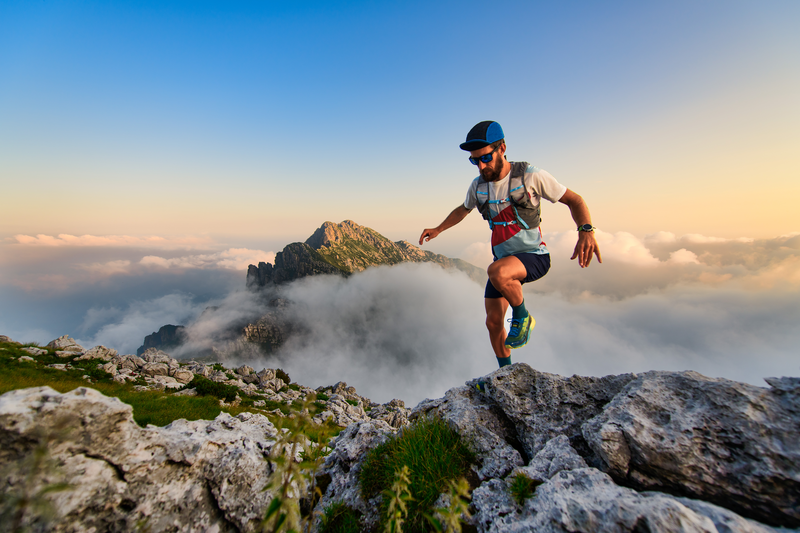
95% of researchers rate our articles as excellent or good
Learn more about the work of our research integrity team to safeguard the quality of each article we publish.
Find out more
ORIGINAL RESEARCH article
Front. Aging Neurosci. , 07 January 2025
Sec. Parkinson’s Disease and Aging-related Movement Disorders
Volume 16 - 2024 | https://doi.org/10.3389/fnagi.2024.1502025
This article is part of the Research Topic Cortico-Muscular Network Interactions View all 4 articles
Background: Previous studies have suggested that neuromuscular junction (NMJ) denervation plays a critical role in amyotrophic lateral sclerosis (ALS). Repetitive nerve stimulation (RNS) has been used as a technique to test neuromuscular transmission, but the sensitivity and stability of its parameters have not been investigated in patients with ALS. In addition, the impact of impaired homeostasis on NMJ stability in patients with ALS remains unclear.
Methods: A total of 421 patients with ALS were enrolled. Data on their clinical, biochemical and electrophysiological indicators were divided into a training set (collected from June 2019 to June 2022) and a test set (collected from July 2022 to June 2023). The coefficient of variation (CV) was used to assess the extent of variability. Stepwise regression was used in independent variable selection and model building.
Results: In patients with ALS, area decrement had a higher rate of abnormal result and a lower CV than amplitude decrement. No significant difference in the rate of abnormal decrement was found when the first compound muscle action potential (CMAP) was compared with either the fourth or fifth one. Moreover, multivariate regression analysis suggests high-density lipoprotein cholesterol (HDL-C) had the greatest impact on decremental response, followed by serum uric acid (UA) and forced vital capacity (FVC). Females had a larger range of area decrement than males.
Conclusion: During RNS test, assessing area decrement significantly enhances our ability to detect the impairment of neuromuscular transmission in patients with ALS. Independent factors contributing to decremental response need to be considered in drug development and clinical trials targeting NMJ in patients with ALS.
Amyotrophic lateral sclerosis (ALS) is the third most common adult-onset neurodegenerative disease. The incidence of ALS exponentially increases with age after 40, peaking in individuals aged 70–79 years (Ingre et al., 2015). The global number of ALS patients is projected to increase by 69% between 2015 to 2040 (Arthur et al., 2016).
Neuromuscular junction (NMJ) degeneration is an early event with complex and dynamic process of continuous denervation and new innervation in ALS (Martineau et al., 2018). Cellular and molecular alterations in presynaptic motor neuron, postsynaptic skeletal muscle, and terminal Schwann cells contribute to NMJ degeneration (Verma et al., 2022). In recent years, peripheral stabilization of NMJs and motor-units (MUs) has been proposed as a potential therapeutic target for patients with ALS (Alhindi et al., 2022; Verma et al., 2022; Martineau et al., 2018).
Repetitive nerve stimulation (RNS) is a widely utilized technique to test neuromuscular transmission. The sensitivity and stability of measurements during RNS test are important issues of concern in clinical practice. Over the past 30 years, most studies have evaluated the decremental response by measuring the reduction in peak-to-peak amplitude of the compound muscle action potential (CMAP) from the first to the fourth (Killian et al., 1994; Hatanaka et al., 2017; Fu et al., 2019) or fifth stimulus (Mori et al., 2016; Wang et al., 2017; Hu et al., 2018), while others have assessed it by analyzing changes in CMAP area (Wang et al., 2001; de Carvalho and Swash, 2019). However, the comparisons of these parameters have not been well investigated in patients with ALS.
