannii, P. aeruginosa and Enterobacteriaceae, are very relevant since these bacterial pathogens are known to evade conventionally used antibiotics in their treatments through drug resistance (Xie et al., 2018). Among them there is the opportunistic bacterium A. baumannii, a human pathogen that has been considered to cause serious medical condition, as it easily develops antimicrobial resistance (Lee et al., 2017) and is increasingly present in cases of hospital infections and intensive care (Anitha et al., 2016).
Bagur et al. (2020b) synthesized Ag NPs from extracts of the tropical endophytic fungus Exserohilum rostrata and reported the inhibition of the pathogens S. aureus, E. coli, K. pneumoniae and P. aeruginosa, in addition to antibiofilm activity. In their work, the authors compared endophytic extracts with and without the nanoparticles and observed that those with Ag NPs had a greater antimicrobial activity. According to them, this combination possibly intensified the natural potential inhibition of the extracts. Additionally, other works also reached similar results. Bagur et al. (2020a), for instance, showed a possible synergism between bioactive compounds of the extract of the endophytic fungus Penicillium sp. and Ag NPs, evidenced by the greater antimicrobial activity of the extracts with Ag NPs against S. aureus, E. coli, P. aeruginosa, and K. pneumoniae. Hemashekhar et al. (2017) also observed that extracts of the endophytic fungus A. niger with silver nanoparticles were able to inhibit E. coli, P. aeruginosa, S. aureus and K. pneumoniae, while the pure extract were not. It is important to mention that both studies used endophytes isolated from tropical plants as well.
CuO NPs synthesized by the endophytic fungus Aspergillus terreus FCBY1 isolated from a tropical plant Aegle marmelos were tested by Mani et al. (2021) against Staphylococcus epidermidis, Candida albicans, Proteus mirabilis, Vibrio cholerae, Salmonella typhi, S. aureus, P. aeruginosa, K. pnuemoniae, E. coli and A. niger. It was shown that all microorganisms had their growth inhibited by CuO NPs – although the inhibition of fungi was lower than that of bacteria. Moreover, no variation was observed in the inhibition of Gram-positive and Gram-negative bacteria. Naturally, the authors pay more attention to bacteria, as the cell walls of both types are morphologically different, which normally contributes to the activity against them. Gram-positive bacteria have a thick layer of peptidoglycan and teichoic acids on their cell wall, while Gram-negative bacteria have a thin layer of peptidoglycan and an outer membrane composed of lipopolysaccharides (LPS), lipoproteins and phospholipids (Tortora et al., 2016). Additionally, Gram-negatives have intracellular efflux pumps in their cytoplasmic membrane that limit the permeability of drugs in their bacterial cell and reduce the action of antibiotics, making the search for new agents against these pathogens more difficult (Page and Heim, 2009; Dorotkiewicz-Jach et al., 2015; Haynes et al., 2017).
Hemashekhar et al. (2019) evaluated the production of Au NPs from the endophytic fungus Alternaria spp. isolated from Rauvolfia tetraphylla in Tumakuru, India. The synthesized NPs were tested against E. coli, P. aeruginosa, K. pneumoniae and S. aureus and were able to inhibit the growth of all strains. Furthermore, the antibiofilm potential of Au NPs, which decreased biofilm formation between E. coli and P. aeruginosa, was also reported. Biofilms can be defined as a community of microorganisms connected through extracellular products that can be found in different types of environments, including human infections (Reid, 1999). It is estimated that approximately 80% of human infections are linked to the formation of biofilms (Hemmati et al., 2021), which contribute to more complicated disease treatments. Antibiofilm assays are usually performed using biosynthesized Ag NPs against A. baumannii (Neethu et al., 2018, 2020), however, they can also be effective against Enterococcus faecalis (Halkai et al., 2018), P. aeruginosa (Ranjani et al., 2021), Bacillus cereus and V. cholerae (Chandankere et al., 2020). In this case, bacterial death is caused by the disruption of bacteria integrity. The same effect is observed in biofilms, showing that it does not constitute a barrier to the bactericidal effect of Ag NPs (Neethu et al., 2020).
The production of Cu NPs by a marine endophytic actinomycete isolated from tropical algae was evaluated by Rasool and Hemalatha (2017), who tested these nanoparticles against some clinical pathogens such as methicillin-resistant Staphylococcus aureus (MRSA) K. pneumoniae, P. mirabilis, E. coli and S. typhimurium. In this work, two volumes were used to analyze the antimicrobial activity of NPs (50 and 100 µl). Even though both of them were able to inhibit all tested pathogens, including MRSA, greater bioactivity was achieved at the highest volume. Ganesan et al. (2020) used extracts of the endophytic Periconium sp. to synthesize ZnO NPs and tested them against E. coli, S. aureus and C. albicans. It was observed that all microorganisms had their growth inhibited by the NPs.
Chowdhury et al. (2016) used Ag NPs synthesized by tropical endophytic Penicillum sp. and Alternaria sp. and obtained increased antibiotic activity against E. coli when compared to Bacillus subtilis. In this case, it was suggested that E. coli was more susceptible to silver nanoparticles than B. subtilis. It is worth mentioning that microorganisms are sources of several secondary metabolites whose production may vary and that synthesized nanoparticles can have different sizes and morphologies, which may interfere with their action (Buzea et al., 2007).
Manjunath et al. (2017) isolated the endophytic fungus Cladosporium cladosporioides from the seaweed Sargassum wightii found in the tropical ocean and evaluated the production and antimicrobial activity of Au NPs by the endophyte. The human pathogens used were Bacillus subtilis (MTCC 441), Aspergillus niger (MTCC 281), E. coli (MTCC 118), S. aureus (MTCC 7443) and P. aeruginosa (MTCC 424), all of which had their growth inhibited by the Ag NPs. Electron microscopy tests showed that the NPs acted on the cell walls of the microorganisms, breaking them down. Ranjani et al. (2021) synthesized Ag NPs using extracts of the tropical endophytic fungus Lasiodiplodia theobromae and tested them against P. aeruginosa ATCC (27853) and P. aeruginosa, which were clinically isolated from a patient. Both strains had their growth inhibited by the NPs and with significant activity when compared to positive controls, in addition to reducing the antibiofilm formed by the pathogens. Another important point in this study was the evaluation of the effects of endophytic nanoparticles on the production of pyocyanin, one of the toxins synthesized by P. aeruginosa. It was observed that Au NPs reduced by 69% the toxin produced by clinically isolated P. aeruginosa, indicating that silver ions possibly crossed the membrane and interfered with the pathogen metabolism.
Antioxidant Activity
The antioxidant activity of NPs has already been explored in several studies (Yang et al., 2019; Bedlovičová et al., 2020), as they protect cells from the damage of ROS. The most common assay used to evaluate the antioxidant activity of biogenic nanoparticles is the DPPH free radical scavenging. This method consists of the reduction of 2,2-diphenyl-1-picrylhydrazyl (DPPH) to methanol in the presence of antioxidants, leading to a color change from violet to yellow. Additionally, the values are calculated through control and sample absorbances (Garcia et al., 2012; Rahman et al., 2015). Another typical technique is the ferric reducing antioxidant power assay (FRAP), which also involves a reduction-dependent color change. The principle of this technique is the reduction caused by antioxidants of ferric 2,4,6-tripyridyl-s-triazine complex [Fe3+-(TPTZ)2]3+ to ferrous complex [Fe2+-(TPTZ)2]2+, which present an intense blue color that can be measured by an spectrophotometer at 593 nm (Gulcin, 2020). The DPPH method is frequently used in studies on the biosynthesis of Ag NPs obtained from Cladosporium cladosporioides extracted from Sargassum wightii (Manjunath and Joshi, 2019) and Aspergillus niger from Simarouba glauca (Hemashekhar et al., 2017); Au NPs obtained from Alternaria spp. isolated from Rauvolfia tetraphylla (Hemashekhar et al., 2019); and CuO, Fe3O4, NiO and ZnO NPs obtained from different endophytes from tropical environments (Ganesan et al., 2020; Mani et al., 2021; Mousa et al., 2021). Nirmala and Sridevi (Nirmala and Sridevi, 2021) isolated the endophytic bacterium Pantoea anthophila from Waltheria indica in order to synthesize Ag NPs and evaluate its antioxidant and antimicrobial ability. To determine the antioxidant potential, the authors used the DPPH, ABTS, H2O2 and superoxide (O2−) radical scavenging assay and FRAP assay. The NPs showed an excellent antioxidant activity that can be applied in various oxidative stress-related degenerative diseases (Nirmala and Sridevi, 2021).
