- 1CERVO Brain Research Center, Quebec City, QC, Canada
- 2Hôpital De L'Enfant-Jésus, CHU de Québec-Université Laval, Quebec City, QC, Canada
- 3Hôpital Fondation Rothschild, Neurochirurgie pédiatrique - Unité Parkinson, Paris, France
- 4Laboratoire d'Anatomie, Université Laval, Quebec City, QC, Canada
The human brainstem harbors neuronal aggregates that ensure the maintenance of several vital functions. It also acts as a major relay structure for the neuronal information that travels between the cerebral cortex, the cerebellum and the spinal cord. As such, this relatively small portion of the human brain houses a multitude of ascending and descending fibers that course among numerous nuclei whose exact boundaries are still uncertain. Such a large number of nuclei and fiber tracts confined to a relatively small and compact brain region imposes upon the brainstem a highly complex cytoarchitectonic organization that still needs to be deciphered. The present work provides a topographic atlas of the human brainstem composed of 45 anatomical plates, each containing a pair of adjacent sections stained with Cresyl Violet and Luxol Fast Blue to help delineating brainstem nuclei and fiber tracts, respectively. The plates, which cover the entire midbrain, pons and medulla oblongata, are composed of equally-spaced sections referenced and aligned parallel to the ponto-mesencephalic junction rather than the fastigium or the obex. This topographic landmark is particularly suitable for neurosurgical interventions aiming at specific nuclei of the mesencephalic tegmentum. In complement, we provide 8 anatomical plates containing adjacent sections stained for choline acetyltransferase and Luxol Fast Blue, taken through the midbrain and the pons. This open access atlas of the human brainstem is intended to assist neuroanatomists, neurosurgeons and neuropathologists in their work.
Introduction
The human brainstem plays a crucial role in the maintenance of vital functions, such as respiratory and cardiovascular activities. Furthermore, it acts as a major relay station between the cerebral cortex, the cerebellum and the spinal cord, as first suggested by the English neurologist Thomas Willis more than 350 years ago (Willis, 1664). Yet, despite its prime importance in the coordination of several basic central nervous system activities, the brainstem is one of the least understood parts of the human brain. The organizational complexity of the brainstem relies, at least in part, from the fact that its houses a multitude of ascending and descending fiber tracts that course among a large number of nuclei whose exact boundaries are still a matter of controversy. Such a large number of nuclei and fiber tracts restricted to a relatively small brain region [the brainstem occupies <3% of the total brain volume (Erbagci et al., 2012)] imposes upon the brainstem a highly complex cytoarchitectonic organization that still poses problems to neuroanatomists, neuropathologists, neurosurgeons, and imaging specialists.
Based on its external appearance along the rostrocaudal axis, the brainstem has traditionally been divided into the midbrain, the pons and the medulla oblongata. However, since the recent discovery of rhomobomeric segmentation based on developmental gene expression, the pons and medulla oblongata are now often referred together as the hindbrain, with the isthmus as its first segment (Watson et al., 2019; Paxinos et al., 2020). Along the dorsoventral axis, the brainstem can be divided into three distinctive parts: (1) a roof plate dorsal to the ventricular system known as the tectum, (2) a central core of cells and fibers beneath the ventricular system known as the tegmentum, and (3) a massive collection of ventrally located fibers derived from the cerebral cortex. The roof plate of the midbrain is represented by the tectum or quadrigeminal plate, consisting of the superior and inferior colliculi. At hindbrain levels, the roof plate is more elaborated and comprises the cerebellum and the tela choroidea, which will not be considered in detail here. The tegmentum of the midbrain and hindbrain contains the brainstem reticular formation or reticular core: a large collection of diffusely distributed cells closely intermingled with fibers that subserve multiple functions, and several more precisely delineated nuclei. The cortically derived ventral fiber system forms the crus cerebri at midbrain levels, one of the principal constituents of the ventral or basilar region at pontine levels, and the pyramids at medullary levels (Parent, 1996).
