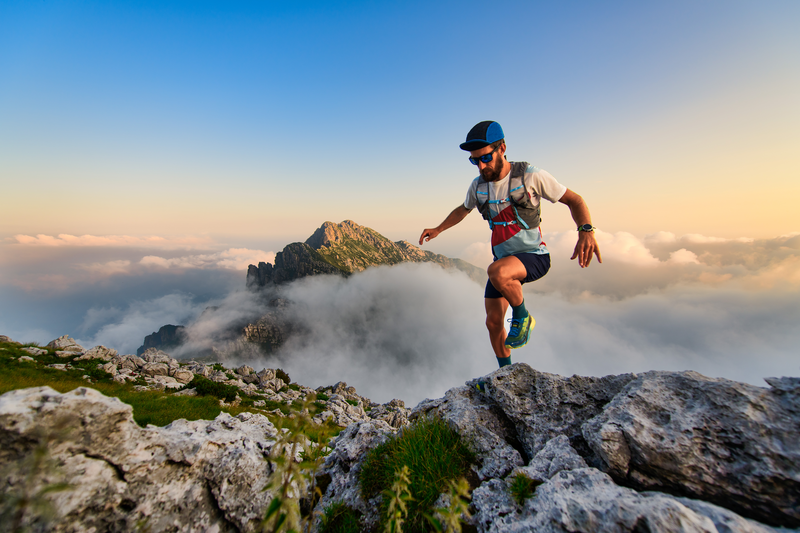
94% of researchers rate our articles as excellent or good
Learn more about the work of our research integrity team to safeguard the quality of each article we publish.
Find out more
ORIGINAL RESEARCH article
Front. Neuroanat. , 15 July 2015
Volume 9 - 2015 | https://doi.org/10.3389/fnana.2015.00089
Although transcranial direct current stimulation (tDCS) studies promise to modulate cortical regions associated with pain, the electric current produced usually spreads beyond the area of the electrodes’ placement. Using a forward-model analysis, this study compared the neuroanatomic location and strength of the predicted electric current peaks, at cortical and subcortical levels, induced by conventional and High-Definition-tDCS (HD-tDCS) montages developed for migraine and other chronic pain disorders. The electrodes were positioned in accordance with the 10–20 or 10–10 electroencephalogram (EEG) landmarks: motor cortex-supraorbital (M1-SO, anode and cathode over C3 and Fp2, respectively), dorsolateral prefrontal cortex (PFC) bilateral (DLPFC, anode over F3, cathode over F4), vertex-occipital cortex (anode over Cz and cathode over Oz), HD-tDCS 4 × 1 (one anode on C3, and four cathodes over Cz, F3, T7, and P3) and HD-tDCS 2 × 2 (two anodes over C3/C5 and two cathodes over FC3/FC5). M1-SO produced a large current flow in the PFC. Peaks of current flow also occurred in deeper brain structures, such as the cingulate cortex, insula, thalamus and brainstem. The same structures received significant amount of current with Cz-Oz and DLPFC tDCS. However, there were differences in the current flow to outer cortical regions. The visual cortex, cingulate and thalamus received the majority of the current flow with the Cz-Oz, while the anterior parts of the superior and middle frontal gyri displayed an intense amount of current with DLPFC montage. HD-tDCS montages enhanced the focality, producing peaks of current in subcortical areas at negligible levels. This study provides novel information regarding the neuroanatomical distribution and strength of the electric current using several tDCS montages applied for migraine and pain control. Such information may help clinicians and researchers in deciding the most appropriate tDCS montage to treat each pain disorder.
Transcranial direct current stimulation (tDCS) continues to be investigated as a therapeutic tool for alleviating symptoms of patients with a large array of neurologic disorders. It has been described as a reliable and well-tolerated brain stimulation technique (Nitsche et al., 2003a,b; Iyer et al., 2005) where a constant low-amperage (usually 1–2 mA) electric current is delivered to the cortex via surface electrodes positioned over the scalp (Tremblay et al., 2014). Since the introduction of this technology in its current form, positive results have been reported in psychiatry (Martin et al., 2011, 2013; Blumberger et al., 2012; Demirtas-Tatlidede et al., 2013; Wolkenstein and Plewnia, 2013) and neurorehabilitation (Webster et al., 2006; Lindenberg et al., 2012). In addition, several studies have explored the contribution of tDCS in the treatment of numerous pain disorders, including fibromyalgia (Fregni et al., 2006b; Valle et al., 2009; Mendonca et al., 2011; Riberto et al., 2011; Villamar et al., 2013b), pain due to traumatic spinal cord injury (Fregni et al., 2006a; Soler et al., 2010; Jensen et al., 2013; Wrigley et al., 2013), chronic pelvic pain (Fenton et al., 2009), refractory orofacial pain (Antal and Paulus, 2011), postherpetic neuralgia (DosSantos et al., 2012), painful diabetic polyneuropathy (Kim et al., 2013), chronic neuropathic pain following burn injury (Portilla et al., 2013), neurogenic pain (Boggio et al., 2009a), trigeminal neuralgia (Hagenacker et al., 2014), low back pain (Schabrun et al., 2014) and migraine (Antal et al., 2011; DaSilva et al., 2012; Viganò et al., 2013). Moreover, according to a recent meta-analysis there is scientific evidence that anodal tDCS decreases pain levels in patients and increases sensory/pain thresholds in healthy individuals (Vaseghi et al., 2014). Table 1 summarizes the results of clinical studies that investigated the effects of tDCS for pain control. While the mechanisms by which tDCS modulates CNS activity are not fully understood, there is evidence that tDCS can modulate endogenous pain networks by affecting mu-opioid and glutamate/GABA neurotransmission, resulting in functional/structural neuroplasticity (DosSantos et al., 2012, 2014; Foerster et al., 2015). In addition, tDCS effects could be associated with thalamic inhibition mediated by cortico-thalamic fibers (Zaghi et al., 2009).
Table 1. Summary of the studies found in the current literature investigating the effects of tDCS in different pain disorders.
The effects produced by tDCS are hypothesized to derive from neuronal membrane polarization, which is determined by the electric field generated in a given brain region. Unlike other methods of supra-threshold brain stimulation, such as deep brain stimulation (DBS) or transcranial magnetic stimulation (TMS), tDCS produces small sub-threshold electric fields (e.g., <1 V/m produced by tDCS vs. 100 V/m produced by other modalities) (Dmochowski et al., 2011). Thus, the degree of brain modulation by tDCS is presumed to monotonically reflect local electric fields. Moreover, while invasive methods (e.g., motor cortex stimulation, MCS) involve directly implanting the electrodes in cortical and/or subcortical structures, in non-invasive approaches (e.g., tDCS) the electric field is not restricted to the target region; instead, it spreads over neighboring cortical and even subcortical regions according to the configuration or montage applied (Bikson et al., 2013). The tDCS montage, as well as other factors such as brain structure (including white and gray matter and regional boundaries) and thickness of the cerebrospinal fluid (CSF) and the skull, determine the electric field generated in each brain region and hence the propensity to modulate regional function (Opitz et al., 2015). Our analysis focuses on the overlap between montage-specific brain current flow and nodes in the pain network. Based on the results of a previous study, we expect to find peaks of electrical current flow in outer cortical areas, including the prefrontal cortex (PFC), primary somatosensory cortex (S1), primary motor cortex (M1) and visual cortex, as well as the insula, cingulate cortex, thalamic nuclei and brainstem. Furthermore, we hypothesize that high-definition (HD-tDCS™) montages will produce more focal effects when compared to conventional tDCS montages.