Besides, accumulating evidence has suggested that homeostasis is impaired in patients with ALS, and several biochemical blood parameters that confirm the presence or progression of a change in body status, have been regarded as possible biomarkers for the prognosis of ALS (Kirk et al., 2019; Monov and Molodozhnikova, 2024; Aydemir et al., 2022; Cheng et al., 2021). However, the impacts of these changes on neuromuscular transmission have not been fully elucidated in patients with ALS.
Therefore, the aim of this study was to investigate the sensitivity and stability of parameters during the RNS test and to identify independent clinical and biochemical factors contributing to the NMJ stability.
This study enrolled patients diagnosed as ALS based on the Awaji criteria (de Carvalho et al., 2008), and who had undergone LF-RNS tests on the accessory nerve at our hospital between June 2019 to June 2023. Exclusion criteria were as follows: (1) patients with a history of poliomyelitis, (2) patients with a spinal cord tumor, (3) patients with other diseases affecting the peripheral nerves, neuromuscular junction (NMJ), or muscles, and (4) patients with incomplete clinical information. This study was approved by the ethics committee of our hospital and written informed consent was obtained from each participant.
Clinical information was collected at the time of diagnosis, including sex, age at onset, onset site, disease duration, diagnostic level, ALSFRS-R score (Cedarbaum et al., 1999), body mass index (BMI), forced vital capacity (FVC), and the results of RNS tests. The rate of disease progression (ΔFS) was calculated through the following formula: ΔFS = [48–ALSFRS-R score]/duration (month) (Labra et al., 2016).
Experienced neurologists followed up on the participants regularly every 6 months with a telephone call or a face-to-face interview until June 30, 2024. For a deceased patient, survival time was defined as the interval time between date of onset and date of death or tracheotomy, which was taken as the equivalent to death. For the surviving patients, survival time was calculated from date of onset to the date of the last follow-up visit (June 30, 2024). Patients who made no contact on two successive occasions were assigned to loss in the follow-up group.
The electrodiagnostic studies were performed on a Keypoint workstation (31A06, Alpine Biomed ApS, Copenhagen, Denmark). Skin temperature over the examined muscle was maintained at 32°C or above throughout the entire period of measurement. Spontaneous activity was considered as present when reproducible trains were observed for at least 300 ms following needle insertion at >2 sites within the same muscle. Ten MUPs were measured in each muscle.
LF-RNS was performed on the side with the more severe symptom involvement, and its results were recorded from the trapezius muscle with stimulation of the accessory nerve, abductor digiti minimi (ADM) muscle for the ulnar nerve, and tibialis anterior muscle (TAM) for the peroneal nerve. For patients asymptomatic on both upper limbs, the RNS test was performed on the right side. A train of 10 3 Hz stimulus was delivered to the nerves, which was repeated three times at an interval of at least 30 s between each stimulus train. The peak-to-peak amplitude decrement and the difference in negative peak area from the first CMAP to the fourth/fifth one were measured and expressed as percentages. A decrement of 10% or more was considered to be abnormal (Kimura, 1989).
Venous blood was collected from the cubital vein of the patient early in the morning after an overnight fast. Biochemical indicators were measured in the department of biochemistry in our hospital. The serum interleukin-6 (IL-6) level was measured using a chemiluminescence immunoassay and with the Immulite 2000 chemiluminescence immunoassay analyzer (Siemens), according to the instructions of the manufacturer. The functional sensitivity (FS) of IL-6 was 2 pg./mL. The detection values of IL-6 lower than FS were recorded as <2 pg./mL. Referring to the methods reported in the previous literature, values lower than FS were calculated as 0.5 × FS without affecting the statistical results. Serum triglyceride (TG), total cholesterol (TC), high-density lipoprotein cholesterol (HDL-C), low-density lipoprotein cholesterol (LDL-C), serum uric acid (UA), serum creatinine, and total serum bilirubin (TSB) were measured using an autoanalyzer (Cobas 8,000 modular analyzer series; Roche Diagnostics, Basel, Switzerland).
The pulmonary function test (PFT) was performed by certified technicians in the pulmonary function laboratory of our hospital. Pulmonary ventilation was measured with a spirometer (MasterScreen Body; Jaeger, Wurzburg, Germany) and the measurement results were analyzed with computer software (Master Lab Manager V5.31.0 software; Jaeger). Each test was repeated three times, and the best result was chosen as the final result. All spirometric values were expressed as a percentage of the predicted values.
MS-Excel 2016 (Microsoft, Redmond, Washington) and MATLAB R2019b (MathWorks, Natick, Massachusetts) were employed for statistical analysis. Measurements that were normally distributed were expressed as mean ± standard deviation (SD), and those non-normally distributed as quartile M (Q1, Q3) values. Medians were compared with the Mann–Whitney U test between two groups. Enumeration data were presented as the number of patients and percentages. The Chi-square test was used for comparison between different enumerative groups. Bonferroni correction was made to p values when several statistical tests were being performed simultaneously. The survival analysis was performed by Kaplan Meier method with a log-rank test. The significance level was set at p < 0.05.
