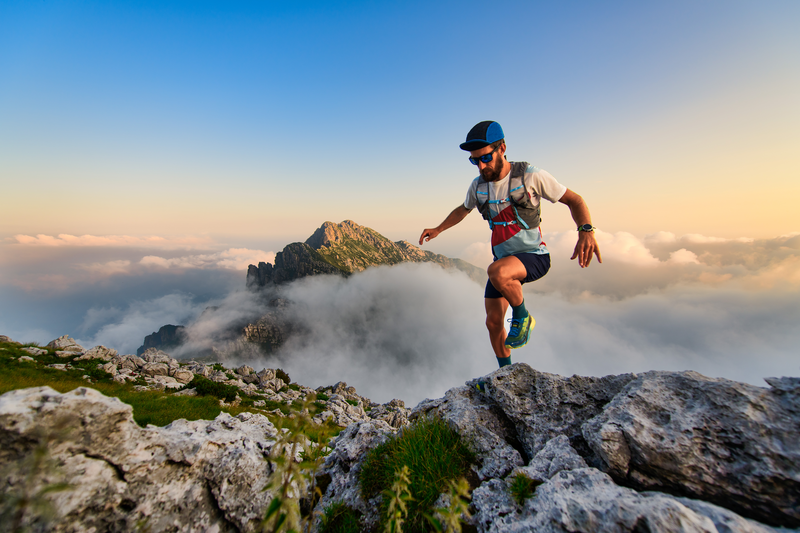
95% of researchers rate our articles as excellent or good
Learn more about the work of our research integrity team to safeguard the quality of each article we publish.
Find out more
ORIGINAL RESEARCH article
Front. Aging Neurosci. , 14 May 2024
Sec. Parkinson’s Disease and Aging-related Movement Disorders
Volume 16 - 2024 | https://doi.org/10.3389/fnagi.2024.1377719
This article is part of the Research Topic Common pathophysiology underpinning Parkinson's disease and other neurodegenerative diseases View all 14 articles
Background: The association between hypothyroidism and Parkinson’s disease (PD) has sparked intense debate in the medical community due to conflicting study results. A better understanding of this association is crucial because of its potential implications for both pathogenesis and treatment strategies.
Methods: To elucidate this complex relationship, we used Bayesian co-localisation (COLOC) and bidirectional Mendelian randomization (MR) analysis. COLOC was first used to determine whether hypothyroidism and PD share a common genetic basis. Subsequently, genetic variants served as instrumental variables in a bidirectional MR to explore causal interactions between these conditions.
Results: COLOC analysis revealed no shared genetic variants between hypothyroidism and PD, with a posteriori probability of hypothesis 4 (PPH4) = 0.025. Furthermore, MR analysis indicated that hypothyroidism does not have a substantial causal effect on PD (OR = 0.990, 95% CI = 0.925, 1.060, p = 0.774). Conversely, PD appears to have a negative causal effect on hypothyroidism (OR = 0.776, 95% CI = 0.649, 0.928, p = 0.005).
Conclusion: Our findings suggest the absence of shared genetic variants between hypothyroidism and PD. Interestingly, PD may inversely influence the risk of developing hypothyroidism, a finding that may inform future research and clinical approaches.
Parkinson’s disease (PD) is the second most common neurodegenerative disease after Alzheimer’s disease, and its incidence continues to increase (Pavlou and Outeiro, 2017). The dyskinesia symptoms of PD–bradykinesia, tremor, rigidity and postural balance deficits–are the result of dopaminergic neuronal deficits and the accumulation of α-synuclein (Jakubowski and Labrie, 2017; Mohd Murshid et al., 2022). Dysregulation of dopamine metabolism, oxidative stress and neuroinflammation are key factors contributing to dopaminergic neuronal deficits (Dexter and Jenner, 2013; Labbé et al., 2016; Chang and Chen, 2020). Hypothyroidism, characterised by low thyroid hormone levels, is associated with oxidative stress and inflammatory responses (Mancini et al., 2016; de Carvalho et al., 2018). There is conflicting evidence about the relationship between hypothyroidism and the risk of PD. For example, a Danish study involving more than 80,000 people found no significant association between hypothyroidism and PD, whereas studies in Taiwan and South Korea have shown that patients with hypothyroidism have an increased risk of PD (Rugbjerg et al., 2009; Chen et al., 2020; Kim et al., 2021). Hypothyroidism is known to increase the risk of anaemia, obesity and hyperlipidaemia, all of which are associated with an increased risk of developing PD (Mohammadi et al., 2021). Given the mixed results of observational studies and potential confounding factors, it remains difficult to establish a causal relationship between hypothyroidism and PD.
