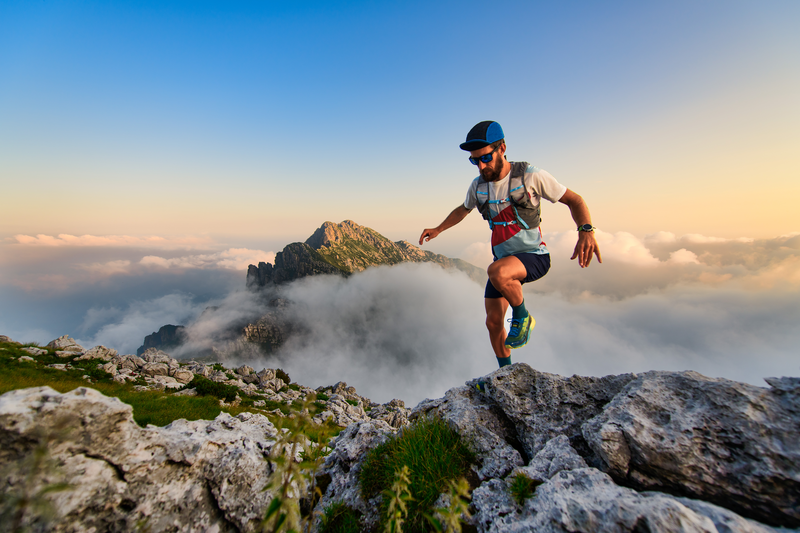
94% of researchers rate our articles as excellent or good
Learn more about the work of our research integrity team to safeguard the quality of each article we publish.
Find out more
ORIGINAL RESEARCH article
Front. Aging Neurosci. , 23 February 2024
Sec. Neurocognitive Aging and Behavior
Volume 16 - 2024 | https://doi.org/10.3389/fnagi.2024.1365086
This article is part of the Research Topic Lifestyle and Healthy Aging to Prevent Cognitive Decline and Dementia View all 24 articles
Introduction: Aging is often linked to oxidative stress, where the body experiences increased damage from free radicals. Plants are rich sources of antioxidants, playing a role in slowing down aging and supporting the proper functioning and longevity of cells. Our study focuses on exploring the impact of Mentha rotundifolia (MR) and Salvia officinalis (SO) hydrosols on aging-related comorbidities.
Methods: The chemical composition of MR and SO hydrosols was analyzed by gas chromatography coupled to mass spectrometry. 2,2-Diphenyl 1-picrylhydrazyl and 2,20-azino-bis 3-ethylbenzothiazoline-6-sulfonic acid radicals scavenging assays were used to assess their in vitro antioxidant activity, and heat induced albumin denaturation test was used to evaluate their anti-inflammatory activity. Subsequently, we administered 5% of each plant hydrosol in the drinking water of 18-month-old rats for six months. We then conducted behavioral tests, including open field, dark/light box, rotarod, and Y-maze assessments, and measured biochemical parameters in plasma, liver and brain tissues.
Results and discussion: At two years old, animals treated with MR and SO hydrosols displayed fewer physical and behavioral impairments, along with well-preserved redox homeostasis in comparison with animals in the control group. These results highlighted the significance of MR and SO hydrosols in addressing various aspects of age-related comorbidities. The study suggests that these plant-derived hydrosols may have potential applications in promoting healthy aging and mitigating associated health challenges.
Aging is a complex physiological process that affects all living organisms, including humans and animals. It is characterized by a progressive decline in physiological function, resulting in an increased susceptibility to age-related diseases and ultimately, death (Khan et al., 2017). Aging is driven by a combination of genetic, environmental, and lifestyle factors, including oxidative stress, inflammation, telomere shortening, mitochondrial dysfunction, and cellular senescence (Song et al., 2020).
The oxidative stress theory of aging suggests that one of the key drivers of the aging process is the accumulation of reactive oxygen species (ROS) in cells (Lin and Flint Beal, 2003). ROS are generated during normal cellular metabolism and can cause damage to cellular structures, including lipids, proteins, and DNA (Checa and Aran, 2020). Over time, this damage accumulates and contributes to the development of aging-related comorbidities such as cardiovascular disease, cancer, and neurodegenerative disorders (Ziada et al., 2020).
While aging cannot be prevented, it is possible to slow down the process and delay the onset of aging-related diseases (Poeggeler, 2005). In this context, bioactive compounds from plants with antioxidant and anti-inflammatory properties might represent a promising approach (Barrajón-Catalán et al., 2014; Spagnuolo et al., 2018; Proshkina et al., 2020). Furthermore, phyto-molecules exhibit extensive structural diversity and demonstrated the capability to interact with and bind to multiple receptors, thereby engaging in multitarget interactions (Panossian and Efferth, 2022). This attribute confers polyvalent pharmacological actions and pleiotropic therapeutic activities.
Plant hydrosols have been extensively investigated for their antioxidant and antimicrobial properties in food and cosmetic industries (Catty, 2001; Wajs-Bonikowska et al., 2015). Nevertheless, their potential pharmacological effects have been acknowledged within the realm of traditional medicine. Functioning as a mild form of aromatherapy, these hydrosols feature minimal concentrations of essential oil molecules (less than 1 g/L), rendering them both secure and user-friendly (Acimovic et al., 2020). Several hydrosols are used as functional drinks for the mitigation of infections, hormonal imbalances, depressive states, sleep disturbances, digestive ailments, and neural illnesses (Yaghoob et al., 2004; Baydar et al., 2013; Kunicka-Styczyńska et al., 2015; Hamedi et al., 2017a,b).
In the light of these data, we aim to evaluate the anti-aging effects of hydrosols derived from two Lamiaceae plants: Mentha rotundifolia (L.) Huds (MR) and Salvia officinalis L. (SO). The selection of these plant species is substantiated by their well-documented antioxidant, anti-inflammatory, neuroprotective, and analgesic effects (Hasanein et al., 2016; Boualam et al., 2023). We here assessed the in-vitro antioxidant and antiinflammatory properties of MR and SO hydrosols. Subsequently, we administered 5% of each plant hydrosol in the drinking water provided to 18-month-old Wistar rats over a period of six months. Upon reaching an age of 24-month-old, we conducted a thorough examination of physical, behavioral, and biochemical changes in the aged rat cohort. Finally, the phytoconstituents present in the plant hydrosols were characterized using gas chromatography coupled with mass spectrometry (GC–MS) technique.
The aerial parts from SO and MR were harvested during their blooming periods (June and September respectively), from Mkam Tolba (33°55′41.9” N, 6°16′34.2” W) in Khemisset, Morocco. The plant samples were dried in shade at room temperature for two weeks. 500 g of each plant powder was distilled separately in 2 L using a Clevenger apparatus for 3 h. At the end of the hydrodistillation process, the essential oils have been removed and 500 mL of each plant’s hydrosols was collected.
