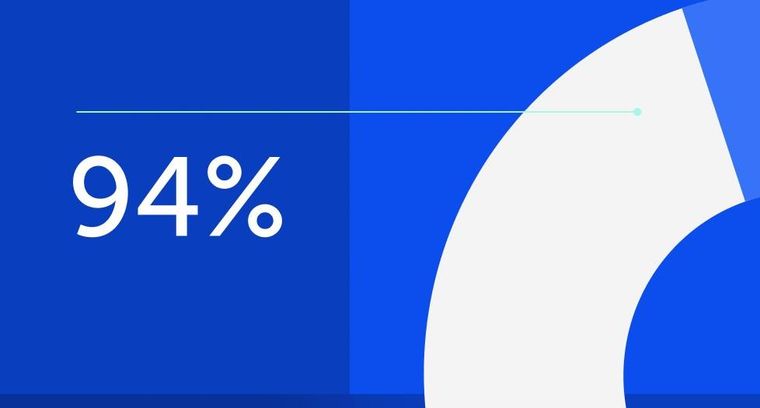
94% of researchers rate our articles as excellent or good
Learn more about the work of our research integrity team to safeguard the quality of each article we publish.
Find out more
ORIGINAL RESEARCH article
Front. Neuroanat., 14 October 2022
Volume 16 - 2022 | https://doi.org/10.3389/fnana.2022.1012422
Alzheimer’s disease (AD) is a neurodegenerative disorder characterized by gradual cognitive decline. Strong antioxidants that inhibit free radicals, such as polyphenols, reduce the likelihood of developing oxidative stress-related degenerative diseases such as AD. Naringin, a flavonoid found in citrus fruit shown to be neuroprotective, reduce oxidative damage and minimize histopathological changes caused by ischemic reperfusion, enhance the long-term memory in AD animal models. This work aimed to comprehend the role of naringin in the defense of the cerebellum against aluminum chloride (AlCl3)-induced AD in rats by investigating the behavioral, neurochemical, immunohistochemical, and molecular mechanisms that underpin its possible neuroprotective effects. Twenty-four adult albino rats were divided into four groups (n = 6/group): (i) Control (C) received saline per oral (p.o.), (ii) Naringin(N)-received naringin (100 mg/kg/d) p.o, (iii) AlCl3-recived AlCl3 (100 mg/kg/d) p.o and (iv) AlCl3 + Naringin (AlCl3 + N) received both AlCl3 and naringin p.o for 21 days. Behavioral tests showed an increase in the time to reach the platform in Morris water maze, indicating memory impairment in the AlCl3-treated group, but co-administration of naringin showed significant improvement. The Rotarod test demonstrated a decrease in muscle coordination in the AlCl3-treated group, while it was improved in the AlCl3 + N group. Neurochemical analysis of the hippocampus and cerebellum revealed that AlCl3 significantly increased lipid peroxidation and oxidative stress and decreased levels of reduced glutathione. Administration of naringin ameliorated these neurochemical changes via its antioxidant properties. Cerebellar immunohistochemical expression for microtubule assembly (tau protein) and oxidative stress (iNOS) increased in A1C13-treated group. On the other hand, the expression of the autophagic marker (LC3) in the cerebellum showed a marked decline in AlCl3-treated group. Western blot analysis confirmed the cerebellar immunohistochemical findings. Collectively, these findings suggested that naringin could contribute to the combat of oxidative and autophagic stress in the cerebellum of AlCl3-induced AD.
Alzheimer’s disease (AD) is the most prevalent neurodegenerative disease that causes memory loss and progressive neurocognitive deterioration in the elderly (Babri et al., 2014). A key risk factor for various age-related neurodegenerative diseases, such as AD, is aluminum (Al) (Kumar et al., 2011). It has been widely used in the industry, and it is currently added to a large number of products available to everyone, including drinking water, many processed foods, infant formulae, cosmetics, toothpaste, antiperspirants, and various medical preparations and medicines (Bondy, 2016).
AlCl3 is a strong neurotoxin that has been linked to the neuropathogenesis of AD (Borai et al., 2017). It is reported to be involved in the etiology of AD as it can easily cross the blood brain barrier (Lakshmi et al., 2015; Borai et al., 2017). Al ions are able to interact with different proteins inducing misfolding and aggregation which are key pathophysiological mechanisms in AD (Colomina and Peris-Sampedro, 2017).
The hippocampus, cerebral cortex, cerebellum, corpus callosum, amygdala, thalamus, and corpus callosum are among the areas of the brain that exhibit slow and progressive neurodegeneration in AD (Akbarpour et al., 2015). Cerebellum has long been known for its role in motor control, but more recently, it has also been regarded important for higher-order cognitive, emotional, and even social processing (Schmahmann, 2019). It exhibits neuropathology in AD which includes structural and functional abnormalities that match the geography of neurodegeneration seen in the cerebral hemispheres (Jacobs et al., 2018).
Motor and cognitive abilities deteriorate with age in both humans and animals, which may be related to a greater vulnerability to the cumulative effects of oxidative stress and inflammation (Zhang et al., 2013). There are evidences that brain tissue in AD patients is exposed to oxidative stress (Gella and Durany, 2009). The most significant feature is that oxidative stress appears to be a primary progenitor of the disease (Bonda et al., 2010).
Polyphenols are strong antioxidants that inhibit developing oxidative stress-related degenerative diseases such as AD (Basli et al., 2012). Their ability to improve neurological health is mediated by several mechanisms, including their interaction with neuronal and glial signaling pathways, reduction in neurotoxins-mediated neuronal damage and loss or neuroinflammation, decrease in reactive oxygen species (ROS) production, and decrease in the accumulation of neuropathological markers like amyloid-β (Aβ) and tau protein (Bensalem et al., 2015).
One of the most significant flavonoids that can be extracted from citrus fruits is naringin (NAR). Due to its potent antioxidant, anti-inflammatory, antiapoptotic, anti-ulcer, anti-osteoporosis, and anticancer properties, it has gained interest (Chen et al., 2016). Previous studies have shown that naringin therapy can reduce oxidative damage and minimize histopathological changes caused by ischemic reperfusion in the brain, striatum, and hippocampus (Cao et al., 2021). Furthermore, previous research has shown that it can enhance long-term memory and act as a neuroprotective agent in transgenic AD mice (Wang et al., 2013).
Tau protein, which plays an essential role in microtubule construction and stabilization, supports appropriate neuronal activity. Aberrant tau protein phosphorylation has been associated with AD progression, as well as its ability to cause cytotoxicity when produced in cultured cell in vitro and animal models (Kolarova et al., 2012).
AD is also associated with autophagic stress in which the rate of autophagosome production is greater than the rate of breakdown in response to protein or organelle damage (Farrag et al., 2021). Furthermore, strong autophagic activity can eliminate damaged mitochondria, so indirectly reducing the amount of ROS production and activation of the inflammasome (Zhao et al., 2019).
Since the neurochemical, immunohistochemical, and molecular effects of naringin on cerebellar neurotoxicity in AlCl3-induced AD have not been investigated yet, this study aims to explore the role of naringin in ameliorating cerebellar changes of AlCl3 rat model of AD.
Following NIH and EU norms for animal care, this study received Institutional Research Board (IRB) approval from Mansoura Faculty of Medicine (Code number: R.21.03.1280). At the Medical Experimental Research Center (MERC), where the experiment was carried out, the rats were housed under veterinary care. The number of animals used and animal discomfort were minimized as far as possible.
