- 1Department of Physics and Chemistry, School of Engineering, São Paulo State University (UNESP), Ilha Solteira, Brazil
- 2Natural Resources Program, Center for Natural Resources Studies (CERNA), Mato Grosso do Sul State University (UEMS), Dourados, Brazil
- 3Department of Bioproducts and Biosystems, School of Chemical Engineering, Aalto University, Espoo, Finland
Controlled release systems of agrochemicals have been developed in recent years. However, the design of intelligent nanocarriers that can be manufactured with renewable and low-cost materials is still a challenge for agricultural applications. Lignocellulosic building blocks (cellulose, lignin, and hemicellulose) are ideal candidates to manufacture ecofriendly nanocarriers given their low-cost, abundancy and sustainability. Complexity and heterogeneity of biopolymers have posed challenges in the development of nanocarriers; however, the current engineering toolbox for biopolymer modification has increased remarkably, which enables better control over their properties and tuned interactions with cargoes and plant tissues. In this mini-review, we explore recent advances on lignocellulosic-based nanocarriers for the controlled release of agrochemicals. We also offer a critical discussion regarding the future challenges of potential bio-based nanocarrier for sustainable agricultural development.
Introduction
The development of environmentally friendly tools that can produce crops or livestock without negative impact on humans and the environment is central to the Sustainable Development Goals. Nowadays, with the development of nanotechnology, researchers are obtaining good outocomes using nanomaterials (NMs) (Mattos et al., 2017; Guo et al., 2021; Sikder et al., 2021). For instance, engineered nanoparticles (ENPs) are capable of improving the efficiency of pesticides and fertilizers through the controlled release of agrochemicals, as well as by providing enhanced plant performance or by enabling plants to act as real-time sensors, actuators, or electronic devices (Baker et al., 2017; Saleem and Zaidi, 2020; Agathokleous et al., 2020; Acharya and Pal, 2020; Usman et al., 2020; Singh et al., 2021; Grillo et al., 2021a). The increased surface area to volume ratio of the ENPs enables a greater control of interfacial interactions with a given cargo to act on demand or specific stimuli as well as many other features. Besides, several factors such as surface charge, particle size, composition, solubility, and manufacturing methods can be exploited to control the interaction of ENPs with specific plants and organisms (Jogaiah et al., 2021), with the goal of developing targeted systems. Although most of the current nanopesticides or nanocarriers are developed from synthetic (e.g., polymers) building blocks, several systems have been developed using biopolymers as tools for the controlled release system of agrochemicals (Mattos et al., 2017). Lignocellulosic-based nanopesticides are biodegradable and offer an interesting platform to produce safe-by-design nanopesticides as they can be non-toxic (Chamundeeswari et al., 2019; Zhang et al., 2019; Bhattacharyya et al., 2020; Shrestha et al., 2021; Ur Rahim et al., 2021) (Figure 1). Moreover, lignocellulosic material can be obtained from agriculture side streams, which is ideal to reduce costs during the production of nanopesticides, which are intended to be applied in large scale agricultural operations. This strategy also indicates a from plant-to-plant effort, ideal for a circular bioeconomy landscape.
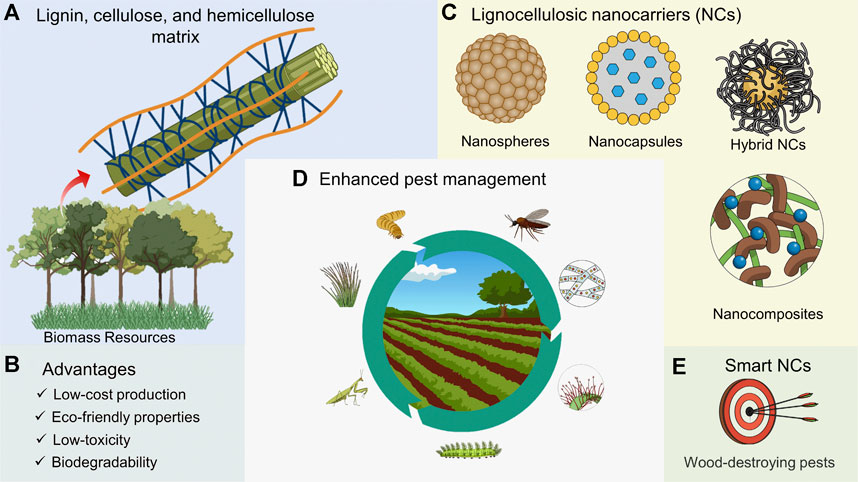
FIGURE 1. Schematic representation of a (A) lignocellulosic building blocks matrix, (B) advantages to produce, and (C) different types of nanopesticides that can (D) enhance pest management (e.g., weeds, viruses, bacteria, fungi, and insects) as well as display unique properties such as (E) targeting wood-destroying pests.
There are mainly three non-edible lignocellulosic biopolymers that have been used in the development of materials: cellulose, lignin, and hemicellulose, along with pectin, tannins, acids, and proteins (Okolie et al., 2021). Even though the manipulation of its feedstock is challenging to engineer, lignocellulosic materials have low-cost production and eco-friendly properties (Li et al., 2021a). Although lignocellulosic-based nanocarriers are still under development for the agricultural sector, several advances have been achieved thus making this technology useful for nano-enabled agriculture. The increased use of lignocellulosics in nano-enabled agriculture is expected to take place because there are new technologies that can 1) increase the extraction efficiency of lignocellulosic materials; 2) modify lignocellulosic structures for desired properties; and 3) design hybrid nanostructures with controllable shape and size. Nonetheless, the application of lignocellulosics in nano-enabled agriculture is currently related to the controlled delivery and release of pesticides and nutrients (Worrall et al., 2018; Papadopoulos et al., 2019; Chen et al., 2020a; Teo and Wahab., 2020). However, lignocellulosic nanomaterials could potentially stabilize emulsions (such as those used in oil borne pesticides) instead conventional surfactants in multiphase systems (Tardy et al., 2021). In this review we summarize and discuss the recent advances in lignocellulosic nanocarriers for agricultural applications; in addition, we offer a critical discussion regarding the future challenges of lignocellulosic nano-enabled materials for sustainable agricultural development.
Types of Lignocellulosic-Based Nanopesticides for Agriculture
Cellulose-Based Nanopesticides
Cellulose is a linear polymer composed of several hundreds of glucose units linked by β-1,4-glycosidic bonds (Figure 2A). Cellulose is highly abundant, being sourced from agro-industrial biomass (from 1st generation to wastes and residues), marine biomasses, and microorganisms. (Kaya and Tabak., 2020; Siqueira et al., 2020; Teo and Wahab., 2020; Bahloul et al., 2021). Cellulose nanomaterials have been marketed in several sectors of the economy, including agriculture. However, cellulose intra- and intermolecular hydrogen bonding interactions hinder its dissolution in water although being highly hydrophilic (Credou and Berthelot, 2014; Shatkin and Kim, 2015). Therefore, several studies have focused on the chemical modification of cellulose using a variety of routes to modify its physicochemical properties, enable dissolution, and therefore to facilitate the conception of nanocarriers that can be applied for improving agrochemical efficacy (Rop et al., 2020; Machado et al., 2021). For instance, dialdehyde carboxymethyl cellulose (DCMC) and carboxymethyl cellulose (CMC) were conjugated with zein (a protein from corn) to develop nanocarriers for avermectin (Chen et al., 2020b; Hao et al., 2020). In both cases, the resulting nanopesticides showed high leaf adhesion as well as efficient protection of the active ingredient against ultraviolet light (UV) when compared to its conventional form. Avermectin has been also encapsulated in CMC-grafted polyethyleneglycol (PEG) nanoparticles (Zhu et al., 2020) and in CMC grafted poly 2,2,3,4,4,4-hexafluorobutyl methacrylate (PHFBA) (Su et al., 2021a); both showed enhanced insecticidal activity against fall webworm (Hyphantria cunea) and an improved of the release profile of the active ingredient for 95 h, respectively. Additionally, glycine methyl ester (GLY) and glycidyl methacrylate (GMA) were used as intermediate and organic nitrogen sources, respectively, to modify CMC during the synthesis of a nanopesticide containing emamectin benzoate. Such nanopesticide and nanofertilizer increased the insecticidal activity against the diamondback moth insect (Plutella xylostella) and showed a potential system to be used as organic nitrogen fertilizer without toxic effect on seed germination (Zhao et al., 2021a).
