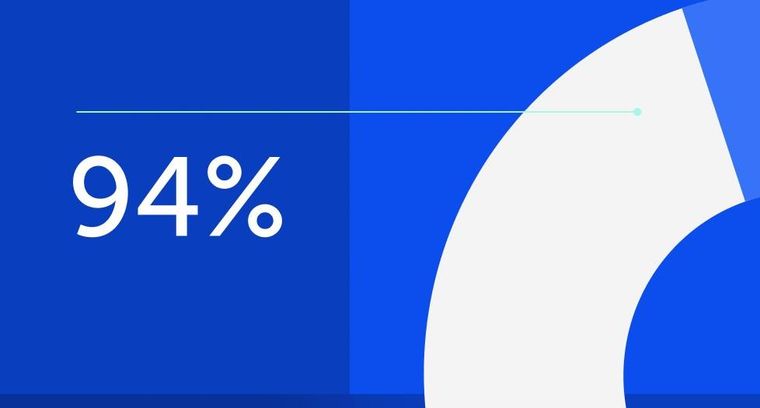
94% of researchers rate our articles as excellent or good
Learn more about the work of our research integrity team to safeguard the quality of each article we publish.
Find out more
REVIEW article
Front. Nanotechnol., 16 November 2020
Sec. Biomedical Nanotechnology
Volume 2 - 2020 | https://doi.org/10.3389/fnano.2020.571284
This article is part of the Research TopicNanotechnology Solutions to Mitigate COVID-19: Detection, Protection, MedicationView all 13 articles
The outbreak of the COVID-19, a human beta coronavirus severe acute respiratory syndrome (SARS-CoV-2) virus infection, has severely affected the world. The pandemic is not yet in full control due to a lack of rapid diagnostics and therapeutics. This viral infection continues to result in a steadily increasing loss of life, and it has also emerged as a significant global socio-economic burden. As result, it has united many countries for the purposes of exploring molecular biology, biomedical science, and the nanotechnology to manage COVID-19 successfully. As of today, the current priority is to investigate novel therapies of high efficacy and smart diagnostics tools for early-stage disease diagnostics along with monitoring. Keeping these advancement and challenges in mind, this perspective article mainly highlights the contribution and possibilities of bio-nanotechnology to manage the COVID-19 pandemic, even in a personalized manner. Authors also pinpoint barriers to the utilization of current bio-nanotechnology to facilitate a more accurate understanding of COVID-19 and to lead the way toward personalized health and wellness. Furthermore, we follow the discussion of the features and challenges in upcoming bio-nanotechnology approaches for COVID-19 management. In this progressive option report, bio-nanotechnologies that have been enriched with the power of artificial intelligence and optimized at the personalized level have been found to lead to a sustainable treatment and cure strategy at a global population scale.
In late December 2019, a considerable number of patients exhibiting respiratory symptoms were admitted to hospitals and healthcare facilities in the city of Wuhan, China. The initial assessment of this outbreak suggested that it was an isolated incident, but the increasing rate of hospitalization that ensued, even after prescribing malaria-specific treatment (Morales-Narváez and Dincer, 2020; Mujawar et al., 2020), took health experts by surprise. Following a multi-dimensional analysis of the symptoms and infection progression, physicians claimed this new respiratory outbreak might be due to a coronavirus infection. This infection appeared to be different compared to previously investigated coronavirus outbreaks, namely, SARS (associated SARS-CoV-1 virus) and middle-east acute respiratory syndrome (MERS), because of a new virus strain, i.e., human beta severe acute respiratory syndrome (SARS-CoV-2) (Rothan and Byrareddy, 2020; Shereen et al., 2020). The infection associated with the SARS-CoV-2 virus infection was then named COVID-19. This COVID-19 viral infection outbreak was later declared to be an international health emergency; soon after, it was further declared a pandemic by the World Health Organization (WHO), as SARS-CoV-2 dramatically affects the respiratory system, which can be lethal (Nadeem et al., 2020). Additionally, experts have suggested that the COVID-19 pandemic is very challenging for manage due to the ease of human-to-human transmission and rapid uptake of SARS-CoV-2 by human cells (Mujawar et al., 2020).
