- 1ICAR-Indian Institute of Wheat and Barley Research, Karnal, India
- 2ICAR-Central Soil Salinity Research Institute, Karnal, India
Globally, between one quarter and one-third of total grains produced each year are lost during storage mainly through infestation of insect pests. Among the available control options such as chemical and physical techniques, fumigation with aluminum phosphide (AlP) is so far considered the best control strategy against storage insect pests. However, these insect pests are now developing resistance against AIP due to its indiscriminate use due to non-availability of any effective alternative control option. Resistance to AIP among storage insect pests is increasing, and its inhalation has shown adverse effects on animals and human beings. Nanotechnology has opened up a wide range of opportunities in various fields such as agriculture (pesticides, fertilizers, etc.), pharmaceuticals, and electronics. One of the applications of nanotechnology is the usage of nanomaterial-based insecticide formulations for mitigating field and storage insect pests. Several formulations, namely, nanoemulsions, nanosuspensions, controlled release formulations, and solid-based nanopesticides, have been developed with different modes of action and application. The major advantage is their small size which helps in proper spreading on the pest surface, and thus, better action than conventional pesticides is achieved. Besides their minute size, these have no or reduced harmful effects on non-target species. Nanopesticides can therefore provide green and efficient alternatives for the management of insect pests of field and storage. However, an outcry against the utilization of nano-based pesticides is also revealed. It is considered by some that nano-insecticides may also have hazardous effects on humans as well as on the environment. Due to limited available data, nanopesticides have become a double-edged weapon. Therefore, nanomaterials need to be evaluated extensively for their large-scale adoption. In this article, we reviewed the nanoformulations that are developed and have proved effective against the insect pests under postharvest storage of grains.
1 Introduction
Grain crops that are most widely grown worldwide include cereals (maize, wheat, millets, rice, etc.) (Awika, 2011; Ye & Fan, 2021); pulses (mung, beans, chickpea, cowpea, black gram, green gram, etc.) (Sharma et al., 2010; Liu et al., 2020); and oilseeds (soybean, sunflower, linseed, groundnut, etc.) (Attia et al., 2021). These crops form a crucial component of our diet as they are rich in proteins, carbohydrates, fats, vitamins, minerals, and oils (Sarwar et al., 2013; Oso & Ashafa, 2021). Due to the presence of these nutrient elements, the grains of these crops are prone to infestation by insect pests during storage. The marketability of the food grains is hampered when insect pests feed on grains and make them unfit for human consumption, leading to huge monetary losses (Sharon et al., 2014; Sharma et al., 2016; Wang et al., 2021). Losses due to insect infestation under storage can go up to 50 to 60% under extreme situations (Kumar et al., 2017; Luo et al., 2020). Sometimes, the postharvest losses can surpass the losses that crops suffer in the field (Mesterházy et al., 2020). Direct losses occur in the form of direct consumption of kernels, while indirect losses include formation of webbing, exuviae, frass, and insect cadavers that significantly hamper seed quality and leave grains unfit for human consumption (Kumar and Kalita., 2017; Mesterházy et al., 2020). Changes in the grain storage environment due to insect infestation result in the formation of warm and moist “hotspots” which further lead to the development of storage fungi. The presence of fungi in stored grain can further increase the level of crop losses (Shankar and Abrol, 2012; Fones et al., 2020).
Storage insect pest species inflicting quantitative and qualitative losses during postharvest storage of grains mainly belong to two insect orders: Coleoptera (around 600 species), Lepidoptera (70 species), and mites (about 355 species) (Rajendran, 2002; Rajendran and Sriranjini, 2008). Insect pests of stored grains are of two types, namely, primary and secondary pests (Banga et al., 2020). Primary pests are those that damage sound or whole grains, while secondary pests damage broken or already damaged grains. Primary pests are further categorized into internal and external feeders based on the place of their attack (Deshwal et al., 2020) (Supplementary Table S1, S2). Several insect control options are available such as physical, mechanical, chemical, and biological practices, but fumigation is considered the most common method of pest control due to its wider application under various storage conditions such as bags, silos, warehouses, and mills (Nguyen et al., 2015; Nayak et al., 2020). However, synthetic pesticides are there in the market for the control of storage pests but these pesticides are costly, ineffective, and are harmful to the environment as well as to human health (Jallow et al., 2017; Poudel et al., 2020).
Aluminum phosphide (AlP) is the key fumigant among all the fumigants available for stored grain pest management as it gives higher grain and stored products protection (Daglish et al., 2002; Collins et al., 2005; Shin et al., 2021). Commodities in bulk quantity can be effectively protected against insect infestation by the usage of AIP. The phosphine gas released by AIP penetrates the grain bulks readily and eliminates rapidly from the grain via aeration leaving no residues. Moreover, there are very limited alternative fumigants available, for instance, methyl bromide and sulfuryl fluoride (Gu, 2020). But, routine fumigation of grains has been discouraged in developed countries since 2005 owing to the concerns of potential fluoride residues (Scheffrahn et al., 1989; Bell, 2000; Oguntade, 2021). Therefore, only dependence on phosphine for fumigation due to non-availability of other effective alternatives has inevitably resulted in insect resistance problems in many storage insect pests (Bengston et al., 1996; Chaudhry, 2000; Mills, 2001; Collins et al., 2002; Daglish et al., 2002; Hasan and Reichmuth, 2004; Pimentel et al., 2007; Wakil et al., 2021). The resistance to phosphine and other fumigants is observed in majority of stored grain pests such as red flour beetle Tribolium castaneum, rice weevil Sitophilus oryzae, and lesser grain borer Rhyzopertha dominica which poses a very serious threat to postharvest grain produce around the globe (Dyte and Halliday, 1985; Badmin, 1990; Bell, 2000; Chaudhry, 2000; Valmas et al., 2008; Newman, 2010; Ali et al., 2013; Holloway et al., 2016; Collins and Schlipalius, 2018; Attia et al., 2020; Nayak et al., 2020; Collins et al., 2017). Thereby, insecticide resistance has become an issue of international importance (Champ and Dyte, 1977; Benhalima et al., 2004; Collins et al., 2005; Wang et al., 2006; Sparks et al., 2021). Conventional techniques for pest management in storage are now inadequate and new innovative management techniques are to be developed.
Under the present scenario wherein agriculture today is facing major challenges of environmental contamination, pest resistance, bioaccumulation, and health hazards, nanotechnology is emanating as a highly attractive tool to achieve the target of lowering the quantity of pesticide used by offering new methods for the formulation and delivery of pesticide active ingredients, as well as novel active ingredients, collectively referred to as nanopesticides (Hayles et al., 2017; Shukla et al., 2019). The main advantage of nanopesticides usage is the reduction in the quantity of pesticide applied for a crop and or stored product protection. Nanotechnology deals with materials at the nanometer scale and is demonstrated to have a great potential in providing novel solutions to pest problems (Sasson et al., 2007; Kashyap et al., 2016; Unsworth et al., 2016; Kashyap et al., 2020). The use of nanotechnology will overcome the limitations associated with conventional pesticides by enhancing pesticide efficacy, improving stability of active ingredients, reducing the required pesticide dose, and conservation of agri inputs (Jasrotia et al., 2018; Rikta and Rajiv, 2021). However, new advanced nano-based formulations are expected to be target-specific, cost-effective, stable, and should have the ability to remain active under different application environments with a novel mode of action (Smith et al., 2008; Singh H et al., 2021). The concept of application of nanopesticides for storage pest management is new, and information related to their mode of action and application is lacking, which is hindering their usage under storage environment (Hischier and Walser, 2012; Kah et al., 2013; Kumar et al., 2018; Singh et al., 2020). However, many laboratory studies determining the efficacy of the nanomaterials have been conducted, but their large-scale application for postharvest management of insect pests is lacking (Hamel et al., 2020). Therefore, investigation of their behavior and ultimate fate in the environment is required that will aid in establishing a regulatory framework for their commercialization as well as will contribute to a sustainable grain protection (Nuruzzaman et al., 2016; Chhipa, 2017a; Bartolucci et al., 2020). This review documents the nanoformulations that have been evaluated for the management of storage insect pests until now and presents the research findings of laboratory studies and safety issues related to nanopesticides use. The article also describes their advantages and limitations followed by conclusions and future perspectives.
2 Advantages of Nanopesticides
Pioneering nanotechnology has opened a new way for the development of nano-sized formulations that are highly effective for pest control with lesser residual toxicity and are environment-friendly (Yadav et al., 2021). These are chemically alterable particles consisting of a large surface-to-volume ratio, and their physical properties make them capable enough to target organisms (Madhuri et al., 2010; Mustafa & Hussein, 2020; Sahoo et al., 2021). Nanoparticles can be engineered in different forms, for example, a capsule acts as a physical shell and can better withstand degradation in the environment. Therefore, nanoparticles offer better long-lasting protection as compared to conventional pesticides (Sushil et al., 2021). Unlike conventional pesticide formulations, nanoformulations are specially designed to increase the solubility of insoluble or poorly soluble active ingredients and to release the biocide in a controlled and targeted manner (Margulis-Goshen & Magdassi, 2013; Ragaei & Sabry, 2014; Garg & Payasi, 2020). Therefore, a smaller amount of active ingredient per area is sufficient for application, and it can provide sustained delivery of active ingredients which may remain effective for extended periods (Singh et al., 2020). Thus, due to the reduced dose requirement, the cost of application is also reduced. Also, it is important for the controlled release formulations that they must remain inactive until the active ingredient is released (Shekhar et al., 2021).
The nanoscale application of agrochemicals in agriculture in the form of nanofertilizers, nanopesticides, nanosensors, and nanoformulations has changed the traditional agro-practices (Aouada and De Moura, 2015; Panpatte et al., 2016; Scott et al., 2018; Pirzadah et al., 2019; Singh R. P et al., 2021). In crop protection, different types of nanoformulations, such as nanoemulsion, nanosuspension, and nanoparticulate, are suggested to tackle the insect pests of field and storage (Islam, 2020; Kashyap et al., 2020; Yadav et al., 2021)). The amalgamation of botanicals with nanopesticides is one of the most powerful tools that provide eco-friendly control routes for insect pest control. Biological pesticides are already being used as an alternative to chemical pesticides (Qazi and Dar, 2020). On top of it, the synthesis of nanoparticles using botanicals has served many potential benefits to agriculture. Several surfactants, polymers, and metal nanoparticles in the nanometer size range are used in their production (Kah et al., 2013; Ragaei and Sabry, 2014; Shaban et al., 2020). These nanomaterials at the nanoscale exhibit some properties which are different from bulk materials, supporting their unique applications for different conditions (Elizabath et al., 2019; Mujtaba et al., 2020). Expansion of nanopesticides through plants, microbes, and their derivatives bring forth dominance of sustainable approach and environment-friendly control. Though nanopesticides have infinite potential, it is still a dream at the moment (Ditta, 2012; Aithal and Aithal, 2015) because of their environmental and ecological impacts (Scrinis and Lyons, 2007; Karn et al., 2009) (Figure 1).
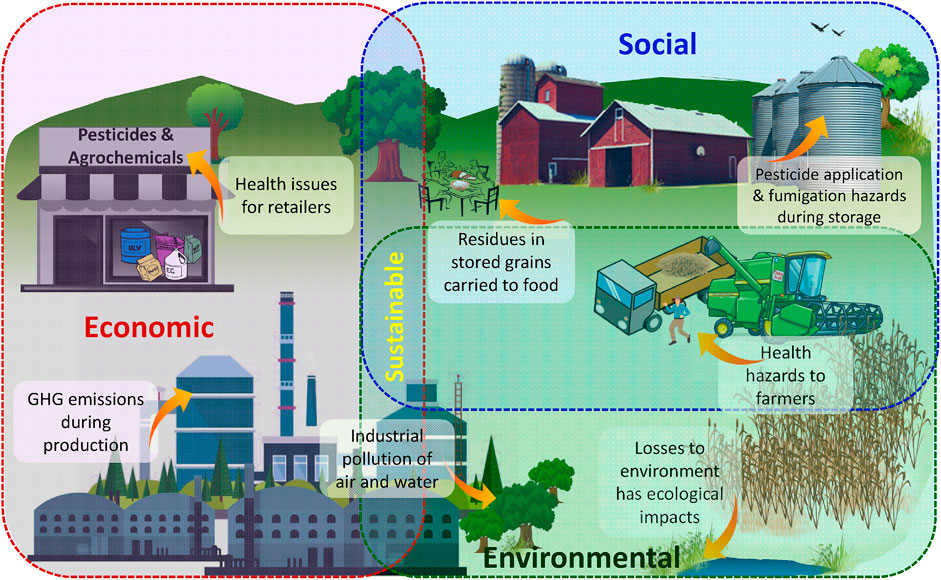
FIGURE 1. Economic, social, and environmental aspects of sustainability of pesticide use in agriculture.
3 Mode of Action of Nanopesticides
Nanoparticles developed through various synthesis routes as novel pesticides have recently attracted high research attention. Various studies have been conducted to test their toxic potential against a wide number of insect pests; however, precise information on their mode of action against insects is lacking (Rai et al., 2014; Athanassiou et al., 2018). Only a few studies have been carried to study the toxicokinetics or toxicodynamics of nanopesticides against storage grain insect pests as these are relatively new materials and have not been studied in great detail. Toxicokinetics basically refers to the movement and changes an insecticide undergoes inside an organism: absorption, distribution, metabolism, and excretion, while toxicodynamics refers to the physiological, biochemical, and molecular effects of the compounds and the mechanisms in which they are involved (Alzogaray & Zerba, 2017). The mode of action of silica-, alumina-, silver-, and graphene oxide nanoparticle–based nanopesticides against insects has been studied in a few studies (Benelli, 2018). Silver nanopesticides decrease acetylcholinesterase activity leading to oxidative stress and cell death by affecting antioxidants and detoxifying enzymes. These are also reported to reduce protein synthesis and gonadotropin release, leading to developmental damages and reproductive failure by either up- or downregulating key insect genes (Nair and Choi, 2011). Metal nanoparticles reduce membrane permeability by binding to S and P in proteins and nucleic acids, respectively, leading to organelle and enzyme denaturation, followed by cell death. Gold nanoparticles can effect development and reproduction and also act as trypsin inhibitors (Patil et al., 2016; Small et al., 2016). Aluminum oxide and silicon dioxide nanopesticides act by binding to the insect cuticle, followed by physico-sorption of waxes and lipids, leading to insect dehydration (Debnath et al., 2011; Arumugam et al., 2016). Nanozeolite formulation acted by attaching itself to the body of T. confusum and then caused scratching and splitting of the cuticle that ultimately led to the dehydration and mortality of the insect (Ibrahim and Salem, 2019). Stadler et al. (2017) reported the toxicity of nanostructured alumina and its mode of action against S. oryzae. The studies indicated that charged nanostructured alumina got attached to the beetle cuticle due to triboelectric forces, leading to insect dehydration through sorbing its wax layer by surface area phenomena (Figure 2).
4 Categories of Nanomaterials
The word “nano” emerged from the Greek word “dwarf” (Bhattacharyya et al., 2010; Anandhi et al., 2020). Nanoparticles refer to materials having nanoscale external dimensions or internal structures. The nanoscale size generally has an upper limit of about 100 nm. Based on the particle size, nanoparticles were classified into three categories: 1) ultrafine particles having a size less than 100 nm in diameter, 2) accumulation-mode particles having a size between 100 nm and 2.5 µm in diameter, and 3) coarse-mode particles having size greater than 2.5 µm in diameter (Sioutas et al., 2005; Kah et al., 2013; Kashyap et al., 2017; Kashyap et al., 2018; Yadav et al., 2021)). A nanosystem unit consists of two basic components: an active ingredient and a carrier. Based on their chemical composition, nanoformulations can also be classified into three basic categories including 1) inorganic-based, solid, and non-biodegradable nanoparticles (gold-, silver-, copper-, iron-, and silica-based nanoparticles), 2) organic-based biodegradable nanoparticles (liposomes, solid lipid, and polymeric nanoparticles), and 3) hybrid (combination of both inorganic and organic components) nanoparticles (Figure 3). For storage insect pest management, inorganic-based nanopesticides have been tested in more cases as compared to other categories. Also, plant-based nanoparticles have attracted more attention for stored grain pest control because these are environmentally safe and are easy to develop as compared to chemical-based nanopesticides (Goodsell 2004; Chen et al., 2019). Nanomaterials can also be developed using microbes or their bioactive compounds (Kashyap et al., 2018; Bazana et al., 2019). For stored insect pest control, various types of formulations have been suggested such as nanoemulsions, polymer-based nanopesticide formulations, and products containing engineered nanoparticles, for example metals and metal oxides (Mondal, 2020; Singh H et al., 2021). In this review, only metal-based nanoparticles, nanoemulsion, and polymer-based nanoformulations will be covered as these have been tested predominantly against storage insect pests. Moreover, a summary of nanopesticides evaluated against storage insect pests is given in Supplementary Table S3.