Mendelian randomization (MR), which uses single-nucleotide polymorphisms (SNPs) as instrumental variables to estimate the causal effect of exposure on outcomes, can help researchers identify causal relationships between diseases and avoid the confounding problems often found in traditional studies (Liu et al., 2021). MR has proven to be a powerful and convenient tool for investigating risk factors associated with PD (Senkevich et al., 2023; Song et al., 2024). Bayesian co-localisation (COLOC) is a method that uses Bayesian statistical principles to assess whether two or more traits share the same genetic variation (Chen et al., 2024). This is important for understanding how different traits are linked by common biological pathways or mechanisms. Some studies have used MR in conjunction with COLOC, an approach that has revealed a common genetic basis between psoriasis and multiple sclerosis and helped to identify potential therapeutic targets for multiple sclerosis (Lin et al., 2023; Patrick et al., 2023).
Traditional observational studies often encounter difficulties in establishing a causal relationship between PD and hypothyroidism, the main obstacle being the exclusion of confounding factors. To overcome this limitation, MR offers a powerful alternative approach that is particularly effective in overcoming the limitations of traditional studies in making causal inferences. Our study uses a genome-wide association study (GWAS) dataset containing two diseases, which provides a good basis for using both COLOC and MR analysis methods. The combination of these two approaches could provide a solid basis for genetic commonality and causal inference to reveal genetic and causal links between hypothyroidism and PD, and provide new biological insights into these two potentially related disorders, thus opening up new avenues for clinical practice and future research directions.
We obtained data from published GWAS,1 focusing on datasets that provide comprehensive insights into PD and hypothyroidism (Table 1). As this study used publicly available data, ethical approval was not required as each dataset had already received ethical approval.
The COLOC analysis was based on the following assumptions (Wallace, 2020). Independence assumption: associations between traits are caused directly by a common genetic variant and not by other indirect factors such as confounding variables. COLOC uses a Bayesian probability model to assess the strength of the evidence that two traits share the same SNPs. COLOC can derive five types of probability, including cases where the two traits are independent and have no common variant, each has its own variant, and one variant is shared. We used the hypothetical posterior probability 4 (PPH4) in the COLOC algorithm to assess whether the two share genetic variants, with PPH4 > 80% defined as the two sharing the same genetic variant as an influence (Deng et al., 2022). This analysis was performed using the “coloc” software package.2
Our MR study design was based on three critical assumptions: (A) SNPs are significantly associated with exposure, serving as an instrumental variable; (B) these SNPs are not associated with known confounders; and (C) the effect of SNPs on outcome is entirely mediated by exposure factors.
SNPs were used as instrumental variables according to the following four criteria: a. p < 5 × 108; b. removal of chain imbalances (kb = 10,000 and r2 = 0.001) (Hu et al., 2023); c. search for these SNPs in PhenoScanner3 and removal of SNPs associated with confounders and outcomes (Hu et al., 2022); d. calculation and selection of SNPs with F-statistics >10, which can mitigate the effects of potential bias (Hu et al., 2023).
We chose inverse variance weighted (IVW), weighted median, MR-Egger and weighted mode for MR analyses, and considered p < 0.05 to be statistically significant (Han et al., 2022). Heterogeneity was assessed using MR-Egger and IVW regressions, with p > 0.05 indicating no heterogeneity. The presence of horizontal pleiotropy was assessed using the Egger intercept, with p > 0.05 indicating the absence of horizontal pleiotropy (Hu et al., 2022, 2023). Visualization results are presented as rejection-by-exclusion tests, forest plots, scatter plots and funnel plots. All statistical analyses were performed using the “TwoSampleMR” package in R (version 4.2.3).