Twenty-four adult (3 months old) male albino rats of average weight of about 200–250 g were used. They were given unrestricted access to food and drink during the regular day-night cycle (12–12 h).
AlCl3 and naringin (N1376) were obtained in powder form from Sigma-Aldrich.
After 2 weeks of acclimatization, the rats were divided into four groups (n = 6/group): (i) Control (C) received saline per oral (p.o.), (ii) Naringin(N)-received naringin (100 mg/kg/d) p.o (Meng et al., 2021), (iii) AlCl3-recived AlCl3 (100 mg/kg/d) p.o (Lakshmi et al., 2015), and (iv) AlCl3 + Naringin (AlCl3 + N) received both AlCl3 and naringin p.o for 21 days. At the end of experiment, body weight was measured and Rotarod and Morris water maze tests were conducted to assess motor and learning abilities, respectively. On sacrifice, the hippocampus was dissected out to confirm neuronal degeneration. The cerebellum also was excised, neurochemical estimation of oxidative stress markers was performed, Histopathological and immunohistochemical evaluation of autophagy, Tau and oxidative stress were done and Western blotting was performed.
At the end of the experiment, rats were weighed to detect if there were significant differences in their weight after exposure to naringin and AlCl3.
Two initial training trials of 5 min each were administered to each rat to maintain posture on the rotarod, which had a diameter of 3 cm and rotated at a constant 20 rev/min. These trials were spaced roughly 10 min apart. After training trials, a trial of 2 min was done for each rat where time spent on the rotarod (grip period) was noted (Ataie et al., 2010).
Morris water maze is composed of learning (acquisition) and retention phases. The Maze consists of a large circular pool (150 cm in diameter, 45 cm in height, filled to a depth of 30 cm with water at 28 ± 1°C) divided into four equal quadrants. For learning of memory, a circular platform (4.5 cm diameter) was placed in one quadrant of the pool 1 cm below the water level. Each rat was subjected to four consecutive trials with a gap of 5 min. The rat was gently placed in the water of the pool between quadrants facing the wall of the pool. Each rat was then allowed 120 s to locate the platform. If the animal failed to reach the platform within 120 s, it was guided to it and allowed to remain there for 20 s. The time taken by each rat in each group to reach the platform (escape latency) was calculated using a stopwatch. For retention of memory (4 h after the last learning session), the platform was removed and the entry latency to the platform quadrant was detected (Laskowitz et al., 2017).
One day after the final dose of the treatment, the rats were anesthetized with 300 mg/kg of intraperitoneal chloral hydrate. The hippocampus and cerebellum were carefully removed. A portion was processed to create the paraffin blocks. Cut sections (4 μm thick) were obtained. Hematoxylin and eosin staining (H & E) was performed for both tissues. Cerebellar sections were also immunostained for the expression of LC3, tau protein, and iNOS. Another cerebellar portion was then preserved in RNA later (for RNA and protein stabilization) (Thermo Fisher Scientific, Waltham, MA, USA) at (−20°C) before being stored at (−80°C) until extraction of protein and subsequent Western blot analysis. Fresh portions of the hippocampus and cerebellum were used to create homogenates for the evaluation of oxidative stress markers.
The tissues of the hippocampus and cerebellum were rinsed with ice and thoroughly cleaned. They were then weighed in an analytical balance after being softly wiped between filter paper folds. A polytron homogenizer was used to prepare 10% of the homogenate at 4°C in 0.05 M phosphate buffer (pH 7). The homogenate was centrifuged at 10,000 rpm for 20 min to separate unbroken cells, mitochondria, erythrocyte nuclei and cell debris. According to the directions in the handbook, the supernatant was aliquoted and kept at −80°C for further analysis of MDA (Barros et al., 2017), NO (Bhidwaria and Ashwlayan, 2017) and GSH (Pires et al., 2014). To estimate their levels, commercial colorimetric kits were used from the Biodiagnostic Company (Cairo, Egypt).
Immunohistochemical localization of LC3, iNOS, and tau protein was performed as previously described. Briefly, 0.03% H2O2 was used to block endogenous peroxidases. The antigens were heated in a microwave for 20 min with sodium citrate buffer (pH 6), and then buffered saline containing 5% bovine serum albumin. Subsequently, sections were incubated with a primary antibody against tau (1:100, ab92676), LC3 (1:300, ab48394), and iNOS (1:2,000, ab283655) for an entire night at 4°C. Following the manufacturer’s instructions, the avidin-biotinylated peroxidase complex (ABC-kit) and DAB substrate (ab64238) were used to detect the response. Finally, hematoxylin was used as a counterstain, and sections were dehydrated in ascending grades of alcohols, cleared in xylene, and mounted (Chen et al., 2010).
To estimate the number of pyramidal cells in the cornu amonnis region 1 (CA1), five randomly spaced H & E-stained hippocampal sections for each rat in each group were examined. Five photomicrographs from each section were used. Then the count of pyramidal neurons was detected in the calibrated area (0.43 mm2) using the Image J program (Version 1.48) and the cell counter plugin. The analysis was done at a magnification of × 200 (Mavroudis et al., 2019).
Five randomly spaced cerebellar sections stained with H & E for each rat in each group were examined. Five photomicrographs from each section were used. The length of the Purkinje cell dendrites (μm) was detected in the calibrated area (area: 0.071 mm2) using the Image J program (Version 1.48). The analysis was done at a magnification of × 400 (Chen H. et al., 2019).
Five randomly spaced sections for each rat in each group were examined. Five photomicrographs from each section were used. The area fraction of immunological expression was calculated using a 40 × objective (area: 0.071 mm2). Immune-positive reaction was analyzed using the Image-j computerized image analysis system (version1.48). Using the color deconvolution plugin and H-DAB vector, three distinct colored images; green, brown, and blue were produced. By calculating area fraction, the DAB pictures (brown in color) were calibrated. The threshold was adjusted for more precision (Helmy et al., 2022).
The expression of p-Tau, Tau, iNOs, and LC3-II/I proteins in the cerebellum was determined using Western blot procedure (Elnagar et al., 2017). Briefly, tissues were homogenized in 250μl pre-cold lysis buffer pH 7.4; 10 mM Tris-Base, 100 mM NaCl, 20 mM Ethylene EGTA, 25 mM EDTA, 2% Triton X-100, and 1:350 protease and phosphatase inhibitor cocktail (Sigma). The tissue homogenates were immediately centrifuged at 12,000 rpm for 15 min. Total proteins were determined using the Pierce 660 nm assay (Thermo Scientific, Rockford, IL). Then, equal amounts (25 μg) of protein were mixed with the loading buffer contains Tris-HCl, dithiothreitol (DTT), sodium dodecyl sulfate (SDS), glycerol, and Bromophenol blue. Protein samples were boiled for 5 min at 95°C, allowed to cool on ice for 5 min, and separated by electrophoresis (Cleaver Scientific Ltd., UK). The proteins on the gel were then electroblotted onto PVDF membranes for 35 min using a Trans-Blot® SD semi-dry transfer cell (Biorad). PVDF membranes were blocked with 5% dry milk (Biorad) in Tris buffered saline supplemented with Tween-20 (TBS-T). The membranes were washed and incubated with antibodies against p-Tau, Tau, iNO, LC3-II/I proteins (1:1,000, Cell Signaling Technology) and β-actin (1:3,000, Sigma) proteins for 13–15 h at 4°C. The membrane blots were incubated with secondary antibodies for 2 h at RT before they visualized with ECL chemiluminescence reagents (Perkin Elmer, USA) for 2 min on the Biorad Chemi-Doc imager, and finally the band intensities were analyzed with ImageLab§ software (Biorad) with normalization to β-actin.
