- Department of Neurology, Beaumont Research Institute, Corewell Health, Royal Oak, MI, United States
Clearance of amyloid-beta (Aβ) from the brain is impaired in both early-onset and late-onset Alzheimer’s disease (AD). Mechanisms for clearing cerebral Aβ include proteolytic degradation, antibody-mediated clearance, blood brain barrier and blood cerebrospinal fluid barrier efflux, glymphatic drainage, and perivascular drainage. ATP-binding cassette (ABC) transporters are membrane efflux pumps driven by ATP hydrolysis. Their functions include maintenance of brain homeostasis by removing toxic peptides and compounds, and transport of bioactive molecules including cholesterol. Some ABC transporters contribute to lowering of cerebral Aβ. Mechanisms suggested for ABC transporter-mediated lowering of brain Aβ, in addition to exporting of Aβ across the blood brain and blood cerebrospinal fluid barriers, include apolipoprotein E lipidation, microglial activation, decreased amyloidogenic processing of amyloid precursor protein, and restricting the entrance of Aβ into the brain. The ABC transporter superfamily in humans includes 49 proteins, eight of which have been suggested to reduce cerebral Aβ levels. This review discusses experimental approaches for increasing the expression of these ABC transporters, clinical applications of these approaches, changes in the expression and/or activity of these transporters in AD and transgenic mouse models of AD, and findings in the few clinical trials which have examined the effects of these approaches in patients with AD or mild cognitive impairment. The possibility that therapeutic upregulation of ABC transporters which promote clearance of cerebral Aβ may slow the clinical progression of AD merits further consideration.
1 Introduction
In 2023 approximately 6.7 million Americans age 65 and older were living with Alzheimer’s disease (AD)–related dementia (Alzheimer’s Association, 2023). Worldwide, AD has been suggested to account for 60–70% of the approximately 55 million cases of dementia (Mayo Clinic, 2023a). AD’s hallmark pathological findings are amyloid beta protein (Aβ)–containing senile plaques (SPs) and tau protein–containing neurofibrillary tangles. The etiology of familial (early onset) AD (AD-related dementia developing before age 65) is thought to be due to mutations in the amyloid precursor protein (APP), Presenilin 1 (PSEN1), and Presenilin 2 (PSEN2) genes (Petit et al., 2022). The etiology of late onset AD (LOAD; AD-related dementia developing at age 65 or later) remains unclear, with multiple factors including gene mutations, environmental toxins, and infectious agents suggested as possible contributing factors to its development or progression (Balin and Hudson, 2014; Chen et al., 2021; Bellenguez et al., 2022). Since publication of the amyloid hypothesis (Hardy and Allsop, 1991; Hardy and Higgins, 1992), which suggested that AD neuropathology was initiated by deposition of insoluble Aβ as SPs, therapeutic approaches have focused primarily on lowering of cerebral Aβ. These approaches have included Aβ vaccination (Gilman et al., 2005), Aβ aggregation inhibitors (Aisen et al., 2011), β-secretase inhibitors (Moussa-Pacha et al., 2020), γ-secretase modulators and inhibitors (Green et al., 2009; Imbimbo et al., 2011), and anti-Aβ monoclonal antibodies (Doody et al., 2014; Panza et al., 2014; Salloway et al., 2014). These approaches failed to slow the clinical progression of AD in large-scale clinical trials until recent trials with two monoclonal anti-Aβ antibodies, Lecanemab and Donanemab. Lecanemab slowed the progression of early stage AD by 27% and Donanemab slowed it by 32% (Sims et al., 2023; van Dyck et al., 2023). Whether these effects are clinically meaningful has been questioned (Alzforum, 2021; Prillaman, 2022).
The findings in the recent clinical trials with Lecanemab and Donanemab support the amyloid hypothesis. However, despite marked lowering of brain levels of PET-detectable Aβ by both antibodies, AD’s clinical progression continues, albeit more slowly than in placebo-treated patients. This suggests that more aggressive targeting of Aβ, including its soluble conformations, might further slow AD clinical progression. Alternatively, targeting of other pathology-related mechanisms suggested by the amyloid hypothesis to occur downstream of Aβ aggregation such as tau phosphorylation and aggregation, oxidative stress, and/or inflammation may be required to achieve this goal.
The literature includes descriptions of many experimental approaches for reducing brain levels of Aβ. Experimental approaches for increasing proteolytic degradation and antibody-mediated clearance of Aβ were recently reviewed by this author (Loeffler, 2023a,b). Additional mechanisms through which Aβ is cleared from the brain include its efflux across the blood brain barrier (BBB) (Qosa et al., 2014; Versele et al., 2022) and blood cerebrospinal fluid barrier (BCSFB) (Crossgrove et al., 2005; Shen et al., 2020), glymphatic drainage (Iliff et al., 2012; Li et al., 2022), and perivascular drainage (Bell et al., 2007; Zhang et al., 2021). ATP-binding cassette transporters, commonly known as ABC transporters, are membrane efflux pumps driven by hydrolysis of ATP (ElAli and Hermann, 2011; ElAli and Rivest, 2013; Erdő and Krajcsi, 2019). Some ABC transporters are present on the BBB and BCSFB (Morris et al., 2017). The present review discusses ABC transporters which have been suggested to promote lowering of cerebral Aβ, changes in their expression and/or activity in AD brain, experimental approaches which have been used to increase their expression, and findings in clinical trials which have explored the effects of these approaches in patients with AD or mild cognitive impairment (MCI).
The main functions of ABC transporters are maintenance of normal brain homeostasis (Jha et al., 2019) and transport of bioactive molecules (Leslie et al., 2005; ElAli and Rivest, 2013; Moore et al., 2023). The ABC transporter superfamily in humans is comprised of 49 proteins, divided into seven subfamilies (Pahnke et al., 2014). These subfamilies have different kinetics for exporting their substrates (Krohn et al., 2011). Much of the early research on ABC transporters focused on their roles in tumor drug resistance (Biedler and Riehm, 1970; Chen et al., 1986; Tan et al., 2000; Szakács et al., 2006). Among the ABC transporters which contribute to clearance of Aβ from the brain, ABCB1 and ABCA1 have been most extensively studied (ElAli and Rivest, 2013). Both are highly expressed on brain capillary endothelial cells. ABCB1 is present on the luminal side of the BBB (Roberts et al., 2008; Hermann and ElAli, 2012; Osgood et al., 2017) and on the apical side of the choroid plexus epithelial cells of the BCSFB (Morris et al., 2017), while ABCA1 is on the abluminal side of the BBB (Panzenboeck et al., 2002). Other ABC transporters suggested to influence cerebral Aβ levels (reviewed by Pahnke et al., 2014) include ABCA7 (Kim et al., 2013; Aikawa et al., 2018), ABCC1 (Krohn et al., 2011; Hofrichter et al., 2013), ABCC5 (Shubbar and Penny, 2020), ABCG1 (Zelcer et al., 2007), ABCG2 (Xiong et al., 2009; Do et al., 2012), and ABCG4 (Do et al., 2012). Reports were found for the expression of each of these transporters on the BBB (ABCB1: Roberts et al., 2008; ABCA1: Panzenboeck et al., 2002; ABCA7: Dib et al., 2021; ABCC1: Bernstein et al., 2014; ABCC5: Jansen et al., 2015; ABCG1: Kober et al., 2017; ABCG2: Schulz et al., 2023; ABCG4: Dodacki et al., 2017) and on the BCSFB (or choroid plexus) (ABCB1: Møllgård et al., 2017; ABCA1: Liddelow et al., 2012; ABCA7: Tijms et al., 2024; ABCC1: Møllgård et al., 2017; ABCC5: Strazielle and Ghersi-Egea, 2015; ABCG1: Liddelow et al., 2012; ABCG2: Møllgård et al., 2017; ABCG4: Fujiyoshi et al., 2007; Liddelow et al., 2012; Matsumoto et al., 2015). Figure 1 shows the subfamily distribution and main efflux functions of ABC transporters suggested in the literature to participate in Aβ clearance, and Table 1 shows the mechanisms of regulation of these transporters and their alterations in expression in AD brain, if known.
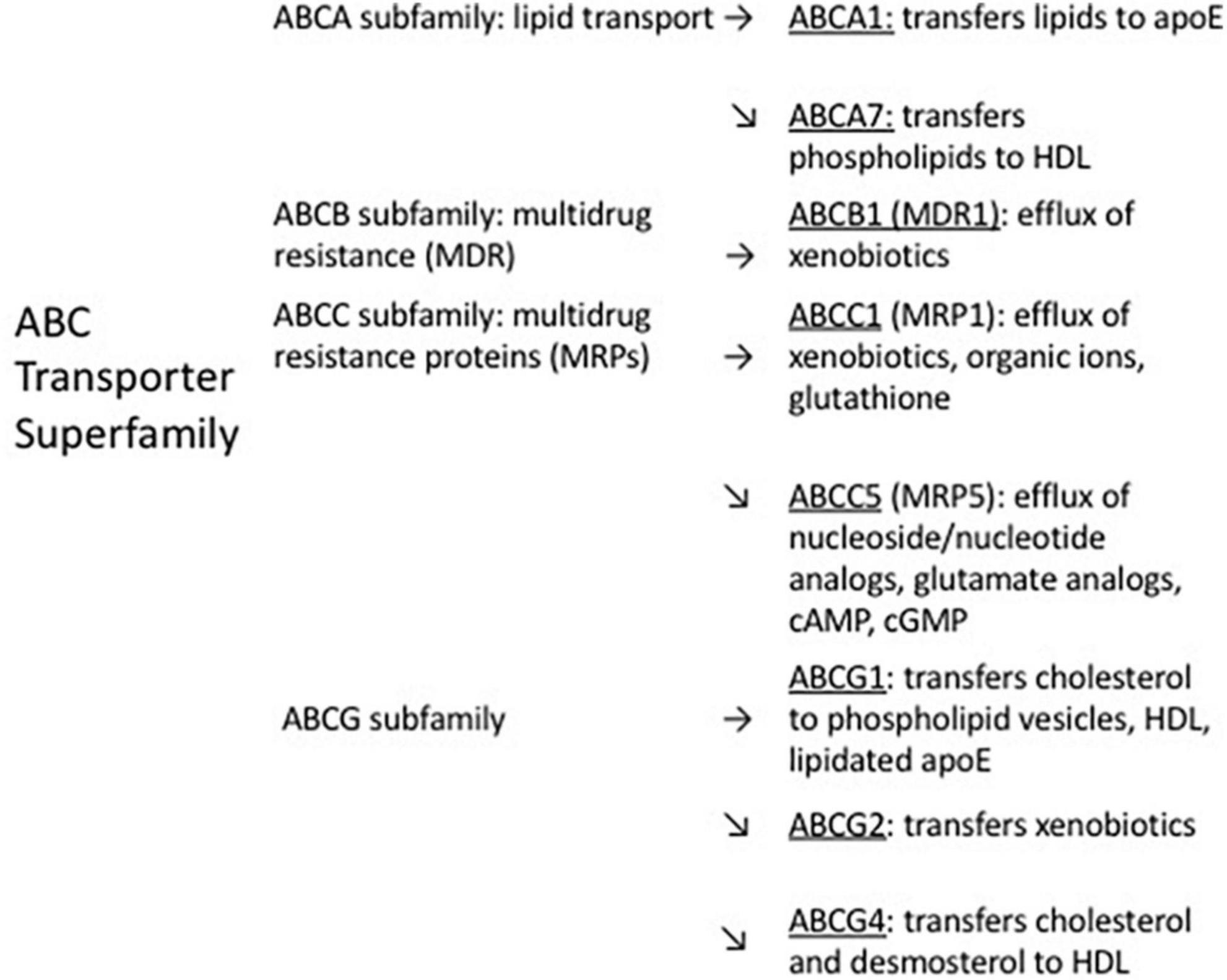
Figure 1. Distribution within ABC transporter subfamilies, and main efflux functions, of ABC transporters suggested to contribute to efflux of cerebral Aβ.
2 Materials and methods
Mechanisms of Aβ clearance from the brain were initially examined by a PubMed search of “Abeta clearance.” Some of the references that were found discussed the effects of various ABC transporters on Aβ clearance and the mechanisms, if known, by which the transporters promote this process. Further PubMed searches were performed using, as search terms, the ABC transporters suggested to be involved in ABC clearance, plus “Aβ clearance” and “experimental approaches.” The initial and subsequent literature searches identified published experimental approaches which have been used to increase the expression of these transporters. Clinical applications of the experimental approaches (including clinical trials and reports or reviews of their clinical use) were found through these PubMed searches and by searching ClinicalTrials.gov for the relevant ABC transporters and for the experimental approaches found to increase the expression of these transporters.
3 ABC transporters involved in clearance of cerebral Aβ
3.1 ABCA1
ABCA1 is expressed in the CNS by glia, neurons, and endothelial cells. It controls efflux of cholesterol, phospholipids, and other lipids from cells to systemic and brain apolipoprotein E (apoE) (Hirsch-Reinshagen et al., 2004; Do et al., 2011; ElAli and Rivest, 2013; Lewandowski et al., 2022). Its expression in mouse lateral ventricular choroid plexus was reported by Liddelow et al. (2012). Of relevance to AD, poor lipidation of apoE4 increases its instability, possibly leading to reduced clearance of Aβ, particularly neurotoxic Aβ oligomers (Tai et al., 2014; Lewandowski et al., 2022). The possibility that ABCA1 deficiency may promote increased aggregation of Aβ was suggested by a study which found that in the APP transgenic mouse model of AD, mice with only one copy of the ABCA1 gene had increased brain levels of oligomeric Aβ (Lefterov et al., 2009). Transcription of the ABCA1 gene is controlled by liver X receptors (LXRs) (Repa et al., 2000), which are present on glial cells and neurons (Jasmin et al., 2014). LXRs respond to elevated intracellular cholesterol by increasing the expression of genes which promote efflux of cholesterol and other lipids from cells (Valledor et al., 2004; Kalaany and Mangelsdorf, 2006; Wente et al., 2007). LXRs form complexes with retinoid X receptors (RXRs) (Heyman et al., 1992; de Urquiza et al., 2000) and these complexes bind to LXR response elements (Song et al., 1994; Willy et al., 1995). Experimental approaches which have been used to increase ABCA1 expression are shown in Table 2.
Two of these approaches, the use of LXR agonists and the retinoid Bexarotene, merit further mention. LXR agonists may cause significant deleterious side effects (Xu et al., 2013) including increased levels of plasma triglycerides (Koldamova et al., 2010). Bexarotene, an RXR agonist (Gaunt et al., 2021), is an FDA-approved drug that stimulates both LXR and peroxisome proliferator-activated receptor gamma (PPAR-γ) pathways. Its administration to transgenic mouse models of AD produced conflicting results regarding its ability to reduce Aβ plaque numbers and slow cognitive decline (Cramer et al., 2012; Tai et al., 2014; Balducci et al., 2015; Skerrett et al., 2015; Muñoz-Cabrera et al., 2019; Ren et al., 2019). The differing results in these studies may have been due to differences in the rate of development or extent of Aβ pathology between the mouse models, or differing Bexarotene doses or timing of its administration. Bexarotene and its derivatives may lower Aβ concentrations in transgenic mouse models of AD via ABCA1- and ABCG1-mediated increase in apoE lipidation, although increased microglial phagocytosis and enzymatic degradation of Aβ has also been detected (Yuan et al., 2019).
Interestingly, the expression of ABCA1 in human neuroglioma cells was decreased by exposure to the pesticide dichlorodiphenyltrichloroethane (DDT) (Li G. et al., 2015), supporting the possibility of an association between AD and DDT exposure which was suggested in an earlier study (Richardson et al., 2014).
3.2 ABCA7
ABCA7 shares 54% sequence homology with ABCA1 (Kaminski et al., 2000). However, ABCA7 expression is regulated by a different mechanism than ABCA1 (Abe-Dohmae and Yokoyama, 2021), namely the sterol-responsive/regulatory element binding protein (SREBP) pathway, which upregulates expression of genes involved in cholesterol synthesis in response to cholesterol deficits (Iwamoto et al., 2006; Dib et al., 2021). Like ABCA1, the main function of ABCA7 may be to regulate lipid metabolism, although the main lipids exported by the two transporters differ (Tomioka et al., 2017; Aikawa et al., 2018): ABCA7 transfers phospholipids to high density lipoprotein (HDL) whereas ABCA1 transfers cholesterol (Dib et al., 2021). ABCA7 is expressed in the CNS by neurons, microglia, astrocytes, and endothelial cells (Kim et al., 2006; Zhang et al., 2014; Fu et al., 2016) and Tijms et al. (2024), in a cerebrospinal fluid (CSF) proteomics study, mentioned its expression in choroid plexus; Liddelow et al. (2012) also reported its detection on mouse choroid plexus. It mediates macrophage and microglial phagocytosis (Fu et al., 2016). ABCA7 gene variants are risk factors for LOAD (Naj et al., 2011; Reitz et al., 2013; Steinberg et al., 2015; Efthymiou and Goate, 2017). In transgenic mouse models of AD, ABCA7 knockout increases SP counts. There are conflicting reports regarding the mechanism responsible for this increase. Knockout of ABCA7 in APP/PS1 mice resulted in increased production of Aβ due to elevated activity of β-site APP cleaving enzyme 1 (Sakae et al., 2016), similar to findings in an in vitro study (Satoh et al., 2015). However, knockout of ABCA7 in J20 mice, which express both the Swedish (K670N/M671L) and the Indiana (V717F) APP mutations, produced no changes in APP processing; increased SP density in J20 mice after ABCA7 knockout was suggested to be due to impaired phagocytic clearance of Aβ (Kim et al., 2013; Li H. et al., 2015). In an in vitro model of the BBB, reduction of ABCA7 expression induced by small interfering RNA decreased basolateral-to-apical transport of Aβ in mouse endothelial cells. ABCA1 expression was also decreased, and this may have contributed to the decrease in Aβ transport (Lamartinière et al., 2018). ABCA7 expression has been reported to be increased in AD brain, possibly as a compensatory mechanism for increased Aβ burden (Vasquez et al., 2013).
Experimental approaches to increase ABCA7 expression appear to have been limited to gene transfection (Chan et al., 2008) and statin-induced blocking of cholesterol synthesis (Tanaka et al., 2010).
3.3 ABCB1
ABCB1, also known as P-glycoprotein (P-gp) and Multi Drug Resistance Protein (Mdr-1), functions together with low density lipoprotein receptor-related protein 1 (LRP1) to transport Aβ from the brain via the BBB (Hartz et al., 2010; ElAli and Rivest, 2013). It is encoded by the ABCB1 gene in humans and by the Abcb1a and Abcb1b genes in rodents (Wang et al., 2016). ABCB1 has a wide range of substrates (Zhou, 2008) and is an important “gatekeeper” of the brain and other organs because of its role in blocking the entrance of many xenobiotics (foreign substances), including some chemotherapeutic agents, into these organs (Wang et al., 2016). It may also restrict entrance of Aβ into the brain (Candela et al., 2010). ABCB1 expression in the brain has been reported in endothelial cells, pericytes, microglia, and astrocytes (Sita et al., 2017), and it was reported on choroid plexus by Møllgård et al., 2017. The expression of ABCB1 on human brain endothelium has been reported to decrease, and to be inversely associated with cerebral Aβ levels, in the BBB during normal aging (Vogelgesang et al., 2002; Toornvliet et al., 2006; Bartels et al., 2009) and in AD (Wijesuriya et al., 2010; Deo et al., 2014; Chiu et al., 2015). Similar findings for ABCB1 expression and/or activity have been reported in animal models (Rosati et al., 2003; Hartz et al., 2010; Pekcec et al., 2011; Mehta et al., 2013; Zoufal et al., 2020a,b). However, opposite age-related results for ABCB1 expression in the BCSFB, i.e., an increase in its expression, were reported in male Brown-Norway/Fischer rats (Pascale et al., 2011). In the APP transgenic mouse model of AD, ABCB1 expression and transport activity at the BBB decreased prior to the development of cognitive deficits (Hartz et al., 2010).
The mechanisms which regulate ABCB1 expression are not well understood (Lim et al., 2008; Do et al., 2016). Schulz et al. (2023), reviewing pathways controlling ABCB1 and ABCG2 at the BBB (the transporters were discussed together in the review by Schulz et al. (2023) because they both restrict entrance of drugs to the brain), listed nuclear receptors [including corticoid receptors, retinoid X receptor (RXR), pregnane X receptor (PXR), constitutive androstane receptor, peroxisome proliferator-activated receptor (PPAR), and thyroid receptors], inflammation [nuclear factor-kappa B (NF-κB), Wnt/β-catenin signaling, TNFα, prostaglandins, and other cytokines], oxidative stress, receptor tyrosine kinases, and growth factor signaling. Vogelgesang et al. (2004), studying the relationship between ABCB1 and Aβ in human brain specimens with cerebral amyloid angiopathy (CAA), reported biphasic regulation: when Aβ was initially deposited in arterioles where ABCB1 expression was low, ABCB1 expression was upregulated in capillaries, but ABCB1 expression then decreased as Aβ accumulated in capillaries. A more recent study involving parietal cortex microvessels (Bourassa et al., 2019) found decreased ABCB1 expression in AD patients compared to its expression in clinically normal subjects and individuals with MCI. The concentration of ABCB1 in microvessels in AD patients was inversely correlated with vascular Aβ40 levels and positively correlated with measures of cognition and memory. The decrease in ABCB1 expression in AD brain may be due to increased cerebral Aβ burden (Brenn et al., 2011; Kania et al., 2011). Mechanisms suggested to account for decreased cerebral ABCB1 expression in AD include activation of the receptor for advanced glycation endproducts (RAGE) in conjunction with NF-κB signaling (Park et al., 2014), and systemic inflammation (Hartz et al., 2006; Salkeni et al., 2009; Erickson et al., 2012), which is present in some AD patients (Holmes and Butchart, 2011). The ubiquitin-proteasome system (UPS) is involved in regulation of ABCB1 expression and activity on the BBB (Loo and Clarke, 1998; Zhang Y. et al., 2004; Hartz et al., 2016), and Aβ-induced ubiquitination of ABCB1 has also been implicated in decreased cerebral expression of ABCB1 in AD (Akkaya et al., 2015; Hartz et al., 2016, 2018). Interestingly, although proteasomal clearance of ubiquitinated proteins has been reported to be decreased in AD brain (Perry et al., 1987; Keck et al., 2003), UPS activity may be increased on the AD BBB (Akkaya et al., 2015; Hartz et al., 2016, 2018).