The extent of variability both in amplitude decrement and area decrement between the first train and the second or third one was investigated by means of coefficient of variation (CV). As to the area decrement from the first CMAP to the fourth, the values of the differences between the first train and the second, as well as between the third one and the first, were used to derive the mean difference value. Standard deviation and CV were derived from the data set that included the mean difference values of all patients. The lower CV indicates greater stability.
Stepwise regression was used for model building. In model validation, the predicted number of patients with abnormal decrement was derived by calculation of the model based on data from patients with abnormal decrements. The predicted number of patients with normal decrements was derived by calculation based on data from patients with normal decrements. Consistency in the same results was calculated as (predicted number of patients with abnormal decrement + predicted number of patients with normal decrement)/(actual number of patients with abnormal decrement + actual number of patients with normal decrement) × 100%.
A total of 421 patients with ALS were enrolled in this study, all having undergone LF-RNS on the trapezius with stimulation on the accessory nerve. Details on the demographics of ALS patients are presented in Table 1. Of the 421 patients, 191 had also undergone LF-RNS on the ADM, and 54 on the TAM.
Two hundred and eight patients attended follow-up visits between June 2019 and June 2024. One hundred and five patients had died or undergone tracheostomy, and 103 were still alive at the last follow-up visit. A total of 225 patients have complete records of biochemical tests. For the multivariate model building and validation, their clinical data were divided into a training set and a test set (Figure 1).
Figure 1. Data splitting. The flow diagram indicates the numbers in the training set and the test set.
The rate of abnormal decrement in trapezius is higher than that in ADM and TAM (Table 2). No significant difference was found in the abnormal rate of either amplitude or area decrement when the first CMAP is compared with either the fourth or fifth one.
The abnormal rate in area decrement is higher than that in amplitude decrement, and 98.8% of the patients with an abnormal amplitude decrement in the accessory nerve also had abnormal decrement measured by the area. However, of the 287 patients with an abnormal area decrement between the first CMAP and the fourth at 3 Hz on the trapezius, 169 (58.9%) had an abnormal amplitude decrement. Of the 248 patients with a normal amplitude decrement, 118 (47.6%) had abnormal area decrement.
Overall, the CV of the area decrement is lower than that of the amplitude decrement (Table 3). Specifically, as far as the decrement response from the first CMAP to fourth CMAP on the trapezius during 3 Hz RNS is concerned, the mean value of CV for area decrement (0.995) is lower than that for amplitude decrement (1.073). The mean absolute differences between stimulus trains in amplitude decrement is significantly different from that in area decrement (Supplementary Table 4). Similar results can be found on ADM and TAM. As such, CMAP area decrement is more stable than amplitude decrement.
A total of 345 patients underwent 3 Hz RNS of the accessory nerve and needle EMG of the ipsilateral trapezius. A total of 48 patients underwent 3 Hz RNS of the peroneal nerve and needle EMG of the ipsilateral TAM. CMAP decrement is correlated with MUP duration and amplitude (Table 4). Patients with fibrillation potentials and/or positive sharp waves (fib-psw) in trapezius have a larger decrement range than those without fib-psw (Table 5).
Since no significant difference was found in the abnormal rate of amplitude or area decrement when the first CMAP is compared with either the fourth or fifth one and the number of patients having abnormal decrement on ADM and TAM was limited, we took the decrement from the first stimuli to the fourth on the trapezius as an example to further investigate the correlations between clinical indicators and the rates of abnormal decrement.
Seven clinical indicators (onset age, sex, onset site, diagnostic level, diagnostic delay, BMI, FVC), and nine biochemical indicators (HDL-C, LDL-C, LDL/HDL, IL-6, TC, TG, TSB, serum creatinine, and serum UA) were considered as components that might contribute to model building. The final results of the stepwise regression (Supplementary Table 1) showed that the following model provided the best fit for the data set:
Where Ys is the standardization of area decrement (Y), X1 is FVC, X2 is HDL-C, X3 is serum UA, D1 = 0 if female, D1 = 1 if male. (D2, D3) represents onset site: upper limb onset = (0, 0), lower limb onset = (1, 0), and bulbar onset = (0, 1). The F-value is 5.713 (p < 0.001, n = 172), Durbin Watson test (DW test) statistic value is 2.029 and the corresponding p-value is 0.5729. T-values for each parameter reach significant level (p < 0.05).
The total consistency between the actual value and the prediction value is 75.581%, where the discrepancy mainly occurs when the decrement is <10% (Table 6). Leave-one-out cross-validation and external validation (Supplementary Table 2) suggest a good and stable prediction performance of model (1).