Antineoplastic Activity
NPs have been increasingly used in therapeutical applications due to their small size and consequent high capacity to interact with cells, proteins and genetic material. Studies have been suggested the electrostatic interaction between the cell surface and NPs and subsequent damage to membrane, ROS generation, protein oxidation, damage to electron transport chain in mitochondria, DNA and cell cycle, and finally induction of apoptosis and necrosis in different cancer cell lines by regulation of essential signaling pathways (El-Sonbaty, 2013; Munawer et al., 2020; Andleeb et al., 2021) (Figure 3).
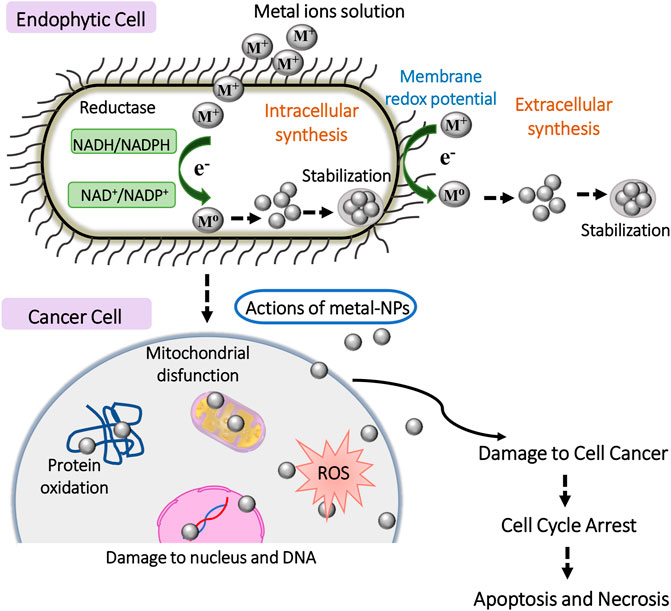
FIGURE 3. Schematic representation of general anticancer mechanisms of metal-based NPs synthetized by intra-or extracellular mechanisms using endophytic cells. The interaction between the cancer cell surface and NPs and subsequent damage of organelles and biomolecules induces apoptosis and necrosis.
Netala et al. (2016) determined the cytotoxicity effect of biosynthesized Ag NPs against B16F10, SKOV3, A549, PC3 and CHO cell lines. They also analyzed their apoptotic potential against human ovarian carcinoma cells and morphological modifications through microscopic analysis and staining assays. The endophytic fungus Penicillium sp. was isolated to produce biogenic Ag NPs, which were submitted to antiproliferative analysis against two types of carcinomas using MTT assay (HepG-2 and MCF-7) (Bagur et al., 2020a). Clarance et al. (2020) used MCF-7 and HeLa cell lines to analyze the anticancer activity of Au NPs synthesized from Fusarium solani isolated from Chonemorpha fragrans. These biosynthesized Au NPs presented effective cytotoxicity activity as well as apoptosis-inducing activity and cell cycle arrestment. Akther et al. (2019) synthesized biogenic Ag NPs from the endophytic fungus Botryosphaeria rhodina isolated from a tropical medicinal plant (Catharanthus roseus), which were found to present high anticancer potential determined through MTT assay, DNA fragmentation assay and evaluation of DNA damage by staining assays. The tests were carried out against human lung carcinoma cells (A549). Similarly, Munawer et al. (2020) produced a biosynthesized Au NPs and demonstrated their anticancer ability against MCF-7. The in vitro studies included MTT assay, staining assays, evaluation of DNA fragmentation and further comet assay, whereas the in vivo studies consisted of acute oral toxicity analysis, treatment exposure after tumor induction, histopathology study and level of apoptosis induction and DNA fragmentation. The authors observed that the in vitro anticancer activity was more relevant in Au NPs synthesized from Cladosporium sp. isolated from Commiphora wightii leaves. The authors concluded that the potential therapeutical application of NPs is, in fact, due to the facilitated interaction between the NPs and a range of molecules within the tumoral milieu.
Challenges Associated With Metal-Based NPs Synthesized by Endophytes
Non-toxic, biocompatible, cost-effective and eco-friendly NPs would be ideal for the safe use in medicine. The potential benefits of metal-based NPs synthesized using endophytes for healthcare applications have been well documented (Rahman et al., 2019; Eid et al., 2020; Rana et al., 2020). However, the knowledge about their precise action mechanisms, specificity and toxicity for humans, as well as non-target organisms/cells and the environment represents a great challenge for healthcare applications.
In general, metal-based NPs for usage in medicine can enter the body applying alternate routes, such as digestive, inhalation and skin penetration (De Matteis, 2017). Their physicochemical properties (e.g., surface-to-volume ratio, shape, charge, composition, concentration, solubilization) are important to mediate the interaction with the surface of the cells and penetration, biocompatibility, accumulation and toxicity (Ajdary et al., 2018). Optimization of the NPs synthesis parameters (e.g., yield, time, temperature, pH, metal ion solution and biomass concentration) also should be considered to obtain different NPs with features, stability and desired activities (Singh et al., 2014). Achieve ideal physicochemical properties and synthesis parameters of metallic NPs is still very challenging for development in large-scale of green nano-formulations, once it includes the integration of methods and process that should be reproducible and viable to reach the pharmaceutical market for a security and efficient administration in humans (Dikshit et al., 2021).
The selective cytotoxicity of metal-based NPs is other essential parameter to be evaluated for healthcare applications, because once into the cell these nanomaterials produce damage (e.g., ROS generation, damage to organelles, DNA and other biomolecules) that can lead to activation of cell death pathways (Ajdary et al., 2018; Attarilar et al., 2020). Thus, caution must be taken in NPs use involving living organisms, and both in vitro and in vivo assays are required.
Although studies using model organisms to assess the cytotoxicity and other effects of NPs are unarguably important and have been carried out (Sayadi et al., 2020; Tortella et al., 2020), to our knowledge the literature available so far to evaluate the NPs synthesized by endophytes from the tropics only addresses in vitro studies. Thus, it is other important challenge to be overcome for future safe and effective use of these green NPs in medicine.
There is a huge expectative surrounding the clinic applications of NPs. However, its regulation still involves gaps and is a worldwide challenge. This scenario reflects the lack of full knowledge of precise interaction of many nanomaterials with biological systems and its potential of accumulation and toxic effects that could be applied to organisms and environment (Foulkes et al., 2020). In addition, the differences in responses in in vitro assays and even between model animals and humans to nano drugs, the variations in their physicochemical properties which can lead to the alteration of the pharmacokinetics and pharmacodynamics assessment, the diverse administration routes, control of the manufacturing process, scale-up and reproducibility, among other factors, can contribute to difficult the development of a nano drug and its regulatory process (Barata-Silva et al., 2021).
The regulation of nanomaterials is necessary to provide security to pharmaceutical industry and patients. In general, the main international regulatory agencies, among them the European Medicines Agency (EMA) and Food and Drug Administration (FDA), making use of scientific and clinical knowledge in regulatory decisions. However, as there is not standardized regulatory guidance for nano drugs development and evaluation, these agencies seek provide guides and technical standards to pharmaceutical industry (Soares et al., 2018; Đorđević et al., 2021).