Because of the expression of ABCB1 on the luminal surface of the BBB, it does not have direct access to Aβ in interstitial fluid, so the mechanism by which it mediates the efflux of Aβ is unclear. Storck et al. (2018) proposed that the Aβ efflux-promoting activity of ABCB1 may be functionally linked to that of LRP1 via phosphatidylinositol binding clathrin assembly protein (PICALM), whose genetic polymorphisms have been identified as risk factors for LOAD (Harold et al., 2009; Xu et al., 2015; Ando et al., 2022). They suggested that transfer of Aβ from LRP1 to ABCB1 may occur in Rab11-positive sorting endosomes, where both proteins are present.
Approaches that have been used to increase ABCB1 expression in experimental systems are shown in Table 3. Although one of the approaches listed is prevention of ABCB1 ubiquitination, effective therapeutic targeting of the UPS is problematic. As discussed by Hartz et al. (2018), designing of small molecule inhibitors for ubiquitination-related enzymes is difficult, and restricting the inhibition of ubiquitination to specific tissues is challenging.
3.4 ABCC1
ABCC1 promotes the efflux of xenobiotic agents (including many chemotherapeutic agents and antibiotics), organic anions, and many compounds including the anti-oxidant glutathione (Wolf et al., 2012; Słomka et al., 2015). Its role as an export protein in AD has been studied mainly by Pahnke and colleagues (Krohn et al., 2011, 2015; Hofrichter et al., 2013; Schumacher et al., 2017; Zoufal et al., 2020c). Sultana and Butterfield (Sultana and Butterfield, 2004) suggested that it may play a neuroprotective role in AD by exporting glutathione-conjugated 4-hydroxy-2-trans-nonenal (HNE), a membrane lipid peroxidation product. HNE can be detoxified via its conjugation to the antioxidant glutathione. This activity is reduced in the AD brain (Lovell et al., 1998; Abuznait and Kaddoumi, 2012). A recent report suggested that in addition to its exporting of Aβ, ABCC1 may also lower Aβ levels by increasing the ratio of α- to β-secretase cleavage of APP (Jepsen et al., 2021).
ABCC1 is expressed in the CNS in many cell types including astrocytes, microglia, neurons, capillary endothelial cells, pericytes, neural stem and progenitor cells, and choroid plexus cells (Dallas et al., 2006; Bernstein et al., 2014; Pahnke et al., 2014; Matsumoto et al., 2015; Møllgård et al., 2017; Schumacher et al., 2017; Zoufal et al., 2020c). Its regulation was reported to be controlled by the transmembrane receptor protein Notch1 as well as transcription factors including GC (Guanine and Cytosine) elements and E-box elements (Cho et al., 2011). ABCC1 was reported to be upregulated in vitro in astrocytes following exposure to monomeric Aβ, resulting in increased release of glutathione; however, prolonged exposure to aggregated Aβ decreased ABCC1 activity (Ye et al., 2015). In the same study, brain ABCC1 transport activity was reported to be transiently increased in the 5×FAD mouse model of AD, with a subsequent decrease to below control levels. A study in APP/PS1 mice in which brain ABCC1 transport activity was measured at a single time point (170 days) found an increase in activity (Zoufal et al., 2020c). Although the cells responsible for this increase were not identified, it was suggested that an increase in ABCC1 activity in astrocytes, with a concomitant increase in their release of glutathione, may have been an effort to protect adjacent neurons from oxidative stress. Knockout of ABCC1 in APP/PS1 mice with a different genetic background resulted in increased brain concentrations of aggregated Aβ40 and Aβ42 (Krohn et al., 2011). The increased cerebral Aβ42 levels in APP/PS1 mice could be prevented by activation of ABCC1, either by an extract of St. John’s wort (Hofrichter et al., 2013) or by the anti-emetic drug Thiethylperazine (Krohn et al., 2011). In the latter study, knockout of ABCC1 in transgenic mice expressing the Dutch APP mutation [which causes CAA (Levy et al., 2006)] resulted in an increase in Aβ-immunoreactive cerebral vessels. Transport of Aβ42 in primary capillary endothelial cells from mouse brains in that study was reduced by approximately 60% by knockout of ABCC1, suggesting a major role for ABCC1 as an Aβ transporter.
The literature contains conflicting reports for the location of ABCC1 on the BBB [reviewed by Wolf et al. (2012)]. Some studies have found it on the luminal surface (Nies et al., 2004; Zhang Z. et al., 2004) while others have detected it on the abluminal surface (Soontornmalai et al., 2006; Roberts et al., 2008). Bernstein et al. (2014) concluded that ABCC1 was present on both luminal and abluminal membranes of brain capillary endothelial cells.
Experimental approaches to increase ABCC1 expression appear to have been limited, as indicated above, to administration of an extract of St. John’s wort (Hofrichter et al., 2013) and Thiethylperazine (Krohn et al., 2011). A phase 2 trial was initiated in November 2017 to investigate the safety and efficacy of Thiethylperazine in subjects with early onset AD (ClinicalTrials.gov Identifier: NCT03417986); although it was completed in 2021, results have apparently not been published. A potential drawback to the use of Thiethylperazine for treatment of AD is that although it promotes ABCC1-mediated transport activity, it inhibits the transport activity of ABCB1 (Wesołowska et al., 2009).
3.5 ABCC5
ABCC5 has been studied mainly because of its role in chemotherapeutic drug resistance. It was initially known as multidrug resistance protein 5 (MRP5) (Wijnholds et al., 2000). ABCC5 reduces access of some chemotherapeutic agents to tumors because of its activity as an efflux transporter of nucleoside/nucleotide analogs (Sampath et al., 2002; Ritter et al., 2005; Fukuda and Schuetz, 2012; Zhang et al., 2018). Another member of the ABC family, ABCC4 (MRP4), plays a similar role (Adachi et al., 2002). ABCC4 and ABCC5 are also efflux transporters for the second messengers 3′,5′-cyclic adenosine monophosphate (cAMP) and 3′,5′-cyclic guanosine monophosphate (cGMP) (Sampath et al., 2002; Wielinga et al., 2003; Ritter et al., 2005). Efflux of cAMP and cGMP may be an alternative mechanism to control their intracellular levels in addition to their degradation by phosphodiesterases (Meyer Zu Schwabedissen et al., 2005). ABCC5 also transports glutamate analogs including the excitotoxins N-methyl-D-aspartic acid and kainic acid (Jansen et al., 2015). ABCC5 expression has been detected in a range of tissues (Stojic et al., 2007); in the CNS it has been reported on the BBB and in neurons, astrocytes, microglia, and pericytes (Hirrlinger et al., 2002; Berezowski et al., 2004; Dallas et al., 2004; Warren et al., 2009; Jansen et al., 2015), as well as on the choroid plexus (Liddelow et al., 2012; Strazielle and Ghersi-Egea, 2015). ABCC5 expression was also detected on the hCMEC/D3 cell line (Carl et al., 2010), an immortalized human endothelial cell line suggested to be an in vitro model for the human BBB (Weksler et al., 2005). Comparison of mRNA levels of seven ABC transporters in human brain found the highest levels for ABCC5 and ABCG2 (Dutheil et al., 2009).
A PubMed search of “ABCC5 and Abeta clearance” found only one study. ABCC5 was reported to mediate transport of Aβ42 out of primary porcine brain endothelial cells (PBECs) (Shubbar and Penny, 2020). Although no reports were found of alterations of ABCC5 expression or activity in AD brain, the possibility that the ability of ABCC5 to prevent entrance of blood-borne Aβ into the brain may be impaired in AD was suggested by a study in which ABCC5 transporter activity was decreased after exposure of PBECs to Aβ42 (Shubbar and Penny, 2018).
ABCC5 expression was increased in CNE2 cells, an epithelial cell line (Sizhong et al., 1983), by increasing the expression of forkhead box M1, a cell growth-specific transcription factor (Hou et al., 2017). In another study, the anti-inflammatory agent dexamethasone increased ABCC5 expression and activity in primary PEBC cultures (Ho et al., 2023). Human chorionic gonadotropin was reported to regulate ABCC5 expression in human trophoblasts (Meyer Zu Schwabedissen et al., 2005).
3.6 ABCG1
ABCG1 is expressed in multiple organs (Nakamura et al., 2004); in the brain, it is present in neurons, astrocytes, and oligodendrocytes (Abildayeva et al., 2006; Kim et al., 2007; Tansley et al., 2007; Tarr and Edwards, 2008) and Liddelow et al. (2012) detected it on mouse choroid plexus. Optimal removal of cholesterol from cells is thought to require coordinated activity of ABCA1 and ABCG1 (Vaughan and Oram, 2005; Gelissen et al., 2006; Jasmin et al., 2014). ABCG1 transfers cholesterol to lipid-containing particles such as high-density lipoprotein (HDL), phospholipid vesicles, and lipidated apoE disks (Nakamura et al., 2004; Baldán et al., 2006; Kim et al., 2008; Tarr et al., 2009; Yu et al., 2010) whereas ABCA1 promotes the transfer of cholesterol and phospholipids to lipid-free (or lipid-poor) apolipoproteins (Oram and Heinecke, 2005; Prinz, 2007). In the brain, both transporters promote efflux of cholesterol from neurons to apoE (Alrosan et al., 2019). The expression of ABCG1, like ABCA1, is regulated by LXRs (Abildayeva et al., 2006; Cao et al., 2007; Burgess et al., 2008). A synthetic LXR activator, T0901317, upregulated expression of ABCG1, ABCA1, and apoE in the APP/PS1 transgenic mouse model of AD. Memory functions were improved but cortical and hippocampal SP counts were unchanged (Vanmierlo et al., 2011). Other regulators of ABCG1 include peroxisome proliferator-activated receptor (PPAR)-delta activators such as pioglitazone (Cocks et al., 2010), cellular sterol levels, and acute permeability barrier disruption (Jiang et al., 2006, 2010). Bexarotene was found to increase the expression of ABCG1 by activating RXR/LXR and RXR/PPAR heterodimers (Cramer et al., 2012; Zhao et al., 2014; Ren et al., 2019). Other RXR agonists have also been reported to increase ABCG1 expression (Boehm et al., 1995; Jung et al., 2010; Chen et al., 2011; Okabe et al., 2013; Sun et al., 2015). Although expression of ABCG1 has been reported in in vitro BBB models (Gosselet et al., 2009; Kober et al., 2017) and in the rat choroid plexus at the BCSFB (Fujiyoshi et al., 2007), ABCG1 does not appear to directly promote Aβ efflux from the brain. Conflicting results have been published regarding its effects on Aβ. Burgess et al. (2008) found that overexpression of ABCG1 in PDAPP mice did not change cerebral Aβ levels, similar to results in APP/PS1 mice in the study with synthetic LXR activator T0901317 discussed above (Vanmierlo et al., 2011). Kim et al. (2007) reported that expressing ABCG1 in APP-expressing Chinese hamster ovary cells reduced Aβ production, although Aβ’s clearance was unchanged, and Sano et al. (2016) found that expressing ABCG1 in human embryonic kidney cells containing the Swedish APP mutation decreased secretion of Aβ by these cells due to suppression of γ-secretase, an activity that was independent of ABCG1’s effects on lipid efflux. Kim et al. (2009) also reported that 27-hydroxycholesterol, which upregulated ABCG1, ABCA1, and apoE expression in primary human neuronal cultures, reduced Aβ in culture supernatants. [Conversely, (Prasanthi et al., 2009) was unable to detect upregulation of ABCG1 or ABCA1 by 27-hydroxycholesterol in SH-SY5Y cells, although cellular Aβ42 concentrations were increased; another oxidized cholesterol derivative, 24-hydroxycholesterol, did increase expression of ABCG1 and ABCA1, but this did not change cellular Aβ42 levels.] In contrast to studies which found upregulation of ABCG1 to be associated with decreased Aβ levels, other studies have found that ABCG1 upregulation increased Aβ levels. Tansley et al. (2007) reported that expressing ABCG1 in HEK cells containing the Swedish APP mutation increased Aβ production, and suggested that ABCG1 may have promoted APP processing by both amyloidogenic and nonamyloidogenic pathways. Dafnis et al. (2018) similarly found that expressing ABCG1 in SK-N-SH cells resulted in a moderate increase in Aβ production, which was suggested to be due to increased β-secretase activity.
In AD patients, the cholesterol efflux activity of cerebrospinal fluid (CSF), which is mediated by both ABCG1 and ABCA1 and promotes transfer of cholesterol from astrocytes to neurons, was reported to be reduced (Marchi et al., 2019). Cholesterol is necessary for optimal neuronal development and function (Dietschy and Turley, 2004; Hayashi et al., 2004; Göritz et al., 2007; Valenza et al., 2015). Because it does not cross the BBB (Vitali et al., 2014), neurons in the brain must be supplied by locally produced cholesterol. Adult neurons lose the ability to synthesize cholesterol (Dietschy and Turley, 2004) and obtain it from glial cells (Mauch et al., 2001; Pfrieger and Ungerer, 2011; Marchi et al., 2019). Significant associations between ABCG1 single nucleotide polymorphisms (SNPs) and AD were detected in some European populations but not others (Wollmer et al., 2007).
3.7 ABCG2
ABCG2, also known as Breast Cancer Resistance Protein (BCRP) (Doyle et al., 1998), is expressed in many tissues including brain, liver, kidney, small intestine, mammary gland, and bone marrow (Zhou et al., 2001; Langmann et al., 2003; Zhang et al., 2003; Sarkadi et al., 2004; Blanco-Paniagua et al., 2021). It is present on the BBB on the luminal surface of microvessel endothelium (Cooray et al., 2002) where it co-localizes with ABCB1 Møllgård et al. (2017) and Wanek et al. (2020) reported its expression in human choroid plexus. Aβ is a substrate for ABCG2 (Xiong et al., 2009; Do et al., 2012; Shubbar and Penny, 2020). Whether ABCG2 transports Aβ out of the brain or prevents it from crossing the BBB into the brain is unclear. Evidence for ABCG2-mediated efflux of Aβ from the brain comes from a study which found that overexpressing human ABCG2 in immortalized rat brain endothelial cells increased abluminal-to-luminal transport of substrates (Zhang et al., 2003). Conversely, the possibility that ABCG2 may limit passage of Aβ from the BBB into the brain was suggested by the finding that inhibiting ABCG2 resulted in increased apical-to-basolateral transport of Aβ in endothelial cells (Tai et al., 2009). This was also suggested by another study which showed that although peripheral injection of Aβ40 resulted in greater brain accumulation of Aβ in ABCG2-knockout mice than in wild-type mice, the lack of ABCG2 did not appear to change the rate of Aβ elimination from the brain (Zhang et al., 2013). The influence of Aβ on ABCG2 expression and activity is unclear. While two studies found that exposure of human brain endothelial cells to Aβ did not influence ABCG2 expression (Xiong et al., 2009; Kania et al., 2011), another study found that ABCG2 efflux activity in porcine endothelial cells decreased following exposure of these cells to Aβ (Shubbar and Penny, 2018).
Conflicting reports have been published regarding changes in ABCG2 expression in transgenic mouse models of AD. Decreased numbers of ABCG2-immunoreactive brain microvessels were reported in APP/PS1 mice (Wanek et al., 2020) but upregulation of ABCG2 was reported in brain specimens from Tg-SwDI and 3×Tg mice (Xiong et al., 2009). Conflicting reports have also been published for changes in ABCG2 expression in brain specimens from individuals with AD and/or CAA (Xiong et al., 2009; Wijesuriya et al., 2010; Carrano et al., 2014; Kannan et al., 2017; Storelli et al., 2021) and associations between ABCG2 variants and the risk for developing AD (Cascorbi et al., 2013; Fehér et al., 2013).
Upregulation of ABCG2 has been suggested to protect neurons against reactive oxygen species and inflammatory cytokines by inhibiting nuclear factor kappa B activation (Shen et al., 2010) and, because ABCG2 is an efflux transporter of glutathione, by increasing extracellular glutathione concentrations (Brechbuhl et al., 2010). A role for ABCG2 in protecting against oxidative stress was also suggested by a study which found increased brain lipid/DNA oxidation in Tg-SwDI mice lacking ABCG2 (Zeng et al., 2012). The possibility that ABCG2 may reduce amyloidogenic processing of APP was suggested by a report that Aβ40 production decreased when ABCG2 was overexpressed in N2a-695 cells (Shen et al., 2010).
ABCG2 expression has been upregulated by exposure of human brain endothelial cells to conditioned medium from Aβ-activated microglia (Xiong et al., 2009), with similar findings in HEK293 cells after exposure to astrocyte-conditioned medium (Hori et al., 2004). Upregulation of ABCG2 in these studies may have been due to its activation by cytokines, because increased ABCG2 expression was detected in the human hCMEC/D3 cell line following exposure to inflammatory cytokines (Poller et al., 2010). Dexamethasone, stated above to increase ABCC5 expression and activity in porcine brain endothelial cells, also increased ABCG2 expression and activity in these cells (Ho et al., 2023). Exposure of brain endothelial cells to glutamate downregulated ABCG2 expression (Salvamoser et al., 2015). Other regulators of ABCG2 expression (discussed by Nakanishi and Ross, 2012) include hypoxia and hypoxia/oxidative-sensitive transcription factors (Krishnamurthy et al., 2004; Zeng et al., 2012), hormones (Yasuda et al., 2005; Wang et al., 2006), growth factors (Meyer zu Schwabedissen et al., 2006), gene amplification, epigenetic regulation (demethylation, histone acetylation, transcription factors including PPARγ, estrogen receptor alpha, progesterone receptor) (Mo and Zhang, 2012), and some chemotherapeutic agents, in agreement with the role of ABCG2 in multidrug resistance (Sukowati et al., 2012).
3.8 ABCG4
The structure of ABCG4 is similar to that of ABCG1. Like ABCG1 it promotes cholesterol synthesis (Tarr and Edwards, 2008) and efflux of cholesterol (as well as desmosterol, an intermediate in cholesterol synthesis) from cells to HDL but not to lipid-poor apolipoproteins (Wang et al., 2004; Vaughan and Oram, 2005; Kim et al., 2008). ABCG4 and ABCG1 have been suggested to play complementary roles in brain cholesterol homeostasis (Sun et al., 2014). ABCG4 may not be involved in the process by which adult neurons in the brain obtain cholesterol via efflux from glial cells (Abildayeva et al., 2006).
ABCG4 is expressed mainly in brain and eye (Annilo et al., 2001; Oldfield et al., 2002; Tarr and Edwards, 2008) although it has also been found in spleen, bone marrow, testes, skin and thymus (Yoshikawa et al., 2002; Wang et al., 2008). In the brain it is present primarily on glial cells and neurons (Wang et al., 2008; Sano et al., 2016). It was not detected in an in vitro model of the pig BBB (Kober et al., 2017) but was found on the mouse BBB, where it promoted efflux of both Aβ and desmosterol; interestingly, desmosterol and Aβ appeared to compete for ABCG4-mediated efflux (Dodacki et al., 2017). These findings followed an earlier report from the same investigators that ABCG4 mediated efflux of Aβ40 from HEK293 cells (Do et al., 2012). ABCG4 was also reported to be present on the choroid plexus, suggesting that it may promote efflux of Aβ out of the brain via the BCSFB (Fujiyoshi et al., 2007; Liddelow et al., 2012; Matsumoto et al., 2015). Both ABCG1 and ABCG4 inhibited γ-secretase activity in HEK 293 cells expressing the Swedish APP mutation (Sano et al., 2016), so this may be an additional mechanism by which they lower Aβ levels. ABCG4 expression was reported to be increased in plaque-associated microglia in AD brain (Uehara et al., 2008) and in brain microvessels from 3-month-old 3×Tg-AD mice (Do et al., 2016). Whether its expression in neurons and astrocytes changes during progression of AD is unknown.
Some but not all studies have found ABCG4 expression to be induced by LXRs (Engel et al., 2001; Abildayeva et al., 2006; Tarr and Edwards, 2008; Wang et al., 2008; Graham, 2015; Yang et al., 2021). Post-translational regulation of ABCG4 has been suggested to be related to its ubiquitination (Alrosan et al., 2019).
4 Discussion
The ABC transporters which influence Aβ levels in the brain have different mechanisms of action, some of which involve lipid metabolism (ABCA1, ABCA7, ABCG1, ABCG4). The expression of some of these transporters is decreased in AD brain and/or transgenic mouse models of AD (ABCB1, ABCG1) while others are increased (ABCA7, ABCC1, ABCG4; conflicting reports for ABCG2). If the expression and/or activity of the ABC transporters which promote efflux of Aβ from the brain or reduce its entrance into the brain could be increased therapeutically in AD patients, this might lower brain levels of soluble Aβ (and by doing so, perhaps the aggregation of Aβ to form SPs would be reduced). Whether such an approach used as “stand-alone therapy” would slow AD’s clinical progression is unknown. As indicated in the Introduction, efforts to slow AD progression by lowering brain Aβ levels failed in large-scale clinical trials until the recent findings with Lecanemab and Donanemab, and although the effects of these monoclonal antibodies on AD progression were statistically significant in their respective phase 3 trials, they were modest. Therefore even if cerebral expression of the ABC transporters discussed above could be increased therapeutically, it is unclear if this would slow AD’s neuropathological or clinical progression. The few studies in which expression of the relevant ABC transporters has been experimentally increased have had inconsistent results. Improvement of cognitive and/or memory impairments, or lowering of brain Aβ, was reported in transgenic mouse models of AD after treatment with ABCB1-upregulating exosomes (Pan et al., 2020), and the M1 receptor antagonist Pirenzepine, which upregulates ABCB1 (Paganetti et al., 2014); however, administration of Bexarotene to transgenic mouse models of AD produced conflicting results, overexpressing of ABCG1 in PDAPP mice did not change brain Aβ levels (Burgess et al., 2008) and treatment of 3×TgAD mice with Dexamethasone, which upregulates ABCC5 and ABCG2, exacerbated Aβ pathology (Green et al., 2006). Possible deleterious side effects would also be a concern with therapeutic increasing of the expression of the Aβ-regulating ABC transporters; as discussed above, treatment with Bexarotene and other LXR agonists can increase plasma triglycerides (Koldamova et al., 2010), and Thiethylperazine inhibits the transport activity of ABCB1 (Wesołowska et al., 2009) although it promotes ABCC1-mediated transport activity. In vitro findings suggesting that upregulation of ABCG1 may increased Aβ levels by increasing β-secretase cleavage of APP (Tansley et al., 2007; Dafnis et al., 2018) would also be a concern.
