- 1Oklahoma Center for Geroscience and Healthy Brain Aging, University of Oklahoma Health Sciences, Oklahoma City, OK, United States
- 2Vascular Cognitive Impairment and Neurodegeneration Program, Department of Neurosurgery, University of Oklahoma Health Sciences, Oklahoma City, OK, United States
- 3International Training Program in Geroscience, Doctoral School of Basic and Translational Medicine/Institute of Preventive Medicine and Public Health, Semmelweis University, Budapest, Hungary
- 4Department of Nutritional Sciences, College of Allied Health, University of Oklahoma Health Sciences, Oklahoma City, OK, United States
- 5Oklahoma Shared Clinical and Translational Resources, University of Oklahoma Health Sciences, Oklahoma City, OK, United States
- 6Department of Medical Engineering and Technomathematics, Aachen University of Applied Sciences, Juelich, Germany
- 7Department of Health Promotion Sciences, College of Public Health, University of Oklahoma Health Sciences, Oklahoma City, OK, United States
- 8Peggy and Charles Stephenson Cancer Center, University of Oklahoma Health Sciences, Oklahoma City, OK, United States
Introduction: Growing aging populations pose new challenges to public health as the number of people living with dementia grows in tandem. To alleviate the burden of dementia, prodromal signs of cognitive impairment must be recognized and risk factors reduced. In this context, non-invasive techniques may be used to identify early changes and monitor disease progression. Dynamic retinal vessel analysis (DVA) provides an opportunity to measure retinal vasoreactivity in a way that may be comparable to cerebral vasoreactivity, thus providing a window to the brain.
Methods: We conducted a literature search on PubMed and Scopus to identify studies utilizing DVA to describe retinal vasoreactivity in central nervous system diseases and compare it with brain function and structure. We included original papers with full text in English.
Results: We identified 11 studies, of which most employed a cross-sectional design (91%). Studies on cerebrovascular diseases reported that retinal vasoreactivity decreased in patient populations compared with that of healthy controls. Studies on cognitive impairment and dementia yielded mixed results, at least in part due to high population heterogeneity. There is also evidence for the association between DVA and brain and cognition parameters such as cerebral blood flow velocity, cerebral microvascular diffusivity, and cognitive function score.
Discussion: The reviewed papers on DVA and brain function, despite the mixed results, have demonstrated the relationship between retinal vasoreactivity and cerebrovascular function and cognition. Heterogeneity in study populations, procedures, and analyses make comparisons difficult. Studies with larger sample size, clear description of the population and methods, and standardized DVA analysis are needed to elucidate the eye–brain connection and to enhance the translational and clinical applications of DVA.
Introduction
Aging populations around the world pose new challenges to public health and healthcare systems. In the latest report of the Global Burden of Disease (Institute for Health Metrics and Evaluation, 2021), an estimated 57 million people were living with dementia in 2021 worldwide, representing a 46% increase from 2010. By 2050, this number is expected to almost triple to 153 million people (GBD 2018 Dementia Forecasting Collaborators, 2022). Importantly, the most prevalent risk factors for dementia are cardiovascular risk factors: fasting hyperglycemia, obesity, smoking, and hypertension (Farnsworth von Cederwald et al., 2022; McGrath et al., 2022; Song et al., 2021; Chang Wong and Chang Chui, 2022). Thus, cardiovascular risk factor reduction and recognition of the prodromal signs of cognitive impairment are crucial for dementia prevention and to alleviate the burden of this disease.
The retina is part of the central nervous system and has the same embryological origin as the brain, and in some applications is considered as a “window to the brain” (Ptito et al., 2021). The eye has long been used to help diagnose and evaluate systemic diseases like diabetes (Lin et al., 2021), hypertension (Dziedziak et al., 2022), and thyroid gland-related diseases (Bojikian and Francis, 2019). This is one of the few organs where we can non-invasively see the small arteries and veins, as well as arterioles, venules and capillaries with our own eyes in vivo, even red blood cells on certain occasions. Several noninvasive and easily performed techniques provide information about the vascular and neuronal function and structure in the eye. Optical coherent tomography (OCT) produces images of the neuronal fibers and retinal layers. Optical coherent tomography angiography (OCTA), ophthalmoscopy and retinal angiography evaluate the health of the eye vasculature. Meanwhile electrophysiology tests (e.g., visual evoked potential, electroretinography) evaluate the neuronal functions of the retina and the visual pathway. A technique termed dynamic vessel analysis (DVA) produces videos of the retina and allows precise measurements of vessel diameter changes over time. Among other applications, this is used to evaluate functional vascular hyperemia responses upon photoreceptor stimulation with flickering light. DVA has been used in research in the context of several diseases, including eye (Selbach et al., 2014; Iacono et al., 2017), metabolic (Kotliar et al., 2011; Schiel et al., 2009), and vascular (Machalinska et al., 2018; Theuerle et al., 2021) pathologies, among others (Sharifizad et al., 2021; Rabiolo et al., 2016; Csipo et al., 2021).
Using DVA to evaluate brain health is of particular interest. Although more common techniques such as OCT and OCTA can be applied to study the connection between the eye, the brain, and cognitive function (Czako et al., 2020), DVA offers an important advantage in its capacity to measure functional hyperemia because this process can also be measured in the brain (Huneau et al., 2015; Csipo et al., 2019). Though, the exact role and importance of each contributor to functional hyperemia–endothelial cells, Müller glial cells, astrocytes, among others–is still under examination in the eye (Newman, 2013; Pournaras et al., 2008), DVA is a good technique to investigate the same response in a different tissue that shares a common origin with the brain. Moreover, pre-clinical and clinical studies show that impaired functional hyperemia in the brain is associated with worse cognitive performance (Mukli et al., 2024; Tarantini et al., 2017). However, whether functional hyperemia in the retinal vessels can be associated with brain and cognitive health remains underexplored. This narrative review aimed to identify and summarize available studies that employed DVA with its flicker stimulation to investigate brain function and cognitive performance in humans. We also aimed to describe areas for improving the translatability of future studies and the clinical relevance of the technique.
DVA: brief technical background
DVA involves illuminating the retina with a bright green light and collecting diameter values from selected vessels, small arteries and veins, branches of central retinal artery and vein, from high-definition image recordings (25 frames per second). The Dynamic Vessel Analyzer software (Imedos Health GmbH, Jena, Germany) is used to set the stimulation parameters and measurement duration, which typically follows the standard DVA protocol and lasts 350 s (Kotliar et al., 2011; Kotliar et al., 2017; Mandecka et al., 2007). During this time, a baseline period of steady bright green light is followed by alternating cycles of rectangular luminant flickering light (stimulus) at a frequency of 12.5 Hz and steady light (resting). Generally, the length of stimulation and rest, and the number of stimulation cycles can be set up in the software and vary greatly within published methodologies (see Discussion for further details). However, most studies use the mentioned standard DVA protocol: 50 s baseline, 20 s flickering period, 80 s recovery, 3 cycles, described in detail elsewhere (Kotliar et al., 2011; Kotliar et al., 2017; Albanna et al., 2016; Mandecka et al., 2007; Kneser et al., 2009). Using the recorded images, the software calculates baseline vessel diameters along with the maximal dilation and maximal constriction values, providing these results as a relative in percentage compared with the baseline value. Other available data include the raw individual diameter values, the smoothed data, and the average diameter per second. Some studies derive additionally more meaningful parameters of retinal vessel diameter response to flicker, e.g., times of maximal constriction and dilation, areas under the flicker response curves, etc. (Kotliar et al., 2011; Kotliar et al., 2017). We direct the reader to a consensus paper by Garhofer et al. (2010) for more details on how to use the DVA device. Figure 1 presents a simplified setup of modern DVA system and output.