Despite the promising advances, the regulatory guidance remains unconsolidated among different regulatory agencies. Therefore, a strategy to evaluate the safety, efficacy, toxicity, compatibility, bioavailability and other parameters of nano drugs for its administration in humans is consider the previous studies and regulation used for the development of traditional medicines by pharmaceutical industry (Barata-Silva et al., 2021). These factors combined with an expensive, complex and time-consuming process of production constitute some of main obstacles for nano drugs clinical translation and their potential entry on the market (Choi and Frangioni, 2010; Hua et al., 2018). However, still there is a great perspective for green synthesis of NPs using endophytes and other microorganisms for future development of new therapeutic agents (Nafeh and Mazhar, 2021; Rehman et al., 2021).
Conclusion
In a scenario in which there is an increasing search for new drugs with greater efficiency, less toxicity and rare adverse effects, the green synthesis of NPs has become of great relevance for the health area. The synthesis of NPs using the biogenic approach is also cost-effective, eco-friendly, and easy to scale-up, overcoming the disadvantages of conventional chemical and physical methods. Considering that most studies on endophytic bacteria and fungi have been carried out using host plants from temperate environments, studies on endophytes from tropical environments offer a great opportunity to explore their potential as nano factories for the synthesis of NPs for application in medicine. In fact, metal-tolerant endophytic are effective tools for the synthesis of metal-based NPs. If appropriately exploited, the green synthesis of NPs using endophytes from the tropics can become a promise way for the numerous therapeutic applications of these materials in the imminent future. Despite it, there are still challenges to be addressed by the researchers to develop green metallic NPs for safe and effective biomedical applications for human and the environment.
Author Contributions
AB conceived the idea of the manuscript. CS supervised the project. AB, SR, MG, MA, EL and CS wrote, revised and approved the final version of the manuscript.
Funding
This work was supported by grants from the São Paulo Research Foundation (FAPESP) No. 2020/16299-9 to SHR, Nos. 2016/13423-5 and 2017/12905-9 to CPS and No. 2013/07296-2 to EL, and from the Coordination for the Improvement of Higher Education Personnel (CAPES) under finance code (001) to MA.
Conflict of Interest
The authors declare that the research was conducted in the absence of any commercial or financial relationships that could be construed as a potential conflict of interest.
Publisher’s Note
All claims expressed in this article are solely those of the authors and do not necessarily represent those of their affiliated organizations, or those of the publisher, the editors and the reviewers. Any product that may be evaluated in this article, or claim that may be made by its manufacturer, is not guaranteed or endorsed by the publisher.
Acknowledgments
This work was partly funded by the São Paulo Research Foundation (FAPESP), the Financier of Studies and Projects (FINEP), the National Council for Scientific and Technological Development (CNPq), and the Coordination for the Improvement of Higher Education Personnel (CAPES).
Supplementary Material
The Supplementary Material for this article can be found online at: https://www.frontiersin.org/articles/10.3389/fnano.2022.823236/full#supplementary-material
References
Abdelnaby, M. A., Shoueir, K. R., Ghazy, A. A., Abdelhamid, S. M., El Kemary, M. A., Mahmoud, H. E., et al. (202110286). Synthesis and Evaluation of Metallic Nanoparticles-Based Vaccines against Candida Albicans Infections. J. Drug Deliv. Sci. Tech., 102862. doi:10.1016/j.jddst.2021.102862
Adeleke, B., and Babalola, O. (2021). Pharmacological Potential of Fungal Endophytes Associated with Medicinal Plants: A Review. JoF 7, 147. doi:10.3390/jof7020147
Ahmed, T., Shahid, M., Noman, M., Bilal Khan Niazi, M., Zubair, M., Almatroudi, A., et al. (2020). Bioprospecting a Native Silver-Resistant Bacillus Safensis Strain for green Synthesis and Subsequent Antibacterial and Anticancer Activities of Silver Nanoparticles. J. Adv. Res. 24, 475–483. doi:10.1016/j.jare.2020.05.011
Ajdary, M., Moosavi, M., Rahmati, M., Falahati, M., Mahboubi, M., Mandegary, A., et al. (2018). Health Concerns of Various Nanoparticles: A Review of Their In Vitro and In Vivo Toxicity. Nanomaterials 8, 634. doi:10.3390/nano8090634
Akther, T., Vabeiryureilai Mathipi, V., Nachimuthu Senthil Kumar, N. S., Davoodbasha, M., and Srinivasan, H. (2019). Fungal-mediated Synthesis of Pharmaceutically Active Silver Nanoparticles and Anticancer Property against A549 Cells through Apoptosis. Environ. Sci. Pollut. Res. 26, 13649–13657. doi:10.1007/s11356-019-04718-w
Alaqad, K., and Saleh, T. A. (2016). Gold and Silver Nanoparticles: Synthesis Methods, Characterization Routes and Applications towards Drugs. J. Environ. Anal. Toxicol. 6, 1000384. doi:10.4172/2161-0525.1000384
Alavi, M., and Varma, R. S. (2021). Antibacterial and Wound Healing Activities of Silver Nanoparticles Embedded in Cellulose Compared to Other Polysaccharides and Protein Polymers. Cellulose 28, 8295–8311. doi:10.1007/s10570-021-04067-3
Andleeb, A., Andleeb, A., Asghar, S., Zaman, G., Tariq, M., Mehmood, A., et al. (2021). A Systematic Review of Biosynthesized Metallic Nanoparticles as a Promising Anti-cancer-strategy. Cancers 13, 2818. doi:10.3390/cancers13112818
Anitha, M., Monisha, D. M., Sulthan, A. M., and Pandurangan, S. (2016). Emergence and Prevalence of Acinetobacter Baumannii in Tertiary Care Hospital Settings. Sch. Acad. J. Biosci. 4, 335–341.
