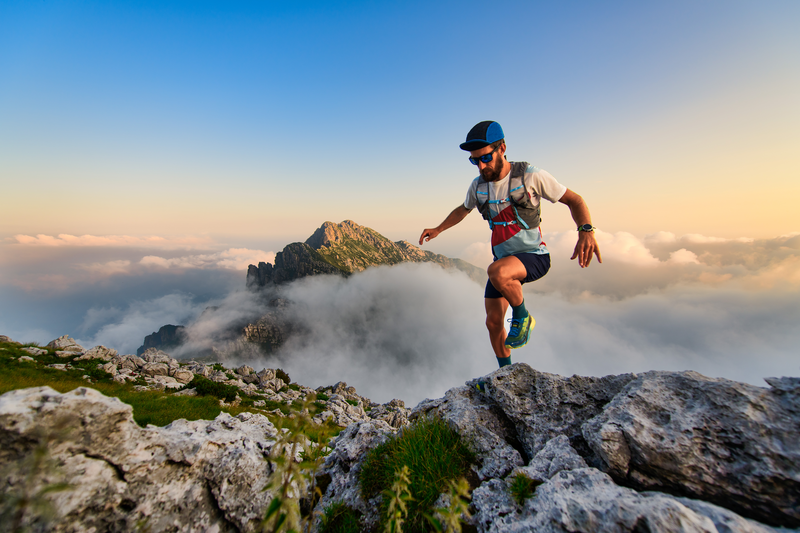
95% of researchers rate our articles as excellent or good
Learn more about the work of our research integrity team to safeguard the quality of each article we publish.
Find out more
ORIGINAL RESEARCH article
Front. Nanotechnol. , 20 May 2021
Sec. Biomedical Nanotechnology
Volume 3 - 2021 | https://doi.org/10.3389/fnano.2021.624564
This article is part of the Research Topic National Conference on Nano/Bio-Technology 2019, India View all 12 articles
Candidiasis caused by Candida albicans is one of the most common microbial infections. Azoles, polyenes, allylamines, and echinocandins are classes of antifungals used for treating Candida infections. Standard drug doses often become ineffective due to the emergence of multidrug resistance (MDR). This leads to the use of higher drug doses for prolonged duration, resulting in severe toxicity (nephrotoxicity and liver damage) in humans. However, combination therapy using very low concentrations of two or more antifungal agents together, can lower such toxicity and limit evolution of drug resistance. Herein, 4–6 nm zinc oxide quantum dots (ZnO QDs) were synthesized and their in vitro antifungal activities were assessed against drug-susceptible (G1, F1, and GU4) and resistant (G5, F5, and GU5) isolates of C. albicans. In broth microdilution assay, ZnO QDs exhibited dose dependent growth inhibition between 0 – 200 µg/ml and almost 90% growth was inhibited in all Candida strains at 200 µg/ml of ZnO QDs. Synergy between ZnO QDs and antifungal drugs at sub-inhibitory concentrations of each was assessed by checkerboard analysis and expressed in terms of the fractional inhibitory concentration (FIC) index. ZnO QDs were used with two different classes of antifungals (azoles and polyenes) against Candida isolates: combination 1 (with fluconazole); combination 2 (with ketoconazole); combination 3 (with amphotericin B), and combination 4 (with nystatin). Results demonstrated that the potency of combinations of ZnO QDs with antifungal drugs even at very low concentrations of each was higher than their individual activities against the fungal isolates. The FIC index was found to be less than 0.5 for all combinations in the checkerboard assay, which confirmed synergism between sub-inhibitory concentrations of ZnO QDs (25 µg/ml) and individual antifungal drugs. Synergism was further confirmed by spot assay where cell viabilities of Candida strains were significantly reduced in all combinations, which was clearly evident from the disappearance of fungal cells on agar plates containing antifungal combinations. For safer clinical use, the in vitro cytotoxic activity of ZnO QDs was assessed against HeLa cell line and it was found that ZnO QDs were non-toxic at 25 µg/ml. Results suggested that the combination of ZnO QDs with drugs potentiate antimicrobial activity through multitargeted action. ZnO QDs could therefore offer a versatile alternative in combination therapy against MDR fungal pathogens, wherein lowering drug concentrations could reduce toxicity and their multitargeted action could limit evolution of fungal drug resistance.
Recurrence of local and systemic fungal infections have posed a life-threatening public health problem globally for immunocompromised individuals, and treatment for fungal infections is estimated to cost around more than $7.2 billion (Benedict et al., 2019). Candida species are the most notorious, opportunistic fungal pathogens, known to cause oral and systemic candidiasis (Lamoth et al., 2018). Individuals with cancer treatment, organ transplantation surgeries, congenital immunodeficiency syndromes, acquired immunodeficiency syndrome (AIDS), and indwelling medical devices are frequently infected by Candida, resulting in morbidity and mortality due to invasive candidiasis (Prasad et al., 2011; Pal et al., 2017; Tso et al., 2018; Xiao et al., 2019). Antifungal drugs such as triazoles (fluconazole, itraconazole, and voriconazole) constitute the first line of standard treatment for candidiasis but due to their fungistatic action, fungal strains acquire resistance in due course toward the standard doses of these drugs (Watt et al., 2013). The next class of antifungal drugs comprises of polyenes (amphotericin B and nystatin) and next-generation echinocandins (caspofungin and micafungin). Although polyenes are effective fungicidal compounds, amphotericin B has toxic side effects such as renal impairment (Spampinato and Leonardi, 2013). Echinocandins are safer than amphotericin B and disrupt fungal cell wall integrity by inhibiting synthesis of β (1,3)-D-glucan (a fungal cell wall component). But echinocandins have a few drawbacks such as poor oral availability, high cost, and administration by intravenous injection only; which limit their use as standard treatment for invasive candidiasis (Spampinato and Leonardi, 2013).
Consequently, due to the limited antifungal arsenal, researchers have sought to improve treatment via different approaches. The approach of combination therapy consisting of two or more drugs in synergism has been considered as an alternative for treatment (Ahmed et al., 2014; Campitelli et al., 2017) to achieve broad spectrum antimicrobial activity and simultaneously reduce the risk of acquiring resistance during therapy. Combination therapy in place of monotherapy was therefore, introduced as a novel, safe, effective therapy, especially to target drug resistant strains and improve survival rate in high-risk life-threatening infections (Tamma et al., 2012). The objective behind this use of combination therapy was to lower the toxicity by reducing the standard administrative doses of drugs and improve the clinical efficacy of existing drugs (Campitelli et al., 2017). It is speculated that combination therapy affects multiple cellular/molecular targets in microbial pathogens, which prevents acquisition of MDR (Worthington and Melander, 2013; Campitelli et al., 2017). In combination therapy, the effect of interactions between two drugs is classified into three types: synergism, indifferent, and antagonism. The combination is called synergistic when the effect of combined drugs is greater than a single drug, indicating two different cellular targets for their action (Campitelli et al., 2017). While if the treatment shows no distinct difference, the combination is considered indifferent and if the effect is smaller than a single drug, then it is called antagonistic (Campitelli et al., 2017). Notably, the antagonistic effect might be due to the competition of both drugs for the same target, which might also be the reason for the antagonistic behavior of the amphotericin B and fluconazole combination against Cryptococcus gattii, where both compete for the same target, viz., ergosterol (Santos et al., 2012).