The composition of MR and SO hydrosols was analyzed using Nexis 2030 gas chromatography system coupled to a TQ8040 NX mass spectrometer (GCMS-TQ8040, SHIMADZU, JAPAN). The separation of the compounds was performed using a Restek RTX-5MS column (30 0.25 mm, film thickness 0.25 μm). The GC temperature was programmed at 50°C for 2 min and increased to 300°C with a rate of 5.5 C/min and stabilized for 3 min at 300°C. Helium was used as the carrier gas with a flow rate of 1.5 mL/min. The sample (1 μL) was injected in split mode (HTA 2800 T injector, HT 250 C). The mass scan was m/z: 50–500. The EO compounds are identified by their column retention indices (RI) determined with reference to the C5-C24 (n-alkane) homolog series, and by comparison of their mass spectra with literature reports using the NIST 2017 and Wiley version libraries (Wiley and NIST, 2017).
Swiss ADME1 tool was used to verify the gastro-intestinal (GI) and the blood brain barrier (BBB) absorptions of the characterized compounds (Daina et al., 2017).
2,2-diphenyl 1-picrylhydrazyl (DPPH) solution was prepared in methanol at the concentration of 79 μg/mL. Then, MR and SO hydrosols solutions were prepared at concentrations of 50–1,000 μL/mL and were mixed with 0.5 mL of DPPH. The reaction mixture was then incubated for 30 min at room temperature in the dark, followed by measurement of the absorbance at 517 nm (Boualam et al., 2023). The scavenging activity was estimated as a percentage using the following formula:
where O.D control and O.D test represent the absorbance values of the control and test samples, respectively.
ABTS assay involves stabilizing the blue-green colored cationic radical 2,20-azino-bis 3-ethylbenzothiazoline-6-sulfonic acid (ABTS+) by an antioxidant, which results in the transformation of the radical to a colorless form. A mixture was prepared by adding 1.9 mL of ABTS+ solution to 600 μL of MR and SO hydrosols solution at various concentrations ranging from 50 to 1,000 μL/mL. It was then incubated at room temperature for 7 min and the absorbance was measured at 734 nm (Boualam et al., 2023). To obtain a blank reading, methanol was used instead of the hydrosols solution. The scavenging activity was estimated as a percentage using the following formula:
where O.D control and O.D test represent the absorbance values of the control and test samples, respectively.
Wistar rats (18-month-old) weighing 400–450 g were selected for the following experiment. They were raised at the central animal care facilities of the Faculty of Sciences, Mohammed V University of Rabat, Morocco and were housed in polyethylene cages in a room with controlled temperature (22 ± 1°C), under a 12 h light–dark cycle. Animals had free access to food and water and were fed on a normal chow diet (Cicalim, Casa Blanca). After two weeks acclimatization, the animals were randomly divided into three groups of six rats each. Due to the chronicity of the treatment period, the treated groups received hydrosols in drinking water to avoid the complications related to long-term oral gavage, namely stress, aspiration pneumonia, unintentional tracheal administration, esophageal trauma, and gastric rupture (Kinder et al., 2014; Dhawan et al., 2018).
- Group 1: Control group, received tap water
- Group 2: received 5% of MR hydrosol in drinking water for six months
- Group 3: received 5% of SO hydrosol in drinking water for six months
Prior to the administration of the hydrosols, the behavioral tests (open field, dark/light box, rotarod, and Y-maze) were performed for all groups, then on day 8, the hydrosols were added to the drinking water of groups 2 and 3 (Figure 1). After a six-month treatment period, we assessed various signs of aging, including physical, cognitive, and biochemical indicators.
At the age of 2 years, the physical changes between the animals treated with the hydrosols and the control rats were analyzed. An observer who was not informed of the study protocol was asked to identify differences. Rats, like other animals, exhibit several signs of aging as they grow older. Some common physical signs of aging in rats include changes in posture, fur, and muscle mass (Phillips et al., 2010). As rats age, they may develop a hunched or stooped posture due to the loss of muscle mass and strength (Baek et al., 2020). This may also impact eyelid muscles leading to ptosis. Their fur may become less shiny and coarse following a lack of grooming routine related to aging (Scimonelli et al., 1999). Additionally, they may experience hair loss in some areas.
The open field test (OF) is a widely utilized method for measuring exploratory behavior and general locomotor activity in mice and rats, as well as assessing anxiety-related behaviors by monitoring defecation and visited areas (Gould et al., 2009; Seibenhener and Wooten, 2015). The OF apparatus employed in this study consists of a black square with an 80 cm2 surface area and a height of 45 cm. The ground of the field is divided into 25 squares of 15 cm × 15 cm each by white strips. The test is conducted in a dimly lit room, and each animal is placed at the center of the field. The field is cleaned after each test, and each animal is allowed to explore the field for 10 min. Locomotor activity is assessed by measuring the distance the animal covers (i.e., the number of tiles crossed) and the number of sit-ups performed. Anxiety-related behavior is evaluated by calculating the number of entries into the central zone of the OF and the duration of time spent in this area.
The assessment of motor coordination in rodents is commonly achieved using the rotarod test (Shiotsuki et al., 2010). The test apparatus consists of a plastic rotating cylinder, measuring 7 cm in diameter and 28 cm in length that is fixed around a horizontal axis elevated by 30 cm and divided into four compartments with a width of 9 cm each (Panlab, Harvard Apparatus). The test is conducted in an isolated room with normal lighting and is composed of two phases: a pre-test phase, during which the animals are habituated to the apparatus, and a test phase, during which rats are placed on the rotating cylinder at a fixed speed of 4 rotations per min (rpm). Once the animal is stabilized, the speed of rotation is progressively increased until reaching a maximum speed of 40 rpm, which is then maintained until the end of the test. The duration of the test is limited to 180 s to prevent fatigue or stress-related effects (Shiotsuki et al., 2010).
The dark/light box test is a commonly used protocol to measure anxiety-like behavior in rodents, involving a two-chambered apparatus consisting of a small dark compartment connected to a larger well-lit compartment by an opening, which the animal can freely explore (Crawley, 1985). In this test, animals typically show a natural aversion to the brightly lit area and prefer to remain in the dark chamber. The test is conducted in a dimly lit room, and the animal is initially placed in the dark compartment and allowed to explore both compartments for 10 min. The amount of time spent in the light compartment and the number of crossings between the two compartments are recorded as measures of anxiety-like behavior.
The Y-maze test is a reliable method for evaluating both spatial working and reference memories in rodents. The Y-maze device consists of a black Y-shaped apparatus with three arms arranged at 120° angles from each other. To assess spatial working memory, spontaneous alternation is calculated by recording the number of arm entries and consecutive entries into all three arms. The percentage of alternation behavior is determined using the formula (Kraeuter et al., 2019):
To assess reference spatial memory, the test is organized into two phases: a training session, during which one arm of the Y-maze is closed off and designated as the novel arm, and a test session, which takes place after a 1-h time interval. During the test session, the animal is placed back in the Y-maze device and is expected to remember the location of the novel arm and visit it more frequently than the other arms. The number of entries into the novel arm is compared to the entries into the other arms to determine the degree of spatial memory (Kraeuter et al., 2019).