FVC, HDL-C, serum UA, sex, and onset site are independent factors contributing to the area decrement (Figure 2). The model (1) suggests that with all other variables being held constant, a one unit increase in FVC (% predicted), HDL-C, or serum UA, were, respectively, associated with a 0.066, −4.4351, or 0.147% decreases in the range of area decrement (Supplementary Table 3). The expected range of area decrement in males was 2.9% lower than that in females. For patients with lower limb onset and bulbar onset, the expected ranges of area decrement decreased by 6.565 and 3.988%, respectively, in comparison with patients with upper limb onset. Therefore, HDL-C had the greatest impact on decremental response as measured by CMAP area, followed by serum UA and FVC; females had a larger range of area decrement than males; upper limb onset contributed more to the decremental response in accessory nerve than lower limb onset and bulbar onset.
Figure 2. Predictor impact on the rate of decrement change. The U/L represents the difference in the rate of decrement change between upper limb onset and lower limb onset when holding other predictors constant.
Notably, in our cohort, there are 118 patients having abnormal decrement in CMAP area but normal decrement in amplitude. To find out their clinical characteristics, we compared them with patients having abnormal decrement in both measurements (Table 7). The results suggested that the patients with abnormal decrement only in area have shorter disease duration, higher ALSFRS-R scores, and a larger percentage of non-definite ALS and upper limb onset. No statistical difference in sex, onset age, BMI, FVC (%), and disease progression rate was observed.
Table 7. Comparison of clinical indicators between patients with normal amplitude decrement but abnormal area decrement and those having abnormal decrement in both measurements.
Finally, we analyzed the correlation between decremental response and survival. The mean survival time was 56.2 months (median: 53; 95%CI: 51.6–60.8) in our cohort. The median and mean survival time in patients with abnormal area decrement during 3 Hz RNS on the trapezius or ADM is shorter than that in patients with normal area decrement, but it did not reach statistical significance (Figure 3).
Figure 3. The impact of abnormal decremental response during 3 Hz RNS on survival. (A) The survival curve for all ALS patients. (B) The survival curves for patients undergone 3 Hz RNS test on the accessory nerve. (C) The survival curves for patients undergone 3 Hz RNS test on the ulnar nerve.
Our study showed that in patients with ALS, area decrement had a higher rate of abnormal result and a lower CV than amplitude decrement. No significant difference in the rate of abnormal decrement was found when the first CMAP was compared with either the fourth or fifth one. HDL-C, FVC, serum UA, sex, and onset site were independent factors contributing to the area decrement. Patients with abnormal decrement only in area had shorter disease duration, higher ALSFRS-R scores, and higher percentage of non-definite ALS than those having abnormal decrement in both measurements.
So far, the precise mechanism leading to CMAP decrement in ALS remains unclear. Previous studies have suggested that deregulation of protein homeostasis, malfunctioning of axonal transportation, cholinergic dysfunction, and impaired mitochondrial dynamics resulting from motor neuron degeneration contribute to NMJ disruption in ALS (Verma et al., 2022). On the other hand, inherent pathological defects in skeletal muscle independently induce NMJ degeneration (Badu-Mensah et al., 2022). ALS muscle cells fail to form large clusters of acetylcholine receptors (Shefner et al., 2023), and they secret excessive Nogo-A (Jokic et al., 2005; Bruneteau et al., 2015). In addition, perisynaptic Schwann cells fail to adopt a phagocytic phenotype on denervated NMJ and to guide compensatory nerve terminal sprouts (Martineau et al., 2020). In this study, correlations of decremental response with abnormal spontaneous activity and MUP have been observed, suggesting that both denervation and re-innervation influence CMAP decrement in patients with ALS.
It is generally assumed that the greatest decrease of acetylcholine release at a slow rate of stimulation occurs during the first four stimuli, and by the fifth or sixth stimulation, the release begins to improve because the mobilization store has begun resupplying the immediately available store (Preston and Shapiro, 2020). The percentage of the decrement is measured between the baseline CMAP and lowest CMAP. Fu et al. (2019) observed the descent pattern of CMAP amplitude in patients with ALS and found that during 3 Hz RNS, the lowest CMAP in the accessory nerve occurred at the 4th stimulation, and that in ulnar nerve was at the 5th. Some researchers have calculated the decrement by comparing baseline CMAP with the CMAP amplitude from the fourth stimulus (Killian et al., 1994; Hatanaka et al., 2017; Fu et al., 2019), while others have chosen the fifth’s (Mori et al., 2016; Wang et al., 2017; Hu et al., 2018). Our study suggests that there is no statistical difference between the two calculation approaches, not only in the aspect of CMAP amplitude but also area.