Version 26.0 of IBM SPSS for Windows was used to analyze the data. The Shapiro test of normality results showed that the data were normally distributed. They were labeled as mean ± SD. The significance was assessed at the (0.05) level. The means of the 4 study groups were compared using the one-way ANOVA test and the Post Hoc Games-Howell test was used to identify pairwise comparisons.
At the end of the experiment, there were significant differences in body weight among the four studied groups (p < 0.001, ANOVA test). There was no significant difference between C and N groups (p = 0.9). Also, the difference between C and AlCl3 + N groups was insignificant (p = 0.1). AlCl3 administration resulted in a significant drop in the body weight as compared to both C and N groups (p < 0.001). Co-administration of naringin with AlCl3 resulted in a significant increase in body weight as compared to AlCl3 group (p = 0.006) (Table 1).
During initial training periods, there were no significant differences among rats in the different studied groups regarding the time required to reach the platform (escape latency) (p = 0.3) or the time spent on the rod (grip period) (p = 0.2) (ANOVA test) (Figures 1, 2). After that, AlCl3-treated rats spent less time on the rotarod (grip period) (p < 0.001) and more time to enter the platform quadrant (entry latency) (p < 0.001) when compared with AlCl3 + N group (Figures 3, 4).
Figure 1. Grip period in the Rotarod test during initial training periods in the studied groups. n = 6 in each group. Data were prescribed as mean ± SD. No significant differences were detected between any groups (p > 0.05, Games-Howell post-hoc test).
Figure 2. Escape latency in the Morris water maze test during initial training periods in the studied groups. n = 6 in each group. Data were prescribed as mean ± SD. No significant differences were detected between any groups (p > 0.05, Games-Howell post-hoc test).
Figure 3. Grip period in the Rotarod test in the studied groups. n = 6 in each group. Data were prescribed as mean ± SD. The intergroup variation was conducted by Games-Howell post-hoc test. *p < 0.001 compared with C group. #p < 0.001 compared with AlCl3. $p = 0.01 compared with AlCl3.
Figure 4. Entry latency in the Morris water maze test in the studied groups. n = 6 in each group. Data were prescribed as mean ± SD. The intergroup variation was conducted by Games-Howell post-hoc test. *p < 0.001 compared with C group. #p < 0.001 compared with AlCl3. $p < 0.001 compared with AlCl3.
Compared to C group, AlCl3 group showed a significant increase in hippocampus MDA, NO, and a significant drop in GSH levels (p < 0.001, p = 0.002, p < 0.001, respectively). Hippocampal MDA and NO levels significantly decreased after NAR administration, while the hippocampal GSH level was significantly increased (Table 2).
Table 2. Effects of naringin administration on MDA, NO, and GSH levels in hippocampal and cerebellar homogenates.
Furthermore, compared to C group, AlCl3 group generated a significant increase in cerebellar MDA, NO, and a significant decrease in GSH levels. When NAR and AlCl3 were administered together, the levels of MDA and NO decreased (p = 0.004, p < 0.001), respectively and GSH level increased significantly in the cerebellum (p = 0.01) than they were in AlCl3 group (Table 2).
Pathological examination of hematoxylin and eosin-stained hippocampal sections was performed to verify the neurochemical findings. Sections of C and N groups showed the pyramidal cell layer, which is composed of tiny pyramidal neurons with large vesicular nuclei and visible nucleoli, as well as the typical architecture of the hippocampal tissue (Figures 5A,B). Most pyramidal neurons in AlCl3 group appeared deeply stained with pyknotic nuclei (Figure 5C). On the other hand, most of pyramidal neurons in AlCl3 + N group appeared normal, with large vesicular nuclei and visible nucleoli. However, some pyramidal neurons had deeply stained pyknotic nuclei (Figure 5D).
Figure 5. H & E-stained hippocampal sections revealing the cornu amonnis region 1 (CA1): (A,B) C and N groups, respectively showed its pyramidal cell layer formed of small pyramidal neurons with large vesicular nuclei and prominent nucleoli (arrows). (C) AlCl3 group showed that most of the pyramidal neurons appeared to be deeply stained with pyknotic nuclei (dotted arrows). (D) The group treated with both AlCl3 and N showed that most of pyramidal neurons appeared normal with large vesicular nuclei and prominent nucleoli (arrows). However, some pyramidal neurons appeared deeply stained with pyknotic nuclei (dotted arrows) (H & E, × 200). (E) Pyramidal cell count of all studied groups. n = 6 in each group. Data were prescribed as mean ± SD. The intergroup variation was conducted by Games-Howell post-hoc test. *p < 0.001 compared with C group. #p = 0.004 compared with AlCl3. $p < 0.001 compared with AlCl3.
The three layers of the cerebellar cortex; the molecular layer, the Purkinje cell layer and the granular layer were seen in the cerebellar sections of C and N groups. Purkinje cells were pyriform in shape and had apical dendrites that extended upwards into the molecular layer (Figures 6A,B). The morphology of Purkinje cells was deformed and they lost their apical dendrites in AlCl3 group (Figure 6C). In AlCl3 + N group, most of Purkinje cells restored their pyriform shape and apical dendrites. Furthermore, there were empty spaces that indicated neuronal degeneration (Figure 6D).
Figure 6. Cerebellar sections stained with H & E-stained cerebellar sections of the studied groups: (A,B) C and N groups, respectively, showed the three layers of the cerebellar cortex: molecular layer (M), Purkinje cell layer (arrows) and granular layer (G). Purkinje cells appeared pyriform in shape, with apical dendrite projecting upwards in the molecular layer. (C) The AlCl3 group showed normal appearance of both molecular (M) and granular (G) layers. Purkinje cells had a distorted shape with lost apical dendrites (arrows). (D) The AlCl3 + N group showed normal appearance of the molecular (M) and granular (G) layers. Most of the Purkinje cells appeared to be normal in shape having apical dendrites (arrows). However, some of the Purkinje cells had a distorted shape with the loss of their apical dendrites (dotted arrows). Also, there were empty spaces (*) indicating neuronal degeneration (H & E, × 400). (E) Length of the Purkinje cell dendrites of all studied groups. n = 6 in each group. Data were prescribed as mean ± SD. The intergroup variation was conducted by Games-Howell post-hoc test. *p < 0.001 compared with C group. #p < 0.001 compared with AlCl3. $p < 0.001 compared with AlCl3.
C group showed weak negative tau reaction in the Purkinje cells (Figure 7A). Rats treated with NAR appeared as having a lower level of anti-tau antibodies than C group (Figure 7B). AlCl3 group revealed strong positive tau immunoreaction in the majority of Purkinje and granule cells (Figure 7C). Unlike AlCl3 group, some Purkinje and granule cells in AlCl3 + N group had weak positive tau immunoreaction (Figure 7D).
Figure 7. Immunohistochemical detection of cerebellar tau protein: (A) The control rat showed weak positive tau immunoreaction in a Purkinje cell (arrow). (B) The rat showed negative tau immunoreaction. (C) AlCl3 group showed a strong positive tau immunoreaction in most Purkinje and granule cells (arrows). (D) The rat treated with combined AlCl3 and N showed weak positive tau immunoreaction in some Purkinje and granule cells (arrows) (Tau × 400). (E) Area% of tau-positive immune reaction in cerebellar sections of all studied groups. n = 6 in each group. Data were prescribed as mean ± SD. The intergroup variation was conducted by Games-Howell post-hoc test. *p < 0.001 compared with C group. #p < 0.001 compared with AlCl3. $p < 0.001 compared with AlCl3.