Use of two different classes of drugs targeting multiple components of fungal cells might be an effective strategy for treatment of fungal infections and in that context nanoparticles (NPs) might be an ideal choice for combination therapy because of their nano size, ease of surface modification, excellent antimicrobial activity, and cost-effective synthesis (Radhakrishnan et al., 2018a; Radhakrishnan et al., 2018b). Smaller, spherical NPs (within 50 nm) were highly permeable even in drug-resistant microbes which showed altered cell membrane/cell wall and overexpression of drug efflux pumps (Baptista et al., 2018). Moreover, the unique surface chemistry of NPs allows their conjugation with biomolecules for targeted drug delivery by facilitating controlled and sustained drug release (Wang et al., 2017). But toxicity due to NPs have been reported, which restricts the use of NPs as preferred antimicrobial agents for treatment. However, among various NPs, the ZnO NP, a semiconductor metal oxide, is extensively used in the medicinal industry and believed to be a biosafe, biocompatible, and non-toxic nanomaterial (Ali et al., 2018). Biocompatibility studies revealed no significant toxicity of ZnO NPs either in cell lines or during topical application for skin infection (Pati et al., 2014; Barman, 2015; Yusof et al., 2019). In addition to having excellent antimicrobial activity and preventing microbial biofilm deposition on implants, ZnO NPs also promoted proliferation of normal cells and angiogenesis (Memarzadeh et al., 2015; Oleshko et al., 2020). Hence, ZnO NPs were used for incorporation in implant and scaffold development (Pati et al., 2014; Barman, 2015; Yusof et al., 2019). Moreover, ZnO NPs displayed the potential for use as a supplement of Zn (essential micronutrient) in diet for regulation of innate and adaptive immune responses, enhancement of growth and development, prevention of local and systemic candidiasis, etc. (Swain et al., 2016; Yusof et al., 2019).
ZnO NPs mediate broad spectrum antimicrobial activity through targeting multiple cellular and molecular mechanisms, which might prevent further acquisition of fungal drug resistance (Sirelkhatim et al., 2015). Therefore, it is likely that ZnO NPs in combination with other antifungal drugs might lead to a reduction in standard doses of antifungals, cost of treatment, and drug toxicity (Benedict et al., 2019). Furthermore, the size-dependent microbial toxicity of ZnO NPs was widely documented, wherein reduction in size increased antimicrobial activity (Preeti et al., 2020a; Jin et al., 2009; Joshi et al., 2009; Palanikumar et al., 2014; Sirelkhatim et al., 2015; da silva et al., 2019.
Spherical nanoparticles (commonly within 10 nm) with size comparable to or less than the Bohr radius of the material, where quantum confinement effects are prominent, are known as “quantum dots” (QDs) (Poulopoulos et al., 2012; Samanta et al., 2018). The quantum confinement in QDs create surface defects and produce active sites, which react with oxygen and hydroxyl ions to form highly reactive hydroxyl and superoxide radicals (Mudunkotuwa et al., 2012; Siddiqi et al., 2018). The smaller size in case of ZnO QDs facilitate higher dissolution of Zn2+ ions in solution, which could be responsible for the augmentation of reactive oxygen species (ROS) and thus resulting in peroxidation of biomolecules and cell death in microbial pathogens (Joshi et al., 2009; Asok et al., 2015). A recent study reported broad spectrum microbicidal activity of ZnO QDs (5–6 nm) against MDR pathogens (E. coli and C. albicans), implying that antimicrobial activity of nano ZnO can be tuned by controlling the size (Preeti et al., 2020b). Antimicrobial activity of ZnO QDs has been reported singly against various microbial pathogens. However, in this study, we have demonstrated the use of ZnO QDs in combination with antifungal drugs of different classes against drug-susceptible and drug-resistant Candida albicans strains, in order to develop a biosafe, nano-based versatile alternative of combination therapy against MDR fungal pathogens, wherein drug concentrations can be lowered to reduce toxicity with the simultaneous advantage of multitargeted action to limit evolution of fungal drug resistance.
Yeast extract, peptone, dextrose, and agar for fungal cell culture were purchased from Fisher Scientific (India) and Hi-Media (India). Absolute ethanol was obtained from Alfa Aesar (MA, United States) and hexane from Merck (NJ, United States). Zinc acetate dihydrate, lithium hydroxide monohydrate, standard antifungal drugs (fluconazole, ketoconazole, amphotericin B, and nystatin), dimethyl sulfoxide (DMSO) solvent, and cell culture media DMEM (Dulbecco’s Modified Eagle’s medium) were procured from Sigma Aldrich (United States). Penicillin-streptomycin antibiotic mix and fetal bovine serum (FBS) were obtained from Fisher Scientific (India) and trypan blue was obtained from Hi-Media (India).
Three isogenic matched pairs of Candida albicans (C. albicans) strains (G1 and G5, F1 and F5, and GU4 and GU5) were used in this study. These strains were provided as a kind gift by Joachim Morschhäuser, University of Würzburg, Germany. The strain description is given in Table 1. These strains were originally isolated after regular intervals from three AIDS patients suffering from oropharyngeal candidiasis (OPC). During the course of azole therapy administered to these OPC patients, drug-susceptible G1, F1, and GU4 evolved after several months to highly drug-resistant G5, F5, and GU5, respectively due to acquisition of MDR (Franz et al., 1998; Franz et al., 1999).
The three isogenic matched pairs of C. albicans strains (G1 and G5, F1 and F5, and GU4 and GU5) were grown on (1% (w/v) yeast extract, 2% (w/v) peptone, and 2% (w/v) dextrose) (YEPD) media at 30°C for 14–16 h under continuous shaking at 140–150 rpm. Exponentially grown log phase microbial cells were used for all experiments. The cells were stored at 4°C on YEPD plates containing 2.5% agar.
Two different classes of standard antifungal drugs: azoles (fluconazole and ketoconazole) and polyenes (amphotericin B and nystatin) and ZnO QDs were used as test compounds. The stock solutions (2 mg/ml) of fluconazole were prepared in deionized (milli Q) water, while ketoconazole, amphotericin B, and nystatin were dissolved in DMSO. The stock solution (4 mg/ml) of ZnO QDs was prepared in deionized water (milli Q) and sonicated for 1 h before use.
Human cervical cancer cell line, HeLa was obtained from the American Type Culture Collection (Manassas, VA) and grown in DMEM media supplemented with 10% heat-inactivated FBS and 1% penicillin-streptomycin. Further, the cell line was incubated and maintained at 37°C under a humidified condition with 5% CO2 (Gurung et al., 2019).