The SARS-CoV-2 virus structure is novel (70–80% similar to SARS-COV-1) because of the excessive presence of a spike protein (S1) and envelop protein (Figure 1A); this makes this virus more sensitive to the host cell receptors, i.e., ACE-2 enzyme and TMPRSS protein (Kim D. et al., 2020; Wrapp et al., 2020). The observed easy binding of the SARS-CoV-2 S1 protein with cell receptors (ACE-2 and TMPRSS2) causes rapid viral replication that leads to severe health-related complications. Along with respiratory-related difficulties, reports have recently emerged about SARS-CoV-2 damaging other organs such as the kidney, liver, lung, intestine, and brain. Experts have demonstrated that organs and various human processes that are also in the presence of the ACE-2 enzyme and TMPRSS protein would undoubtedly be affected by the SARS-CoV-2 virus (Scudellari, 2020; Wadman et al., 2020).
Figure 1. Illustration of SARS-CoV-2 virus structure with active sites useful for diagnostics tool and therapeutic development (A, Source: Biosensors and Bioelectronics 2020) and the significance of using a mask, as it inhibits aerosol inhalation to protect everyone in every circumstance (B, Source: Science 2020) and avoid SARS-CoV-2 virus spread via human-to-human interaction.
As of July 7, 2020, COVID-19 has affected around 10 million people in 220 countries, 30% of which are in the USA alone. The regulations and guidelines suggested by health agencies have been more focused on avoiding human-to-human contact, which affects travel, transportation, and everyday working systems (Mujawar et al., 2020; Prather et al., 2020). The successful implementation of this advice has resulted in staying at home, quarantine if exhibiting symptoms, population tracing, and increased awareness of SARS-CoV-2-related consequences. Besides, significant efforts have been made to explore treatment using available anti-viral drugs related to HIV, ZIKA, Ebola, and malaria-related infectious diseases. Use of personal protection measures as an approach that can be adopted by an individual has also emerged as a solution to control the spread of SARS-CoV-2 in the form of obeying physical/social distance, good hygiene practice, and using a mask. With focus on SARS-CoV-2 spread via human-to-human contact, it was suggested that diagnostics of COVID-19 infection via detection of SARS-CoV-2 is crucial to managing disease progression and determining appropriate therapeutics.
For the successful management of COVID-19, experts have projected bio-nanotechnology as the best scientific tool to investigate novel approaches to accomplish the following: (1) avoid COVID-19 transmission successfully; (2) detect SARS-CoV-2 virus protein selectively at the early stages of infection; (3) hinder SARS-CoV-2 virus progression; and (4) eradicate SARS-CoV-2 virus selectively. The design, development, and execution of a systematic approach must involve experts of various disciplines to achieve this. The process involves an investigation into bio-nanotechnology-assisted novel nano systems to fabricate personal protective equipment (PPE), diagnostics tools, and therapeutics. The existing biotechnologies currently utilized in the management of COVID-19 for either PPE, diagnostics, or therapeutics are summarized below.
The SARS-CoV-2 virus structure and functional mechanism was novel when it was first characterized, and a lack of validated biomarkers has made the testing process and therapeutic invention very challenging (Nadeem et al., 2020). In the early stages of the COVID-19 outbreak, efforts were primarily concentrated toward managing the spread of infection by exploring appropriate precautions, testing systems, and therapeutics (Mujawar et al., 2020). While urgently needed, developing a rapid testing kit and effective therapeutics is a time-consuming process (Morales-Narváez and Dincer, 2020). In such scenarios, experts have suggested that we to pay full attention toward controlling the SARS-CoV-2 virus spread via avoiding human-to-human contact, using a mask, and exploring the possible modes of SARS-CoV-2 virus interaction with the human system (Figure 1B).
The guidelines issued by health agencies have thus prioritized the maintenance of good hygiene (mainly washing hands, as liquid soap breaks down the bond between the virus and wax on the skin). Emphasis has also been put on raising awareness on how to maintain social and physical distance. The need for physical distance has increased the demand for the development and production of PPE to cover the body, and this is mainly recommended for healthcare workers in hospitals and health care facilities. Wearing effective masks was also recommended as an essential tool to be safe while working in various condition involving patient care and testing. Masks should be compatible with humans and have the ability to trap and eradicate SARS-CoV-2 virus proteins (Konda et al., 2020; Leung et al., 2020; Prather et al., 2020; van Doremalen et al., 2020).