Cerebellar sections were immunostained with iNOS to see if the neuroprotective effect of naringin was related to reduced oxidative stress or not. Both C and N groups showed negative iNOS reaction (Figures 8A,B). AlCl3 group showed that most of the Purkinje cells displayed strong positive iNOS immunostaining (Figure 8C). Unlike AlCl3 group, some Purkinje cells in AlCl3 + N group showed weak positive iNOS immunostaining (Figure 8D).
Figure 8. Immunohistochemical detection of cerebellar iNOS (A,B) C and N groups, respectively showed negative iNOS immunostaining. (C) AlCl3 group showed strong positive iNOS immunostaining in most Purkinje cells (arrows). (D) AlCl3 + N group showed weak positive immunostaining for iNOS in some Purkinje cells (arrows) (iNOS × 400). (E) Area% of iNOS positive immune reaction in cerebellar sections of all studied groups. n = 6 in each group. Data were prescribed as mean ± SD. The intergroup variation was conducted by Games-Howell post-hoc test. *p < 0.001 compared with C group. #p < 0.001 compared with AlCl3. $p < 0.001 compared with AlCl3.
To explore whether the neuroprotective effect of naringin was associated with autophagy, the changes of the autophagic vacuoles and the autophagic substrate were studied. Both C and N groups showed strong positive LC3 reaction (Figures 9A,B). Immunohistochemical analysis of AlCl3 group showed negative LC3 immunostaining (Figure 9C). Moreover, AlCl3 + N group showed positive LC3 reaction (Figure 9D).
Figure 9. Immunohistochemical detection of cerebellar LC3: (A,B) C and N groups, respectively showed strong positive LC3 immunostaining in most of the Purkinje cells (arrows). (C) AlCl3 group showed strong negative LC3 immunostaining. (D) AlCl3 + N treated group showed weak positive immunostaining for LC3 in some Purkinje cells (arrows) (LC3 × 400). (E) Area% of LC3 positive immune reaction in cerebellar sections of all studied groups. n = 6 in each group. Data were prescribed as mean ± SD. The intergroup variation was conducted by Games-Howell post-hoc test. *p = 0.008 compared with C group. #p < 0.001 compared with AlCl3. $p < 0.001 compared with AlCl3.
Alcl3 group showed a significant reduction in the hippocampal pyramidal cell count compared to both C and N groups. Co-administration of NAR resulted in a significant increase in the pyramidal cell count as compared to AlCl3 group (p < 0.001) (Figure 5E).
The length of the dendrites of cerebellar Purkinje cells was significantly decreased in AlCl3 group as compared to both C and N groups. AlCl3 + N group showed a significant increase in dendritic length as compared to AlCl3 group (p < 0.001) (Figure 6E).
AlCl3 group showed a significant increase in the% area of tau immunoreaction as compared to both C and N groups (p < 0.001). NAR co-administration for 21 days showed a significant reduction in the% of area of positive tau reaction as compared to AlCl3 group (Figure 7E).
Regarding iNOS, AlCl3 group revealed a substantial increase in iNOS immunostaining as compared to both C and N groups (p < 0.001). NAR administration for 21 days demonstrated a significant decrease in iNOS immunostaining as compared to AlCl3 group (Figure 8E).
Regarding LC3, AlCl3 group revealed that the LC3 immunostaining was significantly reduced (p < 0.001) as compared to both C and N groups. Furthermore, LC3 immunostaining was significantly increased after NAR co-administration when compared with AlCl3 group (Figure 9E).
Compared to C group, AlCl3 group showed a significant increase in p-Tau (Ser-396/404) and p-Tau/Tau and decreased total Tau levels. When NAR and AlCl3 were administered together, the expression of p-Tau (Ser-396/404) and p-Tau/Au decreased, while overall tau levels increased significantly when compared to AlCl3 group (Figure 10).
Figure 10. Western blot analysis. (A) Representative Western blot images of cerebellar tau, iNOS, and LC3 proteins. (B) Quantification of the proteins studied in cerebellar tissue lysates. n = 3 in each group. The control group is set to “1,” and data were prescribed as mean ± SD. The intergroup variation was conducted by Games-Howell post-hoc test. *Significance compared with C group. #Significance compared with AlCl3. $Significance compared with AlCl3.
When NAR and AlCl3 were administered together, iNOS expression was significantly reduced as compared to AlCl3 group. AlCl3, on the other hand, significantly increased the expression of iNOS as compared to C group (p = 0.001) (Table 3).
Table 3. Effects of naringin administration on the expression of cerebellar LC3, tau and iNOS proteins by Western blot analysis.
Combined administration of NAR and AlCl3 resulted in a significantly higher expression of the LC3-i, LC3-ii, and LC3-ii/LC3-i ratio when compared with AlCl3 group. On the other hand, it significantly reduced the expression of LC3-ii and the LC3-ii/LC3-i ratio as compared to C group (p = 0.001) (Table 3).
A graphical abstract was designed to summarize all results of the current study (Figure 11).
Figure 11. Graphical abstract. Naringin improves the cerebellar neurotoxicity induced by AlCl3 evaluated by decrease of the oxidative stress marker (in the form of decreasing the iNOS); decrease the microtubule assembly marker (Tau protein) and increase the autophagy marker (LC3). AlCl3, Aluminum chloride; Inos, Inducible nitric oxide synthase; LC3, Light chain 3; GSH, Reduced glutathione; NO, Nitric oxide and MDA, Malondialdehyde.
The present study was designed to investigate the cerebellar changes in AlCl3 rat model of AD focusing on behavioral, neurochemical, immunohistochemical, and molecular aspects. Furthermore, the neuroprotective role of naringin against AD-related cerebellar changes was estimated.
AD is accompanied by neuronal loss and structural changes (Xiaoguang et al., 2018). Short-term memory impairment is the first clinical sign of AD due to hippocampal neuronal degeneration (Lakshmi et al., 2015). Al is believed to play a role in the development of AD due to its easy entry and accumulation in the central nervous system (Sun et al., 2009). Understanding the pathophysiology in neurodegenerative and neuropsychiatric diseases, particularly AD, has gained considerable interest in this area of research (Jacobs et al., 2018). Um et al. (2022) reviewed an already considerable work on cerebellar neuropathology, structural organization, and functional neuroimaging studies in AD.
According to the findings of the present study, administration of AlCl3 caused a significant decline in learning ability and spatial memory, assessed by the Morris water Maze test. Compared to AlCl3 group, AlCl3 + N group demonstrated a significantly shorter time (p = 0.001) to reach the platform in the Morris water maze in all four quadrants. Also, NAR co-administration improved the retention of memory evaluated by the time needed by rats to enter the platform quadrant. Lakshmi et al. (2015) declared that intracerebral treatment of AlCl3 resulted in learning deficits in rabbits in the Morris water maze test, which was consistent with our findings. This phenomenon could be attributed to the ability of Al to interfere with downstream effector molecules, such as cyclic GMP, involved in long-term memory (Canales et al., 2001). This disturbance could then clarify the memory impairment and neurobehavioral deficits detected. Also, Yang et al. (2014) supported the results of the current study as they mentioned that naringenin (naringin metabolite) can easily cross the blood brain barrier and improve spatial learning and memory in a rat model of AD by regulating the PI3K/AKT/GSK-3 pathway and reducing tau hyperphosphorylation. In addition, naringin improves memory deficits in experimental models of AD by attenuating mitochondrial dysfunction (Sachdeva et al., 2014).