Arnold, A. E., and Lutzoni, F. (2007). Diversity and Host Range of Foliar Fungal Endophytes: Are Tropical Leaves Biodiversity Hotspots? Ecology 88, 541–549. doi:10.1890/05-1459
Arya, S., Sonawane, H., Math, S., Tambade, P., Chaskar, M., and Shinde, D. (2021). Biogenic Titanium Nanoparticles (TiO2NPs) from Tricoderma Citrinoviride Extract: Synthesis, Characterization and Antibacterial Activity against Extremely Drug-Resistant Pseudomonas aeruginosa. Int. Nano Lett. 11, 35–42. doi:10.1007/s40089-020-00320-y
Assis, M., Groppo Filho, F. C., Pimentel, D. S., Robeldo, T., Gouveia, A. F., Castro, T. F. D., et al. (2020). Ag Nanoparticles/AgX (X=Cl, Br and I) Composites with Enhanced Photocatalytic Activity and Low Toxicological Effects. ChemistrySelect 5, 4655–4673. doi:10.1002/slct.202000502
Attarilar, S., Yang, J., Ebrahimi, M., Wang, Q., Liu, J., Tang, Y., et al. (2020). The Toxicity Phenomenon and the Related Occurrence in Metal and Metal Oxide Nanoparticles: A Brief Review from the Biomedical Perspective. Front. Bioeng. Biotechnol. 8, 822. doi:10.3389/fbioe.2020.00822
B, H., C P, C., M, G., N, C., Ganganagappa, N., and Yl, R. (2017). Green Synthesis of Silver Nanoparticles from Endophytic Fungus Aspergillus niger Isolated from Simarouba Glauca Leaf and its Antibacterial and Antioxidant Activity. Ijera 07, 17–24. doi:10.9790/9622-0708011724
Bagur, H., Medidi, R. S., Somu, P., Choudhury, P. W. J., karua, C. S., Guttula, P. K., et al. (2020a). Endophyte Fungal Isolate Mediated Biogenic Synthesis and Evaluation of Biomedical Applications of Silver Nanoparticles. Mater. Tech. 1, 1–12. doi:10.1080/10667857.2020.1819089
Bagur, H., Poojari, C. C., Melappa, G., Rangappa, R., Chandrasekhar, N., and Somu, P. (2020b). Biogenically Synthesized Silver Nanoparticles Using Endophyte Fungal Extract of Ocimum Tenuiflorum and Evaluation of Biomedical Properties. J. Clust. Sci. 31, 1241–1255. doi:10.1007/s10876-019-01731-4
Balakumaran, M. D., Ramachandran, R., and Kalaichelvan, P. T. (2015). Exploitation of Endophytic Fungus, Guignardia Mangiferae for Extracellular Synthesis of Silver Nanoparticles and Their In Vitro Biological Activities. Microbiol. Res. 178, 9–17. doi:10.1016/j.micres.2015.05.009
Bankier, C., Matharu, R. K., Cheong, Y. K., Ren, G. G., Cloutman-Green, E., and Ciric, L. (2019). Synergistic Antibacterial Effects of Metallic Nanoparticle Combinations. Sci. Rep. 9, 16074. doi:10.1038/s41598-019-52473-2
Barabadi, H., Honary, S., Ebrahimi, P., Mohammadi, M. A., Alizadeh, A., and Naghibi, F. (2014). Microbial Mediated Preparation, Characterization and Optimization of Gold Nanoparticles. Braz. J. Microbiol. 45, 1493–1501. doi:10.1590/s1517-83822014000400046
Barata-Silva, C., Santos, L. M. G., Neto, S. A. V., Magalhães, C. D., Jacob, S. C., and Moreira, J. C. (2021). Nano Drugs: Regulation and Quality Control. Vigil. Sanit. Debate. 9, 138–151. doi:10.22239/2317-269x.01765
Bedlovičová, Z., Strapáč, I., Baláž, M., and Salayová, A. (2020). A Brief Overview on Antioxidant Activity Determination of Silver Nanoparticles. Molecules 25, 3191. doi:10.3390/molecules25143191
Bethu, V., Pushpalatha, M., Baki, V. B., Aishwarya, V., Rao, J. V., et al. (2016a). Biogenesis of Silver Nanoparticles Using Endophytic Fungus Pestalotiopsis Microspora and Evaluation of Their Antioxidant and Anticancer Activities. Ijn 11, 5683–5696. doi:10.2147/IJN.S112857
Bundschuh, M., Filser, J., Lüderwald, S., McKee, M. S., Metreveli, G., Schaumann, G. E., et al. (2018). Nanoparticles in the Environment: where Do We Come from, where Do We Go to? Environ. Sci. Eur. 30, 6. doi:10.1186/s12302-018-0132-6
Busi, S., and Rajkumari, J. (2019). Microbially Synthesized Nanoparticles as Next Generation Antimicrobials: Scope and Applications. Nanoparticles Pharm., 485–524. doi:10.1016/B978-0-12-816504-1.00008-9
Buzea, C., Pacheco, I. I., and Robbie, K. (2007). Nanomaterials and Nanoparticles: Sources and Toxicity. Biointerphases 2, MR17–MR71. doi:10.1116/1.2815690
Chandankere, R., Chelliah, J., Subban, K., Shanadrahalli, V. C., Parvez, A., Zabed, H. M., et al. (2020). Pleiotropic Functions and Biological Potentials of Silver Nanoparticles Synthesized by an Endophytic Fungus. Front. Bioeng. Biotechnol. 8, 95. doi:10.3389/fbioe.2020.00095
Choi, H. S., and Frangioni, J. V. (2010). Nanoparticles for Biomedical Imaging: Fundamentals of Clinical Translation. Mol. Imaging 9, 7290.2010.00031–310. doi:10.2310/7290.2010.00031
Chowdhury, D. R., Chatterjee, S. K., and Kanti Roy, S. (2016). Studies on Endophytic Fungi of Calotropis Procera (L.) R.Br. With A View to Their Antimicrobial and Antioxidant Actvities Mediated by Extracellular Synthesised Silver Nanoparticles. Iosr 11, 113–121. doi:10.9790/3008-110502113121
Clarance, P., Luvankar, B., Sales, J., Khusro, A., Agastian, P., Tack, J.-C., et al. (2020). Green Synthesis and Characterization of Gold Nanoparticles Using Endophytic Fungi Fusarium Solani and its In-Vitro Anticancer and Biomedical Applications. Saudi J. Biol. Sci. 27, 706–712. doi:10.1016/j.sjbs.2019.12.026
Danagoudar, A., G K, M., Chatterjee, B., Ghosh, K., Kanade, S. R., and Joshi, C. G. (2020). Cancer Cell Specific Cytotoxic Potential of the Silver Nanoparticles Synthesized Using the Endophytic Fungus, Penicillium citrinum CGJ-C2. Mater. Today Commun. 25, 101442. doi:10.1016/j.mtcomm.2020.101442
De Matteis, V. (2017). Exposure to Inorganic Nanoparticles: Routes of Entry, Immune Response, Biodistribution and In Vitro/In Vivo Toxicity Evaluation. Toxics 5, 29. doi:10.3390/toxics5040029
Devi, L. S., Bareh, D. A., and Joshi, S. R. (2014). Studies on Biosynthesis of Antimicrobial Silver Nanoparticles Using Endophytic Fungi Isolated from the Ethno-Medicinal Plant Gloriosa Superba L. Proc. Natl. Acad. Sci. India, Sect. B Biol. Sci. 84, 1091–1099. doi:10.1007/s40011-013-0185-7
Dikshit, P., Kumar, J., Das, A., Sadhu, S., Sharma, S., Singh, S., et al. (2021). Green Synthesis of Metallic Nanoparticles: Applications and Limitations. Catalysts 11, 902. doi:10.3390/catal11080902
Dong, Z.-Y., Narsing Rao, M. P., Xiao, M., Wang, H.-F., Hozzein, W. N., Chen, W., et al. (2017). Antibacterial Activity of Silver Nanoparticles against Staphylococcus Warneri Synthesized Using Endophytic Bacteria by Photo-Irradiation. Front. Microbiol. 8, 1090. doi:10.3389/fmicb.2017.01090
Đorđević, S., Gonzalez, M. M., Conejos-Sánchez, I., Carreira, B., Pozzo, S., Acúrcio, R. C., et al. (2021). Current Hurdles to the Translation of Nanomedicines from Bench to the Clinic. Drug Deliv. Transl. Res. doi:10.1007/s13346-021-01024-2
Dorotkiewicz-Jach, A., Augustyniak, D., Olszak, T., and Drulis-Kawa, Z. (2015). Modern Therapeutic Approaches against Pseudomonas aeruginosa Infections. Cmc 22, 1642–1664. doi:10.2174/0929867322666150417122531