Some nuclei of the brainstem tegmentum are enriched in dopaminergic, cholinergic, serotoninergic or noradrenergic neurons known to be involved in the control of motor behavior, sleep and waking cycles, as well as various autonomic functions (Parent, 1996). Over the years, many efforts have been made to delineate brainstem nuclei and fiber tracts (Kolliker, 1896; Ziehen, 1903; Jacobsohn, 1909; Riley, 1943). Olszewski and Baxter were the first to provide a comprehensive and detailed description of the cytoarchitecture of the human brainstem illustrated with high-quality photomicrographs and outlined drawings of histological sections taken transversely to the longitudinal axis of the brainstem (Olszewski and Baxter, 1954). Despite the high quality of this work, the fact that no stereotaxic coordinates were provided has led many neurosurgeons to rely on the stereotaxic atlas of the human brain published by Schaltenbrand and Wahren (1977) for their interventions, with the inherent constraint of histological sections being oriented perpendicular to the anterior (ac) and posterior commissural (pc) plane. This coordinate system originally proposed by Talairach (Talairach et al., 1957; Talairach and Szikla, 1967; Talairach and Tournoux, 1988) is not ideally suited for brainstem stereotaxy because landmarks used for stereotaxic coordinates are too distant from regions of interest (Zrinzo et al., 2008; Goetz et al., 2019). The Allen Human Brain Atlas, which uses this sectioning plane, presents an overall view of brainstem structures in relation with the entire human brain by means of Nissl cell stain combined with parvalbumin and SMI-32 immunohistochemical markers (Ding et al., 2016). Efforts have also been deployed to provide brainstem stereotaxic atlases referenced on brainstem landmarks. Afshar et al. (1978), among others, have presented stereotaxic references for the human brainstem and cerebellar nuclei based on the floor of the fourth ventricle, the midline and a plane passing perpendicular to the floor of the fourth ventricle at the level of the fastigium. The stereotaxic atlas of Paxinos and Huang (1995) contains 64 histological sections perpendicular to the long axis of the brainstem, stained for Cresyl Violet and acetylcholine esterase as well as associated diagrams with delineated nuclei and fiber tracts. In their revised version (Paxinos et al., 2020), 159 plates are presented, 31 of which being from a different brain and used to delineate fiber tracts. In this atlas, sections of the brainstem were numbered based on their distance from the obex. More recently, numerous human brainstem descriptions from MRI have been published (Naidich et al., 2007; Deistung et al., 2013; Bianciardi et al., 2015; Tang et al., 2018; Rushmore et al., 2020). Among these, the Duvernoy's non-stereotaxic atlas of the human brainstem correlates transverse histological brainstem sections with corresponding clinical 3T and 9.4T MRI (Naidich et al., 2007) and is still widely used. The recent work of Rushmore and collaborators offers a detailed map of the human brainstem based on MRI dataset composed of 50-micron isotropic voxels from a post-mortem human brain (Rushmore et al., 2020).
In more recent studies (Ferraye et al., 2010; Goetz et al., 2019), the use of a new coordinate system referenced upon the ponto-mesencephalic junction (PMJ, a line that connects the inferior aspect of the quadrigeminal plate posteriorly with the foramen caecum of the interpeduncular fossa anteriorly), the floor of the fourth ventricle and the lateral mesencephalic sulci has been suggested to be more suitable for brainstem stereotaxy. This coordinate system, which refers to mesencephalic landmarks rather than the fastigium or the obex, has been adopted by some research groups (Thevathasan et al., 2011, 2012; Insola et al., 2012). It appears to be particularly suitable for neurosurgical interventions in the mesencephalic reticular formation, mainly because the references used are closer to neurosurgical areas of interest and easy to identify from MRI (Zrinzo et al., 2008). Readers looking for a color atlas of brainstem surgery should refer to the work of Spetzler and collaborators (Spetzler et al., 2017).
Our long-term interest in the basic aspects of the pathogenesis of various neurodegenerative diseases for which patients often present brainstem anomalies or are candidate for brainstem surgical interventions in the case of Parkinson's disease, has led us to produce a topographic atlas of the human brainstem composed of 45 anatomical plates, each containing a pair of adjacent sections stained with Cresyl Violet and Luxol Fast Blue used to delineate brainstem nuclei and fiber tracts, respectively. The plates cover the midbrain, the pons and the medulla oblongata and are composed of transverse sections taken parallel to the PMJ. In complement, we provide eight anatomical plates containing adjacent sections stained for choline acetyltransferase (ChAT) and Luxol Fast Blue taken through the midbrain and the pons. We hope that this open access atlas of the human brainstem will complement the work of our predecessors while providing an additional tool for fundamental and clinical research.
