- 1Research Cell and Department of Physics, Bhagini Nivedita College, University of Delhi, New Delhi, India
- 2SUMAN Laboratory (SUstainable Materials and Advanced Nanotechnology), New Delhi, India
Current theranostics for neurodegenerative diseases (NDD) management are majorly symptomatic due to a lack of identification of early-stage biomarkers and the inefficiency of drugs to penetrate through the blood-brain barrier. Recently, the Neuro-nanotechnology interface has emerged as a potential strategy for diagnosis, monitoring, and treatment of NDDs owing to smaller particle size, high specific surface area, tunable physicochemical attributes and rich surface functionalities. However, toxicity and biocompatibility are two significant challenges restricting their commercial prospect in NDD management. On the contrary, green nanosystems fabricated using plant extracts, microorganisms, biome-based precursors, repurposed-byproducts, exosomes, and protein-based bio-nanomaterials are economical, eco-friendly, biocompatible and renewable due to the abundance of biodiversity. This prospect explores the novel and cutting-edge interface of green nanosystems and NDDs for developing diagnostic and implantable devices, targeted drug delivery strategies, surgical prostheses, therapeutics, treatment, nanoscaffolds for neurogeneration, and immunity development. Besides, it discusses the challenges, alternate solutions and advanced prospects of green nanosystems with the integration of modern-age technologies for the development of sustainable green Neuro-nanotechnology for efficient management of NDDs.
State-of-the-art neurodegenerative diseases theranostics: Limitations and challenges
The population affected with neurodegenerative diseases (NDDs), growing at the pace of tens of millions of individuals yearly, has been declared the most significant global health threat of the twenty-first century by World Health Organization (Hou et al., 2019; Holbrook et al., 2021). NDDs are characterized by the functional loss of brain neurons resulting in diversified clinical and pathological manifestations and vocal, motor, and cognitive dysfunctions. NDDs are a heterogeneous group of either hereditary or sporadic conditions, including Alzheimer’s disease, Amyotrophic lateral sclerosis, Parkinson’s disease, prion disease, or Huntington’s disease (Hou et al., 2019; Holbrook et al., 2021). Though the neurodegeneration and the pathogenesis mechanism are different for each NDD, they are commonly characterized by neuroinflammation, neuronal loss, misfolded protein aggregation, oxidative stress and autophagy dysregulation (Tiwari et al., 2019; Mathieu et al., 2020; Slanzi et al., 2020). The unspecific etiology associated with NDDs makes diagnosing and identifying at an early stage challenging. Moreover, the absence of any early-stage biomarkers has made it difficult to diagnose NDDs accurately and at a preliminary stage (Tiwari et al., 2019; Hansson, 2021). It forces the utilization of peripheral patient-derived biomaterial as a sample to diagnose NDDs, which is insufficient to provide an accurate diagnosis due to the absence of predominantly disease-associated neurons. Furthermore, the present-day treatments are only symptomatic and the entry of drugs into the brain is restricted by a selective mechanism of the blood-brain barrier (BBB) (Kumar et al., 2021). There are only specific ways through which a molecule can cross BBB, including efflux transport, passive transport and active transport (Kadry et al., 2020). Passive transport includes two non-energetic transportation pathways encompassing transcellular and paracellular diffusion pathways, which are only allowed to small hydrophilic/lipophilic molecules. Contrary, active transport necessitates energy for transporting small molecules to cross the BBB through various biological gradients (Kadry et al., 2020; Kumar et al., 2021). Moreover, transport through the BBB also occurs by efflux mechanisms like P-glycoprotein (Pgp) mechanism (Kadry et al., 2020). It indicates that the perquisite to develop drugs and sensors for NDDs with the potential to cross BBB lies in architecting small hydrophilic/lipophilic molecules with appropriate surface chemistries to develop binding potential.