In order to optimize the distribution of the electric current delivered to the central CNS, computational models have been developed, which predict the patterns of the electric current flow (Datta et al., 2011). In this study, we used high-resolution computational models to investigate the neuroanatomical distribution and peak strength of the current flow across regions of interest in pain control during five different types of tDCS: three conventional and two HD-tDCS montages. The electrodes were positioned following the 10–20 or 10–10 electroencephalogram (EEG) landmarks, typically adopted in tDCS administration. In the conventional setup, two large electrodes (5 × 7), corresponding to the anode (positive pole) and cathode (negative pole), were combined to produce three basic configurations: M1-SO (motor cortex-supraorbital: anode positioned over C3 and cathode positioned over Fp2; DaSilva et al., 2011), DLPFC (dorsolateral PFC bilateral: anode over F3, cathode over F4) and Cz-Oz (vertex-occipital cortex: anode and cathode over Cz and Oz, respectively). In addition, two HD montages were applied using ring electrodes, with the purpose of increasing spatial tDCS focality: HD-tDCS 4 × 1 (one anode centered on C3, surrounded by four cathodes over Cz, F3, T7, and P3) and HD-tDCS 2 × 2 (two anodes over C3 and C5 and two cathodes over FC3 and FC5) (Datta et al., 2009; Villamar et al., 2013a).
Brain current flow (electric fields) through cortical and subcortical structures was evaluated based on previous studies and defining putative Brodmann Areas (BA; Wiegell et al., 2003; Afif and Mertens, 2010; Mai and Paxinos, 2012). To compare current flow patterns across the five different electrode montages, we developed an individualized finite element (FE) head model (Huang et al., 2013). Tissues and other materials of varying properties, namely conductivity, were identified and segmented from a high-resolution 1 mm3 T1 MRI of a neurologically normal adult male. Automated segmentation algorithms (SPM8, Wellcome Trust Center for Neuroimaging, London, UK) with customized tissue probability maps and filters specific for FE modeling (Huang et al., 2013) created the initial tissue geometry and ensured continuity in CSF. Additional manual segmentation using a digital pen and tablet resolved finer detail throughout the head including cortical folding and deeper brain structures. Geometric models of sponge electrodes (5 × 7 cm) and HD electrodes (12 mm diameter) were created as computer-aided design (CAD) files and incorporated into the image volume (Truong et al., 2012). The resulting volumetric image data was then converted into meshes using adaptive voxel-based meshing algorithms (Simpleware Ltd, Exeter, UK). The meshes were imported into an FE package (COMSOL Multiphysics 4.3, COMSOL, Inc., Burlington, MA, USA) where electrostatic physics were applied (Datta et al., 2009). The Laplace equation with purely conductive properties was solved for electric field (Miranda et al., 2006; Wagner et al., 2007; Datta et al., 2009) and the following isotropic electrical conductivities were assigned (S/m): skin: 0.465, fat: 0.025, skull: 0.01, CSF: 1.65, gray matter: 0.276, white matter: 0.126, air: 10−15, saline-soaked sponge or conductive gel: 1.4, electrode: 5.99 × 107. Current density corresponding to 1 mA total current was applied at the anode(s), as is typically used for tDCS, and ground applied to the cathode(s). All other surface boundaries, primarily skin in contact with air, were considered electrically insulated. The entire model workflow preserved precision beginning from the 1 mm3 resolution MRI to the induced cortical electric field maps (Bikson and Datta, 2012). Stimulation electrodes for all the montages were positioned in accordance with the 10–20 or 10–10 EEG landmarks as is used in typical tDCS administration.
Five montages were simulated:
• M1-SO: 5 × 7 cm sponges with anode positioned vertically over 10–20 location C3 and cathode positioned horizontally on the contralateral-supraorbital, approximately over 10–20 location Fp2.
• DLPFC (F3-F4): 5 × 7 cm sponges with anode and cathode positioned vertically over 10–20 locations F3 and F4 respectively.
• Cz-Oz: 5 × 7 cm sponges with cathode positioned horizontally over 10–20 location Oz. Anode is centered on the vertex, Cz, with the length of the pad parallel to the line from ear to ear.
• HD-tDCS 4 × 1: 12 mm diameter disk electrodes with the anode centered on 10–20 location C3. Cathodes (4) surround the anode 90° apart at a “4 × 1 ring” radius of approximately 75 mm. Explicit cathode locations were 10–10 locations Cz, F3, T7, and P3.
• H.O.P.E. HD-tDCS 2 × 2 (Donnell et al., 2015): 12 mm diameter disk electrodes with two anodes and two cathodes positioned posterior to anterior across the face/head region of M1. Explicit 10–10 anode locations are C3 and C5. Cathode locations are FC3 and FC5.
The neuroanatomical current distribution and the strength of the predicted electric current peaks related to conventional and High-Definition-tDCS (HD-tDCS) montages are illustrated in Figures 1 and 2, respectively.
Figure 1. Three different conventional tDCS montages are illustrated (first column, from the top to the bottom): M1-SO motor (cortex-supraorbital), DLPFC (dorsolateral prefrontal cortex bilateral) and Cz-Oz (vertex-occipital cortex). Electric field maps generated in outer cortical regions and inner structures (insula, cingulate gyrus, thalamus and brainstem) are illustrated in the next five columns (from the left to the right).
Figure 2. Electric field maps produced by two methods of high-definition (HD)-tDCS: HD-tDCS 4 × 1 and H.O.P.E. HD-tDCS 2 × 2 (first column from the top to the bottom) in outer (second column) and inner structures (third to sixth, from the left to the right).
The frontal lobe received a larger amount of current when compared to other cortical structures. Nonetheless, the current spread over several regions. Peaks of current density and in turn electric field (0.68 V/m) occurred in the PFC, including parts of the superior, middle and inferior frontal gyri, bilaterally. In addition, the current flowed to the premotor cortex (PMC), putative BA 6, and precentral gyrus (primary motor cortex—M1, putative BA 4) particularly on the left side. Peaks of current were also found in the anterior and posterior insula on both sides. Interestingly, the highest insular current density occurred in depth of the anterior insular sulcus (AIS), precentral (preCIS), central (CIS) and postcentral (postCIS) insular sulci, especially on the left side. In the cingulate cortex the current flow was more discrete, extending from the subgenual anterior cingulate cortex (sACC) to the midcingulate cortex (MCC) bilaterally, though with higher intensities on the right side. According to the results of the current study, the thalamus is also directly stimulated in this type of montage. Peaks of current flow were found in the medial pulvinar (PuM), ventral posteromedial (VPM) and anteroventral (AV) nuclei as well as the centromedian (CM) nucleus on the right side. In the brainstem peaks were concentrated in the midbrain, specifically along the interpeduncular fossa. Moreover, few peaks were detected on the left cerebral peduncle. Regions presenting lower densities of current flow were also found in the dorsal pons and medulla.
The peak magnitude of the electric field (0.54 V/m) occurred in the right PFC, mainly in anterior parts of the superior and middle frontal gyri. On the left hemisphere the current was more limited to the anterior part of the superior frontal gyrus. Part of the electric current also spread to the right precentral PMC. A relatively intense electric field (>0.36 V/m) was predicted in the right AIS, while scarce electric fields were predicted at the sACC and pregenual anterior cingulate cortex (pACC). Few thalamic clusters were predicted when DLPFC montage was applied, mainly projected to the right and left PuM and right CM. Electric field peaks (0.18 V/m) were localized in the most lateral aspects of the right and left cerebral peduncles. Current density, however, was relatively low (0.0227 A/m2) due to the lower conductivity used in modeling brainstem (σ = 0.126 S/m vs. 0.276 S/m for cortex, where J = E/σ). Both the ventral and dorsal parts of the pons exhibited peaks of current density. Increased current densities were detected in the superior and inferior cerebellar peduncle, extending to a tiny area in the most cranial part of the medulla, including the olives.
Using this montage, the current flowed mainly to the parietal and occipital lobes with the maximum electric field (0.66 V/m) occurring in the primary visual cortex V1 (putative BA 17) and secondary visual area V2 (putative BA 18). Large areas of intense current flow were found in the inferior peri-insular sulcus (IPS) and posterior insula, bilaterally. Peaks were also detected in the right precentral (preCIG) and postcentral (postCIG) insular gyri. Moreover, peaks of electric field (0.40 V/m) were predicted at several parts of the cingulate cortex, bilaterally—more so than in any other montage tested. Peaks were mainly predicted in the MCC and posterior cingulate cortex (PCC) on both sides. Thalamic clusters were located in the following nuclei: pulvinar (Pu), parafascicular (Pf), mediodorsal (MD) and AV, bilaterally, as well as right CM and PuM. In the brainstem, a cluster of increased current flow occurred in the interpeduncular fossa, while larger areas of great current flow were found in the dorsal pons, medially to the superior cerebellar peduncle. Lower current densities also occurred in the midbrain tectum.