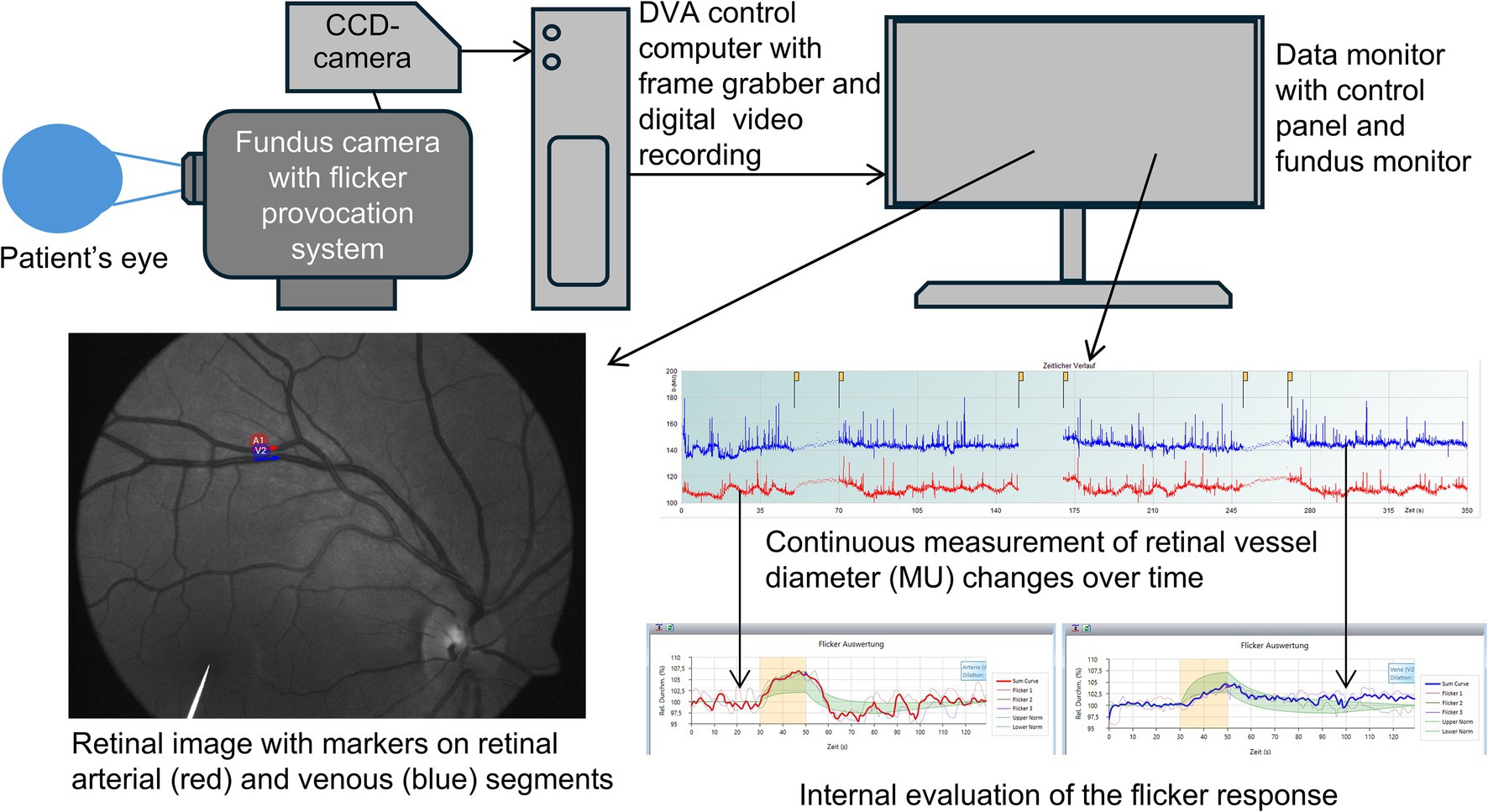
Figure 1. Simplified setup of the dynamic retinal vessel analysis system and representative findings. The image is reproduced with permission from the subject. CCD, charge-coupled device.
DVA and brain function
To identify published studies on DVA and brain function, we searched PubMed and Scopus for interventional and observational human studies using the terms “dynamic vessel analysis,” “brain,” “cognition,” “cognitive,” “flicker,” and “flickering” without publication year restrictions. The search yielded nine publications; of these, one was excluded because it was a conference abstract. Three papers were identified by screening the reference lists. Of the eleven studies, one was longitudinal, the others were cross-sectional, from which two utilized a population-based cohort (the Maastricht study). Table 1 summarizes the characteristics of the retrieved studies. Supplementary Table 1 details the comorbidities of participants reported in the retrieved studies.
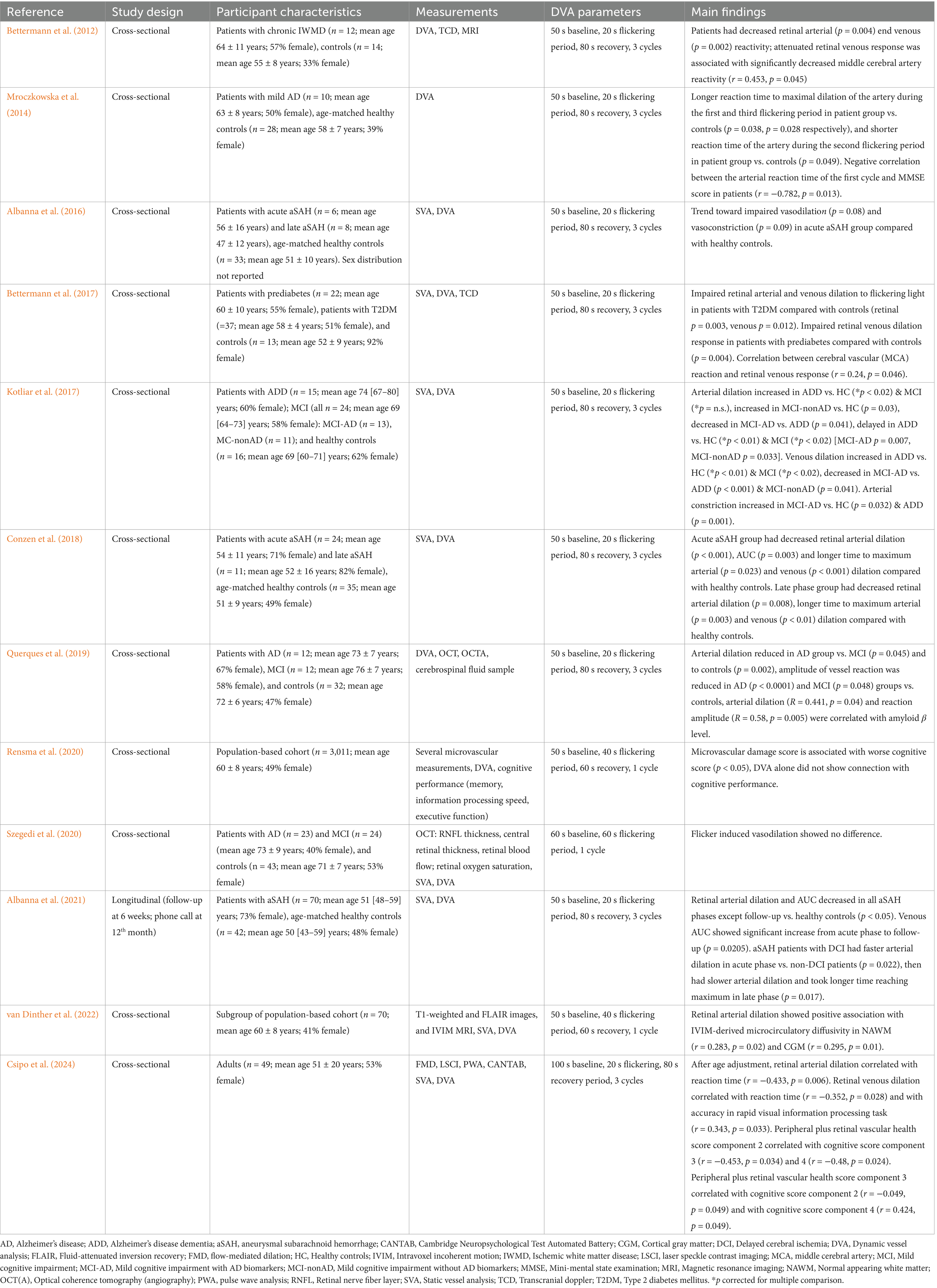
Table 1. Characteristics of studies presented in this review investigating the relationship between retinal vasoreactivity and brain function.