Neuro-nanotechnology: Across the blood-brain barrier
The advent of nanotechnology provides a solution to cater for these challenges by engineering functionalized materials in nanometer dimensions (1–100 nm) that can be soluble in lipids, interact with neural systems at the molecular level, and efficacy to sustain in the in vivo environment for a prolonged time and efficiently crosses BBB (Silva, 2006; Pampaloni et al., 2019). The high effective surface area of nanosystems possesses more enormous possibilities of interaction with biomarkers for diagnosis, with drugs/molecules for drug delivery with more considerable retentive efficacy and with defective cells for a regenerative cure (Chen et al., 2022; Tiwari et al., 2022). Moreover, the physicochemical attributes of nanosystems, including physical properties (magnetic, optical, electrical, thermal, mechanical) and chemical features (surface chemistry, reactivity, solubility), can be tuned per the targeted application. Owing to these tunable and superior physicochemical, morphological and topological features, nanosystems have been utilized as essential vectors for targeted drug delivery, as modalities for neuroimaging, strategies for neuroprotection, tools for neurosurgery, and as scaffolds for neuroregeneration in NDDs with potential to cross BBB (Silva, 2006; Chen et al., 2022; Tiwari and Kaushik, 2022). For instance, magnetic nanosystems are utilized as brain contrast agents in NDD imaging techniques such as magnetic resonance imaging (MRI). Further, the magnetic attribute can also be utilized in treating NDD by regulating it through an external magnetic field. Similarly, the chemical attributes can also be tuned as per desired application, like the solubility of nanosystems can be optimized through surface functionalization. Moreover, desired surface functionalities can be grown over nanosystems for attaching targeted molecules or drugs for treatment or diagnosing an NDD (Silva, 2006; Rahman et al., 2022; Tiwari and Kaushik, 2022). Various nanosystems, including carbon-based nanomaterials, polymeric nanosystems and composites, metal-based nanoparticles, liposomes, dendrimers, quantum dots and recently, 2D nanosystems (like MXenes) have been evaluated for developing NDD’s diagnosing, monitoring and treatment strategies (Feng et al., 2021; Tiwari et al., 2021; Banitaba et al., 2022; Chen et al., 2022; Ghosh et al., 2022; Rahman et al., 2022). For instance, core-shell nanosystems with metal at the core and polymer as a coat are utilized as effective nano-carrier to transport nerve growth factor (promotes neural growth) for crossing BBB (Kumar et al., 2020). However, the toxicity and biocompatibility of these nanosystems are yet to be addressed, which restricts their commercial viability in biomedical sectors (Seaton et al., 2010; Sengul and Asmatulu, 2020). Moreover, these nanosystems can accidentally enter the brain or other human organs during therapy or imaging processes, leading to a disbalance in their functionality. For instance, crossing the BBB through various means, including through adsorption-based transcytosis, through receptors or targeted transporters expressed on endothelial cells of brain capillaries, and through intranasal routes, can induce various neurotoxicity mechanisms such as immune response and inflammation, oxidative stress, activating particular signaling pathways, and induced cell autophagy and apoptosis affecting the BBB functions (Boyes and van Thriel, 2020). Moreover, these toxic effects can directly alter the neural environment and structure and may result in a cascade of effects due to glial neural interactions and activation. Therefore, despite of promising progress of nanosystems in neurosciences, their applications are limited due to neurotoxicity, generic toxicity, biocompatibility and controllable selectivity, which can temporarily or permanently affect the central nervous system or other human functionalities.