Durán, N., Marcato, P. D., Alves, O. L., De Souza, G. I., and Esposito, E. (2005). Mechanistic Aspects of Biosynthesis of Silver Nanoparticles by Several Fusarium Oxysporum Strains. J. Nanobiotechnol 3, 8. doi:10.1186/1477-3155-3-8
Eid, A. M., Fouda, A., Niedbała, G., Hassan, S. E.-D., Salem, S. S., Abdo, A. M., et al. (2020). Endophytic Streptomyces Laurentii Mediated Green Synthesis of Ag-NPs with Antibacterial and Anticancer Properties for Developing Functional Textile Fabric Properties. Antibiotics 9, 641. doi:10.3390/antibiotics9100641
El-Sonbaty, S. M. (2013). Fungus-mediated Synthesis of Silver Nanoparticles and Evaluation of Antitumor Activity. Cancer Nano 4, 73–79. doi:10.1007/s12645-013-0038-3
Fadiji, A. E., and Babalola, O. O. (2020). Elucidating Mechanisms of Endophytes Used in Plant Protection and Other Bioactivities with Multifunctional Prospects. Front. Bioeng. Biotechnol. 8, 467. doi:10.3389/fbioe.2020.00467
Foulkes, R., Man, E., Thind, J., Yeung, S., Joy, A., and Hoskins, C. (2020). The Regulation of Nanomaterials and Nanomedicines for Clinical Application: Current and Future Perspectives. Biomater. Sci. 8, 4653–4664. doi:10.1039/d0bm00558d
Franco-Franklin, V., Moreno-Riascos, S., and Ghneim-Herrera, T. (2021). Are Endophytic Bacteria an Option for Increasing Heavy Metal Tolerance of Plants? A Meta-Analysis of the Effect Size. Front. Environ. Sci. 8, 294. doi:10.3389/fenvs.2020.603668
Ganesan, V., Hariram, M., Vivekanandhan, S., and Muthuramkumar, S. (2020). Periconium Sp. (Endophytic Fungi) Extract Mediated Sol-Gel Synthesis of ZnO Nanoparticles for Antimicrobial and Antioxidant Applications. Mater. Sci. Semiconductor Process. 105, 104739. doi:10.1016/j.mssp.2019.104739
Garcia, E. J., Oldoni, T. L. C., Alencar, S. M. d., Reis, A., Loguercio, A. D., and Grande, R. H. M. (2012). Antioxidant Activity by DPPH Assay of Potential Solutions to Be Applied on Bleached Teeth. Braz. Dent. J. 23, 22–27. doi:10.1590/S0103-64402012000100004
Gond, S. K., Mishra, A., Verma, S. K., Sharma, V. K., and Kharwar, R. N. (2019). Synthesis and Characterization of Antimicrobial Silver Nanoparticles by an Endophytic Fungus Isolated from Nyctanthes Arbor-tristis. Proc. Natl. Acad. Sci. India, Sect. B Biol. Sci. 90, 641–645. doi:10.1007/s40011-019-01137-2
Govindappa, M., Farheen, H., Chandrappa, C. P., ChannabasavaRai, R. V., Rai, R. V., and Raghavendra, V. B. (2016). Mycosynthesis of Silver Nanoparticles Using Extract of Endophytic Fungi, Penicillium Species of Glycosmis Mauritiana , and its Antioxidant, Antimicrobial, Anti-inflammatory and Tyrokinase Inhibitory Activity. Adv. Nat. Sci. Nanosci. Nanotechnol. 7, 035014. doi:10.1088/2043-6262/7/3/035014
Govindappa, M., Lavanya, M., Aishwarya, P., Pai, K., Lunked, P., Hemashekhar, B., et al. (2020). Synthesis and Characterization of Endophytic Fungi, Cladosporium Perangustum Mediated Silver Nanoparticles and Their Antioxidant, Anticancer and Nano-Toxicological Study. BioNanoSci. 10, 928–941. doi:10.1007/s12668-020-00719-z
Gulcin, İ. (2020). Antioxidants and Antioxidant Methods: an Updated Overview. Arch. Toxicol. 94, 651–715. doi:10.1007/s00204-020-02689-3
Gupta, M., and Shukla, K. K. (2020). “Endophytic Fungi: A Treasure Trove of Novel Bioactive Compounds,” in Bioactive Natural Products in Drug Discovery. Editors J. Singh, V. Meshram, and M. Gupta (Singapore: Springer Singapore), 427–449. doi:10.1007/978-981-15-1394-7_14
Halkai, K., Mudda, J., Shivanna, V., Rathod, V., and Halkai, R. (2018). Antibacterial Efficacy of Biosynthesized Silver Nanoparticles against Enterococcus faecalis Biofilm: An In Vitro Study. Contemp. Clin. Dent. 9, 237–241. doi:10.4103/ccd.ccd_828_17
Hallmann, J., Quadt-Hallmann, A., Mahaffee, W. F., and Kloepper, J. W. (1997). Bacterial Endophytes in Agricultural Crops. Can. J. Microbiol. 43, 895–914. doi:10.1139/m97-131
Hassan, S. E.-D., Salem, S. S., Fouda, A., Awad, M. A., El-Gamal, M. S., and Abdo, A. M. (2018). New Approach for Antimicrobial Activity and Bio-Control of Various Pathogens by Biosynthesized Copper Nanoparticles Using Endophytic Actinomycetes. J. Radiat. Res. Appl. Sci. 11, 262–270. doi:10.1016/j.jrras.2018.05.003
Haynes, K. M., Abdali, N., Jhawar, V., Zgurskaya, H. I., Parks, J. M., Green, A. T., et al. (2017). Identification and Structure-Activity Relationships of Novel Compounds that Potentiate the Activities of Antibiotics in Escherichia coli. J. Med. Chem. 60, 6205–6219. doi:10.1021/acs.jmedchem.7b00453
Hemashekhar, B., Chandrappa, C. P., Govindappa, M., and Chandrashekar, N. (2019). Endophytic Fungus Alternaria Spp Isolated from Rauvolfia Tetraphylla Root Arbitrate Synthesis of Gold Nanoparticles and Evaluation of Their Antibacterial, Antioxidant and Antimitotic Activities. Adv. Nat. Sci. Nanosci. Nanotechnol. 10, 035010. doi:10.1088/2043-6254/ab38b0
Hemmati, F., Rezaee, M. A., Ebrahimzadeh, S., Yousefi, L., Nouri, R., Kafil, H. S., et al. (2021). Novel Strategies to Combat Bacterial Biofilms. Mol. Biotechnol. 63, 569–586. doi:10.1007/s12033-021-00325-8
H., L., L.A., M., B., B., Johann, S., M.A., T., L., C., et al. (2011). “Endophytic Fungi of Tropical Forests: A Promising Source of Bioactive Prototype Molecules for the Treatment of Neglected Diseases,” in ” in Drug Development - A Case Study Based Insight Into Modern Strategies. Editor C. Rundfeldt (Rijeka: IntechOpen), 469–486. doi:10.5772/27783
Hua, S., de Matos, M. B. C., Metselaar, J. M., and Storm, G. (2018). Current Trends and Challenges in the Clinical Translation of Nanoparticulate Nanomedicines: Pathways for Translational Development and Commercialization. Front. Pharmacol. 9, 790. doi:10.3389/fphar.2018.00790
Hulkoti, N. I., and Taranath, T. C. (2014). Biosynthesis of Nanoparticles Using Microbes-A Review. Colloids Surf. B: Biointerfaces 121, 474–483. doi:10.1016/j.colsurfb.2014.05.027
Jamuna, B. A., and Ravishankar, R. V. (2014). Environmental Risk, Human Health, and Toxic Effects of Nanoparticles. Nanomater. Environ. Prot., 523–535. doi:10.1002/9781118845530.ch31
Katranidis, A., and Choli-Papadopoulou, T. (2012). “Biofunctionalization of Surfaces with Peptides, Proteins, or Subcellular Organelles: Single-Molecule Studies and Nanomedical Approach,” in Nanomedicine and Nanobiotechnology. Editor S. Logothetidis (Berlin, Heidelberg: Springer Berlin Heidelberg), 57–68. doi:10.1007/978-3-642-24181-9_3
Knetsch, M. L. W., and Koole, L. H. (2011). New Strategies in the Development of Antimicrobial Coatings: The Example of Increasing Usage of Silver and Silver Nanoparticles. Polymers 3, 340–366. doi:10.3390/polym3010340
Kulkarni, P., and Ramakrishna, K. (2020). Biosynthesis of Zinc Oxid Nanoparticles by Endophytic Fungi Aspergillus niger and Their Potential Antibacterial Effects on Propionibacterium Acnes. J. Adv. Sci. Res. 11, 178–183.