As expected, this model limited the anatomical distribution of the electric field to within the ring perimeter. The current flowed to only a few areas in the outer cortical regions and remained exclusively on the side of the stimulation. Peaks of current were located in the depth of the central sulcus and adjoining structures, including the precentral gyrus (M1) and postcentral gyrus (primary somatosensory cortex—S1, putative BA 3, 1, 2). The latter had the highest predicted electric field (0.42 V/m) among the superficial cortical structures. Increased electric current also occurred in the inferior parietal lobule (supramarginal area, putative BA 40) and postcentral sulcus. Clusters of electric activity (0.15 V/m) were also found in the anterior and posterior parts of the left insula (e.g., CIS and postCIS). In the cingulate cortex, the most intense current flow occurred in the right/left MCC, PCC, as well as the right sACC. The left PuM and VPM received the majority of the thalamic current flow. In the brainstem, the electric current flowed to the interpeduncular fossa and left cerebral peduncle. Small clusters occurred on the left dorsal pons.
HD-tDCS can be delivered using many different positions and number of electrodes, with each montage optimized for a specific clinical or experimental objective. With 4 × 1 montage for instance, a wider ring leads to a wider, intense, and deeper region of induced cortical current flow, while decreasing the ring diameter leads to increased focality at the cost of increased current shunting across the scalp (without crossing into the cortex). Therefore, the depth, extent, and maximal intensity of induced cortical current flow can be titrated by simply changing ring diameter (Edwards et al., 2013).
The peak electric field (0.42 V/m) was predicted in the region between the anodes and cathodes. The current flow was even more localized when compared to the 4 × 1 montage, in lieu of the fact that the 4 × 1 montage employed a 15 cm diameter ring. Owing to reduced anode-cathode electrode separation (4 cm), cortical current flow was restricted to a reduced area resulting in increased focality for the 2 × 2 montage. The current flowed to the ipsilateral M1 and PMC. Current density peaks (0.047 A/m2) also occurred along the anterior and posterior left insula, particularly affecting the preCIS, preCIG and postCIG. Current flow occurred in the sACC and MCC, bilaterally. Thalamic clusters were detected in the AV and MD nuclei, on both sides and left CM. Furthermore, peaks of current occurred in the MD and CM/Pf nuclei, bilaterally. However, these were clearly more intense on the right side. In this montage the brainstem peak was very well localized and concentrated in the interpeduncular fossa. Noteworthy, the peaks of current flow found in the cingulate, thalamus and brainstem with HD-tDCS (4 × 1 or 2 × 2) were much lower, even negligible, when compared to those produced by conventional tDCS (M1-SO, F3-F4 or Cz-Oz).
Despite the vast number of studies exploring and challenging the clinical outcomes produced by tDCS (O’Connell et al., 2010, 2014), its impact in the CNS functioning has not been completely established. Hence, with recent evidence that tDCS can directly modify the activity of deeper brain structures through the diffusion of the current from extracranial electrodes (DaSilva et al., 2012), modeling studies have gained considerable importance (Edwards et al., 2013; Truong et al., 2013; Villamar et al., 2013b; Kuo et al., 2014; Ruffini et al., 2014). Previously in a migraine trial, researchers attempted to characterize the tDCS-induced electric current flow to the entire cortical surface and deeper brain structures, and found precisely defined peaks in four brain regions: insula, cingulate, thalamus and brainstem. In that proof of concept study (DaSilva et al., 2012), all areas analyzed contained significant peaks of electric current in subregions related to pain perception and analgesia. However, only the M1-SO montage used in the migraine trial was investigated. Thus, the neuroanatomical patterns of the current flow in other pain modulatory tDCS montages still needed to be explored and compared.
This study aimed to investigate the neuroanatomical distribution of electric current flow in five recently reported tDCS montages used for migraine and/or pain modulation. We hypothesized that peaks of current flow would be observed in outer cortical regions (e.g., PFC, S1, M1, V1 and V2) as well as deeper structures (e.g., insula, cingulate cortex, thalamic nuclei and brainstem), depending on the selected tDCS montage. As expected, there were significant differences in the neuroanatomical maps of current flow generated by each tDCS montage (Figures 1, 2). Confirming the primary hypothesis, HD-tDCS resulted in more focused cortical effects when compared to conventional montages. This finding was particularly evident with HD-TDCS H.O.P.E. 2 × 2 montage (Donnell et al., 2015). The current flow with the 2 × 2 montage was more concentrated than the 4 × 1 montage, as the 2 × 2 montage emulates the effects of implanted motor cortex stimulation by employing a much smaller anode-cathode electrode separation and postero-anterior electric current direction. In both HD-tDCS montages the current peaked in the ipsilateral precentral gyrus (M1) and postcentral gyrus (S1). However, current peaks for 2 × 2 and 4 × 1 HD-tDCS montages became negligible in (sub) cortical regions outside their small HD-tDCS electrodes’ ring, including the thalamus, ACC and insula, when compared to conventional montages. This focused targeting corroborates with the clinical outcome of a recent 4 × 1 M1 HD-tDCS study with fibromyalgia patients where a single session led to significant reduction in overall perceived pain (Villamar et al., 2013b). In another study, daily sessions of 2 × 2 M1 HD-tDCS in chronic temporomandibular disorders (TMD) patients induced significant pain relief—greater than 50% decrease in visual analog scale (VAS) pain ratings—at 4 week follow-up and pain-free mouth opening at 1 week follow-up (Donnell et al., 2015). Furthermore, during the treatment week there was significant improvement in the TMD pain area, intensity and their sum measures in the side contralateral to the M1 stimulation, but not in the ipsilateral side. Interestingly, there were no changes in emotional values between active and placebo groups, which indicates that M1 HD-tDCS montages, at least the 2 × 2, are precise in modulating sensorimotor functions.
Remarkably, in the present study M1-SO and DLPFC montages produced significant peaks of electric current in the PFC, a region intrinsically related to cognitive and emotional functions (Gusnard et al., 2001; Phelps et al., 2004; Tabibnia et al., 2014; Haas et al., 2015; Kong et al., 2015; Nakagawa et al., 2015) and thus important for the modulation of the emotional dimension of pain (Lorenz et al., 2002; Porro et al., 2002; Apkarian et al., 2004; Kuchinad et al., 2007; DaSilva et al., 2008; Boggio et al., 2009b; Metz et al., 2009; Jin et al., 2013; Baliki et al., 2014; Bogdanov et al., 2015). Based on the results of the current study, it is possible to speculate that not only DLPFC tDCS but also M1-SO produces its clinical effects through direct PFC modulation. However, further studies will be necessary to scrutinize this hypothesis.
Furthermore, M1-SO was the conventional montage with more significant effects in the insula, with peaks of current found in both the posterior and anterior insula, while the DLPFC montage resulted in large amounts of electric current in the anterior insula only. Several studies have established the major role of insula in pain processing (Ostrowsky et al., 2002; Craig, 2003; Apkarian et al., 2005; Brooks et al., 2005; Brooks and Tracey, 2007; Henderson et al., 2007; Frot et al., 2014), and structural and functional changes in the insular cortex have been demonstrated in numerous pain conditions, including trigeminal neuropathic pain (DaSilva et al., 2008; Moisset et al., 2011), cluster headache (Sprenger et al., 2007), and migraine (Kim et al., 2008; Schmidt-Wilcke et al., 2008; Valfre et al., 2008; Prescot et al., 2009; Coppola et al., 2014). However, according to neuroimaging studies, there are functional differences between the anterior and posterior insula. While the anterior insula is involved in emotional functions, the posterior insula is more related to visceral symptoms (Dupont et al., 2003). In addition, the nociceptive input is first processed at the posterior insula, which is likely related to the interpretation of the anatomical location and intensity of the stimulus, and then at the anterior insula, mainly involved in emotional reactions (Frot et al., 2014). Other studies revealed that clinical pain is more rostrally located in the anterior insula than acute experimental pain induced in healthy subjects (Schweinhardt et al., 2006; Brooks and Tracey, 2007; Schweinhardt and Bushnell, 2010). Therefore, while both montages (M1-SO and DLPFC) are likely suited to modulate high emotional involvement commonly seen in chronic pain patients, M1-SO should be preferentially used when aiming to treat visceral pain.