Emergence of green nanosystems for efficient NDD management: A green-neuro-nanotechnology
The primary reason associated with the toxicity of conventional nanosystems lies with the precursors and strategies used for their fabrication (Sengul and Asmatulu, 2020; Husain et al., 2021; Verma et al., 2021; Chaudhary et al., 2022c; Umapathi et al., 2022). For instance, the utilization of toxic, volatile and corrosive chemical precursors and their remnant traces in the stoichiometry of nanosystems developed through bottom-up chemical routes are hazardous for humans and the environment. Moreover, the physical techniques, especially top-down methods, are destruction-based approaches that require high energy and induce accidental toxicity by disrupting the nanosystem structure in an uncontrolled manner during their synthesis. Contrarily, green strategies to architect nanosystems involve significantly fewer toxic chemicals and require minimal energy for their fabrication (Verma et al., 2019; Khalaj et al., 2020; Pathania et al., 2022a; Batra et al., 2022). The replacement of harmful chemical precursors with biochemical such as phytochemicals, microorganisms, and biome-extracted precursors caters to the accidental doping side-effects/toxicity induced in nanosystems during their fabrication. Additionally, most phytochemicals possess antimicrobial, neurogeneration, anti-stress and anti-NDD attributes, which are the additive advantage of green synthesized nanosystems accidentally/intentionally doped with their precursors (Singh et al., 2018; Pathania et al., 2022b; Monika et al., 2022). For instance, inhalation aromatherapy of essential oils is proposed as brain-targeted nasal delivery in NDD therapeutics (Cui et al., 2022). These essential oils have also been utilized to fabricate green nanosystems for diversified disease management, and their doping/traces in fabricated nanosystems have exhibited enhanced disorder-combatting efficacies. Apart from essential oils, various phytochemicals (ketones, amines, phenols, flavones, terpenoids, bio-acids) extracted from different part of plants (leaves, fruits, stem, flowers, seed, bark, and roots) serves as multifunctional precursors with capping, reducing and stabilizing actions, which minimizes the chemical/precursors requirements thereby reducing the probability of induced toxicity and secondary contamination to the environment (Khalaj et al., 2020; Palit and Hussain, 2020; Pathania et al., 2022a, 2022b). Similarly, microbial-mediated fabrication (bacteria, yeast, viruses, fungi, algae), biomes-extracted precursors, protein-based nanosystems, exosomes and repurpose-byproducts are green strategies to fabricate eco-friendly bio-nanosystems/green-nanosystems. Moreover, during the fabrication, physicochemical attributes of these nanosystems can be easily optimized by regulating the cellular/phytochemical activities and reaction parameters such as concentration and nature of precursors, reaction medium and time, and reaction temperature and pH. The intelligent selection of biomes/biome-extracted precursors for nanosystem fabrication can be used to control the surface functionalities, optimizing band structures and band gaps, morphological and topological features, ambient and hostile environment stabilities, biocompatibility, toxicity and bioaccumulation for targeted solutions. In recent years, various targeted green nanosystems have been engineered for combatting NDDs, including implantable and diagnostic devices like catheters, biosensors and stunts, drug delivery like nanoliposomes with potential to be functionalized with antibodies and crossing BBB, developing immunity and nanoscaffolds for neurogeneration, controlling and curing primary and secondary symptoms, biocompatibility in surgical prostheses, and targeted therapeutics and imaging eliminating systemic toxicity (Mizrahi et al., 2014; Santhoshkumar et al., 2014; Charbgoo et al., 2017; Maddinedi et al., 2017; Suganthy et al., 2018; Kotcherlakota et al., 2019; Zhang et al., 2020; Ahlawat and Narayan, 2021; Jan et al., 2021; Pathania et al., 2022b, 2022a; Cui et al., 2022; Jasim et al., 2022; Kokturk et al., 2022; Monika et al., 2022). Moreover, their indirect utilization for strengthening present NDD monitoring strategies, such as enhancing the monitoring performance of microelectrodes utilized in electrophysiology, improving laser-mediated vascular anastomoses, and enhancing optical interfaces for neural imaging, is combatting the bottlenecks of controlled monitoring of neuronal ensembles. The essential feature of green nanomaterials for NDDs is their biocompatibility in terms of neurotoxicity, haemo-histocompatibility, cytotoxicity, genotoxicity and carcinogenesis, which implies their immense potential for combatting NDDs. The green nanosystems by addressing the issues of neurotoxicity also possess potential for maintaining the neural health in light of neurogeneration and controlling secretion of neural fluids. Consequently, green nanosystems are energy-efficient, cost-effective, eco-friendly, non-toxic, biocompatible, single-stage processed, multifunctional and renewable strategies for efficient NDD management and treatment with enhanced anti-NDD, antimicrobial, immunity developing, neurogenerative and BBB-crossing efficacies (Figure 1).