To accomplish this, bio-nanotechnology plays a crucial role in the development smart functionalized biocompatible nanostructures with high antibacterial and antiviral capabilities. Such nano systems are being used as a coating material to design and develop next-generation PPE to combat the COVID-19 pandemic (Konda et al., 2020). Using masks is doable for most people, and it has been confirmed to be a manageable and effective method of prevention. Several studies have confirmed that wearing a mask reduces the number COVID-19 cases in several regional and working environments (Prather et al., 2020; van Doremalen et al., 2020). These outcomes have also been supported by findings that the SARS-CoV-2 virus may be airborne (Lewis, 2020); it is very active in aerosol form and viable on various substrates, which are a part of everyday life (van Doremalen et al., 2020). In any environment, the SARS-CoV-2 virus is known to be viable on various substrates and micro-environments containing appropriate heat and moisture. A shield is thus required to avoid SARS-CoV-2 virus inhalation, and such a shield could be an efficient mask with nanoparticles where the SARS-CoV-2 virus is not viable.
Recently, it has been demonstrated that the SARS-CoV-2 virus is not viable on Cu surfaces and loses functional activity at a temperature higher than 40°C (van Doremalen et al., 2020). Besides stimuli-responsive nanoparticles, the photosensitive TiO2 nanoparticle has also exhibited the degradation of bacteria and viruses upon application of optimized stimulation (Vatansever et al., 2013). Keeping this in mind, efforts are being made to design and develop mask-embedded nanoparticles that can trap and eradicate the SARS-CoV-2 virus. Presently, the outcomes of several research studies have confirmed that a selection of a mask fabrics (for example, cotton, silk, and a combination of fibers) and heat- and light-sensitive nano systems (single system or hybrid nanocomposite) are critical factors to the development of an active mask to combat COVID-19 (Mujawar et al., 2020).
However, the anti-viral activity of such a mask has not been reported so far due to a lack of optimized cell types and research infrastructure (BSL-3 Laboratory), which should certainly be an area for future study, but its utilization has certainly reduced SARS-CoV-2 virus spread.
The life-threatening COVID-19 pandemic is very challenging, mainly due to a lack of rapid testing kits for selective detection of the SARS-CoV-2 virus (Morales-Narváez and Dincer, 2020; Mujawar et al., 2020). In the beginning of the outbreak, a biomolecular assay, mainly RT-PCR, was the primary method of COVID-19 diagnostics. The diagnostic performances of these assays are outstanding, but the need for a well-equipped laboratory and expertise to perform testing limits their application. The detection of the SARS-CoV-2 virus protein is crucial for understanding infection progression, evaluating the efficacy of the prescribed therapy, making a timely decision to optimize therapeutics, and ultimately managing the COVID-19 pandemic. Furthermore, asymptomatic carriers of COVID-19 infection are another serious challenge because this virus can stay in a healthy human without showing any symptoms. The probability of SARS-CoV-2 virus spread varies significantly, and, at the same time, false-positive reporting of biomolecular assays has raised more challenges (Morales-Narváez and Dincer, 2020; Mujawar et al., 2020; Yan et al., 2020).
Based on the opinion of health experts, managing the COVID-19 pandemic is a subject of SARS-CoV-2 virus detection on a mass scale. The possibility of testing a large population is more relevant to big cities and urban areas, and it was thus recommended that we investigate a novel, selective, and affordable analytical system to detect SARS-CoV-2 (Mujawar et al., 2020; Seo et al., 2020). Such smart diagnostic systems are not only required for COVID-19 diagnostics but also for generating the bioinformatics required to understand the disease progression and correlate the virus level with its pathogenesis (Allam and Jones, 2020; Cheng et al., 2020; Cohen et al., 2020; Mujawar et al., 2020).
Rapid testing of a large populations in cities and remote areas demands the exploration of potential solutions that involve state-of-the-art bio-nanotechnology (Kaushik and Mujawar, 2018; Kaushik, 2019) with a high performance and reduced form factor. The introduction of bio-nanotechnology to COVID-19 management is mainly based on selecting a nano system (useful to immobilize appropriate bioactives with the best functional structure) and bioactives capable of detecting SARS-CoV-2 virus selectively. Such of compartmentalization-based approach is the foundation of fabricating a nano-enabled miniaturized biosensor for SARS-CoV-2 virus protein detection, even at the site of epidemic outbreak and high-alert areas. Recently, various nano-enabled SARS-CoV-2 biosensors based on the flied-effect-transistor (FET), dual responsive photo-plasmonic, and specific gene sequence principle have been fabricated for the applications related to COVID-19 diagnostics (Ishikawa et al., 2009; Broughton et al., 2020, p. 12; Qiu et al., 2020). These sensors are capable of testing real samples for clinical application.