Regarding the activation of medial neuroanatomical regions, Cz-Oz was the conventional montage with more significant effects in the MCC and PCC, while the M1-SO and DLPFC montages preferentially reached the ACC and the anterior MCC. Previous neuroimaging studies reported changes in the cingulate cortex of migraine patients, mostly in the ACC and MCC (Weiller et al., 1995; Kim et al., 2008; Schmidt-Wilcke et al., 2008; Valfre et al., 2008; May, 2009) but also in the PCC (Kim et al., 2008). Furthermore, the results of a recent investigation suggest that changes in metabolite levels occur in the PCC of patients with chronic pain, including migraine (Fayed et al., 2014). Thus, the decrease of pain found in migraine patients after M1-SO tDCS (DaSilva et al., 2012) could be explained not only by the stimulation of the cortical regions beneath the electrode and indirect activation of more remote regions, but also by a direct effect in the ACC and/or anterior parts of the MCC. On the other hand, the positive results reported with Cz-Oz in migraine (Antal et al., 2011; Siniatchkin et al., 2012; Viganò et al., 2013) could be linked to the direct effects of tDCS in the posterior parts of the MCC or even PCC. Noteworthy, the presence of highly conductive intracerebral artery (which is assigned the conductivity of CSF) directly under the CZ pad provides a preferential conduit for currents to travel into medial brain regions. This likely explains the increased current flow in the posterior cingulate, thalamic nuclei and brainstem when using the Cz-Oz montage. Thus, Cz-Oz could be effective in migraine, not merely because of its action at the occipital (visual) cortex, but also due to its direct influence in medial structures related to pain processing and analgesia, such as the cingulate and thalamus. The thalamus is an important area for pain (Derbyshire et al., 1994; Hsieh et al., 1995; Iadarola et al., 1995; Casey et al., 1996; Vogt et al., 1996; May et al., 1998; Peyron et al., 1998; Petrovic et al., 1999; Rocca et al., 2010) as well as placebo effect (Wager et al., 2007; Zubieta and Stohler, 2009), and the pulvinar has been recently linked to migraine mechanisms (Moulton et al., 2011; Maleki et al., 2012; Schwedt et al., 2013). However, while thalamic current flow was detected in all montages (particularly in the pulvinar), robust levels of electric current were only detected with conventional montages compared to lower levels that occurred with HD-TDCS.
Despite the valuable information provided by modeling studies, it is still not possible to precisely define the extent to which the strength of the electric current correlates to the clinical effects reported with tDCS, as the mechanisms whereby nervous tissue is stimulated by this method are not completely understood. It has been hypothesized that tDCS effects derive from neuronal membrane polarization, which is determined by the electric field generated (Dmochowski et al., 2011). Moreover, evidence from TMS studies indicates that nervous system stimulation takes place at the electrical field peaks (Amassian et al., 1994; Wassermann et al., 1996; Krings et al., 1997; Boroojerdi et al., 1999; Miranda et al., 2003). Nonetheless, further studies are necessary to establish the mechanisms by which tDCS acts at the central nervous system.
The present study provides information on the electric current flow generated by different conventional and HD-tDCS montages applied for migraine/pain control. Five montages were analyzed (M1-SO, DLPFC, Cz-Oz, 4 × 1 HD-tDCS and 2 × 2 HD-tDCS) and all produced significant results. Nevertheless, an increased focality occurred with HD-tDCS. As this study demonstrates, the neuroanatomical approach, based on computational models, is crucial to define the neural networks directly modulated by each type of tDCS montage applied for pain investigation and relief. It is important to emphasize that the single subject models used for this study provide data on broad variations in current flow patterns that are expected to generalize. However, this information ultimately contributes to a broader comprehension of the neurophysiologic aspects as well as central pain mechanisms targeted by tDCS. In the future, the combination of brain-modeling analysis with the evaluation of specific functional and/or structural neuroplastic changes related to pain could contribute to identify the most appropriate tDCS montage to treat each chronic pain disorder.
AFD and MB mentored, conceived, designed, obtained funding and coordinated the study. They also drafted the manuscript. DQT and AD acquired the data, designed the study, analyzed the data and drafted the manuscript. MFD designed the study, analyzed the data, and drafted the manuscript. RLT contributed to the study and helped to draft the manuscript. All authors read and approved the current version of the manuscript.
Dr. Datta and Dr. Bikson are co-founders of Soterix Medical. The other authors declare no conflicts of interest related to this study.
Dr. DaSilva was supported by MICHR Clinical Trial Planning Program/CTSA high-tech funding UL1RR024986, University of Michigan, National Institute of Health—National Institute of Neurological Disorders and Stroke—K23 NS062946, Dana Foundation’s Brain and Immuno-Imaging Award, and the Migraine Research Foundation Research Grant Award.
AIS, anterior insular sulcus; AV, anteroventral; BA, Brodmann area; CAD, computer-aided design; CIS, central insular sulcus; CM, centromedian; CP, cerebral peduncle; CZ, vertex; DLPFC, dorsolateral prefrontal cortex; DP, dorsal pons; EEG, electroencephalogram; HD, High-Definition; IF, interpeduncular fossa; IPS, inferior peri-insular sulcus; MCC, midcingulate Cortex; MD, mediodorsal; M1, primary motor cortex; OZ, occipital cortex; PFC, prefrontal cortex; pACC, pregenual anterior cingulate cortex; PCC, posterior cingulate cortex; Pf, parafascicular; PMC, premotor cortex; postCIS, postcentral insular sulcus; postCIG, postcentral insular gyrus; preCIG, precentral insular sulcus; preCIS, precentral insular sulcus; Pu, pulvinar; PuM, medial pulvinar; P3, left parietal cortex; sACC, subgenual anterior cingulate cortex; SO, supraorbital region; S1, somatosensory cortex; TMD, temporomandibular disorders; T7, left temporal cortex; VPM, ventral posteromedial; V1, primary visual cortex; V2, secondary visual cortex.