ZnO QDs were synthesized using zinc acetate dihydrate as the precursor, as described earlier (Meulenkamp, 1999) with slight modifications. Briefly, the ethanolic solution of zinc acetate dihydrate (0.1 M) was prepared by refluxing the solution for 30 min. After that, the ethanolic solution of lithium hydroxide monohydrate (0.14 M) was added drop-wise on zinc acetate dihydrate solution kept under vigorous stirring for 1 h. Subsequently, ZnO QDs were precipitated by adding n-hexane and collected by centrifugation. After drying at 60°C under vacuum, ZnO QDs were obtained as white powder and their structural, optical, and functional properties were characterized using the following techniques.
The optical property of ZnO QDs was characterized using a UV-Vis spectrophotometer (Perkin Elmer, UV-Vis Spectrophotometer Lambda 35, Singapore). For structural characterization, X-ray diffraction (XRD) pattern was recorded in the scan range of 20°–80° diffraction angle and a step size of 0.016° using an X-ray powder diffractometer with Cu Kα radiation (λ = 1.5418Å) (PANalytical X’Pert PRO diffractometer, Almelo Netherlands). The shape and size of ZnO QDs were analyzed by a transmission electron microscope (TEM) (JEM 2100F; JEOL, Tokyo, Japan) using Image Tool software (multipoint image database software for grain and particle analysis; Dietermann and Heuser Solution GmbH, Greifenstein, Germany). High resolution TEM (HRTEM) analysis was done to visualize planar lattice spacing (d spacing) and electron diffraction pattern. The elemental composition of ZnO QDs was determined by EDX-mapping and spectra was obtained from TEM equipped with an energy-dispersive X-ray (EDX) spectrometer (EDS; Bruker Nano GmbH, Berlin, Germany).
In vitro antifungal activity of ZnO QDs against isogenic matched pairs of C. albicans strains (F1 and F5, G1 and G5, and GU4 and GU5) was assessed by the broth microdilution method, as described by the standard protocol of the Clinical and Laboratory Standards Institute (CLSI) (Clinical and Laboratory Standards Institute, 2008; Prasad et al., 2005; Preeti et al., 2020a; Radhakrishnan et al., 2018a; Radhakrishnan et al., 2018b). The broth microdilution assay was performed in 96-well microtiter plates in triplicate. Candida strains were grown overnight, then resuspended in 0.9% saline in order to achieve an optical density (OD) of 0.1 at 600 nm, which corresponded to 0.5–1 x 106 CFU/ml. These cells were further diluted 100-fold in YEPD media to attain a final concentration of 104 CFU/ml. ZnO QDs ranging in concentration between 0–200 μg/ml were added to the YEPD media in plates containing cells of respective strains and incubated at 30°C for 48 h. Respective growth controls for the fungal strains were maintained without ZnO QDs. Minimum inhibitory concentration (MIC) was determined by measuring the OD at 600 nm at the lowest concentration of ZnO QDs that completely inhibited the growth of the fungal cells, and the percentage of growth inhibition was calculated from the differences in OD between the cells grown in the presence and absence of ZnO QDs.
The synergy between ZnO QDs and antifungal drugs was determined by calculating the fractional inhibitory concentration (FIC) index in a checkerboard assay. The individual potency of four different combinations comprising of ZnO QDs and antifungal drugs (fluconazole, ketoconazole, amphotericin B, and nystatin), where each was used at very low concentrations, was assessed by comparing with their individual activities against isogenic matched pairs of C. albicans strains (G1 and G5, F1 and F5, and GU4 and GU5).
FIC index was determined by a two-dimensional broth microdilution checkerboard assay, as described in the Clinical Microbiology Procedures Handbook (Moody, 2004). The assay was performed in 96-microtiter plates, where YEPD media was added aseptically in all wells and the concentration range was between 0.178–200 µg/ml for ZnO QDs, 0.125–64 µg/ml for fluconazole, and 0.015–8 µg/ml each for ketoconazole, amphotericin B, and nystatin. In each plate, the antifungal drug was serially diluted along the abscissa, while ZnO QDs were serially diluted along the ordinate. Subsequently, inoculum of 104 CFU/ml of each Candida strain was prepared separately in YEPD media. Then, 100 µl of the respective cell suspension was added into each well of the 96-well plates containing test compounds and incubated for 48 h at 30°C. The MICs of each antifungal drug alone, ZnO QDs alone, and their combinations were determined both visually and by measuring the ODs of cells at 600 nm using a microtiter plate reader. The inhibition in growth of fungal cells was calculated with respect to the growth control (absence of both drugs and ZnO QDs).
The ΣFIC index was calculated as follows:
Here
Where
A is the individual antifungal drug (fluconazole, ketoconazole, amphotericin B, and nystatin) and B is ZnO QDs.
Interpretation of interactions in combinations of A (antifungal drug) and B (ZnO QDs) is as follows:
When the FIC index is ≤0.5, the combination is synergistic, at >0.5 to <4.0, the combination is indifferent, and at ≥4.0, the combination is antagonistic.
A spot assay was performed to confirm the synergism between ZnO QDs and the four different antifungal drugs (fluconazole, ketoconazole, amphotericin B, and nystatin) against isogenic matched pairs (G1 and G5, F1 and F5, and GU4 and GU5) of C. albicans (Mukhopadhyay et al., 2004; Prasad et al., 2005; Preeti et al., 2020b; Radhakrishnan et al., 2018a; Radhakrishnan et al., 2018b). In the spot assay, separate YEPD plates were prepared by adding ZnO QDs alone, antifungal drugs alone, and four combinations containing both ZnO QDs and individual drugs (fluconazole, ketoconazole, amphotericin B and nystatin). Overnight grown log phase cells of each strain were separately resuspended in 0.9% saline and OD of each cell suspension at 600 nm was adjusted to 0.1. Subsequently, CFU/ml was calculated and 5-fold serial dilutions containing 1 × 106 cells/ml cells were prepared. A total of 5 μl of each serially diluted cell suspension was spotted onto each YEPD plate containing ZnO QDs alone, antifungal drugs alone, and four combinations containing both ZnO QDs and individual drugs. The plates were incubated for 48 h at 30°C. A separate growth control plate was also maintained without ZnO QDs and drugs. The images were then recorded and growth differences were evaluated by comparison with the growth control.
The in vitro cytotoxic activity of ZnO QDs was evaluated on HeLa cell line using a trypan blue exclusion assay (Gurung et al., 2019). Approximately 4 × 104 cells were plated in a culture dish containing DMEM media and allowed to adhere for 12 h, then ZnO QDs (0 and 25 µg/ml) were added and incubated for 48 h in a humidified incubator (5% CO2) at 37°C. After that, cells were trypsinized, harvested, and resuspended in equal volumes of phosphate buffer saline (PBS) and 0.4% trypan blue for cell counting. Thereafter, viable (unstained) and non-viable (blue-stained) cells were counted using a hemocytometer to find out the total numbers of living and dead cells after treatment with ZnO QDs.