According to Taïwe and Kuete (2017), hyperalgesia is characterized by an increased sensitivity to pain induced by noxious or ordinarily non-noxious stimulation of peripheral tissues. The tail immersion test, first described by Janssen et al. (1963), is a commonly used method for assessing hyperalgesia. In this test, animals are restrained, and the tip of their tails is immersed in a water bath at a temperature of 50 ± 0.5°C. The tail withdrawal reflex, which is mediated by spinal nociceptive reflexes (Bannon and Malmberg, 2007), is elicited as a response to the heat stimulus, and the latency of this reflex is measured. To avoid potential skin damage, each immersion is terminated after 15 s.
The Acetone test was used to assess cold allodynia (Deuis et al., 2017). A drop of acetone was applied to the plantar surface of each hind paw using a pipette. Rats were then placed in individual transparent chambers and the time spent licking, biting or lifting the paw was recorded for 2 min. The same procedure was repeated 3 times at 5 min intervals and the results were averaged. Control tests were performed using saline instead of acetone. An increase in the time spent licking, biting or lifting the paw compared to control tests may indicate cold allodynia.
All animals were sacrificed by decapitation 24 h after the completion of the last test and following an overnight fast. Trunk blood samples were collected in heparinized sample bottles and centrifuged at 2000 rpm for 10 min to obtain plasma which was used for biochemical analysis. The plasma levels of alanine transaminase (ALT), aspartate transaminase (AST), bilirubin, and gamma-glutamyl transferase (GGT) were measured using standard methods. Tissue samples were collected from experimental animals and kept chilled on ice throughout the procedure. Brain and liver tissues were homogenized in 50 mM phosphate buffer, pH 7.4 and centrifuged at 4000 rpm for 15 min at 4°C to obtain the soluble fraction (Mahmoud et al., 2023). Supernatants were collected and protein content was determined by adding alkaline copper tartrate solution and Folin–Ciocalteu reagent. Then, absorbance was measured at 750 nm following the method of (Lowry et al., 1951). Bovine Serum Albumin (BSA) was used as a standard. Superoxide dismutase (SOD), Glutathione peroxidase (GPx), and Glutathione (GSH) concentrations were measured by the corresponding biochemical kits (ab285309, ab281168, and ab239727, Abcam, US) according to the manufacturers’ instructions.
Data were analyzed using GraphPad Prism 8. Normality was assessed by the Shapiro–Wilk test. IC50 values were presented as means ± SD (n = 3) and were compared using t-test. Behavioral tests results were analyzed using two-ways ANOVA considering ‘treatment’ and ‘age’ as variation factors. Biochemical analyses were analyzed using one-way ANOVA. Bonferroni’s multiple comparisons test was used whenever there was significance. Results are expressed as means ± SD (n = 6). The differences were regarded as statistically significant at p ≤ 0.05.
GC–MS analysis of the hydrosols revealed the presence of numerous aromatic compounds, some present in substantial concentrations (Figure 2; Table 1). SO contained notable amounts of α-pinene, camphene, 1,8 cineole, and camphor (Table 1), while MR hydrosols displayed richness in camphor, 1,8 cineole, and (E)-thujone (Table 1). Except for α-pinene and camphene, the identified molecules are characterized by favorable GI and BBB absorption properties (Table 1).
Figure 2. GC–MS chromatograms of the hydrosols of Salvia officinalis (SO, top) and Mentha rotundifolia (MR, bottom).
Table 1. Annotated phytocompounds from hydrosols of Salvia officinalis (SO) and Mentha rotundifolia (MR) with their pharmacokinetics parameters.
MR and SO hydrosols exhibited an interesting scavenging ability against DPPH and ABTS radicals (IC50 ≤ 100 μg/mL), Table 2. The obtained results may be attributed to the presence of terpenes with antioxidant behavior such as 1,8 cineole, camphor, and caryophyllene.
Table 2. IC50 values of the hydrosols of Salvia officinalis (SO) and Mentha rotundifolia (MR) in comparison with ascorbic acid in DPPH and Trolox in ABTS assays.
The in-vitro anti-inflammatory activity of MR and SO hydrosols was evaluated using a thermally induced protein denaturation protocol. Both hydrosols, along with the standard diclofenac, exhibited a dose-dependent anti-inflammatory effect (Figure 3). Remarkably, starting from 120 μg/mL, SO hydrosol demonstrated higher activity compared to MR hydrosol (p ≤ 0.05), a finding corroborated by its significantly lower IC50 value (p ≤ 0.05) (Figure 3).
Figure 3. Inhibitory effects of hydrosols Salvia officinalis (SO, IC50 = 230.6 ± 0.44 μg/mL) and Mentha rotundifolia (MR, IC50 = 346 ± 1.73 μg/mL) on albumin denaturation in comparison with diclofenac (IC50 = 98.63 ± 2.18 μg/mL). Data were analyzed using two-ways ANOVA followed by Bonferroni post-hoc test and were considered as statistically different for p ≤ 0.05. a in comparison with the standard, b in comparison with SO hydrosol. Data are presented as (mean ± SD) for n = 3.
A qualitative physical analysis was performed. Apparent aging signs were evaluated including posture, eyelids muscle, and fur changes, Table 3. All groups presented some or all aging signs as it’s an inevitable process. However, there were substantial differences between the control group and hydrosols treated groups. Treatment with hydrosols prevented aging-related muscle loss, enabling treated animals to maintain firmer posture and more open eyelids (Table 3).
Table 3. Effects of the hydrosols of Salvia officinalis (SO) and Mentha rotundifolia (MR) on aging signs identified in 24-month-old rats.
The impact of treatment with SO and MR hydrosols on aging-related locomotion and motor coordination impairments was evaluated through the open field task and rotarod test. Performance metrics, such as the number of crossed squares and sit-ups in the open field, as well as the duration spent on the rotarod, were quantified (Figure 4). In the open field test, a significant reduction in the number of crossings was observed in aged rats compared to their younger counterparts (p < 0.0001). On the other hand, the administration of both SO and MR hydrosols resulted in a substantial improvement in the number of crossings in aged rats (p < 0.0001 for both groups) (Figure 4A). Similarly, the number of sit-ups significantly decreased in the aged group (p < 0.0001) for both control and MR groups compared to younger rats. Notably, in the SO hydrosol-treated group, no significant difference was observed (p = 0.1636) compared to the control group (Figure 4B), indicating an ameliorative effect. Furthermore, the time spent on the rotarod by young rats significantly exceeded that of aged rats (p < 0.0001). Aging rats treated with SO hydrosol exhibited a significant increase in performance on the rotarod compared to the control aged group (p < 0.0001) and the MR aged group (p = 0.0007) (Figure 4C).
Figure 4. Effects of the hydrosols of Salvia officinalis (SO) and Mentha rotundifolia (MR) on the number of crossed squares (A), the number of set-ups of aged rats in the open field (B); and their time passed on the carrousel of rotarod apparatus (C). Results are presented as means ± SD (n = 6). #p ≤ 0.05 compared to the same young rat groups (control, MR, and SO groups at 18-month-old). ap ≤ 0.05 compared to aged control rats (24- month-old). bp ≤ 0.05 compared to SO-aged treated rats.