In our paired GWAS analysis of hypothyroidism and PD, the COLOC PPH4 was below 80% (PPH4 = 0.024), suggesting that there is insufficient evidence to support that they share a common genetic locus (Figure 1). This finding suggests that there may not be a direct genetic link between hypothyroidism and PD, and that they may affect patients through different biological pathways.
Figure 1. Co-localization between hypothyroidism and PD. PPH4 stands for posterior probability of hypothesis 4. PPH4 > 80% defined as both sharing the same genetic variant as an influencing factor.
When selecting SNPs as instrumental variables from the GWAS dataset, we used methodological mention criteria to ensure that these SNPs were strongly associated with the phenotype, as detailed in Supplementary Table S1. This ensured the precision and reliability of our analysis.
Using a MR analysis with validated instrumental variables, the MR-IVW results showed no causal effect of hypothyroidism on PD (OR = 0.990, 95% CI = 0.925, 1.060, p = 0.774). The MR Egger (OR = 1. 006, 95% CI = 0.824, 1.227, p = 0.953), weighted median (OR = 0.968, 95% CI = 0.899, 1.042, p = 0.389) and weighted mode (OR = 1.034, 95% CI = 0.902, 1.185, p = 0.641) analyses were consistent with the MR-IVW analysis (Table 2). Although our analysis did not show a causal effect of hypothyroidism on PD. This result helps to rule out hypothyroidism as a potential risk factor or protective factor for PD. The Cochran’s Q statistic in the MR-Egger and IVW regressions showed heterogeneity in the Cochran’s Q test (p < 0.05) (Supplementary Table S2). Our horizontal multivariate test showed no horizontal multivariate p > 0.05 in the instrumental variables (Supplementary Table S3). Sensitivity analyses using the leave-one-out method confirmed the stability of our results (Supplementary Figure S1A). Forest, scatter and funnel plots supported the results of our MR analyses (Supplementary Figures S2A–C).
The same MR analysis method was used to infer the causal effect of PD on hypothyroidism. Interestingly, although the MR Egger (OR = 0.886, 95% CI = 0.618, 1.215, p = 0.418), weighted median (OR = 0.829 95% CI = 0.649, 1.058, p = 0.130) and weighted mode (OR = 0.682 95% CI = 0.635, 1.343, p = 0.682) analyses did not agree with the results of the MR-IVW analyses, the MR-IVW results suggested that PD reduced the risk of developing hypothyroidism (OR = 0.776 95% CI = 0.649, 0.928, p = 0.005). The Cochran’s Q statistic for the MR-Egger and IVW regressions suggested that the Cochran’s Q test was not heterogeneous (p > 0.05) (Supplementary Table S2). Our multilevel pleiotropy test indicated no level pleiotropy (p > 0.05) for the instrumental variables used (Supplementary Table S3). This suggests that the instrumental variables were causally related to PD via hypothyroidism, without confounding by other factors. The robustness of our findings was further confirmed in the leave-one-out sensitivity analysis (Supplementary Figure S2B). In addition, forest plots, scatter plots and funnel plots also confirmed the consistency of our MR analysis results (Supplementary Figures S3A–C).
Our study shows that there is no common genetic variant between hypothyroidism and PD. Hypothyroidism does not have a causal effect on PD, but PD has a negative causal effect on hypothyroidism. This finding is inconsistent with previous studies showing that hypothyroidism increases the risk of developing PD (Charoenngam et al., 2022). A concurrent and prospective study using traditional statistics and novel machine learning models found that hypothyroidism is associated with an increased risk of PD (Gialluisi et al., 2023). In vitro and in vivo experiments have shown that thyroid hormones can prevent apoptosis of dopamine neurons (Kincaid, 2001; Lee et al., 2019; Menezes et al., 2019), suggesting a protective role of thyroid hormones on dopamine neurons in PD. However, hypothyroidism, characterised by insufficient thyroid hormone production, may exacerbate or promote the progression of PD. Recent bidirectional MR studies do not support a causal relationship between hypothyroidism and PD (Zeng et al., 2024). Our results are reliable and we used the most recent Finnish database with the largest sample size available for the study. First, in the co-localisation analysis of the two diseases did not find any common genetic variants were found, suggesting that variants in one disease do not increase the risk of the other. Second, despite the heterogeneity in the MR analysis of hypothyroidism on PD, the MR analysis overcame the confounding factors in traditional studies and ensured the reliability of causal inferences.