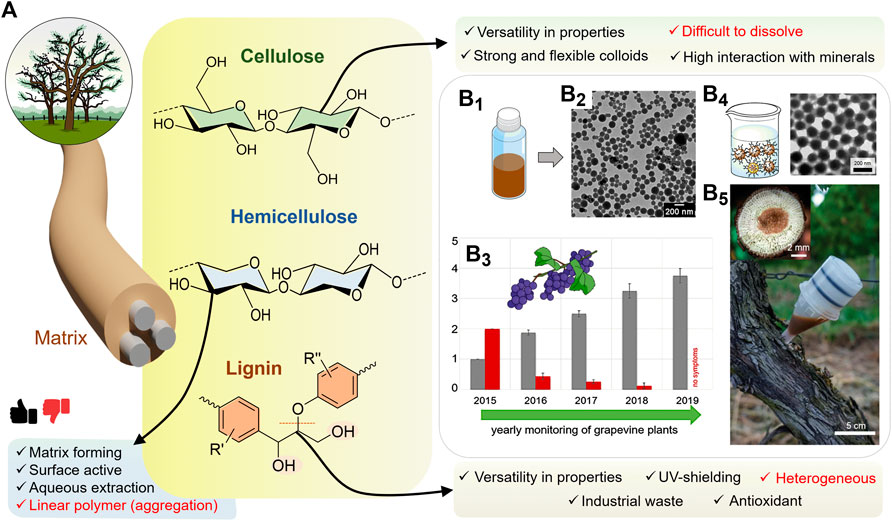
FIGURE 2. (A) Types of lignocellulosic materials (cellulose, hemicellulose (e.g., xylan) and lignin) pointing out their main advantages (black) and disadvantages (red). (B1) Representation of a formulation containing lignin nanoparticles. (B2) Transmission electron microscopy image of pyraclostrobin-loaded lignin nanoparticles in the range of 100—300 nm. (B3) Effect of empty lignin (gray bars) and fungicide-loaded lignin (red bars) NPs on Esca leaf symptoms in four Portugieser grapevine plants monitored yearly from 2015 to 2019. (B4) Transmission electron microscopy image of fungicide-loaded lignin NPs. (B5) Lignin-based fungicide-loaded NPs being applied in a grapevine plant to target Esca. (B2,B3) were reproduced with permission from Machado, 2020, Copyright (2020) American Chemical Society, and (B4,B5) were reproduced with permission from Machado, 2020, Copyright (2019) John Wiley and Sons. Further permissions related to the material excerpted should be directed to the ACS and John Wiley and Sons.
On the other hand, nanoparticles crosslinked by cellulose have received an increased attention in the agricultural sector due to their good stability under environmental conditions (Sun et al., 2020). For instance, stimuli-responsive nanocapsules based on cellulose modified with fatty acid (undec-10-enoic) loaded captan and pyraclostrobin were developed against apple canker (Neonectria ditissima), and the results showed that the active ingredients were released when triggered by the presence of cellulolytic fungi (Machado et al., 2021). Another study showed the fabrication of carboxymethylcellulose sodium salt and hydroxyethyl cellulose-based biodegradable hydrogels using citric acid (CA) as a crosslinker; good water uptake and a sustainable release profile were observed (Das et al., 2021).
A mixture of (nano)cellulose with inorganic (nano)materials (for example clays) opens up strategies for the design of nanopesticides with a widened pallet of biological, thermal, and structural properties. For instance, carboxymethyl cellulose hydrogels filled with nanocellulose and nanoclays (Bauli et al., 2021) or cellulose-g-poly (ammonium acrylate-co-acrylic acid)/nano-hydroxyapatite (Rop et al., 2020) showed good efficiency on hydrogel nutrient release and improved the moisture retention around the plant in the soil. Furthermore, a hybrid nanopesticide composed of hollow mesoporous silica/hydroxypropyl cellulose was reported to control rice blast fungus (Magnaporthe oryzae), and a dual-responsive release profile of the active ingredient was observed when in presence of cellulase (enzyme) or under acid conditions (Gao et al., 2021a). Moreover, layered double hydroxides (LDH) were mixed with CMC to fabricate polymeric nanocomposite able to mitigate the downside effect of herbicides in paddy cultivation (Sharif et al., 2021). In addition, they observed high adhesion of nanocellulose towards hydrophilic surfaces has led to the development of biogenic nanohybrids containing biogenic silica and cellulose nanofibers for the encapsulation of various molecules including a biopesticide (thymol) (Mattos and Magalhães, 2016; Mattos et al., 2018).
The difficulty of promoting dissolution of cellulose, in parallel with the current regulation on bioplastics that defines cellulose derivatives as plastics, will accelerate the development of nanocarriers from cellulose colloids, such as cellulose nanofibers, which have unique physicochemical properties such as higher surface area, nanodimension, high-temperature resistance, and biocompatibility (Shahi et al., 2021; Tardy et al., 2021). Cellulose nanofibers (CNFs) have been reported as potential high performers in nanoformulations of fertilization (do Nascimento et al., 2021), as well as cellulose nanocrystals (CNC) with chitosan to control tomato bacterial speck disease (Schiavi et al., 2021), among others (Table 1). For its diversity and abundance, cellulose is an outstanding material with a list of properties yet to be explored as a nanopesticide thus, understanding better its composition and potential interactions with agrochemicals and non- target and target organisms is essential to the future design of sustainable nano-enabled agriculture.
Lignin-Based Nanopesticides
Lignin is a three-dimensional and complex aromatic biopolymer that is bound to cellulose and hemicellulose within the plant cell wall microstructure (Figure 2A). Furthermore, it has attracted great attention due to its availability at large scales (Lizundia et al., 2021). In the last years, lignin-based nanoparticles (LNPs) have been used in agriculture against fungus, and insects due to their eco-friendly properties, low cost, and good encapsulating properties; however, this formulation is insoluble in the aqueous environment, which raises some technical difficulties when producing nanocarriers or promoting cargo delivery. Nevertheless, production of LNPs has been demonstrated to be possible for upscaling at reasonable cost (Abbati de Assis et al., 2018; Bangalore Ashok et al., 2018). For instance, stem lignin nanoparticles have been used as matrix in the controlled release of the herbicide diuron (Yearla and Padmasree, 2016). Chemical modifications in lignin to control their interactions with water and solubility (Balakshin and Capanema, 2015; Agustin et al., 2019; Falsini et al., 2019; Ma et al., 2020; Machado et al., 2020), also facilitate their use in nano-enabled strategies for agriculture. For instance, sodium lignosulfonate can electrostatically interact with other polymers [e.g., chitosan (Li et al., 2019)], and cationic surfactant [e.g., dodecyl dimethyl benzyl ammonium chloride (Zhang et al., 2021a), cetyltrimethylammonium bromide (Peng et al., 2020)] by self-assembly to form stimuli-responsive nanopesticides (Table 1). Kraft lignin (KL) has a tunable amphiphilic nature due to the abundant phenolic hydroxyl groups capable of forming a stable double-layered nanomaterial with ionic surfactants (Ela et al., 2020), nanocapsules with olive oil (Falsini et al., 2020), and is also able to chelate cationic metals (Sipponen et al., 2017). Other nanopesticides have also been synthesized with alkali lignin (AL) (Yin et al., 2020), organosolv lignin (Zhang et al., 2020a), and methacrylate lignin (Yiamsawas et al., 2021).