Lee, C.-R., Lee, J. H., Park, M., Park, K. S., Bae, I. K., Kim, Y. B., et al. (2017). Biology of Acinetobacter Baumannii: Pathogenesis, Antibiotic Resistance Mechanisms, and Prospective Treatment Options. Front. Cel. Infect. Microbiol. 7, 55. doi:10.3389/fcimb.2017.00055
Mani, V. M., Kalaivani, S., Sabarathinam, S., Vasuki, M., Soundari, A. J. P. G., Ayyappa Das, M. P., et al. (2021). Copper Oxide Nanoparticles Synthesized from an Endophytic Fungus Aspergillus terreus: Bioactivity and Anti-cancer Evaluations. Environ. Res. 201, 111502. doi:10.1016/j.envres.2021.111502
Manjunath, M. H., Joshi, C. G., Danagoudar, A., Poyya, J., Kudva, A. K., and Dhananjaya, B. L. (2017). Biogenic Synthesis of Gold Nanoparticles by Marine Endophytic Fungus-Cladosporium Cladosporioides Isolated from Seaweed and Evaluation of Their Antioxidant and Antimicrobial Properties. Process Biochem. 63, 137–144. doi:10.1016/j.procbio.2017.09.008
Manjunath Hulikere, M., and Joshi, C. G. (2019). Characterization, Antioxidant and Antimicrobial Activity of Silver Nanoparticles Synthesized Using marine Endophytic Fungus- Cladosporium Cladosporioides. Process Biochem. 82, 199–204. doi:10.1016/j.procbio.2019.04.011
Messaoudi, O., and Bendahou, M. (2020). “Biological Synthesis of Nanoparticles Using Endophytic Microorganisms: Current Development,” in “Biological Synthesis of Nanoparticles Using Endophytic Microorganisms: Current Development” in Nanotechnology And the Environment. Editor M. Sen (Rijeka: IntechOpen), 1–19. doi:10.5772/intechopen.93734
Mohamed, N. H., Ismail, M. A., Abdel-Mageed, W. M., and Mohamed Shoreit, A. A. (2019). Antimicrobial Activity of green Silver Nanoparticles from Endophytic Fungi Isolated from Calotropis Procera (Ait) Latex. Microbiology 165, 967–975. doi:10.1099/mic.0.000832
Morais, E. d. S., Holanda, F. H., Ferreira, B. L., Sena, I. S., Lima, A. L., Marinho, V. H. d. S., et al. (2021). “nanopartículas de cobre biossintetizadas pelo fungo endofítico phaeoacremonium sp. Isolado das amêndoas de bertholletia excelsa ducke,” in Trabalhos nas Areas de Fronteira da Química. Editor A. C. Oliveira (Ponta Grossa: Atena Editora), 99–111. doi:10.22533/at.ed.2432122028
Mousa, S. A., El-Sayed, E.-S. R., Mohamed, S. S., Abo El-Seoud, M. A., Elmehlawy, A. A., and Abdou, D. A. M. (2021). Novel Mycosynthesis of Co3O4, CuO, Fe3O4, NiO, and ZnO Nanoparticles by the Endophytic Aspergillus terreus and Evaluation of Their Antioxidant and Antimicrobial Activities. Appl. Microbiol. Biotechnol. 105, 741–753. doi:10.1007/s00253-020-11046-4
Munawer, U., Raghavendra, V. B., Ningaraju, S., Krishna, K. L., Ghosh, A. R., Melappa, G., et al. (2020). Biofabrication of Gold Nanoparticles Mediated by the Endophytic Cladosporium Species: Photodegradation, In Vitro Anticancer Activity and In Vivo Antitumor Studies. Int. J. Pharmaceutics 588, 119729. doi:10.1016/j.ijpharm.2020.119729
Nafeh, A., and Mazhar, S. (2021). Endophytic Nanoparticles: toward a New Therapeutic Future. J. Microbiol. Mol. Gen. 2. doi:10.52700/jmmg.v2i1.23
Navya, P. N., and Daima, H. K. (2016). Rational Engineering of Physicochemical Properties of Nanomaterials for Biomedical Applications with Nanotoxicological Perspectives. Nano Convergence 3, 1. doi:10.1186/s40580-016-0064-z
Neethu, S., Midhun, S. J., Radhakrishnan, E. K., and Jyothis, M. (2018). Green Synthesized Silver Nanoparticles by marine Endophytic Fungus Penicillium polonicum and its Antibacterial Efficacy against Biofilm Forming, Multidrug-Resistant Acinetobacter Baumanii. Microb. Pathogenesis 116, 263–272. doi:10.1016/j.micpath.2018.01.033
Neethu, S., Midhun, S. J., Radhakrishnan, E. K., and Jyothis, M. (2020). Surface Functionalization of central Venous Catheter with Mycofabricated Silver Nanoparticles and its Antibiofilm Activity on Multidrug Resistant Acinetobacter Baumannii. Microb. Pathogenesis 138, 103832. doi:10.1016/j.micpath.2019.103832
Neha Desai, N., Momin, M., Khan, T., Gharat, S., Ningthoujam, R. S., and Omri, A. (2021). Metallic Nanoparticles as Drug Delivery System for the Treatment of Cancer. Expert Opin. Drug Deliv. 18, 1261–1290. doi:10.1080/17425247.2021.1912008
Netala, V. R., Bethu, M. S., Pushpalatha, B., Baki, V. B., Aishwarya, S., Rao, J. V., et al. (2016). Biogenesis of Silver Nanoparticles Using Endophytic Fungus Pestalotiopsis microspora and Evaluation of Their Antioxidant and Anticancer Activities. Int. J. Nanomedicine 11, 5683–5696. doi:10.2147/IJN.S112857
Nirmala, C., and Sridevi, M. (2021). Characterization, Antimicrobial and Antioxidant Evaluation of Biofabricated Silver Nanoparticles from Endophytic Pantoea Anthophila. J. Inorg. Organomet. Polym. 31, 3711–3725. doi:10.1007/s10904-021-01974-7
Ovais, M., Khalil, A., Ayaz, M., Ahmad, I., Nethi, S., and Mukherjee, S. (2018). Biosynthesis of Metal Nanoparticles via Microbial Enzymes: A Mechanistic Approach. Ijms 19, 4100. doi:10.3390/ijms19124100
Page, M. G., and Heim, J. (2009). Prospects for the Next Anti-Pseudomonas Drug. Curr. Opin. Pharmacol. 9, 558–565. doi:10.1016/j.coph.2009.08.006
Pal, G., Rai, P., and Pandey, A. (2019). “Green Synthesis of Nanoparticles: A Greener Approach for a Cleaner Future,” in “Chapter 1 - Green Synthesis of Nanoparticles: A Greener Approach for a Cleaner Future,” in Micro And Nano Technologies. Editors A. K. Shukla,, and S. B. T.-G. S. Iravani (Elsevier), 1–26. doi:10.1016/B978-0-08-102579-6.00001-0
Panda, P. K., Verma, S. K., and Suar, M. (2021). Nanoparticle-biological Interactions: the Renaissance of Bionomics in the Myriad Nanomedical Technologies. Nanomedicine 16, 2249–2254. doi:10.2217/nnm-2021-0174
Pandey, R. P., Mukherjee, R., Priyadarshini, A., Gupta, A., Vibhuti, A., Leal, E., et al. (2021). Potential of Nanoparticles Encapsulated Drugs for Possible Inhibition of the Antimicrobial Resistance Development. Biomed. Pharmacother. 141, 111943. doi:10.1016/j.biopha.2021.111943
Patel, S., and Nanda, R. (2015). Nanotechnology in Healthcare: Applications and Challenges. Med. Chem. 05, 528–533. doi:10.4172/2161-0444.1000312
Patra, J. K., and Baek, K.-H. (2014). Green Nanobiotechnology: Factors Affecting Synthesis and Characterization Techniques. J. Nanomater. 2014, 1–12. doi:10.1155/2014/417305
Pereira, J. O., de Souza, A. Q. L., de Souza, A. D. L., de Castro França, S., and de Oliveira, L. A. (2017). “Overview on Biodiversity, Chemistry, and Biotechnological Potential of Microorganisms from the Brazilian Amazon,” in Diversity and Benefits of Microorganisms from the Tropics. Editors J. L. de Azevedo, and M. C. Quecine (Cham: Springer International Publishing), 71–103. doi:10.1007/978-3-319-55804-2_5