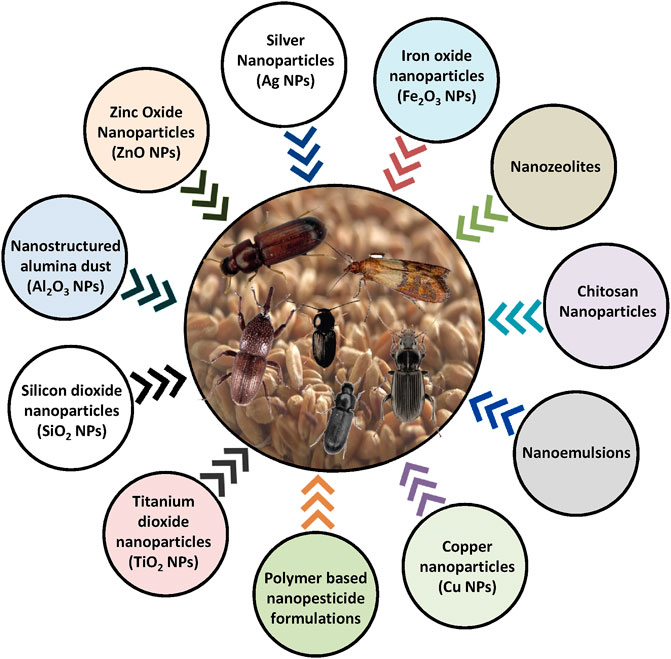
FIGURE 3. Types of potential nanomaterials and nanoformulations suggested for insect pest management under storage.
4.1 Metal-Based Nanomaterials
4.1.1 Silver nanoparticles
Silver nanoparticles (AgNPs) are so far one of the most tested and effective nanomaterials found for insect pest management. These nanoparticles are used carriers for delivering agrochemicals to the targeted site (Gupta et al., 2018; Gour et al., 2019). These exhibit well-built insecticidal, bactericidal, antifungal, and antiviral properties (Chen and Schluesener, 2008; Rai et al., 2014; Saratale et al., 2017) and are found to be very promising against insect pests. Additionally, they have catalytic properties, antimicrobial activity, higher chemical stability, and electrical conductivity that attracted more scientists for their use in pest management studies. The silver-based pesticide formulations deposit more dosage of active ingredients to the target species as compared to conventional pesticides (Ragaei and Sabry, 2014; Athanassiou et al., 2018). These nanoformulations have shown no toxicity to non-target organisms and therefore are considered safe to the environment (Jo et al., 2009; Oliveira et al., 2019).
Silver nanoparticles are reported to effectively control S. oryzae (Goswami et al., 2010; Zahir et al., 2012; Pathipati et al., 2021). The plant-mediated green silver nanoparticles using plant Euphorbia prostrata (Zahir et al., 2012) and Avicennia marina (Vijaya Sankar and Abideen, 2015) resulted in higher mortality of S. oryzae followed by T. castaneum and R. dominica. Silver nanoparticles application at the rate of 1.00 g kg−1 of seed gave 83% mortality of adults and larvae of Callosobruchus maculatus (Rouhani et al., 2013). Vadlapudi and Amanchy (2017) developed silver nanoparticles (AgNPs) utilizing the leaf extracts of Myriostachya wightiana that showed toxic effects against S. oryzae at concentration of 50 µg, and mortality of 29% ± 0.45 was obtained after 24 hrs. Nerium oleander plant extract–mediated AgNPs were found highly effective against larvae of T. castaneum and C. maculatus (Jafer and Annon, 2018). Rashwan and Abu-Zaid (2018) showed that AgNPs synthesized using rosemary executed 100% mortality for adults of S. granarius after 120 h, and the same efficacy of Laura and Cardamom was recorded after 144 h at 1 μg/ml concentration of AgNPs. High insecticidal efficacy was shown by the combination of silver nanoparticles and Malathion against T. castaneum (AS and Thangapandiyan, 2019). Silver nanoparticles fabricated using Annona reticulata leaf extract showed potent insecticidal properties against S. oryzae (Malathi et al., 2019). Clove, Syzygium aromaticum, essential oil-based AgNPs showed 71% repellency at 1% methanol extract concentration and 100% larvicidal mortality at 4% acetone concentration against T. castaneum (Selvaraj et al., 2019). Biogenic silver and gold nanoparticles synthesized from Daphne Mucronata (leaves, bark, and roots) and Monotheca buxifolia (leaves, seeds, and fruits) crude methanolic extracts are 100% effective against T. castaneum, R. dominica, and Callosobruchus analis (Shah, 2019). Silver nanoparticles prepared from silver nitrate using Moringa oleifera (Moringaceae) leaf extract gave the highest mortality (100%) against S. oryzae after 15 days of exposure (Rani et al., 2019). Silver nanoparticles extracted from leaf extracts of Azadirachta indica effectively reduced egg production of T. castaneum and C. maculatus to 85 eggs/female and 18 eggs/female, respectively, at a concentration of 2,000 ppm (Annon et al., 2020). Bio-prepared silver nanoparticles by Beauveria bassiana fungus showed 87.27% repellency and 97.56% mortality against C. maculatus at a concentration of 100% in 20 min soaking period (Al Jubury et al., 2021). Datura stramonium EO–coated silver nanoparticle gave highest mortality of 41.40% against Trogoderma granarium at 300 ppm, and the highest range was 67.89% at 15% concentration of D. stramonium (Gulzar et al., 2020). Silver nanoparticles achieved 100% killing at a concentration of 5,000 ppm within 72 h of application against O. surinamensis (Salman and Hameed, 2020). Silver nanoparticles prepared using ethanolic and hot aqueous extract of leaves of the Damas, Conocarpus lancifolius, showed the maximum sterility index among the stored date moth, Ephestia cautella, and was most effective at 1,500 ppm concentration (Abbood and Ali, 2020). Silver nanoparticles of Camelina sativa extract gave mortality of 60.1% against O. surinamensis and 46.2% against S. granarius at a concentration of 500 ppm after an exposure period of 72 h (ur Rehman et al., 2021). Silver nanoparticles synthesized using extract of Spirogyra hyalina as a capping and reducing agent showed 30% mortality against T. castaneum at 500 ppm (Al Radadi et al., 2021). Curry leaves–mediated silver nanoparticles provided minimum seed damage (38.67%), minimum seed weight loss (0.78%), and absolute mortality (100%) of Callosobruchus chinensis at 70 ppm concentration on seventh day after treatment (Yerragopu et al., 2019). Plant-based silver nanoparticles from Ricinus communis and Citrus paradise gave highest repellency against T. castaneum in the range of 67.89% at 15% concentration of R. communis, and lowest was 28.31% at same concentration of the C. paradise oils (Shahzadi et al., 2019). Green silver nanoparticles prepared with peel extract of sweet orange, Citrus sinensis (L.), caused 83–77% mortality of T. confusum (Sedighi et al., 2019). Silver nanoparticles (AgNPs) at a concentration of 20% using an aqueous extract of hyperpigmented tomato skins resulted in 52% mortality of adults cowpea weevil (C. maculatus) (Carbone et al., 2020). Leaves extract taken from peach tree treated with AgNPs and ZnNPs showed 100% mortality of rice weevil (S.oryzea) and the lesser grain borer (R.dominica) by the fumigation method (Mosa et al., 2021).
4.1.2 Aluminum Oxide Nanoparticles (Al2O3NPs)
Nanostructured alumina (NSA) dust or aluminum nanoparticles are found to protect the grains from stored insect pest infestation (Stadler et al., 2017). Stadler et al. (2012) showed that pesticidal activity of nanoalumina is more effective than diatomaceous earth (DE) formulation against S. oryzae. Sabbour (2012) synthesized two entomotoxins made from nanoparticles of Al2O3 and TiO2 against S. oryzae. Among these, aluminum-based toxin was found more effective against S. oryzae as compared to nano TiO2. Nanoalumina dust of different size and morphology was tested against S. oryzae and R. dominica, and the higher mortality was recorded. But at the same time, it was concluded that reducing the size of the particle and increasing the surface area were not the only dominant factors that influence the effectiveness of insecticides.
Nanoalumina can be a good alternative to harmful dust formulations based on diatomaceous earth. Abo-Arab et al. (2014) reported that aluminum oxide nanoparticles caused 95.33% mortality of S. oryzae and 91.66% moratlity of S. zeamais at a concentration of 1 g kg−1 within 21 days of treatment. Similarly, Nasr (2015) proved the efficacy of Al2O3 nanoparticles against S. oryzae. Nanoaluminium oxide showed mortality of 100.0% when applied at the rate of 2 g kg−1 of rice, after 14 days against rice weevil (El-Bendary et al., 2016). López-García et al. (2018) tested nanostructured alumina dust (NSA) in 400 ml galvanized steel jars on S. oryzae, and it gave effective mortality rates. Comparably, Das et al. (2019) confirmed that treating S. oryzae with tested nanoparticles such as aluminum oxide at a dosage level of 1 g kg−1 killed 90% S. oryzae within 4 days. The insecticidal activity of NSA as seed protectant was evaluated against three insect pests; Oryzaephilus surinamensis, Stegobium paniceum, and Tribolium confusum. The insect mortality was found to be 100.00% when applied at the rate of 400 mg kg−1 for S. paniceum followed by 80.64% in O. surinamensis and 79.41% in T. confusum (Belhamel et al., 2020). Aluminum oxide nanoparticles showed 100% mortality at 8,000 mg/kg of wheat after 7 days of exposure; in addition, after 60 days of exposure, all tested Al2O3-NPs concentrations (1,000, 2000, 4,000, and 8,000 mg/kg grain) significantly decreased the number of S. oryzae offspring in a dose-dependent manner (Ismail et al., 2021).
4.1.3 Titanium Dioxide Nanoparticles (TiO2NPs)
These nanoparticles have low toxicity and minimal non-target biological effects (Ziental et al., 2020). The effectiveness is dependent on the chemical and physical characteristics including size, crystal structure, and photo-activation (Skocaj et al., 2011). The main advantage of using titanium dioxide formulations in storage is lesser ecological harm to non-target species (Norman and Chen, 2011). Abo-Arab et al. (2014) showed that titanium dioxide nanopartciles killed 61.66% of S. oryzae and 60.66% of S. zeamais at a dosage of 1 g kg−1 within 21 days Das et al. (2019) confirmed that treating S. oryzae with tested nanoparticles of titanium dioxide at a dosage level of 2 g kg−1 killed 90% S. oryzae within 14 days Sabbour (2012) demonstrated the toxicity of two NPs, TiO2 and Al2O3, against S. oryzae, both under lab and storage conditions in Egypt. Nano Al2O3 was found more effective than nano TiO2. A high concentration of TiO2 nanoparticles recorded high cumulative mortality of T. castaneum after exposure time 1, 3, and 5 days from treatments (15.30, 23.57, and 29.85%, respectively) (Hilal et al., 2021).
4.1.4 Zinc Oxide Nanoparticles (ZnONPs)
Zinc oxide nanoparticles have been used for developing pesticides because of their remarkable antimicrobial, physical, and optical properties (Akbar et al., 2020; Akintelu and Folorunso, 2020). Different methods can be utilized for their synthesis such as vapor transport and precipitation hydrothermal (Raoufi, 2013). The utilization of plant extracts for the purpose of developing ZnONPs is gaining popularity these days as these are quite safe and eco-friendly. Zinc oxide–based nanoformulations have been found effective in controlling S. oryzae and T. castaneum with higher mortality rates (Hamza 2012; Salem et al., 2015). Nasr (2015) performed a screening test to compare the effects of ZnO nanoparticles with pirmiphos methyl on S. oryzae. The results proved nano ZnO as an alternative to pirmiphos methyl which has harmful effects on human beings. Bacillus thuringiensis–coated zinc oxide nanoparticles (Bt-ZnO NPs) reduces fecundity, total development period, and causes 100% mortality of pulse beetle, C. maculatus, at 25 μg/ml (Malaikozhundan et al., 2017). ZnONPs exhibited a significant toxic effect against S. oryzae and C. maculatus at the highest concentration while T. castaneum showed high resistance (Haroun et al., 2020). Zinc oxide nanoparticles of size 100 nm at a concentration of 1,000 ppm showed 100% mortality of C. maculatus (Mohammed and Aswd, 2019), while in a more updated test, zinc oxide nanoparticles at 200 ppm gave the highest mortality of 100% and lowest egg production against the same pest in green gram (Lakshmi et al., 2020). ZnO nanoparticles in combination with Ricinus communis, Jatropha curcus, and Citrus paradise leaf powder showed 66.32% mortality of T. casteneum and 49.51% of T. granarium (Haider et al., 2020). ZnO prepared using the extract of E. globulus showed 80.5% mortality of R. dominica at 600 ppm after 15 days exposure (Siddique et al., 2021). Similarly, Hilal et al. (2021) concluded that a high concentration of ZnO nanoparticles recorded high cumulative mortality of T. castaneum after the exposure time 1, 3, and 5 days from treatments (20.42, 27.08, and 33.96%, respectively). Chitinase from Lactobacillus coryniformis-incorporated ZnONPs gave 100% mortality of maize weevil, Sitophilus zeamais, at a concentration of 6 mg/L, and the average death rate was 2.4 days (Dikbaş et al., 2021).
However, zinc oxide nanoparticles are found to be less effective in controlling the storage pest’s population as compared to other nanoparticles such as silver, aluminum oxide, and titanium dioxide (Raduw and Mohammed, 2020; Wazid et al., 2020). In a study, Das et al. (2019) evaluated aluminum oxide, titanium dioxide, and zinc oxide nanoparticles against S. oryzae. Nanoaluminium oxide gave 90% mortality at 1 g kg−1 after 4 days, whereas nano zinc oxide and titanium dioxide achieved this level at 2 g kg−1 after 14 days. Therefore, it justifies the fact that zinc oxide nanoparticles are less effective in controlling storage pests. Still, due to its antimicrobial properties, it is recommended for usage in agricultural pest control.
4.1.5 Copper Nanoparticles (CuNPs) and Iron Oxide Nanoparticles (FeNPs)
Biotransformed CuNPs using Pseudomonas fluorescens MAL2, which is almost similar to Pseudomonas fluorescens DSM 12442T DSM, act as a great warrior against T. castaneum (El-Saadony et al., 2020). CuO-NPs fabricated by harnessing metabolites of Aspergillus niger strain (G3-1) gave a mortality percentage of 55–94.4% and 70–90% against S. granaries and R. dominica, respectively (Badawy et al., 2021). A combination of iron oxide nanoparticles and aqueous extract of Anthocephalous cadamba exhibits 100% mortality against S. granarius (Sivapriya et al., 2018).
4.2 Silicon Dioxide Nanoparticles (SiO2NPs)
These are also known as silica nanoparticles and have higher thermal stability, low toxicity, and excellent biocompatibility with a range of molecules and polymers (Rahman and Padavettan, 2012; Huang et al., 2019). These can act as excellent nanocarriers for different agrochemicals due to their mesoporous property (Rastogi et al., 2019). Silica has a wider applicability because of its shape, size, porosity, and crystallinity which can be precisely manipulated. These can act as excellent nanocarriers for the delivery of biopesticides, pheromones, fungicides, and growth promoters (Barik et al., 2008; Cáceres et al., 2019).
Nanotubes reported with aluminosilicate have the capacity to attach to plant surfaces and hair of insects through which they enter into the insect body and affect their body functions (Patil et al., 2009). Silica-based nanoformulation was tested against red flour beetle T. castaneum and gave effective control of the pest (Debnath et al., 2011). SiO2 gave 100% mortality of cowpea weevil, C. maculatus, when applied at the rate of 2.06 g kg−1 (Rouhani et al., 2013). Chlorpyrifos-loaded silica nanoparticles (Ch-SNPs) effectively control R. dominica and T. confusum, and it was found that mortality increased with increased concentration (Babamir-Satehi et al., 2017). Similarly, Kallur and Patil (2019) reported that nano-SiO2–based nanoparticles extracted from Alstonia scholaris showed higher toxicity with a LC50 of 0.8 mg/ml and LC95 of 1.95 mg/ml against R. dominica. Silver nanoparticles in combination with plant oil Ricinus communis showed 67.89% repellency at 15% concentration and 28.31% repellency at the same concentration in combination with Citrus paradise oil against T. castaneum (Shahzadi et al., 2019). SiO2 nanoparticles showed a strong defensive response against Caryedon serratus in groundnut at 0.67 mg/kg and 1.7 mg/kg, and it was observed that mortality increased with time of exposure (Diagne et al., 2019). Inorganic nanosilica killed 50% of adults T. castaneum at 0.90% concentration after 10 days of treatment (Khalil, 2019). SiO2 nanoparticles act as a strong defensive tool against Caryedon serratus in groundnut at 0.67 mg/kg and 1.7 mg/kg, and mortality increased with time of exposure (Diagne et al., 2019). Rouhani et al. (2019) proved that silica nanoparticles (SNPs) are highly effective against S. granarius causing 100% mortality after 2 weeks. Fumed silica (aerosil 200) nanoparticles at a concentration of 1.5 and 2 gm/kg caused 100% mortality of T. granarium and Stegobium paniceum after 5 and 2 days of treatment (Abdelfattah and Zein, 2019).