Literature searches were performed to identify human clinical applications for the experimental approaches which have been used to increase ABC transporter expression. These applications are shown in Tables 4–9. The highest number of human applications were found for approaches which increase the expression of ABCA1 (Table 4), ABCB1 (Table 5), ABCG1 (Table 8), and ABCG2 (Table 9). Clinical applications which would increase ABCC1 expression appear to have been limited to St. John’s wort and Thiethylperazine, and only in vitro studies were found for ABCG4 (Wang et al., 2004; Vaughan and Oram, 2006; Sano et al., 2016). Therapeutic interventions with relatively large numbers of clinical applications include Bexarotene (Table 4), exosomes, PXR agonists, ketone bodies, N-methyl-D-aspartate receptor agonists [which would be contraindicated in AD, because excitotoxicity has been implicated in AD-associated neurotoxicity (Wang and Reddy, 2017)], Pirenzepine (Table 5), Oleocanthal/extra virgin olive oil (Tables 4, 5, 8), Dexamethasone (Tables 7, 9), intermittent hypoxia, Progesterone, Epidermal Growth Factor, and Doxorubicin (Table 9).
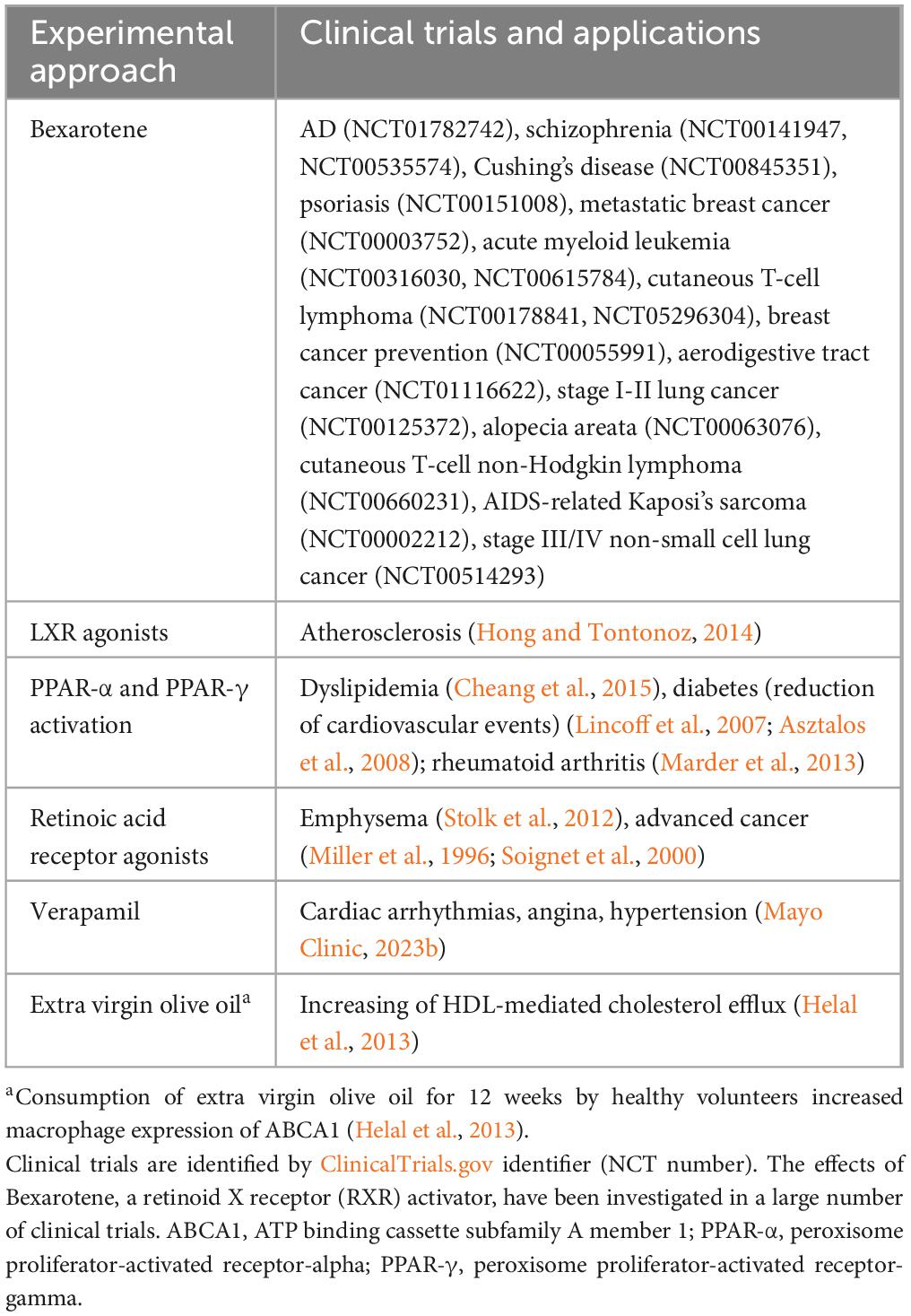
Table 4. Clinical trials and clinical applications of experimental approaches to increase ABCA1 expression.
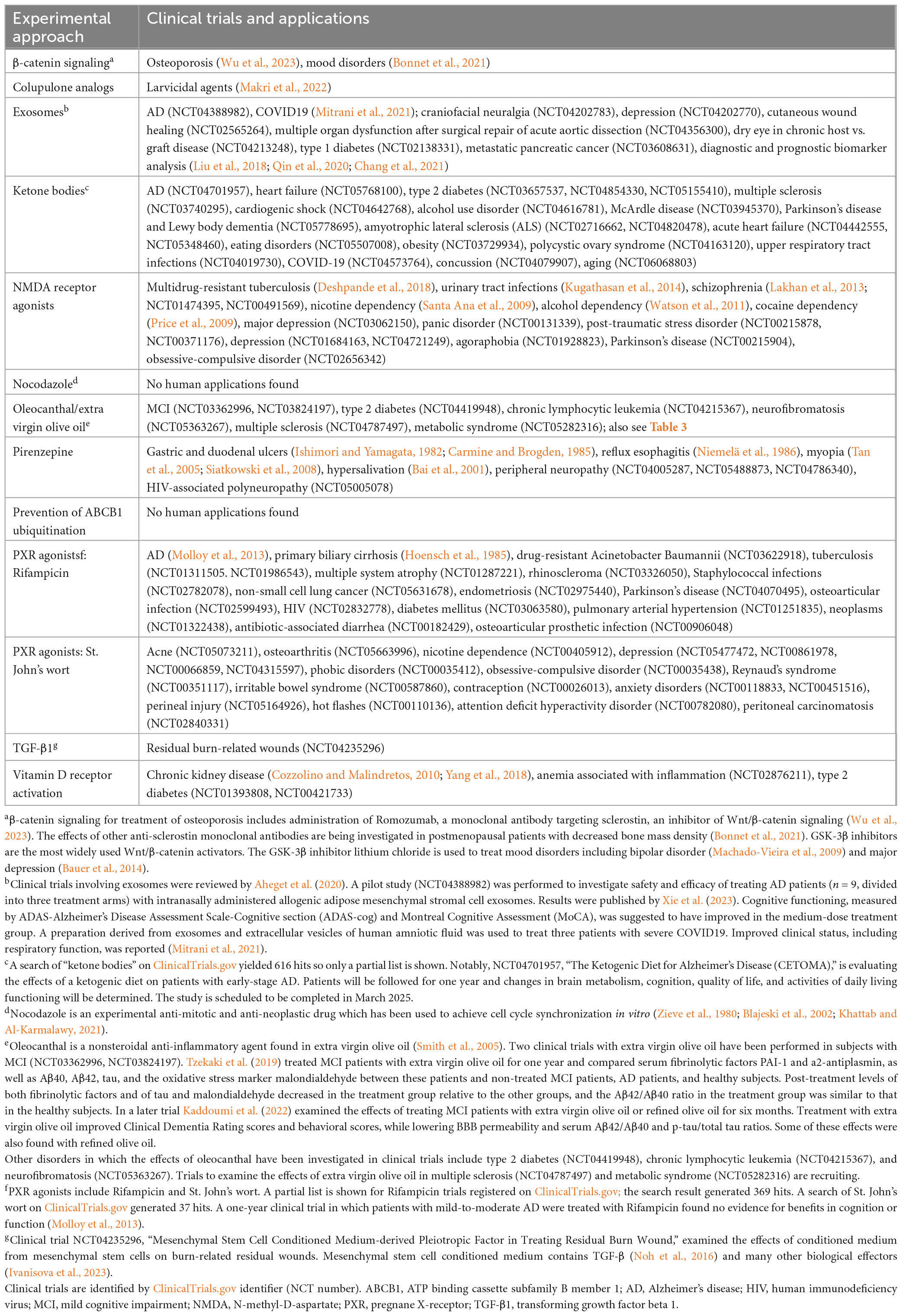
Table 5. Clinical trials and clinical applications of experimental approaches to increase ABCB1 expression.
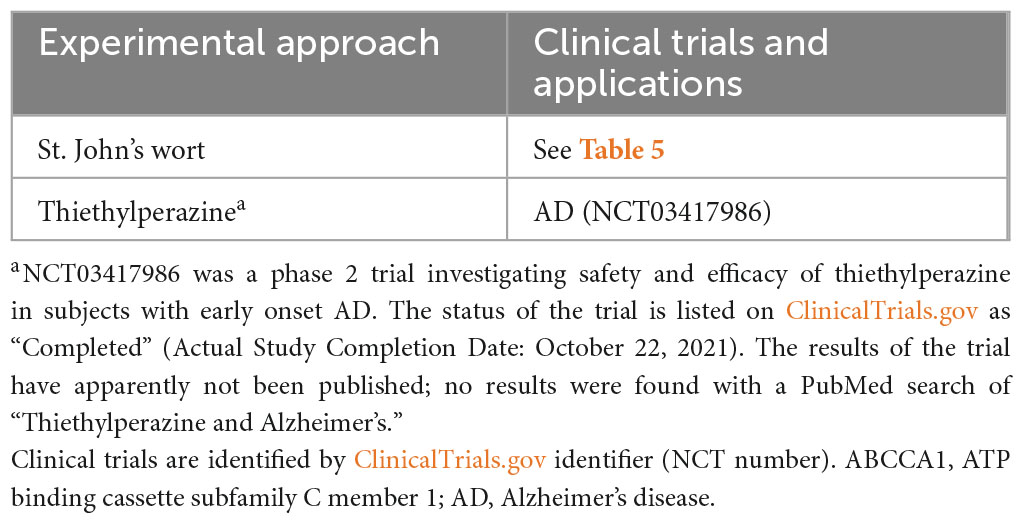
Table 6. Clinical trials and clinical applications of experimental approaches to increase ABCC1 expression.
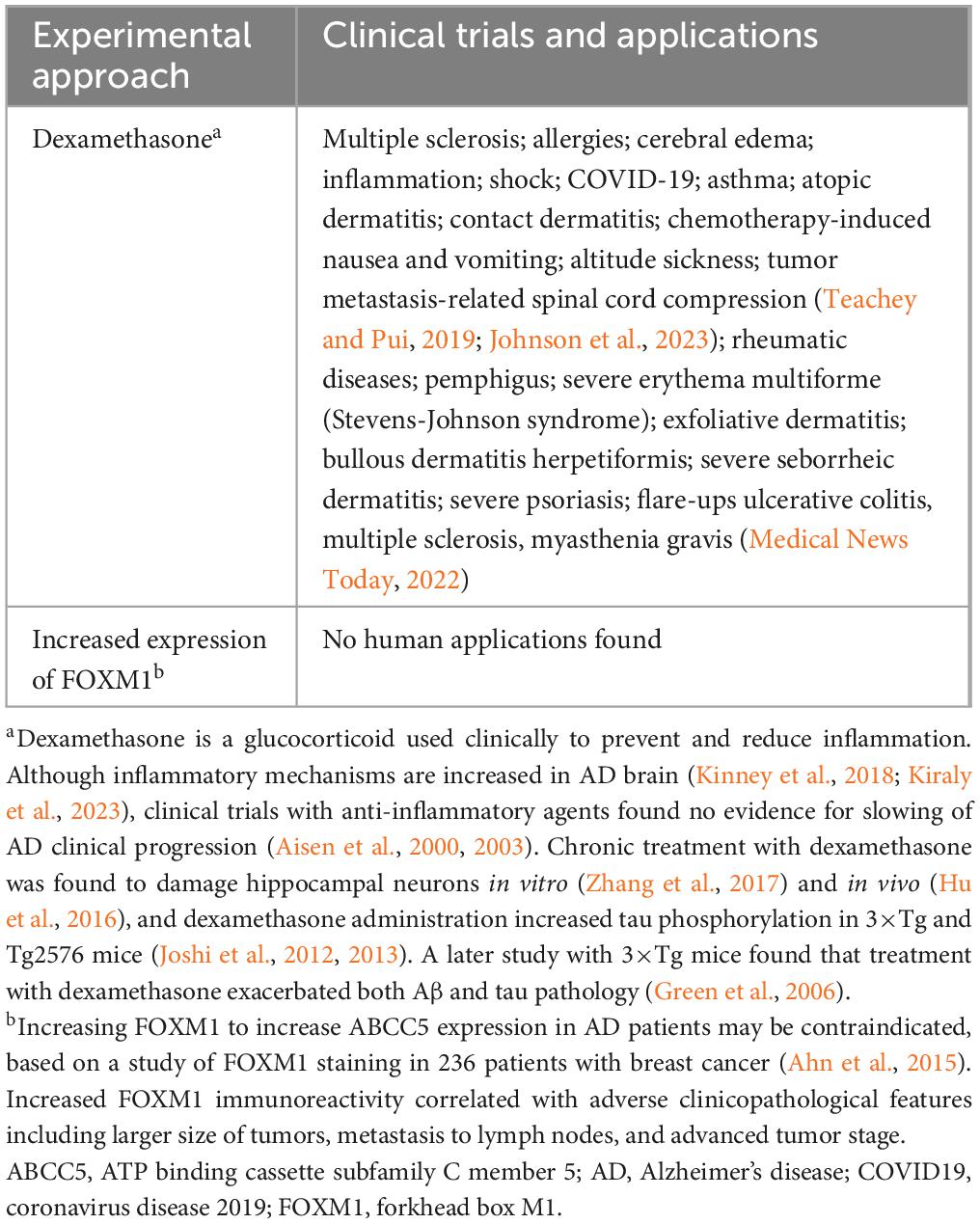
Table 7. Clinical trials and clinical applications of experimental approaches to increase ABCC5 expression.
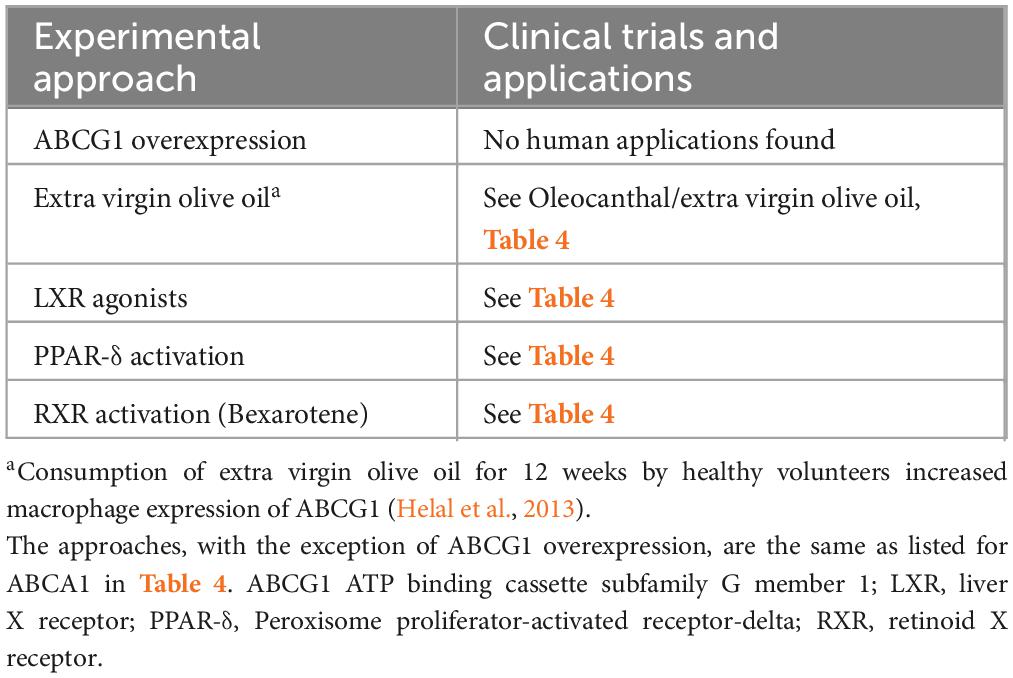
Table 8. Clinical trials and clinical applications of experimental approaches to increase ABCG1 expression.
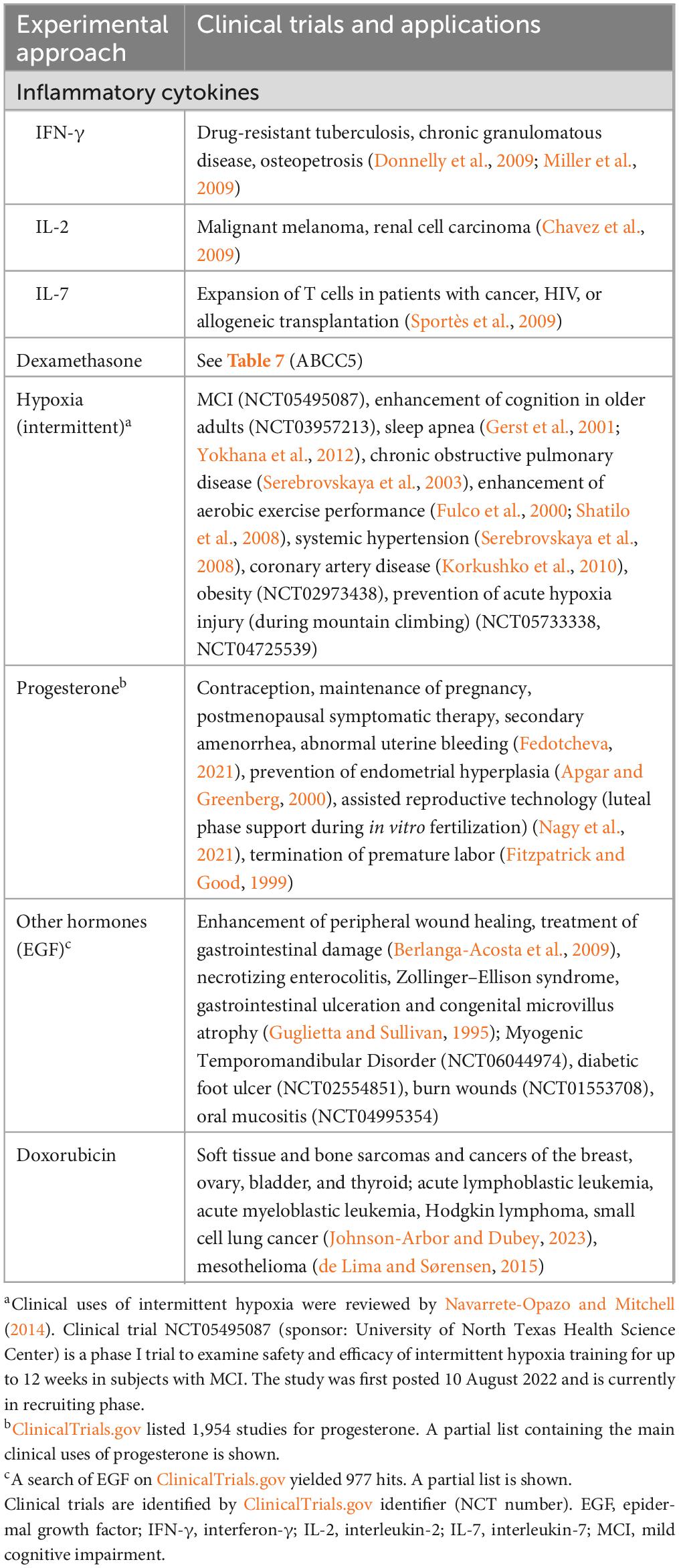
Table 9. Clinical trials and clinical applications of experimental approaches to increase ABCG2 expression.
Few clinical trials have investigated the effects of therapeutic approaches which increase the expression of ABC transporters in AD patients or individuals with MCI. A phase 2 trial with Bexarotene was performed in AD patients (Cummings et al., 2016); lowering of brain Aβ was found in apoE4 noncarriers but not in apoE4 carriers. A clinical trial with the PXR agonist Rifampicin found no benefits on cognition or functioning in AD patients (Molloy et al., 2013). A phase I/II study involving intranasal administration of mesenchymal stromal cell exosomes to AD patients concluded that this approach was safe, and cognitive functioning was suggested to have improved in the medium-dose treatment group (Xie et al., 2023).
Administration of extra virgin olive oil to subjects with MCI for one year decreased serum fibrinolytic factors, the Aβ1-42/Aβ1-40 ratio, and the oxidative stress marker malondialdehyde (Tzekaki et al., 2019), while another study found that six-month administration of extra virgin olive oil to individuals with MCI improved their clinical dementia rating and behavioral scores (Kaddoumi et al., 2022). Among studies investigating the effects of ketogenic diets or ketogenic supplementation in AD patients (reviewed by Lilamand et al., 2022), one study found no cognitive change (Henderson et al., 2020) while others reported improvements in activities of daily living and quality of life (Phillips et al., 2021) and cognitive functioning (Xu et al., 2020). Improved memory or cognition was also found in two studies with ketogenic diet-treated subjects with MCI (Fortier et al., 2020; Neth et al., 2020). As stated above, results have not been published from a phase 2 trial to investigate the safety and efficacy of Thiethylperazine in early onset AD. A trial to investigate the efficacy of intermittent hypoxia in patients with MCI (NCT05495087) is in the recruiting stage. Other approaches for increasing ABC transporter expression which might be worthwhile to evaluate in AD pilot studies include LXR agonists, retinoic acid receptor agonists, β-catenin signaling, Verapamil, and St. John’s wort.
With regard to additional future research directions: evidence for the ability of some of the ABC transporters discussed above to promote clearance of cerebral Aβ is based primarily on findings that knockout of these transporters decreased Aβ efflux or increased Aβ levels in vitro or in transgenic mouse models of AD. The effects of approaches to increase the expression of the relevant ABC transporters could be examined in these models. Studies could also be performed in mouse models of AD to determine if increasing the expression of Aβ-regulating ABC transporters would further slow the progression of AD-related neuropathology or cognitive deficits when combined with administration of Lecanemab or Donanemab; if encouraging findings are obtained, this could be examined further in an AD pilot study.