CMAP area, the product of amplitude and duration, is known to be related most directly to the number of muscle fibers or motor units that contribute to the CMAP (Daube and Rubin, 2009; Asawa et al., 2004). In healthy volunteers, amplitude increases while duration decreases with successive low-frequency stimulation (Asawa et al., 2004; van Dijk et al., 2000). The impact of low-frequency stimulus on CMAP amplitude and duration may counteract each other in calculating CMAP area. In patients with ALS, cellular and molecular alterations in motor neurons, skeletal muscles, and terminal Schwann cells affect neuromuscular transmission (Verma et al., 2022), leading to fewer individual muscle fiber action potentials (MFAPs) contributing to the CMAP through four or five repetitions of low-frequency stimulus. Our study shows that, even when decremental response occurs, parameters of area decrement are more stable than amplitude decrement.
On the other hand, area decrement is more sensitive than amplitude decrement. In our cohort, 98.8% of the patients with abnormal amplitude decrement in the accessory nerve also had abnormal decrement measured by area, while 47.6% of patients with normal amplitude decrement had abnormal area decrement. Similar results were also found in patients with other neuromuscular disorders. Lo et al. (2003) compared area and amplitude of muscle responses to RNS in 87 patients with possible neuromuscular transmission defects and 30 controls. They found that decrement of response area provides additional diagnostic yields of up to 30%. Boonhong (2009) suggested that 23% of RNS reports were negative for amplitude decrement while positive for area decrement. Sixty-nine percent of those patients with inconsistent results were diagnosed with MG or suspected MG by the specialists. Therefore, area decrement greatly improves our ability to evaluate neuromuscular transmission. Moreover, patients with abnormal decrement only in area have a shorter disease duration, higher ALSFRS-R scores, and a larger percentage of non-definite ALS, thus indicating an earlier stage of ALS. This finding supports the suggestion by Ratnagopal (Ratnagopal, 1997) that area decrement may be a helpful indicator of neuromuscular defect at an earlier level.
In addition to the evaluation of the parameters during RNS test, we also investigated the relationship between decrement response and biochemical indicators in the present study. We found that decrement decreases with the increase of the natural antioxidant (serum uric acid), which is compatible with previous studies suggested that NMJ is very sensitive reacting to oxidative stress in ALS (Naumenko et al., 2011; Pollari et al., 2014). Besides, cholesterol is included in all key pre- and postsynaptic processes and plays a crucial role in safety factor at the NMJ (Krivoi and Petrov, 2019). Higher pre-diagnostic HDL-C levels have been found to be associated with a higher risk of ALS (Bjornevik et al., 2021). Statin, a widely used medication that reduces the level of LDL and raises the concentration of HDL (Al-Kuraishy et al., 2024; Barter et al., 2010), is associated with higher incidence (Wang et al., 2023; Skajaa et al., 2021), worse progression and shorter survival of ALS (Zinman et al., 2008; Weisskopf et al., 2022). Thus far, much less is known about how the lipid profile impacts the neuromuscular transmission in patients with ALS. The regression analysis in our study suggests that total cholesterol, LDL-C, and the LDL-C/HDL-C ratio are not independent factors contributing to the decremental response, while the range of decrement increases with HDL-C. The causal relationship between HDL-C elevation and NMJ dysfunction and whether it is an appropriate therapeutic target to improve neuromuscular transmission and produce effective relief for muscle weakness, still need further investigation.
There are some limitations to this study. First, we did not analyze the correlation of survival with decremental response on TAM because of the low abnormal rate and limited number of patients. Second, the number of patients who undergwent EMG test on the ADM is limited in our cohort. The relationship between the decrement and the results of needle EMG tests on the ADM needs further investigation. Third, in addition to the nine biochemical parameters discussed in current study, the relationships between decremental response and other aberrant biochemical parameters need further investigation. Forth, our patients are from different parts of China and are representative only of the ALS cohort in China. The findings of this study cannot be generalized and multicenter retrospective studies are needed in the future to determine whether there are any differences in the stability and sensitivity of measurements during LF-RNS between Chinese cohorts and patients from other countries or regions.
In conclusion, CMAP area decrement is more stable and sensitive than amplitude decrement in patients with ALS. Independent clinical and biochemical factors contributing to the decremental response should be considered in drug development and clinical trials targeting the NMJ in these patients.
The data analyzed in this study is subject to the following licenses/restrictions: The data that support the findings of this study are available from the corresponding author upon reasonable request. Requests to access these datasets should be directed to Qiang Shi, c2hpcTMwMUAxNjMuY29t.
The studies involving humans were approved by the Ethics Committee of Chinese PLA General Hospital. The studies were conducted in accordance with the local legislation and institutional requirements. The participants provided their written informed consent to participate in this study.
JZ: Conceptualization, Data curation, Formal analysis, Investigation, Methodology, Resources, Software, Validation, Visualization, Writing – original draft, Writing – review & editing. YL: Conceptualization, Investigation, Resources, Writing – review & editing. QS: Conceptualization, Data curation, Formal analysis, Investigation, Methodology, Project administration, Resources, Supervision, Validation, Writing – original draft, Writing – review & editing.
The author(s) declare that no financial support was received for the research, authorship, and/or publication of this article.