The impact of SO and MR hydrosols on anxiety-like behavior in both young and aged rats was investigated using the open field and dark/light box tasks, each conducted over a 10-min period. Aged rats exhibited a significant reduction in entries into the inner zone of the open field and fewer transitions between dark/light boxes in comparison to their younger counterparts (p < 0.0001) (Figures 5A,B). Notably, treatment with both SO and MR hydrosols resulted in a significant alleviation of these anxiety-like behavior (p ≤ 0.05).
Figure 5. Effects of the hydrosols of Salvia officinalis (SO) and Mentha rotundifolia (MR) on the number of rats entries in the inner zone in the open field (A), and the number of transitions in the light/dark box task (B). Results are presented as means ± SD (n = 6). #p ≤ 0.05 compared to the young rat groups (control, MR, and SO groups at 18-month-old). ap ≤ 0.05 compared to aged control rats (24- month-old).
Similarly, significant disparities were observed in the time spent within both the inner zone and the light box among the experimental groups (Figures 6A,B). Aged rats demonstrated a significantly reduced duration in both areas compared to their younger counterparts (p ≤ 0.0001), indicative of heightened anxiety levels. In contrary, the administration of SO and MR hydrosols to aged rats resulted in a substantial increase in the time spent in these areas (p < 0.0001 for both) suggesting a potent anxiolytic effect of the treatments.
Figure 6. Effects of the hydrosols of Salvia officinalis (SO) and Mentha rotundifolia (MR) on the time spent in the inner zone by aging rats in the open field (A) and the time spent in the light box in the light/dark box task (B). Results are presented as means ± SD (n = 6). #p ≤ 0.05 compared to the young rat groups (control, MR, and SO groups at 18-month-old). ap ≤ 0.05 compared to aged control rats (24- month-old).
The effect of MR and SO hydrosols on age-related exploratory activity and cognitive impairments was examined using the y-maze task. Assessment included the evaluation of spontaneous alternation behavior (Figure 7) and reference working memory, represented by the number of entries into the novel arm and the corresponding time spent in it (Figure 8). The results revealed a significant decrease in alternation in control aged rats that was reversed in MR and SO hydrosols treated groups (p < 0.0001) (Figure 7B), demonstrating a higher exploratory activity.
Figure 7. Effects of the hydrosols of Salvia officinalis (SO) and Mentha rotundifolia (MR) on aging-related deficiency on the spontaneous alternation y-maze in rats, presented as the number of total entries in different arms (A), and percent alternations (B). Results are presented as means ± SD (n = 6). #p ≤ 0.05 compared to the young rat groups (control, MR, and SO groups at 18-month-old). ap ≤ 0.05 compared to aged control rats (24- month-old).
Figure 8. Effects of the hydrosols of Salvia officinalis (SO) and Mentha rotundifolia (MR) on the reference working memory of aging rats: the number of entries (A) and the time spent (B) in the novel arm in the y-maze task. Results are presented as means ± SD (n = 6). #p ≤ 0.05 compared to the young rat groups (control, MR, and SO group at 18-month-old). ap ≤ 0.05 compared to aged control rats (24- month-old).
Regarding reference working memory, the outcomes indicated a significant reduction in both the number of entries into the novel arm and the corresponding time spent within it among aged rats (p < 0.0001), in contrast to their younger counterparts (Figures 8A,B). Treatment of aged rats with MR and SO hydrosols yielded a noteworthy enhancement in spatial working memory. This improvement was evident through a substantial increase in the number of entries into the novel arms when compared to the aged control group (p = 0.006 and p = 0.0015, respectively) and a heightened duration spent within the novel arm (p = 0.0361 and p = 0.0001, respectively).
Pain sensitivity was evaluated through the tail immersion test. A notable age-related increase in animals’ pain sensitivity was evidenced by a reduction in tail withdrawal latency time within the control group (p ≤ 0.05) (Figure 9A). Groups treated with MR and SO hydrosols exhibited a substantial increase in tolerance to heat stimuli, as reflected by a significantly prolonged tail withdrawal latency time (p ≤ 0.05) (Figure 9A). These findings imply that MR and SO hydrosols exert an analgesic effect, suggesting their potential utility in mitigating age-associated changes in pain sensitivity.
Figure 9. Effects of the hydrosols of Salvia officinalis (SO) and Mentha rotundifolia (MR) against hyperalgesia (A) and cold allodynia (B). #p ≤ 0.05 compared to the young rat groups (control, MR, and SO groups at 18-month-old). ap ≤ 0.05 compared to aged control rats (24- month-old).
Cold allodynia was assessed using the acetone test. Notably, a substantial augmentation in the duration of licking, biting, and paw lifting in response to the stimulus was observed in aged rats belonging to the control group (Figure 9B). In contrast, these behaviors exhibited a marked reduction in groups treated with hydrosols. Specifically, the behavior of the 24-month-old male rats treated with MR hydrosol closely resembled that of 18-month-old rats, indicating a potential ameliorative effect of the hydrosol on cold allodynia in aging rats (p = 0.0061) (Figure 9B).
Aged rats within the control group exhibited significant elevated levels of AST, ALT, and GGT in comparison to groups treated with MR and SO hydrosols (p ≤ 0.05) (Table 4). However, no discernible difference was observed in the levels of bilirubin among the investigated groups (p ≥ 0.05).
Table 4. Effects of the hydrosols of Salvia officinalis (SO) and Mentha rotundifolia (MR) on hepatic biomarkers of aged rats.
The analysis of GSH, GPx, and SOD levels in brain and liver tissues revealed significant antioxidant effects of MR and SO hydrosols, Table 5. Both hydrosols groups exhibited elevated GSH levels, increased GPx activity, and enhanced SOD activity compared to the control group, indicating considerable defense against oxidative stress. Notably, the MR hydrosol treated group displayed particularly robust antioxidant responses in liver tissue. These findings suggest the potential of MR and SO hydrosols to confer antioxidant benefits.
Table 5. Effects of the hydrosols of Salvia officinalis (SO) and Mentha rotundifolia (MR) on antioxidants biomarkers in brain and liver tissues of aged rats.
Primarily composed of water and aromatic active principles, plant hydrosols are ideal candidates for chronic administration as soft functional drinks (Hamedi et al., 2017b). In this study, focus was directed toward Mentha rotundifolia (MR) and Salvia officinalis (SO) hydrosols that demonstrated noteworthy in-vitro antioxidant and anti-inflammatory activities, prompting us to investigate their potential as a long-term solution for preventing aging-related comorbidities.
The study design was conceived to match the human aging process. Laboratory rats, housed in cages with limited space and fed with processed food may replicate the sedentary behavior and imbalanced dietary patterns common in contemporary human lifestyles (Timlin et al., 2020). Such conditions are recognized for promoting oxidative stress, a key contributor to the aging process (Tan et al., 2018). Additionally, a day in the life of a rat approximates 34.8 days in the life of a human (Sengupta, 2013). Therefore, a six-month follow-up of 18-month-old animals is roughly analogous to a two-decade follow-up of a 50-year-old individual until the age of 70.