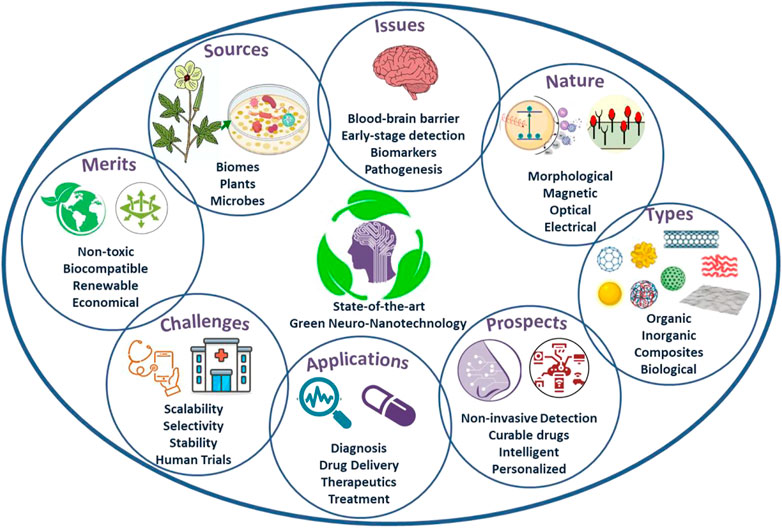
FIGURE 1. State-of-the-art Green Neuro-nanotechnology: Sources, issues, nature, types, merits, challenges, applications and prospects.
Challenges, alternate solutions and prospects: Conclusive outlook
In spite of extraordinary sustainable advantages and significant performances, the assessment of green nanomaterials for diversified application in NDDs, including neurogenesis, diagnosis, imaging-based monitoring, drug delivery and drug development, is in its infancy. There are few reports on the utilization of green nanosystems for combatting NDDs compared to other severe diseases such as cancer. This restricted development of green nanosystems is associated with several challenges related to efficacies, stability, manufacturing, scalability, selectivity and controlled utilization. For instance, the scalable fabrication of green nanosystems is quite difficult due to low yield, the challenge of purification, and altitudinal/regional variation in the composition of biochemical precursors, which limits their commercial applications (Palit and Hussain, 2020; Pathania et al., 2022b). Moreover, low encapsulation efficacy, bioaccumulation, uncontrolled cellular activities, stability on multiple biological variations and the hostile environment, selectivity in target cells/tissues/areas, and strong associations with prominent and early-stage biomarkers are challenging in developing green nanosystem-based strategies for diagnosis, monitoring, management and treatment of NDDs. Additionally, there is a requirement for careful selection of animal models that recapitulate specific human pathology in accordance with green nanomaterial stoichiometry, composition, structure and synthesis (Dawson et al., 2018; Maurya et al., 2022). There is a strong requirement to understand the cellular interaction/mechanism and pathogenesis of green nanomaterials in the human body in the context of NDDs (Mathieu et al., 2020; Tiwari et al., 2021; Chaudhary et al., 2022c). Additionally, there is a large gap between theoretical evaluations, animal modelling, in vitro and in vivo evaluations, animal trials and human clinical trials of green nanosystems for managing NDDs. These challenges can be catered to by focusing research on evaluating the interaction of green nanosystems in multi-bio-variant human-like environments utilizing advanced bioinformatics, analytical docking, machine learning and artificial intelligence techniques (Myszczynska et al., 2020; Tăuţan et al., 2021; Chaudhary et al., 2022b, 2022a; Sonu and Chaudhary, 2022). Moreover, there is a requirement to explore scalable and controllable fabrication of nanosystems with optimized physicochemical attributes and biocompatibilities using modern-age technologies. The abundance of biodiversity can be dedicatedly explored to develop next-generation green nanosystems as a sustainable approach to combat NDDs. As a result, green nanosystems require streamlined, controlled, and directional research and evaluations supported by clinical explorations and are near-future of NDD’s diagnosis, monitoring, management and treatment owing to their enhanced neurogeneration efficacies, biocompatibility, economical and environment-friendly manufacturing, renewable feedstock and non-toxicity, all contributing to form sustainable green-neuro-nanotechnology.
Data availability statement
The original contributions presented in the study are included in the article/supplementary material, further inquiries can be directed to the corresponding author.
Author contributions
VC has worked on entire manuscript.
Acknowledgments
Author wish to acknowledge Department of Science and Technology, Government of India, India, and Vice chancellor, University of Delhi for providing e-resources. Author also wish to acknowledge British Council Going Global Partnership exploratory grant for supporting project entitled Enhancing Commercial acumen and organisational capability for business (ECOBUSS) with grant ID: 877799913.