5 Conclusion
Eight members of the human ABC transporter superfamily have been suggested to participate in clearing of Aβ from the brain. Although these transporters promote Aβ clearance by different mechanisms, several of them do so as a consequence of their involvement in regulation of lipid metabolism, including promoting synthesis and efflux of cholesterol. Some of the transporters may contribute to lowering of cerebral Aβ indirectly, for example by increasing non-amyloidogenic cleavage of APP, increasing phagocytic clearance of Aβ, exerting neuroprotective effects (such as exporting neurotoxic lipid peroxidation products, inhibiting nuclear factor kappa B activation, and increasing extracellular glutathione), and preventing Aβ from entering the brain via the BBB. The expression of ABC transporters involved in cerebral Aβ clearance has been increased through many experimental approaches, some of which are commonly used to treat conditions unrelated to AD; however, few of these approaches have been investigated for efficacy in AD patients. The possibility that therapeutic upregulation of selected ABC transporters might slow AD progression should be further explored.
Author contributions
DL: Conceptualization, Writing – original draft, Writing – review & editing.
Funding
The author declares financial support was received for the research, authorship, and/or publication of this article. Funding was provided by donations to the Beaumont Foundation to support Alzheimer’s research in the Beaumont Research Institute.
Conflict of interest
The author declares that the research was conducted in the absence of any commercial or financial relationships that could be construed as a potential conflict of interest.
Publisher’s note
All claims expressed in this article are solely those of the authors and do not necessarily represent those of their affiliated organizations, or those of the publisher, the editors and the reviewers. Any product that may be evaluated in this article, or claim that may be made by its manufacturer, is not guaranteed or endorsed by the publisher.
References
Abe-Dohmae, S., and Yokoyama, S. (2021). ABCA7 links sterol metabolism to the host defense system: Molecular background for potential management measure of Alzheimer’s disease. Gene 768:145316. doi: 10.1016/j.gene.2020.145316
Abildayeva, K., Jansen, P. J., Hirsch-Reinshagen, V., Bloks, V. W., Bakker, A. H., Ramaekers, F. C., et al. (2006). 24(S)-hydroxycholesterol participates in a liver X receptor-controlled pathway in astrocytes that regulates apolipoprotein E-mediated cholesterol efflux. J. Biol. Chem. 281, 12799–12808. doi: 10.1074/jbc.M601019200
Abuznait, A. H., and Kaddoumi, A. (2012). Role of ABC transporters in the pathogenesis of Alzheimer’s disease. ACS Chem. Neurosci. 3, 820–831. doi: 10.1021/cn300077c
Abuznait, A. H., Qosa, H., Busnena, B. A., El Sayed, K. A., and Kaddoumi, A. (2013). Olive-oil-derived oleocanthal enhances β-amyloid clearance as a potential neuroprotective mechanism against Alzheimer’s disease: In vitro and in vivo studies. ACS Chem. Neurosci. 4, 973–982. doi: 10.1021/cn400024q
Adachi, M., Reid, G., and Schuetz, J. D. (2002). Therapeutic and biological importance of getting nucleotides out of cells: A case for the ABC transporters, MRP4 and 5. Adv. Drug Deliv. Rev. 54, 1333–1342. doi: 10.1016/s0169-409x(02)00166-7
Aheget, H., Tristán-Manzano, M., Mazini, L., Cortijo-Gutierrez, M., Galindo-Moreno, P., Herrera, C., et al. (2020). Exosome: A new player in translational nanomedicine. J. Clin. Med. 9:2380. doi: 10.3390/jcm9082380
Ahn, H., Sim, J., Abdul, R., Chung, M. S., Paik, S. S., Oh, Y. H., et al. (2015). Increased expression of forkhead box M1 is associated with aggressive phenotype and poor prognosis in estrogen receptor-positive breast cancer. J. Korean Med. Sci. 30, 390–397. doi: 10.3346/jkms.2015.30.4.390
Aikawa, T., Holm, M. L., and Kanekiyo, T. (2018). ABCA7 and pathogenic pathways of Alzheimer’s disease. Brain Sci. 8:27. doi: 10.3390/brainsci8020027
Aisen, P. S., Davis, K. L., Berg, J. D., Schafer, K., Campbell, K., Thomas, R. G., et al. (2000). A randomized controlled trial of prednisone in Alzheimer’s disease. Alzheimer’s disease cooperative study. Neurology 54, 588–593. doi: 10.1212/wnl.54.3.588
Aisen, P. S., Gauthier, S., Ferris, S. H., Saumier, D., Haine, D., Garceau, D., et al. (2011). Tramiprosate in mild-to-moderate Alzheimer’s disease – a randomized, double-blind, placebo-controlled, multi-centre study (the alphase study). Arch. Med. Sci. 7, 102–111. doi: 10.5114/aoms.2011.20612
Aisen, P. S., Schafer, K. A., Grundman, M., Pfeiffer, E., Sano, M., Davis, K. L., et al. (2003). Effects of rofecoxib or naproxen vs placebo on Alzheimer disease progression: A randomized controlled trial. JAMA 289, 2819–2826. doi: 10.1001/jama.289.21.2819
Akkaya, B. G., Zolnerciks, J. K., Ritchie, T. K., Bauer, B., Hartz, A. M., Sullivan, J. A., et al. (2015). The multidrug resistance pump ABCB1 is a substrate for the ubiquitin ligase NEDD4-1. Mol. Membr. Biol. 32, 39–45. doi: 10.3109/09687688.2015.1023378
Alrosan, A., Aleidi, S. M., Yang, A., Brown, A. J., and Gelissen, I. C. (2019). The adaptor protein alix is involved in the interaction between the ubiquitin ligase NEDD4-1 and its targets, ABCG1 and ABCG4. Int. J. Mol. Sci. 20:2714. doi: 10.3390/ijms20112714
Alzheimer’s Association (2023). 2023 Alzheimer’s disease facts and figures. Alzheimers Dement. 19, 1598–1695. doi: 10.1002/alz.13016
Ando, K., Nagaraj, S., Küçükali, F., de Fisenne, M. A., Kosa, A. C., Doeraene, E., et al. (2022). PICALM and Alzheimer’s disease: An update and perspectives. Cells 11:3994. doi: 10.3390/cells11243994
Annilo, T., Tammur, J., Hutchinson, A., Rzhetsky, A., Dean, M., and Allikmets, R. (2001). Human and mouse orthologs of a new ATP-binding cassette gene, ABCG4. Cytogenet. Cell Genet. 94, 196–201. doi: 10.1159/000048816
Apgar, B. S., and Greenberg, G. (2000). Using progestins in clinical practice. Am. Fam. Phys. 62, 1839–1846.
Asztalos, B. F., Collins, D., Horvath, K. V., Bloomfield, H. E., Robins, S. J., and Schaefer, E. J. (2008). Relation of gemfibrozil treatment and high-density lipoprotein subpopulation profile with cardiovascular events in the veterans affairs high-density lipoprotein intervention trial. Metabolism 57, 77–83. doi: 10.1016/j.metabol.2007.08.009
Baello, S., Iqbal, M., Bloise, E., Javam, M., Gibb, W., and Matthews, S. G. (2014). TGF-β1 regulation of multidrug resistance P-glycoprotein in the developing male blood-brain barrier. Endocrinology 155, 475–484. doi: 10.1210/en.2013-1472
Bai, Y. M., Lin, C. C., Chen, J. Y., and Liu, W. C. (2001). Therapeutic effect of pirenzepine for clozapine-induced hypersalivation: A randomized, double-blind, placebo-controlled, cross-over study. J. Clin. Psychopharmacol. 21, 608–611. doi: 10.1097/00004714-200112000-00012
Baldán, A., Tarr, P., Lee, R., and Edwards, P. A. (2006). ATP-binding cassette transporter G1 and lipid homeostasis. Curr. Opin. Lipidol. 17, 227–232. doi: 10.1097/01.mol.0000226113.89812.bb
Balducci, C., Paladini, A., Micotti, E., Tolomeo, D., La Vitola, P., Grigoli, E., et al. (2015). The continuing failure of bexarotene in Alzheimer’s disease mice. J. Alzheimers Dis. 46, 471–482. doi: 10.3233/JAD-150029
Balin, B. J., and Hudson, A. P. (2014). Etiology and pathogenesis of late-onset Alzheimer’s disease. Curr. Allergy Asthma Rep. 14:417. doi: 10.1007/s11882-013-0417-1
Bartels, A. L., Kortekaas, R., Bart, J., Willemsen, A. T., de Klerk, O. L., de Vries, J. J., et al. (2009). Blood-brain barrier P-glycoprotein function decreases in specific brain regions with aging: A possible role in progressive neurodegeneration. Neurobiol. Aging 30, 1818–1824. doi: 10.1016/j.neurobiolaging.2008.02.002
Bauer, B., Hartz, A. M., Pekcec, A., Toellner, K., Miller, D. S., and Potschka, H. (2008). Seizure-induced up-regulation of P-glycoprotein at the blood-brain barrier through glutamate and cyclooxygenase-2 signaling. Mol. Pharmacol. 73, 1444–1453. doi: 10.1124/mol.107.041210
Bauer, M., Adli, M., Ricken, R., Severus, E., and Pilhatsch, M. (2014). Role of lithium augmentation in the management of major depressive disorder. CNS Drugs 28, 331–334. doi: 10.1007/s40263-014-0152-8
Bell, R. D., Sagare, A. P., Friedman, A. E., Bedi, G. S., Holtzman, D. M., Deane, R., et al. (2007). Transport pathways for clearance of human Alzheimer’s amyloid beta-peptide and apolipoproteins E and J in the mouse central nervous system. J. Cereb. Blood Flow Metab. 27, 909–918. doi: 10.1038/sj.jcbfm.9600419
Bellenguez, C., Küçükali, F., Jansen, I. E., Kleineidam, L., Moreno-Grau, S., Amin, N., et al. (2022). New insights into the genetic etiology of Alzheimer’s disease and related dementias. Nat. Genet. 54, 412–436. doi: 10.1038/s41588-022-01024-z
Berezowski, V., Landry, C., Dehouck, M. P., Cecchelli, R., and Fenart, L. (2004). Contribution of glial cells and pericytes to the mRNA profiles of P-glycoprotein and multidrug resistance-associated proteins in an in vitro model of the blood-brain barrier. Brain Res. 1018, 1–9. doi: 10.1016/j.brainres.2004.05.092
Berlanga-Acosta, J., Gavilondo-Cowley, J., Lopez-Saura, P., Gonzalez-Lopez, T., Castro-Santana, M. D., Lopez-Mola, E., et al. (2009). Epidermal growth factor in clinical practice–a review of its biological actions, clinical indications and safety implications. Int. Wound J. 6, 331–346. doi: 10.1111/j.1742-481X.2009.00622.x
Bernstein, H. G., Hölzl, G., Dobrowolny, H., Hildebrandt, J., Trübner, K., Krohn, M., et al. (2014). Vascular and extravascular distribution of the ATP-binding cassette transporters ABCB1 and ABCC1 in aged human brain and pituitary. Mech. Ageing Dev. 141-142, 12–21. doi: 10.1016/j.mad.2014.08.003
Bharate, J. B., Batarseh, Y. S., Wani, A., Sharma, S., Vishwakarma, R. A., Kaddoumi, A., et al. (2015). Synthesis and P-glycoprotein induction activity of colupulone analogs. Org. Biomol. Chem. 13, 5488–5496. doi: 10.1039/c5ob00554j
Biedler, J. L., and Riehm, H. (1970). Cellular resistance to actinomycin D in Chinese hamster cells in vitro: Cross-resistance, radioautographic, and cytogenetic studies. Cancer Res. 30, 1174–1184.
Blajeski, A. L., Phan, V. A., Kottke, T. J., and Kaufmann, S. H. (2002). G(1) and G(2) cell-cycle arrest following microtubule depolymerization in human breast cancer cells. J. Clin. Invest. 110, 91–99.
Blanco-Paniagua, E., García-Lino, A. M., García-Mateos, D., Álvarez, A. I., and Merino, G. (2021). Role of the Abcg2 transporter in plasma levels and tissue accumulation of the anti-inflammatory tolfenamic acid in mice. Chem. Biol. Interact. 345:109537. doi: 10.1016/j.cbi.2021.109537
Boehm, M. F., Zhang, L., Zhi, L., McClurg, M. R., Berger, E., Wagoner, M., et al. (1995). Design and synthesis of potent retinoid X receptor selective ligands that induce apoptosis in leukemia cells. J. Med. Chem. 38, 3146–3155. doi: 10.1021/jm00016a018
Bonnet, C., Brahmbhatt, A., Deng, S. X., and Zheng, J. J. (2021). WNT signaling activation: Targets and therapeutic opportunities for stem cell therapy and regenerative medicine. RSC Chem. Biol. 2, 1144–1157. doi: 10.1039/d1cb00063b
Bourassa, P., Tremblay, C., Schneider, J. A., Bennett, D. A., and Calon, F. (2019). Beta-amyloid pathology in human brain microvessel extracts from the parietal cortex: Relation with cerebral amyloid angiopathy and Alzheimer’s disease. Acta Neuropathol. 137, 801–823. doi: 10.1007/s00401-019-01967-4
Brechbuhl, H. M., Gould, N., Kachadourian, R., Riekhof, W. R., Voelker, D. R., and Day, B. J. (2010). Glutathione transport is a unique function of the ATP-binding cassette protein ABCG2. J. Biol. Chem. 285, 16582–16587. doi: 10.1074/jbc.M109.090506
Brenn, A., Grube, M., Peters, M., Fischer, A., Jedlitschky, G., Kroemer, H. K., et al. (2011). Beta-Amyloid downregulates MDR1-P-glycoprotein (Abcb1) expression at the blood-brain barrier in mice. Int. J. Alzheimers Dis. 2011:690121. doi: 10.4061/2011/690121
Burgess, B. L., Parkinson, P. F., Racke, M. M., Hirsch-Reinshagen, V., Fan, J., Wong, C., et al. (2008). ABCG1 influences the brain cholesterol biosynthetic pathway but does not affect amyloid precursor protein or apolipoprotein E metabolism in vivo. J. Lipid Res. 49, 1254–1267. doi: 10.1194/jlr.M700481-JLR200
Burns, M. P., Vardanian, L., Pajoohesh-Ganji, A., Wang, L., Cooper, M., Harris, D. C., et al. (2006). The effects of ABCA1 on cholesterol efflux and abeta levels in vitro and in vivo. J. Neurochem. 98, 792–800. doi: 10.1111/j.1471-4159.2006.03925.x
Candela, P., Gosselet, F., Saint-Pol, J., Sevin, E., Boucau, M. C., Boulanger, E., et al. (2010). Apical-to-basolateral transport of amyloid-beta peptides through blood–brain barrier cells is mediated by the receptor for advanced glycation end-products and is restricted by P-glycoprotein. J. Alzheimers Dis. 22, 849–859. doi: 10.3233/JAD-2010-100462
Cao, G., Bales, K. R., DeMattos, R. B., and Paul, S. M. (2007). Liver X receptor-mediated gene regulation and cholesterol homeostasis in brain: Relevance to Alzheimer’s disease therapeutics. Curr. Alzheimer Res. 4, 179–184. doi: 10.2174/156720507780362173
Carl, S. M., Lindley, D. J., Das, D., Couraud, P. O., Weksler, B. B., Romero, I., et al. (2010). ABC and SLC transporter expression and proton oligopeptide transporter (POT) mediated permeation across the human blood–brain barrier cell line, hCMEC/D3. Mol. Pharm. 7, 1057–1068. doi: 10.1021/mp900178j
Carmine, A. A., and Brogden, R. N. (1985). Pirenzepine. A review of its pharmacodynamic and pharmacokinetic properties and therapeutic efficacy in peptic ulcer disease and other allied diseases. Drugs 30, 85–126. doi: 10.2165/00003495-198530020-00001
Carrano, A., Snkhchyan, H., Kooij, G., van der Pol, S., van Horssen, J., Veerhuis, R., et al. (2014). ATP-binding cassette transporters P-glycoprotein and breast cancer related protein are reduced in capillary cerebral amyloid angiopathy. Neurobiol. Aging 35, 565–575. doi: 10.1016/j.neurobiolaging.2013.09.015
Cascorbi, I., Flüh, C., Remmler, C., Haenisch, S., Faltraco, F., Grumbt, M., et al. (2013). Association of ATP-binding cassette transporter variants with the risk of Alzheimer’s disease. Pharmacogenomics 14, 485–494. doi: 10.2217/pgs.13.18
Chan, S. L., Kim, W. S., Kwok, J. B., Hill, A. F., Cappai, R., Rye, K. A., et al. (2008). ATP-binding cassette transporter A7 regulates processing of amyloid precursor protein in vitro. J. Neurochem. 106, 793–804. doi: 10.1111/j.1471-4159.2008.05433.x
Chang, Y. C., Chan, M. H., Li, C. H., Fang, C. Y., Hsiao, M., and Chen, C. L. (2021). Exosomal components and modulators in colorectal cancer: Novel diagnosis and prognosis biomarkers. Biomedicines 9:931. doi: 10.3390/biomedicines9080931
Chavez, A. R., Buchser, W., Basse, P. H., Liang, X., Appleman, L. J., Maranchie, J. K., et al. (2009). Pharmacologic administration of interleukin-2. Ann. N. Y. Acad. Sci. 1182, 14–27. doi: 10.1111/j.1749-6632.2009.05160.x
Chawla, A., Boisvert, W. A., Lee, C. H., Laffitte, B. A., Barak, Y., Joseph, S. B., et al. (2001). A PPAR gamma-LXR-ABCA1 pathway in macrophages is involved in cholesterol efflux and atherogenesis. Mol. Cell. 7, 161–171. doi: 10.1016/s1097-2765(01)00164-2
Cheang, W. S., Tian, X. Y., Wong, W. T., and Huang, Y. (2015). The peroxisome proliferator-activated receptors in cardiovascular diseases: Experimental benefits and clinical challenges. Br. J. Pharmacol. 172, 5512–5522. doi: 10.1111/bph.13029
Chen, C. J., Chin, J. E., Ueda, K., Clark, D. P., Pastan, I., Gottesman, M. M., et al. (1986). Internal duplication and homology with bacterial transport proteins in the mdr1 (P-glycoprotein) gene from multidrug-resistant human cells. Cell 47, 381–389. doi: 10.1016/0092-8674(86)90595-7
Chen, H. H., Petty, L. E., Sha, J., Zhao, Y., Kuzma, A., Valladares, O., et al. (2021). Genetically regulated expression in late-onset Alzheimer’s disease implicates risk genes within known and novel loci. Transl. Psychiatry 11:618. doi: 10.1038/s41398-021-01677-0
Chen, J., Costa, L. G., and Guizzetti, M. (2011). Retinoic acid isomers up-regulate ATP binding cassette A1 and G1 and cholesterol efflux in rat astrocytes: Implications for their therapeutic and teratogenic effects. J. Pharmacol. Exp. Ther. 338, 870–878. doi: 10.1124/jpet.111.182196
Chinetti, G., Lestavel, S., Bocher, V., Remaley, A. T., Neve, B., Torra, I. P., et al. (2001). PPAR-alpha and PPAR-gamma activators induce cholesterol removal from human macrophage foam cells through stimulation of the ABCA1 pathway. Nat. Med. 7, 53–58. doi: 10.1038/83348
Chiu, C., Miller, M. C., Monahan, R., Osgood, D. P., Stopa, E. G., and Silverberg, G. D. (2015). P-glycoprotein expression and amyloid accumulation in human aging and Alzheimer’s disease: Preliminary observations. Neurobiol. Aging 36, 2475–2482. doi: 10.1016/j.neurobiolaging.2015.05.020
Cho, S., Lu, M., He, X., Ee, P. L., Bhat, U., Schneider, E., et al. (2011). Notch1 regulates the expression of the multidrug resistance gene ABCC1/MRP1 in cultured cancer cells. Proc. Natl. Acad. Sci. U.S.A. 108, 20778–20783. doi: 10.1073/pnas.1019452108
Cocks, G., Wilde, J. I., Graham, S. J., Bousgouni, V., Virley, D., Lovestone, S., et al. (2010). The thiazolidinedione pioglitazone increases cholesterol biosynthetic gene expression in primary cortical neurons by a PPARgamma-independent mechanism. J. Alzheimers Dis. 19, 631–646. doi: 10.3233/JAD-2010-1266
Cooray, H. C., Blackmore, C. G., Maskell, L., and Barrand, M. A. (2002). Localisation of breast cancer resistance protein in microvessel endothelium of human brain. Neuroreport 13, 2059–2063. doi: 10.1097/00001756-200211150-00014
Costet, P., Lalanne, F., Gerbod-Giannone, M. C., Molina, J. R., Fu, X., Lund, E. G., et al. (2003). Retinoic acid receptor-mediated induction of ABCA1 in macrophages. Mol. Cell. Biol. 23, 7756–7766. doi: 10.1128/MCB.23.21.7756-7766.2003
Cozzolino, M., and Malindretos, P. (2010). The role of vitamin D receptor activation in chronic kidney disease. Hippokratia 14, 7–9.
Cramer, P. E., Cirrito, J. R., Wesson, D. W., Lee, C. Y., Karlo, J. C., Zinn, A. E., et al. (2012). ApoE-directed therapeutics rapidly clear β-amyloid and reverse deficits in AD mouse models. Science 335, 1503–1506. doi: 10.1126/science.1217697
Crossgrove, J. S., Li, G. J., and Zheng, W. (2005). The choroid plexus removes beta-amyloid from brain cerebrospinal fluid. Exp. Biol. Med. 230, 771–776. doi: 10.1177/153537020523001011
Cummings, J. L., Zhong, K., Kinney, J. W., Heaney, C., Moll-Tudla, J., Joshi, A., et al. (2016). Double-blind, placebo-controlled, proof-of-concept trial of bexarotene Xin moderate Alzheimer’s disease. Alzheimers Res. Ther. 8:4. doi: 10.1186/s13195-016-0173-2
Dafnis, I., Raftopoulou, C., Mountaki, C., Megalou, E., Zannis, V. I., and Chroni, A. (2018). ApoE isoforms and carboxyl-terminal-truncated apoE4 forms affect neuronal BACE1 levels and Aβ production independently of their cholesterol efflux capacity. Biochem. J. 475, 1839–1859. doi: 10.1042/BCJ20180068
Dallas, S., Miller, D. S., and Bendayan, R. (2006). Multidrug resistance-associated proteins: Expression and function in the central nervous system. Pharmacol. Rev. 58, 140–161. doi: 10.1124/pr.58.2.3
Dallas, S., Schlichter, L., and Bendayan, R. (2004). Multidrug resistance protein (MRP) 4- and MRP 5-mediated efflux of 9-(2-phosphonylmethoxyethyl)adenine by microglia. J. Pharmacol. Exp. Ther. 309, 1221–1229. doi: 10.1124/jpet.103.063966
de Lima, V. A., and Sørensen, J. B. (2015). Third-line chemotherapy with carboplatin, gemcitabine and liposomised doxorubicin for malignant pleural mesothelioma. Med. Oncol. 32:458. doi: 10.1007/s12032-014-0458-x
de Urquiza, A. M., Liu, S., Sjöberg, M., Zetterström, R. H., Griffiths, W., Sjövall, J., et al. (2000). Docosahexaenoic acid, a ligand for the retinoid X receptor in mouse brain. Science 290, 2140–2144. doi: 10.1126/science.290.5499.2140
Deo, A. K., Borson, S., Link, J. M., Domino, K., Eary, J. F., Ke, B., et al. (2014). Activity of P-glycoprotein, a β-amyloid transporter at the blood-brain barrier. Is compromised in patients with mild Alzheimer disease. J. Nucl. Med. 55, 1106–1111. doi: 10.2967/jnumed.113.130161
Deshpande, D., Alffenaar, J. C., Köser, C. U., Dheda, K., Chapagain, M. L., Simbar, N., et al. (2018). d-cycloserine pharmacokinetics/pharmacodynamics, susceptibility, and dosing implications in multidrug-resistant tuberculosis: A faustian deal. Clin. Infect. Dis. 67, S308–S316. doi: 10.1093/cid/ciy624
Dib, S., Pahnke, J., and Gosselet, F. (2021). Role of ABCA7 in human health and in Alzheimer’s disease. Int. J. Mol. Sci. 22:4603.