Silica nanoparticles prepared with the help of sol-gel technique effectively controlled R. dominica, T. castaneum, and O. surinamensis (El-Naggar et al., 2020). Fumigation of maize grains with SiO2-NPs proved very effective against four common stored grain pests, namely, S. oryzae, R. dominica, T. castaneum, and Orizaephilus surinamenisis (El-Naggar et al., 2020). Silica nanoparticles along with neem leaf extracts effectively reduced egg production of T. castaneum and C. maculatus to 58 eggs/female and nine eggs/female, respectively, at a concentration of 2,000 ppm (Annon et al., 2020). Ziaee and Babamir-Satehi (2020) loaded nanosilica with three ingredients deltamethrin, pyriproxyfen, and chlorpyrifos and tested it against the larvae of T. granarium feeding on stored wheat using mosaic and galvanized steel surfaces. Deltamethrin, followed by chlorpyrifos, was found to be the most effective against larvae of T. granarium, and the mortality was significantly higher in galvanized steel than the mosaic ones. Green silica nanoparticles (1,500 ppm) synthesized using spinach leaves, tulsi leaves, and paddy husk proved to be an alternative to Malathion against storage pests (Wazid et al., 2020). Three silica forms, that is, Aerosil 200, chemical, and bio-silica in the form of nanoparticles were tested against C. maculatus, R. dominica, and T. confusum. All the tested materials caused mortality of adult and offspring of the three tested insects, and mortality increased with the increase of concentration and exposure period. The adults C. maculatus were more susceptible followed by R. dominica and T. confusum with the three tested materials at all concentrations used (Salem, 2020).
Insect proof nets coated with silica nanoparticles showed absolute mortality against S. oryzae (Agrafioti et al., 2020). Nanosilica of 30 nm size at a concentration of 0.5 g per kg of rice showed mortality rate of 80 and 97.4% against S. oryzae after 7 and 14 days, respectively (Kar et al., 2021). Nanosilica prepared from sugarcane bagasse ash (SCBA) and used as an additive to the diatomaceous earth (DE) enhanced the insecticidal activity of Mamaghan DE, and after 14 days of exposure, the adult mortality was more than 86 and 95% for T. confusum and R. dominica, respectively (Saed et al., 2021).
4.3 Nanoemulsions
Numerous nanoemulsions have been tested for controlling stored grain pests. These nanoemulsion formulations can improve the effectiveness of botanical insecticide for commercial use and are target-specific (Frederiksen et al., 2003; Anjali et al., 2012). The effectiveness of these formulations can be increased by using adjuvants and surfactants. These are relatively cheaper because their water solubility is high and can solubilize hydrophilic and lipophilic compounds easily, thus requiring less amount of active ingredients and inert material. Moreover, storage stability is quite high under a broad range of temperatures (−10–55°C) (Pavoni et al., 2019). Essential oil nanoformulations have been tested as an alternative for synthetic pesticides. Using such formulations can help in overcoming the problems such as poor water solubility, degradation, and volatility (Martín et al., 2010). These types of formulations are anticipated to be more productive than bulk substances (Anjali et al., 2010, 2012). In the past 10 years, essential oil–based nanoemulsions have proved their strong potency in the management of storage pests (Pavoni et al., 2019). For instance, eucalyptus oil nanoemulsion comprising of Karanja’s and Jatropha’s aqueous filtrate at concentrations of 300 and 1,500 ppm resulted in 88–100% mortality of T. castaneum adults within 24 h duration (Pant et al., 2014); Pimpinella anisum L. (Apiaceae) essential oil–based nanoemulsion consisting of 81.2% of (E)-anethole showed the toxicity of (LC50 = 9.3% v/v) and significantly decreased progeny of rust-red flour beetle (Hashem et al., 2018; Mohammed and Nasr, 2020). Tested essential oil nanoemulsion of eucalyptus oil of a particle size of 8.57 nm was found to have higher insecticidal activity against T. castaneum. Similarly, eucalyptus oil nanoemulsions were passed across centrifugation, thermodynamic stability, heating and cooling, and freezing and thawing experiments and tested at lethal concentrations (LC50) of 1:2 and 1:2.5 against T. castaneum, that is, 0.56 and 0.45 μL/cm2 and were proved to be highly toxic (Adak et al., 2020). Nenaah (2014) showed that nanoemulsions of Achillea biebersteinii, A. santolina, and A. mellifolium essential oils are effective against both larvae and adults of T. castaneum. Furthermore, A. biebersteinii EO nanoemulsion exhibits some additional efficacy against the adults of red flour beetle. Some more essential oil nanoformulations that proved effective against stored insect pests are Jojobe (Simmondasia chinensis) nanoemulsion (Sh et al., 2015); Purslane oil nanoemulsion (Sabbour et al., 2016); and oregano and thyme essential oil–based nanoemulsion (Hossain et al., 2019).
Nanoemulsion developed from sweet orange (Citrus sinensis) EO formulation was reported to be acutely toxic to confused flour beetle and flat grain beetle (Giunti et al., 2019). Dhivya et al. (2019) studied the potential of sweet flag oil (Acorus calamus) nanoemulsion (spontaneous emulsification) against S. oryzae. Mentha spicata L. and Mentha pulegium L. essential oils nanoformulations showed mortality of 95.47 and 86.03%, respectively, against T. castaneum (Heydarzade et al., 2019). Nanoemulsion made from neem oil (Azadirachta indica A. Juss) was 85–100% effective against S. oryzae and 74–100% effective against T. castaneum (Choupanian et al., 2017). Hazomalania voyronii essential oil–based nanoemulsion (HvNE) resulted in 92.1, 97.4, and 100.0% mortality against T. confusum, T. castaneum larvae, and T. molitor adults, respectively, at 1,000 ppm concentration in 7 days (Kavallieratos et al., 2021a). Similarly, Kavallieratos et al. (2021b) developed isofuranodiene-based NE 3% (w/w) derived from Smyrnium olusatrum essential oil (EO) that exhibited high adulticidal effects against T. molitor and larvicidal activity against T. castaneum and T. confusum, reaching 98.6, 97.4, and 93.5% at 1,000 ppm after 7 days of exposure, respectively. Cumin essential oil (Cuminum cyminum L.) nanoemulsion was found to be highly toxic to T. castaneum (Hashem and Ramadan, 2021). Larvae of E. kuehniella were killed with nanoformulation in the form of emulsion using a botanical extract of M. longifolia having a particle range of 14–36 ppm (Louni et al., 2018). Similarly, the same formulation of M. longifolia had higher fumigant toxicity against C. maculatus and was successful in killing 50% egg population at a concentration of 9 μL/L in 4.7 days (Louni et al., 2019). Nanoencapsulated Janesville oil was found more effective against T. confusum and T. castaneum than coriander or black seed oil. (Sabbour et al., 2020a). Nanoformulations of the Tasmanian blue gum (TBG) EO effectively controlled the population of C. maculatus (Ya-Ali et al., 2020). Nanoemulsions prepared by extracting essential oils of Anise (Pimpinella anisum), Artemisia (Artemisia vulgaris), fennel (Foenicum vulgare), garlic (Allium sativum), lavender (Lavandula angustifolia), mint (Mentha piperita), rosemary (Rosmarinus officinalis), and sage (Salvia officinalis) showed considerable toxicity against T. confusum. However, the highest toxicity was observed by the application of garlic oil nanoemulsion, and the best repellent was found to be P. anisum (Palermo et al., 2021).
Very recently, a new study indicated that continuous use of essential oil nanoformulations against storage pests could give rise to habituation. A test conducted against R. dominica by using nanoemulsions of fennel (Foeniculum vulgare), mint (Mentha x piperita), and sweet orange (Citrus sinensis) essential oils showed that habituation occurred in the case of M. piperita and C. sinensis application (Giunti et al., 2021). Cold aerosol and gel nanoemulsion formed using Allium sativum’s oil showed the highest toxicity, and the best repellent was nanoemulsion of Pimpinella anisum against T confusum (Palermo et al., 2021). Nanoemulsion prepared with Achillea biebersteinii essential oil at 10 μL/L air caused 100% mortality of the second larvae of T. castaneum after 4 days of exposure (Almadiy, 2021).
4.4 Polymer-Based Nanopesticide Formulations
The development of controlled release formulations of insecticides, herbicides, and fungicides using polymers for pest management programs is presently being given major attention keeping in mind the protection of the photo-liable active ingredients (Neri-Badang and Chakraborty, 2019). Polymer-based delivery systems increase the dispersion of active ingredients in aqueous media and act as a protective reservoir covering that leads to a controlled release of active ingredients (Kalia et al., 2020) (Figure 4). Slow release of active ingredients depends on nanocarrier’s degradation properties, bonding between active ingredients and the carrier, and the weather factors (Kumar et al., 2019). A number of polymer nanoformulations such as nanocapsules, nanospheres, nanogels, micelles, nanofibers, and chitosan-based nanoformulations have recently been developed. Polymeric nanomaterials are most commonly used for encapsulation of active ingredients mainly due to their eco-friendly and biodegradable nature (Kumar et al., 2017; Ramasamy et al., 2017). The benefit of using polymers is their protection and stability against active ingredients such as essential oils and plant secondary metabolites that have stability issues. These compounds get degraded and evaporated when they come in contact with light, water, air, and high temperatures. According to the current scenario, biodegradability is the most important property that a polymer must have.
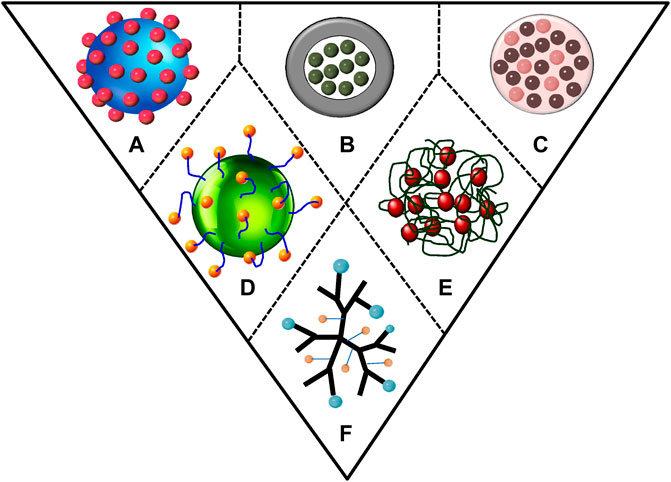
FIGURE 4. Types of polymer-nanoparticles formulations for delivery of pesticides via (A) adsorption on nanoparticle, (B) encapsulation within polymeric spheres (nanocapsule), (C) entrapment within polymeric nanoparticles (nanosphere), (D) attachment on nanoparticle by different linkers, (E) entrapment within polymeric micelles, and (F) branched and ordered polymeric molecules (dendrimers).
Polymer-based nanoformulations can provide improved efficiency of active ingredients with minimized lethal effects on the ecosystem due to reduced use of organic solvents and surfactants in the formulations (Li et al., 2018). Due to polymer-based controlled formulations spatial and temporal doses are reduced and stability and effectiveness are improved (Chen and Yada, 2011). They are also attractive to researchers due to their complex delivery systems by incorporating multiple active ingredients with different modes of action, biocompatibility, and biodegradability (Tong et al., 2017). The polymers deployed for nanopesticide formulations consist mainly of polysaccharides (e.g., chitosan, alginates, and starch) and polyesters (e.g., poly-ε-caprolactone and polyethylene glycol) (Kashyap et al., 2015; Rani et al., 2017; Kumar et al., 2018). Nowadays, there has been an increased trend of using eco-friendly and biodegradable natural materials such as lecithin and corn oil (Nguyen et al., 2012; Kashyap et al., 2015) and cashew gum (Abreu et al., 2012).
Polyethylene glycol–based amphiphilic copolymers exhibit biodegradability, easy processing, and other well-explored properties (Torchilin, 2006). Therefore, they are considered the most attractive polymers. In addition, PEG-based formulations are considered superior in comparison with other commercial products for the purpose of insect pest as well as nematode control (Loha et al., 2012; Pankaj et al., 2012). Polyethylene glycol (PEG)–coated nanoparticles loaded with garlic essential oil effectively control red flour beetle, T. castaneum (Herbst) (Yang et al., 2009). A comparative toxicity study between nanostructured alumina (NSA)- and Protect-It diatomaceous earth (DE)–based products showed that NSA is much more effective in killing R. dominica than Protect-It® (Stadler et al., 2012). Therefore, NSA is an alternative to diatomaceous earth (DE)–based products as it decreases significant percentage of progeny production and can be used for future formulations of pesticides. Vadlapudi and Amanchy (2017) developed a moderate control of R. dominica by the formation of silver nanoparticles (AgNPs) and leaf extracts of Myriostachya wightiana (essential oil). Likewise, nanoemulsion of Eucalyptus essential oil with a particle size of 8.57 nm and polydispersity index (PDI) 0.28 effectively controlled R. dominica as compared to essential oil alone, which shows a great potential of nanoformulation-based products (Mohammed and Nasr, 2020). Very recently, citronella oil, moringa oil, and goatweed extracted from (Ageratum conyzoide) were converted into nano-oils via polymerization technology and were tested at the concentration of (0.5%) on the third instar larvae of R. dominica showed a highest accumulative mortality (99.0%, 89, 87%, respectively) (Sabbour et al., 2020b). In many studies, essential oils that were not much effective in controlling storage pest attack have been proved as a strong product for controlling the same when formulated with nanotechnology. Likewise, Jesser et al. (2020) studied the relationship between insecticidal activities of EO or essential oil–loaded polymeric nanoparticles (EOPN) and also kept notice on post-application temperature in the research. In contact toxicity bioassay, palmorosa proved to be the most efficacious, while in a fumigant bioassay, palmorosa along with peppermint oil were found to be effective. In addition, no effect of environmental variation was noticed. Delivering dsRNA by the utilization of polyamidoamine dendrimer–coated carbon nanotubes (PAMAM-CNT-dsRNA) help in raising the level of RNA interference in T. castaneum (Edwards et al., 2020). Thereby, PAMAM-CNT-dsRNA increases the efficacy of gene knockdown in red flour beetle. Clove essential oil (Syzygium aromaticum)–loaded polyethylene glycol (PEG) nanoparticles shows high rate of mortality against T. castaneum (Ikawati et al., 2021).
4.5 Chitosan-Based Formulations
Chitosan is a bioactive polymer formed by the deacetylation of chitin, which is one of the most abundant natural polysaccharides (Badawy et al., 2011). Only a few studies have been carried out on the efficacy of the chitosan-based formulations against storage insect pests. Myristic acid–chitosan (MA chitosan)–based nanogel loaded with Carum copticum (L.) essential oil (EO) was found effective against S. granarius and T. confusum, and the studies indicated that toxicity effect increased with the increasing exposure time (Ziaee et al., 2014). Peppermint oil (PO)–encapsulated chitosan nanoparticles were found highly toxic against S. Oryzae (Rajkumar et al., 2020). Melissa officinalis essential oil nanoencapsulation in the chitosan matrix improved fumigant activity (LC50 = 0.048 μL/ml air) and acted as a strong antifeedant (EC50 = 0.043 μL/ml) against T. castaneum (Upadhyay et al., 2019). Likewise, chitosan- and polycaprolacton-encapsulated R. officinalis and Zataria multiflora essential oil nanoparticles effectively controlled confused flour beetle (Ahsaei et al., 2020).
4.6 Nanocapsules
Nanoencapsule formulation developed using C. cyminum polymerized oil/water emulsion (using poly(urea-formaldehyde)) when sprayed on rust-red flour beetle continuously for 7 days resulted in LC50 value of 16.25 ppm, which is lower than the value of oil tested alone (Negahban et al., 2012). Nanocapsule of R. officinalis essential oil–loaded formulation found effective against T. castaneum (Khoobdel et al., 2017). Cysteine protease nanocpasule of Albizia procera (ApCP) resulted in 100% mortality of Sitotroga cerealella at 7.0 and 3.5 mg/g concentrations (Batool et al., 2021). Likewise, nanoencapsulated Eucalyptus globulus and Z. multiflora essential oils were found effective for the control of E. kuehniella (Emamjomeh et al., 2021). Nanoparticles encapsulated with Artemisia haussknechtii essential oil showed 100% mortality at 166 ppm (Khanahmadi et al., 2017). The combination of nanocapsulated form of Cuminum cyminum essential oil with reduced amounts of phosphine controlled 50% population of S. granarius and T. castaneum at a concentration of 42.51 and 78.99 μL/L, respectively, and Lavandula angustifolia can also be used as it is on par with cumin oil (Bayramzadeh et al., 2019).
5 Concerns About Nanopesticides Usage
One of the considerations for favoring nanopesticides over conventional pesticides is that these lessen environmental contamination through the reduction in pesticide application rates and losses (Jasrotia et al., 2018; Luiz de Oliveira et al., 2018). Conversely, these may pose a new problem of longer persistence and higher toxicity. A small droplet size may also lead to early evaporation of the nanodroplets before reaching the target. The interaction of nanoformulations with microorganisms, plants, and other animals at different trophic levels is another major area requiring investigation. Moreover, the environmental fate of pesticide nanoformulations on soil, groundwater, and non-target organisms is unknown. The properties of the nanocarriers and the dispersion of the active ingredient within the nanoformulation matrix determine the release of the active ingredients into the environment. It has been reported that delayed release of nanoparticles over an extended period of time may affect non-target organisms (Kah et al., 2013). Nanocarriers mostly used in nanoformulations are either natural polymers or polysaccharides or lipids, which degrade easily but very little concern has been raised toward the use of non-biodegradable nanocarriers such as metal and metal oxides (Kah and Hofmann, 2014). Moreover, most of the synthesized nanocarriers are for controlled release that may cause human body exposure of nanoformulations in a restricted manner (Gahukar and Das, 2020). Conventional formulations require use of harmful organic solvents in developing pesticidal nanoformulations, and these solvents are highly toxic to the environment.