Method
Specimen Preparation
The post-mortem material was gathered from the brain of a 61-year-old woman who died from a pluri-metastatic colorectal cancer with no signs of neurological, neurodegenerative or psychiatric diseases. After a post-mortem delay of 5.5 h, the brain was perfused through the internal carotids and the vertebral arteries with 4 L of cold saline solution (NaCL 0.9% to which 20 units/mL of heparin was added), followed by 6 L of a cold fixative solution containing 4% paraformaldehyde (PFA) diluted in phosphate buffer (PB, 0.1 M, pH 7.4) and 4 L of 4% PFA to which 0.3% of glutaraldehyde was added. We then performed a MRI scan of the head with a 3T Philips Achieva (TE: 17 ms; TR:4000 ms; slice thickness: 500 μm; duration 8 h, see Supplementary Material) before brain extraction. The extracted brain weighed 1,328 g (Figures 1A–F). The brainstem was dissected out and post-fixed by immersion in 4% PFA for 4 days. Tissue samples were obtained from the Human Anatomy Laboratory at Université Laval and kept in the Human Brain Bank of the CERVO research Center. Both Institutions required informed consent before donation of tissues. The Ethics Committee at Université Laval approved the brain collecting procedures, as well as the storage and handling of post-mortem human brain tissues.
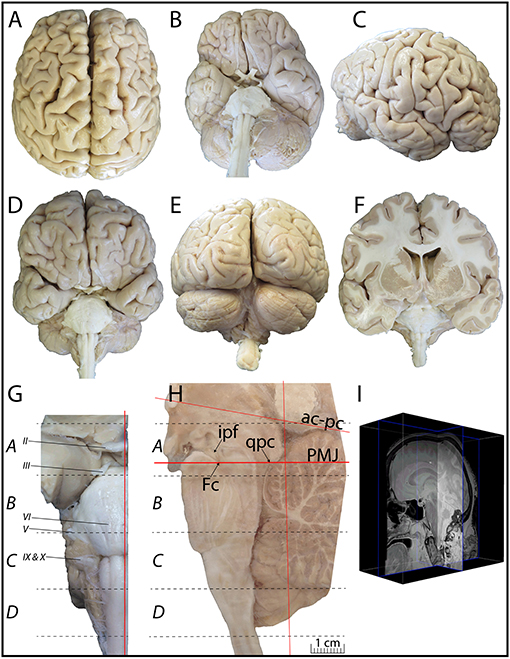
Figure 1. (A–E) Superior (A), inferior (B), lateral (C), anterior (D), and posterior (E) views of the whole brain used. This brain comes from a generous donation of a 61-year-old woman who died from a pluri-metastatic colorectal cancer with no signs of neurological, neurodegenerative or psychiatric diseases. The perfused and extracted brain weighed 1,328 g. (F) Coronal view of the sectioned brain. (G) Picture of the dissected brainstem cut through its longitudinal axis, along a sagittal plane, at 1 mm from the midline. (H) From the right hemi-brainstem containing the midline, four blocks were cut along the ponto-mesencephalic junction plane (PMJ in H). Block A extends from 11.19 to −0.98 mm (plates 1–11), block B from −3.87 to −21.33 mm (plates 12–24), block C from −24.09 to −36.87 mm (plates 25–38), and block D from −39.70 to −51.70 mm (plates 39–45), relative to the PMJ. (I) Brain MRI is available as Supplementary Material.
The brainstem was dissected and cut along a sagittal plane at 1 mm from the midline (Figure 1G). From the right hemi-brainstem that contained the midline, four different blocks were cut along the ponto-mesencephalic junction plane (PMJ), a line that connects the inferior aspect of the quadrigeminal plate (qpc) posteriorly with the foramen caecum (Fc) of the interpeduncular fossa (ipf) anteriorly (Figure 1H). The PMJ is depicted by a red line on the different plates. Its exact position can be found on Figure 1H and on plate 10 (0.00 mm). We estimate that there exists a difference of 10 degrees between the PMJ plane and the ac-pc plane (Figure 1H) and 20 degrees between the PMJ plane and the one used in the atlas of Paxinos (Paxinos et al., 2020). Each of the blocks were then cut at 4°C into 50 μm-thick sections using a vibratome (Leica VT1200S). The sections were all collected serially in phosphate buffer saline (PBS, 0.1 M, pH 7.4). Pairs of adjacent sections were selected out of 22 sections and then processed for Cresyl Violet and Luxol Fast Blue, respectively, providing a mean interval of 1.1 mm between each plate. Because some sections were damaged during the cutting process, in some cases, we decided to choose the next undamaged section instead of the adjacent one, for a maximum of 400 μm interval between Cresyl Violet and Luxol Fast Blue stained sections. Because losses of tissue inevitably occurred between each block, in addition to the known section thickness and intervals, we referred to the MRI in order to confirm the exact position of each section, relative to the PMJ (Figure 1I).