Dietschy, J. M., and Turley, S. D. (2004). Thematic review series: Brain Lipids. Cholesterol metabolism in the central nervous system during early development and in the mature animal. J. Lipid Res. 45, 1375–1397. doi: 10.1194/jlr.R400004-JLR200
Ding, Y., Zhong, Y., Baldeshwiler, A., Abner, E. L., Bauer, B., and Hartz, A. M. S. (2021). Protecting P-glycoprotein at the blood-brain barrier from degradation in an Alzheimer’s disease mouse model. Fluids Barriers CNS 18:10. doi: 10.1186/s12987-021-00245-4
Do, T. M., Dodacki, A., Alata, W., Calon, F., Nicolic, S., Scherrmann, J. M., et al. (2016). Age-dependent regulation of the blood-brain barrier influx/efflux equilibrium of amyloid-β peptide in a mouse model of Alzheimer’s disease (3xTg-AD). J. Alzheimers Dis. 49, 287–300. doi: 10.3233/JAD-150350
Do, T. M., Noel-Hudson, M. S., Ribes, S., Besengez, C., Smirnova, M., Cisternino, S., et al. (2012). ABCG2- and ABCG4-mediated efflux of amyloid-β peptide 1-40 at the mouse blood-brain barrier. J. Alzheimers Dis. 30, 155–166. doi: 10.3233/JAD-2012-112189
Do, T. M., Ouellet, M., Calon, F., Chimini, G., Chacun, H., Farinotti, R., et al. (2011). Direct evidence of abca1-mediated efflux of cholesterol at the mouse blood-brain barrier. Mol. Cell. Biochem. 357, 397–404. doi: 10.1007/s11010-011-0910-6
Dodacki, A., Wortman, M., Saubaméa, B., Chasseigneaux, S., Nicolic, S., Prince, N., et al. (2017). Expression and function of Abcg4 in the mouse blood-brain barrier: Role in restricting the brain entry of amyloid-β peptide. Sci. Rep. 7:13393. doi: 10.1038/s41598-017-13750-0
Donkin, J. J., Stukas, S., Hirsch-Reinshagen, V., Namjoshi, D., Wilkinson, A., May, S., et al. (2010). ATP-binding cassette transporter A1 mediates the beneficial effects of the liver X receptor agonist GW3965 on object recognition memory and amyloid burden in amyloid precursor protein/presenilin 1 mice. J. Biol. Chem. 285, 34144–34154. doi: 10.1074/jbc.M110.108100
Donnelly, R. P., Young, H. A., and Rosenberg, A. S. (2009). An overview of cytokines and cytokine antagonists as therapeutic agents. Ann. N. Y. Acad. Sci. 1182, 1–13. doi: 10.1111/j.1749-6632.2009.05382.x
Doody, R. S., Thomas, R. G., Farlow, M., Iwatsubo, T., Vellas, B., Joffe, S., et al. (2014). Phase 3 trials of solanezumab for mild-to-moderate Alzheimer’s disease. N. Engl. J. Med. 370, 311–321. doi: 10.1056/NEJMoa1312889
Doyle, L. A., Yang, W., Abruzzo, L. V., Krogmann, T., Gao, Y., Rishi, A. K., et al. (1998). A multidrug resistance transporter from human MCF-7 breast cancer cells. Proc. Natl. Acad. Sci. U.S.A. 95, 15665–15670. doi: 10.1073/pnas.95.26.15665
Durk, M. R., Chan, G. N., Campos, C. R., Peart, J. C., Chow, E. C., Lee, E., et al. (2012). 1α,25-Dihydroxyvitamin D3-liganded vitamin D receptor increases expression and transport activity of P-glycoprotein in isolated rat brain capillaries and human and rat brain microvessel endothelial cells. J. Neurochem. 123, 944–953. doi: 10.1111/jnc.12041
Dutheil, F., Dauchy, S., Diry, M., Sazdovitch, V., Cloarec, O., Mellottée, L., et al. (2009). Xenobiotic-metabolizing enzymes and transporters in the normal human brain: Regional and cellular mapping as a basis for putative roles in cerebral function. Drug Metab. Dispos. 37, 1528–1538. doi: 10.1124/dmd.109.027011
Efthymiou, A. G., and Goate, A. M. (2017). Late onset Alzheimer’s disease genetics implicates microglial pathways in disease risk. Mol. Neurodegener. 12:43. doi: 10.1186/s13024-017-0184-x
ElAli, A., and Hermann, D. M. (2011). ATP-binding cassette transporters and their roles in protecting the brain. Neuroscientist 17, 423–436.
ElAli, A., and Rivest, S. (2013). The role of ABCB1 and ABCA1 in beta-amyloid clearance at the neurovascular unit in Alzheimer’s disease. Front. Physiol. 4:45. doi: 10.3389/fphys.2013.00045
Engel, T., Lorkowski, S., Lueken, A., Rust, S., Schlüter, B., Berger, G., et al. (2001). The human ABCG4 gene is regulated by oxysterols and retinoids in monocyte-derived macrophages. Biochem. Biophys. Res. Commun. 288, 483–488. doi: 10.1006/bbrc.2001.5756
Erdő, F., and Krajcsi, P. (2019). Age-related functional and expressional changes in efflux pathways at the blood-brain barrier. Front. Aging Neurosci. 11:196. doi: 10.3389/fnagi.2019.00196
Erickson, M. A., Hartvigson, P. E., Morofuji, Y., Owen, J. B., Butterfield, D. A., and Banks, W. A. (2012). Lipopolysaccharide impairs amyloid β efflux from brain: Altered vascular sequestration, cerebrospinal fluid reabsorption, peripheral clearance and transporter function at the blood-brain barrier. J. Neuroinflamm. 9:150. doi: 10.1186/1742-2094-9-150
Fedotcheva, T. A. (2021). Clinical use of progestins and their mechanisms of action: Present and future (review). Sovrem. Tekhnologii Med. 13, 93–106. doi: 10.17691/stm2021.13.1.11
Fehér, Á, Juhász, A., László, A., Pákáski, M., Kálmán, J., and Janka, Z. (2013). Association between the ABCG2 C421A polymorphism and Alzheimer’s disease. Neurosci. Lett. 550, 51–54. doi: 10.1016/j.neulet.2013.06.044
Fitz, N. F., Cronican, A., Pham, T., Fogg, A., Fauq, A. H., Chapman, R., et al. (2010). Liver X receptor agonist treatment ameliorates amyloid pathology and memory deficits caused by high-fat diet in APP23 mice. J. Neurosci. 30, 6862–6872. doi: 10.1523/JNEUROSCI.1051-10.2010
Fitzpatrick, L. A., and Good, A. (1999). Micronized progesterone: Clinical indications and comparison with current treatments. Fertil. Steril. 72, 389–397. doi: 10.1016/s0015-0282(99)00272-1
Fortier, M., Castellano, C. A., St-Pierre, V., Myette-Côté, E., Langlois, F., and Royet, M. (2020). A ketogenic drink improves cognition in mild cognitive impairment: Results of a 6-month RCT. Alzheimers Dement. 17, 543–552. doi: 10.1002/alz.12206
Fu, Y., Hsiao, J. H., Paxinos, G., Halliday, G. M., and Kim, W. S. (2016). ABCA7 mediates phagocytic clearance of amyloid-β in the brain. J. Alzheimers Dis. 54, 569–584. doi: 10.3233/JAD-160456
Fujiyoshi, M., Ohtsuki, S., Hori, S., Tachikawa, M., and Terasaki, T. (2007). 24S-hydroxycholesterol induces cholesterol release from choroid plexus epithelial cells in an apical- and apoE isoform-dependent manner concomitantly with the induction of ABCA1 and ABCG1 expression. J. Neurochem. 100, 968–978. doi: 10.1111/j.1471-4159.2006.04240.x
Fukuda, Y., and Schuetz, J. D. (2012). ABC transporters and their role in nucleoside and nucleotide drug resistance. Biochem. Pharmacol. 83, 1073–1083. doi: 10.1016/j.bcp.2011.12.042
Fukumoto, H., Deng, A., Irizarry, M. C., Fitzgerald, M. L., and Rebeck, G. W. (2002). Induction of the cholesterol transporter ABCA1 in central nervous system cells by liver X receptor agonists increases secreted Abeta levels. J. Biol. Chem. 277, 48508–48513. doi: 10.1074/jbc.M209085200
Fulco, C. S., Rock, P. B., and Cymerman, A. (2000). Improving athletic performance: Is altitude residence or altitude training helpful? Aviat. Space Environ. Med. 71, 162–171.
Gaunt, C. M., Rainbow, D. B., Mackenzie, R. J., Jarvis, L. B., Mousa, H. S., Cunniffe, N., et al. (2021). The MS remyelinating drug bexarotene (an RXR Agonist) promotes induction of human tregs and suppresses Th17 differentiation in vitro. Front. Immunol. 12:712241. doi: 10.3389/fimmu.2021.712241
Gelissen, I. C., Harris, M., Rye, K. A., Quinn, C., Brown, A. J., Kockx, M., et al. (2006). ABCA1 and ABCG1 synergize to mediate cholesterol export to apoA-I. Arterioscler. Thromb. Vasc. Biol. 26, 534–540. doi: 10.1161/01.ATV.0000200082.58536.e1
Gerst, D. G. III, Yokhana, S. S., Carney, L. M., Lee, D. S., Badr, M. S., Qureshi, T., et al. (2001). The hypoxic ventilatory response and ventilatory long-term facilitation are altered by time of day and repeated daily exposure to intermittent hypoxia. J. Appl. Physiol. 110, 15–28. doi: 10.1152/japplphysiol.00524.2010
Gilman, S., Koller, M., Black, R. S., Jenkins, L., Griffith, S. G., Fox, N. C., et al. (2005). Clinical effects of abeta immunization (AN1792) in patients with AD in an interrupted trial. Neurology 64, 1553–1562. doi: 10.1212/01.WNL.0000159740.16984.3C
Göritz, C., Thiebaut, R., Tessier, L. H., Nieweg, K., Moehle, C., Buard, I., et al. (2007). Glia-induced neuronal differentiation by transcriptional regulation. Glia 55, 1108–1122. doi: 10.1002/glia.20531
Gosselet, F., Candela, P., Sevin, E., Berezowski, V., Cecchelli, R., and Fenart, L. (2009). Transcriptional profiles of receptors and transporters involved in brain cholesterol homeostasis at the blood-brain barrier: Use of an in vitro model. Brain Res. 1249, 34–42. doi: 10.1016/j.brainres.2008.10.036
Graham, A. (2015). Mitochondrial regulation of macrophage cholesterol homeostasis. Free Radic. Biol. Med. 89, 982–992. doi: 10.1016/j.freeradbiomed.2015.08.010
Green, K. N., Billings, L. M., Roozendaal, B., McGaugh, J. L., and LaFerla, F. M. (2006). Glucocorticoids increase amyloid-beta and tau pathology in a mouse model of Alzheimer’s disease. J. Neurosci. 26, 9047–9056. doi: 10.1523/JNEUROSCI.2797-06.2006
Green, R. C., Schneider, L. S., Amato, D. A., Beelen, A. P., Wilcock, G., Swabb, E. A., et al. (2009). Effect of tarenflurbil on cognitive decline and activities of daily living in patients with mild Alzheimer disease: A randomized controlled trial. JAMA 302, 2557–2564. doi: 10.1001/jama.2009.1866
Guglietta, A., and Sullivan, P. B. (1995). Clinical applications of epidermal growth factor. Eur. J. Gastroenterol. Hepatol. 7, 945–950. doi: 10.1097/00042737-199510000-00007
Hardy, J. A., and Higgins, G. A. (1992). Alzheimer’s disease: The amyloid cascade hypothesis. Science 256, 184–185. doi: 10.1126/science.1566067
Hardy, J., and Allsop, D. (1991). Amyloid deposition as the central event in the aetiology of Alzheimer’s disease. Trends Pharmacol. Sci. 12, 383–388. doi: 10.1016/0165-6147(91)90609-v
Harold, D., Abraham, R., Hollingworth, P., Sims, R., Gerrish, A., Hamshere, M. L., et al. (2009). Genome-wide association study identifies variants at CLU and PICALM associated with Alzheimer’s disease. Nat. Genet. 41, 1088–1093. doi: 10.1038/ng.440
Hartz, A. M. S., Zhong, Y., Shen, A. N., Abner, E. L., and Bauer, B. (2018). Preventing P-gp ubiquitination lowers aβ brain levels in an Alzheimer’s disease mouse model. Front. Aging Neurosci. 10:186. doi: 10.3389/fnagi.2018.00186
Hartz, A. M., Bauer, B., Fricker, G., and Miller, D. S. (2006). Rapid modulation of P-glycoprotein-mediated transport at the blood–brain barrier by tumor necrosis factor-alpha and lipopolysaccharide. Mol. Pharmacol. 69, 462–470. doi: 10.1124/mol.105.017954
Hartz, A. M., Miller, D. S., and Bauer, B. (2010). Restoring blood–brain barrier P-glycoprotein reduces brain amyloid-beta in a mouse model of Alzheimer’s disease. Mol. Pharmacol. 77, 715–723. doi: 10.1124/mol.109.061754
Hartz, A. M., Zhong, Y., Wolf, A., LeVine, H. III, Miller, D. S., and Bauer, B. (2016). Aβ40 reduces P-glycoprotein at the blood-brain barrier through the ubiquitin-proteasome pathway. J. Neurosci. 36, 1930–1941. doi: 10.1523/JNEUROSCI.0350-15.2016
Hayashi, H., Campenot, R. B., Vance, D. E., and Vance, J. E. (2004). Glial lipoproteins stimulate axon growth of central nervous system neurons in compartmented cultures. J. Biol. Chem. 279, 14009–14015. doi: 10.1074/jbc.M313828200
Helal, O., Berrougui, H., Loued, S., and Khalil, A. (2013). Extra-virgin olive oil consumption improves the capacity of HDL to mediate cholesterol efflux and increases ABCA1 and ABCG1 expression in human macrophages. Br. J. Nutr. 109, 1844–1855. doi: 10.1017/S0007114512003856
Henderson, S. T., Morimoto, B. H., Cummings, J. L., Farlow, M. R., and Walker, J. (2020). A placebo-controlled, parallel-group, randomized clinical trial of AC-1204 in mild-to-moderate Alzheimer’s disease. J. Alzheimers Dis. 75, 547–557. doi: 10.3233/JAD-191302
Hermann, D. M., and ElAli, A. (2012). The abluminal endothelial membrane in neurovascular remodeling in health and disease. Sci. Signal. 5:re41.
Heyman, R. A., Mangelsdorf, D. J., Dyck, J. A., Stein, R. B., Eichele, G., Evans, R. M., et al. (1992). 9-cis retinoic acid is a high affinity ligand for the retinoid X receptor. Cell 68, 397–406. doi: 10.1016/0092-8674(92)90479-v
Hirrlinger, J., König, J., and Dringen, R. (2002). Expression of mRNAs of multidrug resistance proteins (Mrps) in cultured rat astrocytes, oligodendrocytes, microglial cells and neurones. J. Neurochem. 82, 716–719. doi: 10.1046/j.1471-4159.2002.01082.x
Hirsch-Reinshagen, V., Zhou, S., Burgess, B. L., Bernier, L., McIsaac, S. A., Chan, J. Y., et al. (2004). Deficiency of ABCA1 impairs apolipoprotein E metabolism in brain. J. Biol. Chem. 279, 41197–41207. doi: 10.1074/jbc.M407962200
Ho, Y. S., Torres-Vergara, P., and Penny, J. (2023). Regulation of the ATP-binding cassette transporters ABCB1, ABCG2 and ABCC5 by nuclear receptors in porcine blood-brain barrier endothelial cells. Br. J. Pharmacol. 180, 3092–3109. doi: 10.1111/bph.16196
Hoensch, H. P., Balzer, K., Dylewizc, P., Kirch, W., Goebell, H., and Ohnhaus, E. E. (1985). Effect of rifampicin treatment on hepatic drug metabolism and serum bile acids in patients with primary biliary cirrhosis. Eur. J. Clin. Pharmacol. 28, 475–477. doi: 10.1007/BF00544371
Hofrichter, J., Krohn, M., Schumacher, T., Lange, C., Feistel, B., Walbroel, B., et al. (2013). Reduced Alzheimer’s disease pathology by St. John’s Wort treatment is independent of hyperforin and facilitated by ABCC1 and microglia activation in mice. Curr. Alzheimer Res. 10, 1057–1069. doi: 10.2174/15672050113106660171
Holmes, C., and Butchart, J. (2011). Systemic inflammation and Alzheimer’s disease. Biochem. Soc. Trans. 39, 898–901. doi: 10.1042/BST0390898
Hong, C., and Tontonoz, P. (2014). Liver X receptors in lipid metabolism: Opportunities for drug discovery. Nat. Rev. Drug Discov. 13, 433–444. doi: 10.1038/nrd4280
Hori, S., Ohtsuki, S., Tachikawa, M., Kimura, N., Kondo, T., Watanabe, M., et al. (2004). Functional expression of rat ABCG2 on the luminal side of brain capillaries and its enhancement by astrocyte-derived soluble factor(s). J. Neurochem. 90, 526–536. doi: 10.1111/j.1471-4159.2004.02537.x
Hou, Y., Zhu, Q., Li, Z., Peng, Y., Yu, X., Yuan, B., et al. (2017). The FOXM1-ABCC5 axis contributes to paclitaxel resistance in nasopharyngeal carcinoma cells. Cell Death Dis. 8:e26591.
Hu, W., Zhang, Y., Wu, W., Yin, Y., Huang, D., Wang, Y., et al. (2016). Chronic glucocorticoids exposure enhances neurodegeneration in the frontal cortex and hippocampus via NLRP-1 inflammasome activation in male mice. Brain Behav. Immun. 52, 58–70. doi: 10.1016/j.bbi.2015.09.019
Iliff, J. J., Wang, M., Liao, Y., Plogg, B. A., Peng, W., Gundersen, G. A., et al. (2012). A paravascular pathway facilitates CSF flow through the brain parenchyma and the clearance of interstitial solutes, including amyloid β. Sci. Transl. Med. 4, 1–11. doi: 10.1126/scitranslmed.3003748
Imbimbo, B. P., Panza, F., Frisardi, V., Solfrizzi, V., D’Onofrio, G., Logroscino, G., et al. (2011). Therapeutic intervention for Alzheimer’s disease with γ-secretase inhibitors: Still a viable option? Expert Opin. Investig. Drugs 20, 325–341. doi: 10.1517/13543784.2011.550572
Ishimori, A., and Yamagata, S. (1982). Therapeutic effect of pirenzepine dihydrochloride on gastric ulcer evaluated by a double-blind controlled clinical study. Phase III study. Arzneimittelforschung 32, 556–565.
Ivanisova, D., Bohac, M., Culenova, M., Smolinska, V., and Danisovic, L. (2023). Mesenchymal-stromal-cell-conditioned media and their implication for osteochondral regeneration. Int. J. Mol. Sci. 24:9054. doi: 10.3390/ijms24109054
Iwamoto, N., Abe-Dohmae, S., Sato, R., and Yokoyama, S. (2006). ABCA7 expression is regulated by cellular cholesterol through the SREBP2 pathway and associated with phagocytosis. J. Lipid Res. 47, 1915–1927. doi: 10.1194/jlr.M600127-JLR200
Jansen, R. S., Mahakena, S., de Haas, M., Borst, P., and van de Wetering, K. (2015). ATP-binding cassette subfamily C member 5 (ABCC5) functions as an efflux transporter of glutamate conjugates and analogs. J. Biol. Chem. 290, 30429–30440. doi: 10.1074/jbc.M115.692103
Jasmin, S. B., Pearson, V., Lalonde, D., Domenger, D., Théroux, L., and Poirier, J. (2014). Differential regulation of ABCA1 and ABCG1 gene expressions in the remodeling mouse hippocampus after entorhinal cortex lesion and liver-X receptor agonist treatment. Brain Res. 1562, 39–51. doi: 10.1016/j.brainres.2014.03.016
Jepsen, W. M., De Both, M., Siniard, A. L., Ramsey, K., Piras, I. S., Naymik, M., et al. (2021). Adenosine triphosphate binding cassette subfamily C member 1 (ABCC1) overexpression reduces APP processing and increases alpha- versus beta-secretase activity, in vitro. Biol Open 10:bio0546271.
Jha, N. K., Kar, R., and Niranjan, R. (2019). ABC transporters in neurological disorders: An important gateway for botanical compounds mediated neuro-therapeutics. Curr. Top. Med. Chem. 19, 795–811. doi: 10.2174/1568026619666190412121811
Jiang, Y. J., Lu, B., Kim, P., Elias, P. M., and Feingold, K. R. (2006). Regulation of ABCA1 expression in human keratinocytes and murine epidermis. J. Lipid Res. 47, 2248–2258. doi: 10.1194/jlr.M600163-JLR200
Jiang, Y. J., Lu, B., Tarling, E. J., Kim, P., Man, M. Q., Crumrine, D., et al. (2010). Regulation of ABCG1 expression in human keratinocytes and murine epidermis. J. Lipid Res. 51, 3185–3195. doi: 10.1194/jlr.M006445
Johnson, D. B., Lopez, M. J., and Kelley, B. (2023). Dexamethasone: StatPearls. Treasure Island, FL: StatPearls Publishing.