Regulations for the registration and introduction of nanoproducts into the market are lacking. There is an urgent need to develop standard guidelines for the evaluation of nanochemicals before registration and commercialization as it is pertinent to understand their behavior, efficacy, toxicity, physicochemical properties, and environmental effects. The research studies regarding comparison of nanopesticides with their conventional analogues in all aspects are necessary to guide future research regarding the environmental risks of nanopesticides using the life-cycle analysis. This analysis should take into consideration all the steps of nanoformulations production, application, incorporation levels into food chain, and possible effects on agrosystem conditions that may influence hazardous properties and risk characterization nanomaterials. There is a growing concern in the scientific community about toxicity and impact of nanopesticide formulations on the ecosystem due to their widespread applications, which requires more investigation and research in these areas. Therefore, more efforts should be made to search and find out the way for the development of safer and smarter nanoformulations.
6 Regulations of Nanopesticides
The increasing trend of usage of nanomaterials in various fields is on the rise in today’s hi-tech world. However, at the same time, environmental risk assessment of nanomaterials such as nanopesticides is very essential before a product can be placed on the market worldwide. Risk assessment of nanomaterials, their persistence, behavior, and fate in the environment should be determined prior to their release. The regulatory and legislative bodies around the globe are required to ensure to account for their safe usage and successful risk assessment. Nanoparticles, because of their characteristic features such as size, surface area, and catalytic properties, require additional testing beyond guidelines for setting their toxicity levels (Singh et al., 2020). Various national and international regulatory bodies are trying to ensure safety of nanomaterials. In some regions including Asia, Africa, and Oceania, heterogenous methods are used for the regulation of nanoproducts and these methods vary country-wise (Kookana et al., 2014). For instance, recently in India, guidelines for the evaluation of nano-agri input products and nano-agriproducts have been framed that defined nanomaterials either as a nanofertilizer or nanopesticide (Naqvi and Flora, 2020). The Scientific Advisory Panel of the US Federal Insecticide, Fungicide, and Rodenticide Act (FIFRA) has contemplated pesticides made of nanometals/or metal oxides and has recommended that potential health and environmental risks of such nanomaterials should be taken into consideration before their market release (Grillo et al., 2021). Furthermore, in Europe, plant protection products (PPPs) are mainly regulated by Regulation (EC) No 1107/2009 in which prior authorization is required from the government (Villaverde et al., 2014; Cao et al., 2020). The official control of nanomaterials’ residues in the food is regulated under (EC) No 396/2005 (European Food Safety Authority et al., 2019), and labor safety from these chemicals is covered by the REACH Regulation (EC) No 1907/2006, which gives information to manufacturers and importers about the risks associated with their usage (Tranfo et al., 2020). The Australian Pesticides and Veterinary Medicines Authority (APVMA) also framed regulations for the release of nanomaterials (Bowman & Hodge, 2009; Walker et al., 2017). Priorities in R&D sector of countries around the globe must focus on reducing ecotoxicity of nanopesticides by standardization of testing guidelines for nanopesticides; a better understanding of hazards posed by nanopesticides and their degradation products; increasing time of exposure of nanopesticides tests against particular organisms; and identification of nanopesticide that comes under boundaries for regulatory coverage.
7 Conclusion and Future Directions
Even after countless efforts, food security is still challenging because of limited resources and rising population. The smart nanopesticides can have advantages over conventional agrochemicals such as lower dose requirement, higher solubility, and targeted delivery of active ingredient leading to higher eco-protection with lesser environmental ill-effects (Dubey and Mailapalli, 2016). Despite several advantages, nanoparticles also pose serious drawbacks such as low selective toxicity, low biodegradability of inorganic nanoparticles, and development of pesticide resistance in non-target organisms, if used in an indiscriminate way. Some nanosystems are at their beginning stage or are under development. Moreover, the data concerning the environmental fate of these nanoparticles and their possible negative impact on non-target organisms is scarce, and there is lack of knowledge in this regard. Increased attention must be paid toward the possible impact and reverberations that nanomaterials can have on the environment, non-target organisms, and development of ecologically safer nanopesticides. Scientists need to dwell deeper into research related to precautions to be taken, mutations in food due to nanostructured materials (NSMs), and nanoparticles toxicity in human cells and their environmental impacts (Chhipa et al., 2017b; Sahoo et al., 2021). Only the research community can decide nanotechnology can bring more positive impacts than potential risks. The concept of “green nanotechnology” that is being posed as one of the benefits of nanoproducts need to be assessed by investigating the potential risks involved with their use. The sustainability of these products in addition to their safety and risks should be also need to be assessed, including the cost of production, environmental impact, and renewability of all material used for synthesis and production (Watson et al., 2011; Chowdappa and Gowda, 2013).
Future research in nanotechnology should be focused on 1) development of smart nanopesticide formulations to combat the limitations of conventional formulations, 2) development of environmentally sustainable green nanopesticides chemistry, 3) development of technologies for cheap and commercial nanopesticides production, 4) activity comparison of nanoformulations with conventional analogues at the field level to determine their practical utility, 5) ecotoxicological assessment of nanopesticides, and 6) establishment of legislative and regulatory framework for the safe introduction of nanopesticides in agriculture. Except minuscule limitations, nanomaterials have immense potential of transforming crop protection practices from environment harming to an environment favoring. It makes agriculture cost-effective too. This kind of development will improve the food life, storability, and security and in turn both the costumers and producers will receive more profit.
Author Contributions
PJ: conceptualized the topic of the review and wrote the article; MN: collected and complied all the literature; CM, AS, SK, and UK: assisted in the writing of the manuscript; AB, PK, SK, and GS: assisted in writing, reviewing, and finalized the article for submission.
Conflict of Interest
The authors declare that the research was conducted in the absence of any commercial or financial relationships that could be construed as a potential conflict of interest.
Publisher’s Note
All claims expressed in this article are solely those of the authors and do not necessarily represent those of their affiliated organizations, or those of the publisher, the editors, and the reviewers. Any product that may be evaluated in this article, or claim that may be made by its manufacturer, is not guaranteed or endorsed by the publisher.
Supplementary Material
The Supplementary Material for this article can be found online at: https://www.frontiersin.org/articles/10.3389/fnano.2021.811056/full#supplementary-material
References
Abbood, N. M., and Ali, S. T. (2020). The Role of Silver Nanoparticles and Some Extracts of Damas Conocarpus lancifolius in Controlling Stored Dates Moth Ephestia cautella (Walker). Plant Arch. 20, 3301–3305.
Abdelfattah, N. A., and Zein, D. M. (2019). Biological Studies and Toxicity Experiments of AEROSIL 200 Nanoparticles on Adults and Larvae of Some Stored Grain Insects. Int. J. Entomol. Res. 4 (1), 103–108.
Abo-Arab, R. B., Amal, M. H., and Hashem, A. S. (2014). Comparative Bioactivity of Aluminum Oxide (Al2O3), Titanium Dioxide (TiO2) Nanoparticles and Malathion on Sitophilus Oryzae L. And Sitophilus zeamais (Motsch.). Glob. J. Agric. Food Saf. Sci. 1 (2), 25–37.
Abreu, F. O. M. S., Oliveira, E. F., Paula, H. C. B., and de Paula, R. C. M. (2012). Chitosan/cashew Gum Nanogels for Essential Oil Encapsulation. Carbohydr. Polym. 89 (4), 1277–1282. doi:10.1016/j.carbpol.2012.04.048
Adak, T., Barik, N., Patil, N. B., Govindharaj, G.-P. -P., Gadratagi, B. G., Annamalai, M., et al. (2020). Nanoemulsion of eucalyptus Oil: An Alternative to Synthetic Pesticides against Two Major Storage Insects (Sitophilus Oryzae (L.) and Tribolium castaneum (Herbst)) of rice. Ind. crops Prod. 143, 111849. doi:10.1016/j.indcrop.2019.111849
Agrafioti, P., Faliagka, S., Lampiri, E., Orth, M., Pätzel, M., Katsoulas, N., et al. (2020). Evaluation of Silica-Coated Insect Proof Nets for the Control of Aphis fabae, Sitophilus Oryzae, and Tribolium confusum. Nanomaterials 10 (9), 1658. doi:10.3390/nano10091658
Ahsaei, S. M., Talebi-Jahromi, K., and Amoabediny, G. (2020). Insecticidal Activity of Polycaprolactone Nanoparticles Decorated with Chitosan Containing Two Essential Oils against Tribolium confusum. Int. J. Pest Manag., 1–9. doi:10.1080/09670874.2020.1825875
Aithal, P. S., and Aithal, S. (2015). Ideal Technology Concept & its Realization Opportunity Using Nanotechnology. Int. J. Appl. or Innovation Engineering&Management (Ijaiem) 4 (2), 153–164.
Akbar, S., Tauseef, I., Subhan, F., Sultana, N., Khan, I., Ahmed, U., et al. (2020). An Overview of the Plant-Mediated Synthesis of Zinc Oxide Nanoparticles and Their Antimicrobial Potential. Inorg. Nano-Metal Chem. 50 (4), 257–271. doi:10.1080/24701556.2019.1711121
Akintelu, S. A., and Folorunso, A. S. (2020). A Review on green Synthesis of Zinc Oxide Nanoparticles Using Plant Extracts and its Biomedical Applications. BioNanoScience 10 (4), 848–863. doi:10.1007/s12668-020-00774-6
Al-Jubury, H. M. K., Baker, S. Z., and Ahmed, Z. S. (2020). Studying the Effectiveness of Bio-Prepared Silver Nanoparticles by the Beauveria Bassiana Fungus in Some Biological Aspects for Cowpea Weevil (Callosobruchus maculatus (Fab)) In Vitro. Euphrates J. Agric. Sci. 12 (2).
Al-Radadi, N. S., Hussain, T., Faisal, S., and Shah, S. A. R. (2021). Novel Biosynthesis, Characterization and Bio-Catalytic Potential of green Algae (Spirogyra Hyalina) Mediated Silver Nanomaterials. Saudi J. Biol. Sci. 14, 2021. doi:10.1016/j.sjbs.2021.09.013
Ali, Q. M., Abbas, M., and Arif, S. (2013). Monitoring of Resistance against Phosphine in Stored Grain Insect Pests in Sindh. Middle East. J. Sci. Res. 16, 1501–1507.
Almadiy, A. A. (2021). Insecticidal and Acetylcholinesterase Inhibitory Activities of Achillea biebersteinii Essential Oil and its Nanoemulsion and Major Monoterpenes against Tribolium castaneum. J. Asia-Pacific Entomol. 24 (4), 1170–1178. doi:10.1016/j.aspen.2021.11.002
Alzogaray, R. A., and Zerba, E. N. (2017). Rhodnius prolixus Intoxicated. J. Insect Physiol. 97, 93–113. doi:10.1016/j.jinsphys.2016.04.004
Anandhi, S., Saminathan, V. R., Yasotha, P., Saravanan, P. T., and Rajanbabu, V. (2020). Nano-pesticides in Pest Management. J. Entomol. Zoolog. Stud. 8 (4), 685–690.
Anjali, C. H., Khan, S. S., Margulis-Goshen, K., Magdassi, S., Mukherjee, A., and Chandrasekaran, N. (2010). Formulation of Water-Dispersible Nanopermethrin for Larvicidal Applications. Ecotoxicology Environ. Saf. 73 (8), 1932–1936. doi:10.1016/j.ecoenv.2010.08.039
Anjali, C. H., Sharma, Y., Mukherjee, A., and Chandrasekaran, N. (2012). Neem Oil (Azadirachta indica) Nanoemulsion—A Potent Larvicidal Agent against Culex quinquefasciatus. Pest Manag. Sci. 68 (2), 158–163. doi:10.1002/ps.2233
Annon, M. R., and Jafar, F. S. (2020). “November)The Effectiveness of Silver and Silica Nanoparticles on Productivity and Adult Emergence of T. castaneum and C. maculatus,” in Journal of Physics: Conference Series, Volume 1664, 1st International Virtual Conference on Pure Science 10th -11th June 2020, College of Science, University of Al-Qadisiyah, Iraq (IOP Publishing), 012110. doi:10.1088/1742-6596/1664/1/012110
Aouada, F. A., and De Moura, M. R. (2015). “Nanotechnology Applied in Agriculture: Controlled Release of Agrochemicals,” in Nanotechnologies in Food and Agriculture (Cham: Springer), 103–118. doi:10.1007/978-3-319-14024-7_5
Arumugam, G., Velayutham, V., Shanmugavel, S., and Sundaram, J. (2016). Efficacy of Nanostructured Silica as a Stored Pulse Protector against the Infestation of Bruchid Beetle, Callosobruchus maculatus (Coleoptera: Bruchidae). Appl. nanoscience 6 (3), 445–450. doi:10.1007/s13204-015-0446-2
As, A. A., and Thangapandiyan, S. (2019). Comparative Bioassay of Silver Nanoparticles and Malathion on Infestation of Red Flour Beetle, Tribolium castaneum. J. Basic Appl. Zoolog. 80 (1), 1–10. doi:10.1186/s41936-019-0124-0
Athanassiou, C. G., Kavallieratos, N. G., Benelli, G., Losic, D., Usha Rani, P., and Desneux, N. (2018). Nanoparticles for Pest Control: Current Status and Future Perspectives. J. Pest Sci. 91, 1–15. doi:10.1007/s10340-017-0898-0
Attia, M. A., Wahba, T. F., Shaarawy, N., Moustafa, F. I., Guedes, R. N. C., and Dewer, Y. (2020). Stored Grain Pest Prevalence and Insecticide Resistance in Egyptian Populations of the Red Flour Beetle Tribolium castaneum (Herbst) and the rice weevil Sitophilus oryzae (L.). J. Stored Prod. Res. 87 (3), 101611. doi:10.1016/j.jspr.2020.101611
Attia, Z., Pogoda, C. S., Reinert, S., Kane, N. C., and Hulke, B. S. (2021). Breeding for Sustainable Oilseed Crop Yield and Quality in a Changing Climate. Theor. Appl. Genet., 1–11. doi:10.1007/s00122-021-03770-w
Awika, J. M. (2011). Major Cereal Grains Production and Use Around the World. ACS Symp. Ser. 1089, 1–13. doi:10.1021/bk-2011-1089.ch001
Babamir-Satehi, A., Ziaee, M., and Ashrafi, A. (2017). Synthesis and Toxicological Evaluation of Silica Nanoparticles as Chlorpyrifos Carrier against the Beetle Pests Rhyzopertha dominica and Tribolium confusum. J. Entomol. Soc. Iran 37 (2), 235–247. doi:10.1127/entomologia/2017/0406
Badawy, A. A., Abdelfattah, N. A., Salem, S. S., Awad, M. F., and Fouda, A. (2021). Efficacy Assessment of Biosynthesized Copper Oxide Nanoparticles (Cuo-nps) on Stored Grain Insects and Their Impacts on Morphological and Physiological Traits of Wheat (Triticum aestivum L.) Plant. Biology 10 (3), 233. doi:10.3390/biology10030233
Badmin, J. S. (1990). “IRAC Survey of Resistance of Stored Grain Pests: Results and Progress,” in Proceedings, 5th International Working Conference On Stored-Products Protection (IEEE), 1029–1037.
Banga, K. S., Kumar, S., Kotwaliwale, N., and Mohapatra, D. (2020). Major Insects of Stored Food Grains. Int. J. Chem. Stud. 8 (1), 2380–2384. doi:10.22271/chemi.2020.v8.i1aj.8624
Barik, T. K., Sahu, B., and Swain, V. (2008). Nanosilica-from Medicine to Pest Control. Parasitol. Res. 103 (2), 253. doi:10.1007/s00436-008-0975-7
Bartolucci, C., Antonacci, A., Arduini, F., Moscone, D., Fraceto, L., Campos, E., et al. (2020). Green Nanomaterials Fostering Agrifood Sustainability. Trac Trends Anal. Chem. 125, 115840. doi:10.1016/j.trac.2020.115840
Batool, M., Hussain, D., Akrem, A., ul Haq, M. N., Saeed, S., and Saeed, Q. (2021). Nanoencaspsulation of Cysteine Protease for the Management of Stored Grain Pest, Sitotroga cerealella (Olivier). J. King Saud University-Science 33 (4), 101404. doi:10.1016/j.jksus.2021.101404
Bayramzadeh, N., Mehrkhou, F., Pourmirza, A. A., and Mahmoudian, M. (2019). Fumigant Toxicity of Two Nano-Capsulated Essential Oils with Sublethal Rate of Phosphine against Three Stored-Product Pests. J. Agric. Sci. Tech. 21 (4), 857–872.