All experiments were performed at least three times and results are represented as the mean ± standard deviation (SD). A one-way ANOVA test was performed using GraphPad Prism version 8 (GraphPad Software, San Diego, CA) for validating the reproducibility and significance of results. The difference between the means was considered statistically significant at *p ≤ 0.05.
Figure 1A shows the typical absorption peak of ZnO QDs at 365 nm (corresponding to band gap of 3.4 eV), indicating the formation of ZnO QDs. Figure 1B represents the X-ray diffraction (XRD) peaks at 31.89°, 35.45°, 36.46°, 47.82°, 56.77°, 62.90°, and 68.40°. XRD confirmed a single phase hexagonal wurtzite structure, which was in agreement with JCPDS (Joint Committee on Powder Diffraction Standards), file no. 36–1,451. Furthermore, the TEM image (Figure 2A) revealed the average size of ZnO QDs to be ∼ 4–6 nm (Figure 2B), while HRTEM confirmed the d spacing as 0.201 nm between the atomic planes of ZnO QDs (Figure 2C), which was in accordance with XRD data. The corresponding selected area electron diffraction (SAED) pattern confirmed the crystalline nature of ZnO QDs and the obtained rings matched with the diffraction planes of the XRD spectra (Figure 2D). TEM-EDX mapping revealed the presence of only zinc and oxygen elements in ZnO QDs (Figure 2E), while EDX spectra displayed typical sharp peaks of zinc around 1 and 8.6 keV and a single peak of oxygen at 0.5 keV, indicating no trace of impurity in the sample (Figure 2F).
FIGURE 1. (A) Absorbance spectrum of ZnO QDs and absorbance maxima observed at 365 nm; (B) X-ray diffraction of ZnO QDs.
FIGURE 2. (A) TEM image showing spherical ZnO QDs; (B) histogram depicting mean size (4–6 nm) of ZnO QDs; (C) high-resolution TEM image of ZnO QDs, where lattice spacing d = 0.201 nm (d represents the distance between two lattice fringes); (D) selected area electron diffraction (SAED) pattern of ZnO QDs; (E) TEM-EDX mapping of ZnO QDs; (F) TEM-EDX spectra of ZnO QDs with strong peaks at 0.5, 1.0, and 8.6 keV.
Severe fungal infections occur as a consequence of several health problems in immunocompromised cases due to acquired immunodeficiency syndrome (AIDS), organ transplantation, chemotherapy, cancer, asthma, corticosteroid therapies, etc. Rampant use of antifungals in immunocompromised individuals have led to increasing incidence of fungal drug resistance. The pathogenesis and severity of fungal infection depend on various immunological and non-immunological factors. C. albicans is the most common, opportunistic fungal pathogen and forms a part of human microbiota, however, its pathogenesis depends on the immunity of the individual. Since the last few decades, several MDR strains of Candida spp. causing severe invasive candidiasis have emerged (Franz et al., 1998; Franz et al., 1999; Colombo et al., 2017). A study of Candida species in patients with AIDS showed 33% of late-stage patients with drug-resistant strains of Candida albicans in their oral cavities (White et al., 1998). Oropharyngeal candidiasis (OPC) is one of the most prevalent fungal infections occurring in HIV patients (occurrence range between 0.9 and 83%). Drug resistance in C. albicans is mainly responsible for failure of antifungal therapy when administered in standard doses (Patil et al., 2018). Interestingly, Franz et al. studied the mechanisms for acquired drug resistance in isogenic matched pairs of C. albicans strains (isolated from three AIDS patients, who were suffering from OPC and received azole standard therapy) and found that during the course of treatment, the drug-susceptible strains (G1, F1, and GU4) acquired drug resistance and evolved into drug-resistant G5, F5, and GU5. The proposed primary mechanisms for resistance toward azoles and polyenes include ergosterol gene mutation, alterations in membrane permeability, and efflux pump overexpression, which result in altered drug target (ergosterol), reduced drug uptake, and increased efflux of drugs from the cells (Franz et al., 1998; Franz et al., 1999; Srivastava et al., 2018).
In order to improve the treatment outcome of microbial infections, nano-based drug compositions might be ideal candidates to address the challenges of microbial drug resistance by favoring multitargeted action, broad spectrum activity, and lower host toxicity (Radhakrishnan et al., 2018a; Radhakrishnan et al., 2018b). In addition to standard susceptibility testing and appropriate drug dosing, one of the ways to prevent drug resistance is the use of combination antifungal therapy. Therefore, in order to develop a nano-based therapeutic agent, we first investigated the antimicrobial activity of ZnO QDs against three isogenic matched pairs of C. albicans strains (drug-susceptible G1, F1, GU4 and drug-resistant G5, F5, and GU5) using a broth microdilution assay. Subsequently, synergy of ZnO QDs in different combinations with four antifungal drugs was assessed against the C. albicans strains using a checkerboard assay. Individual potency of these four different combinations of ZnO QDs and antifungal drugs (fluconazole, ketoconazole, amphotericin B, and nystatin), which were used at very low concentrations of each, was assessed by comparing their individual activities against the isogenic matched pairs of C. albicans strains.
The broth microdilution assay was performed in the presence of different concentrations of ZnO QDs between 0–200 μg/ml. Results demonstrated that ZnO QDs exhibited dose-dependent growth inhibition of all C. albicans strains (G1 and G5, F1 and F5, and GU4 and GU5) (Figure 3A). The maximum growth of cells was observed in control (without ZnO QDs), and growth of cells gradually decreased with increasing concentration of ZnO QDs (25–200 μg/ml) (Figure 3A). Further, the percentage of growth inhibition was calculated from the differences in OD at 600 nm between the fungal strains grown in the absence and presence of different concentrations of ZnO QDs. Interestingly, our results revealed almost 90, 90, 87, 85, 75, and 90% growth inhibition, respectively for the strains G1, G5, F1, F5, GU4, and GU5 at 200 μg/ml, however, 25–100 μg/ml of ZnO QDs showed growth inhibition between 30 and 50% for the strains (Figure 3B).
FIGURE 3. Determination of dose-dependent antimicrobial activity of ZnO QDs against C. albicans strains (isogenic matched pairs G1 and G5, F1 and F5, and GU4 and GU5): (A) Determination of growth by measurement of OD600nm using the broth microdilution method for C. albicans cells grown at 30°C in the presence of 0, 25, 50, 100, and 200 µg/ml of ZnO QDs, respectively. The values given are mean ± SD of three independent sets of experiments. **** represents p < 0.0001, calculated with respect to growth control (absence of ZnO QDs); (B) Percentage of growth inhibition of C. albicans strains (isogenic matched pairs) in the presence of ZnO QDs (0, 25, 50, 100, and 200 µg/ml). The values given are mean ± SD of three independent sets of experiments. **** represents p < 0.0001, calculated with respect to growth control (absence of ZnO QDs).
Respective growth controls for the fungal strains were maintained without ZnO QDs.