We sincerely thank Boudewijn T. H. M. Sleutjes at the University Medical Center Utrecht for invaluable and insightful comments. We are grateful to Steven L. Stephenson at the University of Arkansas for his carefully language editing.
The authors declare that the research was conducted in the absence of any commercial or financial relationships that could be construed as a potential conflict of interest.
The authors declare that no Gen AI was used in the creation of this manuscript.
All claims expressed in this article are solely those of the authors and do not necessarily represent those of their affiliated organizations, or those of the publisher, the editors and the reviewers. Any product that may be evaluated in this article, or claim that may be made by its manufacturer, is not guaranteed or endorsed by the publisher.
The Supplementary material for this article can be found online at: https://www.frontiersin.org/articles/10.3389/fnagi.2024.1502025/full#supplementary-material
CMAP, compound muscle action potential; LF-RNS, low-frequency repetitive nerve stimulation; ALS, amyotrophic lateral sclerosis; CV, coefficient of variation; FVC, forced vital capacity; UA, uric acid; NMJ, neuromuscular junction; ACH, acetylcholine; SFEMG, single fiber electromyography; BMI, body mass index; ADM, abductor digiti minimi; TAM, tibialis anterior muscle; IL-6, interleukin-6; TG, serum triglyceride; TC, total cholesterol; HDL-C, high-density lipoprotein cholesterol; LDL-C, low-density lipoprotein cholesterol; TSB, total serum bilirubin; PFT, pulmonary function test; SD, standard deviation; DW test, Durbin Watson test.
Alhindi, A., Boehm, I., and Chaytow, H. (2022). Small junction, big problems: neuromuscular junction pathology in mouse models of amyotrophic lateral sclerosis (ALS). J. Anat. 241, 1089–1107. doi: 10.1111/joa.13463
Al-kuraishy, H., Jabir, M. S., Sulaiman, G. M., Mohammed, H., al-Gareeb, A., Albuhadily, A., et al. (2024). The role of statins in amyotrophic lateral sclerosis: protective or not? Front. Neurosci. 18:1422912. doi: 10.3389/fnins.2024.1422912
Arthur, K. C., Calvo, A., Price, T. R., Geiger, J. T., Chiò, A., Traynor, B. J., et al. (2016). Projected increase in amyotrophic lateral sclerosis from 2015 to 2040. Nat. Commun. 7:12408. doi: 10.1038/ncomms12408
Asawa, T., Shindo, M., and Momoi, H. (2004). Compound muscle action potentials during repetitive nerve stimulation. Muscle Nerve 29, 724–728. doi: 10.1002/mus.20037
Aydemir, D., Surucu, S., Basak, A. N., and Ulusu, N. N. (2022). Evaluation of the hematological and serum biochemistry parameters in the pre-symptomatic and symptomatic stages of ALS disease to support early diagnosis and prognosis. Cells 11:3569. doi: 10.3390/cells11223569
Badu-Mensah, A., Guo, X., Nimbalkar, S., Cai, Y., and Hickman, J. J. (2022). ALS mutations in both human skeletal muscle and motoneurons differentially affects neuromuscular junction integrity and function. Biomaterials 289:121752. doi: 10.1016/j.biomaterials.2022.121752
Barter, P. J., Brandrup-Wognsen, G., Palmer, M. K., and Nicholls, S. J. (2010). Effect of statins on HDL-C: a complex process unrelated to changes in LDL-C: analysis of the VOYAGER database. J. Lipid Res. 51, 1546–1553. doi: 10.1194/jlr.P002816
Bjornevik, K., O'Reilly, É. J., Cortese, M., Furtado, J. D., Kolonel, L. N., Le Marchand, L., et al. (2021). Pre-diagnostic plasma lipid levels and the risk of amyotrophic lateral sclerosis. Amyotroph. Lat. Scler. Frontotemporal. Degener. 22, 133–143. doi: 10.1080/21678421.2020.1822411
Boonhong, J. (2009). Comparison of amplitude and area decrement in repetitive nerve stimulation. J. Med. Assoc. Thail. 92, 96–100.19260249
Bruneteau, G., Bauché, S., Gonzalez de Aguilar, J. L., Brochier, G., Mandjee, N., Tanguy, M. L., et al. (2015). Endplate denervation correlates with Nogo-a muscle expression in amyotrophic lateral sclerosis patients. Ann. Clin. Transl. Neurol. 2, 362–372. doi: 10.1002/acn3.179
Cedarbaum, J. M., Stambler, N., Malta, E., Fuller, C., Hilt, D., Thurmond, B., et al. (1999). The ALSFRS-R: a revised ALS functional rating scale that incorporates assessments of respiratory function. BDNF ALS study group (phase III). J. Neurol. Sci. 169, 13–21. doi: 10.1016/S0022-510X(99)00210-5
Cheng, Y., Chen, Y., and Shang, H. (2021). Aberrations of biochemical indicators in amyotrophic lateral sclerosis: a systematic review and meta-analysis. Transl. Neurodegener. 10:3. doi: 10.1186/s40035-020-00228-9
Daube, J. R., and Rubin, D. I. (2009). Clinical neurophysiology. New York, NY: Oxford University Press.