We also believe that a miniaturized SARS-CoV-2 virus-sensing system is supported by advanced electronics and the internet of things (IoT) technology in relation to the performance of COVID-19 diagnostics for point-of-care (POC) application. The POC diagnostics of the COVID-19 pandemic were proven to be useful when generating the bioinformatics desired to correlate infection progression with race, gender, age, and region. These analytics encourage new ways to optimize the combination of the available drug to decide an appropriate therapy. Such desired testing and analysis have increased the demand for the involvement of computational studies to analyze, generate, store, and share the bioinformatics.
As illustrated in Figure 2, SARS-CoV-2 virus sensing using nano-enabled sensors supported by AI and the IoT have become essential to COVID-19 pandemic management (Mujawar et al., 2020). Such an advanced COVID-19 diagnostics strategy has potential not only for clinical set-up, but it also has the ability to perform targeted testing and tracing of patients that are asymptomatic carries and/or discharged patients who may have the chance to become reinfected with SARS-CoV-2. It has been reported that the SARS-CoV-2 virus can stay in the human body for longer—around 21 days has been reported (Cheng et al., 2020)—and a real-time sensing of this virus has thus also been suggested to be essential, and this can be achieved using AI- and IoT-supported nanosensors (Mujawar et al., 2020). The integration of AI within an analytical system would enable the following: predicting whether a region is safe or not, assessing when someone need to quarantine, suggesting how to manage social distance, sharing information with a medical center, suggesting how to access telemedicine, etc. These sensing systems are also in demand to use to design and develop therapeutic agents to combat the COVID-19 pandemic.
Figure 2. Nano-enabled biosensing system for SARS-CoV-2 detection, a bottom–top approach, showing the efficient integration of artificial intelligence and internet of things for the purposes of personalized COVID-19 management.
Since the COVID-19 pandemic was declared as an international health emergency, significant efforts have been made to design and develop novel therapeutic agents to recognize and eradicate the SARS-CoV-2 virus (Li and De Clercq, 2020). Such agents are urgently required to combat against COVID-19. Experts are therefore exploring all the possible structures and strains of SARS-COV-2 virus protein. This information necessary in order to investigate active sites of the SARS-CoV-2 virus, and this is a requirement when trying to develop an efficient drug, vaccine, and therapeutic agent to combat against COVID-19 selectively. Based on recent investigations (Kaushik et al., 2014, 2016a,b, 2017, 2018c), sincere efforts have been made to explore SARS-CoV-2 virus-related pathogenesis and its probable effect on human systems. It has been found that the SARS-CoV-2 virus can stay in the human body for as long as 21 days, and it can affect the respiratory system, which in turn can damage other organs and alter functional systems within the human body. Such challenging consequences have increased the demand for effective and efficient therapeutic agents. It is suggested that such new agents perform targeted and long-term efficacy with negligible side effects (Chan, 2020; Hu et al., 2020). The design and development of such therapeutic cargo are possible through the exploration of an optimized bio-nanotechnology, i.e., nanomedicine to combat the COVID-19 pandemic (Kaushik, 2019).
Nanomedicine (size ranging from 10 to 200 nm), pharmacologically relevant therapeutic cargo, has shown a significant accomplishment in management of targeted diseases, such as, for example, cancer and viral infections (Nair et al., 2016; Kaushik et al., 2018a,b). It has been suggested that if scientists can investigate a therapeutic agent against the SARS-CoV-2 virus then it is site-specific delivery that will be a challenge. This site-specific delivery of an optimized therapeutic agent and controlled release and maintenance of the therapeutic agent has a vital role in the possible management of COVID-19 (Nair et al., 2016; Kaushik et al., 2018b, 2019a; Vashist et al., 2018).
Optimizing bio-nanotechnology is necessary to the development of nanomedicine against SARS-CoV-2 to manage the COVID-19 pandemic. As we know, the SARS-CoV-2 virus strain variation is dependent on various categories, mainly personal medical history, which makes the optimization of a therapy challenging. Exploring precision nanomedicine can be another way to control COVID-19 infection in a personalized fashion and is possible via investigating bio-nanotechnology and nanomedicine, designed and developed for personalized health wellness.