PD and hypothyroidism share similar pathogenic mechanisms, such as oxidative stress and inflammation. Studies have shown that apo D expression is upregulated in both diseases, and its antioxidant and anti-inflammatory effects provide an interesting avenue for further research (Fyfe-Desmarais et al., 2023). The mechanisms by which PD reduces the risk of hypothyroidism are unknown. We speculate that antioxidant mechanisms upregulated in PD may have a protective effect against the oxidative damage commonly seen in hypothyroidism. Future studies could investigate specific antioxidant levels and markers of oxidative stress in patients with PD compared to those with hypothyroidism. Altered immune-inflammatory pathways in PD may also play a protective role in the development of hypothyroidism. This hypothesis could be explored by examining cytokine levels and inflammatory markers in patients with PD versus those diagnosed with hypothyroidism to see if the reduced inflammatory response in PD is associated with a lower incidence of hypothyroidism. In addition, all current clinical pharmacological treatments can only alleviate the symptoms of PD, rather than slowing and curing the disease process. Investigating the role of oxidative stress and immune-inflammatory responses may provide new targets for PD treatment.
The motor dysfunctions associated with hypothyroidism may resemble those of PD, and given its treatability, accurate clinical diagnosis is important (Schneider et al., 2023). The high prevalence of PD and hypothyroidism in the elderly and of subclinical hypothyroidism in PD patients suggests a complex interaction between the two diseases (Munhoz et al., 2004). There is an association between thyroid hormones and PD, and most of the 12 SNPs may influence PD and thyroid function through immune mechanisms (Xu et al., 2022). The cardiovascular effects of hypothyroidism in PD and the improvement of fluctuating symptoms with thyroxine therapy in some patients further emphasise the need to assess thyroid function in the management of PD (García-Moreno and Chacón-Peña, 2003; Rodrigues et al., 2020). Although we did not find a common genetic variant between PD and hypothyroidism, the interaction between hypothyroidism and PD warrants further investigation.
Nevertheless, our study has limitations, including the heterogeneity of the instrumental variables in our MR analyses, which could be due to unobserved confounders. Also, as our GWAS data are predominantly from European populations, the applicability of our findings to other ethnic groups, such as Asians, remains uncertain. In addition, gender differences in the prevalence of hypothyroidism and PD (Chaker et al., 2017; Cerri et al., 2019), which were not accounted for in our analysis, may influence our results and should be a focus of future research.
In conclusion, our findings suggest that there is no shared genetic variation between hypothyroidism and PD, and interestingly, PD may have an inverse effect on the risk of developing hypothyroidism, a finding that may inform future research and clinical approaches.
Publicly available datasets were analyzed in this study. This data can be found at: https://www.finngen.fi/en/access_results.
JLe: Writing – original draft. WH: Writing – original draft. YaL: Formal analysis, Writing – original draft. QZ: Investigation, Writing – review & editing. YiL: Formal analysis, Writing – original draft. QO: Investigation, Writing – original draft. XW: Formal analysis, Writing – review & editing. FL: Formal analysis, Writing – original draft. JLi: Investigation, Writing – review & editing. YX: Writing – review & editing.
The author(s) declare financial support was received for the research, authorship, and/or publication of this article. This research received funding from the National Natural Science Foundation of China, under Grant/Award Numbers 81801268 and 82060248. Additionally, it was supported by the Clinical Research “Climbing” Program of the First Affiliated Hospital of Guangxi Medical University, Grant/Award Number YYZS2020017. This study was also funded by First-class discipline innovation-driven talentprogram of Guangxi Medical University.