DVA and cerebrovascular function
Magnetic resonance imaging (MRI) offers a good opportunity for evaluating cerebrovascular function. However, this imaging modality is limited to the macrovasculature and the consequences of vascular pathologies, while microvascular changes remain imperceptible (Stringer et al., 2021). In this context, the intravoxel incoherent motion (IVIM) MRI was proposed to assess the brain microvasculature, allowing the detection of blood flow and fast directional changes in small cerebral vessels (Paschoal et al., 2018). Importantly, lower microvascular perfusion in the normal appearing white matter and grey matter measured by IVIM MRI were associated with lower cognitive performance in patients with small vessel disease (Zhang et al., 2017). A subgroup from the population-based cohort of the Maastricht study underwent IVIM MRI and the scan findings were compared with retinal vasoreactivity results (van Dinther et al., 2022). The authors reported that the retinal arterial dilation to flicker stimulus was associated with the pseudo-diffusion in the normal-appearing white matter and the cortical gray matter (adjusted to age, sex, and cardiovascular risk factors). The microcirculatory diffusivity is depending on the microvascular blood flow velocity and architecture of the microvascular bed in the normal-appearing white and cortical matter (van Dinther et al., 2022). With these results, the authors demonstrated that retinal vessel function and cerebral microvasculature properties are related and, with additional longitudinal investigations on a larger population, retinal vasoreactivity is a promising assessment to evaluate cerebral microvascular function (van Dinther et al., 2022).
DVA and cerebrovascular diseases
Chronic ischemic white matter disease
Chronic ischemic white matter disease (IWMD) is a steppingstone in the development of stroke and dementia (Chang Wong and Chang Chui, 2022). Using the eye for detecting early, subclinical signs of IWMD could help prevent or delay the progression of small vessel disease to dementia. A small pilot study found that people with chronic IWMD had decreased retinal arterial and venous reactivity to flickering light stimulus compared with healthy controls (Bettermann et al., 2012). The attenuated retinal venous response was significantly associated with decreased middle cerebral artery reactivity, which was defined as change in flow velocity in response to hyperventilation (breath-hold maneuver), measured by transcranial Doppler sonography. This association persisted after adjustment for age and vascular risk factors. However, the retinal arterial response was not correlated with the middle cerebral artery reactivity. Decreased arterio-venous ratio in the eye around the papilla was also significantly associated with decreased cerebral vasoreactivity in the IWMD group. The smaller arterio-venous ratio was due to increased venous diameters (Bettermann et al., 2012), which is associated as a significant risk factor for stroke (Girach et al., 2024). Importantly, some of the participants were administered phenylephrine for pupil dilation, which is an alfa-receptor agonist and may affect the arteries in the eye and thus influence their diameter and hence the results of DVA measurements (Lanzl et al., 2002; Bettermann et al., 2012).
Acute aneurysmal subarachnoid hemorrhage
Acute aneurysmal subarachnoid hemorrhage (aSAH) can be followed by delayed cerebral ischemia (DCI) in 20–30% of cases, which worsens the prognosis of these patients; therefore, it is crucial that the appropriate therapy is administered in a timely manner (Ikram et al., 2021). A research group conducted cross-sectional (Albanna et al., 2016; Conzen et al., 2018) and prospective observational (Albanna et al., 2021) studies with aSAH patients and age-matched controls. They investigated whether DVA is a feasible assessment of the diameter and function of retinal vessels in this population and whether the technique could be used as a tool for predicting and monitoring the vascular outcomes of patients with aSAH. First, they recruited separate patients in the acute (within 2–14 days (Albanna et al., 2016) and 5–14 days (Conzen et al., 2018) of aSAH) and late [5 ± 2 months (Albanna et al., 2016) and 3 months (Conzen et al., 2018) after aSAH] phases and compared them with healthy age-matched controls in their cross-sectional studies. Interestingly, these two studies found different results in some parameters. The first pilot cross-sectional study (Albanna et al., 2016) found significantly dilated retinal arteries in aSAH patients compared with controls, which persisted when comparing the controls with patients in the late phase, albeit with a smaller but still significant difference. They also found a trend toward impaired arterial vasodilation and-constriction in the acute patient group compared with healthy controls, although this did not reach statistical significance. This impairment was attenuated in patients in the late phase (Albanna et al., 2016). The later cross-sectional study (Conzen et al., 2018) showed that arterial and venous diameters were decreased in the acute phase group compared with those of healthy controls, a difference that lessened to a trend in the late phase group, showing no more significance. The acute phase group had decreased retinal arterial dilation and area under the flicker response curve (AUC) as well as increased time to reach the maximal arterial and venous dilation compared with the healthy control group. Similarly, the late phase group also showed decreased arterial dilation and longer time to reach the maximal arterial and venous dilation than their healthy counterparts, although AUC values were not significantly different between the groups (control vs. late phase SAH: median arterial AUC 85.7 [interquartile range (IQR) 56.8–102.9] vs. 59.9 [IQR 38.5–106.9] %.s; median venous AUC 101 [IQR 81.7–128.1] vs. 91.1 [IQR 43.6–101.8] %.s) (Conzen et al., 2018).
Finally, the research group reported on the findings of a longitudinal study (Albanna et al., 2021) in which 70 patients with aSAH were followed up for more than 6 weeks after primary lesion occurrence. Patients in all phases after SAH had narrower retinal arteries and veins compared with healthy controls. During the acute phase, patients had decreased retinal arterial dilation and AUC compared with their healthy counterparts (control vs. aSAH: median arterial AUC 51.4 [IQR 32.5–69.7] vs. 21.5 [IQR 9.4–35.8] %.s) while the vessel dilation time course remained unchanged. At follow-up, these initial differences were no longer observed. From parameters related to venous reactivity, only the AUC showed a difference between phases, increasing at follow-up (52.4 [IQR 34.2–61.7] %.s) compared with the acute phase (41.3 [IQR 16.4–46.8] %.s). This study also reported differences between patients who did and did not develop DCI after SAH. During the acute phase, patients who later developed DCI had faster arterial dilation compared with those who did not develop DCI. This difference changed its direction in the late phase, when patients with DCI had an increased time to reach the maximal vessel dilation after stimulus (Albanna et al., 2021).
Patients with aSAH often receive nimodipine treatment, which is an L-type Ca2+ channel antagonist and may affect vessel reactivity. However, in the longitudinal study (Albanna et al., 2021), the authors found no evidence that the drug influenced the vessel reactivity parameters. Taken together, these studies demonstrated that DVA is feasible in patients with aSAH, and may work as a simple, non-invasive monitoring technique across different phases of the disease. However, further investigations are needed to place this measurement in the clinical setting.