In addition, the current study revealed that there was a significant decrease in the motor abilities and muscle coordination measured by the Rotarod test after AlCl3 administration. According to Wagner et al. (2019), sporadic AD is characterized by cerebellar atrophy, and cerebellar damage is the cause of motor impairment.
Furthermore, these results were in line with those of Chen C. et al. (2019) who found that daily intragastric administration of naringenin for 7 days attenuated the decrease in the time rats remained on the rotarod. In addition, Meng et al. (2021) found that naringin can significantly improve cognitive, learning, and memory dysfunction in mice with hydrocortisone memory impairment. They concluded that naringin exerted these neuroprotective effects through a variety of mechanisms, including amyloid-β metabolism, tau protein hyperphosphorylation, the acetylcholinergic system, the glutamate receptor system, oxidative stress, and cell apoptosis. Also, naringin is a potent antioxidant which is rapidly absorbed into the blood from the intestinal tract and is then further redistributed to other organs, including the brain (Wang et al., 2021). Feng et al. (2018) excluded that naringin can cross the blood brain barrier. Thus, it can protect the brain tissue and modulate brain chemistry.
The current study demonstrated that MDA and NO levels increased significantly as a result of AlCl3-induced AD. According to Liaquat et al. (2019), prolonged exposure to AlCl3 can harm brain DNA, affect brain neurochemistry, and alter antioxidant enzyme activities. Additionally, aluminum toxicity results in marked oxidative stress by raising pro-oxidant iron levels in the brain and decreasing antioxidant enzyme functions (Praticò et al., 2002).
AlCl3 increases the production of free radicals, which eventually causes oxidative stress and neurotoxicity. The brain is particularly susceptible to oxidative stress, which results in toxicity when free radicals increase and the antioxidant status declines (Kumar and Gill, 2014). MDA accumulates as a result of ROS production. It damages and deteriorates membranes by lipid peroxidation (Busch and Binder, 2017). In the same line, current results demonstrated a significant drop in GSH levels in AlCl3 group. Glutathione in its reduced form is the most abundant intracellular antioxidant and is involved in the direct scavenging of free radicals or serving as a substrate for the glutathione peroxidase enzyme that catalyzes H2O2 detoxification. Catalase is also known to be a protective enzyme and functions for the detoxification of highly reactive free radicals (Mačičková et al., 2005).
In the present study, naringin restored reduced glutathione and decreased NO and MDA levels in rats treated with both AlCl3 and NAR. Liaquat et al. (2018) reported that NAR was found to be the most effective antioxidant of polyphenols. Additionally, it has estrogenic properties, which alter NO generation by activating estrogen receptors (Ghofrani et al., 2015). Furthermore, NAR antioxidant effects modulate oxidative stress and inflammatory responses in the adult brain. Its neuroprotective effects are also controlled by induction of neurotrophic factors and activation of anti-apoptotic pathways (Kim et al., 2016).
According to the current work, AlCl3 caused an increase in iNOS expression which was studied at immunohistochemical and molecular levels in the cerebellar tissue, both of which were alleviated by NAR administration. In agreement with these findings, Dou et al. (2013) showed that in many forms of inflammation, naringenin has also been demonstrated to suppress iNOS expression. Furthermore, in mice whose brains had been exposed to 1-methyl-4-phenyl-1, 2, 3, 6-tetrahydropyridine (MPTP), pretreatment with naringenin reduced the degree of iNOS expression (Sugumar et al., 2019).
Histopathological studies were performed on the rat hippocampus to assess the morphological changes that confirmed the establishment of AD. Hippocampal neuronal degeneration was detected in the form of dark stained nuclei and increased pyramidal cell degeneration with noticeable neuronal distortion. These findings were confirmed by quantitative measurement of the hippocampal pyramidal cell count. The count was significantly reduced in AlCl3 group as compared to AlCl3 + N group (p < 0.001). Chen et al. (2018) reported similar histological alterations like hippocampal neuronal degeneration as one of the main pathological hallmarks of AD. Furthermore, Haider et al. (2020) showed that pretreatment with naringin greatly inhibited AlCl3-caused Histological changes.
In the current study, Histological examination of cerebellar sections revealed that most of Purkinje cells in rats treated with AlCl3 had deformed shapes and lost their apical dendrites. These changes improved in AlCl3 + N group. In addition, AlCl3-treated rats had shorter dendritic length when compared with AlCl3 + N group (p < 0.001). These neuropathological and morphological alterations were in agreement with Baloyannis et al. (2000) who revealed reduced Purkinje cell densities and significant morphological alterations in AD, such as loss of distal dendritic segments, lower dendritic densities and fewer dendritic branches and spines. Furthermore, the pattern of neurodegeneration in the cerebellum in AD may be explained by the dissemination of neurotoxic chemicals through neuronal pathways that connect dispersed nodes to functional modules through self-propagation or prion-like mechanisms (Mavroudis et al., 2019).
In the current study, the cerebellum of rats treated with AlCl3 had significantly higher levels of expression of p-Tau and p-Tau/Tau and the levels decreased significantly after receiving NAR. In the normal brain, tau binds to microtubules to stabilize them and accelerate axonal transport (Kontaxi et al., 2017). However, tau is hyperphosphorylated in AD, which causes it to separate from microtubules and assemble in the paired helical filaments and dystrophic neurites (Spillantini and Goedert, 2013). These results were in agreement with Ballaed et al. (2011) who found that activation of phosphokinase glycogen synthase kinase-3 (GSK-3) contributes to tau hyperphosphorylation of tau and is linked to the suppression of the PI3K/AKT pathway. Interestingly, the administration of naringin improved AD by lowering tau hyperphosphorylation (Yang et al., 2014).
For evaluating autophagy, the current work studied the expression of the LC3-II/I protein in cerebellar tissues using Western blot method. Furthermore, cerebellar sections were immunostained with LC3. Immunohistochemical examination of the cerebellum of AlCl3-treated rats revealed strong negative immunostaining of LC3 which became strong positive on NAR intake. These results indicated that AlCl3-induced AD created a state of autophagic stress in the cerebellum. Keller et al. (2004) supported our findings when they explained that autophagic stress generally refers to a relatively sustained imbalance in which the rate of autophagosome formation exceeds the rate of its degradation. In addition, neurons are vulnerable to defective autophagy due to their special features like having fewer lysosomes in distal axons and the axonal autophagosomes must be transported to the cell body to be combined with lysosomes (Cheng et al., 2015).
The current study revealed that the combined administration of NAR and AlCl3 resulted in significant higher expression of the LC3-i, LC3-ii, and LC3-ii/LC3-i ratio as compared to AlCl3-treated rats. These findings were in agreement with Boland et al. (2018) who established AD model in mice and found that the brain exhibits failure of lysosomal proteolysis. Park and Chung (2019) confirmed that there was a strong relationship between defective autophagy and AD pathogenesis. In addition, Jeong et al. (2015) declared that NAR may have beneficial effects in preventing neuronal death through anti-autophagic stress and anti-neuroinflammation in the hippocampus in vivo.