In this regard, some interesting studies have been using lignin nanoparticles for the controlled release of fungicide to target Esca (a type of grapevine trunk disease that negatively impacts grape yields and the wine industry around the world). For instance, Machado et al. (2020) developed several fungicide-loaded lignin nanocarriers (Figure 2B1,2) to be applied in a single injection into Vitis vinifera (“Portugieser”) plants, and the results showed successfully inhibition of lignase-producing fungi (e.g., Phaeomoniella chlamydospora and Phaeoacremonium minimum) as well as fungicide efficiency for at least 4 years against Esca (Figure 2B3). Furthermore, two other stimuli-responsive lignin-based nanocarriers were developed for the treatment of Esca (Fischer et al., 2019; Peil et al., 2020). In both studies, the fungi associated with Esca degraded lignin through secretion of ligninolytic enzymes (e.g., laccases and peroxidases) and, thus, released fungal spores (Trichoderma reesei) (Peil et al., 2020) and the hydrophobic fungicide pyraclostrobin (Fischer et al., 2019) loaded in lignin nanocarriers (Figure 2B4,5). Moreover, another triggered strategy is based on the controlled release of micronutrients , such as copper and iron, to fertilize and protect plants against various pathogens (Gazzurelli et al., 2020; Li et al., 2021b).
Despite the development of numerous lignin-based nanocarriers for agriculture, the great challenge of these nanoformulations is still the complexity and variability of chemical structure of this resource given the batch-to-batch variations in the extraction process. In this context, studies such as those by Beckers et al. (2021) have been important as it is possible to synthesize lignin-like monomers (e.g., phenylcoumaran and β-O-4-aryl ether) able to comprise linkages found in native lignin with promising results. Nevertheless, lignin-first biorefining approaches have recently been proposed to produce well-defined lignin structures that can be further utilized in a more systematic way (Lourencon et al., 2019).
Hemicellulose-Based Nanopesticides
Hemicellulose is a biopolymer with a degree of polymerization of ca. 50–200 and molecular weight below 90 kDa, thus a much smaller building block when compared to cellulose or lignin (Ye et al., 2021). Hemicelluloses represent 15–25% of the wood cell-wall but can reach up to 45% in annual, seasonal plants. Such biopolymers include xyloglucans, xylans (Figure 2A), mannans and glucomannans, and beta-glucans. A wide range of applications has been associated with hemicellulose, such as the generation of chemical products, packaging materials, drug delivery systems, and more recently as pesticide delivery systems (Naidu et al., 2018; Wijaya et al., 2021). The extraction of hemicellulose comes from lignocellulosic biomass, wood, foliage, grass, and agricultural residues and can be carried out using organic solvent but more commonly with hydrothermal extractions (Naidu et al., 2018).
Due to the low water solubility of hemicelluloses, there are still some challenges to produce stable hemicellulose-based nanocarriers for the controlled release of agrochemicals. However, several examples have been demonstrated in recent years. Beckers et al. (2020a) described the first synthesis of nanocarriers built from xylan extracted from corn cobs to contain the fungicide pyraclostrobin, by interfacial polymerization method of diisocyanate of toluene (TDI) in an inverse emulsion. Hence, such nanopesticide was colloidally stable in water and cyclohexane for several weeks as well as it was efficient against phytopathogenic fungi (Botrytis cinerea) as the biocide release from the xylan nanocarriers was stimulated by the fungi. In another study, xylan-based nanoparticles (without active ingredients) were fabricated and their antifungal effect was studied on corn husk fiber and on the high-density polyethylene (HDPE) composite. Such nanoparticles prevented the formation of hyphae in wood as well as increased the strength of the composite (Gao et al., 2021b). Additionally, lignin sulfonate-based nanocarriers containing hemicellulose residues showed potential for controlled delivery of agrochemicals such as the fungicides pyraclostrobin or prothioconazole (Beckers et al., 2020b). In addition, xylan-based nanoparticles are advantageous for their biocompatibility, biodegradability, and low-cost biological material (Beckers et al., 2020b). Recently, lignin-xylan and arabinoxylan nanoparticles were also reported as an enzymatic-responsive for pesticides release (Jiang et al., 2020) (Table 1) as well as a gene delivery system for CRISR-Cas9 DNA (Sarker et al., 2020), respectively.
β-Glucan is a homopolymer, an abundant class of polysaccharides in plants, fungi, and bacteria. Most recently, ß-Glucan-based nanocarriers have been extensively studied as drug delivery systems (Su et al., 2021b). For instance, Kaziem et al. (2022) developed a smart-delivery formulation based on carboxymethylated-β-glucans on the mesoporous silica nanoparticles (MSNs) surfaces after loading chlorothalonil (CHT) fungicide, with bioactivity against phytopathogens better than commercial formulation and lower toxicity to manure worm (Eisenia fetida) and zebra fish (Danio rerio). In another study with the same formulation, the authors observed that CHT@MSNs-β-glucans showed 2.6 times lower toxicity to the planktonic crustacean (Daphnia magna) and also exhibited lower effects on soil microbial abundance than commercial chlorothalonil (Kaziem et al., 2021), which improves its use as a nano-enabled agrochemical.
Other Lignocellulosic Materials-Based Nanopesticides
Other lignocellulosic materials, even in minor quantities, are rising in agriculture as compounds of nanocarriers. Pectin (Pec), a structural compound present in the primary cell walls of higher plants has been applied for the development of nano-enabled delivery systems for agrochemicals. Pectin has been used to configure an intelligent stimuli-responsive carrier triggered by pectinase, an enzymes produced by plants, filamentous fungi, bacteria, and yeasts. Moreover, a hybrid system comprising mesoporous silica nanoparticles and pectin (MSN-Pec) could delivery prochloraz (a fungicide) slowly, showing potential to be used in rice crops (Abdelrahman et al., 2021). Pectin-based nanocarriers showed promising behavior to mitigate drought stress in plants of arid and semi-arid environments (Sharma et al., 2017). Carbendazim-loaded chitosan-pectin nanoparticles showed a good response against pathogenic fungi Fusarium pxysporum and Aspergillus parasiticus (Kumar et al., 2017). Composite systems, such as chitosan/tripolyphosphate/pectin nanoparticles, were reported as a delivery system for paraquat herbicide to reduce the toxic behavior to alveolar and mouth cell lines, as well as to enhance the herbicidal activity against maize and mustard plants (Rashidipour et al., 2019). Thus, the nanoencapsulation of paraquat improves its herbicidal activity and reduces its toxic and mutagenic effects (Grillo et al., 2015; Pontes et al., 2021; Rashidipour et al., 2021).