Bazana, M. T., Codevilla, C. F., and de Menezes, C. R. (2019). Nanoencapsulation of Bioactive Compounds: Challenges and Perspectives. Curr. Opin. Food Sci. 26, 47–56. doi:10.1016/j.cofs.2019.03.005
Belhamel, C., Boulekbache–Makhlouf, L., Bedini, S., Tani, C., Lombardi, T., Giannotti, P.,, et al. (2020). Nanostructured Alumina as Seed Protectant against Three Stored-Product Insect Pests. J. Stored Prod. Res. 87, 101607. doi:10.1016/j.jspr.2020.101607
Bell, C. H. (2000). Fumigation in the 21st century. Crop Prot. 19 (8-10), 563–569. doi:10.1016/S0261-2194(00)00073-9
Benelli, G. (2018). Mode of Action of Nanoparticles against Insects. Environ. Sci. Pollut. Res. 25 (13), 12329–12341. doi:10.1007/s11356-018-1850-4
Bengston, M., Sidik, M., Halid, H., and Alip, E. (1996). “Efficacy of Phosphine Fumigations on Bagged Milled rice under Polyethylene Sheeting in Indonesia,” in Proceedings of the International Conference on Controlled Atmospheres and Fumigation in Stored Products (IEEE), 21–26.
Benhalima, H., Chaudhry, M. Q., Mills, K. A., and Price, N. R. (2004). Phosphine Resistance in Stored-Product Insects Collected from Various Grain Storage Facilities in Morocco. J. Stored Prod. Res. 40 (3), 241–249. doi:10.1016/S0022-474X(03)00012-2
Bhattacharyya, A., Bhaumik, A., Rani, P. U., Mandal, S., and Epidi, T. T. (2010). Nano-particles-A Recent Approach to Insect Pest Control. Afr. J. Biotechnol. 9 (24), 3489–3493.
Bowman, D., and Hodge, G. (2009). 12. Nanotechnology Products in Australia: Chemicals, Cosmetics and Regulatory Character. New Glob. Front. Regul. Age Nanotechnology 239, 1.
Buteler, M., Sofie, S. W., Weaver, D. K., Driscoll, D., Muretta, J., and Stadler, T. (2015). Development of Nanoalumina Dust as Insecticide against Sitophilus Oryzae and. Rhyzopertha dominica. Int. J. Pest Manag. 61 (1), 80–89. doi:10.1080/09670874.2014.1001008
Cáceres, M., Vassena, C. V., Garcerá, M. D., and Santo-Orihuela, P. L. (2019). Silica Nanoparticles for Insect Pest Control. Curr. Pharm. Des. 25 (37), 4030–4038.
Carbone, K., Santangelo, E., De Angelis, A., Micheli, L., Frosinini, R., Gargani, E., et al. (2020). “Green Synthesis of Silver Nanoparticles from Hyperpigmented Tomato Skins and Preliminary Evaluation of the Insecticidal Activity,” in 28th European Biomass Conference and Exhibition, 6–9 July 2020, Virtual.
Champ, B. R., and Dyte, C. E. (1977). FAO Global Survey of Pesticide Susceptibility of Stored Grain Pests. FAO Plant Prot. Bull. 25 (2), 49–67.
Chen, H., and Yada, R. (2011). Nanotechnologies in Agriculture: New Tools for Sustainable Development. Trends Food Sci. Tech. 22 (11), 585–594. doi:10.1016/j.tifs.2011.09.004
Chen, X., Chen, Y., Zou, L., Zhang, X., Dong, Y., Tang, J., et al. (2019). Plant-based Nanoparticles Prepared from Proteins and Phospholipids Consisting of a Core–Multilayer-Shell Structure: Fabrication, Stability, and Foamability. J. Agric. Food Chem. 67 (23), 6574–6584. doi:10.1021/acs.jafc.9b02028
Chen, X., and Schluesener, H. J. (2008). Nanosilver: a Nanoproduct in Medical Application. Toxicol. Lett. 176 (1), 1–12. doi:10.1016/j.toxlet.2007.10.004
Chhipa, H. (2017a). Nanofertilizers and Nanopesticides for Agriculture. Environ. Chem. Lett. 15 (1), 15–22. doi:10.1007/s10311-016-0600-4
Chhipa, H. (2017b). Nanopesticide: Current Status and Future Possibilities. Agric. Res. Technol. 5 (1), 1–4. doi:10.19080/ARTOAJ.2017.05.555651
Choupanian, M., Omar, D., Basri, M., and Asib, N. (2017). Preparation and Characterization of Neem Oil Nanoemulsion Formulations against Sitophilus oryzae and Tribolium castaneum Adults. J. Pestic. Sci., 42 (4), 158–165. doi:10.1584/jpestics
Chowdappa, P., and Gowda, S. (2013). Nanotechnology in Crop protection: Status and Scope. Pest Manag. Hortic. Ecosyst. 19 (2), 131–151.
Côa, F., Bortolozzo, L. S., Petry, R., Da Silva, G. H., Martins, C. H., de Medeiros, A. M., et al. (2020). Environmental Toxicity of Nanopesticides against Non-target Organisms: The State of the Art. Nanopesticides, 227–279.
Collins, P. J., Daglish, G. J., Bengston, M., Lambkin, T. M., and Pavic, H. (2002). Genetics of Resistance to Phosphine in Rhyzopertha dominica (Coleoptera: Bostrichidae). J. Econ. Entomol. 95 (4), 862–869. doi:10.1603/0022-0493-95.4.862
Collins, P. J., Daglish, G. J., Pavic, H., and Kopittke, R. A. (2005). Response of Mixed-Age Cultures of Phosphine-Resistant and Susceptible Strains of Lesser Grain Borer, Rhyzopertha dominica, to Phosphine at a Range of Concentrations and Exposure Periods. J. Stored Prod. Res. 41 (4), 373–385. doi:10.1016/j.jspr.2004.05.002
Collins, P. J., Falk, M. G., Nayak, M. K., Emery, R. N., and Holloway, J. C. (2017). Monitoring Resistance to Phosphine in the Lesser Grain Borer, Rhyzopertha dominica, in Australia: a National Analysis of Trends, Storage Types and Geography in Relation to Resistance Detections. J. Stored Prod. Res. 70, 25–36. doi:10.1016/j.jspr.2016.10.006
Collins, P. J., and Schlipalius, D. I. (2018). “Insecticide Resistance,” in Recent Advances in Stored Product protection (Berlin, Heidelberg: Springer), 169–182. doi:10.1007/978-3-662-56125-6_8
Daglish, G. J., Collins, P. J., Pavic, H., and Kopittke, R. A. (2002). Effects of Time and Concentration on Mortality of Phosphine‐resistant Sitophilus oryzae (L) Fumigated with Phosphine. Pest Manag. Sci. formerly Pestic. Sci. 58 (10), 1015–1021. doi:10.1002/ps.532
Das, S., Yadav, A., and Debnath, N. (2019). Entomotoxic Efficacy of Aluminium Oxide, Titanium Dioxide and Zinc Oxide Nanoparticles against Sitophilus oryzae (L.): A Comparative Analysis. J. Stored Prod. Res. 83, 92–96. doi:10.1016/j.jspr.2019.06.003
Debnath, N., Das, S., Seth, D., Chandra, R., Bhattacharya, S. C., and Goswami, A. (2011). Entomotoxic Effect of Silica Nanoparticles against Sitophilus oryzae (L.). J. Pest Sci. 84 (1), 99–105. doi:10.1007/s10340-010-0332-3
Deshwal, R., Vaibhav, V., Kumar, N., Kumar, A., and Singh, R. (2020). Stored Grain Insect Pests and Their Management: An Overview. J. Entomol. Zoolog. 8 (5), 969–974.
Dhivya, V., Nelson, S. J., Subramanian, K. S., Edward, Y. S. J. T., Rajamani, K., Santhanakrishnan, V. P., et al. (2019). Formulation of Sweet Flag Oil (Acorus calamus) Nanoemulsion by Spontaneous Emulsification Method for the Management of Sitophilus oryzae. IJCS 7 (3), 2072–2076.
Diagne, A., Diop, B. N., Ndiaye, P. M., Andreazza, C., and Sembene, M. (2019). Efficacy of Silica Nanoparticles on Groundnut Bruchid, Caryedon serratus (Olivier)(Coleoptera, Bruchidae). Afr. Crop Sci. J. 27 (2), 229–235. doi:10.4314/acsj.v27i2.8
Dikbaş, N., Uçar, S., Tozlu, G., Öznülüer Özer, T., and Kotan, R. (2021). Bacterial Chitinase Biochemical Properties, Immobilization on Zinc Oxide (ZnO) Nanoparticle and its Effect on Sitophilus zeamais as a Potential Insecticide. World J. Microbiol. Biotechnol. 37 (10), 1–14.
Ditta, A. (2012). How Helpful Is Nanotechnology in Agriculture? Adv. Nat. Sci. Nanoscience Nanotechnology 3 (3), 033002. doi:10.1088/2043-6262/3/3/033002
Dubey, A., and Mailapalli, D. R. (2016). “Nanofertilisers, Nanopesticides, Nanosensors of Pest and Nanotoxicity in Agriculture,” in Sustainable Agriculture Reviews (Cham: Springer), 307–330. doi:10.1007/978-3-319-26777-7_7
Dyte, C. E., and Halliday, D. (1985). Problems of Development of Resistance to Phosphine by Insect Pests of Stored Grains 1. EPPO Bull. 15 (1), 51–57. doi:10.1111/j.1365-2338.1985.tb00200.x
Edwards, C. H., Christie, C. R., Masotti, A., Celluzzi, A., Caporali, A., and Campbell, E. M. (2020). Dendrimer-coated Carbon Nanotubes Deliver dsRNA and Increase the Efficacy of Gene Knockdown in the Red Flour Beetle Tribolium castaneum. Scientific Rep. 10 (1), 1–11. doi:10.1038/s41598-020-69068-x
El-Bendary, H. M., Abdel-Wahab, A. S., and El-Helaly, A. A. (2016). Entomo-toxic Assay of Nano-Aluminum Oxide and Nano-Zinc Oxide against Sitophilus oryzae as a Promising Insecticide. Egypt. J. Zoolog. 174 (4083), 1–8. doi:10.12816/0034708
El-Naggar, M. E., Abdelsalam, N. R., Fouda, M. M., Mackled, M. I., Al-Jaddadi, M. A., Ali, H. M., et al. (2020). Soil Application of Nano Silica on maize Yield and its Insecticidal Activity against Some Stored Insects after the post-harvest. Nanomaterials 10 (4), 739. doi:10.3390/nano10040739
El-Saadony, M. T., El-Hack, A., Mohamed, E., Taha, A. E., Fouda, M. M., Ajarem, J. S., et al. (2020). Ecofriendly Synthesis and Insecticidal Application of Copper Nanoparticles against the Storage Pest Tribolium castaneum. Nanomaterials 10 (3), 587. doi:10.3390/nano10030587
Elizabath, A., Babychan, M., Mathew, A. M., and Syriac, G. M. (2019). Application of Nanotechnology in Agriculture. Int. J. Pure Appl. Biosci. 7(2), 131–139. doi:10.18782/2320-7051.6493
Emamjomeh, L., Imani, S., Talebi Jahromi, K., and Moharramipour, S. (2021). Nanoencapsulation Enhances the Contact Toxicity of Eucalyptus globulus Labill and Zataria multiflora Boiss Essential Oils against the Third Instar Larvae of Ephestia kuehniella (Lepidoptera: Pyralidae). Int. J. Pest Manag., 1–9. doi:10.1080/09670874.2020.1871529
European Food Safety Authority (Efsa), , Anastassiadou, M., Brancato, A., Brocca, D., Carrasco Cabrera, L., Ferreira, L., et al. (2019). Reporting Data on Pesticide Residues in Food and Feed According to Regulation (EC) No 396/2005 (2018 Data Collection). EFSA J. 17 (4), e05655. doi:10.2903/j.efsa.2019.5655
Fones, H. N., Bebber, D. P., Chaloner, T. M., Kay, W. T., Steinberg, G., and Gurr, S. J. (2020). Threats to Global Food Security from Emerging Fungal and Oomycete Crop Pathogens. Nat. Food 1 (6), 332–342. doi:10.1038/s43016-020-0075-0
Frederiksen, H. K., Kristensen, H. G., and Pedersen, M. (2003). Solid Lipid Microparticle Formulations of the Pyrethroid Gamma-Cyhalothrin—Incompatibility of the Lipid and the Pyrethroid and Biological Properties of the Formulations. J. controlled release 86 (2-3), 243–252. doi:10.1016/S0168-3659(02)00406-6
Gahukar, R. T., and Das, R. K. (2020). Plant-derived Nanopesticides for Agricultural Pest Control: Challenges and Prospects. Nanotechnology Environ. Eng. 5 (1), 1–9. doi:10.1007/s41204-020-0066-2
Garg, D., and Payasi, D. K. (2020). Nanomaterials in Agricultural Research: an Overview. Environ. Nanotechnology 3, 243–275. doi:10.1007/978-3-030-26672-1_8
Giunti, G., Campolo, O., Laudani, F., Zappalà, L., and Palmeri, V. (2021). Bioactivity of Essential Oil-Based Nano-Biopesticides toward Rhyzopertha dominica (Coleoptera: Bostrichidae). Ind. Crops Prod. 162, 113257. doi:10.1016/j.indcrop.2021.113257
Giunti, G., Palermo, D., Laudani, F., Algeri, G. M., Campolo, O., and Palmeri, V. (2019). Repellence and Acute Toxicity of a Nano-Emulsion of Sweet orange Essential Oil toward Two Major Stored Grain Insect Pests. Ind. Crops Prod. 142, 111869. doi:10.1016/j.indcrop.2019.111869
Goswami, A., Roy, I., Sengupta, S., and Debnath, N. (2010). Novel Applications of Solid and Liquid Formulations of Nanoparticles against Insect Pests and Pathogens. Thin solid films 519 (3), 1252–1257. doi:10.1016/j.tsf.2010.08.079
Gour, N., Upadhyaya, P., and Patel, J. (2019). “Nanomaterials as Therapeutic and Diagnostic Tool for Controlling Plant Diseases,” in Comprehensive Analytical Chemistry (Elsevier), 225–261. doi:10.1016/bs.coac.2019.04.003
Grillo, R., Fraceto, L. F., Amorim, M. J., Scott-Fordsmand, J. J., Schoonjans, R., and Chaudhry, Q. (2021). Ecotoxicological and Regulatory Aspects of Environmental Sustainability of Nanopesticides. J. Hazard. Matter. 404, 124148. doi:10.1016/j.jhazmat.2020.124148
Gu, C. (2020). Evaluation of Ethyl Formate for Management of Both Resistant and Susceptible Strains of Cryptolestes Pusillus and Cryptolestes ferrugineus. Doctoral dissertation, Murdoch University. Perth, Australia.
Gulzar, M. U., Zia, T., Shahzad, M., Ibrahim, M. U., and Ihsan, T. (2020). Impacts of Bio Synthesized Silver-Nanoparticles (AgNO3) and Plant Oils against. Trogoderma granariumGSC Biol. Pharm. Sci. 10 (3), 010–015. doi:10.30574/gscbps.2020.10.3.0044
Gupta, N., Upadhyaya, C. P., Singh, A., Abd-Elsalam, K. A., and Prasad, R. (2018). “Applications of Silver Nanoparticles in Plant protection,” in Nanobiotechnology Applications in Plant Protection (Cham: Springer), 247–265. doi:10.1007/978-3-319-91161-8_9
Haider, Z., Ayub, A. R., Tahir, M. U., Sajid, S. A., and Khalid, M. (2020). Efficacy and Characterization of Synthesized Zinc Oxide Nanoparticles against Tribolium Castaneum and Trogoderma Granarium. Int. J. Scientific Res. 10, 9912. doi:10.29322/IJSRP.10.03.2020.p9912
Hamel, D., Rozman, V., and Liška, A. (2020). Storage of Cereals in Warehouses with or without Pesticides. Insects 11 (12), 846. doi:10.3390/insects11120846
Hamza, A. M. (2012). Efficacy and Safety of Non-traditional Methods as Alternatives for Control of Sitophilus Oryzae (L.)(Coleoptera: Curculionidae) in rice Grains. Egypt. J. Biol. Pest Control. 22 (2), 103.
Haroun, S. A., Elnaggar, M. E., Zein, D. M., and Gad, R. I. (2020). Insecticidal Efficiency and Safety of Zinc Oxide and Hydrophilic Silica Nanoparticles against Some Stored Seed Insects. J. Plant Prot. Res. 60, 77–85.
Hasan, M. M., and Reichmuth, C. (2004). Relative Toxicity of Phosphine against the Bean Bruchid Acanthoscelides obtectus (Say)(Col., Bruchidae). J. Appl. Entomol. 128 (5), 332–336. doi:10.1111/j.1439-0418.2004.00850.x
Hashem, A. S., Awadalla, S. S., Zayed, G. M., Maggi, F., and Benelli, G. (2018). Pimpinella Anisum Essential Oil Nanoemulsions against Tribolium castaneum Insecticidal Activity and Mode of Action. Environ. Sci. Pollut. Res. 25 (19), 18802–18812. doi:10.1007/s11356-018-2068-1
Hashem, A. S., and Ramadan, M. M. (2021). Nanoemulsions of Chamomile and Cumin Essential Oils: As an Alternative Bio-Rational Control Approach against the Red Flour Beetle, Tribolium castaneum. J. Plant Prot. Pathol. 12 (1), 11–17. doi:10.21608/jppp.2021.149515
Hayles, J., Johnson, L., Worthley, C., and Losic, D. (2017). “Nanopesticides: a Review of Current Research and Perspectives,” in New Pesticides and Soil Sensors. Editor A. M. Grumezescu (Academic Press), 193–225. doi:10.1016/B978-0-12-804299-1.00006-0
Heydarzade, A., Valizadegan, O., Negahban, M., and Mehrkhou, F. (2019). Efficacy of Mentha spicata and Mentha pulegium Essential Oil Nanoformulation on Mortality and Physiology of Tribolium castaneum (Col.: Tenebrionidae). J. Crop Prot. 8 (4), 501–520.