The effect of interaction of drugs in combination is assessed by FIC index in the checkerboard assay (Doern, 2014). In this study, synergy between ZnO QDs and different antifungal drugs against C. albicans strains (G1 and G5, F1 and F5, and GU4 and GU5) was assessed by checkerboard assay and expressed in terms of FIC index. Firstly, MIC was determined for each drug (fluconazole, ketoconazole, amphotericin B, and nystatin) against C. albicans strains (G1 and G5, F1 and F5, and GU4 and GU5). Table 2 lists the MIC for each drug against respective Candida strains. Subsequently, based on MIC obtained for ZnO QDs and each antifungal drug, singly and in combination, the FIC index for each combination was calculated as given in the Material and Methods section. Results demonstrated that the potency of combinations of ZnO QDs and antifungal drugs even at very low concentrations of each was higher than their individual activities against the fungal isolates. FIC index obtained in the checkerboard assay was less than 0.5 for all combinations tested (ZnO QDs + fluconazole; ZnO QDs + ketoconazole; ZnO QDs + amphotericin B and ZnO QDs + nystatin), which confirmed synergism between sub-inhibitory concentrations of both ZnO QDs (25 µg/ml) and individual antifungal drugs (Table 3). ZnO QDs and fluconazole exhibited synergism in combination, at a concentration of fluconazole which was 2 to 8-fold lower than the respective MIC values for fluconazole alone against the fungal strains tested (Figure 4A). Similarly, ZnO QDs displayed good synergistic activity in combination with very low concentrations of 0.015 µg/ml of ketoconazole, 0.4 µg/ml of amphotericin B, and 0.5 µg/ml of nystatin, and the concentration of each antifungal drug used in combination was much lower than the respective individual MIC values obtained for each drug against Candida strains (Figures 4B–D). Moreover, an increase in growth inhibition by 10–20% was observed in all strains for the four combinations of ZnO QDs and antifungal drugs, as compared to the strains grown in the presence of either individual drugs or only ZnO QDs (Figure 4).
TABLE 3. FIC index for different combinations of ZnO QDs and antifungal drugs tested against C. albicans strains.
FIGURE 4. | Percentage of growth inhibition in C. albicans strains (isogenic matched pairs G1 and G5, F1 and F5, and GU4 and GU5) in the presence of both drugs and ZnO QDs as compared to growth control (absence of drugs and ZnO QDs): (A) Fluconazole + ZnO QDs; (B) Ketoconazole + ZnO QDs; (C) Amphotericin B + ZnO QDs; (D) Nystatin + ZnO QDs. The values are mean ± SD of three independent sets of experiments. **** represents p < 0.0001, *** represents p < 0.0002, ** represents p < 0.0046, * represents p < 0.0408, calculated with respect to growth control.
Synergism observed in combinations indicates that ZnO QDs and individual drugs (fluconazole, ketoconazole, amphotericin B, and nystatin) probably share a non-competitive cellular target, which potentiates the antifungal activity against both drug-susceptible (G1, G5, and F1) and resistant (G5, F5, and GU5) strains, even at concentrations much lower than their respective individual MICs. However, the FIC index for the checkerboard assay has certain limitations since it only tests antimicrobials for a fixed incubation time rather than a continuous time frame and is also not capable of testing more than two antimicrobials at a time (combinations of three and four antimicrobials cannot be tested), which can slow down the assessment of potential synergistic combinations appropriately (Rybak and McGrath, 1996; Doern, 2014). Therefore, spot assay was further performed in this study to validate the results.
The spot assay (Figure 5) further confirmed the synergism exhibited between ZnO QDs and antifungal drugs in combinations (Table 4). As compared to growth control (without ZnO QDs or drugs) in Figure 5A, a slight growth inhibition and reduction in fungal colonies was observed for all strains in the presence of sub-inhibitory concentration of ZnO QDs (Figure 5B) and individual antifungals drugs (Figure 5C). However, this reduction in cell viabilities was further increased for all C. albicans strains in the presence of different combinations of ZnO QDs and antifungal drugs, which was clearly evident from the disappearance of fungal cells on agar plates (Figure 5D).
FIGURE 5. Spot assay for C. albicans clinical isolates (G1 and G5, F1 and F5, and GU4 and GU5) in the presence of: (A) Growth control (absence of both ZnO QDs and drugs); (B) ZnO QDs (25 µg/ml) only; (C) drug alone: fluconazole (2 µg/ml), ketoconazole (0.015 µg/ml), amphotericin B (0.4 µg/ml), and nystatin (0.5 µg/ml); (D) different combinations containing both ZnO QDs (25 µg/ml) and antifungal drugs [(fluconazole (2 µg/ml) or ketoconazole (0.015 µg/ml) or amphotericin B (0.4 µg/ml) or nystatin (0.5 µg/ml)].
TABLE 4. Concentrations of ZnO QDs and antifungal drugs used in different combinations for spot assays.
The FIC index (Table 3) and spot assay (Figure 5) results indicate that ZnO QDs might be a potential adjuvant for antifungal combination therapy. The standard antifungal classes of drugs, i.e., azoles and polyenes act by inhibiting ergosterol biosynthesis and targeting the ergosterol moiety, respectively in the fungal membrane, whereas multiple mechanisms of action are proposed for ZnO QDs such as high penetrability, greater retention, generation of oxidative stress, etc. When combined together, ZnO QDs and antifungal drugs might have exerted a concerted impact and were able to counter MDR in drug-resistant Candida strains (G5, F5, and GU5) (Asok et al., 2015; Sirelkhatim et al., 2015; da Silva et al., 2019; Gold et al., 2018; Tiwari et al., 2018).
This study demonstrates the promising potential of ZnO QDs for both single and combination antifungal therapy against MDR fungal pathogens. Since, ZnO QDs in combination with respective antifungal drugs exhibit synergism and can potentiate antifungal activity even at very low concentrations, use of ZnO QDs in combination therapy can reduce the toxicity of antifungal compounds. Additionally, simultaneous multitargeted action in combination therapy can prevent the development of fungal drug resistance.
However, for safer clinical use of ZnO QDs, we further investigated the in vitro cytotoxic activity of ZnO QDs against the HeLa cell line.
The in vitro cytotoxic activity of ZnO QDs (0, 5, and 25 μg/ml) was assessed by trypan blue assay in HeLa cell line and expressed as the percentage of viable cells after treatment with ZnO QDs, as compared to control cells without any treatment. Almost 93 and 86% cell viability was observed in the presence of 5 and 25 μg/ml of ZnO QDs, respectively, indicating that there was negligible toxicity to mammalian cells at 25 μg/ml of ZnO QDs (Figure 6) and hence, ZnO QDs at this concentration exhibited synergism with antifungal drugs and therefore, may be suitable for combination therapy.
FIGURE 6. In vitro cytotoxic activity of ZnO QDs against HeLa cell line. The values are mean ± SD of three independent sets of experiments. ** represents p < 0.001, * represents p < 0.01, calculated with respect to growth control.