Tannins, especially tannic acid, have been used in nanopesticide formulations (Table 1) as an additive to promote better foliage adhesion of particulates (Yu et al., 2019; Zhi et al., 2020). The ability of tannins to promote multiple and diverse secondary interactions towards virtually any surface has warranted their utilization to modify nanocarriers surfaces while adding UV protection and antioxidant properties (both useful to increase the lifetime of the active ingredient) (Guo et al., 2016). Finally, acids such as rosin (abietic acid) and salicylic acid are used in the attempt to create efficient nanocarriers (Table 1) (Zhao et al., 2021b; Su et al., 2021c).
Concluding Remarks and Challenges Ahead
Current research on the smart nano-enabled delivery systems for pesticides has opened a new way to view the stimuli-responsive controlled release of crop protectants and to the development of novel agro-technological products. Despite the complexity of biomolecules and the challenges on generating stable nanoformulations, lignocellulosic-based nanocarriers have shown promising features that can be further explored in advanced, and renewable nanocarriers for agriculture. These carriers display unique properties (e.g., targeting wood-destroying pests), low cost, and good biodegradability rate. However, the number of studies on the mechanism of action of these nanopesticides as well as their toxicity impacts in the environment is still very limited (Grillo et al., 2021b). Moreover, little is known about the biodegradation of biopolymers after their modification or compositing, which still restricts their wider utilization in large-scale platforms such as crop protection. Therefore, further research on lignocellulosic nanomaterials is necessary in order to improve the efficient use of biomass resources as well as achieving environmental sustainability in agriculture.
Author Contributions
PL, DA, and MF writing, review and editing the manuscript, MP and BM review and editing the manuscript, and RG conceptualization, writing, review, editing and supervision the manuscript. All authors contributed to manuscript revision, read, and approved the submitted version.
Funding
The authors acknowledge funding from National Council for Scientific and Technological Development, CNPq (Grant no. #427498/2018-0), São Paulo Research Foundation, FAPESP (#2020/12769-0), and Coordenação de Aperfeiçoamento de Pessoal de Nível Superior—Brasil, CAPES—Finance Code 001.
Conflict of Interest
The authors declare that the research was conducted in the absence of any commercial or financial relationships that could be construed as a potential conflict of interest.
Publisher’s Note
All claims expressed in this article are solely those of the authors and do not necessarily represent those of their affiliated organizations, or those of the publisher, the editors and the reviewers. Any product that may be evaluated in this article, or claim that may be made by its manufacturer, is not guaranteed or endorsed by the publisher.
Acknowledgments
MF thanks FAPESP for scholarship (#2020/12769-0).
References
Abbati de Assis, C., Greca, L. G., Ago, M., Balakshin, M. Y., Jameel, H., Gonzalez, R., et al. (2018). Techno-Economic Assessment, Scalability, and Applications of Aerosol Lignin Micro- and Nanoparticles. ACS Sust. Chem. Eng. 6 (9), 11853–11868. doi:10.1021/acssuschemeng.8b02151
Abdelrahman, T. M., Qin, X., Li, D., Senosy, I. A., Mmby, M., Wan, H., et al. (2021). Pectinase-responsive Carriers Based on Mesoporous Silica Nanoparticles for Improving the Translocation and Fungicidal Activity of Prochloraz in rice Plants. Chem. Eng. J. 404, 126440. doi:10.1016/j.cej.2020.126440
Acharya, A., and Pal, P. K. (2020). Agriculture Nanotechnology: Translating Research Outcome to Field Applications by Influencing Environmental Sustainability. NanoImpact 19, 100232. doi:10.1016/j.impact.2020.100232
Agathokleous, E., Feng, Z., Iavicoli, I., and Calabrese, E. J. (2020). Nano-pesticides: A Great challenge for Biodiversity? the Need for a Broader Perspective. Nano Today 30, 100808. doi:10.1016/j.nantod.2019.100808
Agustin, M. B., Penttilä, P. A., Lahtinen, M., and Mikkonen, K. S. (2019). Rapid and Direct Preparation of Lignin Nanoparticles from Alkaline Pulping Liquor by Mild Ultrasonication. ACS Sust. Chem. Eng. 7 (24), 19925–19934. doi:10.1021/acssuschemeng.9b05445
Bahloul, A., Kassab, Z., El Bouchti, M., Hannache, H., Qaiss, A. E. K., Oumam, M., et al. (2021). Micro- and Nano-Structures of Cellulose from Eggplant Plant (Solanum Melongena L) Agricultural Residue. Carbohydr. Polym. 253, 117311. doi:10.1016/j.carbpol.2020.117311
Baker, S., Volova, T., Prudnikova, S. V., Satish, S., and Prasad M.N., N. (2017). Nanoagroparticles Emerging Trends and Future prospect in Modern Agriculture System. Environ. Toxicol. Pharmacol. 53, 10–17. doi:10.1016/j.etap.2017.04.012
Balakshin, M., and Capanema, E. (2015). On the Quantification of Lignin Hydroxyl Groups With31P and13C NMR Spectroscopy. J. Wood Chem. Techn. 35 (3), 220–237. doi:10.1080/02773813.2014.928328
Bangalore Ashok, R. P., Oinas, P., Lintinen, K., Sarwar, G., Kostiainen, M. A., and Österberg, M. (2018). Techno-economic Assessment for the Large-Scale Production of Colloidal Lignin Particles. Green. Chem. 20 (21), 4911–4919. doi:10.1039/c8gc02805b
Bauli, C. R., Lima, G. F., de Souza, A. G., Ferreira, R. R., and Rosa, D. S. (2021). Eco-friendly Carboxymethyl Cellulose Hydrogels Filled with Nanocellulose or Nanoclays for Agriculture Applications as Soil Conditioning and Nutrient Carrier and Their Impact on Cucumber Growing. Colloids Surf. A: Physicochemical Eng. Aspects 623, 126771. doi:10.1016/j.colsurfa.2021.126771
Beckers, S. J., Fischer, J., and Wurm, F. R. (2021). Synthetic Lignin-like and Degradable Nanocarriers. Polym. Chem. 12 (32), 4661–4667. doi:10.1039/d1py00818h