Stroke
Stroke risk is increased for people with diabetes as hyperglycemia injuries blood vessels, causing changes in microvascular behavior that are evident in both retinal and cerebrovascular vessels (Kim et al., 2008; Mandecka et al., 2007). Therefore, a study investigated whether the responsiveness of the retinal and cerebrovascular vessels is correlated in participants with prediabetes and type 2 diabetes (Bettermann et al., 2017). The study used DVA to assess the retinal vascular response to flickering light stimuli, and transcranial Doppler sonography to measure middle cerebral artery reactivity at rest and after a hyperventilation/breath-hold maneuver. They found that patients with prediabetes had attenuated retinal vascular reaction to flickering light stimulation compared with controls, whereas patients with type 2 diabetes had a decrease in both arterial and venous response after stimulation compared with controls. The authors also reported that cerebral vessel reactivity was directly correlated with retinal venous vasodilation in these patients. These results persisted after adjusting for age, hypertension, and other comorbidities (Bettermann et al., 2017). However, the authors reported that around half of the participants with type 2 diabetes needed extra phenylephrine eye drops for pupil dilation, which may affect the tone of the retinal arteries and influence the results of DVA. Further, it is unclear whether DVA results were adjusted for systemic blood pressure even though they reported continuously monitoring blood pressure during the measurements (Bettermann et al., 2017).
DVA and cognitive function and dementia
Alzheimer’s disease
Alzheimer’s disease (AD) is considered a neurodegenerative disease, but emerging evidence shows that it is a multifactorial disease with vascular contributions (Mehta and Mehta, 2023; Dumitrascu et al., 2024), with preclinical evidence indicating that endothelial dysfunction precedes the accumulation of amyloid beta plaques (Waigi et al., 2024). Although structural changes of AD and related dementias are detectable in the retina, such as the thinning of the peripapillary retinal nerve fiber layer and the macula, decreasing complexity of the vasculature, increased tortuosity (Czako et al., 2020), and amyloid plaque accumulations around the periarteriolar region (Dumitrascu et al., 2024), whether functional impairments in vasoreactivity can also be detected in the retina in early stages of cognitive impairment and dementia remains unclear. Kotliar et al. (2017) used DVA to investigate the differences in retinal reactivity in patients with AD dementia (ADD), mild cognitive impairment (MCI), and cognitively intact controls. The MCI group was divided to two additional groups based on the presence (MCI-AD) or absence (MCI-nonAD) of AD biomarkers for certain analysis. They found that both arterial and venous dilation was increased in the ADD group compared with the MCI and control groups. The reaction amplitude of the arteries both in the ADD and MCI groups were enhanced compared with controls. Additionally, they observed that the course of events during the DVA procedure was different in patients with ADD compared with patients with MCI and controls, with delayed arterial dilation and no rapid constriction following stimulation. In contrast, patients with MCI-AD exhibited stronger constrictions after the flickering light stimulation compared with controls and the ADD group, however patients with MCI-AD had decreased both arterial and venous dilation compared with patients with ADD, and decreased venous dilation compared with MCI-nonAD group. Lastly, the MCI-nonAD group showed increased arterial dilation compared with controls (Kotliar et al., 2017). Although these results were surprising, the authors suggest that the enhanced arterial and venous dilations and delayed vessel responses in MCI and ADD are related to neuronal components that are known to be impaired in AD. Moreover, it has been shown that the blood-oxygen-level-dependent (BOLD) response in the brain of patients with AD is also delayed (Rombouts et al., 2005).
Interestingly, Querques et al. (2019) found contrasting results when using the same DVA measurement protocol to assess arterial reactivity in patients with AD, MCI, and healthy controls. They reported that arterial dilation was decreased during flickering light stimulus in the AD group, and that the reaction amplitude was smaller in the AD and MCI groups compared with that observed in the control group. They also assessed amyloid-beta levels in the cerebrospinal fluid of participants and reported a direct correlation between amyloid-beta levels and arterial dilation and reaction amplitude (Querques et al., 2019). Importantly, some concerns regarding the characterization of the cohorts in this study warrant caution when interpreting their findings: the AD and MCI groups were not well characterized in terms of disease stage and underlying disorder, and the prevalence of hypertension was specified in the AD and MCI groups, but not in the control group. Hypertension influences retinal arterial reactions, representing an important confounder (Machalinska et al., 2018; Peregud-Pogorzelska et al., 2020).
A third study led by Szegedi et al. (2020) also investigated retinal vessel diameter reactivity to flickering light in patients with AD, MCI, and healthy controls. The authors reported no significant differences in retinal reactivity between patient and control groups. However, it is important to note that they did not define the maximal dilation calculation in their report. Lastly, a brief report authored by Mroczkowska et al. (2014) investigated the association between mild AD and retinal vasoreactivity. Their results showed that the arterial reaction of patients with mild AD was slower during the first and third (last) flickering period compared with that of healthy controls. In contrast, the arterial reaction was quicker during the second flickering period in the mild AD group compared with the control group. Furthermore, the arterial reaction time during the first flickering period was negatively correlated with the Mini Mental State score in patients with mild AD, but no correlation was observed with the following two flickering periods. The authors did not discuss possible explanations for these different associations between cognitive performance and flickering periods. These disparate results reflect the heterogeneity in DVA studies. For instance, while three of the four studies used the same DVA protocol (Kotliar et al., 2017; Querques et al., 2019; Mroczkowska et al., 2014), the third study administered a different protocol with only one 60-s baseline cycle and then one 60-s flickering light stimulation period (Szegedi et al., 2020). Further complicating direct comparisons between the studies, that each of them analyzed the DVA data with a different method. Finally, only one of the studies attempted to correlate retinal vasoreactivity with cognitive performance. Future studies on DVA and ADD should characterize their patients in terms of disease stage and comorbidities as these may affect flicker responses and contribute to the heterogeneity in study findings.
DVA and cognitive performance
In a large population-based cohort study (the Maastricht study), researchers devised a microvascular damage score based on several microvascular measurements, including DVA. This score was compared with cognitive performance assessed with a neuropsychological battery in over 3,000 adults (Rensma et al., 2020). The authors found that the microvascular damage score showed a significant inverse association with cognitive performance (i.e., a higher microvascular damage score was associated with a lower cognitive performance score). However, when they analyzed retinal vasodilation response alone, they found no correlation with cognitive performance (Rensma et al., 2020).
Recently, a pilot cross-sectional study investigated the relationship of peripheral and retinal vascular health with cognitive performance (Csipo et al., 2024). This study utilized macro-and microvascular endothelial function measurements, static retinal vessel analysis, and DVA to assess vascular health, and a cognitive battery to assess cognitive performance in domains such as memory, attention, processing speed, and reaction time. When comparing DVA parameters with cognitive performance, age-adjusted analyses showed that retinal arterial and venous dilation were significantly associated with reaction time, and retinal venous dilation was significant correlated with accuracy in the rapid visual processing task. Next, the authors compared cognitive performance parameters with vascular health composite scores with and without retinal vessel measurements as loading variables in the principal component analysis. Interestingly, the authors reported more significant associations with cognitive performance parameters when retinal vascular analyses were included in the composite scores, indicating the importance of including retinal vessel analyses when investigating vascular health and cognitive performance.
Discussion
In this review, we presented and discussed several studies that investigated the connection between retinal vasoreactivity using DVA and brain function. Although the significance of connection remains unclear, most studies reported changes in retinal vasoreactivity in disease states, thereby meriting larger studies designed to elucidate the many different factors that influence retinal vasoreactivity in health and disease. Several questions remain that limit the clinical utility of DVA: (i) What is the normal range of retinal vessel vasodilation as a response to flickering light stimulation? (ii) How does systemic blood pressure affect retinal responsiveness to stimulation? (iii) What is the best approach to analyze DVA data? (iv) Are there other methodological approaches to investigate brain function using DVA technology that can give us a clue to brain health? Regarding the first question, Streese et al. (2021) conducted a study with 277 participants to assess the normal dilation and constriction responses across different age groups in healthy participants aged between 20 and 82 years old. They reported that the maximal arterial dilation induced by flickering light stimulation did not change significantly with age, whereas the venous dilation response to stimulation decreased with increasing age. They also reported that arterial and venous vessel diameters decreased with aging. However, the number of participants in the study is too small for generating normative data and larger, population-based studies are needed to answer this question. Other studies investigating the association between age and DVA parameters found that the retinal arterial constriction was significantly decreased in older compared with younger individuals (Kneser et al., 2009; Seshadri et al., 2016). A study performed by our group reported decreased maximal retinal arterial dilation to flickering light stimulation in older compared with young participants (Lipecz et al., 2019).