The authors declare that the research was conducted in the absence of any commercial or financial relationships that could be construed as a potential conflict of interest.
All claims expressed in this article are solely those of the authors and do not necessarily represent those of their affiliated organizations, or those of the publisher, the editors and the reviewers. Any product that may be evaluated in this article, or claim that may be made by its manufacturer, is not guaranteed or endorsed by the publisher.
The Supplementary material for this article can be found online at: https://www.frontiersin.org/articles/10.3389/fnagi.2024.1377719/full#supplementary-material
Cerri, S., Mus, L., and Blandini, F. (2019). Parkinson’s disease in women and men: What’s the difference? J. Parkinsons Dis. 9, 501–515. doi: 10.3233/JPD-191683
Chaker, L., Bianco, A. C., Jonklaas, J., and Peeters, R. P. (2017). Hypothyroidism. Lancet 390, 1550–1562. doi: 10.1016/S0140-6736(17)30703-1
Chang, K. H., and Chen, C. M. (2020). The role of oxidative stress in Parkinson’s disease. Antioxidants (Basel) 9:597. doi: 10.3390/antiox9070597
Charoenngam, N., Rittiphairoj, T., Ponvilawan, B., and Prasongdee, K. (2022). Thyroid dysfunction and risk of Parkinson’s disease: a systematic review and Meta-analysis. Front. Endocrinol. (Lausanne) 13:863281. doi: 10.3389/fendo.2022.863281
Chen, Y., Fan, C., and Liu, J. (2024). Investigating the shared genetic architecture between COVID-19 and obesity: a large-scale genome wide cross-trait analysis. Front. Endocrinol. (Lausanne) 15:1325939. doi: 10.3389/fendo.2024.1325939
Chen, S. F., Yang, Y. C., Hsu, C. Y., and Shen, Y. C. (2020). Risk of Parkinson’s disease in patients with hypothyroidism: a nationwide population-based cohort study. Parkinsonism Relat. Disord. 74, 28–32. doi: 10.1016/j.parkreldis.2020.04.001
de Carvalho, G. P.-F. G. A., Junior, C. M., and Graf, H. (2018). Management of endocrine disease: pitfalls on the replacement therapy for primary and central hypothyroidism in adults. Eur. J. Endocrinol. 178, R231–R244. doi: 10.1530/EJE-17-0947
Deng, Y.-T., Ou, Y.-N., Wu, B.-S., Yang, Y.-X., Jiang, Y., Huang, Y.-Y., et al. (2022). Identifying causal genes for depression via integration of the proteome and transcriptome from brain and blood. Mol. Psychiatry 27, 2849–2857. doi: 10.1038/s41380-022-01507-9
Dexter, D. T., and Jenner, P. (2013). Parkinson disease: from pathology to molecular disease mechanisms. Free Radic. Biol. Med. 62, 132–144. doi: 10.1016/j.freeradbiomed.2013.01.018
Fyfe-Desmarais, G., Desmarais, F., Rassart, É., and Mounier, C. (2023). Apolipoprotein D in oxidative stress and inflammation. Antioxidants 12:1027. doi: 10.3390/antiox12051027
García-Moreno, J. M., and Chacón-Peña, J. (2003). Hypothyroidism and Parkinson’s disease and the issue of diagnostic confusion. Mov. Disord. 18, 1058–1059. doi: 10.1002/mds.10475
Gialluisi, A., De Bartolo, M. I., Costanzo, S., Belvisi, D., Falciglia, S., Ricci, M., et al. (2023). Risk and protective factors in Parkinson’s disease: a simultaneous and prospective study with classical statistical and novel machine learning models. J. Neurol. 270, 4487–4497. doi: 10.1007/s00415-023-11803-1
Han, F., Zhang, C., Xuan, M., Xie, Z., Zhang, K., and Li, Y. (2022). Effects of hyperthyroidism on venous thromboembolism: a Mendelian randomization study. J Immunol Res 2022:2339678. doi: 10.1155/2022/2339678