Aged rats displayed significant motor impairment assessed by the open field and rotarod tests. With advancing age, the loss of muscle mass, known as sarcopenia, can lead to weaker muscles, making movement and physical tasks more challenging (Volpi et al., 2004; Larsson et al., 2019). Numerous studies have linked oxidative stress to sarcopenia, demonstrating its role in increasing proteolysis and/or reducing protein synthesis (Meng and Yu, 2010; Wiedmer et al., 2021). Aged rats also manifested elevated anxious behaviors evaluated through the open field and dark/light box tests. Elevated levels of oxidative stress and reduced telomere length have been proven to be associated with anxiety disorders, both of which characterize aging (Simon et al., 2006; Pousa et al., 2021). Finally, aged rats exhibited cognitive deficits related to spontaneous alternation and spatial working memory evaluated by the Y-maze test. Indeed, the capacity for learning gradually diminishes with aging (Klencklen et al., 2017). The decline in memory retention capacity has been shown to result from oxidative stress-induced delayed-type apoptosis observed in the hippocampal CA1 region (Kandlur et al., 2020). In contrast, all observed behavioral impairments were averted in MR and SO hydrosols treated groups. The chemical composition of these hydrosols revealed the presence of various compounds that likely contributed to the deceleration of the aging process. 1,8 Cineole, documented for its robust antioxidant, anti-inflammatory, and anxiolytic properties, was found to exhibit anti-aging effect by inducing G0/G1 cell cycle arrest in stress-induced senescence in HepG2 cells (Juergens et al., 2004; Greiner et al., 2013; Dougnon and Ito, 2020; Rodenak-Kladniew et al., 2020). Camphor and camphene, along with 1,8 cineole, have been investigated for their anti-acetylcholinesterase and cognition enhancement effects (Werner et al., 2009; Babazadeh et al., 2023; Ertosun et al., 2023). Additionally, ɣ-terpinene and terpinen-4-ol have demonstrated neuroprotective effects against oxidative stress by inhibiting the generation of pro-inflammatory cytokines (Ayaz et al., 2017; Asikin et al., 2022; Deen et al., 2023).
As anticipated, aged rats demonstrated elevated pain sensitivity, as evaluated through the tail immersion test and exposure to acetone. The findings align with established knowledge indicating an age-related increase in pain threshold, predisposing individuals to neuropathic pain, characterized by hyperalgesia and allodynia (Yezierski, 2012; Lautenbacher et al., 2017). These effects were completely reversed in groups treated with MR and SO hydrosols showcasing a significant analgesic effect. The presence of camphor, a predominant component in both hydrosols, has been documented to alleviate hyperalgesia symptoms in various neuropathic pain models by modulating the excitability of dorsal root ganglion neurons (dos Santos et al., 2021; Li et al., 2023). Similarly, 1,8 cineole has been shown to inhibit the overexpression of the P2X2 receptor protein and mRNA, which plays a role in the transmission of algesia and nociception information by primary sensory neurons in the spinal cord and dorsal horn in CCI rats (Zheng et al., 2019).
Finally, the measurement of hepatic markers (ALT, AST, and GGT) as well as antioxidants (GSH, GPx, and SOD) in cerebral and hepatic tissues aligns consistently with the outcomes observed in behavioral tests. In accordance with the oxidative stress theory, the aged control rats manifested an augmented state of oxidative stress, as evidenced by a decline in antioxidant concentrations and a substantial elevation in ALT, AST, and GGT levels (Le Couteur et al., 2010; Kozakiewicz et al., 2019; Warraich et al., 2020). In contrast, administration of hydrosols effectively mitigated these adverse effects. The beneficial impact of hydrosols can be attributed to their role as a persistent source of antioxidants, potentially alleviating the age-related decline in liver detoxification functions. The intricate process of detoxification involves synergistic interactions between biotransformation enzymes and endogenous antioxidants. With progressive aging, a discernible reduction in endogenous antioxidant levels ensues, leading to a deceleration of the detoxification process (Vyskočilová et al., 2013). Consequently, the perturbation in the liver’s redox equilibrium and the ensuing oxidative stress culminate in the release of ROS and other deleterious molecules, including lipid peroxidation products and aldehydes, exerting systemic effects on distant organs and physiological systems (Adibhatla and Hatcher, 2010). Moreover, hepatic oxidative stress prompts the release of pro-inflammatory cytokines and mediators into the bloodstream (Dludla et al., 2020). These inflammatory signals possess the capacity to traverse the blood–brain barrier, contributing to neuroinflammation and influencing the antioxidant defenses within the cerebral milieu (Sankowski et al., 2015; Chen et al., 2022), as substantiated by our findings.
While aging remains an inexorable aspect of life, the primary objective of this study was not to impede its course but rather to attenuate the onset of consequential complications. The comprehensive evaluation encompassing physical, behavioral, and biochemical parameters revealed a compelling outcome. At the chronological age of 2 years, all animals exhibited discernible signs of aging. However, the groups treated with Salvia officinalis (SO) and Mentha rotundifolia (MR) hydrosols were biologically younger. These findings suggest that the hydrosols treatment holds promise in mitigating aging-related effects and offers a potential avenue for influencing the aging process. Our results contribute valuable insights for future research seeking interventions to promote healthier aging outcomes and address aging-related physiological changes.
The raw data supporting the conclusions of this article will be made available by the authors, without undue reservation.
The animal study was approved by Ethical Committee for Animal Veterinary Science and Public health, Institut Agronomique et Vétérinaire Hassan II, Rabat-Kingdom of Morocco. Approval No CESASPV_2023_A08. The study was conducted in accordance with the local legislation and institutional requirements.
KB: Conceptualization, Data curation, Formal analysis, Funding acquisition, Investigation, Methodology, Project administration, Resources, Software, Supervision, Validation, Visualization, Writing – original draft, Writing – review & editing. HI: Data curation, Formal analysis, Investigation, Methodology, Writing – original draft. ZL: Conceptualization, Investigation, Methodology, Writing – original draft. MS: Investigation, Methodology, Software, Supervision, Validation, Visualization, Writing – original draft, Writing – review & editing. KT: Supervision, Validation, Visualization, Writing – review & editing.
The author(s) declare that no financial support was received for the research, authorship, and/or publication of this article.
ChatGPT was used to improve the English of the manuscript.
The authors declare that the research was conducted in the absence of any commercial or financial relationships that could be construed as a potential conflict of interest.
All claims expressed in this article are solely those of the authors and do not necessarily represent those of their affiliated organizations, or those of the publisher, the editors and the reviewers. Any product that may be evaluated in this article, or claim that may be made by its manufacturer, is not guaranteed or endorsed by the publisher.