Petrini, O. (1991). “Fungal Endophytes of Tree Leaves,” in Microbial Ecology of Leaves. Editors J. H. Andrews, and S. S. Hirano (New York, NY: Springer New York), 179–197. doi:10.1007/978-1-4612-3168-4_9
Raffa, V., Vittorio, O., Riggio, C., and Cuschieri, A. (2010). Progress in Nanotechnology for Healthcare. Minimally Invasive Ther. Allied Tech. 19, 127–135. doi:10.3109/13645706.2010.481095
Rahman, M. M., Islam, M. B., Biswas, M., and Khurshid Alam, A. H. M. (2015). In Vitro antioxidant and Free Radical Scavenging Activity of Different Parts of Tabebuia Pallida Growing in Bangladesh. BMC Res. Notes 8, 621. doi:10.1186/s13104-015-1618-6
Rahman, S., Rahman, L., Khalil, A. T., Ali, N., Zia, D., Ali, M., et al. (2019). Endophyte-mediated Synthesis of Silver Nanoparticles and Their Biological Applications. Appl. Microbiol. Biotechnol. 103, 2551–2569. doi:10.1007/s00253-019-09661-x
Ramos, M. M., dos S. Morais, E., da S. Sena, I., Lima, A. L., de Oliveira, F. R., de Freitas, C. M., et al. (2020). Silver Nanoparticle from Whole Cells of the Fungi Trichoderma Spp. Isolated from Brazilian Amazon. Biotechnol. Lett. 42, 833–843. doi:10.1007/s10529-020-02819-y
Rana, K. L., Kour, D., Yadav, N., and Yadav, A. N. (2020). “Endophytic Microbes in Nanotechnology: Current Development, and Potential Biotechnology Applications,” in ” in Woodhead Publishing Series In Food Science, Technology And Nutrition. Editors A. Kumar, and V. K. Singh (Cambridge, United Kingdom: Woodhead Publishing), 231–262. doi:10.1016/B978-0-12-818734-0.00010-3
Rasool, U., and Hemalatha, S. (2017). Marine Endophytic Actinomycetes Assisted Synthesis of Copper Nanoparticles (CuNPs): Characterization and Antibacterial Efficacy against Human Pathogens. Mater. Lett. 194, 176–180. doi:10.1016/j.matlet.2017.02.055
Ratti, R. P., Serrano, N. F. G., Hokka, C. O., and Sousa, C. P. (2008). Antagonistic Properties of Some Microorganisms Isolated from Brazilian Tropical savannah Plants against Staphylococcus Coagulase-Positive Strain. J. Venom. Anim. Toxins Incl. Trop. Dis. 14, 294–302. doi:10.1590/s1678-91992008000200007
Rehman, S., Ansari, M. A., Al-Dossary, H. A., Fatima, Z., Hameed, S., Ahmad, W., et al. (2021). “Current Perspectives on Mycosynthesis of Nanoparticles and Their Biomedical Application,” in Modeling and Control of Drug Delivery Systems. Editor A. T. Azar (Academic Press), 301–311. doi:10.1016/B978-0-12-821185-4.00005-1
Reid, G. (1999). Biofilms in Infectious Disease and on Medical Devices. Int. J. Antimicrob. Agents 11, 223–226. doi:10.1016/S0924-8579(99)00020-5
Rezvani, E., Rafferty, A., McGuinness, C., and Kennedy, J. (2019). Adverse Effects of Nanosilver on Human Health and the Environment. Acta Biomater. 94, 145–159. doi:10.1016/j.actbio.2019.05.042
Rodrigues, A. G., Ping, L. Y., Marcato, P. D., Alves, O. L., Silva, M. C. P., Ruiz, R. C., et al. (2013). Biogenic Antimicrobial Silver Nanoparticles Produced by Fungi. Appl. Microbiol. Biotechnol. 97, 775–782. doi:10.1007/s00253-012-4209-7
Romano, L. H. (2014). Bioprospecção de microrganismos endofíticos isolados de Tabebuia spp. e Hymenaea courbaril e identificação da produção de metabólitos de interesse biotecnológico. [thesis]. [São Carlos (SP)]. São Carlos, (Brazil): Federal University of São Carlos.
Roy, S., and Banerjee, D. (2018, Diversity of Endophytes in Tropical Forests). “Diversity of Endophytes in Tropical Forests BT - Endophytes of Forest Trees: Biology and Applications,” in, eds. A. M. Pirttilä, and A. C. Frank (Cham: Springer International Publishing), 43–62. doi:10.1007/978-3-319-89833-9_3
S, R., M, S. A., Adnan, M., N, S. K., K, R., and S, H. (2021). Synthesis, Characterization and Applications of Endophytic Fungal Nanoparticles. Inorg. Nano-Metal Chem. 51, 280–287. doi:10.1080/24701556.2020.1784231
Salesa, B., Assis, M., Andrés, J., and Serrano-Aroca, Á. (2021). Carbon Nanofibers versus Silver Nanoparticles: Time-dependent Cytotoxicity, Proliferation, and Gene Expression. Biomedicines 9, 1155. doi:10.3390/biomedicines9091155
Satisha, S., Syed, B., and Prasad, N. N. (2016). Endogenic Mediated Synthesis of Gold Nanoparticles Bearing Bactericidal Activity. J. Microsc. Ultrastruct. 4, 162–166. doi:10.1016/j.jmau.2016.01.004
Sayadi, M. H., Mansouri, B., Shahri, E., Tyler, C. R., Shekari, H., and Kharkan, J. (2020). Exposure Effects of Iron Oxide Nanoparticles and Iron Salts in Blackfish (Capoeta Fusca): Acute Toxicity, Bioaccumulation, Depuration, and Tissue Histopathology. Chemosphere 247, 125900. doi:10.1016/j.chemosphere10.1016/j.chemosphere.2020.125900
Sebastianes, F. L. S., de Azevedo, J. L., and Lacava, P. T. (2017). “Diversity and Biotechnological Potential of Endophytic Microorganisms Associated with Tropical Mangrove Forests,” in Diversity and Benefits of Microorganisms from the Tropics. Editors J. L. de Azevedo, and M. C. Quecine (Cham: Springer International Publishing), 37–56. doi:10.1007/978-3-319-55804-2_3
Seetharaman, P. K., Chandrasekaran, R., Periakaruppan, R., Gnanasekar, S., Sivaperumal, S., Abd-Elsalam, K. A., et al. (2021). Functional Attributes of Myco-Synthesized Silver Nanoparticles from Endophytic Fungi: A New Implication in Biomedical Applications. Biology 10, 473. doi:10.3390/biology10060473
Shaikh, S., Nazam, N., Rizvi, S. M. D., Ahmad, K., Baig, M. H., Lee, E. J., et al. (2019). Mechanistic Insights into the Antimicrobial Actions of Metallic Nanoparticles and Their Implications for Multidrug Resistance. Ijms 20, 2468. doi:10.3390/ijms20102468
Shariq Ahmed, M., Soundhararajan, R., Akther, T., Kashif, M., Khan, J., Waseem, M., et al. (2019). Biogenic AgNPs Synthesized via Endophytic Bacteria and its Biological Applications. Environ. Sci. Pollut. Res. 26, 26939–26946. doi:10.1007/s11356-019-05869-6
Singh, D., Rathod, V., Ninganagouda, S., Hiremath, J., Singh, A. K., and Mathew, J. (2014). Optimization and Characterization of Silver Nanoparticle by Endophytic FungiPenicilliumsp. Isolated fromCurcuma longa(Turmeric) and Application Studies against MDRE. coliandS. Aureus. Bioinorganic Chem. Appl. 2014, 1–8. doi:10.1155/2014/408021
Singh, T., Jyoti, K., Patnaik, A., Singh, A., Chauhan, R., and Chandel, S. S. (2017). Biosynthesis, Characterization and Antibacterial Activity of Silver Nanoparticles Using an Endophytic Fungal Supernatant of Raphanus Sativus. J. Genet. Eng. Biotechnol. 15, 31–39. doi:10.1016/j.jgeb.2017.04.005
Soares, S., Sousa, J., Pais, A., and Vitorino, C. (2018). Nanomedicine: Principles, Properties, and Regulatory Issues. Front. Chem. 6, 360. doi:10.3389/fchem.2018.00360
Sousa, C. P., Carvalho, C. M., and Kurachi, C. (2021). Método para biossíntese de nanopartículas de ouro por fitobactérias endofíticas. BR. Patent No 10202100836. Brazil: INPI - Instituto Nacional da Propriedade Industrial.