In line with our earlier work in the development nanomedicine for neuro HIV/AIDS management (Kaushik et al., 2019b), we suggested that the SARS-COV-2 virus can be up-taken by a specific cell because it can securely bind with this virus and cell surface receptors (Hu et al., 2020). Then, the SARS-CoV-2 virus hijacked cell bio-functionality to create more and more SARS-COV-2 virus copies, and over time, this virus severely affects the respiratory system of humans. The SARS-CoV-2 virus also affects other bodily functions and damages various organs. Future therapy for COVID-19 should thus also be developed to support the immune system as well. Developing an effective therapy for COVID-19 will therefore be a compartmentalization-based approach that will recognize and eradicate the virus and provide support for quick recovery.
Presently, Dr. Hu has explained the mechanism of chloroquine, an anti-malarial drug, against the SARS-COV-2 virus (Hu et al., 2020). The research suggested that viruses of a nanoparticle size ranging from 2 to 60 nm can adopt the conventional approach of cell-uptake. Thus, developing bio-compatible nanoparticles, which can act as inhibitors, is a viable approach to combating infection. Besides, developing various drugs, vaccines, genes, and antibodies that can knock down the SARS-COV-2 virus is the ultimate objective of experts. The selection of such agents and maintaining their functionality in the human body is very challenging. Moreover, a high dose of these agents due to off-targeting may cause several side effects, which can be worse than SARS-COV-2 virus infection. As a recent development, microneedle array (MNA)-based recombinant coronavirus vaccine delivery has been demonstrated by Kim et al. In this research, the coronavirus S-1 subunit vaccine used a MNA to elicit antigen-specific antibody responses, which was observed in the beginning of 2 weeks after immunization (Kim E. et al., 2020). However, developing such immunotherapy for the clinic is a motivational approach (Kim E. et al., 2020)
These obstacles can be overcome if we can deliver an optimized therapeutic agent at a targeted site with control over the drug release as per patient disease progression. These essential tasks can be achieved via introducing nanomedicine fabricated using a stimuli-responsive nano system, and an optimized therapeutic agent can be developed to combat SARS-COV-2 virus-associated symptoms. Such therapeutic cargos (as illustrated in Figure 3) with tunable drug payload and controlled drug release features can eradicate the SARS-COV-2 virus. Based on patient medical history, nanomedicine can be prepared to achieve maximum efficacy and the least amount of adverse effects. Personalized health management in the case of the COVID-19 pandemic is thus possible through the adoption of nanomedicine as a future therapy. Such approaches, however, are still in the early stages of development, and they need public-private involvement to conduct systematic research, followed by FDA approval, before they can be promoted for clinical application.
This perspective article summarizes the potential of bio-nanotechnology to combat the COVID-19 pandemic through the avoidance of virus transmission, selective detection of the SARS-COV-2 virus, and novel therapies that can recognize and eradicate the SARS-COV-2 virus with negligible side effects. Successful efforts have been made to demonstrate the capacities of bio-nanotechnology to handle the COVID-19 outbreak in a personalized manner. Bio-nanotechnology-assisted approaches have successfully investigated novel and smart nano systems needed to develop effective masks, efficient diagnostics tools, and therapies of higher efficacy. Following advancements in bio-nanotechnology, the impact and effect of the SARS-COV-2 virus upon the major organs of interest and behavioral aspects have been successfully explored.
Such accomplishments are, however, very motivational at the preliminary stage but cannot be recommended for global use because of the variables found within populations, including region, race, gender, and age. The battle against COVID-19 will therefore be a personalized one and requires the help of technology, principally an integration of bio-nanotechnology and computational intelligence. Future research must involve massive private-public partnerships and should be directed toward introducing AI-enabled bio-nanotechnology in every aspect of SARS-COV-2 virus-related investigation. An enormous amount of data and biological samples are being collected, which facilitates data analytics as well as data-driven and evidence-based planning for COVID-19 management. In brief, AI-enabled bio-nanotechnology optimized for personalized prevention and treatment can pave the way to a population-based cure.
Taking this into consideration, we request that experts initiate a multi-disciplinary project where bio-nanotechnology, integrated with artificial intelligence, can be implemented on the IoT platform and used in a reduced form factor (least adverse effects but maximum desired effects) to develop effective and affordable technologies and therapies to combat the SARS-COV-2-associated COVID-19 pandemic.