de Carvalho, M., Dengler, R., Eisen, A., England, J. D., Kaji, R., Kimura, J., et al. (2008). Electrodiagnostic criteria for diagnosis of ALS. Clin. Neurophysiol. 119, 497–503. doi: 10.1016/j.clinph.2007.09.143
de Carvalho, M., and Swash, M. (2019). The split hand in amyotrophic lateral sclerosis: a possible role for the neuromuscular junction. Amyotroph. Lateral. Scler. Frontotemporal. Degener. 20, 368–375. doi: 10.1080/21678421.2019.1606245
Fu, L. L., Yin, H. X., Liu, M. S., and Cui, L. Y. (2019). Study on variation trend of repetitive nerve stimulation waveform in amyotrophic lateral sclerosis. Chin. Med. J. 132, 542–550. doi: 10.1097/CM9.0000000000000117
Hatanaka, Y., Higashihara, M., Chiba, T., Miyaji, Y., Kawamura, Y., and Sonoo, M. (2017). Utility of repetitive nerve stimulation test for ALS diagnosis. Clin. Neurophysiol. 128, 823–829. doi: 10.1016/j.clinph.2017.02.021
Hu, F., Jin, J., Kang, L., Jia, R., Qin, X., Liu, X., et al. (2018). Decremental responses to repetitive nerve stimulation in amyotrophic lateral sclerosis. Eur. Neurol. 80, 151–156. doi: 10.1159/000494670
Ingre, C., Roos, P. M., Piehl, F., Kamel, F., and Fang, F. (2015). Risk factors for amyotrophic lateral sclerosis. Clin. Epidemiol. 7, 181–193. doi: 10.2147/CLEP.S37505
Jokic, N., Gonzalez de Aguilar, J. L., Pradat, P. F., Dupuis, L., Echaniz-Laguna, A., Muller, A., et al. (2005). Nogo expression in muscle correlates with amyotrophic lateral sclerosis severity. Ann. Neurol. 57, 553–556. doi: 10.1002/ana.20420
Killian, J. M., Wilfong, A. A., Burnett, L., Appel, S. H., and Boland, D. (1994). Decremental motor responses to repetitive nerve stimulation in ALS. Muscle Nerve 17, 747–754. doi: 10.1002/mus.880170708
Kimura, J. (1989). “Techniques of repetitive stimulation” in Electrodiagnosis in diseases of nerve and muscle: Principal and practice. ed. J. Kimura. 2nd ed (Philadelphia, PA: FA Davis Company).
Kirk, S. E., Tracey, T. J., Steyn, F. J., and Ngo, S. T. (2019). Biomarkers of metabolism in amyotrophic lateral sclerosis. Front. Neurol. 10:191. doi: 10.3389/fneur.2019.00191
Krivoi, I. I., and Petrov, A. M. (2019). Cholesterol and the safety factor for neuromuscular transmission. Int. J. Mol. Sci. 20:1046. doi: 10.3390/ijms20051046
Labra, J., Menon, P., Byth, K., Morrison, S., and Vucic, S. (2016). Rate of disease progression: a prognostic biomarker in ALS. J. Neurol. Neurosurg. Psychiatry 87, 628–632. doi: 10.1136/jnnp-2015-310998
Lo, Y. L., Dan, Y. F., Leoh, T. H., Tan, Y. E., and Ratnagopal, P. (2003). Decrement in area of muscle responses to repetitive nerve stimulation. Muscle Nerve 27, 494–496. doi: 10.1002/mus.10339
Martineau, É., Arbour, D., Vallée, J., and Robitaille, R. (2020). Properties of glial cell at the neuromuscular junction are incompatible with synaptic repair in the SOD1G37R ALS mouse model. J. Neurosci. 40, 7759–7777. doi: 10.1523/JNEUROSCI.1748-18.2020
Martineau, É., Di Polo, A., Vande Velde, C., and Robitaille, R. (2018). Dynamic neuromuscular remodeling precedes motor-unit loss in a mouse model of ALS. eLife 7:e41973. doi: 10.7554/eLife.41973
Monov, D., and Molodozhnikova, N. (2024). Biochemical parameters as a tool to assess the nutritional status of patients with amyotrophic lateral sclerosis. Front. Neurol. 14:1258224. doi: 10.3389/fneur.2023.1258224
Mori, A., Yamashita, S., Nakajima, M., Hori, H., Tawara, A., Matsuo, Y., et al. (2016). CMAP decrement as a potential diagnostic marker for ALS. Acta Neurol. Scand. 134, 49–53. doi: 10.1111/ane.12510
Naumenko, N., Pollari, E., Kurronen, A., Giniatullina, R., Shakirzyanova, A., Magga, J., et al. (2011). Gender-specific mechanism of synaptic impairment and its prevention by GCSF in a mouse model of ALS. Front. Cell. Neurosci. 5:26. doi: 10.3389/fncel.2011.00026
Pollari, E., Goldsteins, G., Bart, G., Koistinaho, J., and Giniatullin, R. (2014). The role of oxidative stress in degeneration of the neuromuscular junction in amyotrophic lateral sclerosis. Front. Cell. Neurosci. 8:131. doi: 10.3389/fncel.2014.00131
Preston, D. C., and Shapiro, B. E. (2020). Electromyography and neuromuscular disorders: Clinical-Electrophysiologic-ultrasound correlations. Amsterdam: Elsevier.