Hu, H., Mei, J., Cai, Y., Ding, H., Niu, S., Zhang, W., et al. (2023). No genetic causal association between Alzheimer’s disease and osteoporosis: a bidirectional two-sample Mendelian randomization study. Front. Aging Neurosci. 15:1090223. doi: 10.3389/fnagi.2023.1090223
Hu, M. J., Tan, J. S., Gao, X. J., Yang, J. G., and Yang, Y. J. (2022). Effect of cheese intake on cardiovascular diseases and cardiovascular biomarkers. Nutrients 14:2936. doi: 10.3390/nu14142936
Jakubowski, J. L., and Labrie, V. (2017). Epigenetic biomarkers for Parkinson’s disease: from diagnostics to therapeutics. J. Parkinsons Dis. 7, 1–12. doi: 10.3233/JPD-160914
Kim, J. H., Lee, H. S., Ahn, J. H., Oh, J. K., Chang, I. B., Song, J. H., et al. (2021). Association between thyroid diseases and Parkinson’s disease: a nested case-control study using a National Health Screening Cohort. J. Parkinsons Dis. 11, 211–220. doi: 10.3233/JPD-202265
Kincaid, A. E. (2001). Spontaneous circling behavior and dopamine neuron loss in a genetically hypothyroid mouse. Neuroscience 105, 891–898. doi: 10.1016/S0306-4522(01)00229-9
Labbé, C., Lorenzo-Betancor, O., and Ross, O. A. (2016). Epigenetic regulation in Parkinson’s disease. Acta Neuropathol. 132, 515–530. doi: 10.1007/s00401-016-1590-9
Lee, E. H., Kim, S. M., Kim, C. H., Pagire, S. H., Pagire, H. S., Chung, H. Y., et al. (2019). Dopamine neuron induction and the neuroprotective effects of thyroid hormone derivatives. Sci. Rep. 9:13659. doi: 10.1038/s41598-019-49876-6
Lin, J., Zhou, J., and Xu, Y. (2023). Potential drug targets for multiple sclerosis identified through Mendelian randomization analysis. Brain 146, 3364–3372. doi: 10.1093/brain/awad070
Liu, H., Zhang, Y., Zhang, H., Wang, L., Wang, T., Han, Z., et al. (2021). Effect of plasma vitamin C levels on Parkinson’s disease and age at onset: a Mendelian randomization study. J. Transl. Med. 19:221. doi: 10.1186/s12967-021-02892-5
Mancini, A., Di Segni, C., Raimondo, S., Olivieri, G., Silvestrini, A., Meucci, E., et al. (2016). Thyroid hormones, oxidative stress, and inflammation. Mediat. Inflamm. 2016:6757154. doi: 10.1155/2016/6757154
Menezes, E. C., Santos, P. R., Goes, T. C., Carvalho, V. C. B., Teixeira-Silva, F., Stevens, H. E., et al. (2019). Effects of a rat model of gestational hypothyroidism on forebrain dopaminergic, GABAergic, and serotonergic systems and related behaviors. Behav. Brain Res. 366, 77–87. doi: 10.1016/j.bbr.2019.03.027
Mohammadi, S., Dolatshahi, M., and Rahmani, F. (2021). Shedding light on thyroid hormone disorders and Parkinson disease pathology: mechanisms and risk factors. J. Endocrinol. Investig. 44, 1–13. doi: 10.1007/s40618-020-01314-5
Mohd Murshid, N., Aminullah Lubis, F., and Makpol, S. (2022). Epigenetic changes and its intervention in age-related neurodegenerative diseases. Cell. Mol. Neurobiol. 42, 577–595. doi: 10.1007/s10571-020-00979-z
Munhoz, R. P., Teive, H. A., Troiano, A. R., Hauck, P. R., Herdoiza Leiva, M. H., Graff, H., et al. (2004). Parkinson’s disease and thyroid dysfunction. Parkinsonism Relat. Disord. 10, 381–383. doi: 10.1016/j.parkreldis.2004.03.008
Patrick, M. T., Nair, R. P., He, K., Stuart, P. E., Billi, A. C., Zhou, X., et al. (2023). Shared genetic risk factors for multiple sclerosis/psoriasis suggest involvement of Interleukin-17 and Janus kinase-signal transducers and activators of transcription signaling. Ann. Neurol. 94, 384–397. doi: 10.1002/ana.26672