Acimovic, M., Tešević, V., Smiljanic, K., Cvetkovic, M., Stankovic, J., Kiprovski, B., et al. (2020). Hydrolates: by-products of essential oil distillation: chemical composition, biological activity and potential uses. Adv. Technol. 9, 54–70. doi: 10.5937/savteh2002054A
Adibhatla, R. M., and Hatcher, J. F. (2010). Lipid oxidation and peroxidation in CNS health and disease: from molecular mechanisms to therapeutic opportunities. Antioxid. Redox Signal. 12, 125–169. doi: 10.1089/ars.2009.2668
Asikin, Y., Shimizu, K., Iwasaki, H., Oku, H., and Wada, K. (2022). Stress amelioration and anti-inflammatory potential of Shiikuwasha (Citrus depressa Hayata) essential oil, limonene, and γ-terpinene. J. Food Drug Anal. 30, 454–465. doi: 10.38212/2224-6614.3414
Ayaz, M., Sadiq, A., Junaid, M., Ullah, F., Subhan, F., and Ahmed, J. (2017). Neuroprotective and anti-aging potentials of essential oils from aromatic and medicinal plants. Front. Aging Neurosci. 9:168. doi: 10.3389/fnagi.2017.00168
Babazadeh, A., Vahed, F. M., Liu, Q., Siddiqui, S. A., Kharazmi, M. S., and Jafari, S. M. (2023). Natural bioactive molecules as Neuromedicines for the treatment/prevention of neurodegenerative diseases. ACS Omega 8, 3667–3683. doi: 10.1021/acsomega.2c06098
Baek, K.-W., Jung, Y.-K., Kim, J.-S., Park, J. S., Hah, Y.-S., Kim, S.-J., et al. (2020). Rodent model of muscular atrophy for sarcopenia study. J. Bone Metab. 27, 97–110. doi: 10.11005/jbm.2020.27.2.97
Bannon, A. W., and Malmberg, A. B. (2007). Models of nociception: hot-plate, tail-flick, and formalin tests in rodents. Curr. Protoc. Neurosci. Chapter 41:ns0809s41. doi: 10.1002/0471142301.ns0809s41
Barrajón-Catalán, E., Herranz-López, M., Joven, J., Segura-Carretero, A., Alonso-Villaverde, C., Menéndez, J. A., et al. (2014). “Molecular promiscuity of plant polyphenols in the Management of age-Related Diseases: far beyond their antioxidant properties” in Oxidative stress and inflammation in non-communicable diseases – molecular mechanisms and perspectives in therapeutics advances in experimental medicine and biology. ed. J. Camps (Cham: Springer International Publishing), 141–159.
Baydar, H., Sangun, M. K., Erbas, S., and Kara, N. (2013). Comparison of aroma compounds in distilled and extracted products of sage (salvia officinalis L.). J. Essent. Oil Bear. Plants 16, 39–44. doi: 10.1080/0972060X.2013.764175
Boualam, K., Bouhaddou, N., Sobeh, M., Tabyaoui, M., and Taghzouti, K. (2023). Mentha rotundifolia (L.) Huds. Aqueous extract attenuates H2O2 induced oxidative stress and neurotoxicity. Front. Neurosci. 17, 17:1121029. doi: 10.3389/fnins.2023.1121029
Checa, J., and Aran, J. M. (2020). Reactive oxygen species: drivers of physiological and pathological processes. J. Inflamm. Res. 13, 1057–1073. doi: 10.2147/JIR.S275595
Chen, S., Li, L., Peng, C., Bian, C., Ocak, P. E., Zhang, J. H., et al. (2022). Targeting oxidative stress and inflammatory response for blood–brain barrier protection in intracerebral hemorrhage. Antioxid. Redox Signal. 37, 115–134. doi: 10.1089/ars.2021.0072
Crawley, J. N. (1985). Exploratory behavior models of anxiety in mice. Neurosci. Biobehav. Rev. 9, 37–44. doi: 10.1016/0149-7634(85)90030-2
Daina, A., Michielin, O., and Zoete, V. (2017). SwissADME: a free web tool to evaluate pharmacokinetics, drug-likeness and medicinal chemistry friendliness of small molecules. Sci. Rep. 7:42717. doi: 10.1038/srep42717
Deen, J. I., Zawad, A. N. M. S., Uddin, M., Chowdhury, M. A. H., Al Araby, S. Q., and Rahman, M. A. (2023). Terpinen-4-ol, a volatile terpene molecule, extensively electrifies the biological systems against the oxidative stress-linked pathogenesis. Adv. Redox Res. 9:100082. doi: 10.1016/j.arres.2023.100082
Deuis, J. R., Dvorakova, L. S., and Vetter, I. (2017). Methods used to evaluate pain behaviors in rodents. Front. Mol. Neurosci. 10:284. doi: 10.3389/fnmol.2017.00284
Dhawan, S. S., Xia, S., Tait, D. S., Bundgaard, C., Bowman, E., and Brown, V. J. (2018). Oral dosing of rodents using a palatable tablet. Psychopharmacology 235, 1527–1532. doi: 10.1007/s00213-018-4863-2
Dludla, P. V., Nkambule, B. B., Mazibuko-Mbeje, S. E., Nyambuya, T. M., Marcheggiani, F., Cirilli, I., et al. (2020). N-acetyl cysteine targets hepatic lipid accumulation to curb oxidative stress and inflammation in NAFLD: a comprehensive analysis of the literature. Antioxidants 9:1283. doi: 10.3390/antiox9121283
dos Santos, E., Leitão, M. M., Aguero Ito, C. N., Silva-Filho, S. E., Arena, A. C., Silva-Comar, F. M. D. S., et al. (2021). Analgesic and anti-inflammatory articular effects of essential oil and camphor isolated from Ocimum kilimandscharicum Gürke leaves. J. Ethnopharmacol. 269:113697. doi: 10.1016/j.jep.2020.113697
Dougnon, G., and Ito, M. (2020). Inhalation Administration of the Bicyclic Ethers 1,8- and 1,4-cineole prevent anxiety and depressive-like Behaviours in mice. Molecules 25:1884. doi: 10.3390/molecules25081884
Ertosun, G. B., Ergen, M., Bardakci, H., Barak, T. H., and Suyen, G. (2023). Cholinergic cognitive enhancer effect of Salvia triloba L. essential oil inhalation in rats. Marmara Med. J. 36, 361–370. doi: 10.5472/marumj.1368345
Gould, T. D., Dao, D. T., and Kovacsics, C. E. (2009). “The open field test” in Mood and anxiety related phenotypes in mice: Characterization using behavioral tests Neuromethods. ed. T. D. Gould (Totowa, NJ: Humana Press), 1–20.