Conflict of interest
The author declares that the research was conducted in the absence of any commercial or financial relationships that could be construed as a potential conflict of interest.
Publisher’s note
All claims expressed in this article are solely those of the authors and do not necessarily represent those of their affiliated organizations, or those of the publisher, the editors and the reviewers. Any product that may be evaluated in this article, or claim that may be made by its manufacturer, is not guaranteed or endorsed by the publisher.
References
Ahlawat, J., and Narayan, M. (2021). “Organic carbon dots for mitigating neurodegenerative diseases,” in Biobased nanotechnology for green applications (Cham: Springer), 273–287. doi:10.1007/978-3-030-61985-5_10
Banitaba, S. N., Ebadi, S. V., Salimi, P., Bagheri, A., Gupta, A., Arifeen, W. U., et al. (2022). Biopolymer-based electrospun fibers in electrochemical devices: Versatile platform for energy, environment, and health monitoring. Mat. Horiz. doi:10.1039/D2MH00879C
Batra, V., Kaur, I., Pathania, D., and Chaudhary, V. (2022). Efficient dye degradation strategies using green synthesized ZnO-based nanoplatforms: A review. Appl. Surf. Sci. Adv. 11, 100314. doi:10.1016/j.apsadv.2022.100314
Boyes, W. K., and van Thriel, C. (2020). Neurotoxicology of nanomaterials. Chem. Res. Toxicol. 33, 1121–1144. doi:10.1021/acs.chemrestox.0c00050
Charbgoo, F., Ahmad, M., and Darroudi, M. (2017). Cerium oxide nanoparticles: Green synthesis and biological applications. Int. J. Nanomedicine 12, 1401–1413. doi:10.2147/IJN.S124855
Chaudhary, V., Gautam, A., Silotia, P., Malik, S., de Oliveira Hansen, R., Khalid, M., et al. (2022a). Internet-of-nano-things (IoNT) driven intelligent face masks to combat airborne health hazard. Mat. Today. doi:10.1016/j.mattod.2022.08.019
Chaudhary, V., Kaushik, A., Furukawa, H., and Khosla, A. (2022b). Review—towards 5th generation AI and IoT driven sustainable intelligent sensors based on 2D MXenes and borophene. ECS Sens. Plus 1, 013601. doi:10.1149/2754-2726/ac5ac6
Chaudhary, V., Mostafavi, E., and Kaushik, A. (2022c). De-coding Ag as an efficient antimicrobial nano-system for controlling cellular/biological functions. Matter 5, 1995–1998. doi:10.1016/j.matt.2022.06.024
Chen, J., Zhou, Z., Luo, S., Liu, G., Xiang, J., and Tian, Z. (2022). Progress of advanced nanomaterials in diagnosis of neurodegenerative diseases. Biosens. Bioelectron. X. 217, 114717. doi:10.1016/j.bios.2022.114717
Cui, J., Li, M., Wei, Y., Li, H., He, X., Yang, Q., et al. (2022). Inhalation aromatherapy via brain-targeted nasal delivery: Natural volatiles or essential oils on mood disorders. Front. Pharmacol. 13, 860043. doi:10.3389/fphar.2022.860043
Dawson, T. M., Golde, T. E., and Lagier-Tourenne, C. (2018). Animal models of neurodegenerative diseases. Nat. Neurosci. 21, 1370–1379. doi:10.1038/s41593-018-0236-8
Feng, W., Han, X., Hu, H., Chang, M., Ding, L., Xiang, H., et al. (2021). 2D vanadium carbide MXenzyme to alleviate ROS-mediated inflammatory and neurodegenerative diseases. Nat. Commun. 12, 2203. doi:10.1038/s41467-021-22278-x
Ghosh, S., Sachdeva, B., Sachdeva, P., Chaudhary, V., Rani, G. M., and Sinha, J. K. (2022). Graphene quantum dots as a potential diagnostic and therapeutic tool for the management of Alzheimer’s disease. Carbon Lett. 2022, 1–14. doi:10.1007/s42823-022-00397-9