Monotherapy against microbial infections often allow for the emergence of MDR in microbes with time and makes the standard drug doses ineffective leading to failure in treatment. Additionally, the high rates of morbidity and mortality caused by fungal infections are associated with availability of the limited antifungal arsenal and high toxicity of the compounds. Because of many similarities between fungal and human cells, identifying novel drug targets remains challenging. Owing to the limited availability of antifungal compounds, researchers have sought to improve treatment via different approaches. Therefore, to overcome these problems, combination therapy is considered for treatment (Ahmed et al., 2014; Campitelli et al., 2017). The combination therapy approach, however, is likely to offer a broader antimicrobial spectrum, lower toxicity of the antimicrobial compounds due to synergism at much lower concentrations in combination, and reduce the risk of acquiring resistance during therapy. Herein, we report synergism between sub-inhibitory concentrations of both ZnO QDs and individual antifungal drugs against all drug-susceptible and drug-resistant isolates of C. albicans tested. ZnO QDs thus appear to be a potential adjuvant in combination antifungal therapy against MDR fungal pathogens (Scheme 1), wherein drug toxicity can be reduced and their simultaneous multitargeted synergistic action can limit development of fungal drug resistance.
The raw data supporting the conclusions of this article will be made available by the authors, without undue reservation.
The Candida albicans clinical isolates were kind gifts from Joachim Morschhauser, University of Wurzburg, Germany. These isolates have been extensively studied and their molecular characterization has been reported by various researchers all over the world, which is also mentioned in the materials and methods section of this study. The present study only involved C. albicans strains and did not require any samples from patients or involvement of any animal or human subject in the study. Therefore, no ethical approval was required.
PC, SPS, and TP contributed to the concept and design of the study. PC conducted all experiments. PC and SK performed cytotoxicity assay on HeLa cells. PC and TP participated in the acquisition of data and statistical analysis. PC, NM, SPS, and TP participated in interpretation of data and drafting of the manuscript. SPS and TP contributed to editing the manuscript and revised it critically for significant intellectual content. All authors read and approved the final manuscript.
This work has been supported by grants to TP from Department of Biotechnology (BT/PR5110/MED/29/497/2012 and BT/BI/12/045/2008), JNU-UPOE II scheme (ID-161) and JNU-DST-PURSE Phase-II, India. Preeti gratefully acknowledges Council of Scientific and Industrial Research (CSIR), India for the award of Senior Research Fellowship.
The authors declare that the research was conducted in the absence of any commercial or financial relationships that could be construed as a potential conflict of interest.
We thank Joachim Morschhauser, University of Wurzburg, Germany for generously providing clinical isogenic isolates of Candida albicans. SPS acknowledges CSIR-NPL for providing the nanoparticle synthesis and characterization infrastructure.
The Supplementary Material for this article can be found online at: https://www.frontiersin.org/articles/10.3389/fnano.2021.624564/full#supplementary-material
Ahmed, A., Azim, A., Gurjar, M., and Baronia, A. K. (2014). Current Concepts in Combination Antibiotic Therapy for Critically Ill Patients. Indian J. Crit. Care Med. 18, 310–314. doi:10.4103/0972-5229.132495
Ali, A., Phull, A.-R., and Zia, M. (2018). Elemental Zinc to Zinc Nanoparticles: Is ZnO NPs Crucial for Life? Synthesis, Toxicological, and Environmental Concerns. Nanotechnol. Rev. 7, 413–441. doi:10.1515/ntrev-2018-0067
Asok, A., Ghosh, S., More, P. A., Chopade, B. A., Gandhi, M. N., and Kulkarni, A. R. (2015). Surface Defect Rich ZnO Quantum Dots as Antioxidants Inhibiting α-amylase and α-glucosidase: a Potential Anti-diabetic Nanomedicine. J. Mater. Chem. B 3, 4597–4606. doi:10.1039/c5tb00407a
Baptista, P. V., McCusker, M. P., Carvalho, A., Ferreira, D. A., Mohan, N. M., Martins, M., et al. (2018). Nano-Strategies to Fight Multidrug Resistant Bacteria-“A Battle of the Titans”. Front. Microbiol. 9, 1441. doi:10.3389/fmicb.2018.01441
Barman, A. (2015). “Review on Biocompatibility of ZnO Nano Particles,” in Advancements of Medical Electronics. Lecture Notes in Bioengineering. Editors S. Gupta, S. Bag, K. Ganguly, I. Sarkar, and P. Biswas (New Delhi: Springer), 343–352. doi:10.1007/978-81-322-2256-9_32
Benedict, K., Jackson, B. R., Chiller, T., and Beer, K. D. (2019). Estimation of Direct Healthcare Costs of Fungal Diseases in the United States. Clin. Infect. Dis. 68 (11), 1791–1797. doi:10.1093/cid/ciy776
Campitelli, M., Zeineddine, N., Samaha, G., and Maslak, S. (2017). Combination Antifungal Therapy: a Review of Current Data. J. Clin. Med. Res. 9, 451–456. doi:10.14740/jocmr2992w
Clinical and Laboratory Standards Institute (2008). Reference Method for Broth Dilution Antifungal Susceptibility Testing of Filamentous Fungi; Approved Standard-Second Edition. CLSI Document M38-A2. Wayne: Clinical and Laboratory Standards InstituteAvailable at: https://clsi.org/media/1455/m38a2_sample.pdf
Colombo, A. L., de Almeida Júnior, J. N., Slavin, M. A., Chen, S. C., and Sorrell, T. C. (2017). Candida and Invasive Mould Diseases in Non-Neutropenic Critically Ill Patients and Patients with Haematological Cancer. Lancet Infect. Dis. 17, e344–e356. doi:10.1016/S1473-3099(17)30304-3