Beckers, S. J., Wetherbee, L., Fischer, J., and Wurm, F. R. (2020a). Fungicide‐loaded and Biodegradable Xylan‐based Nanocarriers. Biopolymers 111 (12), e23413. doi:10.1002/bip.23413
Beckers, S., Peil, S., and Wurm, F. R. (2020b). Pesticide-Loaded Nanocarriers from Lignin Sulfonates-A Promising Tool for Sustainable Plant Protection. ACS Sust. Chem. Eng. 8 (50), 18468–18475. doi:10.1021/acssuschemeng.0c05897
Bhattacharyya, C., Roy, R., Tribedi, P., Ghosh, A., and Ghosh, A. (2020). “Biofertilizers as Substitute to Commercial Agrochemicals,” in Agrochemicals Detection, Treatment and Remediation (Kolkata, India: Butterworth-Heinemann), 263–290. doi:10.1016/B978-0-08-103017-2.00011-8
Chamundeeswari, M., Jeslin, J., and Verma, M. L. (2019). Nanocarriers for Drug Delivery Applications. Environ. Chem. Lett. 17 (2), 849–865. doi:10.1007/s10311-018-00841-1
Chen, H., Lin, G., Zhou, H., Zhou, X., Xu, H., and Huang, S. (2018). Preparation of Avermectin/grafted CMC Nanoparticles and Their Sustained Release Performance. J. Polym. Environ. 26, 2945–2953. doi:10.1007/s10924-018-1182-y
Chen, L., Zhou, H., Hao, L., Li, Z., Xu, H., Chen, H., et al. (2020b). Dialdehyde Carboxymethyl Cellulose-Zein Conjugate as Water-Based Nanocarrier for Improving the Efficacy of Pesticides. Ind. Crops Prod. 150, 112358. doi:10.1016/j.indcrop.2020.112358
Chen, Z., Ragauskas, A., and Wan, C. (2020a). Lignin Extraction and Upgrading Using Deep Eutectic Solvents. Ind. Crops Prod. 147, 112241. doi:10.1016/j.indcrop.2020.112241
Credou, J., and Berthelot, T. (2014). Cellulose: from Biocompatible to Bioactive Material. J. Mater. Chem. B 2 (30), 4767–4788. doi:10.1039/c4tb00431k
Cui, J., Mo, D., Jiang, Y., Gan, C., Li, W., Wu, A., et al. (20192019). Fabrication, Characterization, and Insecticidal Activity Evaluation of Emamectin Benzoate−Sodium Lignosulfonate Nanoformulation with pH-Responsivity. Ind. Eng. Chem. Res. 58, 19741–19751. doi:10.1021/acs.iecr.9b03171
Das, D., Prakash, P., Rout, P. K., and Bhaladhare, S. (2021). Synthesis and Characterization of Superabsorbent Cellulose‐Based Hydrogel for Agriculture Application. Starch‐Stärke 73 (1-2), 1900284. doi:10.1002/star.201900284
do Nascimento, D. M., Nunes, Y. L., de Almeida, J. S., Leitão, R. C., Feitosa, J., Dufresne, A., et al. (2021). Development of an Integrated Process to Produce CNFs and Lignin and its Potential Applications for Agrochemical Delivery. Cellulose 28, 1–14. doi:10.1007/s10570-021-04200-2
Dong, J., Chen, W., Feng, J., Liu, X., Xu, Y., Wang, C., et al. (2021). Facile, Smart, and Degradable Metal–Organic Framework Nanopesticides Gated with FeIII-Tannic Acid Networks in Response to Seven Biological and Environmental Stimuli. ACS Appl. Mater. Inter. 13 (16), 19507–19520. doi:10.1021/acsami.1c04118
Ela, R. C. A., Tajiri, M., Newberry, N. K., Heiden, P. A., and Ong, R. G. (2020). Double-shell Lignin Nanocapsules Are a Stable Vehicle for Fungicide Encapsulation and Release. ACS Sust. Chem. Eng. 8 (46), 17299–17306. doi:10.1021/acssuschemeng.0c06686
Falsini, S., Tani, C., Schiff, S., Gonnelli, C., Clemente, I., Ristori, S., et al. (2020). A New Method for the Direct Tracking of In Vivo Lignin Nanocapsules in Eragrostis Tef (Poaceae) Tissues. Eur. J. Histochem. 64 (2). doi:10.4081/ejh.2020.3112
Falsini, S., Clemente, I., Papini, A., Tani, C., Schiff, S., Salvatici, M. C., et al. (2019). When Sustainable Nanochemistry Meets Agriculture: Lignin Nanocapsules for Bioactive Compound Delivery to Plantlets. ACS Sust. Chem. Eng. 7 (24), 19935–19942. doi:10.1021/acssuschemeng.9b05462
Fischer, J., Beckers, S. J., Yiamsawas, D., Thines, E., Landfester, K., and Wurm, F. R. (2019). Targeted Drug Delivery in Plants: Enzyme-Responsive Lignin Nanocarriers for the Curative Treatment of the Worldwide Grapevine Trunk Disease Esca. Adv. Sci. (Weinh) 6, 1802315. doi:10.1002/advs.201802315
Gao, X., Fan, S., Pang, J., Rahman, M. Z., Zhu, D., Guo, S., et al. (2021b). Preparation of Nano-Xylan and its Influences on the Anti-fungi Performance of Straw Fiber/HDPE Composite. Ind. Crops Prod. 171, 113954. doi:10.1016/j.indcrop.2021.113954
Gao, Y., Liu, Y., Qin, X., Guo, Z., Li, D., Li, C., et al. (2021a). Dual Stimuli-Responsive Fungicide Carrier Based on Hollow Mesoporous Silica/hydroxypropyl Cellulose Hybrid Nanoparticles. J. Hazard. Mater. 414, 125513. doi:10.1016/j.jhazmat.2021.125513
Gazzurelli, C., Migliori, A., Mazzeo, P. P., Carcelli, M., Pietarinen, S., Leonardi, G., et al. (2020). Making Agriculture More Sustainable: an Environmentally Friendly Approach to the Synthesis of Lignin@ Cu Pesticides. ACS Sust. Chem. Eng. 8 (39), 14886–14895. doi:10.1021/acssuschemeng.0c04645
Grillo, R., Clemente, Z., de Oliveira, J. L., Campos, E. V., Chalupe, V. C., Jonsson, C. M., et al. (2015). Chitosan Nanoparticles Loaded the Herbicide Paraquat: the Influence of the Aquatic Humic Substances on the Colloidal Stability and Toxicity. J. Hazard. Mater. 286, 562–572. doi:10.1016/j.jhazmat.2014.12.021
Grillo, R., Mattos, B. D., Antunes, D. R., Forini, M. M., Monikh, F. A., and Rojas, O. J. (2021a). Foliage Adhesion and Interactions with Particulate Delivery Systems for Plant Nanobionics and Intelligent Agriculture. Nano Today 37, 101078. doi:10.1016/j.nantod.2021.101078
Grillo, R., Fraceto, L. F., Amorim, M. J. B., Scott-Fordsmand, J. J., Schoonjans, R., and Chaudhry, Q. (2021b). Ecotoxicological and Regulatory Aspects of Environmental Sustainability of Nanopesticides. J. Hazard. Mater. 404, 124148. doi:10.1016/j.jhazmat.2020.124148
Guo, J., Tardy, B. L., Christofferson, A. J., Dai, Y., Richardson, J. J., Zhu, W., et al. (2016). Modular Assembly of Superstructures from Polyphenol-Functionalized Building Blocks. Nat. Nanotechnol 11 (12), 1105–1111. doi:10.1038/nnano.2016.172
Guo, S., He, F., Song, B., and Wu, J. (2021). Future Direction of Agrochemical Development for Plant Disease in China. Food and Energy Security 10(4), e293. doi:10.1002/fes3.293