Hilal, S. M., Mohmed, A. S., Barry, N. M., and Ibrahim, M. H. (2021). “Entomotoxicity of TiO2 and ZnO Nanoparticles against Adults Tribolium castaneum (Herbest)(Coleoptera: Tenebrionidae),” in IOP Conference Series: Earth and Environmental Science (IOP Publishing Bristol, UK), 012088. doi:10.1088/1755-1315/910/1/012088
Hischier, R., and Walser, T. (2012). Life Cycle Assessment of Engineered Nanomaterials: State of the Art and Strategies to Overcome Existing Gaps. Sci. total Environ. 425, 271–282. doi:10.1016/j.scitotenv.2012.03.001
Holloway, J. C., Falk, M. G., Emery, R. N., Collins, P. J., and Nayak, M. K. (2016). Resistance to Phosphine in Sitophilus oryzae in Australia: A National Analysis of Trends and Frequencies over Time and Geographical Spread. J. Stored Prod. Res. 69, 129–137. doi:10.1016/j.jspr.2016.07.004
Hossain, F., Follett, P., Salmieri, S., Vu, K. D., Harich, M., and Lacroix, M. (2019). Synergistic Effects of Nanocomposite Films Containing Essential Oil Nanoemulsions in Combination with Ionizing Radiation for Control of rice Weevil Sitophilus oryzae in Stored Grains. J. Food Sci. 84 (6), 1439–1446. doi:10.1111/1750-3841.14603
Huang, L., Yang, S., Chen, J., Tian, J., Huang, Q., Huang, H., et al. (2019). A Facile Surface Modification Strategy for Fabrication of Fluorescent Silica Nanoparticles with the Aggregation-Induced Emission Dye through Surface-Initiated Cationic Ring Opening Polymerization. Mater. Sci. Eng. C 94, 270–278. doi:10.1016/j.msec.2018.09.042
Ibrahim, S. S., and Salem, N. Y. (2019). Insecticidal Efficacy of Nano Zeolite against Tribolium confusum (Col., Tenebrionidae) and Callosobruchus maculatus (Col., Bruchidae). Bull. Natl. Res. Centre 43 (1), 1–8. doi:10.1186/s42269-019-0128-4
Ikawati, S., Himawan, T., Abadi, A. L., and Tarno, H. (2021). Toxicity Nanoinsecticide Based on Clove Essential Oil against Tribolium castaneum (Herbst). J. Pestic. Sci. 46 (2), 222–228. doi:10.1584/jpestics.d20-059
Islam, M. T. (2020). Application of Nanomaterials in Plant Protection. Doctoral dissertation, Bangabandhu Sheikh Mujibur Rahman Agricultural University Gazipur City, Bangladesh.
Ismail, T., Salama, M. A., and El-Ebiary, M. (2021). Entomotoxic Effects of Synthesized Aluminum Oxide Nanoparticles against Sitophilus oryzae and Their Toxicological Effects on Albino Rats. Toxicol. Ind. Health 37 (10), 594–602. doi:10.1177/07482337211035000
Jafer, F. S., and Annon, M. R. (2018). Larvicidal Effect of Pure and green-synthesized Silver Nanoparticles against Tribolium castaneum (herb.) and Callosobruchus maculatus (fab.). J. Glob. Pharma Technol 10 (3), 448–454.
Jallow, M. F., Awadh, D. G., Albaho, M. S., Devi, V. Y., and Thomas, B. M. (2017). Pesticide Risk Behaviors and Factors Influencing Pesticide Use Among Farmers in Kuwait. Sci. Total Environ. 574, 490–498. doi:10.1016/j.scitotenv.2016.09.085
Jasrotia, P., Kashyap, P. L., Bhardwaj, A. K., Kumar, S., and Singh, G. P. (2018). Scope and Applications of Nanotechnology for Wheat Production: a Review of Recent Advances. Wheat Barley Res. 10 (1), 1–14. doi:10.25174/2249-4065/2018/76672
Jesser, E., Yeguerman, C., Stefanazzi, N., Gomez, R., Murray, A. P., Ferrero, A. A., et al. (2020). Ecofriendly Approach for the Control of a Common Insect Pest in the Food Industry, Combining Polymeric Nanoparticles and Post-application Temperatures. J. Agric. Food Chem. 68 (21), 5951–5958. doi:10.1021/acs.jafc.9b06604
Jo, Y. K., Kim, B. H., and Jung, G. (2009). Antifungal activity of silver ions and nanoparticles on phytopathogenic fungi. Plant disease, 93 (10), 1037–1043.
Kah, M., Beulke, S., Tiede, K., and Hofmann, T. (2013). Nanopesticides: State of Knowledge, Environmental Fate, and Exposure Modeling. Crit. Rev. Environ. Sci. Tech. 68, 16. doi:10.1080/10643389.2012.671750
Kah, M., and Hofmann, T. (2014). Nanopesticide Research: Current Trends and Future Priorities. Environ. Int. 63, 224–235. doi:10.1016/j.envint.2013.11.015
Kalia, A., Sharma, S. P., Kaur, H., and Kaur, H. (2020). “Novel Nanocomposite-Based Controlled-Release Fertilizer and Pesticide Formulations: Prospects and Challenges,” in Multifunctional Hybrid Nanomaterials for Sustainable Agri-Food and Ecosystems (Elsevier), 99–134. doi:10.1016/b978-0-12-821354-4.00005-4
Kallur, R., and Patil, S. (2019). To Evaluate the Effect of Pesticide Activity of of Nanoformulations of Alstonia scholaris, against Pest-Rhyzopertha dominica Using HPLC and Fourier Transform Infrared (FT-IR) Spectra Analysis. Int. J. 2 (3), 294.
Kar, S., Nayak, R. N., Sahoo, N. R., Bakhara, C. K., Panda, M. K., Pal, U. S., et al. (2021). Rice Weevil Management through Application of Silica Nano Particle and Physico-Chemical and Cooking Characterization of the Treated rice. J. Stored Prod. Res. 94, 101892. doi:10.1016/j.jspr.2021.101892
Karn, B., Kuiken, T., and Otto, M. (2009). Nanotechnology and In Situ Remediation: a Review of the Benefits and Potential Risks. Environ. Health Perspect. 117 (12), 1813–1831. doi:10.1289/ehp.0900793
Kashyap, P. L., Kumar, S., and Srivastava, A. K. (2017). Nanodiagnostics for Plant Pathogens. Environ. Chem. Lett. 15, 7–13. doi:10.1007/s10311-016-0580-4
Kashyap, P. L., Rai, P., Kumar, R., Sharma, S., Jasrotia, P., Srivastava, A. K., et al. (2018). “Microbial Nanotechnology for Climate Resilient Agriculture,” in Microbes for Climate Resilient Agriculture (Hoboken: Wiley), 279–344. doi:10.1002/9781119276050.ch13
Kashyap, P. L., Rai, P., Sharma, S., Chakdar, H., Kumar, S., Pandiyan, K., et al. (2016). “Nanotechnology for the Detection and Diagnosis of Plant Pathogens,” in Nanoscience in Food and Agriculture 2, Sustainable Agriculture Reviews 21 (Basel: Springer), 253–276. doi:10.1007/978-3-319-39306-3_8
Kashyap, P. L., Kumar, S., Jasrotia, P., Singh, D. P., and Singh, G. P. (2020). “Nanotechnology in Wheat Production and Protection,” in Environmental Nanotechnology Volume 4. Editors N. Dasgupta, S. Ranjan, and E. Lichtfouse (Cham: Springer), 165–194. doi:10.1007/978-3-030-26668-4_5
Kashyap, P. L., Xiang, X., and Heiden, P. (2015). Chitosan Nanoparticle Based Delivery Systems for Sustainable Agriculture. Int. J. Biol. macromolecules 77, 36–51. doi:10.1016/j.ijbiomac.2015.02.039
Kavallieratos, N. G., Nika, E. P., Skourti, A., Ntalli, N., Boukouvala, M. C., Ntalaka, C. T., et al. (2021a). Developing a Hazomalania voyronii Essential Oil Nanoemulsion for the Eco-Friendly Management of Tribolium confusum, Tribolium castaneum and Tenebrio molitor Larvae and Adults on Stored Wheat. Molecules 26 (6), 1812. doi:10.3390/molecules26061812
Kavallieratos, N. G., Skourti, A., Nika, E. P., Ntalaka, C. T., Boukouvala, M. C., Bonacucina, G., et al. (2021b). Isofuranodiene-based Nanoemulsion: Larvicidal and Adulticidal Activity against Tenebrionid Beetles Attacking Stored Wheat. J. Stored Prod. Res. 93, 101859. doi:10.1016/j.jspr.2021.101859
Khalil, M. S. (2019). Efficacy of Some Nanoparticles against the Adults of Red Flour Beetle Tribolium castaneum (Herbst) under Laboratory Conditions. Ann. Agric. Sci. Moshtohor 57 (2), 535–540. doi:10.21608/assjm.2019.44940
Khanahmadi, M., Pakravan, P., Hemati, A., Azandaryani, M. N., and Ghamari, E. (2017). Fumigant Toxicity of Artemisia haussknechtii Essential Oil and its Nano-Encapsulated Form. Pharma 2, 1776–1783.
Khoobdel, M., Ahsaei, S. M., and Farzaneh, M. (2017). Insecticidal Activity of Polycaprolactone Nanocapsules Loaded with Rosmarinus officinalis Essential Oil in Tribolium castaneum (Herbst). Entomol. Res. 47 (3), 175–184. doi:10.1111/1748-5967.12212
Kookana, R. S., Boxall, A. B., Reeves, P. T., Ashauer, R., Beulke, S., Chaudhry, Q., et al. (2014). Nanopesticides: Guiding Principles for Regulatory Evaluation of Environmental Risks. J. Agric. Food Chem. 62 (19), 4227–4240. doi:10.1021/jf500232f
Kumar, D., and Kalita, P. (2017). Reducing Postharvest Losses during Storage of Grain Crops to Strengthen Food Security in Developing Countries. Foods 6 (1), 8. doi:10.3390/foods6010008
Kumar, S., Bhanjana, G., Sharma, A., Dilbaghi, N., Sidhu, M. C., and Kim, K. H. (2017). Development of Nanoformulation Approaches for the Control of Weeds. Sci. Total Environ. 586, 1272–1278. doi:10.1016/j.scitotenv.2017.02.138
Kumar, S., Nehra, M., Dilbaghi, N., Marrazza, G., Hassan, A. A., and Kim, K. H. (2019). Nano-based Smart Pesticide Formulations: Emerging Opportunities for Agriculture. J. Controlled Release 294, 131–153. doi:10.1016/j.jconrel.2018.12.012
Kumar, S., Nehra, M., Kedia, D., Dilbaghi, N., Tankeshwar, K., and Kim, K. H. (2018). Carbon Nanotubes: A Potential Material for Energy Conversion and Storage. Prog. Energ. Combustion Sci. 64, 219–253. doi:10.1016/j.pecs.2017.10.005
Lakshmi, S. J., Roopa Bai, R. S., Sharanagouda, H., Ramachandra, C. T., and Nadagouda, S. (2020). Effect of Zinc Oxide Nanoparticles on Pulse Beetle (Callosobruchus maculatus)(Col.: Chrysomelidae) in Greengram. J. Entomol. Zoolog. Stud. 8, 297–300.
Li, X., Ke, M., Zhang, M., Peijnenburg, W. J. G. M., Fan, X., Xu, J., et al. (2018). The Interactive Effects of Diclofop-Methyl and Silver Nanoparticles on Arabidopsis thaliana: Growth, Photosynthesis and Antioxidant System. Environ. Pollut. 232, 212–219. doi:10.1016/j.envpol.2017.09.034
Liu, K., Bandara, M., Hamel, C., Knight, J. D., and Gan, Y. (2020). Intensifying Crop Rotations with Pulse Crops Enhances System Productivity and Soil Organic Carbon in Semi-arid Environments. Field Crops Res. 248, 107657. doi:10.1016/j.fcr.2019.107657
Loha, K. M., Shakil, N. A., Kumar, J., Singh, M. K., and Srivastava, C. (2012). Bio-efficacy Evaluation of Nanoformulations of β-cyfluthrin against Callosobruchus maculatus (Coleoptera: Bruchidae). J. Environ. Sci. Health B 47 (7), 687–691. doi:10.1080/03601234.2012.669254
López-García, G. P., Buteler, M., and Stadler, T. (2018). Testing the Insecticidal Activity of Nanostructured Alumina on Sitophilus oryzae (L.)(Coleoptera: Curculionidae) under Laboratory Conditions Using Galvanized Steel Containers. Insects 9 (3), 87.
Louni, M., Shakarami, J., and Negahban, M. (2018). Insecticidal Efficacy of Nanoemulsion Containing Mentha longifolia Essential Oil against Ephestia Kuehniella (Lepidoptera: Pyralidae). J. Crop Prot. 7 (2), 171–182.
Louni, M., Shakarami, J., and Negahban, M. (2019). Study on Insecticidal Properties of Nanoemulsion Mentha Longifolia L.(Lamiaceae) Essential Oil against Callosobruchus maculatus (Fabricius)(Coleoptera: Chrysomelidae). J. Entomol. Soc. Iran 39 (2), 151–163.
Luiz de Oliveira, J., Ramos Campos, E. V., and Fraceto, L. F. (2018). Recent Developments and Challenges for Nanoscale Formulation of Botanical Pesticides for Use in Sustainable Agriculture. J. Agric. Food Chem. 66 (34), 8898–8913. doi:10.1021/acs.jafc.8b03183
Luo, Y., Huang, D., Li, D., and Wu, L. (2020). On Farm Storage, Storage Losses and the Effects of Loss Reduction in China. Resour. Conservation Recycling 162, 105062. doi:10.1016/j.resconrec.2020.105062
Madhuri, S., Choudhary, A. K., and Rohit, K. (2010). Nanotechnology in Agricultural Diseases and Food Safety. J. Phytology 2 (4), 78–82.
Malaikozhundan, B., Vaseeharan, B., Vijayakumar, S., and Thangaraj, M. P. (2017). Bacillus thuringiensis Coated Zinc Oxide Nanoparticle and its Biopesticidal Effects on the Pulse Beetle, Callosobruchus maculatus. J. Photochem. Photobiol. B: Biol. 174, 306–314. doi:10.1016/j.jphotobiol.2017.08.014
Malathi, S., Rameshkumar, G., Rengarajan, R. L., Rajagopal, T., Muniasamy, S., and Ponmanickam, P. (2019). Phytofabrication of Silver Nanoparticles Using Annona reticulata and Assessment of Insecticidal and Bactericidal Activities. J. Environ. Biol. 40 (4), 626–633. doi:10.22438/jeb/40/4/mrn-934
Margulis-Goshen, K., and Magdassi, S. (2013). “Nanotechnology: an Advanced Approach to the Development of Potent Insecticides,” in Advanced Technologies for Managing Insect Pests. Editors I. Ishaaya, S. Palli, and A. Horowitz (Dordrecht: Springer), 295–314. doi:10.1007/978-94-007-4497-4_15
Martín, Á., Varona, S., Navarrete, A., and Cocero, M. J. (2010). Encapsulation and Co-precipitation Processes with Supercritical Fluids: Applications with Essential Oils. Open Chem. Eng. J. 4 (1). doi:10.2174/1874123101004010031
Mesterházy, Á., Oláh, J., and Popp, J. (2020). Losses in the Grain Supply Chain: Causes and Solutions. Sustainability 12 (6), 2342.
Mills, K. A. (2001). “October). Phosphine Resistance: where to Now,” in Proceedings, International Conference on Controlled Atmosphere and Fumigation in Stored Products (IEEE), 583–591.