All authors contributed equally in visualizing, writing, revising, summarizing challenges, and proposing aspects of Bio-nanotechnology to manage COVID-19 pandemic.
CD employed by the company Q&I Diagnostics.
The remaining authors declare that the research was conducted in the absence of any commercial or financial relationships that could be construed as a potential conflict of interest.
The handling editor is currently organizing a Research Topic with one of the authors AK.
The authors acknowledge respective institutions and departments for providing facilities and support. AK acknowledges a research grant, namely, GR-2000024, funded by Florida Polytechnic University, Lakeland, FL, USA.
Allam, Z., and Jones, D. S. (2020). On the coronavirus (COVID-19) outbreak and the smart city Network: universal data sharing standards coupled with Artificial Intelligence (AI) to benefit urban health monitoring and management. Healthcare 8:46. doi: 10.3390/healthcare8010046
Broughton, J. P., Deng, X., Yu, G., Fasching, C. L., Servellita, V., Singh, J., et al. (2020). CRISPR–Cas12-based detection of SARS-CoV-2. Nat. Biotechnol. 38, 870–874. doi: 10.1038/s41587-020-0513-4
Chan, W. C. W. (2020). Nano research for COVID-19. ACS Nano 14, 3719–3720. doi: 10.1021/acsnano.0c02540
Cheng, H.-Y., Jian, S.-W., Liu, D.-P., Ng, T.-C., Huang, W.-T., Lin, H.-H., et al. (2020). Contact tracing assessment of COVID-19 transmission dynamics in taiwan and risk at different exposure periods before and after symptom onset. JAMA Intern. Med. 180, 1156–1163. doi: 10.1001/jamainternmed.2020.2020
Cohen, I. G., Gostin, L. O., and Weitzner, D. J. (2020). Digital smartphone tracking for COVID-19: public health and civil liberties in tension. JAMA 323, 2371–2372. doi: 10.1001/jama.2020.8570
Hu, T. Y., Frieman, M., and Wolfram, J. (2020). Insights from nanomedicine into chloroquine efficacy against COVID-19. Nat. Nanotechnol. 15, 247–249. doi: 10.1038/s41565-020-0674-9
Ishikawa, F. N., Chang, H.-K., Curreli, M., Liao, H.-I., Olson, C. A., Chen, P.-C., et al. (2009). Label-free, electrical detection of the SARS virus N-protein with nanowire biosensors utilizing antibody mimics as capture probes. ACS Nano 3, 1219–1224. doi: 10.1021/nn900086c
Kaushik, A. (2019). Biomedical nanotechnology related grand challenges and perspectives. Front. Nanotechnol. 1:1. doi: 10.3389/fnano.2019.00001
Kaushik, A., Jayant, R. D., Bhardwaj, V., and Nair, M. (2018a). Personalized nanomedicine for CNS diseases. Drug Discov. Today 23, 1007–1015. doi: 10.1016/j.drudis.2017.11.010
Kaushik, A., Jayant, R. D., and Nair, M. (2018b). Nanomedicine for neuroHIV/AIDS management. Nanomedicine 13, 669–673. doi: 10.2217/nnm-2018-0005
Kaushik, A., Jayant, R. D., Tiwari, S., Vashist, A., and Nair, M. (2016a). Nano-biosensors to detect beta-amyloid for Alzheimer's disease management. Biosens. Bioelectr. 80, 273–287. doi: 10.1016/j.bios.2016.01.065
Kaushik, A., and Mujawar, M. (2018). Point of care sensing devices: better care for everyone. Sensors 18:4303. doi: 10.3390/s18124303
Kaushik, A., Rodriguez, J., Rothen, D., Bhardwaj, V., Jayant, R. D., Pattany, P., et al. (2019a). MRI-guided, noninvasive delivery of magneto-electric drug nanocarriers to the brain in a nonhuman primate. ACS Appl. Bio Mater. 2, 4826–4836. doi: 10.1021/acsabm.9b00592
Kaushik, A., Tiwari, S., Dev Jayant, R., Marty, A., and Nair, M. (2016b). Towards detection and diagnosis of Ebola virus disease at point-of-care. Biosens. Bioelectr. 75, 254–272. doi: 10.1016/j.bios.2015.08.040