da Silva, B. L., Caetano, B. L., Chiari-Andréo, B. G., Pietro, R. C. L. R., and Chiavacci, L. A. (2019). Increased Antibacterial Activity of ZnO Nanoparticles: Influence of Size and Surface Modification. Colloids Surf.B Biointerfaces 177, 440–447. doi:10.1016/j.colsurfb.2019.02.013
Doern, C. D. (2014). When Does 2 Plus 2 Equal 5? A Review of Antimicrobial Synergy Testing. J. Clin. Microbiol. 52, 4124–4128. doi:10.1128/JCM.01121-14
Franz, R., Kelly, S. L., Lamb, D. C., Kelly, D. E., Ruhnke, M., and Morschhäuser, J. (1998). Multiple Molecular Mechanisms Contribute to a Stepwise Development of Fluconazole Resistance in Clinical C. albicans Strains. Antimicrob. Agents Chemother. 42, 3065–3072. doi:10.1128/AAC.42.12.3065
Franz, R., Ruhnke, M., and Morschhauser, J. (1999). Molecular Aspects of Fluconazole Resistance Development in Candida albicans. Mycoses 42, 453–458. doi:10.1046/j.1439-0507.1999.00498.x
Gold, K., Slay, B., Knackstedt, M., and Gaharwar, A. K. (2018). Antimicrobial Activity of Metal and Metal-Oxide Based Nanoparticles. Adv. Therap. 1, 1700033. doi:10.1002/adtp.201700033
Gurung, S. K., Dana, S., Mandal, K., Mukhopadhyay, P., and Mondal, N. (2019). Downregulation of C-Myc and p21 Expression and Induction of S Phase Arrest by Naphthalene Diimide Derivative in Gastric Adenocarcinoma Cells. Chemico-Biol. Inter. 304, 106–123. doi:10.1016/j.cbi.2019.02.010
Husen, A., Mahmud, S., Seeni, A., Kaus, N. H. M., Ann, L. C., Bakhori, S. K. M., et al. (2015). Review on Zinc Oxide Nanoparticles: Antibacterial Activity and Toxicity Mechanism. Nano-Micro Lett. 7(3), 219–242. doi:10.1007/s40820-015-0040-x
Jin, T., Sun, D., Su, J. Y., Zhang, H., and Sue, H.-J. (2009). Antimicrobial efficacy of Zinc Oxide quantum dots against Listeria monocytogenes, Salmonella enteritidis, and Escherichia coli O157: H7. J. Food Sci. 74 (1), M46–M52. doi:10.1111/j.1750-3841.2008.01013.x
Joshi, P., Chakraborti, S., Chakrabarti, P., Haranath, D., Shanker, V., Ansari, Z. A., et al. (2009). Role of Surface Adsorbed Anionic Species in Antibacterial Activity of ZnO Quantum Dots against Escherichia coli. J. Nanosci. Nanotech. 9 (11), 6427–6433. doi:10.1166/jnn.2009.1584
Lamoth, F., Lockhart, S. R., Berkow, E. L., and Calandra, T. (2018). Changes in the Epidemiological Landscape of Invasive Candidiasis. J. Antimicrob. Chemother. 73, i4–i13. doi:10.1093/jac/dkx444
Memarzadeh, K., Sharili, A. S., Huang, J., Rawlinson, S. C. F., and Allaker, R. P. (2015). Nanoparticulate Zinc Oxide as a Coating Material for Orthopedic and Dental Implants. J. Biomed. Mater. Res. 103, 981–989. doi:10.1002/jbm.a.35241
Meulenkamp, E. A. (1999). Electron Transport in Nanoparticulate ZnO Films. J. Phys. Chem. B 103 (37), 7831–7838. doi:10.1021/jp9914673
Moody, J. A. (2004). Synergism Testing: Broth Microdilution Checkboard and Broth Macrodilution Methods in Clinical Microbiology Procedures Handbook. Editor H. D. Isenberg (Washington, DC: ASM Press), 1–28. doi:10.1128/9781555818814.ch5.16
Mudunkotuwa, I. A., Rupasinghe, T., Wu, C.-M., and Grassian, V. H. (2012). Dissolution of ZnO Nanoparticles at Circumneutral pH: A Study of Size Effects in the Presence and Absence of Citric Acid. Langmuir 28 (1), 396–403. doi:10.1021/la203542x
Mukhopadhyay, K., Prasad, T., Saini, P., Pucadyil, T. J., Chattopadhyay, A., and Prasad, R. (2004). Membrane Sphingolipid-Ergosterol Interactions Are Important Determinants of Multidrug Resistance in Candida albicans. Antimicrob. Agents Chemother. 48, 1778–1787. doi:10.1128/aac.48.5.1778-1787.2004
Oleshko, O., Husak, Y., Korniienko, V., Pshenychnyi, R., Varava, Y., Kalinkevich, O., et al. (2020). Biocompatibility and antibacterial properties of ZnO-incorporated anodic oxide coatings on TiZrNb Alloy. Nanomaterials 10, 2401. doi:10.3390/nano10122401
Pal, M. (2017). Morbidity and Mortality Due to Fungal Infections. J. Appl. Microbiol. Biochem. 1, 1–3. doi:10.21767/2576-1412.100002
Palanikumar, L., Ramasamy, S. N., and Balachandran, C. (2014). Size‐dependent Antimicrobial Response of Zinc Oxide Nanoparticles. IET Nanobiotechnol. 8 (2), 111–117. doi:10.1049/iet-nbt.2012.0008
Pati, R., Mehta, R. K., Mohanty, S., Padhi, A., Sengupta, M., Vaseeharan, B., et al. (2014). Topical Application of Zinc Oxide Nanoparticles Reduces Bacterial Skin Infection in Mice and Exhibits Antibacterial Activity by Inducing Oxidative Stress Response and Cell Membrane Disintegration in Macrophages. Nanomedicine: Nanotechnology, Biol. Med. 10, 1195–1208. doi:10.1016/j.nano.2014.02.012
Patil, S., Majumdar, B., Sarode, S. C., Sarode, G. S., and Awan, K. H. (2018). Oropharyngeal Candidosis in HIV-Infected Patients-An Update. Front. Microbiol. 9, 980. doi:10.3389/fmicb.2018.00980
Poulopoulos, P., Lewitz, B., Straub, A., Pappas, S. D., Droulias, S. A., Baskoutas, S., et al. (2012). Band-gap Tuning at the Strong Quantum Confinement Regime in Magnetic Semiconductor EuS Thin Films. Appl. Phys. Lett. 100 (21), 211910. doi:10.1063/1.4720167
Prasad, T., Saini, P., Gaur, N. A., Vishwakarma, R. A., Khan, L. A., Haq, Q. M. R., et al. (2005). Functional Analysis of CaIPT1, a Sphingolipid Biosynthetic Gene Involved in Multidrug Resistance and Morphogenesis of Candida albicans. Antimicrob. Agents Chemother. 49, 3442–3452. doi:10.1128/AAC.49.8.3442-3452.2005
Prasad, T., Sethumadhavan, S., and Fatima, Z. (2011). “Altered Ergosterol Biosynthetic Pathway–An Alternate Multidrug Resistance Mechanism Independent of Drug Efflux Pump in Human Pathogenic Fungi C. albicans,” in Science against Microbial Pathogens: Communicating Current Research and Technological Advances, Microbiology Series. Editor A. Méndez-Vilas Badajoz (Spain: Formatex Research Center), 757–768.
Preeti, , Prasad, T., Magoo, D., Meena, K., Ghorai, S. M., and Kaur, H. (2020a). Elucidation of the Antimicrobial Capabilities of Spirooxindole Fused Heterocycles Synthesized via Catalyst Free Method. Indian J. Nat. Sci. 10, 27323–27330.