Mohammed, A. M., and Aswd, S. A. (2019). Effect of Some Nanoparticles on the Stages Biology of the Southern Cowpea Beetle Callosobruchus maculatus (Fab.)(Coleoptera: Bruchidae). J. Edu. Sci. 28 (3), 188–199. doi:10.33899/edusj.2019.162956
Mohammed, T. G., and Nasr, M. E. H. (2020). Preparation, Characterization and Biological Efficacy of Eucalyptus Oil Nanoemulsion against the Stored Grain Insects. Asian J. Adv. Agric. Res, 13 (2), 41–51. doi:10.9734/ajaar/2020/v13i230102
Mondal, S. (2020). Potential of Nanotechnology for Rural Applications. Arabian J. Sci. Eng. 45 (7), 5011–5042. doi:10.1007/s13369-019-04332-5
Mosa, W. F., El-Shehawi, A. M., Mackled, M. I., Salem, M. Z., Ghareeb, R. Y., Hafez, E. E., et al. (2021). Productivity Performance of Peach Trees, Insecticidal and Antibacterial Bioactivities of Leaf Extracts as Affected by Nanofertilizers Foliar Application. Scientific Rep. 11 (1), 1–19. doi:10.1038/s41598-021-89885-y
Mujtaba, M., Khawar, K. M., Camara, M. C., Carvalho, L. B., Fraceto, L. F., Morsi, R. E., and Wang, D. (2020). Chitosan-based Delivery Systems for Plants: A Brief Overview of Recent Advances and Future Directions. Int. J. Biol. macromolecules 154, 683–697. doi:10.1016/j.ijbiomac.2020.03.128
Mustafa, I. F., and Hussein, M. Z. (2020). Synthesis and Technology of Nanoemulsion-Based Pesticide Formulation. Nanomaterials 10 (8), 1608. doi:10.3390/nano10081608
Nair, P. M. G., and Choi, J. (2011). Identification, Characterization and Expression Profiles of Chironomus Riparius Glutathione S-Transferase (GST) Genes in Response to Cadmium and Silver Nanoparticles Exposure. Aquat. Toxicol. 101 (3), 550–560. doi:10.1016/j.aquatox.2010.12.006
Naqvi, S., and Flora, S. J. S. (2020). Nanomaterial's Toxicity and its Regulation Strategies. J. Environ. Biol. 41 (4), 659–671. doi:10.22438/jeb/41/4/mrn-1394
Nasr, M. (2015). Impact of Nanoparticle Zinc Oxide and Aluminum Oxide against Rice Weevil Sitophilus oryzae (Coleoptera: Curculionidae) under Laboratory Conditions. Egy. J. Plant Pro. Res. 3 (3), 30–38.
Nayak, M. K., Daglish, G. J., Phillips, T. W., and Ebert, P. R. (2020). Resistance to the Fumigant Phosphine and its Management in Insect Pests of Stored Products: a Global Perspective. Annu. Rev. Entomol. 65, 333–350. doi:10.1146/annurev-ento-011019-025047
Negahban, M., Moharramipour, S., Zandi, M., Hashemi, S. A., and Ziayee, F. (2012). “October)Nano-insecticidal Activity of Essential Oil from Cuminum cyminum on Tribolium castaneum,” in Proc 9th. Int. Conf. On Controlled Atmosphere and Fumigation in Stored Products (Antalya, Turkey: IEEE), 15–19.
Nenaah, G. E. (2014). Chemical Composition, Toxicity and Growth Inhibitory Activities of Essential Oils of Three Achillea Species and Their Nano-Emulsions against Tribolium castaneum (Herbst). Ind. Crops Prod. 53, 252–260. doi:10.1016/j.indcrop.2013.12.042
Neri-Badang, M. C., and Chakraborty, S. (2019). Carbohydrate Polymers as Controlled Release Devices for Pesticides. J. Carbohydr. Chem. 38 (1), 67–85. doi:10.1080/07328303.2019.1568449
Newman, C. R. (2010). A Novel Approach to Limit the Development of Phosphine Resistance in Western Australia. Julius-Kühn-Archiv 425, 1038. doi:10.5073/jka.2010.425.072
Nguyen, H. M., Hwang, I. C., Park, J. W., and Park, H. J. (2012). Photo protection for Deltamethrin Using Chitosan‐coated Beeswax Solid Lipid Nanoparticles. Pest Manag. Sci. 68 (7), 1062–1068. doi:10.1002/ps.3268
Nguyen, T. T., Collins, P. J., and Ebert, P. R. (2015). Inheritance and Characterization of strong Resistance to Phosphine in Sitophilus oryzae (L.). PloS one 10 (4), e0124335. doi:10.1371/journal.pone.0124335
Norman, D. J., and Chen, J. (2011). Effect of Foliar Application of Titanium Dioxide on Bacterial Blight of geranium and Xanthomonas Leaf Spot of Poinsettia. HortScience 46 (3), 426–428. doi:10.21273/hortsci.46.3.426
Nuruzzaman, M. D., Rahman, M. M., Liu, Y., and Naidu, R. (2016). Nanoencapsulation, Nano-Guard for Pesticides: a New Window for Safe Application. J. Agric. Food Chem. 64 (7), 1447–1483. doi:10.1021/acs.jafc.5b05214
Oguntade, A. S. (2021). Assessment of Active Compounds Present in Preservatives Used for Grain Storage in Selected Markets in Ilorin, Kwara State (Doctoral dissertation, Kwara State University (Nigeria).
Oliveira, C. R., Domingues, C. E., de Melo, N. F., Roat, T. C., Malaspina, O., Jones-Costa, M., et al. (2019). Nanopesticide Based on Botanical Insecticide Pyrethrum and its Potential Effects on Honeybees. Chemosphere 236, 124282. doi:10.1016/j.chemosphere.2019.07.013
Oso, A. A., and Ashafa, A. O. (2021). Nutritional Composition of Grain and Seed Proteins. IntechOpen London, UK. doi:10.5772/intechopen.97878
Palermo, D., Giunti, G., Laudani, F., Palmeri, V., and Campolo, O. (2021). Essential Oil-Based Nano-Biopesticides: Formulation and Bioactivity against the Confused Flour Beetle Tribolium confusum. Sustainability 13 (17), 9746. doi:10.3390/su13179746
Pankaj, , Shakil, N. A., Kumar, J., Singh, M. K., and Singh, K. (2012). Bioefficacy Evaluation of Controlled Release Formulations Based on Amphiphilic Nano-Polymer of Carbofuran against Meloidogyne incognita Infecting Tomato. J. Environ. Sci. Health Part B 47 (6), 520–528. doi:10.1080/03601234.2012.665667
Panpatte, D. G., Jhala, Y. K., Shelat, H. N., and Vyas, R. V. (2016). “Nanoparticles: the Next Generation Technology for Sustainable Agriculture,” in Microbial Inoculants in Sustainable Agricultural Productivity (New Delhi: Springer), 289–300. doi:10.1007/978-81-322-2644-4_18
Pant, M., Dubey, S., Patanjali, P. K., Naik, S. N., and Sharma, S. (2014). Insecticidal Activity of eucalyptus Oil Nanoemulsion with Karanja and Jatropha Aqueous Filtrates. Int. biodeterioration biodegradation 91, 119–127. doi:10.1016/j.ibiod.2013.11.019
Pathipati, U. R., and Kanuparthi, P. L. (2021). Silver nanoparticles for insect control: Bioassays and mechanisms. In Silver Nanomaterials for Agri-Food Applications, (pp. 471–494). Elsevier.
Patil, C. D., Borase, H. P., Suryawanshi, R. K., and Patil, S. V. (2016). Trypsin Inactivation by Latex Fabricated Gold Nanoparticles: A New Strategy towards Insect Control. Enzyme Microb. Technol. 92, 18–25. doi:10.1016/j.enzmictec.2016.06.005
Patil, N., Lin, A., Myers, E. R., Ryu, K., Badmaev, A., Zhou, C., et al. (2009). Wafer-scale Growth and Transfer of Aligned Single-Walled Carbon Nanotubes. IEEE Trans. Nanotechnology 8 (4), 498–504. doi:10.1109/tnano.2009.2016562
Pavoni, L., Pavela, R., Cespi, M., Bonacucina, G., Maggi, F., Zeni, V., et al. (2019). Green Micro-and Nanoemulsions for Managing Parasites, Vectors and Pests. Nanomaterials 9 (9), 1285. doi:10.3390/nano9091285
Pimentel, M. A. G., Faroni, L. R. D. A., Tótola, M. R., and Guedes, R. N. C. (2007). Phosphine Resistance, Respiration Rate and Fitness Consequences in Stored‐product Insects. Pest Manag. Sci. formerly Pestic. Sci. 63 (9), 876–881. doi:10.1002/ps.1416
Pirzadah, T. B., Malik, B., Maqbool, T., and Rehman, R. U. (2019). “Development of Nanobioformulations of Nutrients for Sustainable Agriculture,” in Nanobiotechnology inBioformulations (Cham: Springer), 381–394. doi:10.1007/978-3-030-17061-5_16
Poudel, S., Poudel, B., Acharya, B., and Poudel, P. (2020). Pesticide Use and its Impacts on Human Health and Environment. Environ. Ecosyst. Sci. 4, 47–51. doi:10.26480/ees.01.2020.47.51
Qazi, G., and Dar, F. A. (2020). Nano-agrochemicals: Economic Potential and Future Trends. Nanobiotechnology in Agriculture, pp.185–193. doi:10.1007/978-3-030-39978-8_11
Raduw, G. G., and Mohammed, A. A. (2020). Insecticidal Efficacy of Three Nanoparticles for the Control of Khapra Beetle (Trogoderma Granarium) on Different Grains. J. Agric. Urban Entomol. 36 (1), 90–100. doi:10.3954/1523-5475-36.1.90
Ragaei, M., and Sabry, A. K. H. (2014). Nanotechnology for Insect Pest Control. Int. J. Sci. Environ. Technol. 3 (2), 528–545.
Rahman, I. A., and Padavettan, V. (2012). Synthesis of Silica Nanoparticles by Sol-Gel: Size-dependent Properties, Surface Modification, and Applications in Silica-Polymer Nanocomposites—A Review. J. Nanomater. 2012, 132424. doi:10.1155/2012/132424
Rai, M., Kon, K., Ingle, A., Duran, N., Galdiero, S., and Galdiero, M. (2014). Broad-spectrum Bioactivities of Silver Nanoparticles: the Emerging Trends and Future Prospects. Appl. Microbiol. Biotechnol. 98 (5), 1951–1961. doi:10.1007/s00253-013-5473-x
Rajendran, S. (20022002). “Postharvest Pest Losses,” in Encyclopedia of Pest Management. Editor D. Pimentel (New York: Marcel Dekker), 654–656. doi:10.1201/noe0824706326.ch302
Rajendran, S., and Sriranjini, V. (2008). Plant Products as Fumigants for Stored-Product Insect Control. J. stored Prod. Res. 44 (2), 126–135. doi:10.1016/j.jspr.2007.08.003
Rajkumar, V., Gunasekaran, C., Paul, C. A., and Dharmaraj, J. (2020). Development of Encapsulated Peppermint Essential Oil in Chitosan Nanoparticles: Characterization and Biological Efficacy against Stored-Grain Pest Control. Pestic. Biochem. Physiol. 170, 104679. doi:10.1016/j.pestbp.2020.104679
Ramasamy, T., Ruttala, H. B., Gupta, B., Poudel, B. K., Choi, H. G., Yong, C. S., et al. (2017). Smart Chemistry-Based Nanosized Drug Delivery Systems for Systemic Applications: a Comprehensive Review. J. Controlled Release 258, 226–253. doi:10.1016/j.jconrel.2017.04.043
Rani, R., Dahiya, S., Dhingra, D., Dilbaghi, N., Kim, K. H., and Kumar, S. (2017). Evaluation of Anti-diabetic Activity of Glycyrrhizin-Loaded Nanoparticles in Nicotinamide-Streptozotocin-Induced Diabetic Rats. Eur. J. Pharm. Sci. 106, 220–230. doi:10.1016/j.ejps.2017.05.068
Rani, S. S., Justin, C. G. L., Gunasekaran, K., and Joyce, S. S. (2019). Efficacy of green Synthesized Silver Nanoparticle, Plant Powders and Oil against rice Weevil Sitophilus oryzae L.(Coleoptera: Curculionidae) on Sorghum Seeds. J. Pharmacognosy Phytochemistry 8 (5), 38–42.
Raoufi, D. (2013). Synthesis and Microstructural Properties of ZnO Nanoparticles Prepared by Precipitation Method. Renew. Energ. 50, 932–937. doi:10.1016/j.renene.2012.08.076
Rashwan, R. S., and Abu-Zaid, A. A. (2018). Evaluation of Nutritive Values and Microbial Content of Wheat Grains Treated with Some Types Nano Particles to Control Sitophilus granaries (L.). Arab Universities J. Agric. Sci. 26 (1), 293–301. doi:10.21608/ajs.2018.14006
Rastogi, A., Tripathi, D. K., Yadav, S., Chauhan, D. K., Živčák, M., Ghorbanpour, M., et al. (2019). Application of Silicon Nanoparticles in Agriculture. 3 Biotech. 9 (3), 1–11. doi:10.1007/s13205-019-1626-7
Rikta, S. Y., and Rajiv, P. (2021). “Applications of Silver Nanomaterial in Agricultural Pest Control,” in Silver Nanomaterials for Agri-Food Applications (Elsevier), 453–470. doi:10.1016/b978-0-12-823528-7.00002-0
Rouhani, M., Samih, M. A., and Kalantari, S. (2013). Insecticidal Effect of Silica and Silver Nanoparticles on the Cowpea Seed Beetle, Callosobruchus maculatus F. (Col.: Bruchidae). J. Entomol. Res. 4, 297–305.
Rouhani, M., Samih, M. A., Zarabi, M., Beiki, K., Gorji, M., and Aminizadeh, M. R. (2019). Synthesis and Entomotoxicity Assay of Zinc and Silica Nanoparticles against Sitophilus granarius (Coleoptera: Curculionidae). J. Plant Prot. Res. 59, 26–31.
Sabbour, M. M., and Abd El-Aziz, S. E. A. (2016). Efficacy of three essential oils and their nano-particles against Sitophilus granarius under laboratory and store conditions.. Journal of Entomological Research, 40 (3), 229–234.
Sabbour, M. M., and Abd El-Aziz, S. E. S. (2019). Impact of Certain Nano Oils against Ephestia kuehniella and Ephestia cautella (Lepidoptera-Pyralidae) under Laboratory and Store Conditions. Bull. Natl. Res. Centre 43 (1), 1–7. doi:10.1186/s42269-019-0129-3
Sabbour, M. M. A. (2020b). Efficacy of Nano-Formulated Certain Essential Oils on the Red Flour Beetle Tribolium castaneum and Confused Flour Beetle, Tribolium confusum (Coleoptera: Tenebrionidae) under Laboratory and Storage Conditions. Bull. Natl. Res. Centre 44 (1), 1–7. doi:10.1186/s42269-020-00336-6
Sabbour, M. M. (2020a). Effect of Some Essential Oils on Rhyzopertha dominica (Coleoptera: Bostrichidae). Int. Resaerch J. Biol. Sci. 2 (1), 32–37.
Sabbour, M. M. (2012). Entomotoxicity Assay of Two Nanoparticle Materials 1-(Al2O3and TiO2) against Sitophilus oryzae under Laboratory and Store Conditions in Egypt. J. Nov Appl. Sci. 1 (4), 103–108.
Saed, B., Ziaee, M., Kiasat, A. R., and Jafari nasab, M. (2021). Preparation of Nanosilica from Sugarcane Bagasse Ash for Enhanced Insecticidal Activity of Diatomaceous Earth against Two Stored-Products Insect Pests. Toxin Rev., 1–7. doi:10.1080/15569543.2021.1903038
Sahoo, M., Vishwakarma, S., Panigrahi, C., and Kumar, J. (2021). Nanotechnology: Current Applications and Future Scope in Food. Food Front. 2 (1), 3–22. doi:10.1002/fft2.58
Salem, A. A. (2020). Comparative Insecticidal Activity of Three Forms of Silica Nanoparticles on Some Main Stored Product Insects. J. Plant Prot. Pathol. 11 (4), 225–230. doi:10.21608/jppp.2020.96009
Salem, A. A., Hamzah, A. M., and El-Taweelah, N. M. (2015). Aluminum and Zinc Oxides Nanoparticles as A New Methods for Controlling the Red Flour Beetles, Tribolium Castaneum (Herbest) Compared to Malathion Insecticide. J. Plant Prot. Pathol. 6 (1), 129–137. doi:10.21608/jppp.2015.53186
Salman, W. L., and Hameed, H. N. (2020). The Effect of Efficacy of Some Plant Powders, Biological Treatemnts and Some Nanoscale Applications in the Control of Oryzaephilus Serrinamensis Beetle. Eur. J. Mol. Clin. Med. 7 (9), 157–163.