Hansson, O. (2021). Biomarkers for neurodegenerative diseases. Nat. Med. 27, 954–963. doi:10.1038/s41591-021-01382-x
Holbrook, J. A., Jarosz-Griffiths, H. H., Caseley, E., Lara-Reyna, S., Poulter, J. A., Williams-Gray, C. H., et al. (2021). Neurodegenerative disease and the NLRP3 inflammasome. Front. Pharmacol. 12, 643254. doi:10.3389/fphar.2021.643254
Hou, Y., Dan, X., Babbar, M., Wei, Y., Hasselbalch, S. G., Croteau, D. L., et al. (2019). Ageing as a risk factor for neurodegenerative disease. Nat. Rev. Neurol. 15, 565–581. doi:10.1038/s41582-019-0244-7
Husain, S., Verma, S. K., HemlataAzam, M., Sardar, M., Haq, Q. M. R., and Fatma, T (2021). Antibacterial efficacy of facile cyanobacterial silver nanoparticles inferred by antioxidant mechanism. Mater. Sci. Eng. C 122, 111888. doi:10.1016/j.msec.2021.111888
Jan, H., Shah, M., Andleeb, A., Faisal, S., Khattak, A., Rizwan, M., et al. (2021). Plant-based synthesis of zinc oxide nanoparticles (ZnO-NPs) using aqueous leaf extract of aquilegia pubiflora: Their antiproliferative activity against HepG2 cells inducing reactive oxygen species and other in vitro properties. Oxid. Med. Cell. Longev. 2021, 1–14. doi:10.1155/2021/4786227
Jasim, S. A., Patra, I., Opulencia, M. J. C., Hachem, K., Parra, R. M. R., Ansari, M. J., et al. (2022). Green synthesis of spinel copper ferrite (CuFe 2 O 4 ) nanoparticles and their toxicity. Nanotechnol. Rev. 11, 2483–2492. doi:10.1515/ntrev-2022-0143
Kadry, H., Noorani, B., and Cucullo, L. (2020). A blood–brain barrier overview on structure, function, impairment, and biomarkers of integrity. Fluids Barriers CNS 17, 69. doi:10.1186/s12987-020-00230-3
Khalaj, M., Kamali, M., Costa, M. E. V., and Capela, I. (2020). Green synthesis of nanomaterials - a scientometric assessment. J. Clean. Prod. 267, 122036. doi:10.1016/j.jclepro.2020.122036
Kokturk, M., Yıldırım, S., Nas, M. S., Ozhan, G., Atamanalp, M., Bolat, I., et al. (2022). Investigation of the oxidative stress response of a green synthesis nanoparticle (RP-Ag/ACNPs) in zebrafish. Biol. Trace Elem. Res. 200, 2897–2907. doi:10.1007/s12011-021-02855-3
Kotcherlakota, R., Das, S., and Patra, C. R. (2019). “Therapeutic applications of green-synthesized silver nanoparticles,” in Green synthesis, characterization and applications of nanoparticles (Elsevier), 389–428. doi:10.1016/B978-0-08-102579-6.00017-4
Kumar, R., Aadil, K. R., Mondal, K., Mishra, Y. K., Oupicky, D., Ramakrishna, S., et al. (2021). Neurodegenerative disorders management: State-of-art and prospects of nano-biotechnology. Crit. Rev. Biotechnol. 2021, 1–33. doi:10.1080/07388551.2021.1993126
Kumar, R., Mondal, K., Panda, P. K., Kaushik, A., Abolhassani, R., Ahuja, R., et al. (2020). Core–shell nanostructures: Perspectives towards drug delivery applications. J. Mat. Chem. B 8, 8992–9027. doi:10.1039/D0TB01559H
Maddinedi, S. B., Mandal, B. K., and Anna, K. K. (2017). Tyrosine assisted size controlled synthesis of silver nanoparticles and their catalytic, in-vitro cytotoxicity evaluation. Environ. Toxicol. Pharmacol. 51, 23–29. doi:10.1016/j.etap.2017.02.020
Mathieu, C., Pappu, R. V., and Taylor, J. P. (2020). Beyond aggregation: Pathological phase transitions in neurodegenerative disease. Science 370, 56–60. doi:10.1126/science.abb8032
Maurya, S. K., Baghel, M. S., GauravChaudhary, V., Kaushik, A., and Gautam, A. (2022). Putative role of mitochondria in SARS-CoV-2 mediated brain dysfunctions: A prospect. Biotechnol. Genet. Eng. Rev. 2022, 1–26. doi:10.1080/02648725.2022.2108998