Cresyl Violet Staining
Sections intended for Cresyl Violet staining were first mounted on gelatin-coated microscope slides, air dried at room temperature and incubated overnight in 95% ethanol at 56°C. The slides were then rinsed 15 times in distilled water and immersed for 3 min at room temperature in a pre-heated and filtered solution of Cresyl Violet acetate (catalog no. C5042, Sigma, St-Louis, MO, USA) dissolved at 0.1% in distilled water. Sections were then rinsed in distilled water followed by 10 times in ethanol 95%, two times in a solution of ethanol/acid acetic 0.5%, five times in ethanol 95%. The rinses in ethanol 95% and ethanol/acid acetic were repeated until the desired staining intensity was obtained. Sections were dehydrated in ethanol and coverslipped with Permount (Permount Mounting Medium, catalog no. SP15, Thermo Fisher Scientific, Waltman, MA, USA).
Luxol Fast Blue Staining
Sections intended for Luxol Fast Blue staining were first mounted on gelatin-coated microscope slides, air dried at room temperature and rinsed 10 times in ethanol 95%. They were then incubated overnight at 56°C in Luxol Fast Blue (Solvent Blue 38, catalog no. S3382, Sigma, St-Louis, MO, USA) dissolved at 0.1% in ethanol. They were rinsed once in ethanol 95%, 10 times in lithium carbonate (0.01%) and 10 times in ethanol 70%. When needed, rinses in lithium carbonate (0.01–0.1%) and ethanol were repeated until the desired staining intensity was obtained. Sections were dehydrated in ethanol and coverslipped with Permount. To increase contrast, five sections stained for Luxol Fast Blue had to be also stained with Cresyl Violet (Plates 9, 10, 26, 31, 37).
Choline Acetyltransferase Immunostaining
Eight additional equally-spaced sections taken trough the midbrain and the pons (between +4.88 mm and −10.59 mm) were stained for choline acetyltransferase (ChAT), the enzyme responsible for the synthesis of acetylcholine (plates A-H). After three rinses in PBS, the sections were placed for 20 min at room temperature in hydrogen peroxide (3% H2O2 diluted in ethanol) to eliminate endogenous peroxidase activity. The free-floating sections were then placed in sodium borohydride (0.5% diluted in PBS) for 30 min. After three rinses in PBS, the sections were preincubated for 1 h at room temperature in a PBS solution containing 2% normal rabbit serum and 0.5% Triton X-100, and incubated 48 h at 4°C in the same solution to which the goat anti-ChAT antibody was added (catalog no. AB144P, EMD Milipore Corporation, Billerica, MA, 1:20). The sections were then rinsed, reincubated for 1 h at room temperature with a rabbit anti-goat biotinylated IgG (catalog # BA-5000; Vector Labs, Burlingame, CA, USA; 1:200). After three rinses in PBS, the sections were reincubated for 1 h at room temperature in 2% avidin-biotin-peroxidase complex (catalog # PK-4000; Vector Labs). The bound peroxidase was revealed by placing the sections in a medium containing 0.05% 3,3′-diaminobenzidine tetrahydrochloride (DAB, catalog #D5637; Sigma) and 0.005% H2O2 in 0.05 M Tris buffer, pH 7.6. The reaction was stopped after 8 min by several washes in Tris buffer and PBS. Immunostained sections were mounted on gelatine-coated slides, dehydrated in ethanol and coverslipped with Permount.
Cresyl Violet, Luxol Fast Blue and ChAT-stained sections were digitalized at 1200 dpi (pixel resolution of 1 μm) using a slide scanner (TISSUEscopeTM 4000, Huron Technologies, Waterloo, Ontario, Canada) equipped with a 10X objective.