Kaushik, A., Tiwari, S., Jayant, R. D., Vashist, A., Nikkhah-Moshaie, R., El-Hage, N., et al. (2017). Electrochemical biosensors for early stage zika diagnostics. Trends Biotechnol. 35, 308–317. doi: 10.1016/j.tibtech.2016.10.001
Kaushik, A., Vasudev, A., Arya, S. K., Pasha, S. K., and Bhansali, S. (2014). Recent advances in cortisol sensing technologies for point-of-care application. Biosens. Bioelectr. 53, 499–512. doi: 10.1016/j.bios.2013.09.060
Kaushik, A., Yndart, A., Atluri, V., Tiwari, S., Tomitaka, A., Gupta, P., et al. (2019b). Magnetically guided non-invasive CRISPR-Cas9/gRNA delivery across blood-brain barrier to eradicate latent HIV-1 infection. Sci. Rep. 9:3928. doi: 10.1038/s41598-019-40222-4
Kaushik, A., Yndart, A., Kumar, S., Jayant, R. D., Vashist, A., Brown, A. N., et al. (2018c). A sensitive electrochemical immunosensor for label-free detection of Zika-virus protein. Sci. Rep. 8:9700. doi: 10.1038/s41598-018-28035-3
Kim, D., Lee, J.-Y., Yang, J.-S., Kim, J. W., Kim, V. N., and Chang, H. (2020). The Architecture of SARS-CoV-2 transcriptome. Cell 181, 914–921.e10. doi: 10.1101/2020.03.12.988865
Kim, E., Erdos, G., Huang, S., Kenniston, T. W., Balmert, S. C., Carey, C. D., et al. (2020). Microneedle array delivered recombinant coronavirus vaccines: immunogenicity and rapid translational development. EBioMedicine 55:102743. doi: 10.1016/j.ebiom.2020.102743
Konda, A., Prakash, A., Moss, G. A., Schmoldt, M., Grant, G. D., and Guha, S. (2020). Aerosol filtration efficiency of common fabrics used in respiratory cloth masks. ACS Nano 14, 6339–6347. doi: 10.1021/acsnano.0c04676
Leung, N. H. L., Chu, D. K. W., Shiu, E. Y. C., Chan, K.-H., McDevitt, J. J., Hau, B. J. P., et al. (2020). Respiratory virus shedding in exhaled breath and efficacy of face masks. Nat. Med. 26, 676–680. doi: 10.1038/s41591-020-0843-2
Lewis, D. (2020). Is the coronavirus airborne? Experts can't agree. Nature 580, 175–175. doi: 10.1038/d41586-020-00974-w
Li, G., and De Clercq, E. (2020). Therapeutic options for the 2019 novel coronavirus (2019-nCoV). Nat. Rev. Drug Discov. 19, 149–150. doi: 10.1038/d41573-020-00016-0
Morales-Narváez, E., and Dincer, C. (2020). The impact of biosensing in a pandemic outbreak: COVID-19. Biosens. Bioelectr. 163:112274. doi: 10.1016/j.bios.2020.112274
Mujawar, M. A., Gohel, H., Bhardwaj, S. K., Srinivasan, S., Hickman, N., and Kaushik, A. (2020). Aspects of nano-enabling biosensing systems for intelligent healthcare; towards COVID-19 management. Mater. Today Chem. 17:100306. doi: 10.1016/j.mtchem.2020.100306
Nadeem, M. S., Zamzami, M. A., Choudhry, H., Murtaza, B. N., Kazmi, I., Ahmad, H., et al. (2020). Origin, potential therapeutic targets and treatment for coronavirus disease (COVID-19). Pathogens 9:307. doi: 10.3390/pathogens9040307
Nair, M., Jayant, R. D., Kaushik, A., and Sagar, V. (2016). Getting into the brain: potential of nanotechnology in the management of NeuroAIDS. Adv. Drug Deliv. Rev. 103, 202–217. doi: 10.1016/j.addr.2016.02.008
Prather, K. A., Wang, C. C., and Schooley, R. T. (2020). Reducing transmission of SARS-CoV-2. Science 368, 1422–1424. doi: 10.1126/science.abc6197
Qiu, G., Gai, Z., Tao, Y., Schmitt, J., Kullak-Ublick, G. A., and Wang, J. (2020). Dual-functional plasmonic photothermal biosensors for highly accurate severe acute respiratory syndrome coronavirus 2 detection. ACS Nano 14, 5268–5277. doi: 10.1021/acsnano.0c02439