Hao, L., Lin, G., Lian, J., Chen, L., Zhou, H., Chen, H., et al. (2020). Carboxymethyl Cellulose Capsulated Zein as Pesticide Nano-Delivery System for Improving Adhesion and Anti-UV Properties. Carbohydr. Polym. 231, 115725. doi:10.1016/j.carbpol.2019.115725
Jiang, Y., Chen, Y., Tian, D., Shen, F., Wan, X., Xu, L., et al. (2020). Fabrication and Characterization of Lignin-Xylan Hybrid Nanospheres as Pesticide Carriers with Enzyme-Mediated Release Property. Soft Matter 16 (39), 9083–9093. doi:10.1039/d0sm01402h
Jogaiah, S., Paidi, M. K., Venugopal, K., Geetha, N., Mujtaba, M., Udikeri, S. S., et al. (2021). Phytotoxicological Effects of Engineered Nanoparticles: An Emerging Nanotoxicology. Sci. Total Environ. 801, 149809. doi:10.1016/j.scitotenv.2021.149809
Kaya, M., and Tabak, A. (2020). Recycling of an Agricultural Bio-Waste as a Novel Cellulose Aerogel: A green Chemistry Study. J. Polym. Environ. 28 (1), 323–330. doi:10.1007/s10924-019-01609-6
Kaziem, A. E., Yang, L., Lin, Y., Kazem, A. E., Xu, H., and Zhang, Z. X. (2021). Pathogenic Invasion-Responsive Carrier Based on Mesoporous Silica/β-Glucan Nanoparticles for Smart Delivery of Fungicides. ACS Sust. Chem. Eng. 9 (27), 9126–9138. doi:10.1021/acssuschemeng.1c02962
Kaziem, A. E., Yang, L., Lin, Y., Song, Z., Xu, H., and Zhang, Z. (2022). Efficiency of Mesoporous Silica/carboxymethyl β-glucan as a Fungicide Nano-Delivery System for Improving Chlorothalonil Bioactivity and Reduce Biotoxicity. Chemosphere 287, 131902. doi:10.1016/j.chemosphere.2021.131902
Kumar, S., Kumar, D., and Dilbaghi, N. (2017). Preparation, Characterization, and Bio-Efficacy Evaluation of Controlled Release Carbendazim-Loaded Polymeric Nanoparticles. Environ. Sci. Pollut. Res. 24 (1), 926–937. doi:10.1007/s11356-016-7774-y
Li, B., Lin, G., Duan, W., Wang, X., and Cen, B. (2021c). Synthesis of Myrtenal-Based Nanocellulose/Diacylhydrazine Complexes with Antifungal Activity for Plant Protection. J. Agric. Food Chem. 69 (44), 12956–12965. doi:10.1021/acs.jafc.1c02694
Li, P., Huang, Y., Fu, C., Jiang, S. X., Peng, W., Jia, Y., et al. (2021a). Eco‐friendly Biomolecule‐nanomaterial Hybrids as Next‐generation Agrochemicals for Topical Delivery. EcoMat 3, e12132. doi:10.1002/eom2.12132
Li, T., Lü, S., Yan, J., Bai, X., Gao, C., and Liu, M. (2019). An Environment-Friendly Fertilizer Prepared by Layer-By-Layer Self-Assembly for pH-Responsive Nutrient Release. ACS Appl. Mater. Inter. 11 (11), 10941–10950. doi:10.1021/acsami.9b01425
Li, T., Lü, S., Wang, Z., Huang, M., Yan, J., and Liu, M. (2021b). Lignin-based Nanoparticles for Recovery and Separation of Phosphate and Reused as Renewable Magnetic Fertilizers. Sci. Total Environ. 765, 142745. doi:10.1016/j.scitotenv.2020.142745
Liang, Y., Song, J., Dong, H., Huo, Z., Gao, Y., Zhou, Z., et al. (2021). Fabrication of pH-Responsive Nanoparticles for High Efficiency Pyraclostrobin Delivery and Reducing Environmental Impact. Sci. Total Environ. 787, 147422. doi:10.1016/j.scitotenv.2021.147422
Lizundia, E., Sipponen, M. H., Greca, L. G., Balakshin, M., Tardy, B. L., Rojas, O. J., et al. (2021). Multifunctional Lignin-Based Nanocomposites and Nanohybrids. Green. Chem. 23, 6698–6760. doi:10.1039/d1gc01684a
Lourencon, T. V., Greca, L. G., Tarasov, D., Borrega, M., Tamminen, T., Rojas, O. J., et al. (2019). Lignin-first Integrated Hydrothermal Treatment (HTT) and Synthesis of Low-Cost Biorefinery Particles. ACS Sust. Chem. Eng. 8 (2), 1230–1239. doi:10.1021/acssuschemeng.9b06511
Luo, J., Zhang, D. X., Jing, T., Liu, G., Cao, H., Li, B. X., et al. (2020). Pyraclostrobin Loaded Lignin-Modified Nanocapsules: Delivery Efficiency Enhancement in Soil Improved Control Efficacy on Tomato Fusarium crown and Root Rot. Chem. Eng. J. 394, 124854. doi:10.1016/j.cej.2020.124854
Ma, M., Dai, L., Xu, J., Liu, Z., and Ni, Y. (2020). A Simple and Effective Approach to Fabricate Lignin Nanoparticles with Tunable Sizes Based on Lignin Fractionation. Green. Chem. 22, 2011–2017. doi:10.1039/d0gc00377h
Machado, T. O., Beckers, S. J., Fischer, J., Müller, B., Sayer, C., de Araújo, P. H. H., et al. (2020). Bio-Based Lignin Nanocarriers Loaded with Fungicides as a Versatile Platform for Drug Delivery in Plants. Biomacromolecules 21, 2755–2763. doi:10.1021/acs.biomac.0c00487
Machado, T. O., Beckers, S. J., Fischer, J., Sayer, C., de Araújo, P. H. H., Landfester, K., et al. (2021). Cellulose Nanocarriers via Miniemulsion Allow Pathogen-specific Agrochemical Delivery. J. Colloid Interf. Sci. 601, 678–688. doi:10.1016/j.jcis.2021.05.030
Mattos, B. D., Greca, L. G., Tardy, B. L., Magalhães, W. L. E., and Rojas, O. J. (2018). Green Formation of Robust Supraparticles for Cargo Protection and Hazards Control in Natural Environments. Small 14 (29), e1801256. doi:10.1002/smll.201801256
Mattos, B. D., Tardy, B. L., Magalhães, W. L. E., and Rojas, O. J. (2017). Controlled Release for Crop and wood protection: Recent Progress toward Sustainable and Safe Nanostructured Biocidal Systems. J. Control. Release 262, 139–150. doi:10.1016/j.jconrel.2017.07.025
Mattos, B. D., and Magalhães, W. L. (2016). Biogenic Nanosilica Blended by Nanofibrillated Cellulose as Support for Slow-Release of Tebuconazole. J. Nanoparticle Res. 18 (9), 1–10. doi:10.1007/s11051-016-3586-8
Naidu, D. S., Hlangothi, S. P., and John, M. J. (2018). Bio-based Products from Xylan: A Review. Carbohydr. Polym. 179, 28–41. doi:10.1016/j.carbpol.2017.09.064
Okolie, J. A., Nanda, S., Dalai, A. K., and Kozinski, J. A. (2021). Chemistry and Specialty Industrial Applications of Lignocellulosic Biomass. Waste Biomass Valor. 12 (5), 2145–2169. doi:10.1007/s12649-020-01123-0
Papadopoulos, A. N., Bikiaris, D. N., Mitropoulos, A. C., and Kyzas, G. Z. (2019). Nanomaterials and Chemical Modifications for Enhanced Key Wood Properties: A Review. Nanomaterials (Basel) 9 (4), 607. doi:10.3390/nano9040607