Johnson-Arbor, K., and Dubey, R. (2023). Doxorubicin: StatPearls. Treasure Island, FL: StatPearls Publishing.
Joshi, Y. B., Chu, J., and Praticò, D. (2012). Stress hormone leads to memory deficits and altered tau phosphorylation in a model of Alzheimer’s disease. J. Alzheimers Dis. 31, 167–176. doi: 10.3233/JAD-2012-120328
Joshi, Y. B., Chu, J., and Praticò, D. (2013). Knockout of 5-lipoxygenase prevents dexamethasone-induced tau pathology in 3xTg mice. Aging Cell 12, 706–711. doi: 10.1111/acel.12096
Jung, C. G., Horike, H., Cha, B. Y., Uhm, K. O., Yamauchi, R., Yamaguchi, T., et al. (2010). Honokiol increases ABCA1 expression level by activating retinoid X receptor beta. Biol. Pharm. Bull. 33, 1105–1111. doi: 10.1248/bpb.33.1105
Kaddoumi, A., Denney, T. S. Jr., Deshpande, G., Robinson, J. L., Beyers, R. J., Redden, D. T., et al. (2022). Extra-virgin olive oil enhances the blood-brain barrier function in mild cognitive impairment: A randomized controlled trial. Nutrients 14:5102. doi: 10.3390/nu14235102
Kalaany, N. Y., and Mangelsdorf, D. J. (2006). LXRS and FXR: The yin and yang of cholesterol and fat metabolism. Annu. Rev. Physiol. 68, 159–191. doi: 10.1146/annurev.physiol.68.033104.152158
Kaminski, W. E., Orsó, E., Diederich, W., Klucken, J., Drobnik, W., and Schmitz, G. (2000). Identification of a novel human sterol-sensitive ATP-binding cassette transporter (ABCA7). Biochem. Biophys. Res. Commun. 273, 532–538. doi: 10.1006/bbrc.2000.2954
Kania, K. D., Wijesuriya, H. C., Hladky, S. B., and Barrand, M. A. (2011). Beta amyloid effects on expression of multidrug efflux transporters in brain endothelial cells. Brain Res. 1418, 1–11. doi: 10.1016/j.brainres.2011.08.044
Kannan, P., Schain, M., Kretzschmar, W. W., Weidner, L., Mitsios, N., Gulyás, B., et al. (2017). An automated method measures variability in P-glycoprotein and ABCG2 densities across brain regions and brain matter. J. Cereb. Blood Flow Metab. 37, 2062–2075. doi: 10.1177/0271678X16660984
Keck, S., Nitsch, R., Grune, T., and Ullrich, O. (2003). Proteasome inhibition by paired helical filament-tau in brains of patients with Alzheimer’s disease. J. Neurochem. 85, 115–122. doi: 10.1046/j.1471-4159.2003.01642.x
Khattab, M., and Al-Karmalawy, A. A. (2021). Revisiting activity of some nocodazole analogues as a potential anticancer drugs using molecular docking and DFT calculations. Front. Chem. 9:628398. doi: 10.3389/fchem.2021.628398
Kim, W. S., Chan, S. L., Hill, A. F., Guillemin, G. J., and Garner, B. (2009). Impact of 27-hydroxycholesterol on amyloid-beta peptide production and ATP-binding cassette transporter expression in primary human neurons. J. Alzheimers Dis. 16, 121–131. doi: 10.3233/JAD-2009-0944
Kim, W. S., Guillemin, G. J., Glaros, E. N., Lim, C. K., and Garner, B. (2006). Quantitation of ATP-binding cassette subfamily-A transporter gene expression in primary human brain cells. Neuroreport 17, 891–896. doi: 10.1097/01.wnr.0000221833.41340.cd
Kim, W. S., Li, H., Ruberu, K., Chan, S., Elliott, D. A., Low, J. K., et al. (2013). Deletion of Abca7 increases cerebral amyloid-β accumulation in the J20 mouse model of Alzheimer’s disease. J. Neurosci. 33, 4387–4394. doi: 10.1523/JNEUROSCI.4165-12.2013
Kim, W. S., Rahmanto, A. S., Kamili, A., Rye, K. A., Guillemin, G. J., Gelissen, I. C., et al. (2007). Role of ABCG1 and ABCA1 in regulation of neuronal cholesterol efflux to apolipoprotein E discs and suppression of amyloid-beta peptide generation. J. Biol. Chem. 282, 2851–2861. doi: 10.1074/jbc.M607831200
Kim, W. S., Weickert, C. S., and Garner, B. (2008). Role of ATP-binding cassette transporters in brain lipid transport and neurological disease. J. Neurochem. 104, 1145–1166. doi: 10.1111/j.1471-4159.2007.05099.x
Kinney, J. W., Bemiller, S. M., Murtishaw, A. S., Leisgang, A. M., Salazar, A. M., and Lamb, B. T. (2018). Inflammation as a central mechanism in Alzheimer’s disease. Alzheimers Dement. 4, 575–590. doi: 10.1016/j.trci.2018.06.014
Kiraly, M., Foss, J. F., and Giordano, T. (2023). Neuroinflammation, its role in Alzheimer’s disease and therapeutic strategie. J. Prev. Alzheimers Dis. 10, 686–698. doi: 10.14283/jpad.2023.109
Kober, A. C., Manavalan, A. P. C., Tam-Amersdorfer, C., Holmér, A., Saeed, A., Fanaee-Danesh, E., et al. (2017). Implications of cerebrovascular ATP-binding cassette transporter G1 (ABCG1) and apolipoprotein M in cholesterol transport at the blood-brain barrier. Biochim. Biophys. Acta Mol. Cell. Biol. Lipids 1862, 573–588. doi: 10.1016/j.bbalip.2017.03.003
Koldamova, R. P., Lefterov, I. M., Ikonomovic, M. D., Skoko, J., Lefterov, P. I., Isanski, B. A., et al. (2003). 22R-hydroxycholesterol and 9-cis-retinoic acid induce ATP-binding cassette transporter A1 expression and cholesterol efflux in brain cells and decrease amyloid beta secretion. J. Biol. Chem. 278, 13244–13256. doi: 10.1074/jbc.M300044200
Koldamova, R., Fitz, N. F., and Lefterov, I. (2010). The role of ATP-binding cassette transporter A1 in Alzheimer’s disease and neurodegeneration. Biochim. Biophys. Acta 1801, 824–830. doi: 10.1016/j.bbalip.2010.02.010
Korkushko, O. V., Shatilo, V. B., and Ishchuk, V. A. (2010). Effectiveness of intermittent normabaric hypoxic trainings in elderly patients with coronary artery disease. Adv. Gerontol. 23, 476–482.
Krishnamurthy, P., Ross, D. D., Nakanishi, T., Bailey-Dell, K., Zhou, S., Mercer, K. E., et al. (2004). The stem cell marker Bcrp/ABCG2 enhances hypoxic cell survival through interactions with heme. J. Biol. Chem. 279, 24218–24225. doi: 10.1074/jbc.M313599200
Krohn, M., Bracke, A., Avchalumov, Y., Schumacher, T., Hofrichter, J., Paarmann, K., et al. (2015). Accumulation of murine amyloid-β mimics early Alzheimer’s disease. Brain 138, 2370–2382. doi: 10.1093/brain/awv137
Krohn, M., Lange, C., Hofrichter, J., Scheffler, K., Stenzel, J., Steffen, J., et al. (2011). Cerebral amyloid-β proteostasis is regulated by the membrane transport protein ABCC1 in mice. J. Clin. Invest. 121, 3924–3931. doi: 10.1172/JCI57867
Kugathasan, R., Wootton, M., and Howe, R. (2014). Cycloserine as an alternative urinary tract infection therapy: Susceptibilities of 500 urinary pathogens to standard and alternative therapy antimicrobials. Eur. J. Clin. Microbiol. Infect. Dis. 33, 1169–1172. doi: 10.1007/s10096-014-2051-9
Lakhan, S. E., Caro, M., and Hadzimichalis, N. (2013). NMDA receptor activity in neuropsychiatric disorders. Front. Psychiatry 4:52. doi: 10.3389/fpsyt.2013.00052
Lamartinière, Y., Boucau, M. C., Dehouck, L., Krohn, M., Pahnke, J., Candela, P., et al. (2018). ABCA7 downregulation modifies cellular cholesterol homeostasis and decreases amyloid-β peptide efflux in an in vitro model of the blood-brain barrier. J. Alzheimers Dis. 64, 1195–1211. doi: 10.3233/JAD-170883
Langmann, T., Mauerer, R., Zahn, A., Moehle, C., Probst, M., Stremmel, W., et al. (2003). Real-time reverse transcription-PCR expression profiling of the complete human ATP-binding cassette transporter superfamily in various tissues. Clin. Chem. 49, 230–238. doi: 10.1373/49.2.230
Lefterov, I., Fitz, N. F., Cronican, A., Lefterov, P., Staufenbiel, M., and Koldamova, R. (2009). Memory deficits in APP23/Abca1+/- mice correlate with the level of Aβ oligomers. ASN Neuro 1:e00006. doi: 10.1042/AN20090015
Lemmen, J., Tozakidis, I. E., and Galla, H. J. (2013). Pregnane X receptor upregulates ABC-transporter Abcg2 and Abcb1 at the blood-brain barrier. Brain Res. 1491, 1–13. doi: 10.1016/j.brainres.2012.10.060
Leslie, E. M., Deeley, R. G., and Cole, S. P. (2005). Multidrug resistance proteins: Role of P-glycoprotein, MRP1, MRP2, and BCRP (ABCG2) in tissue defense. Toxicol. Appl. Pharmacol. 204, 216–237. doi: 10.1016/j.taap.2004.10.012
Levy, E., Prelli, F., and Frangione, B. (2006). Studies on the first described Alzheimer’s disease amyloid beta mutant, the Dutch variant. J. Alzheimers Dis. 9, 329–339. doi: 10.3233/jad-2006-9s337
Lewandowski, C. T., Laham, M. S., and Thatcher, G. R. J. (2022). Remembering your A, B, C’s: Alzheimer’s disease and ABCA1. Acta Pharm. Sin. B 12, 995–1018. doi: 10.1016/j.apsb.2022.01.011
Li, G., Kim, C., Kim, J., Yoon, H., Zhou, H., and Kim, J. (2015). Common Pesticide, Dichlorodiphenyltrichloroethane (DDT), increases amyloid-β levels by impairing the function of ABCA1 and IDE: Implication for Alzheimer’s disease. J. Alzheimers Dis. 46, 109–122. doi: 10.3233/JAD-150024
Li, H., Karl, T., and Garner, B. (2015). Understanding the function of ABCA7 in Alzheimer’s disease. Biochem. Soc. Trans. 43, 920–923. doi: 10.1042/BST20150105
Li, M., Kitamura, A., Beverley, J., Koudelka, J., Duncombe, J., Lennen, R., et al. (2022). Impaired glymphatic function and pulsation alterations in a mouse model of vascular cognitive impairment. Front. Aging Neurosci. 13:788519. doi: 10.3389/fnagi.2021.788519
Liddelow, S. A., Temple, S., Møllgård, K., Gehwolf, R., Wagner, A., Bauer, H., et al. (2012). Molecular characterisation of transport mechanisms at the developing mouse blood-CSF interface: A transcriptome approach. PLoS One 7:e33554. doi: 10.1371/journal.pone.0033554
Lilamand, M., Mouton-Liger, F., Di Valentin, E., Sànchez Ortiz, M., and Paquet, C. (2022). Efficacy and safety of ketone supplementation or ketogenic diets for Alzheimer’s disease: A mini review. Front. Nutr. 8:807970. doi: 10.3389/fnut.2021.807970
Lim, J. C., Kania, K. D., Wijesuriya, H., Chawla, S., Sethi, J. K., Pulaski, L., et al. (2008). Activation of beta-catenin signalling by GSK-3 inhibition increases p-glycoprotein expression in brain endothelial cells. J. Neurochem. 106, 1855–1865. doi: 10.1111/j.1471-4159.2008.05537.x
Lincoff, A. M., Wolski, K., Nicholls, S. J., and Nissen, S. E. (2007). Pioglitazone and risk of cardiovascular events in patients with type 2 diabetes mellitus: A meta-analysis of randomized trials. JAMA 298, 1180–1188. doi: 10.1001/jama.298.10.1180
Liu, C., Zeng, X., An, Z., Yang, Y., Eisenbaum, M., Gu, X., et al. (2018). Sensitive detection of exosomal proteins via a compact surface plasmon resonance biosensor for cancer diagnosis. ACS Sens. 3, 1471–1479. doi: 10.1021/acssensors.8b00230
Loane, D. J., Washington, P. M., Vardanian, L., Pocivavsek, A., Hoe, H. S., Duff, K. E., et al. (2011). Modulation of ABCA1 by an LXR agonist reduces β-amyloid levels and improves outcome after traumatic brain injury. J. Neurotrauma 28, 225–236. doi: 10.1089/neu.2010.1595
Loeffler, D. A. (2023a). Experimental approaches for altering the expression of abeta-degrading enzymes. J. Neurochem. 164, 725–763. doi: 10.1111/jnc.15762
Loeffler, D. A. (2023b). Antibody-mediated clearance of brain amyloid-β: Mechanisms of action, effects of natural and monoclonal anti-aβ antibodies, and downstream effects. J. Alzheimers Dis. Rep. 7, 873–899. doi: 10.3233/ADR-230025
Loo, T. W., and Clarke, D. M. (1998). Quality control by proteases in the endoplasmic reticulum. Removal of a protease-sensitive site enhances expression of human P-glycoprotein. J. Biol. Chem. 273, 32373–32376. doi: 10.1074/jbc.273.49.32373
Lovell, M. A., Xie, C., and Markesbery, W. R. (1998). Decreased glutathione transferase activity in brain and ventricular fluid in Alzheimer’s disease. Neurology 51, 1562–1566. doi: 10.1212/wnl.51.6.1562
Machado-Vieira, R., Manji, H. K., and Zarate, C. A. Jr. (2009). The role of lithium in the treatment of bipolar disorder: Convergent evidence for neurotrophic effects as a unifying hypothesis. Bipolar Disord. 11, 92–109. doi: 10.1111/j.1399-5618.2009.00714.x
Makri, A., Papachristos, D. P., Michaelakis, A., and Vidali, V. P. (2022). Colupulone, colupone and novel deoxycohumulone geranyl analogues as larvicidal agents against Culex pipiens. Pest Manag. Sci. 78, 4217–4222. doi: 10.1002/ps.7039
Marchi, C., Adorni, M. P., Caffarra, P., Ronda, N., Spallazzi, M., Barocco, F., et al. (2019). ABCA1- and ABCG1-mediated cholesterol efflux capacity of cerebrospinal fluid is impaired in Alzheimer’s disease. J. Lipid Res. 60, 1449–1456. doi: 10.1194/jlr.P091033
Marder, W., Khalatbari, S., Myles, J. D., Hench, R., Lustig, S., Yalavarthi, S., et al. (2013). The peroxisome proliferator activated receptor-γ pioglitazone improves vascular function and decreases disease activity in patients with rheumatoid arthritis. J. Am. Heart Assoc. 2:e000441.
Matsumoto, K., Chiba, Y., Fujihara, R., Kubo, H., Sakamoto, H., and Ueno, M. (2015). Immunohistochemical analysis of transporters related to clearance of amyloid-β peptides through blood-cerebrospinal fluid barrier in human brain. Histochem. Cell. Biol. 144, 597–611. doi: 10.1007/s00418-015-1366-7
Mauch, D. H., Nagler, K., Schumacher, S., Goritz, C., Muller, E. C., Otto, A., et al. (2001). CNS synaptogenesis promoted by glia-derived cholesterol. Science 294, 1354–1357. doi: 10.1126/science.294.5545.1354
Mayo Clinic (2023a). Alzheimer’s disease – Symptoms and causes. Available online at: https://www.mayoclinic.org/diseases-conditions/alzheimers-disease/symptoms-causes/syc-20350447 (accessed December 24, 2023).
Mayo Clinic (2023b). Drugs and supplements. Verapamil (oral route). Available online at: https://www.mayoclinic.org/drugs-supplements/verapamil-oral-route/side-effects/drg-20071728?p=1) (accessed October 29, 2023).
Medical News Today (2022). Dexamethasone, oral tablet. Available online at: https://www.medicalnewstoday.com/articles/322409 (accessed November 5, 2023).
Mehta, D. C., Short, J. L., and Nicolazzo, J. A. (2013). Altered brain uptake of therapeutics in a triple transgenic mouse model of Alzheimer’s disease. Pharm. Res. 30, 2868–2879. doi: 10.1007/s11095-013-1116-2
Meyer zu Schwabedissen, H. E., Grube, M., Dreisbach, A., Jedlitschky, G., Meissner, K., Linnemann, K., et al. (2006). Epidermal growth factor-mediated activation of the map kinase cascade results in altered expression and function of ABCG2 (BCRP). Drug Metab. Dispos. 34, 524–533.
Meyer Zu Schwabedissen, H. E., Grube, M., Heydrich, B., Linnemann, K., and Fusch, C. (2005). Expression, localization, and function of MRP5 (ABCC5), a transporter for cyclic nucleotides, in human placenta and cultured human trophoblasts: Effects of gestational age and cellular differentiation. Am. J. Pathol. 166, 39–48. doi: 10.1016/S0002-9440(10)62230-4
Miller, C. H. T., Maher, S. G., and Young, H. A. (2009). Clinical use of interferon-γ. Ann. N. Y. Acad. Sci. 1182, 69–79. doi: 10.1111/j.1749-6632.2009.05069.x
Miller, V. A., Rigas, J. R., Benedetti, F. M., Verret, A. L., Tong, W. P., Kris, M. G., et al. (1996). Initial clinical trial of the retinoid receptor pan agonist 9-cis retinoic acid. Clin. Cancer Res. 2, 471–475.
Mitrani, M. I., Bellio, M. A., Sagel, A., Saylor, M., Kapp, W., VanOsdol, K., et al. (2021). Case report: Administration of amniotic fluid-derived nanoparticles in three severely ill COVID-19 patients. Front. Med. 8:583842. doi: 10.3389/fmed.2021.583842
Mo, W., and Zhang, J. T. (2012). Human ABCG2: Structure, function, and its role in multidrug resistance. Int. J. Biochem. Mol Biol. 3, 1–27.
Møllgård, K., Dziegielewska, K. M., Holst, C. B., Habgood, M. D., and Saunders, N. R. (2017). Brain barriers and functional interfaces with sequential appearance of ABC efflux transporters during human development. Sci. Rep. 7:11603. doi: 10.1038/s41598-017-11596-0
Molloy, D. W., Standish, T. I., Zhou, Q., and Guyatt, G., Darad Study, and Group. (2013). A multicenter, blinded, randomized, factorial controlled trial of doxycycline and rifampin for treatment of Alzheimer’s disease: The DARAD trial. Int. J. Geriatr. Psychiatry 28, 463–470. doi: 10.1002/gps.3846
Moore, J. M., Bell, E. L., Hughes, R. O., and Garfield, A. S. (2023). ABC transporters: Human disease and pharmacotherapeutic potential. Trends Mol. Med. 29, 152–172. doi: 10.1016/j.molmed.2022.11.001
Morris, M. E., Rodriguez-Cruz, V., and Felmlee, M. A. (2017). SLC and ABC transporters: Expression, localization, and species differences at the blood-brain and the blood-cerebrospinal fluid barriers. AAPS J. 19, 1317–1331. doi: 10.1208/s12248-017-0110-8
Moussa-Pacha, N. M., Abdin, S. M., Omar, H. A., Alniss, H., and Al-Tel, T. H. (2020). BACE1 inhibitors: Current status and future directions in treating Alzheimer’s disease. Med. Res. Rev. 40, 339–384. doi: 10.1002/med.21622
Muñoz-Cabrera, J. M., Sandoval-Hernández, A. G., Niño, A., Báez, T., Bustos-Rangel, A., Cardona-Gómez, G. P., et al. (2019). Bexarotene therapy ameliorates behavioral deficits and induces functional and molecular changes in very-old triple transgenic mice model of Alzheimer’s disease. PLoS One 14:e0223578. doi: 10.1371/journal.pone.0223578
Nagy, B., Szekeres-Barthó, J., Kovács, G. L., Sulyok, E., Farkas, B., Várnagy, Á, et al. (2021). Key to life: Physiological role and clinical implications of progesterone. Int. J. Mol. Sci. 22:11039. doi: 10.3390/ijms222011039
Naj, A. C., Jun, G., Beecham, G. W., Wang, L. S., Vardarajan, B. N., Buros, J., et al. (2011). Common variants at MS4A4/MS4A6E, CD2AP, CD33 and EPHA1 are associated with late-onset Alzheimer’s disease. Nat. Genet. 43, 436–441. doi: 10.1038/ng.801
Nakamura, K., Kennedy, M. A., Baldan, A., Bojanic, D. D., Lyons, K., and Edwards, P. A. (2004). Expression and regulation of multiple murine ATP-binding cassette transporter G1 mRNAs/isoforms that stimulate cellular cholesterol efflux to high density lipoprotein. J. Biol. Chem. 279, 45980–45989. doi: 10.1074/jbc.M408652200
Nakanishi, T., and Ross, D. D. (2012). Breast cancer resistance protein (BCRP/ABCG2): Its role in multidrug resistance and regulation of its gene expression. Chin. J. Cancer 31, 73–99. doi: 10.5732/cjc.011.10320
Navarrete-Opazo, A., and Mitchell, G. S. (2014). Therapeutic potential of intermittent hypoxia: A matter of dose. Am. J. Physiol. Regul. Integr. Comp. Physiol. 307, R1181–R1197. doi: 10.1152/ajpregu.00208.2014
Neth, B. J., Mintz, A., Whitlow, C., Jung, Y., Solingapuram Sai, K., Register, T. C., et al. (2020). Modified ketogenic diet is associated with improved cerebrospinal fluid biomarker profile, cerebral perfusion, and cerebral ketone body uptake in older adults at risk for Alzheimer’s disease: A pilot study. Neurobiol. Aging 86, 54–63. doi: 10.1016/j.neurobiolaging.2019.09.015
Niemelä, S., Jääskeläinen, T., Lehtola, J., Martikainen, J., Krekelä, I., Sarna, S., et al. (1986). Pirenzepine in the treatment of reflux oesophagitis. A placebo-controlled, double-blind study. Scand. J. Gastroenterol. 21, 1193–1199. doi: 10.3109/00365528608996442
Nies, A. T., Jedlitschky, G., König, J., Herold-Mende, C., Steiner, H. H., Schmitt, H. P., et al. (2004). Expression and immunolocalization of the multidrug resistance proteins, MRP1-MRP6 (ABCC1-ABCC6), in human brain. Neuroscience 129, 349–360. doi: 10.1016/j.neuroscience.2004.07.051
Noh, M. Y., Lim, S. M., Oh, K. W., Cho, K. A., Park, J., Kim, K. S., et al. (2016). Mesenchymal stem cells modulate the functional properties of microglia via TGF-β secretion. Stem Cells Transl. Med. 5, 1538–1549. doi: 10.5966/sctm.2015-0217
Okabe, A., Urano, Y., Itoh, S., Suda, N., Kotani, R., Nishimura, Y., et al. (2013). Adaptive responses induced by 24S-hydroxycholesterol through liver X receptor pathway reduce 7-ketocholesterol-caused neuronal cell death. Redox Biol. 2, 28–35. doi: 10.1016/j.redox.2013.11.007
Oldfield, S., Lowry, C., Ruddick, J., and Lightman, S. (2002). ABCG4: A novel human white family ABC-transporter expressed in the brain and eye. Biochim. Biophys. Acta 1591, 175–179. doi: 10.1016/s0167-4889(02)00269-0
Oram, J. F., and Heinecke, J. W. (2005). ATP-binding cassette transporter A1: A cell cholesterol exporter that protects against cardiovascular disease. Physiol. Rev. 85, 1343–1372. doi: 10.1152/physrev.00005.2005
Osgood, D., Miller, M. C., Messier, A. A., Gonzalez, L., and Silverberg, G. D. (2017). Aging alters mRNA expression of amyloid transporter genes at the blood-brain barrier. Neurobiol. Aging 57, 178–185. doi: 10.1016/j.neurobiolaging.2017.05.011
Paganetti, P., Antoniello, K., Devraj, K., Toni, N., Kieran, D., Madani, R., et al. (2014). Increased efflux of amyloid-β peptides through the blood-brain barrier by muscarinic acetylcholine receptor inhibition reduces pathological phenotypes in mouse models of brain amyloidosis. J. Alzheimers Dis. 38, 767–786. doi: 10.3233/JAD-131091
Pahnke, J., Langer, O., and Krohn, M. (2014). Alzheimer’s and ABC transporters–new opportunities for diagnostics and treatment. Neurobiol. Dis. 72, 54–60. doi: 10.1016/j.nbd.2014.04.001
Pan, J., He, R., Huo, Q., Shi, Y., and Zhao, L. (2020). Brain microvascular endothelial cell derived exosomes potently ameliorate cognitive dysfunction by enhancing the clearance of Aβ through up-regulation of P-gp in mouse model of AD. Neurochem. Res. 45, 2161–2172. doi: 10.1007/s11064-020-03076-1
Panza, F., Solfrizzi, V., Imbimbo, B. P., Tortelli, R., Santamato, A., and Logroscino, G. (2014). Amyloid-based immunotherapy for Alzheimer’s disease in the time of prevention trials: The way forward. Expert Rev. Clin. Immunol. 10, 405–419. doi: 10.1586/1744666X.2014.883921
Panzenboeck, U., Balazs, Z., Sovic, A., Hrzenjak, A., Levak-Frank, S., Wintersperger, A., et al. (2002). ABCA1 and scavenger receptor class B, type I, are modulators of reverse sterol transport at an in vitro blood-brain barrier constituted of porcine brain capillary endothelial cells. J. Biol. Chem. 277, 42781–42789. doi: 10.1074/jbc.M207601200
Park, R., Kook, S. Y., Park, J. C., and Mook-Jung, I. (2014). Aβ1-42 reduces P-glycoprotein in the blood-brain barrier through RAGE-NF-κB signaling. Cell Death. Dis. 5:e12991.