The present study highlights that naringin improves behavioral, neurochemical, immunohistochemical, and molecular parameters in the rat cerebellum of AlCl3-induced AD; these effects may be largely attributed to its antioxidant and autophagic regulatory properties.
To fully understand how naringin affects oxidative and autophagic stress in various experimental circumstances, more research is necessary.
The raw data supporting the conclusions of this article will be made available by the authors, without undue reservation.
Following NIH and EU norms for animal care, this study received Institutional Research Board (IRB) approval from the Faculty of Medicine at Mansoura University (Code number: R.21.03.1280). At the Medical Experimental Research Center (MERC), where the experiment was conducted, the rats were housed under veterinary care. The number of animals used and animal discomfort were both minimized as far as possible.
HH and ME designed the study. HH examined the hippocampal and cerebellar tissue specimens, interpreted the histological and immunohistochemical results, and performed and interpreted the morphometric studies. EA and EE established the study model. MRE and EH performed and interpreted the neurochemical and molecular results. ZA-Q interpreted the results of behavioral tests. EMEN, MA, KA-K, and ZA-Q shared project administration, funding acquisition, investigation, methodology, writing, reviewing, and editing. All authors contributed to the conception of the study, revised the manuscript, and approved the submitted manuscript.
This research was funded by the Deanship of Scientific Research at King Khalid University through a large groups project (research group program) (grant number RGP. 2/143/43).
We express our gratitude to the Mansoura University, Egypt and the King Khalid University, Saudi Arabia, for providing administrative and technical support.
The authors declare that the research was conducted in the absence of any commercial or financial relationships that could be construed as a potential conflict of interest.
The reviewer AE-k declared a shared affiliation with the authors EMEN, MA, and ZA-Q to the handling editor at the time of review.
All claims expressed in this article are solely those of the authors and do not necessarily represent those of their affiliated organizations, or those of the publisher, the editors and the reviewers. Any product that may be evaluated in this article, or claim that may be made by its manufacturer, is not guaranteed or endorsed by the publisher.
Akbarpour, T., Shamsi, M., and Daneshvar, S. (2015). “Extraction of brain regions affected by Alzheimer disease via fusion of brain multispectral MR images,” in Proceedings of the 2015 7th conference on information and knowledge technology (IKT) (Urmia: IEEE), 1–6. doi: 10.1109/IKT.2015.7288773
Ataie, A., Sabetkasaei, M., Haghparast, A., Moghaddam, A. H., Ataee, R., and Moghaddam, S. N. (2010). Curcumin exerts neuroprotective effects against homocysteine intracerebroventricular injection-induced cognitive impairment and oxidative stress in rat brain. J. Med. Food 13, 821–826. doi: 10.1089/jmf.2009.1278
Babri, S., Mohaddes, G., Feizi, I., Mohammadnia, A., Niapour, A., Alihemmati, A., et al. (2014). Effect of troxerutin on synaptic plasticity of hippocampal dentate gyrus neurons in a β-amyloid model of Alzheimer’s disease: An electrophysiological study. Eur. J. Pharmacol. 732, 19–25. doi: 10.1016/j.ejphar.2014.03.018
Ballaed, C., Gauthier, S., Corbett, A., Brayne, C., Aarsland, D., and Jones, E. (2011). Alzheimer’s disease. Lancet 377, 1019–1031. doi: 10.1016/S0140-6736(10)61349-9
Baloyannis, S. J., Manolidis, S. L., and Manolidis, L. S. (2000). Synaptic alterations in the vestibulocerebellar system in Alzheimer’s disease-a Golgi and electron microscope study. Acta Otolaryngol. 120, 247–250. doi: 10.1080/000164800750001026
Barros, A. S., Crispim, R. Y. G., Cavalcanti, J. U., Souza, R. B., Lemos, J. C., Cristino Filho, G., et al. (2017). Impact of the chronic omega-3 fatty acids supplementation in Hemiparkinsonism model induced by 6-hydroxydopamine in rats. Basic Clin. Pharmacol. Toxicol. 120, 523–531. doi: 10.1111/bcpt.12713
Basli, A., Soulet, S., Chaher, N., Mérillon, J. M., Chibane, M., Monti, J. P., et al. (2012). Wine polyphenols: Potential agents in neuroprotection. Oxid. Med. Cell. Longev. 2012:805762. doi: 10.1155/2012/805762
Bensalem, J., Dal-Pan, A., Gillard, E., Calon, F., and Pallet, V. (2015). Protective effects of berry polyphenols against age-related cognitive impairment. Nutr. Aging 3, 89–106. doi: 10.1007/978-3-030-42667-5_7
Bhidwaria, S., and Ashwlayan, V. D. (2017). Neuroprotective effect of Brasicca olarecae L. Var. Botrytis (Brassicaceae) flowers extract on memory deficit in aged & young rats. Glob. J. Pharm. Pharm. Sci. 3, 105–114. doi: 10.19080/GJPPS.2017.03.555620
Boland, B., Yu, W. H., Corti, O., Mollereau, B., Henriques, A., Bezard, E., et al. (2018). Promoting the clearance of neurotoxic proteins in neurodegenerative disorders of ageing. Nat. Rev. Drug Discov. 17, 660–688.
Bonda, D. J., Wang, X., Perry, G., Nunomura, A., Tabaton, M., Zhu, X., et al. (2010). Oxidative stress in Alzheimer disease: A possibility for prevention. Neuropharmacology 59, 290–294. doi: 10.1016/j.neuropharm.2010.04.005
Bondy, S. C. (2016). Low levels of aluminum can lead to behavioral and morphological changes associated with Alzheimer’s disease and age-related neurodegeneration. Neurotoxicology 52, 222–229. doi: 10.1016/j.neuro.2015.12.002
Borai, I. H., Ezz, M. K., Rizk, M. Z., Aly, H. F., El-Sherbiny, M., Matloub, A. A., et al. (2017). Therapeutic impact of grape leaves polyphenols on certain biochemical and neurological markers in AlCl3-induced Alzheimer’s disease. Biomed. Pharmacother. 93, 837–851. doi: 10.1016/j.biopha.2017.07.038
Busch, C. J., and Binder, C. J. (2017). Malondialdehyde epitopes as mediators of sterile inflammation. Biochim. Biophy. Acta Mol. Cell Biol. Lipids 1862, 398–406. doi: 10.1016/j.bbalip.2016.06.016
Canales, J. J., Corbalán, R., Montoliu, C., Llansola, M., Monfort, P., Erceg, S., et al. (2001). Aluminium impairs the glutamate-nitric oxide-cGMP pathway in cultured neurons and in rat brain in vivo: Molecular mechanisms and implications for neuropathology. J. Inorg. Biochem. 87, 63–69. doi: 10.1016/s0162-0134(01)00316-6
Cao, W., Feng, S. J., and Kan, M. C. (2021). Naringin targets NFKB1 to alleviate oxygen-glucose deprivation/reoxygenation–induced injury in PC12 cells via modulating HIF-1α/AKT/mTOR-signaling pathway. J. Mol. Neurosci. 71, 101–111. doi: 10.1007/s12031-020-01630-8
Chen, C., Wei, Y. Z., He, X. M., Li, D. D., Wang, G. Q., Li, J. J., et al. (2019). Naringenin produces neuroprotection against LPS-induced dopamine neurotoxicity via the inhibition of microglial NLRP3 inflammasome activation. Front. Immunol. 10:936. doi: 10.3389/fimmu.2019.00936
Chen, H., Cheng, R., Zhao, X., Zhang, Y., Tam, A., Yan, Y., et al. (2019). An injectable self-healing coordinative hydrogel with antibacterial and angiogenic properties for diabetic skin wound repair. NPG Asia Mater. 11, 1–12.