Peil, S., Beckers, S. J., Fischer, J., and Wurm, F. R. (2020). Biodegradable, Lignin-Based Encapsulation Enables Delivery of Trichoderma Reesei with Programmed Enzymatic Release against grapevine Trunk Diseases. Mater. Today Bio 7, 100061. doi:10.1016/j.mtbio.2020.100061
Peng, R., Yang, D., Qiu, X., Qin, Y., and Zhou, M. (2020). Preparation of Self-Dispersed Lignin-Based Drug-Loaded Material and its Application in Avermectin Nano-Formulation. Int. J. Biol. Macromol 151, 421–427. doi:10.1016/j.ijbiomac.2020.02.114
Pontes, M. S., Antunes, D. R., Oliveira, I. P., Forini, M. M., Santos, J. S., Arruda, G. J., et al. (2021). Chitosan/tripolyphosphate Nanoformulation Carrying Paraquat: Insights on its Enhanced Herbicidal Activity. Environ. Sci. Nano 8 (5), 1336–1351. doi:10.1039/d0en01128b
Rashidipour, M., Maleki, A., Kordi, S., Birjandi, M., Pajouhi, N., Mohammadi, E., et al. (2019). Pectin/Chitosan/Tripolyphosphate Nanoparticles: Efficient Carriers for Reducing Soil Sorption, Cytotoxicity, and Mutagenicity of Paraquat and Enhancing its Herbicide Activity. J. Agric. Food Chem. 67 (20), 5736–5745. doi:10.1021/acs.jafc.9b01106
Rashidipour, M., Rasoulian, B., Maleki, A., Davari, B., Pajouhi, N., and Mohammadi, E. (2021). Pectin/chitosan/tripolyphosphate Encapsulation Protects the Rat Lung from Fibrosis and Apoptosis Induced by Paraquat Inhalation. Pestic. Biochem. Physiol. 178, 104919. doi:10.1016/j.pestbp.2021.104919
Rop, K., Mbui, D., Karuku, G. N., Michira, I., and Njomo, N. (2020). Characterization of Water Hyacinth Cellulose-G-Poly (Ammonium Acrylate-Co-Acrylic Acid)/nano-Hydroxyapatite Polymer Hydrogel Composite for Potential Agricultural Application. Results Chem. 2, 100020. doi:10.1016/j.rechem.2019.100020
Saleem, H., and Zaidi, S. J. (2020). Recent Developments in the Application of Nanomaterials in Agroecosystems. Nanomaterials (Basel) 10 (12), 2411. doi:10.3390/nano10122411
Sarker, N. C., Ray, P., Pfau, C., Kalavacharla, V., Hossain, K., and Quadir, M. (2020). Development of Functional Nanomaterials from Wheat Bran Derived Arabinoxylan for Nucleic Acid Delivery. J. Agric. Food Chem. 68 (15), 4367–4373. doi:10.1021/acs.jafc.0c00029
Schiavi, D., Balbi, R., Giovagnoli, S., Camaioni, E., Botticella, E., Sestili, F., et al. (2021). A Green Nanostructured Pesticide to Control Tomato Bacterial Speck Disease. Nanomaterials 11 (7), 1852. doi:10.3390/nano11071852
Shahi, N., Wang, P., Adhikari, S., Min, B., and Rangari, V. K. (2021). Biopolymers Fractionation and Synthesis of Nanocellulose/Silica Nanoparticles from Agricultural Byproducts. ACS Sustainable. Chem. Eng. 9 (18), 6284–6295. doi:10.1021/acssuschemeng.0c09342
Sharif, S. N. M., Hashim, N., Isa, I. M., Bakar, S. A., Saidin, M. I., Ahmad, M. S., et al. (2021). Polymeric Nanocomposite-Based Herbicide of Carboxymethyl Cellulose Coated-Zinc/Aluminium Layered Double Hydroxide-Quinclorac: A Controlled Release Purpose for Agrochemicals. J. Polym. Environ. 29 (6), 1817–1834. doi:10.1007/s10924-020-01997-0
Sharma, R., Bajpai, J., Bajpai, A. K., Acharya, S., Kumar, B., and Singh, R. K. (2017). Assessment of Water Retention Performance of Pectin-Based Nanocarriers for Controlled Irrigation in Agriculture. Agric. Res. 6 (2), 139–149. doi:10.1007/s40003-017-0257-7
Shatkin, J. A., and Kim, B. (2015). Cellulose Nanomaterials: Life Cycle Risk Assessment, and Environmental Health and Safety Roadmap. Environ. Sci. Nano 2 (5), 477–499. doi:10.1039/c5en00059a
Shrestha, S., Kognou, A. L. M., Zhang, J., and Qin, W. (2021). Different Facets of Lignocellulosic Biomass Including Pectin and its Perspectives. Waste Biomass Valor. 12 (9), 4805–4823. doi:10.1007/s12649-020-01305-w
Sikder, A., Pearce, A. K., Parkinson, S. J., Napier, R., and O’Reilly, R. K. (2021). Recent Trends in Advanced Polymer Materials in Agriculture Related Applications. ACS Appl. Polym. Mater. 3 (3), 1203–1217. doi:10.1021/acsapm.0c00982
Singh, H., Sharma, A., Bhardwaj, S. K., Arya, S. K., Bhardwaj, N., and Khatri, M. (2021). Recent Advances in the Applications of Nano-Agrochemicals for Sustainable Agricultural Development. Environ. Sci. Process. Impacts 23 (2), 213–239. doi:10.1039/d0em00404a
Sipponen, M. H., Rojas, O. J., Pihlajaniemi, V., Lintinen, K., and Österberg, M. (2017). Calcium Chelation of Lignin from Pulping Spent Liquor for Water-Resistant Slow-Release Urea Fertilizer Systems. ACS Sust. Chem. Eng. 5 (1), 1054–1061. doi:10.1021/acssuschemeng.6b02348
Siqueira, J. G. W., Rodrigues, C., de Souza Vandenberghe, L. P., Woiciechowski, A. L., and Soccol, C. R. (2020). Current Advances in On-Site Cellulase Production and Application on Lignocellulosic Biomass Conversion to Biofuels: a Review. Biomass and Bioenergy 132, 105419. doi:10.1016/j.biombioe.2019.105419
Su, S., Chen, L., Hao, L., Chen, H., Zhou, X., and Zhou, H. (2021a). Fluorinated Sodium Carboxymethyl Cellulose Nanoparticles as Carrier for Improving Adhesion and Sustaining Release of AVM. J. Macromolecular Sci. A 58 (4), 219–231. doi:10.1080/10601325.2020.1840922
Su, S., Chen, L., Hao, L., Chen, H., Zhou, X., and Zhou, H. (2021c). Preparation of P-Amino Salicylic Acid-Modified Polysuccinimide as Water-Based Nanocarriers for Enhancing Pesticide Stability and Insecticidal Activity. Colloids Surf. B: Biointerfaces 207, 111990. doi:10.1016/j.colsurfb.2021.111990
Su, Y., Chen, L., Yang, F., and Cheung, P. C. K. (2021). Beta-d-glucan-based Drug Delivery System and its Potential Application in Targeting Tumor Associated Macrophages. Carbohydr. Polym. 253, 117258. doi:10.1016/j.carbpol.2020.117258
Sun, H., Erdman, W., Yuan, Y., Mohamed, M. A., Xie, R., Wang, Y., et al. (2020). Crosslinked Polymer Nanocapsules for Therapeutic, Diagnostic, and Theranostic Applications. Wiley Interdiscip. Rev. Nanomed Nanobiotechnol 12 (6), e1653. doi:10.1002/wnan.1653