Afif, A., and Mertens, P. (2010). Description of sulcal organization of the insular cortex. Surg. Radiol. Anat. 32, 491–498. doi: 10.1007/s00276-009-0598-4
Amassian, V. E., Maccabee, P. J., Cracco, R. Q., Cracco, J. B., Somasundaram, M., Rothwell, J. C., et al. (1994). The polarity of the induced electric field influences magnetic coil inhibition of human visual cortex: implications for the site of excitation. Electroencephalogr. Clin. Neurophysiol. 93, 21–26. doi: 10.1016/0168-5597(94)90087-6
Antal, A., Kriener, N., Lang, N., Boros, K., and Paulus, W. (2011). Cathodal transcranial direct current stimulation of the visual cortex in the prophylactic treatment of migraine. Cephalalgia 31, 820–828. doi: 10.1177/0333102411399349
Antal, A., and Paulus, W. (2011). A case of refractory orofacial pain treated by transcranial direct current stimulation applied over hand motor area in combination with NMDA agonist drug intake. Brain Stimul. 4, 117–121. doi: 10.1016/j.brs.2010.09.003
Antal, A., Terney, D., Kühnl, S., and Paulus, W. (2010). Anodal transcranial direct current stimulation of the motor cortex ameliorates chronic pain and reduces short intracortical inhibition. J. Pain Symptom Manage. 39, 890–903. doi: 10.1016/j.jpainsymman.2009.09.023
Apkarian, A. V., Bushnell, M. C., Treede, R. D., and Zubieta, J. K. (2005). Human brain mechanisms of pain perception and regulation in health and disease. Eur. J. Pain 9, 463–484. doi: 10.1016/j.ejpain.2004.11.001
Apkarian, A. V., Sosa, Y., Sonty, S., Levy, R. M., Harden, R. N., Parrish, T. B., et al. (2004). Chronic back pain is associated with decreased prefrontal and thalamic gray matter density. J. Neurosci. 24, 10410–10415. doi: 10.1523/jneurosci.2541-04.2004
Baliki, M. N., Mansour, A. R., Baria, A. T., and Apkarian, A. V. (2014). Functional reorganization of the default mode network across chronic pain conditions. PLoS One 9:e106133. doi: 10.1371/journal.pone.0106133
Bikson, M., and Datta, A. (2012). Guidelines for precise and accurate computational models of tDCS. Brain Stimul. 5, 430–431. doi: 10.1016/j.brs.2011.06.001
Bikson, M., Dmochowski, J., and Rahman, A. (2013). The “quasi-uniform” assumption in animal and computational models of non-invasive electrical stimulation. Brain Stimul. 6, 704–705. doi: 10.1016/j.brs.2012.11.005
Blumberger, D. M., Tran, L. C., Fitzgerald, P. B., Hoy, K. E., and Daskalakis, Z. J. (2012). A randomized double-blind sham-controlled study of transcranial direct current stimulation for treatment-resistant major depression. Front. Psychiatry 3:74. doi: 10.3389/fpsyt.2012.00074
Bogdanov, V. B., Viganò, A., Noirhomme, Q., Bogdanova, O. V., Guy, N., Laureys, S., et al. (2015). Cerebral responses and role of the prefrontal cortex in conditioned pain modulation: an fMRI study in healthy subjects. Behav. Brain Res. 281, 187–198. doi: 10.1016/j.bbr.2014.11.028
Boggio, P. S., Amancio, E. J., Correa, C. F., Cecilio, S., Valasek, C., Bajwa, Z., et al. (2009a). Transcranial DC stimulation coupled with TENS for the treatment of chronic pain: a preliminary study. Clin. J. Pain 25, 691–695. doi: 10.1097/AJP.0b013e3181af1414
Boggio, P. S., Zaghi, S., and Fregni, F. (2009b). Modulation of emotions associated with images of human pain using anodal transcranial direct current stimulation (tDCS). Neuropsychologia 47, 212–217. doi: 10.1016/j.neuropsychologia.2008.07.022
Boroojerdi, B., Foltys, H., Krings, T., Spetzger, U., Thron, A., and Töpper, R. (1999). Localization of the motor hand area using transcranial magnetic stimulation and functional magnetic resonance imaging. Clin. Neurophysiol. 110, 699–704. doi: 10.1016/s1388-2457(98)00027-3
Brooks, J. C., and Tracey, I. (2007). The insula: a multidimensional integration site for pain. Pain 128, 1–2. doi: 10.1016/j.pain.2006.12.025
Brooks, J. C., Zambreanu, L., Godinez, A., Craig, A. D., and Tracey, I. (2005). Somatotopic organisation of the human insula to painful heat studied with high resolution functional imaging. Neuroimage 27, 201–209. doi: 10.1016/j.neuroimage.2005.03.041
Casey, K. L., Minoshima, S., Morrow, T. J., and Koeppe, R. A. (1996). Comparison of human cerebral activation pattern during cutaneous warmth, heat pain and deep cold pain. J. Neurophysiol. 76, 571–581.
Coppola, G., Di Renzo, A., Tinelli, E., Iacovelli, E., Lepre, C., Di Lorenzo, C., et al. (2014). Evidence for brain morphometric changes during the migraine cycle: a magnetic resonance-based morphometry study. Cephalalgia doi: 10.1177/0333102414559732 [Epub ahead of print].
Craig, A. D. (2003). Interoception: the sense of the physiological condition of the body. Curr. Opin. Neurobiol. 13, 500–505. doi: 10.1016/s0959-4388(03)00090-4
DaSilva, A. F., Becerra, L., Pendse, G., Chizh, B., Tully, S., and Borsook, D. (2008). Colocalized structural and functional changes in the cortex of patients with trigeminal neuropathic pain. PLoS One 3:e3396. doi: 10.1371/journal.pone.0003396
DaSilva, A. F., Mendonca, M. E., Zaghi, S., Lopes, M., DosSantos, M. F., Spierings, E. L., et al. (2012). tDCS-induced analgesia and electrical fields in pain-related neural networks in chronic migraine. Headache 52, 1283–1295. doi: 10.1111/j.1526-4610.2012.02141.x
DaSilva, A. F., Volz, M. S., Bikson, M., and Fregni, F. (2011). Electrode positioning and montage in transcranial direct current stimulation. J. Vis. Exp. 51:2744. doi: 10.3791/2744
Datta, A., Baker, J. M., Bikson, M., and Fridriksson, J. (2011). Individualized model predicts brain current flow during transcranial direct-current stimulation treatment in responsive stroke patient. Brain Stimul. 4, 169–174. doi: 10.1016/j.brs.2010.11.001
Datta, A., Bansal, V., Diaz, J., Patel, J., Reato, D., and Bikson, M. (2009). Gyri-precise head model of transcranial direct current stimulation: improved spatial focality using a ring electrode versus conventional rectangular pad. Brain Stimul. 2, 201–207.e1. doi: 10.1016/j.brs.2009.03.005
Demirtas-Tatlidede, A., Vahabzadeh-Hagh, A. M., and Pascual-Leone, A. (2013). Can noninvasive brain stimulation enhance cognition in neuropsychiatric disorders? Neuropharmacology 64, 566–578. doi: 10.1016/j.neuropharm.2012.06.020
Derbyshire, S. W., Jones, A. K., Devani, P., Friston, K. J., Feinmann, C., Harris, M., et al. (1994). Cerebral responses to pain in patients with atypical facial pain measured by positron emission tomography. J. Neurol. Neurosurg. Psychiatry 57, 1166–1172. doi: 10.1136/jnnp.57.10.1166
Dmochowski, J. P., Datta, A., Bikson, M., Su, Y., and Parra, L. C. (2011). Optimized multi-electrode stimulation increases focality and intensity at target. J. Neural Eng. 8:046011. doi: 10.1088/1741-2560/8/4/046011
Donnell, A., Nascimento, T., Lawrence, M., Gupta, V., Zieba, T., Truong, D. Q., et al. (2015). High-Definition and Non-Invasive Brain Modulation of Pain and Motor Dysfunction in Chronic TMD. Brain Stimul. In Press doi: 10.1016/j.brs.2015.06.008
DosSantos, M. F., Love, T. M., Martikainen, I. K., Nascimento, T. D., Fregni, F., Cummiford, C., et al. (2012). Immediate effects of tDCS on the μ-opioid system of a chronic pain patient. Front. Psychiatry 3:93. doi: 10.3389/fpsyt.2012.00093
DosSantos, M. F., Martikainen, I. K., Nascimento, T. D., Love, T. M., DeBoer, M. D., Schambra, H. M., et al. (2014). Building up analgesia in humans via the endogenous μ-opioid system by combining placebo and active tDCS: a preliminary report. PLoS One 9:e102350. doi: 10.1371/journal.pone.0102350
Dupont, S., Bouilleret, V., Hasboun, D., Semah, F., and Baulac, M. (2003). Functional anatomy of the insula: new insights from imaging. Surg. Radiol. Anat. 25, 113–119. doi: 10.1007/s00276-003-0103-4