Regarding the effect of blood pressure on retinal vasoreactivity, the above mentioned Streese et al. (2021) explained the unchanged arterial response with age with the increasing blood pressure in the older population. The higher blood pressure was also associated with the decreasing vessel diameters. Additionally, blood pressure and the central retinal arterial equivalent (summarized diameters of the arteries around the papilla) were positively and negatively associated, respectively, with flickering light-induced retinal arterial vasodilation (Streese et al., 2021). Another study by the same group showed that exercise-induced increase in blood pressure during DVA (in between baseline and flickering light period) increased the maximal retinal arterial dilation, and that healthy athletic individuals have a smaller baseline arterial diameter (Streese et al., 2021). However, it is important to distinguish chronic high blood pressure (uncontrolled hypertension) from acute higher blood pressure (e.g., due to stress and nervousness from the measurement, or due to exercise), as the former causes permanent narrowing and arteriosclerosis in the wall of the retinal arteries/arterioles over time (Cheung et al., 2022). Other studies that investigated the effect of blood pressure during or before DVA on retinal vessel diameters and vasoreactivity to flickering light in healthy individuals found no significant association (Nagel et al., 2006; Garhofer et al., 2003; Sorensen et al., 2017). Finally, while some studies (Bettermann et al., 2017; Bettermann et al., 2012) presented in this review collected blood pressure data and used it to normalize DVA results, this is not standard practice as continuous blood pressure monitoring requires an additional device connected to the DVA system. However, because of the above-mentioned reasons, recording the blood pressure at least at the beginning and at the end of the DVA measurement is advised by the manufacturer (Vilser, 2019).
Regarding analytical approaches, there are several differences between reports, contributing to the variety in results within this topic and precluding direct comparisons between studies on the same topic. Analyses differ in baseline length, maximal vascular dilation and constriction method of calculation, use of absolute or mean diameter values, and parameters calculated from raw diameter values. Moreover, descriptions of analytical procedures, data preprocessing, and calculations are not always reported in sufficient detail. Some research groups have attempted to provide solutions for standardizing the protocol and analysis of DVA measurements (Streese et al., 2021; Kotliar et al., 2011), but no widely accepted standard currently exists. Kotliar et al. (2011) have reported perhaps the most thorough DVA protocol in their methodology paper, which was used by some of the reviewed papers (Albanna et al., 2016; Kotliar et al., 2017). One reviewed study used a different approach, which is called sequential and diameter response analysis (Mroczkowska et al., 2014). This method considers the fluctuation of the baseline diameter and separates the analysis of the 3 cycles, resulting in additional variables, such as baseline-corrected flicker response and baseline diameter fluctuation next to the commonly used maximal dilation, constriction and other variables explaining the time course (Heitmar et al., 2010). Adjustment of the dilation with the baseline diameter was proposed by Nagel and colleagues, and although their results did not offer significant evidence for the effect of baseline diameter on maximal dilation (Nagel et al., 2004), other studies found a connection between baseline diameters and stimulation-induced maximal vessel dilation in small (Streese et al., 2021) and large vessels (Rembold et al., 2003). Of note, the inventors of DVA technology advise utilizing signal averaging when performing functional analyses of endothelial response to flicker stimulation (Vilser, 2019). This smoothing processing makes the DVA signal more stable and, where feasible, corrects inaccuracies and measurement errors.
The papers described in this review did not utilize the baseline-corrected flicker response analysis method except for Mroczkowska et al. (2014). Along with the variations in analytical approaches, we also observed considerable variations in the stimulus protocol. Table 1 includes the DVA protocol parameters used by each study. The flickering duration, recovery period, and number of cycles varied among studies. These differences may influence the physiological response induced with the flickering light and may diminish the meaning of comparisons between studies, ultimately affecting the clinical utility potential of DVA.
Finally, alternative methodological approaches may offer additional advantages compared with the most used flickering light assessment. Notably, flickering light-induced vasoreactivity must account for the baseline vessel diameter, which varies depending on systemic blood pressure (Dziedziak et al., 2022; Tapp et al., 2019) and physical activity (Streese et al., 2019), among other factors. Furthermore, the specific effect of the baseline diameter on the features of the dilation and the underlying mechanisms are still not fully understood. Thus, for flickering light-induced reactivity to truly reflect brain function, it must be adjusted accordingly. To gain further insight into the physiological and pathophysiological processes at play, it is essential to assess the retinal vessel behavior in greater depth. To overcome the limitations of the current approach and achieve more accurate results, it would be beneficial to consider additional aspects of retinal vessel dynamics and include complementary assessments such as oscillations and vasomotions. An animal study using an Alzheimer’s mice model reported that increased arterial vasomotions in mouse brain were associated with paravascular clearance (van Veluw et al., 2020), which is comparable with human studies utilizing DVA in patients diagnosed with AD reporting enhanced retinal arterial vasomotion compared with healthy individuals (Kotliar et al., 2022) and positively correlating arterial pulsation amplitude with neocortical amyloid beta scores (Golzan et al., 2017). Investigating pulsations and vasomotions of retinal vessels requires the assessment of the corresponding magnitudes, periodicity of the oscillations, their spectral characteristics, and wave forms, which can indirectly provide valuable information about vascular stiffness and the contractile state of the selected vessels (Kotliar et al., 2022; Vilser et al., 2002).
Another alternative methodological approach is combining other retinal imaging techniques with DVA to improve the visualization of vascular pathologies in the retina. Although the possibility of generating compact scores using static analyses such as OCT, OCTA, and static vessel analysis could simplify the interpretation of comprehensive vascular assessments, it is unclear how DVA parameters correlate with those obtained with other retinal imaging techniques. Among the studies summarized in this review, only one compared DVA with another retinal imaging method (OCT). Szegedi et al. (2020) reported no significant association between vessel reactivity and retinal nerve fiber layer thickness.
In conclusion, DVA provides an accessible method for non-invasive functional measurement of the retinal vasoreactivity. As part of the central nervous system, the retina has potential to become a surrogate tissue to assess cerebrovascular function and pathology. However, there is still a long road ahead to elucidate details of the relationship between retinal and cerebral functional vascular reactivity and how it relates to brain health. Methodological consensus as well as larger studies are needed to further promote the clinical utility of DVA.
Author contributions
AP: Conceptualization, Data curation, Investigation, Methodology, Visualization, Writing – original draft, Writing – review & editing. AP-L: Visualization, Writing – review & editing. ZS: Writing – review & editing. MM: Writing – review & editing. ZK: Writing – review & editing. CA: Writing – review & editing. CP: Writing – review & editing. PM: Writing – review & editing. KK: Supervision, Visualization, Writing – review & editing. AY: Conceptualization, Funding acquisition, Methodology, Supervision, Writing – review & editing.
Funding
The author(s) declare that financial support was received for the research, authorship, and/or publication of this article. The authors declare that this study received funding from National Institute on Aging of the National Institutes of Health under Award Number R01AG075834, R21AG080775, K99AG088478, and by the American Heart Association under Award Number 966924, 24CDA1276855, 24DIVSUP1282484. The funder was not involved in the study design, collection, analysis, interpretation of data, the writing of this article, or the decision to submit it for publication.
Conflict of interest
The authors declare that the research was conducted in the absence of any commercial or financial relationships that could be construed as a potential conflict of interest.
Generative AI statement
The authors declare that no Gen AI was used in the creation of this manuscript.