Mizrahi, M., Friedman-Levi, Y., Larush, L., Frid, K., Binyamin, O., Dori, D., et al. (2014). Pomegranate seed oil nanoemulsions for the prevention and treatment of neurodegenerative diseases: The case of genetic CJD. Nanomedicine Nanotechnol. Biol. Med. 10, 1353–1363. doi:10.1016/j.nano.2014.03.015
Monika, P., Chandraprabha, M. N., Hari Krishna, R., Vittal, M., Likhitha, C., Pooja, N., et al. (2022). Recent advances in pomegranate peel extract mediated nanoparticles for clinical and biomedical applications. Biotechnol. Genet. Eng. Rev. 2022, 1–29. doi:10.1080/02648725.2022.2122299
Myszczynska, M. A., Ojamies, P. N., Lacoste, A. M. B., Neil, D., Saffari, A., Mead, R., et al. (2020). Applications of machine learning to diagnosis and treatment of neurodegenerative diseases. Nat. Rev. Neurol. 16, 440–456. doi:10.1038/s41582-020-0377-8
Palit, S., and Hussain, C. M. (2020). “Green nanomaterials: A sustainable perspective,” in Green nanomaterials (Cham: Springer), 23–41. doi:10.1007/978-981-15-3560-4_2
Pampaloni, N. P., Giugliano, M., Scaini, D., Ballerini, L., and Rauti, R. (2019). Advances in nano neuroscience: From nanomaterials to nanotools. Front. Neurosci. 12, 953. doi:10.3389/fnins.2018.00953
Pathania, D., Kumar, S., Thakur, P., Chaudhary, V., Kaushik, A., Varma, R. S., et al. (2022a). Essential oil-mediated biocompatible magnesium nanoparticles with enhanced antibacterial, antifungal, and photocatalytic efficacies. Sci. Rep. 12, 11431. doi:10.1038/s41598-022-14984-3
Pathania, D., Sharma, M., Thakur, P., Chaudhary, V., Kaushik, A., Furukawa, H., et al. (2022b). Exploring phytochemical composition, photocatalytic, antibacterial, and antifungal efficacies of Au NPs supported by Cymbopogon flexuosus essential oil. Sci. Rep. 12, 14249. doi:10.1038/s41598-022-15899-9
Rahman, M. M., Islam, M. R., Akash, S., Harun-Or-Rashid, M., Ray, T. K., Rahaman, M. S., et al. (2022). Recent advancements of nanoparticles application in cancer and neurodegenerative disorders: At a glance. Biomed. Pharmacother. 153, 113305. doi:10.1016/j.biopha.2022.113305
Santhoshkumar, T., Rahuman, A. A., Jayaseelan, C., Rajakumar, G., Marimuthu, S., Kirthi, A. V., et al. (2014). Green synthesis of titanium dioxide nanoparticles using Psidium guajava extract and its antibacterial and antioxidant properties. Asian pac. J. Trop. Med. 7, 968–976. doi:10.1016/S1995-7645(14)60171-1
Seaton, A., Tran, L., Aitken, R., and Donaldson, K. (2010). Nanoparticles, human health hazard and regulation. J. R. Soc. Interface 7, S119–S129. doi:10.1098/rsif.2009.0252.focus
Sengul, A. B., and Asmatulu, E. (2020). Toxicity of metal and metal oxide nanoparticles: A review. Environ. Chem. Lett. 18, 1659–1683. doi:10.1007/s10311-020-01033-6
Silva, G. A. (2006). Neuroscience nanotechnology: Progress, opportunities and challenges. Nat. Rev. Neurosci. 7, 65–74. doi:10.1038/nrn1827
Singh, A. K., Kumar, S., and Vinayak, M. (2018). Recent development in antihyperalgesic effect of phytochemicals: Anti-inflammatory and neuro-modulatory actions. Inflamm. Res. 67, 633–654. doi:10.1007/s00011-018-1156-5
Slanzi, A., Iannoto, G., Rossi, B., Zenaro, E., and Constantin, G. (2020). In vitro models of neurodegenerative diseases. Front. Cell Dev. Biol. 8, 328. doi:10.3389/fcell.2020.00328
Sonu, S., and Chaudhary, V. (2022). A paradigm of internet-of-nano-things inspired intelligent plant pathogen-diagnostic biosensors. ECS Sens. Plus 1, 031401. doi:10.1149/2754-2726/ac92ed