Edwards, D., Cortes, M., Datta, A., Minhas, P., Wassermann, E. M., and Bikson, M. (2013). Physiological and modeling evidence for focal transcranial electrical brain stimulation in humans: a basis for high-definition tDCS. Neuroimage 74, 266–275. doi: 10.1016/j.neuroimage.2013.01.042
Fayed, N., Andrés, E., Viguera, L., Modrego, P. J., and Garcia-Campayo, J. (2014). Higher glutamate+glutamine and reduction of N-acetylaspartate in posterior cingulate according to age range in patients with cognitive impairment and/or pain. Acad. Radiol. 21, 1211–1217. doi: 10.1016/j.acra.2014.04.009
Fenton, B. W., Palmieri, P. A., Boggio, P., Fanning, J., and Fregni, F. (2009). A preliminary study of transcranial direct current stimulation for the treatment of refractory chronic pelvic pain. Brain Stimul. 2, 103–107. doi: 10.1016/j.brs.2008.09.009
Foerster, B. R., Nascimento, T. D., Deboer, M., Bender, M. A., Rice, I. C., Truong, D. Q., et al. (2015). Excitatory and inhibitory brain metabolites as targets of motor cortex transcranial direct current stimulation therapy and predictors of its efficacy in fibromyalgia. Arthritis Rheumatol. 67, 576–581. doi: 10.1002/art.38945
Fregni, F., Boggio, P., Lima, M., Ferreira, M., Wagner, T., Rigonatti, S., et al. (2006a). A sham-controlled, phase II trial of transcranial direct current stimulation for the treatment of central pain in traumatic spinal cord injury. Pain 122, 197–209. doi: 10.1016/j.pain.2006.02.023
Fregni, F., Gimenes, R., Valle, A. C., Ferreira, M. J., Rocha, R. R., Natalle, L., et al. (2006b). A randomized, sham-controlled, proof of principle study of transcranial direct current stimulation for the treatment of pain in fibromyalgia. Arthritis Rheum. 54, 3988–3998. doi: 10.1002/art.22195
Frot, M., Faillenot, I., and Mauguière, F. (2014). Processing of nociceptive input from posterior to anterior insula in humans. Hum. Brain Mapp. 35, 5486–5499. doi: 10.1002/hbm.22565
Gusnard, D. A., Akbudak, E., Shulman, G. L., and Raichle, M. E. (2001). Medial prefrontal cortex and self-referential mental activity: relation to a default mode of brain function. Proc. Natl. Acad. Sci. U S A 98, 4259–4264. doi: 10.1073/pnas.071043098
Haas, B. W., Ishak, A., Anderson, I. W., and Filkowski, M. M. (2015). The tendency to trust is reflected in human brain structure. Neuroimage 107, 175–181. doi: 10.1016/j.neuroimage.2014.11.060
Hagenacker, T., Bude, V., Naegel, S., Holle, D., Katsarava, Z., Diener, H. C., et al. (2014). Patient-conducted anodal transcranial direct current stimulation of the motor cortex alleviates pain in trigeminal neuralgia. J. Headache Pain 15:78. doi: 10.1186/1129-2377-15-78
Henderson, L. A., Gandevia, S. C., and Macefield, V. G. (2007). Somatotopic organization of the processing of muscle and cutaneous pain in the left and right insula cortex: a single-trial fMRI study. Pain 128, 20–30. doi: 10.1016/j.pain.2006.08.013
Hsieh, J. C., Belfrage, M., Stone-Elander, S., Hansson, P., and Ingvar, M. (1995). Central representation of chronic ongoing neuropathic pain studied by positron emission tomography. Pain 63, 225–236. doi: 10.1016/0304-3959(95)00048-w
Huang, Y., Dmochowski, J. P., Su, Y., Datta, A., Rorden, C., and Parra, L. C. (2013). Automated MRI segmentation for individualized modeling of current flow in the human head. J. Neural Eng. 10:066004. doi: 10.1088/1741-2560/10/6/066004
Iadarola, M. J., Max, M. B., Berman, K. F., Byas-Smith, M. G., Coghill, R. C., Gracely, R. H., et al. (1995). Unilateral decrease in thalamic activity observed with positron emission tomography in patients with chronic neuropathic pain. Pain 63, 55–64. doi: 10.1016/0304-3959(95)00015-k
Iyer, M. B., Mattu, U., Grafman, J., Lomarev, M., Sato, S., and Wassermann, E. M. (2005). Safety and cognitive effect of frontal DC brain polarization in healthy individuals. Neurology 64, 872–875. doi: 10.1212/01.wnl.0000152986.07469.e9
Jensen, M. P., Sherlin, L. H., Askew, R. L., Fregni, F., Witkop, G., Gianas, A., et al. (2013). Effects of non-pharmacological pain treatments on brain states. Clin. Neurophysiol. 124, 2016–2024. doi: 10.1016/j.clinph.2013.04.009
Jin, C., Yuan, K., Zhao, L., Yu, D., von Deneen, K. M., Zhang, M., et al. (2013). Structural and functional abnormalities in migraine patients without aura. NMR Biomed. 26, 58–64. doi: 10.1002/nbm.2819
Kim, Y. J., Ku, J., Kim, H. J., Im, D. J., Lee, H. S., Han, K. A., et al. (2013). Randomized, sham controlled trial of transcranial direct current stimulation for painful diabetic polyneuropathy. Ann. Rehabil. Med. 37, 766–776. doi: 10.5535/arm.2013.37.6.766
Kim, J. H., Suh, S. I., Seol, H. Y., Oh, K., Seo, W. K., Yu, S. W., et al. (2008). Regional grey matter changes in patients with migraine: a voxel-based morphometry study. Cephalalgia 28, 598–604. doi: 10.1111/j.1468-2982.2008.01550.x
Kong, F., Hu, S., Xue, S., Song, Y., and Liu, J. (2015). Extraversion mediates the relationship between structural variations in the dorsolateral prefrontal cortex and social well-being. Neuroimage 105, 269–275. doi: 10.1016/j.neuroimage.2014.10.062
Krings, T., Buchbinder, B. R., Butler, W. E., Chiappa, K. H., Jiang, H. J., Cosgrove, G. R., et al. (1997). Functional magnetic resonance imaging and transcranial magnetic stimulation: complementary approaches in the evaluation of cortical motor function. Neurology 48, 1406–1416. doi: 10.1212/wnl.48.5.1406
Kuchinad, A., Schweinhardt, P., Seminowicz, D. A., Wood, P. B., Chizh, B. A., and Bushnell, M. C. (2007). Accelerated brain gray matter loss in fibromyalgia patients: premature aging of the brain? J. Neurosci. 27, 4004–4007. doi: 10.1523/jneurosci.0098-07.2007
Kuo, M. F., Paulus, W., and Nitsche, M. A. (2014). Therapeutic effects of non-invasive brain stimulation with direct currents (tDCS) in neuropsychiatric diseases. Neuroimage 85, 948–960. doi: 10.1016/j.neuroimage.2013.05.117
Lindenberg, R., Zhu, L. L., and Schlaug, G. (2012). Combined central and peripheral stimulation to facilitate motor recovery after stroke: the effect of number of sessions on outcome. Neurorehabil. Neural Repair 26, 479–483. doi: 10.1177/1545968311427568
Lorenz, J., Cross, D. J., Minoshima, S., Morrow, T. J., Paulson, P. E., and Casey, K. L. (2002). A unique representation of heat allodynia in the human brain. Neuron 35, 383–393. doi: 10.1016/s0896-6273(02)00767-5
Maleki, N., Becerra, L., Upadhyay, J., Burstein, R., and Borsook, D. (2012). Direct optic nerve pulvinar connections defined by diffusion MR tractography in humans: implications for photophobia. Hum. Brain Mapp. 33, 75–88. doi: 10.1002/hbm.21194
Martin, D. M., Alonzo, A., Ho, K. A., Player, M., Mitchell, P. B., Sachdev, P., et al. (2013). Continuation transcranial direct current stimulation for the prevention of relapse in major depression. J. Affect. Disord. 144, 274–278. doi: 10.1016/j.jad.2012.10.012
Martin, D. M., Alonzo, A., Mitchell, P. B., Sachdev, P., Gálvez, V., and Loo, C. K. (2011). Fronto-extracephalic transcranial direct current stimulation as a treatment for major depression: an open-label pilot study. J. Affect. Disord. 134, 459–463. doi: 10.1016/j.jad.2011.05.018
May, A. (2009). New insights into headache: an update on functional and structural imaging findings. Nat. Rev. Neurol. 5, 199–209. doi: 10.1038/nrneurol.2009.28
May, A., Bahra, A., Büchel, C., Frackowiak, R. S., and Goadsby, P. J. (1998). Hypothalamic activation in cluster headache attacks. Lancet 352, 275–278. doi: 10.1016/s0140-6736(98)02470-2
Mendonca, M. E., Santana, M. B., Baptista, A. F., Datta, A., Bikson, M., Fregni, F., et al. (2011). Transcranial DC stimulation in fibromyalgia: optimized cortical target supported by high-resolution computational models. J. Pain 12, 610–617. doi: 10.1016/j.jpain.2010.12.015