Greiner, J. F.-W., Müller, J., Zeuner, M.-T., Hauser, S., Seidel, T., Klenke, C., et al. (2013). 1,8-cineole inhibits nuclear translocation of NF-κB p65 and NF-κB-dependent transcriptional activity. Biochim. Biophys. Acta BBA Mol. Cell Res. 1833, 2866–2878. doi: 10.1016/j.bbamcr.2013.07.001
Hamedi, A., Moheimani, S. M., Sakhteman, A., Etemadfard, H., and Moein, M. (2017a). An overview on indications and chemical composition of aromatic waters (hydrosols) as functional beverages in Persian nutrition culture and folk medicine for hyperlipidemia and cardiovascular conditions. J. Evid.-Based Complement. Altern. Med. 22, 544–561. doi: 10.1177/2156587216686460
Hamedi, A., Pasdaran, A., Zebarjad, Z., and Moein, M. (2017b). A survey on chemical constituents and indications of aromatic waters soft drinks (hydrosols) used in Persian nutrition culture and folk medicine for neurological disorders and mental health. J. Evid.-Based Complement. Altern. Med 22, 744–752. doi: 10.1177/2156587217714145
Hasanein, P., Felehgari, Z., and Emamjomeh, A. (2016). Preventive effects of Salvia officinalis L. against learning and memory deficit induced by diabetes in rats: possible hypoglycaemic and antioxidant mechanisms. Neurosci. Lett. 622, 72–77. doi: 10.1016/j.neulet.2016.04.045
Janssen, P. A., Niemegeers, C. J., and Dony, J. G. (1963). The inhibitory effect of fentanyl and other morphine-like analgesics on the warm water induced tail withdrawl reflex in rats. Arzneimittelforschung 13, 502–507.
Juergens, U. R., Engelen, T., Racké, K., Stöber, M., Gillissen, A., and Vetter, H. (2004). Inhibitory activity of 1,8-cineole (eucalyptol) on cytokine production in cultured human lymphocytes and monocytes. Pulm. Pharmacol. Ther. 17, 281–287. doi: 10.1016/j.pupt.2004.06.002
Kandlur, A., Satyamoorthy, K., and Gangadharan, G. (2020). Oxidative stress in cognitive and epigenetic aging: a retrospective glance. Front. Mol. Neurosci. 13:41. doi: 10.3389/fnmol.2020.00041
Khan, S. S., Singer, B. D., and Vaughan, D. E. (2017). Molecular and physiological manifestations and measurement of aging in humans. Aging Cell 16, 624–633. doi: 10.1111/acel.12601
Kinder, J. M., Then, J. E., Hansel, P. M., Molinero, L. L., and Bruns, H. A. (2014). Long-term repeated daily use of Intragastric gavage hinders induction of Oral tolerance to ovalbumin in mice. Comp. Med. 64, 369–376.
Klencklen, G., Banta Lavenex, P., Brandner, C., and Lavenex, P. (2017). Working memory decline in normal aging: is it really worse in space than in color? Learn. Motiv. 57, 48–60. doi: 10.1016/j.lmot.2017.01.007
Kozakiewicz, M., Kornatowski, M., Krzywińska, O., and Kędziora-Kornatowska, K. (2019). Changes in the blood antioxidant defense of advanced age people. Clin. Interv. Aging 14, 763–771. doi: 10.2147/CIA.S201250
Kraeuter, A.-K., Guest, P. C., and Sarnyai, Z. (2019). The Y-maze for assessment of spatial working and reference memory in mice. Methods Mol. Biol. Clifton NJ 1916, 105–111. doi: 10.1007/978-1-4939-8994-2_10
Kunicka-Styczyńska, A., Śmigielski, K., Prusinowska, R., Rajkowska, K., Kuśmider, B., and Sikora, M. (2015). Preservative activity of lavender hydrosols in moisturizing body gels. Lett. Appl. Microbiol. 60, 27–32. doi: 10.1111/lam.12346
Larsson, L., Degens, H., Li, M., Salviati, L., Lee, Y. I., Thompson, W., et al. (2019). Sarcopenia: aging-related loss of muscle mass and function. Physiol. Rev. 99, 427–511. doi: 10.1152/physrev.00061.2017
Lautenbacher, S., Peters, J. H., Heesen, M., Scheel, J., and Kunz, M. (2017). Age changes in pain perception: a systematic-review and meta-analysis of age effects on pain and tolerance thresholds. Neurosci. Biobehav. Rev. 75, 104–113. doi: 10.1016/j.neubiorev.2017.01.039
Le Couteur, D. G., Blyth, F. M., Creasey, H. M., Handelsman, D. J., Naganathan, V., Sambrook, P. N., et al. (2010). The Association of Alanine Transaminase with Aging, frailty, and mortality. J. Gerontol. A Biol. Sci. Med. Sci. 65A, 712–717. doi: 10.1093/gerona/glq082
Li, Z., Gan, Y., Kang, T., Zhao, Y., Huang, T., Chen, Y., et al. (2023). Camphor attenuates hyperalgesia in neuropathic pain models in mice. J. Pain Res. 16, 785–795. doi: 10.2147/JPR.S398607
Lin, M. T., and Flint Beal, M. (2003). The oxidative damage theory of aging. Clin. Neurosci. Res. 2, 305–315. doi: 10.1016/S1566-2772(03)00007-0
Lowry, O. H., Rosebrough, N. J., Farr, A. L., and Randall, R. J. (1951). Protein measurement with the FOLIN phenol reagent. J. Biol. Chem. 193, 265–275. doi: 10.1016/S0021-9258(19)52451-6
Mahmoud, M. F., Elrashidy, R. A., Mohammed, H. O., Drissi, B., Mahdi, I., and Sobeh, M. (2023). Essential oil and polyphenolics from Thymus satureioides Coss. Counteract acrylamide-induced liver toxicity through suppression of NLRP3 inflammasome/NF-κB axis. J. Funct. Foods 107:105641. doi: 10.1016/j.jff.2023.105641
Meng, S.-J., and Yu, L.-J. (2010). Oxidative stress, molecular inflammation and sarcopenia. Int. J. Mol. Sci. 11, 1509–1526. doi: 10.3390/ijms11041509
Panossian, A., and Efferth, T. (2022). Network pharmacology of Adaptogens in the assessment of their pleiotropic therapeutic activity. Pharmaceuticals 15:1051. doi: 10.3390/ph15091051
Phillips, P. M., Jarema, K. A., Kurtz, D. M., and MacPhail, R. C. (2010). An observational assessment method for aging laboratory rats. J. Am. Assoc. Lab. Anim. Sci. 49, 792–799.
Poeggeler, B. (2005). Melatonin, aging, and age-related diseases. Endocrine 27, 201–212. doi: 10.1385/ENDO:27:2:201
Pousa, P. A., Souza, R. M., Melo, P. H. M., Correa, B. H. M., Mendonça, T. S. C., Simões-e-Silva, A. C., et al. (2021). Telomere shortening and psychiatric disorders: a systematic review. Cell 10:1423. doi: 10.3390/cells10061423
Proshkina, E., Plyusnin, S., Babak, T., Lashmanova, E., Maganova, F., Koval, L., et al. (2020). Terpenoids as potential Geroprotectors. Antioxidants 9:529. doi: 10.3390/antiox9060529
Rodenak-Kladniew, B., Castro, A., Stärkel, P., Galle, M., and Crespo, R. (2020). 1,8-cineole promotes G0/G1 cell cycle arrest and oxidative stress-induced senescence in HepG2 cells and sensitizes cells to anti-senescence drugs. Life Sci. 243:117271. doi: 10.1016/j.lfs.2020.117271
Sankowski, R., Mader, S., and Valdés-Ferrer, S. I. (2015). Systemic inflammation and the brain: novel roles of genetic, molecular, and environmental cues as drivers of neurodegeneration. Front. Cell. Neurosci. 9:28. doi: 10.3389/fncel.2015.00028
Scimonelli, T., Marucco, M., and Celis, M. E. (1999). Age-related changes in grooming behavior and motor activity in female rats. Physiol. Behav. 66, 481–484. doi: 10.1016/s0031-9384(98)00314-x
Seibenhener, M. L., and Wooten, M. C. (2015). Use of the open field maze to measure locomotor and anxiety-like behavior in mice. J. Vis. Exp. JoVE 52434:e52434. doi: 10.3791/52434
Sengupta, P. (2013). The laboratory rat: relating its age with Human’s. Int. J. Prev. Med. 4, 624–630.