Suganthy, N., Sri Ramkumar, V., Pugazhendhi, A., Benelli, G., and Archunan, G. (2018). Biogenic synthesis of gold nanoparticles from Terminalia arjuna bark extract: Assessment of safety aspects and neuroprotective potential via antioxidant, anticholinesterase, and antiamyloidogenic effects. Environ. Sci. Pollut. Res. 25, 10418–10433. doi:10.1007/s11356-017-9789-4
Tăuţan, A.-M., Ionescu, B., and Santarnecchi, E. (2021). Artificial intelligence in neurodegenerative diseases: A review of available tools with a focus on machine learning techniques. Artif. Intell. Med. 117, 102081. doi:10.1016/j.artmed.2021.102081
Tiwari, S., Atluri, V., Kaushik, A., Yndart, A., and Nair, M. (2019). <p>Alzheimer’s disease: Pathogenesis, diagnostics, and therapeutics</p>. Int. J. Nanomedicine 14, 5541–5554. doi:10.2147/IJN.S200490
Tiwari, S., Juneja, S., Ghosal, A., Bandara, N., Khan, R., Wallen, S. L., et al. (2022). Antibacterial and antiviral high-performance nanosystems to mitigate new SARS-CoV-2 variants of concern. Curr. Opin. Biomed. Eng. 21, 100363. doi:10.1016/j.cobme.2021.100363
Tiwari, S., and Kaushik, A. (2022). Nano-neurogenesis for CNS diseases and disorders. Front. Nanotechnol. 4. doi:10.3389/fnano.2022.931259
Tiwari, V., Koganti, R., Russell, G., Sharma, A., and Shukla, D. (2021). Role of tunneling nanotubes in viral infection, neurodegenerative disease, and cancer. Front. Immunol. 12, 680891. doi:10.3389/fimmu.2021.680891
Umapathi, A., Kumawat, M., and Daima, H. K. (2022). Engineered nanomaterials for biomedical applications and their toxicity: A review. Environ. Chem. Lett. 20, 445–468. doi:10.1007/s10311-021-01307-7
Verma, S. K., Jha, E., Panda, P. K., Thirumurugan, A., and Suar, M. (2019). “Biological effects of green-synthesized metal nanoparticles: A mechanistic view of antibacterial activity and cytotoxicity,” in Advanced nanostructured materials for environmental remediation (Cham: Springer), 145–171. doi:10.1007/978-3-030-04477-0_6
Verma, S. K., Panda, P. K., Kumari, P., Patel, P., Arunima, A., Jha, E., et al. (2021). Determining factors for the nano-biocompatibility of cobalt oxide nanoparticles: Proximal discrepancy in intrinsic atomic interactions at differential vicinage. Green Chem. 23, 3439–3458. doi:10.1039/D1GC00571E
Keywords: neurodegenerative diseases, nanotechnology, blood-brain barrier, green nanomaterials, toxicity
Citation: Chaudhary V (2022) Prospects of green nanotechnology for efficient management of neurodegenerative diseases. Front. Nanotechnol. 4:1055708. doi: 10.3389/fnano.2022.1055708
Received: 28 September 2022; Accepted: 20 October 2022;
Published: 01 November 2022.
Edited by:
Sudip Mukherjee, Rice University, United StatesReviewed by:
Suresh K. Verma, Uppsala University, SwedenRaj Kumar, University of Nebraska Medical Center, United States
Copyright © 2022 Chaudhary. This is an open-access article distributed under the terms of the Creative Commons Attribution License (CC BY). The use, distribution or reproduction in other forums is permitted, provided the original author(s) and the copyright owner(s) are credited and that the original publication in this journal is cited, in accordance with accepted academic practice. No use, distribution or reproduction is permitted which does not comply with these terms.
*Correspondence: Vishal Chaudhary, Q2hhdWRoYXJ5MDB2aXNoYWxAZ21haWwuY29t