Saratale, G. D., Saratale, R. G., Benelli, G., Kumar, G., Pugazhendhi, A., Kim, D. S., et al. (2017). Anti-diabetic Potential of Silver Nanoparticles Synthesized with Argyreia Nervosa Leaf Extract High Synergistic Antibacterial Activity with Standard Antibiotics against Food Borne Bacteria. J. Cluster Sci. 28 (3), 1709–1727. doi:10.1007/s10876-017-1179-z
Sarwar, M. F., Sarwar, M. H., Sarwar, M., Qadri, N. A., and Moghal, S. (2013). The Role of Oilseeds Nutrition in Human Health: A Critical Review. J. Cereals oilseeds 4 (8), 97–100. doi:10.5897/jco12.024
Sasson, Y., Levy-Ruso, G., Toledano, O., and Ishaaya, I. (2007). “Nanosuspensions: Emerging Novel Agrochemical Formulations,” in Insecticides Design Using Advanced Technologies. Editors I. Ishaaya, A. R. Horowitz, and R. Nauen (Berlin, Heidelberg: Springer), 1–39. doi:10.1007/978-3-540-46907-0_1
Scheffrahn, R. H., Hsu, R. C., Osbrink, W. L., and Su, N. Y. (1989). Fluoride and Sulfate Residues in Foods Fumigated with Sulfuryl Fluoride. J. Agric. Food Chem. 37 (1), 203–206. doi:10.1021/jf00085a046
Scott, N. R., Chen, H., and Cui, H. (2018). Nanotechnology Applications and Implications of Agrochemicals toward Sustainable Agriculture and Food Systems. J. Agric. Food Chem. 66, 6451–6456. doi:10.1021/acs.jafc.8b00964
Scrinis, G., and Lyons, K. (2007). The Emerging Nano-Corporate Paradigm: Nanotechnology and the Transformation of Nature, Food and Agri-Food Systems. Int. J. Sociol. Agric. Food 15(2), 22–44. doi:10.48416/ijsaf.v15i2.293
Sedighi, A., Imani, S., Moshtaghi Kashanian, G. R., Najafi, H., and Fathipour, Y. (2019). Efficiency of Green Synthesized Silver Nanoparticles with Sweet Orange, Citrus Sinensis (L.)(Rutaceae, Sapindales) against Tribolium confusum Duval.(Coleoptera, Tenebrionidae). J. Agric. Sci. Tech. 21 (6), 1485–1494.
Selvaraj, P., Christina Angelin, P., and Princy Rathnamala Jayaseeli, J. (2019). Insecticidal and Antibacterial Potential of Syzygium aromaticum (L.) merrill and perry. J. Biopesticides 12 (2), 191–196.
Sh, A., Abdelrazeik, A. B., and Rakha, O. M. (2015). Nanoemulsion of Jojoba Oil, Preparation, Characterization and Insecticidal Activity against Sitophilus oryzae (Coleoptera: Curculionidae) on Wheat. Intern. J. Agri. Innov. Res. 4, 72–75.
Shaban, S. M., Kang, J., and Kim, D. H. (2020). Surfactants: Recent advances and their applications. Composites Communications 22, 100537.
Shah, A. (2019). Biomedical Potential of Silver and Gold Nanoparticles Synthesized from Daphne mucronata and Monotheca buxifolia as New Precursors. Peshawar: Doctoral dissertation, University of Peshawar.
Shahzadi, K., Bashir, F., Nazir, K., Seemab, S., Shaker, M. A. A., Waheed, I., et al. (2019). Impacts of green Synthesized Silver-Nanoparticles against Tribolium castaneum (Coleoptera: Tenebrionidae). Int. J. Entomol. Res. 4 (6), 114–117.
Shankar, U., and Abrol, D. P. (2012). 14 Integrated Pest Management in Stored Grains. Integrated pest management: principles and practice, 386. United Kingdom: CAB International. doi:10.1079/9781845938086.0386
Sharma, H. C., Srivastava, C. P., Durairaj, C., and Gowda, C. L. L. (2010). “Pest Management in Grain Legumes and Climate Change,” in Climate Change and Management of Cool Season Grain Legume Crops (Dordrecht: Springer), 115–139. doi:10.1007/978-90-481-3709-1_7
Sharma, S., Thakur, A. K., and Maiti, R. (2016). Post-harvest Technology for Reducing Stress on Bioresource: Recent Advances and Future Needs. In: R Maiti, A Kumari, A Thakur, and N Sarkar. (eds) Bioresource and Stress Management. Springer, Singapore. pp 229–256. doi:10.1007/978-981-10-0995-2_12
Sharon, M., Abirami, C. V., and Alagusundaram, K. (2014). Grain Storage Management in India. J. Postharvest Tech. 2 (1), 12–24.
Shekhar, S., Sharma, S., Kumar, A., Taneja, A., and Sharma, B. (2021). The Framework of Nanopesticides: a Paradigm in Biodiversity. Mater. Adv. 2 (20), 6569–6588. doi:10.1039/d1ma00329a
Shin, H. Y., An, J. S., Lee, J. M., You, S. G., and Shin, I. S. (2021). Phosphine Residues and Physicochemical Stability of Hwangtae after Fumigation. Food Sci. Biotechnol. 30 (8), 1025–1031. doi:10.1007/s10068-021-00944-6
Shukla, P., Chaurasia, P., Younis, K., Qadri, O. S., Faridi, S. A., and Srivastava, G. (2019). Nanotechnology in Sustainable Agriculture: Studies from Seed Priming to post-harvest Management. Nanotechnology Environ. Eng. 4 (1), 11. doi:10.1007/s41204-019-0058-2
Siddique, M. A., Sagheer, M., and Sahi, S. T. (2021). Comparative Toxic Effects of Eucalyptus globulus L.(Myrtales: Myrtaceae) and its green Synthesized Zinc Oxide Nanoparticles (ZnONPs) against Rhyzopertha dominica (F.)(Coleoptera: Bostrichidae). Int. J. Trop. Insect Sci. doi:10.1007/s42690-021-00691-5
Singh, A., Dhiman, N., Kar, A. K., Singh, D., Purohit, M. P., Ghosh, D., et al. (2020). Advances in Controlled Release Pesticide Formulations: Prospects to Safer Integrated Pest Management and Sustainable Agriculture. J. Hazard. Mater. 385, 121525. doi:10.1016/j.jhazmat.2019.121525
Singh, H., Sharma, A., Bhardwaj, S. K., Arya, S. K., Bhardwaj, N., and Khatri, M. (2021). Recent Advances in the Applications of Nano-Agrochemicals for Sustainable Agricultural Development. Environ. Sci. Process. Impacts 23 (2), 213–239. doi:10.1039/D0EM00404ASingh
Singh, R. P., Handa, R., and Manchanda, G. (2021). Nanoparticles in Sustainable Agriculture: An Emerging Opportunity. J. Controlled Release 329, 1234–1248. doi:10.1016/j.jconrel.2020.10.051
Sioutas, C., Delfino, R. J., and Singh, M. (2005). Exposure Assessment for Atmospheric Ultrafine Particles (UFPs) and Implications in Epidemiologic Research. Environ. Health Perspect. 113, 947–955. doi:10.1289/ehp.7939
Sivapriya, V., Azeez, A. N., and Deepa, S. V. (2018). Phyto Synthesis of Iron Oxide Nano Particles Using the Agro Waste of Anthocephalus cadamba for Pesticidal Activity against Sitophilus granaries. J. Entomol. Zool. Stud. 6, 1050–1057.
Skocaj, M., Filipic, M., Petkovic, J., and Novak, S. (2011). Titanium Dioxide in Our Everyday Life; Is it Safe? Radiol. Oncol. 45 (4), 227. doi:10.2478/v10019-011-0037-0
Small, T., Ochoa-Zapater, M. A., Gallello, G., Ribera, A., Romero, F. M., Torreblanca, A., et al. (2016). Gold-nanoparticles Ingestion Disrupts Reproduction and Development in the German Cockroach. Sci. Total Environ. 565, 882–888. doi:10.1016/j.scitotenv.2016.02.032
Smith, K., Evans, D. A., and El-Hiti, G. A. (2008). Role of Modern Chemistry in Sustainable Arable Crop protection. Phil. Trans. R. Soc. B: Biol. Sci. 363 (1491), 623–637. doi:10.1098/rstb.2007.2174
Sparks, T. C., Storer, N., Porter, A., Slater, R., and Nauen, R. (2021). Insecticide Resistance Management and Industry: the Origins and Evolution of the I Nsecticide R Esistance A Ction C Ommittee (IRAC) and the Mode of Action Classification Scheme. Pest Manag. Sci. 77 (6), 2609–2619. doi:10.1002/ps.6254
Stadler, T., Buteler, M., Weaver, D. K., and Sofie, S. (2012). Comparative Toxicity of Nanostructured Alumina and a Commercial Inert Dust for Sitophilus oryzae (L.) and Rhyzopertha dominica (F.) at Varying Ambient Humidity Levels. J. stored Prod. Res. 48, 81–90. doi:10.1016/j.jspr.2011.09.004
Stadler, T., Lopez Garcia, G. P., Gitto, J. G., and Buteler, M. (2017). Nanostructured Alumina: Biocidal Properties and Mechanism of Action of a Novel Insecticide Powder. Bull. Insectol 70 (1), 17–25.
Sushil, A., Kamla, M., Nisha, K., Karmal, M., and Sandeep, A. (2021). Nano-Enabled Pesticides in Agriculture: Budding Opportunities and Challenges. J. Nanoscience Nanotechnology 21 (6), 3337–3350. doi:10.1166/jnn.2021.19018
Tong, Y., Wu, Y., Zhao, C., Xu, Y., Lu, J., Xiang, S., et al. (2017). Polymeric Nanoparticles as a Metolachlor Carrier: Water-Based Formulation for Hydrophobic Pesticides and Absorption by Plants. J. Agric. Food Chem. 65 (34), 7371–7378. doi:10.1021/acs.jafc.7b02197
Torchilin, V. (2006). “Introduction. Nanocarriers for Drug Delivery: Needs and Requirements,” in Nanoparticulates as Drug Carriers (Springer), 1–8. doi:10.1142/9781860949074_0001
Tranfo, G., Manni, V., Brusco, A., Bucci, G., Cabella, R., Fizzano, M. R., et al. (2020). A Pilot Survey on Usability and Effectiveness of Extended Data Sheets of Chemicals. Ital. J. Occup. Environ. Hyg. 11 (2), 56.
Unsworth, J. B., Corsi, C., Van Emon, J. M., Farenhorst, A., Hamilton, D. J., Howard, C. J., et al. (2016). Developing Global Leaders for Research, Regulation, and Stewardship of Crop protection Chemistry in the 21st century. J. Agric. Food Chem. 64 (1), 52–60. doi:10.1021/jf5060744
Upadhyay, N., Singh, V. K., Dwivedy, A. K., Das, S., Chaudhari, A. K., and Dubey, N. K. (2019). Assessment of Melissa officinalis L. Essential Oil as an Eco-Friendly Approach against Biodeterioration of Wheat Flour Caused by Tribolium castaneum Herbst. Environ. Sci. Pollut. Res. 26 (14), 14036–14049. doi:10.1007/s11356-019-04688-z
ur Rehman, H., Majeed, B., Farooqi, M. A., Rasul, A., Sagheer, M., Ali, Q., et al. (2021). Green Synthesis of Silver Nitrate Nanoparticles from Camelina sativa (L.) and its Effect to Control Insect Pests of Stored Grains. Int. J. Trop. Insect Sci. doi:10.1007/s42690-021-00495-7
Vadlapudi, V., and Amanchy, R. (2017). Phytofabrication of Silver Nanoparticles Using Myriostachya wightiana as a Novel Bioresource, and Evaluation of Their Biological Activities. Braz. Arch. Biol. Tech. 60. doi:10.1590/1678-4324-2017160329
Valmas, N., Zuryn, S., and Ebert, P. R. (2008). Mitochondrial Uncouplers Act Synergistically with the Fumigant Phosphine to Disrupt Mitochondrial Membrane Potential and Cause Cell Death. Toxicology 252 (1-3), 33–39. doi:10.1016/j.tox.2008.07.060
Vijaya Sankar, M., and Abideen, S. (2015). Pesticidal Effect of green Synthesized Silver and lead Nanoparticles Using Avicennia marina against Grain Storage Pest Sitophilus oryzae. Int. J. Nanomater. Biostruct 5 (3), 32–39.
Villaverde, J. J., Sevilla‐Morán, B., Sandín‐España, P., López‐Goti, C., and Alonso‐Prados, J. L. (2014). Biopesticides in the Framework of the European Pesticide Regulation (EC) No. 1107/2009. Pest Manag. Sci. 70 (1), 2–5. doi:10.1002/ps.3663
Wakil, W., Kavallieratos, N. G., Usman, M., Gulzar, S., and El-Shafie, H. A. (2021). Detection of Phosphine Resistance in Field Populations of Four Key Stored-Grain Insect Pests in Pakistan. Insects 12 (4), 288. doi:10.3390/insects12040288
Walker, G. W., Kookana, R. S., Smith, N. E., Kah, M., Doolette, C. L., Reeves, P. T., and Navarro, D. A. (2017). Ecological Risk Assessment of Nano-Enabled Pesticides: a Perspective on Problem Formulation. J. Agric. Food Chem. 66 (26), 6480–6486. doi:10.1021/acs.jafc.7b02373
Wang, J., Zhu, F., Zhou, X. M., Niu, C. Y., and Lei, C. L. (2006). Repellent and Fumigant Activity of Essential Oil from Artemisia vulgaris to Tribolium castaneum (Herbst)(Coleoptera: Tenebrionidae). J. Stored Prod. Res. 42 (3), 339–347. doi:10.1016/j.jspr.2005.06.001
Wang, Y., Yuan, Z., and Tang, Y. (2021). Enhancing Food Security and Environmental Sustainability: A Critical Review of Food Loss and Waste Management. Resour. Environ. Sustainability 4, 100023. doi:10.1016/j.resenv.2021.100023
Watson, S. B., Gergely, A., and Janus, E. R. (2011). Where Is Agronanotechnolgoy Heading in the United States and European Union. Nat. Resour. Env't 26, 8.
Wazid, S. N., Prabhuraj, A., Naik, R. H., Shakuntala, N. M., and Sharanagouda, H. (2020). The Persistence of Residual Toxicity of Zinc, Copper and Silica green Nanoparticles against Important Storage Pests. J. Entomol. Zoolog. Stud. 8, 1207–1211.
Ya-Ali, P., Yarahmadi, F., and Mehrnia, M. A. (2020). Efficacies of Two Nano-Formulations of Tasmanian Blue Gum Essential Oil to Control Callosobruchus maculatus. J. Econ. Entomol. 113 (3), 1555–1562. doi:10.1093/jee/toaa069
Yadav, J., Jasrotia, P., Bhardwaj, A. K., Kashyap, P. L., Kumar, S., Singh, M., et al. (2021). Nanopesticides: Current Status and Scope for Application in Agriculture. Plant Prot. Sci. 58, 1–17. doi:10.17221/102/2020-PPS
Yang, F. L., Li, X. G., Zhu, F., and Lei, C. L. (2009). Structural Characterization of Nanoparticles Loaded with Garlic Essential Oil and Their Insecticidal Activity against Tribolium castaneum (Herbst)(Coleoptera: Tenebrionidae). J. Agric. Food Chem. 57 (21), 10156–10162. doi:10.1021/jf9023118
Ye, C. Y., and Fan, L. (2021). Orphan Crops and Their Wild Relatives in the Genomic Era. Mol. Plant 14 (1), 27–39. doi:10.1016/j.molp.2020.12.013
Yerragopu, P. S., Hiregoudar, S., Nidoni, U., Ramappa, K. T., Sreenivas, A. G., and Doddagoudar, S. R. (2019). Effect of Plant-Mediated Synthesized Silver Nanoparticles on Pulse Beetle, Callosobruchus chinensis (L.). Int. J. Curr. Microbiol. App. Sci, 8 (9), 1965–1972.
Zahir, A. A., Bagavan, A., Kamaraj, C., Elango, G., and Rahuman, A. A. (2012). Efficacy of Plant-Mediated Synthesized Silver Nanoparticles against Sitophilus oryzae. J. Biopesticides 5, 95.
Ziaee, M., and Babamir-Satehi, A. (2020). Insecticidal Efficacy of Silica Nanoparticles Loaded with Several Insecticides in Controlling Khapra Beetle Larvae, Trogoderma granarium on Mosaic and Galvanized Steel Surfaces. Plant Prot. 43 (2), 35–47. doi:10.22055/ppr.2020.15975
Ziaee, M., Moharramipour, S., and Mohsenifar, A. (2014). Toxicity of Carum Copticum Essential Oil‐loaded Nanogel against Sitophilus Granarius and Tribolium confusum. J. Appl. Entomol. 138 (10), 763–771. doi:10.1111/jen.12133
Keywords: nanotechnology, storage, insect control, nanopesticides, formulation
Citation: Jasrotia P, Nagpal M, Mishra CN, Sharma AK, Kumar S, Kamble U, Bhardwaj AK, Kashyap PL, Kumar S and Singh GP (2022) Nanomaterials for Postharvest Management of Insect Pests: Current State and Future Perspectives. Front. Nanotechnol. 3:811056. doi: 10.3389/fnano.2021.811056
Received: 08 November 2021; Accepted: 13 December 2021;
Published: 03 February 2022.
Edited by:
Kamel A. Abd-Elsalam, Agricultural Research Center, EgyptReviewed by:
Vijayakumar Sekar, Shandong University, ChinaMontcharles da Silva Pontes, State University of Mato Grosso do Sul, Brazil
Copyright © 2022 Jasrotia, Nagpal, Mishra, Sharma, Kumar, Kamble, Bhardwaj, Kashyap, Kumar and Singh. This is an open-access article distributed under the terms of the Creative Commons Attribution License (CC BY). The use, distribution or reproduction in other forums is permitted, provided the original author(s) and the copyright owner(s) are credited and that the original publication in this journal is cited, in accordance with accepted academic practice. No use, distribution or reproduction is permitted which does not comply with these terms.
*Correspondence: Poonam Jasrotia, cG9vbmFtamFzcm90aWFAZ21haWwuY29t