Metz, A. E., Yau, H. J., Centeno, M. V., Apkarian, A. V., and Martina, M. (2009). Morphological and functional reorganization of rat medial prefrontal cortex in neuropathic pain. Proc. Natl. Acad. Sci. U S A 106, 2423–2428. doi: 10.1073/pnas.0809897106
Miranda, P. C., Hallett, M., and Basser, P. J. (2003). The electric field induced in the brain by magnetic stimulation: a 3-D finite-element analysis of the effect of tissue heterogeneity and anisotropy. IEEE Trans. Biomed. Eng. 50, 1074–1085. doi: 10.1109/tbme.2003.816079
Miranda, P. C., Lomarev, M., and Hallett, M. (2006). Modeling the current distribution during transcranial direct current stimulation. Clin. Neurophysiol. 117, 1623–1629. doi: 10.1016/j.clinph.2006.04.009
Moisset, X., Villain, N., Ducreux, D., Serrie, A., Cunin, G., Valade, D., et al. (2011). Functional brain imaging of trigeminal neuralgia. Eur. J. Pain 15, 124–131. doi: 10.1016/j.ejpain.2010.06.006
Moulton, E. A., Becerra, L., Maleki, N., Pendse, G., Tully, S., Hargreaves, R., et al. (2011). Painful heat reveals hyperexcitability of the temporal pole in interictal and ictal migraine states. Cereb. Cortex 21, 435–448. doi: 10.1093/cercor/bhq109
Nakagawa, S., Takeuchi, H., Taki, Y., Nouchi, R., Sekiguchi, A., Kotozaki, Y., et al. (2015). Comprehensive neural networks for guilty feelings in young adults. Neuroimage 105, 248–256. doi: 10.1016/j.neuroimage.2014.11.004
Nitsche, M. A., Liebetanz, D., Antal, A., Lang, N., Tergau, F., and Paulus, W. (2003a). Modulation of cortical excitability by weak direct current stimulation–technical, safety and functional aspects. Suppl. Clin. Neurophysiol. 56, 255–276. doi: 10.1016/s1567-424x(09)70230-2
Nitsche, M., Liebetanz, D., Lang, N., Antal, A., Tergau, F., and Paulus, W. (2003b). Safety criteria for transcranial direct current stimulation (tDCS) in humans. Clin. Neurophysiol. 114, 2220–2222; author reply 2222–2223. doi: 10.1016/s1388-2457(03)00235-9
O’Connell, N. E., Wand, B. M., Marston, L., Spencer, S., and Desouza, L. H. (2010). Non-invasive brain stimulation techniques for chronic pain. Cochrane Database Syst. Rev. 8:CD008208. doi: 10.1002/14651858.CD008208.pub2
O’Connell, N. E., Wand, B. M., Marston, L., Spencer, S., and Desouza, L. H. (2014). Non-invasive brain stimulation techniques for chronic pain. Cochrane Database Syst. Rev. 4:CD008208. doi: 10.1002/14651858.CD008208.pub3
Opitz, A., Paulus, W., Will, S., Antunes, A., and Thielscher, A. (2015). Determinants of the electric field during transcranial direct current stimulation. Neuroimage 109, 140–150. doi: 10.1016/j.neuroimage.2015.01.033
Ostrowsky, K., Magnin, M., Ryvlin, P., Isnard, J., Guenot, M., and Mauguière, F. (2002). Representation of pain and somatic sensation in the human insula: a study of responses to direct electrical cortical stimulation. Cereb. Cortex 12, 376–385. doi: 10.1093/cercor/12.4.376
Petrovic, P., Ingvar, M., Stone-Elander, S., Petersson, K. M., and Hansson, P. (1999). A PET activation study of dynamic mechanical allodynia in patients with mononeuropathy. Pain 83, 459–470. doi: 10.1016/s0304-3959(99)00150-5
Peyron, R., GarcÍa-Larrea, L., Grégoire, M. C., Convers, P., Lavenne, F., Veyre, L., et al. (1998). Allodynia after lateral-medullary (Wallenberg) infarct. A PET study. Brain 121, 345–356. doi: 10.1093/brain/121.2.345
Phelps, E. A., Delgado, M. R., Nearing, K. I., and LeDoux, J. E. (2004). Extinction learning in humans: role of the amygdala and vmPFC. Neuron 43, 897–905. doi: 10.1016/j.neuron.2004.08.042
Porro, C. A., Baraldi, P., Pagnoni, G., Serafini, M., Facchin, P., Maieron, M., et al. (2002). Does anticipation of pain affect cortical nociceptive systems? J. Neurosci. 22, 3206–3214.
Portilla, A. S., Bravo, G. L., Miraval, F. K., Villamar, M. F., Schneider, J. C., Ryan, C. M., et al. (2013). A feasibility study assessing cortical plasticity in chronic neuropathic pain following burn injury. J. Burn Care Res. 34, e48–e52. doi: 10.1097/BCR.0b013e3182700675
Prescot, A., Becerra, L., Pendse, G., Tully, S., Jensen, E., Hargreaves, R., et al. (2009). Excitatory neurotransmitters in brain regions in interictal migraine patients. Mol. Pain 5:34. doi: 10.1186/1744-8069-5-34
Riberto, M., Marcon Alfieri, F., Monteiro de Benedetto Pacheco, K., Dini Leite, V., Nemoto Kaihami, H., Fregni, F., et al. (2011). Efficacy of transcranial direct current stimulation coupled with a multidisciplinary rehabilitation program for the treatment of fibromyalgia. Open Rheumatol. J. 5, 45–50. doi: 10.2174/1874312901105010045
Rocca, M. A., Valsasina, P., Absinta, M., Colombo, B., Barcella, V., Falini, A., et al. (2010). Central nervous system dysregulation extends beyond the pain-matrix network in cluster headache. Cephalalgia 30, 1383–1391. doi: 10.1177/0333102410365164
Ruffini, G., Fox, M. D., Ripolles, O., Miranda, P. C., and Pascual-Leone, A. (2014). Optimization of multifocal transcranial current stimulation for weighted cortical pattern targeting from realistic modeling of electric fields. Neuroimage 89, 216–225. doi: 10.1016/j.neuroimage.2013.12.002
Schabrun, S. M., Jones, E., Elgueta Cancino, E. L., and Hodges, P. W. (2014). Targeting chronic recurrent low back pain from the top-down and the bottom-up: a combined transcranial direct current stimulation and peripheral electrical stimulation intervention. Brain Stimul. 7, 451–459. doi: 10.1016/j.brs.2014.01.058
Schmidt-Wilcke, T., Gänssbauer, S., Neuner, T., Bogdahn, U., and May, A. (2008). Subtle grey matter changes between migraine patients and healthy controls. Cephalalgia 28, 1–4. doi: 10.1111/j.1468-2982.2007.01428.x
Schwedt, T. J., Schlaggar, B. L., Mar, S., Nolan, T., Coalson, R. S., Nardos, B., et al. (2013). Atypical resting-state functional connectivity of affective pain regions in chronic migraine. Headache 53, 737–751. doi: 10.1111/head.12081
Schweinhardt, P., and Bushnell, M. C. (2010). Pain imaging in health and disease–how far have we come? J. Clin. Invest. 120, 3788–3797. doi: 10.1172/JCI43498
Schweinhardt, P., Glynn, C., Brooks, J., McQuay, H., Jack, T., Chessell, I., et al. (2006). An fMRI study of cerebral processing of brush-evoked allodynia in neuropathic pain patients. Neuroimage 32, 256–265. doi: 10.1016/j.neuroimage.2006.03.024
Siniatchkin, M., Sendacki, M., Moeller, F., Wolff, S., Jansen, O., Siebner, H., et al. (2012). Abnormal changes of synaptic excitability in migraine with aura. Cereb. Cortex 22, 2207–2216. doi: 10.1093/cercor/bhr248
Soler, M. D., Kumru, H., Pelayo, R., Vidal, J., Tormos, J. M., Fregni, F., et al. (2010). Effectiveness of transcranial direct current stimulation and visual illusion on neuropathic pain in spinal cord injury. Brain 133, 2565–2577. doi: 10.1093/brain/awq184
Sprenger, T., Ruether, K. V., Boecker, H., Valet, M., Berthele, A., Pfaffenrath, V., et al. (2007). Altered metabolism in frontal brain circuits in cluster headache. Cephalalgia 27, 1033–1042. doi: 10.1111/j.1468-2982.2007.01386.x
Tabibnia, G., Creswell, J. D., Kraynak, T., Westbrook, C., Julson, E., and Tindle, H. A. (2014). Common prefrontal regions activate during self-control of craving, emotion and motor impulses in smokers. Clin. Psychol. Sci. 2, 611–619. doi: 10.1177/2167702614522037
Tremblay, S., Lepage, J. F., Latulipe-Loiselle, A., Fregni, F., Pascual-Leone, A., and Théoret, H. (2014). The uncertain outcome of prefrontal tDCS. Brain Stimul. 7, 773–783. doi: 10.1016/j.brs.2014.10.003
Truong, D. Q., Datta, A., Xu, J., Fregni, F., and Bikson, M. (2012). “Prefrontal cortex transcranial direct current stimulation via a combined high definition and conventional electrode montage: a FEM modeling studying,” in New York City, NY: 34th Annual International Conference of the IEEE Engineering in Medicine and Biology Society. EMBS ’12.