Chen, P., Chen, F., and Zhou, B. (2018). Antioxidative, anti-inflammatory and anti-apoptotic effects of ellagic acid in liver and brain of rats treated by D-galactose. Sci. Rep. 8:1865.
Chen, R., Qi, Q. L., Wang, M. T., and Li, Q. Y. (2016). Therapeutic potential of naringin: An overview. Pharm. Biol. 54, 3203–3210. doi: 10.1080/13880209.2016.1216131
Chen, X., Cho, D. B., and Yang, P. C. (2010). Double staining immunohistochemistry. N. Am. J. Med. Sci. 2, 241–245.
Cheng, X. T., Zhou, B., Lin, M. Y., Cai, Q., and Sheng, Z. H. (2015). Axonal autophagosomes recruit dynein for retrograde transport through fusion with late endosomes. J. Cell Biol. 209, 377–386. doi: 10.1083/jcb.201412046
Colomina, M. T., and Peris-Sampedro, F. (2017). Aluminum and Alzheimer’s disease. Neurotox. Met. 18, 183–197. doi: 10.1007/978-3-319-60189-2_9
Dou, W., Zhang, J., Sun, A., Zhang, E., Ding, L., Mukherjee, S., et al. (2013). Protective effect of naringenin against experimental colitis via suppression of toll-like receptor 4/NF-κB signalling. Br. J. Nutr. 110, 599–608. doi: 10.1017/S0007114512005594
Elnagar, M. R., Walls, A. B., Helal, G. K., Hamada, F. M., Thomsen, M. S., and Jensen, A. A. (2017). Probing the putative α7 nAChR/NMDAR complex in human and murine cortex and hippocampus: Different degrees of complex formation in healthy and Alzheimer brain tissue. PLoS One 12:e0189513. doi: 10.1371/journal.pone.0189513
Farrag, E. A., Abdelrazikb, E., Abdallahc, Z., and Hassan, H. M. (2021). Vitamin E attenuates cardiomyopathy via alleviation of autophagic stress. Int. J. Sci. Basic Appl. Res. 60, 67–86.
Feng, J., Chen, X., Lu, S., Li, W., Yang, D., Su, W., et al. (2018). Naringin attenuates cerebral ischemia-reperfusion injury through inhibiting peroxynitrite-mediated mitophagy activation. Mol. Neurobiol. 55, 9029–9042. doi: 10.1007/s12035-018-1027-7
Gella, A., and Durany, N. (2009). Oxidative stress in Alzheimer disease. Cell Adhes. Migr. 3, 88–93.
Ghofrani, S., Joghataei, M. T., Mohseni, S., Baluchnejadmojarad, T., Bagheri, M., Khamse, S., et al. (2015). Naringenin improves learning and memory in an Alzheimer’s disease rat model: Insights into the underlying mechanisms. Eur. J. Pharmacol. 764, 195–201. doi: 10.1016/j.ejphar.2015.07.001
Haider, S., Liaquat, L., Ahmad, S., Batool, Z., Siddiqui, R. A., Tabassum, S., et al. (2020). Naringenin protects AlCl3/D-galactose induced neurotoxicity in rat model of AD via attenuation of acetylcholinesterase levels and inhibition of oxidative stress. PLoS One 15:e0227631. doi: 10.1371/journal.pone.0227631
Helmy, M. A., Hassan, H. M., Elsherbeny, M., Ali, F., Mhanna, R. M., and Taalab, Y. M. (2022). Toxic effects of aramadol abuse and withdrawal on histopathological structure of tongue in Sprague Dawley rats. Zagazig J. For. Med. 20, 67–81.
Jacobs, H. I., Hopkins, D. A., Mayrhofer, H. C., Bruner, E., van Leeuwen, F. W., Raaijmakers, W., et al. (2018). The cerebellum in Alzheimer’s disease: Evaluating its role in cognitive decline. Brain 141, 37–47.
Jeong, K. H., Jung, U. J., and Kim, S. R. (2015). Naringin attenuates autophagic stress and neuroinflammation in kainic acid-treated hippocampus in vivo. Evid. Based Complement. Alternat. Med. 2015:354326. doi: 10.1155/2015/354326
Keller, J. N., Dimayuga, E., Chen, Q., Thorpe, J., Gee, J., and Ding, Q. (2004). Autophagy, proteasomes, lipofuscin, and oxidative stress in the aging brain. Int. J. Biochem. Cell Biol. 36, 2376–2391. doi: 10.1016/j.biocel.2004.05.003
Kim, H. D., Jeong, K. H., Jung, U. J., and Kim, S. R. (2016). Naringin treatment induces neuroprotective effects in a mouse model of Parkinson’s disease in vivo, but not enough to restore the lesioned dopaminergic system. J. Nutr. Biochem. 28, 140–146. doi: 10.1016/j.jnutbio.2015.10.013
Kolarova, M., García-Sierra, F., Bartos, A., Ricny, J., and Ripova, D. (2012). Structure and pathology of tau protein in Alzheimer disease. Int. J. Alzheimers Dis. 2012:731526.
Kontaxi, C., Piccardo, P., and Gill, A. C. (2017). Lysine-directed post-translational modifications of tau protein in Alzheimer’s disease and related tauopathies. Front. Mol. Biosci. 4:56. doi: 10.3389/fmolb.2017.00056
Kumar, A., Prakash, A., and Dogra, S. (2011). Neuroprotective effect of carvedilol against aluminium induced toxicity: Possible behavioral and biochemical alterations in rats. Pharmacol. Rep. 63, 915–923.
Kumar, V., and Gill, K. D. (2014). Oxidative stress and mitochondrial dysfunction in aluminium neurotoxicity and its amelioration: A review. Neurotoxicology 41, 154–166. doi: 10.1016/j.neuro.2014.02.004
Lakshmi, B. V. S., Sudhakar, M., and Prakash, K. S. (2015). Protective effect of selenium against aluminum chloride-induced Alzheimer’s disease: Behavioral and biochemical alterations in rats. Biol. Trace Elem. Res. 165, 67–74. doi: 10.1007/s12011-015-0229-3
Laskowitz, D. T., Wang, H., Chen, T., Lubkin, D. T., Cantillana, V., Tu, T. M., et al. (2017). Neuroprotective pentapeptide CN-105 is associated with reduced sterile inflammation and improved functional outcomes in a traumatic brain injury murine model. Sci. Rep. 7:46461. doi: 10.1038/srep46461
Liaquat, L., Batool, Z., Sadir, S., Rafiq, S., Shahzad, S., Perveen, T., et al. (2018). Naringenin-induced enhanced antioxidant defence system meliorates cholinergic neurotransmission and consolidates memory in male rats. Life Sci. 194, 213–223. doi: 10.1016/j.lfs.2017.12.034
Liaquat, L., Sadir, S., Batool, Z., Tabassum, S., Shahzad, S., Afzal, A., et al. (2019). Acute aluminum chloride toxicity revisited: Study on DNA damage and histopathological, biochemical and neurochemical alterations in rat brain. Life Sci. 217, 202–211. doi: 10.1016/j.lfs.2018.12.009
Mačičková, T., Pečivová, J., Nosáĺ, R., and Holomáňová, D. (2005). Influence of carvedilol on superoxide generation and enzyme release from stimulated human neutrophils. Biomed. Pap. Med. Fac. Univ. Palacky Olomouc Czech. Repub. 149, 389–392.