Rothan, H. A., and Byrareddy, S. N. (2020). The epidemiology and pathogenesis of coronavirus disease (COVID-19) outbreak. J. Autoimmun. 109:102433. doi: 10.1016/j.jaut.2020.102433
Scudellari, M. (2020). The sprint to solve coronavirus protein structures — and disarm them with drugs. Nature 581, 252–255. doi: 10.1038/d41586-020-01444-z
Seo, G., Lee, G., Kim, M. J., Baek, S.-H., Choi, M., Ku, K. B., et al. (2020). Rapid detection of COVID-19 causative virus (SARS-CoV-2) in human nasopharyngeal swab specimens using field-effect transistor-based biosensor. ACS Nano 14, 5135–5142. doi: 10.1021/acsnano.0c02823
Shereen, M. A., Khan, S., Kazmi, A., Bashir, N., and Siddique, R. (2020). COVID-19 infection: origin, transmission, and characteristics of human coronaviruses. J. Adv. Res. 24, 91–98. doi: 10.1016/j.jare.2020.03.005
van Doremalen, N., Bushmaker, T., Morris, D. H., Holbrook, M. G., Gamble, A., Williamson, B. N., et al. (2020). Aerosol and surface stability of SARS-CoV-2 as compared with SARS-CoV-1. N. Engl. J. Med. 382, 1564–1567. doi: 10.1056/NEJMc2004973
Vashist, A.rti, Kaushik, A., Vashist, A.tul, Bala, J., Nikkhah-Moshaie, R., Sagar, V., and Nair, M. (2018). Nanogels as potential drug nanocarriers for CNS drug delivery. Drug Discov. Today 23, 1436–1443. doi: 10.1016/j.drudis.2018.05.018
Vatansever, F., Ferraresi, C., de Sousa, M. V. P., Yin, R., Rineh, A., Sharma, S. K., et al. (2013). Can biowarfare agents be defeated with light? Virulence 4, 796–825. doi: 10.4161/viru.26475
Wadman, M., Couzin-Frankel, J., Kaiser, J., and Matacic, C. (2020). A rampage through the body. Science 368, 356–360. doi: 10.1126/science.368.6489.356
Wrapp, D., Wang, N., Corbett, K. S., Goldsmith, J. A., Hsieh, C.-L., Abiona, O., et al. (2020). Cryo-EM structure of the 2019-nCoV spike in the prefusion conformation. Science 367, 1260–1263. doi: 10.1126/science.abb2507
Keywords: SARS-COV-2 virus infection, COVID-19 pandemic, bio-nanotechnology, nanotherapeutics, nano-sensors, artificial intelligence, COVID-19 management
Citation: Paliwal P, Sargolzaei S, Bhardwaj SK, Bhardwaj V, Dixit C and Kaushik A (2020) Grand Challenges in Bio-Nanotechnology to Manage the COVID-19 Pandemic. Front. Nanotechnol. 2:571284. doi: 10.3389/fnano.2020.571284
Received: 10 June 2020; Accepted: 28 August 2020;
Published: 16 November 2020.
Edited by:
Bingqing Wei, University of Delaware, United StatesReviewed by:
Vivek Gupta, St. John's University, United StatesCopyright © 2020 Paliwal, Sargolzaei, Bhardwaj, Bhardwaj, Dixit and Kaushik. This is an open-access article distributed under the terms of the Creative Commons Attribution License (CC BY). The use, distribution or reproduction in other forums is permitted, provided the original author(s) and the copyright owner(s) are credited and that the original publication in this journal is cited, in accordance with accepted academic practice. No use, distribution or reproduction is permitted which does not comply with these terms.
*Correspondence: Ajeet Kaushik, YWthdXNoaWtAZmxvcmlkYXBvbHkuZWR1
Disclaimer: All claims expressed in this article are solely those of the authors and do not necessarily represent those of their affiliated organizations, or those of the publisher, the editors and the reviewers. Any product that may be evaluated in this article or claim that may be made by its manufacturer is not guaranteed or endorsed by the publisher.
Research integrity at Frontiers
Learn more about the work of our research integrity team to safeguard the quality of each article we publish.