Pavlou, M. A. S., and Outeiro, T. F. (2017). Epigenetics in Parkinson’s disease. Adv. Exp. Med. Biol. 978, 363–390. doi: 10.1007/978-3-319-53889-1_19
Rodrigues, L. D., Oliveira, L. F., Scorza, C. A., Scorza, F. A., Andersen, M. L., Tufik, S., et al. (2020). Sudden unexpected death in Parkinson’s disease: who would think of the thyroid gland? Parkinsonism Relat. Disord. 81, 54–55. doi: 10.1016/j.parkreldis.2020.10.010
Rugbjerg, K., Friis, S., Ritz, B., Schernhammer, E. S., Korbo, L., and Olsen, J. H. (2009). Autoimmune disease and risk for Parkinson disease: a population-based case-control study. Neurology 73, 1462–1468. doi: 10.1212/WNL.0b013.e3181c06635
Schneider, S. A., Tschaidse, L., and Reisch, N. (2023). Thyroid disorders and movement disorders-a systematic review. Mov. Disord. Clin. Pract. 10, 360–368. doi: 10.1002/mdc3.13656
Senkevich, K., Alipour, P., Chernyavskaya, E., Yu, E., Noyce, A. J., and Gan-Or, Z. (2023). Potential protective link between type I diabetes and Parkinson’s disease risk and progression. Mov. Disord. 38, 1350–1355. doi: 10.1002/mds.29424
Song, J., Qin, Y., Wang, L., Quan, W., Xu, J., Li, J., et al. (2024). Exploring the causal relationship between B lymphocytes and Parkinson’s disease: a bidirectional, two-sample Mendelian randomization study. Sci. Rep. 14:2783. doi: 10.1038/s41598-024-53287-7
Wallace, C. (2020). Eliciting priors and relaxing the single causal variant assumption in colocalisation analyses. PLoS Genet. 16:e1008720. doi: 10.1371/journal.pgen.1008720
Xu, J., Zhao, C., Liu, Y., Xu, C., Qin, B., and Liang, H. (2022). Genetic correlation between thyroid hormones and Parkinson’s disease. Clin. Exp. Immunol. 208, 372–379. doi: 10.1093/cei/uxac044
Keywords: Parkinson’s disease, Mendelian randomization, single-nucleotide polymorphism, hypothyroidism, co-localization
Citation: Lei J, He W, Liu Y, Zhang Q, Liu Y, Ou Q, Wu X, Li F, Liao J and Xiao Y (2024) The potential protective role of Parkinson’s disease against hypothyroidism: co-localisation and bidirectional Mendelian randomization study. Front. Aging Neurosci. 16:1377719. doi: 10.3389/fnagi.2024.1377719
Received: 28 January 2024; Accepted: 29 April 2024;
Published: 14 May 2024.
Edited by:
Sheila Pirooznia, National Institutes of Health (NIH), United StatesReviewed by:
Guohao Wang, National Institutes of Health (NIH), United StatesCopyright © 2024 Lei, He, Liu, Zhang, Liu, Ou, Wu, Li, Liao and Xiao. This is an open-access article distributed under the terms of the Creative Commons Attribution License (CC BY). The use, distribution or reproduction in other forums is permitted, provided the original author(s) and the copyright owner(s) are credited and that the original publication in this journal is cited, in accordance with accepted academic practice. No use, distribution or reproduction is permitted which does not comply with these terms.
*Correspondence: Jiajia Liao, TGppYWppYTIxN0AxNjMuY29t; Yousheng Xiao, eHlzMTM1QDEyNi5jb20=
†These authors share first authorship
Disclaimer: All claims expressed in this article are solely those of the authors and do not necessarily represent those of their affiliated organizations, or those of the publisher, the editors and the reviewers. Any product that may be evaluated in this article or claim that may be made by its manufacturer is not guaranteed or endorsed by the publisher.
Research integrity at Frontiers
Learn more about the work of our research integrity team to safeguard the quality of each article we publish.