Methodological Considerations and Limitations
Chemical fixation of the brain and section processing inevitably lead to shrinkage. In order to minimize shrinkage, blocks were cut at 4°C with a vibratome, precluding the need of cryoprotection in sucrose solution and freezing. A comparison of the size of the red nucleus as it appears on post-mortem brain MRI images and on Cresyl Violet stained sections, has revealed the shrinkage to be of the order of 4%. No pre-mortem MRI was available to assess shrinkage that might have been caused by the perfusion. It should be reminded that this atlas is based on a single brain of a 61-year-old woman and that existing inter-individual variations should carefully be taken into account using provided MRI (see Supplementary Material). Three different magnifications had to be used to provide plates presenting histological sections of adequate size (plates 1–6, plates 7–29, and plates 30–45). Therefore, readers should pay close attention to individual scale bars when comparisons are made between plates. Segmentation of brainstem nuclei and fiber tracts from Cresyl Violet and Luxol Fast Blue stained sections were performed following careful examination of histological sections, so as to avoid arbitrary delineation. Only structures that could be easily delineated in our preparations are identified and dashed lines are used when brainstem regions don't show clear histological boundaries. For example, the cuneiform nucleus (CnF) and the tegmental part of pontine reticular nucleus (PnTn) are broadly delineated with dashed lines because their boundaries with surrounding structures could not be clearly established. Therefore, the CnF, as defined in the present study, might comprise a portion of the mesencephalic reticular formation, whereas the PnTn could include parts of the isthmic reticular formation, the retroisthmic nucleus, the retrorubral field and the ventrolateral tegmental nucleus, as defined by Paxinos (Paxinos et al., 2020). Our segmentation was confirmed with the help of other human brainstem atlases (Olszewski and Baxter, 1954; Schaltenbrand and Wahren, 1977; Afshar et al., 1978; Paxinos and Huang, 1995; Naidich et al., 2007; Paxinos et al., 2020). Readers might refer to other human brainstem atlases for more extensive delineation of brainstem subnuclei and additional plates, in different sectioning planes. The nomenclature and abbreviations used in the present study are largely based on those of Paxinos and Watson (1982). For brainstem nuclei that didn't show clear subdivisions, we used single abbreviations to identify structures composed of several subnuclei. For example, the abbreviation Sp5 for spinal trigeminal nucleus, as delineated in the present atlas, includes the oral (Sp5O), interpolar (Sp5I), and caudal (Sp5C) parts of the nucleus, and the abbreviation VC for ventral cochlear nucleus includes the anterior (VCA) and posterior (VCP) parts of the nucleus that are delineated in other atlases. Likewise, the abbreviation PAG for periaqueductal gray refers to all PAG columns. A complete list of abbreviations and equivalent Latin names, as published in Terminologia Anatomica (Terminology, 1998) is also provided. The asterisks added to some abbreviations indicate that these abbreviations are slightly different from those used in the human brainstem atlas of Paxinos (Paxinos et al., 2020) or point to structures that are not identified in that atlas.
Data Availability Statement
The original contributions presented in the study are included in the article/Supplementary Material, further inquiries can be directed to the corresponding author.
Ethics Statement
The studies involving human participants were reviewed and approved by Ethics Committee at Université Laval. The patients/participants provided their written informed consent to participate in this study.
Author Contributions
MT and ÉP provided post-mortem human brain tissue. LG and MP were in charge of MRI and proceeded with brain dissection. MT, LG, and MP perfused and extracted the brain. LG cut the brainstem and stained sections with Cresyl Violet and Luxol Fast Blue. VC stained sections with ChAT and acquired and edited images. SS, AP, and MP were in charge of brainstem nuclei and fiber tracts segmentation. VC, AP, and MP wrote the manuscript. All authors contributed to the article and approved the submitted version.
Funding
This work was supported by research grants from the Quebec Bio-Imaging Network (QBIN) and the Natural Sciences and Engineering Research Council of Canada (NSERC) to MP who benefited from a senior career award from the Fonds de Recherche du Québec-Santé (FRQ-S). VC was the recipient of MSc and BSc fellowships from FRQ-S and NSERC.
Conflict of Interest
The authors declare that the research was conducted in the absence of any commercial or financial relationships that could be construed as a potential conflict of interest.