Preeti, , Radhakrishnan, V. S., Radhakrishnan, S., Mukherjee, S., Singh, S. P., and Prasad, T. (2020b). ZnO Quantum Dots: Broad Spectrum Microbicidal Agent against Multidrug Resistant Pathogens E. coli and C. albicans. Front. Nanotechnol. 2, 576342. doi:10.3389/fnano.2020.576342
Radhakrishnan, V. S., Dwivedi, S. P., Siddiqui, M. H., and Prasad, T. (2018a). In Vitro studies on Oxidative Stress-Independent, Ag Nanoparticles-Induced Cell Toxicity of C. albicans, an Opportunistic Pathogen. Int. J. Nanomedicine 13, 91–96. doi:10.2147/IJN.S125010
Radhakrishnan, V. S., Reddy Mudiam, M. K., Kumar, M., Dwivedi, S. P., Singh, S. P., and Prasad, T. (2018b). Silver Nanoparticles Induced Alterations in Multiple Cellular Targets, Which Are Critical for Drug Susceptibilities and Pathogenicity in Fungal Pathogen (Candida albicans). Int. J. Nanomed. 13, 2647–2663. doi:10.2147/IJN.S150648
Rybak, M. J., and McGrath, B. J. (1996). Combination Antimicrobial Therapy for Bacterial Infections. Drugs 52, 390–405. doi:10.2165/00003495-199652030-00005
Samanta, P. K. (2018). Strong and Weak Quantum Confinement and Size Dependent Optoelectronic Properties of Zinc Oxide. Ann. Univ. Craiova Phys. 28, 17–23.
Santos, J. R. A., Gouveia, L. F., Taylor, E. L. S., Resende-Stoianoff, M. A., Pianetti, G. A., César, I. C., et al. (2012). Dynamic Interaction between Fluconazole and Amphotericin B against Cryptococcus Gattii. Antimicrob. Agents Chemother. 56, 2553–2558. doi:10.1128/AAC.06098-11
Siddiqi, K. S., ur Rahman, A., Tajuddin , , and Husen, A. (2018). Properties of Zinc Oxide Nanoparticles and Their Activity against Microbes. Nanoscale Res. Lett. 13, 141. doi:10.1186/s11671-018-2532-3
Sirelkhatim, A., Mahmud, S., Seeni, A., Kaus, N., Ann, L. C., Bakhori, S., et al. (2015). Review on Zinc Oxide Nanoparticles: Antibacterial Activity and Toxicity Mechanism. Nanomicro Lett. 7 (3), 219–242. doi:10.1007/s40820-015-0040-x
Spampinato, C., and Leonardi, D. (2013). Candida Infections, Causes, Targets, and Resistance Mechanisms: Traditional and Alternative Antifungal Agents. BioMed. Res. Int. 2013, 204237. doi:10.1155/2013/204237
Srivastava, V., Singla, R. K., and Dubey, A. K. (2018). Emerging Virulence, Drug Resistance and Future Anti-fungal Drugs for Candida Pathogens. Curr. Top Med. Chem. 18 (9), 759–778. doi:10.2174/1568026618666180528121707
Swain, P. S., Rao, S. B., Rajendran, D., Dominic, G., and Selvaraju, S. (2016). Nano Zinc, An Alternative to Conventional Zinc as Animal Feed Supplement: A Review. Anim. Nutr. 2 (3), 134–141. doi:10.1016/j.aninu.2016.06.003
Tamma, P. D., Cosgrove, S. E., and Maragakis, L. L. (2012). Combination Therapy for Treatment of Infections with Gram-Negative Bacteria. Clin. Microbiol. Rev. 25(3), 450–470. doi:10.1128/CMR.05041-11
Tiwari, V., Mishra, N., Gadani, K., Solanki, P. S., Shah, N. A., and Tiwari, M. (2018). Mechanism of Anti-bacterial Activity of Zinc Oxide Nanoparticle against Carbapenem-Resistant Acinetobacter Baumannii. Front. Microbiol. 9, 1218. doi:10.3389/fmicb.2018.01218
Tso, G. H. W., Reales-Calderon, J. A., and Pavelka, N. (2018). The Elusive Anti-Candida Vaccine: Lessons from the Past and Opportunities for the Future. Front. Immunol. 9, 897. doi:10.3389/fimmu.2018.00897
Wang, L., Hu, C., and Shao, L. (2017). The Antimicrobial Activity of Nanoparticles: Present Situation and Prospects for the Future. Int. J. Nanomedicine 12, 1227–1249. doi:10.2147/IJN.S121956
Watt, K., Manzoni, P., Cohen-Wolkowiez, M., Rizzollo, S., Boano, E., Jacqz-Aigrain, E., et al. (2013). Triazole Use in the Nursery: Fluconazole, Voriconazole, Posaconazole, and Ravuconazole. Curr. Drug Metab. 14 (2), 193–202. doi:10.2174/138920013804870583
White, T. C., Marr, K. A., and Bowden, R. A. (1998). Clinical, Cellular, and Molecular Factors that Contribute to Antifungal Drug Resistance. Clin. Microbiol. Rev. 11 (2), 382–402. doi:10.1128/cmr.11.2.382
Worthington, R. J., and Melander, C. (2013). Combination Approaches to Combat Multidrug-Resistant Bacteria. Trends Biotechnology 31 (3), 177–184. doi:10.1016/j.tibtech.2012.12.006
Xiao, Z., Wang, Q., Zhu, F., and An, Y. (2019). Epidemiology, Species Distribution, Antifungal Susceptibility and Mortality Risk Factors of Candidemia Among Critically Ill Patients: a Retrospective Study from 2011 to 2017 in a Teaching Hospital in China. Antimicrob. Resist. Infect. Control. 8, 89. doi:10.1186/s13756-019-0534-2
Keywords: ZnO QDs, Candida albicans, synergism, antifungals, multidrug resistance, fractional inhibitory concentration
Citation: Chand P, Kumari S, Mondal N, Singh SP and Prasad T (2021) Synergism of Zinc Oxide Quantum Dots with Antifungal Drugs: Potential Approach for Combination Therapy against Drug Resistant Candida albicans. Front. Nanotechnol. 3:624564. doi: 10.3389/fnano.2021.624564
Received: 31 October 2020; Accepted: 09 April 2021;
Published: 20 May 2021.
Edited by:
Anil Kumar, National Institute of Immunology (NII), IndiaReviewed by:
Jyotsana Mehta, Guru Jambheshwar University of Science and Technology Hisar, IndiaCopyright © 2021 Chand, Kumari, Mondal, Singh and Prasad. This is an open-access article distributed under the terms of the Creative Commons Attribution License (CC BY). The use, distribution or reproduction in other forums is permitted, provided the original author(s) and the copyright owner(s) are credited and that the original publication in this journal is cited, in accordance with accepted academic practice. No use, distribution or reproduction is permitted which does not comply with these terms.
*Correspondence: Tulika Prasad, cHJhc2FkdHVsaWthQGhvdG1haWwuY29t; Surinder P. Singh, c2luZ2gudXBybUBnbWFpbC5jb20=
Disclaimer: All claims expressed in this article are solely those of the authors and do not necessarily represent those of their affiliated organizations, or those of the publisher, the editors and the reviewers. Any product that may be evaluated in this article or claim that may be made by its manufacturer is not guaranteed or endorsed by the publisher.
Research integrity at Frontiers
Learn more about the work of our research integrity team to safeguard the quality of each article we publish.