Ratnagopal, P. (1997). Repetitive nerve stimulation - amplitude vs area decrement [abstract]. Electroencephalogr. Clin. Neurophysiol. 103:126. doi: 10.1016/S0013-4694(97)88559-1
Shefner, J. M., Musaro, A., Ngo, S. T., Lunetta, C., Steyn, F. J., Robitaille, R., et al. (2023). Skeletal muscle in amyotrophic lateral sclerosis. Brain 146, 4425–4436. doi: 10.1093/brain/awad202
Skajaa, N., Bakos, I., Horváth-Puhó, E., Henderson, V. W., Lash, T. L., and Sørensen, H. T. (2021). Statin initiation and risk of amyotrophic lateral sclerosis: a Danish population-based cohort study. Epidemiology 32, 756–762. doi: 10.1097/EDE.0000000000001384
van Dijk, J. G., van der Hoeven, B. J., and van der Hoeven, H. (2000). Repetitive nerve stimulation: effects of recording site and the nature of 'pseudofacilitation'. Clin. Neurophysiol. 111, 1411–1419. doi: 10.1016/S1388-2457(00)00331-X
Verma, S., Khurana, S., Vats, A., Sahu, B., Ganguly, N. K., Chakraborti, P., et al. (2022). Neuromuscular junction dysfunction in amyotrophic lateral sclerosis. Mol. Neurobiol. 59, 1502–1527. doi: 10.1007/s12035-021-02658-6
Wang, F. C., De Pasqua, V., Gérard, P., and Delwaide, P. J. (2001). Prognostic value of decremental responses to repetitive nerve stimulation in ALS patients. Neurology 57, 897–899. doi: 10.1212/WNL.57.5.897
Wang, Y., Xiao, Z., Chu, H., Liang, J., Wu, X., Dong, H., et al. (2017). Correlations between slow-rate repetitive nerve stimulation and characteristics associated with amyotrophic lateral sclerosis in Chinese patients. J. Phys. Ther. Sci. 29, 737–743. doi: 10.1589/jpts.29.737
Wang, W., Zhang, L., Xia, K., Huang, T., and Fan, D. (2023). Mendelian randomization analysis reveals statins potentially increase amyotrophic lateral sclerosis risk independent of peripheral cholesterol-lowering effects. Biomedicines. 11:1359. doi: 10.3390/biomedicines11051359
Weisskopf, M. G., Levy, J., Dickerson, A. S., Paganoni, S., and Leventer-Roberts, M. (2022). Statin medications and amyotrophic lateral sclerosis incidence and mortality. Am. J. Epidemiol. 191, 1248–1257. doi: 10.1093/aje/kwac054
Keywords: amyotrophic lateral sclerosis, repetitive nerve stimulation, decremental response, electromyography, modeling
Citation: Zhang J, Li Y and Shi Q (2025) Decremental response in patients with amyotrophic lateral sclerosis during repetitive nerve stimulation and its relationships with impaired homeostasis. Front. Aging Neurosci. 16:1502025. doi: 10.3389/fnagi.2024.1502025
Received: 26 September 2024; Accepted: 17 December 2024;
Published: 07 January 2025.
Edited by:
Franca Tecchio, Consiglio Nazionale delle Ricerche CNR, ItalyReviewed by:
José Castro, Universidade de Lisboa, PortugalCopyright © 2025 Zhang, Li and Shi. This is an open-access article distributed under the terms of the Creative Commons Attribution License (CC BY). The use, distribution or reproduction in other forums is permitted, provided the original author(s) and the copyright owner(s) are credited and that the original publication in this journal is cited, in accordance with accepted academic practice. No use, distribution or reproduction is permitted which does not comply with these terms.
*Correspondence: Qiang Shi, c2hpcTMwMUAxNjMuY29t
†ORCID: Qiang Shi, orcid.org/0000-0002-4694-4272
Disclaimer: All claims expressed in this article are solely those of the authors and do not necessarily represent those of their affiliated organizations, or those of the publisher, the editors and the reviewers. Any product that may be evaluated in this article or claim that may be made by its manufacturer is not guaranteed or endorsed by the publisher.
Research integrity at Frontiers
Learn more about the work of our research integrity team to safeguard the quality of each article we publish.