Publisher's Note
All claims expressed in this article are solely those of the authors and do not necessarily represent those of their affiliated organizations, or those of the publisher, the editors and the reviewers. Any product that may be evaluated in this article, or claim that may be made by its manufacturer, is not guaranteed or endorsed by the publisher.
Acknowledgments
The authors are very grateful for brain donation. The authors thank Marie-Josée Wallman and Sylvain Courcy (IRM Québec) for technical assistance.
Supplementary Material
The Supplementary Material for this article can be found online: https://www.dropbox.com/sh/ln9zqv4dkygol2q/AAC80leqF0bYcI8ZLWERCIRXa?dl=0
References
Afshar, F., Watkins, E. S., and Yap, J. C. (1978). Stereotaxic Atlas of the Human Brainstem and Cerebellar Nuclei. New York, NY: Raven Press.
Bianciardi, M., Toschi, N., Edlow, B. L., Eichner, C., Setsompop, K., Polimeni, J. R., et al. (2015). Toward an in vivo neuroimaging template of human brainstem nuclei of the ascending arousal, autonomic, and motor systems. Brain Connect. 5, 597–607. doi: 10.1089/brain.2015.0347
Deistung, A., Schafer, A., Schweser, F., Biedermann, U., Gullmar, D., Trampel, R., et al. (2013). High-resolution MR imaging of the human brainstem in vivo at 7 tesla. Front. Hum. Neurosci. 7:710. doi: 10.3389/fnhum.2013.00710
Ding, S. L., Royall, J. J., Sunkin, S. M., Ng, L., Facer, B. A., Lesnar, P., et al. (2016). Comprehensive cellular-resolution atlas of the adult human brain. J. Comp. Neurol. 524, 3127–3481. doi: 10.1002/cne.24080
Erbagci, H., Keser, M., Kervancioglu, S., and Kizilkan, N. (2012). Estimation of the brain stem volume by stereological method on magnetic resonance imaging. Surg. Radiol. Anat. 34, 819–824. doi: 10.1007/s00276-012-0966-3
Ferraye, M. U., Debu, B., and Pollak, P. (2010). Deep brain stimulation and gait disorders in Parkinson disease. Rev. Neurol. 166, 178–187. doi: 10.1016/j.neurol.2009.07.018
Goetz, L., Bhattacharjee, M., Ferraye, M. U., Fraix, V., Maineri, C., Nosko, D., et al. (2019). Deep brain stimulation of the pedunculopontine nucleus area in Parkinson disease: MRI-based anatomoclinical correlations and optimal target. Neurosurgery 84, 506–518. doi: 10.1093/neuros/nyy151
Insola, A., Valeriani, M., and Mazzone, P. (2012). Targeting the pedunculopontine nucleus: a new neurophysiological method based on somatosensory evoked potentials to calculate the distance of the deep brain stimulation lead from the Obex. Neurosurgery 71, 96–103. doi: 10.1227/NEU.0b013e318249c726
Jacobsohn, L. (1909). Über die Kerne des Menschlichen Hirnstamms (Medulla oblongata, Pons, und Pedunculus cerebri). Berlin: Verlag der Konigl Akademie der Wissenschaften.
Naidich, T. P., Duvernoy, H. M., Delman, B. M., Sorensen, A. G., Kollias, S. S., and Haacke, E. M. (2007). Duvernoy's Atlas of the Human Brain Stem and Cerebellum. Wien: Springer Verlag.
Olszewski, J., and Baxter, D. (1954). Cytoarchitecture of the Human Brain Stem. Basel: J.B. Lippincott Company.
Paxinos, G., Furlong, T. M., and Watson, C. (2020). Human Brainstem: Cytoarchitecture, Chemoarchitecture, Myeloarchitecture, Revised Edition. San Diego, CA: Academic Press.
Paxinos, G., and Watson, C. (1982). The Rat Brain in Stereotaxic Coordinates. Sydney, NSW: Academic Press.
Riley, H. A. (1943). An Atlas of the Basal Ganglia, Brain Stem and Spinal Cord. New York, NY: Hafner Publishing Company.