Truong, D. Q., Magerowski, G., Blackburn, G. L., Bikson, M., and Alonso-Alonso, M. (2013). Computational modeling of transcranial direct current stimulation (tDCS) in obesity: impact of head fat and dose guidelines. Neuroimage Clin. 2, 759–766. doi: 10.1016/j.nicl.2013.05.011
Valfre, W., Rainero, I., Bergui, M., and Pinessi, L. (2008). Voxel-based morphometry reveals gray matter abnormalities in migraine. Headache 48, 109–117. doi: 10.1111/j.1526-4610.2007.00723.x
Valle, A., Roizenblatt, S., Botte, S., Zaghi, S., Riberto, M., Tufik, S., et al. (2009). Efficacy of anodal transcranial direct current stimulation (tDCS) for the treatment of fibromyalgia: results of a randomized, sham-controlled longitudinal clinical trial. J. Pain Manag. 2, 353–361.
Vaseghi, B., Zoghi, M., and Jaberzadeh, S. (2014). Does anodal transcranial direct current stimulation modulate sensory perception and pain? A meta-analysis study. Clin. Neurophysiol. 125, 1847–1858. doi: 10.1016/j.clinph.2014.01.020
Viganò, A., D’elia, T. S., Sava, S. L., Auvé, M., De Pasqua, V., Colosimo, A., et al. (2013). Transcranial Direct Current Stimulation (tDCS) of the visual cortex: a proof-of-concept study based on interictal electrophysiological abnormalities in migraine. J. Headache Pain 14:23. doi: 10.1186/1129-2377-14-23
Villamar, M. F., Volz, M. S., Bikson, M., Datta, A., Dasilva, A. F., and Fregni, F. (2013a). Technique and considerations in the use of 4x1 ring high-definition transcranial direct current stimulation (HD-tDCS). J. Vis. Exp. 77:e50309. doi: 10.3791/50309
Villamar, M. F., Wivatvongvana, P., Patumanond, J., Bikson, M., Truong, D. Q., Datta, A., et al. (2013b). Focal modulation of the primary motor cortex in fibromyalgia using 4×1-ring high-definition transcranial direct current stimulation (HD-tDCS): immediate and delayed analgesic effects of cathodal and anodal stimulation. J. Pain 14, 371–383. doi: 10.1016/j.jpain.2012.12.007
Vogt, B. A., Derbyshire, S., and Jones, A. K. (1996). Pain processing in four regions of human cingulate cortex localized with co-registered PET and MR imaging. Eur. J. Neurosci. 8, 1461–1473. doi: 10.1111/j.1460-9568.1996.tb01608.x
Wager, T. D., Scott, D. J., and Zubieta, J. K. (2007). Placebo effects on human mu-opioid activity during pain. Proc. Natl. Acad. Sci. U S A 104, 11056–11061. doi: 10.1073/pnas.0702413104
Wagner, T., Fregni, F., Fecteau, S., Grodzinsky, A., Zahn, M., and Pascual-Leone, A. (2007). Transcranial direct current stimulation: a computer-based human model study. Neuroimage 35, 1113–1124. doi: 10.1016/j.neuroimage.2007.01.027
Wassermann, E. M., Wang, B., Zeffiro, T. A., Sadato, N., Pascual-Leone, A., Toro, C., et al. (1996). Locating the motor cortex on the MRI with transcranial magnetic stimulation and PET. Neuroimage 3, 1–9. doi: 10.1006/nimg.1996.0001
Webster, B. R., Celnik, P. A., and Cohen, L. G. (2006). Noninvasive brain stimulation in stroke rehabilitation. NeuroRx 3, 474–481. doi: 10.1016/j.nurx.2006.07.008
Weiller, C., May, A., Limmroth, V., Jüptner, M., Kaube, H., Schayck, R. V., et al. (1995). Brain stem activation in spontaneous human migraine attacks. Nat. Med. 1, 658–660. doi: 10.1038/nm0795-658
Wiegell, M. R., Tuch, D. S., Larsson, H. B., and Wedeen, V. J. (2003). Automatic segmentation of thalamic nuclei from diffusion tensor magnetic resonance imaging. Neuroimage 19, 391–401. doi: 10.1016/s1053-8119(03)00044-2
Wolkenstein, L., and Plewnia, C. (2013). Amelioration of cognitive control in depression by transcranial direct current stimulation. Biol. Psychiatry 73, 646–651. doi: 10.1016/j.biopsych.2012.10.010
Wrigley, P. J., Gustin, S. M., McIndoe, L. N., Chakiath, R. J., Henderson, L. A., and Siddall, P. J. (2013). Longstanding neuropathic pain after spinal cord injury is refractory to transcranial direct current stimulation: a randomized controlled trial. Pain 154, 2178–2184. doi: 10.1016/j.pain.2013.06.045
Zaghi, S., Heine, N., and Fregni, F. (2009). Brain stimulation for the treatment of pain: a review of costs, clinical effects and mechanisms of treatment for three different central neuromodulatory approaches. J. Pain Manag. 2, 339–352.
Keywords: neuromodulation, finite-element modeling, transcranial direct current stimulation, pain, HD-tDCS
Citation: DaSilva AF, Truong DQ, DosSantos MF, Toback RL, Datta A and Bikson M (2015) State-of-art neuroanatomical target analysis of high-definition and conventional tDCS montages used for migraine and pain control. Front. Neuroanat. 9:89. doi: 10.3389/fnana.2015.00089
Received: 10 April 2015; Accepted: 23 June 2015;
Published: 15 July 2015.
Edited by:
James A. Bourne, Australian Regenerative Medicine Institute, AustraliaReviewed by:
James C. Vickers, University of Tasmania, AustraliaCopyright © 2015 DaSilva, Truong, DosSantos, Toback, Datta and Bikson. This is an open-access article distributed under the terms of the Creative Commons Attribution License (CC BY). The use, distribution and reproduction in other forums is permitted, provided the original author(s) or licensor are credited and that the original publication in this journal is cited, in accordance with accepted academic practice. No use, distribution or reproduction is permitted which does not comply with these terms.
*Correspondence: Alexandre F. DaSilva, Headache and Orofacial Pain Effort (H.O.P.E.), Department of Biologic and Materials Sciences and Michigan Center for Oral Health Research (MCOHR), School of Dentistry, University of Michigan, 1011 N. University Avenue, Room 1014A, Ann Arbor, MI 48109-1078, USA,YWRhc2lsdmFAdW1pY2guZWR1
† These authors have contributed equally to this work.
Disclaimer: All claims expressed in this article are solely those of the authors and do not necessarily represent those of their affiliated organizations, or those of the publisher, the editors and the reviewers. Any product that may be evaluated in this article or claim that may be made by its manufacturer is not guaranteed or endorsed by the publisher.
Research integrity at Frontiers
Learn more about the work of our research integrity team to safeguard the quality of each article we publish.