Pascale, C. L., Miller, M. C., Chiu, C., Boylan, M., Caralopoulos, I. N., Gonzalez, L., et al. (2011). Amyloid-beta transporter expression at the blood-CSF barrier is age-dependent. Fluids Barriers CNS 8:21. doi: 10.1186/2045-8118-8-21
Pekcec, A., Schneider, E. L., Baumgärtner, W., Stein, V. M., Tipold, A., and Potschka, H. (2011). Age-dependent decline of blood-brain barrier P-glycoprotein expression in the canine brain. Neurobiol. Aging 32, 1477–1485.
Perry, G., Friedman, R., Shaw, G., and Chau, V. (1987). Ubiquitin is detected in neurofibrillary tangles and senile plaque neurites of Alzheimer disease brains. Proc. Natl. Acad. Sci. U.S.A. 84, 3033–3036. doi: 10.1073/pnas.84.9.3033
Petit, D., Fernández, S. G., Zoltowska, K. M., Enzlein, T., Ryan, N. S., O’Connor, A., et al. (2022). Aβ profiles generated by Alzheimer’s disease causing PSEN1 variants determine the pathogenicity of the mutation and predict age at disease onset. Mol. Psychiatry 27, 2821–2832. doi: 10.1038/s41380-022-01518-6
Pfrieger, F. W., and Ungerer, N. (2011). Cholesterol metabolism in neurons and astrocytes. Prog. Lipid Res. 50, 357–371. doi: 10.1016/j.plipres.2011.06.002
Phillips, M. C., Deprez, L. M., Mortimer, G. M., Murtagh, D. K., McCoy, S., Mylchreest, R., et al. (2021). Randomized crossover trial of a modified ketogenic diet in Alzheimer’s disease. Alzheimers Res. Ther. 13:51. doi: 10.1186/s13195-021-00783-x
Poller, B., Drewe, J., Krähenbühl, S., Huwyler, J., and Gutmann, H. (2010). Regulation of BCRP (ABCG2) and P-glycoprotein (ABCB1) by cytokines in a model of the human blood-brain barrier. Cell. Mol. Neurobiol. 30, 63–70. doi: 10.1007/s10571-009-9431-1
Prasanthi, J. R., Huls, A., Thomasson, S., Thompson, A., Schommer, E., and Ghribi, O. (2009). Differential effects of 24-hydroxycholesterol and 27-hydroxycholesterol on beta-amyloid precursor protein levels and processing in human neuroblastoma SH-SY5Y cells. Mol. Neurodegener. 4:1. doi: 10.1186/1750-1326-4-1
Price, K. L., McRae-Clark, A. L., Saladin, M. E., Maria, M. M., DeSantis, S. M., Back, S. E., et al. (2009). D-cycloserine and cocaine cue reactivity: Preliminary findings. Am. J. Drug Alcohol Abuse 35, 434–438. doi: 10.3109/00952990903384332
Prillaman, M. (2022). Alzheimer’s drug slows mental decline in trial – but is it a breakthrough? Nature 610, 15–16. doi: 10.1038/d41586-022-03081-0
Prinz, W. A. (2007). Non-vesicular sterol transport in cells. Prog. Lipid Res. 46, 297–314. doi: 10.1016/j.plipres.2007.06.002
Qin, D., Yang, W., Pan, Z., Zhang, Y., Li, X., and Lakshmanan, S. (2020). Differential proteomics analysis of serum exosomein burn patients. Saudi J. Biol. Sci. 27, 2215–2220. doi: 10.1016/j.sjbs.2020.06.024
Qosa, H., Abuasal, B. S., Romero, I. A., Weksler, B., Couraud, P. O., Keller, J. N., et al. (2014). Differences in amyloid-β clearance across mouse and human blood-brain barrier models: Kinetic analysis and mechanistic modeling. Neuropharmacology 79, 668–678. doi: 10.1016/j.neuropharm.2014.01.023
Reitz, C., Jun, G., Naj, A., Rajbhandary, R., Vardarajan, B. N., Wang, L. S., et al. (2013). Variants in the ATP-binding cassette transporter (ABCA7), apolipoprotein E 4, and the risk of late-onset Alzheimer disease in African Americans. JAMA 309, 1483–1492. doi: 10.1001/jama.2013.2973
Ren, G., Bao, W., Zeng, Z., Zhang, W., Shang, C., Wang, M., et al. (2019). Retinoid X receptor alpha nitro-ligand Z-10 and its optimized derivative Z-36 reduce β-amyloid plaques in Alzheimer’s disease mouse model. Mol. Pharm. 16, 480–488. doi: 10.1021/acs.molpharmaceut.8b00096
Repa, J. J., Turley, S. D., Lobaccaro, J. A., Medina, J., Li, L., Lustig, K., et al. (2000). Regulation of absorption and ABC1-mediated efflux of cholesterol by RXR heterodimers. Science 289, 1524–1529. doi: 10.1126/science.289.5484.1524
Richardson, J. R., Roy, A., Shalat, S. L., von Stein, R. T., Hossain, M. M., Buckley, B., et al. (2014). Elevated serum pesticide levels and risk for Alzheimer disease. JAMA Neurol. 71, 284–290. doi: 10.1001/jamaneurol.2013.6030
Ritter, C. A., Jedlitschky, G., Meyer zu Schwabedissen, H., Grube, M., Köck, K., and Kroemer, H. K. (2005). Cellular export of drugs and signaling molecules by the ATP-binding cassette transporters MRP4 (ABCC4) and MRP5 (ABCC5). Drug Metab. Rev. 37, 253–278. doi: 10.1081/dmr-200047984
Roberts, L. M., Black, D. S., Raman, C., Woodford, K., Zhou, M., Haggerty, J. E., et al. (2008). Subcellular localization of transporters along the rat blood-brain barrier and blood-cerebral-spinal fluid barrier by in vivo biotinylation. Neuroscience 155, 423–438. doi: 10.1016/j.neuroscience.2008.06.015
Rosati, A., Maniori, S., Decorti, G., Candussio, L., Giraldi, T., and Bartoli, F. (2003). Physiological regulation of P-glycoprotein, MRP1, MRP2 and cytochrome P450 3A2 during rat ontogeny. Dev. Growth Differ. 45, 377–387. doi: 10.1046/j.1440-169x.2003.00699.x
Sakae, N., Liu, C. C., Shinohara, M., Frisch-Daiello, J., Ma, L., Yamazaki, Y., et al. (2016). ABCA7 deficiency accelerates amyloid-β generation and Alzheimer’s neuronal pathology. J. Neurosci. 36, 3848–3859. doi: 10.1523/JNEUROSCI.3757-15.2016
Salkeni, M. A., Lynch, J. L., Otamis-Price, T., and Banks, W. A. (2009). Lipopolysaccharide impairs blood-brain barrier P-glycoprotein function in mice through prostaglandin- and nitric oxide-independent pathways. J. Neuroimmune Pharmacol. 4, 276–282. doi: 10.1007/s11481-008-9138-y
Salloway, S., Sperling, R., Fox, N. C., Blennow, K., Klunk, W., Raskind, M., et al. (2014). Two phase 3 trials of bapineuzumab in mild-to-moderate Alzheimer’s disease. N. Engl. J. Med. 370, 322–333. doi: 10.1056/NEJMoa1304839
Salvamoser, J. D., Avemary, J., Luna-Munguia, H., Pascher, B., Getzinger, T., Pieper, T., et al. (2015). Glutamate-mediated down-regulation of the multidrug-resistance protein BCRP/ABCG2 in porcine and human brain capillaries. Mol. Pharm. 12, 2049–2060. doi: 10.1021/mp500841w
Sampath, J., Adachi, M., Hatse, S., Naesens, L., Balzarini, J., Flatley, R. M., et al. (2002). Role of MRP4 and MRP5 in biology and chemotherapy. AAPS Pharm. Sci. 4:E14. doi: 10.1208/s040314
Sano, O., Tsujita, M., Shimizu, Y., Kato, R., Kobayashi, A., Kioka, N., et al. (2016). ABCG1 and ABCG4 suppress γ-secretase activity and amyloid β production. PLoS One 11:e0155400. doi: 10.1371/journal.pone.0155400
Santa Ana, E. J., Rounsaville, B. J., Frankforter, T. L., Nich, C., Babuscio, T., Poling, J., et al. (2009). D-Cycloserine attenuates reactivity to smoking cues in nicotine dependent smokers: A pilot investigation. Drug Alcohol Depend. 104, 220–227. doi: 10.1016/j.drugalcdep.2009.04.023
Sarkadi, B., Ozvegy-Laczka, C., Német, K., and Váradi, A. (2004). ABCG2 – a transporter for all seasons. FEBS Lett. 567, 116–120. doi: 10.1016/j.febslet.2004.03.123
Satoh, K., Abe-Dohmae, S., Yokoyama, S., St George-Hyslop, P., and Fraser, P. E. (2015). ATP-binding cassette transporter A7 (ABCA7) loss of function alters Alzheimer amyloid processing. J. Biol. Chem. 290, 24152–24165. doi: 10.1074/jbc.M115.655076
Schulz, J. A., Hartz, A. M. S., and Bauer, B. (2023). ABCB1 and ABCG2 regulation at the blood-brain barrier: Potential new targets to improve brain drug delivery. Pharmacol. Rev. 75, 815–853. doi: 10.1124/pharmrev.120.000025
Schumacher, T., Krohn, M., Hofrichter, J., Lange, C., Stenzel, J., Steffen, J., et al. (2017). ABC transporters B1, C1 and G2 differentially regulate neuroregeneration in mice. PLoS One 7:e35613. doi: 10.1371/journal.pone.0035613
Serebrovskaya, T. V., Manukhina, E. B., Smith, M. L., Downey, H. F., and Mallet, R. T. (2008). Intermittent hypoxia: Cause of or therapy for systemic hypertension? Exp. Biol. Med. Maywood 233, 627–650. doi: 10.3181/0710-MR-267
Serebrovskaya, T. V., Swanson, R. J., and Kolesnikova, E. E. (2003). Intermittent hypoxia: Mechanisms of action and some applications to bronchial asthma treatment. J. Physiol. Pharmacol. 54, 35–41.
Shatilo, V. B., Korkushko, O. V., Ischuk, V. A., Downey, H. F., and Serebrovskaya, T. V. (2008). Effects of intermittent hypoxia training on exercise performance, hemodynamics, and ventilation in healthy senior men. High Alt. Med. Biol. 9, 43–52. doi: 10.1089/ham.2007.1053
Shen, S., Callaghan, D., Juzwik, C., Xiong, H., Huang, P., and Zhang, W. (2010). ABCG2 reduces ROS-mediated toxicity and inflammation: A potential role in Alzheimer’s disease. J. Neurochem. 114, 1590–1604. doi: 10.1111/j.1471-4159.2010.06887.x
Shen, X., Xia, L., Liu, L., Jiang, H., Shannahan, J., Du, Y., et al. (2020). Altered clearance of beta-amyloid from the cerebrospinal fluid following subchronic lead exposure in rats: Roles of RAGE and LRP1 in the choroid plexus. J. Trace Elem. Med. Biol. 61:126520. doi: 10.1016/j.jtemb.2020.126520
Shubbar, M. H., and Penny, J. I. (2018). Effect of amyloid beta on ATP-binding cassette transporter expression and activity in porcine brain microvascular endothelial cells. Biochim. Biophys. Acta Gen. Subj. 1862, 2314–2322. doi: 10.1016/j.bbagen.2018.07.021
Shubbar, M. H., and Penny, J. I. (2020). Therapeutic drugs modulate ATP-Binding cassette transporter-mediated transport of amyloid beta(1-42) in brain microvascular endothelial cells. Eur. J. Pharmacol. 874:173009. doi: 10.1016/j.ejphar.2020.173009
Siatkowski, R. M., Cotter, S. A., Crockett, R. S., Miller, J. M., Novack, G. D., Zadnik, K., et al. (2008). Two-year multicenter, randomized, double-masked, placebo-controlled, parallel safety and efficacy study of 2% pirenzepine ophthalmic gel in children with myopia. J. AAPOS 12, 332–339. doi: 10.1016/j.jaapos.2007.10.014
Sims, J. R., Zimmer, J. A., Evans, C. D., Lu, M., Ardayfio, P., Sparks, J., et al. (2023). Donanemab in early symptomatic Alzheimer disease: The TRAILBLAZER-ALZ 2 randomized clinical trial. JAMA 330, 512–527. doi: 10.1001/jama.2023.13239
Sita, G., Hrelia, P., Tarozzi, A., and Morroni, F. (2017). P-glycoprotein (ABCB1) and oxidative stress: Focus on Alzheimer’s disease. Oxid. Med. Cell. Longev. 2017:905486. doi: 10.1155/2017/7905486
Sizhong, Z., Xiukung, G., and Yi, Z. (1983). Cytogenetic studies on an epithelial cell line derived from poorly differentiated nasopharyngeal carcinoma. Int. J. Cancer 31, 587–590. doi: 10.1002/ijc.2910310509
Skerrett, R., Pellegrino, M. P., Casali, B. T., Taraboanta, L., and Landreth, G. E. (2015). Combined liver X receptor/peroxisome proliferator-activated receptor gamma agonist treatment reduces amyloid beta levels and improves behavior in amyloid precursor protein/presenilin 1 mice. J. Biol. Chem. 290, 21591–21602. doi: 10.1074/jbc.M115.652008
Słomka, M., Sobalska-Kwapis, M., Korycka-Machała, M., Bartosz, G., Dziadek, J., and Strapagiel, D. (2015). Genetic variation of the ABC transporter gene ABCC1 (multidrug resistance protein 1-MRP1) in the polish population. BMC Genet. 16:114. doi: 10.1186/s12863-015-0271-3
Smith, A. B. III, Han, Q., Breslin, P. A., and Beauchamp, G. K. (2005). Synthesis and assignment of absolute configuration of (-)-oleocanthal: A potent, naturally occurring non-steroidal anti-inflammatory and anti-oxidant agent derived from extra virgin olive oils. Org. Lett. 7, 5075–5078. doi: 10.1021/ol052106a
Soignet, S. L., Miller, V. A., Pfister, D. G., Bienvenu, B. J., Ho, R., Parker, B. A., et al. (2000). Initial clinical trial of a high-affinity retinoic acid receptor ligand (LGD1550). Clin. Cancer Res. 6, 1731–1735.
Song, C., Kokontis, J. M., Hiipakka, R. A., and Liao, S. (1994). Ubiquitous receptor: A receptor that modulates gene activation by retinoic acid and thyroid hormone receptors. Proc. Natl. Acad. Sci. U.S.A. 91, 10809–10813. doi: 10.1073/pnas.91.23.10809
Soontornmalai, A., Vlaming, M. L., and Fritschy, J. M. (2006). Differential, strain-specific cellular and subcellular distribution of multidrug transporters in murine choroid plexus and blood-brain barrier. Neuroscience 138, 159–169. doi: 10.1016/j.neuroscience.2005.11.011
Sportès, C., Gress, R. E., and Mackall, C. L. (2009). Perspective on potential clinical applications of recombinant human interleukin-7. Ann. N. Y. Acad. Sci. 1182, 28–38. doi: 10.1111/j.1749-6632.2009.05075.x
Steinberg, S., Stefansson, H., Jonsson, T., Johannsdottir, H., Ingason, A., Helgason, H., et al. (2015). Loss-of-function variants in ABCA7 confer risk of Alzheimer’s disease. Nat. Genet. 47, 445–447. doi: 10.1038/ng.3246
Stojic, J., Stöhr, H., and Weber, B. H. (2007). Three novel ABCC5 splice variants in human retina and their role as regulators of ABCC5 gene expression. BMC Mol. Biol. 8:42. doi: 10.1186/1471-2199-8-42
Stolk, J., Stockley, R. A., Stoel, B. C., Cooper, B. G., Piitulainen, E., Seersholm, N., et al. (2012). Randomised controlled trial for emphysema with a selective agonist of the γ-type retinoic acid receptor. Eur. Respir. J. 40, 306–312. doi: 10.1183/09031936.00161911
Storck, S. E., Hartz, A. M. S., Bernard, J., Wolf, A., Kachlmeier, A., Mahringer, A., et al. (2018). The concerted amyloid-beta clearance of LRP1 and ABCB1/P-gp across the blood-brain barrier is linked by PICALM. Brain Behav. Immun. 73, 21–33. doi: 10.1016/j.bbi.2018.07.017
Storelli, F., Billington, S., Kumar, A. R., and Unadkat, J. D. (2021). Abundance of P-glycoprotein and other drug transporters at the human blood-brain barrier in Alzheimer’s disease: A quantitative targeted proteomic study. Clin. Pharmacol. Ther. 109, 667–675. doi: 10.1002/cpt.2035
Strazielle, N., and Ghersi-Egea, J. F. (2015). Efflux transporters in blood-brain interfaces of the developing brain. Front. Neurosci. 9:21. doi: 10.3389/fnins.2015.00021
Sukowati, C. H., Rosso, N., Pascut, D., Anfuso, B., Torre, G., Francalanci, P., et al. (2012). Gene and functional up-regulation of the BCRP/ABCG2 transporter in hepatocellular carcinoma. BMC Gastroenterol. 12:160. doi: 10.1186/1471-230X-12-160
Sultana, R., and Butterfield, D. A. (2004). Oxidatively modified GST and MRP1 in Alzheimer’s disease brain: Implications for accumulation of reactive lipid peroxidation products. Neurochem. Res. 29, 2215–2220. doi: 10.1007/s11064-004-7028-0
Sun, H.-M., Chen, L., and Miao, J.-W. (2014). The role of transporters ABCG1/4 and ABCA1 in brain cholesterol metabolism. Progr. Biochem. Biophys. 41, 765–776.
Sun, Y., Fan, J., Zhu, Z., Guo, X., Zhou, T., Duan, W., et al. (2015). Small molecule TBTC as a new selective retinoid X receptor α agonist improves behavioral deficit in Alzheimer’s disease model mice. Eur. J. Pharmacol. 762, 202–213. doi: 10.1016/j.ejphar.2015.05.050
Sun, Y., Yao, J., Kim, T. W., and Tall, A. R. (2003). Expression of liver X receptor target genes decreases cellular amyloid beta peptide secretion. J. Biol. Chem. 278, 27688–27694. doi: 10.1074/jbc.M300760200
Suzuki, S., Nishimaki-Mogami, T., Tamehiro, N., Inoue, K., Arakawa, R., Abe-Dohmae, S., et al. (2004). Verapamil increases the apolipoprotein-mediated release of cellular cholesterol by induction of ABCA1 expression via liver X receptor-independent mechanism. Arterioscler. Thromb. Vasc. Biol. 24, 519–525. doi: 10.1161/01.ATV.0000117178.94087.ba
Szakács, G., Paterson, J. K., Ludwig, J. A., Booth-Genthe, C., and Gottesman, M. M. (2006). Targeting multidrug resistance in cancer. Nat. Rev. Drug Discov. 5, 219–234. doi: 10.1038/nrd1984
Tai, L. M., Loughlin, A. J., Male, D. K., and Romero, I. A. (2009). P-glycoprotein and breast cancer resistance protein restrict apical-to-basolateral permeability of human brain endothelium to amyloid-beta. J. Cereb. Blood Flow Metab. 29, 1079–1083. doi: 10.1038/jcbfm.2009.42
Tai, L. M., Mehra, S., Shete, V., Estus, S., Rebeck, G. W., Bu, G., et al. (2014). Soluble apoE/Aβ complex: Mechanism and therapeutic target for APOE4-induced AD risk. Mol. Neurodegener. 9:2. doi: 10.1186/1750-1326-9-2
Tan, B., Piwnica-Worms, D., and Ratner, L. (2000). Multidrug resistance transporters and modulation. Curr. Opin. Oncol. 12, 450–458.