Publisher’s note
All claims expressed in this article are solely those of the authors and do not necessarily represent those of their affiliated organizations, or those of the publisher, the editors and the reviewers. Any product that may be evaluated in this article, or claim that may be made by its manufacturer, is not guaranteed or endorsed by the publisher.
Supplementary material
The Supplementary material for this article can be found online at: https://www.frontiersin.org/articles/10.3389/fnagi.2024.1517368/full#supplementary-material
References
Albanna, W., Conzen, C., Weiss, M., Clusmann, H., Fuest, M., Mueller, M., et al. (2016). Retinal vessel analysis (RVA) in the context of subarachnoid hemorrhage - a proof of concept study. PLoS One 11:e0158781. doi: 10.1371/journal.pone.0158781
Albanna, W., Conzen, C., Weiss, M., Seyfried, K., Kotliar, K., Schmidt, T. P., et al. (2021). Non-invasive assessment of neurovascular coupling after aneurysmal subarachnoid hemorrhage: a prospective observational trial using retinal vessel analysis. Front. Neurol. 12:690183. doi: 10.3389/fneur.2021.690183
Bettermann, K., Slocomb, J. E., Shivkumar, V., and Lott, M. E. (2012). Retinal vasoreactivity as a marker for chronic ischemic white matter disease? J. Neurol. Sci. 322, 206–210. doi: 10.1016/j.jns.2012.05.041
Bettermann, K., Slocomb, J., Shivkumar, V., Quillen, D., Gardner, T. W., and Lott, M. E. (2017). Impaired retinal Vasoreactivity: an early marker of stroke risk in diabetes. J. Neuroimaging 27, 78–84. doi: 10.1111/jon.12412
Bojikian, K. D., and Francis, C. E. (2019). Thyroid eye disease and myasthenia gravis. Int. Ophthalmol. Clin. 59, 113–124. doi: 10.1097/IIO.0000000000000277
Chang Wong, E., and Chang Chui, H. (2022). Vascular Cognitive Impairment and Dementia. Continuum (Minneap Minn) 28, 750–780. doi: 10.1212/CON.0000000000001124
Cheung, C. Y., Biousse, V., Keane, P. A., Schiffrin, E. L., and Wong, T. Y. (2022). Hypertensive eye disease. Nat. Rev. Dis. Primers 8:14. doi: 10.1038/s41572-022-00342-0
Conzen, C., Albanna, W., Weiss, M., Kurten, D., Vilser, W., Kotliar, K., et al. (2018). Vasoconstriction and impairment of neurovascular coupling after subarachnoid hemorrhage: a descriptive analysis of retinal changes. Transl. Stroke Res. 9, 284–293. doi: 10.1007/s12975-017-0585-8
Csipo, T., Lipecz, A., Mukli, P., Péterfi, A., Szarvas, Z., Ungvari, A., et al. (2024). Advancing prediction of age-related vascular cognitive impairment based on peripheral and retinal vascular health in a pilot study: a novel comprehensive assessment developed for a prospective workplace-based cohort (the Semmelweis study). Gero Science. doi: 10.1007/s11357-024-01447-y
Csipo, T., Lipecz, A., Owens, C., Mukli, P., Perry, J. W., Tarantini, S., et al. (2021). Sleep deprivation impairs cognitive performance, alters task-associated cerebral blood flow and decreases cortical neurovascular coupling-related hemodynamic responses. Sci. Rep. 11:20994. doi: 10.1038/s41598-021-00188-8
Csipo, T., Mukli, P., Lipecz, A., Tarantini, S., Bahadli, D., Abdulhussein, O., et al. (2019). Assessment of age-related decline of neurovascular coupling responses by functional near-infrared spectroscopy (fNIRS) in humans. Gero Science 41, 495–509. doi: 10.1007/s11357-019-00122-x
Czako, C., Kovacs, T., Ungvari, Z., Csiszar, A., Yabluchanskiy, A., Conley, S., et al. (2020). Retinal biomarkers for Alzheimer’s disease and vascular cognitive impairment and dementia (VCID): implication for early diagnosis and prognosis. Gero Science 42, 1499–1525. doi: 10.1007/s11357-020-00252-7
Dumitrascu, O. M., Doustar, J., Fuchs, D. T., Koronyo, Y., Sherman, D. S., Miller, M. S., et al. (2024). Retinal peri-arteriolar versus peri-venular amyloidosis, hippocampal atrophy, and cognitive impairment: exploratory trial. Acta Neuropathol. Commun. 12:109. doi: 10.1186/s40478-024-01810-2
Dziedziak, J., Zaleska-Zmijewska, A., Szaflik, J. P., and Cudnoch-Jedrzejewska, A. (2022). Impact of arterial hypertension on the eye: a review of the pathogenesis, diagnostic methods, and treatment of hypertensive retinopathy. Med. Sci. Monit. 28:e935135. doi: 10.12659/MSM.935135
Farnsworth von Cederwald, B., Josefsson, M., Wahlin, A., Nyberg, L., and Karalija, N. (2022). Association of Cardiovascular Risk Trajectory with Cognitive Decline and Incident Dementia. Neurology 98, e2013–e2022. doi: 10.1212/WNL.0000000000200255
Garhofer, G., Bek, T., Boehm, A. G., Gherghel, D., Grunwald, J., Jeppesen, P., et al. (2010). Use of the retinal vessel analyzer in ocular blood flow research. Acta Ophthalmol. 88, 717–722. doi: 10.1111/j.1755-3768.2009.01587.x
Garhofer, G., Zawinka, C., Huemer, K. H., Schmetterer, L., and Dorner, G. T. (2003). Flicker light-induced vasodilatation in the human retina: effect of lactate and changes in mean arterial pressure. Invest. Ophthalmol. Vis. Sci. 44, 5309–5314. doi: 10.1167/iovs.03-0587
GBD 2018 Dementia Forecasting Collaborators (2022). Estimation of the global prevalence of dementia in 2019 and forecasted prevalence in 2050: an analysis for the Global Burden of Disease Study 2019. Lancet Public Health 7, e105–e125. doi: 10.1016/S2468-2667(21)00249-8
Girach, Z., Sarian, A., Maldonado-Garcia, C., Ravikumar, N., Sergouniotis, P. I., Rothwell, P. M., et al. (2024). Retinal imaging for the assessment of stroke risk: a systematic review. J. Neurol. 271, 2285–2297. doi: 10.1007/s00415-023-12171-6
Golzan, S. M., Goozee, K., Georgevsky, D., Avolio, A., Chatterjee, P., Shen, K., et al. (2017). Retinal vascular and structural changes are associated with amyloid burden in the elderly: ophthalmic biomarkers of preclinical Alzheimer’s disease. Alzheimers Res. Ther. 9:13. doi: 10.1186/s13195-017-0239-9
Heitmar, R., Blann, A. D., Cubbidge, R. P., Lip, G. Y., and Gherghel, D. (2010). Continuous retinal vessel diameter measurements: the future in retinal vessel assessment? Invest. Ophthalmol. Vis. Sci. 51, 5833–5839. doi: 10.1167/iovs.09-5136
Huneau, C., Benali, H., and Chabriat, H. (2015). Investigating human neurovascular coupling using functional neuroimaging: a critical review of dynamic models. Front. Neurosci. 9:467. doi: 10.3389/fnins.2015.00467
Iacono, P., Parodi, M. B., La Spina, C., Zerbini, G., and Bandello, F. (2017). Dynamic and static vessel analysis in patients with retinitis Pigmentosa: a pilot study of vascular diameters and functionality. Retina 37, 998–1002. doi: 10.1097/IAE.0000000000001301
Ikram, A., Javaid, M. A., Ortega-Gutierrez, S., Selim, M., Kelangi, S., Anwar, S. M. H., et al. (2021). Delayed cerebral ischemia after subarachnoid hemorrhage. J. Stroke Cerebrovasc. Dis. 30:106064. doi: 10.1016/j.jstrokecerebrovasdis.2021.106064
Institute for Health Metrics and Evaluation. (2021). Alzheimer’s disease and other dementias - level 3 cause. Available at: https://www.healthdata.org/research-analysis/diseases-injuries-risks/factsheets/2021-alzheimers-disease-and-other-dementias [Accessed August 6, 2024].