Tardy, B. L., Mattos, B. D., Otoni, C. G., Beaumont, M., Majoinen, J., Kämäräinen, T., et al. (2021). Deconstruction and Reassembly of Renewable Polymers and Biocolloids into Next Generation Structured Materials. Chem. Rev. 121 (22), 14088–14188. doi:10.1021/acs.chemrev.0c01333
Teo, H. L., and Wahab, R. A. (2020). Towards an Eco-Friendly Deconstruction of Agro-Industrial Biomass and Preparation of Renewable Cellulose Nanomaterials: A Review. Int. J. Biol. macromolecules 161, 1414–1430. doi:10.1016/j.ijbiomac.2020.08.076
Ur Rahim, H., Qaswar, M., Uddin, M., Giannini, C., Herrera, M. L., and Rea, G. (2021). Nano-Enable Materials Promoting Sustainability and Resilience in Modern Agriculture. Nanomaterials 11 (8), 2068. doi:10.3390/nano11082068
Usman, M., Farooq, M., Wakeel, A., Nawaz, A., Cheema, S. A., Rehman, H. U., et al. (2020). Nanotechnology in Agriculture: Current Status, Challenges and Future Opportunities. Sci. Total Environ. 721, 137778. doi:10.1016/j.scitotenv.2020.137778
Wijaya, C. J., Ismadji, S., and Gunawan, S. (2021). A Review of Lignocellulosic-Derived Nanoparticles for Drug Delivery Applications: Lignin Nanoparticles, Xylan Nanoparticles, and Cellulose Nanocrystals. Molecules 26 (3), 676. doi:10.3390/molecules26030676
Worrall, E. A., Hamid, A., Mody, K. T., Mitter, N., and Pappu, H. R. (2018). Nanotechnology for Plant Disease Management. Agronomy 8 (12), 285. doi:10.3390/agronomy8120285
Ye, L., Han, Y., Wang, X., Lu, X., Qi, X., and Yu, H. (2021). Recent Progress in Furfural Production from Hemicellulose and its Derivatives: Conversion Mechanism, Catalytic System, Solvent Selection. Mol. Catal. 515, 111899. doi:10.1016/j.mcat.2021.11189
Yearla, S. R., and Padmasree, K. (2016). Exploitation of Subabul Stem Lignin as a Matrix in Controlled Release Agrochemical Nanoformulations: a Case Study with Herbicide Diuron. Environ. Sci. Pollut. Res. Int. 23 (18), 18085–18098. doi:10.1007/s11356-016-6983-8
Yiamsawas, D., Kangwansupamonkon, W., and Kiatkamjornwong, S. (2021). Lignin-based Nanogels for the Release of Payloads in Alkaline Conditions. Eur. Polym. J. 145, 110241. doi:10.1016/j.eurpolymj.2020.110241
Yin, J. M., Wang, H. L., Yang, Z. K., Wang, J., Wang, Z., Duan, L. S., et al. (2020). Engineering Lignin Nanomicroparticles for the Antiphotolysis and Controlled Release of the Plant Growth Regulator Abscisic Acid. J. Agric. Food Chem. 68 (28), 7360–7368. doi:10.1021/acs.jafc.0c02835
Yu, M., Sun, C., Xue, Y., Liu, C., Qiu, D., Cui, B., et al. (2019). Tannic Acid-Based Nanopesticides Coating with Highly Improved Foliage Adhesion to Enhance Foliar Retention. RSC Adv. 9 (46), 27096–27104. doi:10.1021/10.1039/c9ra05843e
Zhang, D. X., Liu, G., Jing, T. F., Luo, J., Wei, G., Mu, W., et al. (2020). Lignin-Modified Electronegative Epoxy Resin Nanocarriers Effectively Deliver Pesticides against Plant Root-Knot Nematodes (Meloidogyne incognita). J. Agric. Food Chem. 68 (47), 13562–13572. doi:10.1021/acs.jafc.0c01736
Zhang, D. X., Du, J., Wang, R., Luo, J., Jing, T. F., Li, B. X., et al. (2021a). Core/Shell Dual‐Responsive Nanocarriers via Iron‐Mineralized Electrostatic Self‐Assembly for Precise Pesticide Delivery. Adv. Funct. Mater. 31, 2102027. doi:10.1002/adfm.202102027
Zhang, D. X., Wang, R., Cao, H., Luo, J., Jing, T. F., Li, B. X., et al. (2021b). Emamectin Benzoate Nanogel Suspension Constructed from Poly (Vinyl Alcohol)-Valine Derivatives and Lignosulfonate Enhanced Insecticidal Efficacy. Colloids Surf. B: Biointerfaces 209, 112166. doi:10.1016/j.colsurfb.2021.112166
Zhang, L., Peng, X., Zhong, L., Chua, W., Xiang, Z., and Sun, R. (2019). Lignocellulosic Biomass Derived Functional Materials: Synthesis and Applications in Biomedical Engineering. Curr. Med. Chem. 26 (14), 2456–2474. doi:10.2174/0929867324666170918122125
Zhang, S., Fu, X., Tong, Z., Liu, G., Meng, S., Yang, Y., et al. (2020a). Lignin–Clay Nanohybrid Biocomposite-Based Double-Layer Coating Materials for Controllable-Release Fertilizer. ACS Sust. Chem. Eng. 8 (51), 18957–18965. doi:10.1021/acssuschemeng.0c06472
Zhao, M., Zhou, H., Hao, L., Chen, H., and Zhou, X. (2021a). A High‐efficient Nano Pesticide‐fertilizer Combination Fabricated by Amino Acid‐modified Cellulose Based Carriers. Pest Manag. Sci. doi:10.1002/ps.6655
Zhao, M., Zhou, H., Hao, L., Chen, H., and Zhou, X. (2021b). Natural Rosin Modified Carboxymethyl Cellulose Delivery System with Lowered Toxicity for Long-Term Pest Control. Carbohydr. Polym. 259, 117749. doi:10.1016/j.carbpol.2021.117749
Zhi, H., Yu, M., Yao, J., Sun, C., Cui, B., Zhao, X., et al. (2020). A Facile Approach to Increasing the Foliage Retention of Pesticides Based on Coating with a Tannic acid/Fe3+ Complex. Coatings 10 (4), 359. doi:10.3390/coatings10040359
Keywords: nanopesticides, biopolymers, lignocellulosic materials, nanotechnology, nano-enabled agriculture, sustainable agriculture, circular bioeconomy
Citation: Lima PHC, Antunes DR, Forini MML, Pontes MS, Mattos BD and Grillo R (2021) Recent Advances on Lignocellulosic-Based Nanopesticides for Agricultural Applications. Front. Nanotechnol. 3:809329. doi: 10.3389/fnano.2021.809329
Received: 04 November 2021; Accepted: 22 November 2021;
Published: 17 December 2021.
Edited by:
Amitava Mukherjee, VIT University, IndiaReviewed by:
Suresh Kaushik, Indian Agricultural Research Institute (ICAR), IndiaCopyright © 2021 Lima, Antunes, Forini, Pontes, Mattos and Grillo. This is an open-access article distributed under the terms of the Creative Commons Attribution License (CC BY). The use, distribution or reproduction in other forums is permitted, provided the original author(s) and the copyright owner(s) are credited and that the original publication in this journal is cited, in accordance with accepted academic practice. No use, distribution or reproduction is permitted which does not comply with these terms.
*Correspondence: Renato Grillo, cmVuYXRvLmdyaWxsb0B1bmVzcC5icg==, cmVuYXRvLmdyaWxsb0B5bWFpbC5jb20=