Tan, D. T., Lam, D. S., Chua, W. H., Shu-Ping, D. F., Crockett, R. S., et al., Asian Pirenzepine (2005). One-year multicenter, double-masked, placebo-controlled, parallel safety and efficacy study of 2% pirenzepine ophthalmic gel in children with myopia. Ophthalmology 112, 84–91. doi: 10.1016/j.ophtha.2004.06.038
Tanaka, N., Abe-Dohmae, S., Iwamoto, N., Fitzgerald, M. L., and Yokoyama, S. (2010). Helical apolipoproteins of high-density lipoprotein enhance phagocytosis by stabilizing ATP-binding cassette transporter A7. J. Lipid Res. 51, 2591–2599. doi: 10.1194/jlr.M006049
Tansley, G. H., Burgess, B. L., Bryan, M. T., Su, Y., Hirsch-Reinshagen, V., Pearce, J., et al. (2007). The cholesterol transporter ABCG1 modulates the subcellular distribution and proteolytic processing of beta-amyloid precursor protein. J. Lipid Res. 48, 1022–1034 doi: 10.1194/jlr.M600542-JLR200
Tarr, P. T., and Edwards, P. A. (2008). ABCG1 and ABCG4 are coexpressed in neurons and astrocytes of the CNS and regulate cholesterol homeostasis through SREBP-2. J. Lipid Res. 49, 169–182. doi: 10.1194/jlr.M700364-JLR200
Tarr, P. T., Tarling, E. J., Bojanic, D. D., Edwards, P. A., and Baldán, A. (2009). Emerging new paradigms for ABCG transporters. Biochim. Biophys. Acta 1791, 584–593. doi: 10.1016/j.bbalip.2009.01.007
Teachey, D. T., and Pui, C. H. (2019). Comparative features and outcomes between paediatric T-cell and B-cell acute lymphoblastic leukaemia. Lancet Oncol. 20, e142–e154. doi: 10.1016/S1470-2045(19)30031-2
Tijms, B. M., Vromen, E. M., Mjaavatten, O., Holstege, H., Reus, L. M., van der Lee, S., et al. (2024). Cerebrospinal fluid proteomics in patients with Alzheimer’s disease reveals five molecular subtypes with distinct genetic risk profiles. Nat. Aging 4, 33–47. doi: 10.1038/s43587-023-00550-7
Tomioka, M., Toda, Y., Mañucat, N. B., Akatsu, H., Fukumoto, M., Kono, N., et al. (2017). Lysophosphatidylcholine export by human ABCA7. Biochim. Biophys. Acta Mol. Cell. Biol. Lipids 1862, 658–665. doi: 10.1016/j.bbalip.2017.03.012
Toornvliet, R., van Berckel, B. N., Luurtsema, G., Lubberink, M., Geldof, A. A., Bosch, T. M., et al. (2006). Effect of age on functional P-glycoprotein in the blood-brain barrier measured by use of (R)-[(11)C]verapamil and positron emission tomography. Clin. Pharmacol. Ther. 79, 540–548. doi: 10.1016/j.clpt.2006.02.004
Tzekaki, E. E., Tsolaki, M., Pantazaki, A. A., Geromichalos, G., Lazarou, E., Kozori, M., et al. (2019). Administration of the extra virgin olive oil (EVOO) in mild cognitive impairment (MCI) patients as a therapy for preventing the progress to AD. Hell. J. Nucl. Med. 22:181.
Uehara, Y., Yamada, T., Baba, Y., Miura, S., Abe, S., Kitajima, K., et al. (2008). ATP-binding cassette transporter G4 is highly expressed in microglia in Alzheimer’s brain. Brain Res. 1217, 239–246. doi: 10.1016/j.brainres.2008.04.048
Valenza, M., Marullo, M., Di Paolo, E., Cesana, E., Zuccato, C., Biella, G., et al. (2015). Disruption of astrocyte-neuron cholesterol cross talk affects neuronal function in Huntington’s disease. Cell Death Differ. 22, 690–702. doi: 10.1038/cdd.2014.162
Valledor, A. F., Hsu, L. C., Ogawa, S., Sawka-Verhelle, D., Karin, M., and Glass, C. K. (2004). Activation of liver X receptors and retinoid X receptors prevents bacterial-induced macrophage apoptosis. Proc. Natl. Acad. Sci. U.S.A. 101, 17813–17818. doi: 10.1073/pnas.0407749101
van Dyck, C. H., Swanson, C. J., Aisen, P., Bateman, R. J., Chen, C., Gee, M., et al. (2023). Lecanemab in early Alzheimer’s disease. N. Engl. J. Med. 388, 9–21. doi: 10.1056/NEJMoa2212948
Vanmierlo, T., Rutten, K., Dederen, J., Bloks, V. W., van Vark-van, der Zee, L. C., et al. (2011). Liver X receptor activation restores memory in aged AD mice without reducing amyloid. Neurobiol. Aging 32, 1262–1272. doi: 10.1016/j.neurobiolaging.2009.07.005
Vasquez, J. B., Fardo, D. W., and Estus, S. (2013). ABCA7 expression is associated with Alzheimer’s disease polymorphism and disease status. Neurosci. Lett. 556, 58–62. doi: 10.1016/j.neulet.2013.09.058
Vaughan, A. M., and Oram, J. F. (2005). ABCG1 redistributes cell cholesterol to domains removable by high density lipoprotein but not by lipid-depleted apolipoproteins. J. Biol. Chem. 280, 30150–30157. doi: 10.1074/jbc.M505368200
Vaughan, A. M., and Oram, J. F. (2006). ABCA1 and ABCG1 or ABCG4 act sequentially to remove cellular cholesterol and generate cholesterol-rich HDL. J. Lipid Res. 47, 2433–2443. doi: 10.1194/jlr.M600218-JLR200
Versele, R., Corsi, M., Fuso, A., Sevin, E., Businaro, R., Gosselet, F., et al. (2020). Ketone bodies promote amyloid-β1-40 clearance in a human in vitro blood-brain barrier model. Int. J. Mol. Sci. 21:934. doi: 10.3390/ijms21030934
Versele, R., Sevin, E., Gosselet, F., Fenart, L., and Candela, P. (2022). TNF-α and IL-1β modulate blood-brain barrier permeability and decrease amyloid-β peptide efflux in a human blood-brain barrier model. Int. J. Mol. Sci. 23:10235. doi: 10.3390/ijms231810235
Vitali, C., Wellington, C. L., and Calabresi, L. (2014). HDL and cholesterol handling in the brain. Cardiovasc. Res. 103, 405–413. doi: 10.1093/cvr/cvu148
Vogelgesang, S., Cascorbi, I., Schroeder, E., Pahnke, J., Kroemer, H. K., Siegmund, W., et al. (2002). Deposition of Alzheimer’s beta-amyloid is inversely correlated with P-glycoprotein expression in the brains of elderly non-demented humans. Pharmacogenetics 12, 535–541. doi: 10.1097/00008571-200210000-00005
Vogelgesang, S., Warzok, R. W., Cascorbi, I., Kunert-Keil, C., Schroeder, E., Kroemer, H. K., et al. (2004). The role of P-glycoprotein in cerebral amyloid angiopathy; implications for the early pathogenesis of Alzheimer’s disease. Curr. Alzheimer Res. 1, 121–125. doi: 10.2174/1567205043332225
Wahrle, S. E., Jiang, H., Parsadanian, M., Kim, J., Li, A., Knoten, A., et al. (2008). Overexpression of ABCA1 reduces amyloid deposition in the PDAPP mouse model of Alzheimer disease. J. Clin. Invest. 118, 671–682. doi: 10.1172/JCI33622
Wanek, T., Zoufal, V., Brackhan, M., Krohn, M., Mairinger, S., Filip, T., et al. (2020). Brain distribution of dual ABCB1/ABCG2 substrates is unaltered in a beta-amyloidosis mouse model. Int. J. Mol. Sci. 21:8245. doi: 10.3390/ijms21218245
Wang, H., Zhou, L., Gupta, A., Vethanayagam, R. R., Zhang, Y., Unadkat, J. D., et al. (2006). Regulation of BCRP/ABCG2 expression by progesterone and 17beta-estradiol in human placental BeWo cells. Am. J. Physiol. Endocrinol. Metab. 290, E798–E807. doi: 10.1152/ajpendo.00397.2005
Wang, N., Lan, D., Chen, W., Matsuura, F., and Tall, A. R. (2004). ATP-binding cassette transporters G1 and G4 mediate cellular cholesterol efflux to high-density lipoproteins. Proc. Natl. Acad. Sci. U. S. A. 101, 9774–9779. doi: 10.1073/pnas.0403506101
Wang, N., Yvan-Charvet, L., Lütjohann, D., Mulder, M., Vanmierlo, T., Kim, T. W., et al. (2008). ATP-binding cassette transporters G1 and G4 mediate cholesterol and desmosterol efflux to HDL and regulate sterol accumulation in the brain. FASEB J. 22, 1073–1082. doi: 10.1096/fj.07-9944com
Wang, R., and Reddy, P. H. (2017). Role of glutamate and NMDA receptors in Alzheimer’s disease. J. Alzheimers Dis. 57, 1041–1048. doi: 10.3233/JAD-160763
Wang, W., Bodles-Brakhop, A. M., and Barger, S. W. (2016). A role for P-glycoprotein in clearance of alzheimer amyloid β -peptide from the brain. Curr. Alzheimer Res. 13, 615–620. doi: 10.2174/1567205013666160314151012
Warren, M. S., Zerangue, N., Woodford, K., Roberts, L. M., Tate, E. H., Feng, B., et al. (2009). Comparative gene expression profiles of ABC transporters in brain microvessel endothelial cells and brain in five species including human. Pharmacol. Res. 59, 404–413. doi: 10.1016/j.phrs.2009.02.007
Watson, B. J., Wilson, S., Griffin, L., Kalk, N. J., Taylor, L. G., Munafò, M. R., et al. (2011). A pilot study of the effectiveness of D-cycloserine during cue-exposure therapy in abstinent alcohol-dependent subjects. Psychopharmacology 216, 121–129. doi: 10.1007/s00213-011-2199-2
Weksler, B. B., Subileau, E. A., Perrière, N., Charneau, P., Holloway, K., Leveque, M., et al. (2005). Blood-brain barrier-specific properties of a human adult brain endothelial cell line. FASEB J. 19, 1872–1874. doi: 10.1096/fj.04-3458fje
Wente, W., Brenner, M. B., Zitzer, H., Gromada, J., and Efanov, A. M. (2007). Activation of liver X receptors and retinoid X receptors induces growth arrest and apoptosis in insulin-secreting cells. Endocrinology 148, 1843–1849. doi: 10.1210/en.2006-1247
Wesołowska, O., Molnar, J., Ocsovszki, I., and Michalak, K. (2009). Differential effect of phenothiazines on MRP1 and P-glycoprotein activity. In Vivo 23, 943–947.
Wielinga, P. R., van der Heijden, I., Reid, G., Beijnen, J. H., Wijnholds, J., and Borst, P. (2003). Characterization of the MRP4- and MRP5-mediated transport of cyclic nucleotides from intact cells. J. Biol. Chem. 278, 17664–17671. doi: 10.1074/jbc.M212723200
Wijesuriya, H. C., Bullock, J. Y., Faull, R. L., Hladky, S. B., and Barrand, M. A. (2010). ABC efflux transporters in brain vasculature of Alzheimer’s subjects. Brain Res. 1358, 228–238. doi: 10.1016/j.brainres.2010.08.034
Wijnholds, J., Mol, C. A., van Deemter, L., de Haas, M., Scheffer, G. L., Baas, F., et al. (2000). Multidrug-resistance protein 5 is a multispecific organic anion transporter able to transport nucleotide analogs. Proc. Natl. Acad. Sci. U.S.A. 97, 7476–74781. doi: 10.1073/pnas.120159197
Willy, P. J., Umesono, K., Ong, E. S., Evans, R. M., Heyman, R. A., and Mangelsdorf, D. J. (1995). LXR, a nuclear receptor that defines a distinct retinoid response pathway. Genes Dev. 9, 1033–1045. doi: 10.1101/gad.9.9.1033
Wolf, A., Bauer, B., and Hartz, A. M. (2012). ABC transporters and the Alzheimer’s disease enigma. Front. Psychiatry 3:54. doi: 10.3389/fpsyt.2012.00054
Wollmer, M. A., Sleegers, K., Ingelsson, M., Zekanowski, C., Brouwers, N., Maruszak, A., et al. (2007). Association study of cholesterol-related genes in Alzheimer’s disease. Neurogenetics 8, 179–188. doi: 10.1007/s10048-007-0087-z
Wu, D., Li, L., Wen, Z., and Wang, G. (2023). Romosozumab in osteoporosis: Yesterday, today and tomorrow. J. Transl. Med. 21:668. doi: 10.1186/s12967-023-04563-z
Xia, M., Hou, M., Zhu, H., Ma, J., Tang, Z., Wang, Q., et al. (2005). Anthocyanins induce cholesterol efflux from mouse peritoneal macrophages: The role of the peroxisome proliferator-activated receptor {gamma}-liver X receptor {alpha}-ABCA1 pathway. J. Biol. Chem. 280, 36792–36801. doi: 10.1074/jbc.M505047200
Xie, X., Song, Q., Dai, C., Cui, S., Tang, R., Li, S., et al. (2023). Clinical safety and efficacy of allogenic human adipose mesenchymal stromal cells-derived exosomes in patients with mild to moderate Alzheimer’s disease: A phase I/II clinical trial. Gen. Psychiatr. 36:e101143.
Xiong, H., Callaghan, D., Jones, A., Bai, J., Rasquinha, I., Smith, C., et al. (2009). ABCG2 is upregulated in Alzheimer’s brain with cerebral amyloid angiopathy and may act as a gatekeeper at the blood-brain barrier for Abeta(1-40) peptides. J. Neurosci. 29, 5463–5475.
Xu, P., Li, D., Tang, X., Bao, X., Huang, J., Tang, Y., et al. (2013). LXR agonists: New potential therapeutic drug for neurodegenerative diseases. Mol. Neurobiol. 48, 715–728. doi: 10.1007/s12035-013-8461-3
Xu, Q., Zhang, Y., Zhang, X., Liu, L., Zhou, B., Mo, R., et al. (2020). Medium-chain triglycerides improved cognition and lipid metabolomics in mild to moderate Alzheimer’s disease patients withAPOE4/: A double-blind, randomized, placebo-controlled crossover trial. Clin. Nutr. 39, 2092–2105.
Xu, W., Tan, L., and Yu, J. T. (2015). The role of PICALM in Alzheimer’s disease. Mol. Neurobiol. 52, 399–413.
Yang, A., Alrosan, A. Z., Sharpe, L. J., Brown, A. J., Callaghan, R., and Gelissen, I. C. (2021). Regulation of ABCG4 transporter expression by sterols and LXR ligands. Biochim. Biophys. Acta Gen. Subj. 1865:129769.
Yang, S., Li, A., Wang, J., Liu, J., Han, Y., Zhang, W., et al. (2018). Vitamin D receptor: A novel therapeutic target for kidney diseases. Curr. Med. Chem. 25, 3256–3271. doi: 10.2174/0929867325666180214122352
Yasuda, S., Itagaki, S., Hirano, T., and Iseki, K. (2005). Expression level of ABCG2 in the placenta decreases from the mid stage to the end of gestation. Biosci. Biotechnol. Biochem. 69, 1871–1876.
Ye, B., Shen, H., Zhang, J., Zhu, Y. G., Ransom, B. R., Chen, X. C., et al. (2015). Dual pathways mediate β-amyloid stimulated glutathione release from astrocytes. Glia 63, 2208–2219.
Yokhana, S. S., Gerst, D. G. III, Lee, D. S., Badr, M. S., Qureshi, T., and Mateika, J. H. (2012). Impact of repeated daily exposure to intermittent hypoxia and mild sustained hypercapnia on apnea severity. J Appl Physiol 112, 367–377.
Yoshikawa, M., Yabuuchi, H., Kuroiwa, A., Ikegami, Y., Sai, Y., Tamai, I., et al. (2002). Molecular and cytogenetic characterization of the mouse ATP-binding cassette transporter Abcg4. Gene 293, 67–75.
Yu, C., Youmans, K. L., and LaDu, M. J. (2010). Proposed mechanism for lipoprotein remodelling in the brain. Biochim. Biophys. Acta 1801, 819–823.
Yuan, C., Guo, X., Zhou, Q., Du, F., Jiang, W., Zhou, X., et al. (2019). OAB-14, a bexarotene derivative, improves Alzheimer’s disease-related pathologies and cognitive impairments by increasing β-amyloid clearance in APP/PS1 mice. Biochim. Biophys. Acta Mol. Basis Dis. 1865, 161–180.
Zelcer, N., Khanlou, N., Clare, R., Jiang, Q., Reed-Geaghan, E. G., Landreth, G. E., et al. (2007). Attenuation of neuroinflammation and Alzheimer’s disease pathology by liver x receptors. Proc. Natl. Acad. Sci. U.S.A. 104, 10601–10606.
Zeng, Y., Callaghan, D., Xiong, H., Yang, Z., Huang, P., and Zhang, W. (2012). Abcg2 deficiency augments oxidative stress and cognitive deficits in Tg-SwDI transgenic mice. J. Neurochem. 122, 456–469.
Zhang, B., Zhang, Y., Xu, T., Yin, Y., Huang, R., Wang, Y., et al. (2017). Chronic dexamethasone treatment results in hippocampal neurons injury due to activate NLRP1 inflammasome in vitro. Int. Immunopharmacol. 49, 222–230.
Zhang, H., Lian, Z., Sun, G., Liu, R., and Xu, Y. (2018). Loss of miR-516a-3p mediates upregulation of ABCC5 in prostate cancer and drives its progression. Onco Targets Ther. 11, 3853–3867.
Zhang, W., Mojsilovic-Petrovic, J., Andrade, M. F., Zhang, H., Ball, M., and Stanimirovic, D. B. (2003). The expression and functional characterization of ABCG2 in brain endothelial cells and vessels. FASEB J. 17, 2085–2087.
Zhang, W., Xiong, H., Callaghan, D., Liu, H., Jones, A., Pei, K., et al. (2013). Blood-brain barrier transport of amyloid beta peptides in efflux pump knock-out animals evaluated by in vivo optical imaging. Fluids Barriers CNS 10:13.
Zhang, X., O’Callaghan, P., Li, H., Tan, Y., Zhang, G., Barash, U., et al. (2021). Heparanase overexpression impedes perivascular clearance of amyloid-β from murine brain: Relevance to Alzheimer’s disease. Acta Neuropathol. Commun. 9:84. doi: 10.1186/s40478-021-01182-x
Zhang, Y., Chen, K., Sloan, S. A., Bennett, M. L., Scholze, A. R., O’Keeffe, S., et al. (2014). An RNA-sequencing transcriptome and splicing database of glia, neurons, and vascular cells of the cerebral cortex. J. Neurosci. 34, 11929–11947.
Zhang, Y., Schuetz, J. D., Elmquist, W. F., and Miller, D. W. (2004). Plasma membrane localization of multidrug resistance-associated protein homologs in brain capillary endothelial cells. J. Pharmacol. Exp. Ther. 311, 449–455.
Zhang, Z., Wu, J. Y., Hait, W. N., and Yang, J. M. (2004). Regulation of the stability of P-glycoprotein by ubiquitination. Mol. Pharmacol. 66, 395–403.
Zhao, J., Fu, Y., Liu, C. C., Shinohara, M., Nielsen, H. M., Dong, Q., et al. (2014). Retinoic acid isomers facilitate apolipoprotein E production and lipidation in astrocytes through the retinoid X receptor/retinoic acid receptor pathway. J. Biol. Chem. 289, 11282–11292.
Zhou, S. F. (2008). Structure, function and regulation of P-glycoprotein and its clinical relevance in drug disposition. Xenobiotica 38, 802–832. doi: 10.1080/00498250701867889
Zhou, S., Schuetz, J. D., Bunting, K. D., Colapietro, A. M., Sampath, J., Morris, J. J., et al. (2001). The ABC transporter Bcrp1/ABCG2 is expressed in a wide variety of stem cells and is a molecular determinant of the side-population phenotype. Nat. Med. 7, 1028–1034.
Zieve, G. W., Turnbull, D., Mullins, J. M., and McIntosh, J. R. (1980). Production of large numbers of mitotic mammalian cells by use of the reversible microtubule inhibitor nocodazole. Exp. Cell. Res. 126, 397–405.
Zoufal, V., Wanek, T., Krohn, M., Mairinger, S., Filip, T., Sauberer, M., et al. (2020a). Age dependency of cerebral P-glycoprotein function in wild-type and APPPS1 mice measured with PET. J. Cereb. Blood Flow Metab. 40, 150–162.
Zoufal, V., Mairinger, S., Krohn, M., Wanek, T., Filip, T., Sauberer, M., et al. (2020c). Measurement of cerebral ABCC1 transport activity in wild-type and APP/PS1-21 mice with positron emission tomography. J. Cereb. Blood Flow Metab. 40, 954–965.
Keywords: ABC transporters, Abeta, Alzheimer’s, cerebral clearance, experimental approaches
Citation: Loeffler DA (2024) Enhancing of cerebral Abeta clearance by modulation of ABC transporter expression: a review of experimental approaches. Front. Aging Neurosci. 16:1368200. doi: 10.3389/fnagi.2024.1368200
Received: 10 January 2024; Accepted: 01 May 2024;
Published: 30 May 2024.
Edited by:
Joon W. Shim, Marshall University, United StatesReviewed by:
Siranjeevi Nagaraj, Université libre de Bruxelles, BelgiumAmal Kaddoumi, Auburn University, United States
Copyright © 2024 Loeffler. This is an open-access article distributed under the terms of the Creative Commons Attribution License (CC BY). The use, distribution or reproduction in other forums is permitted, provided the original author(s) and the copyright owner(s) are credited and that the original publication in this journal is cited, in accordance with accepted academic practice. No use, distribution or reproduction is permitted which does not comply with these terms.
*Correspondence: David A. Loeffler, loefflerdavid6@gmail.com