Kim, Y. S., Immink, R. V., Stok, W. J., Karemaker, J. M., Secher, N. H., and van Lieshout, J. J. (2008). Dynamic cerebral autoregulatory capacity is affected early in type 2 diabetes. Clin. Sci. (Lond.) 115, 255–262. doi: 10.1042/CS20070458
Kneser, M., Kohlmann, T., Pokorny, J., and Tost, F. (2009). Age related decline of microvascular regulation measured in healthy individuals by retinal dynamic vessel analysis. Med. Sci. Monit. 15:CR436-441
Kotliar, K., Hauser, C., Ortner, M., Muggenthaler, C., Diehl-Schmid, J., Angermann, S., et al. (2017). Altered neurovascular coupling as measured by optical imaging: a biomarker for Alzheimer’s disease. Sci. Rep. 7:12906. doi: 10.1038/s41598-017-13349-5
Kotliar, K., Lanzl, I. M., Schmidt-Trucksass, A., Sitnikova, D., Ali, M., Blume, K., et al. (2011). Dynamic retinal vessel response to flicker in obesity: a methodological approach. Microvasc. Res. 81, 123–128. doi: 10.1016/j.mvr.2010.11.007
Kotliar, K., Ortner, M., Conradi, A., Hacker, P., Hauser, C., Gunthner, R., et al. (2022). Altered retinal cerebral vessel oscillation frequencies in Alzheimer’s disease compatible with impaired amyloid clearance. Neurobiol. Aging 120, 117–127. doi: 10.1016/j.neurobiolaging.2022.08.012
Lanzl, I., Kotliar, K., and Vilser, W. (2002). Are retinal branch artery diameters influenced by phenylephrine 10% eye drop application? Invest. Ophthalmol. Vis. Sci. 43:3290.
Lin, K. Y., Hsih, W. H., Lin, Y. B., Wen, C. Y., and Chang, T. J. (2021). Update in the epidemiology, risk factors, screening, and treatment of diabetic retinopathy. J. Diabetes Investig. 12, 1322–1325. doi: 10.1111/jdi.13480
Lipecz, A., Csipo, T., Tarantini, S., Hand, R. A., Ngo, B. N., Conley, S., et al. (2019). Age-related impairment of neurovascular coupling responses: a dynamic vessel analysis (DVA)-based approach to measure decreased flicker light stimulus-induced retinal arteriolar dilation in healthy older adults. Geroscience 41, 341–349. doi: 10.1007/s11357-019-00078-y
Machalinska, A., Pius-Sadowska, E., Babiak, K., Salacka, A., Safranow, K., Kawa, M. P., et al. (2018). Correlation between flicker-induced retinal vessel vasodilatation and plasma biomarkers of endothelial dysfunction in hypertensive patients. Curr. Eye Res. 43, 128–134. doi: 10.1080/02713683.2017.1358372
Mandecka, A., Dawczynski, J., Blum, M., Muller, N., Kloos, C., Wolf, G., et al. (2007). Influence of flickering light on the retinal vessels in diabetic patients. Diabetes Care 30, 3048–3052. doi: 10.2337/dc07-0927
McGrath, E. R., Beiser, A. S., O’Donnell, A., Himali, J. J., Pase, M. P., Satizabal, C. L., et al. (2022). Determining vascular risk factors for dementia and dementia risk prediction across mid-to later life: the Framingham heart study. Neurology 99, e142–e153. doi: 10.1212/WNL.0000000000200521
Mehta, R. I., and Mehta, R. I. (2023). The vascular-immune hypothesis of Alzheimer’s disease. Biomedicines 11, 1–18. doi: 10.3390/biomedicines11020408
Mroczkowska, S., Benavente-Perez, A., Patel, S., Qin, L., Bentham, P., and Gherghel, D. (2014). Retinal vascular dysfunction relates to cognitive impairment in Alzheimer disease. Alzheimer Dis. Assoc. Disord. 28, 366–367. doi: 10.1097/WAD.0b013e3182a2e221
Mukli, P., Pinto, C. B., Owens, C. D., Csipo, T., Lipecz, A., Szarvas, Z., et al. (2024). Impaired neurovascular coupling and increased functional connectivity in the frontal cortex predict age-related cognitive dysfunction. Adv. Sci. 11:2303516. doi: 10.1002/advs.202303516
Nagel, E., Vilser, W., Fink, A., Riemer, T., and Lanzl, I. (2006). Blood pressure effects on retinal vessel diameter and flicker response: a 1.5-year follow-up. Eur. J. Ophthalmol. 16, 560–565. doi: 10.1177/112067210601600410
Nagel, E., Vilser, W., and Lanzl, I. (2004). Age, blood pressure, and vessel diameter as factors influencing the arterial retinal flicker response. Invest. Ophthalmol. Vis. Sci. 45, 1486–1492. doi: 10.1167/iovs.03-0667
Newman, E. A. (2013). Functional hyperemia and mechanisms of neurovascular coupling in the retinal vasculature. J. Cereb. Blood Flow Metab. 33, 1685–1695. doi: 10.1038/jcbfm.2013.145
Paschoal, A. M., Leoni, R. F., Dos Santos, A. C., and Paiva, F. F. (2018). Intravoxel incoherent motion MRI in neurological and cerebrovascular diseases. Neuroimage Clin. 20, 705–714. doi: 10.1016/j.nicl.2018.08.030
Peregud-Pogorzelska, M., Zielska, M., Kawa, M. P., Babiak, K., Safranow, K., Machalinski, B., et al. (2020). Association between light-induced dynamic dilation of retinal vessels and echocardiographic parameters of the left ventricular function in hypertensive patients. Medicina (Kaunas) 56, 1–13. doi: 10.3390/medicina56120704
Pournaras, C. J., Rungger-Brandle, E., Riva, C. E., Hardarson, S. H., and Stefansson, E. (2008). Regulation of retinal blood flow in health and disease. Prog. Retin. Eye Res. 27, 284–330. doi: 10.1016/j.preteyeres.2008.02.002
Ptito, M., Bleau, M., and Bouskila, J. (2021). The retina: a window into the brain. Cells 10, 1–11. doi: 10.3390/cells10123269
Querques, G., Borrelli, E., Sacconi, R., De Vitis, L., Leocani, L., Santangelo, R., et al. (2019). Functional and morphological changes of the retinal vessels in Alzheimer’s disease and mild cognitive impairment. Sci. Rep. 9:63. doi: 10.1038/s41598-018-37271-6
Rabiolo, A., Corvi, F., Monteduro, D., Benatti, L., Cicinelli, M. V., Fogliato, G., et al. (2016). Impact of combined hormonal contraceptives on vessels functionality. Arch. Gynecol. Obstet. 294, 1317–1322. doi: 10.1007/s00404-016-4170-2
Rembold, K. E., Ayers, C. R., Wills, M. B., and Rembold, C. M. (2003). Usefulness of carotid intimal medial thickness and flow-mediated dilation in a preventive cardiovascular practice. Am. J. Cardiol. 91, 1475–1477. doi: 10.1016/s0002-9149(03)00403-x
Rensma, S. P., van Sloten, T. T., Houben, A., Kohler, S., van Boxtel, M. P. J., Berendschot, T., et al. (2020). Microvascular dysfunction is associated with worse cognitive performance: the Maastricht study. Hypertension 75, 237–245. doi: 10.1161/HYPERTENSIONAHA.119.13023
Rombouts, S. A., Goekoop, R., Stam, C. J., Barkhof, F., and Scheltens, P. (2005). Delayed rather than decreased BOLD response as a marker for early Alzheimer’s disease. NeuroImage 26, 1078–1085. doi: 10.1016/j.neuroimage.2005.03.022