Rushmore, R. J., Wilson-Braun, P., Papadimitriou, G., Ng, I., Rathi, Y., Zhang, F., et al. (2020). 3D Exploration of the brainstem in 50-micron resolution MRI. Front. Neuroanat. 14:40. doi: 10.3389/fnana.2020.00040
Schaltenbrand, G., and Wahren, W. (1977). Atlas for Stereotaxy of the Human Brain. Chicago, IL: Thieme.
Spetzler, R. F., Kalani, M. Y. S., Nakaji, P., and Yagmurlu, K. (2017). Color Atlas of Brainstem Surgery. New York, NY: Thieme.
Talairach, J., David, M., Tournoux, P., Corredor, H., and Kvasina, T. (1957). Atlas d'anatomie stéréotaxique. Repérage radiologique indirect des noyaux gris centraux des régions mésencephalo-sousoptique et hypothalamique de l'homme. Paris: Masson and Cie.
Talairach, J., and Szikla, G. (1967). Atlas of Stereotaxic Anatomy of the Telencephalon. Anatomo-Radiological Studies. Paris: Masson and Cie.
Talairach, J., and Tournoux, P. (1988). Co-Planar Stereotactic Atlas of the Human Brain. 3-Dimensional Proportional System: An Approach to Cerebral Imaging. Stuttgart: Georg Thieme Verlag.
Tang, Y., Sun, W., Toga, A. W., Ringman, J. M., and Shi, Y. (2018). A probabilistic atlas of human brainstem pathways based on connectome imaging data. Neuroimage 169, 227–239. doi: 10.1016/j.neuroimage.2017.12.042
Terminology, F. C. O. A. (1998). Terminologia Anatomica: International Anatomical Terminology. New York, NY: Thieme Stuttgart.
Thevathasan, W., Coyne, T. J., Hyam, J. A., Kerr, G., Jenkinson, N., Aziz, T. Z., et al. (2011). Pedunculopontine nucleus stimulation improves gait freezing in Parkinson disease. Neurosurgery 69, 1248–1253. discussion 1254. doi: 10.1227/NEU.0b013e31822b6f71
Thevathasan, W., Pogosyan, A., Hyam, J. A., Jenkinson, N., Foltynie, T., Limousin, P., et al. (2012). Alpha oscillations in the pedunculopontine nucleus correlate with gait performance in parkinsonism. Brain 135, 148–160. doi: 10.1093/brain/awr315
Watson, C., Bartholomaeus, C., and Puelles, L. (2019). Time for radical changes in brain stem nomenclature-applying the lessons from developmental gene patterns. Front. Neuroanat. 13:10. doi: 10.3389/fnana.2019.00010
Willis, T. (1664). Cerebri Anatome: Cui Accessit Nervorum Descriptio et Usus. London: Martyn and Allestry.
Zrinzo, L., Zrinzo, L. V., Tisch, S., Limousin, P. D., Yousry, T. A., Afshar, F., et al. (2008). Stereotactic localization of the human pedunculopontine nucleus: atlas-based coordinates and validationof a magnetic resonance imaging protocol for direct localization. Brain 131, 1588–1598. doi: 10.1093/brain/awn075
Keywords: midbrain, pons, medulla oblongata, cytoarchitecture, reticular formation, neurosurgery, neuropathology
Citation: Coulombe V, Saikali S, Goetz L, Takech MA, Philippe É, Parent A and Parent M (2021) A Topographic Atlas of the Human Brainstem in the Ponto-Mesencephalic Junction Plane. Front. Neuroanat. 15:627656. doi: 10.3389/fnana.2021.627656
Received: 09 November 2020; Accepted: 24 May 2021;
Published: 13 August 2021.
Edited by:
Kathleen S. Rockland, Boston University, United StatesReviewed by:
Henry Evrard, Center for Integrative Neuroscience & Max Planck Institute, GermanySong-Lin Ding, Allen Institute for Brain Science, United States
Copyright © 2021 Coulombe, Saikali, Goetz, Takech, Philippe, Parent and Parent. This is an open-access article distributed under the terms of the Creative Commons Attribution License (CC BY). The use, distribution or reproduction in other forums is permitted, provided the original author(s) and the copyright owner(s) are credited and that the original publication in this journal is cited, in accordance with accepted academic practice. No use, distribution or reproduction is permitted which does not comply with these terms.
*Correspondence: Martin Parent, bWFydGluLnBhcmVudEBmbWVkLnVsYXZhbC5jYQ==