Mavroudis, I., Petridis, F., Kazis, D., Njau, S. N., Costa, V., and Baloyannis, S. J. (2019). Purkinje cells pathology in Alzheimer’s disease. Am. J. Alzheimers Dis. Other Dement. 34, 439–449.
Meng, X., Fu, M., Wang, S., Chen, W., Wang, J., and Zhang, N. (2021). Naringin ameliorates memory deficits and exerts neuroprotective effects in a mouse model of Alzheimer’s disease by regulating multiple metabolic pathways. Mol. Med. Rep. 23, 1–13. doi: 10.3892/mmr.2021.11971
Park, E., and Chung, S. W. (2019). ROS-mediated autophagy increases intracellular iron levels and ferroptosis by ferritin and transferrin receptor regulation. Cell Death Dis. 10, 1–10. doi: 10.1038/s41419-019-2064-5
Pires, L. F., Costa, L. M., de Almeida, A. A. C., Silva, O. A., Cerqueira, G. S., de Sousa, D. P., et al. (2014). Is there a correlation between in vitro antioxidant potential and in vivo effect of carvacryl acetate against oxidative stress in mice hippocampus? Neurochem. Res. 39, 758–769. doi: 10.1007/s11064-014-1267-5
Praticò, D., Uryu, K., Sung, S., Tang, S., Trojanowski, J. Q., and Lee, V. M. Y. (2002). Aluminum modulates brain amyloidosis through oxidative stress in APP transgenic mice. FASEB J. 16, 1138–1140. doi: 10.1096/fj.02-0012fje
Sachdeva, A. K., Kuhad, A., and Chopra, K. (2014). Naringin ameliorates memory deficits in experimental paradigm of Alzheimer’s disease by attenuating mitochondrial dysfunction. Pharmacol. Biochem. Behav. 127, 101–110. doi: 10.1016/j.pbb.2014.11.002
Schmahmann, J. D. (2019). The cerebellum and cognition. Neurosci. Lett. 688, 62–75. doi: 10.1016/j.neulet.2018.07.005
Spillantini, M. G., and Goedert, M. (2013). Tau pathology and neurodegeneration. Lancet Neurol. 12, 609–622.
Sugumar, M., Sevanan, M., and Sekar, S. (2019). Neuroprotective effect of naringenin against MPTP-induced oxidative stress. Int. J. Neurosci. 129, 534–539. doi: 10.1080/00207454.2018.1545772
Sun, Z. Z., Chen, Z. B., Jiang, H., Li, L. L., Li, E. G., and Xu, Y. (2009). Alteration of Aβ metabolism-related molecules in predementia induced by AlCl3 and d-galactose. Age 31, 277–284. doi: 10.1007/s11357-009-9099-y
Um, Y. H., Wang, S. M., Kang, D. W., Kim, N. Y., and Lim, H. K. (2022). Subcortical and cerebellar neural correlates of prodromal Alzheimer’s disease with prolonged sleep latency. J. Alzheimers Dis. 86, 1–14. doi: 10.3233/JAD-215460
Wagner, J. M., Sichler, M. E., Schleicher, E. M., Franke, T. N., Irwin, C., Löw, M. J., et al. (2019). Analysis of motor function in the Tg4-42 mouse model of Alzheimer’s disease. Front. Behav. Neurosci. 13:107. doi: 10.3389/fnbeh.2019.00107
Wang, D. M., Yang, Y. J., Zhang, L., Zhang, X., Guan, F. F., and Zhang, L. F. (2013). Naringin enhances CaMKII activity and improves long-term memory in a mouse model of Alzheimer’s disease. Int. J. Mol. Sci. 14, 5576–5586. doi: 10.3390/ijms14035576
Wang, M. H., Yang, C. C., Tseng, H. C., Fang, C. H., Lin, Y. W., and Soung, H. S. (2021). Naringin ameliorates haloperidol-induced neurotoxicity and orofacial dyskinesia in a rat model of human tardive dyskinesia. Neurotox. Res. 39, 774–786. doi: 10.1007/s12640-021-00333-1
Xiaoguang, W., Jianjun, C., Qinying, C., Hui, Z., Lukun, Y., and Yazhen, S. (2018). Establishment of a valuable mimic of Alzheimer’s Disease in rat animal model by intracerebroventricular injection of composited amyloid Beta Protein. JoVE (J. Vis. Exp.) e56157. doi: 10.3791/56157
Yang, W., Ma, J., Liu, Z., Lu, Y., Hu, B., and Yu, H. (2014). Effect of naringenin on brain insulin signaling and cognitive functions in ICV-STZ induced dementia model of rats. Neurol. Sci. 35, 741–751. doi: 10.1007/s10072-013-1594-3
Zhang, J., Zhen, Y. F., Song, L. G., Kong, W. N., Shao, T. M., Li, X., et al. (2013). Salidroside attenuates beta amyloid-induced cognitive deficits via modulating oxidative stress and inflammatory mediators in rat hippocampus. Behav. Brain Res. 244, 70–81. doi: 10.1016/j.bbr.2013.01.037
Keywords: naringin, autophagy, cerebellum, Alzheimer’s disease, oxidative stress, aluminum chloride
Citation: Hassan HM, Elnagar MR, Abdelrazik E, Mahdi MR, Hamza E, Elattar EM, ElNashar EM, Alghamdi MA, Al-Qahtani Z, Al-Khater KM, Aldahhan RA and ELdesoqui M (2022) Neuroprotective effect of naringin against cerebellar changes in Alzheimer’s disease through modulation of autophagy, oxidative stress and tau expression: An experimental study. Front. Neuroanat. 16:1012422. doi: 10.3389/fnana.2022.1012422
Received: 05 August 2022; Accepted: 26 September 2022;
Published: 14 October 2022.
Edited by:
Zhiyou Yang, Guangdong Ocean University, ChinaReviewed by:
Aminu Imam, University of Ilorin, NigeriaCopyright © 2022 Hassan, Elnagar, Abdelrazik, Mahdi, Hamza, Elattar, ElNashar, Alghamdi, Al-Qahtani, Al-Khater, Aldahhan and ELdesoqui. This is an open-access article distributed under the terms of the Creative Commons Attribution License (CC BY). The use, distribution or reproduction in other forums is permitted, provided the original author(s) and the copyright owner(s) are credited and that the original publication in this journal is cited, in accordance with accepted academic practice. No use, distribution or reproduction is permitted which does not comply with these terms.
*Correspondence: Hend M. Hassan, SGVuZG1vaGFtbWVkQG1hbnMuZWR1; Eman Mohamed ElNashar, ZW5zaGFyQGtrdS5lZHUuc2E=
†ORCID: Hend M. Hassan, orcid.org/0000-0001-9390-8226; Mohamed R. Elnagar, orcid.org/0000-0003-0434-2369; Eman Mohamed ElNashar, orcid.org/0000-0002-2883-6761; Zainah Al-Qahtani, orcid.org/0000-0002-1024-6376
Disclaimer: All claims expressed in this article are solely those of the authors and do not necessarily represent those of their affiliated organizations, or those of the publisher, the editors and the reviewers. Any product that may be evaluated in this article or claim that may be made by its manufacturer is not guaranteed or endorsed by the publisher.
Research integrity at Frontiers
Learn more about the work of our research integrity team to safeguard the quality of each article we publish.