Shiotsuki, H., Yoshimi, K., Shimo, Y., Funayama, M., Takamatsu, Y., Ikeda, K., et al. (2010). A rotarod test for evaluation of motor skill learning. J. Neurosci. Methods 189, 180–185. doi: 10.1016/j.jneumeth.2010.03.026
Simon, N. M., Smoller, J. W., McNamara, K. L., Maser, R. S., Zalta, A. K., Pollack, M. H., et al. (2006). Telomere shortening and mood disorders: preliminary support for a chronic stress model of accelerated aging. Biol. Psychiatry 60, 432–435. doi: 10.1016/j.biopsych.2006.02.004
Song, S., Lam, E. W.-F., Tchkonia, T., Kirkland, J. L., and Sun, Y. (2020). Senescent cells: emerging targets for human aging and age-related diseases. Trends Biochem. Sci. 45, 578–592. doi: 10.1016/j.tibs.2020.03.008
Spagnuolo, C., Moccia, S., and Russo, G. L. (2018). Anti-inflammatory effects of flavonoids in neurodegenerative disorders. Eur. J. Med. Chem. 153, 105–115. doi: 10.1016/j.ejmech.2017.09.001
Taïwe, G. S., and Kuete, V. (2017). “Chapter 2 – Management of Inflammatory and Nociceptive Disorders in Africa” in Medicinal spices and vegetables from Africa. ed. V. Kuete (Cambridge, MA: Academic Press), 73–92.
Tan, B. L., Norhaizan, M. E., and Liew, W.-P.-P. (2018). Nutrients and oxidative stress: friend or foe? Oxidative Med. Cell. Longev. 2018:e9719584, 1–24. doi: 10.1155/2018/9719584
Timlin, D., McCormack, J. M., Kerr, M., Keaver, L., and Simpson, E. E. A. (2020). Are dietary interventions with a behaviour change theoretical framework effective in changing dietary patterns? A systematic review. BMC Public Health 20:1857. doi: 10.1186/s12889-020-09985-8
Volpi, E., Nazemi, R., and Fujita, S. (2004). Muscle tissue changes with aging. Curr. Opin. Clin. Nutr. Metab. Care 7, 405–410. doi: 10.1097/01.mco.0000134362.76653.b2
Vyskočilová, E., Szotáková, B., Skálová, L., Bártíková, H., Hlaváčová, J., and Boušová, I. (2013). Age-related changes in hepatic activity and expression of detoxification enzymes in male rats. Biomed. Res. Int. 2013:408573, 1–10. doi: 10.1155/2013/408573
Wajs-Bonikowska, A., Sienkiewicz, M., Stobiecka, A., Maciąg, A., Szoka, Ł., and Karna, E. (2015). Chemical composition and biological activity of Abies alba and A. koreana seed and cone essential oils and characterization of their seed hydrolates. Chem. Biodivers. 12, 407–418. doi: 10.1002/cbdv.201400167
Warraich, U.-A., Hussain, F., and Kayani, H. U. R. (2020). Aging – oxidative stress, antioxidants and computational modeling. Heliyon 6:e04107. doi: 10.1016/j.heliyon.2020.e04107
Werner, N. S., Duschek, S., and Schandry, R. (2009). D-camphor-crataegus berry extract combination increases blood pressure and cognitive functioning in the elderly – a randomized, placebo controlled double blind study. Phytomedicine 16, 1077–1082. doi: 10.1016/j.phymed.2009.05.006
Wiedmer, P., Jung, T., Castro, J. P., Pomatto, L. C. D., Sun, P. Y., Davies, K. J. A., et al. (2021). Sarcopenia – molecular mechanisms and open questions. Ageing Res. Rev. 65:101200. doi: 10.1016/j.arr.2020.101200
Wiley and NIST (2017). Wiley Registry, 11e / NIST 2017 Mass Spectral Library Upgrade. 11th edition. Wiley.
Yaghoob, A., Zahra, A., Hajimehdipoor, H., and Faramarzi, M. (2004). GC/MS analysis of Citrus aurantium L. Hydrolate and its comparison with the commercial samples GC/MS analysis of Citrus aurantium L. Hydrolate and its comparison with the commercial samples. Iran. J. Pharm. Res. 3, 177–179.
Yezierski, R. P. (2012). The effects of age on pain sensitivity: pre-clinical studies. Pain Med. Malden Mass 13 Suppl 2, S27–S36. doi: 10.1111/j.1526-4637.2011.01311.x
Zheng, X., Zhang, Y., Li, Q., Liu, Y., Wang, X., Yang, B., et al. (2019). Effects of 1,8-cineole on neuropathic pain mediated by P2X2 receptor in the spinal cord dorsal horn. Sci. Rep. 9:7909. doi: 10.1038/s41598-019-44282-4
Keywords: Mentha rotundifolia, Salvia officinalis, aging, hydrosols, healthy aging
Citation: Boualam K, Ibork H, Lahboub Z, Sobeh M and Taghzouti K (2024) Mentha rotundifolia (L.) Huds. and Salvia officinalis L. hydrosols mitigate aging related comorbidities in rats. Front. Aging Neurosci. 16:1365086. doi: 10.3389/fnagi.2024.1365086
Received: 03 January 2024; Accepted: 12 February 2024;
Published: 23 February 2024.
Edited by:
Celeste Annemarie De Jager Loots, Imperial College London, United KingdomReviewed by:
Chandra Prakash, Jawaharlal Nehru University, IndiaCopyright © 2024 Boualam, Ibork, Lahboub, Sobeh and Taghzouti. This is an open-access article distributed under the terms of the Creative Commons Attribution License (CC BY). The use, distribution or reproduction in other forums is permitted, provided the original author(s) and the copyright owner(s) are credited and that the original publication in this journal is cited, in accordance with accepted academic practice. No use, distribution or reproduction is permitted which does not comply with these terms.
*Correspondence: Khadija Boualam, a2hhZGlqYWJvdWFsYW05NEBnbWFpbC5jb20=
Disclaimer: All claims expressed in this article are solely those of the authors and do not necessarily represent those of their affiliated organizations, or those of the publisher, the editors and the reviewers. Any product that may be evaluated in this article or claim that may be made by its manufacturer is not guaranteed or endorsed by the publisher.
Research integrity at Frontiers
Learn more about the work of our research integrity team to safeguard the quality of each article we publish.