Sudarsan, S., Kumar Shankar, M., Kumar Belagal Motatis, A., Shankar, S., Krishnappa, D., Mohan, C. D., et al. (2021). Green Synthesis of Silver Nanoparticles by Cytobacillus Firmus Isolated from the Stem Bark of Terminalia Arjuna and Their Antimicrobial Activity. Biomolecules 11, 259. doi:10.3390/biom11020259
Sumanth, B., Lakshmeesha, T. R., Ansari, M. A., Alzohairy, M. A., Udayashankar, A. C., Shobha, B., et al. (2020). Mycogenic Synthesis of Extracellular Zinc Oxide Nanoparticles from Xylaria Acuta and its Nanoantibiotic Potential. Ijn 15, 8519–8536. doi:10.2147/IJN.S271743
Sunkar, S., and Nachiyar, C. V. (2012). Biogenesis of Antibacterial Silver Nanoparticles Using the Endophytic Bacterium Bacillus Cereus Isolated from Garcinia Xanthochymus. Asian Pac. J. Trop. Biomed. 2, 953–959. doi:10.1016/S2221-1691(13)60006-4
Taran, M., Rad, M., and Alavi, M. (2017). Antibacterial Activity of Copper Oxide (CuO) Nanoparticles Biosynthesized by Bacillus Sp. FU4: Optimization of Experiment Design. Pharm. Sci. 23, 198–206. doi:10.15171/PS.2017.30
Tortella, G. R., Rubilar, O., Durán, N., Diez, M. C., Martínez, M., Parada, J., et al. (2020). Silver Nanoparticles: Toxicity in Model Organisms as an Overview of its hazard for Human Health and the Environment. J. Hazard. Mater. 390, 121974. doi:10.1016/j.jhazmat.2019.121974
Tortella, G., Rubilar, O., Fincheira, P., Pieretti, J. C., Duran, P., Lourenço, I. M., et al. (2021). Bactericidal and Virucidal Activities of Biogenic Metal-Based Nanoparticles: Advances and Perspectives. Antibiotics 10, 783. doi:10.3390/antibiotics10070783
Tortora, G. J., Funke, B. R., and Case, C. L. (2016). Microbiology: An Introduction. 12th ed. Boston, USA: Pearson.
Uddandarao, P., Balakrishnan, R. M., Ashok, A., Swarup, S., and Sinha, P. (2019). Bioinspired ZnS:Gd Nanoparticles Synthesized from an Endophytic Fungi Aspergillus flavus for Fluorescence-Based Metal Detection. Biomimetics 4, 11. doi:10.3390/biomimetics4010011
Uddandarao, P. (2016). ZnS Semiconductor Quantum Dots Production by an Endophytic Fungus Aspergillus flavus. Mater. Sci. Eng. B 207, 26–32. doi:10.1016/j.mseb.2016.01.013
Verma, V. C., Singh, S. K., Solanki, R., and Prakash, S. (2010). Biofabrication of Anisotropic Gold Nanotriangles Using Extract of Endophytic Aspergillus clavatus as a Dual Functional Reductant and Stabilizer. Nanoscale Res. Lett. 6, 16. doi:10.1007/s11671-010-9743-6
Vijayanandan, A. S., and Balakrishnan, R. M. (2018). Biosynthesis of Cobalt Oxide Nanoparticles Using Endophytic Fungus Aspergillus nidulans. J. Environ. Manage. 218, 442–450. doi:10.1016/j.jenvman.2018.04.032
Wang, L., Hu, C., and Shao, L. (2017). The Antimicrobial Activity of Nanoparticles: Present Situation and Prospects for the Future. Int. J. Nanomedicine 12, 1227–1249. doi:10.2147/IJN.S121956
Wong, K. K. Y., Cheung, S. O. F., Huang, L., Niu, J., Tao, C., Ho, C.-M., et al. (2009). Further Evidence of the Anti-inflammatory Effects of Silver Nanoparticles. ChemMedChem 4, 1129–1135. doi:10.1002/cmdc.200900049
Xie, R., Zhang, X. D., Zhao, Q., Peng, B., and Zheng, J. (2018). Analysis of Global Prevalence of Antibiotic Resistance in Acinetobacter Baumannii Infections Disclosed a Faster Increase in OECD Countries. Emerg. Microbes Infect. 7, 1–10. doi:10.1038/s41426-018-0038-9
Xu, L., Wang, Y.-Y., Huang, J., Chen, C.-Y., Wang, Z.-X., and Xie, H. (2020). Silver Nanoparticles: Synthesis, Medical Applications and Biosafety. Theranostics 10, 8996–9031. doi:10.7150/thno.45413
Xu, R., Li, T., Cui, H., Wang, J., Yu, X., Ding, Y., et al. (2015). Diversity and Characterization of Cd-Tolerant Dark Septate Endophytes (DSEs) Associated with the Roots of Nepal Alder (Alnus Nepalensis) in a Metal Mine Tailing of Southwest China. Appl. Soil Ecol. 93, 11–18. doi:10.1016/j.apsoil.2015.03.013
Yang, B., Chen, Y., and Shi, J. (2019). Reactive Oxygen Species (ROS)-Based Nanomedicine. Chem. Rev. 119, 4881–4985. doi:10.1021/acs.chemrev.8b00626
Keywords: endophyte, tropics, bioactive compounds, green synthesis, nanoparticles, medicine
Citation: Bogas AC, Henrique Rodrigues S, Gonçalves MO, De Assis M, Longo E and Paiva De Sousa C (2022) Endophytic Microorganisms From the Tropics as Biofactories for the Synthesis of Metal-Based Nanoparticles: Healthcare Applications. Front. Nanotechnol. 4:823236. doi: 10.3389/fnano.2022.823236
Received: 26 November 2021; Accepted: 19 January 2022;
Published: 24 February 2022.
Edited by:
Vijayakumar Sekar, Shandong University, Weihai, ChinaReviewed by:
Suresh K. Verma, Uppsala University, SwedenSilvana Alborés, Universidad de la República, Uruguay
Di Huang, Massachusetts Eye & Ear Infirmary and Harvard Medical School, United States
Copyright © 2022 Bogas, Henrique Rodrigues, Gonçalves, De Assis, Longo and Paiva De Sousa. This is an open-access article distributed under the terms of the Creative Commons Attribution License (CC BY). The use, distribution or reproduction in other forums is permitted, provided the original author(s) and the copyright owner(s) are credited and that the original publication in this journal is cited, in accordance with accepted academic practice. No use, distribution or reproduction is permitted which does not comply with these terms.
*Correspondence: Andréa Cristina Bogas, acbogas@gmail.com