Schiel, R., Vilser, W., Kovar, F., Kramer, G., Braun, A., and Stein, G. (2009). Retinal vessel response to flicker light in children and adolescents with type 1 diabetes mellitus and overweight or obesity. Diabetes Res. Clin. Pract. 83, 358–364. doi: 10.1016/j.diabres.2008.11.001
Selbach, J., Schallenberg, M., Kramer, S., Anastassiou, G., Steuhl, K. P., Vilser, W., et al. (2014). Trabeculectomy improves vessel response measured by dynamic vessel analysis (DVA) in Glaucoma patients. Open Ophthalmol. J. 8, 75–81. doi: 10.2174/1874364101408010075
Seshadri, S., Ekart, A., and Gherghel, D. (2016). Ageing effect on flicker-induced diameter changes in retinal microvessels of healthy individuals. Acta Ophthalmol. 94, e35–e42. doi: 10.1111/aos.12786
Sharifizad, M., Schmidl, D., Werkmeister, R. M., Zeisler, H., Told, R., Binder, J., et al. (2021). Retinal vessel diameters, flicker-induced retinal vasodilation and retinal oxygen saturation in high-and low-risk pregnancy. Acta Ophthalmol. 99, 628–636. doi: 10.1111/aos.14696
Song, R., Pan, K. Y., Xu, H., Qi, X., Buchman, A. S., Bennett, D. A., et al. (2021). Association of cardiovascular risk burden with risk of dementia and brain pathologies: a population-based cohort study. Alzheimers Dement. 17, 1914–1922. doi: 10.1002/alz.12343
Sorensen, B. M., Houben, A., Berendschot, T., Schouten, J., Kroon, A. A., van der Kallen, C. J. H., et al. (2017). Cardiovascular risk factors as determinants of retinal and skin microvascular function: the Maastricht study. PLoS One 12:e0187324. doi: 10.1371/journal.pone.0187324
Streese, L., Kotliar, K., Deiseroth, A., Infanger, D., Vilser, W., and Hanssen, H. (2019). Retinal endothelial function, physical fitness and cardiovascular risk: a diagnostic challenge. Front. Physiol. 10:831. doi: 10.3389/fphys.2019.00831
Streese, L., Lona, G., Wagner, J., Knaier, R., Burri, A., Neve, G., et al. (2021). Normative data and standard operating procedures for static and dynamic retinal vessel analysis as biomarker for cardiovascular risk. Sci. Rep. 11:14136. doi: 10.1038/s41598-021-93617-7
Streese, L., Vaes, A., Infanger, D., Roth, R., and Hanssen, H. (2021). Quantification of retinal vessel myogenic constriction in response to blood pressure peaks: implications for flicker light-induced dilatation. Front. Physiol. 12:608985. doi: 10.3389/fphys.2021.608985
Stringer, M. S., Lee, H., Huuskonen, M. T., MacIntosh, B. J., Brown, R., Montagne, A., et al. (2021). A review of translational magnetic resonance imaging in human and rodent experimental models of small vessel disease. Transl. Stroke Res. 12, 15–30. doi: 10.1007/s12975-020-00843-8
Szegedi, S., Dal-Bianco, P., Stogmann, E., Traub-Weidinger, T., Rainer, M., Masching, A., et al. (2020). Anatomical and functional changes in the retina in patients with Alzheimer’s disease and mild cognitive impairment. Acta Ophthalmol. 98, e914–e921. doi: 10.1111/aos.14419
Tapp, R. J., Owen, C. G., Barman, S. A., Welikala, R. A., Foster, P. J., Whincup, P. H., et al. (2019). Associations of retinal microvascular diameters and tortuosity with blood pressure and arterial stiffness: United Kingdom biobank. Hypertension 74, 1383–1390. doi: 10.1161/HYPERTENSIONAHA.119.13752
Tarantini, S., Tran, C. H. T., Gordon, G. R., Ungvari, Z., and Csiszar, A. (2017). Impaired neurovascular coupling in aging and Alzheimer’s disease: contribution of astrocyte dysfunction and endothelial impairment to cognitive decline. Exp. Gerontol. 94, 52–58. doi: 10.1016/j.exger.2016.11.004
Theuerle, J. D., Al-Fiadh, A. H., Amirul Islam, F. M., Patel, S. K., Burrell, L. M., Wong, T. Y., et al. (2021). Impaired retinal microvascular function predicts long-term adverse events in patients with cardiovascular disease. Cardiovasc. Res. 117, 1949–1957. doi: 10.1093/cvr/cvaa245
van Dinther, M., Voorter, P. H. M., Schram, M. T., Berendschot, T., Houben, A., Webers, C. A. B., et al. (2022). Retinal microvascular function is associated with the cerebral microcirculation as determined by intravoxel incoherent motion MRI. J. Neurol. Sci. 440:120359. doi: 10.1016/j.jns.2022.120359
van Veluw, S. J., Hou, S. S., Calvo-Rodriguez, M., Arbel-Ornath, M., Snyder, A. C., Frosch, M. P., et al. (2020). Vasomotion as a driving force for Paravascular clearance in the awake mouse brain. Neuron 105:e545, 549–561.e5. doi: 10.1016/j.neuron.2019.10.033
Vilser, W. (2019) in “methods of retinal vessel analysis,” in retinal vessel analysis - a new method of diagnostics and risk prediction. eds. H. Hanssen and W. Vilser (Bremen, Germany: UNI-MED), 17–29.
Vilser, W., Nagel, E., and Lanzl, I. (2002). Retinal vessel analysis--new possibilities. Biomed Tech (Berl) 47, 682–685. doi: 10.1515/bmte.2002.47.s1b.682
Waigi, E. W., Pernomian, L., Crockett, A. M., Costa, T. J., Townsend, P. Jr., Webb, R. C., et al. (2024). Vascular dysfunction occurs prior to the onset of amyloid pathology and Abeta plaque deposits colocalize with endothelial cells in the hippocampus of female APPswe/PSEN1dE9 mice. Geroscience 46, 5517–5536. doi: 10.1007/s11357-024-01213-0
Keywords: dementia, brain, dynamic vessel analysis, retinal vasoreactivity, aging
Citation: Peterfi A, Pinaffi-Langley ACdC, Szarvas Z, Muranyi M, Kaposzta Z, Adams C, Pinto CB, Mukli P, Kotliar K and Yabluchanskiy A (2025) Dynamic retinal vessel analysis: flickering a light into the brain. Front. Aging Neurosci. 16:1517368. doi: 10.3389/fnagi.2024.1517368
Edited by:
Enzo Emanuele, 2E Science, ItalyReviewed by:
Bhakta Prasad Gaire, Cedars Sinai Medical Center, United StatesHamidreza Ashayeri, Tabriz University of Medical Sciences, Iran
Copyright © 2025 Peterfi, Pinaffi-Langley, Szarvas, Muranyi, Kaposzta, Adams, Pinto, Mukli, Kotliar and Yabluchanskiy. This is an open-access article distributed under the terms of the Creative Commons Attribution License (CC BY). The use, distribution or reproduction in other forums is permitted, provided the original author(s) and the copyright owner(s) are credited and that the original publication in this journal is cited, in accordance with accepted academic practice. No use, distribution or reproduction is permitted which does not comply with these terms.
*Correspondence: Andriy Yabluchanskiy, YW5kcml5LXlhYmx1Y2hhbnNraXlAb3Voc2MuZWR1; Konstantin Kotliar, a290bGlhckBmaC1hYWNoZW4uZGU=